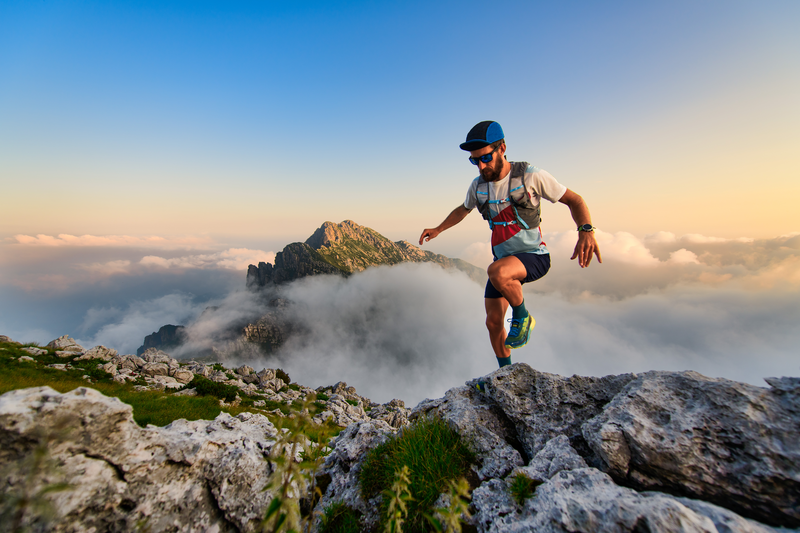
94% of researchers rate our articles as excellent or good
Learn more about the work of our research integrity team to safeguard the quality of each article we publish.
Find out more
ORIGINAL RESEARCH article
Front. Environ. Sci. , 04 March 2024
Sec. Toxicology, Pollution and the Environment
Volume 12 - 2024 | https://doi.org/10.3389/fenvs.2024.1284198
This article is part of the Research Topic Toxic Effects of Emerging Pollutants on Crop Plants and Soil Biological Remediation Technologies View all articles
This study aimed to investigate whether and how nighttime warming (NW) influences the copper (Cu) bioaccumulation of wheat (Triticum aestivum L.) under Cu pollution. We examined plant growth, Cu concentration, and antioxidant enzymes of wheat after 6 months of Cu exposure (0, 100, 400, and 800 mg/kg) under NW. The results indicated that, NW of 1.54°C significantly promoted Cu translocation from root to shoot through an increasing root system under increased Cu treatments (p < 0.05). The Cu contents of grains were significantly increased by NW under 800 mg/kg Cu treatment (p < 0.05). NW decreased superoxide dismutase (SOD) activity under increased Cu treatments (p < 0.05). There were significantly negative correlations between biomass and Cu concentrations of the same part under NW (p < 0.05). The root biomass significantly increased by 51% in NW compared with nonnighttime warming (CK) (p < 0.05). These results provided evidence that wheat might respond to NW by altering their roots so that they were larger, thinner, and longer, suggesting that soil Cu translocation from root to shoot would be accelerated resulting from the expanded roots under elevated temperatures, and thus forewarned the food safety risks of climate change in agricultural ecosystem.
According to the IPCC Sixth Assessment Report, the average global surface temperature has increased by 1.04 °C from 2014 to 2018, and the annual global warming was expected to increase at 1.5°C from 2021 to 2030 (Intergovernmental Panel on Climate Change, IPCC, 2021). Climate warming has obvious asymmetric warming characteristics, that is, the temperature increase at night is greater than that during the day, and the temperature increase in winter and spring is significantly higher than that in summer and autumn (Moore et al., 2021). Temperature is an important factor affecting crop growth, physiology, and yield (Hoegh-Guldberg, et al., 2019). “Chemical time bombs” have indicated the intrinsic relationship between global warming and soil heavy metal pollution (Stigliani et al., 1991). Climate warming may directly lead to changes in the physical and chemical properties of soil, and the transformation and availability of heavy metals in soil will increase, which will increase the possibility of metal transfer from soils to plants. Nighttime warming (NW) affects crop growth and development, which will change the element (e.g., carbon, and nitrogen) absorption of roots (Sadok and Jagadish, 2020) and potentially affect the redistribution of elements in plants (Ge et al., 2016). Previous reports found that NW led to the acidification of the soil, and affected the bioavailability and mobilization of heavy metals (Bibi and Rahman, 2023). So far, it is rare to study the effects of root growth and morphological changes on heavy metal accumulation and transport in plants under NW.
Copper (Cu) pollution in agricultural soils from sewage sludge and fertilizer application has been a major environmental problem, which is deleterious to crop safety and production. Cu has been widely used in agriculture as a trace element additive to increase growth performance and prevent disease in livestock and poultry production (El Sabry et al., 2021). Wide abuse of Cu additives enriches Cu ions in animal manure and litter, which results in high levels of Cu entering the agricultural environments (Xiang et al., 2021). In addition, Cu fertilizers have been widely adopted to prevent Cu deficiency in crops on Cu-deficient soils (Kumar et al., 2021). Repeated use of Cu fertilizer can also lead to Cu accumulation in soils. In summary, the continued application of Cu additive feed and fertilizer in agricultural environment will cumulatively elevate the Cu loading in soil, which may increase the potential of Cu uptake by plants and the opportunity for Cu entrance into the food chain.
Wheat (Triticum aestivum L.) is one of the major staple foods all over the world, with approximately 30% of the world’s cereal plants. The migration and enrichment of Cu in soil–crop systems are recognized to be the main sources of human health risk. Previous studies have indicated that wheat is more likely to accumulate heavy metals than other grain plants, such as corn (Wan et al., 2017). In recent years, selecting and breeding wheat cultivars to minimize heavy metals have been important tools for reducing the risk of heavy metal movement into the human diet (Li et al., 2022). For instance, Zhongmai 175 has been identified as a variety of low accumulation of heavy metals in grain and straw (Liu et al., 2020). The asymmetric warming overlaps with the growth period of wheat. Asymmetric climate warming will inevitably pose a great potential impact on the growth and development of wheat (Misiou and Koutsoumanis, 2022). Under the irreversible trend of global warming, no relevant report exists on the effect of increasing temperature on the enrichment of Cu in wheat. Climate warming brings great uncertainty to wheat quality, so it is imperative to understand the accumulation patterns of Cu in wheat to cope with climate change and ensure food security.
This study was designed to investigate whether and how climate warming will change the impact of Cu pollution on the growth, Cu concentration, and antioxidant defense of wheat (T. aestivum L.) under Cu pollution, which was created by the dilution of pH-neutral soils from Cu-processing plant. We hypothesized that i) NW can regulate the growth and development of wheat, in which increasing Cu concentrations enhance the root biomass and absorption area; ii) changes in root biomass and absorption area will promote Cu translocation from root to shoot due to the linkage of root biomass and translocation factor; and iii) NW deteriorates Cu tolerance by inhibiting antioxidant enzyme due to the induction of oxide damage by Cu ions.
The soil was collected from the topsoil (0–20 cm) layer of an experimental farm in Luoyang (34°38′N, 112°22′E) of China, which is a rainfed agricultural area with an annual average temperature of 13.7°C and an annual average rainfall of 650.2 mm. The soil used in this experiment was classified as fluvo-aquic soil (Inceptisol). Before the pot experiment, visible rocks, roots, and fresh litter were removed. The soil was sieved to 2 mm and air-dried at room temperature to a constant weight. Soil pH was measured in soil: water suspension (1:2.5, v/v) with a pH meter. Soil cation exchange capacity was determined by ammonium acetate method. Soil total carbon (TC) and total nitrogen (TN) were measured with a CN-element analyzer (Elementar VarioMax CN, New Jersey, United States). Nitrate nitrogen (
Wheat (Zhongmai 175) was chosen as the experimental material, which has been identified as the variety of low accumulation of heavy metals, and is widely grown in China. Seeds were obtained from the Henan Academy of Agricultural Sciences. Wheat seeds of similar size were selected. After 20 min of disinfection on the surface with 0.2% sodium hypochlorite, the seeds were soaked in water for 10 min to remove bacteria from the epidermis of the seed and air dried. The seeds germinated on the well-drained plastic tray and incubated in an artificial climate chamber in the dark with a relative humidity of 90% and a temperature of 25°C. Wheat seedlings were transplanted to pots when the wheat seedling has three true leaves.
The analytically pure reagent CuSO4-5H2O was dissolved in water to make a solution, which was applied to the pots to set four levels of Cu treatments: no Cu application (Control), 100, 400, and 800 mg kg-1, mixed thoroughly. The experiment was carried out in a plastic pot (height: 16 cm, bottom diameter: 12 cm, caliber: 22 cm), filled with fresh soil (2 kg dry weight equivalent) in every pot. The three Cu applications represented three exposure scenarios: the predicted environmental background, the possible heavy metal hotspots in soil, and the relatively high dose to explore potential toxic mechanisms (Warne et al., 2008). The soil with no Cu addition was the control. Then, the soil was placed for over a year to allow metal equilibrium. The soil water content was maintained by weighing the pots at 2 week intervals and adding water to replace lost water. No additional fertilizer was added in the pots. After that, every pot was transferred with 12 seedlings (seedling preparation described as before). Each treatment had four replicates. The pot experiment was started from 11 November 2021 to 31 May 2022 in Luoyang City, Henan Province of China. The soil water content was maintained by weighing the pots at a week and adding water to replace lost water. No additional fertilizer was added in the pots during the experiment.
Two levels of temperature treatments were set: nonnighttime warming (CK) and nighttime warming (NW). The field-warming zone adopted a passive nighttime warming system that intercepted surface long-wave radiation at night to increase the temperature (Speights et al., 2018). The system was mainly composed of a horizontal frame that could adjust the lifting height and a covering material that could be retracted on the frame, which was used to increase the temperature during the entire growth period. The temperature coverage time was from 19:00 in the evening on the first day to 7:00 on the second day, and the coverage height was 20 cm directly above the canopy. No obstruction existed around the wheat canopy, and the warming zone was not covered to reduce the influence of precipitation difference. During the experiment, an automatic temperature recorder (JC12BZDR-41, Beijing Jingcheng Huatai Instrument Co., Ltd.) was used to measure the temperatures of the soil plow layer, which were recorded every 10 min, and the recorded temperatures were averaged to obtain the average soil temperatures during the daytime and nighttime periods of each treatment.
After 6 months of Cu treatments and temperature treatment, the above-ground part of six seedlings were harvested before mature time. The fresh leaves were sampled for the determination of antioxidant capacity. The content of malondialdehyde (MDA) was determined by thiobarbituric acid method; the activity of superoxide dismutase (SOD) was determined by nitro-blue tetrazole method; the activity of peroxidase (POD) was measured with guaiacol (Aazami et al., 2021).
Plants were harvested after 6 months of Cu treatments and temperature treatment. The plants were initially washed in diluted water to remove the soil, then soaked in 1.0 mol/L CaCl2 solution, and discarded Cu2+ ion around the roots, and finally washed continuously three times in diluted water to remove CaCl2. The harvested plants were separated into their leaf, stem, and root parts and placed into different envelopes. The dry weights of roots, stems, and leaves were obtained after oven drying from 55°C to 60°C until their weight reached constant, and the plant weights were then determined.
The root systems were scanned at a resolution of 3,000 dpi using an Epson Expression 10000XL scanner (Seiko Epson Corporation, Nagano, Japan). Root morphology included root length, surface area, average diameter, and volume. Root-specific area was the ratio of surface area and volume and was determined by analyzing the scanned images using WinRHIZO version Pro 2007d software (Regent Instruments Inc., Quebec Canada). The roots were collected according to a previous study (Kou et al., 2019).
Dried plant samples (approximately 0.5 g) were ashed by heating at 250°C for 0.5 h, which was then gradually increased to 500°C for 2 h. Ashed samples were digested in 8 mL of mixed acid [V (HClO4): V (HNO3) = 1:1] for 1 h. The digests were analyzed using ICP/AES and MS techniques for Cu concentration. Shoot Cu concentration was calculated as (leaf mass×leaf concentration + straw mass×straw concentration)/(leaf mass + straw mass). The translocation factor (TF) is the ratio of shoot metal concentration to root metal concentration. TF is usually greater than 1 for tolerant plants and less than 1 for nontolerant plants.
The promotion rate of the above parameters, defined as [(NW−CK)/CK]×100, was linearly regressed against the Cu concentration in the soils to explore whether NW could regulate the effect of Cu pollution on biomass, Cu concentration, and antioxidant responses of wheat. After data normality distribution and variance homogeneity were satisfied, two-way ANOVA was conducted to analyze the effects of Cu pollution, NW, and their interactions on biomass, Cu concentration, and antioxidant responses of wheat. One-way ANOVA, in conjunction with a post hoc least significant difference test, was used to determine the significant differences (p < 0.05) of the above parameters among treatments. The PCoA based on the Bray–Curtis distance matrices was performed to visualize the overall differences in Cu concentrations of wheat across treatments. Spearman’s correlation analysis was conducted to analyze the relationship among biomass, Cu concentration, and antioxidant responses of wheat. Analyses were conducted using either R (http://www.r-project.org/) or SPSS (SPSS, Chicago, United States).
During the entire wheat growth period, the soil temperature difference between the warming zone and normal temperature treatments varied from −0.09°C to 3.48°C, and the average temperature was increased by 1.54°C (Figure 1A), which was within the range of climatic warming (1.5°C–2°C) predicted to occur by 2030 (IPCC, 2021). This result indicated that the passive NW system in this study could achieve the effect of increasing the soil-plant system temperature. The pattern of diurnal temperature change of the nighttime warming treatment was −0.37°C to 3.20°C, and the average temperature was increased by 1.23°C (Figure 1B). The curtains were not spread out to cover the field when it rained or snowed; consequently, the difference in the mean air temperature among the treatments was relatively low on some days (Figure 1). Thus, the variations in the warming effect of the NW facility were acceptable for this field experiment.
FIGURE 1. Differences in nighttime mean temperature between the warmed and unwarmed plots from 2017 to 2018 growing seasons. (A) Differences in nighttime mean temperature between the nighttime warming and nonnighttime warming pots, and (B) Diurnal changes of wheat canopy mean air temperatures under the passive nighttime warming facility.
In this study, NW did not affact the biomass of the aboveground parts (e.g., leaf, stem, glume, and grain, p > 0.05; Supplementary Table S2). The regression analysis was applied to compare the different effects of warming treatments (CK and NW) on wheat biomass under Cu treatments. A significant linear regression was found between the promotion rate of root biomass and Cu treatments (R2 = 0.420, p = 0.046; Figure 2A and Supplementary Table S3), indicating that NW promoted the root growth of wheat in the presence of Cu pollution. One-way ANOVA was used to illustrate how the NW was involved in the promotion of the root growth under Cu treatments. At 800 mg/kg Cu treatment (the highest Cu pollution), the root biomass significantly increased by 51% in NW compared with CK (p = 0.038; Supplementary Figure S1). By contrast, previous studies have documented that decreases in root biomass, root number, total length, and metabolic activities of the root system are evidenced as typically injurious effects of NW (Wu et al., 2014). Nevertheless, heavy metal could stimulate lateral root formation, increase the number of fine roots and root hairs, and accelerate the turnover of fine roots to increase the root absorption area (Hu et al., 2013). Consistently, we found that NW increased the fine roots (decreased the root diameter, R2 = 0.508, p = 0.018; Figure 2B and Supplementary Table S3), the root length (R2 = 0.598, p = 0.006; Figure 2C and Supplementary Table S3) and enlarged the active sites (specific area, R2 = 0.412, p = 0.048; Figure 2D and Supplementary Table S3) with increasing Cu treatment. Plants could invest in expanding their root system to increase the uptake of water, nutrients, and trace elements to promote plant growth (Jiang et al., 2022). For example, grain biomass of 400 mg/kg treatment was increased by 59% (p = 0.021, Supplementary Figure S2A) in NW compared with CK.
FIGURE 2. Effects of nighttime warming on root morphology under Cu treatments. (A) The regression of the promotion rates of root biomass and Cu treatments. (B) The regression of the promotion rates of root diameter and Cu treatments. (C) The regression of the promotion rates of root length and Cu treatments. (D) The regression of the promotion rates of root specific area and Cu treatments. Error bars indicate the standard deviation of four independent replicates. Cu treatments refers to Control (non-Cu addition), L (100 mg/kg Cu treatment), M (400 mg/kg Cu treatment), and H (800 mg/kg Cu treatment).
The root system is responsible for the absorption of trace elements from soils. A large root system (large biomass and absorption area) is expected to have a strong capacity for element uptake. Correspondingly, we found a significant positive correlation between root biomass and TF (R = 0.762, p < 0.001; Figure 3A). An increase of trace element absorption was expected under climate warming, given the increases in soil enzyme activity and resource demands by the photosynthetic and growing tissues. A significant linear regression was found between the promotion rate of TF and Cu treatments (R2 = 0.780, p < 0.001; Figure 4A). However, no linear regression was found in the promotion rate of root, grain, and shoot Cu concentration with Cu treatments (p > 0.05; Supplementary Table S3). The TF of wheat in NW was significantly higher than that in CK under Cu treatments (p < 0.05 for 100, 400, and 800 mg/kg; Figure 4B). These results indicated that NW would promote Cu translocation from root to shoot with increasing Cu treatment. NW promoted Cu accumulation in the edible parts of wheat (e.g., grain, and straw), which would increase the opportunity for heavy metal entry into humans by the way of the food chain. Furthermore, climatic warming can also affect plant metabolism and the internal distribution of elements, thereby changing the mobilization and translocation of elements within plant organs (Anderegg et al., 2015; Querejeta et al., 2021). Consistently, we found a significant positive correlation between root biomass and grain Cu content (R = 0.437, p = 0.033; Figure 3B). Also, we further found that NW enhanced the Cu bioaccumulation of grain at the control and the highest Cu treatment (800 mg/kg, Supplementary Figure S2A and Supplementary Table S4). The effect of warming on increasing Cu in aboveground parts can enhance the future retention capacity of the anthropogenic source of these elements if warming continues in the next decades. There were significant differences in root Cu concentration and grain Cu concentration between CK and NW, but there were no significant differences in the Cu concentration of other parts, including leaf, stem, and husk between CK and NW (Supplementary Figure S2). Since leaves, stems, and husks represented the majority of biomass, we found no significant difference between CK and NW before planting and after harvest (Supplementary Table S5).
FIGURE 3. Larger root biomass would promote Cu translocation from root to grain. (A) Spearman correlation between root biomass and translocation factor of wheat (n = 21). (B) Spearman correlation between root biomass and grain Cu concentration of wheat (n = 24). Grain Cu content was calculated as grain Cu concentration × grain biomass.
FIGURE 4. Effects of nighttime warming on the translocation factor of wheat under Cu treatments. (A) The regression of the promotion rates of translocation factor and Cu treatment. (B) The effects of nighttime warming on translocation factor illustrated by one way ANOVA. Error bars indicate the standard deviation of four independent replicates. Warming treatments refers to nonnighttime warming (CK), and nighttime warming (NW). Cu treatments refers to non-Cu addition (Control), 100 mg/kg Cu treatment (L), 400 mg/kg Cu treatment (M), and 800 mg/kg Cu treatment (H). *p < 0.05.
The responses of plants to adversary environments involve a sequential reaction of regulation of enzyme production and activity, and the extent varies depending on the plant species and their tissues and corresponding stress types and intensity (Nahar et al., 2016). Considerable studies have documented that plants develop defense or protective mechanisms against reactive oxygen species, including H2O2, OH, and
FIGURE 5. Regression of the promotion rates of superoxide dismutase (SOD) activity and Cu treatments. Error bars indicate the standard deviation of four independent replicates.
The relationships of all indicators (e.g., antioxidant resistance, biomass, and Cu concentration) under CK and NW were analyzed through spearman correlation. The results showed that the correlation between biomass and Cu concentration was shifted by NW. For instance, the positive correlation between root biomass and TF in CK was altered in NW (no correlation), and the same result was found in the correlation between shoot-to-root ratio and stem Cu concentration. NW increased the negative correlations between the biomass and Cu concentration. Twenty-three significant negative correlations existed between plant growth and Cu bioaccumulation under NW. Among them, only 2 negative correlations (root biomass and Cu concentration and leaf biomass and Cu concentration) were shown under CK, whereas the other 21 correlations were nonexistent in CK (Figure 6). For instance, no correlation occurred between grain biomass and grain Cu concentration in CK, although a negative correlation between them emerged in NW (Figure 6). Previous studies found NW enhanced antioxidative activities (Farooq et al., 2014; Fan et al., 2017). The most probable reason is that plants might respond with greater production of SOD to combat heat-induced injuries. However, an increase in the activity of ROS (reactive oxygen species) of plants occurs under the dual pressure of NW and Cu treatment. In such circumstances, high temperatures might deteriorate the tolerance of wheat by inhibiting SOD activity under increasing Cu treatment. NW acted as a stimulator to promote wheat growth (Chen et al., 2014), which, in turn, reduced the wheat Cu concentration to alleviate the influence of Cu on the growth and development of wheat. In case of the same heavy metal content in plants, increased biomass would correspondingly reduce the heavy metal concentration of wheat. NW might alleviate the damage of Cu lions to wheat by increasing biomass in this way to reduce Cu concentrations of wheat.
FIGURE 6. Spearman’s correlations between plant biomass, root morphology, Cu concentration, and antioxidant resistance (n = 24). Only statistically-significant relationships (p < 0.05) are shown, and the colored (red/blue) plots represent significant correlation. The lower part was extracted from nonnighttime warming treatment, and the upper part was extracted from nighttime warming treatment. TF: Translocation factor; MDA: malondialdehyde; SOD: Superoxide dismutase; POD: Peroxidase.
NW significantly increased root biomass (p = 0.046), fine roots (decreased the root diameter, p = 0.018), and the root length (p = 0.006), and enlarged the active sites (specific area, p = 0.048) as increased Cu treatments. Root biomass was significantly correlated with TF (R = 0.636, p < 0.001), and grain Cu content (R = 0.437, p = 0.033). NW significantly increased the Cu concentration of grain in 800 mg/kg Cu treatment (p < 0.05). NW stimulated more extensive root systems and higher Cu accumulation in grain of wheat as increased Cu pollution. Such evidence suggested that climate warming would deteriorate high-quality wheat production with increasingly heavy Cu pollution in soil, which would pose the potential risk of Cu pollution for wheat grain production. These findings would shed light on the future development of new adaptation strategies under the warming climate.
The raw data supporting the conclusion of this article will be made available by the authors, without undue reservation.
LQ: Writing–original draft, Writing–review and editing. CL: Data curation, Formal Analysis, Writing–review and editing. LL: Data curation, Methodology, Writing–review and editing. TK: Data curation, Formal Analysis, Funding acquisition, Investigation, Writing–original draft. YD: Investigation, Methodology, Writing–review and editing.
The author(s) declare that financial support was received for the research, authorship, and/or publication of this article. This work was supported by Natural Science Foundation of Henan Province (222300420147), Youth Foundation of Henan University of Science and Technology (2014QN065), Henan University of Science and Technology Discipline Improvement and Promotion Plan A (13660001), and the Foundation of National Research and Observation Station of Shangqiu Agro-ecology System, Chinese Academy of Agricultural Sciences (FIRI2019-02-0104).
The authors declare that the research was conducted in the absence of any commercial or financial relationships that could be construed as a potential conflict of interest.
All claims expressed in this article are solely those of the authors and do not necessarily represent those of their affiliated organizations, or those of the publisher, the editors and the reviewers. Any product that may be evaluated in this article, or claim that may be made by its manufacturer, is not guaranteed or endorsed by the publisher.
The Supplementary Material for this article can be found online at: https://www.frontiersin.org/articles/10.3389/fenvs.2024.1284198/full#supplementary-material
Aazami, M. A., Rasouli, F., and Ebrahimzadeh, A. (2021). Oxidative damage, antioxidant mechanism and gene expression in tomato responding to salinity stress under in vitro conditions and application of iron and zinc oxide nanoparticles on callus induction and plant regeneration. BMC Plant Biol. 21, 597. doi:10.1186/s12870-021-03379-7
Anderegg, W. R. L., Ballantyne, A. P., Smith, W. K., Majkut, J., Rabin, S., Beaulieu, C., et al. (2015). Tropical nighttime warming as a dominant driver of variability in the terrestrial carbon sink. Proc. Natl. Acad. Sci. U. S. A. 112, 15591–15596. doi:10.1073/pnas.1521479112
Bibi, F., and Rahman, A. (2023). An overview of climate change impacts on agriculture and their mitigation strategies. Agriculture 13, 1508. doi:10.3390/agriculture13081508
Chen, J., Tian, Y., Zhang, X., Zheng, C., Song, Z., and Deng, A. (2014). Nighttime warming will increase winter wheat yield through improving plant development and grain growth in North China. J. Plant Growth Regul. 33, 397–407. doi:10.1007/s00344-013-9390-0
El Sabry, M. I., Stino, F. K. R., and El-Ghany, W. A. A. (2021). Copper: benefits and risks for poultry, livestock, and fish production. Trop. Anim. Health Prod. 53, 487. doi:10.1007/s11250-021-02915-9
Fan, Y., Miguez-Macho, G., Jobbágy, E. G., Jackson, R. B., Otero-Casal, C., et al. (2017). Hydrologic regulation of plant rooting depth. Proc. Natl. Acad. Sci. U. S. A. 114(40), 10572–10577. doi:10.1073/pnas.1712381114
Fan, Y., Lv, Z., Ge, T., Li, T., Yang, W., Zhang, W., et al. (2021). Night-warming priming at the vegetative stage alleviates damage to the flag leaf caused by post-anthesis warming in winter wheat (Triticum aestivum L.). Front. plant Sci. 12, 706567. doi:10.3389/fpls.2021.706567
Farooq, M., Hussain, M., and Siddique, K. H. M. (2014). Drought stress in wheat during flowering and grain-filling periods. Crit. Rev. Plant Sci. 33, 331–349. doi:10.1080/07352689.2014.875291
Ge, L. Q., Cang, L., Liu, H., and Zhou, D. M. (2016). Effects of warming on uptake and translocation of cadmium (Cd) and copper (Cu) in a contaminated soil-rice system under Free Air Temperature Increase (FATI). Chemosphere 155, 1–8. doi:10.1016/j.chemosphere.2016.04.032
Hoegh-Guldberg, O., Jacob, D., Taylor, M., Bolaños, T. G., Bindi, M., Brown, S., et al. (2019). The human imperative of stabilizing global climate change at 1.5°C. Science 365, eaaw6974. doi:10.1126/science.aaw6974
Hu, Y., Zhou, G., Na, X., Yang, L., Nan, W., Liu, X., et al. (2013). Cadmium interferes with maintenance of auxin homeostasis in arabidopsis seedlings. J. Plant Physiol. 170, 965–975. doi:10.1016/j.jplph.2013.02.008
IPCC (2021). Climate change 2021: the physical science Ba-sis. Summary for policymakers. Cambridge: CambridgeUniversity Press.
Jiang, Q., Jia, L., Wang, X., Chen, W., Xiong, D., Chen, S., et al. (2022). Soil warming alters fine root lifespan, phenology, and architecture in a Cunninghamia lanceolata plantation. Agric. For. Meteorol. 327, 109201. doi:10.1016/j.agrformet.2022.109201
Kou, T., Lai, L., Lam, S., Chen, D., and He, J. (2019). Increased carbon loss via root respiration and impaired root morphology under free -air ozone enrichment adversely affect rice (Oryza Sativa L.) Production. Exp. Agric. 55, 500–508. doi:10.1017/s0014479718000170
Kumar, V., Pandita, S., Sidhu, G. P. S., Sharma, A., Khanna, K., Kaur, P., et al. (2021). Copper bioavailability, uptake, toxicity and tolerance in plants: a comprehensive review. Chemosphere 262, 127810. doi:10.1016/j.chemosphere.2020.127810
Li, S., Huang, X., Liu, N., Chen, Y., He, H., Cao, X., et al. (2022). Selection of low-cadmium and high-micronutrient wheat cultivars and exploration of the relationship between agronomic traits and grain cadmium. Environ. Sci. Pollut. R. 29, 42884–42898. doi:10.1007/s11356-022-18763-5
Liu, N., Huang, X., Sun, L., Li, S., Chen, Y., Cao, X., et al. (2020). Screening stably low cadmium and moderately high micronutrients wheat cultivars under three different agricultural environments of China. Chemosphere 241, 125065. doi:10.1016/j.chemosphere.2019.125065
Manquián-Cerda, K., Escudey, M., Zúñiga, G., Arancibia-Miranda, N., Molina, M., and Cruces, E. (2016). Effect of cadmium on phenolic compounds, antioxidant enzyme activity and oxidative stress in blueberry (Vaccinium corymbosum L.) plantlets grown in vitro. Ecotox Environ. Safe 133, 316–326. doi:10.1016/j.ecoenv.2016.07.029
Misiou, O., and Koutsoumanis, K. (2022). Climate change and its implications for food safety and spoilage. Trends Food Sci. Tech. 126, 142–152. doi:10.1016/j.tifs.2021.03.031
Moore, C. E., Meacham-Hensold, K., Lemonnier, P., Slattery, R. A., Benjamin, C., Bernacchi, C. J., et al. (2021). The effect of increasing temperature on crop photosynthesis: from enzymes to ecosystems. J. Exp. Bot. 72, 2822–2844. doi:10.1093/jxb/erab090
Nahar, K., Hasanuzzaman, M., Alam, M. M., Rahman, A., Suzuki, T., and Fujita, M. (2016). Polyamine and nitric oxide crosstalk: antagonistic effects on cadmium toxicity in mung bean plants through upregulating the metal detoxification, antioxidant defense and methylglyoxal detoxification systems. Ecotox Environ. Safe 126, 245–255. doi:10.1016/j.ecoenv.2015.12.026
Querejeta, J. I., Ren, W., and Prieto, I. (2021). Vertical decoupling of soil nutrients and water under climate warming reduces plant cumulative nutrient uptake, water-use efficiency and productivity. New Phytol. 230, 1378–1393. doi:10.1111/nph.17258
Sadok, W., and Jagadish, S. V. K. (2020). The hidden costs of nighttime warming on yields. Trends Plant Sci. 25, 644–651. doi:10.1016/j.tplants.2020.02.003
Sharma, P., Kumar, A., and Bhardwaj, R. (2016). Plant steroidal hormone epibrassinolide regulate – heavy metal stress tolerance in Oryza Sativa L. by modulating antioxidant defense expression. Environ. Exp. Bot. 122, 1–9. doi:10.1016/j.envexpbot.2015.08.005
Speights, C. J., Wolff, C. L., Barton, M. E., and Barton, B. T. (2018). Why and how to create nighttime warming treatments for ecological field experiments. Yale J. Biol. Med. 91, 471–480.
Stigliani, W. M., Doelman, P., Salomons, W., Schulin, R., Smidt, G. R. B., and Van der Zee, SEATM (1991). Chemical time bombs. Predicting the unpredictable. Environment 33 (5 -9), 4–30. doi:10.1080/00139157.1991.9931383
Wan, S., Wu, W., Liu, F., Liao, R., and Hu, Y. (2017). Accumulation of heavy metals in soil-crop systems: a review for wheat and corn. Environ. Sci. Pollut. R. 24, 15209–15225. doi:10.1007/s11356-017-8909-5
Warne, M. S. J., Heemsbergen, D., Stevens, D., Cozens, M. M. G., Whatmuff, M., Broos, K., et al. (2008). Modeling the toxicity of copper and zinc salts to wheat in 14 soils. Environ. Toxicol. Chem. 27, 786–792. doi:10.1897/07-294.1
Wu, Y., Zhang, J., Deng, Y., Wu, J., Wang, S., Tang, Y., et al. (2014). Effects of warming on root diameter, distribution, and longevity in an alpine meadow. Plant Ecol. 215, 1057–1066. doi:10.1007/s11258-014-0364-5
Keywords: nighttime warming (NW), copper pollution, bioaccumulation, wheat, root system
Citation: Qi L, Li C, Lai L, Kou T and Deng Y (2024) Nighttime warming promotes copper translocation from root to shoot of wheat (Triticum aestivum L.) through enlarging root systems. Front. Environ. Sci. 12:1284198. doi: 10.3389/fenvs.2024.1284198
Received: 28 August 2023; Accepted: 21 February 2024;
Published: 04 March 2024.
Edited by:
MinLing Gao, Shantou University, ChinaReviewed by:
Xiao-San Luo, Nanjing University of Information Science and Technology, ChinaCopyright © 2024 Qi, Li, Lai, Kou and Deng. This is an open-access article distributed under the terms of the Creative Commons Attribution License (CC BY). The use, distribution or reproduction in other forums is permitted, provided the original author(s) and the copyright owner(s) are credited and that the original publication in this journal is cited, in accordance with accepted academic practice. No use, distribution or reproduction is permitted which does not comply with these terms.
*Correspondence: Taiji Kou, dGprb3VAaGF1c3QuZWR1LmNu
Disclaimer: All claims expressed in this article are solely those of the authors and do not necessarily represent those of their affiliated organizations, or those of the publisher, the editors and the reviewers. Any product that may be evaluated in this article or claim that may be made by its manufacturer is not guaranteed or endorsed by the publisher.
Research integrity at Frontiers
Learn more about the work of our research integrity team to safeguard the quality of each article we publish.