- 1Forest Science Department, NEIKER-Basque Institute for Agricultural Research and Development, Basque Research and Technology Alliance (BRTA), Bizkaia Science and Technology Park, Derio, Spain
- 2Amillena Koop Elkarte Txikia, Popular Lab for Food Sovereignty and (trans)formation, Atxondo, Spain
- 3Public Health Division of Bizkaia, Basque Government, Bilbao, Spain
Forest biomass is considered an alternative to fossil fuels in energy production, as part of global strategies for climate change mitigation. Application of by-products such as wood ash (WA) and biochar (BC) to soil could replace the nutrients removed by tree harvesting and could also increase soil carbon stocks. However, the extent to which these amendments can provide benefits depends on how the by-products interact with the soil-water-plant system. We studied the short-term responses of WA and BC application in two different mineral soil-water-plant systems in temperate forests: A. Typic Udorthent (TU) with mature Pinus radiata; B. Typic Dystrudept (TD) with young Quercus pyrenaica, to test the following hypotheses: (1) the application of WA and BC will increase nutrient uptake by plants, but (2) these products could induce toxicity in the soil-water-plant system, and (3) in case of no toxicity, plant biomass growth in these temperate forest soils will increase due to increased plant nutrient uptake. Biochar was applied at rates of 3.5, 10, and 20 Mg ha–1 and WA at rates of 1.5, 4.5, and 9 Mg ha–1 (calcium equivalent). A nitrogen enriched treatment was applied with the intermediate doses. Ecotoxicity testing indicated that WA and BC were not toxic, although Ni uptake increased in biomass of the TU after BC + N application. BC increased SOC stocks of both sites, depending on treatment. In TD BC increased K uptake by plants, but did not increase biomass. In summary, this study shows that the application of BC and WA had different effects on the soil -water-plant system in two different forest soils. This difference was attributed to (i) the soil characteristics, (ii) the application rates and (iii) whether or not nitrogen was applied. Long-term field experiments are required to test the performance and potential toxicity of these by-products as soil enhancers.
1. Introduction
Forest tree biomass has become a substantial source of biomass-based energy in the northern countries over last decades (Pugliese et al., 2014). Increased production energy based on forest biomass is envisaged in either international or national climate and energy strategies (e.g., EVE, 2017), as an alternative to the use of fossil fuels and as part of global climate change mitigation strategies, such as the target of carbon neutrality by 2050 proposed by the European Union within the “Green Deal.” Biomass production, the raw material for fulfilling these targets, are mainly determined by climate and soil.
Application to soil of products derived from bioenergy production, such as wood ash (WA) and biochar (BC), has been proposed as a means of enhancing soil quality within the circular economy framework, as well as a solution for disposing of the residues (Insam and Knapp, 2011). These products can replace the nutrients removed by tree harvesting, as well as increase soil carbon stocks, particularly BC (e.g., Sohi, 2012). However, the extent to which soil amendments can provide benefits to soil fertility and plant nutritional status depends on the interactions between the added products and the soil-water-plant environment.
Wood ash has been used in both the USA and Europe to replace nutrients in forest soils (e.g., Pitman, 2006). Calcium (Ca) is the most abundant element in WA, followed by potassium (K), magnesium (Mg), and phosphorus (P) (Reid and Watmough, 2014), as well as increasing pH directly through the reaction with water of the anion bound to the Ca. Although WA affects soil physical properties (Moragues-Saitua et al., 2017), it is mainly used to modify the chemical properties of soil (Demeyer et al., 2001).
Many governments have become interested in the production and use of BC, especially because of its potentiality in climate change mitigation, mainly by reducing greenhouse gas emissions in energy production, as well as increasing C persistence within the soil (Lehmann et al., 2021). Biochar is derived from a pyrolysis process and could be used to increase organic matter stocks in soils while increasing soil quality (Lehmann and Joseph, 2009). Biochar has the potential to increase the cation exchange capacity of soil (Liang et al., 2013) and to enhance nutrient retention (Biederman and Harpole, 2013) because of its characteristic properties, such as porous structure, large surface area and negative surface charge. Biochar can also supply nutrients and liming compounds, depending on the ash content and how fresh the product is (Verheijen et al., 2009).
In addition to nutrients, hazardous heavy metals, such as cadmium (Cd), chromium (Cr), zinc (Zn), and nickel (Ni), are also concentrated in WA and BC (e.g., Reimann et al., 2008). WA application guidelines were developed in many countries, some as essential components of biomass harvesting guidelines (Titus et al., 2021) and its use on forestry and agriculture is regulated in some European countries, such as Denmark, Finland, Lithuania, Sweden, Austria, and Germany (Hannam et al., 2018). However, chemical analysis alone is not sufficient to estimate the risk associated with the use of these materials as soil fertilizers (Malara and Oleszczuk, 2013). Interactions between the soil and products applied may make it difficult to predict how these compounds will behave in the ecosystem (Oleszczuk et al., 2013). In this respect, biological assays may complement chemical analysis and provide a wider overview to increase knowledge about possible interactions between various contaminants and demonstrate the existence or absence of toxic effects in a wide range of organisms (Oleszczuk et al., 2013).
Some studies have examined the effects of WA on heavy metal contents in soil, water and forest biota (Olsson et al., 2017). By contrast, studies reporting toxic or beneficial effects of the application of BC to forest soils remain scarce (Gogoi et al., 2019). There is also a lack of research examining both toxicological and nutritional effects simultaneously in the soil-water plant environment of temperate forests where WA or BC have been applied. The existing meta-analysis based on studies of the application WA and BC in the field could provide an overview of the significant effects. For example, Jeffery et al. (2011) reported an overall small, but positive effect of BC application on crop production, suggesting liming and increased water holding capacity of the soil as the main underlying mechanisms. On the other hand, Augusto et al. (2008) reported that WA application only increased stand production in organic boreal soils, and that it should primarily be considered a liming treatment. However, most authors agree that the effects BC or WA are not universally beneficial (Augusto et al., 2008; Novak et al., 2010; Jeffery et al., 2011; Reid and Watmough, 2014). Therefore, whether WA and BC act as sources or sinks for nutrients and toxic compounds depends on, as pointed out by Clough et al. (2013) and Malara and Oleszczuk (2013), (i) feedstock material, (ii) burning processes, (iii) soil characteristics such as pH, surface area, porosity, cation exchange capacity, (iv) transfer of nutrients into the amended soil, (v) vegetation (Schiemenz et al., 2011), and (vi) climate.
In the present study, we examined the short-term response (during the first 3 years after application) of the soil-water-plant environment in two forests, to address the following objectives: (1) evaluate if application of WA and BC increase plant nutrient uptake from forest soils improving the nutritional status of the vegetation, (2) evaluate if these products, mainly at high doses, induce toxicity in the soil-water-plant system, and (3) evaluate if WA and BC increase the SOC stocks in temperate forests.
2. Materials and methods
2.1. Biochar and wood ash production
Biochar was produced by pyrolysis (450°C) of Myscanthus sp. in a Pyreg® pyrolysis unit. WA was produced by combustion of Pinus radiata D. Don harvest residues in a commercial biomass boiler. The type of WA used in this study was bottom ash. The chemical characteristics of the WA and BC used are summarized in Table 1.
2.2. Experimental design and study sites
This study was conducted in two experimental sites located on the Atlantic side of the Basque country (northern Iberian Peninsula). The sites are both - characterized by a mean annual temperature of 10.5°C and a mean annual precipitation of around 1,200 mm (EUSKALMET, 2012–2014).
The treatments consisted of spreading different doses of BC and WA (calcium equivalent) on the soil surface, with and without nitrogen. The product was spread on the topsoil. Biochar doses of 3.5, 10, and 20 Mg ha–1 corresponded to low BC(L), intermediate BC(I), and high BC(H) treatments, respectively, although these treatments were not applied at both sites (see below). Wood ash doses of 1.5, 4.5, and 9 Mg ha–1 of WA corresponded to treatments WA(L), WA(I), and WA(H). Nitrogen [115 Kg ha–1 of nitrogen (N) in the form of ammonium nitrate] was applied with the intermediate doses [BC(I) and WA(I)] to produce treatments BC(I)N and WA(I)N. Control (Ctrl) treatment, with no addition of any compound, was also considered.
Two experimental sites (ES) were established in different soil types, and four treatments WA(I), WA(I)N, BC(I) and BC(I)N plus the control were common in both. The Karrantza experimental site (ES-K) was established in May 2012, in a 25 years old Pinus radiata D. Don stand, in the Karrantza valley (at 280 m a. s. l, 30N ETRS89 475081, 4786389). The soil was characterized as a Typic Udorthent (Soil Survey Staff, 2014) (Table 2), with a 3.9% SOC content, C:N ratio of 14.4 and pH 4.8. Two additional treatments, BC(L) and WA(L), were applied in this site. The high dose treatments BC(H) and WA(H) were not applied. A complete randomized block design with three blocks running perpendicular to the slope (25%) was established. In each block there were three plots per treatment. The size of each plot was 8 × 8 m. A buffer distance of at least 10 m was left between plots. Therefore, in each block there were 21 plots.
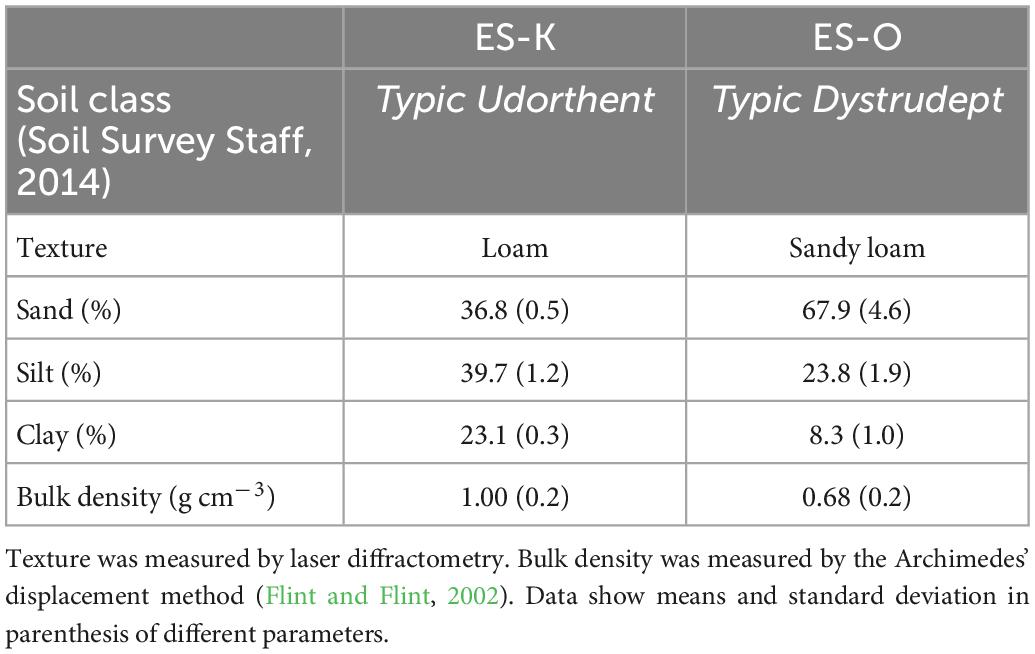
Table 2. General characterization of the soil in each experimental site of Karrantza and Oiz (ES-K and ES-O).
The second experimental site (ES-O) was established in September 2013 on the southern slope of the Oiz Mountain (760 m a. s. l. 30N ETRS89 532673, 4785572) in a 2 years old Quercus pyrenaica Willd. plantation mainly covered by shrubs and grass. The soil was characterized as a Typic Dystrudept (Soil Survey Staff, 2014; Table 2). It contains a 10.8% of SOC characterized by a C:N ratio of 16.8 and a pH 3.8. Two additional treatments, BC(H) and WA(H), were applied in this site. The low dose treatments BC(L) and WA(L) were not applied. Four plots per treatment were established in a complete randomized design. The size of the plots in ES-O was 3 × 3 m, leaving a buffer distance of at least 1 m between plots.
2.3. Sampling, processing, and analysis
2.3.1. Soil sampling, processing, and analysis
In ES-K, the soil was sampled 6, 18, and 30 months after treatment application, always in November. In ES-O the first soil sampling was performed 6 months after treatment application, and the following two sampling periods 15 and 26 after treatment application, in November too. Samples of soil (upper 10 cm after separated the forest floor layer by hand) were collected at ten randomly selected points in each plot, with a gouge auger (D = 2 cm). The soil samples were air dried, sieved (2 mm) and stored dry at room temperature until analysis. In the case of the ES-K, samples of the three plots per block were compiled, resulting in three composite samples per treatment.
Soil pH was determined in water (1:2.5). Total carbon, hydrogen and total nitrogen were determined in a LECO TruSPEC® CHN-S elemental analyzer. The total carbon was considered equivalent to the soil organic carbon (SOC) content, due to the absence of carbonates in both soils. The chemical composition (pseudo-total amounts of P, K, Mg, Ca, Ni, Cd, Cr, and Pb) was measured by ICP-AES with CCD (Varian, Inc., California, CA, USA). The samples were previously digested by acidic digestion (mixture of nitric-perchloric acid, 85–15%) (Croisé et al., 1999). Nutrient and metal contents in soil were converted to absolute values (kg ha–1) considering the bulk density of each sampling unit. Bulk density was measured by the Archimedes’ displacement method (Flint and Flint, 2002).
2.3.2. Vegetation sampling, processing, and analysis
Aboveground part of the vegetation present in the studied sites was sampled to determine biomass production and available nutrient content via plant uptake. In ES-K, the understorey vegetation and tree foliage were sampled 6, 18, and 30 months after treatment application. In ES-O only aboveground biomass of understorey vegetation was sampled (trees in ES-O were too young for foliage sampling) 15 and 26 months after treatment application.
Foliage samples were collected from the trees in each experimental plot in ES-K. One hundred needles were randomly collected from randomly selected branches bearing current-year needles. In both sites, samples of understorey vegetation were collected from a randomly selected 50 × 50 cm quadrat in each plot. Before analysis, the foliar and vegetation samples were oven-dried (70°C) to a constant weight, milled (0.50 mm) and stored dry at room temperature for nutrient analysis. In the case of the ES-K, as performed with soil samples, samples of the three replicate plots (same treatment) within the same block were mixed to perform composite samples per block, resulting in three composite samples per treatment.
Dry weights of oven dried understorey biomass and needle samples were recorded and converted to production units: kg ha–1 in the case of understorey biomass, and kg 100 needle–1 for pine tree foliage. Nutrient and metal content in aboveground biomass (tree foliage and understorey biomass, P, K, Mg, Ca, Ni, Cd, Cr, and Pb) were determined by ICP-AES with CCD (Varian, Inc., California, CA, USA). The samples were previously digested by acidic digestion (mixture of nitric-perchloric acid, 85–15%) (Croisé et al., 1999). Nutrient and metal contents in soil were converted to absolute values (kg ha–1, for understorey biomass) considering the production units.
2.3.3. Pore water sampling, processing, and analysis
Water from soil pores was sampled twice after treatment application. The first sampling was performed after the first prolonged winter rainfall after treatment application, after 8 months in ES-K and after 4 months in Es-O, and the second sampling was performed 32 months after treatment application in ES-K, and 17 months after in ES-O. Pore water was extracted using MacroRhizon™ samplers (Rhizosphere Research Products, Wageningen, Netherlands) inserted at 30 cm depth and connected via 50 ml disposable syringes. The samples were frozen until analysis, 30 ml per sample.
The pore water samples were subjected to eco-toxicity screening. Two commercial microbial bioassays were used: a growth assay (MARA, Microbial Assay for Risk Assessment) with the first pore water samples collected and a bioluminescence assay (LumiMARA) with the samples of the second pore waters collection event.
In the MARA, toxicity was evaluated by measuring inhibition of the growth of pellets formed by ten bacterial strains and one yeast inoculated with undiluted soil pore water samples, as described by Gabrielson et al. (2003). The pH of the pore water was previously adjusted and the samples prepared for inoculation as described by Wadhia et al. (2007). We examined pellet formation by colorimetry. Absorbance at 620 nm was measured in a microplate reader (Zenyth 3100. Anthos Labtec Instruments GmbH, Salzburg. Austria). Inhibition of pellet growth was assessed for each individual species, by examining the mean values of all constituent species in the test.
The LumiMARA was also performed with undiluted soil pore water samples, for toxicity screening. Eleven bioluminescent bacterial strains (nine marine and two freshwater bacteria) were incubated with pore water samples, following the manufacturer’s protocol for environmental samples (Jung et al., 2015). The toxicity was indicated by the reduction in luminescence. Light from the luminescent bacteria was measured in a microplate reader (Zenyth 3100. Anthos Labtec Instruments GmbH, Salzburg. Austria) and data were processed with the LumiMARA software (NCIMB Ltd., Bucksburn, Aberdeen. UK).
The pore water samples were treated with nitric acid prior to chemical analysis. The chemical composition (P, K, Mg, Ca, Ni, Cd, Cr, and Pb concentrations) of pore water in the first sampling was determined by simultaneous inductively coupled plasma atomic emission spectrometry (ICP-AES) with charge coupled devices (CCD) (Varian, Inc., California, CA, USA).
2.4. Statistical analysis
Linear mixed-effects models (LMMs) were used through REML (Restricted Maximum Likelihood) using lme4 v.1.1-30 (Bates et al., 2015) and lmerTest v.3.1-3 (Kuznetsova et al., 2017) packages, to investigate the effects of treatments (fixed effect) on each soil parameter, and each experimental site was analyzed separately.
Multiple models have been adjusted for each of the data sets (soil, needles, understorey biomass) in which treatment, time and interaction have been included as fixed factors, and block as random factor. On the other hand, multiple models have been adjusted for the pore water dataset where treatment and block have been included as a fixed factor.
For each of the models, the overall effects of each factor have been analyzed using a type III ANOVA. A post-hoc pairwise comparison of the estimated marginal means (EMM) with the emmeans package v.1.7.5 (Lenth et al., 2022) has been performed to identify significant differences between each treatment and its respective control. Differences were considered significant at P < 0.05.
3. Results
3.1. Soil pH and soil organic carbon
Figure 1 shows the pH in soil of the two experimental sites: ES-K and ES-O. A treatment effect is observed (F = 9.13, df = 6, p < 0.001). Soil pH increased in ES-K only when treated with BC(I)N (median values of 5.2 and 4.96, in month 6 and 18, respectively), over Ctrl (medians of 4.94 and 4.64, months 6 and 18) although pH values equated at the end of the research period (Figure 1C). In ES-O application of high doses of WA and BC [WA(H) and BC(H)], increased soil pH (Figure 1; F = 2.86, df = 6, p = 0.02). Being the medians of Ctrl of 3.72 and 3.96 in the first two sampling times and of 3.89 and 4.24 for WA(H) and of 3.88 and 4.17 for BC(H) (months 5 and 15, respectively). In ES-K, BC affected SOC content (F = 3.03, df = 6, p = 0.02). BC(I)N presented a significant increase of 34% over control [estimated marginal mean (emm) of 12.1 ± 0.77 Mg⋅ha–1]. In ES-O, only the highest dose of BC increased in a 42% SOC content over control (emm of 38.3 ± 2.95 Mg⋅ha–1; F = 3.4, df = 6, p = 0.01).
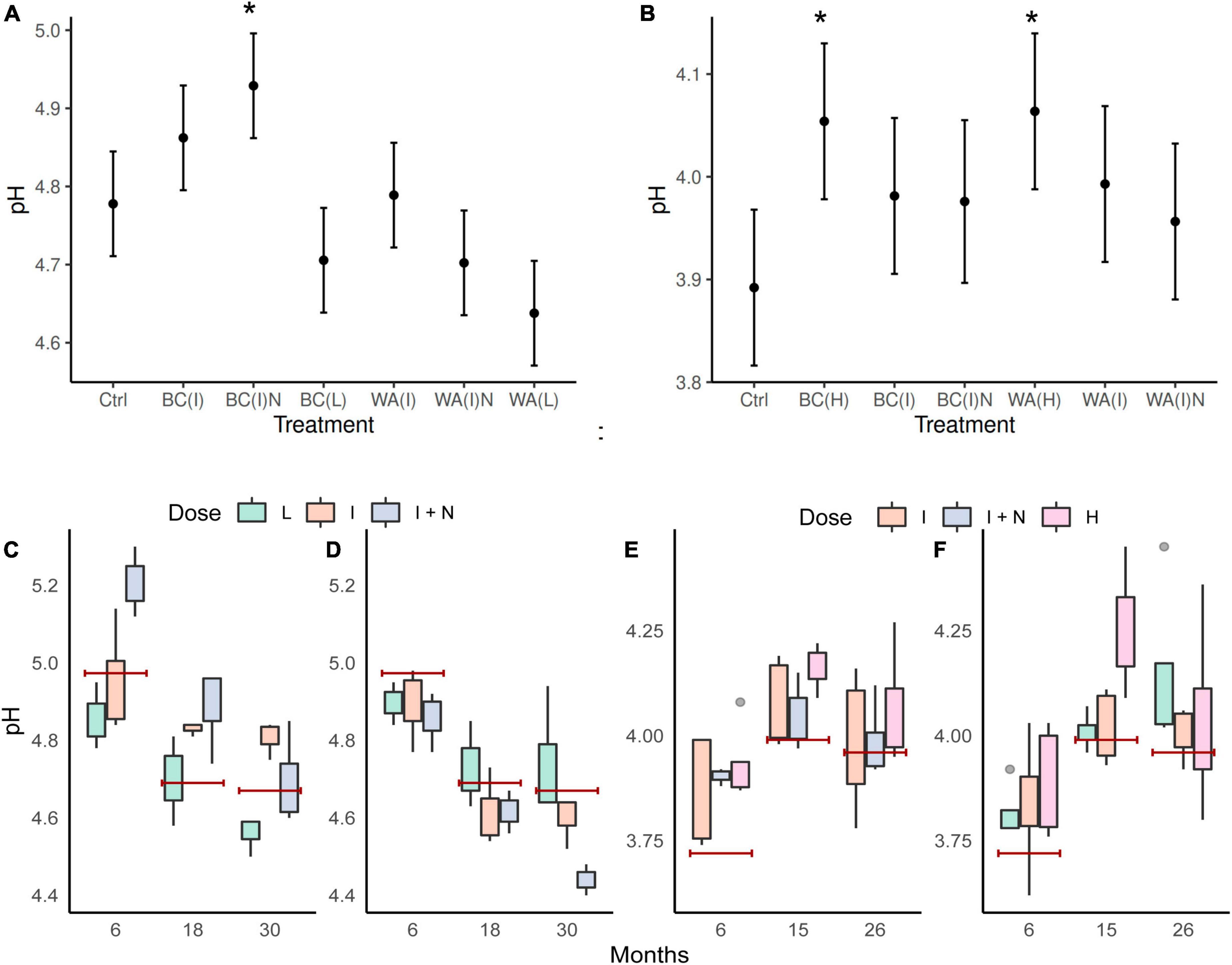
Figure 1. Representation of estimated Marginal Means (EMM) of soil pH (top) by treatment and experimental site: Experimental site of Karrantza [ES-K, (A) and Experimental site of Oiz (ES-O, (B)], and boxplots showing soil pH values of each experimental site (bottom): ES-K (C,D) and ES-O (E,F) during time (X-axis). Values for biochar (BC) treatments are represented by boxplots c and e, and wood ash (WA) treatments are represented by boxplots (D,F). EMM of control is represented in the boxplots by red lines, to provide a visual comparison. High dose (H, purple), intermediate dose (I, pink) and low dose (L, green); 0.8% of N (always with intermediate rate), (I + N, blue). Boxes show the density data within the first to the third quartile of the dataset for each treatment. The horizontal line inside the box represents the median value. The upper and lower whiskers extend from the higher to the lower pH data. Means which differ significantly from control are in bold and mark with asterisks (*p < 0.05).
3.2. Nutrients in the soil-water-plant system
Table 3 summarizes treatment effects, estimated marginal means of nutrient content in soil (control), and the differences of each treatment.
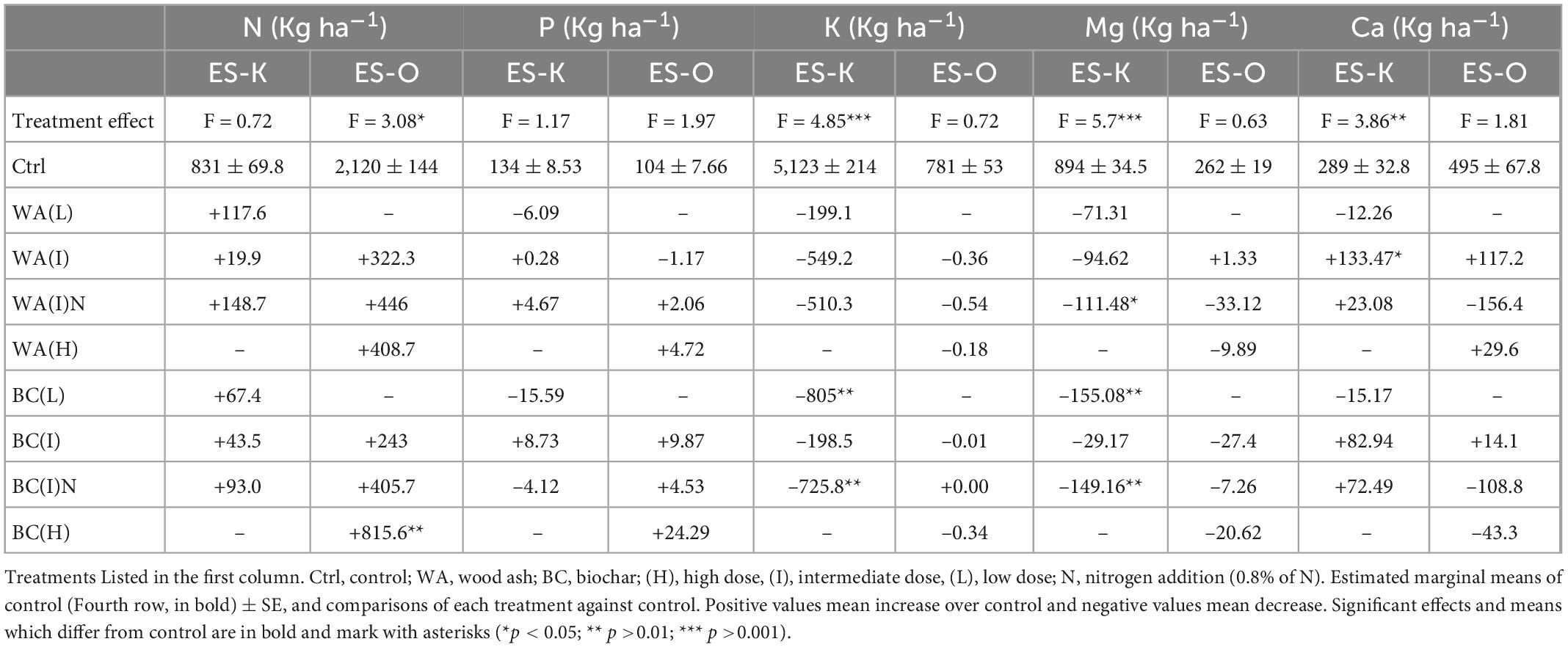
Table 3. Effects of the treatments (Third row) in soil nutrients, reported by F-values of the ANOVAs (df = 6).
Biochar(I) treated soils in ES-O present significantly superior values of K content in understorey biomass (medians of 431 and 841 Kg ha–1, for months 15 and 26, respectively), if compared to control (1,001 and 1,020 Kg ha–1 months 15 and 26; Figure 2; t-ratio = –3.085, df = 39, p = 0.05), although no evidence of treatment effect has been found (Supplementary Table 2). The rest of the treatments did not affect understorey biomass nutrient content in none of the experimental sites (Supplementary Table 2). Nutrient content in needles was not affected either, by none of the treatments (Supplementary Table 3).
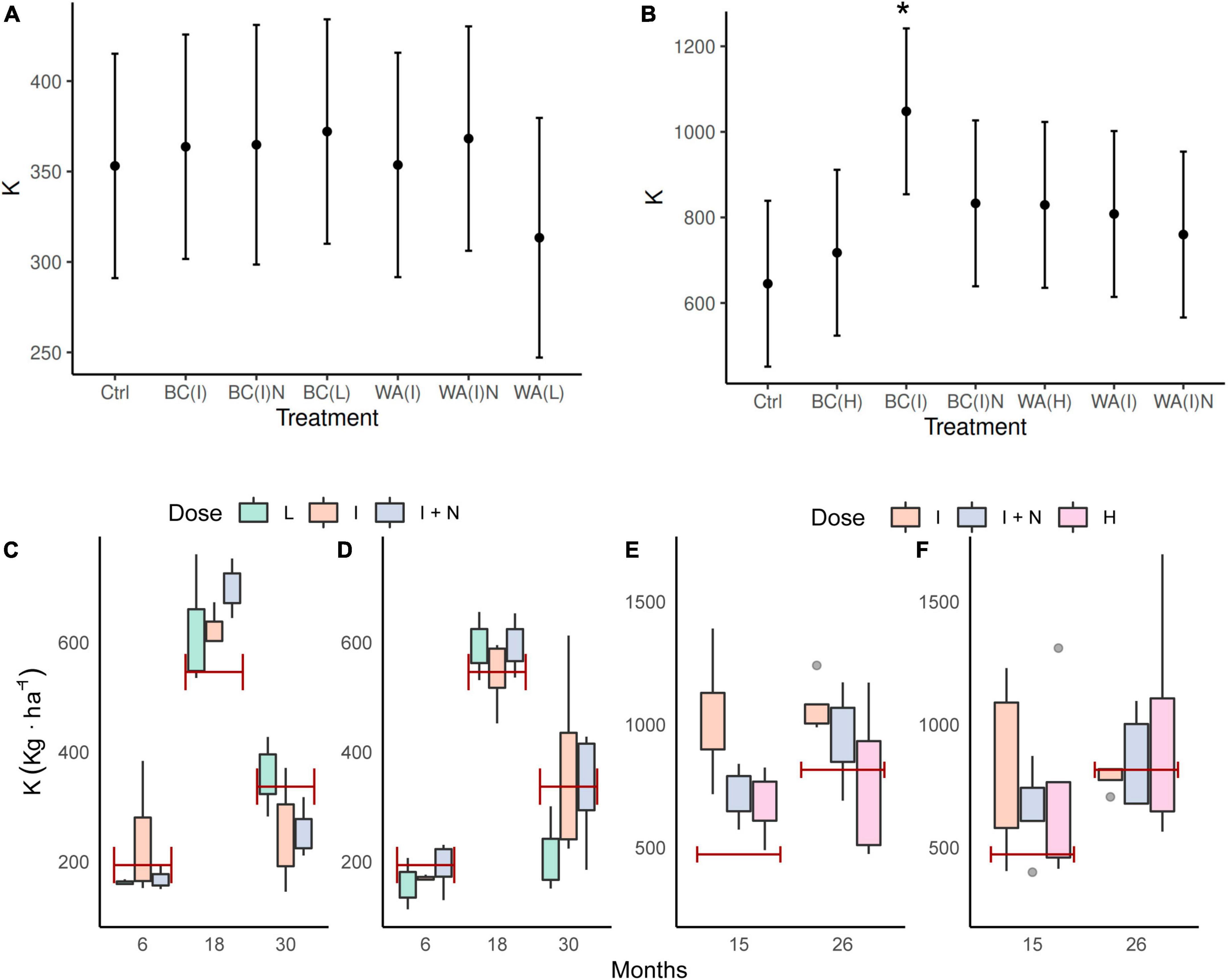
Figure 2. Representation of Estimated Marginal Means (EMM) of K content (Kg ha–1) in understorey biomass (top) by treatment and experimental site: Experimental site of Karrantza [ES-K, (A)] and Experimental site of Oiz [ES-O, (B)], and boxplots showing K content (Kg ha–1) of understorey biomass at each experimental site: ES-K (C,D) and ES-O (E,F) during the time (X-axis). Values for biochar (BC) treatments are represented by boxplots (C,E), and wood ash (WA) treatments are represented by boxplots (D,F). EMM of control is represented in the boxplots by red lines, to provide a visual comparison. High dose (H, purple), intermediate dose (I, pink) and low dose (L, green); 0.8% of N (always with intermediate rate), (I + N, blue). Boxes show the density data within the first to the third quartile of the dataset for each treatment. The horizontal line inside the box represents the median value. The upper and lower whiskers extend from the higher to the lower K content data. Means which differ significantly from control are in bold and mark with asterisks (*p < 0.05).
Pore waters did not contain differences in macronutrient content between treated and not treated samples, in none of the experimental sites (Supplementary Table 4).
3.3. Heavy metals in the soil-water-plant system
Figure 3 shows the Cd in soil of the ES-K and the ES-O, and changes observed in BC treated plots in ES-K, e.g., medians of 0.13, 0.17, and 0.11 g⋅ha–1 in month 30 [BC(L), BC(I), BC(I)N, respectively], significantly lower (F = 3.29, df = 6, p = 0.01) than control (e.g., 0.2 g⋅ha–1, month 30). No more changes in heavy metal content were observed in none of the soils after application of any treatment (Supplementary Table 1).
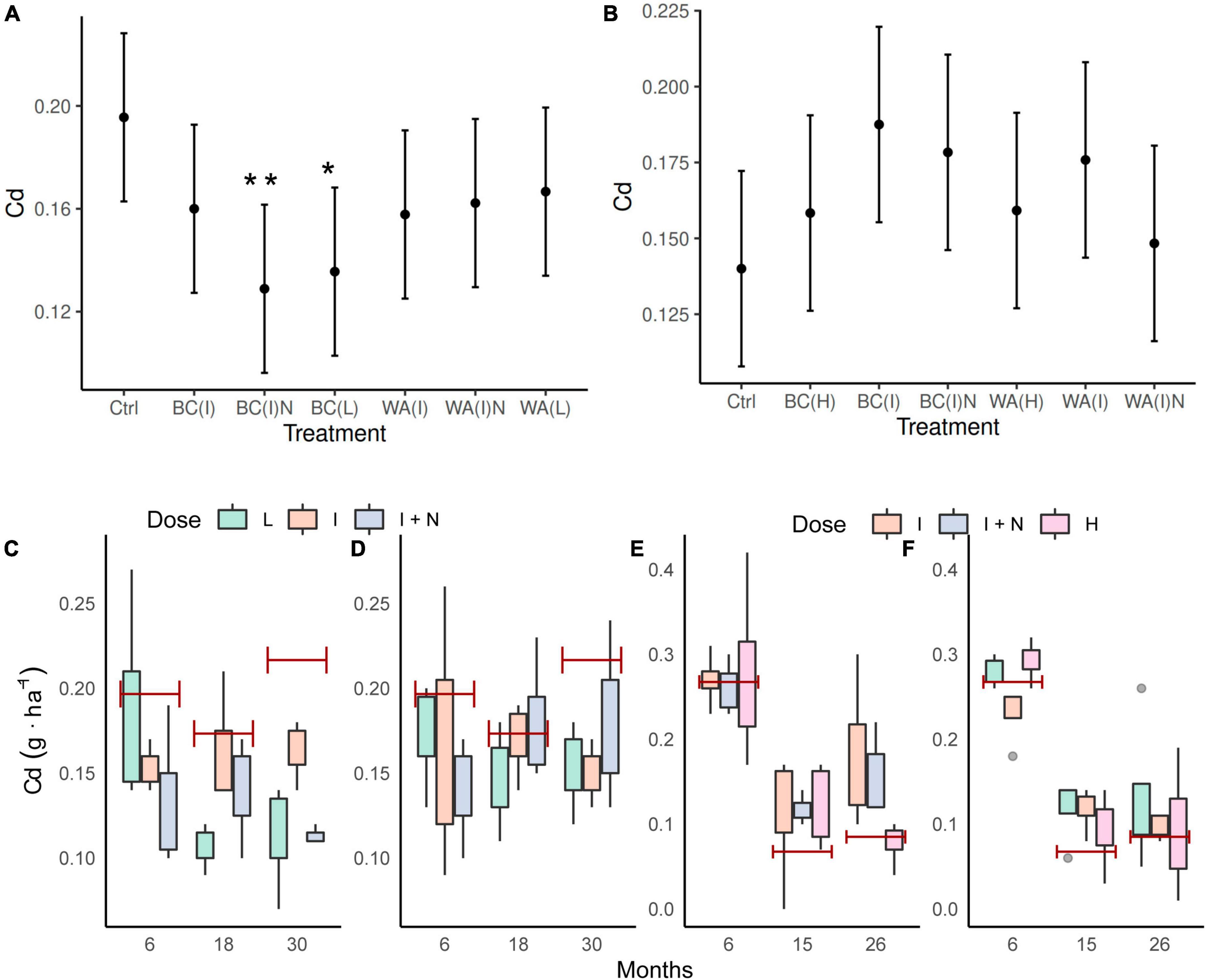
Figure 3. Estimated Marginal Means (EMM) of Cd content (g⋅ha–1) in soil (top) in ES-K soil (A) and in ES-O (B), and boxplots showing Cd content in soil at each experimental site: ES-K (C,D) and ES-O (E,F) during the time (X-axis). Values for biochar (BC) treatments are represented by boxplots c and e, and wood ash (WA) treatments are represented by boxplots (D,F). EMM of control is represented in the boxplots by red lines, to provide a visual comparison. High dose (H, purple), intermediate dose (I, pink) and low dose (L, green); 0.8% of N (always with intermediate rate), (N, blue). Boxes show the density data within the first to the third quartile of the dataset for each treatment. The upper and lower whiskers extend from the higher to the lower Cd content data. Means which differ significantly from control are in bold and mark with asterisks (*p < 0.05; **p > 0.01).
Understorey biomass from ES-K increased 1.5 times Ni content after BC(I)N application (F = 16.01, df = 6, p < 0.001) if compared with Crtl (emm of 259 ± 41.4 g⋅ha–1). No more effects were observed in aboveground biomass heavy metal content (Supplementary Tables 2, 3).
Pore waters collected from BC(L) treated plots in ES-K, presented an estimated marginal mean of 0.06 ± 0.01 mg Mn cm–3, 6 times higher than Ctrl (0.01 mg⋅ha–1). No more effects were observed in pore waters after treatment application (Supplementary Table 4).
3.4. Ecotoxicity testing
WA and BC did not induce toxic effects in the pore waters (Figure 4). The MARA indicated that application of WA and BC caused very low growth inhibitions rates that were not different from those in the control treatments. The LumiMARA showed that application of WA to ES-K yielded significantly greater reduction of luminescence in plots amended with WA(L) than in control plots. However, the overall mean inhibition of luminescence and growth did not exceed the 40% in either of the experimental sites.
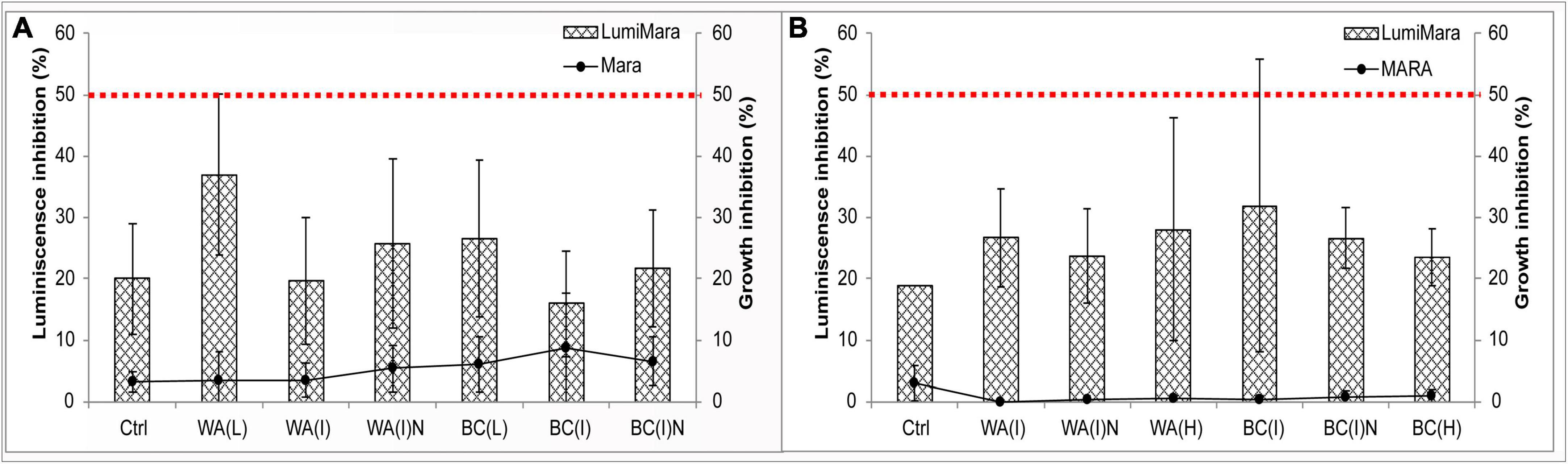
Figure 4. Effect of the treatments (Ctrl, control; BC, biochar; WA, wood ash; (H), high dose; (I), intermediate dose; (L), low dose; N = 0.8% of N) on bioluminescence inhibition (left y axis) measured with LumiMARA (bars) and pellet mean growth inhibition represented by dots (right y axis) determined by the MARA bioassay. Treatment effect was analyzed for both experimental sites: ES-K (A) on the left side, and ES-O (B) soil on the right side. Red flashing line (dotted red line) represents the 50% of growth and luminescence inhibition threshold for being toxic).
3.5. Plant biomass growth
No significant differences were observed in understorey biomass or needle production in relation to WA and BC application (Supplementary Figure 1).
4. Discussion
4.1. Vegetation response to soil amendments
The increase in K uptake by plants observed in ES-O after BC application suggests a fertilization effect of BC, as reported by other authors (e.g., Ingerslev et al., 2014). Contrary, when WA was applied to ES-O, even though the total content of K was greater in WA than in BC (Table 1), no increased K uptake by plants was observed after application of WA to the ES-O. These differences in nutrient release from WA and BC might be complex, due to chemistry of the product (Verheijen et al., 2009) and its interaction with soil biota (e.g., Anderson et al., 2011) or organo-mineral associations (Fernández-Ugalde et al., 2017). Buss et al. (2019) suggested an improved fertilizing effect of biochar, derived from a moderate supply of K instead of instant leaching of K from WA. The increased K uptake by plants observed after BC application was not proportional to the dose applied, contrary to what observed by many authors (Clapham and Zibilske, 1992; Demeyer et al., 2001; Park et al., 2012). This suggests that the highest doses of both WA and BC applied to the Typic Dystrudept may have resulted in formation of a thick amendment layer on top of the soil, as reported in some studies after forest fires (Bodí et al., 2014) or may have prolonged changed the physicochemical properties of the soil (Brais et al., 2015) thus affecting the processes taking place in the soil, as a different dose-depended response was observed in soil too, in ES-O. Indeed, the system in ES-O is probably adapted to make use of K more efficiently (Cornut et al., 2021), as K was efficiently taken up by plants and prevented its loss via leaching, despite the low energy of adsorption of K (Brais et al., 2015). This is consistent with some studies analyzing the effects of wood ash, were increase in exchangeable K concentration in the forest floor was reported, and revealed its fertilizing potential in relation to stand growth in K deficient forests (Augusto et al., 2008; Solla-Gullón et al., 2008; Brais et al., 2015).
However, we did not observe biomass growth after the increased nutrient content in biomass. A review on the effects of BC application in forest ecosystems (Li et al., 2018) concluded that BC addition alone is not sufficient to meet the nutrient needs for tree growth and productivity. In fact, in an analysis of P radiata D. Don stands in the Basque Country, most of the soils were found to be deficient in P, and fertilization with between 20 and 150 Kg P ha–1 was recommended (Martínez de Arano, 1999). Considering that in our field experiment the largest dose applied was 31.6 Kg P ha–1 (treatment BC(H) in ES-O) and that Martínez de Arano (1999) recommended 50–100 Kg P ha–1 for this type of soil, pine growth was probably limited by lack of P. It is therefore important to know which are the limiting nutrients in each soil, as well as to identify the needs of the system itself.
The observed increase in Ni uptake by understorey plants in ES-K when BC(I)N was applied is another example of the complexity of the interactions that occur after the soil amendments have been applied. Even though BC(I)N contains less Ni than WA(I) and BC(I), it is the only treatment in ES-K that directly contributes to Ni uptake by understorey biomass in ES-K. This could be due to the increase in soil pH observed. Ni absorption usually increases with increasing pH up to five (Pandaa et al., 2007). It could be also linked to the decrease in Mg, Cd, and K content observed in soil and subsequent reduction of their inhibitory effect on the absorption and translocation of Ni2+ from roots to shoots (Temp, 1991), or probably, it could be due to the interaction of the mentioned reasons above. However, more research and time is needed in order to prevent toxicity, since Yusuf et al. (2011) suggested that Ni is a high mobile trace metal that tends to accumulate in newly formed plant parts as well to seeds. Ni uptake and accumulation in plants depend on plant species, soil pH, quantity, and form of Ni present in the soil (Hassan et al., 2019). Still more research is needed to understand all processes, since Ni, in contrast to other toxic heavy metals like cadmium, lead, mercury, copper and chromium has received little attention from plant scientists due to its dual character (essential-toxic) and complex electronic chemistry which is a major hurdle in disclosing its toxicity mechanism in plants (Yusuf et al., 2011).
Although nutrition and toxicity are key factors determining plant growth, the yearly fluctuations in biomass growth suggest that water availability is a more constraining factor than nutrients in these ESs, as the variations in needle and understorey biomass production in ES-K (Supplementary Figure 1). Table clearly coincide with the variations in annual rainfall: 1,413 mm in 2013, 978 mm in 2012 and 945 mm in 2014 (data obtained by the closest meteorological stations [(<10 km), EUSKALMET, 2012–2014]. A positive net primary productivity-precipitation linear relationship has been widely recognized across many ecosystem types (Sala et al., 2012; Felton et al., 2021). The distribution of rainfall will be increasingly variable and possibilities for the disappearance of this linearity are expected as response of complex interactions (e.g., Felton et al., 2021). It is therefore important, from an ecosystem resilience point of view, to increase water holding capacity if needed, in order to maintain as much as possible the soil moisture during drought periods. BC has widely considered enhancer of the hydraulic properties due to its porosity and soil structuring capacity. However, Moragues-Saitua et al. (2017) did not observe any increase in water holding capacity after 1–2 years in the same experiment.
4.2. Soil response to amendments
The increase in soil pH observed after WA and BC application may just have been too small to increase nutrient uptake by plants. Even considering WA or BC simply as liming treatments for temperate forest soils (Augusto et al., 2008), our findings show that these by-products cannot be generally applied, at least in the short term. As many authors (e.g., Augusto et al., 2008; Godlewska et al., 2021) have mentioned, the effects of amendments are soil and dosage dependent (Hansen et al., 2017), and for example large quantities of BC must be applied in order to detect any increase in pH in the mineral layers of the Typic Dystrudept. This places in doubt the overall effectiveness of BC or WA for liming temperate forest soils in areas with high precipitation rates. Some authors have reported that WA application affects mainly the pH of the topsoil, being the depth of the effect largely dosage dependent (Jacobson et al., 2004; Hansen et al., 2017). Further research observing effects on the organic layers are also needed to complement this type of studies, since these are the horizons that are in direct contact with the amendment and the local changes can lead to effects on the overall ecosystem functioning (Hansen et al., 2017).
Biochar increased SOC stocks in both experimental sites, although the effect varied according to the site and treatment. In the case of ES-K, the increase in SOC was observed when BC(I)N was applied, while in ES-O the same treatment had no effect and the increase in SOC in the highest doses was accompanied by an increase in nitrogen content. This suggests different organic matter dynamics in both sites. Depending on soil properties and characteristics of the site, the biotic community present varies and together determine the pathway that SOM decomposition and transformation follows on each site (Prescott and Vesterdal, 2021). The release of compounds from WA and BC in the field is subjected to many variables on a site-to-site basis, with time since application also playing an important role (Reid and Watmough, 2014). Zhang et al. (2017), Knoblauch et al. (2010), Wardle et al. (2008) concluded that BC or WA addition increases leaf litter and deadwood decomposition at the soil surface, enhancing soil microbial biomass and activity, and thus, increasing the mean concentration of DOC (Gömöryová et al., 2016) that would be translocated through the soil profile. On the other hand, in ES-K, the increase in SOC may be due to the contribution of C through direct incorporation of the biochar in the mineral layer. Fernández-Ugalde et al. (2017) investigated the incorporation of BC-derived C (C4 type plant feedstock) into various particle-size fractions and examined the effect of BC on the storage of total OC in the particle-size fractions in the Karrantza experimental site (ES-K, dominated by C3 type Pinus radiata). These authors observed that in the first year, part of the BC spread on the field was degraded and incorporated into the mineral soil. Although increased SOC did not affect plant responses during this field experiment, it may have a long-term fertilization effect (Bot and Benites, 2005) and could contribute to mitigating climate change via sequestration of SOC if the incorporation and stabilization rate of SOC in the mineral soil layers is higher than the decomposition rate occurring in the organic layer of the soil. Addition of biochar could also contribute to soil health, soil structure (Six et al., 2004), water holding capacity and other processes (Bronick and Lal, 2005; Moragues-Saitua et al., 2017) that are important for enabling ecosystems to adapt to climate change.
In ES-K, the application of both BC and WA seem to mobilize Cd, K, and Mg in soil, since less content of this nutrients have been observed after applying the amendments. The fate of these elements remains unknown as the concentrations did not increase in any of the fractions analyzed (needles, understorey o pore waters) Some of these nutrients could have accumulated in other parts of the plants, such as roots. However, it is possible that they could have been translocated deeper into the soil adsorbed to DOC (Loganathan et al., 2012) or to the negatively charged small fractions of BC and then translocated deeper into the soil, since Cao et al. (2021) observed that the transport of Cd2+ is facilitated in the cotransport of BC.
4.3. Water responses to soil amendments
Pore water analysis and ecotoxicological tests did not provide any evidence of toxicological effects at either of the experimental sites. However, some studies have reported leaching and increased bioavailability of hazardous trace elements after application of BC (Kloss et al., 2015) or WA (Williams, 1997; Ingerslev et al., 2014). Since we observed increased Mn content in pore waters of plots treated with BC(L) in ES-K, this could indicate a potential availability of this toxic element, it is important to conduct long-term field experiments to ensure that substances of this nature are not harmful to water and soil resources or to the entire ecosystem. This is essential for the safe application of BC or WA to soil.
In summary, this research provides further information regarding the effectiveness of using by-products of biomass-based energy production as soil amendments in temperate forests.
The amendments applied do not seem to be toxic or harmful in the two types of forest plantations under study, as revealed by MARA and LumiMARA testing with pore water samples. Concerns about the risks of increase in Ni mobility and availability derived from BC(I)N application are highlighted, although this did not limit the growth of understorey biomass.
These findings show that the addition of biochar and wood ash to temperate humid forest soils affects different sites in different ways depending on the soil type and system, as well as on application rates and whether nitrogen was added. Biochar addition increased K uptake by plants only in ES-O and with the intermediate rate. The biggest rates increase SOC content and pH of the Typic Dystrudept, and similar effects were observed in the Typic Udorthent, but with the intermediate dose combined with N. Application of WA contributed to increase soil pH in the Typic Dystrudept, but not of the Typic Udorthent, and only when high doses were applied. Widespread use of these by-products in forestry plantations on steep slopes in remote locations may be limited because the high cost of transporting and applying large amounts of the products that would reduce the cost-effectiveness of the treatments. On the other hand, WA or BC could be used as liming treatments, or applied to soil as a carbon sink, the effects cannot be generalized and have been shown to be depend on soil type.
It is important to conduct appropriate long-term field experiments to determine whether by-products can be used in climate change adaptation strategies, as well as to ensure that substances of this nature are not harmful to water, soil or the entire ecosystems, as this is essential for the safe application of BC or WA to soil.
Data availability statement
The raw data supporting the conclusions of this article will be made available by the authors, without undue reservation.
Author contributions
LM-S, NG-B, and AA-G contributed to conception and design of the study. GB-C and FB optimized and performed the ecotoxicology tests. LM-S organized the database and performed the statistical analysis and wrote the first draft of the manuscript. All authors contributed to manuscript revision, read, and approved the submitted version.
Funding
This study was formed part of the Charfor project (RTA2012-00048-00-00) funded by the Spanish National Institute for Agronomic Research INIA. The Karrantza experimental site was established by the Reinfforce Project Reinfforce (REsource INFrastructure for monitoring and adapting European Atlantic FORests under Changing climate; DS21 Demostration Site) funded by INTERREG IVB EA Atlantic space. The Economic Development and Competitiveness Department of the Basque Government awarded Lur Moragues Saitua a pre-doctoral scholarship. Open access publishing was covered by URLUR project (PID2019-109726RR-100) funded by the Spanish state R + D + I Program Oriented to the Challenges of Society of Society.
Acknowledgments
We thank Kevin Alconada and Ianire Santesteban for indispensable assistance with sampling and processing. We are grateful to the provincial council of Bizkaia, for providing access to the land to establish the experimental sites.
Conflict of interest
The authors declare that the research was conducted in the absence of any commercial or financial relationships that could be construed as a potential conflict of interest.
Publisher’s note
All claims expressed in this article are solely those of the authors and do not necessarily represent those of their affiliated organizations, or those of the publisher, the editors and the reviewers. Any product that may be evaluated in this article, or claim that may be made by its manufacturer, is not guaranteed or endorsed by the publisher.
Supplementary material
The Supplementary Material for this article can be found online at: https://www.frontiersin.org/articles/10.3389/ffgc.2022.878217/full#supplementary-material
References
Anderson, C. R., Condron, L. M., Clough, T. J., Fiers, M., Stewart, A., Hill, R. A., et al. (2011). Biochar induced soil microbial community change: Implications for biogeochemical cycling of carbon, nitrogen and phosphorus. Pedobiologia 54, 309–320.
Augusto, L., Bakker, M. R., and Meredieu, C. (2008). Wood ash applications to temperate forest ecosystems — potential benefits and drawbacks. Plant Soil 306, 181–198. doi: 10.1007/s11104-008-9570-z
Bates, D., Mächler, M., Bolker, B., and Walker, S. (2015). Fitting linear mixed-effects models using lme4. J. Stat. Softw. 67, 1–48.
Biederman, L. A. O., and Harpole, W. S. (2013). Biochar and its effects on plant productivity and nutrient cycling: A meta-analysis. GCB Bioenergy 5, 202–214. doi: 10.1111/gcbb.12037
Bodí, M. B., Martin, D. A., Balfour, V. N., Santín, C., Doerr, S. H., Pereira, P., et al. (2014). Earth-science reviews wildland fire ash: Production, composition and eco-hydro-geomorphic effects. Earth Sci. Rev. 130, 103–127. doi: 10.1016/j.earscirev.2013.12.007
Bot, A., and Benites, J. (2005). The importance of soil organic matter: Key to drought-resistant soil and sustained food and production. FAO soils bulletin 80. Rome: FAO.
Brais, S., Bélanger, N., and Guillemette, T. (2015). Wood ash and N fertilization in the Canadianboreal forest: Soil properties and response of jack pine and black spruce. For. Ecol. Manag. 348, 1–14.
Bronick, C. J., and Lal, R. (2005). Soil structure and management: A review. Geoderma 124, 3–22. doi: 10.1016/j.geoderma.2004.03.005
Buss, W., Jansson, S., and Masek, O. (2019). Unexplored potential of novel biochar-ash composites for use as organo-mineral fertilizers. J. Clean. Prod. 208, 960–967. doi: 10.1016/j.jclepro.2018.10.189
Cao, G., Sun, J., Chen, M., Sun, H., and Zhang, G. (2021). Co-transport of ball-milled biochar and Cd2+ in saturated porous media. J. Hazard. Mater. 416:125725. doi: 10.1016/j.jhazmat.2021.125725
Clapham, W. M., and Zibilske, L. M. (1992). Wood ash as a liming amendment. Commun. Soil Sci. Plant 23, 1209–1227. doi: 10.1080/00103629209368661
Clough, T. J., Condron, L. M., Kammann, C., and Müller, C. (2013). A review of biochar and soil nitrogen dynamics. Agronomy 3, 275–293.
Cornut, I., Le Maire, G., Laclau, J. P., Guillemot, J., Mareschal, L., Nouvellon, Y., et al. (2021). Potassium limitation of wood productivity: A review of elementary processes and ways forward to modelling illustrated by Eucalyptus plantations. For. Ecol. Manag. 494:119275. doi: 10.1016/j.foreco.2021.119275
Croisé, L., Cluzeau, C., Ulrich, H., Lanier, M., and Gomez, A. (1999). RENECOFOR -interprétation des analyses foliaires réalisées dans les 102 peuplements du réseau de 1993 a 1997 et premières évaluations interdisciplinaires. Fontainebleau: Office National des Forêts, Département Recherche et Développement, 413.
Demeyer, A., Nkana, J. C. V., and Verloo, M. G. (2001). Characteristics of wood ash and influence on soil properties and nutrient uptake: An overview. Bioresour. Technol. 77, 287–295. doi: 10.1016/S0960-8524(00)00043-2
EUSKALMET Euskal Meteorologia Agentzia (2012–2014). Meteorological annual reports. Miñano Mayor: EUSKALMET Euskal Meteorologia Agentzia
EVE (2017). Ente Vasco de la Energía. The Energy Strategy of the Basque Country 2030. Gobierno vasco, Departamento de desarrollo económico e infraestructuras. Available online at: https://www.eve.eus/EveWeb/media/EVE/pdf/3E2030/EVE-3E2030-Ingles.pdf (accessed December 30, 2022)
Felton, A. J., Knapp, A. K., and Smith, M. D. (2021). Precipitation–productivity relationships and the duration of precipitation anomalies: An underappreciated dimension of climate change. Glob. Change Biol. 27, 1127–1140. doi: 10.1111/gcb.15480
Fernández-Ugalde, O., Gartzia-Bengoetxea, N., Arostegi, J., Moragues, L., and Arias-González, A. (2017). Storage and stability of biochar-derived carbon and total organic carbon in relation to minerals in an acid forest soil of the Spanish Atlantic area. Sci. Total Environ. 587-588, 204–213. doi: 10.1016/j.scitotenv.2017.02.121
Flint, A. L., and Flint, L. E. (2002). “Particle density,” in Methods of soil analysis. Part 4. Physical methods, eds J. H. Dane and G. C. Topp (Madison, WI: Soil Science Society of America), 229–240.
Gabrielson, J., Kuhn, I., Colque-Navarro, P., Hart, M., Iversen, A., McKenzie, D., et al. (2003). Microplate-based microbial assay for risk assessment and (eco) toxic fingerprinting of chemicals. Anal. Chim. Acta 485, 121–130. doi: 10.1016/S0003-2670(03)00404-5
Godlewska, P., Ok, Y. S., and Oleszczuk, P. (2021). The dark side of black gold: Ecotoxicological aspects of biochar and biochar-amended soils. J. Hazard. Mater. 403:123833. doi: 10.1016/j.jhazmat.2020.123833
Gogoi, L., Narzari, R., Gogoi, N., Farooq, M., and Kataki, R. (2019). Biochar production and application in forest soils: A critical review. Phyton Int. J. Exp. Bot. 88, 349–635. doi: 10.32604/phyton.2019.08406
Gömöryová, E., Pichler, V., Tóthová, S., and Gömöry, D. (2016). Changes of chemical and biological properties of distinct forest floor layers after wood ash application in a norway spruce stand. Forests 7:108. doi: 10.3390/f7050108
Hannam, K. D., Venier, L., Allen, D., Deschamps, C., Hope, E., Jull, M., et al. (2018). Wood ash as soil amendment in Canadian forests: What are the barriers to utilization? Can. J. For. Res. 48, 442–450.
Hansen, M., Bang-Andreasen, T., Sørensen, H., and Ingerslev, M. (2017). Micro vertical changes in soil pH and base cations over time after application of wood ash on forest soil. For. Ecol. Manag. 406, 274–280. doi: 10.1016/j.foreco.2017.09.069
Hassan, M. U., Chattha, M. U., Khan, I., Chattha, M. B., Aamer, M., Nawaz, M., et al. (2019). Nickel toxicity in plants: Reasons, toxic effects, tolerance mechanisms, and remediation possibilities—a review. Environ. Sci. Pollut. Res. 26, 12673–12688. doi: 10.1007/s11356-019-04892-x
Ingerslev, M., Hansen, M., Pedersen, L. B., and Skov, S. (2014). Effects of wood chip ash fertilization on soil chemistry in a Norway spruce plantation on a nutrient-poor soil. For. Ecol. Manag. 334, 10–17. doi: 10.1016/j.foreco.2014.08.034
Insam, H., and Knapp, B. A. (2011). Recycling of biomass ashes. Heidelberg: Springer. doi: 10.1007/978-3-642-19354-5
Jacobson, S., Högbom, L., Ring, E., and Nohrstedt, H. Ö. (2004). Effects of wood ash dose and formulation on soil chemistry at two coniferous forest sites. Wat. Air Soil Pollut. 158:113125. doi: 10.1023/B:WATE.0000044834.18338.a0
Jeffery, S., Verheijen, F. G. A., van der Velde, M., and Bastos, A. C. (2011). A quantitative review of the effects of biochar application to soils on crop productivity using meta-analysis. Agric. Ecosyst. Environ. 144, 175–187. doi: 10.1016/j.agee.2011.08.015
Jung, Y., Park, C.-B., Kim, Y., Kim, S., Pflugmacher, S., and Baik, S. (2015). Application of multi-species microbial bioassay to assess the effects of engineered nanoparticles in the aquatic environment: Potential of a luminous microbial array for toxicity risk assessment (LumiMARA) on testing for surface-coated silver nanoparticle. Int. J. Environ. Res. Public Health 12, 8172–8186. doi: 10.3390/ijerph120708172
Kloss, S., Zehetner, F., Buecker, J., Oburger, E., Wenzel, W. W., Enders, A., et al. (2015). Trace element biogeochemistry in the soil-water-plant system of a temperate agricultural soil amended with different biochars. Environ. Sci. Pollut. Res. 22, 4513–4526. doi: 10.1007/s11356-014-3685-y
Knoblauch, C., Maarifat, A. A., Pfeiffer, E. M., and Haefele, M. S. (2010). Degradability of black carbon and its impact on trace gas fluxes and carbon turnover in paddy soils. Soil Biol. Biochem. 43, 1768–1778.
Kuznetsova, A., Brockhoff, P. B., and Christensen, R. H. B. (2017). lmerTest package: Tests in linear mixed effects models. J. Stat. Softw. 82, 1–26.
Lehmann, J., and Joseph, S. (2009). “Biochar for environmental management: An introduction,” in Biochar for environmental management: Science and technology, eds J. Lehmann and S. Joseph (London: Earthscan), 1–9.
Lehmann, J., Cowie, A., Masiello, C., Kammann, C., Woolf, D., Amonette, J., et al. (2021). Biochar in climate change mitigation. Nat. Geosci. 14:883892. doi: 10.1038/s41561-021-00852-8
Lenth, R. V., Buerkner, P., Giné-Vázquez, I., Herve, M., Jung, M., Love, J., et al. (2022). Emmeans: Estimated marginal means, aka least-squares means. R package version 1.8.3. Available online at: https://cran.r-project.org/web/packages/emmeans/emmeans.pdf
Li, Y., Hu, S., Chen, J., Müller, K., Li, Y., Fu, W., et al. (2018). Effects of biochar application in forest ecosystems on soil properties and greenhouse gas emissions: A review. J. Soils Sediments 18, 546–563. doi: 10.1007/s11368-017-1906-y
Liang, B., Wang, C., Solomon, D., Kinyangi, J., Luiz, F. J., Wirick, S., et al. (2013). Oxidation is key for black carbon surface functionality and nutrient retention in amazon anthrosols. Bri. J. Environ. Clim. Change 3, 9–23. doi: 10.9734/BJECC/2013/2267
Loganathan, P., Vigneswaran, S., Kandasamy, J., and Naidu, R. (2012). Cadmium sorption and desorption in soils: A review. Crit. Rev. Environ. Sci. Technol. 42, 489–533.
Malara, A., and Oleszczuk, P. (2013). Application of a battery of biotests for the determination of leachate toxicity to bacteria and invertebrates from sewage sludge-amended soil. Environ. Sci. Pollut. R. 20, 3435–3446. doi: 10.1007/s11356-012-1268-3
Martínez de Arano, I. (1999). Diagnóstico nutritivo y necesidades de fertilización de plantaciones de Pinus radiata de bizkaia y Araba. Final Report. Project founded by the I+D/1999 call of the (Decreto 367/1995). Vitoria-Gasteiz: Departament of Agriculture and fisherie of the Basque Goberment.
Moragues-Saitua, L., Arias-González, A., and Gartzia-Bengoetxea, N. (2017). Effects of biochar and wood ash on soil hydraulic properties: A field experiment involving contrasting temperate soils. Geoderma 305, 144–152.
Novak, J. M., Busscher, W. J., Watts, D. W., Laird, D. A., Ahmedna, M. A., and Niandou, M. A. S. (2010). Short-term CO2 mineralisation after additions of biochar and switchgrass to a typic Kandiudult. Geoderma 154, 281–288. doi: 10.1016/j.geoderma.2009.10.014
Oleszczuk, P., Josko, I., and Kusmierz, M. (2013). Biochar properties regarding to contaminants content and ecotoxicological assessment. J. Hazard. Mater. 260, 375–382. doi: 10.1016/j.jhazmat.2013.05.044
Olsson, B. A., Åkerblom, S., Bishop, K., Eklöf, K., and Ring, E. (2017). Does the harvest of logging residues and wood ash application affect the mobilization and bioavailability of trace metals? For. Ecol. Manag. 383, 61–72. doi: 10.1016/j.foreco.2016.09.017
Pandaa, G., Dasa, S., Bandopadhyayb, T., and Guha, A. (2007). Adsorption of nickel on husk of Lathyrus sativus: Behavior and binding mechanism. Colloids Surf. B Biointerfaces 57, 135–142. doi: 10.1016/j.colsurfb.2007.01.022
Park, N. D., Rutherford, P. M., Thring, R. W., and Helle, S. S. (2012). Chemosphere wood pellet fly ash and bottom ash as an effective liming agent and nutrient source for rye grass (Lolium perenne L.) and oats (Avena sativa). Chemosphere 86:427432. doi: 10.1016/j.chemosphere.2011.10.052
Pitman, R. (2006). Wood ash use in forestry. A review of the environmental impacts. Forestry 79, 563–588. doi: 10.1093/forestry/cpl041
Prescott, C. E., and Vesterdal, L. (2021). Decomposition and transformations along the continuum from litter to soil organic matter in forest soils. For. Ecol. Manag. 498:119522. doi: 10.1016/j.foreco.2021.119522
Pugliese, S., Jones, T., Preston, M. D., Hazlett, P., Tran, H., and Basiliko, N. (2014). Wood ash as a forest soil amendment: The role of boiler and soil type on soil property response. Can. J. Soil Sci. 94, 621–634. doi: 10.4141/cjss-2014-037
Reid, C., and Watmough, S. A. (2014). Evaluating the effects of liming and wood-ash treatment on forest ecosystems through systematic meta-analysis. Can. J. For. Res. 44, 867–885. doi: 10.1139/cjfr-2013-0488
Reimann, C., Ottesen, R. F., Andersson, M., Arnoldussen, A., Koller, F., and Englmaier, P. (2008). Element levels in birch and spruce wood ashes – green energy? Sci. Total Environ. 393, 191–197. doi: 10.1016/j.scitotenv.2008.01.015
Sala, O. E., Gherardi, L. A., Reichmann, L., Jobbagy, E., and Peters, D. (2012). Legacies of precipitation fluctuations on primary production: Theory and data synthesis. Philosoph. Trans. R. Soc. B Biol. Sci. 367, 3135–3144. doi: 10.1098/rstb.2011.0347
Schiemenz, K., Kern, J., Paulsen, H. P., Bachmann, S., and Eichler-Löbermann, B. (2011). “Phosphorus fertilizing effects of biomass ashes,” in Recycling of biomass ashes, eds H. Insam and B. A. Knapp (Heidelberg: Springer), 17–31. doi: 10.1007/978-3-642-19354-5_2
Six, J., Bossuyt, H., Degryze, S., and Denef, K. (2004). A history of research on the link between (micro)aggregates, soil biota, and soil organic matter dynamics. Soil Tillage Res. 79, 7–31. doi: 10.1016/j.still.2004.03.008
Soil Survey Staff (2014). Keys to soil taxonomy, 12th Edn. Washington, DC: USDA-Natural Resources Conservation Service.
Solla-Gullón, F., Santalla, M., Pérez-Cruzado, C., Merino, A., and Rodríguez-Soalleiro, R. (2008). Response of Pinus radiata seedlings to application of mixed wood-bark ash at planting in a temperate region: Nutrition and growth. For. Ecol. Manag. 255, 3873–3884. doi: 10.1016/j.foreco.2008.03.035
Temp, G. (1991). “Nickel in plants and its toxicity,” in Ustoichivost’k tyazhelym metallam dikorastushchikh vidov (Resistance of wild species to heavy metals), ed. N. Alekseeva-Popova (Leningrad: Lenuprizdat), 139–146.
Titus, B. D., Brown, K., Helmisaari, H. S., Vanguelova, E., Stupak, I., Evans, A., et al. (2021). Sustainable forest biomass: A review of current residue harvesting guidelines. Energy Sustain. Soc. 11, 1–32. doi: 10.1186/s13705-021-00281-w
Verheijen, F. G. A., Jeffery, S., Bastos, A. C., van der Velde, M., and Diafas, I. (2009). Biochar application to soils–a critical scientific review of effects on soil properties, processes and functions. EUR 24099 EN. Luxembourg: Office for the Official Publications of the European Communities, 149.
Wadhia, K., Dando, T., and Thompson, K. C. (2007). Intra-laboratory evaluation of microbial assay for risk assessment (MARA) for potential application in the implementation of the water framework directive (WFD). J. Environ. Monitor. 9, 953–958. doi: 10.1039/b704059h
Wardle, D. A., Nilsson, M. C., and Zackrisson, O. (2008). Fire-derived charcoal causes loss of forest humus. Science 320:629.
Williams, T. M. (1997). Obtaining water quality permits for land application of biomass broiler ash. Biomass Bioenergy 13, 279–287.
Yusuf, M., Fariduddin, Q., Hayat, S., and Ahmad, A. (2011). Nickel: An overview of uptake, essentiality and toxicity in plants. Bull. Environ. Contam. Toxicol. 86, 1–17. doi: 10.1007/s00128-010-0171-1
Keywords: biochar, wood ash, fertilization, toxicity, SOC, soil pH, pore water
Citation: Moragues-Saitua L, Arias-González A, Blanco F, Benito-Carnero G and Gartzia-Bengoetxea N (2023) Effects of biochar and wood ash amendments in the soil-water-plant environment of two temperate forest plantations. Front. For. Glob. Change 5:878217. doi: 10.3389/ffgc.2022.878217
Received: 17 February 2022; Accepted: 30 December 2022;
Published: 19 January 2023.
Edited by:
Manfred J. Lexer, University of Natural Resources and Life Sciences Vienna, AustriaReviewed by:
Nicole Wellbrock, Thuenen Instutitute of Forest Ecology, GermanyZachary E. Kayler, University of Idaho, United States
Copyright © 2023 Moragues-Saitua, Arias-González, Blanco, Benito-Carnero and Gartzia-Bengoetxea. This is an open-access article distributed under the terms of the Creative Commons Attribution License (CC BY). The use, distribution or reproduction in other forums is permitted, provided the original author(s) and the copyright owner(s) are credited and that the original publication in this journal is cited, in accordance with accepted academic practice. No use, distribution or reproduction is permitted which does not comply with these terms.
*Correspondence: Nahia Gartzia-Bengoetxea, bmdhcnR6aWFAbmVpa2VyLmV1cw==
†These authors have contributed equally to this work and share senior authorship