- 1Sky Islands Alliance, Tucson, AZ, United States
- 2Wildlands Network, Salt Lake City, UT, United States
Geopolitical boundaries can present challenges to wildlife conservation because of varying environmental regulations, and increasingly, the existence of border barriers. As of 2024, approximately 1,023 km of border walls (i.e., steel bollard walls 5.5-9.1 m tall with interstitial spaces ≤10 cm) and 169 km of vehicle barriers (i.e., variable steel structures designed to stop vehicles but not pedestrians) exist along the USA-Mexico border. Some small wildlife passages (21.5 x 27.8 cm) were installed in border walls but few other accommodations for wildlife connectivity exist. As such, ecological consequences of border barriers may be severe and documenting the ability of wildlife to traverse these barriers will be essential to conservation efforts. We placed 36 wildlife cameras across 163.5 km of the USA-Mexico border in Arizona, USA and Sonora, MX to evaluate crossing rates through border barriers for 20 terrestrial species. We observed 9,240 wildlife events, including 1,920 successful crossing events. All focal species crossed through vehicle barriers, whereas white-tailed deer, mule deer, American black bear, American badger, wild turkey, and mountain lion appeared unable to cross through interstitial spaces in border walls. Small wildlife passages improved crossing rates for several species, including American badger, collared peccary, coyote, and mountain lion. Yet, small wildlife passages were scarce with only 13 along >130 km of continuous border wall and failed to allow American black bear, deer, and wild turkey to cross. Additional research on the impacts of border barriers and potential mitigation strategies will be critical for effective transboundary conservation.
Introduction
The ability to move is crucial for the survival of nearly all wildlife species. Yet, human development has fragmented natural habitats and created barriers to wildlife movement across much of the Earth’s surface (Barnosky et al., 2012; Cozzi et al., 2013; Davis et al., 2024). The loss of habitat connectivity and landscape permeability can reduce survival of individuals (Coppola et al., 2021), decrease the viability of populations (Fahrig and Merriam, 1994; Olson et al., 2009), suppress gene flow (Aspi et al., 2009; Sawaya et al., 2019), and increase extinction risk (Benson et al., 2016; Clobert, 2012; Gilpin, 1986). Hence, maintaining and increasing habitat connectivity and understanding barriers to movement are vital to conservation efforts (Abrahms et al., 2017; Ford et al., 2020; Heller and Zavaleta, 2009).
Conservation efforts across geopolitical boundaries can be hindered by varying environmental regulations and customs (Rick et al., 2004; Thornton and Branch, 2019). Geopolitical boundaries may also act as physical barriers to conservation and wildlife movement because of the growing presence of infrastructure designed to control the movements of humans and goods. Indeed, construction of border barriers has accelerated globally in recent decades with barriers now present in 74 countries (Vallet, 2022). The ecological impacts of such border barriers can be severe as barriers are often long, continuous, and built with minimal accommodations for wildlife due to security concerns (Aspi et al., 2009; Linnell et al., 2016).
The USA-Mexico border exemplifies the recent proliferation of border barriers (Lasky et al., 2011; McCallum et al., 2014). After the passage of the Secure Fence Act in 2006, 1,053 km of border barriers were built, including 570 km of pedestrian border walls and 483 km of vehicle barriers (Isacson, 2023; US Government Accountability Office, 2017, 2023). Pedestrian border walls (hereafter referred to as, “border walls”) vary but are typically steel bollard walls, constructed with square carbon steel tubing 15 cm wide and 5.5-9.1 m tall. Interstitial spaces between bollards measure ≤10 cm to stop all human crossings. Vehicle barriers are a variable system of steel posts arranged to stop vehicles but not pedestrians (US Government Accountability Office, 2021). Between 2017 and 2020, the USA replaced most vehicle barriers with border walls and built new walls in previously open areas (all border walls built between 2017 and 2020 were 9.1 m tall). As a result, 1,023 km of border walls and 169 km of vehicle barriers exist along the USA-Mexico border in 2024 (US Government Accountability Office, 2023).
Generally, wildlife movements have not been considered a priority when border barriers have been constructed along the USA-Mexico border. On some federal conservation lands (e.g., National Wildlife Refuges, etc.), small wildlife passages measuring roughly 21.5 x 27.8 cm were added to border walls to facilitate movements of endangered species like ocelots (Leopardus pardalis) in south Texas, USA (US Fish and Wildlife Service, 2016). Additionally, floodgates were installed where the border wall crosses arroyos and riverbeds. These gates are opened to accommodate seasonal floods but remain closed most of the year. A settlement in July of 2023 with the Department of Homeland Security will allow select floodgates to remain open longer (Sierra Club v. Biden, 2023), however, most gates remain closed except during high water events. As such, crossing opportunities are limited for wildlife along most of the border wall to the interstitial spaces between bollards, scarce small wildlife passages, and, rarely, open floodgates.
The USA-Mexico border crosses some of the most biologically diverse regions in North America. For example, the Madrean Sky Islands span the USA-Mexico border in southeastern Arizona, USA, and northeastern Sonora, MX. These mountains are recognized as a global biodiversity hotspot and are home to the highest density of bee species in North America (Minckley and Radke, 2021), the highest diversity of mammals, reptiles, and ants in the USA (Warshall, 1994), and one of the highest rates of plant endemism in the USA (McLaughlin, 1994). In its totality, the USA-Mexico border intersects the geographic ranges of 1,077 native animals and bisects important habitat for numerous federally listed species (Peters et al., 2018). Border barriers, especially border walls, may severely limit movements of many of these native animals, including American black bear (Ursus americanus), mountain lion (Puma concolor), desert bighorn sheep (Ovis canadensis nelsoni), mule deer (Odocoileus hemionus), white-tailed deer (O. virginianus), and federally listed species such as jaguar (Panthera onca), Sonoran pronghorn (Antilocapra americana sonoriensis), and Mexican gray wolf (Canis lupus baileyi). Border barriers may hinder recovery efforts for these listed species and threaten other species not currently listed by restricting movement, isolating populations, severing corridors, and limiting gene flow (Chambers et al., 2022; Lasky et al., 2011; Peters et al., 2018).
Presently, land managers and conservation practitioners have insufficient information to manage or mitigate the effects of the USA-Mexico border wall on wildlife populations. Further, the efficacy of small wildlife passages to facilitate movement of wildlife is untested in this region. To address such knowledge gaps, we deployed motion-activated cameras near border barriers along 163.5 km of the USA-Mexico border to: 1) evaluate crossing rates for 20 terrestrial species through border walls and vehicle barriers, and 2) quantify use of the small wildlife passages.
Materials and methods
Field methods
We maintained non-baited, motion-activated cameras (Browning Trail Cameras) at 36 locations spanning 163.5 km of the USA-Mexico border between the Patagonia Mountains in Santa Cruz County, Arizona and the San Bernardino National Wildlife Refuge in Cochise County, Arizona (Figure 1). Three camera locations were in Sonora, MX and 33 locations were in Arizona, USA. We selected camera sites opportunistically across multiple types of border barriers where we were permitted access, could locate a secure mounting structure for the camera with a clear view of the barrier, and could minimize risk of camera theft or disturbance. We maintained 18 cameras facing vehicle barriers, 7 cameras at border walls, and 11 cameras at small wildlife passages (Figures 1, 2). Elevations ranged from 1,140–1,638 m and dominant biotic communities included Madrean evergreen woodlands, plains and great basin grasslands, semidesert grasslands, and Chihuahuan desert-scrub (Brown and Lowe, 1980).
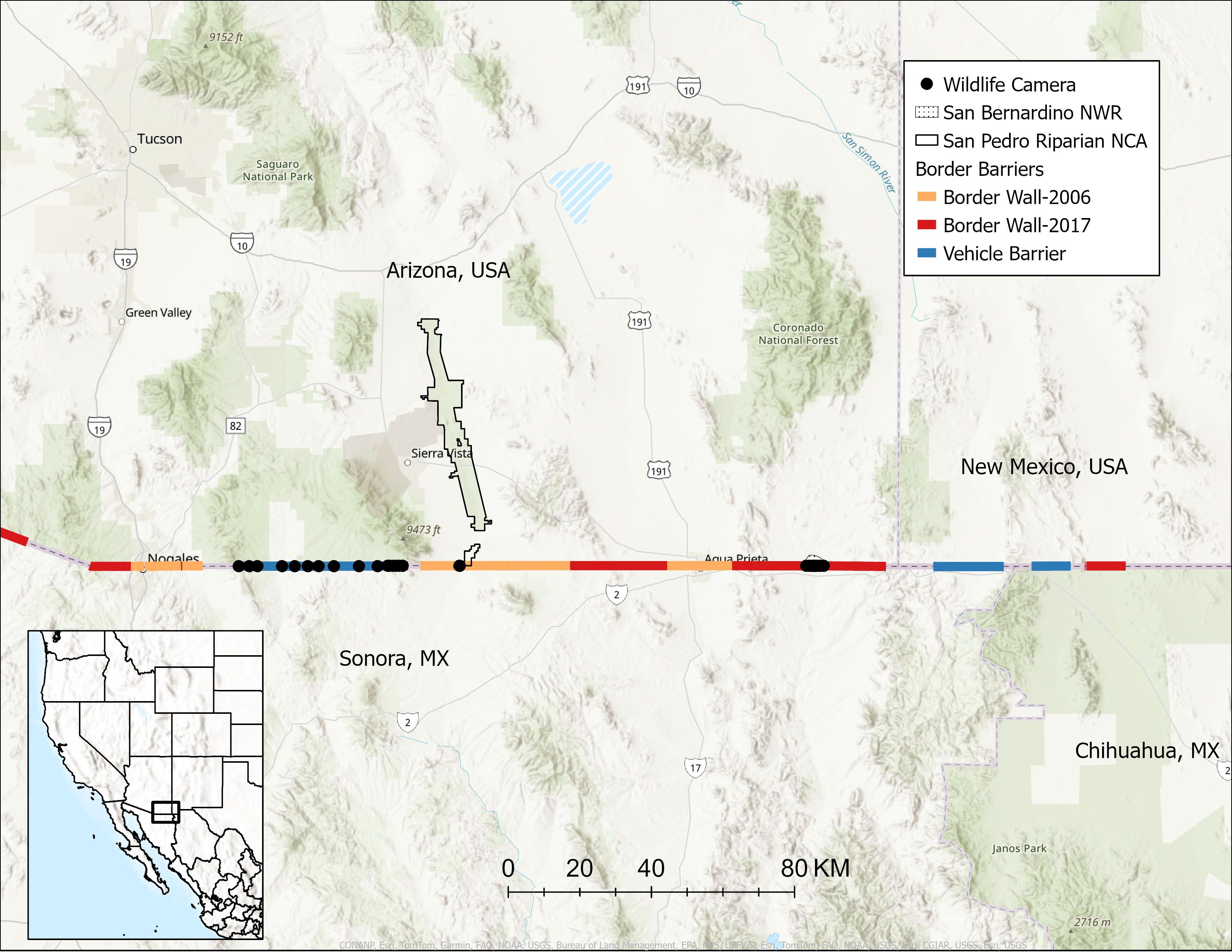
Figure 1. We maintained wildlife cameras at 36 locations along 163.5 km of the USA-Mexico border barrier in southern Arizona, USA and northern Sonora, MX between August 2022 and July 2024. Border walls built between 2006-2010 (Border Wall-2006) measure 5.5 m tall and border walls built after 2017 (Border Wall-2017) are 9.1 m tall, both with interstitial spaces between bollards measuring ≤ 10 cm. NCA is National Conservation Area and NWR is National Wildlife Refuge. At the scale of this map, some camera points represent multiple camera locations.
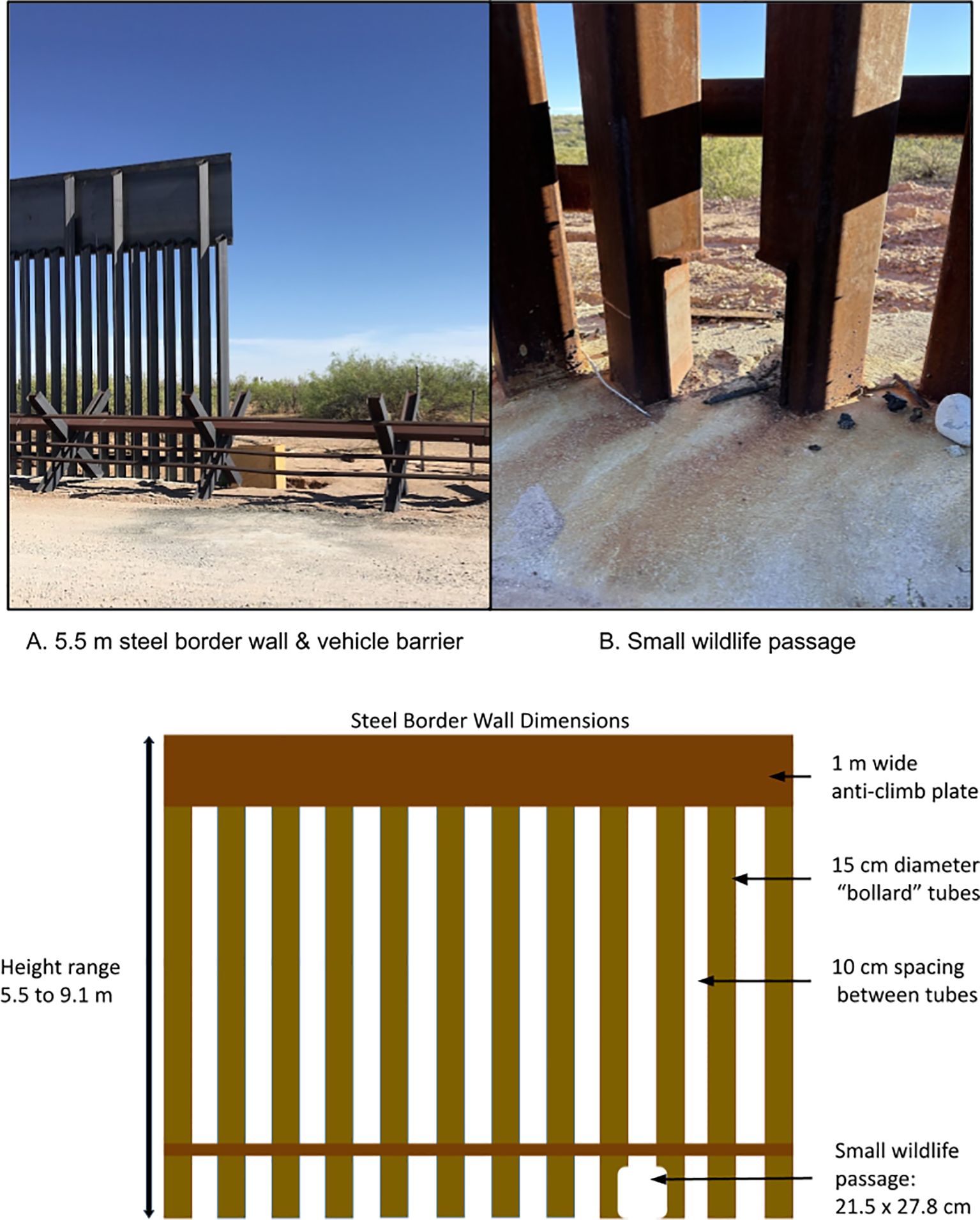
Figure 2. Border barriers in our study consisted of vehicle barriers (foreground in A), border wall between 5.5-9.1 m tall (A), and small wildlife passages (B).
We mounted cameras 1.5–20.0 m from the border barriers and 0.2–1.5 m high based on local conditions (e.g., terrain, vegetation, etc.) to ensure each camera had a clear view of the base of the barrier. We programmed cameras to operate 24 hr/day and capture 20-sec videos with a 1-sec to 30-sec trigger delay to minimize false detections. We used video rather than photographs to better document wildlife behaviors near border barriers. We selected longer trigger delays to preserve battery life and storage on memory cards where vegetation caused excessive false triggers (e.g., in areas with dense shrub cover in the camera viewshed, etc.). We considered terrestrial mammals >1 kg as well as wild turkey (Meleagris gallopavo mexicana) in our analyses of wildlife crossing rates as we hypothesized such species could be hindered by the interstitial spaces of the border walls (Table 1).
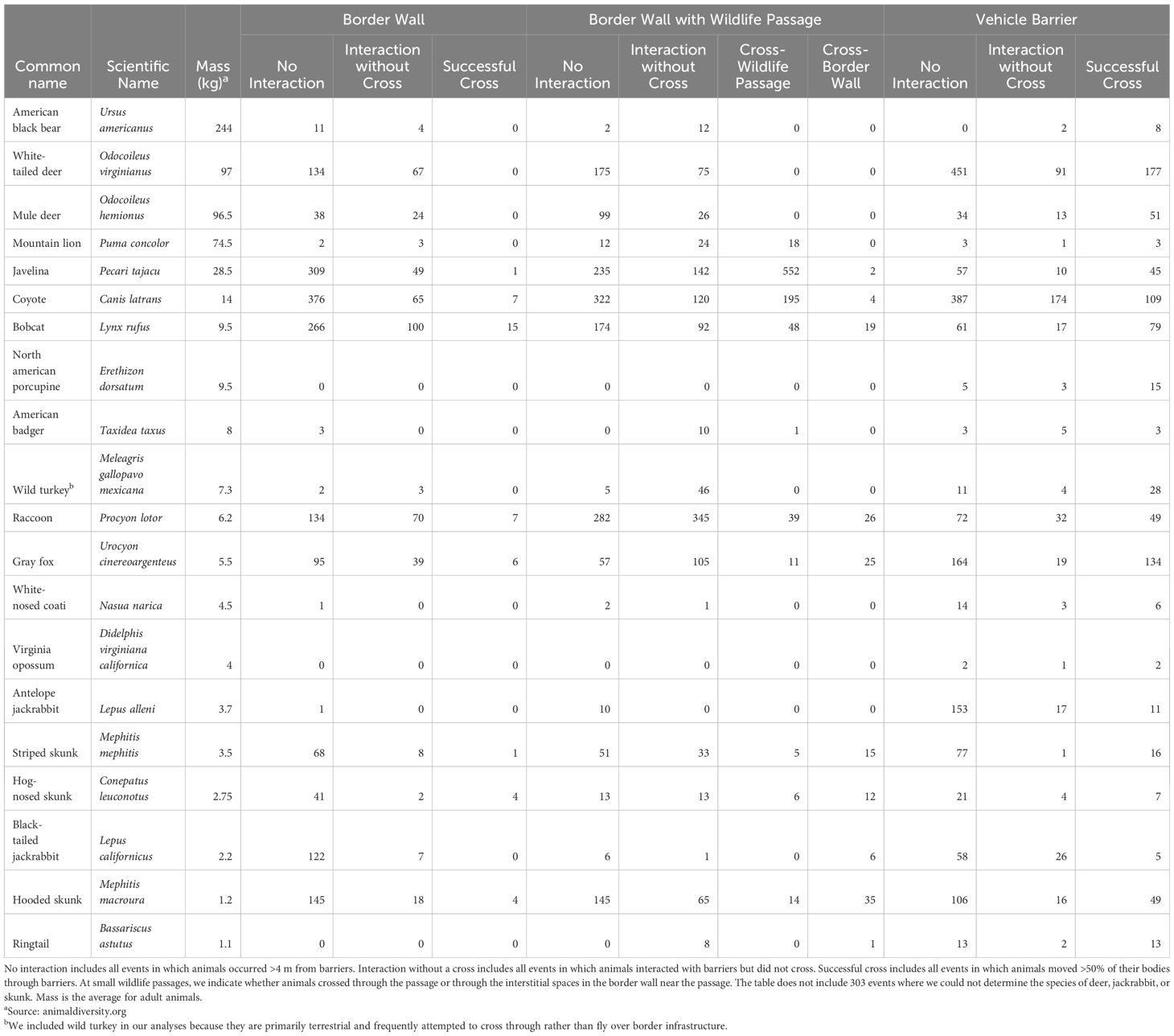
Table 1. Number of events for 19 terrestrial mammals and the wild turkey (Meleagris gallopavo mexicana) at three types of border barriers across 163.5 km of the USA-Mexico border in Arizona between August 2022 and July 2024.
We maintained cameras for 1-23 months between August 2022 and July 2024 and collected memory cards every 4-8 weeks (Supplementary Table S1). Effort varied because of different permitting requirements across land ownership and camera theft. We processed all videos through a multi-step process. First, we sorted all videos into general categories of false triggers (i.e., empty), vehicles, pedestrians, livestock, and wildlife. For terrestrial wildlife >1 kg, we grouped sequential videos of the same species into events, where events began with the first video of an animal and included all subsequent videos within 15 minutes of the initial observation or all videos until a different species occurred (Jones et al., 2020). We then quantified the number of individuals, assigned direction of movement, and categorized behavior relative to the border barrier for each event into three general categories:
● No interaction – animals occur >4 m from, and make no movement towards, the border barrier.
● Successful cross – animals move more than 50% of their body through or under border barrier. We created a threshold of 50% of the body because videos occasionally ended before animals moved 100% of their body through a barrier.
● Interaction without a cross – animals occur within 4 m of the border barrier but do not cross the barrier. We further classified these interactions into the following categories:
• Move parallel – animals move parallel to the barrier.
• Deflect – animals approach and turn roughly 90 degrees (parallel) to move along the barrier.
• Repel – animals approach and turn between 90-180 degrees to move away from the barrier.
• Move perpendicular – animals move towards or away from the border barrier with at least part of the movement within 4 m of the border barrier.
• Investigate – animals actively smell or visually search the border barrier.
We assigned only one behavior to each wildlife event and prioritized crossing behaviors. For example, if an animal spent 15 seconds moving parallel and crossed during the final 5 seconds of the event, we categorized this event as a successful cross. For animals we observed in groups, we assigned direction and behavior based on group majority and noted the number of the group that crossed, if any. Finally, we noted the structure through which the animal crossed if appropriate (i.e., small wildlife passage or interstitial spaces between bollards). For vehicles, pedestrians, livestock, and all non-target wildlife species, we identified species but did not assign movement directions or behaviors.
Statistical analyses
For the purposes of this study, we treated all interactions without a cross (e.g., deflect, move parallel, etc.) as a single category and excluded obvious juveniles (i.e., individuals markedly smaller than adults) from our analyses. We calculated crossing rates as the number of crossing events divided by the total number of barrier interactions (i.e., interactions without a cross and successful crosses). We calculated crossing rates separately for each species, camera, and deployment, where a deployment was defined as the dates between successive camera checks. We then averaged crossing rates across all cameras and deployments for each species and barrier type. We calculated wildlife activity rates for each camera as the number of events divided by the number of days a camera was active. We evaluated differences among barrier types in activity rates and average number of crosses per camera trap-day with Kruskal-Wallis (KW) tests because data were not normally distributed. We established a null hypothesis of no difference between groups (e.g., border wall vs. vehicle barrier) and rejected this null when alpha values ≤0.05. We used R v4.1.2 (R Core Team, 2021) for all analyses and plotted all results with the ggplot2 package (Wickham, 2016). We present mean values and use SE to indicate standard error.
Results
We collected 12,780 videos of the 20 focal species, resulting in 9,240 events. Most events (5,225 of 9,240; 56.6%) involved no interaction with border barriers, whereas 22.7% (2,095 of 9,240) of events featured an animal interacting but not crossing, and 20.8% (1,920 of 9,240) of events featured a successful crossing. We documented one fatality of an adult collared peccary that became stuck between bollards in the border wall and subsequently died from exposure. Coyote was the most common species with 1,759 events, followed by collared peccary (n = 1,402), and white-tailed deer (n = 1,170; Table 1). We documented all 20 focal species at vehicle barriers, 90% (18 of 20) at small wildlife passages, and 85% (17 of 20) at border walls. When considering all focal species together, average daily activity (as number of barrier interactions per camera trap-day) varied significantly by barrier type (KW2 = 20.7, p = <0.001) as did the average number of crosses per camera trap-day (KW2 = 50.2, p = <0.001). Specifically, average daily activity was 3.2 times higher near small wildlife passages (µ = 0.60, SE = 0.08) than at border walls (µ = 0.19, SE = 0.03) and the average number of crosses per camera trap-day was 16.7 times greater at small wildlife passages (µ = 0.25, SE = 0.05) than at border walls (µ = 0.015, SE = 0.004; Supplementary Table S1).
Of the focal species we documented at each barrier, 55.6% (10 of 18) crossed through small wildlife passages, 58.8% (10 of 17) crossed through the interstitial spaces in the border wall, and 100% (20 of 20) crossed through the vehicle barrier (Table 1). Within species, crossing rates were consistently highest at vehicle barriers and lowest at border walls (Figure 3). This was evident even for smaller species (e.g., skunk species, bobcat, etc.) that could easily cross through interstitial spaces in the border wall. For example, bobcats showed no physical limitations when crossing through interstitial spaces of border walls but were still 5.1 times more likely to cross through vehicle barriers than border walls (0.82, SE = 0.06 vs 0.16, SE = 0.05). Although many relatively small species were able to cross through all border barriers, we did not observe larger species crossing through border walls. Indeed, white-tailed deer, mule deer, American black bear, American badger, wild turkey, and mountain lion never successfully crossed (crossing rate = 0.0) through interstitial spaces in the border wall but crossed during >50% of events at vehicle barriers (crossing rates of 0.5-0.79).
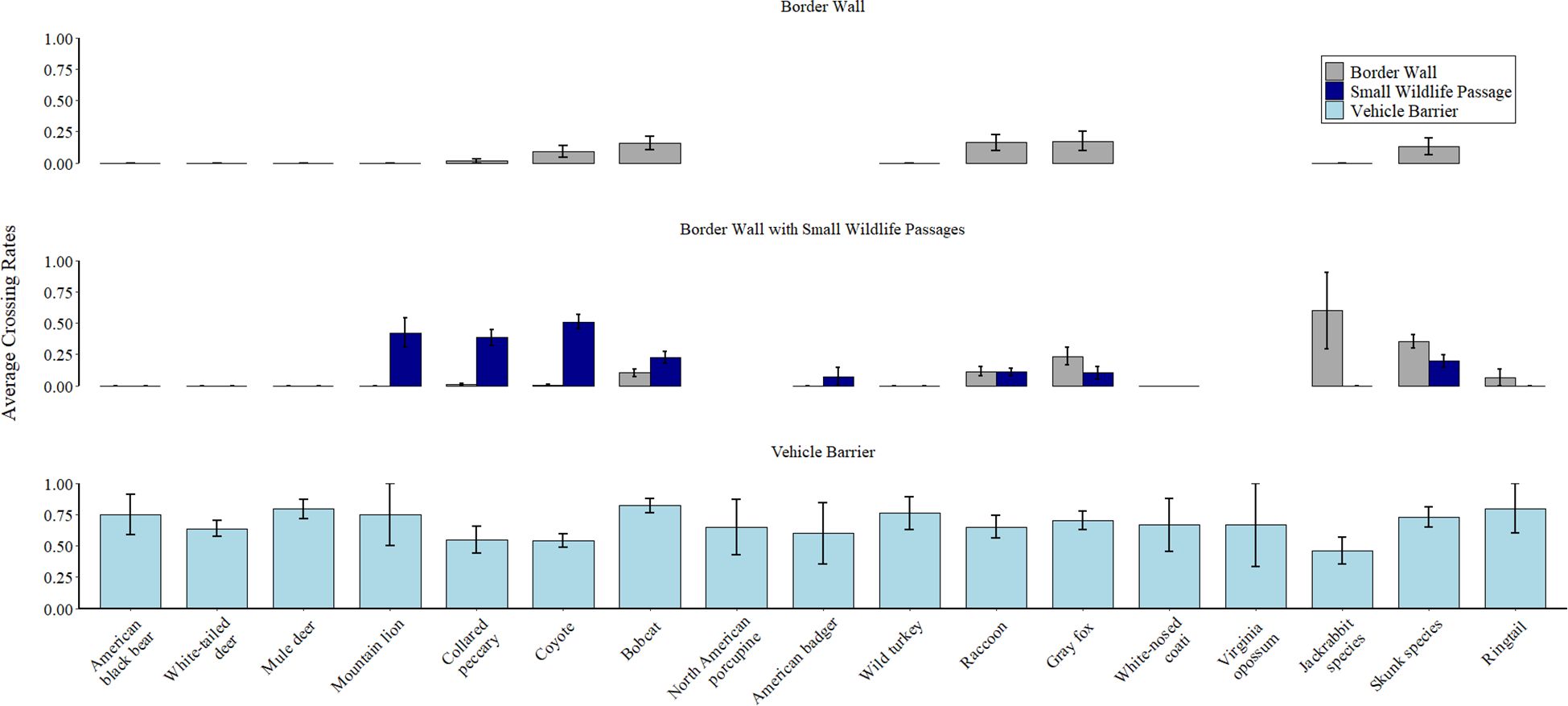
Figure 3. Average crossing rates for focal species in our study along 163.5 km of the USA-Mexico border between August 2022-July 2024. We calculated crossing rate as the number of crosses divided by the number of barrier interactions for each species. We averaged crossing rates across all cameras within each barrier type. Species are sorted in descending order by average mass from left to right. We grouped antelope and black-tailed jackrabbits as “Jackrabbit species” and hooded skunk, striped skunk, and hog-nosed skunk as “Skunk species” for sample size considerations. Gaps indicate the species was not observed at the barrier type (e.g., ringtail was not observed on cameras facing border walls without small wildlife passages). For cameras facing small wildlife passages, we categorized crosses as either through the passages or through the interstitial spaces between the bollards adjacent to the passage (indicated as Border Wall crossing rates in the second row). Error bars represent 95% confidence intervals. A library of example videos from this study can be found at https://skyislandalliance.imagerelay.com/fl/4c85eaa9e1c34762be6f476391ec9c30.
Small wildlife passages benefited larger-bodied species than we expected. Indeed, the crossing rate of collared peccary (average mass = 28.5 kg) was 24 times greater through small wildlife passages than through interstitial spaces in the border wall (0.38, SE = 0.06 vs 0.016, SE = 0.016; Figure 3). Furthermore, mountain lions (average mass = 74.5 kg) never crossed through interstitial spaces in border walls but had a crossing rate of 0.42, SE = 0.12 at small wildlife passages (Figure 3). However, small wildlife passages did not offer any crossing opportunities for white-tailed deer, mule deer, American black bear, or wild turkey, despite many interactions from these species near the passages. Smaller species such as gray fox, northern raccoon, and skunks had similar crossing rates through interstitial spaces in the border wall and small wildlife passages. Finally, we did not observe a single crossing event by a human through any border barrier.
Discussion
We present a novel assessment of crossing rates for 20 terrestrial wildlife species through barriers across 163.5 km of the USA-Mexico border. We demonstrate that several large terrestrial species are unable to cross through border walls that now bisect some of the most biodiverse regions of North America and bound approximately 1,023 km of the USA-Mexico border. Moreover, we evaluate for the first time the efficacy of the small wildlife passages and show that they are used frequently by several species. Nevertheless, these small openings are inadequate for large carnivores and ungulates that may have the greatest need to move to find mates, secure resources, or avoid competition. Additional research efforts such as this study are needed to elucidate the ecological consequences of border barriers and potential mitigation strategies.
We observed 10 focal species crossing through the small wildlife passages including two species apparently unable or unwilling to cross through interstitial spaces in the border wall (i.e., mountain lion and American badger) and two species unlikely to cross through interstitial spaces (i.e., collared peccary and coyote). However, only 13 small wildlife passages exist in our study area, with 8 distributed across 6.3 km near the San Bernardino National Wildlife Refuge and 5 distributed across 0.5 km at the San Pedro Riparian National Conservation Area. These clusters of openings are separated by 95 km of continuous border wall, thereby limiting their utility to individuals that do not reside near one of these conservation areas. Moreover, current small wildlife passages measure only 21.5 x 27.8 cm (roughly the size of a piece of A4 printer paper) and provided no crossing opportunities for American black bear, white-tailed deer, mule deer or wild turkey in our study. It is unlikely existing passages would provide crossing opportunities for similarly sized mammals of conservation concern in the area including pronghorn, desert bighorn sheep, jaguar, and Mexican gray wolves. The latter two species are larger than mountain lions and coyotes we observed crossing through the small wildlife passages (De La Torre and Rivero, 2017), and probably would not fit through existing passages. Gaps between constructed sections of border walls remain in some regions of the border (Traphagen, 2021), but the border wall section we monitored is uninterrupted for 130.2 km (Figure 1). Beyond the 13 small wildlife passages, no permanent openings exist for wildlife along this entire stretch of wall. More small wildlife passages should be installed (e.g., every 5-10 m) and different sized passages should be considered to more meaningfully mitigate the impacts of the border wall on wildlife connectivity.
The impacts of the border wall may manifest at multiple levels. At the demographic level, border walls may affect survival and reproductive rates by impeding access to resources and mating opportunities (Cozzi et al., 2013; Hering et al., 2022; Safner et al., 2021). At the population level, border walls may limit dispersal, thereby increasing isolation and decreasing viability of subpopulations (Flesch et al., 2010). For example, American black bears disperse regularly between mountain ranges in the USA and Mexico, and genetic analyses show populations in southeastern Arizona are closely connected with those of northern Sonora, Mexico (Atwood et al., 2011; Gould et al., 2022; Lara-Díaz et al., 2013; Varas et al., 2010). However, our data indicate that the border wall is mostly impermeable for American black bears and will thus hinder their dispersal between the USA and Mexico, where they are listed as federally endangered (SEMARNAT, 2010). Border walls could thus threaten the population viability of American black bears in Mexico. Border walls may also influence community composition and structure by excluding some species but not others from adjacent habitats. For example, many mesocarnivores (e.g., bobcat, gray fox, etc.) had relatively high crossing rates through the border walls, whereas large carnivores were effectively excluded. Finally, climate change is driving the distribution of many species to higher latitudes and elevations (Chen et al., 2011; Thornton and Branch, 2019). The presence of largely impermeable barriers along 1,023 km of the USA-Mexico border may hinder the ability of many species to reach climate refuges, thereby increasing their vulnerability to such changes (Chambers et al., 2022; Davis et al., 2024; Egan et al., 2018).
Other factors associated with the border barriers may influence crossing rates and warrant further research. For example, we considered only the barriers themselves when evaluating crossing rates and use of the small wildlife passages. Border barriers are often accompanied by parallel roads (10-18 m wide in our study area) and a network of access roads with varying levels of law enforcement and maintenance traffic (Sayre and Knight, 2010; US Government Accountability Office, 2021). Moreover, some areas of the USA-Mexico border have high-intensity security lighting that illuminates the barriers (US Government Accountability Office, 2021). We did not include lighting in our analyses because lighting infrastructure has been installed but not activated in our study area. Furthermore, landscape characteristics (e.g., terrain) and vegetation conditions (e.g., vegetation cover adjacent to border barriers) probably affect wildlife occurrence and crossing rates. Future work should evaluate the influence of human activity, additional security infrastructure such as lighting (including for taxonomic groups like birds, bats, and insects that may not be as directly affected by border barriers), and environmental covariates on wildlife crossing rates through border barriers. Additionally, we considered all non-crossing interactions as a single category in our analyses. However, further insights into the impacts of the border barriers could be gained by evaluating non-crossing interactions in greater detail. Finally, we evaluated crossing rates of 20 wildlife species, but many other species may be impacted by border barriers that we could not evaluate because they did not occur in our study area (e.g., peninsular bighorn sheep, Mexican gray wolf, etc.) or because we did not detect them on our cameras, such as desert tortoise (Gopherus agassizii), pronghorn, ocelot, jaguar, and ferruginous pygmy owl (Glaucidium brasilianum; Flesch et al., 2010). The impacts of border barriers on these species should not be overlooked.
Approximately 1,023 km of the USA-Mexico border (including 63% of the southern border of Arizona) is now bounded by border walls 5.5-9.1 m tall with interstitial spaces between bollards measuring ≤10 cm. Our study is among the first to explicitly evaluate the permeability of these barriers for terrestrial species along the USA-Mexico border. Our results indicate border walls are largely impermeable to many terrestrial mammals, including several federally listed species in the USA and MX. We further demonstrate that existing wildlife passages have the potential to improve crossing rates for some species, but more passages (including larger openings) are needed. Our work may help conservation practitioners and land managers to understand the impacts of current border barriers on wildlife movement and improve design and distribution of wildlife passages. As construction of border barriers accelerates globally, research into the full extent of ecological consequences of these barriers and effective mitigation strategies will be paramount for transboundary conservation efforts throughout the world.
Data availability statement
The original contributions presented in the study are included in the article/Supplementary Material. Further inquiries can be directed to the corresponding author.
Author contributions
EJH: Conceptualization, Data curation, Formal Analysis, Investigation, Methodology, Project administration, Visualization, Writing – original draft, Writing – review & editing. MT: Conceptualization, Investigation, Methodology, Writing – review & editing. MB: Data curation, Investigation, Writing – review & editing. ANF: Investigation, Formal Analysis, Methodology, Writing – review & editing. MD: Writing – review & editing, Funding acquisition, Resources. EB: Funding acquisition, Resources, Writing – review & editing, Investigation, Methodology, Project administration, Conceptualization.
Funding
The author(s) declare financial support was received for the research, authorship, and/or publication of this article. This research was completed in compliance with the following permits: US Forest Service Special Use Permit #SUP2349, US Fish & Wildlife Service Permit #SBSUP24-04, Bureau of Land Management Research Permit #20221221, Arizona State Parks and Trails Commercial Rental Permit for Research and Monitoring #2020-00, and the National Park Service Special Use Permit #CORO-0051. Funding was provided by the Wilburforce Foundation (Grants #SKY12211A and #SKY12311A), Carroll Petrie Foundation (Grants #2022-106 and #2023-132), New York Community Trust (Grant #P21-000540), Clif Family Foundation (Grant #72606543), Brabson Family Foundation, the Wilderness Society, Arizona Community Foundation of Cochise (Grant #31014915), Dr. Scholl Foundation, National Park Service Southwest Border Resource Project Program (Grant #P22AC00512-00), and many individual supporters.
Acknowledgments
Miguel Enriquez Galaz, Oskar Anderson, McKinsey Tighe, Chloe Atkins, Jakelynn Wert, and McKenna Carter assisted with data processing. Bryon Lichtenhan, Brit Rosso, Elizabeth Taylor, John Nametz, and many volunteers assisted with camera maintenance. We received logistical support from San Bernardino National Wildlife Refuge and Cuenca Los Ojos, A.C. Associate editor (M. Di Febbraro) and two reviewers provided valuable feedback to improve a previous version of this manuscript. Charlie Turner provided technical assistance with data management and Dan Morris assisted with adapting MegaDetector for video sorting.
Conflict of interest
The authors declare that the research was conducted in the absence of any commercial or financial relationships that could be construed as a potential conflict of interest.
Publisher’s note
All claims expressed in this article are solely those of the authors and do not necessarily represent those of their affiliated organizations, or those of the publisher, the editors and the reviewers. Any product that may be evaluated in this article, or claim that may be made by its manufacturer, is not guaranteed or endorsed by the publisher.
Supplementary material
The Supplementary Material for this article can be found online at: https://www.frontiersin.org/articles/10.3389/fevo.2024.1487911/full#supplementary-material
References
Abrahms B., Sawyer S. C., Jordan N. R., McNutt J. W., Wilson A. M., Brashares J. S. (2017). Does wildlife resource selection accurately inform corridor conservation? J. Appl. Ecol. 54, 412–422. doi: 10.1111/1365-2664.12714
Aspi J., Roininen E., Kiiskilä J., Ruokonen M., Kojola I., Bljudnik L., et al. (2009). Genetic structure of the northwestern Russian wolf populations and gene flow between Russia and Finland. Conserv. Genet. 10, 815–826. doi: 10.1007/s10592-008-9642-x
Atwood T. C., Young J. K., Beckmann J. P., Breck S. W., Fike J., Rhodes O. E., et al. (2011). Modeling connectivity of black bears in a desert sky island archipelago. Biol. Conserv. 144, 2851–2862. doi: 10.1016/j.biocon.2011.08.002
Barnosky A. D., Hadly E. A., Bascompte J., Berlow E. L., Brown J. H., Fortelius M., et al. (2012). Approaching a state shift in Earth’s biosphere. Nature 486, 52–58. doi: 10.1038/nature11018
Benson J. F., Mahoney P. J., Sikich J. A., Serieys L. E. K., Pollinger J. P., Ernest H. B., et al. (2016). Interactions between demography, genetics, and landscape connectivity increase extinction probability for a small population of large carnivores in a major metropolitan area. Proc. R. Soc. B: Biol. Sci. 283, 20160957. doi: 10.1098/rspb.2016.0957
Brown D. E., Lowe C. H. Jr. (1980). Biotic communities of the southwest (Salt Lake City, UT: USDA Forest Service and University of Utah).
Chambers S. N., Villarreal M. L., Norman L. M., Bravo J. C., Traphagen M. B. (2022). Spatial models of jaguar energy expenditure in response to border wall construction and remediation. Front. Conserv. Sci. 3. doi: 10.3389/fcosc.2022
Chen I.-C., Hill J. K., Ohlemüller R., Roy D. B., Thomas C. D. (2011). Rapid range shifts of species associated with high levels of climate warming. Science 333, 1024–1026. doi: 10.1126/science.1206432
Clobert J. (2012). Dispersal ecology and evolution (Oxford, United Kingdom: Oxford University Press).
Coppola P. M., Williams C. K., Terhune T. M., Parke J., Cecil J. (2021). Landscape connectivity influences survival and resource use following long-distance translocation of northern bobwhite. J. Wildlife Manage. 85, 369–383. doi: 10.1002/jwmg.21975
Cozzi G., Broekhuis F., Mcnutt J. W., Schmid B. (2013). Comparison of the effects of artificial and natural barriers on large African carnivores: Implications for interspecific relationships and connectivity. J. Anim. Ecol. 82, 707–715. doi: 10.1111/1365-2656.12039
Davis F., Szopa-Comley A., Rouse S., Caromel A., Arnell A., Basrur S., et al. (2024). “State of the world’s migratory species,” in Convention on the Conservation of Migratory Species of Wild Animals. (Cambridge, United Kingdom: UNEP-WCMC). Available online at: www.unep-wcmc.org. State of the World’s Migratory Species.
De La Torre J. A., Rivero M. (2017). A morphological comparison of jaguars and pumas in southern Mexico. Therya 8, 117–122. doi: 10.12933/therya-17-456
Egan S. P., Comerford M., Hood G. R., Zhang L., Weinersmith K. L. (2018). Borders on the rise: the unintended evolutionary consequences of border barriers on natural populations (Houston, TX: James A. Baker III Institute for Public Policy. Rice University).
Fahrig L., Merriam G. (1994). Conservation of fragmented populations. Conserv. Biol. 8, 50–59. doi: 10.1046/j.1523-1739.1994.08010050.x
Flesch A. D., Epps C. W., Cain J. W., Clark M., Krausman P. R., Morgart J. R. (2010). Potential effects of the United States-Mexico border fence on wildlife: Contributed paper. Conserv. Biol. 24, 171–181. doi: 10.1111/j.1523-1739.2009.01277.x
Ford A. T., Sunter E. J., Fauvelle C., Bradshaw J. L., Ford B., Hutchen J., et al. (2020). Effective corridor width: linking the spatial ecology of wildlife with land use policy. Eur. J. Wildlife Res. 66, 69. doi: 10.1007/s10344-020-01385-y
Gilpin M. E. (1986). Minimum viable populations: processes of species extinction. Conserv. Biology: Sci. Scarcity Diversity, 19–34.
Gould M. J., Cain J. W., Atwood T. C., Harding L. E., Johnson H. E., Onorato D. P., et al. (2022). Pleistocene–Holocene vicariance, not Anthropocene landscape change, explains the genetic structure of American black bear (Ursus americanus) populations in the American Southwest and northern Mexico. Ecol. Evol. 12, e9406. doi: 10.1002/ece3.9406
Heller N. E., Zavaleta E. S. (2009). Biodiversity management in the face of climate change: A review of 22 years of recommendations. Biol. Conserv. 142, 14–32. doi: 10.1016/j.biocon.2008.10.006
Hering R., Hauptfleisch M., Kramer-SChadt S., Stiegler J., Blaum N. (2022). Effects of fences and fence gaps on the movement behavior of three southern African antelope species. Front. Conserv. Sci. 3. doi: 10.3389/fcosc.2022.959423
Isacson A. (2023). US-Mexico border barriers now total 741 miles https://adamisacson.com/u-s-Mexico-border-barriers-now-total-741-miles/. September 10, 2023.
Jones P. F., Jakes A. F., MacDonald A. M., Hanlon J. A., Eacker D. R., Martin B. H., et al. (2020). Evaluating responses by sympatric ungulates to fence modifications across the northern great plains. Wildlife Soc. Bull. 44, 130–141. doi: 10.1002/wsb.1067
Lara-Díaz N. E., López-González C. A., Coronel-Arellano H., González-Bernal A. (2013). “Black bear population and connectivity in the Sky Islands of Mexico and the United States,” in Merging Science and Management in a Rapidly Changing World. Eds. Gottfried G. J., Ffolliott, Peter F., Gebow, Brooke S., Eskew, Lane G., Collins L. C. (US Department of Agriculture, Forest Service, Rocky Mountain Research Station, Fort Collins, CO, USA). Biodiversity and management of the Madrean Archipelago III and 7th Conference on Research and Resource Management in the Southwestern Deserts; 2012 May 1-5; Tucson, AZ. Proceedings. RMRS-P-67.
Lasky J. R., Jetz W., Keitt T. H. (2011). Conservation biogeography of the US-Mexico border: A transcontinental risk assessment of barriers to animal dispersal. Diversity Distributions 17, 673–687. doi: 10.1111/j.1472-4642.2011.00765.x
Linnell J. D. C., Trouwborst A., Boitani L., Kaczensky P., Huber D., Reljic S., et al. (2016). Border security fencing and wildlife: the end of the transboundary paradigm in Eurasia? PloS Biol. 14, e1002483. doi: 10.1371/journal.pbio.1002483
McCallum J. W., Rowcliffe J. M., Cuthill I. C. (2014). Conservation on international boundaries: The impact of security barriers on selected terrestrial mammals in four protected areas in Arizona, USA. PloS One 9, e93679. doi: 10.1371/journal.pone.0093679
McLaughlin S. P. (1994). “An overview of the flora of the Sky Islands, southeastern Arizona: Diversity, affinities, and insularity,” in Biodiversity and Management of the Madrean Archipelago: The Sky Islands of the Southwestern United States and Northwestern Mexico. Eds. DeBano L., Ffolliott P. F., Ortega-Rubio A., Gottfried G. J., Hamre R. H., Edminster C. B. (US Department of Agriculture, Fort Collins, CO, USA), Pp. 6–Pp.18.
Minckley R. L., Radke W. R. (2021). Extreme species density of bees (Apiformes, Hymenoptera) in the warm deserts of North America. J. Hymenoptera Res. 82, 317–345. doi: 10.3897/JHR.82.60895
Olson K. A., Mueller T., Leimgruber P., Nicolson C., Fuller T. K., Bolortsetseg S., et al. (2009). Fences impede long-distance Mongolian gazelle (Procapra gutturosa) movements in drought-stricken landscapes. Mongolian J. Biol. Sci. 7, (1–2). doi: 10.22353/mjbs.2009.07.07
Peters R., Ripple W. J., Wolf C., Moskwik M., Carreón-Arroyo G., Ceballos G., et al. (2018). Nature divided, scientists united: US–Mexico border wall threatens biodiversity and binational conservation. BioScience 68, 740–743. doi: 10.1093/biosci/biy063
R Core Team (2021). R: A language and environment for statistical computing (Vienna, Austria: R Foundation for Statistical Computing). Available at: https://www.R-project.org/.
Rick D., Schoik V., Lelea E., Cunningham J. (2004). “Sovereignty, borders, and transboundary biodiversity: turning a potential tragedy into a true partnership an introduction to the theory and practice of conservation biology in the security bisected US-Mexican border region,” in The US-Mexican Border Environment: Transboundary Ecosystem Management. Eds. Hoffman K.. (San Diego, CA, USA: San Diego State University Press).
Safner T., Gracanin A., Gligora I., Pokorny B., Flajšman K., Apollonio M., et al. (2021). State border fences as a threat to habitat connectivity: a case study from south-eastern Europe. Sumarski List 145, 269–278. doi: 10.31298/SL.145.5-6.6
Sawaya M. A., Clevenger A. P., Schwartz M. K. (2019). Demographic fragmentation of a protected wolverine population bisected by a major transportation corridor. Biol. Conserv. 236, 616–625. doi: 10.1016/j.biocon.2019.06.030
Sayre N. F., Knight R. L. (2010). Potential effects of United States-Mexico border hardening on ecological and human communities in the Malpai borderlands. Conserv. Biol. 24, 345–348. doi: 10.1111/j.1523-1739.2009.01381.x
SEMARNAT (2010). Norma Oficial Mexicana NOM-059-SEMARNAT-2010, Protección ambiental-Especies nativas de México de flora y fauna silvestres - Categorías de riesgo especificaciones para su inclusión, exclusión o cambio- lista de especies en riesgo. Diario Oficial de la Federación, 1.
Thornton D. H., Branch L. C. (2019). Transboundary mammals in the Americas: Asymmetries in protection challenge climate change resilience. Diversity Distributions 25, 674–683. doi: 10.1111/ddi.12880
Traphagen M. (2021). The border wall in Arizona and New Mexico. Available online at: https://storymaps.arcgis.com/stories/8532c503c2084293bb8847407245228d (Accessed May 1, 2024).
US Fish and Wildlife Service (2016). Recovery plan for the Ocelot (Leopardus pardalis), first revision Vol. 217 (Albuquerque, NM, USA: US Fish and Wildlife Service Southwest Region Albuquerque).
US Government Accountability Office (2017). Additional actions needed to better assess fencing's contributions to operations and provide guidance for identifying capability gaps. Available online at: https://www.gao.gov/assets/gao-17-331.pdf (Accessed May 1, 2024).
US Government Accountability Office (2021). Schedule considerations drove army corps of engineers' approaches to awarding construction contracts through 2020. Available online at: https://www.gao.gov/assets/gao-21-372.pdf (Accessed May 1, 2024).
US Government Accountability Office (2023). Southwest border: cultural and natural resource impacts from barrier construction. Available online at: https://www.gao.gov/assets/d24107127.pdf (Accessed May 1, 2024).
Vallet E. (2022). The world is witnessing a rapid proliferation of border walls (Washington, DC, USA: Migration Policy Institute). Available at: https://www.migrationpolicy.org/article/rapid-proliferation-number-border-walls.
Varas C., López-González C. A., Ramirez J., Krausman P., Culver M., Halvorson W., et al. (2010). “Conservation genetics of black bears in the Sky Islands of Arizona and Northern México,” in Southwestern Desert Resources (University of Arizona Press, Tucson, AZ, USA), 275–281.
Warshall P. (1994). “The Madrean sky island archipelago: A planetary overview,” in Biodiversity and Management of the Madrean Archipelago: The Sky Islands of the Southwestern United States and Northwestern Mexico. Eds. DeBano L., Ffolliott P. F., Ortega-Rubio A., Gottfried G. J., Hamre R. H., Edminster C. B. (US Department of Agriculture, Fort Collins, CO, USA), pp 6–pp18.
Keywords: border wall, border barrier, connectivity, wildlife crossing, movement, transboundary conservation
Citation: Harrity EJ, Traphagen M, Bethel M, Facka AN, Dax M and Burns E (2024) USA-Mexico border wall impedes wildlife movement. Front. Ecol. Evol. 12:1487911. doi: 10.3389/fevo.2024.1487911
Received: 29 August 2024; Accepted: 30 October 2024;
Published: 21 November 2024.
Edited by:
Mirko Di Febbraro, University of Molise, ItalyReviewed by:
Rafael Carballeira, University of Valencia, SpainErin Koen, Cherokee Nation System Solutions, United States
Copyright © 2024 Harrity, Traphagen, Bethel, Facka, Dax and Burns. This is an open-access article distributed under the terms of the Creative Commons Attribution License (CC BY). The use, distribution or reproduction in other forums is permitted, provided the original author(s) and the copyright owner(s) are credited and that the original publication in this journal is cited, in accordance with accepted academic practice. No use, distribution or reproduction is permitted which does not comply with these terms.
*Correspondence: Eamon J. Harrity, ZWFtb25Ac2t5aXNsYW5kYWxsaWFuY2Uub3Jn