- 1South Australian Museum, Adelaide, SA, Australia
- 2Invertebrate Systematics and Biodiversity Laboratory, School of Biological Sciences, The University of Adelaide, Adelaide, SA, Australia
- 3Molecular Biodiversity Laboratory, Department of Environment and Genetics, La Trobe University, Melbourne, VIC, Australia
- 4Trace and Environmental DNA (TrEnD) Laboratory, School of Molecular and Life Sciences, Curtin University, Bentley, WA, Australia
The Great Artesian Basin of Australia represents one of the largest and deepest basins of freshwater on Earth. Thousands of springs fed by the Basin are scattered across Australia’s arid zone, often representing the sole sources of freshwater for thousands of kilometers. As “islands” in the desert, the springs support endemic fauna and flora that have undergone millions of years of evolution in almost total isolation. Here, we review the current body of knowledge surrounding Great Artesian Basin springs and their significance from ecological, evolutionary, and cultural perspectives using South Australian spring wetlands as a case study. We begin by identifying the status of these springs as critical sources of groundwater, the unique biodiversity they support, and their cultural significance to the Arabana people as Traditional Custodians of the land. We then summarize known threats to the springs and their biota, both exogenous and endogenous, and the potential impacts of such processes. Finally, considering the status of these at-risk habitats as time capsules of biodiversity, we discuss lessons that can be learnt from current conservation and management practices in South Australia. We propose key recommendations for improved biodiversity assessment and monitoring of Great Artesian Basin springs nationwide, including 1) enhanced legal protections for spring biota; 2) increased taxonomic funding and capacity; 3) improved biodiversity monitoring methods, and 4) opportunities for reciprocal knowledge-sharing with Aboriginal peoples when conducting biodiversity research.
1 Introduction
The Great Artesian Basin (GAB) is an expansive aquifer spanning an area of 1.7 million km2 in arid and semi-arid regions of Australia. In addition to representing one of the world’s largest sources of groundwater the GAB feeds around 6,300 individual springs, approximately 80% of which occur in South Australia (SA) (National Parks South Australia, 2017) with the remainder in Queensland and New South Wales. GAB springs, also called mound springs in certain states (Fensham et al., 2010), are often the only permanent bodies of freshwater for thousands of kilometers in the arid interior of the country. Springs have persisted for upwards of 1 million years at certain sites (Love et al., 1993) and represent immeasurable value from both biodiversity and cultural perspectives (e.g. the Environment Protection and Biodiversity Conservation Act 1999, hereafter EPBC Act; Dodd v State of South Australia [2012] FCA 519; Malone v State of Queensland [2019] FCA 2115), supporting extraordinary numbers of endemic species not found elsewhere on Earth (Murphy et al., 2015a; CAPAD, 2016; Rossini et al., 2018) and serving as the foci of ancient songlines vital to Aboriginal cultural identity (Nursey-Bray et al., 2020).
The GAB springs have been managed using traditional practices by Aboriginal peoples for at least 5,000 years (Florek, 1993). However, substantial modifications have been made to these water sources since European colonization, particularly via the sinking of bores, which have lowered aquifer pressure and reduced emergent water from springs. Contemporary threats to the springs include extraction of water for industrial and pastoral practices, grazing and trampling of wetlands by livestock, the presence of invasive (or overabundant native) fauna and flora, disruption of habitat by tourists, and climate change (Brake, 2020). All of these processes have the potential to impact spring environments in the long term. Indeed, changes have already been observed at certain sites: these include declines and extinctions of fauna, decreased water flow rate, and disturbance of spring sediment (Mudd, 2000; Noack, 2003; Guzik et al., 2012). Unfortunately, the exceptionally diverse GAB spring fauna and flora are not adequately protected in the face of these threats under existing federal Australian environmental legislation (Rossini, 2020), and many springs of high biodiversity and cultural value sit outside of protected areas such as conservation parks (Rossini et al., 2018; Habermehl, 2020a). The cultural heritage of GAB springs have also not been appropriately respected or recognised, either by government legislation or industrial stakeholders (Parliament of Australia, 2021).
The GAB springs clearly require our renewed attention and protection. However, the majority of existing conservation efforts to ameliorate threats to GAB springs have primarily focused on improving artesian pressure—and therefore the flow of emergent water—and the fencing of wetlands, to varying degrees of success (Fatchen and Fatchen 1993; Fensham et al., 2019; GABCC 2019). At the heart of these efforts is a national strategic management plan that has facilitated the rehabilitation of over 700 bores through a GAB-wide capping program (Great Artesian Basin Coordinating Committee, 2019). The rehabilitation of bores has led to increased water flow rates at certain springs (Fensham et al., 2019), associated with healthier wetlands (Fensham and Laffineur, 2022). However, approximately a third of bores still flow uncontrollably and other remaining threats, such as surface disturbances caused by livestock, have received comparatively little attention to date (Brake, 2020). As a result, an adaptive management plan was recently proposed to ensure the future survival of GAB springs (Brake et al., 2020). This integrated approach advocates for a range of measures, including a stronger evidence base related to spring environmental characteristics, the ongoing monitoring of spring condition, and improved biodiversity assessments. Nonetheless, major knowledge gaps mean that our capacity to improve and implement biodiversity assessment and monitoring is limited due to poor understandings of GAB spring biodiversity in the first place.
A comprehensive biodiversity assessment of GAB springs is critical because species reliant on such vulnerable habitats are at risk of decline themselves. This absence of taxonomic reference information has led to a paucity of biodiversity knowledge for the GAB springs more broadly and is a well-recognized “taxonomic impediment” to robust understandings of similarly underexplored environments (Ficetola et al., 2019). If the biotic inventory of a habitat is poorly known, such an impediment can render any spring bioassessment or monitoring program lacking in ecological sensitivity. Extreme vulnerabilities therefore exist for GAB spring endemic fauna in the face of the above-mentioned habitat degradation, as the vast majority cannot and have not been effectively assessed regarding their extinction risk (Rossini et al., 2018; Rossini, 2020). Further, without formal names and/or identification methods, these fauna also cannot be effectively protected by environmental legislation such as the EPBC Act, exacerbating their vulnerability. Ultimately, one of the most fundamental issues facing scientists, managers, and legislators is that a unified mechanism by which GAB spring biodiversity value can be assessed and monitored does not currently exist.
Several robust reviews on GAB springs as “oases of life” have been conducted from diverse angles in response to concerns surrounding their survival. These have included retrospectives, papers on legal mechanisms of protection, the cultural significance of springs, spring hydrogeology, and the biogeography and biodiversity of ecological communities dependent on springs (Nursey-Bray and Arabana Aboriginal Corporation, 2015a; Nursey-Bray and Arabana Aboriginal Corporation, 2015b; Rossini et al., 2018; Habermehl, 2020a; 2020b; Harris, 2020; Kerezsy, 2020; Moggridge, 2020; Nursey-Bray et al., 2020; Pointon and Rossini, 2020; Rossini, 2020). Clear issues and gaps in knowledge have been identified, primarily in relation to management directives, awareness, and conservation of the system as a whole or endemic species therein. However, most reviews on spring biodiversity have primarily concentrated on Queensland wetlands, in stark contrast to the fact that approximately 80% of GAB springs are found in South Australia (Rossini et al., 2017, 2018, 2020; Pointon and Rossini, 2020). The ecological communities reliant on GAB springs in South Australia are some of the best-characterized in the system from a taxonomic and genetic standpoint. However, even in these springs true measures of diversity of fauna and flora are no doubt vastly underestimated due to the widespread presence of undescribed species that cannot be distinguished from one another morphologically (i.e., cryptic species) (Murphy et al., 2009; 2015b; Guzik et al., 2012; Guzik and Murphy, 2013). Advancements towards better understandings of these biota offer a roadmap ahead for characterizing and conserving the biodiversity of springs in other states. In South Australia, much of the GAB spring fauna consists of invertebrates that are ultra-short-range endemics (i.e., with distributions <100 km2) (Guzik et al., 2019) and at risk of decline, though the majority remain undescribed and lack formal names. Springs in Queensland are similarly ecologically diverse, but almost no genetic or taxonomic research has been conducted on that fauna to examine the presence of cryptic or undescribed species (Rossini 2020). To our knowledge no such work has been conducted on the New South Wales springs.
In addition to being well-characterized from a biodiversity standpoint, a substantial body of knowledge exists regarding the Aboriginal significance of South Australian GAB springs. The involvement of Aboriginal peoples in natural resource management, including the GAB springs, has been intentionally limited on a systematic level in Australia (Parliament of Australia, 2021; Samuel, 2021). However, this status quo is slowly changing. In the case of the GAB springs, Aboriginal names and stories associated with springs have been collated on a fine geographic scale (Hercus and Sutton, 1985)—of note as many Australian Aboriginal languages are threatened with extinction (McConvell and Thieberger, 2001)—and the potential and realized cultural impacts of spring loss have been documented in collaboration with Traditional Custodians (Nursey-Bray et al., 2020). Frameworks have also been proposed for the co-management of GAB springs that utilize Aboriginal knowledge alongside Western scientific understandings (Nursey-Bray and Arabana Aboriginal Corporation, 2015a, 2015b). As such, South Australian GAB springs provide an opportunity to amplify Aboriginal voices and develop understandings of spring biodiversity in partnership with Aboriginal knowledge.
Compared to other springs fed by the GAB nationwide, those found in South Australia are some of the best-studied from biodiversity and taxonomic standpoints. This is particularly the case for cryptic species, which to our knowledge have not been characterized in other states and hint at a much more diverse biota than is currently known. With this in mind, we believe South Australian GAB springs represent an underutilized resource for the development of a roadmap ahead to conserve and characterize groundwater-dependent ecosystems GAB-wide. In the current review, we use South Australian GAB (hereafter SA GAB) springs as a case study to propose future directions for GAB spring biota within the state and beyond. We begin by providing an overview of the biodiversity of the SA GAB springs and their cultural significance. We then discuss current threats to SA springs, the potential impacts of such threats, and lessons that can be applied to the GAB as a broader system, particularly with respect to biodiversity and taxonomic research. In doing so, we provide recommendations to facilitate future research on groundwater-dependent ecosystems supported by the GAB by proposing: 1) enhanced legal protections of GAB spring taxa under federal environmental legislation; 2) increased taxonomic funding and capacity to ensure members of these communities are named; 3) mechanisms for effective monitoring of such taxa using novel, less invasive technologies compared to traditional surveys; and finally 4) opportunities for collaboration with Aboriginal peoples to inform spring management and biodiversity research.
2 SA GAB spring terminology
Great Artesian Basin-fed springs can either be generally categorized as recharge springs, which form due to overspilling of groundwater at recharge points, or discharge springs, which form at geological faults (Fensham and Fairfax, 2003). Springs fed by the GAB in South Australia are predominantly the latter and, being at the extreme westerly margin of the GAB, are reliant on water which has traveled thousands of kilometers through aquifers from the western slopes of the Great Dividing Range in eastern Australia. South Australian GAB springs are generally grouped together using a hierarchical framework established in the 1980s (Ponder, 1986) and standardized relatively recently by the Allocating Water and Maintaining Springs in the Great Artesian Basin (AWMSGAB) project (Lewis et al., 2013). Discrete discharge points of water from the GAB, also called vents, are the smallest unit used when referring to springs from a policy standpoint and are joined together by permanent wetland vegetation to form springs. In some cases, springs are represented by a single vent. Tails are the distal ends of wetlands that flow away from vents (see Figure 1). Springs are clustered together to form spring groups, which themselves form spring complexes. Supergroups represent the broadest geographic classification of GAB springs. Figure 2.
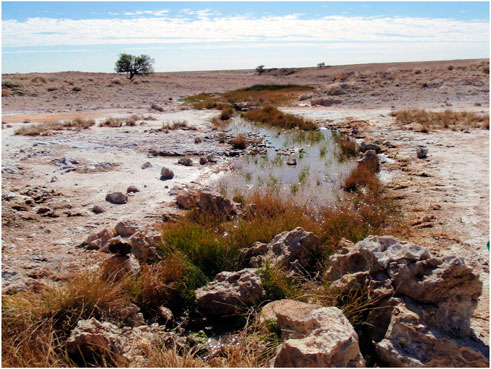
FIGURE 1. A Great Artesian Basin-fed spring vent (foreground) in the Elizabeth North spring group (Kati Thanda–Lake Eyre supergroup), South Australia with a distinctive distal “tail” (background) formed by the overflow of groundwater. Tails support wetlands that house endemic fauna and flora and act as refuges for migratory species such as waterbirds. Photo credit Rachael King.
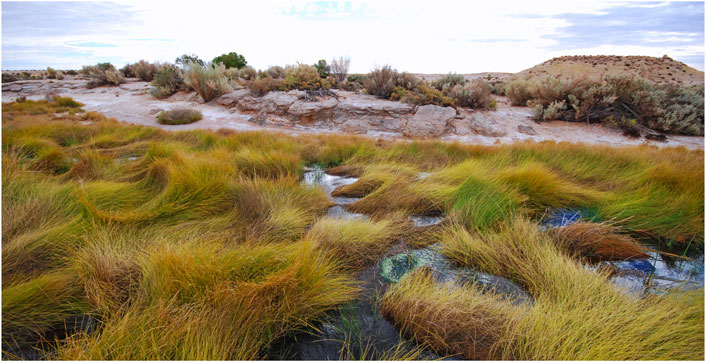
FIGURE 2. View of vegetation in the Jersey spring group (Kati Thanda–Lake Eyre supergroup) dominated by bore-drain sedge (Cyperus laevigatus). Photo credit Rachael King.
The above hierarchical classification also considers hydrogeological factors: spring groups and complexes cluster spring units together that broadly share water chemistry, geomorphology, or source water from the same fault or structure (Fatchen and Fatchen, 1993). For consistency we here employ the spring hierarchy and naming conventions of the AWMSGAB project. However, other classification systems or clustering definitions have been used historically (Habermehl, 1982; Zeidler and Ponder, 1989; Kinhill Engineers, 1997; Fensham and Fairfax, 2003), and may also differ when referring to discharge points of the GAB in other Australian states (Fensham and Price, 2004; Rossini et al., 2018). Three spring supergroups exist in South Australia: Dalhousie, Lake Eyre, and Lake Frome (Figure 3). We note that since 2012, the salt lake basin Lake Eyre has been officially known by the dual name Kati Thanda–Lake Eyre in acknowledgement of the traditional lands of the Arabana people (detailed below). We will therefore refer to that supergroup as Kati Thanda–Lake Eyre. Per the naming system of Lewis et al. (2013), the three South Australian supergroups contain 22 spring complexes, which themselves encompass 169 individual spring groups. Prior to the above-mentioned standardization, other complexes or groups were infrequently recognized in the literature but have since been subsumed within other sites: for example, the Mount Hopeless complex in the Lake Frome supergroup is now split into the Petermorra, Reedy, and Lake Blanche complexes (Social and Ecological Assessment Pty Ltd, 1986).
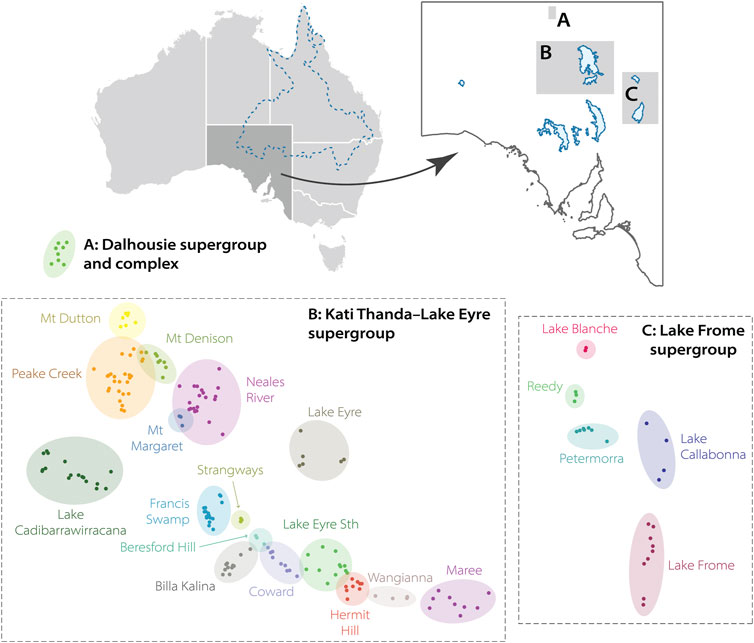
FIGURE 3. Location and naming of the three Great Artesian Basin (GAB) spring supergroups in South Australia following Lewis et al. (2013): (A) Dalhousie, (B) Kati Thanda–Lake Eyre, (C) Lake Frome. The approximate extent of the GAB is indicated top-left. Spring complexes (coloured circles) contain spring groups (points). The Maree complex is also referred to as Marree under certain classifications.
3 Biodiversity
The SA GAB springs are a critical source of freshwater in an otherwise arid landscape for a diverse biota including invertebrates, birds, mammals, fishes and a wide range of unique wetland vegetation. Springs also support a large number of species representing short-range endemics, with some restricted to single spring groups (Murphy et al., 2015a; Guzik et al., 2019). A preliminary checklist of species endemic to the GAB springs Australia-wide has shown a large number of putatively undescribed species exist in springs (Rossini et al., 2018), but the South Australian fauna and flora (both endemic and non-endemic) remain to be systematically collated and standardized. Here, we outline the current body of knowledge regarding SA GAB taxa (summarized in Figure 4) including their conservation and taxonomic status. A more detailed description of taxonomic groups mentioned here are provided in the Supplementary Material.
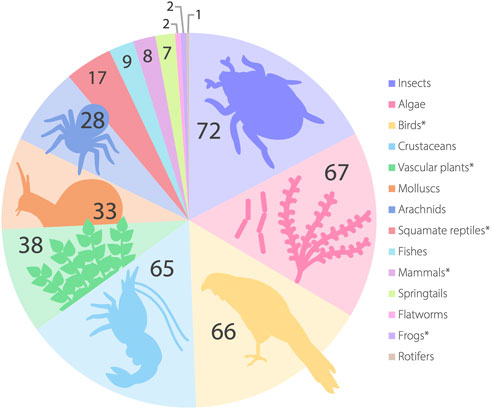
FIGURE 4. Broad taxonomic groups known to occur in the SA GAB springs. Taxon numbers are listed for each group; divergent genetic lineages or morphological forms explicitly stated as putatively undescribed species in the literature are included as separate taxa following Rossini et al. (2018). A full summary of the taxa detailed here is supplied in the electronic supplementary material. Here, algae refer to a paraphyletic grouping including both micro-algae and filamentous algae (see text). *Group is not known to contain representatives endemic to SA GAB springs.
South Australian GAB-fed springs can be viewed as “museums of biodiversity, preserving lineages that would have otherwise gone extinct” (Murphy et al., 2015a). Research over the last 15 years has demonstrated that fauna endemic to the springs have undergone millions of years of evolution in almost complete isolation, reflected in an extraordinary genetic diversity and degree of local endemism, adaptation, and mutational change (Murphy et al., 2009, 2012, 2013, 2015a; Guzik et al., 2012, 2019; DeBoo et al., 2019). Due to the extent of this endemism, it is also likely that in the event of local extinction, particular sites might represent complete species extinctions in certain cases. There are currently nine described endemic animal taxa of formal conservation concern in the SA GAB. Three fish, the Dalhousie goby (Chlamydogobius gloveri), hardyhead (Craterocephalus dalhousiensis), and gudgeon (Mogurnda thermophila) are critically endangered and restricted to the Dalhousie spring supergroup/complex (Hammer et al., 2019; Unmack et al., 2019; Whiterod et al., 2019). Six snail taxa in the genera Trochidrobia and Fonscochlea, which may represent many additional species based on molecular data (Ponder et al., 1995; Rossini et al., 2018), are either endangered or vulnerable (Ponder, 1996a, 1996b, 1996c; Mollusc Specialist Group, 1996; Ponder et al., 1996; Clark, 2011). However, no insect or crustacean in the SA GAB, the system’s two most speciose groups containing endemics, has been evaluated on a per-taxon basis to determine if it is at risk of decline, either at an Australian federal legislative or global level (e.g. the EPBC Act or The International Union for Conservation of Nature’s Red List of Threatened Species). It is likely that many other species associated with GAB springs are also at risk of decline in SA but have not been formally investigated in this context.
In addition to the described species presently recognised as endemic to the SA GAB springs, a significant number of undescribed species have been identified from molecular studies (Murphy et al., 2009; 2015b; Guzik et al., 2012; Guzik and Murphy, 2013) However, three major limiting factors impede our ability to quickly and effectively describe species from GAB springs. Firstly, the rigors of formal taxonomic work and a global lack of taxonomic expertise mean the description of species often occurs decades after their discovery. For example, 32 putative species from SA GAB springs need formal taxonomic descriptions at the time of writing; almost all are divergent lineages of isopods and molluscs discovered using molecular data (Rossini et al., 2018) though this list also includes beetles (DeBoo et al., 2019), sedges (Department of Climate Change, Energy, the Environment and Water, 2022a), and microalgae (Ling et al., 1989). All but two of these taxa are not known to occur outside of SA GAB springs. Secondly, a large number of putatively undescribed endemics are morphologically cryptic and can only be discriminated using robust DNA sequence data rather than morphological characters (Fišer et al., 2018), as demonstrated by the Wangiannachiltonia guzikae King, 2009 cryptic species complex (Murphy et al., 2015b). Finally, microbial biodiversity of springs is likely to be high but remains poorly characterized for the SA GAB (Love et al., 1993; Andrews and Patel, 1996; Byers et al., 1998; Kimura et al., 2005; Ogg and Patel, 2009).
Understandings of determinants of GAB spring species distributions and their biogeography are limited. A publicly available database cataloging environmental attributes of GAB springs only exists for such habitats in Queensland (http://data.qld.gov.au/dataset/springs). The lack of a system-wide dataset currently represents a major impediment to our knowledge of these ecosystems. Broad environmental factors (e.g., spring vent morphology, mean annual rainfall) have been explored as determinants of species distributions of GAB springs in the past (Rossini et al., 2018), but no strong relationship was found when examining supergroups across the GAB as a whole. At the smaller scale of spring complexes, distributions of endemic snails are correlated with sections of wetlands they occupy (Rossini et al., 2017), and wetland size is associated with invertebrate taxonomic richness (Negus and Blessing, 2022).
4 Cultural significance
There are two groups of peoples indigenous to Australia: Torres Strait Islanders from the Torres Strait Islands north of Cape York in Queensland and Aboriginal peoples from all other parts of Australia. Collectively, these groups may be referred to as Aboriginal and Torres Strait Islander peoples. The alternate term Indigenous peoples is sometimes discouraged in an Australian context as it is seen as generalizing the two groups (Australian Institute of Aboriginal and Torres Strait Islander Studies, 2020), though it may be used as a broader term to refer to First Nations peoples worldwide (e.g., Bennett, 2022). Traditional Custodians refer to descendants of the group of Aboriginal and Torres Strait Islander peoples that occupied a region prior to European colonization. Country is a mass noun used by Aboriginal and Torres Strait Islander peoples to describe their traditional land and seas and their relationship with them. Dreaming(s) refer to unique stories and beliefs, such as world-creation narratives or personal totemic symbols, held by different Aboriginal and Torres Strait Islander groups. All of the above terms are capitalized by convention. Here, we will use Aboriginal and Torres Strait Islander peoples when referring to First Nations peoples of all of Australia, Aboriginal peoples when referring to First Nations peoples to the exclusion of the Torres Strait, and Indigenous when referring to First Nations peoples in a global context. Where possible, in this review we will use Aboriginal names of springs, as contributed by Arabana, Kuyani, Southern Aranda, Wangkangurru, and Yarluyandi people throughout South Australia (Hercus and Sutton, 1985), alongside English names.
The Arabana people are the Traditional Custodians of much of the area containing the SA GAB springs and, alongside the South Australian Government Department for Environment and Water, co-manage almost 69,000 km2 of land spanning from Maree in the southeast, Oodnadatta in the west, Kati Thanda–Lake Eyre (including the Wabma Kadarbu Mound Springs Conservation Park) and the boundary of the Macumba River in the north (Nursey-Bray and Arabana Aboriginal Corporation, 2015b). A Native Title Determination Application was filed by the Arabana people in 1998 and they were officially recognised as Traditional Custodians of their Country at Finniss Springs in 2012. Here, we will focus on the Arabana people as the Traditional Custodians of the bulk of the SA GAB springs but acknowledge the Dieri people are native title holders of Country encompassing a portion of the Maree spring complex (National Native Title Tribunal, 2022) and that the SA GAB springs are also an important water resource to the Antakarinja, Dhirari, Karangura, Kokatha, Kuyani, Lower Southern Arrernte, Ngamini, Pirlatapa, Southern Aranda, Thirrari, Wangkangurru, and Yandruwandha peoples (Hercus and Sutton, 1985; Brake et al., 2020). Certain Aboriginal groups not holding native title should not be interpreted as that group lacking strong cultural connections to GAB springs, nor the Country encompassing them.
The Arabana people have deep and ongoing cultural connections to the SA GAB springs. Springs are the only permanent source of freshwater for thousands of kilometers in the arid South Australian interior and the Arabana have relied on them for water, food, and other resources, such as reeds to weave baskets and nets, for generations (National Parks South Australia, 2017). The springs, which Arabana people describe as “like a living pulse that provides life for everything”, are also a central theme in Dreaming stories that have been exchanged for thousands of years to facilitate trade and extend cultural ties (National Parks South Australia, 2017). Individual spring groups usually have their own names and unique Dreaming stories (Hercus and Sutton, 1985; Nursey-Bray and Arabana Aboriginal Corporation, 2015b). Springs are visited and used for cultural activities such as knowledge sharing, which includes the expression of collective memory and the transmission of culturally sensitive or privileged knowledge (Nursey-Bray and Arabana Aboriginal Corporation, 2015b). Western archaeological evidence suggests widespread use of the SA GAB springs as places central to social and ancestral identity by Arabana people began upwards of 5,000 years before present. Prior to European colonization, the Arabana people managed threats to the SA GAB springs with burning and excavation practices to ensure access to water (Davies, 2005; Lewis and Packer, 2020). This management continued until the mid-19th century, when many springs were appropriated by pastoralists and for the laying of railway lines (Hughes and Lampert, 1985; Harris, 1992; Florek, 1993). Today, this degradation has continued through the removal of water from aquifers for mining and pastoral purposes by the sinking of bores, decreasing the pressure of emergent water from vents and therefore the volume of water in springs (known as drawdown). Drawdown is a key concern for the Arabana with respect to the conservation of SA GAB springs (Nursey-Bray et al., 2020) and one of the major threats to the system we identify in this review.
5 Threats to SA GAB springs
5.1 Water use by industry
Contemporary use of SA GAB springs is not as intensive as in the past with respect to the volume of extracted water, but it is still a major threat to springs due to pastoral, agricultural, and mining practices. Intensive extraction of water via bores by pastoralists for livestock and irrigation represents a significant source of drawdown for the SA GAB springs. At present, the volume of stock-accessible water across the GAB is not formally metered and generally estimated based on regional characteristics such as stocking rates. In South Australia, approximately 10 megaliters (ML) of water per annum is allocated to stock access (Frontier Economics, 2016), but due to a lack of regulation the real volume may be much higher than estimated and/or reported. Notably, a significant proportion of water extracted for pastoralism has historically been wasted: in the mid-1990s, up to 80% or more was left to evaporate, in part due to the uncontrolled flow of high-yield bores (Hillier, 1996); many on pastoral land have now been capped, substantially curtailing water wastage. Water is also extracted from the GAB for agriculture and irrigation. In South Australia, approximately 115 ML of water is thought to be extracted from the GAB for irrigation, which far exceeds the license volumes of up to ∼76 ML/year in other states (Frontier Economics, 2016).
The largest known user of water extracted from the GAB in South Australia is the mining industry, representing approximately 24,200 ML a year (Frontier Economics, 2016). Compared to pastoral impacts, mining-associated water extraction in the southern GAB, whilst substantial, is currently strictly licensed with allocations to individual mining companies. However, these allocations do not necessarily account for possible future expansions of mines and still have the potential to substantially reduce spring flow and pressure in local aquifers, especially around extraction wellfields. For example, the Olympic Dam mine managed by BHP is the largest mine in the state and, accordingly, the largest single user of SA GAB water (Frontier Economics, 2016). In springs outside of the company’s two primary borefields, BHP predicted spring flow declines of up to 20% due to drawdown and observed actual declines of up to 10% between 2016 and 2018 (BHP, 2018). While increases in flow rate in those same springs have occurred since (BHP, 2021), it is also important to note that pastoral bores tend to be spread diffusely across the landscape and are of low water yield.
A relatively new industry to South Australia is the extraction of coal seam gas (CSG) (Cosens, 2015). CSG is currently much more prevalent in other Australian states and territories and uses large amounts of groundwater for the life of such projects; as of 2016 in Queensland, for instance, annual estimated GAB water use for CSG was almost double that of irrigation and agriculture (de Rijke et al., 2016; Frontier Economics, 2016). Use of GAB water in South Australia by such operations may increase in coming years to facilitate the extraction of natural gas from the underlying geological Cooper Basin (Menpes and Hill, 2012). Whilst there is an extraction component to CSG processes which may impact flow rates of SA GAB springs, an additional issue is the reinjection of water into GAB aquifers via surface bores (Moran and Vink, 2010). Re-injection aims to replace water extracted for CSG, thereby mitigating aquifer drawdown and avoiding decreases in artesian pressure. However, it is unknown whether re-injected water would reach discharge points at spring vents and if so, how this might impact GAB spring faunal communities. More robust field data related to the hydrological processes of GAB springs, as well as long-term monitoring programs of fauna and flora within these habitats, is needed to better understand potential cumulative impacts on groundwater resources by CSG developments (Williams et al., 2012). The extraction of CSG has been identified as an “emerging challenge” for GAB preservation nationwide (Great Artesian Basin Coordinating Committee, 2019).
5.2 Invasive or overabundant species
Overabundant native and introduced species form a significant threat to the SA GAB springs. This is chiefly due to the fact that most springs fall outside of areas that are protected from stock and pest animals, e.g., national/conservation parks (in which grazing is prevented), fenced areas on pastoral lease land, or areas that have been de-stocked (Rossini et al., 2018; Harris, 2020; Lewis and Harris, 2020). Stock and large-bodied feral animals including cattle, donkeys, goats, pigs, and camels can graze on or trample tail vegetation and disturb spring substrate, causing clogging of spring vents and impacting natural spring flows. Large vertebrates are also known to impact water quality in arid zone freshwater bodies more directly through the input of dung, which can lead to water fouling and negatively impact macroinvertebrate communities (Brim-Box et al., 2010; McBurnie et al., 2015). The above impacts may have already led to local extinctions of spring fauna and flora such as fish (Kodric-Brown et al., 2007), spiders (Kovac and Mackay, 2009), and salt pipewort (Fatchen and Fatchen, 1993; Davies, 2005) at a number of sites. To reduce the negative effects of trampling on wetland communities, it is possible to fence springs such that stock and other herbivores are excluded (Dobkin et al., 1998; Yates et al., 2000). Depending on the method of fencing used, species diversity in GAB spring wetlands has substantial capacity for recovery following the removal of disturbance pressures from invasives (Lewis, 2001; Gotch et al., 2016; Peck, 2020).
In addition to terrestrial vertebrates, the invasive mosquitofish Gambusia holbrooki is also likely to pose a significant risk to the fauna of the SA GAB springs. The impact of mosquitofish incursion has not been robustly assessed in South Australia, though it is currently known in four spring complexes within the Kati Thanda–Lake Eyre supergroup (Gotch et al., 2016; Rossini et al., 2018). Mosquitofish has also been implicated in the demise of Australia’s most endangered freshwater fish, the red-finned blue-eye, from Queensland GAB springs (Kerezsy, 2015). In that state, the red-finned blue-eye has been translocated to springs without mosquitofish to avoid further extirpations and fencing has been employed to prevent subsequent overland dispersals of mosquitofish after rain (Kerezsy, 2015). Mosquitofish are known to prey on several of the invertebrate groups represented as endemics in the SA GAB springs—for example, hydrobiid snails and crustaceans—and their aggressive behavior towards other fish may negatively impact endemics like the desert goby (Chlamydogobius eremius) in much the same way Queensland species have been affected (Gotch et al., 2016).
Although fencing protects springs from damage caused by stock and other invasives, it is not without its drawbacks. For example, fences can cause more damage to sites than if a wetland was not fenced at all if invasives are unable to escape those areas and become trapped (Negus et al., 2019). The long-term effects of stock removal on wetland vegetation communities within arid regions via fencing are also poorly understood (Lewis and Packer, 2020) and a reduction in grazing pressure can lead to undesirable expansion of plant species such as the native Phragmites reed and the invasive date palm (Fatchen, 2001). These species can cause significant change to spring habitats by outcompeting other flora and limiting available habitat for aquatic species. In extreme cases, Phragmites can effectively suck springs dry by greatly increasing evapotranspiration and/or forming mats of rotting stems that soak up water and risk blocking vents (Fatchen, 2000; Harris and Lewis, 2006; Munro et al., 2009; Witjira National Park Co-management Board et al., 2018). Phragmites overgrowth can also push certain GAB spring fauna to the margin of wetlands, exposing them to increased grazing pressures (Gotch et al., 2016). Whether species such as Phragmites have always played such a large role in SA GAB spring ecosystems is unclear. However, oral histories and archaeological evidence suggest Aboriginal peoples controlled Phragmites growth with traditional burning regimes (Hercus and Sutton, 1985; Boyd, 1990; Boyd, 1994; Davies, 2005). Following European colonization this shift, concurrent with the introduction of invasive grazers and a reduction in water flow due to drawdown, may have exacerbated the impacts of overabundant plant species (Lewis and Packer, 2020). Local managers consider a mixed management scheme of temporary stock grazing valuable in maintaining spring flow and reducing Phragmites populations (Lewis and Packer, 2020).
5.3 Climate change
Under all likely emissions scenarios, the Earth is expected to experience a 3°C increase in mean global surface temperatures by 2,100 compared to pre-industrial levels (Allan et al., 2021; Australian Academy of Science, 2021). Arid Australia is likely to experience more frequent heatwaves, often of longer duration compared to historical trends (Australian Academy of Science, 2021). Annual rainfall will decline while rare, major (1-in-20-year) rainfall events will become more intense, potentially leading to flooding (Australian Academy of Science, 2021). Southern arid zone rainfall patterns are also likely to shift from being predominantly dominated by low-intensity winter events, to higher-intensity events during the summer (Alexander and Arblaster, 2009; Malerba et al., 2022). These changes have high potential to negatively impact the biota of the SA GAB springs. Indeed, some of the most widespread extinctions of local plant and animal populations have been observed in freshwater habitats as a result of extreme climate events (Pörtner et al., 2022).
The climate of arid Australia is generally characterized by long periods of dry conditions lasting months or years, interspersed by unpredictable rainfall events caused by tropical monsoon systems from the north and north-west of the continent (Morton, 2022). In the SA arid zone, rainfall varies greatly and ranges from 125 to 250 mm a year (Ponder, 1995). Unfortunately, research on the impacts of climate change on the Australian arid zone and its freshwater systems is lacking. Based on early predictions, the southern portion of the GAB may experience substantial drying while the northern basin will be subject to higher intensity rainfall and recharge (Cosens, 2015). As the GAB is recharged by rainfall from a wetter climatic zone in the east of the continent, the volume and pressure of emergent water in the SA springs is therefore not necessarily dependent on local rainfall at short timescales (Davis et al., 2013). However, on a long enough time scale decreasing annual rainfall in recharge areas of the GAB could potentially lead to groundwater extraction levels exceeding replenishment, lowering groundwater levels and leading to a reduction in flow rate of SA GAB springs (Welsh et al., 2012). The level of emergent groundwater being decoupled from local rainfall in the short term also does not mean that SA GAB spring biota will be protected from other impacts of climate change, such as flooding or rising surface temperatures.
The response of GAB spring endemic fauna to flooding is complex. Historically, major flooding events of SA GAB springs have occurred approximately once per decade (Ponder, 1986) and resulted in greater temporary connectivity between springs. However, these events can also lead to the extinction of populations (particularly invertebrates) due to springs essentially being flushed clean. Floods have been proposed as a mechanism that encourages gene flow between otherwise isolated GAB spring populations, but evidence for this is mixed (Worthington Wilmer and Wilcox, 2007; Wilmer et al., 2011). Dramatic population crashes have been observed in SA GAB invertebrates post-flood, with populations requiring years to recover (Wilmer et al., 2011). Even so, genetic data from spring invertebrates indicates a resilience to disturbance given the extent of old and new genetic diversity in major phylogeographic groups (Guzik et al., 2012; Murphy et al., 2015a), suggesting a climatic buffering of species over evolutionary time. Fishes fare better in such precarious habitats during flooding events compared to smaller animals like invertebrates (Larson, 1995) and it has been proposed they move between GAB springs during these periods (Kodric-Brown and Brown, 1993) albeit with subsequent population declines (Fairfax et al., 2007). Flooding is responsible for well-documented “boom and bust” cycles for vertebrates in Australia’s arid zone (Kingsford et al., 1999; Roshier et al., 2002), and as such is likely to also impact species with strongholds outside of these regions that use SA GAB wetlands to breed, such as waterbirds (Badman, 1985).
Higher local temperatures in Australia’s arid lands have the potential to increase spring evaporative rates and water temperatures to the detriment of spring-dependent ecosystems. Increases in evapotranspiration stemming from higher ground temperatures, for instance, may negatively impact springs (particularly those with low flows) and the taxa they support by increasing salinity or drying wetlands entirely (Ponder, 1986; Gotch et al., 2016). The impact of changing temperatures on spring endemics and their life cycles is poorly known and will depend on baseline temperatures in specific spring groups. For most GAB springs, water temperatures are generally stable close to spring vents (Ponder, 1986). Away from the vent, spring water temperatures approach ambient temperatures and can vary within a 24-h period and across seasons and geographic locations (Smith, 1989; Keppel et al., 2011, 2012; Rossini et al., 2017). In South Australia’s warmest springs, at the Dalhousie supergroup/complex, temperatures can exceed 40°C due to warmer groundwater from the Pedirka Basin partially contributing to spring flows (Fensham et al., 2010; Wolaver et al., 2013; Gotch et al., 2016). Hence, a more immediate threat to the high temperature-dependent fauna at Dalhousie is in fact a reduction in water temperature posed by drawdown from the Pedirka Basin through coal or mining developments (Gotch et al., 2016). Taxa from the comparatively milder Lake Eyre and Lake Frome supergroups (≤30°C) may be more negatively affected by the warming of spring water via increased ambient temperatures (Ponder, 1986; Keppel et al., 2012).
5.4 Tourist and visitor activities
Inappropriate visitor activity has the potential to negatively impact the GAB springs, especially at sites endorsed as tourist destinations. Three key protected areas overlap with springs in South Australia: Witjira National Park (the entire Dalhousie supergroup/complex), Wabma Kadarbu Mound Springs Conservation Park (a subset of the Coward complex, Kati Thanda–Lake Eyre supergroup), and Kati Thanda–Lake Eyre National Park (a subset of the Lake Eyre and Lake Eyre South complexes, Kati Thanda–Lake Eyre supergroup). Of these three groups, only springs in the Kati Thanda–Lake Eyre National Park are not tourist destinations.
Swimming is permitted at the Main Pool spring of Dalhousie in the Witjira National Park, which has led to the erosion of substrate at spring banks (Noack, 2003) and caused concern for Aboriginal peoples and conservationists (Ah Chee, 2002; Harris, 2020). Long-term impacts of these practices on GAB spring chemistry or the disturbance of spring sediment remains unknown. Tourist activities are supported by a campground at Dalhousie, which is thought to have released potentially contaminated runoff into the springs and impacted resident populations of spike-rush plants (Noack, 2003). The National Park’s management plan does not acknowledge these threats beyond noting that “swimming is [generally] not compatible with maintaining healthy mound springs'' and cites the Main Pool as an exception due to its size and flow (Witjira National Park Co-management Board, 2022).
Further south in the Wabma Kadarbu Mound Springs Conservation Park, the above activities are forbidden, and the Park is restricted to day-visitors. Nonetheless, springs such as Pirdali-nha (The Bubbler) and Thirrka/Thirka (Blanche Cup) have boardwalks installed to enable tourists to view active springs at a distance. Visitors have the capacity to come into contact with spring water, compact soil adjacent to springs as a result of foot traffic and degrade ecologically significant wetland tails. These risks are explicitly listed as a threat to the Conservation Park’s protection in its management plan (National Parks South Australia, 2017) and World Heritage assessment (Morton et al., 1995). Overall, such behavior from visitors—either deliberate or accidental—has the potential not only to degrade springs from an ecological standpoint, but compromise sites of immense cultural significance.
6 Discussion
Here, we have used the South Australian GAB springs as a case study to illustrate the immense biodiversity and cultural value of artesian springs in arid and semi-arid Australia. The threatening processes detailed here pose similar risks to the remainder of GAB springs across Queensland and New South Wales. The well-studied nature of the South Australian GAB wetlands provides valuable lessons applicable to GAB springs nationwide and may inform their future study and custodianship. What might become of these habitats if the above threats eventuate? In light of the current state of SA GAB spring management, below we discuss potential, and in some cases already realized, impacts of these threatening processes and propose recommendations to aid the ongoing monitoring and conservation of ecological communities within GAB springs.
6.1 Impact of threats on GAB springs
6.1.1 Risk of extinction of endemic taxa
Arguably the most pressing environmental and conservation impact of the threats above is the risk of extinction of GAB spring endemic species. Most at risk are the endemic invertebrates, which have some of the smallest distributions of GAB spring taxa yet are not well-monitored or managed, and for which a systematic data deficiency exists (Rossini, 2020). This deficiency is of particular concern due to the slow pace of invertebrate species discovery, the overlooking of invertebrates in conservation legislation, and the fact that preliminary data suggest invertebrates are proxies for GAB spring health (Lydeard et al., 2004; Cardoso et al., 2011; Eisenhauer et al., 2019). All of these factors have the strong potential to lead to invertebrate species going extinct before they can be described and adequately conserved. Indeed, in the SA GAB springs a localized extinction, if not a probable species extinction, has already occurred concerning the isopod Phreatomerus latipes from the Hergott spring bore in Maree (Figure 5). Recent collections by the authors failed to find P. latipes at its type locality and revealed the original bore it was described from had been capped. A subsequent molecular analysis of almost 500 specimens from additional locations indicated the existence of up to 10 evolutionarily distinct lineages representing putative species, all isolated within separate spring groups (Guzik et al., 2012). Thus, the population from which type specimens of this species were collected may represent a separate species that has since become extinct. Given the geographic isolation and exceptional levels of phylogeographic structure observed in P. latipes (Guzik et al., 2012), it is unlikely that the “original” species exists elsewhere and has little chance of recolonisation in its type locality, irrespective of reinvigorated water flow.
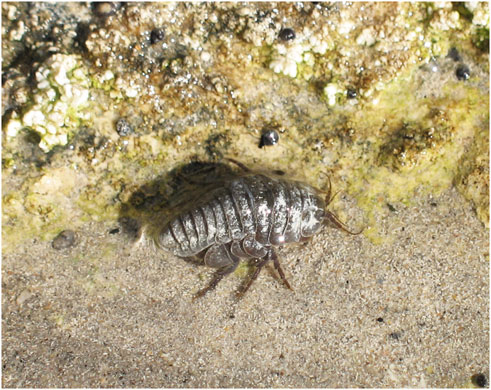
FIGURE 5. The isopod Phreatomerus latipes, a species endemic to the SA GAB springs that might represent a localized, if not species-wide, extinction as a result of the degradation of springs via threats detailed in this review. Photo credit Rachael King.
Although invertebrates are perhaps at the highest risk of extinction in GAB wetlands, processes which threaten these ecosystems do not occur in a vacuum and act at the whole-community level. Some degree of loss of local populations might be recoverable over time via migration and re-colonisation (Fairfax et al., 2007; Kodric-Brown et al., 2007), but new populations of colonizers, such as fish (Fatchen and Fatchen, 1993), have not been observed to survive long-term and instead losses are likely to represent permanent extinctions of evolutionarily distinct species with little to no chance of recovery to their original state. The probability of local extinctions is inversely proportional to spring size, and can also be driven by major changes in habitat (e.g., large increases in the abundance or area of Phragmites), decreases in the area and volume of open water, and increases in evaporation, all factors influenced by the threats we have discussed above (Kodric-Brown and Brown, 1993; Kodric-Brown et al., 2007).
6.1.2 Cultural ramifications of spring water loss
The possibility of spring extinction or loss has the potential to have deeply negative impacts on Traditional Custodians. For the Arabana people in South Australia, water is embedded in identity. Water is not distinguished as belonging to different ecosystems, per Western scientific classifications, but instead understood by the Arabana as a single, unified resource (Nursey-Bray and Arabana Aboriginal Corporation, 2015a); any loss of GAB water may therefore be culturally catastrophic. The Arabana have expressed a great loss in response to spring flow rate decreasing in SA GAB springs, with particular concern about current mining practices. A respondent from Maree described this decrease as “terrible, really sad to go out there now in terms of water. It is not only The Bubbler [...] but [the] other one at Finniss there - it is very dry but used to run.” (Nursey-Bray et al., 2020). Further loss of SA GAB springs would lead to an erosion of cultural identity for the Arabana, the destruction of key foci of dreaming stories, and ultimately a potentially permanent disconnection from traditional lands: “People can not live in or go back to dead Country.” (Nursey-Bray and Arabana Aboriginal Corporation, 2015b). The negative impacts of this loss will not be restricted to native title holders such as the Arabana and Dieri, but affect all Traditional Custodians with connections to Country encompassing GAB springs. While less published information is available regarding the Aboriginal cultural significance of GAB springs in Queensland and New South Wales, Western archaeological evidence suggests Aboriginal peoples in those states have, like the Arabana, relied upon these water sources for thousands of years (Robins, 1995; Noble et al., 1998; Powell and Powell, 2012; Powell et al., 2015).
6.2 A roadmap ahead for GAB spring-dependent ecosystems
6.2.1 Enhanced legal protections for GAB spring biota
Since 2001, the biological community of native species dependent on GAB springs has been recognised as endangered under the EPBC Act. While we note the Act does not concern all GAB springs Australia-wide (Habermehl, 2020a), a corresponding recovery plan has been published with the aim of enhancing groundwater supplies to spring wetlands, maintaining or increasing habitat area and health, and increasing populations of endemic organisms (Fensham et al., 2010). Nonetheless, the listing of these communities as a whole (as opposed to on a per-species basis) has been criticized as offering insufficient protection of endemic species, particularly the invertebrates (Rossini, 2020). Listing of species enables a concentrated direction of conservation and policy efforts, without which they may go extinct (Stuart et al., 2010). We agree with the assessment of Rossini (2020) that these species deserve individual EPBC assessments, and argue this issue is symptomatic of a broader trend that sees invertebrates overlooked in conservation legislation and threatened species lists.
Species formally considered at risk of extinction, such as via the List of Threatened Fauna in Australia’s EPBC Act and the IUCN Red List, are overwhelmingly vertebrates (Eisenhauer et al., 2019; Department of Climate Change, Energy, the Environment and Water, 2022a). In contrast, many invertebrate groups contain a large amount of undescribed genetic and taxonomic diversity but are not often listed as species of conservation concern. In part, this is likely due to a general lack of data, funding, knowledge, and support for experienced invertebrate taxonomists capable of intergenerational knowledge transfer (Cardoso et al., 2011). In Australia, legal structural issues have reinforced this bias. The EPBC Act replicates the IUCN’s criteria to assess species as being at risk with two exceptions: 1) the Act does not allow species to be listed as Data Deficient, ignoring that for invertebrates this tends to be the norm, and 2) species cannot be listed as vulnerable on the basis of short range endemism alone, despite the fact that short range endemics are more likely than not to be invertebrates (Harvey, 2002; Braby, 2018; Taylor et al., 2018). Progress towards equitable listings of GAB spring invertebrate species should begin with the assessment of taxa on a case-by-case basis where possible, but will also require a review of the way Australian conservation legislation is currently designed (Pointon and Rossini, 2020). A better understanding of species diversity and distributions will also significantly contribute to this undertaking, detailed below.
6.2.2 Taxonomic funding and capacity
Around 70% of Australian and New Zealand species are thought to yet be discovered, formally described, or documented (Taxonomy Decadal Plan Working Group, 2018). Without formal acknowledgement and description of species via this taxonomic process, the undescribed majority is essentially invisible—i.e., not included or acknowledged by stakeholders outside the research sphere, especially under existing legislation—and this has serious implications for their conservation. Tackling such an impediment is imperative if we are to gain a greater understanding of current environmental crises and apply sound scientific evidence to industry, conservation, and management practices moving forward. For every dollar invested, a thirty-five-fold return has been estimated if all remaining Australian species were discovered, potentially representing tens of billions of dollars in economic benefits (Deloitte Access Economics, 2020). Such an undertaking, as proposed by the Taxonomy Australia initiative (taxonomyaustralia.org.au), could bring value to sectors including biosecurity, drug development, industry, and society more broadly by stimulating connections to nature (Taxonomy Decadal Plan Working Group, 2018). Even outside the conservation sphere, there is a clear incentive to invigorate taxonomic research in Australia and this would hugely benefit the protection and management of GAB springs nationwide. A comprehensive understanding of the biodiversity and ecology of the GAB springs will not be possible without rigorous and rapid taxonomic research underpinned by strong species delimitation methods. To assist in breaching this gap, here we make recommendations for the use of genetic data in distinguishing cryptic species and the translation of those data into taxonomic descriptions.
Virtually all ecological monitoring studies require species-level identifications. However, these are almost impossible for some spring taxa due to the taxonomic impediment mentioned previously earlier. This barrier has the potential to impact environmental impact assessment, monitoring, and legislative protections at all levels due to 1) a paucity of formal taxonomic descriptions for most faunal groups; 2) morphological homogeneity amongst cryptic species; 3) low quality, or absent, identification keys and 4) few accurate databases of occurrence records. Genetic data can resolve these roadblocks and in doing so, illuminate population genetic structure, distinct phylogeographic lineages, putative cryptic species, and as-yet unknown biodiversity within springs. Much foundational genetic data already exists for GAB-dependent ecosystems (e.g., Murphy et al., 2009; Murphy et al., 2012, 2013, 2015a; Guzik et al., 2012, 2019; DeBoo et al., 2019) but is yet to be implemented at the level of species descriptions or bioassessment protocols (Brake et al., 2019).
Existing genetic surveys of endemic macro-invertebrate spring fauna have demonstrated evidence of at least 42 evolutionarily significant units (ESUs) in SA GAB springs (Murphy et al., 2009; 2015b; Guzik et al., 2012; Guzik and Murphy, 2013). ESUs represent genetic diversity equivalent to species for conservation purposes, and in many cases, they are readily distinguishable from one another using morphological characters. In order to translate these groupings into taxonomic descriptions, adoption of an abbreviated or faster taxonomy is required (Riedel et al., 2013). In recent studies (Murphy et al., 2015b; King et al., 2022), robust examples of a total evidence approach to species description from genetic lineages, especially in situations of cryptic species complexes, have been provided. Recommendations in such studies have included: standard generic descriptions to establish a strong taxonomic framework; use of integrative approaches (combining DNA, morphological characters, and locality data) to describe species; and Open Access publication practices for efficient dissemination. Macro-invertebrate taxa that require taxonomic attention based on current evidence include, but are certainly not limited to, chiltoniid amphipods, Phreatomerus isopods, Trochidrobia snails, Ngarawa ostracods, Fonscochlea snails, and hydraenid beetles.
For cryptic species that are not morphologically distinguishable from others, a multi-tiered approach of genetic data can be implemented for taxonomic diagnosis. This may include the use of genomic data for species-level delineation (e.g., whole mitochondrial genomes, genome skimming approaches), and population genetic and phylogeographic analyses to differentiate fauna from different geographic locations (e.g., the use of single nucleotide polymorphisms). Other approaches—such as metabarcoding, which we detail in Section 6.2.3—may be required to characterize GAB spring fauna more holistically, e.g., in the case of the minimally-known meio- and micro-faunal communities (Love et al., 1993; Andrews and Patel, 1996; Byers et al., 1998; Kimura et al., 2005; Ogg and Patel, 2009). In addition to an increased use of genetic data for species discovery, we also recommend that biodiversity assessment becomes a proactive, government-funded priority at the state and federal level in Australia. A comprehensive biodiversity collecting framework would permit planned collections, curation of specimens in state institutions, and a long-term biobank record of endemic fauna from a range of broad geographic areas. Current practices couple biodiversity assessment with land-use applications and their environmental assessments. This approach unfortunately often leads to discrete and limited sampling as well as specimens and data that can take years to be appropriately housed within museums and similar institutions, potentially limiting their value for future molecular research. Biodiversity assessment and monitoring practices can also be assisted by next-generation DNA sequencing methods, which can feed back into the species discovery process.
6.2.3 Improving biodiversity monitoring methods
The emergence of next-generation DNA sequencing (hereafter NGS) platforms over the last decade has resulted in the capacity to produce large amounts of DNA sequence data in rapid timeframes. Metabarcoding is one method reliant on NGS that can be applied to ecological questions of organismal detection and involves the simultaneous identification of multiple species from a single complex sample, whether the sample contains entire organisms or fragments of DNA (Taberlet et al., 2012). Metabarcoding can be applied at a relatively low cost in a wide range of contexts, ranging from the detection of microbiota in gut samples to that of higher organisms which have “shed” DNA (also known as environmental DNA, or eDNA) into their environment, e.g., in water, leaf litter, and soil samples. eDNA metabarcoding methods have clear applications for biomonitoring, in which the resulting DNA sequence data can be translated into ecological inventories of known and putative species (Brandt et al., 2021; Mousavi-Derazmahalleh et al., 2021). These approaches also represent a major advancement in the speed and accuracy of the identification and characterisation of ecological communities in diverse and difficult-to-sample environments (Zepeda Mendoza et al., 2015).
Instances in which eDNA metabarcoding has been successfully used in Australian groundwater-dependent ecosystems such as the GAB springs include the calcrete aquifers of Western Australia (Saccò et al., 2020), alluvial aquifers in eastern Australia (Korbel et al., 2017), karst systems on Christmas Island (West et al., 2020), and Beetaloo sub-basin environments in the Northern Territory (Oberprieler et al., 2021). In these habitats, rare and elusive species and their distributions have been examined in a way that has previously been impossible, such as the detection of the rare Pilbara blind cave eel (White et al., 2020) and the olm in Croatia (Vörös, 2017) in deep groundwater. eDNA metabarcoding can also offer invaluable insights into groundwater microbial communities (Smith et al., 2015; Smith et al., 2018) and their potential associations with macro-fauna (Smith et al., 2016). This rapid and cost-effective methodology has the potential to advance the monitoring of GAB spring dependent fauna by providing a complimentary, standardized, and affordable biomonitoring tool in addition to traditional survey-based approaches. eDNA is increasingly recognized as a powerful, but underutilized, tool for biomonitoring of delicate ecosystems (Saccò et al., 2022). We propose that eDNA metabarcoding has the potential to revolutionize the characterisation and monitoring of taxa present in the SA GAB springs by enabling the detection of at-risk species, overcoming several impediments to the taxonomic process and accelerating species discovery efforts in the process.
Accurate detection of species from eDNA amplicon data relies on matching millions of anonymous reads (fragments of sequenced DNA) to a reference collection of verified sequences with known taxonomic identities. For eDNA metabarcoding to be an effective tool for biomonitoring, an accurate barcode reference library is required: a database containing DNA sequences annotated with taxonomic information such that new sequences may be referenced against it to determine the identity of the organism they originated from. For GAB spring taxa, much of existing publically available DNA sequence data corresponds to the mitochondrial cytochrome c oxidase subunit 1 (CO1) mitochondrial gene, limiting the ease of taxonomic identification of eDNA data represented by other genes or loci (i.e., nuclear or other mitochondrial genes, commonly 12S, 16S, or 18S rRNA) or for organisms lacking a mitochondrion such as bacteria (Page et al., 2007; King, 2009; Murphy et al., 2009; Guzik et al., 2012; Murphy et al., 2013; King et al., 2014; Murphy et al., 2015a; Guzik et al., 2019; Stringer et al., 2019). If eDNA metabarcoding were to be implemented as a biomonitoring tool in GAB springs, DNA sequencing for other barcoding genes would be required to establish robust and taxonomically accurate barcode reference libraries (Saccò et al., 2022).
6.2.4 Partnership with Traditional Custodians
In this section we defer to published Indigenous expertise to summarize how Traditional Custodians are currently recognized in GAB spring management practices, frameworks co-developed with Aboriginal peoples to improve this status quo, and ways in which reciprocal information-sharing can be facilitated by the incorporation of traditional knowledge into biodiversity and taxonomic research.
Aboriginal and Torres Strait Islander peoples have been systematically excluded from yielding power in natural resource management decisions in Australia. A key example is the EPBC Act, a core piece of federal environmental legislation containing a framework for the protection of biodiversity and locations of natural and cultural significance nationwide. In a recent independent review, the Act was criticized for consistently devaluing Aboriginal and Torres Strait Islander knowledge and values in its decision-making processes, failing Aboriginal and Torres Strait Islander peoples with respect to protection of their cultural heritage, and creating unbalanced power relations with leaseholders of protected areas (Samuel, 2021). Unfortunately, frameworks designed to conserve the GAB springs have been similarly lacking. In South Australia, for instance, authorisation of water use from the GAB is at ministerial discretion (Aboriginal Heritage Act 1988 (SA) s14 (2)) provided consultation is conducted with the Arabana people, even if the Arabana do not permit such a decision. Any subsequent damage to springs only incurs a maximum penalty of $50,000 AUD, at odds with the cultural and environmental significance of these ecosystems (Parliament of Australia, 2021). The chair of the Arabana Aboriginal Corporation has stated that a discussion has been initiated on a national level regarding how the protection of SA GAB springs from ecological, cultural, and spiritual standpoints might be implemented (Parliament of Australia, 2021).
The Arabana people have developed a proposal in collaboration with non-Aboriginal researchers for the co-development of cultural indicators for water sites in the Kati Thanda–Lake Eyre region, including the SA GAB springs (Nursey-Bray and Arabana Aboriginal Corporation, 2015a). As stressed by the Arabana people and their co-authors, Aboriginal and Torres Strait Islander traditional ecological and cultural knowledge should be recognized as a separate body that is equally powerful, testable, and able to guide conservation practices in concert with existing Eurocentric scientific systems, “link[ing] the two systems like the sleepers on the railway lines that snake across their country” (Nursey-Bray and Arabana Aboriginal Corporation, 2015a; Nursey-Bray et al., 2020). Collaboration with Traditional Custodians should not be limited to the management of the GAB springs. For example, one way in which Traditional Custodians may be acknowledged is by the incorporation of Aboriginal names or words in species epithets by taxonomists. The use of Indigenous names can act as conduits of traditional knowledge, encourage a sense of ownership over the species and epithet itself, and affirm the expertise of Indigenous peoples, a much-needed contrast to the overwhelmingly Eurocentric history of biodiversity and taxonomy research (Veale et al., 2019; Gillman and Wright, 2020; Knapp et al., 2020; Galbreath, 2021; Sterner and Elliott, 2022).
Best practices do not currently exist regarding how taxonomic research may be conducted in partnership with Aboriginal peoples in Australia. However, recommendations have been published regarding the involvement of Indigenous Māori peoples in taxonomic research in Aotearoa/New Zealand (Veale et al., 2019). A key recommendation therein was that Indigenous names should not only be considered in the taxonomic process, but that active discussions with Indigenous peoples should take place to facilitate the selection of species names and reciprocal learning. In the absence of such discussions, epithets may prove insulting to Indigenous peoples if they are poorly researched or constructed (Veale et al., 2019). These recommendations provide a robust starting point for a similar framework in Australia. In addition to the co-development of cultural indicators of health for the GAB springs, the co-designing of species epithets with Traditional Custodians is one way in which Aboriginal and Western bodies of knowledge can exist together and ensure groundwater-dependent communities are adequately cared for into the future.
7 Concluding remarks
Here, we have reviewed current understandings of the significance of South Australian Great Artesian Basin-fed springs, remaining knowledge gaps, and a way forward regarding biodiversity research on the groundwater-dependent ecosystems they support. We have also outlined the cultural significance of these environments to Traditional Custodians of the land, namely the Arabana people, and discussed ways in which Aboriginal knowledge can be affirmed and acknowledged in future studies of these ecosystems. There are clear threatening processes that pose impending–and in some cases, already realized–risks to these habitats. Not only is a holistic characterization of the fauna and flora that rely on GAB springs necessary, but so too are additional protective mechanisms to buffer springs against such processes. Here we have largely concentrated on the former, as GAB spring ecosystems cannot be adequately protected if we do not have a detailed understanding of their biota in the first place.
In our view, key barriers exist to a robust understanding of the ecological communities reliant on GAB-fed springs: 1) spring fauna are not adequately protected under Australian federal environmental legislation and 2) a substantial taxonomic impediment exists to robustly naming and characterizing GAB springs fauna, particularly short-range endemic species. The way in which Australian fauna and flora are listed under legislation needs to be revisited such that short range endemics and data deficient species, the norm for many GAB spring-reliant taxa, are considered. An increase in taxonomic funding and capacity, in line with nationwide programs such as Taxonomy Australia, would enable new species to be described well before extinctions occur. Taxonomic and biodiversity research also represents an opportunity for reciprocal knowledge-sharing with Traditional Custodians.
The GAB springs are hotspots of biodiversity supporting unique ecological communities that have evolved in isolation for millions of years. In many cases, species housed in these vulnerable habitats are not found elsewhere on Earth. If we are to effectively conserve these plants and animals they must first be characterized, and in many cases their existence cannot be confirmed without the use of emerging genetic techniques as detailed here. A robust understanding of the species supported by these “oases of life” will ensure their corresponding ecological communities can be effectively managed and monitored into the future. The GAB springs hold universal biodiversity and cultural value, and the conservation management and characterization of the ecosystems they support should ultimately be a social responsibility shared by all stakeholders.
Author contributions
PBH and MG conceived the study. PBH provided expertise on spring endemic flora and fauna, the cultural significance of springs, and tourism impacts. MG provided expertise on short-range endemic spring taxa more broadly. NM provided expertise regarding industrial water use from the Great Artesian Basin. RK provided expertise regarding spring endemics, with specific focus on the crustaceans, and the cultural significance of springs. BH, SC, and AA provided expertise regarding the impact of climate change on freshwater bodies. All authors edited the manuscript and approved the final draft. The authors declare that they have no competing interests.
Funding
Funding for MG was provided by the Australian Research Council (grant LP190100555) in partnership with Curtin University, The University of Adelaide, BHP Billiton Iron Ore Pty Ltd., Rio Tinto Ltd., Chevron Australia Pty Ltd., Western Australian Museum, South Australian Museum, the Department for Biodiversity, Conservation and Attractions (WA), the Western Australian Biodiversity Science Institute, Department of Water and Environmental Regulation (WA). Funding for PBH was provided by contract research via BHP Billiton Pty Ltd. Funding for BH was provided by an Australian Government Research Training Program (RTP) Scholarship, the Roy and Marjory Edwards Scholarship as administered by Nature Foundation, and the Justin Costelloe Scholarship as administered by the Kati Thanda—Lake Eyre Basin Authority, Department of Agriculture, Water and the Environment.
Conflict of interest
The authors declare that the research was conducted in the absence of any commercial or financial relationships that could be construed as a potential conflict of interest.
Publisher’s note
All claims expressed in this article are solely those of the authors and do not necessarily represent those of their affiliated organizations, or those of the publisher, the editors and the reviewers. Any product that may be evaluated in this article, or claim that may be made by its manufacturer, is not guaranteed or endorsed by the publisher.
Supplementary Material
The Supplementary Material for this article can be found online at: https://www.frontiersin.org/articles/10.3389/fenvs.2022.1021987/full#supplementary-material
References
Ah Chee, D. (2002). Indigenous people’s connection with kwatye (water) in the Great Artesian Basin. Environ. S. Aust. 9, 20–21.
Alexander, L. V., and Arblaster, J. M. (2009). Assessing trends in observed and modelled climate extremes over Australia in relation to future projections. Int. J. Climatol. 29, 417–435. doi:10.1002/joc.1730
Allan, R. P., Cassou, C., Chen, D., Cherchi, A., Connors, L., Doblas-Reyes, F. J., et al. (2021). “IPCC, 2021: Summary for policymakers,” in Climate change 2021: The physical science basis. Contribution of working group I to the sixth assessment report of the intergovernmental panel on climate change (IPCC), 3–32. doi:10.1017/9781009157896.001
Andrews, K. T., and Patel, B. K. (1996). Fervidobacterium gondwanense sp. nov., a new thermophilic anaerobic bacterium isolated from nonvolcanically heated geothermal waters of the Great Artesian Basin of Australia. Int. J. Syst. Bacteriol. 46, 265–269. doi:10.1099/00207713-46-1-265
Australian Academy of Science (2021). The risks to Australia of a 3°C warmer world. Available at: www.science.org.au/warmerworld (Accessed August 11, 2022).
Australian Institute of Aboriginal and Torres Strait Islander Studies (2020). Indigenous Australians: Aboriginal and Torres Strait Islander people. Available at: https://aiatsis.gov.au/explore/indigenous-australians-aboriginal-and-torres-strait-islander-people (Accessed October 25, 2022).
Badman, F. J. (1985). “Birds of the mound springs and bores south and west of Lake Eyre with special reference to the Coward Spring area,” in South Australia’s mound springs (Plympton, South Australia): Nature Conservation Society of South Australia Inc., Hyde Park Press.
Bennett, B. (2022). Australian social work: Proposed guidelines for articles by Aboriginal and Torres Strait Islander authors and about Aboriginal and Torres Strait Islander issues. Aust. Soc. Work 75, 273–279. doi:10.1080/0312407X.2021.2013511
Boyd, W. E. (1990). Quaternary pollen analysis in the arid zone of Australia: Dalhousie Springs, Central Australia. Rev. Palaeobot. Palynology 64, 331–341. doi:10.1016/0034-6667(90)90149-D
Boyd, W. e. (1994). Quaternary pollen analysis in the arid zone of Australia: Further results from Dalhousie springs, central Australia. Aust. Geogr. Stud. 32, 274–280. doi:10.1111/j.1467-8470.1994.tb00676.x
Braby, M. F. (2018). Threatened species conservation of invertebrates in Australia: An overview. Austral Entomol. 57, 173–181. doi:10.1111/aen.12324
Brake, L., Harris, C., Jensen, A., Keppel, M., Lewis, M., and Lewis, S. (2020). GAB adaptive management plan and template. Report prepared for the Australian Government Department of Agriculture, South Australian Department for Environment and Water, Queensland Department of Natural Resources, Mines and Energy, New South Wales Department of Planning, Industry and Environment, Northern Territory Department of Environment.
Brake, L., Harris, C., Jensen, A., Keppel, M., Lewis, M., and Lewis, S. (2019). Great Artesian Basin springs: A plan for the future. Evidence-Based methodologies for managing risks to spring values. Report prepared for the Australian Government Department of Agriculture, South Australian Department for Environment and Water, Queensland Department of Natural Resources, Mines and Energy, New South Wales Department of Planning, Industry and Environment. Northern Territory Department of Environment and Natural Resources.
Brake, L. K. (2020). Development, management and rehabilitation of water bores in the Great Artesian Basin, 1878–2020. Proc. R. Soc. Qld. 126, 153–175.
Brandt, M. I., Pradillon, F., Trouche, B., Henry, N., Liautard-Haag, C., Cambon-Bonavita, M.-A., et al. (2021). Evaluating sediment and water sampling methods for the estimation of deep-sea biodiversity using environmental DNA. Sci. Rep. 11, 7856. doi:10.1038/s41598-021-86396-8
Brim-Box, J., Guest, T., Barker, P., Jambrecina, M., Moran, S., Kulitja, R., et al. (2010). Camel usage and impacts at a permanent spring in central Australia: A case study. Rangel. J. 32, 55–62. doi:10.1071/RJ09042
Byers, H. K., Stackebrandt, E., Hayward, C., and Blackall, L. L. (1998). Molecular investigation of a microbial mat associated with the Great Artesian Basin. FEMS Microbiol. Ecol. 25, 391–403. doi:10.1111/j.1574-6941.1998.tb00491.x
CAPAD (2016). Terrestrial protected areas of South Australia. Available at: https://web.archive.org/web/20170716113120/http://www.environment.gov.au/system/files/pages/761994ab-42cc-4f24-952c-c21221861884/files/capad2016sa.xlsx (Accessed August 10, 2022).
Cardoso, P., Erwin, T. L., Borges, P. A. V., and New, T. R. (2011). The seven impediments in invertebrate conservation and how to overcome them. Biol. Conserv. 144, 2647–2655. doi:10.1016/j.biocon.2011.07.024
Clark, S. (2011). IUCN Red List of Threatened Species: Fonscochlea aquatica. IUCN Red List of Threatened Species. Available at: https://www.iucnredlist.org/en (Accessed August 11, 2022).
Cosens, B. (2015). Application of the adaptive water governance project to the management of the Lake Eyre Basin and its connections to the Great Artesian Basin. Adelaide, Australia: Flinders University and the Goyder Institution. Available at: http://goyderinstitute.org/.
Davies, R. J.-P. (2005). Conservation biology of the nationally endangered mound spring endemic forb, Eriocaulon carsonii (Eriocaulaceae). PhD thesis. Adelaide, South Australia: Flinders University.
Davis, J., Pavlova, A., Thompson, R., and Sunnucks, P. (2013). Evolutionary refugia and ecological refuges: Key concepts for conserving Australian arid zone freshwater biodiversity under climate change. Glob. Chang. Biol. 19, 1970–1984. doi:10.1111/gcb.12203
de Rijke, K., Munro, P., and Zurita, M. de L. M. (2016). The great Artesian Basin: A contested resource environment of subterranean water and coal seam gas in Australia. Soc. Nat. Resour. 29, 696–710. doi:10.1080/08941920.2015.1122133
DeBoo, M. L., Murphy, N. P., Austin, A. D., Watts, C. H. S., and Guzik, M. T. (2019). Arid zone island hopping: The impact of dispersal on endemism in hydraenid beetles (Coleoptera: Hydraenidae) found in isolated desert springs. Austral Entomol. 58, 886–896. doi:10.1111/aen.12426
Deloitte Access Economics (2020). Cost benefit analysis of a mission to discover and document Australia’s species. Prepared for the Australian Academy of Science. Available at: https://www2.deloitte.com/au/en/pages/economics/articles/cost-benefit-analysis-mission-discover-document-australia-species.html (Accessed June 4, 2022).
Department of Climate Change, Energy, the Environment and Water (2022a). The community of native species dependent on natural discharge of groundwater from the Great Artesian Basin. Community and Species Profile and Threats Database. Canberra: Department of Climate Change, Energy, the Environment and Water, Australian Government. Available at: http://www.environment.gov.au/cgi-bin/sprat/public/publicshowcommunity.pl?id=26& status=Endangered (Accessed May 17, 2022).
Dobkin, D. S., Rich, A. C., and Pyle, W. H. (1998). Habitat and avifaunal recovery from livestock grazing in a riparian meadow system of the northwestern Great Basin. Conserv. Biol. 12, 209–221. doi:10.1111/j.1523-1739.1998.96349.x
Eisenhauer, N., Bonn, A., and Guerra, A. C. (2019). Recognizing the quiet extinction of invertebrates. Nat. Commun. 10, 50. doi:10.1038/s41467-018-07916-1
Fairfax, R., Fensham, R., Wager, R., Brooks, S., Webb, A., and Unmack, P. (2007). Recovery of the red-finned blue-eye: An endangered fish from springs of the Great Artesian Basin. Wildl. Res. 34, 156–166. doi:10.1071/WR06086
Fatchen, T. J. (2001). “Competitive exclusions and dominance changeovers on mound springs after stocking,” in Proceedings of the 4th Mound Spring Researchers Forum 2001 (Adelaide: Department of Environment and Heritage), 9–15.
Fatchen, T. J., and Fatchen, D. H. (1993). “Dynamics of vegetation on mound springs in the Hermit Hill region, northern South Australia,” in Report prepared for WMC (Olympic Dam Operations) Pty. Ltd. (Adelaide: TJ Fatchen & Associates).
Fatchen, T. J. (2000). Mound springs management planning: Management issues, strategies, and prescriptions for mound springs in far north South Australia. Report prepared for the South Australian Department of Environment and Heritage with the support of the Natural Heritage Trust.
Fensham, R. J., and Fairfax, R. J. (2003). Spring wetlands of the Great Artesian Basin, Queensland, Australia. Wetl. Ecol. Manag. 11, 343–362. doi:10.1023/B:WETL.0000005532.95598.e4
Fensham, R. J., Laffineur, B., and Collingwood, T. (2019). IUCN Red List of Threatened Species: Eucalyptus camaldulensis. IUCN Red List of Threatened Species. Available at: https://www.iucnredlist.org/en (Accessed August 11, 2022).
Fensham, R. J., and Laffineur, B. (2022). Response of spring wetlands to restored aquifer pressure in the Great Artesian Basin, Australia. J. Hydrology 612, 128152. doi:10.1016/j.jhydrol.2022.128152
Fensham, R. J., Ponder, W. F., and Fairfax, R. J. (2010). Recovery plan for the community of native species dependent on natural discharge of groundwater from the Great Artesian Basin. Report prepared for the Department of the Environment, Water, Heritage and the Arts, Canberra. Queensland Department of Environment and Resource Management, Brisbane.
Fensham, R. J., and Price, R. J. (2004). Ranking spring wetlands in the Great Artesian Basin of Australia using endemicity and isolation of plant species. Biol. Conserv. 119, 41–50. doi:10.1016/j.biocon.2003.10.019
Ficetola, G. F., Canedoli, C., and Stoch, F. (2019). The Racovitzan impediment and the hidden biodiversity of unexplored environments. Conserv. Biol. 33, 214–216. doi:10.1111/cobi.13179
Fišer, C., Robinson, C. T., and Malard, F. (2018). Cryptic species as a window into the paradigm shift of the species concept. Mol. Ecol. 27, 613–635. doi:10.1111/mec.14486
Florek, S. (1993). Archaeology of the mound spring campsites near Lake Eyre in South Australia. PhD thesis. Sydney, Australia: University of NSW.
Frontier Economics (2016). Economic output of groundwater dependent sectors in the Great Artesian Basin: A report commissioned by the Australian Government and Great Artesian Basin jurisdictions based on advice from the Great Artesian Basin Coordinating Committee. Australia: Frontier Economics Pty. Ltd.
Galbreath, R. (2021). Why have so few Māori or Moriori names been used in taxonomic description? N. Z. J. Ecol. 45, 1–3. doi:10.20417/nzjecol.45.16
Gillman, L. N., and Wright, S. D. (2020). Restoring indigenous names in taxonomy. Commun. Biol. 3, 609–613. doi:10.1038/s42003-020-01344-y
Gotch, T., Keppel, M., Fels, K., and McKenzie, J. (2016). Lake Eyre Basin springs assessment: Ecohydrological conceptual models of springs in the Western Lake Eyre basin, south Australia. DEWNR Technical report 2016/02. Adelaide: Government of South Australia, through Department of Environment, Water and Natural Resources.
Great Artesian Basin Coordinating Committee (2019). Great Artesian Basin strategic management plan. Department of Climate Change, Energy, the Environment and Water. Available at: https://www.agriculture.gov.au/sites/default/files/documents/strategic-management-plan.pdf.
Guzik, M. T., Adams, M. A., Murphy, N. P., Cooper, S. J. B., and Austin, A. D. (2012). Desert springs: Deep phylogeographic structure in an ancient endemic crustacean (Phreatomerus latipes). PLOS ONE 7, e37642. doi:10.1371/journal.pone.0037642
Guzik, M. T., and Murphy, N. P. (2013). “Fauna of the GAB springs: Comparative phylogeography of GAB spring invertebrates,” in Allocating water and maintaining springs in the Great Artesian Basin (Canberra: National Water Commission).
Guzik, M. T., Stringer, D. N., Murphy, N. P., Cooper, S. J. B., Taiti, S., King, R. A., et al. (2019). Molecular phylogenetic analysis of Australian arid-zone oniscidean isopods (Crustacea: Haloniscus) reveals strong regional endemicity and new putative species. Invert. Syst. 33, 556–574. doi:10.1071/IS18070
Habermehl, M. A. (2020a). Hydrogeological overview of springs in the Great Artesian Basin. Proc. R. Soc. Qld. 126, 29–46.
Habermehl, M. A. (2020b). Review: The evolving understanding of the Great Artesian Basin (Australia), from discovery to current hydrogeological interpretations. Hydrogeol. J. 28, 13–36. doi:10.1007/s10040-019-02036-6
Habermehl, M. A. (1982). Springs in the Great Artesian Basin, Australia: Their origin and nature. Canberra: Australian Geological Survey Organisation.
Hammer, M., Unmack, P., Whiterod, N., Unni, R. M., Flinders, R., and Gotch, T. (2019). IUCN Red List of Threatened Species: Chlamydogobius gloveri. IUCN Red List of Threatened Species. Available at: https://www.iucnredlist.org/en (Accessed August 11, 2022).
Harris, C. (2020). Five decades of watching mound springs in South Australia. Proc. R. Soc. Qld. 126, 1–11.
Harris, C., and Lewis, S. (2006). “Great Artesian Basin (GAB) springs protection and monitoring program,” in Proceedings of the 6th Mound Spring Researchers Forum, Adelaide. South Australian Arid Lands Natural Resources Management Board.
Harris, C. R. (1992). Mound springs: South Australian conservation initiatives. Rangel. J. 14, 157–173. doi:10.1071/rj9920157
Harvey, M. S. (2002). Short-range endemism amongst the Australian fauna: Some examples from non-marine environments. Invert. Syst. 16, 555–570. doi:10.1071/is02009
Hercus, L., and Sutton, P. (1985). “The assessment of Aboriginal cultural significance of mound springs in South Australia,” in Heritage of the mound springs (South Australia: Department of Environment and Planning).
Hillier, J. (1996). The Great Artesian Basin management of water resources after 100 years of development. Mesoz. Geol. East. Aust. Plate Conf. 43, 251–255.
Hughes, P. J., and Lampert, J. (1985). The assessment of Aboriginal archaeological significance of mound springs in South Australia. Adelaide: Prepared by Kinhill Stearns for the South Australian Department of Planning and Environment.
Keppel, M. N., Clarke, J. D. A., Halihan, T., Love, A. J., and Werner, A. D. (2011). Mound springs in the arid Lake Eyre south region of South Australia: A new depositional tufa model and its controls. Sediment. Geol. 240, 55–70. doi:10.1016/j.sedgeo.2011.08.001
Keppel, M. N., Post, V. E. A., Love, A. J., Clarke, J. D. A., and Werner, A. D. (2012). Influences on the carbonate hydrochemistry of mound spring environments, Lake Eyre South region, South Australia. Chem. Geol. 296–297, 50–65. doi:10.1016/j.chemgeo.2011.12.017
Kerezsy, A. (2015). Development of a technique for quarantining Great Artesian Basin springs from colonisation by the invasive fish Eastern Gambusia (Gambusia holbrooki). Ecol. Manag. Restor. 16, 229–232. doi:10.1111/emr.12174
Kerezsy, A. (2020). Fishes of Australia’s Great Artesian Basin springs – an overview. Proc. R. Soc. Qld. 126, 117–127.
Kimura, H., Sugihara, M., Yamamoto, H., Patel, B. K. C., Kato, K., and Hanada, S. (2005). Microbial community in a geothermal aquifer associated with the subsurface of the Great Artesian Basin, Australia. Extremophiles 9, 407–414. doi:10.1007/s00792-005-0454-3
King, R. (2009). Two new genera and species of chiltoniid amphipods (Crustacea: Amphipoda: Talitroidea) from freshwater mound springs in South Australia. Zootaxa 2293, 35–52. doi:10.11646/zootaxa.2293.1.2
King, R. A., Fagan-Jeffries, E. P., Bradford, T. M., Stringer, D. N., Finston, T. L., Halse, S. A., et al. (2022). Cryptic diversity down under: Defining species in the subterranean amphipod genus Nedsia Barnard & Williams, 1995 (Hadzioidea: Eriopisidae) from the Pilbara, Western Australia. Invertebr. Syst. 36, 113–159. doi:10.1071/IS21041
King, R., Leys, R., King, R. A., and Leys, R. (2014). Molecular evidence for mid-Pleistocene divergence of populations of three freshwater amphipod species (Talitroidea: Chiltoniidae) on Kangaroo Island, South Australia, with a new spring-associated genus and species. Aust. J. Zool. 62, 137–156. doi:10.1071/ZO13099
Kingsford, R. T., Curtin, A. L., and Porter, J. (1999). Water flows on Cooper Creek in arid Australia determine ‘boom’ and ‘bust’ periods for waterbirds. Biol. Conserv. 88, 231–248. doi:10.1016/S0006-3207(98)00098-6
Kinhill Engineers (1997). Olympic Dam expansion project: Environmental impact statement. Prepared for WMC (Olympic Dam Corporation) Pty. Ltd. South Australia: Olympic Dam.
Knapp, S., Vorontsova, M. S., and Turland, N. J. (2020). Indigenous species names in algae, fungi and plants: A comment on Gillman & Wright (2020). TAXON 69, 1409–1410. doi:10.1002/tax.12411
Kodric-Brown, A., and Brown, J. H. (1993). Highly structured fish communities in Australian desert springs. Ecology 74, 1847–1855. doi:10.2307/1939942
Kodric-Brown, A., Wilcox, C., Bragg, J. G., and Brown, J. H. (2007). Dynamics of fish in Australian desert springs: Role of large-mammal disturbance. Divers. Distributions 13, 789–798. doi:10.1111/j.1472-4642.2007.00395.x
Korbel, K., Chariton, A., Stephenson, S., Greenfield, P., and Hose, G. C. (2017). Wells provide a distorted view of life in the aquifer: Implications for sampling, monitoring and assessment of groundwater ecosystems. Sci. Rep. 7, 40702. doi:10.1038/srep40702
Kovac, K.-J., and Mackay, D. A. (2009). An experimental study of the impacts of cattle on spider communities of artesian springs in South Australia. J. Insect Conserv. 13, 57–65. doi:10.1007/s10841-007-9117-2
Larson, H. K. (1995). A review of the Australian endemic gobiid fish genus Chlamydogobius, with description of five new species. Beagle Rec. Museums Art Galleries North. Territ. 12, 19–51. doi:10.5962/p.264275
Lewis, M. M., White, D. C., and Gotch, T. (2013). “Allocating water and maintaining springs in the Great Artesian Basin,” in Spatial survey and remote sensing of artesian springs of the Western Great Artesian Basin (Canberra: National Water Commission).
Lewis, S. (2001). “Department for Environment and Heritage mound springs protection program,” in Proceedings of the 4th Mound Spring Researchers Forum (Adelaide: Department of Environment and Heritage), 28–30.
Lewis, S., and Harris, C. (2020). Improving conservation outcomes for Great Artesian Basin springs in South Australia. Proc. R. Soc. Qld. 126, 271–287. doi:10.3316/informit.626794538781042
Lewis, S., and Packer, J. G. (2020). Decadal changes in Phragmites australis performance in Lake Eyre supergroup spring communities following stock exclusion. Proc. R. Soc. Qld. 126, 193–211.
Ling, H. U., Thomas, D. P., and Tyler, P. A. (1989). “Micro-algae,” in Natural History of Dalhousie springs. Special publication, Adelaide, South Australia: South Australian Museum.
Love, C. A., Patel, B. K. C., Nichols, P. D., and Stackebrandt, E. (1993). Desulfotomaculum australicum, sp. nov., a thermophilic sulfate-reducing bacterium isolated from the Great Artesian Basin of Australia. Syst. Appl. Microbiol. 16, 244–251. doi:10.1016/S0723-2020(11)80475-3
Lydeard, C., Cowie, R. H., Ponder, W. F., Bogan, A. E., Bouchet, P., Clark, S. A., et al. (2004). The global decline of nonmarine mollusks. BioScience 54, 321–330. doi:10.1641/0006-3568(2004)054[0321:TGDONM]2.0.CO;2
Malerba, M. E., Wright, N., and Macreadie, P. I. (2022). Australian farm dams are becoming less reliable water sources under climate change. Sci. Total Environ. 829, 154360. doi:10.1016/j.scitotenv.2022.154360
McBurnie, G., Davis, J., Thompson, R. M., Nano, C., and Brim-Box, J. (2015). The impacts of an invasive herbivore (Camelus dromedaries) on arid zone freshwater pools: An experimental investigation of the effects of dung on macroinvertebrate colonisation. J. Arid Environ. 113, 69–76. doi:10.1016/j.jaridenv.2014.09.011
McConvell, P., and Thieberger, N. (2001). State of Indigenous languages in Australia - 2001. Australia State of the Environment Second Technical Paper Series (Natural and Cultural Heritage). Canberra: Department of the Environment and Heritage.
Menpes, S., and Hill, A. (2012). Emerging continuous gas plays in the Cooper Basin, South Australia. APPEA J. 52, 671. doi:10.1071/AJ11085
Mollusc Specialist Group (1996). IUCN Red List of Threatened Species: Fonscochlea conica. IUCN Red List of Threatened Species. Available at: https://www.iucnredlist.org/en (Accessed August 11, 2022).
Moran, C., and Vink, S. (2010). Assessment of impacts of the proposed coal seam gas operations on surface and groundwater systems in the Murray-Darling Basin. Report prepared by the Centre for Water in the Minerals Industry Sustainable Minerals Institute, The University of Queensland.
Morton, S. (2022). Australian deserts: Ecology and landscapes, CSIRO Publishing, Victoria. Available at: https://www.publish.csiro.au/book/7568 (Accessed August 11, 2022).
Morton, S., Doherty, M. D., and Barker, R. D. (1995). Natural heritage values of the Lake Eyre Basin in South Australia: World heritage assessment. Canberra: Department of the Environment, Sport and Territories.
Mousavi-Derazmahalleh, M., Stott, A., Lines, R., Peverley, G., Nester, G., Simpson, T., et al. (2021). eDNAFlow, an automated, reproducible and scalable workflow for analysis of environmental DNA sequences exploiting Nextflow and Singularity. Mol. Ecol. Resour. 21, 1697–1704. doi:10.1111/1755-0998.13356
Mudd, G. M. (2000). Mound springs of the Great Artesian Basin in South Australia: A case study from Olympic Dam. Environ. Geol. 39, 463–476. doi:10.1007/s002540050452
Munro, N. T., Kovac, K.-J., Niejalke, D., and Cunningham, R. B. (2009). The effect of a single burn event on the aquatic invertebrates in artesian springs. Austral Ecol. 34, 837–847. doi:10.1111/j.1442-9993.2009.01989.x
Murphy, N. P., Adams, M., and Austin, A. D. (2009). Independent colonization and extensive cryptic speciation of freshwater amphipods in the isolated groundwater springs of Australia’s Great Artesian Basin. Mol. Ecol. 18, 109–122. doi:10.1111/j.1365-294X.2008.04007.x
Murphy, N. P., Adams, M., Guzik, M. T., and Austin, A. D. (2013). Extraordinary micro-endemism in Australian desert spring amphipods. Mol. Phylogenet. Evol. 66, 645–653. doi:10.1016/j.ympev.2012.10.013
Murphy, N. P., Breed, M. F., Guzik, M. T., Cooper, S. J. B., and Austin, A. D. (2012). Trapped in desert springs: Phylogeography of Australian desert spring snails. J. Biogeogr. 39, 1573–1582. doi:10.1111/j.1365-2699.2012.02725.x
Murphy, N. P., Guzik, M. T., Cooper, S. J. B., and Austin, A. D. (2015a). Desert spring refugia: Museums of diversity or evolutionary cradles? Zool. Scr. 44, 693–701. doi:10.1111/zsc.12129
Murphy, N. P., King, R. A., Delean, S., Murphy, N. P., King, R. A., and Delean, S. (2015b). Species, ESUs or populations? Delimiting and describing morphologically cryptic diversity in Australian desert spring amphipods. Invert. Syst. 29, 457–467. doi:10.1071/IS14036
National Native Title Tribunal (2022). South Australia: Native Title claimant applications and determination areas as per the Federal Court (30 June 2022), Perth, Australia. Available at: http://www.nntt.gov.au/Maps/SA_NTDA_Schedule.pdf.
National Parks South Australia (2017). Wabma Kadarbu Mound Springs Conservation Park management plan 2017. Department of Environment, Water and Natural Resources, Government of South Australia.
Negus, P., and Blessing, J. (2022). Girt by dirt: Island biogeography and relationships between invertebrate richness and wetted area of spring wetlands. Aquat. Ecol. 56, 299–310. doi:10.1007/s10452-021-09920-y
Negus, P. M., Marshall, J. C., Clifford, S. E., Blessing, J. J., and Steward, A. L. (2019). No sitting on the fence: Protecting wetlands from feral pig damage by exclusion fences requires effective fence maintenance. Wetl. Ecol. Manag. 27, 581–585. doi:10.1007/s11273-019-09670-7
Noack, D. (2003). “Monitoring of Eleocharis geniculata,” in Expedition Witjira: Interim report (Unley, South Australia: Scientific Expedition Group with National Parks and Wildlife and Department of Environment and Heritage).
Noble, J. C., Habermehl, M. A., James, C. D., Landsberg, J., Langston, A. C., and Morton, S. R. (1998). Biodiversity implications of water management in the Great Artesian Basin. Rangel. J. 20, 275–300. doi:10.1071/rj9980275
Nursey-Bray, M., Arabana Aboriginal Corporation (2015a). Cultural indicators, country and culture: The Arabana, change and water. Rangel. J. 37, 555. doi:10.1071/RJ15055
Nursey-Bray, M., Palmer, R., Stuart, A., Arbon, V., and Rigney, L.-I. (2020). Scale, colonisation and adapting to climate change: Insights from the Arabana people, South Australia. Geoforum 114, 138–150. doi:10.1016/j.geoforum.2020.05.021
Nursey-Bray, M.Arabana Aboriginal Corporation (2015b). The Arabana people, water and developing cultural indicators for country. Adelaide, South Australia: Goyder Institute for Water Research.
Oberprieler, S., Rees, G., Nielsen, D., Shackleton, M., Watson, G., Chandler, L., et al. (2021). Connectivity, not short-range endemism, characterises the groundwater biota of a northern Australian karst system. Sci. Total Environ. 796, 148955. doi:10.1016/j.scitotenv.2021.148955
Ogg, C. D., and Patel, B. K. C. Y. (2009). Caloramator australicus sp. nov., a thermophilic, anaerobic bacterium from the Great Artesian Basin of Australia. Int. J. Syst. Evol. Microbiol. 59, 95–101. doi:10.1099/ijs.0.000802-0
Page, T. J., Rintelen, K. V., and Hughes, J. (2007). An island in the stream: Australia’s place in the cosmopolitan world of indo-west pacific freshwater shrimp (Decapoda: Atyidae: Caridina). Mol. phylogenetics Evol. 43, 645–659. doi:10.1016/J.YMPEV.2006.08.007
Parliament of Australia (2021). Joint standing Committee on Northern Australia - destruction of 46,000-year-old caves at the Juukan Gorge (Hansard). Canberra: House of Representatives, Parliament of Australia, October 16, 2021.
Peck, S. (2020). Evaluating the effectiveness of fencing to manage feral animal impacts on high conservation value artesian spring wetland communities of Currawinya National Park. Proc. R. Soc. Qld. 126, 177–191.
Pointon, R. K., and Rossini, R. A. (2020). Legal mechanisms to protect Great Artesian Basin springs: Successes and shortfalls. Proc. R. Soc. Qld. 126, 249–269.
Ponder, W. F., Colgan, D. J., Terzis, T., Clark, S. A., and Miller, A. C. (1996). Three new morphologically and genetically determined species of hydrobiid gastropods from Dalhousie Springs, northern South Australia, with the description of a new genus. Molluscan Res. 17, 49–109. doi:10.1080/13235818.1996.10673675
Ponder, W. F., Eggler, P., and Colgan, D. J. (1995). Genetic differentiation of aquatic snails (Gastropoda: Hydrobiidae) from artesian springs in arid Australia. Biol. J. Linn. Soc. Lond. 56, 553–596. doi:10.1111/j.1095-8312.1995.tb01110.x
Ponder, W. F. (1996a). IUCN Red List of Threatened Species: Fonscochlea accepta. IUCN Red List of Threatened Species. Available at: https://www.iucnredlist.org/en (Accessed August 11, 2022).
Ponder, W. F. (1996b). IUCN Red List of Threatened Species: Fonscochlea billakalina. IUCN Red List of Threatened Species. Available at: https://www.iucnredlist.org/en (Accessed August 11, 2022).
Ponder, W. F. (1996c). IUCN red list of threatened species: Trochidrobia inflata. IUCN red list of threatened species. Available at: https://www.iucnredlist.org/en (Accessed August 11, 2022).
Ponder, W. F. (1996d). IUCN Red List of Threatened Species: Trochidrobia smithi. IUCN Red List of Threatened Species. Available at: https://www.iucnredlist.org/en (Accessed August 11, 2022).
Ponder, W. F. (1995). “Mound spring snails of the Australian Great Artesian Basin,” in The conservation biology of molluscs (Gland, Switzerland: IUCN), 13–18.
Ponder, W. F. (1986). “Mound springs of the Great Artesian Basin,” in Limnology in Australia Editors P. De Deckker, and W. D. Williams (Dordrecht: Springer Netherlands), 403–420. doi:10.1007/978-94-009-4820-4_25
Pörtner, H.-O., Roberts, D. C., Poloczanska, E. S., Mintenbeck, K., Tignor, M., Alegría, A., et al. (2022). Climate change 2022: Impacts, adaptation, and vulnerability. Contribution of working group II to the sixth assessment report of the intergovernmental panel on climate change. Cambridge University Press. In Press.
Powell, O. C., and Powell, O. C. (2012). Song of the artesian water: Aridity, drought and disputation along Queensland’s pastoral frontier in Australia. Rangel. J. 34, 305–317. doi:10.1071/RJ12014
Powell, O., Silcock, J., and Fensham, R. (2015). Oases to oblivion: The rapid demise of springs in the South-eastern Great Artesian Basin, Australia. Groundwater 53, 171–178. doi:10.1111/gwat.12147
Riedel, A., Sagata, K., Suhardjono, Y. R., Tänzler, R., and Balke, M. (2013). Integrative taxonomy on the fast track - towards more sustainability in biodiversity research. Front. Zool. 10, 15. doi:10.1186/1742-9994-10-15
Robins, R. P. (1995). Archaeological investigations at Youlain springs, southwest Queensland. Memoirs Qld. Mus. Cult. 1, 57–74. doi:10.3316/informit.888788023974365
Roshier, D. A., Robertson, A. I., and Kingsford, R. T. (2002). Responses of waterbirds to flooding in an arid region of Australia and implications for conservation. Biol. Conserv. 106, 399–411. doi:10.1016/S0006-3207(01)00268-3
Rossini, R. A., Fensham, R., J. R., and Walter, H. G. (2020). Different species requirements within a heterogeneous spring complex affects patch occupancy of threatened snails in Australian desert springs. Water 12, 2942. doi:10.3390/w12102942
Rossini, R. A. (2020). Current state and reassessment of threatened species status of invertebrates endemic to Great Artesian Basin springs. Proc. R. Soc. Qld. 126, 225–248.
Rossini, R. A., Fensham, R. J., Stewart-Koster, B., Gotch, T., and Kennard, M. J. (2018). Biogeographical patterns of endemic diversity and its conservation in Australia’s artesian desert springs. Divers. Distrib. 24, 1199–1216. doi:10.1111/ddi.12757
Rossini, R. A., Fensham, R. J., and Walter, G. H. (2017). Spatiotemporal variance of environmental conditions in Australian artesian springs affects the distribution and abundance of six endemic snail species. Aquat. Ecol. 51, 511–529. doi:10.1007/s10452-017-9633-4
Saccò, M., Blyth, A. J., Humphreys, W. F., Karasiewicz, S., Meredith, K. T., Laini, A., et al. (2020). Stygofaunal community trends along varied rainfall conditions: Deciphering ecological niche dynamics of a shallow calcrete in Western Australia. Ecohydrology 13, e2150. doi:10.1002/eco.2150
Saccò, M., Guzik, M. T., van der Heyde, M., Nevill, P., Cooper, S. J. B., Austin, A. D., et al. (2022). eDNA in subterranean ecosystems: Applications, technical aspects, and future prospects. Sci. Total Environ. 820, 153223. doi:10.1016/j.scitotenv.2022.153223
Samuel, G. (2021). Independent review of the EPBC Act – final report. Canberra: Department of Agriculture, Water and the Environment.
Smith, P. C. (1989). “Hydrology,” in Natural history of Dalhousie springs (Adelaide, South Australia: Special Publication, South Australian Museum).
Smith, R. J., Paterson, J. S., Launer, E., Tobe, S. S., Morello, E., Leijs, R., et al. (2016). Stygofauna enhance prokaryotic transport in groundwater ecosystems. Sci. Rep. 6, 32738. doi:10.1038/srep32738
Smith, R. J., Paterson, J. S., Sibley, C. A., Hutson, J. L., and Mitchell, J. G. (2015). Putative effect of aquifer recharge on the abundance and taxonomic composition of endemic microbial communities. PLOS ONE 10, e0129004. doi:10.1371/journal.pone.0129004
Smith, R. J., Paterson, J. S., Wallis, I., Launer, E., Banks, E. W., Bresciani, E., et al. (2018). Southern South Australian groundwater microbe diversity. FEMS Microbiol. Ecol. 94, 158. doi:10.1093/femsec/fiy158
Social and Ecological Assessment Pty Ltd (1986). Mound springs heritage survey: Biological assessment of South Australian mound springs. Adelaide, South Australia: Report prepared for the South Australian Department of Environment and Planning.
Sterner, B., and Elliott, S. (2022). How data governance principles influence participation in biodiversity science. Available at: http://philsci-archive.pitt.edu/21039/ (Accessed October 25, 2022).
Stringer, D. N., King, R. A., Taiti, S., Guzik, M. T., Cooper, S. J. B., and Austin, A. D. (2019). Systematics of Haloniscus Chilton, 1920 (Isopoda: Oniscidea: Philosciidae), with description of four new species from threatened Great Artesian Basin springs in South Australia. J. Crustac. Biol. 39, 651–668. doi:10.1093/jcbiol/ruz044
Stuart, S. N., Wilson, E. O., McNeely, J. A., Mittermeier, R. A., and Rodríguez, J. P. (2010). The barometer of life. Science 328, 177. doi:10.1126/science.1188606
Taberlet, P., Coissac, E., Pompanon, F., Brochmann, C., and Willerslev, E. (2012). Towards next-generation biodiversity assessment using DNA metabarcoding. Mol. Ecol. 21, 2045–2050. doi:10.1111/j.1365-294X.2012.05470.x
Taxonomy Decadal Plan Working Group (2018). Discovering biodiversity: A decadal plan for taxonomy and biosystematics in Australia and New Zealand 2018–2027. Canberra and Wellington: Australian Academy of Science and Royal Society Te Apārangi.
Taylor, G. S., Braby, M. F., Moir, M. L., Harvey, M. S., Sands, D. P. A., New, T. R., et al. (2018). Strategic national approach for improving the conservation management of insects and allied invertebrates in Australia. Austral Entomol. 57, 124–149. doi:10.1111/aen.12343
Unmack, P., Whiteroad, N., Hammer, M., Mathwin, R., and Gotch, T. (2019). IUCN Red List of Threatened Species: Mogurnda thermophila. IUCN Red List of Threatened Species. Available at: https://www.iucnredlist.org/en (Accessed August 11, 2022).
Veale, A. J., de Lange, P., Buckley, T. R., Cracknell, M., Hohaia, H., Parry, K., et al. (2019). Using te reo Māori and ta re Moriori in taxonomy. N. Z. J. Ecol. 43, 1–11. doi:10.20417/nzjecol.43.30
Welsh, W. D., Moore, C. R., Turnadge, C. J., Smith, A. J., and Barr, T. M. (2012). “Modelling of climate and development,” in A technical report to the Australian Government from the CSIRO Great Artesian Basin Water Resource Assessment (Australia: CSIRO Water for a Healthy Country Flagship).
West, K. M., Richards, Z. T., Harvey, E. S., Susac, R., Grealy, A., and Bunce, M. (2020). Under the karst: Detecting hidden subterranean assemblages using eDNA metabarcoding in the caves of Christmas Island, Australia. Sci. Rep. 10, 21479. doi:10.1038/s41598-020-78525-6
White, N. E., Guzik, M. T., Austin, A. D., Moore, G. I., Humphreys, W. F., Alexander, J., et al. (2020). Detection of the rare Australian endemic blind cave eel (Ophisternon candidum) with environmental DNA: Implications for threatened species management in subterranean environments. Hydrobiologia 847, 3201–3211. doi:10.1007/s10750-020-04304-z
Whiterod, N., Hammer, M., Unmack, P., Mathwin, R., and Gotch, T. (2019). IUCN Red List of Threatened Species: Craterocephalus dalhousiensis. IUCN Red List of Threatened Species. Available at: https://www.iucnredlist.org/en (Accessed August 11, 2022).
Williams, J., Stubbs, T., and Milligan, A. (2012). An analysis of coal seam gas production and natural resource management in Australia. A report prepared for the Australian Council of Environmental Deans and Directors (Canberra, Australia: John Williams Scientific Services Pty Ltd).
Wilmer, J. W., Murray, L., Elkin, C., Wilcox, C., Niejalke, D., and Possingham, H. (2011). Catastrophic floods may pave the way for increased genetic diversity in endemic artesian spring snail populations. PLOS ONE 6, e28645. doi:10.1371/journal.pone.0028645
Witjira National Park Co-management Board (2018). “Irrwanyere Aboriginal Corporation, Department for Environment and Water,” in Witjira waru pulka (fire) management strategy 2018–2028 (Port Augusta: South Australian Department for Environment and Water).
Witjira National Park Co-management Board (2022). Witjira National Park management plan. South Australia: National Parks and Wildlife Service.
Wolaver, B., Keppel, M., and Love, A. (2013). “Hydrogeology of Dalhousie springs,” in Allocating water and maintaining springs in the Great Artesian Basin (Canberra: National Water Commission),
Worthington Wilmer, J., and Wilcox, C. (2007). Fine scale patterns of migration and gene flow in the endangered mound spring snail, Fonscochlea accepta (Mollusca: Hydrobiidae) in arid Australia. Conserv. Genet. 8, 617–628. doi:10.1007/s10592-006-9206-x
Yates, C. J., Norton, D. A., and Hobbs, R. J. (2000). Grazing effects on plant cover, soil and microclimate in fragmented woodlands in South-Western Australia: Implications for restoration. Austral Ecol. 25, 36–47. doi:10.1046/j.1442-9993.2000.01030.x
Zeidler, W., and Ponder, W. F. (1989). The natural history of Dalhousie Springs. Adelaide, South Australia: Special Publication, South Australian Museum.
Keywords: Great Artesian Basin, groundwater-dependent ecosystems, springs, biodiversity, taxonomy, aquifers, climate change, eDNA
Citation: Beasley-Hall PG, Murphy NP, King RA, White NE, Hedges BA, Cooper SJB, Austin AD and Guzik MT (2023) Time capsules of biodiversity: Future research directions for groundwater-dependent ecosystems of the Great Artesian Basin. Front. Environ. Sci. 10:1021987. doi: 10.3389/fenvs.2022.1021987
Received: 18 August 2022; Accepted: 15 November 2022;
Published: 09 February 2023.
Edited by:
Jenny Davis, Charles Darwin University, AustraliaReviewed by:
Peter Mervyn Negus, Queensland Government, AustraliaRoderick Fensham, University of Queensland and Queensland Museum, Australia
Copyright © 2023 Beasley-Hall, Murphy, King, White, Hedges, Cooper, Austin and Guzik. This is an open-access article distributed under the terms of the Creative Commons Attribution License (CC BY). The use, distribution or reproduction in other forums is permitted, provided the original author(s) and the copyright owner(s) are credited and that the original publication in this journal is cited, in accordance with accepted academic practice. No use, distribution or reproduction is permitted which does not comply with these terms.
*Correspondence: P. G. Beasley-Hall, cGVycnkuYmVhc2xleS1oYWxsQGFkZWxhaWRlLmVkdS5hdQ==