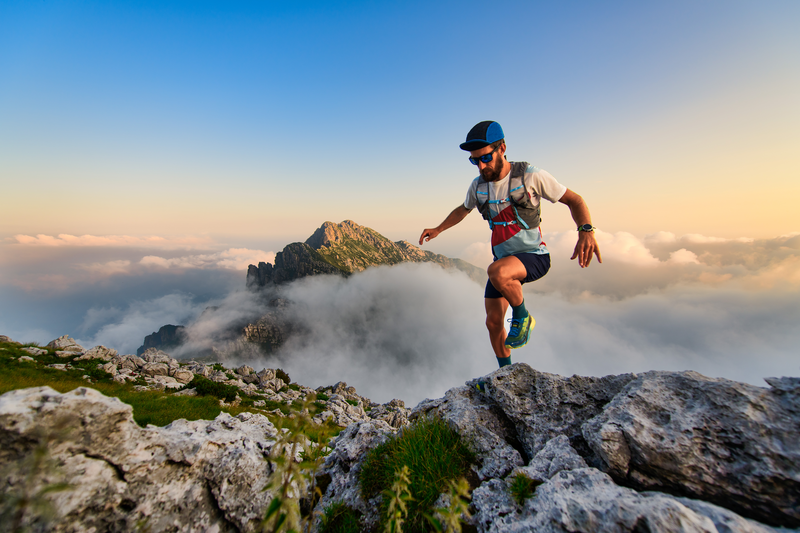
95% of researchers rate our articles as excellent or good
Learn more about the work of our research integrity team to safeguard the quality of each article we publish.
Find out more
ORIGINAL RESEARCH article
Front. Educ. , 07 April 2025
Sec. Teacher Education
Volume 10 - 2025 | https://doi.org/10.3389/feduc.2025.1512320
Introduction: Science teacher education programs play a crucial role in shaping future educators. Pre-service Teachers’ (PSTs) attitudes toward science and their self-efficacy beliefs are pivotal in determining the extent to which they engage with and benefit from their academic training. The Flipped Classroom Model (FCM) offers a technology-enhanced, student-centered approach with the potential to enhance PSTs’ self-efficacy beliefs and attitudes.
Methods: This study explores the effect of an FCM approach in a general science course on the PSTs science teaching self-efficacy beliefs and attitudes toward science. The study used a mixed methods approach with 39 PSTs. The quantitative dimension adopted a semi-experimental design with a pretest-posttest control group, and qualitative data were collected through semi-structured interviews.
Results: Results suggest that the FCM had a significant effect on PSTs’ science teaching self-efficacy beliefs, particularly in the personal science teaching efficacy, and on their attitudes toward science, particularly in their perceptions of the science teacher. The themes of supportive environment and hands-on activities emerged from the qualitative analysis.
Discussion: The implementation features of the FCM, combined with teacher support, appeared to foster a more productive classroom environment that had mobilized multiple sources of self-efficacy. This environment stimulated PSTs’ interest in science and increased their confidence, thereby cultivating a greater sense of investment in their science learning. Incorporating the FCM into teacher education programs offers significant advantages in PSTs’ academic training.
Despite many classrooms being well-equipped with technology, many educators still feel unprepared to fully leverage their potential to transform teaching, expand classroom boundaries, and enhance student learning experiences. These challenges, coupled with the ever-evolving expectations of what it means to be a teacher, presents a need for “new skills, new ways of thinking and innovative approaches to view the interaction of teaching and learning” (p. 560), which constitute important challenges and opportunities for teacher education (Livingston and Flores, 2017). Thus, effective educational technologies should be integrated into pre-service education, and innovative learning environments should be designed and implemented (Debbağ and Yıldız, 2021).
Research has consistently demonstrated that high-quality science courses can significantly influence pre-service teachers’ (PST) science self-efficacy beliefs (Tschannen-Moran and Johnson, 2011; Menon and Sadler, 2016; González-Gómez et al., 2022). Experience in teacher education programs can enhance self-efficacy by involving PSTs in inquiry-based science activities (Menon and Azam, 2021; Ingram et al., 2024). Particularly because the learning experiences that PSTs have before entering university play a crucial role in shaping their attitudes toward science (Palmer, 2006). In primary education, where teachers often exhibit a negative attitude toward science education (Tosun, 2000), it is essential to enhance efficacy beliefs about teaching and learning by relying on instructional methodologies that prioritize inquiry-based, student-centered, cooperative, and hands-on approaches (van Aalderen-Smeets and Walma van der Molen, 2015). However, according to Ulukök and Sari (2016), there is a lack of research on PSTs’ attitudes toward science teaching, despite the significant influence of teacher training programs and instructor attitudes on shaping their perspectives.
In recent years, there has been a notable increase in the popularity of the Flipped Classroom Model (FCM) (Hwang et al., 2019; Lo and Hew, 2021; Latorre-Cosculluela et al., 2022; Wilson and Hobbs, 2023; Ribeirinha and Silva, 2024). As a blended learning model, FCM can be implemented across various educational levels (e.g., Hwang et al., 2019; Ribeirinha and Silva, 2024) and aligns with the growing demand for technology-enhanced, student-centered learning environments (Lai et al., 2021). In light of the aforementioned gaps in the literature and the evidence derived from numerous studies indicating the pedagogical advantages of the FCM - including its positive effects on students’ academic achievement (van Alten et al., 2019), learner motivation and self-paced learning (Nikitova et al., 2020), student autonomy (Lee and Martin, 2020), as well as, its potential to enhance student self-efficacy (González-Gómez et al., 2022; Latorre-Cosculluela et al., 2022), and attitudes toward science (Jeong et al., 2021; González-Gómez et al., 2022) - this study explores the effect of FCM approach in a general science course on the PSTs science teaching self-efficacy beliefs and attitudes toward science. Specifically, it aims to: (1) examine the effects of the FCM on PSTs’ science teaching self-efficacy and attitudes toward science and (2) gain insight into the perceptions of PSTs regarding the utilization of the FCM for the science course.
The term self-efficacy can be defined as an individual’s conviction in their personal capabilities to motivate themselves and mobilize the requisite cognitive resources and action plans to effectively navigate a given situation (Bandura, 1986; Tschannen-Moran and Johnson, 2011). Individuals who possess a more robust perception of self-efficacy are more likely to demonstrate perseverance and exert greater effort, as perceived self-efficacy plays a critical role in shaping motivation levels and influencing performance accomplishments (Bandura, 1977). According to Bandura (1977, 1986, 1997), self-efficacy perceptions are derived from a variety of sources, including mastery experiences, vicarious experiences, verbal persuasion, and emotional and physiological states. Of these, mastery experiences are considered the most important factor in the formation of a strong sense of self-efficacy (Bandura, 1997). Mastery experiences are influenced by the individual’s past successes and failures in similar situations; it is the experience gained from tasks that a person has completed alone (Bandura, 1997). Vicarious experience involves observing the behavior of someone the individual holds up as a model and making a judgment about what kind of outcome the individual will achieve in similar situations (Bandura, 1997). Verbal persuasion encompasses both positive and negative verbal messages received from the environment regarding task performance (Bandura, 1997). Finally, emotional and physiological states are characterized by a reduction/increase in emotional barriers, which can be understood as an inability/ability to perform an action (Bandura, 1997).
The concept of self-efficacy has gained prominence in the field of teacher education (Twohill et al., 2023; Menon et al., 2024) and it has been associated with teachers’ decisions regarding instructional activities, classroom organization, and their capacity to cope with challenging circumstances (Bandura, 1986). Additionally, teacher self-efficacy is a critical factor in student engagement and learning (Coladarci, 1992). Teachers who demonstrate confidence and enthusiasm in their teaching foster a positive learning environment that enhances student engagement; conversely, uncertainty or defensiveness may be perceived by students as a lack of confidence, potentially hindering their learning experience (Coladarci, 1992). Bandura’s (1977) theory of social learning provides a valuable framework for examining teacher self-efficacy from a cognitive perspective. In accordance with this theory, teacher self-efficacy can be conceptualized as a bidimensional construct comprising personal efficacy and outcome expectancy. Personal efficacy pertains to one’s confidence in attaining a specific level of performance and involves teachers’ beliefs in their ability to teach effectively; outcome expectancy encompasses judgments regarding the probable consequences of specific behaviors and relates to student outcomes as a consequence of their teaching (Bandura, 1977).
Science teachers’ perceived beliefs about their ability to create an effective and productive learning environment and increase student success and motivation are expressed as science teaching self-efficacy (Durak et al., 2023). Science teachers’ self-efficacy emerges as an important predictor of their performance, influencing their confidence in implementing innovative teaching practices (Rittmayer and Beier, 2009). Research has demonstrated that elementary teachers with low science self-efficacy tend to avoid science instruction, teach it sporadically, or provide inadequate time for it (Bursal and Paznokas, 2006). On the other hand, there is evidence that supports a direct positive correlation between science teaching self-efficacy and the use of inquiry-based science teaching practices, job satisfaction, and student academic success (Perera et al., 2022). Consequently, enhancing the self-efficacy of PSTs has been identified as a crucial aspect of teacher education (Saçkes et al., 2012; Yerdelen et al., 2024). In this context, high levels of science teaching self-efficacy among PSTs enable them to face challenges with confidence and facilitate effective management of the learning environment; conversely, low levels of self-efficacy in science teaching may induce anxiety in PSTs leading them to perceive tasks as personal threats (Oppermann et al., 2019).
A considerable number of studies have employed the Science Teaching Efficacy Belief Instrument-Preservice (STEBI-B) (Riggs and Enochs, 1990) as a means of measuring the efficacy beliefs of PSTs. For instance, in a study conducted by González-Gómez et al. (2022), the impact of the flipped classroom approach on the science self-efficacy of PSTs was evaluated. The findings revealed that there were significant differences in the students’ self-efficacy levels before and after the completion of the course. However, as the study design did not include a control group, it is not possible to attribute the observed results exclusively to flipped classroom approach. In another study, Menon and Azam (2021) investigated the development of 55 PSTs’ science teaching self-efficacy beliefs by analyzing their reflective practices in a science methods course. The results indicated a statistically significant increase in the participants’ science teaching self-efficacy beliefs. The authors emphasized the importance of field experiences and reflective practices for pre-service elementary teachers’ preparation and science teaching self-efficacy. Similarly, a study conducted to determine if 30 teacher candidates’ confidence would increase during teacher preparation if they were exposed to authentic teaching practices throughout their field-based science methods course revealed mixed results (Flores, 2015). A pretest-posttest administration of the STEBI-B revealed that personal science teaching efficacy (PSTE) increased; however, science teaching outcome expectancy (STOE) increased, but to a lesser extent. Also, Ingram et al. (2024) investigated the effectiveness of garden-based technology integration in increasing the science teaching self-efficacy of 39 pre-service elementary teachers. Results showed that participants in the Garden TOOLS (Garden Technology Opportunities in Outdoor Learning Spaces) professional development workshop experienced a statistically significant increase in their PSTE from pre- to post-workshop. Participants who also implemented the Garden TOOLS lesson showed increases in both PSTE and STOE.
These findings lend support to the efficacy of high-quality science teacher training programs as a means of enhancing self-efficacy (Tschannen-Moran and Johnson, 2011; Menon and Sadler, 2016; González-Gómez et al., 2022). Participation in experiential learning activities, observation of expert instructors, and engagement in guided practice sessions within these science courses provide concrete mastery experiences that enhance PSTs’ understanding of scientific content and effective pedagogical strategies, thereby exerting a significant influence on their self-efficacy beliefs (Bandura, 1986). Beyond enhancing self-efficacy, these programs play a pivotal role in shaping PSTs’ attitudes toward science, an essential factor influencing how they approach science instruction and engage their students.
In the past few decades, researchers have proposed a variety of definitions of attitudes (Osborne et al., 2003). For example, Eagly and Chaiken (1993) defined attitude as a psychological tendency that is expressed by evaluating a particular entity with some degree of favor or disfavor. This definition identifies the core feature of an attitude as the propensity to produce an evaluative response. In this sense, attitudes toward science generally refer to individuals’ beliefs, feelings, and values about science, school science, and/ or the influence of science on society or scientists (Osborne et al., 2003).
The ABC model of attitudes (Myers, 2010) posits that attitudes are comprised of three fundamental components: affect, behavior, and cognition. Affect is used to describe an individual’s emotional response or feelings towards an object, person, or situation. The spectrum of emotional responses may encompass a range of positive and negative affect, including feelings of enjoyment and anxiety, respectively. Behavior encompasses the actions or observable behaviors that result from an attitude. For instance, recycling may be a behavior that stems from a strong belief in protecting the environment. Finally, cognition refers to beliefs, thoughts, and knowledge about a particular subject. For example, the belief that recycling helps to protect the planet is an example of cognition. These three components interact with one another to shape attitudes and influence how individuals feel, think, and behave in different contexts.
In the context of learning and education, students’ attitudes toward a subject are used to describe a student’s evaluation of learning content, methods, teacher, and their own feelings about the learning context (Li et al., 2024). Moreover, several studies have demonstrated a clear connection between teachers’ attitudes towards a given subject and the outcomes observed in their students (Riggs and Enochs, 1990; Mattern and Schau, 2002; Ulukök and Sari, 2016). These outcomes encompass both the extent to which students are able to engage with scientific learning and the evolution of their own attitudes. For instance, teachers with positive attitudes toward science and science teaching provided a more productive classroom environment, which in turn fostered positive attitudes toward the course and teacher in their students and increased their success and motivation in studying science (Mattern and Schau, 2002). Conversely, negative teacher attitudes toward science are likely to lead to a reduction in the amount of content covered and to teaching methods that are less engaging and less effective (Riggs and Enochs, 1990; Ibourk and Mathis, 2024). Furthermore, negative attitudes toward science among teachers can influence students to develop similar negative attitudes, as students often model and internalize the perspectives of their role models (McKown and Weinstein, 2002).
Notably, these attitudes toward science were shaped during pre-service times (Palmer, 2006). Consequently, during their university education, teacher education programs and their content, as well as the attitudes of instructors who teach science courses or any courses on science and science education, exert an influence on PSTs’ attitudes toward science education (Ulukök and Sari, 2016).
Nevertheless, research has demonstrated that teacher expertise is not merely contingent on comprehensive subject matter expertise (e.g., Menon and Sadler, 2016). Instead, it is also shaped by the development of pedagogical content knowledge (encompassing inquiry-based teaching and learning pedagogies), the cultivation of ICT proficiency, and a sense of agency and dedication to science education (Marco-Bujosa and Levy, 2016). Building on this idea, the Riegle-Crumb et al. (2015) study investigates the potential of inquiry-based science courses to enhance the attitudes of pre-service elementary teachers toward science. A comparison of over 200 students in an inquiry-based program with those in traditional lecture-based classes revealed that participants in the inquiry-based courses exhibited a statistically significant increase in their scores on scales measuring confidence, enjoyment, anxiety, and perceptions of relevance. Conversely, those in the comparison group demonstrated a decline in favorable attitudes toward science. In addition, a study published in 2016, Ulukök and Sari (2016) investigated the impact of computer-assisted laboratory applications on the attitudes of pre-service science educators towards science teaching. The study involved 46 students who participated in an eight-week computer-assisted course. The results indicated that the computer-assisted applications had a significant impact on the attitudes and motivation of the PSTs. Furthermore, the authors indicated that the participants perceived the simulations to be advantageous, citing that they facilitated learning, supported knowledge retention, and positively influenced their attitudes and creativity.
These findings lend support to the efficacy of high-quality science teacher training programs as a means of enhancing attitudes toward science. Consequently, to enhance PSTs attitudes toward science, the instructional methodology should focus on science learning through a more inquiry-based, student-centered, and hands-on approach, while also promoting cooperative learning activities (van Aalderen-Smeets and Walma van der Molen, 2015). One pedagogical approach that aligns well with these principles is the Flipped Classroom Model, which emphasizes active, student-centered learning through collaboration.
In recent years, FCM has emerged as a central pedagogical approach in the educational landscape, attracting considerable interest from researchers and educators alike (Hwang et al., 2019; Lo and Hew, 2021; Latorre-Cosculluela et al., 2022; Wilson and Hobbs, 2023; Ribeirinha and Silva, 2024). The FCM is a pedagogical model originally proposed by Bergmann and Sams in 2012 in their book ‘Flip Your Classroom: Reach Every Student in Every Class Every Day’ (Bergmann and Sams, 2012). According to the authors, FCM reverses the conventional sequence of learning activities presented to students, i.e., activities such as lectures or teacher explanations are conducted at home using video lessons (or other resources selected by the teacher), and the traditional concept of ‘homework’ is transformed into an integral part of the in-class sessions. It is therefore anticipated that students will have completed the requisite tasks prior to the lesson. The lesson itself should be dedicated to constructivist activities, such as discussion, practical activities, and problem-based learning. The main goal is to enhance the quality of face-to-face instruction in the classroom by allocating the component that encompasses advanced competencies, such as knowledge assimilation and persistence, to the classroom setting, while assigning the component that requires basic knowledge acquisition to outside the classroom (Bergmann and Sams, 2012).
Horn and Staker (2015) conceptualized FCM as a blended learning approach that responds to the demand for learning environments enhanced by technology and centered on the student. Firstly, the integration of technology in pre-class sessions allows the teacher to deliver multimodal content, thereby creating opportunities for students to practice, interact and receive feedback (Ribeirinha et al., 2022). Secondly, the utilization of technological resources (platforms and instructional videos) in pre-class sessions provides students with enhanced control over their learning, enabling them to regulate both the pace and frequency of their interactions with the content, as well as the feedback they receive (Lai et al., 2021). Finally, the model optimizes in-class time by providing more opportunities for peer and instructor interaction, thereby facilitating collaborative learning and the development of higher-order skills (van Alten et al., 2019). This is because the model recognizes the pivotal role of students in their own educational achievement, placing trust in their capacity to work autonomously and their contribution to the group’s learning (FLN, 2014).
Since the introduction of FCM, there has been a notable increase in the number of research studies conducted across various disciplines that have documented its favorable impact on learning outcomes. The research evidence on FCM demonstrates that it has a positive impact on students’ academic achievement (van Alten et al., 2019), learner motivation and the ability to learn at their own pace (Nikitova et al., 2020). It enhances student autonomy, learning by doing, and mitigates cognitive overload in teacher education (Lee and Martin, 2020). Furthermore, it has the potential to augment student engagement (Lo and Hew, 2021) and self-efficacy (González-Gómez et al., 2022; Latorre-Cosculluela et al., 2022) and attitudes toward science (Jeong et al., 2021; González-Gómez et al., 2022). In addition, several meta-analyses have been conducted with the objective of synthesizing the existing evidence on this topic. In a study published in 2019, Shi et al. (2020). collated and analyzed the effect sizes of 33 studies comparing FCM with traditional learning. Their findings indicated that FCM facilitates cognitive learning in college students across a diverse range of disciplines. In their 2021 meta-analysis, Lo and Hew (2021) drew upon a substantial body of research on FCM to examine student engagement across three key dimensions. The findings indicated that the implementation of the FCM may enhance certain facets of behavioral engagement (e.g., interaction and attention/participation), emotional engagement (e.g., course satisfaction) and cognitive engagement (e.g., understanding of mathematics). However, it was also observed that several aspects (e.g., students’ attendance, mathematics anxiety and self-regulation) of student engagement have not been sufficiently investigated and warrant further examination. Nevertheless, it should be noted that not all studies on flipped learning have yielded consistent, positive results. Indeed, several studies have indicated that there is no significant difference in students’ academic achievement between flipped classrooms and traditional environments (Fraga and Harmon, 2014; Smith et al., 2020; Wilson and Hobbs, 2023), or in students’ satisfaction (van Alten et al., 2019). Therefore, further research is required to ascertain the specific advantages of the flipped classroom approach in comparison to traditional teaching methods (Zhao et al., 2021; Latorre-Cosculluela et al., 2022). Particularly, there is limited research analyzing the impact of FCM on the self-efficacy of PSTs in science teaching (González-Gómez et al., 2022).
The study was conducted over a period of 14 weeks, employing a mixed-methods research approach (Creswell, 2012). The quantitative dimension was based on a semi-experimental design with a pretest-post-test control group. For the qualitative dimension, semi-structured interviews were carried out with the experimental group, providing additional insights that complemented the quantitative findings.
The study involved 39 PSTs from two classes who were enrolled in a general science course as part of the basic education program at the School of Education of the Santarém Polytechnic University in Portugal during the 2023/2024 academic year.
The two classes had the same instructor responsible and constituted the sole cohort in the inaugural year of the basic education program. The experimental and control groups were assigned based on the results of a diagnostic achievement test, which assessed the PSTs’ prior science knowledge before the course commenced (see section 3.3 for more information). This test also allowed researchers to determine whether the two classes were equivalent in terms of initial science knowledge. As no significant differences were found in the pretest scores between the two classes, group assignment was conducted randomly within them. Consequently, Class 1 was entirely designated as the experimental group, while Class 2 served as the control group.
The experimental group, comprising 22 PSTs (all female), had an average age of 21.64 years (SD = 6.57). The experimental group participated in a general science course following the FCM. Prior to the in-class sessions, the group received individualized instruction via internet-connected devices, typically in the form of video lessons tailored to different learning paces, interactive quizzes providing immediate feedback, and supplementary readings and exercises based on individual progress. During the in-class sessions, the group engaged in inquiry-based, student-centered, and hands-on learning activities, such as designing and conducting simple experiments, collaboratively solving real-world problems through case studies, and using modeling tools to explore physical processes.
The control group comprised 17 PSTs (one male and 16 female), with an average age of 21.06 years (SD = 6.47). They had completed a general science course following the same curriculum as the experimental group, but without any intervention prior to the in-class sessions. Although they participated in practical, group-based activities during the in-class sessions, these activities were implemented within a standard, teacher-led instructional framework without the preparatory, inquiry-based, or student-centered components provided to the experimental group.
In order to capture the PSTs’ science teaching efficacy beliefs, the Science Teaching Efficacy Belief Instrument-Preservice (STEBI-B) (Riggs and Enochs, 1990) was adapted for use this study. The original instrument consists of 23 items, which are rated on a 5-point Likert scale, ranging from “strongly disagree” to “strongly agree.” It comprises two subscales: The Personal Science Teaching Efficacy (PSTE) and the Science Teaching Outcome Expectancy (STOE). The PSTE comprises 13 items and measures PSTs’ beliefs about teaching science (e.g., “I know how to teach science concepts effectively”). The STOE comprises 10 items and measures elementary student outcomes as a result of science teaching (e.g., “The inadequacy of a learner’s science background can be overcome by good teaching”). The instrument utilizes forward and reverse-phrased items, with their associated scores being reversed in accordance with the STEBI-B implementation guidelines. The STEBI-B is a well-established instrument that has been developed and validated by Riggs and Enochs (1990), and translated versions of the instrument have been used in several studies internationally (e.g., González-Gómez et al., 2022). The original version of the survey instrument was translated into Portuguese. To ensure the survey instruments could be read and understood, three PTSs were invited to complete the survey and provide feedback on the instructions, question format, and wording. Based on their feedback, additional revisions were made before the survey was finalized and administered to the participants. The reliability of the STEBI-B was satisfactory (Cronbach’s α = 0.80), with the same evaluation being achieved in both subscales [Cronbach’s α (PSTE) = 0.78; Cronbach’s α (STOE) = 0.84]. These values exceed the threshold of 0.70, which is considered acceptable (Cohen, 1988).
Conversely, PSTs’ attitudes toward science were evaluated by using the Attitude Toward Science Inventory (ATSI) (Gogolin and Swartz, 1992). The ATSI is a 48-item inventory, with responses rated on a 5-point Likert scale, ranging from “strongly disagree” to “strongly agree.” The inventory comprises six subscales (perception of the science teacher, anxiety toward science, value of science in society, self-concept in science, enjoyment of science, and motivation in science) and exhibits high construct validity; for the six subscales, Cronbach’s alpha coefficients ranged from 0.73 to 0.90 (Gogolin and Swartz, 1992). The ASTI comprises negatively worded items, and their associated scores were reversed. Higher numerical scores indicate more positive attitudes in all areas except anxiety, where a lower numerical score reflects a more positive attitude (less anxiety). In line with the findings of Schruba (2008), this study employed a condensed version of the ASTI comprising 36 items, distributed across four subscales: perception of science teacher (e.g., “Science teachers show little interest in their students”), anxiety toward science (e.g., “It makes me nervous to even think about doing science”), value of science in society (e.g., “Science is useful for solving the problems of everyday life”) and enjoyment of science (e.g., “I enjoy talking to other people about science”). The condensed version of the survey instrument was translated into Portuguese. To guarantee that the inventory could be read and understood, the same procedure that was applied in STEBI-B was used (three PTSs were invited to complete the inventory and provide feedback on the instructions, question format, and wording; based on their feedback, additional revisions were made before the inventory was finalized and administered to the participants). The reliability of the four subscales was satisfactory [Cronbach’s α (science teacher) = 0.837; Cronbach’s α (anxiety toward science) = 0.867; Cronbach’s α (enjoyment of science) = 0.864 and Cronbach’s α (science in society) = 0.772], with values greater than 0.70 being considered as acceptable (Cohen et al., 2003).
Furthermore, the PSTs were required to complete two academic achievement tests, which were designed by the instructor. The first assessment was a diagnostic achievement test consisting of 20 multiple-choice questions designed to identify the science knowledge that the students had acquired prior to the start of the course. This test also allowed the researchers to determine whether the two groups were equivalent in terms of their initial science knowledge. The second achievement test consisted of 25 questions presented in a variety of formats and it was developed considering the learning outcomes of the general science course. Both tests were scored on a 200-point scale.
Finally, to obtain qualitative data, a semi-structured interview script was developed by the researcher and subsequently reviewed with the main researcher, who provided feedback on the content and suggested necessary modifications. The interview questions were designed to elicit the perceptions of the PSTs in the experimental group regarding the implementation process of the FCM. In particular, the questions were structured around three distinct dimensions: (1) General experience, this dimension assessed the comprehensive influence of the FCM implementation on the learning and engagement of the PSTs; it encompassed both the challenges encountered and the positive aspects perceived by the PSTs, as well as an evaluation of the extent to which the FCM facilitated enhanced preparation for future endeavors, such as the instruction of science (e.g., “What were the most positive and challenging aspects of using FCM in the science classroom?”); (2) Pre-class interactions, this dimension related to the preparatory phase preceding the classroom sessions, during which the PSTs had access to learning resources designed for autonomous exploration; it included an evaluation of the effectiveness of the materials provided, the PSTs’ self-management of their time and learning effort, and the impact these preparatory activities may have had on their understanding of the concepts and their approach to the classroom sessions (e.g., “To what extent were the learning resources provided in the pre-class useful for learning the scientific concepts?”); (3) In-class interactions, this dimension is concerned with the observable changes in the classroom environment, particularly in terms of the dynamics between the teacher and the PSTs, and between the PSTs themselves; it included an analysis of the evolution of these interactions in the context of FCM implementation, with a specific focus on the role of teacher support in facilitating learning and the impact of peer collaboration in resolving difficulties and clarifying doubts (e.g., “Compared to traditional approaches to science teaching, what has changed in the classroom with the use of FCM and how have these changes affected your learning?”).
The two groups (experimental and control) were enrolled in a 14-week general science course comprising four lessons per week. The course was designed to provide PSTs with a general understanding of scientific content. The course covers a range of topics, including the composition and structure of the universe, the nature of matter and its transformation, the properties and transformation of energy, and the interaction between matter and energy.
The teaching instruction for the experimental group was based on the FCM approach and comprised two distinct phases: an online pre-class session conducted by the PSTs at home and a subsequent face-to-face in-class session held at the educational institution. The online pre-class session, which was made available 1 week prior via an e-learning platform, comprised a set of materials that focused on theoretical content. The materials comprise a didactic video, written texts, a monitoring quiz, and a list of exercises. In conjunction with the exploration of the aforementioned materials, the PSTs were encouraged to synthesize the theoretical content and complete the quiz on the platform 1 day prior to the in-class session. This allowed the instructor to identify any potential learning difficulties that could be addressed during the in-class session. None of these activities were compulsory or had any weight in the final grade for the term. In the in-class session, the instructor and PSTs first discussed the pre-class session, namely the contents of the videos and any misunderstandings. Subsequently, the PSTs were divided into groups for the purpose of engaging in inquiry-based and problem-based activities. These practical activities were directly related to the theoretical content of the pre-class session and constituted obligatory components of the final grade for the course. In these in-class sessions, the instructor’s role was to provide support to PSTs in their work and to deliver brief explanations when necessary.
The control group was not subjected to any form of individualized or pre-class intervention throughout the course duration. The curriculum was followed using predominantly teacher-centered methods, such as direct lectures, presentations, and demonstrations. However, to align with the course structure and learning objectives, both groups participated in practical, group-based activities during the in-class sessions. The key distinction is that, unlike the experimental group, the control group engaged in these activities without prior exposure to individualized instruction, inquiry-based learning, or student-centered approaches. Their participation in group activities was guided primarily by teacher-led instruction, without the emphasis on self-directed exploration and scaffolding that characterized the experimental condition.
The experimental and control groups administered the academic achievement test and questionnaire (STEBI-B and ATSI) at the outset of the course (pre-test, September 2023) and at its conclusion (post-test, January 2024). The questionnaire was administered in person under the supervision of the researchers. Before administering the questionnaire, a brief description of the purpose of the study and an informed consent process were given to the participants. The questionnaire took about 25 min (STEBI-B: 10 min; ATSI: 15 min) to complete. The pre- and post-tests were matched using the PSTs’ identification numbers.
Additionally, the experimental group participants were invited to share their perceptions of the FCM implementation process at the conclusion of the course. All PSTs who participated in the interview had volunteered to do so. The interviews were conducted online via Zoom® with the 16 PSTs, who were divided into groups of four. The average duration of the interviews was 30 min, and they were audio-recorded with the consent of the participants. The research pattern is illustrated in Figure 1.
The results of the questionnaire were subjected to analysis using Jamovi ® 2.2.5.0 software. The reliability of the subscales was evaluated through the calculation of Cronbach’s alpha (α). The normality assumption of the data was assessed through the utilization of the Shapiro–Wilk test. The descriptive statistics were examined, and the mean score was obtained for the questionnaires, for each subscale of the questionnaires, and for the academic achievement tests. For the intergroup analyses, an independent samples t-test was used was used if the assumptions of normality (Shapiro–Wilk test) and equal variances (Levene’s test) were met. Otherwise, the Mann–Whitney U test was employed.
A thematic analysis was conducted on the qualitative data set. A deductive thematic analysis was undertaken, with the researcher’s preconceptions and ideas, developed through the literature review, informing the coding process. Nevertheless, any codes or themes that were not aligned with the preconceived concepts were employed to guarantee that the data collection process was adaptable to the PST’s voice. The Braun and Clarke (2006) six-phase framework was employed for thematic analysis. In the initial stages of data analysis, transcripts were produced from the interviews. The coding process was conducted in a systematic manner, with the transcripts being highlighted and assigned their initial codes. Subsequently, the data were categorized based on their collective meaning. The coded data were then rearranged into more refined themes. Subsequently, the resulting themes were subjected to a process of reflection and review by two researchers, with the objective of ensuring accuracy and relevance.
This section presents the findings of the study, structured into quantitative and qualitative results. The quantitative analysis examines the effects of the FCM on PSTs’ science teaching self-efficacy and attitudes toward science. The qualitative analysis explores PSTs’ perceptions of utilizing the FCM in the science course. The results are organized as follows: first, the quantitative findings, including pre-test and post-test comparisons, are presented. This is followed by qualitative findings, which provide insight into participants’ experiences and perspectives.
Table 1 presents the mean values obtained by each group in the various instruments administered at the pre-test stage, together with the results of the comparative analysis of these values. Since the pre-test data were not normally distributed, Mann–Whitney test was used for comparison.
Table 1. Mann–Whitney test results related to STEBI-B, ASTI, and academic achievement test pre-test scores of the experimental and control groups.
No statistically significant difference was identified between the experimental and control groups with respect to either of the STEBI-B subscales or the STEBI-B total score. [PSTE: U(37) = 179, p = 0.595; STOE: U(37) = 159, p = 0.213; Total: U(37) = 154, p = 0.357].
Furthermore, no statistically significant difference was observed between the experimental and control groups with regard to the ATSI subscales or the ASTI total score [Science Teacher: U(37) = 164.5, p = 0.530; Anxiety toward science: U(37) = 179.5, p = 0.842; Enjoyment of science: U(37) = 177.0, p = 0.787; Science in society: U(37) = 176.0, p = 0.764; Total: U(37) = 159.5, p = 0.444].
Finally, as previously stated, the Diagnostic achievement test was utilized to assess the two groups in terms of their initial scientific knowledge. The results of the Mann–Whitney test indicate that the two groups exhibited no statistically significant difference in terms of initial scientific knowledge [U(37) = 388; p = 0.662].
The mean values obtained by the control and experimental groups in the various instruments administered at the post-test stage are presented in Table 2, together with the results of the comparative analysis of these values.
Table 2. Mann–Whitney test results related to STEBI-B, ASTI and academic achievement tests scores of the experimental and control groups at post-test stage.
Regarding STEBI-B, a statistically significant difference was observed between the STEBI-B total post-test scores of the experimental and control groups, in favor of the experimental group [U(37) = 115.5, p = 0.044]. The same was verified to the PSTE post-test scores [U(37) = 127, p = 0.044]. It can thus be stated that the FCM had a positive effect on PSTs’ science teaching self-efficacy beliefs, specifically in PSTE subscale. Nevertheless, no notable discrepancy was observed in the STOE post-test scores between the two groups [U(37) = 153.0, p = 0.171].
A statistically significant difference was identified between the experimental and control groups in the ATSI total score and in the Science Teacher scale, in favor of the experimental group [U(37) = 97.5, p = 0.012; U(37) = 82.0, p = 0.003]. It can therefore be proposed that the FCM had a positive effect on PSTs’ attitudes toward science, particularly regarding the perceptions of the science teacher subscale. However, no differences were identified in the remaining subscales [Anxiety toward science: U(37) = 159.0, p = 0.431; Enjoyment of science: U(37) = 161.0, p = 0.468; Science in society: U(37) = 129.5, p = 0.103].
Finally, with respect to the academic achievement test, a statistically significant difference was identified between the experimental and control groups, in favor of the experimental group [U(37) = 145.0, p < 0.001]. It can thus be proposed that the FCM had a positive effect on the academic achievement of PSTs.
The semi-structured interview provided a deeper insight into the perceptions of the PSTs regarding the utilization of the FCM for the science course. Overall, the PSTs outlined several characteristics of their flipped learning environment and how it impacted their attitude toward science and their beliefs about science teaching self-efficacy (see Table 3). Thematic analysis identified six themes that facilitate comprehension of PSTs’ perceptions regarding the utilization of the FCM for the science course. Each theme was subsequently subdivided into subcategories, representing distinct aspects of the theme.
The initial theme to emerge from the experimental group interviews was personalization, which was subsequently divided into two subcategories: freedom of choice and learning style. This concept refers to the way the pre-class materials enabled the PSTs to personalize the educational experience, aligning it with their individual needs, preferences and interests. The PSTs indicated that they had the option to participate in the activities offered in the pre-class, which were not mandatory. If they chose to engage in these activities, they were free to decide when, where and how. This was made possible by the time flexibility afforded to them, the digital learning content, and the variety of content formats (videos, readings, interactive tools) through which they could chart their learning path according to their learning style.
The following quotations from PST’s interview serve to illustrate this theme:
I think this method, made my learning much easier. I like to take notes, and I could stop the videos as many times as I wanted until I understood (PST4).
I liked that the content was available on video because it's more engaging than reading. There were also slides and notes for those who wanted to read, but I preferred the videos because I understood the content better. It's more immediate when you hear it and see it (PST12).
The second theme was behavioral change, broken down into the subcategories of study routines and autonomy. PSTs reported that FCM required them to prepare for in-class sessions by engaging with the material independently. This shift gave them greater responsibility for managing their learning outside the classroom. Over time, they began to develop a sense of accountability, understanding that the success of their in-class sessions depended on their preparation. Such regular engagement with content outside the classroom encouraged the development of routine study practices, fostered independence and improved time management habits as they learned to balance multiple tasks, prioritize effectively, and meet deadlines.
The following quotations from PST’s interview serve to illustrate this theme:
The in-class session without the pre-class preparation wasn’t as productive for me …. so, for me the responsibility of preparing was really worth it (PST6).
I’d say this experience was challenging, but in a good way. It was a chance for us to work on our autonomy. We set aside time each week to watch the videos, take notes, and do the quizzes, even though it wasn’t required. We really threw ourselves into it (PST5).
After that, I even liked doing them [pre-class tasks] because I could see that they were useful for my performance in class (PST9).
A further emerging theme was that of emotions, which was divided into two subcategories: anxiety levels and confidence in learning. PSTs indicated that the FCM allowed them to engage with new content at their own pace prior to class, thereby reducing the pressure often associated with traditional classroom settings where learners are expected to grasp complex concepts immediately during live lectures. The self-paced nature of the pre-class learning environment was found to help reduce anxiety levels among PSTs, as it allowed them to revisit material until they felt sufficiently prepared. Furthermore, the prompt feedback provided by the quizzes enabled the PSTs to overcome challenges in a supportive environment. By allowing PSTs to check their answers immediately and correct mistakes themselves, these quizzes helped them to identify areas of misunderstanding, adjust their learning strategies and set specific goals for improvement. As they master content independently, they gain confidence in their abilities and knowledge.
The following quotations from PST’s interview serve to illustrate this theme:
The model instilled a greater sense of confidence. Initially, I felt some uncertainty when I learned that my course would include science. However, as the semester progressed, I found that I was able to understand the science content, which led to a reduction in my feelings of insecurity. (PTS2).
As the preparation undertaken prior to the class was not included in the assessment, there was no concern about the potential for failure. In this sense, the tasks were completed without undue pressure, as the quizzes served merely to alert us to areas requiring improvement. Naturally, we would prefer to have demonstrated a higher level of knowledge and competence (PTS14).
Another theme that emerged from the interviews with the experimental group was that of a supportive environment, which was subsequently divided into three subcategories: teacher support, peer support and complementarity. PSTs described that the FCM fostered a supportive environment in in-class sessions, characterized by the establishment of robust teacher and peer support networks. It was emphasized that the complementarity between pre-class preparation and in-class activities ensured that they arrived at the in-class session adequately prepared to engage deeply with the activities. Consequently, the in-class time was utilized to provide individualized guidance, addressing specific student requirements, and reinforcing challenging concepts. It was also highlighted that PSTs collaborate on problem-solving activities, sharing knowledge and learning from each other. This comprehensive support system elevated PSTs’ confidence and engagement, resulting in enhanced levels of satisfaction and success in their learning journey.
The following quotations from PST’s interview serve to illustrate this theme:
In the practical activities, I assisted the group and the group assisted me. I believe this was a productive process for all involved, as we were able to address the questions and felt a sense of accomplishment in doing so (PST7).
The teacher was great at creating engaging scenarios. For example, in one exercise, we were instructed to interact as atoms of different species, with the objective of forming simple, double, and triple bonds. I found this approach highly engaging and think it would be beneficial to incorporate more activities like this into the curriculum (PST9).
In class, the teacher would go over things we already knew from home, which made us feel more comfortable. We often had doubts, which allowed us to discuss the content in a different, more thoughtful way. Sometimes, during class, more doubts would come up, which probably would not have come up if we were hearing the content for the first time (PST2).
A further emerging theme was that hands on which was divided into three subcategories: involvement, understanding and mastery experience. PSTs indicated that the in-class sessions facilitated active learning, with the class time allocated to discussion, problem-solving, and the application of knowledge. This approach facilitated involvement with the activities, prompting critical questioning and thinking, as opposed to passive information absorption. As they progress through these activities, they move from theoretical understanding to practical application, thereby deepening their understanding of the subject matter. Furthermore, the completion of challenging tasks provided the opportunity for mastery experiences that boosted their confidence and increased their sense of accomplishment. This cyclical process of involvement, deeper understanding and mastery ultimately results in a more complete and empowering learning experience.
The following quotations from PST’s interview serve to illustrate this theme:
(…) There was more discussion, more interaction in the class. Because we already knew something, we answered more of the teacher’s questions and were able to clarify our colleagues’ doubts (PST4).
I think it [FCM] made it easier to understand the content because we were not hearing it for the first time. Because the lesson was built on the knowledge we brought with us, there was more time for hands-on activities where we could apply and develop that knowledge (PST6).
The final theme to emerge from the interviews with the experimental group was that of innovation, which was subsequently divided into three subcategories: meaningful learning, change (behavioral, affective, and cognitive), and vicarious experience. This concept concerns the implementation of creative and inspiring methodologies that have transformed the way PSTs learn, creating meaningful learning experiences and equipping them with the necessary skills to respond innovatively and effectively to the needs of their students. PSTs posited that the FCM constituted an educational innovation that transformed their learning experience. The course content was accessible remotely, utilizing a range of resources, thereby enabling learners to personalize their learning experience and proceed at their own pace. The self-paced nature of the pre-class learning environment helped to reduce anxiety levels by allowing learners to revisit material until they felt sufficiently prepared (affective change). Such regular engagement with content outside the classroom encouraged the development of routine learning practices and fostered a sense of independence from the teacher (behavioral change). In-class time was devoted to interactive, hands-on activities that promoted more meaningful learning. The completion of challenging tasks, supported by the teacher and peers, provided the opportunity for mastery experiences that boosted their confidence and increased their sense of accomplishment (cognitive change). This cyclical process of involvement, deeper understanding and mastery ultimately results in a more complete and empowering learning experience. The inspiration provided by the teacher when utilizing the FCM in the PSTs teaching and learning process was evident in their discourse. The experience was regarded as so constructive that the PSTs expressed a willingness to reproduce the model when they assumed the role of teacher (vicarious experience).
The following quotations from PST’s interview serve to illustrate this theme:
The model is more effective than traditional classes in preparing students for teaching and is particularly beneficial for subjects that are challenging to learn, such as science and mathematics. I intend to utilize this model when I become a teacher (PST12).
Initially, I was uncertain about my ability to complete the subject. However, I believe that the model’s effectiveness is responsible for the positive outcome. This experience has enhanced my understanding of science and enhanced my capacity to educate future students in this field (PST16).
The main objectives of this study were to (1) examine the effects of the FCM on PSTs’ science teaching self-efficacy and attitudes toward science; (2) gain insight into the perceptions of PSTs regarding the utilization of the FCM for the science course.
The results indicated that the FCM had a statistically significant impact on enhancing science teaching self-efficacy beliefs particularly about the PSTE of the PSTs. This finding is consistent with previous studies that have demonstrated the positive effect of FCM on increasing PSTs’ science self-efficacy beliefs (González-Gómez et al., 2022; Latorre-Cosculluela et al., 2022). However, the results also indicated that the FCM did not have a statistically significant impact on the STOE of the PSTs. In other words, the FCM led to an increase in the PSTs’ confidence in their abilities to teach science, yet it did not result in a change in their belief that their teaching practices would lead to improved student achievement and learning.
By the time the study was conducted, the PSTs had not yet initiated their teaching practice in an educational setting. As outcome expectancy encompasses judgments regarding the capacity of teaching to bring about change in children’s understanding (Bandura, 1977), it is possible that they have not yet developed the ability to make judgments about the consequences of their behavior as teachers. This notion is corroborated by prior research employing STEBI-B to assess PSTs’ self-efficacy beliefs during field experiences (teaching practice in an educational setting), wherein alterations in the PSTE and STOE of PSTs were discerned (Flores, 2015; Menon and Sadler, 2016; Menon and Azam, 2021). Furthermore, the study conducted by Ingram et al. (2024) indicated that PSTs in a professional development workshop exhibited a statistically significant increase in their PSTE from pre- to post-workshop; additionally, those who implemented the workshop lesson demonstrated increases in both PSTE and STOE. In this sense, field experiences can be expected to produce changes in STOE, as these experiences provide PSTs with first-hand teaching experience.
Another pertinent factor that contributes to an understanding of these findings is the academic performance of the PSTs during the course. The implementation of FCM had a significant impact on the academic performance of PSTs, a fact that has been reported in previous studies (e.g., van Alten et al., 2019). Twohill et al. (2023) found that personal mathematics teaching efficacy was positively correlated with students’ performance in mathematics, whereby students with higher prior attainment in mathematics exhibited stronger self-efficacy beliefs, but there was no correlation between mathematics performance and mathematics teaching outcome expectancy. Thus, it can be argued that the FCM promoted the development of advanced scientific knowledge, which in turn increased PSTs’ confidence in their ability to teach science effectively. However, a lack of field experience could lead to a prevailing sense of uncertainty among PSTs regarding their ability to influence student learning outcomes.
The interviews offer valuable insight into the specific characteristics of experimental course design that contributed to the increase in PSTE. A variety of sources of self-efficacy, including mastery experiences, vicarious experiences, verbal persuasion, and emotional and physiological states (Bandura, 1977, 1986), were identified through the analysis of interviews with the experimental group. Firstly, the interviews revealed that the self-paced nature of the pre-class learning environment helped PSTs to reduce anxiety levels by allowing them to revisit material until they felt sufficiently prepared. Prior research has already highlighted the advantages of the self-paced nature of FCM (e.g., Lai et al., 2021; Nikitova et al., 2020). As PSTs achieved proficiency in the subject matter through independent study, they may have developed confidence in their abilities and subject knowledge. The emotional and psychological states aroused by the PSTs’ participation in the pre-class sessions (reduced anxiety and confidence) may have served to reduce or eliminate emotional barriers that might otherwise inhibit the performance of an action (Bandura, 1977). Consequently, this may have increased the PSTs’ self-efficacy beliefs. Secondly, this growing self-assurance was further reinforced during in-class sessions, wherein PSTs were able to actively participate in hands-on activities, pose questions, and apply their learning without the anxiety associated with being unprepared. The completion of hands-on science activities provided the opportunity for mastery experiences (Menon and Azam, 2021), which may have served to enhance the confidence of PSTs and augment their self-efficacy beliefs. Thirdly, PSTs indicated that the FCM facilitated the creation of a supportive environment within in-class sessions, characterized by the establishment of robust teacher and peer support networks. The verbal persuasion employed by the teacher to clarify or affirm PSTs’ abilities may have enhanced their perseverance and equipped them to overcome challenges. The optimization of in-class time through the provision of enhanced opportunities for peer and instructor interaction has been demonstrated to facilitate the development of higher-order skills (van Alten et al., 2019). Ultimately, the inspiration provided by the teacher in using the FCM in the teaching and learning process of the PSTs was evident in the experimental group interviews. The experience was perceived as so beneficial that the PSTs expressed a willingness to reproduce the FCM when they assumed the role of teacher (vicarious experience). Although the quantitative results indicate that the FCM had no impact on STOE, the PSTs’ discourse demonstrates their capacity to anticipate positive outcomes should they utilize the model with their students. It can thus be proposed that the implementation characteristics of the FCM, when combined with the teacher’s support, play a pivotal role in enhancing the PSTs’ science self-efficacy.
Regarding attitudes towards science, the results showed that the FCM had a positive effect on PSTs’ attitudes toward science. This finding is consistent with previous studies that have demonstrated the positive effect of FCM on increasing PSTs’ attitudes toward science (González-Gómez et al., 2022; Jeong et al., 2021). Upon analysis of the ATSI by subscale, it was verified that the FCM exerted a pronounced influence on PSTs perceptions of the science teacher. Several studies have demonstrated a clear connection between teachers’ attitudes towards a given subject and the extent to which their students are able to engage with scientific learning and the evolution of their own attitudes (Riggs and Enochs, 1990; Mattern and Schau, 2002; Ulukök and Sari, 2016). Given that FCM is a student-centered pedagogical approach, its successful implementation necessitates that the teacher assumes the role of a professional educator (FLN, 2014). This entails the management and guidance of the entire learning process. Initially, it encompasses the selection and creation of relevant content to facilitate student learning. Subsequently, it includes the capacity to develop an integrated learning experience, wherein a robust complementarity between the core components (in-class sessions and pre-class sessions) is essential. Additionally, it requires the ability to provide prompt and adaptive feedback, as well as to engage in reflective practice to ensure continuous improvement. Thus, it can be hypothesized that the flipped science course was implemented by a professional educator (with a positive attitude toward science and science teaching) who was able to create a more productive classroom environment, thereby fostering positive attitudes toward science and the teacher among the PSTs and stimulating their interest in studying science.
The analysis of the interviews with the experimental group offers further evidence to support the hypothesis that positive attitudes toward science were fostered. Myers’ ABC theory of attitudes posits that attitudes are typically coordinated with emotions, behavioral tendencies, and cognition. Additionally, it suggests that the affective component plays the most pivotal role among the three (Myers, 2010). In order to restore equilibrium among the three components — that is, to achieve a pleasant mood, recognize the significance of a situation, and take action — it is necessary first to alter one’s emotions (Li et al., 2024). In the interviews, complementarity between the pre-class and in-class sessions was identified as a subcategory of the broader theme of supportive environment, which includes other subcategories such as teacher support and peer support. This complementarity led the PSTs to perceive the completion of the pre-class tasks as a beneficial and essential element for the success of the in-class sessions. This belief consequently fostered a sense of responsibility for engaging with the material prior to the in-class sessions, as they recognized the value of having a firm grasp on the material before engaging in the in-class discussions. Upon arrival at the in-class session, PSTs typically demonstrated a satisfactory level of preparation for engagement with the hands-on activities. The in-class period was then utilized by the teacher to provide individualized guidance, addressing specific PSTs’ requirements and reinforcing challenging concepts. Another piece of evidence that supports the notion that the implementation of FCM changed PSTs’ emotional response toward science (e.g., stimulated interest) is the significant impact that the model had on PSTs’ academic achievement. As observed by Li et al. (2024), there is a positive correlation between students’ interest in learning science and their academic achievement in science. It can thus be concluded that the complementarity between the pre-class sessions and in-class sessions, along with teacher support, stimulated PSTs’ interest (affective response), facilitated their understanding of the relevance (cognitive response) of what they were learning, and increased their confidence, thereby fostering a greater sense of investment in their education (behavioral response).
It is important to recognize the limitations of this study. Firstly, the course instructor was an experienced science teacher whose flipped teaching method has been formally recognized as highly effective. Therefore, different results might have been obtained if a less effective teacher had been involved. Secondly, the study was confined to a single general science course within a basic education program at the higher education level, was conducted over a 14-week period, and did not track participants beyond this timeframe—either through the remainder of their teacher preparation program or into their early teaching careers. Consequently, there is no guarantee that the participants’ improved science teaching efficacy beliefs and attitudes toward science will persist once participants begin teaching, given potential influences such as school culture, curriculum constraints, and available professional support. To address these limitations, future research should explore the long-term impact of science teacher training by conducting longitudinal research that follows PSTs into their early teaching years. Comparative studies involving different instructional approaches or instructors with varying levels of experience could also provide insights into how teacher preparation influences self-efficacy and attitudes toward science in diverse educational contexts. Additionally, research examining external factors, such as mentorship programs or school environments, shape the retention of positive attitudes and teaching efficacy over time would be valuable. Such studies would provide a more comprehensive perspective on the enduring impact of high-quality science teacher training programs on self-efficacy and attitudes toward science.
This study contributes to the understanding of how a general science course designed using the FCM can impact PSTs’ self-efficacy beliefs and attitudes toward science.
The results suggest that the FCM is associated with enhanced science teaching self-efficacy beliefs, particularly regarding the PSTE. The observed implementation features of the FCM, in conjunction with teacher support, may have contributed to the activation of various sources of self-efficacy, which are linked to an increase in PSTs’ personal science teaching efficacy. However, the limited field experience of the PSTs may also be related to a sense of uncertainty about their ability to influence student learning outcomes. In the absence of field experience, PSTs may draw upon their academic performance and vicarious experiences as a foundation for their self-efficacy judgments. This underscores the potential for teacher education programs enhanced by FCM to foster robust personal efficacy beliefs, thereby enabling PSTs to excel in science and inspiring their future practice as teachers.
In terms of attitudes toward science, the results suggest that the FCM exerted a significant influence on PSTs’ attitudes toward science, particularly regarding their perceptions of the science teacher. Once again, the role of the teacher as a professional educator, as defined by FLN (2014), with a positive attitude toward science and science teaching, fostered a more productive classroom environment that stimulated PSTs’ interest in science, increased their confidence, and enhanced a greater sense of investment in the learning of science.
These findings have the potential to inform the design of future teacher education programs, and the learning environments experienced by future teachers. As research indicates that PSTs often adopt pedagogical approaches similar to those, they experienced during their own education (Palmer, 2006; Ulukök and Sari, 2016), science courses designed using the FCM can serve as an exemplar for implementing inquiry-based, student-centered, and hands-on approaches while promoting cooperative learning in their own classrooms. This ultimately contributes to the development of pedagogical content knowledge (encompassing inquiry-based teaching and learning pedagogies), the cultivation of ICT proficiency, and a sense of agency and dedication to science education (Marco-Bujosa and Levy, 2016).
The raw data supporting the conclusions of this article will be made available by the authors, without undue reservation.
Written informed consent was obtained from the individual(s) for the publication of any potentially identifiable images or data included in this article.
TR: Conceptualization, Formal analysis, Investigation, Methodology, Writing – original draft, Writing – review & editing. MC: Supervision, Validation, Writing – original draft, Writing – review & editing.
The author(s) declare that financial support was received for the research and/or publication of this article. This research was funded by national funds through FCT—Portuguese Foundation for Science and Technology, I.P., under the scope of: Life Quality Research Centre (CIEQV) (UIDP/04748/2020).
The authors declare that the research was conducted in the absence of any commercial or financial relationships that could be construed as a potential conflict of interest.
The authors declare that Generative AI was used in the creation of this manuscript. Generative AI was used solely to enhance the English language quality of this manuscript, without contributing to its content or ideas.
All claims expressed in this article are solely those of the authors and do not necessarily represent those of their affiliated organizations, or those of the publisher, the editors and the reviewers. Any product that may be evaluated in this article, or claim that may be made by its manufacturer, is not guaranteed or endorsed by the publisher.
Bandura, A. (1977). Self-efficacy: toward a unifying theory of behavioral change. Psychol. Rev. 84, 191–215. doi: 10.1037/0033-295X.84.2.191
Bandura, A. (1986). Social foundations of thought and action: A social cognitive theory. Englewood Cliffs, NJ: Prentice-Hall.
Bergmann, J., and Sams, A. (2012). Flip your classroom: Reach every student in every class every day. First Edn. Washington, DC: International Society for Technology in Education.
Braun, V., and Clarke, V. (2006). Using thematic analysis in psychology. Qual. Res. Psychol. 3, 77–101. doi: 10.1191/1478088706qp063oa
Bursal, M., and Paznokas, L. (2006). Mathematics anxiety and preservice elementary teachers’ confidence to teach mathematics and science. Sch. Sci. Math. 106, 173–180. doi: 10.1111/j.1949-8594.2006.tb18073.x
Cohen, J. (1988). Statistical power analysis for the behavioral sciences. Hillsdale, NJ: Lawrence Erlbaum Associates.
Cohen, J., Cohen, P., West, S. G., and Aiken, L. S. (2003). Applied multiple regression/correlation analysis for the behavioral sciences. 3rd Edn. Mahwah, NJ: Lawrence Erlbaum Associates Publishers.
Coladarci, T. (1992). Teachers’ sense of efficacy and commitment to teaching. J. Exp. Educ. 60, 323–337. doi: 10.1080/00220973.1992.9943869
Creswell, J. W. (2012). Educational research: Planning, conducting and evaluating quantitative and qualitative research. 4th Edn. Boston: Pearson.
Debbağ, M., and Yıldız, S. (2021). Effect of the flipped classroom model on academic achievement and motivation in teacher education. Educ Inf Technol (Dordr) 26, 3057–3076. doi: 10.1007/s10639-020-10395-x
Durak, H. Y., Uslu, N. A., Bilici, S. C., and Güler, B. (2023). Examining the predictors of TPACK for integrated STEM: science teaching self-efficacy, computational thinking, and design thinking. Educ Inf Technol (Dordr) 28, 7927–7954. doi: 10.1007/s10639-022-11505-7
Eagly, A. H., and Chaiken, S. (1993). The psychology of attitudes. Fort Worth, TX: Harcourt Brace Jovanovich.
FLN (2014). The four pillars of F-L-I-P. Available online at: https://flippedlearning.org/definition-of-flipped-learning/ (Accessed August 14, 2024).
Flores, I. M. (2015). Developing preservice teachers’ self-efficacy through field-based science teaching practice with elementary students. Res. High. Educ. J. 17, 1–19.
Fraga, L. M., and Harmon, J. (2014). The flipped classroom model of learning in higher education: an investigation of preservice teachers’ perspectives and achievement. J. Digit. Learn. Teach. Educ. 31, 18–27. doi: 10.1080/21532974.2014.967420
Gogolin, L., and Swartz, F. (1992). A quantitative and qualitative inquiry into the attitudes toward science of nonscience college students. J. Res. Sci. Teach. 29, 487–504. doi: 10.1002/tea.3660290505
González-Gómez, D., Jeong, J. S., and Cañada-Cañada, F. (2022). Enhancing science self-efficacy and attitudes of pre-service teachers (PST) through a flipped classroom learning environment. Interact. Learn. Environ. 30, 896–907. doi: 10.1080/10494820.2019.1696843
Horn, M. B., and Staker, H. (2015). Blended: Using disruptive innovation to improve schools. San Francisco: Jossey-Bass.
Hwang, G.-J., Yin, C., and Chu, H.-C. (2019). The era of flipped learning: promoting active learning and higher order thinking with innovative flipped learning strategies and supporting systems. Interact. Learn. Environ. 27, 991–994. doi: 10.1080/10494820.2019.1667150
Ibourk, A., and Mathis, C. (2024). Developing preservice elementary teachers’ self-efficacy toward teaching science. Int. J. Sci. Educ. 1–24, 1–24. doi: 10.1080/09500693.2024.2347154
Ingram, E., Hill, T. W., Harshbarger, D., and Keshwani, J. (2024). Improving elementary pre-service teachers’ science teaching self-efficacy through garden-based technology integration. Educ. Sci. (Basel) 14:65. doi: 10.3390/educsci14010065
Jeong, J. S., González-Gómez, D., and Cañada-Cañada, F. (2021). How does a flipped classroom course affect the affective domain toward science course? Interact. Learn. Environ. 29, 707–719. doi: 10.1080/10494820.2019.1636079
Lai, H.-M., Hsieh, P.-J., Uden, L., and Yang, C.-H. (2021). A multilevel investigation of factors influencing university students’ behavioral engagement in flipped classrooms. Comput. Educ. 175:104318. doi: 10.1016/j.compedu.2021.104318
Latorre-Cosculluela, C., Suárez, C., Quiroga, S., Anzano-Oto, S., Lira-Rodríguez, E., and Salamanca-Villate, A. (2022). Facilitating self-efficacy in university students: an interactive approach with flipped classroom. Higher Educ. Res. Develop. 41, 1603–1617. doi: 10.1080/07294360.2021.1937067
Lee, Y., and Martin, K. I. (2020). The flipped classroom in ESL teacher education: an example from CALL. Educ. Inf. Technol. 25, 2605–2633. doi: 10.1007/s10639-019-10082-6
Li, Z., Ji, S., Zhang, Y., Du, C., Chen, T., Liu, T., et al. (2024). Associations among science study attitudes, habits and science academic performance of elementary school students: a latent profile approach. Int. J. Sci. Educ. 1–22, 1–22. doi: 10.1080/09500693.2024.2369725
Livingston, K., and Flores, M. A. (2017). Trends in teacher education: a review of papers published in the European journal of teacher education over 40 years. Eur. J. Teach. Educ. 40, 551–560. doi: 10.1080/02619768.2017.1387970
Lo, C. K., and Hew, K. F. (2021). Student engagement in mathematics flipped classrooms: implications of journal publications from 2011 to 2020. Front. Psychol. 12:672610. doi: 10.3389/fpsyg.2021.672610
Marco-Bujosa, L. M., and Levy, A. J. (2016). Caught in the balance: an organizational analysis of science teaching in schools with elementary science specialists. Sci. Educ. 100, 983–1008. doi: 10.1002/sce.21239
Mattern, N., and Schau, C. (2002). Gender differences in science attitude-achievement relationships over time among white middle-school students. J. Res. Sci. Teach. 39, 324–340. doi: 10.1002/tea.10024
McKown, C., and Weinstein, R. S. (2002). Modeling the role of child ethnicity and gender in Children’s differential response to teacher expectations 1. J. Appl. Soc. Psychol. 32, 159–184. doi: 10.1111/j.1559-1816.2002.tb01425.x
Menon, D., and Azam, S. (2021). Investigating preservice teachers’ science teaching self-efficacy: an analysis of reflective practices. Int. J. Sci. Math. Educ. 19, 1587–1607. doi: 10.1007/s10763-020-10131-4
Menon, D., and Sadler, T. D. (2016). Preservice elementary teachers’ science self-efficacy beliefs and science content knowledge. J. Sci. Teach. Educ. 27, 649–673. doi: 10.1007/s10972-016-9479-y
Menon, D., Wieselmann, J. R., Haines, S., and Asim, S. (2024). A Meta-synthesis of the literature on science & engineering teaching self-efficacy: current gaps and future research directions. J. Sci. Teach. Educ. 35, 480–503. doi: 10.1080/1046560X.2023.2297499
Nikitova, I., Kutova, S., Shvets, T., Pasichnyk, O., and Matsko, V. (2020). Flipped learning methodology in professional training of future language teachers. European. J. Educ. Res. 9, 19–31. doi: 10.12973/eu-jer.9.1.19
Oppermann, E., Brunner, M., and Anders, Y. (2019). The interplay between preschool teachers’ science self-efficacy beliefs, their teaching practices, and girls’ and boys’ early science motivation. Learn. Individ. Differ. 70, 86–99. doi: 10.1016/j.lindif.2019.01.006
Osborne, J., Simon, S., and Collins, S. (2003). Attitudes towards science: a review of the literature and its implications. Int. J. Sci. Educ. 25, 1049–1079. doi: 10.1080/0950069032000032199
Palmer, D. H. (2006). Sources of self-efficacy in a science methods course for primary teacher education students. Res. Sci. Educ. 36, 337–353. doi: 10.1007/s11165-005-9007-0
Perera, H. N., Maghsoudlou, A., Miller, C. J., McIlveen, P., Barber, D., Part, R., et al. (2022). Relations of science teaching self-efficacy with instructional practices, student achievement and support, and teacher job satisfaction. Contemp. Educ. Psychol. 69:102041. doi: 10.1016/j.cedpsych.2021.102041
Ribeirinha, T., Alves, R., and Duarte Silva, B. S. (2022). Impacto do modelo Flipped Classroom na experiência de aprendizagem dos alunos em contexto online. Pixel-Bit Revista Medios y Educ. 65, 65–93. doi: 10.12795/pixelbit.93519
Ribeirinha, T., and Silva, B. (2024). O envolvimento do aluno no modelo Flipped Classroom implementado na aprendizagem online. Revista Latinoamericana Tecnología Educativa 23, 43–59. doi: 10.17398/1695-288X.23.1.43
Riegle-Crumb, C., Morton, K., Moore, C., Chimonidou, A., Labrake, C., and Kopp, S. (2015). Do inquiring minds have positive attitudes? The science education of preservice elementary teachers. Sci. Educ. 99, 819–836. doi: 10.1002/sce.21177
Riggs, I. M., and Enochs, L. G. (1990). Toward the development of an elementary teacher’s science teaching efficacy belief instrument. Sci. Educ. 74, 625–637. doi: 10.1002/sce.3730740605
Rittmayer, A. D., and Beier, M. E. B. (2009). “Self-efficacy in STEM” in Applying research to practice (ARP) resources. eds. B. Bogue and E. Cady (Washington, DC: SWE-AWE and NAE CASEE), 3–12.
Saçkes, M., Flevares, L. M., Gonya, J., and Trundle, K. C. (2012). Preservice early childhood teachers’ sense of efficacy for integrating mathematics and science: impact of a methods course. J. Early Child. Teach. Educ. 33, 349–364. doi: 10.1080/10901027.2012.732666
Schruba, A. E. (2008). Evaluation of student attitude toward science and self-efficacy in biology in a non-majors college biology course. Fort Worth, Texas: Texas Christian University.
Shi, Y., Ma, Y., MacLeod, J., and Yang, H. H. (2020). College students’ cognitive learning outcomes in flipped classroom instruction: a meta-analysis of the empirical literature. J. Comput. Educ. 7, 79–103. doi: 10.1007/s40692-019-00142-8
Smith, K. M., Geletta, S., and Duelfer, K. (2020). Flipped classroom in podiatric medical education. J. Am. Podiatr. Med. Assoc. 110, 1–7. doi: 10.7547/19-060
Tosun, T. (2000). The beliefs of preservice elementary teachers toward science and science teaching. Sch. Sci. Math. 100, 374–379. doi: 10.1111/j.1949-8594.2000.tb18179.x
Tschannen-Moran, M., and Johnson, D. (2011). Exploring literacy teachers’ self-efficacy beliefs: potential sources at play. Teach. Teach. Educ. 27, 751–761. doi: 10.1016/j.tate.2010.12.005
Twohill, A., NicMhuirí, S., Harbison, L., and Karakolidis, A. (2023). Primary preservice teachers’ mathematics teaching efficacy beliefs: the role played by mathematics attainment, educational level, preparedness to teach, and gender. Int. J. Sci. Math. Educ. 21, 601–622. doi: 10.1007/s10763-022-10259-5
Ulukök, Ş., and Sari, U. (2016). The effect of simulation-assisted laboratory applications on pre-service teachers’ attitudes towards science teaching. Univ. J. Educ. Res. 4, 465–474. doi: 10.13189/ujer.2016.040301
van Aalderen-Smeets, S. I., and Walma van der Molen, J. H. (2015). Improving primary teachers’ attitudes toward science by attitude-focused professional development. J. Res. Sci. Teach. 52, 710–734. doi: 10.1002/tea.21218
van Alten, D. C. D., Phielix, C., Janssen, J., and Kester, L. (2019). Effects of flipping the classroom on learning outcomes and satisfaction: a meta-analysis. Educ. Res. Rev. 28:100281. doi: 10.1016/j.edurev.2019.05.003
Wilson, K. E., and Hobbs, J. R. (2023). Innovative use of a flipped-classroom approach to teach fundamental nursing skills. Teach. Learn. Nurs. 18, 144–147. doi: 10.1016/j.teln.2022.08.002
Yerdelen, S., Tas, Y., and Osmanoglu, A. (2024). Investigating the change in prospective science teachers’ self-efficacy beliefs and the sources of this change during the teaching practice course. Front. Educ. 9, 1–14. doi: 10.3389/feduc.2024.1427513
Keywords: attitudes toward science, flipped classroom model, pre-service teachers, science education, self-efficacy, teacher education
Citation: Ribeirinha T and Correia M (2025) Enhancing pre-service teachers’ science teaching efficacy beliefs and attitudes toward science using the flipped classroom model. Front. Educ. 10:1512320. doi: 10.3389/feduc.2025.1512320
Received: 16 October 2024; Accepted: 24 March 2025;
Published: 07 April 2025.
Edited by:
Marco Ferreira, Higher Institute of Education and Science (ISEC), PortugalReviewed by:
Elisabetta Conte, University of Bergamo, ItalyCopyright © 2025 Ribeirinha and Correia. This is an open-access article distributed under the terms of the Creative Commons Attribution License (CC BY). The use, distribution or reproduction in other forums is permitted, provided the original author(s) and the copyright owner(s) are credited and that the original publication in this journal is cited, in accordance with accepted academic practice. No use, distribution or reproduction is permitted which does not comply with these terms.
*Correspondence: Marisa Correia, bWFyaXNhLmNvcnJlaWFAZXNlLmlwc2FudGFyZW0ucHQ=
Disclaimer: All claims expressed in this article are solely those of the authors and do not necessarily represent those of their affiliated organizations, or those of the publisher, the editors and the reviewers. Any product that may be evaluated in this article or claim that may be made by its manufacturer is not guaranteed or endorsed by the publisher.
Research integrity at Frontiers
Learn more about the work of our research integrity team to safeguard the quality of each article we publish.