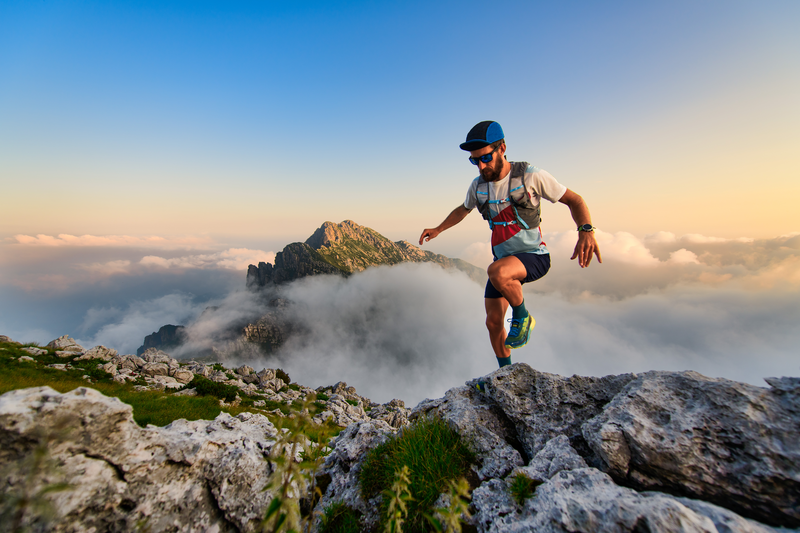
94% of researchers rate our articles as excellent or good
Learn more about the work of our research integrity team to safeguard the quality of each article we publish.
Find out more
ORIGINAL RESEARCH article
Front. Ecol. Evol. , 06 October 2023
Sec. Conservation and Restoration Ecology
Volume 11 - 2023 | https://doi.org/10.3389/fevo.2023.1246824
This article is part of the Research Topic Regeneration Mechanisms and Tradeoffs of Ecosystem Function after Drastic Environmental Changes View all 7 articles
Oxyria sinensis has the characteristics of drought resistance, barren resistance, pollution resistance and clonal reproduction, and has the potential to be developed as a restoration plant (pioneer plant) for vegetation restoration and heavy metal pollution control in mining wasteland. In this study, the rhizosphere microbial communities of the pioneer plant O. sinensis growing in a lead-zinc mine wasteland and the rhizosphere microbial communities of O. sinensis growing in adjacent non-mining areas were studied by field investigation. There were significant differences in the composition of microbial communities between the rhizosphere soil of O. sinensis and the bare soil. There were significant differences in the composition of soil microbial communities between the non-mining area control and the lead-zinc mine wasteland. Compared with the non-mining area control, the rhizosphere soil of O. sinensis in the lead-zinc mine wasteland had a unique microbial community. The dominant bacteria were Nitrospirae, Chloroflexi, Proteobacteria, Actinobacteria, Ascomycota and Kickxellomycota. Further gene function prediction showed that the metabolic pathway sequences related to heavy metal ion transport, heavy metal resistance and repair in the rhizosphere soil of O. sinensis were more abundant than those in the control. The dominant ecological functional groups of fungi in the rhizosphere soil of O. sinensis were saprophytic flora and pathological flora. Plenodomus, Surfurifusis, Sphingomonas, Filobasidium and Articulospora were enriched in the rhizosphere soil of O. sinensis in the abandoned land of lead-zinc mining area, and had high tolerance to heavy metals in the soil, indicating that O. sinensis can recruit heavy metal-tolerant microbial groups to promote its survival and adaptation in the abandoned land of the mining area.
Abandoned land in mining areas refers to the land that has been destroyed in mining, transportation, smelting and other mining processes and cannot exert normal ecological functions without restoration (Saavedra-Mella et al., 2022). Abandoned land, tailings and waste residues often remain after mining, causing serious soil pollution, water pollution and air pollution (Wu et al., 2023), posing a major threat to human health and environmental safety (Briffa et al., 2020). Therefore, the restoration and stabilization of mining wasteland has become one of the environmental issues of global concern. So far, the research on the restoration and management of mining wasteland can be divided into four main directions (Sebelikova et al., 2019; Wu et al., 2021): (1) Waste land reuse; (2) Physical repair method; (3) Chemical repair method; (4) Phytoremediation. Combined with the comprehensive analysis of policy, time, cost and effect, phytoremediation is considered to be the most widely used method for the restoration of abandoned land in mining areas (Saini et al., 2021). By introducing and screening hyperaccumulator-tolerant plants, it is particularly effective in dealing with large-scale mining wastelands polluted by organic matter, nutrients and metal pollutants (Stumbea et al., 2019). Relevant studies have shown that large-scale planting of hyperaccumulators cannot form stable and sustainable plant communities, and the soil of mining wasteland often faces factors such as extreme pH, high salinity, low water retention capacity, high concentration of heavy metals, and insufficient soil organic matter and fertility, which is not conducive to the growth of hyperaccumulators (Wu et al. 2021; Wang et al., 2022).
Choosing the right plant species is one of the most important considerations in the process of plant repair. Appropriate plant species should be able to tolerate high metal content and extreme soil conditions, such as high acidity, salinity or alkalinity (Wu et al., 2021). In addition, plants used for revegetation should have other favorable attributes, such as dense roots, relatively fast growth rates, and high biomass production (Jiang et al., 2022). The use of native species in the process of plant restoration has a great advantage (Yuan et al., 2022). Studies have found that native pioneer material species in arid areas have developed several adaptive abilities that enable them to resist extreme environments and create better conditions for the emergence of other species through natural evolution, tending to the process of ecosystem restoration (Wang et al., 2022). For native plants in the mining area, the high content of heavy metal elements in the soil is a stress and a selective pressure, which provides impetus for the adaptive evolution of some plants under adversity (Rahmonov et al., 2022). Stress-resistant plants that can naturally settle on abandoned land in mining areas have their own unique adaptive stress-resistant mechanisms (Frérot et al., 2018), so that they can survive, grow and reproduce under heavy metal pollution and poor environmental conditions (Sha et al., 2023), and gradually become dominant species.
Plant rhizosphere microbial communities have been shown to play an important role in promoting plant growth and heavy metal uptake (Liu et al., 2023). The composition and function of plant rhizosphere microorganisms often change dramatically during long-term adaptation to the mine environment to tolerate high heavy metal concentrations (Zhong et al., 2022). Rhizosphere soil area refers to the soil area adjacent to plant roots and affected by root growth activities. The diversity of microbial community is high (Lan et al., 2021), which is considered to be one of the most complex and meaningful areas in soil science research. Plant rhizosphere microbial communities establish a complex bond between plants and soil (Wang et al., 2022). Beneficial soil microorganisms can improve plant nutrient uptake (Abdelkrim et al., 2020) and enhance plant tolerance to different biotic or abiotic stresses, such as heavy metals, drought, high temperature, acidity, alkalinity, salinity, etc. (Bang et al., 2022). In the plant rhizosphere environment, on the one hand, plant roots can release root exudates and recruit special microorganisms to construct rhizosphere microbial communities (Hu et al., 2018; Jiang et al., 2022); on the other hand, rhizosphere microorganisms can also regulate the interaction between plants and the environment through various biochemical reactions (Zhen et al., 2019), thereby promoting plant growth and improving ecological environment functions (Gazitua et al., 2019). Rhizosphere soil includes plant growth-promoting rhizobacteria (PGPR), such as Pesudomonas sp, Bacillus sp, and AM fungi, which are involved in the cycle of nitrogen, phosphorus, sulfur and some important elements. PGPR can improve vegetation in mining areas by absorbing and fixing nitrogen for plants, providing bioavailable phosphorus and inhibiting soil-borne pathogens of plants (Guo et al., 2022), and is related to plant tolerance to heavy metals. It can improve the tolerance of plants and plays an important role in phytoremediation of heavy metal-contaminated environments (Bierza et al., 2020). EM fungi can promote the establishment of plant species in heavy metal contaminated sites by immobilizing heavy metals in soil, thereby reducing the availability of metals to plants (Zhang Y. H. et al., 2022).
Yunnan Province is known as the “non-ferrous metal kingdom”. Huize County in the jurisdiction is an important mining area of lead-zinc mineral resources in China. Continuous development has provided strong resource support for China’s industrial development, but the resulting soil pollution problems have also increased, and left a large area of wasteland. Due to the low intensity of human disturbance in the later period, many pioneer plants with strong resistance were naturally settled in the Huize lead-zinc mining area. Oxyria sinensis is a perennial herb of the genus Polygonum. It can be cloned and grown through rhizomes, and has strong drought resistance (Lei et al., 2013). It has the characteristics of growth and clonal reproduction in the mining area, and can be used as an important pioneer plant for vegetation restoration in the mining area. However, the tolerance characteristics of O. sinensis world at present are still unclear. In order to explore the tolerance and adaptation mechanism of local stress-resistant plants to long-term continuous heavy metal pollution and its influence on the shaping of rhizosphere microbial community, this study decided to take the pioneer plant O. sinensis in the abandoned land of Huize lead-zinc mine area as the research object after long-term field investigation. Through the exploration and analysis of its rhizosphere microbial community, the adaptive mechanism of extreme habitats in the abandoned land of the mining area was revealed, so as to provide theoretical reference for the subsequent restoration of plant communities in the region.
The study area is located in Kuangshan Town, Huize County, Yunnan Province (103.03° E, 25.48° N). It is located in the northeast of Yunnan Province and belongs to the plateau monsoon climate. The average altitude is 2300 m, the average annual temperature is 12.5°C, the annual precipitation is 800 mm, and the natural soil type is yellow brown soil. It has been about 70 years (1950~1990) of lead-zinc smelting history and is now mostly abandoned. The vegetation in this area is sparse, dominated by shrubs and herbs. O. sinensis is widely distributed in slag heap and abandoned land of lead-zinc mine with different years.
In May 2022, the distribution of O. sinensis in the abandoned land of Huize lead-zinc mine area was investigated through field investigation. Combined with the results of heavy metal content and altitude slope survey in the whole soil surface of the sample area, the slag heap (BT), abandoned for 5 years (NR5), abandoned for 15 years (NR15) and abandoned for 25 years (NR25) were selected as four experimental plots, and the uncontaminated area was used as the control plot (CK). A total of five plots were set up for research. Five healthy and similar-sized O. sinensis were randomly selected as sampling points in each plot. The rhizosphere soil and bare soil (located next to O. sinensis and not covered by plants) of O. sinensis were collected respectively, and a total of 50 samples of rhizosphere soil and bare soil of O. sinensis were collected. During sampling, the rhizomes of O. sinensis were carefully excavated and tracked. After accurately finding the roots of O. sinensis, the foreign bodies and foreign soil were removed, and the soil tightly bound to the roots was brushed with a clean brush as a rhizosphere soil sample. All samples were divided into two parts, one was used for the determination of soil physical and chemical properties and heavy metal content, and the other was divided into three parts after mixing the soil at different points in each of the five plots. A total of 30 samples were used for rhizosphere microbial extraction and measurement.
The collected soil samples were naturally dried and ground in the laboratory. The soil samples were screened by 2 mm sieve and 0.1 mm sieve. After mixing, they were placed in a self-sealing bag and numbered and marked for testing. Soil moisture content by drying method; soil bulk density, porosity and water holding capacity were measured by ring knife method. Soil pH was measured by acid–base indicator method, and soil organic carbon was oxidized by potassium dichromate. Soil total nitrogen was determined by Kjeldahl method; soil total phosphorus using acid soluble-molybdenum antimony colorimetric method; the content of heavy metal elements in soil was determined by nitric acid-perchloric acid-hydrofluoric acid digestion flame atomic absorption spectrometry. The available state of heavy metals in soil was determined by inductively coupled plasma mass spectrometry (ICP-MS).
FastPrep DNA extraction kit was used to extract DNA from the rhizosphere soil and bare soil samples of Polygonum cuspidatum. The quality of DNA extraction was detected by 1% agarose gel electrophoresis. The V3–V4 fragments of the extracted DNA were amplified by bacteria. Universal primers 338F: (5’-ACTCCTACGGGAGGCAGCA-3’), 806R: (5’-GGACTACHVGGGTWTCTAAT-3’); fungi amplification ITS1 fragment was extracted, universal primers ITS1F (5’-CTTGGTCATTTAGAGGAAGTAA-3’), ITS2R (5’-GCTGCGTTCTTCATCGATGC-3’). The qualified primers were sequenced using Illumina HiSeq 2500. (Beijing BioMike Biotechnology Co., Ltd.). Raw reads were filtered by Trimmomatic (v0.33), primer fragments were removed by cutadapt (v1.9.1), and Clean reads were spliced in FLASH (v1.2.7). The bacterial 16S rDNA was extracted for high-throughput sequencing, and a total of 3,60,484 pairs of Reads were obtained. After quality control and splicing of double-ended Reads, a total of 3,593,964 Clean Reads were generated. Each sample produced at least 79,419 Clean Reads, with an average of 79,866 Clean Reads. Fungal 16S rDNA was extracted from all samples for high-throughput sequencing. A total of 3123940 pairs of Reads were obtained from 30 samples. After quality control and splicing of double-ended Reads, a total of 3,114,027 Clean Reads were generated. Each sample produced at least 42,127 Clean Reads, with an average of 69,201 Clean Reads.
The data of heavy metal content and physical and chemical properties of the soil were statistically analyzed and sorted by Excel 2020 software. Statistical analysis was performed using IBM SPSS 26.0 software. Origin 2023 software was used to draw the diagram. One-way ANOVA was used to test the differences in heavy metal content and soil physical and chemical properties between plants and soils.
The pH value of the rhizosphere soil of O. sinensis was between 6.22 and 8.12 (Figure 1). With the increase of the abandoned years of the lead-zinc mining area, the pH value gradually decreased. The pH value of the rhizosphere soil of O. sinensis was significantly lower than that of the slag heap soil. The total carbon, total phosphorus and total nitrogen contents were 7.78~34.23 g · kg−1, 0.39~0.94 g · kg-−1, 0.31~0.80 g · kg−1, respectively. The total carbon and total phosphorus contents increased with the increase of the abandoned years, and the total nitrogen content increased first and then decreased. The average pH value of the bare soil was between 6.75 and 8.30. With the increase of the abandoned years of the lead-zinc mining area, the pH value gradually decreased. The pH value of the bare soil was significantly lower than that of the slag heap soil. The total carbon, total phosphorus and total nitrogen contents were 5.61~25.12, 0.50~1.31 g · kg−1 and 0.54~1.44 g · kg−1, respectively. The total carbon and total nitrogen contents increased with the increase of the abandoned years, and the total phosphorus content increased first and then decreased. The contents of Pb, Zn, Cd, Cu and Mn in the rhizosphere soil samples of O. sinensis were 158.3~8406.6, 381.5~13906.9, 4.5~38.6, 22.0~247.9 and 182.3~897.1 mg · kg−1, respectively (Figure 2). The content of heavy metals in the rhizosphere soil of lead-zinc mining area was significantly different from that of non-mining area. The available contents of Pb, Zn, Cd, Cu and Mn in the rhizosphere soil samples of O. sinensis were 27.3~189.7, 37.6~171.5, 0.2~0.4, 0.3~1.0 and 0.4~3.5 mg · kg−1, respectively (Figure 3). The available contents of heavy metals in the rhizosphere soil of abandoned lead-zinc mining areas were significantly different from those in non-mining areas.
Figure 1 Soil nutrient content in rhizosphere (R)/bare land (B) of Polygonum sinense in lead-zinc mining areas with different abandoned years. Different letters indicated that there were significant differences between different plots based on 95% confidence interval (p < 0.05). CK: non-mining area; BT: slag heap; NR5: abandoned for 5 years; NR15: 15 years abandoned; NR25: abandoned for 25 years. (A) pH; (B) TN; (C) TC; (D) TP.
Figure 2 The content of heavy metals in the rhizosphere (R)/bare land (B) soil of Polygonum huashanense in lead-zinc mining areas with different years: (A) soil Pb; (B) soil Zn; (C) soil Cd; (D) soil Cu; (E) soil Mn. Different letters indicated that there were significant differences between different plots based on 95% confidence interval (p < 0.05). CK: non-mining area; BT: slag heap; NR5: abandoned for 5 years; NR15: 15 years abandoned; NR25: abandoned for 25 years.
Figure 3 Heavy metal content in rhizosphere (R)/bare land (B) of Polygonum cuspidatum in different years of lead-zinc mining area in rainy season: (A) soil Pb; (B) soil Zn; (C) soil Cd; (D) soil Cu; (E) soil Mn. Different letters indicated that there were significant differences between different plots based on 95% confidence interval (p < 0.05). CK: non-mining area; BT: slag heap; NR5: abandoned for 5 years; NR15: 15 years abandoned; NR25: abandoned for 25 years.
The Chao1 index reflecting the richness was used to estimate the total number of species in a single sample (Table 1). There were significant differences among the three sample groups in each plot. With the increase of abandoned years, the richness of bacterial communities in the rhizosphere soil and bare soil of O. sinensis increased gradually. The two diversity indices showed similar trends, and the diversity index values of non-mining control and mining wasteland were significantly different. In all samples, the coverage Coverage was close to 1, indicating that the accuracy of sequencing and alignment results in this study was high and close to the true value. From the perspective of species richness, diversity or evenness, the rhizosphere soil of O. sinensis was larger than that of bare soil. In general, the results of α diversity analysis showed that the soil bacterial diversity of the five habitats was significantly different, and the soil bacterial richness of the slag heap was the lowest.
As shown in Table 2, there was no significant difference between the rhizosphere soil and bare soil samples in the non-contaminated control area in the fungal community. From the comprehensive comparison of the community richness ACE and Chao1 indexes, it can be seen that the ITS fungal abundance was the highest in the rhizosphere soil samples of the 15-year and 25-year lead-zinc mine wasteland, and the lowest in the slag heap. From the comprehensive comparison of Shannon and Simpson indexes of community species diversity, it can be seen that the ITS fungal biodiversity was the highest in the rhizosphere soil samples of the 15-year lead-zinc mine wasteland, and the lowest in the slag heap. The coverage of all samples was close to 1, indicating that the accuracy of sequencing and alignment results was high and close to the true value.
The results of bacterial OTUs obtained by clustering were plotted by Venn diagram (Figure 4). The number of OTUs in each sample in the Venn diagram was represented by a closed ellipse (set), and the number of OTUs shared by the samples was represented by an elliptical overlap (digital representation). From the number of OTUs, non-mining area > mining wasteland, rhizosphere soil > bare land. From the common and unique OTU analysis, there were 64 OTUs in 10 sample types, 175 OTUs in different rhizosphere soil samples, and 106 OUTs in different bare soil samples.
Figure 4 Common and unique OTU analysis of bacterial community sample groups. (A) Common and unique OTUs in 10 sample groups; histogram represents the total number of OTUs in soil samples in different plots; (B) Common and unique OTUs in the rhizosphere soil sample group of O. sinensis in lead-zinc mine wastelands with different abandoned years. (C) Common and unique OTUs in the bare soil sample group of lead-zinc mine wastelands with different abandoned years. CK: non-mining area; BT: slag heap; NR5: abandoned for 5 years; NR15: 15 years abandoned; NR25: abandoned for 25 years; R: Rhizosphere soil; N: Bare soil.
After homogenizing the collected soil samples, the results of fungal OTUs obtained by clustering were plotted by Venn diagram (Figure 5). As shown in Figure 5, there are 2439 OTUs in the rhizosphere soil samples and bare soil samples of O. sinensis. In addition, except for the soil samples of the abandoned land in the 5-year lead-zinc mining area, the number of OTUs in the rhizosphere soil samples of each sample is higher than that of the bare soil samples. From the number of OTUs, 15 years lead-zinc mine wasteland > slag heap, rhizosphere soil > bare soil. From the analysis of common and unique OTUs, there were 33 OTUs in 10 sample types, 89 OTUs in rhizosphere soil samples and 48 OUTs in bare soil samples.
Figure 5 Common and unique OTU analysis of fungal community sample groups. (A) Common and unique OTUs in 10 sample groups; histogram represents the total number of OTUs in soil samples in different plots; (B) Common and unique OTUs in the rhizosphere soil sample group of O. sinensis in lead-zinc mine wastelands with different abandoned years. (C) Common and unique OTUs in the bare soil sample group of lead-zinc mine wastelands with different abandoned years. CK: non-mining area; BT: slag heap; NR5: abandoned for 5 years; NR15: 15 years abandoned; NR25: abandoned for 25 years; R: Rhizosphere soil; N: Bare soil.
The classification and richness of bacterial communities at the level of phylum classification in each sample plot were analyzed. The “others” was used to represent the phylum with less than 1% abundance in all samples. The rank sum test was used to analyze the differences of different bacteria in each sample. The soil samples contained abundant bacterial phyla (Figure 6). Proteobacteria, Acidobacteriota, Gemmatimonadetes, Bacteroidetes, Actinobacteriota and Chloroflexi were distributed in all soils. Proteobacteria, Acidobacteriota and Bacteroidetes were the main dominant bacteria in the rhizosphere soil of O. sinensis, and Proteobacteria, Acidobacteriota and Gemmatimonadetes were the main dominant bacteria in the bare soil. Among them, Proteobacteria had the highest abundance and the highest proportion. The abundance of Proteobacteria was about 35.90%~48.87% in non-mining control soil and 37.95%~51.97% in mining wasteland soil. In general, the abundance of Proteobacteria in mining wasteland soil samples was higher than that in non-mining control soi. The abundance of Gemmatimonadetes in bare soil was significantly higher than that in rhizosphere soil. The relative abundances of Chloroflexi, Acidobacteriota and Bacteroideteswere increased by the colonization of O. sinensis in the mining wasteland. The relative abundance of Actinobacteriota was reduced. Among them, Acidobacteriota reached 20.3% in the rhizosphere soil of the abandoned mining area abandoned for 15 years, 14.8% in the slag pile, and Chloroflexi accounted for 6.5% in the slag pile rhizosphere soil.
Figure 6 The phylum level community composition of soil bacteria in different abandoned years of lead-zinc mine wasteland. CK: non-mining area; bT: slag heap; nR5: abandoned for 5 years; nR15: 15 years abandoned; nR25: 25 years abandoned; r: Rhizosphere soil; g: bare land; n: Bare soil. The same below.
Ascomycota (36.57%~75.84%) and Basidiomycota (10.26%~59.33%) were the main dominant phyla in the rhizosphere soil fungi of O. sinensis (Figure 7). Ascomycota (10.41%~60.82%), unclassified Fungi (10.21%~57.14%) and Basidiomycota (16.80%~23.38%) were the dominant phyla in the bare soil in the five plots. The effect of pollution conditions on the composition of fungal community was also obvious. Among them, the relative abundance of Ascomycota in the rhizosphere soil of slag heap was the highest (75.84%), and the lowest was 10.41% in the bare soil of 25-year lead-zinc mine wasteland; the relative abundance of Basidiomycota was the highest in non-mining control rhizosphere soil (59.33%) and the lowest in slag heap bare soil (6.80%). Some fungal phyla were only found in the soil of lead-zinc mine wasteland, such as Mucoromycota and Kickxellomycota. Olpidiomycota was only compared in the control rhizosphere soil of non-mining area, as well as in the rhizosphere soil of 15-year lead-zinc mine wasteland and 25-year lead-zinc mine wasteland.
Figure 7 The phylum level community composition of soil fungi in different abandoned years of lead-zinc mine wasteland.
The LEfSe significantly different bacteria at different classification levels were analyzed between the rhizosphere and bare soil of the five plots (Figure 8). There were 36 significantly different bacteria in the rhizosphere soil of five different plots (Figure 8A). There were 4 bacteria in the rhizosphere soil of the non-mining area, including Rhizobiales (Proteobacteria, Rhizobium) and Comamonadaceae (Proteobacteria, Comamonadaceae). There were 14 rhizosphere soils in the slag heap, including Chloroflexi, Nitrospira (Nitrospira, Nitrospira), Sphingomonas (Proteobacteria, Sphingomonas), etc. There were 7 rhizosphere soils in the abandoned land of lead-zinc mine in 5 years, including Chitinophagaceae (Bacteroidetes, Chitinophagaceae) and Hymenobacter. There were 8 rhizosphere soils in 15 years of lead-zinc mine wasteland, including Gemmatimonas (Gemmatimonas, Gemmatimonas), Xanthobacteraceae (Proteobacteria, Xanthobacteraceae), etc. There were three kinds of rhizosphere soil in the abandoned land of lead-zinc mine in 25 years, including Flavobacterium (Bacteroidetes, Flavobacteriaceae) and Flavobacteriaceae. There were 57 significantly different bacteria between the five different bare soil (Figure 8B), and 9 in the bare soil of the non-mining area, including Subgroup 7, Ellin6067, MND1; there are 19 species in the bare land of slag heap, including Chloroflexi, Chitinophageles (Bacteroidetes, Chitinophaga), Nitrospira (Nitrospira, Nitrospira), etc. There were 6 bare lands in the abandoned land of lead-zinc mine in 5 years, including Vicinamibacteraceae, Comamonadaceae (Proteobacteria, Comamonadaceae), etc. There were 11 bare lands in the abandoned land of lead-zinc mine in 15 years, including Acidobacteriales (Acidobacteriales, Acidobacteriales), Myxococcales (Proteobacteria, Myxococcales), etc. There were 12 bare lands in the abandoned land of lead-zinc mine in 25 years, including Cytophagales and Ehodospirillales (Proteobacteria, Marine Spiromycota).
Figure 8 LEfSe analysis of bacterial (A) and fungal (B) communities in rhizosphere soil of O. sinensis with different abandoned years.
There were 67 significantly different fungi among the five different rhizosphere soils (Figure 9A). There were 10 fungi in the rhizosphere soil of the non-mining area, including Aureobasidium, Dothideales and Lepiota. There were 16 genera in the rhizosphere soil of slag heap, including Plenodomus, Leptodontidium, Glomerellales and Chaetothyriales. There were 13 rhizosphere soils in the abandoned land of the lead-zinc mining area in the past 5 years, including Didymellaceae (Ascomycota, subseptococcaceae), Helotiaceae (Ascomycota, lepidomycota); a total of 13 rhizosphere soils in the abandoned land of the lead-zinc mining area in the past 15 years, including Pezizales (Ascomycota, Pedigree), Mortierella; there were 15 rhizosphere soils in the 25-year lead-zinc mine wasteland, including Nectriaceae and Botryotinia. There were 57 significantly different fungi among the five different bare soils (Figure 9B), and 21 fungi in the bare soil of the non-mining area, including Cladospsrium (Deuteromycotina, Cladosporium), Dothideales (Ascomycota, Ascomycota), etc.; a total of 11 slag heap bare land, including Cladosprium (Deuteromycetes, Cladosporium), Lecanicillium, etc.; there were 12 bare lands in the abandoned land of the lead-zinc mine in 5 years, including Pezizales (Ascomycota, Didymellaceae); there were 10 bare lands in the abandoned land of lead-zinc mine in 15 years, including Herpotrichiellaceae and Trechisporales (Agaricales, Pitylosporales). There are three bare lands in the abandoned land of lead-zinc mine in 25 years, including Basidiomycota.
Figure 9 LEfSe analysis of bacterial (A) and fungal (B) communities in rhizosphere soil of O. sinensis in bare land with different abandoned years.
PICRUSt function prediction analysis was performed on the 16 SrDNA fragment of bacterial sequence of 10 groups of soil samples. From the COG function (Figure 10) analysis, it can be seen that the metabolic related functions in 10 groups of samples are very rich. It mainly includes: General function prediction only, Amino acid transport and metabolism, Translation, ribosome structure and biogenesis, Energy production and conversion, Cell wall/membrane/envelope biogenesis. It is inferred that the microbial metabolism in the control area and the lead-zinc mining area is strong.
Figure 10 Relative abundance of COG gene function prediction in 10 sample groups. Different colors represent different gene functions.
KEGG database was used to compare the sequencing data, and different pathway levels (1–3) information and abundance of 10 groups of samples were obtained. There were 6 metabolic pathways in pathway level 1, 45 metabolic pathways in pathway level 2, and 320 metabolic pathways in pathway level 3. In different groups of soil samples, the metabolic pathway categories of each group were similar, but the abundance values were different. It can be seen from Table 3 that the metabolic pathways related to heavy metal ion transport, heavy metal resistance and remediation in soil samples are more abundant in lead-zinc mine wasteland. Compared with the non-mining control group (Table 4). The number of pathway sequences such as Basal transcription factors, Cysteine and methionine metabolism, Glutathione metabolism, Metabolism of xenobiotics by cytochrome P450, Calcium signaling pathway, Aminoacyl-tRNA biosynthesis and Sulfur metabolism was up-regulated in all samples of lead-zinc mine wasteland.
Table 3 Predicting the number of heavy metal-related sequences in rhizosphere soil at level 3 of KEGG metabolic pathway.
Table 4 The number of sequences related to heavy metals in bare soil predicted by KEGG metabolic pathway level 3.
According to the results of database annotation, the functional abundance of Trophic Mode and Guild was counted. The statistical results are as follows: As shown in Figure 11A, the main nutritional types of rhizosphere soil fungal community in the study area are pathotroph, saprotroph and symbiotroph. Plant Pathogen accounted for the highest proportion (31.6%) in the slag heap, and wood Sparotroph accounted for the highest proportion (48.6%) in the non-contaminated control. The main nutritional types of the bare soil fungal community (Figure 11B) in the study area are pathotroph, saprotroph and symbiotroph. Undefined Saprotroph fungi accounted for the highest proportion (53.1%) in the 15-year wasteland, and plant pathogens accounted for the highest proportion (36.8%) in the 25-year wasteland.
Figure 11 Prediction of fungal community function in rhizosphere soil (A) and bare soil (B) of lead-zinc mine wasteland with different abandoned years.
The bacterial community was mainly affected by heavy metals (Figure 12A). Chloroflexi and Myxomycota had strong tolerance to heavy metals, and Chloroflexi was significantly positively correlated with Cd, Pb, Zn and Cdexe. Myxococcota was significantly positively correlated with Cdexe, Znexe and Cuexe. However, heavy metal pollution has a significant inhibitory effect on some unclassified bacteria. Nutrient elements also significantly affected bacterial groups. TN and TP were negatively correlated with Gemmatimonadetes, and positively correlated with Chloroflexi and Bacteroidota. Among the top 30 soil bacterial genera (Figure 12B), 6 genera were significantly positively correlated with pH value and Cd, Pb and Zn, and negatively correlated with most soil physical and chemical properties, mainly including Bryobacter, Sphingomonas, Sulfurifustis, Thiobacillus, unclassified Chloroflexi and unclassified Mircroscillaceae. Among them, Sphingomonas and Sulfurifustis are the core or high-abundance species of lead-zinc mine wasteland soil. The core or high abundance species in the control soil of non-mining area were positively correlated with soil nutrient factors, and the contents of Cd, Pb and Zn were significantly negatively correlated.
Figure 12 Correlation heat map of bacterial phylum (A) and genus (B) with heavy metals. The number on the right side of the figure is the correlation coefficient, above 0 is positive correlation, below 0 is negative correlation; TC, total carbon; TN, total nitrogen; TP, total phosphorus; Pbexe, available lead; Cdexe, available cadmium; Znexe, available zinc; Cuexe, effective copper; Mnexe, effective state; *0.01 < p ≤ 0.05, **0.001 < p ≤ 0.01, ***p ≤ 0.001; the same below.
The fungal community was mainly affected by heavy metals (Figure 13A). Ascomycota had strong tolerance to heavy metals, and had a significant positive correlation with Cd and Pb. Heavy metal pollution of Cd, Pb and Zn has obvious inhibitory effect on Basidiomycetes. In addition, nutrients also significantly affected individual bacterial groups. TC and WAF were significantly positively correlated with Basidiomycota and Olpidomycota (Figure 13B); tN was significantly negatively correlated with Ascomycota. The correlation between soil dominant fungal genera (top 30) and environmental factors among them, 7 genera were significantly positively correlated with soil pH and the contents of Cd, Pb and Zn, and negatively correlated with most soil physical and chemical properties, mainly Plenodomus, Articulospora, unclassified Ascomycots, Filobasidium, Paraphoma and unclassified Chaetothyriales. Gymnopus and Lepiota were significantly negatively correlated with Cd, Pb and Zn, and positively correlated with soil nutrient factors.
Figure 13 Correlation heat map of fungal phylum (A) and genus (B) with heavy metals. * significant at 5% level, p < 0.05; ** significant at 1% level, p < 0.01; *** significant at the 0.1% significance level, p < 0.001.
The abandoned land of lead-zinc mining area in Huize mine town is polluted by several heavy metals such as Pb, Zn, Cd, Mn and Cu, and the concentration of Pb, Zn and Cd is far higher than the local soil background value and the national background value. The total amount of Pb, Cd and Zn in slag heap is higher than that in other plots, but the content of Mn is low, which may be related to the low content of loam and clay in slag heap and high pH (Lu et al., 2022). Soil contaminated by heavy metals has various adverse effects on plant growth, such as the reduction of plant nutrients, toxic concentration of heavy metals, salinity and alkalinity (Wu et al., 2023). Despite being contaminated by heavy metals, different plant species have achieved their adaptation through microevolutionary processes (Buch et al., 2020), which are determined by specific cellular mechanisms, including limiting and/or excluding free radical absorption of heavy metals, fixing heavy metals in the external environment through free radical exudates (Elizabeth et al., 2020), chelating ions in the epidermis, vacuoles and trichomes, and transferring heavy metals through xylem to branches (Singh et al., 2020). In general, the phytotoxicity of soil is about Zn (300 mg · kg−1), Pb (500~1000 mg · kg−1), Cd (1 mg · kg−1) (Saavedra-Mella et al., 2022). Therefore, the soil of the abandoned land in the Huize lead-zinc mining area will have a toxic effect on the plant. However, the field survey found that O. sinensis can grow normally in the abandoned land of the lead-zinc mining area. The observation results show that O. sinensis grown in the abandoned land of the lead-zinc mining area can tolerate higher heavy metal concentrations.
The content of nutrient elements such as TN and TP in soil is low, and the degree of soil pollution is very high. The pH value in the abandoned land of the lead-zinc mine area decreased significantly with the increase of the abandoned years. The total nitrogen and total carbon content increased with the increase of the abandoned years, and the total phosphorus content increased first and then decreased with the increase of the abandoned years. Compared with the rhizosphere soil, the total nitrogen and total phosphorus in the bare soil of the abandoned land in the mining area did not change significantly in the rainy season and the dry season, which may be related to the limited influence range of the root system (Zhang D. Z. et al., 2022). The root system can only affect the rhizosphere soil, so the nutrient content of some rhizosphere soil has significant differences in the abandoned lead-zinc mine areas with different abandoned years. The nutrient content of bare soil did not change much. In this study, the abandoned land in the Huize lead-zinc mining area is alkaline, and the colonization of O. sinensis in the abandoned land of the lead-zinc mining area can reduce the soil pH value to a certain extent, indicating that O. sinensis may change the conditions of the abandoned land of the mining area to promote its growth, and has a certain acidification effect on its rhizosphere soil (Frérot et al., 2018), which may be related to the release of H+ from the roots of O. sinensis, and then neutralize the charge imbalance caused by the uneven absorption of NH4-N and NO3-N (Antoniadis et al., 2021). With the increase of abandoned years, the total nitrogen and total carbon contents in the rhizosphere soil of the lead-zinc mine wasteland increased. This may be due to the large root system of P. sinensis, and the activity of the root system caused more nitrogen to accumulate in its roots (Navarro-Cano et al., 2019). At the same time, the plant roots will produce nitrogen-containing secretions to gradually increase the total nitrogen content. At the same time, as a perennial herb, P. sinensis has more litters and leaves, and its litters can be more returned to the soil, thereby increasing the total carbon content in the soil (Yin et al., 2023). Vegetation restoration can improve soil nutrient status, promote feedback between plants and soil, and then trigger basic ecosystem functions. Developed vegetation restoration can promote long-term and sustainable restoration of abandoned land in mining areas. The selection of plant species is the key to plant stability in abandoned land in mining areas (Zhu et al., 2021). As a local species in abandoned land of lead-zinc mining areas, P. zhonghuashanense has high tolerance to heavy metals such as Pb, Zn, Cd, Mn and Cu, and can adapt to local climatic conditions and soil environment, and increase the accumulation of total carbon and nutrients in soil to a certain extent. It is a suitable choice for vegetation restoration in abandoned land of lead-zinc mine area.
The diversity of soil bacterial and fungal communities in lead-zinc mine wasteland may be affected by a variety of changing environmental factors, including heavy metal pollution, soil physical and chemical properties and vegetation conditions (Yung et al., 2021). The obvious gradient of microbial diversity among soil habitats in different years of lead-zinc mine wasteland may be related to the strong environmental gradient caused by long-term heavy metal pollution in soil ecosystem (Zhen et al., 2019). Analysis of α diversity and β diversity. The composition structure of bacterial community between non-mining control samples and other samples was quite different, and the composition structure of bacterial community and fungal community between rhizosphere soil and bare soil in different plots was quite different. The fungal diversity of rhizosphere soil in lead-zinc mine wasteland was higher than that of non-mining control rhizosphere soil. Except for slag heap, the bacterial diversity of rhizosphere soil in other lead-zinc mine wasteland was higher than that of non-mining control rhizosphere soil. With the increase of abandoned years, the bacterial diversity of rhizosphere soil showed an increasing trend, indicating the adaptability of rhizosphere soil microorganisms to mine wasteland soil. The comparison between ACE index and Chao1 index showed that the richness of bacterial community in rhizosphere soil and bare soil of P. sinensis increased gradually with the increase of abandoned years. There were some differences in the characteristics of fungal and bacterial communities in different habitats and different types of soils, indicating that heavy metal pollution, different habitats, plant rhizosphere effects and other factors may affect the composition and species distribution of communities.
In this study, the highest relative abundance of soil bacterial community was Proteobacteria, and the other dominant phylums included Acidobacteriota and Actinobacteria. In the mining wasteland, the colonization of O. sinensis increased the relative abundance of Chloroflexi, Acidobacteria and Bacteroidetes. The study found that these bacterial populations can adapt to heavy metal stress to a certain extent and play an important role in maintaining soil ecological balance. The abandoned land of lead-zinc mine area is characterized by high concentration of heavy metals in soil. In this study, the content of Cd, Pb and Zn in soil is high, which is the main factor to promote the change of microbial community. The abundant genera in the rhizosphere soil of O. sinensis are positively correlated with heavy metal components. These genera can tolerate high concentrations of heavy metals and promote plant growth, which is of great benefit to the ecological restoration process of mining wasteland. These bacterial groups may also establish a good symbiotic relationship with O. sinensis in the process of long-term adaptation to the abandoned land of lead-zinc mine, which promotes the higher tolerance of O. sinensis and successful colonization in the abandoned land of lead-zinc mine. Ascomycota and Basidiomycota accounted for the main proportion in the soil fungal community. Ascomycota was the largest and most diverse phylum, accounting for the highest proportion in the soil of several plots. Ascomycota includes saprophytes, parasites and symbionts that can interfere with ecosystem functions (Thavamani et al., 2017). Basidiomycetes and Mortierella are also the dominant phyla in this study. Studies have shown that Ascomycota and Basidiomycetes often appear in soils contaminated by heavy metals (Huang et al., 2020). Studies have shown that ascomycetes and basidiomycetes are the most abundant in mining-contaminated soil, and other taxa Eurotiomycetes, Agaricomycetes, Nectriaceae, Mortierellaceae, Trichocomaceae and Fusarium (Paukszto and Miroslawski, 2019) are also present, which are consistent with this study. Leptodontidium, Hypocreales, Nectriaceae, Clavicipitaceae, Naganishia, Exophiala, Acrostalagmus and Capronia were significantly different in the rhizosphere soil of O. sinensis in the lead-zinc mine wasteland. Fungi Exophiala, Phialophora and Leptodontidium can colonize the roots of plants in places contaminated by lead and zinc (Zhang D. Z. et al., 2022). Exophiala can endow its host plants with obvious tolerance to Cd and also play a functional role as a beneficial fungus (Sharma et al., 2023). In the lead-zinc mine wasteland in this study, the extreme conditions of the soil matrix showed a natural weakening, which may be related to the microorganisms involved in the stabilization or accumulation of heavy metals and the plants naturally growing in the area (Singh et al., 2020). The bacterial and fungal communities in the rhizosphere of O. sinensis may be involved in the establishment of the plant in the lead-zinc mine wasteland. In turn, O. sinensis also provides a resource-rich place, which is conducive to the richness and diversity of the fungal community.
Different rhizosphere soil microbial community composition is different. Proteobacteria, Chloroflexi, Actinobacteria, Nitrospirae and Ascomycota are the dominant phyla in the rhizosphere soil of O. sinensis in the abandoned lead-zinc mining area. The relative abundance of these phyla in the rhizosphere soil of the abandoned lead-zinc mining area is higher than that of the non-mining area control O. sinensis rhizosphere soil. These phyla have been confirmed to be the dominant microorganisms in the mining area environment, which are related to the formation of soil aggregates, inorganic nitrogen fixation, plant pathogen control and residue decomposition (Thavamani et al., 2017), which plays an important role in the colonization of plants contaminated by high concentrations of heavy metals. The rhizosphere soil of O. sinensis in the lead-zinc mining area is significantly different from other soils at the genus level. For example, Sphingomonas is a significant difference genus. Sphingomonas contains a large amount of glycosphingolipids and can be combined with heavy metal ions (Liu et al., 2020). Microbial communities are usually sensitive to heavy metal stress in the mine environment, resulting in changes in microbial community structure (Gazitua et al., 2019; Picariello et al., 2021). In this study, microbial communities were driven by heavy metals and soil nutrients. The genera Plenodomus, Surfurifusis, Sphingomonas, Filobasidium and Articulospora of Chloroflexi, Acidobacteria and Ascomycota in the lead-zinc mine wasteland have high tolerance to extremely high concentrations of heavy metals and are enriched in the rhizosphere soil of O. sinensis, indicating that O. sinensis can recruit heavy metal-resistant microorganisms to provide nutrients for them or help them survive under stress conditions.
In this study, the soil of lead-zinc mine wasteland is lack of nutrition and the content of heavy metals is high. O. sinensis can survive and colonize in the abandoned land of the mining area and occupy a dominant position in the natural community, indicating that O. sinensis has strong tolerance to mining area pollution. With the increase of abandoned years, the content of heavy metals in vegetation patches gradually decreased. O. sinensis cuspidatum can form new microhabitats, improve soil environment, and promote the establishment of other species in mining wasteland. It can be used as a pioneer greening plant to form a special group. The microbial diversity in the rhizosphere soil of O. sinensis was quite different from that in the bare soil. The composition of bacterial and fungal communities in different plots was different, which was mainly affected by Cd, Pb, Zn and pH, TN, TP and other nutritional factors. Plenodomus, Surfurifusis, Sphingomonas, Filobasidium and Articulospora were enriched in the rhizosphere soil of O. sinensis. The unique high-tolerant microbial community had a great effect on the growth and metal tolerance of O. sinensis.
The original contributions presented in the study are included in the article/supplementary files. Further inquiries can be directed to the corresponding author.
HT: conceptualization, methodology, software, writing original draft, visualization, and investigation. YJ: investigation and software. C-QD: methodology, validation, revision, review and editing, supervision, and funding acquisition. X-QY, S-CW, and L-YL: investigation and methodology. All authors contributed to the article and approved the submitted version.
This research was supported by the National Natural Science Foundation of China (U2002208 and 32260315) and China Yunnan Provincial R&D Programs (202101AS070033, 202201AS070016, and 202201BF070001-002).
The authors declare that the research was conducted in the absence of any commercial or financial relationships that could be construed as a potential conflict of interest.
All claims expressed in this article are solely those of the authors and do not necessarily represent those of their affiliated organizations, or those of the publisher, the editors and the reviewers. Any product that may be evaluated in this article, or claim that may be made by its manufacturer, is not guaranteed or endorsed by the publisher.
Abdelkrim S., Harzalli S., Saadani J. O., Abid G., Taamalli W., Zemni H., et al. (2020). In situ effects of Lathyrus sativus-PGPR to remediate and restore quality and fertility of Pb and Cd polluted soils. Ecotoxicology Environ. Saf. 192, 110260. doi: 10.1016/j.ecoenv.2020.110260
Antoniadis V., Shaheen S. M., Stark H. J., Wennrich R.., Levizou E., Merbach I., et al. (2021). Phytoremediation potential of twelve wild plant species for toxic elements in a contaminated soil. Environ. Int. 146, 106233. doi: 10.1016/j.envint.2020.106233
Bang L., Jun Y., Chen Z. H., Bo M., Hao L., Pang W. C., et al. (2022). Biogeography, assembly processes and species coexistence patterns of microbial communities in metalloids-laden soils around mining and smelting sites. J. Hazardous Materials 425, 127945. doi: 10.1016/j.jhazmat.2021.127945
Bierza W., Bierza K., Trzebny A., Gren I., Dabert M., Ciepal R., et al. (2020). The communities of ectomycorrhizal fungal species associated with Betula pendula Roth and Pinus sylvestris L.growing in heavy-metal contaminated soils. Plant Soil 457 (1-2), 321–338. doi: 10.1007/s11104-020-04737-4
Briffa J., Sinagra E., Blundell R. (2020). Heavy metal pollution in the environment and their toxicological effects on humans. Heliyon 6 (9), e04691. doi: 10.1016/j.heliyon.2020.e04691
Buch A. C., Niemeyer J. C., Marques E. D., Silva E. V. (2020). Ecological risk assessment of trace metals in soils affected by mine tailings. J. Hazardous Materials 403, 123852. doi: 10.1016/j.jhazmat.2020.123852
Elizabeth M., Kelly S., Tandra D., Wall D. H. (2020). Soil biodiversity integrates solutions for a sustainable future. Sustainability 12 (7), 2662–2675. doi: 10.3390/su12072662
Frérot H., Hautekèete N. C., Decombeix I., Bouchet M. H., Creach A., Saumitou-Laprade P., et al. (2018). Habitat heterogeneity in the pseudometallophyte Arabidopsis halleri and its structuring effect on natural variation of zinc and cadmium hyperaccumulation. Plant Soil 423 (1), 157–174. doi: 10.1007/s11104-017-3509-1
Gazitua M. C., Morgante V., Poupin M. J., Ledger T., Rodriguez-Valdecantos G., Herrera C., et al. (2019). The microbial community from the early-plant colonizer (Baccharis linearis) is required for plant establishment on copper mine tailings. Sci. Rep. 11 (1), 10448. doi: 10.1038/s41598-021-89769-1
Guo M. N., Zhong X., Liu W. S., Wang G. B., Chao Y. Q., Huot H., et al. (2022). Biogeochemical dynamics of nutrients and rare earth elements (REEs) during natural succession from biocrusts to pioneer plants in REE mine tailings in southern China. Sci. Total Environ. 828, 154361. doi: 10.1016/j.scitotenv.2022.154361
Hu L. F., Robert C. A. M., Cadot S., Zhang X., Ye M., Li B. B., et al (2018). Root exudate metabolites drive plant-soil feedbacks on growth and defense by shaping the rhizosphere microbiota. Nat. Commun. 9 (1), 2738–2750. doi: 10.1038/s41467-018-05122-7
Huang X., Liu S., Liu X., Zhang S. R., Li L., Zhao H. T., et al. (2020). Plant pathological condition is associated with fungal community succession triggered by root exudates in the plant-soil system. Soil Biol. Biochem. 151, 108046. doi: 10.1016/j.soilbio.2020.108046
Jiang X. S., Guo Y., Li H. X., Li X. M., Liu J. (2022). Ecological evolution during the three-year restoration using rhizosphere soil cover method at a Lead-Zinc tailing pond in Karst areas. Sci. Total Environ. 853, 158219. doi: 10.1016/j.scitotenv.2022.158291
Lan J. R., Zhang S. S., Dong Y. Q., Li J. H., Li S. Y., Feng L., et al. (2021). Stabilization and passivation of multiple heavy metals in soil facilitating by pinecone-based biochar: Mechanisms and microbial community evolution. J. Hazardous Materials 420, 126588. doi: 10.1016/j.jhazmat.2021.126588
Lei Q., Xie C. Q., Liu L. F., Zhan F. D., Zu Y. Q., He Y. M. (2013). Effects of cadmium on the hydrogen ion secretion of rhizosphere fungi of Oxyria sinensis Hemsl. in abandoned lead-zinc mining area in Huize, Yunnan. Chinese Agricultural Science Bulletin 29 (17), 177–181. doi: 1000-6850(2013)29:17<177:GDQXKQ>2.0.TX;2-H
Liu K. H., Zhang H. C., Liu Y. F., Yu F. (2020). Investigation of plant species and their heavy metal accumulation in manganese mine tailings in Pingle Mn mine, China. Environ. Sci. pollut. Res. 27 (16), 19933–19945. doi: 10.1007/s11356-020-08514-9
Lu M. X., Wang X. X., Li Y., Liu H. L., An X. C., Lian B. (2022). Soil microbial community structure and environmental effects of serpentine weathering under different vegetative covers in the serpentine mining area of Donghai County, China. Sci. Total Environ. 835, 155452. doi: 10.1016/j.scitotenv.2022.155452
Liu X., Xu X., Ma T., Zhou S. W., Bi X. L., He H. B., et al. (2023). Linking microbial carbon pump capacity and efficacy to soil organic carbon storage and stability under heavy metal pollution. Soil Ecol. Lett. 5 (2), 220140. doi: 10.1007/s42832-022-0140-8
Navarro-Cano J. A., Goberna M., Verdú M. (2019). Using plant functional distances to select species for restoration of mining sites. J. Appl. Ecoligy 56 (10), 2353–2362. doi: 10.1111/1365-2664.13453
Paukszto A., Miroslawski J. (2019). Using stinging nettle (Urtica dioica L.) to assess the influence of long term emission upon pollution with metals of the Tatra National Park area (Poland). J. Hazardous Materials 379 (5), 120813.
Picariello E., Baldantoni D., Izzo F., Langella A., De Nicola F. (2021). Soil organic matter stability and microbial community in relation to different plant cover: A focus on forests characterizing Mediterranean area. Appl. Soil Ecol. 162, 103897. doi: 10.1016/j.apsoil.2021.103897
Rahmonov O., Cabala J., Krzysztofik R. (2022). Vegetation and environmental changes on contaminated soil formed on waste from an historic Zn-Pb ore-washing plant. Biology-Basel 10 (12), 1242. doi: 10.3390/biology10121242
Saavedra-Mella F., Liu Y. J., Southam G., Huang L. B. (2022). Phosphate treatment alleviated acute phytotoxicity of heavy metals in sulfidic Pb-Zn mine tailings. Environ. Pollut. 250, 676–689. doi: 10.1016/j.envpol.2019.04.100
Saini S., Kaur N., Pati P. K. (2021). Phytohormones: Key players in the modulation of heavy metal stress tolerance in plants. Ecotoxicology Environ. Saf. 223, 112578. doi: 10.1016/j.ecoenv.2021.112578
Sebelikova L., Csicsek G., Kirmer A., Vitovcova K., Ortmann-Ajkai A., Prach K., et al. (2019). Spontaneous revegetation versus forestry reclamation-Vegetation development in coal mining spoil heaps across Central Europe. Land Degradation Dev. 30 (3), 348–356. doi: 10.1002/ldr.3233
Sha H. C., Li J., Wang L. Q., Nong H. D., Wang G. H., Zeng T. T. (2023). Preparation of phosphorus-modified biochar for the immobilization of heavy metals in typical lead-zinc contaminated mining soil: Performance, mechanism and microbial community. Environ. Res. 218, 114769. doi: 10.1016/j.envres.2022.114769
Sharma P., Chaturvedi P., Chandra R., Kumar S. (2023). Identification of heavy metals tolerant Brevundimonas sp. from rhizospheric zone of Saccharum munja L. and their efficacy in in-situ phytoremediation. Chemosphere 295, 133823. doi: 10.1016/j.chemosphere.2022.133823
Singh B. K., Trivedi P., Egidi E., Macdonald C. A., Delgado-Baquerizo M. (2020). Crop microbiome and sustainable agriculture. Nat. Rev. Microbiol. 19 (1), 601–602. doi: 10.1038/s41579-020-00446-y
Stumbea D., Chicos M. M., Nica V. (2019). Effects of waste deposit geometry on the mineralogical and geochemical composition of mine tailings. J. Hazardous Materials 368, 496–505. doi: 10.1016/j.jhazmat.2019.01.071
Thavamani P., Samkumar R. A., Satheesh V., Subashchandrabose S. R., Ramadass K., Naidu R., et al. (2017). Microbes from mined sites: Harnessing their potential for reclamation of derelict mine sites. Environ. pollut. 230, 495–505. doi: 10.1016/j.envpol.2017.06.056
Wang F. F., Li Z. M., Fu B. J., Lu Y. H., Liu G. P., Wang D. B., et al. (2022). Short-term grazing exclusion alters soil bacterial co-occurrence patterns rather than community diversity or composition in temperate grasslands. Front. Microbiol. 13, 824192. doi: 10.3389/fmicb.2022.824192
Wu B. H., Peng H., Sheng M. P., Luo H. Y., Wang X. T., Zhang R., et al. (2021). Evaluation of phytoremediation potential of native dominant plants and spatial distribution of heavy metals in abandoned mining area in Southwest China. Ecotoxicology Environ. Saf. 220, 112368. doi: 10.1016/j.ecoenv.2021.112368
Wu S. L., You F., Huang L. B., Huang L. B. (2021). Native plant Maireana brevifolia drives prokaryotic microbial community development in alkaline Fe ore tailings under semi-arid climatic conditions. Sci. Total Environ. 760, 144019. doi: 10.1016/j.scitotenv.2020.144019
Wu L. J., Yue W. F., Wu J., Cao C. M., Liu H., Teng Y. G. (2023). Metal-mining-induced sediment pollution presents a potential ecological risk and threat to human health across China: A meta-analysis. J. Environ. Manage. 329, 117058. doi: 10.1016/j.jenvman.2022.117058
Yin Y., Wang X. J., Hu Y. A., Li F. D., Cheng H. F. (2023). Soil bacterial community structure in the habitats with different levels of heavy metal pollution at an abandoned polymetallic mine. J. Hazardous Materials 442 (15), 130063. doi: 10.1016/j.jhazmat.2022.130063
Yuan X. Q., Guo Z. L., Duan C. Q., Yang J., Tang H., Li L. Y., et al (2022). Alleviation of heavy metal stress and enhanced plant complex functional restoration in abandoned Pb-Zn mining areas by the nurse plant Coriaria nepalensis. Front. Ecol. Evol. 10, 1006468. doi: 10.3389/fevo.2022.1006468
Yung L., Bertheau C., Tafforeau F., Zappelini C., Valot B., Maillard F., et al. (2021). Partial overlap of fungal communities associated with nettle and poplar roots when co-occurring at a trace metal contaminated site. Sci. Total Environ. 782, 146692. doi: 10.1016/j.scitotenv.2021.146692
Zhang Y. H., Huang H. G., Zhan J., Yu H. Y., Ye D. H., Zhang X. Z., et al. (2022). Indigenous rhizosphere microbial community characteristics of the phytostabilizer Athyrium wardii (Hook.) grown in a Pb/Zn mine tailing. Chemosphere 308 (3), 136552. doi: 10.1016/j.chemosphere.2022.136552
Zhang D. Z., Zhou H., Shao L. L., Wang H. R., Zhang Y. B., Zhu T., et al. (2022). Root characteristics critical for cadmium tolerance and reduced accumulation in wheat (Triticum aestivum L.). J. Environ. Manage. 305, 114365. doi: 10.1016/j.jenvman.2021.114365
Zhen Z., Wang S. B., Luo S. W., Ren L., Liang Y. Q., Yang R. C., et al. (2019). Significant impacts of both total amount and availability of heavy metals on the functions and assembly of soil microbial communities in different land use patterns. Front. Microbiol. 10, 2293. doi: 10.3389/fmicb.2019.02293
Zhong X., Chen Z. W., Ding K. B., Liu W. S., Baker A. J. M., Fei Y. H., et al. (2022). Heavy metal contamination affects the core microbiome and assembly processes in metal mine soils across Eastern China. J. Hazardous Materials 443, 130241. doi: 10.1016/j.jhazmat.2022.130241
Keywords: Oxyria sinensis Hemsl., heavy metals, tolerance characteristics, microbial community, abandoned Pb-Zn mining area
Citation: Tang H, Yang J, Yuan X-q, Wang S-c, Li L-y and Duan C-q (2023) Rhizosphere microbial characteristics of pioneer plant Oxyria sinensis Hemsl. in abandoned Pb-Zn mining area. Front. Ecol. Evol. 11:1246824. doi: 10.3389/fevo.2023.1246824
Received: 24 June 2023; Accepted: 13 September 2023;
Published: 06 October 2023.
Edited by:
Kang Di, China West Normal University, ChinaCopyright © 2023 Tang, Yang, Yuan, Wang, Li and Duan. This is an open-access article distributed under the terms of the Creative Commons Attribution License (CC BY). The use, distribution or reproduction in other forums is permitted, provided the original author(s) and the copyright owner(s) are credited and that the original publication in this journal is cited, in accordance with accepted academic practice. No use, distribution or reproduction is permitted which does not comply with these terms.
*Correspondence: Chang-qun Duan, Y2hxZHVhbkB5bnUuZWR1LmNu
Disclaimer: All claims expressed in this article are solely those of the authors and do not necessarily represent those of their affiliated organizations, or those of the publisher, the editors and the reviewers. Any product that may be evaluated in this article or claim that may be made by its manufacturer is not guaranteed or endorsed by the publisher.
Research integrity at Frontiers
Learn more about the work of our research integrity team to safeguard the quality of each article we publish.