- Instituto de Lactología Industrial (INLAIN, UNL-CONICET), Facultad de Ingeniería Química, Santa Fe, Argentina
Lactic acid bacteria (LAB), essential in fermenting milk, produce an array of antimicrobial compounds, notably bacteriocins, which contribute to the extended shelf life of dairy goods. Bacteriocins, ribosomally synthesized peptides, display broad or narrow-spectrum antimicrobial activity, thus holding promise in food preservation. The classification of LAB bacteriocins is intricate, reflecting evolving genomic insights and biosynthesis mechanisms. Strategies for integrating bacteriocins into dairy products include purified forms, bacteriocin-producing LAB, and bacteriocin-containing fermentates, each with distinct advantages and considerations. Optimization of fermentation conditions, encompassing time, temperature, pH, and culture medium, is essential for maximizing bacteriocin production. This optimization facilitates enhanced quality and safety of fermented dairy items, aligning with the growing consumer preference for natural, minimally processed foods. Furthermore, the incorporation of bacteriocins into a hurdle approach alongside thermal and non-thermal treatments holds promise for augmenting food bioprotection while reducing reliance on chemical preservatives. This comprehensive overview underscores the potential of LAB bacteriocins as a natural, effective alternative to conventional food preservatives, offering insights into their application and optimization in dairy product preservation.
1 Introduction
Lactic acid bacteria (LAB) are a heterogeneous group of non-spore forming, Gram-positive, catalase-negative without cytochromes, anaerobic or aerotolerant, fastidious, acid tolerant, and strictly fermentative bacteria with lactic acid as the major product during sugar fermentation. LAB have long been revered for their crucial role in the fermentation of dairy products, contributing not only to flavor and texture but also to the preservation of these foods (Deegan et al., 2006; Favaro et al., 2015). The latter characteristic primarily stems from LAB’s production of antimicrobial compounds, predominantly including organic acids, hydrogen peroxide, diacetyl, acetoin, ethanol, antifungal peptides, and bacteriocins. Among these, bacteriocins stand out as potent antimicrobial peptides with diverse applications in food preservation.
Bacteriocins are ribosomally synthesized antimicrobial peptides able to kill microorganisms that are closely related or not (narrow or broad spectrum, respectively) (Alvarez-Sieiro et al., 2016; O’Connor et al., 2020). Many LAB and their products fulfill the status of Generally Regarded As Safe (GRAS) of the Food and Drug Administration (FDA, USA) or the Qualified Presumption of Safety (QPS) from the European Food Safety Authority (EFSA), for microorganisms intentionally introduced into the food chain. Therefore, LAB can be used in the food industry for the manufacture of fermented products, due to their biopreservative abilities. Particularly in fermented dairy products, bacteriocins offer an attractive solution. These peptides not only inhibit the growth of pathogens post-processing but also regulate adventitious microorganisms and enhance the fermentation process, ultimately enriching the sensory attributes of cheeses and similar products (O’Connor et al., 2020; Simons et al., 2020). Bacteriocins from LAB exhibit remarkable heat resistance, can withstand a broad spectrum of pH levels, possess no discernible color, odor, or taste, and are vulnerable to degradation by human digestive proteases, thereby preserving the balance of gut microbiota. These attributes render bacteriocins well-suited for use in food preservation (Daba and Elkhateeb, 2020). The utilization of bacteriocins in the dairy industry reflects a broader societal shift towards natural, minimally processed foods devoid of synthetic preservatives.
In light of expanding consumer demand for natural, additive-free foods, the incorporation of bacteriocins represents a promising opportunity for safeguarding the integrity and healthiness of dairy products.
2 Bacteriocins from LAB
2.1 Classification
The classification of bacteriocins from LAB is a complex landscape, enriched by recent genomic studies and evolving understanding of these antimicrobial peptides. Since Klaenhammer’s initial classification in 1993, several schemes have been proposed, focusing on biosynthesis mechanisms and biological activities (Simons et al., 2020).
Although there is still no full consensus, some authors (Arnison et al., 2013; Cotter et al., 2013; Alvarez-Sieiro et al., 2016) proposed a classification based on biological activity and mechanisms of biosynthesis. Here we present a brief overview:
Class I, lantibiotics, or RiPPs (ribosomally produced and post-translationally modified peptides, heat resistant) bacteriocins which includes: class Ia, which are peptides undergoing post-translational modifications with lanthionine or (methyl)lanthionine addition (e.g., nisin); class Ib (head-to-tail cyclized peptides) formed by circular peptides linked N- and C-terminally. They form pores in bacterial membranes; and class Ic, which are sulfur-to-α-carbon-containing peptides with hairpin structures. Class II, non-lantibiotics (or unmodified bacteriocins, heat resistant), encompasses class IIa or pediocin-like, which are broad-spectrum bacteriocins active mostly against Listeria species (e.g., pediocin PA-1); class IIb comprises two different peptides (e.g., lacticin F); class IIc, are cyclic peptides (e.g., enterocin AS-48) and class IId or non pediocin-like bacteriocins, are linear peptides. Class III are large, heat sensitive proteins that comprise class IIIa or bacteriolysins (e.g., enterolysin A) and class IIIb are non-lytic proteins. Class IV are complex, heat sensitive proteins with lipid or carbohydrate moieties (lipo- or glycoproteins; e.g., lactocin 27) (Figure 1).
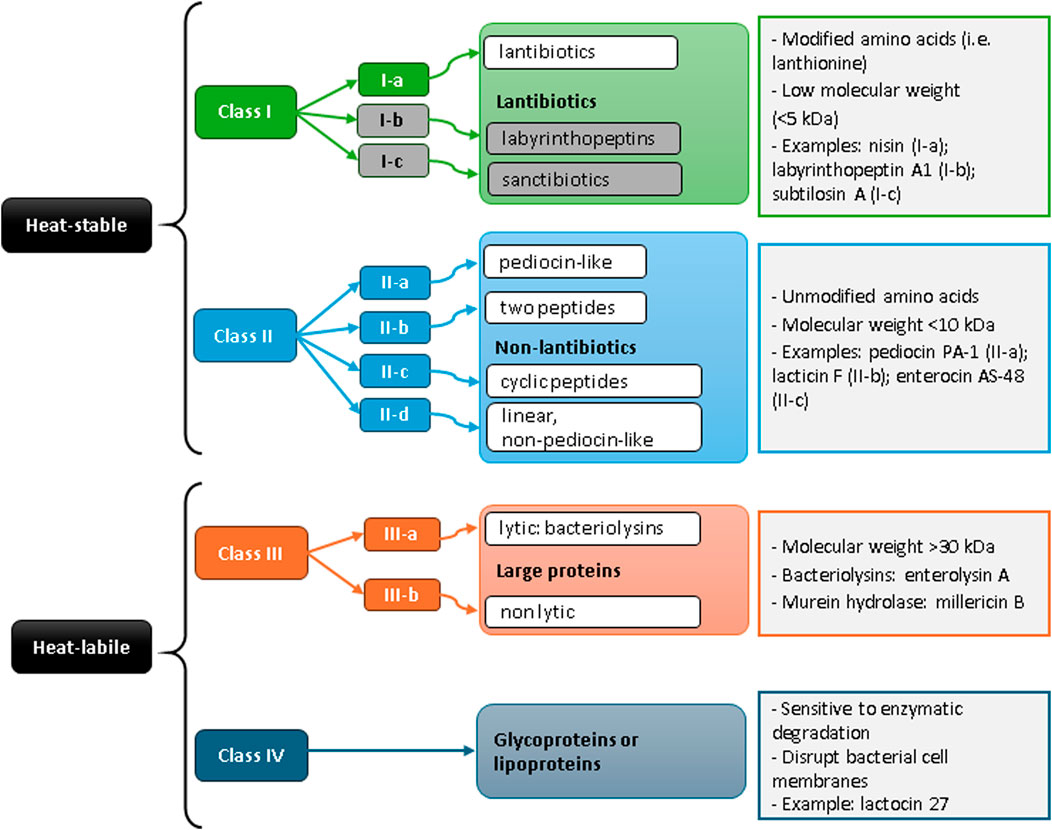
Figure 1. Overall classification of bacteriocins from lactic acid bacteria. Sub-classes I-b (labyrinthopeptins) and I-c (sanctibiotics) were included in the scheme (in grey), as well as example molecules, though they were not reported in lactic acid bacteria to date.
2.2 Use of bacteriocin in dairy foods
Bacteriocins can be used for food biopreservation, either alone or in combination with other methods of protection. However, in vitro results must be confirmed in the food matrix to assure bacteriocin effectiveness. To apply bacteriocins to foods, certain important criteria must be met. The producing strains should be food-grade (GRAS or QPS), exhibit a broad spectrum of inhibition, have high specific activity, pose no health risks, offer beneficial effects (such as improving food safety, quality, and flavor), and demonstrate stability in heat and pH, as well as optimal solubility and stability for the specific food application (Silva et al., 2018).
Numerous studies have been conducted on bacteriocin activity, characterization, target organisms, and applications; however, only a few have been commercialized. Before reaching that stage, various concerns must be addressed. Bacteriocins used in foods must be safe and have no toxicity for human cells. Some studies reported low cytotoxicity in both in vitro and in vivo assays, but only after using high bacteriocin amounts (beyond the required minimum inhibitory concentrations for pathogen or spoilage organisms) and long-time incubation. In addition, the presence of some compounds (such as proteases) in the food matrix can rapidly degrade bacteriocins, preventing from their bioprotective activity (Soltani et al., 2021). Lastly, the commercial production of bacteriocin will depend on the cost-benefit balance of producing large quantities of purified bacteriocin. This involves large-scale production, appropriate purification processes, and proper storage to ensure bacteriocin stability (Reuben et al., 2024).
For centuries, bacteriocins have been consumed in fermented and non-fermented foods. More recently, more people are looking for natural foods without artificial preservatives. This trend is particularly evident in the dairy industry, where consumption of naturally fermented milks is growing, prompting a shift toward exploring new and more natural preservation methods.
Fermented dairy products require refrigerated storage, which is optimal for the survival and proliferation of psychrotrophic bacteria such as L. monocytogenes. Consequently, contamination can occur during later stages of dairy product processing (Carpentier and Cerf, 2011). Hence, bacteriocins emerge as an optimal solution for inhibiting the growth of this pathogen post-cooking (Cotter et al., 2005). Furthermore, bacteriocins can serve to regulate adventitious microorganisms such as non-starter lactic acid bacteria (NSLAB) in cheese. Moreover, bacteriocins can be helpful in accelerating food fermentation, expedite cheese ripening, and even augment its flavor profile (O’Sullivan et al., 2002).
Bacteriocins can be included into dairy products as purified compounds, by adding a bacteriocin-producer LAB (as adjunct culture), or a fermentate containing bacteriocin (as an additive or ingredient) (Silva et al., 2018; O’Connor et al., 2020) (Figure 2; Table 1).
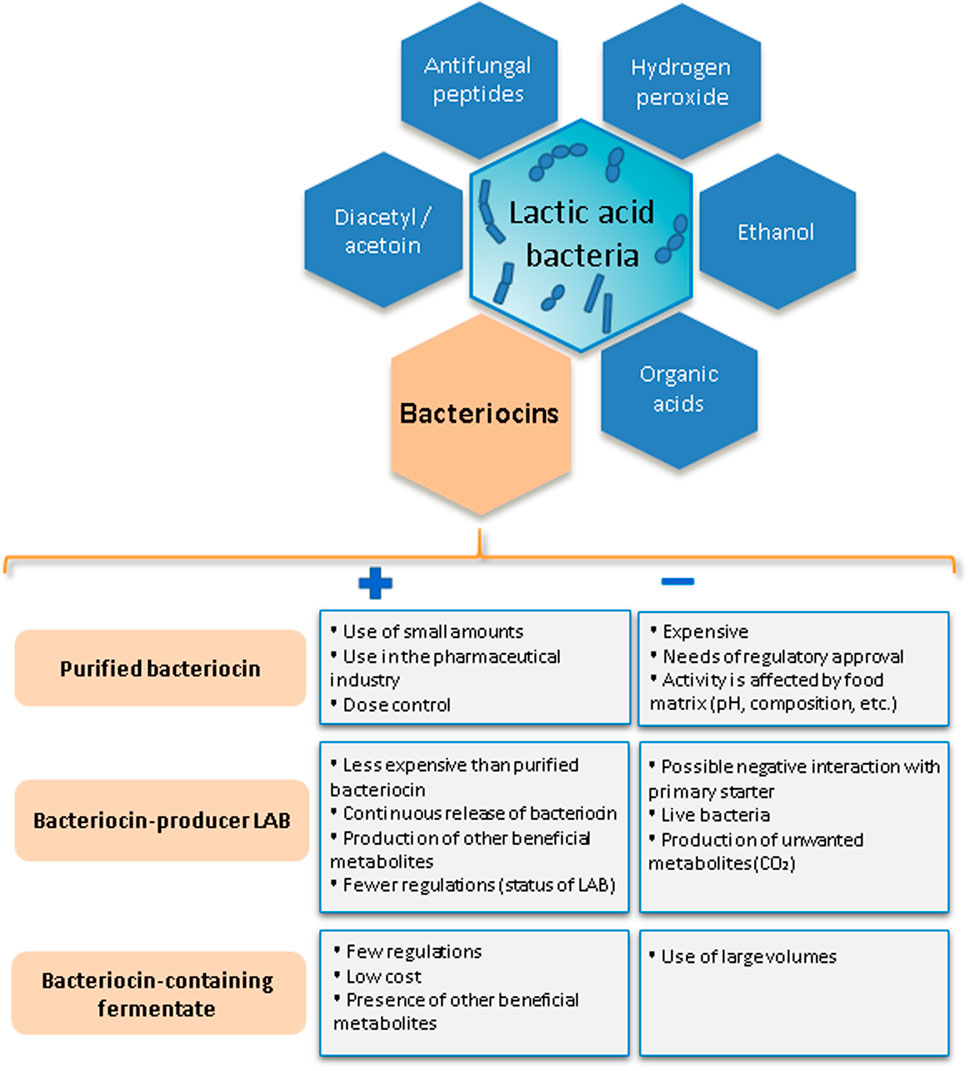
Figure 2. Antimicrobial compounds produced by lactic acid bacteria. Benefits and drawbacks of diverse approaches for incorporating bacteriocins into dairy products.
2.2.1 Purified bacteriocins
Purified bacteriocins employed in dairy products offer some advantages compared to the use of fermented product-containing bacteriocin or bacteriocin-producer LAB. Firstly, purification processes ensure the removal of impurities, resulting in a higher concentration of active bacteriocin. This increased potency allows for more effective inhibition of pathogenic and spoilage bacteria, thereby enhancing the shelf life and safety of dairy products. Moreover, purified bacteriocins exhibit improved stability and consistency in their antimicrobial activity, making them reliable and predictable agents for food preservation (Leroy and De Vuyst, 2010). Additionally, purification facilitates the removal of any unwanted taste or odor that may be associated with unpurified bacteriocins, thereby preserving the authentic flavor profile of the dairy product. Overall, the use of purified bacteriocins represents a superior approach to enhancing the quality, safety, and sensory attributes of dairy products compared to their unpurified counterparts. However, they require expensive regulatory approval. Up to date, nisin is the only commercial purified bacteriocin used. Nisin is a type IA bacteriocin produced by Lactococcus lactis (Egan et al., 2016; O’Connor et al., 2020). There were discovered more than ten nisin variants. Nisin A, F, Q and Z are produced by L. lactis, nisin U/U2, H, P, and G are produced by Streptococcus species, while nisin J, O and kunkecin A are produced by additional bacteria (Sugrue et al., 2023). Nisin holds approval for commercial use in over 60 countries as a food additive. Its commercial application falls under the European Food Safety Authority (EFSA) regulation, where it is licensed as a food preservative (E234). Moreover, nisin is recognized as a safe biopreservative in foods by the Joint Food and Agriculture Organization/World Health Organization (FAO/WHO) Expert Committee on Food Additives. At present, commercial nisin is offered by several companies: Nisaplin® (Danisco), NisinA® (Handary), or Niseen® (Siveele) are some examples (Table 1). Nisin is effective against numerous Gram-positive bacteria (including LAB), pathogens such as Staphylococcus and Listeria, and spore-forming bacteria like Bacillus and Clostridium. The use of nisin is extensive, going from refrigerated milk to combat the growth of pathogenic Listeria monocytogenes and Staphylococcus aureus, to diverse cheese varieties to prevent late blowing, a condition caused by Clostridium species (Silva et al., 2018; O’Connor et al., 2020).
2.2.2 Bacteriocin-producer LAB
The addition of LAB-producing bacteriocins to the food matrix has no regulatory limitations, and offers the advantage of localized and continuous release of antibacterial agents as the strain proliferates. Furthermore, it is less expensive than purified bacteriocins because there is no need for costly purification processes (Pujato et al., 2014). Bacteriocin synthesis depends on various factors including environmental conditions and bacterial growth phase. These variables may affect the consistency and effectiveness of bacteriocin activity in dairy products. Together, bacteriocin-producing bacteria could also generate unwanted metabolites that could affect organoleptic characteristics of the final product, such as taste, flavor and texture. Although, companies producing commercial ferments guarantee that protective cultures do not negatively influence on sensory characteristics of the product.
There are some commercially available bacteriocin-producer cultures (Table 1). BIOSAFE® (Chr. Hansen) contains a nisin A-producing strain, L. lactis subsp. lactis BS-10, that is used in hard and semi-hard cheese manufacture to prevent late blowing defects. HOLDBAC® (Danisco) is a series of protective cultures containing undefined bacteriocins from Propionibacterium freudenreichii subsp. shermanii DSM 706 and Lacticaseibacillus rhamnosus DSM 7061. They can be used in several foods including cheeses, meats, fermented vegetables, and beverages (O’Connor et al., 2020), generating various metabolites including bacteriocins, acids and other beneficial compounds that enhance their biocontrol capabilities. It is worth noting that more than 230 bacteriocin-producing bacteria have been isolated and studied in dairy products (Silva et al., 2018).
2.2.3 Bacteriocin-containing fermentates
Some commercial food-grade fermentates containing bacteriocins are available for use in the dairy industry (Table 1). This innovative antimicrobial solution is obtained from cultivating a bacteriocin-producing strain within a food-grade medium. They offer numerous advantages over the addition of bacteriocinogenic strains, such as cost-effectiveness, minimal regulatory obstacles, and the presence of other beneficial metabolites in the fermented product. Additionally, it allows adjusting the best conditions for bacteriocin production and minimizing undesirable by-products (e.g., CO2). Moreover, heat treatment could deactivate bacteriocin-producing bacteria without affecting heat-resistant bacteriocins, thus preventing competition with starter strains (Pujato et al., 2022). However, additional time is required to produce the fermentate separately. Despite the benefits, this extra step could lengthen the production process. Some examples of commercial available options are MicroGARD® (Danisco) and DuraFresh™ (Kerry Group P.L.C., Kerry, Ireland). MicroGARD® is a line of fermentates containing bacteriocins and other metabolites produced by Pediococcus acidilactici that offers a convenient way to introduce antimicrobial compounds into food products, helping in shelf-life extension of dairy foods and chocolate sweets (Makhal et al., 2015; Mills et al., 2017).
2.3 Optimization in bacteriocin production
Optimal production of bacteriocins depends on meticulous control of several physical and nutritional conditions. Firstly, fermentation time is crucial for maximizing bacteriocin production. Bacteriocins, generally considered primary metabolites, are mainly produced during the exponential growth phase of bacteria. The production rate of these metabolites often correlates with the growth rate of the bacterial cells. Numerous studies document bacteriocins that follow this pattern, such as nisin A/Z and lactococcin 140 (Parente and Ricciardi, 1994), pediocin PA1/SA-1 (Anastasiadou et al., 2008), mesenterocin 5 (Daba et al., 1993), lactocin 705 (Vignolo et al., 1995) lactostrepcin (De Vuyst, 1994) and leucocin (Geisen et al., 1993). However, some LAB produce bacteriocins during the stationary phase when conditions such as lower pH and reduced nutrient availability prevail. Examples include Lactobacillus plantarum LPCO10 (Jimenez-Díaz et al., 1993), L. lactis subsp. lactis (Cheigh et al., 2002), Lactobacillus pentosus B96 (Delgado et al., 2005). This variation requires an understanding of growth kinetics and metabolic processes for optimization (Sidooski et al., 2019).
Secondly, bacteriocin production is highly sensitive to temperature. Temperature directly influences enzymatic activity and cell growth rates of microorganisms. Studies suggest that optimal bacteriocin production often occurs at temperatures below the optimal growth temperature. For instance, Leuconostoc mesenteroides MB1 (Pujato et al., 2022) and Streptococcus macedonicus ACA-DC 198 (Van den Berghe et al., 2006) grew best at 30°C and 42°C, respectively, but their bacteriocin production peaked at 25°C. However, this is not a universal rule. Some LAB strains, such as Enterococcus faecium RZS C5 (Leroy and De Vuyst, 2002), P. acidilactici 13 (Altuntas et al., 2010), showed no significant differences in bacteriocin production between optimal and suboptimal growth temperatures. Therefore, it is essential to investigate the best temperature conditions for each case to maximize bacteriocin production.
In addition, pH affects enzymatic activity, growth rate, and metabolite production, including bacteriocins. High acidity can impair nutrient transport and essential cytoplasmic processes, leading to reduced cell growth and bacteriocin synthesis (Guerra, 2014). Excessive acidification, often due to high lactic acid levels, negatively influences bacteriocin production by decreasing the release of immune peptides in growing cells. Sometimes it is required the acidification of the medium to stimulate the bacteriocin making. In these cases, a buffered process would negatively affect their production (Guerra et al., 2008). For example, in Leuconostoc citreum MB1 the optimal pH for bacteriocin production was lower (pH 5 and 6) than that needed for maximum bacterial growth (pH 7) (Pujato et al., 2022). Therefore, to optimize bacteriocin production, it is crucial to control pH during fermentation (Sidooski et al., 2019).
One of the most influential factors in bacteriocin production by LAB is the culture medium. Supplementing the medium with key nutrients such as carbohydrates, nitrogen sources, vitamins, and salts can significantly enhance bacteriocin production. Although complex media like MRS are effective, they are costly and contain proteins that complicate downstream processing. Therefore, simpler, supplemented media offer a cost-effective alternative (Garsa et al., 2014). Moreover, optimizing carbon sources such as glucose and lactose is crucial as they influence enzyme production and metabolic activity. However, too high concentrations can inhibit bacteriocin production due to increased lactic acid levels. Nitrogen sources like yeast extract and tryptone, are also essential, providing amino acids and peptides that promote cell growth and bacteriocin synthesis (Leães et al., 2011). Minerals like magnesium and manganese are vital for enzymatic activities and the overall metabolism of LAB, supporting the structural and functional integrity of bacteriocins. Specific amino acids and vitamins also play a role in enhancing production, acting as precursors and co-factors in bacteriocin biosynthetic pathways (Moretro et al., 2000; Sidooski et al., 2019).
Fermentation conditions significantly affect bacteriocin production. Batch fermentation, where all nutrients are provided at the beginning, is straightforward but might result in lower yields due to nutrient depletion. Continuous fermentation, which maintains a steady supply of nutrients and removes waste products, often leads to more consistent and higher bacteriocin synthesis (Guerra, 2014).
Optimization of physical and nutritional conditions makes it possible to maximize bacteriocin production by LAB, making the process more efficient and economically viable for applications in food preservation.
3 Conclusion
From some time now, there is an increased interest in returning to less processed, chemical-free and more natural foods. In this sense, the use of bacteriocins in foods can be an effective alternative to chemical preservatives that could fulfill the current healthy lifestyle standards required. Depending on the need, cost and food type, bacteriocins can be included in food matrix as purified compound, by adding bacteriocinogenic LAB -with in situ and continuous release of the antimicrobial compound-, or by adding a fermentate-containing bacteriocin. Additionally, optimizing fermentation conditions, including time, temperature, pH, and culture medium, is crucial to maximize bacteriocin production, thereby enhancing the quality and safety of fermented dairy products. The use of bacteriocins into a hurdle approach including thermal and non-thermal treatments could increase food bioprotection and reduce or eliminate the use of potentially dangerous chemical preservatives.
Author contributions
SP: Conceptualization, Data curation, Investigation, Supervision, Visualization, Writing–original draft, Writing–review and editing. DM: Data curation, Investigation, Writing–original draft, Writing–review and editing. MB: Writing–review and editing. MC: Funding acquisition, Writing–review and editing. AQ: Funding acquisition, Project administration, Writing–review and editing. DG: Conceptualization, Data curation, Investigation, Supervision, Visualization, Writing–original draft, Writing–review and editing.
Funding
The author(s) declare that financial support was received for the research, authorship, and/or publication of this article. This work was supported by the Agencia Nacional de Promoción Científica y Tecnológica (ANPCyT; Projects PICT 2018-02935 and PICT 2019-0077; Argentina).
Conflict of interest
The authors declare that the research was conducted in the absence of any commercial or financial relationships that could be construed as a potential conflict of interest.
Publisher’s note
All claims expressed in this article are solely those of the authors and do not necessarily represent those of their affiliated organizations, or those of the publisher, the editors and the reviewers. Any product that may be evaluated in this article, or claim that may be made by its manufacturer, is not guaranteed or endorsed by the publisher.
References
Altuntas, E. G., Cosansu, S., and Ayhan, K. (2010). Some growth parameters and antimicrobial activity of a bacteriocin-producing strain Pediococcus acidilactici 13. Int. J. Food Microbiol. 141 (1–2), 28–31. doi:10.1016/j.ijfoodmicro.2010.04.024
Alvarez-Sieiro, P., Montalbán-López, M., Mu, D., and Kuipers, O. P. (2016). Bacteriocins of lactic acid bacteria: extending the family. Appl. Microbiol. Biotechnol. 100 (7), 2939–2951. doi:10.1007/s00253-016-7343-9
Anastasiadou, S., Papagianni, M., Filiousis, G., Ambrosiadis, I., and Koidis, P. (2008). Growth and metabolism of a meat isolated strain of Pediococcus pentosaceus in submerged fermentation. Enzyme Microb. Technol. 43 (6), 448–454. doi:10.1016/j.enzmictec.2008.05.007
Arnison, P. G., Bibb, M. J., Bierbaum, G., Bowers, A. A., Bugni, T. S., Bulaj, G., et al. (2013). Ribosomally synthesized and post-translationally modified peptide natural products: overview and recommendations for a universal nomenclature. Nat. Prod. Rep. 30, 108–160. doi:10.1039/c2np20085f
Aspri, M., O’Connor, P. M., Field, D., Cotter, P. D., Ross, P., Hill, C., et al. (2017). Application of bacteriocin-producing Enterococcus faecium isolated from donkey milk, in the bio-control of Listeria monocytogenes in fresh whey cheese. Int. Dairy J. 73, 1–9. doi:10.1016/j.idairyj.2017.04.008
Carpentier, B., and Cerf, O. (2011). Persistence of Listeria monocytogenes in food industry equipment and premises. Int. J. Food Microbiol. 145 (1), 1–8. doi:10.1016/j.ijfoodmicro.2011.01.005
Cheigh, C.-I., Choi, H.-J., Park, H., Kim, S.-B., Kook, M.-C., Kim, T.-S., et al. (2002). Influence of growth conditions on the production of a nisin-like bacteriocin by Lactococcus lactis subsp. lactis A164 isolated from kimchi. J. Biotechnol. 95 (3), 225–235. doi:10.1016/S0168-1656(02)00010-X
Cotter, P. D., Hill, C., and Ross, R. P. (2005). Bacteriocins: developing innate immunity for food. Nat. Rev. Microbiol. 3, 777–788. doi:10.1038/nrmicro1273
Cotter, P. D., Ross, R. P., and Hill, C. (2013). Bacteriocins - a viable alternative to antibiotics? Nat. Rev. Microbiol. 11, 95–105. doi:10.1038/nrmicro2937
Daba, G. M., and Elkhateeb, W. A. (2020). Bacteriocins of lactic acid bacteria as biotechnological tools in food and pharmaceuticals: current applications and future prospects. Biocatal. Agric. Biotechnol. 28, 101750. doi:10.1016/j.bcab.2020.101750
Daba, H., Lacroix, C., Huang, J., and Simard, R. (1993). Influence of growth conditions on production and activity of mesenterocin 5 by a strain of Leuconostoc mesenteroides. Appl. Microbiol. Biotechnol. 39 (2), 166–173. doi:10.1007/BF00228601
Dal Bello, B., Cocolin, L., Zeppa, G., Field, D., Cotter, P. D., and Hill, C. (2012). Technological characterization of bacteriocin producing Lactococcus lactis strains employed to control Listeria monocytogenes in cottage cheese. Int. J. Food Microbiol. 153, 58–65. doi:10.1016/j.ijfoodmicro.2011.10.016
Deegan, L. H., Cotter, P. D., Hill, C., and Ross, P. (2006). Bacteriocins: biological tools for biopreservation and shelf-life extension. Int. Dairy J. 16 (9), 1058–1071. doi:10.1016/j.idairyj.2005.10.026
Delgado, A., Brito, D., Peres, C., Noé-Arroyo, F., and Garrido-Fernández, A. (2005). Bacteriocin production by Lactobacillus pentosus B96 can be expressed as a function of temperature and NaCl concentration. Food Microbiol. 22 (6), 521–528. doi:10.1016/j.fm.2004.11.015
De Vuyst, L. (1994). “Lactostrepcins, bacteriocins produced by Lactococcus lactis strains,” in Bacteriocins of lactic acid bacteria. Editors L. De Vuyst, and E. J. Vandamme (Boston, MA, U.S.: Springer), 291–299. doi:10.1007/978-1-4615-2668-1_9
Egan, K., Field, D., Rea, M. C., Ross, R. P., Hill, C., and Cotter, P. D. (2016). Bacteriocins: novel solutions to age old spore-related problems? Front. Microbiol. 7, 461. doi:10.3389/fmicb.2016.00461
Favaro, L., Barretto Penna, A. L., and Todorov, S. D. (2015). Bacteriocinogenic LAB from cheeses – application in biopreservation? Trends Food Sci. Technol. 41 (1), 37–48. doi:10.1016/j.tifs.2014.09.001
Garsa, A. K., Kumariya, R., Sood, S. K., Kumar, A., and Kapila, S. (2014). Bacteriocin production and different strategies for their recovery and purification. Probiotics Antimicrob. Proteins 6 (1), 47–58. doi:10.1007/s12602-013-9153-z
Geisen, R., Becker, B., and Holzapfel, W. H. (1993). Bacteriocin production of Leuconostoc carnosum LA54A at different combinations of pH and temperature. J. Ind. Microbiol. 12 (3–5), 337–340. doi:10.1007/BF01584211
Guerra, N. P. (2014). Modeling the batch bacteriocin production system by lactic acid bacteria by using modified three-dimensional Lotka-Volterra equations. Biochem. Eng. J. 88, 115–130. doi:10.1016/j.bej.2014.04.010
Guerra, N. P., Bernárdez, P. F., and Castro, L. P. (2008). Modelling the stress inducing biphasic growth and pediocin production by Pediococcus acidilactici NRRL B-5627 in re-alkalized fed-batch cultures. Biochem. Eng. J. 40 (3), 465–472. doi:10.1016/j.bej.2008.02.001
Hartmann, H., Wilke, T., and Erdmann, R. (2011). Efficacy of bacteriocin-containing cell-free culture supernatants from lactic acid bacteria to control Listeria monocytogenes in food. Int. J. Food Microbiol. 146, 192–199. doi:10.1016/j.ijfoodmicro.2011.02.031
Jimenez-Díaz, R., Rios-Sánchez, R. M., Desmazeaud, M., Ruiz-Barba, J. L., and Piard, J. C. (1993). Plantaricins S and T, two new bacteriocins produced by Lactobacillus plantarum LPCO10 isolated from a green olive fermentation. Appl. Environ. Microbiol. 59 (5), 1416–1424. doi:10.1128/aem.59.5.1416-1424.1993
Kondrotiene, K., Kasnauskyte, N., Serniene, L., Gölz, G., Alter, T., Kaskoniene, V., et al. (2018). Characterization and application of newly isolated nisin producing Lactococcus lactis strains for control of Listeria monocytogenes growth in fresh cheese. LWT Food Sci. Technol. 87, 507–514. doi:10.1016/j.lwt.2017.09.021
Leães, F. L., Vanin, N. G., Sant'anna, V., and Brandelli, A. (2011). Use of byproducts of food industry for production of antimicrobial activity by Bacillus sp. P11. Food Bioprocess Tech. 4, 822–828. doi:10.1007/s11947-010-0410-9
Leroy, F., and De Vuyst, L. (2002). Bacteriocin production by Enterococcus faecium RZS C5 is cell density limited and occurs in the very early growth phase. Int. J. Food Microbiol. 72 (1–2), 155–164. doi:10.1016/S0168-1605(01)00635-3
Leroy, F., and De Vuyst, L. (2010). Bacteriocins of lactic acid bacteria to combat undesirable bacteria in dairy products. Aust. J. Dairy Technol. 65, 143–149.
Liu, L., O'Conner, P., Cotter, P. D., Hill, C., and Ross, R. P. (2008). Controlling Listeria monocytogenes in Cottage cheese through heterologous production of enterocin A by Lactococcus lactis. J. Appl. Microbiol. 104 (4), 1059–1066. doi:10.1111/j.1365-2672.2007.03640.x
Makhal, S., Kanawjia, S. K., and Giri, A. (2015). Effect of microGARD on keeping quality of direct acidified Cottage cheese. J. Food Sci. Technol. 52 (2), 936–943. doi:10.1007/s13197-013-1055-2
Mills, S., Ross, R. P., and Hill, C. (2017). Bacteriocins and bacteriophage; a narrow-minded approach to food and gut microbiology. FEMS Microbiol. Rev. 41, S129-S153–S153. doi:10.1093/femsre/fux022
Moretro, T., Aasen, I. M., Storro, I., and Axelsson, L. (2000). Production of sakacin P by Lactobacillus sakei in a completely defined medium. J. Appl. Microbiol. 88 (3), 536–545. doi:10.1046/j.1365-2672.2000.00994.x
Muñoz, A., Ananou, S., Gálvez, A., Martínez-Bueno, M., Rodríguez, A., Maqueda, M., et al. (2007). Inhibition of Staphylococcus aureus in dairy products by enterocin AS-48 produced in situ and ex situ: bactericidal synergism with heat. Int. Dairy J. 17, 760–769. doi:10.1016/j.idairyj.2006.09.006
O’Connor, P. M., Kuniyoshi, T. M., Oliveira, R. P. S., Hill, C., Ross, R. P., and Cotter, P. D. (2020). Antimicrobials for food and feed; a bacteriocin perspective. Curr. Opin. Biotechnol. 61, 160–167. doi:10.1016/j.copbio.2019.12.023
O’Sullivan, L., Ross, R. P., and Hill, C. (2002). Potential of bacteriocin-producing lactic acid bacteria for improvements in food safety and quality. Biochimie 84, 593–604. doi:10.1016/s0300-9084(02)01457-8
Parente, E., and Ricciardi, A. (1994). Influence of pH on the production of enterocin 1146 during batch fermentation. Lett. Appl. Microbiol. 19 (1), 12–15. doi:10.1111/j.1472-765X.1994.tb00891.x
Pucci, M. J., Vedamuthu, E. R., Kunka, B. S., and Vandenbergh, P. A. (1988). Inhibition of Listeria monocytogenes by using bacteriocin PA-1 produced by Pediococcus acidilactici PAC 1.0. Appl. Environ. Microbiol. 54, 2349–2353. doi:10.1128/AEM.54.10.2349-2353.1988
Pujato, S. A., Quiberoni, A. del L., Candioti, M. C., Reinheimer, J. A., and Guglielmotti, D. M. (2014). Leuconostoc citreum MB1 as biocontrol agent of Listeria monocytogenes in milk. J. Dairy Res. 81, 137–145. doi:10.1017/S002202991300068X
Pujato, S. A., Quiberoni, A. del L., and Guglielmotti, D. M. (2022). “Characterization of bacteriocins produced by lactic acid bacteria of industrial interest,” in Biomolecules from natural sources. Editors V. K. Gupta, S. D. Sarker, M. Sharma, M. E. Pirovani, Z. Usmani, and C. Jayabaskaran (John Wiley and Sons, Inc.), 458–469. doi:10.1002/9781119769620.ch16
Ribeiro, S. C., O’Connor, P. M., Ross, R. P., Stanton, C., and Silva, C. C. (2016). An anti-listerial Lactococcus lactis strain isolated from Azorean Pico cheese produces lacticin 481. Int. Dairy J. 63, 18–28. doi:10.1016/j.idairyj.2016.07.017
Ribeiro, S. C., Ross, R. P., Stanton, C., and Silva, C. C. (2017). Characterization and application of antilisterial enterocins on model fresh cheese. J. Food Prot. 80, 1303–1316. doi:10.4315/0362-028X.JFP-17-031
Reuben, R. C., Torres, C., and Yildirim, (2024). Bacteriocins: potentials and prospects in health and agrifood systems. Arch. Microbiol. 206 (5), 233. doi:10.1007/s00203-024-03948-y
Sidooski, T., Brandelli, A., Bertoli, S. L., Krebs de Souza, C., and Fernandes de Carvalho, L. (2019). Physical and nutritional conditions for optimized production of bacteriocins by lactic acid bacteria – a review. Crit. Rev. Food Sci. Nutr. 59 (17), 2839–2849. doi:10.1080/10408398.2018.1474852
Silva, C. C. G., Silva, S. P. M., and Ribeiro, S. C. (2018). Application of bacteriocins and protective cultures in dairy food preservation. Front. Microbiol. 9, 594. doi:10.3389/fmicb.2018.00594
Simons, A., Alhanout, K., and Duval, R. E. (2020). Bacteriocins, antimicrobial peptides from bacterial origin: overview of their biology and their impact against multidrug-resistant bacteria. Microorganisms 8 (5), 639. doi:10.3390/microorganisms8050639
Soltani, S., Hammami, R., Cotter, P. D., Rebuffat, S., Said, L. B., Gaudreau, H., et al. (2021). Bacteriocins as a new generation of antimicrobials: toxicity aspects and regulations. FEMS Microbiol. Rev. 8 (1), fuaa039. doi:10.1093/femsre/fuaa039
Sugrue, I., Hill, D., O'Connor, P. M., Day, L., Stanton, C., Hill, C., et al. (2023). Nisin E is a novel nisin variant produced by multiple Streptococcus equinus strains. Microorganisms 11 (2), 427. doi:10.3390/microorganisms11020427
Van den Berghe, E., Skourtas, G., Tsakalidou, E., and De Vuyst, L. (2006). Streptococcus macedonicus ACA-DC 198 produces the lantibiotic, macedocin at temperature and pH conditions that prevail during cheese manufacture. Int. J. Food Microbiol. 107 (2), 138–147. doi:10.1016/j.ijfoodmicro.2005.08.023
Vandera, E., Lianou, A., Kakouri, A., Feng, J., Koukkou, A.-I., and Samelis, J. (2017). Enhanced control of Listeria monocytogenes by Enterococcus faecium KE82, a multiple enterocin–producing strain, in different milk environments. J. Food Prot. 80, 74–85. doi:10.4315/0362-028X.JFP-16-082
Verma, S. K., Sood, S. K., Saini, R. K., and Saini, N. (2017). Pediocin PA-1 containing fermented cheese whey reduces total viable count of raw buffalo (Bubalis bubalus) milk. LWT Food Sci. Technol. 83, 193–200. doi:10.1016/j.lwt.2017.02.031
Vignolo, G. M., de Kairuz, M. N., de Ruiz Holgado, A. A. P., and Oliver, G. (1995). Influence of growth conditions on the production of lactocin 705, a bacteriocin produced by Lactobacillus casei CRL 705. J. Appl. Bacteriol. 78 (1), 5–10. doi:10.1111/j.1365-2672.1995.tb01665.x
Keywords: lactic acid bacteria, antimicrobial activity, bacteriocin, bioprotection, dairy foods
Citation: Pujato SA, Mercanti DJ, Briggiler Marcó M, Capra ML, Quiberoni A and Guglielmotti DM (2024) Bacteriocins from lactic acid bacteria: strategies for the bioprotection of dairy foods. Front. Food. Sci. Technol. 4:1439891. doi: 10.3389/frfst.2024.1439891
Received: 28 May 2024; Accepted: 07 August 2024;
Published: 20 August 2024.
Edited by:
Norbert Raak, University of Copenhagen, DenmarkReviewed by:
Monika Garbowska, Warsaw University of Life Sciences, PolandCopyright © 2024 Pujato, Mercanti, Briggiler Marcó, Capra, Quiberoni and Guglielmotti. This is an open-access article distributed under the terms of the Creative Commons Attribution License (CC BY). The use, distribution or reproduction in other forums is permitted, provided the original author(s) and the copyright owner(s) are credited and that the original publication in this journal is cited, in accordance with accepted academic practice. No use, distribution or reproduction is permitted which does not comply with these terms.
*Correspondence: Daniela Marta Guglielmotti, ZGd1Z2xpZWxAZmlxLnVubC5lZHUuYXI=