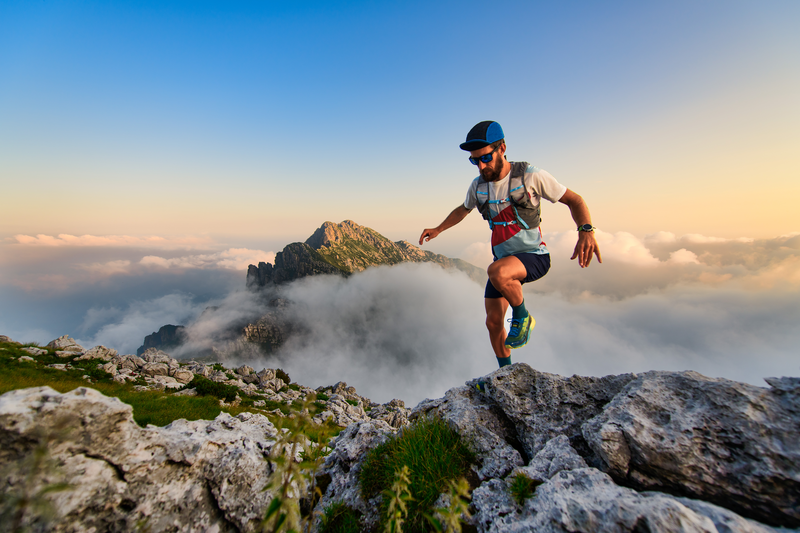
94% of researchers rate our articles as excellent or good
Learn more about the work of our research integrity team to safeguard the quality of each article we publish.
Find out more
ORIGINAL RESEARCH article
Front. Food. Sci. Technol. , 06 January 2023
Sec. Food Biotechnology
Volume 2 - 2022 | https://doi.org/10.3389/frfst.2022.1082490
This article is part of the Research Topic Algae as Food and Ingredient: From Production to Consumer Acceptance View all 4 articles
This study aimed at extracting lipophilic and hydrophilic compounds from Nordic brown seaweed Fucus vesiculosus and to evaluate the extract’s ability to maintain the physical and oxidative stability of fish oil-enriched mayonnaise (80% fat, 1:4 fish oil: rapeseed oil) during storage (dark, up to 28 days). Three different types of extracts were obtained, one using supercritical carbon dioxide (lipophilic extract) and two using subcritical water extraction (hydrophilic extracts)—one on dry seaweed (Subcritical water extract (SCWE) and one on the residue from supercritical carbon dioxide extraction after extracting the lipophilic compounds (Subcritical water extract (SCWER). The extracts were characterized with respect to their antioxidant composition and in vitro antioxidant properties. Moreover, the extracts were added in concentrations of 2 g/kg mayonnaise, both individually or in combination to study synergistic effects between antioxidants with different polarity and locations in the mayonnaise system. Results showed that both types of extractsdelayed the oxidation of lipids; The hydrophilic extracts (SCWE and SCWER) were able toretard the formation of hydroperoxides, and subsequent formation of secondary oxidation products. However, no synergetic effect was found for the hydrophilic and hydrophobic extracts when they were applied in different phases of the mayonnaise. The metal chelating ability is suggested to be responsible for the observed better performance of the hydrophilic extracts. However, further studies are required to understand which specific components in the extract have contributed to metal chelating ability. In conclusion, the findings of the present study suggest hydrophilic and hydrophobic compounds from the Nordic seaweed F. vesiculosus can retard lipid oxidation in mayonnaise.
Lipid oxidation in foods, cosmetics, and consumer products is a major factor for the quality deterioration of these products, giving rise to unwanted off-odors and -flavors (Frankel, 1998a). Lipid oxidation of food oil-in-water (o/w) emulsions, like mayonnaise, can be controlled by the addition of antioxidants. The partitioning of these antioxidants in o/w emulsions is crucial for the overall antioxidant efficacy. Depending on the polarity of the antioxidants they can be located in either the water or oil phases, and/or at the oil-water interface. The presence of transition metals such as iron in mayonnaise has shown that metal-chelating antioxidants in the water phase, are the most efficient in these food systems [e.g., ethylenediaminetetraacetic acid (EDTA)], to hinder Fe2+ catalyzing lipid oxidation (Jacobsen et al., 2001). However, other studies show that surface active compounds like lyophilized phenolic compounds have promising antioxidant efficacy in mayonnaise due to their location at the oil/water interface and also potential synergistic effect with other antioxidants, e.g., tocopherols in the oil phase (Alemán et al., 2015). Lastly, lipid soluble antioxidants like carotenoids and tocopherols have the advantage to be located in the oil phase where the propagation of lipid oxidation can take place, and from where these antioxidants can work as radical scavengers (Huang et al., 1996). However, tocopherols alone are not efficient antioxidants in mayonnaise (Jacobsen et al., 2000).
Several antioxidants, like EDTA, are very efficient synthetic antioxidants, designed to retard lipid oxidation. However, due to the restricted use of some synthetic antioxidants and increased awareness among consumers, there is a trend towards the use of natural additives and clean labeling. Thus, in the past decades, several studies have been published on the extraction and application of antioxidants obtained from natural sources in food and related products (Sabeena Farvin et al., 2012). The marine environment is a relatively less explored source of natural bioactive compounds with antioxidant properties including phenolic compounds, terpenoids, sulfated polysaccharides, and pigments to replace synthetic compounds (Tziveleka et al., 2021; Oliveira et al., 2022). These compounds can be found in e.g., seaweeds.
Fucus vesiculosus is a brown seaweed found in the intertidal waters of e.g., Nordic countries, such as the Baltic Sea. Brown seaweed, like F. vesiculosus, contains several bioactive compounds such as carotenoid pigments, phenolic compounds, polyunsaturated fatty acids, and polysaccharides (Holdt and Kraan, 2011). Traditionally seaweed bioactive compounds have been extracted using organic solvents. However, the use of organic solvents has several disadvantages including the use of large volumes of solvents, longer extraction time, and degradation of oxygen-sensitive compounds as the extraction is conducted in an open system. Another problem is the low selectivity of organic solvents (Getachew et al., 2018). To mitigate these challenges, several studies have been conducted in recent years on the extraction of bioactive compounds from seaweeds using emerging technologies (Getachew et al., 2020). These technologies include microwave-assisted extraction (Charoensiddhi et al., 2015), ultrasound-assisted extraction (Moreira et al., 2017), pulsed electric field assisted extraction (Robin et al., 2018), ohmic heating (Pereira et al., 2021), deep eutectic and natural deep eutectic solvents (Das et al., 2016; Obluchinskaya et al., 2019), supercritical carbon dioxide extraction (Saravana et al., 2017), and subcritical water (pressurized liquid extraction) (Sumampouw et al., 2021). Among these extraction technologies, extraction with sub and supercritical fluids is getting increasing attention. The use of these extraction technologies can help to mitigate the toxicity problem associated with the use of organic solvents and the selectivity problem. Extraction using supercritical carbon dioxide can selectively extract lipophilic compounds such as pigments and other lipid-soluble compounds (Getachew et al., 2020).
A previous study evaluated extracts from F. vesiculosus obtained by solid-liquid extraction (SLE), as antioxidants in fish oil-enriched mayonnaise (15% FO model system) during 28 days of storage (dark and room temperature) (Hermund et al., 2015). The obtained water extract showed antioxidant activity against the formation of both lipid hydroperoxides and secondary volatile oxidation products in fish oil enriched mayonnaise (concentration: 2.0 g dry extract/kg mayonnaise). This was associated with the metal chelating ability of the water extract since metal chelation is an important antioxidant mechanism in mayonnaise to hinder lipid oxidation.
SLE is not as specific an extraction method as the others mentioned above. Therefore, in the present study, the aim was to extract two different fractions of antioxidants with different polarities and evaluate their antioxidant efficacy in mayonnaise. Hence, a lipophilic fraction of F. vesiculosus was obtained using supercritical CO2 with ethanol as a co-solvent. Water-soluble antioxidants were extracted using subcritical water extraction on dry F. vesiculosus or on the residue from supercritical CO2 extraction, for a potential multi-extraction approach. The extracts were added to fish oil-enriched mayonnaise model systems (2 g/kg mayonnaise) to evaluate their ability to improve the oxidative and physical stability of the mayonnaise during storage. The synergistic effect between lipophilic and hydrophilic extracts was also evaluated in the mayonnaise model system (2 + 2 g/kg mayonnaise). The antioxidant efficacy of the extracts was evaluated against a well-known antioxidant in mayonnaise formulations, EDTA, as a positive control, and no antioxidant added to mayonnaise was included as a negative control. The results obtained in this study are the first of their kind and will contribute to the research on seaweed-based antioxidants in foods prone to lipid oxidation.
Brown seaweed Fucus vesiculosus was harvested in May 2020 from Bellevue beach, (55°46′17.4″N 12° 35′48.4″E), Denmark. After harvesting the seaweed, it was immediately taken to the laboratory rinsed with tap water to remove sand and epiphytes attached to the seaweed and immediately frozen at −40°C. The time from harvesting to freezing took approximately 2 h. The frozen seaweed was later freeze-dried in Christ Freeze Dryer Beta 1–8 (Germany) for 2 days, then powdered with Waring blender (VWR), and sieved to remove large particles (>Ø1 mm). The powdered seaweed was stored frozen at −20°C after being purged with nitrogen to avoid oxidation.
Three types of extracts were obtained using advanced, highly efficient, green extraction techniques. Supercritical CO2 extraction was used to extract the lipid fraction and high amount of lipid soluble antioxidants such as carotenoids. Subcritical water extraction extracted polar compounds, among other phenolic antioxidants, and possibly also antioxidative polysaccharides and proteins/peptides. Potential multi-extraction of lipid soluble and polar antioxidants were included in the study as subcritical water extraction was performed on both dry seaweed and the residue after supercritical CO2 extraction.
The extraction of F. vesiculosus was conducted using SFE system connected MV-10 ASFE System (Waters, Milford, MA 01757, United States). The extraction was conducted with previously optimized extraction conditions. Briefly, 10 g of dried and grounded seaweed powder (<1 mm) was packed in 25 mL extraction vessels connected to the supercritical CO2 inlet and extracts outlet line then kept in the oven. CO2 was pumped to the extraction vessel to attain the desired pressure using a Fluid delivery module (Waters Corp., Manchester, United Kingdom) after passing through a cooling heat exchanger. The CO2 and the co-solvent (ethanol) flow rate were 7 mL/min and 1.5 mL/min respectively. The extraction temperature and pressure were 40°C and 250 bar respectively. The extraction was conducted in one cycle for both static (10 min) and dynamic extractions (110 min). The dynamic extraction stage was conducted under different conditions. For the first 100 min of extraction, the extraction was conducted in the presence of the co-solvent and for the last 10 min, the flow of the co-solvent was stopped while keeping the same flow rate of CO2 to remove all the remaining solvents from the extraction. After the extraction was completed, the system was depressurized to collect the extract and residue. The remaining solvent was removed from the extract using a stream of nitrogen to obtain the final extract coded SCCO2E. Finally, both the extract and the residue were kept in a freezer at −20°C until needed for further analysis.
The extraction was performed using accelerated solvent extractor ASE (ASE 350, Dionex Sunnyvale, CA, United States). For each experiment, 1 g of dried seaweed powder (code: SCWE) or seaweed powder residue (code: SCWE-R) after supercritical CO2 extraction and 2 g of Ottawa sand were mixed and loaded into a 10 mL extraction cell equipped with a glass fiber filter on its bottom. Each experiment used maximum operating pressure of 10 bar, 60% rinsing volume, and purge time of 100 s. The extraction temperature was 160°C. The extraction time was 5 min. The time needed for equilibration of the extraction conditions was 9 min. After the extraction was completed, the extract was collected automatically in extract collection vials and stored at −20°C. The extract was dried in a freeze dryer (Christ Freeze Dryer Beta 1–8, Germany) for 1 day after being frozen at −20°C.
The total phenolic content (TPC) was determined using Folin-Ciocaltau reagent for quantification. The protocol was according to our previous study (Sabeena Farvin and Jacobsen, 2013). Briefly, 100 µL of the appropriately diluted extract was mixed with 750 µL of Folin–Ciocalteu reagent (10% v/v in water) in a 1.5 mL plastic microcuvette. After incubating the mixture for 5 min in the dark and at room temperature 750 µL of sodium carbonate (Na2CO3, 7.5% w/v in water) was added. This solution was thoroughly mixed and incubated for 90 min more. Finally, the absorbance of this mixture was measured at 725 nm using a spectrophotometer (Shimadzu UV mini 1,240, Duisburg, Germany). The TPC value of each extract was measured in triplicate (n = 3) and reported as a mean ± standard deviation. The TPC was calculated as milligrams of gallic acid equivalents (mg GAE) per 100 g of dry seaweed or residue from the first extraction based on a six-point standard curve of different gallic concentrations (7.81–250 μg/mL)
Pigment analysis was done according to (Ljubic et al., 2019) with minor modifications. Briefly, approximately, 10 mg of the SCCO2E was weighed in a centrifugation tube and 10 mL of methanol containing BHT was added, and the mixture was kept on a sonication bath (Buch & Holm A/S, Denmark) for 10 min at 5 ± 2°C to completely dissolve the extract. Pigment analysis was performed by HPLC using Agilent 1,100 Liquid Chromatograph with diode array detector (DAD) (Agilent Technologies, Santa Clara, CA, United States). Separation was carried out on a Zorbax Eclipse C8 column 150 mm × 46 mm × 3.5 μm (Phenomenex Inc., Santa Clara, CA United States) at 60°C. The mobile phase was a mixture of 70% methanol + 30% of 0.028 M tertiary butyl ammonium acetate in water and methanol at a flow rate of 1.1 mL min−1 with a total acquisition time of 40 min. DHI pigment standard mix (DHI LAB Products, Horsholm, Denmark) was used for the identification of peaks. Detection of chlorophylls and carotenoids was done at 660 nm and 440 nm, respectively, and for internal standard (BHT) at 280 nm. Pigments are reported as mg/g of the extract. Analyses were carried out in duplicate (n = 2) and results were reported as means ± SD.
The DPPH radical scavenging activity of the extracts was evaluated following our previous study (Getachew et al., 2022). Briefly, an aliquot (100 µL) of extract at a concentration of 2 mg/mL in distilled water was transferred into a 96-well microtiter plate. 100 µL of DPPH (0.1 mM in ethanol) was added to each well. These mixtures were incubated for 30 min at room temperature in dark conditions Afterwards, the absorbance of each mixture was measured at a wavelength of 517 nm in a BioTek Eon Microplate reader (Winooski, VT, United States). The analysis was performed in triplicates (n = 3) and results were given at inhibition% at a concentration of 2 mg extract/mL methanol/deionized water. BHT was included as a radical scavenging control (0.2 mg/g) for valid results (inhibition 74.07%).
The ferrous iron chelating ability of the extracts was evaluated following our previous study (Getachew et al., 2022). In brief, 100 μL of antioxidant extracts with concentrations of 2 mg/mL were pipetted into a 96-well microtiter plate followed by the addition of 110 μL distilled water and 20 μL ferrous chloride solution (0.5 mM). After 3 min of incubation, 20 μL ferrozine solution (2.5 mM) was added to each well. The plate was incubated for 10 min in dark at room temperature. Finally, the absorbance was measured at 562 nm in a BioTek Eon Microplate reader (Winooski, VT, United States). The analysis was performed in triplicates (n = 3) and results were given at inhibition% at a concentration of 2 mg extract/mL methanol/deionized water EDTA was included as a metal chelating control (0.2 mg/g) for valid results (inhibition% = 99.8).
Six different types of mayonnaise (80% oil w/w, 1:4 fish oil:rapeseed oil) were prepared according to (Meyer et al., 1996) with some modifications. Table 1 shows the different formulations, and to which phase the different antioxidants are added. The color of the mayonnaises was measured immediately after the preparation according to Poyato et al. (2017) to obtain the color coordinates L, a, and b. The measurements were performed in triplicates.
TABLE 1. The different mayonnaise, the type and amount of antioxidants added, and the phases in which the antioxidants were added.
For droplet size analysis, each mayonnaise (1 g) was dispersed in 9 g of SDS buffer (10 mM NaH2PO4, 5 mM SDS, pH 7) and the solution was whirly mixed for 0.5 min at room temperature. After that, the samples were placed in an ultrasonic bath (30°C, 20 min). This mixing procedure was repeated once more. The oil droplet size of the mayonnaises was determined using laser diffraction in a Mastersizer 2000 (Malvern Instruments, Ltd., Worcestershire, United Kingdom) according to the method described by Jacobsen et al. (1999) on days 7 and 28. Results were presented as the surface weighted (D [3,2]) and volume-weighted (D [4,3]) (n = 2).
The lipid phase of the mayonnaise was separated by centrifugation (1,620 g, 10 min, 4°C) after defrosting the frozen mayonnaise. The supernatant oil phase was collected and used for tocopherol and peroxide value analysis (Hermund et al., 2015).
The oil phase from mayonnaises was dissolved in heptane and analyzed by HPLC (Agilent 1,100 Series, Agilent Technology) according to(AOCS Press, 1997) to quantify the contents of α-, β-, γ- and δ-tocopherols in the samples (results for γ- and δ-tocopherols are in Supplementary Table S1). These tocopherol homologs were separated using a silica column (Waters, Dublin, Ireland, 150 mm, 4.6 mm, 3 µm silica film). A stock solution added 10 mg tocopherols (mixture of α-, β-, γ- and δ-tocopherols) per liter was prepared and used for quantification. The analysis was done in duplicates (n = 2) and the results were reported as µg tocopherol/g mayonnaise. The relative change (RC%) in tocopherols from day 0 to 28 was calculated.
The PV of all mayonnaises was determined using a method described by Shantha and Decker (1994). The oil phase obtained as described in section 2.6 was used for analysis PV was subsequently measured on the lipid extracts by colorimetric determination of iron thiocyanate on a spectrophotometer (Shimadzu, UV mini 1,240, Kyoto, Japan) at 500 nm. Duplicate (n = 2) measurements from the same sample were carried out. Results for the PV are expressed as meq O2/kg oil.
Tenax GR™ packed tubes were used to collect volatile compounds by dynamic headspace. The collection of the volatile compounds was carried out using 4 g of mayonnaise sample (incl. 30 mg int.std., 4-methyl-1-pentanol). The volatile secondary oxidation products were collected at 60°C followed by flushing with nitrogen (flow of 150 mL/min) for 30 min to remove water from the Tenax tube (n = 3). For the mayonnaise sample, volatile acids were removed by 0.1 g KOH during the headspace collection, by the connection of an S-tube with KOH, as described by Hartvigsen et al. (2000). The trapped volatiles were desorbed using an automatic thermal desorber (ATD-400, Perkin-Elmer, Norwalk, CT) connected to an Agilent 5890 IIA model gas chromatograph equipped with a HP 5972 mass selective detector. Chromatographic separation of volatile compounds was performed on a DB1701 column (30 m × ID 0.25 mm × 0.5 µm film thickness, J&W Scientific, Folsom, CA, United States) using helium gas flow (1.3 mL/min). The temperature program for mayonnaise was as follows: 3 min at 35°C, 3°C/min from 35 to 120°C, 7°C/min to 120–160°C, 15°C/min 160–200°C and hold for 4 min at 200°C. For milk the temperature programs: 5 min at 45°C, 1.5°C/min from 45 to 55°C, 2.5°C/min from 55 to 90°C, 12°C/min from 90 to 200°C and hold for 4 min at 200°C. The autosampler collectors setting details are 9.2 psi, outlet split: 5.0 mL/min, and desorption flow: 60 mL/min. For quantification, a calibration curve was prepared from external standards added to mayonnaise without added seaweed extracts. Then, the volatiles were collected in the same way as the samples. The analysis was performed in triplicate (n = 3) and the results are given in ng/g of mayonnaise.
The statistical analysis was performed using Origin software (OriginPro 2021). Depending on the different data sets one-way and two-way ANOVA was performed followed by Bonferroni post hoc analysis to determine the presence of significant differences among the means of the different values. All significant levels were analyzed at p values of ≤ .05.
Three extracts were obtained in the present study; SCCO2E, the lipophilic fraction, which was obtained using supercritical CO2 extraction, and SCWE and SCWE-R, the polar fraction, from extraction with subcritical water. SCWE was from dry F. vesiculosus and SCWE-R was from the residue after supercritical CO2 extraction after extraction of the lipophilic fraction.
Figure 1 shows the results from the characterization of the extracts. The SCCO2E and SCWE showed the highest and the lowest TPC with 85.31 ± 0.48 and 35.78 ± 0.67 mg GAE/g extract respectively with GAE meaning gallic acid equivalent. The extraction with supercritical CO2 was supplemented with ethanol as a cosolvent. This could enhance the solubility of the phenolic compounds and thereby increase the TPC (Saravana et al., 2017). A much lower TPC (36.9 mg GAE/g seaweed) was reported by Sumampouw et al. (2021) who extracted using pressurized liquid extraction with hydroethanolic solvent. In another study, Agregán et al. (2017) reported 11.5 mg GAE/g of seaweed for the same type of seaweed species extracted using water as solvent at room temperature. However, a higher TPC was reported by Poyato et al. (2017), who reported 143.7 mg GAE/g from F. vesiclusos extracted using the conventional method employing 100% water as an extraction solvent. The TPC depends on the type of seaweed species used, the geographic location, the harvest season, the harvest stage of the seaweed, and the extraction method used to obtain the extract (Getachew et al., 2022). Unlike the solvent extraction method, the methods used in this study were able to selectively extract both the lipophilic and hydrophilic fractions.
FIGURE 1. Total phenolic contents of the different extracts. The values are represented as mg GAE/g dried extract. The error bars show the standard deviation between treatments (n = 3) Different letters on the top of the bar graphs indicate significant differences (p < . 05).
Table 2 shows the pigment content of SCCO2E. In total nine different pigments were identified. Chlorophyll a showed the highest value followed by fucoxanthin and pheophytin with 130.75 ± 11.07, 44.86 ± .09, and 22.59 ± 0.52 mg/g of extract respectively. These values are different from those reported by Hermund et al. (2015) who found a much lower value for chlorophyll of 10.0 ± 1.5 mg/g extract and nearly the same values of fucoxanthin (43.0 ± 1.9 mg/g extract). The difference could be due to the extraction method used to obtain the extracts. In Hermund et al. (2015) the extracts were recovered using conventional SLE. Another reason for the observed discrepancy could be the harvesting season of the seaweed. The extracts obtained by subcritical water extraction did not contain any chlorophyll or were not analyzed.
TABLE 2. Pigments content of the F. vesiculosus lipid fraction (SCCO2E) obtained using supercritical CO2 extraction.
Table 3 shows the antioxidant activities of the extracts. The antioxidants were measured in terms of DPPH radical scavenging capacity and ferrous iron chelating ability at a concentration of 2 mg/mL. SCWER and SCCO2E showed the highest and the lowest DPPH radical scavenging capacity with 83.5 ± 1.7 and 77.5 ± 22.8%, respectively. One would expect a high antioxidant activity for SCWE because unlike the SCWER, compounds such as TPC, which contribute to the antioxidant activity were, not removed. However, there was no significant difference (p < .05) between the SCWE and SCWER. The reason for this could be the opening of the structure of the cells by the supercritical CO2, which would make the remaining compounds more acceptable for extraction. Similarly, these two extracts also showed the highest ferrous iron chelating ability with ca. 50% inhibition. The ferrous iron chelating ability of SCCO2E was significantly different from the extracts obtained by subcritical water extraction. Our previous study (Getachew et al., 2022) on subcritical water extraction on F. vesiculosus showed a high amount of polysaccharides alginate and fucoidan in the final extract. In this study, the extracts showed a DPPH radical scavenging activity with an IC50 value of 0.14 mg/mL and metal chelating ability with an IC50 value of 1.39 mg/mL for extracts obtained at 160°C and 120°C respectively. Polysaccharides such as fucoidan and alginate, protein, and peptides can have metal chelating abilities. The difference in the ferrous iron chelating ability of the extract could be due to the presence of such polysaccharides and other metal chelating species in the subcritical water extracts.
TABLE 3. In vitro antioxidant properties of the extracts; DPPH radical scavenging capacity and ferrous iron chelating ability. The values are for extract concentrations of 2 mg extract/mL Values are mean ± SD (n = 3). Values flowed by different small letters are significantly different (p < .05).
As mentioned above the SCCO2E extract had a high chlorophyll a content and therefore had a green color. Hence, addition of SCCO2E resulted in a green color of the mayonnaise. The subcritical water extracts (SCWE and SCWE-R) gave the mayonnaise a brownish color (no pigment data included). Poyato et al. (2017) found brown pigments to be dominant in water extracts from Fucus vesiculosus (xanthophylls, 2.17 mg/mg extract, and carotenes, 1.72 mg/mg extract) compared to green pigments (chlorophylls, 0.46 mg/mg extract) resulting in a brownish color when the extracts were added to skin care emulsions. The control mayonnaise without extract and the mayonnaise with EDTA added were more white (high L-value in Table 4).
TABLE 4. The color values of mayonnaise with different antioxidants added (and control without antioxidant added) were measured at the beginning of the storage period (on day 1). L* for lightness, a* for green/redness, and b* blueness/yellowness.
In Table 4 the color measurements of the mayonnaise samples are shown. The green colored mayonnaises with SCCO2E added (also when in combination with SCWE-R) had a significantly higher b-value compared to the other samples. Moreover, these samples were greener (p < .05) shown by a lower a-value and were the darkest of all samples (significantly lower L-value). The green color of SCCO2E was associated with a high content of chlorophyll especially chlorophyll a (130.75 ± 11.07 mg/g), which to a higher extent was extracted with super critical CO2 extraction in the lipophilic fraction. When added alone, the water extracts (SCWE and SCWE-R) increased the red color significantly (a-value) of the mayonnaise compared to the other samples, resulting in brownish mayonnaise samples. Moreover, the mayonnaise samples with SCWE and SCWE-R added were significantly darker (L-value) than the control and the mayonnaise with EDTA added.This shows that the water extracts contained more water soluble pigments compared to SCCO2E. Honold et al. (2016) showed that water extracts obtained from SPE contained carotene a and b whereas ethanolic extracts contained more chlorophylls. These results support the difference in pigment composition depending on extraction solvent.
Oil droplet size distribution (DSD) was also affected by the addition of extracts to the mayonnaise. Figure 2 shows the D[4,3] (a) and D[3,2] (b) values of the mayonnaise samples after 7 and 28 days of storage. D[3,2], the surface mean of the droplets, did not change significantly from day 7 to day 28, and there was no significant difference between samples, indicating that the oil droplet size in the mayonnaise samples did not change during storage. D[3,2] of mayonnaise with SCCO2E and SCWER added in combination had a large standard deviation indicating that there could be phase separation, which makes it difficult to measure the DSD. When looking at the D[4,3] values, mayonnaise with SCCO2E added showed a significantly higher value compared to the control (and sample added EDTA). Moreover, the value increased significantly from day 7 to 28 only for the mayonnaise sample with SCCO2E added. When adding SCCO2E in combination with SCWE-R the value was 8x higher than all other samples, but the value did not increase from day 7 to 28. Hence, this sample had a high proportion of large droplets than the other samples, resulting in a higher D[4,3] value. Large droplets have a much higher weight for D[4,3] than for D[3,2] and this explains why the same differences between samples and storage days were not observed for D[3,2] as for D[4,3]. The D[4,3] and D[3,2]values of the mayonnaise samples at day 7 (except for mayonnaise with SCCO2E and SCWER added in combination), are in agreement withpreviousfindings for this type of food system (Jacobsen et al., 2001). The increase in DSD in some of the mayonnaise samples from day 7 to day 28, indicates destabilization of the emulsions.
FIGURE 2. Droplet size (µm) in mayonnaise with different antioxidants added (and control without antioxidant added) on day 7 and 28 (stored dark at room temperature). (A) D [4,3] volume-weighted mean and (B) D [3,2] surface means droplet sizes. Mean ± sd (n = 3).
Oxidative changes in the mayonnaises were determined during storage by the development of lipid hydroperoxides, tocopherols, and secondary volatile oxidation products.
The development of lipid hydroperoxides in mayonnaise during storage was determined as the development in peroxide value (PV) for each sample as shown in Figure 3. The lipid hydroperoxides in the control mayonnaise increased significantly (p < .05) during storage from .0 ± .0 to 22.8 ± 0.5 meq O2/kg oil. No significant (p < .05) increase in PV was observed in mayonnaise with 75 ppm EDTA added, whereas in mayonnaise with seaweed extracts added (2 g/kg SCWE, SCCO2E, SCWE-R or SCCO2E + SCWE-R) a significant increase (p < .05) in PV was found. PV for samples with EDTA, SCWE, or SCWE-R added increased from approximately 0.0 meq O2/kg oil to 2.1 ± 0.5, 15.8 ± 0.5, and 14.5 ± 0.3 meq O2/kg oil at day 28, respectively. PV for samples with SCCO2E or SCCO2E in combination with SCWE-R (SCCO2E + SCWE-R) added had significantly higher initial PV (PV at day 0) (p < .05) of 5.1 ± 0.2 and 4.4 ± 0.1 meq O2/kg oil, respectively, compared to the other samples. The higher initial PV of these samples indicates the formation of lipid hydroperoxides already during the production of the mayonnaise or that SCCO2E contains peroxides itself. Moreover, a decrease in PV was observed after day 21 for the sample with SCCO2E added, indicating the breakdown of lipid hydroperoxides to secondary oxidation products.
FIGURE 3. Development in peroxide value (PV) in mayonnaise with different antioxidants added (and control without antioxidants added) stored up to 28 days (dark, room temperature). Values with the same symbols are not significantly different (p < .05). Mean ± sd (n = 3).
Lag phases for the development in PV were observed for all samples, except the one with EDTA added, as no significant increase in PV from day 0 to day 28 was found. A lag phase of up to 3 days was found for mayonnaise with SCCO2E or when SCCO2E was added in combination with SCWE-R. For the control and mayonnaise with SCWE added the lag phase was up to 7 days, and when SCWE-R was added the lag phase was up to 14 days. EDTA, SCWE, and SCWE-R showed antioxidant activity against the formation of hydroperoxides, as a lower formation of PV was observed when these compounds were added to the mayonnaise compared to the control. On the other hand, in samples with SCCO2E or SCCO2E + SCWE-R added, prooxidant activity was observed as the formation of PV was higher in these samples compared to the control. This indicates that SCCO2E was prooxidative.Based on these results it seems that only SCWE and SCWE-R were able to decrease the formation of lipid hydroperoxides. SCWE and SCWE-R had very similar antioxidant properties (Table 3), such as moderate to high metal chelating ability. Both were soluble in the water phase, where they can work as secondary antioxidants by preventing the initiation of lipid oxidation by chelating transition metals such as iron. Other studies also found a lag phase in formation of primary oxidation products of up to 7 days when water extracts from Fucus vesiculosus were added to mayonnaise as antioxidants (Hermund et al., 2015; Honold et al., 2016). However, Hermund et al. (2015) found that PV at day 28 in mayonnaise with water extract added (concentration of 2 g/kg), were similar to PV for mayonnaise added EDTA in the present study, indicating lower antioxidant efficacy of the extracts obtained by subcritical water extraction compared to traditional SPE.
Even though SCCO2E did not show antioxidant activity against the formation of lipid hydroperoxides, this extract may work against the breakdown of lipid hydroperoxides into secondary oxidation products. SCCO2E is expected to be located in the oil phase where compounds like fucoxanthin (Table 2) may scavenge lipid oxidation intermediates by hydrogen donation. Hence, to better understand the antioxidant mechanisms of the seaweed extracts added to the mayonnaise, we investigated the formation of secondary oxidation products.
Odor and flavor deterioration of lipid containing products is caused mainly by the presence of volatile secondary oxidation compounds, which have an impact on odor and flavor at extremely low concentrations. Compounds formed from the decomposition of lipid hydroperoxides during storage can either react with unsaturated lipids to form stable and innocuous compounds or undergo fragmentations into aldehydes and ketones causing rancidity in unsaturated matrices (Frankel, 1998a). In the present study, 12 volatile secondary oxidation products were identified from the samples, most of them have previously been associated with oxidation of fish-oil-enriched mayonnaise (Hartvigsen et al., 2000). Figure 4 shows two representatives (pentanal and 1-penten-3-ol) of the development of volatile secondary oxidation products.
FIGURE 4. Development in concentration (ng/g mayonnaise) of two representative volatile compounds (A) pentanal and (B) 1-penten-3-ol in mayonnaise with different antioxidants added (and control without antioxidant added) during 4 weeks of storage (dark, room temperature). Mean ± sd (n = 3).
The same trend as for the development of lipid hydroperoxides (Figure 3) was found for the development of pentanal, with one exception; namely that SCWE had a slight prooxidative effect on pentanal formation (significantly higher pentanal concentration at day 3 and 21 (p < .05) compared to the control) and antioxidant effect on the formation of lipid hydroperoxides. Mayonnaise with SCCO2E added alone or in combination with SCWE-R showed higher initial concentration and no lag phase for pentanal, and the concentration of pentanal was 5–6 times higher at the end of the storage compared to the other samples, clearly indicating prooxidant activity of SCCO2E.
The higher initial values when SCCO2E was added could indicate that this extract contains some oxidation products itself. SCCO2E is produced under high pressure, which could cause some degree of oxidation in the extract and therefore oxidation products may be present in the final extract. Hermund et al. (2015) also found high initial concentration of both primary and secondary oxidation products when adding extracts obtained by SPE from Fucus vesiculosus to fish-oil-enriched milk. These milk samples were obtained by high-pressure homogenization with fish oil, giving rise to formation of oxidation products already during production of the samples. This indicates that some compounds in the seaweed extract are prooxidative under certain conditions. Similar reactions can occur in the lipophilic SCCO2E during production and oxidation products could be formed already at this stage. However, this deserves additional research.When EDTA was added to the mayonnaise no significant increase of either pentanal or 1-penten-3-ol was observed. For the formation of 1-penten-3-ol all samples with extracts addedshowed a lag phase of up to 7 days, but the final concentration was lower in all samples compared to the control. Hence, unlike for pentanal, no prooxidant activity of SCCO2E was observed for the formation of 1-penten-3-oil. Hence, all the seaweed extracts showed antioxidant activity against the formation of 1-penten-3-ol from day 14 (p < .05). Since, lipid hydroperoxides from n-3 PUFA undergo β-scission to form both pentanal and 1-penten-3-ol (which can be further reduced to 1-penten-3-one), the higher pentanal concentration in mayonnaise with SCCO2E added indicated that pentanal is present in the extract, as also discussed above.
Figure 5 shows a PCA bi-plot of the different secondary volatile oxidation products and how they relate to the samples. The sample with EDTA added was far away from the volatile compounds, meaning that they had low values of all volatiles, which was also shown in Figure 5 by very low concentrations of volatiles in this sample throughout the storage. In the samples with SCCO2E added pentanal, 1-pentanol and 2-heptenal were highly present, whereas the control and samples with SCWE or SCWE-R extract added contained other volatile compounds (2-ethylfuran, 1-penten-3-one, pentanal, 1-penten-3-ol, 2-pentenal, 1-pentanol, hexanal, 2-hexenal, heptanal, 2-heptenal, 2,4-heptadienal, t,c-2,6-nonadienal).
FIGURE 5. Principal component analysis (PCA) for the different volatile compounds. The blue arrows indicate the loadings. The different ellipse represents the confidence intervals of the analysis (95%). The arrow (→) shows the loadings of different volatiles analysed (2-ethlfuran, 1-penten-3-one, Pentanal, 1-penten-3-ol, 2-pentenal, 1-pentanol, Hexanal, 2-hexenal, Heptanal, 2-heptenal, 2,4-heptadienal, t,c-2,6-nonadienal).
The antioxidant activity of SCCO2E against the formation of 1-penten-3-ol can be related to the location of the extract, in the oil phase, where it reduced the decomposition of the lipid hydroperoxides to 1-penten-3-ol to a higher extent compared to the control than it could prevent the decomposition of lipid hydroperoxides to other volatile oxidation products such as pentanal. The precise mechanism for this difference needs to be further investigated.
No synergistic effect was observed by using the combination of water and oil soluble extracts. However, no clear conclusions can be made since SCCO2E itself may have had a high concentration of oxidation products. In a previous study on seaweed extracts added to skin care emulsions (oil-in-water emulsion with almond oil), synergistic effects between tocopherol in the oil phase (naturally present) and surface active phenolic compounds or pigments (from the seaweed extract) were found and were suggested to be due to regeneration of tocopherols from their oxidized tocopheroxyl radicals formed during oxidation in skin care emulsions containing seaweed extracts (Poyato et al., 2017). Therefore, it cannot be ruled out that more amphiphilic antioxidant compounds would be more efficient instead of having oil- or water-soluble antioxidants in the mayonnaise. This would need further investigation, e.g., in a partitioning study of the antioxidants in the extracts.
Table 5 shows the different tocopherol homologs (alfa, beta, and gamma) found in the samples, including the relative changes (RC%) observed during storage. Tocopherols are present in the oils used to produce the mayonnaise. The consumption of tocopherols during storage of the samples indicates the use of these as antioxidants and that oxidation is taking place (Frankel, 1998b). Alpha-tocopherol content decreased from day 0 to day 28 in the control mayonnaise, and in mayonnaise with SCCO2E or SCWE-R added, or when these two extracts were combined. This indicates that alpha-tocopherols are used as antioxidants and that the oxidation level might be higher in these samples. Alemán et al. (2015) also found degradation of α-tocopherol in mayonnaise during storage of 28 days. The findings are partly in agreement with the other oxidation results in the present study, which showed more oxidation when SCCO2E was added to the mayonnaise.
TABLE 5. Alpha-tocopherol content of the different mayonnaise during different storage days (day 0–28), including the relative tocopherol consumption during storage (RC%).
Lipid oxidation and subsequent quality degradation of foods and other consumer products is a major challenge in products containing unsaturated lipids. To address this challenge, antioxidants—mostly of synthetic origin-are added to slow down the formation of lipid hydroperoxides and their degradation/secondary oxidation products. However, the growing consumer awareness of the possible side effects of synthetic antioxidants has driven the quest for antioxidants from natural origins. In this study, hydrophilic and hydrophobic antioxidants were extracted from a Nordic seaweed F. vesiculosus using supercritical and carbon dioxide and subcritical water extraction technology. The extracts were added in different phases of mayonnaise formulations aiming to retard lipid oxidation in mayonnaise. Both hydrophilic and hydrophobic fractions showed retardation of lipid oxidation with hydrophilic extracts showing a better performance. Both SCWE and SCWER provided equally good antioxidant effects, probably due to the high level of metal chelating species in these extracts. No synergetic effect was observed between the hydrophilic and lipophilic extracts. However, further studies are required to understand which classes of compounds in the extracts are responsible for the retardation of lipid oxidation in the formulated mayonnaise.
The raw data supporting the conclusions of this article will be made available by the authors, without undue reservation.
Conceptualization, DH, AG, and CJ; formal analysis. WL; writing—original draft preparation, DH and AG; writing—review and editing, CJ and SH. All authors have read and agreed to the published version of the manuscript.
This work has received funding from the European Union’s Horizon 2020 research and innovation program under the Marie Sklodowska-Curie grant agreement no. 713683 (COFUNDfellowsDTU).
The authors declare that the research was conducted in the absence of any commercial or financial relationships that could be construed as a potential conflict of interest.
All claims expressed in this article are solely those of the authors and do not necessarily represent those of their affiliated organizations, or those of the publisher, the editors and the reviewers. Any product that may be evaluated in this article, or claim that may be made by its manufacturer, is not guaranteed or endorsed by the publisher.
The Supplementary Material for this article can be found online at: https://www.frontiersin.org/articles/10.3389/frfst.2022.1082490/full#supplementary-material
Agregán, R., Munekata, P. E., Domínguez, R., Carballo, J., Franco, D., and Lorenzo, J. M. (2017). Proximate composition, phenolic content and in vitro antioxidant activity of aqueous extracts of the seaweeds Ascophyllum nodosum, Bifurcaria bifurcata and Fucus vesiculosus. Effect of addition of the extracts on the oxidative stability of canola oil under accelerated storage conditions. Food Res. Int. 99, 986–994. doi:10.1016/J.FOODRES.2016.11.009
Alemán, M., Bou, R., Guardiola, F., Durand, E., Villeneuve, P., Jacobsen, C., et al. (2015). Antioxidative effect of lipophilized caffeic acid in fish oil enriched mayonnaise and milk. Food Chem. 167, 236–244. doi:10.1016/J.FOODCHEM.2014.06.083
Aocs Press, C. I. U. (1997). AOCS official method Ce 8-89 determination of tocopherols and tocotrienols in vegetable oils and fats, Champaign, IL: USA.
Charoensiddhi, S., Franco, C., Su, P., and Zhang, W. (2015). Improved antioxidant activities of Brown seaweed Ecklonia radiata extracts prepared by microwave-assisted enzymatic extraction. J. Appl. Phycol. 27, 2049–2058. doi:10.1007/s10811-014-0476-2
Das, A. K., Sharma, M., Mondal, D., and Prasad, K. (2016). Deep eutectic solvents as efficient solvent system for the extraction of κ-carrageenan from Kappaphycus alvarezii. Carbohydr. Polym. 136, 930–935. doi:10.1016/j.carbpol.2015.09.114
Getachew, A. T., Holdt, S. L., Meyer, A. S., and Jacobsen, C. (2022). Effect of extraction temperature on pressurized liquid extraction of bioactive compounds from Fucus vesiculosus. Mar. Drugs 20, 263. Page 263 20. doi:10.3390/MD20040263
Getachew, A. T., Jacobsen, C., and Holdt, S. L. (2020). Emerging technologies for the extraction of marine phenolics: Opportunities and challenges. Mar. Drugs 18, 389. doi:10.3390/md18080389
Getachew, A. T., Saravana, P. S., Cho, Y. J., Woo, H. C., and Chun, B. S. (2018). Concurrent extraction of oil from roasted coffee (Coffea arabica) and fucoxanthin from Brown seaweed (Saccharina japonica) using supercritical carbon dioxide. J. CO2 Util. 25, 137–146. doi:10.1016/j.jcou.2018.03.018
Hartvigsen, K., Lund, P., Hansen, L. F., and Holmer, G. (2000). Dynamic headspace gas chromatography/mass spectrometry characterization of volatiles produced in fish oil enriched mayonnaise during storage. J. Agric. Food Chem. 48, 4858–4867. doi:10.1021/JF991385B/ASSET/IMAGES/LARGE/JF991385BF00005
Hermund, D. B., Yeşiltaş, B., Honold, P., Jónsdóttir, R., Kristinsson, H. G., and Jacobsen, C. (2015). Characterisation and antioxidant evaluation of Icelandic F. vesiculosus extracts in vitro and in fish-oil-enriched milk and mayonnaise. J. Funct. Foods 19, 828–841. doi:10.1016/J.JFF.2015.02.020
Holdt, S. L., and Kraan, S. (2011). Bioactive compounds in seaweed: Functional food applications and legislation. J. Appl. Phycol. 23, 543–597. doi:10.1007/s10811-010-9632-5
Honold, P. J., Jacobsen, C., Jónsdóttir, R., Kristinsson, H. G., and Hermund, D. B. (2016). Potential seaweed-based food ingredients to inhibit lipid oxidation in fish-oil-enriched mayonnaise. Eur. Food Res. Technol. 242, 571–584. doi:10.1007/s00217-015-2567-y
Huang, S. W., Hopia, A., Schwarz, K., Frankel, E. N., and German, J. B. (1996). Antioxidant activity of α-tocopherol and trolox in different lipid substrates: Bulk oils vs oil-in-water emulsions. J. Agric. Food Chem. 44, 444–452. doi:10.1021/JF9505685
Jacobsen, C., Hartvigsen, K., Lund, P., Adler-Nissen, J., Hølmer, G., Meyer, A. S., et al. (2000). Oxidation in fish-oil-enriched mayonnaise. Eur. Food Res. Technol. 2104 210, 242–257. doi:10.1007/S002179900070
Jacobsen, C., Hartvigsen, K., Lund, P., Meyer, A. S., Adler-Nissen, J., Holstborg, J., et al. (1999). Oxidation in fish-oil-enriched mayonnaise. Eur. Food Res. Technol. 1 210, 13–30. doi:10.1007/S002170050526
Jacobsen, C., Hartvigsen, K., Thomsen, M. K., Hansen, L. F., Lund, P., Skibsted, L. H., et al. (2001). Lipid oxidation in fish oil enriched mayonnaise: Calcium disodium ethylenediaminetetraacetate, but not gallic acid, strongly inhibited oxidative deterioration. J. Agric. Food Chem. 49, 1009–1019. doi:10.1021/JF000729R
Ljubic, A., Safafar, H., and Jacobsen, C. (2019). Recovery of microalgal biomass and metabolites from homogenized, swirl flash-dried microalgae. J. Appl. Phycol. 31, 2355–2363. doi:10.1007/s10811-019-1733-1
Meyer, A. S., Jacobsen’, C., Meyer, A. S., and Jacobsen, C. (1996). The fate the synergistic antioxidant system ascorbic acid, lecithin, and tocopherol in mayonnaise: Partition of ascorbic acid. J. Food Lipids 3, 139–147. doi:10.1111/J.1745-4522.1996.TB00061.X
Moreira, R., Sineiro, J., Chenlo, F., Arufe, S., and Díaz-Varela, D. (2017). Aqueous extracts of Ascophyllum nodosum obtained by ultrasound-assisted extraction: Effects of drying temperature of seaweed on the properties of extracts. J. Appl. Phycol. 29, 3191–3200. doi:10.1007/s10811-017-1159-6
Obluchinskaya, E. D., Daurtseva, A. v., Pozharitskaya, O. N., Flisyuk, E. v., and Shikov, A. N. (2019). Natural deep eutectic solvents as alternatives for extracting phlorotannins from Brown algae. Pharm. Chem. J. 53, 243–247. doi:10.1007/s11094-019-01987-0
Oliveira, C. Y. B., Abreu, J. L., Santos, E. P., Matos, Â. P., Tribuzi, G., Oliveira, C. D. L., et al. (2022). Light induces peridinin and docosahexaenoic acid accumulation in the dinoflagellate Durusdinium glynnii. Appl. Microbiol. Biotechnol. 106, 6263–6276. doi:10.1007/s00253-022-12131-6
Pereira, S. G., Teixeira-Guedes, C., Souza-Matos, G., Maricato, É., Nunes, C., Coimbra, M. A., et al. (2021). Influence of ohmic heating in the composition of extracts from Gracilaria vermiculophylla. Algal Res. 58, 102360. doi:10.1016/J.ALGAL.2021.102360
Poyato, C., Thomsen, B. R., Hermund, D. B., Ansorena, D., Astiasarán, I., Jónsdóttir, R., et al. (2017). Antioxidant effect of water and acetone extracts of Fucus vesiculosus on oxidative stability of skin care emulsions. Eur. J. Lipid Sci. Technol. 119, 1600072. doi:10.1002/EJLT.201600072
Robin, A., Kazir, M., Sack, M., Israel, A., Frey, W., Mueller, G., et al. (2018). Functional protein concentrates extracted from the green marine macroalga ulva sp., by high voltage pulsed electric fields and mechanical press. ACS Sustain. Chem. Eng. 6, 13696–13705. doi:10.1021/acssuschemeng.8b01089
Sabeena Farvin, K. H., Grejsen, H. D., and Jacobsen, C. (2012). Potato peel extract as a natural antioxidant in chilled storage of minced horse mackerel (Trachurus trachurus): Effect on lipid and protein oxidation. Food Chem. 131, 843–851. doi:10.1016/J.FOODCHEM.2011.09.056
Sabeena Farvin, K. H., and Jacobsen, C. (2013). Phenolic compounds and antioxidant activities of selected species of seaweeds from Danish coast. Food Chem. 138, 1670–1681. doi:10.1016/j.foodchem.2012.10.078
Saravana, P. S., Getachew, A. T., Cho, Y.-J., Choi, J. H., Park, Y. B., Woo, H. C., et al. (2017). Influence of co-solvents on fucoxanthin and phlorotannin recovery from Brown seaweed using supercritical CO2. J. Supercrit. Fluids 120, 295–303. doi:10.1016/j.supflu.2016.05.037
Shantha, N. C., and Decker, E. A. (1994). Rapid, sensitive, iron-based spectrophotometric methods for determination of peroxide values of food lipids. J. AOAC Int. 77, 421–424. doi:10.1093/JAOAC/77.2.421
Sumampouw, G. A., Jacobsen, C., and Getachew, A. T. (2021). Optimization of phenolic antioxidants extraction from Fucus vesiculosus by pressurized liquid extraction. J. Appl. Phycol. 33, 1195–1207. doi:10.1007/s10811-020-02362-3
Keywords: Fucus vesiculosus, lipid oxidation, Brown seaweed, sub and supercritical fluids, green extraction technology, omega-3
Citation: Hermund DB, Liu W, Holdt SL, Jacobsen C and Getachew AT (2023) Extraction of bioactives from brown seaweed using sub and supercritical fluids: Influence of the extract on the storage stability of fish oil enrich mayonnaise. Front. Food. Sci. Technol. 2:1082490. doi: 10.3389/frfst.2022.1082490
Received: 28 October 2022; Accepted: 22 December 2022;
Published: 06 January 2023.
Edited by:
Giustino Tribuzi, Federal University of Santa Catarina, BrazilReviewed by:
Rui M. S. Cruz, Universidade do Algarve, PortugalCopyright © 2023 Hermund, Liu, Holdt, Jacobsen and Getachew. This is an open-access article distributed under the terms of the Creative Commons Attribution License (CC BY). The use, distribution or reproduction in other forums is permitted, provided the original author(s) and the copyright owner(s) are credited and that the original publication in this journal is cited, in accordance with accepted academic practice. No use, distribution or reproduction is permitted which does not comply with these terms.
*Correspondence: Adane Tilahun Getachew, YXRpZ2VAZm9vZC5kdHUuZGs=
Disclaimer: All claims expressed in this article are solely those of the authors and do not necessarily represent those of their affiliated organizations, or those of the publisher, the editors and the reviewers. Any product that may be evaluated in this article or claim that may be made by its manufacturer is not guaranteed or endorsed by the publisher.
Research integrity at Frontiers
Learn more about the work of our research integrity team to safeguard the quality of each article we publish.