- Institute of Environmental Engineering, Wrocław University of Environmental and Life Sciences, Wrocław, Poland
Most phytoremediation studies are limited to several of the most common plants and in most cases these studies are performed to a very limited extent. There are also few publications on the assessment of the effectiveness of the removal of pollutants, performed simultaneously with various plants and using physicochemical analyses as well as toxicity tests. Therefore, the conducted studies focused on assessing the removal of pollutants from leachate by physicochemical analyses and toxicity tests. The leachate was collected from four municipal waste landfills of different ages (2 non-operational facilities and 2 active). The studies were conducted for two biological systems: using emergent macrophytes (Phragmites australis) and submergent macrophytes (Ceratophyllum demersum L.). It has been observed that efficiency of biological treatment of leachates with Phragmites australis and Ceratophyllum demersum shows significant differences at lower concentrations of solutions. Even after the treatment with Phragmites australis and Ceratophyllum demersum, the analyzed leachate samples produced a toxic effect on the test organisms. A reduction or no toxicity of the leachate after the biological treatment was observed only in some cases. The observed effects of the biological leachate treatment were not conclusive. Particular attention should be paid to the persistent toxicity, which can pose a real threat to the environment when discharging leachates after treatment.
Introduction
The reduction of municipal waste landfilling has recently become increasingly important. However, even after major changes in waste management and closure of some landfills, management of leachate from these facilities will still remain a challenge (Białowiec et al., 2019).
Leachate can be characterized as an aqueous solution containing dissolved organic and inorganic compounds, heavy metals, suspended particles and hazardous substances (Christensen et al., 2001; Kjeldsen et al., 2002; Aziz et al., 2010; Naveen et al., 2017). Before discharging into the environment, leachates require treatment because they can be a serious pollutant (Kjeldsen et al., 2002). The literature distinguishes three main groups of processes used to treat landfill leachate: biological (aerobic or anaerobic), physicochemical and hybrid (combination of physicochemical and biological processes) (Thomas et al., 2009).
Physicochemical methods are effective in removing most pollutants, particularly in the treatment of leachate from old landfills and the reduction of compounds resistant to biodegradation, but their use generates high costs and additional pollution (Renou et al., 2008). For treatment of leachate from young landfills, mainly biological methods are used, but their selection should be preceded by an analysis of leachate properties, technical possibilities and the cost-effectiveness of a given method (Kurniawan et al., 2010).
Biological methods have become the most popular technique in leachate treatment due to low costs and high efficiency in the removal of nitrogen (Jemec et al., 2012; Miao et al., 2019). Their additional advantage is the possibility to be applied on-site. Although treatment at the site of formation is the best solution, treatment together with wastewater in municipal treatment plants still remains the most popular option (Yalcuk, Ugurlu, 2009).
One of the good alternatives to treatment plants, enabling on-site use, is a constructed wetlands (CW). It is a solution justified not only for economic, but also for technical reasons, as these systems do not have high operating and maintenance requirements (Yalcuk, Ugurlu, 2009; Białowiec et al., 2012). In CW, macrophytes are used: emergent (e.g., common reed, broadleaf cattail, reed sweet-grass); floating-leaved (e.g., European white water lily and yellow water lily); submergent (e.g., rigid hornwort) and free-floating (e.g., common duckweed) (Ravi et al., 1998; Rahman et al., 2020). The most popular ones include: broadleaf cattail (Typha latifolia L.), common reed (Phragmites australis), common rush (Juncus effusus L.), yellow flag (Iris pseudacorus L.) and reed sweet-grass (Glyceria maxima) as well as willow (Salix sp.) (Białowiec et al., 2012).
Before choosing a treatment method or discharging into the environment, tests are required to study pollution of the leachate. Although the conducted physicochemical analyses allow for identification of most of the pollutants, in many cases a significant proportion of toxic substances remain undetected. Toxicity tests are helpful in this situation. It is a well-known method, but very rarely used in practice (Jemec et al., 2012), despite the fact that the tests are very important from the point of view of the organisms living in the environment (Hassan et al., 2016). Toxicity tests use organisms representative of different trophic levels.
For the assessment of complex mixtures, such as landfill leachate, among the most commonly used organisms are Daphnia magna crustaceans (Persoone et al., 2009; Barrios Restrepo et al., 2017; Przydatek, 2019b), which are presented as a model species in the international toxicity testing guidelines (Persoone et al., 2009; Cui et al., 2017). Their advantage is a small size, a short life cycle, allowing for rapid testing and ease of cultivation under laboratory conditions (Manakul et al., 2017; Lari et al., 2018).
The second common type is the phytotoxicity test involving terrestrial plants. One of the species used is Sinapis alba L, which has gained popularity due to the sensitivity to a wide range of substances (Zloch et al., 2018; Vaverková et al., 2020). Phytotoxicity tests are most frequently performed in the early development phase and include seed germination as well as growth of roots and shoots (Maiorana et al., 2019). Their advantage is a short time, ease of execution and low costs (Šourková et al., 2020). In addition, the use of phytotoxicity tests helps to determine and maintain appropriate concentration of leachate, which is important for the effectiveness of phytoremediation (Kalčíková et al., 2012).
Most phytoremediation studies are limited to several of the most common plants and in most cases these studies are performed to a very limited extent (Abdallah, 2012). There are also few publications on the assessment of the effectiveness of the removal of pollutants, performed simultaneously with various plants and using physicochemical analyses as well as toxicity tests. Therefore, the conducted studies focused on assessing the removal of pollutants from leachate by physicochemical analyses, but also toxicity tests. The conducted study is important in supplementing the information on the effectiveness of removing pollutants from landfill leachate from objects of different ages (2 non-operational facilities and 2 active), using various plants, i.e. emergent macrophytes (Phragmites australis) and submergent macrophytes (Ceratophyllum demersum L.).
Materials and Methods
Leachate Samples
The leachate samples were collected in June 2020, from four municipal waste landfills located in the Lower Silesian Voivodeship in Poland. Two landfills are non-operational, i.e. a landfill located in Wrocław and a landfill in Bielawa. The oldest landfill was established in 1966, in Wrocław, and it operated until 2000. Its area occupies 11.7 ha, and the landfill capacity is about 2 million m3. The second non-operational landfill is located in Bielawa, the facility operated from 2001 to 2011. The landfill area is 0.86 ha, and its capacity is 37.8 thousand m3.
The largest active landfill is in Legnica, with the area of 14.12 ha, and the total capacity of the facility is 2.34 million m3. The landfill has been operating since 1977. The second active landfill, in Jawor, has existed since 1997. The area occupied by waste is 3.37 ha. The total capacity of the landfill is 231.3 thousand m3.
More detailed characteristics of the research objects are presented in the article (Wdowczyk and Szymańska, 2021a).
Physicochemical Composition of Leachate
Immediately after collecting, the samples were transported to the Environmental Research Laboratory of the Institute of Environmental Engineering at Wrocław University of Environmental and Life Sciences. The analyses of physicochemical properties were conducted by commonly used methods, in accordance with the ISO (International Organization for Standardization) standards. Laboratory analyses not requiring mineralization of samples were performed within 24 h of their collection (Tomczyk and Wiatkowski, 2021). Simultaneously, mineralization was performed, and subsequently, analyses requiring mineralization were conducted.
The raw and post-treatment leachate was tested for: pH, electrical conductivity (EC), Chemical Oxygen Demand (COD), biochemical oxygen demand and concentration (BOD5), total Kjeldahl nitrogen (TKN), organic nitrogen (ON), ammonium nitrogen (AN), total phosphorus (TP), total solids (TS), total dissolved solids (TDS), total suspended solids (TSS), sulphates, chlorides, sodium, potassium, calcium, magnesium, iron, manganese, zinc, lead and nickel, cadmium.
Organization and Course of the Experiment
Laboratory tests included the exposure of the seedlings of common reed (Phragmites australis) and rigid hornwort (Ceratophyllum demersum L.) to the increasing concentrations of landfill leachate.
Phragmites australis was selected for research due to the fact that for many years it has been used in phytoremediation for the treatment of various types of wastewater. It has a high capacity to accumulate nutrients, heavy metals and micropollutants, in this respect surpassing other aquatic plants (Milke et al., 2020). The second plant selected for research was Ceratophyllum demersum L. It is a completely submerged plant, commonly found in water bodies with moderate and high levels of nutrients (Foroughi et al., 2013). It is capable of absorbing even high concentrations of various components, including phosphorus and nitrogen, without additional aeration (Foroughi et al., 2010).
Figure 1 shows a diagram of the conducted experiment. The experiment was aimed at checking the effectiveness of landfill leachate treatment by physicochemical analyses and toxicity tests, using various concentrations of leachate.
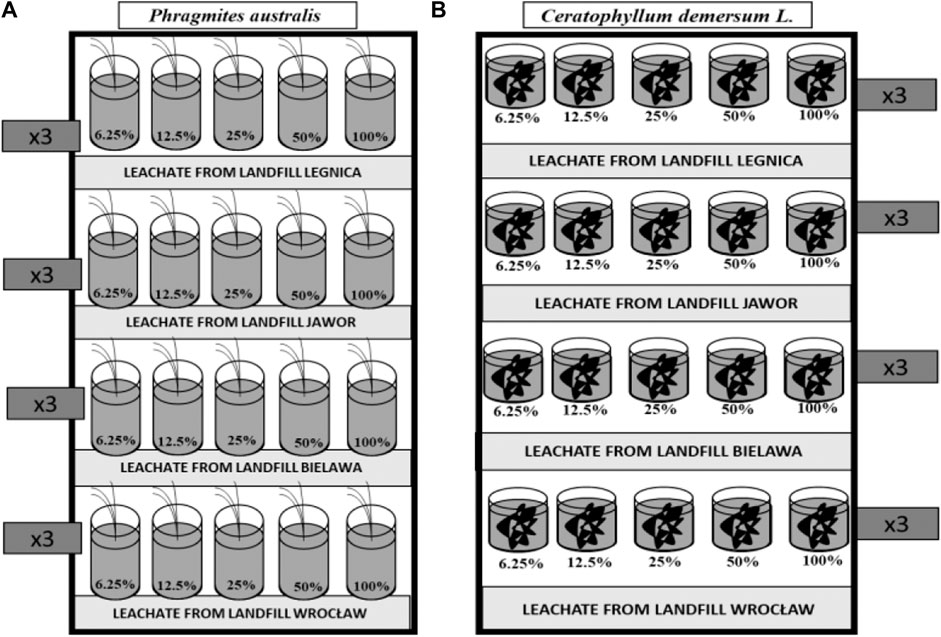
FIGURE 1. Diagram of the experiment on the treatment of landfill leachate with: (A) Phragmites australis, (B) Ceratophyllum demersum L.
Experiment With Phragmites australis
The transported seedlings of Phragmites australis after watering with tap water during the period of 14 days were being adapted to laboratory conditions. Then, from the seedlings that had acclimatized, 60 plants of similar sizes were selected and transferred individually to containers with a volume of 1.5 dm3. The containers were filled with the leachate from the four landfills. The series for each landfill consisted of solutions with increasing concentration from 0% (tap water) through 6.25; 12.5; 25; 50–100% (Figure 1A).
The choice of dilution occurred on the basis of previously performed toxicity analyses. In order to facilitate the calculations, the series consisted of solutions with decreasing concentrations, each successive one constituting 50% of the previous one. A similar range of sample concentrations was used in other Białowiec i Kasiński, who conducted research on the neutralization of landfill leachate in wetlands systems (Białowiec and Kasiński, 2009). Exposure to the leachate lasted another 14 days. The same exposure time was also chosen in the studies (Tahmasbian, Safari Sinegani, 2016; Antić et al., 2018; Daud et al., 2018). No additional aeration was used during the experiment. Each variant was performed in 3 replications.
After the plants had been exposed to the leachate, the same volume of solution was withdrawn from each of the three containers. After mixing, they were samples for physicochemical composition and toxicity tests.
Experiment With Ceratophyllum demersum L
The second part of the experiment was conducted with rigid hornwort Ceratophyllum demersum L. The plants after transportation to the laboratory were watered with tap water for the period of 14 days. Of the plants, 120 were selected with an average length of approx. 20 cm. Two plants were placed in each container with a volume 0.5 dm3. The containers were filled with the leachate from the four landfills with increasing concentration from 0% (tap water) through 6.25; 12.5; 25; 50–100% (Figure 1B). Exposure to the leachate lasted another 2 weeks; no additional aeration was used during the experiment. Each variant was performed in 3 replications. After the plants had been exposed to the leachate, the same volume of solution was withdrawn from each of the three containers. After mixing, they were samples for physicochemical composition and toxicity tests.
Toxicity Tests
Acute toxicity tests were performed on white mustard (Sinapis alba L.) and on the freshwater crustaceans Daphnia magna Straus, for the raw leachate samples and the leachates after the biological treatment with Phragmites australis and Ceratophyllum demersum L.
Acute Toxicity Test on White Mustard Sinapis alba L
For the leachate samples from the four landfills, 3-days acute toxicity tests were conducted on white mustard seeds (Sinapis alba L.) based on the Phytotoxkit™ methodology (MicroBioTests Inc.). The test was performed twice, i.e. before the treatment and after the biological treatment with Phragmites australis and Ceratophyllum demersum L.
White mustard was chosen for this study due to its sensitivity to a number of substances and its frequent use in toxicity testing (Zloch et al., 2018; Vaverková et al., 2020).
The acute toxicity tests were conducted in flat boxes with foam pads and paper filters. On the basis of previous analyses and data presented in the literature, a definitive range of dilutions was selected for the actual study. For most landfills the range was: 100, 50, 25, 12.5, 6.3, 3.2%. However, for the leachate from the Wrocław landfill, the range of concentrations was limited, and it amounted to: 100 and 50% (which resulted from the age of the landfill and the previously conducted analyses). A similar range of concentrations was also used in other studies of acute toxicity of leachate (Sackey et al., 2020). For dilution, dechlorinated tap water was used, which was also a control sample. Each filter was wetted with 20 cm3 of diluted leachate. For dilutions, dechlorinated tap water was used, which also constituted a control sample.
On each pre-soaked plate, 10 Sinapis alba seeds of equal size and weight were placed. The samples prepared in this way were kept in the dark for a period of 3 days. The test consisted of 3 replications.The experiment was performed at room temperature (±25°C). After 3 days, the number of germinating seeds as well as the growth of roots and shoots were recorded. For a comprehensive interpretation, data on seed germination and root length gain were used to calculate the germination index (GI) according to the pattern eq. 1:
where: Gs and Ls are seed germination (%) and root length gain (mm) in the tested sample, and Gc and Lc are the respective control values (García, 2014).
Depending on the value of the GI germination index, leachate toxicity can be divided into:
— high phytotoxicity: GI < 50%,
— moderate phytotoxicity: GI 50–80%,
— no phytotoxicity: GI > 80%,
— stimulating effect for plants: GI > 100% (Zucconi et al., 1984; Bożym et al., 2020).
Acute Toxicity Test on Freshwater Crustaceans Daphnia magna Straus
Toxicity tests on D. magna crustaceans have been widely used in toxicity testing for over 30 years. This method has been standardized by OECD 202 (2004) and is recommended for toxicity testing by the Environment Agency, SEPA and most other national environmental authorities (Thomas et al., 2009).
Toxicity tests were performed in accordance with the applicable ISO 6341:2012 standard (European Committee for Standardization, 2012). The actual toxicity tests were conducted based on the concentration range established during the preliminary tests. The test consisted in preparing the following solutions of the tested sample: 100, 50, 25, 12.5, 6.3 and 3.2%. For dilution of the leachate, dechlorinated tap water was used, which also constituted a control sample. In each replication, 5 organisms were subjected to the toxicity test. The preliminary test was performed with one replication (5 organisms); the actual test consisted of 4 replications (5 each, 20 organisms in total) for each dilution.
In each prepared test solution with a volume of 100 cm3, 5 Daphnia magna Straus organisms were placed for a period of 24 h. After 24-h exposure, the degree of immobilization of organisms exposed to the individual test solutions was visually assessed. The toxic effect was expressed as the mean percentage of the immobilized subjects. Immobilized organisms were considered to be those that were unable to swim for 15 s after shaking the sample, even if they could still move the antennae. The control sample, with dechlorinated tap water meeting the criteria specified in the standard was similarly analyzed. If the number of immobilized organisms exceeded 10%, the tested control sample was considered unrepresentative (European Committee for Standardization, 2012).
Acute toxicity for Daphnia magna organisms was defined as the mean concentration that caused the immobilization effect in 50% of the tested organisms. On the basis of the conducted analyses, EC50 values were determined, defining the concentration of the leachate, which caused the immobilization effect in 50% of the tested organisms. Based on the EC50 value, the TU (Toxic Unit) value was calculated, according to eq. 2:
Depending on the TU value, leachate toxicity can be divided into:
— no acute toxicity: TU < 0.4,
— slight acute toxicity: 0.4 < TU < 1,
— acute toxicity: 1.0 < TU < 10,
— high acute toxicity: 10 < TU < 100,
— very high acute toxicity: TU > 100 (Persoone et al., 2003; Barrios Restrepo et al., 2017; Przydatek, 2019b).
Data Treatment and Statistical Analysis
The obtained results of the studies of leachate properties and phytotoxicity tests from landfills in the raw samples and post-treatment with Phragmites australis and Ceratophyllum demersum were subjected to statistical analysis, using Statistica 13.1 software (StatSoft Polska, StatSoft, Inc, Tulsa, OK, United States).
The analysis of the differences between the properties of the leachate from the active landfills (Legnica, Jawor) and the closed landfills (Wrocław, Bielawa), treated with Phragmites australis (P) and Ceratophyllum demersum (C), was conducted using the parametric t-test for dependent groups, frequently applied in many studies.
Results and Discussion
Physicochemical Properties of Leachate
Analysis of Physicochemical Properties in Raw Leachate
Table 1 presents the results of physicochemical analyses of the raw leachate from the active and closed landfills.
As part of the studies of the physicochemical composition of the leachate, 25 selected parameters were analyzed. The pH values of the leachate from the landfills where the research was conducted ranged from 8.11 to 8.84. The alkaline nature of the leachate indicates a mature stage of landfilling and is characteristic of older landfills (Jorstad et al., 2004). All the landfills where the research was conducted can be classified as mature facilities, i.e. operating for >10 years (Nájera-Aguilar, 2019).
Larger differences between landfills occurred in the case of EC. The leachate from the non-operational landfills was characterized by values in the range 1800–2,318 μS/cm, while for the leachate from the active landfills, the EC values were higher and ranged from 3,919 to 7,791 μS/cm. Similar trends can be observed in the case of the TDS content, changing with the age of landfills, which can be explained by the decrease in the concentration of organic and inorganic solutes (Tatsi and Zouboulis, 2002). The highest value of TDS was observed in the active landfill in Legnica (7,065 mg/dm3), and the lowest in the landfill in Wrocław (1,690 mg/dm3). The high content of solid solutes is reflected in the high EC values of the leachate (Wdowczyk and Szymańska, 2020).
The leachate from the active landfills was characterized by high COD values, much higher than those recorded in the closed facilities. In the active landfills, COD values ranged from 1765.9 mg O2/dm3 to 2007 mg O2/dm3. In contrast, in the non-operational facilities, COD ranged from 127.6 mg O2/dm3 (Wrocław) to 321.8 mg O2/dm3 (Bielawa). Lower COD values in the closed landfills result from the fact that the susceptibility of the leachate to biological degradation processes decreases with age (Mohammad-Pajooh, 2017). The presented results are consistent with the literature data indicating the presence of a high content of organic matter (Vedrenne et al., 2012). The values obtained in this study do not differ from the COD values found in leachates by other authors (Kjeldsen et al., 2002; Tatsi, Zouboulis, 2002).
In the leachate from the active landfills, high BOD values can also be observed. The highest BOD was recorded in the landfill in Jawor, and it was 288 mg O2/dm3, while the lowest value was found in the non-operational, oldest landfill in Wrocław, and it was only 1.8 mg O2/dm3. The low COD value and the low BOD/COD ratio prove the dominance of the methanogenic phase inside the landfill and the low biological activity due to the low concentration of volatile fatty acids and a relatively higher amount of fulvic and humic compounds (Öman and Junestedt, 2008).
Leachate may pose a potential threat to the environment due to the high content of organic matter, high load of nitrates and ammoniacal nitrogen (Jabłońska-Trypuć, 2021). The main factor influencing the presence of nitrogen in leachate is the decomposition of complex nitrogen compounds in the solid waste (Al Yaqout and Hamoda, 2020). Much higher nitrogen contents were observed in the leachate from the active facilities. In the active landfills, TKN ranged from 269.85 mg N/dm3 (landfill in Legnica) to 310.16 mg N/dm3 (landfill in Jawor). In contrast, in the closed landfills, the TKN value was much lower, ranging from 1.09 mg N/dm3 (landfill in Wrocław) to 51.12 mg N/dm3 (landfill in Bielawa).
Among the analyzed forms of nitrogen, AN had the highest share, except for the oldest landfill in Wrocław, where the dominant share can be attributed to ON, which is consistent with the results obtained by other authors, who also indicated AN as the main form of nitrogen occurring in landfill leachate (Kjeldsen et al., 2002; Tatsi, Zouboulis, 2002; Thomsen et al., 2012). The high concentration of ammonium nitrogen is often attributed to acute toxicity of leachate (Isidori et al., 2003; Dave, Nilsson, 2005; Kalka, 2012).
The values of other indicators, such as the content of TP and chlorides, decrease in the emerging leachate with the age of the stored waste. According to the data presented in the literature, the content of chlorides in mature leachate is on average 10 times lower than in young leachate (Ding et al., 2017). This is confirmed by the obtained results: the lowest among the recorded contents of chlorides and TP were recorded in the leachate from the oldest landfill in Wrocław. The highest contents of chlorides were observed in the leachate from the active landfill in Legnica (2,160 mg/dm3), while in the second active landfill, in Jawor, the content of chlorides was lower and amounted to 660 mg/dm3. All the analyzed landfills can be classified as middle-aged facilities (i.e. > 5 years). According to the data presented in the literature, the content of chlorides in the leachate from such facilities usually ranges up to 400 mg/dm3, but these values have also been found to reach up to even 8,000 mg/dm3 (Bowman et al., 2002).
Low concentrations of Ca, Mg, Fe and Mn cations were observed in the leachate from the active and closed landfills. All the obtained values were within the range of values reported in the literature, characteristic for leachates from landfills in the methanogenic phase (Ehrig, 1983). It can be assumed that the low concentrations of Ca, Mg, Fe and Mn cations are related to the high pH values found in the leachate from all the landfills. In alkaline solutions, the intensity of sorption and precipitation increases, and the content of dissolved organic matter decreases (Öman and Junestedt, 2008).
In the active landfills, the contents of sulphates were much lower than in the closed landfills, ranging up to 374 mg/dm3 (Kulikowska, Klimiuk, 2008), which may also confirm that the landfill is in the methanogenic phase and there was a reduction of sulphates in the previous, acidic anaerobic phase (Öman,Junestedt, 2008). In contrast, in the leachate from the closed landfills, higher contents of sulphates were recorded, reaching 1,481 mg/dm3 (in the leachate from the Bielawa landfill), which in turn can be explained by the high content of organic matter, which is associated with a high concentration of sulphates (Liu et al., 2011; Wdowczyk and Szymańska-Pulikowska, 2021a).
The contents of sodium and potassium in the samples from the active landfills were higher than those recorded in the non-operational landfills. The mean sodium concentrations in the active landfills ranged from 162.9 mg/dm3 (Jawor) to 177.8 mg/dm3 (Legnica), while in the closed facilities these values were lower and ranged from 91.2 mg/dm3 (Wrocław) to 151.8 mg/dm3 (Bielawa). The mean potassium concentrations were similar, the highest values were observed in the active landfills, from 410 mg/dm3 (Jawor) to 507.6 mg/dm3 (Legnica). The lowest value was obtained for the non-operational landfill in Wrocław and it amounted to 61.2 mg/dm3, while in the second non-operational landfill in Bielawa that value was much higher and amounted to 256.2 mg/dm3. The contents of sodium and potassium are not significantly influenced by microbiological changes occurring inside the landfill. These ions play an important role in plant physiology, and their presence in leachate may indicate that the landfill contained plant residues and other bio-waste (Christensen et al., 2001; Wdowczyk and Szymańska-Pulikowska, 2021b).
Researchers emphasize that the content of heavy metals in leachate is an important issue, because metals can cause its toxicity, accumulate in aquatic organisms, which in turn may lead to biomagnification and transfer in the subsequent links of the food chain (Alkassasbeh et al., 2009; Fauziah et al., 2013). Leachate was classified as one of the main sources of heavy metal transfer to the environment (Gworek et al., 2016; Przydatek, 2019b), and their presence as a potential hazard to human health (Borjac et al., 2019).
In recent years, increasingly more studies have shown that the content of heavy metals ceases to be the most serious threat posed by landfill leachate (Christensen et al., 2001; Przydatek, 2019a; Wdowczyk and Szymańska-Pulikowska, 2020). However, studies showing a serious threat of heavy metal contamination of soil, surface water and groundwater by landfill leachate can still be found. This is especially the case of some developing countries, e.g. Ghana (Boateng et al., 2019), where storage is carried out in an uncontrolled manner (Borjac et al., 2019). The prevailing redox conditions, anaerobic environment, pH, the degree of oxidation of metals and microbiological activity play an important role in the leaching of metals (Hussein et al., 2021).
The results of the conducted research show that the content of heavy metals in the leachate from the studied active and closed landfills in Poland remains at a very low level, i.e. in each case <1 mg/dm3. Low concentrations of heavy metals in the tested leachate confirm that the deposited waste was mainly municipal waste (Naveen et al., 2017). Other researchers also found low contents of heavy metals in landfill leachate (Tatsi, Zouboulis, 2002; Zainol et al., 2012; Przydatek, 2021).
Differences Between Physicochemical Properties of the Leachate After the Treatment With Phragmites australis and Ceratophyllum demersum
The results of analyzes of the physicochemical properties of raw leachate and after treatment with Phragmites australis (P) and Ceratophyllum demersum are presented in Supplementary Tables S1–S4 (for each landfill), included in the supplementary materials. They also marked statistically significant differences between the results of studies on the composition of leachate samples with such a dilution, treated with different plants. Differences were assessed using a paired t-test.
The following parameters were included in the test: pH, EC, COD, BOD5, TKN, ON, AN, TP, TS, TDS, TSS, sulphates, chlorides, sodium, potassium, calcium, magnesium, iron, manganese, zinc, lead, nickel and cadmium. The parameters were determined for all the tested concentrations (6.25, 12.5, 25, 50, 100%).
The conducted analysis showed several cases of significant differences between the properties of solutions with the same concentration of leachate, treated with different plants, i.e. Phragmites australis (P) and Ceratophyllum demersum (C). In the non-operational landfills, significant differences were seen in the leachate dosed at the lowest concentration, i.e. 6.25%. However, in both active landfills, significant differences were observed for solutions containing 12.5% leachate; in addition, in the samples for the Legnica landfill, a significant difference occurred at a concentration of 50%. In the samples for which significant differences were found, lower values of the tested parameters occurred mainly in the treatment of the leachate with C. demersum. This concerned the leachate solutions from the closed landfills (6.25%) and the active landfill in Jawor (12.5%). Only in the case of the active landfill in Legnica, the statistical analysis showed better treatment efficiency for the samples treated with P. australis (samples with a concentration of 12.5 and 50%). The conducted studies showed that despite many favorable properties, P. australis might not always be the most effective species in removing pollutants from leachate. Liang et al. report that P. australis and other plants are able to remove from 40 to 80% of organic substances, such as COD, BOD and AN from landfill leachate (Liang et al., 2017).
Leachate Toxicity
Toxicity Test on Dicotyledonous Plant Sinapis alba L
Of the many toxicity indicators based on germination and early growth of higher plants, the GI germination index was used to assess the phytotoxicity of the leachate. Figure 2 shows the GI values for the leachate from the four landfills, before and after the biological treatment, dosed at different concentrations. The graphs also show the classification of phytotoxicity based on the GI values, where: GI < 50% - medium high phytotoxicity, GI within 50–80% medium moderate phytotoxicity, GI > 80% no phytotoxicity, a GI > 100% - a plant stimulating effect (Zucconi et al., 1984; Bożym et al., 2020).
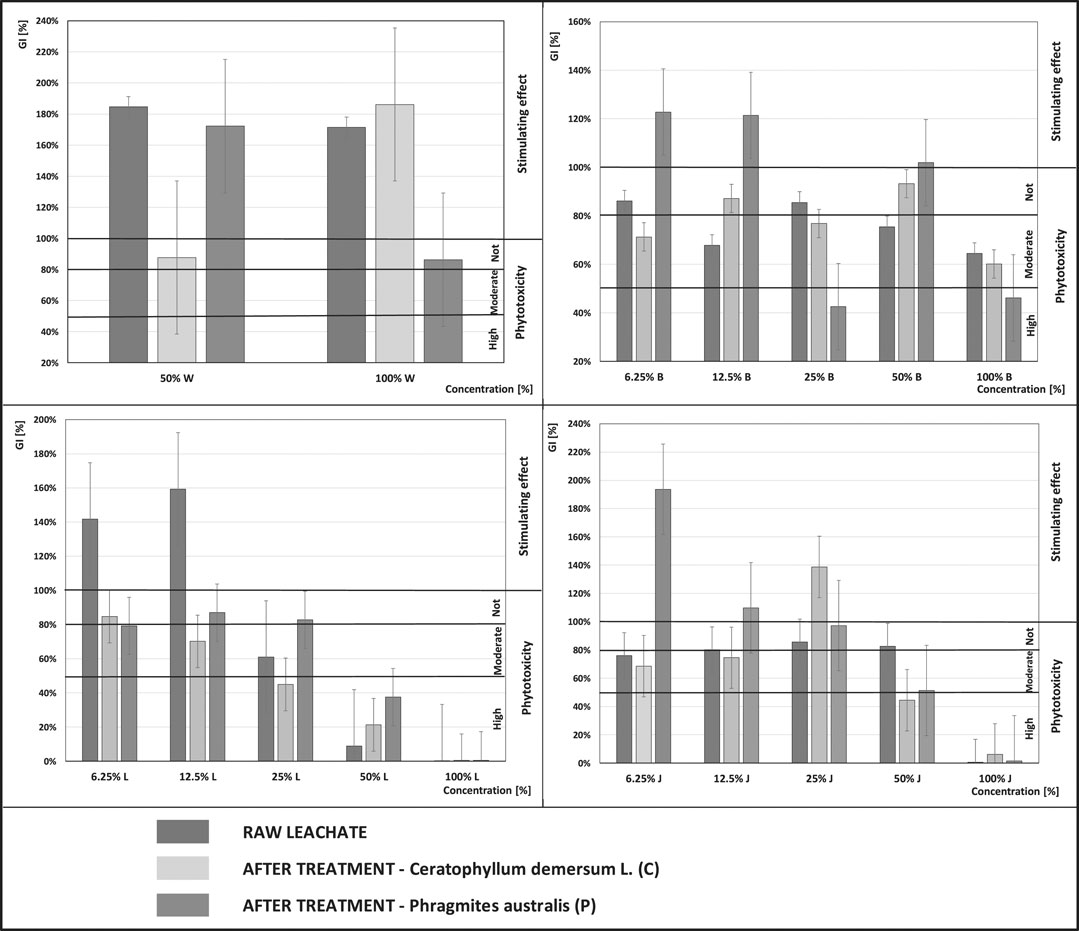
FIGURE 2. Germination index (GI) of Sinapis alba L. in the raw leachate from the active landfills (L-Legnica, J-Jawor) and the closed landfills (W-Wrocław, B-Bielawa), and after the treatment with Phragmites australis (P) and Ceratophyllum demersum L (C) at various concentrations.
Leachate is a complex mixture, containing a wide variety of different substances. Its composition changes over time and depends on many factors. Among them are, inter alia, location, age of the landfill or landfilling technology (Kheradmand et al., 2010; Fitzke et al., 2013; Xaypanya et al., 2018). The conducted research shows a large variation in the values of the GI between individual landfills. The greatest difference can be observed depending on the stage of operation (active, closed), the age of the landfill and the concentration of leachate.
The highest toxicity was observed in the leachate from the active landfills, at concentrations of 100%. In all the samples, both before and after the treatment, the leachate had a toxic effect on plants. Also Šourková et al. during the research conducted in the Bukov landfill showed a significant growth inhibition of Sinapis alba L. when dosing higher concentrations of leachate (Šourková et al., 2020).
The leachate from the non-operational landfills was not as toxic to the Sinapis alba seeds as the leachate from the active facilities. A significant difference can also be noticed between the two closed landfills. The leachate from the Bielawa landfill showed higher toxicity than the leachate from the Wrocław landfill, which can be explained by the age of the facilities. The landfill in Wrocław was closed in 2000, and the facility in Bielawa 11 years later. During the studies of the leachate from the landfills in Slovenia, significant differences were also observed in the composition and toxicity of the leachate, depending on the stage of landfill operation. The leachate from the active part of the landfill had a negative impact on the tested plants (Lens esculentum, Triticum aestivum, Sinapis alba). In contrast, the leachate from the closed part of the landfill did not have a toxic effect on the tested plants due to the stabilization of the occurring processes (Kalčíková et al., 2012).
The leachate from the younger landfill out of the non-operational landfills (in Bielawa) at a concentration of 100% caused a moderate toxic effect in the raw samples and post-treatment with Ceratophyllum demersum L., and a high toxic effect in the samples after the treatment with Phragmites australis. In the second non-operational landfill in Wrocław (in the raw samples and post-treatment with Ceratophyllum demersum L.) the leachate caused a stimulating effect for Sinapis alba seeds. However, after the treatment with Phragmites australis its positive impact on Sinapis alba seeds decreased, but the leachate still did not cause a toxic effect.
The observed increase in toxicity of the leachate after the treatment with Phragmites australis could have been related to a strong phytotoxic effect on germination and growth of other aquatic plants from these plant organs extracts, which was confirmed in previous studies (Rudrappa et al., 2007; Uddin et al., 2014).
The GI values of the raw leachate from the non-operational landfill in Bielawa ranged from 64% (at a leachate concentration of 100%) to 86% (at a concentration of 6.25%). The raw leachate showed moderate and no phytotoxic effect on the seeds of the Sinapis alba plant. In the leachate treated with Ceratophyllum demersum L., GI values were similar to those in the raw leachate. The GI values ranged from 60% (at the leachate concentration of 100%) to 93% (at a concentration of 50%). The post-treatment leachate also demonstrated moderate or no phytotoxic effect on the seeds of the Sinapis alba plant.
Better results were obtained with low leachate concentrations (from 6.25 to 12.5%), after the treatment with Phragmites australis, where a stimulating effect on plant growth was observed. The GI ranged from 123% (at a concentration of 6.25%) to 121% (at a concentration of 12.5%). A positive effect of the leachate on Sinapis alba seeds after the treatment with Phragmites australis was also noted at a concentration of 50%, where the GI was 102%. In the remaining cases (i.e. at leachate concentrations of 25 and 100%), a toxic effect on the seeds was observed, which was not recorded even for the raw leachate.
The GI values of the raw leachate from the Jawor landfill ranged from 0% (at a leachate concentration of 100%) to 86% (at a concentration of 25%). Thus, the raw leachate at various concentrations showed a toxic, moderate and no toxic effect on the seeds of the Sinapis alba plant. Even after the biological treatment with Ceratophyllum demersum L. and Phragmites australis the leachate from the Jawor landfill at a concentration of 100% caused severe toxicity to Sinapis alba seeds, ranging from 2% (Phragmites australis) to 6% (Ceratophyllum demersum L.).
In contrast, at lower concentrations, the raw leachate from the Jawor landfill showed a moderate and no phytotoxic effect on Sinapis alba seeds. In the leachate treated with Ceratophyllum demersum at low concentrations (i.e. 6.25% and 12.5%) the GI values were very similar to those in the raw leachate. The GI values ranged from 69% (at the leachate concentration of 6.25%) to 74% (at a concentration of 12.5%), causing moderate toxicity to Sinapis alba. At a higher leachate concentration (25%), a significant improvement could be observed after the treatment with Ceratophyllum demersum compared to the raw leachate. The GI reached the level of 139%, thus stimulating the seeds of Sinapis alba. Good results were also obtained with low concentrations of the leachate from Jawor (within the range 6.25–12.5%), after the treatment with Phragmites australis. In both cases, a stimulating effect on plant growth was observed. The best treatment result was obtained for Phragmites australis at a leachate concentration of 6.25%, where the GI was the highest among all those recorded in the Jawor landfill and amounted to 194%. The obtained results confirm the thesis that leachate may have a positive effect on plant growth. This may be due to the high nitrogen levels and the presence of other nutrients necessary for plant growth (Calabrese and Blain, 2005; Vaisi and Žaltauskaitė, 2010; Guerrero, 2014). In both cases, after treating the leachate at a concentration of 50%, a higher phytotoxicity was observed than before the treatment.
GI values in the raw leachate from the active landfill in Legnica ranged from 0% (at a concentration of 100%) to 159% (at a concentration of 12.5%). Depending on the concentration, the leachate demonstrated a toxic to a stimulating effect on Sinapis alba seeds. There was no improvement after either treatment: with Phragmites australis and with Ceratophyllum demersum L., at a leachate concentration of 100%. The GI after the treatment was 0%, and the leachate was still highly toxic to Sinapis alba seeds. At a leachate concentration of 50%, improvement could be seen after the treatment of the leachate, with both the first and the second plant. A particularly noticeable improvement was noted after the treatment with Phragmites australis, the GI increased from 9% (in the raw leachate) to 38%. A lower result was obtained after the treatment with Ceratophyllum demersum L., where the GI was 21%. Despite the improvement achieved after the treatment of the leachate, in both cases it showed a toxic effect on Sinapis alba seeds. At lower concentrations (6.25 and 12.5%), the post-treatment leachate in both cases showed a worse effect on Sinapis alba seeds than in the raw samples. A reduction in the GI from the growth stimulating one (in the case of the raw leachate) to the GI characteristic of moderate and no toxicity was observed. At a leachate concentration of 25%, an improvement was noted after the treatment with Phragmites australis from 61% (in the raw leachate) to 83% (after the treatment). However, in the case of Ceratophyllum demersum L., the GI deteriorated, from the level of moderate toxicity to high toxicity (after the treatment).
The scope of the toxicity tests in the leachate from the closed landfill in Wrocław was limited to 50 and 100%, which resulted from previous analyses. The raw leachate in both concentrations showed a stimulating effect on the germination of Sinapis alba seeds. Also other authors, during the conducted research, noted the growth stimulation of Sinapis alba compared to the control sample (Šourková et al., 2020). The stimulating effect of leachates on plant growth can be explained by the fact that they contain a large amount of nutrients, e.g. carbon and nitrogen as well as low concentrations of heavy metals (Morozesk et al., 2017). Only in the case of a concentration of 100% of the leachate from Wrocław, the treatment with Ceratophyllum demersum L. improved the GI from 171% (the raw leachate) to 186% (after the treatment). In the remaining cases, the treatment did not have satisfactory results and adversely affected toxicity to Sinapis alba seeds.
When determining toxicity in higher plants, the following parameters of seed germination, root or shoot length are commonly used. Research shows that the seed germination parameter itself is less sensitive to leachate toxicity, which results from the fact that substances considered to be toxic do not directly impact the seed germination process (Klauck et al., 2015; Wdowczyk and Szymańska, 2020). Direct contact of meristematic tissue of the root with leachate causes increased sensitivity, influencing permeability, growth hormone production and cell differentiation (Kummerová and Kmentová, 2004). Therefore, in addition to the GI parameter, Figure 3 shows the root lengths [mm] after exposure to the leachate from the four landfills, dosed at various concentrations. The graphs also show the root lengths before and after the biological treatment with Ceratophyllum demersum L. and Phragmites australis, as well as in the control sample.
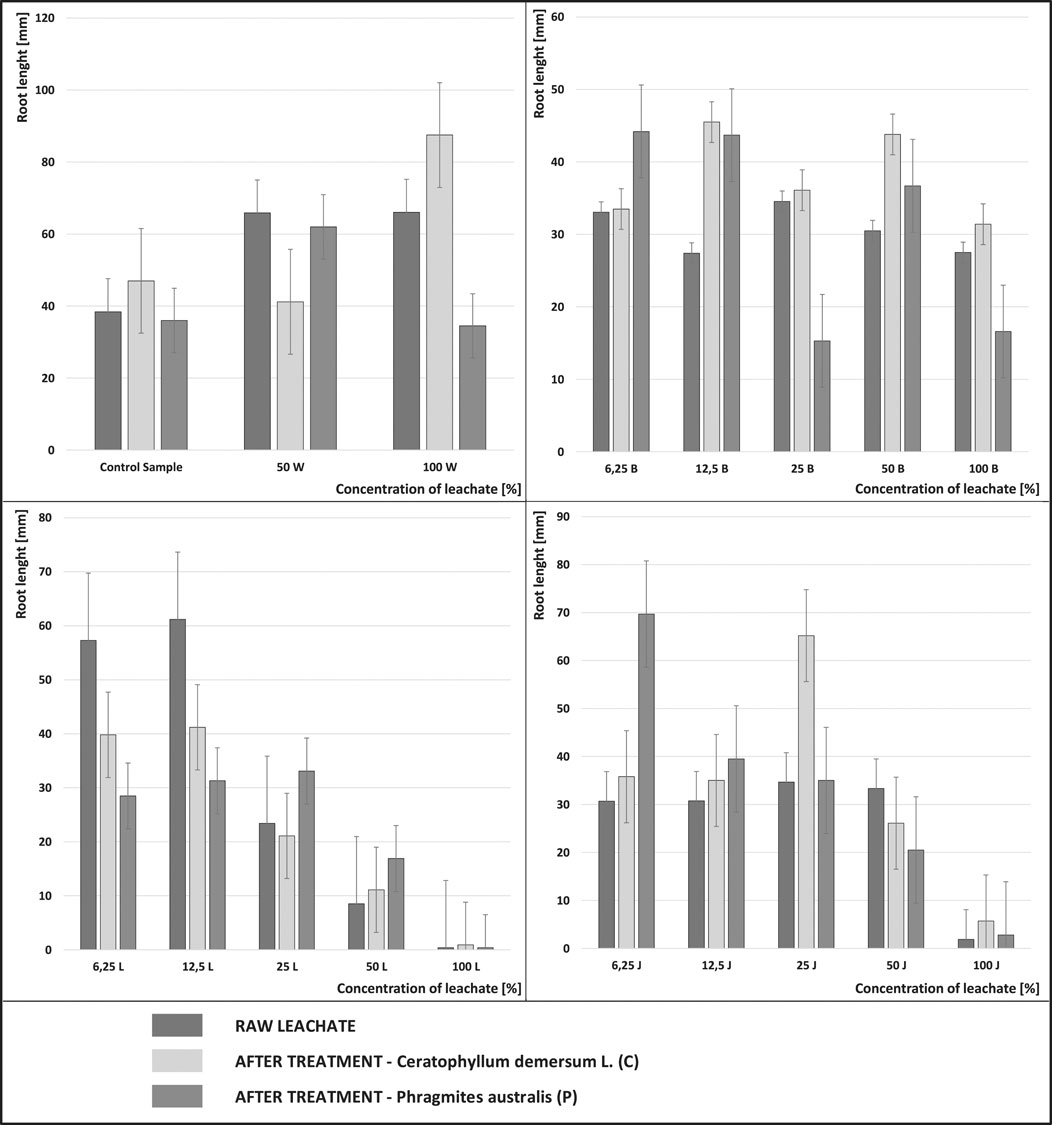
FIGURE 3. Root length of Sinapis alba L. for the raw leachate from the active landfills (L-Legnica, J-Jawor) and the closed landfills (W-Wrocław, B-Bielawa), and after the treatment with Phragmites australis (P) and Ceratophyllum demersum L (C) at various concentrations.
In the leachate from the active landfills (Legnica, Jawor), it can be observed that the length of the roots of Sinapis alba L. decreases with the increase in leachate concentration. Vaisi and Žaltauskaitė (Vaisi and Žaltauskaitė, 2010) also observed such a dependency during the studies conducted on Lactuca sativa L. The mean root length of the control samples for Sinapis alba was 38 mm. In the active landfills (Legnica, Jawor), the mean root length at the leachate concentrations of 100% before and after the treatment was much lower than in the control sample and ranged from 0.4 mm (100 L) to 5.7 mm (100 JC).
Despite the applied treatment, the increase in the root growth of Sinapis alba was small compared to the control sample. Kwarciak-Kozłowska et al. found a high degree of pollutant removal during the research on the effectiveness of landfill leachate treatment with the advanced oxidation process (AOP) with biochar adsorption, but despite this, at a concentration of 100% before and after the treatment they did not find any germination of Lepidium sativum and Sinapis alba seeds (Kwarciak-Kozłowska and Fijałkowski, 2021).
The conducted analyses showed that not in all cases the leachate treatment had a positive effect on Sinapis alba seeds. Although in many cases, after the treatment, an increase in the length of Sinapis alba roots was recorded compared to the raw leachate, it cannot be concluded that it was a permanent dependency. Similarly, Klauck et al. during the growth assessment of Lactuca sativa and Allium cepa roots showed that those are sensitive to the toxic effects of substances present both in raw and treated leachate. Even after biological treatment, the leachate was toxic to Allium cepa (Klauck et al., 2015). Other authors showed during their research that after the treatment of leachate with biological and mechanical methods, the negative effect on root growth was less pronounced (Vaisi and Žaltauskaitė, 2010).
In the control samples, the highest growth of Sinapis alba roots was observed in the sample with Ceratophyllum demersum L., and the lowest growth with the use of Phragmites australis. The lower growth of Sinapis alba roots in aqueous solutions with Phragmites australis may have been due to the fact that aqueous extracts from the organs of P. australis may have a phytotoxic effect on the growth of other plant species (Rudrappa et al., 2007; Uddin et al., 2014).
Tests on Freshwater Crustaceans Daphnia magna Straus
Daphnia magna invertebrates were selected from the many species commonly used for toxicity testing. In the conducted study, immobilization of D. magna after 48 h of exposure to landfill leachate was assumed for determining toxicity. Figure 4 shows the results of the conducted acute toxicity tests with Daphnia magna for the raw samples and after the treatment with Phragmites australis and Ceratophyllum demersum L. at various concentrations.
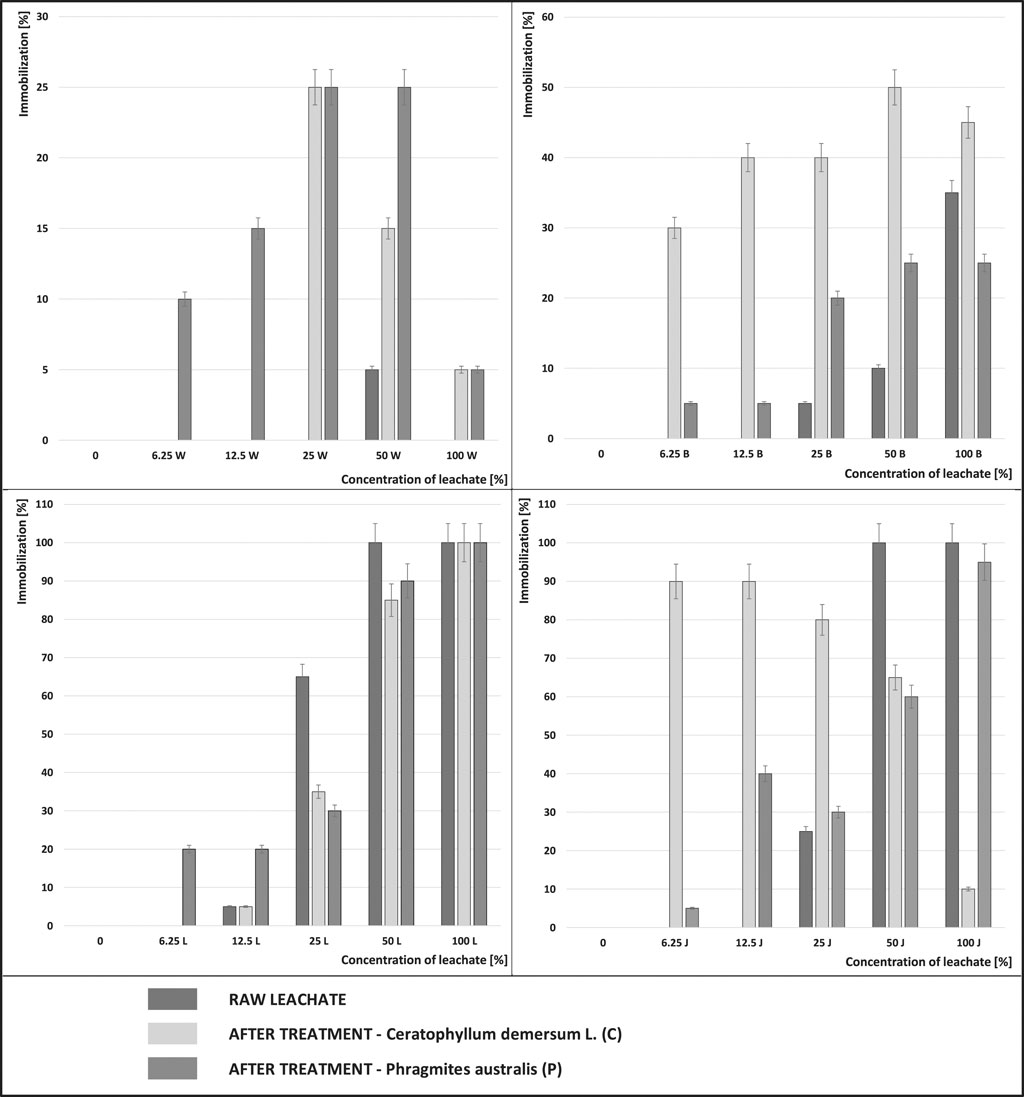
FIGURE 4. Percentage of immobilization of water fleas (Daphnia magna) after exposure to the leachate from the active landfills (L-Legnica, J-Jawor) and the closed landfills (W-Wrocław, B-Bielawa): raw and after the treatment with Phragmites australis (P) and Ceratophyllum demersum L (C) at various concentrations.
The percentage of immobilization of Daphnia magna in the leachate from the active landfills (Legnica, Jawor) was much higher than that determined in the samples from the closed landfills (Wrocław, Bielawa). No test organism survived in a concentration of 100% of leachate from Legnica. Even after the biological treatment of the leachate from the Legnica landfill, at a concentration of 100%, no improvement was noted. The lack of improvement after the biological treatment proves that the applied treatment was not a good solution for the leachate from that landfill. Biological treatment is known to have the best results in the treatment of young leachate (from landfills <5 years of operation) with a high BOD to COD ratio (>0,6) (Jemec et al., 2012). However, the effectiveness of biological treatment is limited in the case of high toxicity of leachate and a low BOD to COD ratio (Goi et al., 2009; Jemec et al., 2012), which was the case for the leachate from the stabilized landfill in Legnica.
The test organisms were also completely immobilized in the raw (100%) leachate from the second active landfill (in Jawor). High toxicity can arise for a number of reasons, In the literature, high levels of AN and heavy metals are often indicated as the main cause of toxicity (Sackey et al., 2020). In the raw leachate from the Jawor landfill, 246.62 mg AN/dm3 was recorded and it was the highest content noted in the leachate from the landfills where the research was conducted. However, the content of heavy metals in the leachate from all the facilities was very low. Therefore, it can be concluded that it was the high AN content that caused a complete immobilization of the test organisms.
However, after the treatment, an improvement in the survival of the test organisms was observed. The treatment with Phragmites australis reduced immobilization of Daphnia magna by 5%. The treatment with Ceratophyllum demersum brought much better results, reducing the immobilization of organisms by 90%.
At a concentration of 100%, the lowest percentage of immobilization of test organisms was recorded in the leachate from the Wrocław landfill. In the raw (100%) leachate from Wrocław, the survival rate of organisms was 100%, but after the treatment with both plants, the survival rate decreased by 5% compared to the raw samples. In the second non-operational landfill (in Bielawa), immobilization in the raw 100% samples was 35%. After the treatment with Ceratophyllum demersum, a 10% improvement in the survival of the organisms was noted. However, after treatment with Phragmites australis the survival rate of organisms decreased by 10% compared to the raw leachate.
In the concentrations of 50% of the leachate from the Legnica landfill, a 10% improvement after the treatment with Phragmites australis and a 15% improvement after the treatment with Ceratophyllum demersum can be noted.
In the second active landfill in Jawor, much better results were obtained with leachate concentrations of 50% after the treatment. From the 100% immobilization of test organisms, in leachate concentrations of 50%, after the treatment, immobilization decreased to 60% (Phragmites australis) and to 65% (Ceratophyllum demersum). In contrast, in the leachate from the non-operational landfills, in concentrations of 50%, after the treatment, greater immobilization of test organisms was observed than in the raw samples. Similarly, in the case of the remaining, lower concentrations (i.e. 25, 12.5, 6.25%), after the treatment, an increase in immobilization of Daphnia magna was observed compared to the raw samples.
In the active landfill in Legnica, even with a leachate concentration of 25%, after the treatment, an improvement by 30% (Ceratophyllum demersum) and by 35% (Phragmites australis) can be noted compared to the raw samples. In contrast, at lower concentrations (12.5%, 6.25%), the leachate after the treatment caused greater immobilization of organisms. The deterioration of the survival rate of the test organisms was particularly visible in the leachate from the Jawor landfill (at a concentration of 12.5 and 6.25%) after the treatment with Ceratophyllum demersum, which increased immobilization of organisms by 90%.
The toxicity test results were also examined for their environmental relevance, calculating the toxicity unit (TU) values. Table 2 shows the TU values in the landfills covered by the study, calculated for the raw leachate and after the biological treatment with Phragmites australis and Ceratophyllum demersum L.
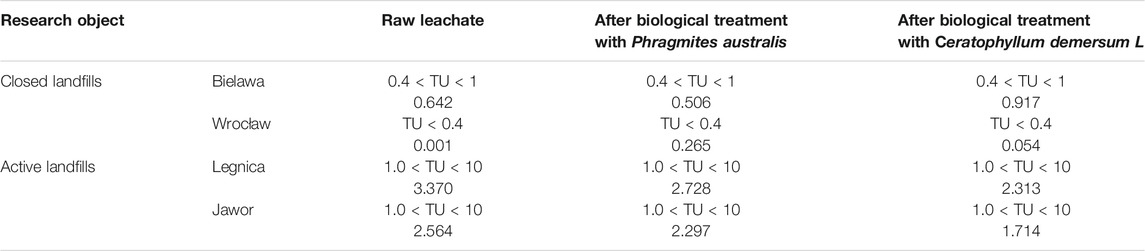
TABLE 2. TU values in the landfill leachate before and after the biological treatment with Phragmites australis and Ceratophyllum demersum L.
The TU values of the raw leachate from the active landfills ranged from 2.56 to 3.37. The TU values in the raw leachate from the closed landfills were much lower and ranged from 0.001 to 0.642. Also other authors during the research found that the TU values in the leachate from active landfills were much higher than the TU values for the leachate from old landfills (Kalka, 2012).
The obtained results indicate that the untreated leachate from the active landfills (Legnica, Jawor) shows acute toxicity of the tested aquatic invertebrates TU > 1 (Persoone et al., 2009; Barrios Restrepo et al., 2017; Przydatek, 2019b). The TU values only slightly improved in the leachate after the biological treatment with Phragmites australis. Toxicity was successfully reduced by 0.267 in the leachate from the Jawor landfill, and by 0.642 in the leachate from the Legnica landfill. Unfortunately, even after the treatment, the leachate produced acute toxicity (TU > 1) to Daphnia magna organisms. Better results were obtained after the treatment with Ceratophyllum demersum, where toxicity was reduced by 0.99 (Legnica) and by 0.85 (Jawor). However, the leachate still remained toxic to Daphnia magna organisms.
In the raw leachate from the non-operational landfill in Wrocław, the TU values were <0.4, which means no acute toxicity. In the raw leachate from the second non-operational landfill (in Bielawa), a slight acute toxicity was demonstrated (Persoone et al., 2003; Barrios Restrepo et al., 2017; Przydatek, 2019b). In the leachate from the oldest, non-operational landfill in Wrocław, after the treatment, a slight increase in the TU values was observed compared to the raw samples. However, it was still non-toxic to Daphnia magna. After the leachate from the Bielawa landfill had been treated with Ceratophyllum demersum, the TU value increased. The increase in the toxicity of leachate after treatment with Ceratophyllum demersum may result from the direct and mutual interaction of natural organic matter on Daphnia magna organisms - subjected to toxicity tests. Meems et al. and investigated the direct and interplay of natural organic matter on the toxicological effects of Daphnia magna. Demonstrated that natural organic matter can have a direct effect on Daphnia magna organisms, causing acute stress and toxic reactions (Meems et al., 2004).
A slight improvement was noted only in the case of the treatment of the leachate from Bielawa with Phragmites australis. After the treatment, the leachate still showed a slight acute activity. The applied biological treatment did not significantly reduce toxicity of the leachate, while other authors, during the research on biological treatment of leachates observed a complete absence or reduction of leachate toxicity to water fleas (Marttinen et al., 2002).
Conclusion
(1) Properties of landfill leachates (e.g. the content of AN, heavy metals, toxicity) may interfere with the processes of their biological treatment.
(2) The efficiency of biological treatment of leachates with Phragmites australis and Ceratophyllum demersum shows significant differences at lower concentrations of solutions.
(3) Even after the treatment with Phragmites australis and Ceratophyllum demersum, the analyzed leachate samples produced a toxic effect on the test organisms. A reduction or no toxicity of the leachate after the biological treatment was observed only in some cases.
(4) The conducted analyses showed that in some of the analyzed cases the biological treatment had a positive effect on the growth of the roots of Sinapis alba seed.
(5) The observed effects of the biological leachate treatment were not conclusive. Particular attention should be paid to the persistent toxicity, which can pose a real threat to the environment when discharging leachates after treatment. Therefore, it is worth extending the scope of further research with indicators demonstrating the possible impact of leachates on living organisms.
Data Availability Statement
The raw data supporting the conclusions of this article will be made available by the authors, without undue reservation.
Author Contributions
Conceptualization AS-P. AW, Methodology AS-P., AW, Validation AS-P Formal analysis AS-P. Investigation AW. Resources AW, AS-P. Data Curation AS-P., AW Writing-AW. Writing-Review and Editing AS-P. Visualization AS-P., AW, Supervision AS-P. Project administration AW.
Funding
B010/0004/20 Maintaining and developing the research potential of departments and institutes–subsidy 2020–IKSIG–Institute of Environmental Engineering. Environmental Engineering. Wrocław University of Environmental and Life Sciences (Poland) as the Ph.D. research program “Innowacyjny Doktorat”, no. N070/0001/21.
Conflict of Interest
The authors declare that the research was conducted in the absence of any commercial or financial relationships that could be construed as a potential conflict of interest.
Publisher’s Note
All claims expressed in this article are solely those of the authors and do not necessarily represent those of their affiliated organizations, or those of the publisher, the editors and the reviewers. Any product that may be evaluated in this article, or claim that may be made by its manufacturer, is not guaranteed or endorsed by the publisher.
Supplementary Material
The Supplementary Material for this article can be found online at: https://www.frontiersin.org/articles/10.3389/fenvs.2021.739562/full#supplementary-material
References
Abdallah, M. A. M. (2012). Phytoremediation of heavy metals from aqueous solutions by two aquatic macrophytes, Ceratophyllum demersumand Lemna gibbaL. Environ. Tech. 33, 1609–1614. doi:10.1080/09593330.2011.640354
Al-Yaqout, A., and Hamoda, M. F. (2020). Long-term temporal variations in characteristics of leachates from a closed landfill in an Arid Region. Water Air Soil Pollut. 231, 6. doi:10.1007/s11270-020-04688-7
Alkassasbe, J. Y. M., Heng, L. Y., and Surif, S. (2009). Toxicity testing and the effect of landfill leachate in Malaysia on behavior of common carp (Cyprinus carpio L., 1758; Pisces, Cyprinidae). Am. J. Environ. Sci. 5, 209–217. doi:10.3844/ajessp.2009.209.217
Antić, K., Pap, S., Novaković, M., Sekulić, M. T., Adamović, D., and Radonić, J. (2018). Removal of heavy metal ions from landfill leachate by phytoremediation using Eichhornia crassipes. Ind. Wastewater Treat. Other Top. Remov., 427–433.
Barrios Restrepo, J. J., Flohr, L., Melegari, S. P., da Costa, C. H., Fuzinatto, C. F., de Castilhos, A. B., et al. (2017). Correlation between acute toxicity for Daphnia magna, Aliivibrio fischeri and physicochemical variables of the leachate produced in landfill simulator reactors. Environ. Tech. 38, 2898–2906. doi:10.1080/09593330.2017.1281352
Białowiec, A., Davies, L., Albuquerque, A., and Randerson, P. F. (2012). Nitrogen removal from landfill leachate in constructed wetlands with reed and willow: Redox potential in the root zone. J. Environ. Manage. 97, 22–27. doi:10.1016/j.jenvman.2011.11.014
Białowiec, A., and Kasiński, S. (2009). Neutralization of landfill leachate in plant-ground systems - Possibilities of selecting leachate dose in the initial phase of plant development. Annu. Set Env. Prot. 11, 1267–1278. (in Polish).
Białowiec, A., Koziel, J., and Manczarski, P. (2019). Stomatal Conductance Measurement for Toxicity Assessment in Zero-Effluent Constructed Wetlands: Effects of Landfill Leachate on Hydrophytes. Ijerph 16, 468. doi:10.3390/ijerph16030468
Boateng, T. K., Opoku, F., and Akoto, O. (2019). Heavy metal contamination assessment of groundwater quality: a case study of Oti landfill site, Kumasi. Appl. Water Sci. 9, 1–15. doi:10.1007/s13201-019-0915-y
Borjac, J., El Joumaa, M., Kawach, R., Youssef, L., and Blake, D. A. (2019). Heavy metals and organic compounds contamination in leachates collected from Deir Kanoun Ras El Ain dump and its adjacent canal in South Lebanon. Heliyon 5, e02212. doi:10.1016/j.heliyon.2019.e02212
Bowman, M. S., Clune, T. S., and Sutton, B. G. (2002). Sustainable management of landfill leachate by irrigation. Water. Air Soil Pollut. 134, 81–96. doi:10.1023/A:1014114500269
Bożym, M., Król, A., and Mizerna, K. (2020). Leachate and contact test with Lepidium sativum L. to assess the phytotoxicity of waste. Int. J. Environ. Sci. Technol. 18, 1975–1990. doi:10.1007/s13762-020-02980-x
Calabrese, E., and Blain, R. (2005). The occurrence of hormetic dose responses in the toxicological literature, the hormesis database: An overview. Toxicol. Appl. Pharmacol. 202, 289–301. doi:10.1016/j.taap.2004.06.023
Christensen, T. H., Kjeldsen, P., Bjerg, P. L., Jensen, D. L., Christensen, J. B., Baun, A., et al. (2001). Biogeochemistry of landfill leachate plumes. Appl. Geochem.. doi:10.1016/S0883-2927(00)00082-2
Cui, R., Chae, Y., and An, Y.-J. (2017). Dimension-dependent toxicity of silver nanomaterials on the cladocerans Daphnia magna and Daphnia galeata. Chemosphere 185, 205–212. doi:10.1016/j.chemosphere.2017.07.011
Daud, M. K., Ali, S., Abbas, Z., Zaheer, I. E., Riaz, M. A., Malik, A., et al. (2018). Potential of Duckweed (Lemna minor) for the phytoremediation of landfill leachate. J. Chem. 2018, 1–9. doi:10.1155/2018/3951540
Dave, G., and Nilsson, E. (2005). Increased reproductive toxicity of landfill leachate after degradation was caused by nitrite. Aquat. Toxicol. 73, 11–30. doi:10.1016/j.aquatox.2005.02.006
Ding, K., Lu, L., Wang, J., Wang, J., Zhou, M., Zheng, C., et al. (2017). In vitro and in silico investigations of the binary-mixture toxicity of phthalate esters and cadmium (II) to Vibrio qinghaiensis sp.-Q67. Sci. Total Environ. 580, 1078–1084. doi:10.1016/j.scitotenv.2016.12.062
Ehrig, H. (1983). Quality and quantity of sanitary landfill leachate. Waste Manag. Res. 1, 53–68. doi:10.1016/0734-242x(83)90024-1
European Committee for Standardization (2012). Water Quality - Determination of the Inhibition of the mobility of Daphnia magna Straus (Cladocera, Crustacea) - Acute toxicity test. Belgium: Brussels. doi:10.9774/GLEAF.978-1-907643-27-9_9
Fauziah, S. H., Izzati, M. N., and Agamuthu, P. (2013). Toxicity on Anabas Testudineus: a case study of sanitary landfill leachate. Proced. Environ. Sci. 18, 14–19. doi:10.1016/j.proenv.2013.04.003
Fitzke, B., Blume, T., Wienands, H., and Cambiella, Á. (2013). Hybrid processes for the treatment of leachate from landfills. NATO Sci. Peace Secur. Ser. C Environ. Secur., 107–126. doi:10.1007/978-94-007-5079-1_6
Foroughi, M., Najafi, P., Toghiani, A., and Honarjoo, N. (2010). Analysis of pollution removal from wastewater by Ceratophyllum demersum. Afr. J. Biotechnol. 9, 2125–2128.
Foroughi, M., Najafi, P., Toghiani, S., Toghiani, A., and Honarjoo, N. (2013). Nitrogen Removals by Ceratophyllum Demersum from Wastewater. J. Residuals Sci. Technol. 10, 63–68.
García-Lorenzo, M. L., Martínez-Sánchez, M. J., and Pérez-Sirvent, C. (2014). Application of a plant bioassay for the evaluation of ecotoxicological risks of heavy metals in sediments affected by mining activities. J. Soils Sediments 14, 1753–1765. doi:10.1007/s11368-014-0942-0
Goi, A., Veressinina, Y., and Trapido, M. (2009). Combination of ozonation and the Fenton processes for landfill leachate treatment: Evaluation of treatment efficiency. Ozone: Sci. Eng. 31, 28–36. doi:10.1080/01919510802582011
Guerrero-Rodríguez, D., Sánchez-Yáñez, J. M., Buenrostro-Delgado, O., and Márquez-Benavides, L. (2014). Phytotoxic effect of landfill leachate with different pollution indexes on common bean. Water Air Soil Pollut. 225, 2002. doi:10.1007/s11270-014-2002-1
Gworek, B., Dmuchowski, W., Koda, E., Marecka, M., Baczewska, A., Brągoszewska, P., et al. (2016). Impact of the Municipal Solid Waste Łubna Landfill on Environmental Pollution by Heavy Metals. Water 8, 470. doi:10.3390/w8100470
Hassan, S. H. A., Van Ginkel, S. W., Hussein, M. A. M., Abskharon, R., and Oh, S.-E. (2016). Toxicity assessment using different bioassays and microbial biosensors. Environ. Int. 92-93, 106–118. doi:10.1016/j.envint.2016.03.003
Hussein, M., Yoneda, K., Mohd-Zaki, Z., Amir, A., and Othman, N. (2021). Heavy metals in leachate, impacted soils and natural soils of different landfills in Malaysia: An alarming threat. Chemosphere 267, 128874. doi:10.1016/j.chemosphere.2020.128874
Isidori, M., Lavorgna, M., Nardelli, A., and Parrella, A. (2003). Toxicity identification evaluation of leachates from municipal solid waste landfills: A multispecies approach. Chemosphere 52, 85–94. doi:10.1016/S0045-6535(03)00298-4
Jabłońska-Trypuć, A., Wydro, U., Wołejko, E., Pietryczuk, A., Cudowski, A., Leszczyński, J., et al. (2021). Potential Toxicity of Leachate from the Municipal Landfill in View of the Possibility of their Migration to the Environment Through Infiltration into Groundwater. Environ. Geochem. Health 43, 3683–3698. doi:10.1007/s10653-021-00867-5
Jemec, A., Tišler, T., and Žgajnar-Gotvajn, A. (2012). Assessment of landfill leachate toxicity reduction after biological treatment. Arch. Environ. Contam. Toxicol. 62, 210–221. doi:10.1007/s00244-011-9703-x
Jorstad, L. B., Jankowski, J., and Acworth, R. I. (2004). Analysis of the distribution of inorganic constituents in a landfill leachate-contaminated aquifer: Astrolabe Park, Sydney, Australia. Env Geol. 46, 263–272. doi:10.1007/s00254-004-0978-3
Kalčíková, G., Zagorc-Končan, J., Zupančič, M., and Gotvajn, A. Ž. (2012). Variation of landfill leachate phytotoxicity due to landfill ageing. J. Chem. Technol. Biotechnol. 87, 1349–1353. doi:10.1002/jctb.3776
Kalka, J. (2012). Landfill leachate toxicity removal in combined treatment with municipal wastewater. Scientific World J. 2012, 1–7. doi:10.1100/2012/202897
Kheradmand, S., Karimi-Jashni, A., and Sartaj, M. (2010). Treatment of municipal landfill leachate using a combined anaerobic digester and activated sludge system. Waste Manag. 30, 1025–1031. doi:10.1016/j.wasman.2010.01.021
Kjeldsen, P., Barlaz, M. A., Rooker, A. P., Baun, A., Ledin, A., and Christensen, T. H. (2002). Present and long-term composition of MSW landfill leachate: A review. Crit. Rev. Environ. Sci. Tech. 32, 297–336. doi:10.1080/10643380290813462
Klauck, C., Rodrigues, M., and Silva, L. (2015). Evaluation of phytotoxicity of municipal landfill leachate before and after biological treatment. Braz. J. Biol. 75, 57–62. doi:10.1590/1519-6984.1813
Kulikowska, D., and Klimiuk, E. (2008). The effect of landfill age on municipal leachate composition. Bioresour. Tech. 99, 5981–5985. doi:10.1016/j.biortech.2007.10.015
Kummerová, M., and Kmentová, E. (2004). Photoinduced Toxicity of Fluoranthene on Germination and Early Development of Plant Seedling. Chemosphere 56, 837–393. doi:10.1016/j.chemosphere.2004.01.007
Kurniawan, T. A., Lo, W., Chan, G., and Sillanpää, M. E. T. (2010). Biological processes for treatment of landfill leachate. J. Environ. Monit. 12, 2032. doi:10.1039/c0em00076k
Kwarciak-Kozłowska, A., and Fijałkowski, K. L. (2021). Efficiency assessment of municipal landfill leachate treatment during advanced oxidation process (AOP) with biochar adsorption (BC). J. Environ. Manage. 287, 112309. doi:10.1016/j.jenvman.2021.112309
Lari, E., Steinkey, D., Steinkey, R. J., and Pyle, G. G. (2018). Daphnia magna increase feeding activity in the presence of four amino acids. J. Plankton Res. 40, 537–543. doi:10.1093/plankt/fby038
Liang, Y., Zhu, H., Bañuelos, G., Yan, B., Zhou, Q., Yu, X., et al. (2017). Constructed wetlands for saline wastewater treatment: A review. Ecol. Eng. 98, 275–285. doi:10.1016/j.ecoleng.2016.11.005
Liu, J., Hu, J., Zhong, J., Luo, J., Zhao, A., Liu, F., et al. (2011). The effect of calcium on the treatment of fresh leachate in an expanded granular sludge bed bioreactor. Bioresour. Tech. 102, 5466–5472. doi:10.1016/j.biortech.2010.11.056
Maiorana, S., Teoldi, F., Silvani, S., Mancini, A., Sanguineti, A., Mariani, F., et al. (2019). Phytotoxicity of wear debris from traditional and innovative brake pads. Environ. Int. 123, 156–163. doi:10.1016/j.envint.2018.11.057
Manakul, P., Peerakietkhajorn, S., Matsuura, T., Kato, Y., and Watanabe, H. (2017). Effects of symbiotic bacteria on chemical sensitivity of Daphnia magna. Mar. Environ. Res. 128, 70–75. doi:10.1016/j.marenvres.2017.03.001
Marttinen, S. K., Kettunen, R. H., Sormunen, K. M., Soimasuo, R. M., and Rintala, J. A. (2002). Screening of physical-chemical methods for removal of organic material, nitrogen and toxicity from low strength landfill leachates. Chemosphere 46, 851–858. doi:10.1016/S0045-6535(01)00150-3
Meems, N., Steinberg, C. E., and Wiegand, C. (2004). Direct and interacting toxicological effects on the waterflea (Daphnia magna) by natural organic matter, synthetic humic substances and cypermethrin. Sci. Total Environ. 319, 123–136. doi:10.1016/S0048-9697(03)00445-5
Miao, L., Yang, G., Tao, T., and Peng, Y. (2019). Recent advances in nitrogen removal from landfill leachate using biological treatments - A review. J. Environ. Manage. 235, 178–185. doi:10.1016/j.jenvman.2019.01.057
Milke, J., Gałczyńska, M., and Wróbel, J. (2020). The Importance of Biological and Ecological Properties of Phragmites Australis (Cav.) Trin. Ex Steud., in Phytoremendiation of Aquatic Ecosystems-The Review., in phytoremendiation of aquatic ecosystems-the review. Water 12, 1770. doi:10.3390/w12061770
Mohammad-pajooh, E., Weichgrebe, D., and Cuff, G. (2017). Municipal landfill leachate characteristics and feasibility of retrofitting existing treatment systems with deammonification - A full scale survey. J. Environ. Manage. 187, 354–364. doi:10.1016/j.jenvman.2016.10.061
Morozesk, M., Bonomo, M. M., Souza, I. d. C., Rocha, L. D., Duarte, I. D., Martins, I. O., et al. (2017). Effects of humic acids from landfill leachate on plants: An integrated approach using chemical, biochemical and cytogenetic analysis. Chemosphere 184, 309–317. doi:10.1016/j.chemosphere.2017.06.007
Nájera-Aguilar, H. A., Gutiérrez-Hernández, R. F., Bautista-Ramírez, J., Martínez-Salinas, R. I., Escobar-Castillejos, D., Borraz-Garzón, R., et al. (2019). Treatment of low biodegradability leachates in a serial system of aged refuse-filled bioreactors. Sustainability 11, 3193. doi:10.3390/su11113193
Naveen, B. P., Mahapatra, D. M., Sitharam, T. G., Sivapullaiah, P. V., and Ramachandra, T. V. (2017). Physico-chemical and biological characterization of urban municipal landfill leachate. Environ. Pollut. 220, 1–12. doi:10.1016/j.envpol.2016.09.002
Öman, C. B., and Junestedt, C. (2008). Chemical characterization of landfill leachates - 400 parameters and compounds. Waste Manag. 28, 1876–1891. doi:10.1016/j.wasman.2007.06.018
Persoone, G., Baudo, R., Cotman, M., Blaise, C., Thompson, K. C., Moreira-Santos, M., et al. (2009). Review on the acuteDaphnia magnatoxicity test - Evaluation of the sensitivity and the precision of assays performed with organisms from laboratory cultures or hatched from dormant eggs. Knowl. Managt. Aquat. Ecosyst., 01. doi:10.1051/kmae/2009012
Persoone, G., Marsalek, B., Blinova, I., Törökne, A., Zarina, D., Manusadzianas, L., et al. (2003). A practical and user-friendly toxicity classification system with microbiotests for natural waters and wastewaters. Environ. Toxicol. 18, 395–402. doi:10.1002/tox.10141
Przydatek, G. (2019a). Multi-indicator analysis of the influence of old municipal landfill sites on the aquatic environment: case study. Environ. Monit. Assess. 191, 773. doi:10.1007/s10661-019-7814-4
Przydatek, G. (2019b). The analysis of the possibility of using biological tests for assessment of toxicity of leachate from an active municipal landfill. Environ. Toxicol. Pharmacol. 67, 94–101. doi:10.1016/j.etap.2019.01.013
Przydatek, G. (2021). Using advanced statistical tools to assess the impact of a small landfill site on the aquatic environment. Environ. Monit. Assess., 193. doi:10.1007/s10661-021-08850-4
Rahman, M. E., Bin Halmi, M. I. E., Bin Abd Samad, M. Y., Uddin, M. K., Mahmud, K., Abd Shukor, M. Y., et al. (2020). Design, operation and optimization of constructed wetland for removal of pollutant. Ijerph 17, 8339–8340. doi:10.3390/ijerph17228339
Ravi, V., Williams, J. R., States, U., and Management, N. R. (1998). Estimation of infiltration rate in the vadose zone: compilation of simple mathematical models. Washington, DC: US Environmental Protection Agency I, 26.
Renou, S., Givaudan, J. G., Poulain, S., Dirassouyan, F., and Moulin, P. (2008). Landfill leachate treatment: Review and opportunity. J. Hazard. Mater. 150, 468–493. doi:10.1016/j.jhazmat.2007.09.077
Rudrappa, T., Bonsall, J., Gallagher, J. L., Seliskar, D. M., and Bais, H. P. (2007). Root-secreted allelochemical in the noxious weed Phragmites australis deploys a reactive oxygen species response and microtubule assembly disruption to execute rhizotoxicity. J. Chem. Ecol. 33, 1898–1918. doi:10.1007/s10886-007-9353-7
Sackey, L. N. A., Kočí, V., and van Gestel, C. A. M. (2020). Ecotoxicological effects on Lemna minor and Daphnia magna of leachates from differently aged landfills of Ghana. Sci. Total Environ. 698, 134295. doi:10.1016/j.scitotenv.2019.134295
Šourková, M., Adamcová, D., Zloch, J., Skutnik, Z., and Vaverková, M. D. (2020). Evaluation of the phytotoxicity of leachate from a municipal solid waste landfill: The case study of Bukov landfill. Environments 7, 111–114. doi:10.3390/environments7120111
Tahmasbian, I., and Safari Sinegani, A. A. (2016). Improving the efficiency of phytoremediation using electrically charged plant and chelating agents. Environ. Sci. Pollut. Res. 23, 2479–2486. doi:10.1007/s11356-015-5467-6
Tatsi, A. A., and Zouboulis, A. I. (2002). A field investigation of the quantity and quality of leachate from a municipal solid waste landfill in a Mediterranean climate (Thessaloniki, Greece)field investigation of the quantity and quality of leachate from a municipal solid waste landfill in a Mediterranean climate (Thessaloniki, Greece). Adv. Environ. Res. 6 (3), 207–219. doi:10.1016/S1093-0191(01)00052-1
Thomas, D. J. L., Tyrrel, S. F., Smith, R., and Farrow, S. (2009). Bioassays for the evaluation of landfill leachate toxicity. J. Toxicol. Environ. Health B 12, 83–105. doi:10.1080/10937400802545292
Thomsen, N. I., Milosevic, N., and Bjerg, P. L. (2012). Application of a contaminant mass balance method at an old landfill to assess the impact on water resources. Waste Manag. 32, 2406–2417. doi:10.1016/j.wasman.2012.06.014
Tomczyk, P., and Wiatkowski, M. (2021). The effects of hydropower plants on the physicochemical parameters of the Bystrzyca river in Poland. Energies 14, 2075. doi:10.3390/en14082075
Uddin, M. N., Robinson, R. W., and Caridi, D. (2014). Phytotoxicity induced by Phragmites australis: An assessment of phenotypic and physiological parameters involved in germination process and growth of receptor plant. J. Plant Interactions 9, 338–353. doi:10.1080/17429145.2013.835879
Umar, M., Aziz, H. A., and Yusoff, M. S. (2010). Variability of parameters involved in leachate pollution index and determination of LPI from four landfills in Malaysia. Int. J. Chem. Eng. 2010, 1–6. doi:10.1155/2010/747953
Vaisi, R., and Žaltauskaitė, J. (2010). Evaluation of municipal effluent toxicity using higher plants and invertebrates. Environ. Res. Eng. Manag. 3, 17–23.
Vaverková, M. D., Elbl, J., Koda, E., Adamcová, D., Bilgin, A., Lukas, V., et al. (2020). Chemical composition and hazardous effects of leachate from the active municipal solid waste landfill surrounded by farmlands. Sustainability 12, 4531. doi:10.3390/su12114531
Vedrenne, M., Vasquez-Medrano, R., Prato-Garcia, D., Frontana-Uribe, B. A., and Ibanez, J. G. (2012). Characterization and detoxification of a mature landfill leachate using a combined coagulation-flocculation/photo Fenton treatment. J. Hazard. Mater. 205-206, 208–215. doi:10.1016/j.jhazmat.2011.12.060
Wdowczyk, A., and Szymańska-Pulikowska, A. (2021a). Analysis of the possibility of conducting a comprehensive assessment of landfill leachate contamination using physicochemical indicators and toxicity test. Ecotoxicology Environ. Saf. 221, 112434. doi:10.1016/j.ecoenv.2021.112434
Wdowczyk, A., and Szymańska-Pulikowska, A. (2021b). Comparison of Landfill Leachate Properties by LPI and Phytotoxicity-A Case Study. Front. Environ. Sci. 9, 1–14. doi:10.3389/fenvs.2021.693112
Wdowczyk, A., and Szymańska-Pulikowska, A. (2020). Differences in the composition of leachate from active and non-operational municipal waste landfills in Poland. Water 12 (11), 3129. doi:10.3390/w12113129
Xaypanya, P., Takemura, J., Chiemchaisri, C., Seingheng, H., and Tanchuling, M. (2018). Characterization of Landfill Leachates and Sediments in Major Cities of Indochina Peninsular Countries-Heavy Metal Partitioning in Municipal Solid Waste Leachate. Environments 5, 65. doi:10.3390/environments5060065
Yalcuk, A., and Ugurlu, A. (2009). Comparison of horizontal and vertical constructed wetland systems for landfill leachate treatment. Bioresour. Tech. 100, 2521–2526. doi:10.1016/j.biortech.2008.11.029
Zainol, N. A., Aziz, H. A., and Yusoff, M. S. (2012). Characterization of leachate from Kuala Sepetang and Kulim landfills: A Comparative Study. Eer 2. doi:10.5539/eer.v2n2p45
Zloch, J., Vaverková, M. D., Adamcová, D., Radziemska, M., Vyhnánek, T., Trojan, V., et al. (2018). Seasonal changes and toxic potency of landfill leachate for white mustard (Sinapis alba L.). Acta Univ. Agric. Silvic. Mendelianae Brun. 66, 235–242. doi:10.11118/actaun201866010235
Keywords: landfill leachate, biological treatment, Phragmites australis, Ceratophyllum demersum, toxicity tests, physicochemical composition
Citation: Szymańska-Pulikowska A and Wdowczyk A (2021) Changes of a Landfill Leachate Toxicity as a Result of Treatment With Phragmites australis and Ceratophyllum demersum–A Case Study. Front. Environ. Sci. 9:739562. doi: 10.3389/fenvs.2021.739562
Received: 11 July 2021; Accepted: 30 August 2021;
Published: 10 September 2021.
Edited by:
Ahmed El Nemr, National Institute of Oceanography and Fisheries, EgyptReviewed by:
Anshu Gupta, Government of Jammu and Kashmir, IndiaYoucai Zhao, Tongji University, China
Copyright © 2021 Szymańska-Pulikowska and Wdowczyk. This is an open-access article distributed under the terms of the Creative Commons Attribution License (CC BY). The use, distribution or reproduction in other forums is permitted, provided the original author(s) and the copyright owner(s) are credited and that the original publication in this journal is cited, in accordance with accepted academic practice. No use, distribution or reproduction is permitted which does not comply with these terms.
*Correspondence: Aleksandra Wdowczyk, YWxla3NhbmRyYS53ZG93Y3p5a0B1cHdyLmVkdS5wbA==