- 1U.S. Geological Survey, Fort Collins Science Center, Fort Collins, CO, United States
- 2Biology Department, Colorado State University, Fort Collins, CO, United States
- 3Department of Environmental Sciences, Western Washington University, Bellingham, WA, United States
- 4Yurok Tribe, Revegetation and Monitoring Branch, Fisheries Technical Services Program, Klamath, CA, United States
- 5Department of Biology, Eastern Washington University, Cheney, WA, United States
Large dam removal can trigger changes to physical and biological processes that influence vegetation dynamics in former reservoirs, along river corridors downstream of former dams, and at a river’s terminus in deltas and estuaries. We present the first comprehensive review of vegetation response to major fluvial disturbance caused by the world’s largest dam removal. After being in place for nearly a century, two large dams were removed along the Elwha River, Washington, USA, between 2011 and 2014. The exposure, erosion, transport, and deposition of large volumes of sediment and large wood that were impounded behind the dams created new fluvial surfaces where plant colonization and growth have occurred. In the former reservoirs, dam removal exposed ~290 ha of unvegetated sediment distributed on three main landforms: valley walls, high terraces, and dynamic floodplains. In addition to natural revegetation in the former reservoirs, weed control and seeding and planting of desirable plants influenced vegetation trajectories. In early years following dam removal, ~20.5 Mt of trapped sediment were eroded from the former reservoirs and transported downstream. This sediment pulse, in combination with transport of large wood, led to channel widening, an increase in gravel bars, and floodplain deposition. The primary vegetation responses along the river corridor were a reduction in vegetated area associated with channel widening, plant establishment on new gravel bars, increased hydrochory, and altered plant community composition on gravel bars and floodplains. Plant species diversity increased in some river segments. In the delta, sediment deposition led to the creation of ~26.8 ha of new land surfaces and altered the distribution and dynamics of intertidal water bodies. Vegetation colonized ~16.4 ha of new surfaces: mixed pioneer vegetation colonized supratidal beach, river bars, and river mouth bars, and emergent marsh vegetation colonized intertidal aquatic habitats. In addition to the sediment-dominated processes that have created opportunities for plant colonization and growth, biological processes such as restored hydrochory and anadromous fish passage with associated delivery of marine-derived nutrients may influence vegetation dynamics over time. Rapid changes to landforms and vegetation growth were related to the large sediment pulse in the early years following dam removal, and the rate of change is expected to attenuate as the system adjusts to natural flow and sediment regimes.
1 Introduction
Dam removal has occurred at an increasing pace over recent decades and is expected to continue as many aging dams cease to perform useful functions, and as the safety hazards, maintenance costs, and ecological consequences of keeping dams in place come to outweigh the benefits (O’Connor et al., 2015; Duda and Bellmore, 2022; American Rivers, 2023). Dam removal can trigger a range of responses in physical and biological components of river systems, driving new ecosystem dynamics and trajectories, including differing short- and long-term responses (Foley et al., 2017a; Major et al., 2017; Bellmore et al., 2019). Despite the ubiquity of dam removal, relatively few cases have been studied, and even fewer have been studied over a long period of time, including before, during and after dam removal (Bellmore et al., 2017; Foley et al., 2017a).
Riparian, wetland, and coastal vegetation may all change in response to dam removal (Shafroth et al., 2002), due to alterations of various physical and biological processes. One of the primary ways that dam removal affects vegetation is by changing the distribution, abundance, and character of landforms on which plants grow (Shafroth et al., 2002; Bellmore et al., 2019). In former reservoirs, water drawdown associated with dam removal exposes formerly submerged landforms that become available for plant colonization. In cases where a significant amount of sediment was trapped behind the dam, the mobilization, transport, and deposition of sediment downstream can trigger changes to active channels and near-channel bars, floodplain aggradation, or aggradation and new landform development in the river’s delta (Pizzuto, 2002; Major et al., 2017). These kinds of changes can affect existing vegetation through burial or erosion/removal and provide opportunities for colonization of new plants via creation of new surfaces and landforms (Shafroth et al., 2002). The new surfaces and landforms may have environmental conditions that particularly favor early-successional, disturbance-adapted plant species, including weedy, non-native species (Shafroth et al., 2002; Tullos et al., 2016). Changes to flow regime associated with dam removal could also lead to vegetation changes over time, although we are unaware of any dam-removal case studies with significantly altered flow regimes (Foley et al., 2017a). Dam removal also restores connectivity along the river for organisms that were unable to pass through a dam in the up- or downstream direction (Bellmore et al., 2019). Seed or propagule dispersal of some riparian plants is accomplished by downstream transport in the river, a form of hydrochory (Nilsson et al., 2010). Dam removal can restore hydrochory, allowing seeds to be transported downstream past former dam sites. Transport and deposition of large wood past former dam sites may also influence vegetation via interactions with fluvial processes and landform dynamics (Francis et al., 2008; Naiman et al., 2010; Leung, 2019). Restored upstream movement of anadromous fish may lead to deposition of marine-derived nutrients on floodplains, potentially affecting growth of riparian plants (Helfield and Naiman, 2001; Quinn et al., 2018).
Ecosystem responses to dam removal may vary spatially along the river continuum – from former reservoir(s), along downstream river corridors, to deltas and estuaries. Most studies of vegetation responses to dam removal have focused on short-term colonization and growth of plants on formerly submerged surfaces associated with drained reservoirs (e.g., Orr and Stanley, 2006; Lisius et al., 2018; Ravot et al., 2020; Chenoweth et al., 2023). Others have examined specific aspects of vegetation in other parts of the river system, such as plant community composition and diversity (Schmitz et al., 2009; Brown et al., 2022). There have been no published reviews that synthesize results of vegetation change associated with dam removal in multiple riverine landscape positions.
The Elwha River (Washington, USA) is the site of two large dam removals that occurred between 2011 and 2014 and is the most studied dam-removal case study globally. The principal objective of the dam removals was to restore the Elwha’s native anadromous salmon populations, but a secondary goal was to evaluate ecosystem responses to dam removal and the return of salmon (U.S. Department of the Interior and epartment of Commerce and Lower Elwha S’Klallam Tribe, 1994; Ward et al., 2008). Because most of the Elwha watershed is protected within a national park, ecosystem responses may be evaluated without the confounding influence of the various anthropogenic disturbances that occur in most American river systems (Duda et al., 2008). Moreover, whereas vegetation in the majority of the former reservoirs was actively managed through invasive vegetation control, and planting and seeding of native vegetation (funded as part of the dam removal; Chenoweth et al., 2011), the river reaches and delta were largely left to recover on their own. As a result, the Elwha dam removals offer a unique opportunity to assess how restoring natural fluvial processes affects riparian vegetation communities in a coastal temperate ecosystem. This work has important implications for biodiversity conservation as well as salmon recovery because coastal temperate riparian zones support diverse plant communities (Naiman et al., 1993; Naiman et al., 1998) and provide essential habitat functions for aquatic ecosystems (Gregory et al., 1991; Naiman and Decamps, 1997). There are more than 250 publications related to the Elwha dams and their removal (https://www.zotero.org/groups/4740476/elwha_bibliography), including studies of effects of dam removal on various aspects of the physical environment, and various terrestrial, aquatic, and marine communities. With respect to vegetation, previous research has examined ecosystem responses upstream, between, and downstream of the former dams, and within the former reservoirs (e.g., Brown et al., 2022; Chenoweth et al., 2023; Perry et al., 2023), but a comprehensive review of Elwha vegetation studies has not been conducted.
In this paper, we review and synthesize previous studies of vegetation responses to dam removal along the Elwha River, focusing on the important connections between fluvial processes and vegetation dynamics. We discuss vegetation dynamics in three major landscape positions along the river continuum (Figure 1): (1) the former reservoirs, (2) along the river corridor, and (3) in the Elwha delta and estuary. We gathered all available literature on Elwha River vegetation prior to and following dam removal, 39 sources in total (Table 1). Most of this literature focused on vegetation development in the former reservoirs following dam removal, but some sources examined vegetation along downstream river segments or in the river delta and estuary before and/or after dam removal. We also drew on published studies of geomorphic effects of the Elwha River dam removals, in order to place vegetation responses within the context of landform and sediment dynamics.
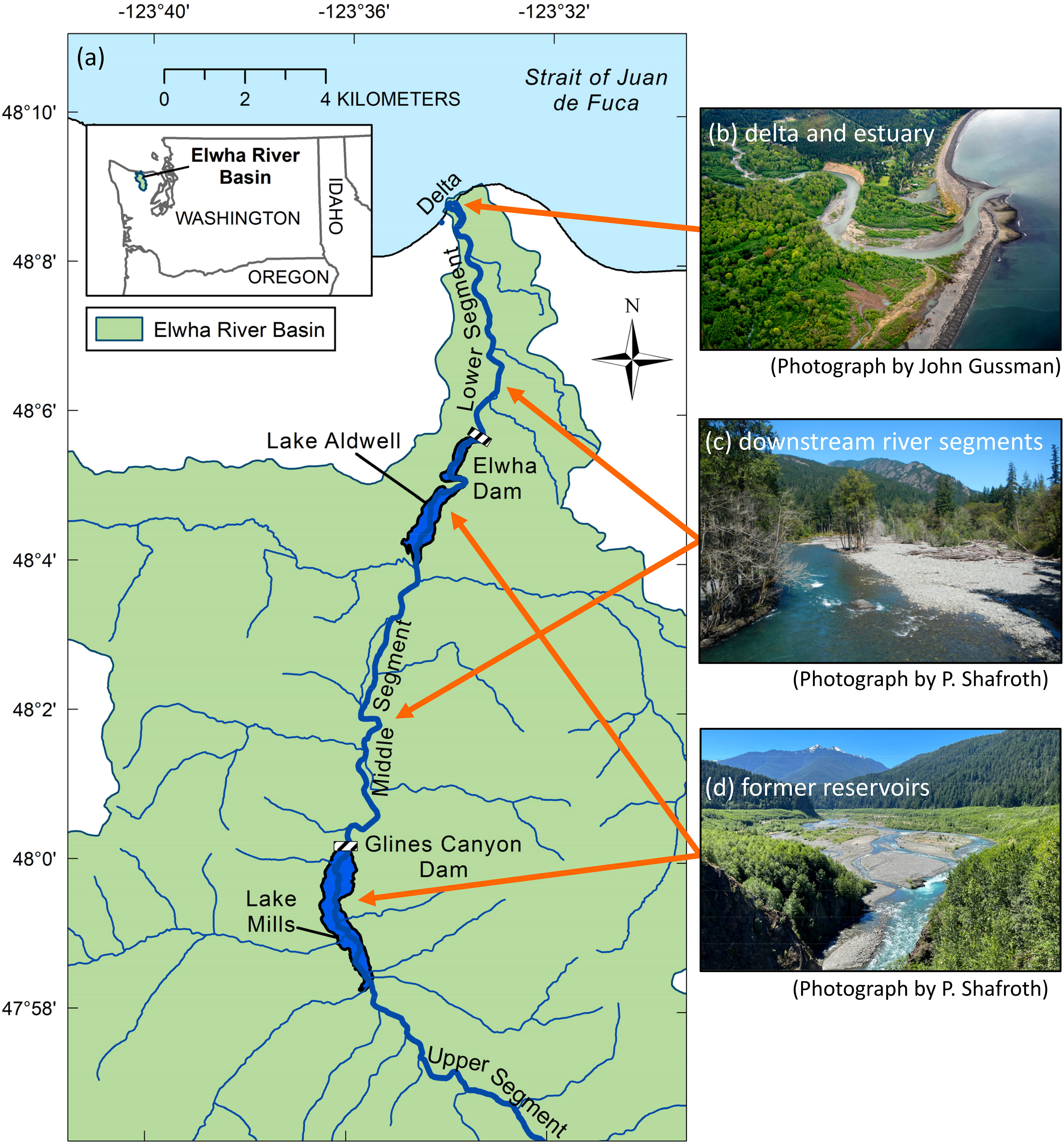
Figure 1 (A) Map of the Elwha River study area. Inset map shows the location of the Elwha River Basin within the Pacific Northwest, United States. Areas where riparian and wetland vegetation were potentially affected by dam removal are labelled in the larger map: the river delta and estuary (delta), river segments downstream of and between the former dams (lower and middle segments), and the former reservoirs (Lake Aldwell and Lake Mills). The upper river segment used as a reference condition in some studies is also labeled. Photographs depict examples of these areas: (B) oblique image of the Elwha River delta and estuary on May 8, 2023, (C) oblique image of the Elwha River ~1.5 km downstream of former Glines Canyon Dam on August 22, 2014, and (D) oblique image of the former Lake Mills on July 11, 2022.
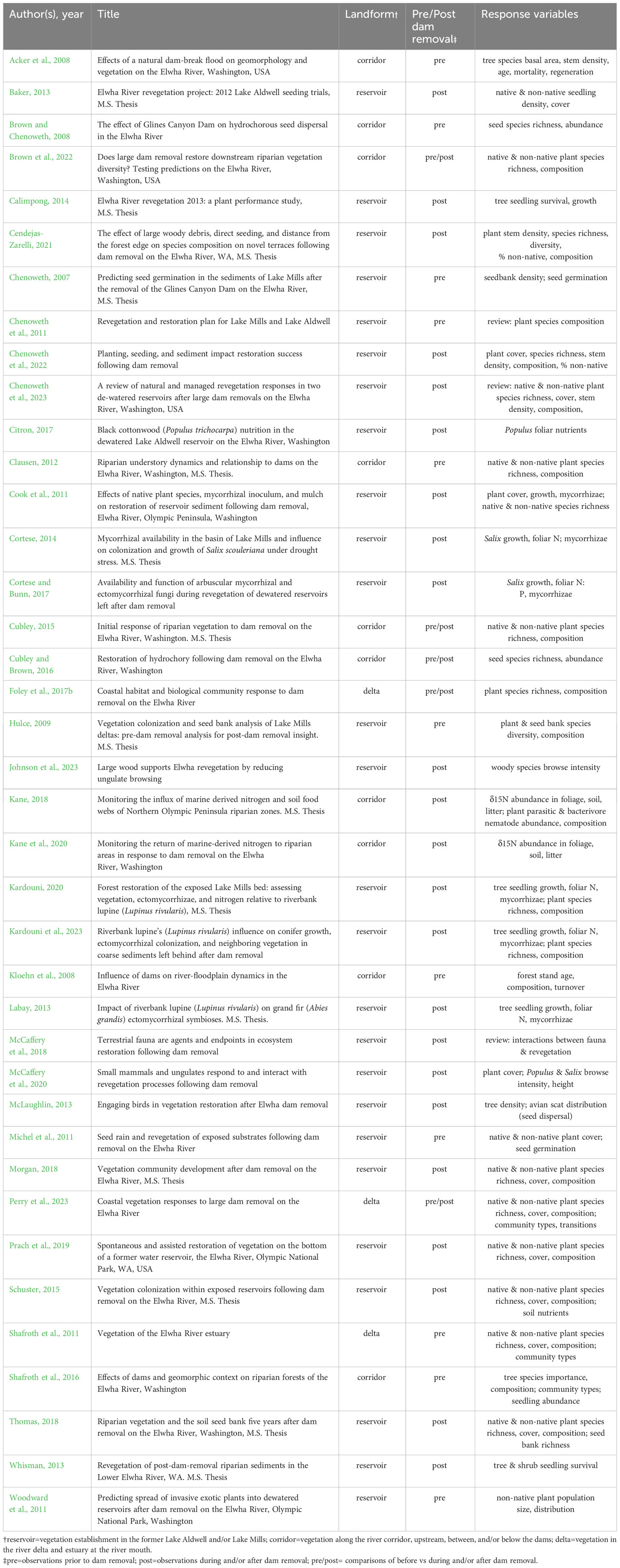
Table 1 Comprehensive list of publications on vegetation along the Elwha River, Washington, USA, before and after two dams were removed in 2011-2014.
We created separate causal-loop feedback diagrams for each of the three major landscape positions to illustrate expected or hypothesized relationships between riparian vegetation and hydrology, sediment, large wood, and marine influences following dam removal (Figure 2), informed by prior reviews of dam removal effects on river systems (Shafroth et al., 2002; Foley et al., 2017a; Major et al., 2017). The structure of these diagrams was loosely based on diagrams of ecosystem effects of dam removal published in Bellmore et al. (2019). The locations of the different landscape positions relative to the former dam, the river mouth, and each other result in distinct roles of sediment, wood, and propagule transport downstream and marine-derived nutrient transport upstream at each landscape position.
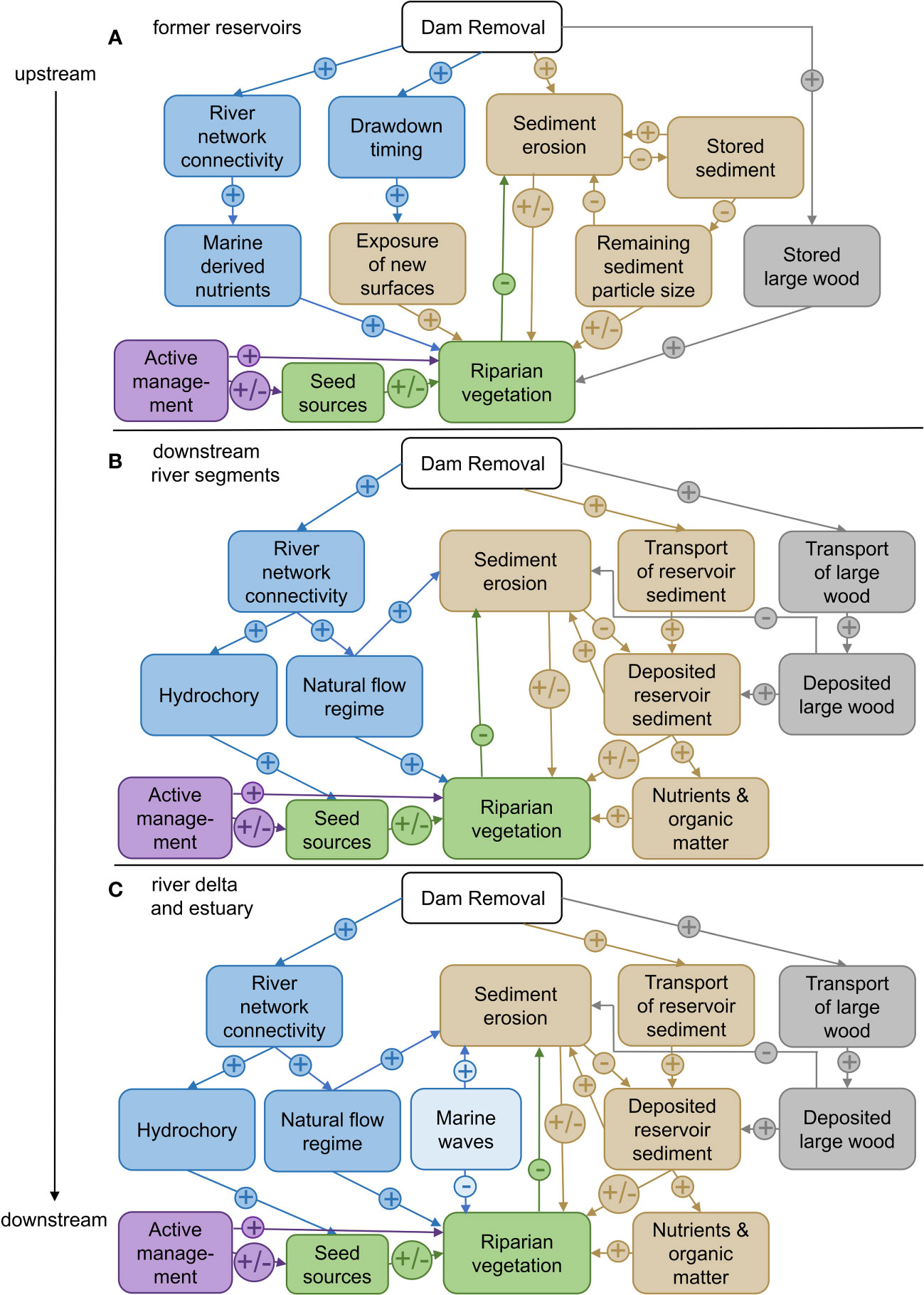
Figure 2 Causal-loop diagrams depicting cause-and-effect links and feedback loops influencing vegetation response to dam removal within (A) former reservoirs, (B) downstream river segments, and (C) coastal deltas and estuaries. The arrows indicate the direction of influence, and the plus and minus signs indicate whether the influence is positive (+; i.e., increases in the causal variable lead to increases in the response variable), negative (-), or positive for some plant taxa and negative for others (+/-). Sediment-driven processes are shown in brown, wood-driven processes are shown in grey, hydrology-driven processes are shown in blue, human-driven processes are shown in purple, and vegetation-driven processes are shown in green. The diagrams are loosely based on diagrams in Bellmore et al. (2019), modified to focus on vegetation responses.
In the review that follows, we (1) briefly summarize expected effects of dam removal on vegetation based on the causal-loop feedback diagrams in Figure 2, emphasizing changes that were particularly likely to occur in the context of the Elwha River dam removals, (2) describe observed sediment and landform dynamics following dam removal that were likely to influence vegetation, and (3) review vegetation responses to dam removal and post-dam-removal sediment and landform dynamics. For the former reservoirs, we also describe vegetation management activity and review vegetation responses to active management following dam removal. This review is intended to help guide future dam removal and ecosystem restoration efforts, and also to elucidate fundamental relationships between riparian vegetation and fluvial processes along a coastal temperate river.
2 Study area
The Elwha River flows 72 km from its headwaters in the Olympic Mountains to the Strait of Juan de Fuca, draining a total area of 833 km2, >80% of which are within Olympic National Park. Average instantaneous discharge is 43 m3 s-1. Two large dams were constructed for local hydropower along the Elwha River in 1913 (Elwha Dam, 32 m tall, 7.9 km from the river mouth) and 1927 (Glines Canyon Dam, 64 m tall, 21.6 km from the river mouth). The dams did not provide substantial flood control or water supply and, after 1975, regulated river flows were largely “run-of-the-river”. However, the dams prevented upstream fish passage and restricted downstream transport of sediment and wood, impounding ~30 Mt of sediment within the reservoirs during the 84-98 years that the dams were in place (Randle et al., 2015). Approximately 45% of the stored sediment was fine sediment (silt and clay) and ~55% coarser sediment (Randle et al., 2015).
Both dams were removed between 2011 and 2014. In late May 2011, water levels in both reservoirs were reduced by approximately 4.5 m in preparation for dam removal, exposing a narrow band of the valley wall. Removal of the two dams began simultaneously in September 2011. Removal of the Elwha Dam was relatively rapid, completed in March 2012. Removal of Glines Canyon Dam was designed to be slower to allow the Elwha River to gradually erode the larger quantity of sediment impounded in the upstream reservoir delta. The reservoir pool was drained slowly over a period of 13 months (September 2011-October 2012) (Randle et al., 2015) and dam removal was completed in August 2014. Dam removal released ~20.5 ± 3.2 Mt of sediment downstream over the first five years (2012-2016) (Ritchie et al., 2018). More detailed descriptions of the dam removal process are provided by Randle et al. (2015) and Warrick et al. (2015).
The former reservoirs, Lake Mills (Glines Canyon Dam) and Lake Aldwell (Elwha Dam) (Figure 1), are located at 166 m and 57 m above sea level, respectively. When full, Lake Mills occupied 1.68 km2 of Olympic National Park, flooding ~4.5 km of the former river (Duda et al., 2008). Lake Aldwell occupied 1.08 km2 downstream of the Park boundary, flooding ~4 km of the former river (Duda et al., 2008). The forests around the former reservoirs are generally dominated by mature conifers [Pseudotsuga menziesii (Douglas fir), Abies grandis (grand fir), Tsuga heterophylla (western hemlock)], with thin bands of deciduous trees [Alnus rubra (red alder), Acer macrophyllum (bigleaf maple), Populus balsamifera spp. trichocarpa (black cottonwood), and Salix spp. (willow)] along the former shoreline (Chenoweth et al., 2022). Prior to dam removal, Lake Mills and Lake Aldwell held an estimated 23 ± 6 Mt and 7 ± 2 Mt of impounded sediment, respectively (Warrick et al., 2015).
River corridors downstream of the former dams included a ~10-km “middle segment” between the dams and a ~7.9-km “lower segment” downstream of the Elwha Dam site (Figure 1). Prior to dam removal, geomorphic surfaces along these river segments were composed of a mixture of developing and mature floodplains and terraces and infrequent gravel bars (Shafroth et al., 2016). Riparian forests were composed of a mixture of deciduous and coniferous trees, most commonly A. rubra, A. macrophyllum, and P. menziesii on the middle segment and A. rubra and P. balsamifera spp. trichocarpa on the lower segment (Shafroth et al., 2016). To evaluate effects of the dams, some pre-dam-removal studies compared vegetation responses in the middle and lower segments to a reference segment upstream of Lake Mills (“upper segment”; Figure 1). Low sediment supply while the dams were in place resulted in channel narrowing, incision, armoring, and channel bed coarsening downstream of the dams, particularly along the middle segment and upper portions of the lower segment, where there were fewer remaining sources of fine, unconsolidated sediment in channel margins and floodplains (Pohl, 2004; Draut et al., 2011). Reduced channel mobility and fluvial disturbance in turn resulted in older forest stand ages, reduced pioneer tree seedling establishment, and reduced native plant diversity downstream of the dams compared to upstream, particularly along the middle segment (Shafroth et al., 2016; Brown et al., 2022).
The river delta and estuary (Figure 1) are constrained by a levee on the west side constructed in 1964 and a second levee set back from the main channel on the east side, constructed in 1985 (Warrick et al., 2009). Both levees were raised approximately 1 m in 2010–2011 in preparation for dam removal, anticipating that bed aggradation due to the dam-removal sediment pulse could raise the water-surface elevations. The location of the main channel in the delta has changed repeatedly over time, resulting in dynamic coastal lakes along the shoreline created by abandoned channels. In particular, the predominant eastern channel was blocked by dike construction in 1950 and the western channel was redirected by levee construction in 1964. After levee construction, the channel still meandered considerably (Warrick et al., 2011), for example with lateral channel migration of several meters per year in the years immediately prior to dam removal (Draut et al., 2011). Channel movement in the lowermost river and delta, fed by sediment from eroding channel-margin bluffs and floodplains along the lower segment (Draut et al., 2011), maintained a mosaic of plant community types and stand ages, including younger riparian shrub-dominated communities and willow-alder forest, older mixed riparian forest, dunegrass communities at the tops of the beach fronts, and estuarine marsh vegetation at the margins of the coastal lakes (Shafroth et al., 2011). However, low sediment supply resulted in substantial and accelerating erosion east of the river mouth during the lifetime of the dams, with mean annual shoreline erosion of 0.9 ± 0.2 m per year from 1939 to 1990 and 1.2 ± 0.2 m per year from 1990 to 2006, resulting in loss of >8.7 ha of delta land surface between 1939 and 2006 (Warrick et al., 2009).
3 Former reservoirs
3.1 Expected effects of dam removal on vegetation in the former reservoirs
The draining of the two former reservoirs was certain to expose large areas of sediment where plants could become established and grow (Figure 2A). Several variables were expected to influence the details of natural, unmanaged vegetation succession and dynamics, including reservoir drawdown timing, distance from seed sources and seed banks, landform characteristics, and sediment texture (fine versus coarse) (Chenoweth et al., 2023). In addition, active management in the former reservoirs was aimed at promoting native vegetation colonization and growth (Chenoweth et al., 2023).
3.2 Sediment and landform dynamics in the former reservoirs
The exposed former reservoir areas provided extensive bare surfaces for vegetation establishment on three general landform types: (1) valley walls; (2) terraces (of variable elevation); and (3) dynamic floodplains and channels (Figure 3). The three landform types varied with respect to stability, slope, sediment texture, and elevation above the channel. Fine-textured sediments were predominant on the steep valley walls. Terraces of variable thickness and elevation above the channel formed as a result of sediment erosion, redistribution, and progradation within the former reservoirs during dam removal, and were modified by erosion and redeposition at lower elevations as the river responded to dam removal (Randle et al., 2015). These processes resulted in relatively flat, perched terraces, 6-18 m thick, topped with predominantly coarse sediments farther from the reservoir margins. Many of the terraces eroded away during high flow events in the first few years after dam removal (Ritchie et al., 2018). Finally, the lowest positions in the valley included dynamic braided channels and floodplains characterized by coarse sediments and extensive lateral mobility (Randle et al., 2015; Chenoweth et al., 2022).
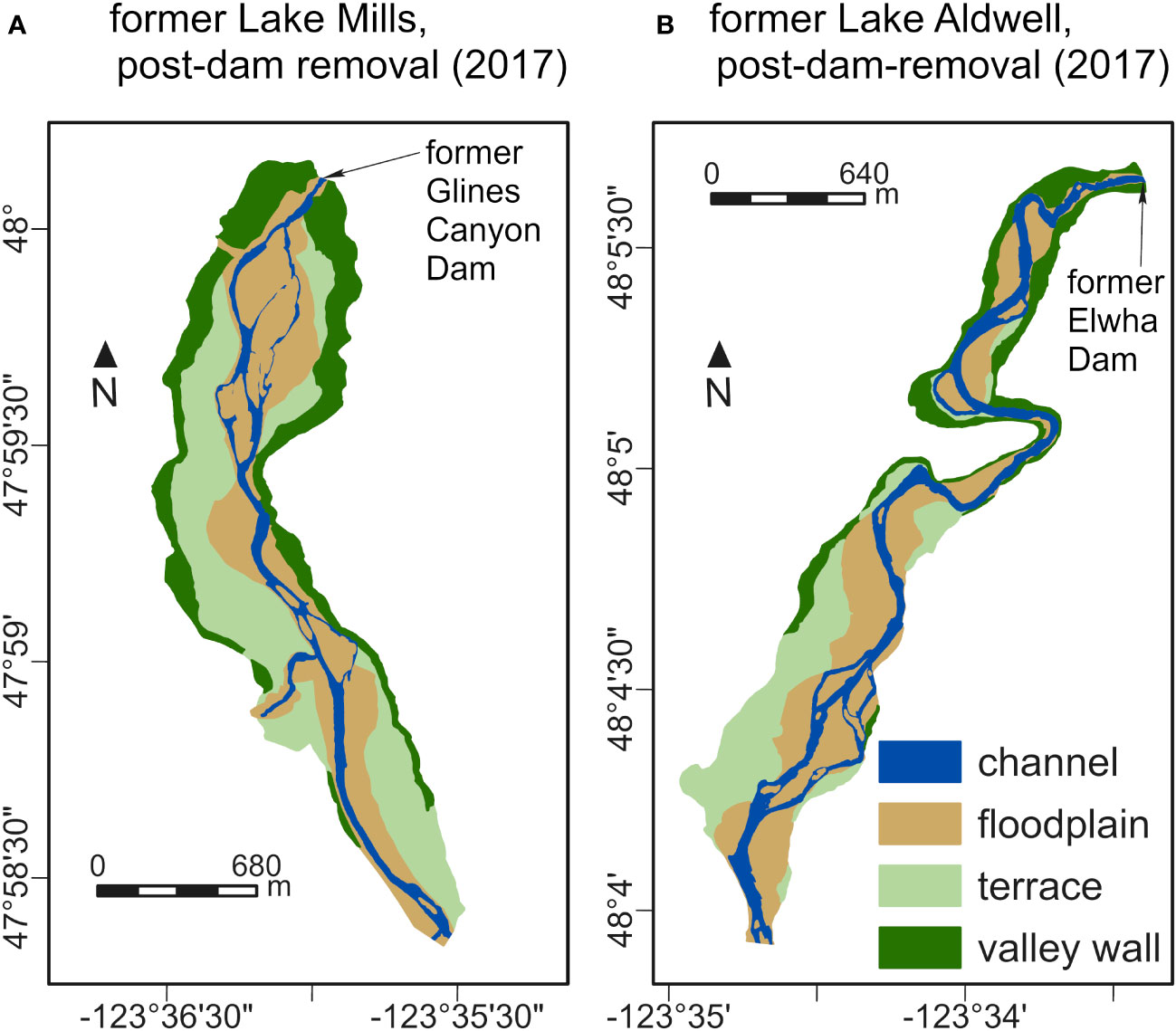
Figure 3 Cover by different landforms following dam removal in the former (A) Lake Mills and (B) Lake Aldwell along the Elwha River. Removal of Glines Canyon Dam was completed in 2014, and the Elwha Dam removal was completed in 2012. High valley wall surfaces were formed by initial, gradual drawdowns of the reservoirs. Terraces, varying in elevation, were formed by sediment erosion, redistribution, and progradation during dam removal. Low floodplain surfaces remained dynamic, with substantial, ongoing channel migration and sediment erosion, redistribution, and progradation. Polygons were drawn roughly from National Agriculture Imagery Program (NAIP) imagery taken on August 22, 2017. See Figure 1 for locations of the former reservoirs along the Elwha River.
3.3 Vegetation management in the former reservoirs
Four types of active management were implemented to influence revegetation in the former reservoirs (Chenoweth et al., 2011): 1) invasive vegetation control; 2) seeding of desirable plant species; 3) planting of containerized or bare root plants of desirable species; and 4) placement of large wood (hereafter, LW; e.g., tree boles, and boles with root wads) to create microclimatic complexity and protection from wind and herbivory for new plants.
Invasive vegetation control, which consisted of both chemical control and manual removal, started before dam removal in an effort to reduce seed sources of various species of concern (Woodward et al., 2011). Invasive vegetation control continued within the former reservoirs as sediments were gradually exposed, informed by annual invasive vegetation mapping efforts (Chenoweth et al., 2023). Planting of containerized plants began in November 2011 and seeding began in Fall 2012, except for a small seeding trial that occurred in Spring 2012 in the former Lake Aldwell (Baker, 2013).
In the former Lake Mills, 218,116 plants were installed, and 2,193 kg of seed were sown on 14.1 ha of the valley wall and 44.0 ha of terraces. Remaining areas were left to naturally revegetate, including 25.6 ha of valley wall landforms and 18.7 ha of terraces. In the former Lake Aldwell, 86,064 rooted plants were installed, and 716 kg of seed were sown on 16.7 ha, leaving 103.7 ha to revegetate naturally. Species composition and planting density varied by site and by year. Most of the plantings were trees and shrubs representing 60 native species (Chenoweth et al., 2022). The seed mixes varied but were composed primarily of nine native species (Chenoweth et al., 2022).
The Lower Elwha Klallam Tribe [Lower Elwha Tribe] and the Olympic National Park in 2012 and 2014 translocated 835 logs (log boles and logs with attached root-wads) by helicopter into the former Lake Mills onto the coarse-textured terraces formed during dam removal. The logs were arranged in single, parallel, and overlapping configurations (Chenoweth et al., 2023). Roughly two-thirds of the translocation area was planted and seeded.
3.4 Vegetation responses in the former reservoirs
Natural revegetation (i.e., passive revegetation) in the former reservoirs was studied during and 3-5 years after dam removal in plots along a series of transects on the three general landform types (valley walls, terraces, floodplains/channels) through areas that were not planted or seeded (Schuster, 2015; Morgan, 2018), and in “control” (untreated) plots along a separate series of transects through areas that had been planted and seeded (Prach et al., 2019; Chenoweth et al., 2022). Effects of planting and seeding (i.e., active revegetation) were examined in plots along the latter series of transects, in comparison to the “control” plots (Morgan, 2018; Prach et al., 2019; Chenoweth et al., 2022). Some studies differed with respect to plot sizes, years sampled, response variables, and other details, making comparisons among studies challenging in some cases (Chenoweth et al., 2023).
Within the unplanted portions of the former reservoirs, natural revegetation depended on sediment texture, landform, and the timing of landform development during reservoir drawdown (Figure 2A). The rate of natural revegetation was fastest on areas with fine sediment, such as valley walls and portions of terraces in the former Lake Aldwell, which tended to have finer sediment than the former Lake Mills because coarser sediments were preferentially trapped upstream in Lake Mills (Randle et al., 2015; Schuster, 2015; Morgan, 2018). On valley walls and terraces with fine sediment in Lake Aldwell, initial stands of Juncus spp. (rushes), Carex spp. (sedges) and Equisetum spp. (horsetails), likely germinated from in situ seed banks (Brown and Chenoweth, 2008), rapidly gave way to dense stands of Alnus rubra (red alder) interspersed with Populus balsamifera ssp. trichocarpa (black cottonwood) and Salix sitchensis (Sitka willow), which persist today and are self-thinning. On terraces with coarse sediment, natural revegetation was slow and, in some locations, still had relatively low plant cover six years after dam removal. However, one terrace landform in former Lake Mills, which formed in spring during initial dam removal, supported abundant black cottonwood and Sitka willow seedling establishment (Chenoweth et al., 2022). This establishment occurred during a drawdown hold period in May/June 2012 (Bountry et al., 2015), providing ideal conditions for the seedlings. However, when the drawdown resumed, the terrace quickly became perched, eventually ~18 meters above the final channel elevation. The tree seedlings eventually grew into woodlands with no cottonwood mortality observed in long-term monitoring plots established by the National Park Service (Chenoweth et al., 2022). On all terraces, bands of willow and cottonwood also formed in moisture-holding channels stemming from the valley wall. Open terrace areas not formed during the cottonwood and willow seed dispersal period (May-June) that were not seeded or planted continued to have low vegetation cover (Chenoweth et al., 2022) with sparse, drought-tolerant vegetation, including non-native annual grasses (e.g., Vulpia myuros (rat-tail fescue), Aira caryophyllea (silver hairgrass)), and biological soil crust (mostly bryophytes). Where larger plants and LW provided protection (Figure 2A), vegetation was denser (Cendejas-Zarelli, 2021). By 2016, an average of 13 native species had established per 100 m2 on exposed new terraces in Lake Aldwell and Lake Mills (Figure 4). In the zone with dynamic channels and floodplains, bands of willow and cottonwood gradually established on stable gravel bar landforms, with herbaceous pioneer vegetation on less stable surfaces (Schuster, 2015; Morgan, 2018). Due to ongoing channel and floodplain dynamics, vegetation cover remained relatively low in these low-elevation parts of the former reservoirs.
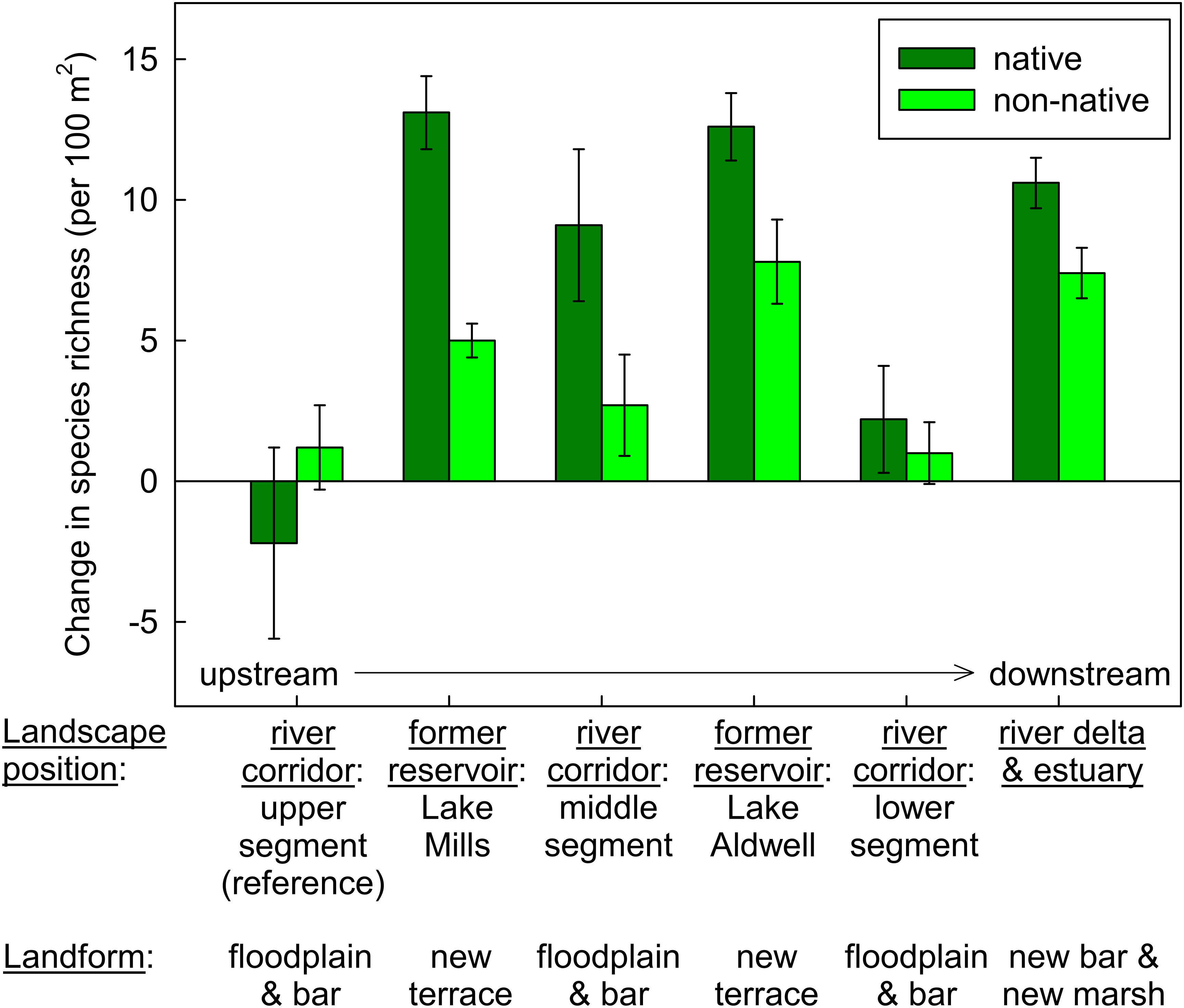
Figure 4 Change in mean native and non-native plant species richness in 100-m2 plots between 2010 (before dam removal) and 2016-2018 (several years after the two dams were removed) at different landscape positions along the Elwha River. For river corridors, species richness was measured in 2017 on floodplains and gravel bars upstream of both former dams (upper segment) as a reference condition, between the two former dams (middle segment), and downstream of the former lower dam (lower segment) (Brown et al., 2022). For former reservoirs, species richness was measured in 2016 on terraces that formed during reservoir drawdown (Morgan, 2018). For the river delta and estuary, species richness was measured in 2018 on new river bars, river mouth bars, and intertidal aquatic marsh that formed from sediment eroded from the former reservoirs (Perry et al., 2023). Native species richness increased significantly between 2010 and 2017 on the middle river segment only (Brown et al., 2022). For the former reservoirs and delta, we treated mean species richness prior to dam removal as equal to zero, because these surfaces did not exist prior to dam removal, and, therefore, statistical analyses of temporal change were not possible. Error bars are one standard error of the mean. For consistency, we limited this figure to Elwha River studies that examined native and non-native species richness in 100-m2 plots in 2016 or later.
Within the planted portions of the former reservoirs, effects of planting and seeding also varied with sediment texture and landform (Figure 2A), as well as among studies. In fine sediment sites, seeding reduced non-native frequency (Chenoweth et al., 2022) but not non-native cover (Morgan, 2018; Prach et al., 2019). Seeding also did not affect overall vegetation cover in fine sediment sites (Chenoweth et al., 2022). Planting trees and shrubs on fine sediments increased species richness in some studies (Chenoweth et al., 2022), but this effect was not detected in other studies (Morgan, 2018). Seeding and planting had the greatest effect on revegetation on the coarse-textured terraces (Cendejas-Zarelli, 2021; Chenoweth et al., 2022). Seeding increased vegetation cover relative to unseeded sites from 2012 to 2016 and affected species composition (Chenoweth et al., 2022). The most influential species seeded was Lupinus rivularis (riverbank lupine) (Morgan, 2018; Kardouni, 2020; Chenoweth et al., 2022). Planting trees and shrubs on coarse terraces affected species composition but did not significantly increase stem densities (Chenoweth et al., 2022), likely due to the low survival rate of plantings installed 2+ years after dam removal (Chenoweth et al., 2023).
Efforts to control invasive exotic vegetation focused on the more common non-native species of the region, such as Phalaris arundinacea (reed canary grass) and Cytisus scoparius (scotch broom) and were largely successful in that these species did not come to dominate the former reservoirs (Chenoweth et al., 2022). However, these control efforts were undertaken independently from vegetation monitoring and were not integrated into the monitoring plots in any consistent way. Their method of application by different field crews in different years and minimal documentation makes it a challenge to draw definitive conclusions about their effects on native vegetation trajectories. Initially, Lake Aldwell had more non-native species than Lake Mills, possibly due to (1) the higher levels of human development near the reservoir (Woodward et al., 2011) and (2) its relatively fine sediment, which promoted rapid colonization (Schuster, 2015). By 2017, this difference had largely disappeared due to non-native species establishment on the coarse sediments of Lake Mills (Morgan, 2018), such as the non-native annual grasses described above. Non-native species did not dominate vegetation on either of the former reservoirs.
Installations of LW were used in the former reservoirs to enhance revegetation efforts by creating favorable microclimates (i.e., “safe sites”) for seedlings through shading and protection from wind and erosion (Figure 2A; Chenoweth et al., 2011; Calimpong, 2014). In Lake Mills, LW was associated with reductions in wind speed, soil temperature and evaporative stress (Colton, 2018), all of which can inhibit germination and contribute to plant mortality (MaChado and Paulsen, 2001; Wahid et al., 2007). Clusters of LW also likely enhanced seedling growth by impeding browsing by large ungulates (Johnson et al., 2023). At planting sites in Lake Mills, the presence of LW was associated with increased survivorship through the first growing season after planting (Calimpong, 2014). Plant diversity and species composition were also affected by LW. In Lake Mills, LW trapped wind-blown seeds, particularly of grasses and forbs, resulting in greater overall species richness but also increased representation of non-native species (Cendejas-Zarelli, 2021). In the Cendejas-Zarelli (2021) study, interaction effects were observed for LW and seeding treatments, as LW-associated increases in non-native species were most prevalent in unseeded areas, and the greatest increases in species richness were observed where seeding was paired with LW. In Lake Mills, LW also attracted avian seed dispersers, resulting in greater local abundance of native woody plants (McLaughlin, 2013; Cendejas-Zarelli, 2021).
4 River segments downstream of dams
4.1 Expected effects of dam removal on vegetation downstream of dams
River segments downstream of the dams were expected to become more dynamic in response to dam removal due to the effects of transport and deposition of large quantities of reservoir sediment and LW, and potential bed elevation changes (aggradation) as an expected sediment pulse moved downstream (Figure 2B). These processes were expected to promote channel widening, new bar formation, and sediment deposition, particularly fine sediment, on floodplains (Draut et al., 2011). Increased abundance of gravel bars, which experience frequent flood disturbance, was expected to support more pioneer riparian vegetation (Shafroth et al., 2002) and thus a younger overall forest community age structure (Shafroth et al., 2016). Where sediment deposition was significant, existing plants that were less well adapted to sediment burial were expected to be stressed or killed (Shafroth et al., 2002). Water transport of seeds (hydrochory) was expected to increase with dam removal (Figure 2B; Brown and Chenoweth, 2008), and along with gravel bar formation, was expected to increase downstream plant diversity (Clausen, 2012). Occasional, targeted invasive vegetation control and small-scale vegetation plantings occurred in the lower river segment, but effects could not be quantified (Brown et al., 2022).
4.2 Sediment and landform dynamics downstream of dams
Channel dynamics (largely associated with high sediment loads) during and after dam removal increased the abundance of young landforms downstream along the river, especially in the middle segment. An estimated 0.38 Mt and 0.98 Mt of sediment were deposited in the mainstem channel in the middle and lower segments, respectively, during the first five years of dam removal (2012-2016) (Ritchie et al., 2018). Widespread mainstem bed aggradation of 1-2 m and subsequent channel avulsion resulted in substantially increased channel width and braiding in the second year of dam removal (East et al., 2015). These processes, together with increased LW deposits (logjams, Leung, 2019), resulted in extensive, new bars along the mainstem channel (Figure 5) (East et al., 2015; Warrick et al., 2015). The initial downstream deposition included substantial fine (silt and clay) material (Draut and Ritchie, 2015) and most sediment deposited during the peak of the sediment pulse was sand and gravel, resulting in finer-textured channel beds and gravel bars compared to the armored, cobble surfaces that predominated prior to dam removal (East et al., 2015). The fine-textured, aggraded channels became incised, and some were abandoned between 2013 and 2017. On the lower river segment, channel width and braiding quickly returned to that of the dammed condition (East et al., 2018). However, on the middle segment, channel width and braiding remained higher relative to the dammed condition at least through 2017, probably because sediment was most limited along this segment when the dams were in place (Figure 5) (East et al., 2018).
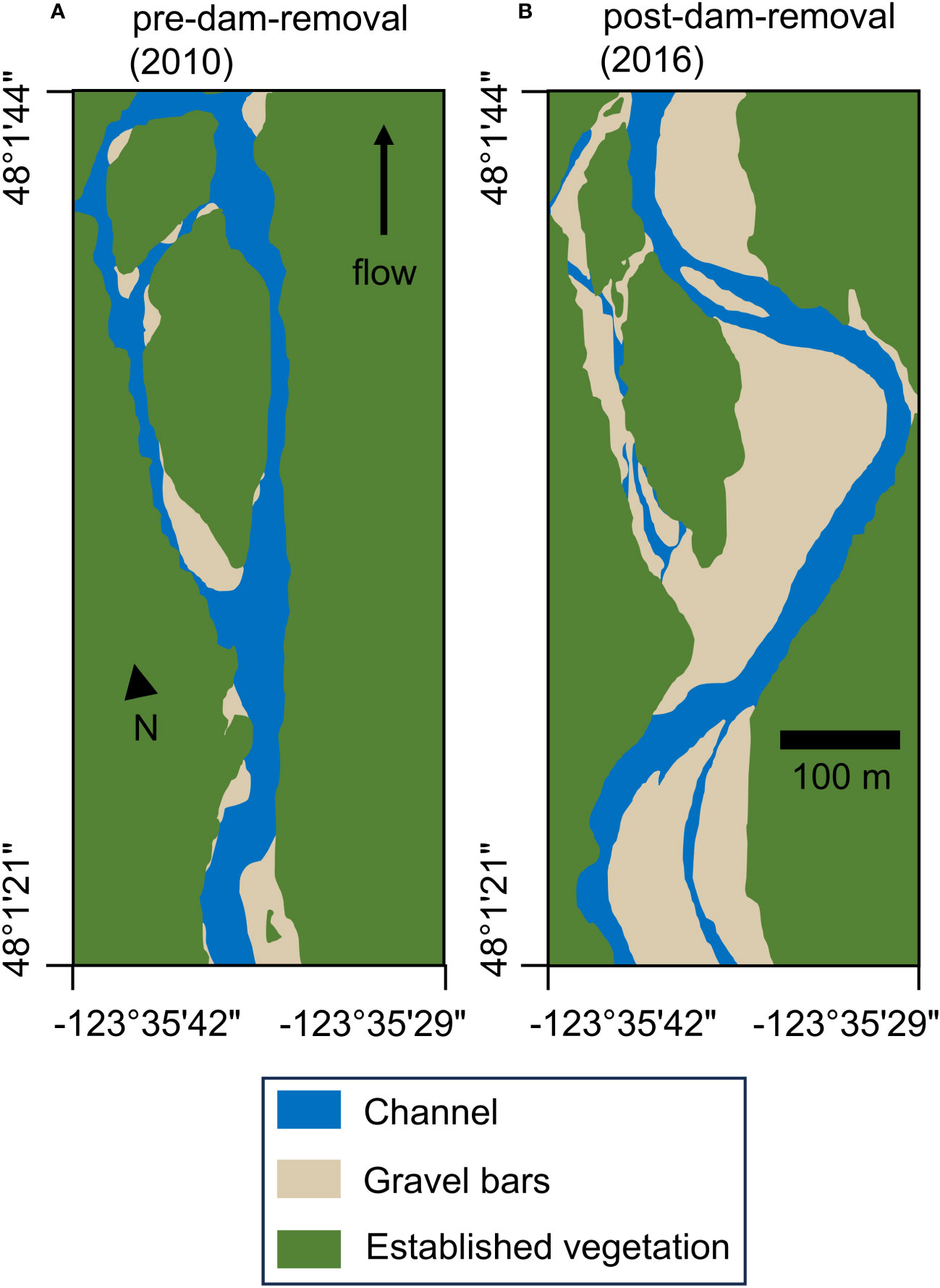
Figure 5 Change in cover by the channel, gravel bars, and established vegetation along an approximately 0.75-km reach of the middle segment of the Elwha River (A) before dam removal (2010) versus (B) after dam removal (2016). Polygons were drawn from imagery published in Ritchie et al. (2018). See Figure 1 for location of the middle segment of the Elwha River.
In addition to the mainstem channel, established landforms along the river corridor also received substantial sediment deposition during dam removal. An estimated 0.24 Mt and 0.54 Mt of sediment were deposited on the floodplain on the middle and lower segments, respectively, during the first five years during and after dam removal (Ritchie et al., 2018). Mainstem bed aggradation redirected streamflow to floodplain side-channels even during low and moderate discharge, depositing a mean of 50 ± 38 cm of mainly fine sediment in floodplain channels, particularly along the middle segment, in the first two years of dam removal (East et al., 2015). Correspondingly, between 2010 and 2017, vegetation plots aggraded by 24 ± 8, 21 ± 16, and 17 ± 33 cm on bars, floodplains and terraces, respectively, on the middle segment, and by 61 ± 38, 35 ± 27, and 26 ± 42 cm on bars, floodplains and terraces, respectively, on the lower segment (Brown et al., 2022). Ten plots, mainly on the lower segment, aggraded by >50 cm.
4.3 Vegetation responses downstream of dams
Vegetation responses to post-dam-removal landform dynamics and sediment deposition along river corridors downstream from the dams were examined using field plot sampling on terraces, floodplains, gravel bars, and abandoned secondary channels. The plots were arranged along fifteen transects that spanned the river valley within the upper, middle and lower segments (Shafroth et al., 2016; Brown et al., 2022). These transects were repeatedly sampled before (2005, 2010) and after (2013, 2014, 2016, 2017) dam removal.
Native species richness on the middle segment increased by 31% during and after dam removal (2013-2017), as expected, partially mitigating what had been substantially lower species richness downstream of the dams compared to upstream prior to dam removal (Figure 4; Brown et al., 2022). By contrast, native plant diversity did not increase significantly on the lower segment during and after dam removal (Figure 4). Plant species composition on both the middle and lower segments also changed significantly on floodplains and bars during and after dam removal, but not on terraces (Brown et al., 2022). Herbaceous species changed the most in terms of both which species were most abundant, and which were significant indicator species for floodplains and bars (based on frequency and abundance), with fewer changes in shrubs and trees. Non-native species richness along the middle and lower segments did not significantly change following dam removal (Figure 4), while non-native species cover increased to a similar extent both upstream and downstream of the former dams (Brown et al., 2022).
Plant establishment on new gravel bars and fresh sediment deposits created during dam removal likely influenced changes in species richness and composition along the downstream river corridor (Figure 2B). New bars, varying spatially in elevation, flood disturbance, sediment texture, and overstory shade, can provide an array of niches and microsites suitable for establishment of different plant species. Likewise, fine sediment deposits on extant gravel bars and floodplains increase spatial variation in environmental conditions suitable for different plant species. These disturbed conditions are particularly suitable for establishment of early-successional plant communities, which were notably rare downstream of the dams compared to upstream prior to dam removal (Shafroth et al., 2016).
Changes in species richness and composition may also have been influenced by increases in hydrochorous seed dispersal (Figure 2B). Dams form a barrier to downstream seed transport, affecting downstream plant species occurrence and abundance (Nilsson et al., 2010). Prior to dam removal on the Elwha River, Glines Canyon Dam reduced species richness and abundance of floating and submerged seeds in the middle river segment by >80% compared to upstream of the dams (Brown and Chenoweth, 2008). After dam removal, seed abundance and richness in the middle segment increased by ~30x and ~6x, respectively, leading to higher seed abundance and richness downstream of the former dam than upstream (Cubley and Brown, 2016). This substantial hydrochory may have been supplied by a combination of seed produced by established upstream plant communities, seed produced by nascent plant communities in the former Lake Mills, and mobilization of seeds stored in former reservoir sediment.
Finally, some changes in plant community composition along the downstream river corridor may have been driven by negative effects of sediment burial on established plants (Figure 2B). On the lower river segment, native species richness was negatively associated with greater sediment deposition on terraces and bars, suggesting that high rates of sediment deposition on this segment may have reduced the frequency of some species (Brown et al., 2022). For example, sediment deposition on some lower segment terraces led to die-back of dense Polystichum munitum (western sword fern) stands and replacement by early-successional species. Negative effects of sediment deposition on some plants may have counterbalanced positive effects of sediment deposition on others better adapted to riparian sediment dynamics, perhaps explaining in part the lack of change in overall native species richness on the lower segment following dam removal (Brown et al., 2022).
5 Delta and estuary
5.1 Expected effects of dam removal on vegetation in the delta and estuary
Much of the sediment and LW stored behind the two dams was expected to be deposited in the river delta and nearshore (Czuba et al., 2011; Warrick et al., 2011), creating landforms that could be colonized by plants (Figure 2C). Where sediment deposition was significant, existing plants that were less well adapted to sediment burial were expected to be stressed or killed (Shafroth et al., 2002). Based on studies of sediment deposition in Pacific Northwest tidal wetlands, rapid sediment deposition in the delta had the potential to cause rapid vegetation change (Shafroth et al., 2011). Occasional, targeted invasive vegetation control and small-scale vegetation plantings occurred in the delta but effects could not be quantified (Perry et al., 2023).
5.2 Sediment and landform dynamics in the delta and estuary
Dam removal created abundant new surfaces suitable for riparian and wetland vegetation in the delta and estuary (Figure 6). Approximately 5.4 Mt of sediment were deposited in the delta and estuary in the first five years of dam removal (2012-2016) (Ritchie et al., 2018), forming new intertidal and supratidal river bars, river mouth bars, and beaches, as well as new intertidal aquatic habitats (Foley et al., 2017b; Perry et al., 2023). The total area of intertidal and supratidal surfaces increased by ~31.3 ha during this period, mainly seaward of the former shoreline, expanding the 97.5 ha pre-dam-removal sampling area for the delta and estuary by >30% (Perry et al., 2023). However, between 2016 and 2018, this trend reversed and the total area of intertidal and supratidal surfaces in the delta decreased by ~4.5 ha (Perry et al., 2023), as sediments at the river mouth were eroded and deposited further east along the shoreline (Warrick et al., 2019).
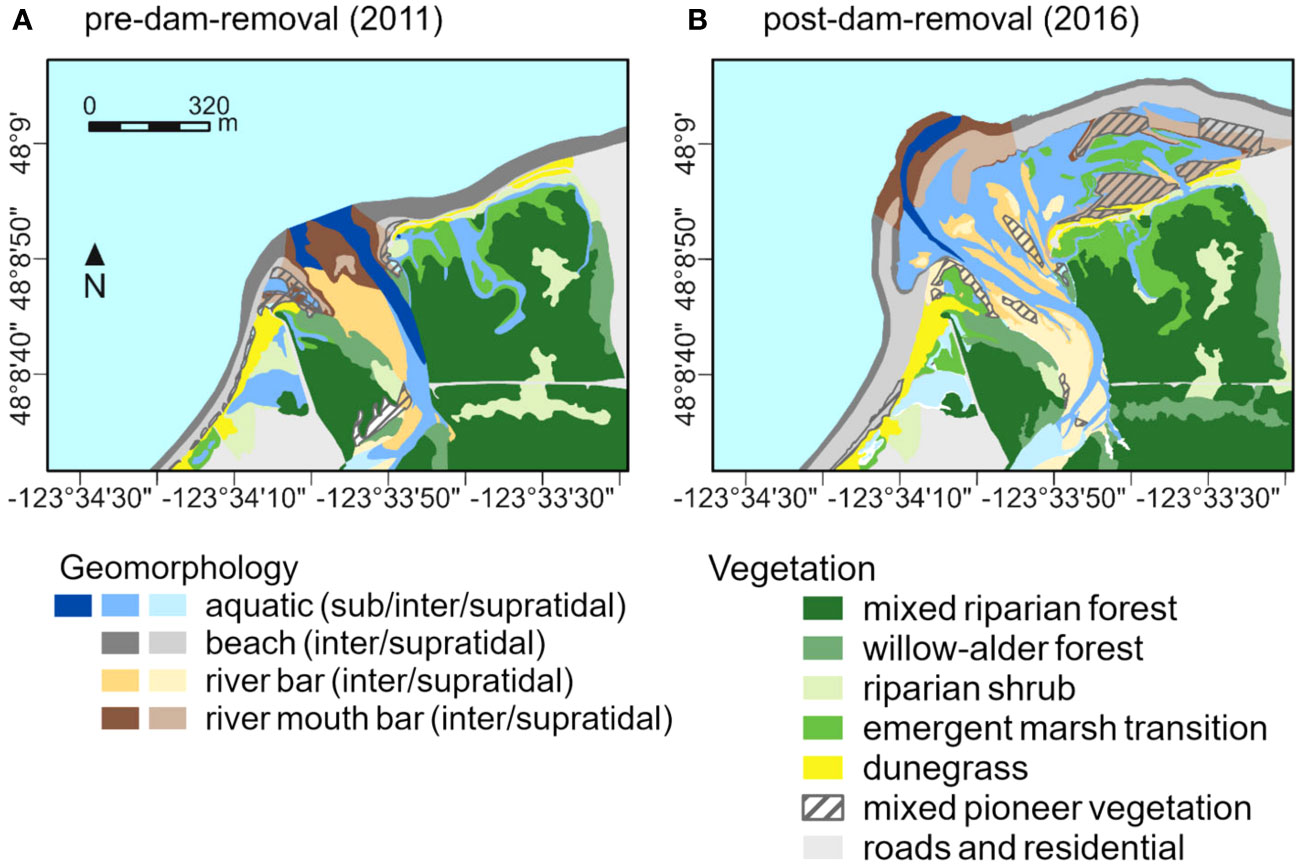
Figure 6 Change in vegetation and geomorphic surface cover in the Elwha River delta and estuary (A) before dam removal (2011) versus (B) after dam removal (2016). Geomorphic surfaces were separated into subtidal, intertidal, and supratidal areas based on elevations of mean lower low water and mean higher high water. New surfaces that formed in the delta and estuary following dam removal were colonized by mixed pioneer and emergent marsh vegetation. Polygons were drawn from aerial imagery; the figure is a modified version of a figure in Perry et al. (2023).
Surfaces in the delta and estuary that already existed prior to dam removal also received former reservoir sediments during and after dam removal. During the first five years of dam removal, an estimated 0.02 Mt of sediment were deposited within the pre-dam-removal delta and estuary, and what had been estuary area before dam removal was considered part of the lower river after 2012, as the new estuary developed 500 m farther seaward (Ritchie et al., 2018). Correspondingly, surface elevations in established emergent marsh, dunegrass, and riparian shrub communities in the delta and estuary increased by 31 ± 20 cm (range=2-67 cm) between 2007 and 2018 (Perry et al., 2023).
5.3 Vegetation responses in the delta and estuary
Vegetation responses to sediment deposition and landform dynamics in the delta and estuary were examined using (1) time series of aerial imagery to assess vegetation establishment on new surfaces and changes in cover of different vegetation types on older surfaces and (2) time series of field plot sampling to assess developing plant community composition on new surfaces and changes in community composition on older surfaces (Shafroth et al., 2011; Foley et al., 2017b; Perry et al., 2023).
By 2018, vegetation had established on 16.4 ha that had been unvegetated prior to dam removal, on both new surfaces in the delta and estuary and extant surfaces that aggraded, stabilized, and/or became protected from wave action during dam removal (Figure 2C; Perry et al., 2023). Surfaces that supported new vegetation tended to have been relatively stable in elevation for at least three years prior to vegetation establishment and were higher in elevation and farther from the new shoreline than unvegetated new surfaces. On-going channel migration and sediment reworking between 2013 and 2018 led to destruction and/or turnover of establishing vegetation on some new surfaces, especially during winter storms (Figure 2C). In particular, between 2016 and 2018, ~1.6 ha of new-surface vegetation reverted to unvegetated surfaces, as coastal erosion and sediment redistribution moved the outermost new river mouth bars and beaches inland towards the former shoreline. These trends of net shoreline erosion and loss of new vegetation have continued through 2022 (P. Shafroth, personal observation).
Vegetation development on stable new surfaces in the delta and estuary followed early-successional trajectories, with mixed pioneer vegetation (i.e., vegetation too young and undeveloped to be defined as a particular established community type on aerial imagery) on new supratidal beaches, river bars, and river mouth bars, and early-successional emergent marsh vegetation (i.e., communities dominated by obligate wetland species but with lower perennial, graminoid, and native plant cover and higher annual/biennial cover than well-established emergent marsh) in new intertidal aquatic habitats (Perry et al., 2023). Compositional differences between vegetation on new surfaces and well-established community types in the delta and estuary decreased over time following surface stabilization, as plant cover and species richness on new surfaces increased for graminoids, herbaceous forbs, perennials, annual/biennials, native species, and non-native species. By 2018, an average of 11 ± 1 native species had established per 100 m2 on vegetated new surfaces (Figure 4). Further, nearly one hectare that initially established as mixed pioneer vegetation had matured into dunegrass communities and willow-alder forest discernible on aerial imagery, suggesting that given time, vegetation on persistent new surfaces will mature into typical, later-successional delta and estuarine community types.
By contrast, sediment deposition within plant communities that were already established in the delta and estuary prior to dam removal had few discernible effects on vegetation abundance or composition (Foley et al., 2017b; Perry et al., 2023). Contrary to expectations, negative effects of sediment deposition on plant survival and cover were not apparent. Most temporal changes in established community composition during and after dam removal likely reflected natural successional processes, including increases in woody cover, decreases in non-native species richness, increases in wetland adaptation (community-weighted mean wetland indicator value) in emergent marshes, and development of riparian shrub communities into willow-alder forest (Perry et al., 2023). However, decreases in Leymus mollis (American dunegrass) and increases in shrub cover in dunegrass communities east of the river mouth were not typical of natural dunegrass succession and may have been related to dam removal. The extensive new surfaces that formed seaward of the dunes in this portion of the delta shielded these dunegrass communities from wave action and salt spray to which they are adapted. This, perhaps together with changes in soil chemistry or texture caused by deposition of former reservoir sediments (Figure 2C), may have facilitated shrub establishment, growth, and competitive ability, altering community composition on the former dunes (Perry et al., 2023). Shrub cover continued to increase in these former dunegrass communities from 2018 to 2022 (L. Perry, personal observation).
Bare ground on new surfaces and on sediment deposits in established communities may have facilitated invasion by short-lived, disturbance-adapted non-native species in the delta and estuary. During and after dam removal (2014, 2018), 34 non-native species were observed in the delta and estuary vegetation plots that were not observed prior to dam removal (2007) (Perry et al., 2023). The majority were annual/biennial grasses and forbs. Over half of these species also occurred upstream along the Elwha River, suggesting that their propagules may have reached the delta via hydrochory following dam removal (Figure 2C; Brown et al., 2022; Perry et al., 2023). While one third of these new species were observed only in plots on new surfaces, the other two thirds invaded established community types, most often dunegrass communities, in addition to or instead of new surfaces. However, neither the proportion of total species richness in the delta that was composed of non-native species nor the number of non-native species per plot increased following dam removal, suggesting that local extinction of other non-native species was sufficient to counterbalance the increase in non-native annual/biennials.
6 Discussion
Our review of vegetation changes associated with dam removals on the Elwha River – the world’s largest dam removal to date – was based on >35 publications covering vegetation responses in different landscape positions along the river and various before/after-control/impact studies, with and without active management (Table 1). Knowledge of vegetation dynamics is important not only for understanding plant communities, but also for better understanding other riverine ecosystem responses given the strong connections between vegetation, physical processes, aquatic and terrestrial habitats, and biota (Figure 2; Bellmore et al., 2019). Our review and synthesis provide new insights regarding the connections between fluvial geomorphic processes and vegetation responses and the effects of active management (e.g., weed control, vegetation planting), with implications and lessons that can help to inform dam removal monitoring efforts world-wide.
6.1 Connections between sediment, river morphodynamics, and vegetation following dam removal
In the case of the Elwha River dam removals, vegetation changes were primarily driven by processes related to the exposure, erosion, transport, and deposition of the large volume of sediment that had accumulated in the two reservoirs for nearly a century. These sediment-related processes connected the vegetation responses longitudinally (up- to downstream) in three different landscape contexts along the river: the former reservoirs, the river corridor, and the delta and estuary (Figure 2). In all three landscape positions, many plant species established on fresh sediment deposits (Figure 4), expanding vegetated habitat on new surfaces in the former reservoirs (Figure 3) and the river delta and estuary (Figure 6), and increasing species richness on floodplains and gravel bars along the river corridor between the two former dams (Figure 4). Sediment and landform dynamics during and after dam removal and throughout the affected parts of the Elwha River system underpinned the vegetation responses.
The connections between sediment dynamics and vegetation responses in multiple parts of a river system have not been reported in other dam-removal studies, though they are not surprising given the myriad relationships and feedbacks that commonly characterize fluvial geomorphic processes and riparian vegetation (Hupp and Osterkamp, 1996; Gurnell et al., 2016; Merritt, 2022). The large dam sizes and large volume of sediment released on the Elwha River (the most of any dam removal worldwide) led to the strong, system-wide signal. Virtually all other published studies of vegetation responses to dam removal have been associated with small dam removals and restricted to former reservoirs (e.g., Orr and Stanley, 2006). Geomorphic change downstream of small dam-removal sites, or large dam removals where the sediment release is deliberately limited, is typically minimal (Collins et al., 2020; Cashman et al., 2021; East et al., 2023), which translates to minor vegetation change. Also, riparian vegetation responses to dam removal have been studied much less than physical environmental variables or other biota such as fish or aquatic macroinvertebrates (Bellmore et al., 2017).
In natural riparian ecosystems, flood disturbance and fluvial dynamics drive spatiotemporal patterns, which have been described as a “shifting habitat mosaic” or “dynamic patch mosaic” (Stanford et al., 2005; Latterell et al., 2006). New patches of riparian vegetation are typically composed of pioneer species that establish on bare, moist sediments on landforms created by flood disturbance, such as gravel bars or low floodplains. Over time, landforms that support young stands of vegetation tend to aggrade, rendering them less vulnerable to destruction by future floods and providing conditions for vegetation to grow, further stabilize the landform, and facilitate establishment and growth of later-seral species (Naiman et al., 2010; Merritt, 2022). The character of the shifting habitat mosaic had been altered by the dams on the Elwha River (Shafroth et al., 2016).
The timeline of vegetation responses to dam removal on the Elwha River was closely related to the timeline of sediment and LW processes and dynamics (Ritchie et al., 2018; Leung, 2019). The pulse of sediment and LW caused the river corridor and delta downstream of the former reservoirs to be in a transport-limited state temporarily after dam removal began (particularly over the winter of 2012–2013), and then a sediment-supply-limited state beginning ~4-6 years after the start of dam removal (East et al., 2018). Surfaces on which vegetation can grow and persist need to be stable for months to years; thus, the transport-limited period when channels and near-channel landforms were unstable on the Elwha River (East et al., 2018) was characterized by conditions that were unfavorable for new vegetation establishment. During the ~6-month-long transport-limited period, significant geomorphic change occurred even in the absence of large peak flows. Rapid erosion and transport of reservoir sediment within the first three years following dam removal, often in the absence of high flows, has been documented in numerous cases (Wilcox et al., 2014; Foley et al., 2017a; Major et al., 2017), suggesting that the timeline on the Elwha River was not unique, although the sediment mass and response magnitude were larger than in any previous dam removal. LW transport and new logjam formation within the former reservoirs and in the two river segments and delta downstream likely interacted with sediment to promote the development of new fluvial surfaces where vegetation could establish near the main channel, in side channels, and in the delta (Figure 2; Fetherston et al., 1995; Abbe and Montgomery, 1996; Naiman et al., 2010; Leung, 2019).
Dam removal and the associated large disturbance event led to a large short-term increase in young fluvial surfaces that were colonized by pioneer vegetation, but in the longer term a return to more of a quasi-equilibrium, shifting habitat mosaic is expected. Over time on the new pioneer bars and former reservoir terraces and valley walls associated with dam removal, the importance of sediment-related processes should decrease relative to biological processes such as vegetation growth and plant community succession over decades to centuries (Latterell et al., 2006; Van Pelt et al., 2006). Conceptual models of ecological responses suggest that different potential trajectories could follow dam removal depending on variables such as the duration of downstream sediment effects, the abundance of non-native species, and whether other significant anthropogenic perturbations persist after dam removal (Bellmore et al., 2019).
6.2 Species composition considerations
The combination of new pioneer surfaces, sediment deposition and plant burial on extant surfaces, and expanded hydrochory was expected to influence vegetation community composition following dam removal along the Elwha River (Figure 2). For example, sediment burial was expected to reduce red alder survival and growth while new surfaces and sediment dynamics were expected to favor greater black cottonwood and willow establishment, potentially altering riparian forest composition and structure (Shafroth et al., 2002). Rather than declining, red alder increased in cover on bars and floodplains along the middle and lower river segments (Brown et al., 2022). Sediment burial had few large or lasting effects on downstream vegetation following dam removal (Brown et al., 2022; Perry et al., 2023), perhaps because typical burial depths (~15-60 cm; Brown et al., 2022; Perry et al., 2023) were insufficient to influence plants other than herbaceous species and woody seedlings (Lowe et al., 2010; Kui and Stella, 2016; Politti et al., 2018). As expected, however, cottonwood and willow established on new surfaces in the former reservoirs, on new gravel bars along the river corridor, and on new river mouth bars in the delta (Brown et al., Chenoweth et al., 2023; Perry et al., 2023). This was part of a broader trend of increased abundance of early-successional species from the former reservoirs downstream to the delta because of the increase in new, young landforms. Similarly, flushing of sediment from dams and associated deposition and bar development downstream promoted colonization of pioneer plants along the Kurobe River, Japan (Asaeda and Rashid, 2012).
The prevalence of disturbed pioneer habitat associated with dam removal has made potential invasion by non-native species a common management concern (Tullos et al., 2016). Along the Elwha River, pioneer surfaces created during and after dam removal were often invaded by non-native species, particularly weedy annuals (Schuster, 2015; Brown et al., 2022; Perry et al., 2023). However, early-successional native species were generally more abundant, and non-native species did not come to dominate pioneer vegetation in any of the three landscape positions, at least not within the first seven years during and after dam removal (Figure 4). Invasive vegetation control efforts in the former reservoirs may have reduced hydrochorous seed dispersal to downstream areas for at least some non-native species. The rapid, natural establishment of early-successional, native species also may have reduced opportunities for expansion of non-native species populations, emphasizing the benefits of local seed sources for disturbance-adapted native species in the context of dam removals (Figure 2).
In the longer term, vegetation dynamics and species composition might be influenced by the return of anadromous fish and corresponding deliveries of marine-derived nutrients (Figure 2). Nutrient subsidies from anadromous fish carcasses can affect riparian plant growth (Helfield and Naiman, 2001) and community composition (Mathewson et al., 2003; Bartz and Naiman, 2005; Wilkinson et al., 2005), with effects becoming evident within two decades (Quinn et al., 2018). In the years following the Elwha River dam removals, anadromous Pacific salmon (Oncorhynchus spp.) have returned to spawn above the former dams (Duda et al., 2021). Thus far there is limited evidence to suggest that riparian soils upstream of the former dams have been enriched with marine-derived nutrients (Kane et al., 2020), but marine-derived nutrients have been re-incorporated into freshwater and riparian food webs (Tonra et al., 2015). Returning salmon provide a seasonal food source for numerous mammal and bird species (Cederholm et al., 1989), many of which affect plant communities by disseminating marine-derived nutrients in their wastes (Ben-David et al., 1998; Hilderbrand et al., 1999; Helfield and Naiman, 2006). Animals that forage on salmon may also affect plant communities through zoochory. For example, salmon-supported bears (Ursus spp.) secondarily consume large quantities of fruit, and changes in salmon abundance may alter local bear distributions and patterns of seed dispersal (Harrer and Levi, 2018). Taken together with the observed effects of wildlife on revegetation in and around LW accumulations in the former reservoirs (Johnson et al., 2023), these findings illustrate the reciprocal roles that can be played by vegetation and wildlife in ecological restoration: wildlife benefit from restored habitats, and their activities in turn affect patterns of vegetation growth and restoration outcomes (McCaffery et al., 2018).
6.3 Managing vegetation in former reservoirs
The timing and duration of reservoir drawdown are important variables influencing vegetation trajectories in former reservoirs (Figure 2A; Shafroth et al., 2002). Slow reservoir drawdowns over several months are more likely to promote colonization by a variety of species (Chenoweth et al., 2023). On the Elwha River, timing the reservoir drawdown during Salicaceae (cottonwood and willow) seed dispersal led to rapid vegetation development on newly exposed landforms (Chenoweth et al., 2022). Given the ubiquity of cottonwood and willow taxa across the northern hemisphere and strong interest in restoring forests dominated by these genera (González et al., 2018), results from the Elwha River could help to inform management decisions in other dam removal contexts. The successful establishment of cottonwood despite the eventually deep water table and relatively rapid water decline was somewhat surprising, since these variables have been shown to limit cottonwood establishment in many other situations (Mahoney and Rood, 1998). However, Auble et al. (2007) also reported successful cottonwood establishment beyond these limits following a reservoir drawdown in Colorado. Relatively high annual precipitation along the Elwha River may have enabled survival of cottonwoods and willows even on landforms > 6 m above the alluvial water table.
Active management in the former reservoirs generally enhanced revegetation efforts. Inconsistencies in the results of seeding and planting may have been due to variations in conditions during plant establishment, such as those related to drawdown timing and moisture availability (Shafroth et al., 2002; Auble et al., 2007). Some inconsistency may also have been due to variations in monitoring methods, variation in sampling intensity on different landforms, differing years sampled, or discrepancies between planting and monitoring locations, such as in cases where plots established for monitoring planting success might have been only partially planted and included naturally-occurring vegetation (Chenoweth et al., 2023). Invasive vegetation control was largely effective, as evidenced by the fact that neither of the former reservoirs was dominated by non-native species, and these efforts were bolstered by other forms of active management, particularly seeding (Morgan, 2018; Cendejas-Zarelli, 2021). Similarly, seeding and planting efforts were bolstered by LW placement, as evidenced by the increased rates of survivorship observed in planting sites with LW installations (Calimpong, 2014; Johnson et al., 2023) and the increased species richness observed where seeding was paired with LW (Cendejas-Zarelli, 2021). Together, these findings point to the benefits of multiple forms of active management, undertaken in concert, for revegetating large areas exposed after dam removal.
The strong influence of sediment texture on natural revegetation in the former reservoirs (Schuster, 2015; Morgan, 2018; Prach et al., 2019; Chenoweth et al., 2022) suggests that considering sediment texture is important when planning active revegetation in former reservoirs following dam removal (Figure 2A). Seeding and planting efforts tended to be most effective and necessary on coarse sediments, where natural revegetation was more limited. The fact that fine sediment did not inhibit natural revegetation ran counter to pre-dam-removal predictions based on planting trials using dredged reservoir sediments in pots and raised beds (Chenoweth et al., 2011; Michel et al., 2011). This may have been due to artificial conditions that altered sediment characteristics or plant performance within the growing containers (Poorter et al., 2012; Kawaletz et al., 2014), suggesting that field experiments may be more useful for predicting revegetation success under different former reservoir conditions.
6.4 Monitoring vegetation responses to future dam removals
Studies of vegetation along the Elwha River before, during, and after two large dam removals captured many of the key responses and indicate methodological strengths and weaknesses that could provide insights when planning other dam-removal studies. Multiple factors led to a several year delay in the start of dam removals, which had the positive effect of providing more time and opportunity for pre-dam-removal data collection, including Before-After-Control-Impact study designs in some cases (e.g., East et al., 2018; Brown et al., 2022). These baseline data enabled more confident interpretations of dam-removal effects (e.g., Foley et al., 2017b; Brown et al., 2022; Perry et al., 2023); studies of future dam removals would benefit from similar pre-dam-removal data collection efforts. Some of the vegetation studies on the Elwha used similar sampling methodologies (e.g., Schuster, 2015; Morgan, 2018; Brown et al., 2022; Perry et al., 2023), which could facilitate future comparisons of vegetation in the different landscape positions along the river. In contrast, use of different methodologies complicated comparisons of multiple vegetation datasets in the former reservoirs (Chenoweth et al., 2023). We suggest that analysis of remotely sensed data (e.g., aerial imagery) to assess vegetation changes associated with dam removal along the Elwha River has been underutilized thus far (but see Perry et al., 2023). Assessments using unmanned aerial vehicles (UAVs; drones) could be particularly fruitful and have been used to assess changes in topography and vegetation in two small dam-removal case studies in New Hampshire, USA (Evans et al., 2022). Finally, given the close connections and interactions between fluvial geomorphic dynamics and vegetation dynamics on the Elwha, more interdisciplinary collaborations among physical and biological scientists could have strengthened the understanding and interpretations of vegetation responses. That said, monitoring on the Elwha River has been very successful largely due to effective collaborations within a diverse coalition of researchers and resource managers (Eitzel et al., 2023).
7 Conclusions
On the Elwha River, erosion, transport, and deposition of reservoir sediment were key drivers of vegetation responses to dam removal, from the former reservoirs to the river delta. Consistent with predictions made prior to dam removal, there was rapid revegetation of drained reservoirs; increased bar formation, hydrochory, and plant diversity in the river segment below Glines Canyon Dam; and colonization of new delta surfaces by emergent marsh and pioneer plant communities, an indication that dam removal has been effective thus far for restoring native plant communities. Comparing the dam-affected portions of the river with an upstream reference reach before and after dam removal led to the conclusion that the observed increases in species richness below Glines Canyon Dam were due to dam removal (Figure 4). However, plant species richness did not increase in the lower river segment (Figure 4), potentially due to burial-related mortality of some species and because this reach had more gravel bars and higher tributary sediment supply than the middle segment before dam removal. Non-native species did not come to dominate newly exposed or deposited landforms following dam removal on the Elwha River (Figure 4), another positive outcome. Active management (invasive vegetation control and planting and seeding) within the drained reservoirs may have reduced the abundance of target invasive species and may have limited downstream spread of invasive species onto newly formed river bar and delta surfaces. Plant communities may continue to change and diversify in the lower river segment as the river channel adjusts to a higher sediment supply post-dam removal. Our review covers the first 5-7 years after dam removal, but given the time required for forest succession, full understanding of recovery on the Elwha River will require long-term monitoring over decades.
As the frequency and scale of dam removal increases globally, detailed information about the ecological responses to past dam removals is needed to inform future research and management efforts. The differences in vegetation responses among river segments as well as among major landscape positions highlight the importance of understanding the unique setting of any dam removal. Factors such as reservoir sediment storage, the degree to which the dam altered the natural flow regime, constraints on channel migration, and surrounding land use can all affect vegetation dynamics. With most of its watershed contained within Olympic National Park, the Elwha River provided a unique opportunity to understand how vegetation responds to dam removal in a relatively natural, forested ecosystem. This allowed researchers to better isolate dam and dam-removal effects from other factors, such as urbanization, roadways, or agricultural development. Other systems may have more complex vegetation responses and higher risk of plant invasion after dam removal, such as the Klamath River, where large dam removal is currently underway. In contrast to the Elwha, the Klamath R. has a larger watershed that crosses several ecoregions with extensive agricultural development and grazing, as well as historic logging and mining (East and Grant, 2023). Going forward, it will be important to conduct multi-factorial studies to examine how dam removal interacts with other types of land use to anticipate and mitigate undesirable effects.
Author contributions
PS: Conceptualization, Project administration, Writing – original draft, Writing – review & editing. LP: Writing – original draft, Writing – review & editing, Visualization, Project administration. JH: Writing – original draft, Writing – review & editing. JC: Writing – original draft. RB: Writing – review & editing, Visualization.
Funding
The author(s) declare financial support was received for the research, authorship, and/or publication of this article. The U.S. Geological Survey supported LP’s participation, and publication costs.
Acknowledgments
Thanks to the organizers of the Elwha River ScienceScape Symposium (August 2022), which inspired the development of this manuscript. Thanks also to the Resources Legacy Fund and Lower Elwha Klallam Tribe for their support of the overall Elwha River ScienceScape project. Funding to support LP was provided by the U.S. Geological Survey. We thank J. Bauman and C.M. Werner for feedback on the initial outline of this manuscript. Thanks to A. East and two journal reviewers for helpful suggestions on earlier versions of the manuscript. Any use of trade, firm, or product names is for descriptive purposes only and does not imply endorsement by the U.S. Government.
Conflict of interest
The authors declare that the research was conducted in the absence of any commercial or financial relationships that could be construed as a potential conflict of interest.
Publisher’s note
All claims expressed in this article are solely those of the authors and do not necessarily represent those of their affiliated organizations, or those of the publisher, the editors and the reviewers. Any product that may be evaluated in this article, or claim that may be made by its manufacturer, is not guaranteed or endorsed by the publisher.
References
Abbe T. B., Montgomery D. R. (1996). Large woody debris jams, channel hydraulics and habitat formation in large rivers. Regulated Rivers-Research Manage. 12, 201–221. doi: 10.1002/(Sici)1099-1646(199603)12:2/3<201::Aid-Rrr390>3.0.Co;2-A
Acker S. A., Beechie T. J., Shafroth P. B. (2008). Effects of a natural dam-break flood on geomorphology and vegetation on the Elwha River, Washington, USA. Northwest Sci. 82, 210–223. doi: 10.3955/0029-344x-82.S.I.210
American Rivers (2023) Database of U.S. dam removals. Available at: https://figshare.com/articles/dataset/American_Rivers_Dam_Removal_Database/5234068.
Asaeda T., Rashid M. H. (2012). The impacts of sediment released from dams on downstream sediment bar vegetation. J. Hydrology 430, 25–38. doi: 10.1016/j.jhydrol.2012.01.040
Auble G. T., Shafroth P. B., Scott M. L., Roelle J. E. (2007). Early vegetation development on an exposed reservoir: Implications for dam removal. Environ. Manage. 39, 806–818. doi: 10.1007/s00267-006-0018-z
Baker R. (2013). Elwha River revegetation project: 2012 Lake Aldwell seeding trials (Seattle, WA: M.S. Thesis University of Washington).
Bartz K. K., Naiman R. J. (2005). Effects of salmon-borne nutrients on riparian soils and vegetation in southwest Alaska. Ecosystems 8, 529–545. doi: 10.1007/s10021-005-0064-z
Bellmore J. R., Duda J. J., Craig L. S., Greene S. L., Torgersen C. E., Collins M. J., et al. (2017). Status and trends of dam removal research in the United States. WIREs Water 4. doi: 10.1002/wat2.1164
Bellmore J. R., Pess G. R., Duda J. J., O’Connor J. E., East A. E., Foley M. M., et al. (2019). Conceptualizing ecological responses to dam removal: if you remove it, what’s to come? Bioscience 69, 26–39. doi: 10.1093/biosci/biy152
Ben-David M., Hanley T. A., Schell D. M. (1998). Fertilization of terrestrial vegetation by spawning Pacific salmon: the role of flooding and predator activity. Oikos 83, 47–55. doi: 10.2307/3546545
Bountry J., Crain P., Chenoweth J., Randle T., Ritchie A. (2015). “Role of adaptive sediment management in Elwha dam removal,” in 10th Federal Interagency Sedimentation Conference and 5th Federal Interagency Hydrologic Modeling Conference, Reno, Nevada. (Reno, NV: Advisory Committee on Water Information). Available at: https://acwi.gov/sos/pubs/3rdJFIC/Contents/10B-Bountry.pdf.
Brown R. L., Chenoweth J. (2008). The effect of Glines Canyon Dam on hydrochorous seed dispersal in the Elwha River. Northwest Sci. 82, 197–209. doi: 10.3955/0029-344x-82.S.I.197
Brown R. L., Thomas C. C., Cubley E. S., Clausen A. J., Shafroth P. B. (2022). Does large dam removal restore downstream riparian vegetation diversity? Testing predictions on the Elwha River, Washington, USA. Ecol. Appl. 32. doi: 10.1002/eap.2591
Calimpong C. (2014). Elwha River revegetation 2013: a plant performance study. M.S. Thesis (Seattle, WA: University of Washington).
Cashman M. J., Gellis A. C., Boyd E., Collins M. J., Anderson S. W., McFarland B. D., et al. (2021). Channel response to a dam-removal sediment pulse captured at high-temporal resolution using routine gage data. Earth Surface Processes Landforms 46, 1145–1159. doi: 10.1002/esp.5083
Cederholm C. J., Houston D. B., Cole D. L., Scarlett W. J. (1989). Fate of coho salmon (Oncorhynchus-Kisutch) carcasses in spawning streams. Can. J. Fisheries Aquat. Sci. 46, 1347–1355. doi: 10.1139/f89-173
Cendejas-Zarelli S. (2021). The effect of large woody debris, direct seeding, and distance from the forest edge on species composition on novel terraces following dam removal on the Elwha River, WA. M.S. Thesis (Bellingham, WA: Western Washington University). Available at: https://cedar.wwu.edu/wwuet/1003.
Chenoweth J. (2007). Predicting seed germination in the sediments of Lake Mills after the removal of the Glines Canyon Dam on the Elwha River. M.S. Thesis (Seattle, WA: University of Washington).
Chenoweth J., Acker S. A., McHenry M. L. (2011). Revegetation and restoration plan for Lake Mills and Lake Aldwell (Port Angeles, WA: Olympic National Park and the Lower Elwha Klallam Tribe).
Chenoweth J., Bakker J. D., Acker S. A. (2022). Planting, seeding, and sediment impact restoration success following dam removal. Restor. Ecol. 30. doi: 10.1111/rec.13506
Chenoweth J., Shafroth P. B., Brown R. L., Helfield J. M., Bauman J. M., Cendejas-Zarelli S., et al. (2023). A review of natural and managed revegetation responses in two de-watered reservoirs after large dam removals on the Elwha River, Washington, USA. Front. Ecol. Evol., 11. doi: 10.3389/fevo.2023.1268969
Citron E. (2017). Black cottonwood (Populus trichocarpa) nutrition in the dewatered Lake Aldwell reservoir on the Elwha River, Washington. M.S. Thesis (Bellingham, WA: Western Washington University). Available at: https://cedar.wwu.edu/wwuet/466
Clausen A. J. (2012). Riparian understory dynamics and relationship to dams on the Elwha River, Washington. M.S. Thesis (Cheney, WA: Eastern Washington University). Available at: https://dc.ewu.edu/theses/78.
Collins M. J., Kelley A. R., Lombard P. J. (2020). River channel response to dam removals on the lower Penobscot River, Maine,United States. River Res. Appl. 36, 1778–1789. doi: 10.1002/rra.3700
Colton M. (2018). Daytime summer microclimate influence of large woody debris on dewatered sediments in Lake Mills, WA, following dam removal. M.S. Thesis (Bellingham, WA: Western Washington University). Available at: https://cedar.wwu.edu/wwuet/712/.
Cook K. L., Wallender W. W., Bledsoe C. S., Pasternack G., Upadhyaya S. K. (2011). Effects of native plant species, mycorrhizal inoculum, and mulch on restoration of reservoir sediment following dam removal, Elwha River, Olympic Peninsula, Washington. Restor. Ecol. 19, 251–260. doi: 10.1111/j.1526-100X.2009.00559.x
Cortese A. (2014). Mycorrhizal availability in the basin of Lake Mills and influence on colonization and growth of Salix scouleriana under drought stress. M.S. Thesis (Bellingham, WA: Western Washington University). Available at: https://cedar.wwu.edu/wwuet/340.
Cortese A. M., Bunn R. A. (2017). Availability and function of arbuscular mycorrhizal and ectomycorrhizal fungi during revegetation of dewatered reservoirs left after dam removal. Restor. Ecol. 25, 63–71. doi: 10.1111/rec.12406
Cubley E. S. (2015). Initial response of riparian vegetation to dam removal on the Elwha River, Washington. M.S. Thesis (Cheney, WA: Eastern Washington University).
Cubley E. S., Brown R. L. (2016). Restoration of hydrochory following dam removal on the Elwha River, Washington. River Res. Appl. 32, 1566–1575. doi: 10.1002/rra.2999
Czuba C. R., Randle T. J., Bountry J. A., Magirl C. S., Czuba J. A., Curran C. A., et al. (2011). “Anticipated sediment delivery to the lower Elwha River during and following dam removal,” in Coastal habitats of the Elwha river, Washington - biological and physical patterns and processes prior to dam removal. US geological survey scientific investigations report 2011-5120. Eds. Duda J. J., Warrick J. A., Magirl C. S. (Reston, Virginia: US Geological Survey), 27–46.
Draut A. E., Logan J. B., Mastin M. C. (2011). Channel evolution on the dammed Elwha River, Washington, USA. Geomorphology 127, 71–87. doi: 10.1016/j.geomorph.2010.12.008
Draut A. E., Ritchie A. C. (2015). Sedimentology of new fluvial deposits on the Elwha River, Washington, USA, formed during large-scale dam removal. River Res. Appl. 31, 42–61. doi: 10.1002/rra.2724
Duda J. J., Bellmore J. R. (2022). “Dam removal and river restoration,” in Encyclopedia of inland waters, 2nd ed. Eds. Mehner T., Tockner K. (Amsterdam: Elsevier), 576–585. doi: 10.1016/B978-0-12-819166-8.00101-8
Duda J. J., Freilich J. E., Schreiner E. G. (2008). Baseline studies in the Elwha River ecosystem prior to dam removal: Introduction to the special issue. Northwest Sci. 82, 1–12. doi: 10.3955/0029-344x-82.S.I.1
Duda J. J., Torgersen C. E., Brenkman S. J., Peters R. J., Sutton K. T., Connor H. A., et al. (2021). Reconnecting the Elwha River: spatial patterns of fish response to dam removal. Front. Ecol. Evol. 09. doi: 10.3389/fevo.2021.765488
East A. E., Grant G. E. (2023). A watershed moment for western U.S. dams. Water Resour. Res. 59. doi: 10.1029/2023WR035646
East A. E., Harrison L. R., Smith D. P., Logan J. B., Bond R. M. (2023). Six years of fluvial response to a large dam removal on the Carmel River, California, USA. Earth Surface Processes Landforms 48, 1487–1501. doi: 10.1002/esp.5561
East A. E., Logan J. B., Mastin M. C., Ritchie A. C., Bountry J. A., Magirl C. S., et al. (2018). Geomorphic evolution of a gravel-bed river under sediment-starved versus sediment-rich conditions: river response to the world’s largest dam removal. J. Geophysical Research-Earth Surface 123, 3338–3369. doi: 10.1029/2018jf004703
East A. E., Pess G. R., Bountry J. A., Magirl C. S., Ritchie A. C., Logan J. B., et al. (2015). Large-scale dam removal on the Elwha River, Washington, USA: river channel and floodplain geomorphic change. Geomorphology 228, 765–786. doi: 10.1016/j.geomorph.2014.08.028
Eitzel M. V., Meyer R., Morley S., Miller I., Shafroth P. B., Behymer C., et al. (2023). Lessons learned from community and citizen science monitoring on the Elwha River restoration project. Front. Ecol. Evol. 11. doi: 10.3389/fevo2023/1216080
Evans A. D., Gardner K. H., Greenwood S., Still B. (2022). UAV and structure-from-motion photogrammetry enhance river restoration monitoring: a dam removal study. Drones 6. doi: 10.3390/drones6050100
Fetherston K. L., Naiman R. J., Bilby R. E. (1995). Large woody debris, physical process, and riparian forest development in montane river networks of the Pacific-Northwest. Geomorphology 13, 133–144. doi: 10.1016/0169-555x(95)00033-2
Foley M. M., Bellmore J. R., O’Connor J. E., Duda J. J., East A. E., Grant G. E., et al. (2017a). Dam removal: listening in. Water Resour. Res. 53, 5229–5246. doi: 10.1002/2017wr020457
Foley M. M., Warrick J. A., Ritchie A., Stevens A. W., Shafroth P. B., Duda J. J., et al. (2017b). Coastal habitat and biological community response to dam removal on the Elwha River. Ecol. Monogr. 87, 552–577. doi: 10.1002/ecm.1268
Francis R. A., Tibaldeschi P., McDougall L. (2008). Fluvially-deposited large wood and riparian plant diversity. Wetlands Ecol. Manage. 16, 371–382. doi: 10.1007/s11273-007-9074-2
González E., Martínez-Fernández V., Shafroth P. B., Sher A. A., Henry A. L., Garófano-Gómez V., et al. (2018). Regeneration of Salicaceae riparian forests in the Northern Hemisphere: a new framework and management tool. J. Environ. Manage. 218, 374–387. doi: 10.1016/j.jenvman.2018.04.069
Gregory S. V., Swanson F. J., Mckee W. A., Cummins K. W. (1991). An ecosystem perspective of riparian zones. Bioscience 41, 540–551. doi: 10.2307/1311607
Gurnell A. M., Corenblit D., De Jalón D. G., Del Tánago M. G., Grabowski R. C., O’Hare M. T., et al. (2016). A conceptual model of vegetation-hydrogeomorphology interactions within river corridors. River Res. Appl. 32, 142–163. doi: 10.1002/rra.2928
Harrer L. E. F., Levi T. (2018). The primacy of bears as seed dispersers in salmon-bearing ecosystems. Ecosphere 9. doi: 10.1002/ecs2.2076
Helfield J. M., Naiman R. J. (2001). Effects of salmon-derived nitrogen on riparian forest growth and implications for stream productivity. Ecology 82, 2403–2409. doi: 10.1890/0012-9658(2001)082[2403:Eosdno]2.0.Co;2
Helfield J. M., Naiman R. J. (2006). Keystone interactions: salmon and bear in riparian forests of alaska. Ecosystems 9, 167–180. doi: 10.1007/s10021-004-0063-5
Hilderbrand G. V., Hanley T. A., Robbins C. T., Schwartz C. C. (1999). Role of brown bears (Ursus arctos) in the flow of marine nitrogen into a terrestrial ecosystem. Oecologia 121, 546–550. doi: 10.1007/s004420050961
Hulce H. (2009). Vegetation colonization and seed bank analysis of Lake Mills deltas: pre-dam removal analysis for post-dam removal insight. M.S. Thesis (Cheney, WA: Eastern Washington University).
Hupp C. R., Osterkamp W. R. (1996). Riparian vegetation and fluvial geomorphic processes. Geomorphology 14, 277–295. doi: 10.1016/0169-555X(95)00042-4
Johnson C., Douglas C., Mansmith T., McLaughlin J. (2023). Large wood supports Elwha revegetation by reducing ungulate browsing. Front. Ecol. Evol. 11. doi: 10.3389/fevo.2023.1215144
Kane W. (2018). Monitoring the influx of marine derived nitrogen and soil food webs of Northern Olympic Peninsula riparian zones. M.S. Thesis (Cheney, WA: Eastern Washington University).
Kane W., Brown R., Bastow J. (2020). Monitoring the return of marine-derived nitrogen to riparian areas in response to dam removal on the Elwha River, Washington. Northwest Sci. 94, 118–128. doi: 10.3955/046.094.0203
Kardouni J. (2020). Forest restoration of the exposed Lake Mills bed: assessing vegetation, ectomycorrhizae, and nitrogen relative to riverbank lupine (Lupinus rivularis). M.S. Thesis (Bellingham, WA: Western Washington University).
Kardouni J., Danilchik Lindsay M., Labay A., Bauman J. M. (2023). Riverbank lupine’s (Lupinus rivularis) influence on conifer growth, ectomycorrhizal colonization, and neighboring vegetation in coarse sediments left behind after dam removal. Front. Ecol. Evol. 11. doi: 10.3389/fevo.2023.1214117
Kawaletz H., Mölder I., Annighöfer P., Terwei A., Zerbe S., Ammer C. (2014). Pot experiments with woody species - a review. Forestry 87, 482–491. doi: 10.1093/forestry/cpu017
Kloehn K. K., Beechie T. J., Morley S. A., Coe H. J., Duda J. J. (2008). Influence of dams on river-floodplain dynamics in the Elwha River, Washington. Northwest Sci. 82, 224–235. doi: 10.3955/0029-344x-82.S.I.224
Kui L., Stella J. C. (2016). Fluvial sediment burial increases mortality of young riparian trees but induces compensatory growth response in survivors. For. Ecol. Manage. 366, 32–40. doi: 10.1016/j.foreco.2016.02.001
Labay A. (2013). Impact of riverbank lupine (Lupinus rivularis) on grand fir (Abies grandis) ectomycorrhizal symbioses. M.S. Thesis (Bellingham, WA: Western Washington University). Available at: https://cedar.wwu.edu/wwuet/1165.
Latterell J. J., Bechtold J. S., O’Keefe T. C., Van Pelt R., Naiman R. J. (2006). Dynamic patch mosaics and channel movement in an unconfined river valley of the Olympic Mountains. Freshw. Biol. 51, 523–544. doi: 10.1111/j.1365-2427.2006.01513.x
Leung V. (2019). Large woody debris and river morphology in scour pool formation, dam removal, and delta formation. Ph.D. Dissertation (Seattle, WA: University of Washington).
Lisius G. L., Snyder N. P., Collins M. J. (2018). Vegetation community response to hydrologic and geomorphic changes following dam removal. River Res. Appl. 34, 317–327. doi: 10.1002/rra.3261
Lowe B. J., Watts R. J., Roberts J., Robertson A. (2010). The effect of experimental inundation and sediment deposition on the survival and growth of two herbaceous riverbank plant species. Plant Ecol. 209, 57–69. doi: 10.1007/s11258-010-9721-1
MaChado S., Paulsen G. M. (2001). Combined effects of drought and high temperature on water relations of wheat and sorghum. Plant Soil 233, 179–187. doi: 10.1023/A:1010346601643
Mahoney J. M., Rood S. B. (1998). Streamflow requirements for cottonwood seedling recruitment – an integrative model. Wetlands 18, 634–645. doi: 10.1007/BF03161678
Major J. J., East A. E., O’Connor J. E., Grant G. E., Wilcox A. C., Magirl C. S., et al. (2017). “Geomorphic responses to dam removal in the United States: a two-decade perspective,” in Gravel-bed rivers: processes and disasters. Eds. Tsutsumi D., Laronne J. B. (Chichester, UK: Wiley-Blackwell), 355–383.
Mathewson D. D., Hocking M. D., Reimchen T. E. (2003). Nitrogen uptake in riparian plant communities across a sharp ecological boundary of salmon density. BMC Ecol. 3, 4–15. doi: 10.1186/1472-6785-3-4
McCaffery R., Jenkins K. J., Cendejas-Zarelli S., Happe P. J., Sager-Fradkin K. A. (2020). Small mammals and ungulates respond to and interact with revegetation processes following dam removal. Food Webs 25. doi: 10.1016/j.fooweb.2020.e00159
McCaffery R., McLaughlin J., Sager-Fradkin K., Jenkins K. J. (2018). Terrestrial fauna are agents and endpoints in ecosystem restoration following dam removal. Ecol. Restor. 36, 97–107. doi: 10.3368/er.36.2.97
McLaughlin J. F. (2013). Engaging birds in vegetation restoration after Elwha dam removal. Ecol. Restor. 31, 46–56. doi: 10.3368/er.31.1.46
Merritt D. M. (2022). “Reciprocal relations between riparian vegetation, fluvial landforms, and channel processes,” in Treatise on geomorphology, 2nd edition. Ed. Shroder J. F. (Cambridge, MA: Elsevier), 269–297.
Michel J. T., Helfield J. M., Hooper D. U. (2011). Seed rain and revegetation of exposed substrates following dam removal on the Elwha River. Northwest Sci. 85, 15–29. doi: 10.3955/046.085.0102
Morgan O. A. (2018). Vegetation community development after dam removal on the Elwha River. M.S. Thesis (Cheney, WA: Eastern Washington University). Available at: https://dc.ewu.edu/theses/523/.
Naiman R. J., Bechtold J. S., Beechie T. J., Latterell J. J., Van Pelt R. (2010). A process-based view of floodplain forest patterns in coastal river valleys of the Pacific Northwest. Ecosystems 13, 1–31. doi: 10.1007/s10021-009-9298-5
Naiman R. J., Decamps H. (1997). The ecology of interfaces: riparian zones. Annu. Rev. Ecol. Systematics 28, 621–658. doi: 10.1146/annurev.ecolsys.28.1.621
Naiman R. J., Décamps H., Pollock M. M. (1993). The role of riparian corridors in maintaining regional biodiversity. Ecol. Appl. 3, 209–212. doi: 10.2307/1941822
Naiman R. J., Fetherston K. L., McKay S. J., Chen J. (1998). “Riparian forests,” in River ecology and management: lessons from the pacific coastal ecoregion. Eds. Naiman R. J., Bilby R. E. (New York, NY: Springer-Verlag), 289–323.
Nilsson C., Brown R. L., Jansson R., Merritt D. M. (2010). The role of hydrochory in structuring riparian and wetland vegetation. Biol. Rev. 85, 837–858. doi: 10.1111/j.1469-185X.2010.00129.x
O’Connor J. E., Duda J. J., Grant G. E. (2015). 1000 dams down and counting. Science 348, 496–497. doi: 10.1126/science.aaa9204
Orr C. H., Stanley E. H. (2006). Vegetation development and restoration potential of drained reservoirs following dam removal in Wisconsin. River Res. Appl. 22, 281–295. doi: 10.1002/rra.891
Perry L. G., Shafroth P. B., Alfieri S. J., Miller I. M. (2023). Coastal vegetation responses to large dam removal on the Elwha River. Front. Ecol. Evol. 11. doi: 10.3389/fevo.2023.1233903
Pizzuto J. (2002). Effects of dam removal on river form and process. Bioscience 52, 683–691. doi: 10.1641/0006-3568(2002)052[0683:Eodror]2.0.Co;2
Pohl M. (2004). Channel bed mobility downstream from the Elwha dams, Washington. Prof. Geographer 56, 422–431. doi: 10.1111/j.0033-0124.2004.05603010.x
Politti E., Bertoldi W., Gurnell A., Henshaw A. (2018). Feedbacks between the riparian Salicaceae and hydrogeomorphic processes: a quantitative review. Earth-Science Rev. 176, 147–165. doi: 10.1016/j.earscirev.2017.07.018
Poorter H., Bühler J., van Dusschoten D., Climent J., Postma J. A. (2012). Pot size matters: a meta-analysis of the effects of rooting volume on plant growth. Funct. Plant Biol. 39, 839–850. doi: 10.1071/Fp12049
Prach K., Chenoweth J., del Moral R. (2019). Spontaneous and assisted restoration of vegetation on the bottom of a former water reservoir, the Elwha River, Olympic National Park, WA, USA. Restor. Ecol. 27, 592–599. doi: 10.1111/rec.12915
Quinn T. P., Helfield J. M., Austin C. S., Hovel R. A., Bunn A. G. (2018). A multidecade experiment shows that fertilization by salmon carcasses enhanced tree growth in the riparian zone. Ecology 99, 2433–2441. doi: 10.1002/ecy.2453
Randle T. J., Bounty J. A., Ritchie A., Wille K. (2015). Large-scale dam removal on the Elwha River, Washington, USA: erosion of reservoir sediment. Geomorphology 246, 709–728. doi: 10.1016/j.geomorph.2014.12.045
Ravot C., Laslier M., Hubert-Moy L., Dufour S., Le Coeur D., Bernez I. (2020). Large dam removal and early spontaneous riparian vegetation recruitment on alluvium in a former reservoir: Lessons learned from the pre-removal phase of the Sélune River project (France). River Res. Appl. 36, 894–906. doi: 10.1002/rra.3535
Ritchie A. C., Warrick J. A., East A. E., Magirl C. S., Stevens A. W., Bountry J. A., et al. (2018). Morphodynamic evolution following sediment release from the world’s largest dam removal. Sci. Rep. 8. doi: 10.1038/s41598-018-30817-8
Schmitz D., Blank M., Ammondt S., Patten D. T. (2009). Using historic aerial photography and paleohydrologic techniques to assess long-term ecological response to two Montana dam removals. J. Environ. Manage. 90, S237–S248. doi: 10.1016/j.jenvman.2008.07.028
Schuster J. L. (2015). Vegetation colonization within exposed reservoirs following dam removal on the Elwha River, Washington (Cheney, Washington: Eastern Washington University). Available at: https://dc.ewu.edu/theses/310/.
Shafroth P. B., Friedman J. M., Auble G. T., Scott M. L., Braatne J. H. (2002). Potential responses of riparian vegetation to dam removal. Bioscience 52, 703–712. doi: 10.1641/0006-3568(2002)052[0703:Prorvt]2.0.Co;2
Shafroth P. B., Fuentes T. L., Pritekel C., Beirne M., Beauchamp V. B. (2011). “Vegetation of the elwha river estuary,” in Coastal habitats of the Elwha river, Washington - biological and physical patterns and processes prior to dam removal. US geological survey scientific investigations report 2011-5120. Eds. Duda J. J., Warrick J. A., Magirl C. S. (Reston, VA: US Geological Survey), 225–247.
Shafroth P. B., Perry L. G., Rose C. A., Braatne J. H. (2016). Effects of dams and geomorphic context on riparian forests of the Elwha River, Washington. Ecosphere, 7. doi: 10.1002/ecs2.1621
Stanford J. A., Lorang M. S., Hauer F. R. (2005). The shifting habitat mosaic of river ecosystems. Internationale Vereinigung für theoretische und angewandte Limnologie: Verhandlungen 29, 123–136. doi: 10.1080/03680770.2005.11901979
Thomas C. (2018). Riparian vegetation and the soil seed bank five years after dam removal on the Elwha River, Washington (Cheney, WA: Eastern Washington University). Available at: https://dc.ewu.edu/theses/530.
Tonra C. M., Sager-Fradkin K., Morley S. A., Duda J. J., Marra P. P. (2015). The rapid return of marine-derived nutrients to a freshwater food web following dam removal. Biol. Conserv. 192, 130–134. doi: 10.1016/j.biocon.2015.09.009
Tullos D. D., Collins M. J., Bellmore J. R., Bountry J. A., Connolly P. J., Shafroth P. B., et al. (2016). Synthesis of common management concerns associated with dam removal. J. Am. Water Resour. Assoc. 52, 1179–1206. doi: 10.1111/1752-1688.12450
U.S. Department of the Interior, U.S, Department of Commerce and Lower Elwha S’Klallam Tribe (1994). The Elwha Report: Restoration of the Elwha River Ecosystem and Native Anadromous Fisheries. Report submitted pursuant to Public Law 102–495 (Port Angeles: Washington).
Van Pelt R., O’Keefe T. C., Latterell J. J., Naiman R. J. (2006). Riparian forest stand development along the Queets River in Olympic National Park, Washington. Ecol. Monogr. 76, 277–298. doi: 10.1890/05-0753
Wahid A., Gelani S., Ashraf M., Foolad M. R. (2007). Heat tolerance in plants: an overview. Environ. Exp. Bot. 61, 199–223. doi: 10.1016/j.envexpbot.2007.05.011
Ward L., Crain P., Freymond B., McHenry M., Morrill D., Pess G., et al. (2008). Elwha river fish restoration plan–developed pursuant to the Elwha river ecosystem and fisheries restoration act, public law 102-495. U.S. Dept. Commer., NOAA Tech. Memo. NMFS-NWFSC-90, 168 p. Available at: https://repository.library.noaa.gov/view/noaa/3569.
Warrick J. A., Bountry J. A., East A. E., Magirl C. S., Randle T. J., Gelfenbaum G., et al. (2015). Large-scale dam removal on the Elwha River, Washington, USA: source-to-sink sediment budget and synthesis. Geomorphology 246, 729–750. doi: 10.1016/j.geomorph.2015.01.010
Warrick J. A., Draut A. E., McHenry M. L., Miller I. M., Magirl C. S., Beirne M. M., et al. (2011). “Geomorphology of the Elwha River and its delta,” in Coastal habitats of the Elwha river, Washington - biological and physical patterns and processes prior to dam removal. US geological survey scientific investigations report 2011-5120. Eds. Duda J. J., Warrick J. A., Magirl C. S. (Reston, Virginia: US Geological Survey), 47–73.
Warrick J. A., George D. A., Gelfenbaum G., Ruggiero P., Kaminsky G. M., Beirne M. (2009). Beach morphology and change along the mixed grain-size delta of the dammed Elwha River, Washington. Geomorphology 111, 136–148. doi: 10.1016/j.geomorph.2009.04.012
Warrick J. A., Stevens A. W., Miller I. M., Harrison S. R., Ritchie A. C., Gelfenbaurn G. (2019). World’s largest dam removal reverses coastal erosion. Sci. Rep. 9. doi: 10.1038/s41598-019-50387-7
Whisman M. (2013). Revegetation of post-dam-removal riparian sediments in the Lower Elwha River, WA. M. S. Thesis (Olympia, WA: Evergreen State College).
Wilcox A. C., O’Connor J. E., Major J. J. (2014). Rapid reservoir erosion, hyperconcentrated flow, and downstream deposition triggered by breaching of 38 m tall Condit Dam, White Salmon River, Washington. JGR Earth Surface 119, 1376–1394. doi: 10.1002/2013JF003073
Wilkinson C. E., Hocking M. D., Reimchen T. E. (2005). Uptake of salmon-derived nitrogen by mosses and liverworts in coastal British Columbia. Oikos 108, 85–98. doi: 10.1111/j.0030-1299.2005.13277.x
Woodward A., Torgersen C., Chenoweth J., Beirne K., Acker S. (2011) Predicting spread of invasive exotic plants into dewatered reservoirs after dam removal on the Elwha River, Olympic National Park, Washington. Open-File Report 2011-1048. U.S. Geological Survey. Available at: https://pubs.usgs.gov/of/2011/1048/pdf/ofr20111048.pdf.
Keywords: ecogeomorphology, sediment pulse, riparian vegetation, river restoration, drained reservoir, coastal vegetation, river delta, active revegetation
Citation: Shafroth PB, Perry LG, Helfield JM, Chenoweth J and Brown RL (2024) Vegetation responses to large dam removal on the Elwha River, Washington, USA. Front. Ecol. Evol. 12:1272921. doi: 10.3389/fevo.2024.1272921
Received: 04 August 2023; Accepted: 26 January 2024;
Published: 13 February 2024.
Edited by:
Jean-Marc Roussel, IFREMER, FranceReviewed by:
Lorena Rodríguez-Gallego, Universidad de la República, UruguayLoïs Morel, Institut Agro Rennes-Angers, France
Copyright © 2024 Shafroth, Perry, Helfield, Chenoweth and Brown. This is an open-access article distributed under the terms of the Creative Commons Attribution License (CC BY). The use, distribution or reproduction in other forums is permitted, provided the original author(s) and the copyright owner(s) are credited and that the original publication in this journal is cited, in accordance with accepted academic practice. No use, distribution or reproduction is permitted which does not comply with these terms.
*Correspondence: Patrick B. Shafroth, shafrothp@usgs.gov