- 1Hawkesbury Institute for the Environment, Western Sydney University, Penrith, NSW, Australia
- 2Department of Pathobiology and Population Health, School of Animal and Veterinary Sciences, University of Adelaide, Roseworthy, SA, Australia
- 3Institute of Science and Learning, Taronga Conservation Society Australia, Mosman, NSW, Australia
- 4Currency Creek Arboretum, Melrose Park, SA, Australia
Generally, urbanization is a major threat to biodiversity; however, urban areas also provide habitats that some species can exploit. Flying-foxes (Pteropus spp.) are becoming increasingly urbanized; which is thought to be a result of increased availability and temporal stability of urban food resources, diminished natural food resources, or both. Previous research has shown that urban-roosting grey-headed flying-foxes (Pteropus poliocephalus) preferentially forage in human-modified landscapes. However, which land-use areas and food plants support its presence in urban areas is unknown. We tracked nine P. poliocephalus roosting in Adelaide, South Australia, between December 2019 and May 2020, using global positioning systems (GPS), to investigate how individuals used the urban landscape mosaic for feeding. The most frequently visited land-use category was “residential” (40% of fixes) followed by “road-side,” “reserves” and “primary production” (13–14% each). However, “reserves” were visited four times more frequently than expected from their areal availability, followed by the “residential” and “road-side” categories that were visited approximately twice more than expected each; in contrast, the “primary production” category was visited approximately five times less than expected. These results suggest that while residential areas provide most foraging resources supporting Adelaide’s flying-fox population, reserves contain foraging resources that are particularly attractive to P. poliocephalus. Primary production land was relatively less utilized, presumably because it contains few food resources. Throughout, flying-foxes visited an eclectic mixture of diet plants (49 unique species), with a majority of feeding fixes (63%) to locally indigenous Australian native species; however, in residential areas 53% of feeding visits were to non-locally indigenous species, vs only 13% in reserves. Flowering and fruiting phenology records of the food plants visited further indicated that non-locally indigenous species increase the temporal availability of foraging resources for P. poliocephalus in urban Adelaide. Our findings demonstrate the importance of residential areas for urban-roosting P. poliocephalus, and suggest that the anthropogenic mixture of food resources available in the urban landscape mosaic supports the species’ year-round presence in urban areas. Our results further highlight the importance of conserving natural habitats within the urban landscape mosaic, and stress the need for accounting for wildlife responses to urban greening initiatives.
Introduction
Generally, urbanization is one of the most detrimental processes to wildlife and the ecosystem services they provide (McKinney, 2002; Grimm et al., 2008; Fenoglio et al., 2021), because it results in the replacement of natural habitat with concrete and buildings, interspersed by human-modified “greenspaces” such as parks and gardens (Marzluff and Ewing, 2001). While urban greenspaces provide habitats for adaptable wildlife, they are often distributed patchily throughout the urban landscape (Callaghan et al., 2019), making them difficult for less mobile species to exploit (Öckinger et al., 2010; Concepción et al., 2015; Jung and Threlfall, 2018). Additionally, urban clearing promotes the establishment of disturbance tolerant, “weedy” non-native plants, and this can result in biotic homogenization of urban habitat (McKinney, 2002) poorly suited to many wildlife species (McKinney and Lockwood, 1999). However, the occurrence of locally indigenous remnant trees and the active planting of aesthetically pleasing locally non-indigenous native and non-indigenous species in urban greenspaces (Kendal et al., 2012), can provide a wide range of foraging opportunities for mobile nectivorous and frugivorous vertebrates, including birds (e.g., Evans et al., 2012) and bats (e.g., Lim et al., 2018; de Souza Laurindo and Vizentin-Bugoni, 2020; Chan et al., 2021; Meade et al., 2021). Thus, green spaces can contribute to the persistence of such species. Increased temperatures associated with the urban heat island effect (Magee et al., 1999), as well as the use of artificial watering systems and fertilizers (e.g., Neil and Wu, 2006; Zhao et al., 2016) can enhance the productivity of plants in urban areas relative to their wild counterparts, likely resulting in more temporally reliable urban food supplies. However, urban habitats can expose wildlife to additional anthropogenic threats (Taylor-Brown et al., 2019), including predation by domestic pets (e.g., van Heezik et al., 2010), electrocution on powerlines (Dwyer and Mannan, 2007), vehicle and object collisions (e.g., Chace and Walsh, 2006), and exacerbate human-wildlife conflict (e.g., Tillman et al., 2000; Delahay et al., 2009; Soulsbury and White, 2015). Thus, wildlife urbanization is an important issue requiring precarious balancing of conservation and management concerns. However, mitigation strategies often focus on the human side of conflict rather than addressing the underlying factors that drive wildlife urbanization, which can pose critical impediments to the sound conservation management of urban wildlife (e.g., Baruch-Mordo et al., 2014; Fernández-Gil et al., 2016; Broekhuis et al., 2017).
Australian flying-foxes (Pteropus spp.) are large bats that forage by night on blossom and fruit resources and rest by day in arboreal roosts that may support colonies of many thousands of individuals (Hall and Richards, 2000). They are extremely mobile species that travel nomadically among networks of roosts within their ranges (Welbergen et al., 2020), and their movements are likely driven by changes in the availability of foraging resources in the landscape (Eby, 1991; Parry-Jones and Augee, 1992). However, while two of Australia’s four mainland species are listed as threatened under federal legislation (Threatened Species Scientific Committee, 2001, 2019) and the IUCN Red List (International Union for Conservation of Nature [IUCN], 2021), flying-foxes are becoming increasingly common in Australia’s urban environments (Tait et al., 2014; Meade et al., 2021; Timmiss et al., 2021; Yabsley et al., 2021), and this creates important challenges for their management and conservation (Scheelings and Frith, 2015; Mo et al., 2020; Roberts et al., 2021). Roosts were traditionally thought to be occupied on a seasonal basis, possibly as a result of both climatic variables and the irregular, asynchronous flowering of many native diet species across space (Nelson, 1965). However, in the last few decades, multiple new colonies have become permanently occupied in urban areas (Plowright et al., 2011), including, but not limited to, the centers of the major cities of Brisbane (Markus and Hall, 2004), Sydney (Richards, 2002), Melbourne (Aston, 1987; McDonald-Madden et al., 2005; Williams et al., 2006), and Adelaide, with the latter being well-outside the former range of any Pteropus species (Boardman et al., 2021). The documented increase in flying-fox urbanization has exacerbated human-wildlife conflict, largely due to a loss of social amenity as a result of objectionable noise and smell (Currey et al., 2018) and fear of zoonotic disease transmission (Kung et al., 2015). However, present methods used to control flying-foxes in urban environments are commonly met with very limited success (Roberts et al., 2021) as they do not account for the highly nomadic behaviors of these species (Welbergen et al., 2020), nor address the underlying drivers of flying-fox urbanization (Meade et al., 2021; Yabsley et al., 2021).
The drivers behind flying-fox urbanization are presently unknown; however, it has been hypothesized that flying-fox urbanization is a response to the year-round availability and stability of urban food resources from urban plantings (Williams et al., 2006; Yabsley et al., 2021). While there is little detailed documented information to indicate that the spatiotemporal availability and stability of food plant resources has increased in Australian cities in recent decades, Australian native trees have become increasingly popular as candidates for street trees (Yau, 1982). As a result, many locally indigenous and non-indigenous Australian native diet plant species (e.g., Eucalypts, Corymbias, and Angophoras of the Myrtaceae family) have been planted since the 1970’s (Yau, 1982). These plants will have since matured, and as such have likely increased the availability of food to flying-foxes in urban areas as mature individuals produce more flowers, flower more frequently, and flower for longer than younger individuals (see Wilson, 2002). The increase in abundance of airborne Myrtaceous pollen in Melbourne since the 1960s indicates increasing flowering; this has been attributed to the maturation of planted eucalypts (Williams et al., 2006). In addition to pollen and nectar, the availability of fruit for flying-foxes has increased in urban areas with plantings of non-indigenous Australian native fruit-bearing trees including Ficus macrophylla and Acmena smithii in streets and parks, and the cultivation of non-Australian species such as apples, plums, and figs (Williams et al., 2006). Furthermore, plantings of non-indigenous Australian native and non-Australian species may increase the period of time food is available for flying-foxes roosting in urban areas as these species may flower or fruit at different times of the year compared to locally indigenous species (e.g., Williams et al., 2006), thus potentially extending the temporal availability of food. The above suggests that the spatiotemporal availability and stability of food resources for flying-foxes in urban areas has likely increased since the 1980s, but thus far we do not know how flying-foxes use urban landscape mosaics for feeding or the composition of their diet when roosting in urban areas.
Human-modified landscapes are important foraging and roosting habitat for grey-headed flying-foxes (Pteropus poliocephalus) (Timmiss et al., 2021; Yabsley et al., 2021). Previous work has shown that the majority of P. poliocephalus colonies are found in urban environments (Timmiss et al., 2021); that urban roosts are becoming occupied increasingly permanently (van der Ree et al., 2006; Williams et al., 2006; Plowright et al., 2011; Boardman et al., 2021); and that individuals exhibit higher fidelity to urban roosts, and forage over shorter distances when roosting in major-urban areas (Meade et al., 2021). Pteropus poliocephalus roosting in major-urban areas forage preferentially in human-modified landscapes, and likely forage on a different set of food plants when roosting in urban versus natural areas (Yabsley et al., 2021). A spring case study in Adelaide found that most P. poliocephalus foraging sites were in residential areas, and that the food plants most commonly visited included non-indigenous Australian native species (Boardman et al., 2021). Taken together, these studies indicate differences in urban and non-urban landscape use by P. poliocephalus. However, while it is now clear that human-modified areas support a large proportion of the P. poliocephalus population, with only 5.2% of P. poliocephalus roosts occurring in protected habitat (Timmiss et al., 2021), it is imperative to the conservation management of this species to better understand which areas and resources support P. poliocephalus in urban landscape mosaics. This information would inform management of human-versus flying-fox conflict and other anthropogenic threats including electrocution on powerlines, fruit tree netting entanglement, and vehicle collisions, and would potentially facilitate targeted redistribution of food plant resources for a more balanced approach to managing urban flying-fox populations.
In this study, we used paired global positioning system (GPS) and accelerometer data to investigate the diet and landscape use of P. poliocephalus roosting at a recently established colony in the Adelaide Botanic Park, South Australia. We further examined flowering and fruiting phenology records to study the temporal availability of identified P. poliocephalus food plant species in the broader Adelaide region. We predicted that P. poliocephalus would preferentially feed in highly human-modified areas, particularly residential areas, and that food plant species visited in these habitats would comprise a wide range of locally indigenous, non-indigenous Australian native, and non-Australian species. We also predicted that flowering and fruiting phenology records of P. poliocephalus food plants would point to year-round availability of food resources. We discuss our findings in the context of the observed P. poliocephalus urbanization and the implications for the conservation management of urban flying-fox populations.
Materials and methods
Study area
The focal flying-fox roost used for this study (Adelaide Botanic Park; −34.916, 138.607) represents the furthest south-west documented colony of P. poliocephalus. The Adelaide Botanic Park colony was established in 2011, and the roost is now occupied year-round (Boardman et al., 2021). Adelaide Botanic Park comprises a range of locally indigenous plant species including Eucalyptus camaldulensis, locally non-indigenous Australian natives including Corymbia citriodora and Angophora costata, and a wide collection of non-Australian plant species from around the world (Lothian, 1980). The flying-foxes primarily roost in Aleppo pine (Pinus halepensis) within Adelaide Botanic Park (Boardman et al., 2021).
Adelaide is an isolated city located in South Australia, bordered by a range of hills to the East and the Australian coastline to the west (Tait et al., 2005). It has a Mediterranean climate exhibiting cool, wet winters and hot, dry summers (Tait et al., 2005; Milazzo et al., 2016). The dominant land-use for the Adelaide region is cropping and pasture, followed closely by urban habitat (Tait et al., 2005). Other land-uses include remnant vegetation, orchards, irrigated areas, water bodies, quarries, swamp, hardwood and pine plantations (Tait et al., 2005). Residential areas in the region are largely characterized by gardens; the metropolitan area has a range of parks and gardens that support a wide range of vegetation types comprised of Australian locally indigenous, non-indigenous Australian native, and non-Australian species.
Capture and deployment of transmitters
Pteropus poliocephalus individuals were captured at the Adelaide Botanic Park on 10 and 11 December 2019. Flying-foxes returning to the roost before dawn were captured using two double banked mist nets (18 m × 5 m and 12 m × 5 m, 38 mm mesh, Ecotone Telemetry, Poland) suspended 15 m high in the canopy of the colony. Nets were run on pulley systems that were continuously monitored by volunteers and two or three trained researchers. Each flying-fox was removed from the net by researchers and placed into a pillowcase hung from a horizontal pole. Females that were lactating, pregnant or carrying a pup were released immediately upon capture.
Ten focal individuals (five adult males and five non-reproductively active adult females) were transported to Adelaide Zoo where they were processed. Individuals were anesthetized using 5% vaporized isoflurane via facemask and maintained at 2% isoflurane until processing was complete. While anesthetized, morphometrics were taken and all bats were banded with a single stainless-steel band (Australian Bird and Bat Banding Scheme). The ten individuals were fitted with a collar supporting a GPS and accelerometry unit (CREX GPS Logger, Ecotone Telemetry, Poland, hereafter: transmitter). The five females had a mass of 725 ± 93.1 g (568–806) and the five males had a body mass of 818 ± 127.0 g (685–954). The transmitter and collar weighed 10 and 3 g, respectively, giving a total weight of 13 g, representing 1.6–2.3 and 1.4–1.9% of the body weight of females and males, respectively. After animals recovered from anesthesia, they were placed in animal holding facilities at the Adelaide Zoo for recovery. The five male flying-foxes required surgery to implant a temperature-sensitive VHF FM radio transmitter (model PD-2THX, 3.9 g; battery life: 5 months; Holohil) as part of another study (Walker, unpublished)1. Tracking devices represented <3.0% of the body mass of the lightest individual. Individuals that did not require surgery (n = 5 females) were released back into the colony within 6 h of capture; the five males were released back into the colony the next morning following an assessment by a wildlife veterinarian (WB).
Research was conducted under Animal Research Authority no. A12217, issued by Western Sydney University.
Data collection
Global positioning systems and accelerometer data were collected for 5 months from 13 December 2019 to 23 May 2020 (Austral Summer and Autumn). Accelerometer data were recorded on three orthogonal axes and were used to identify the GPS fixes associated with feeding, as opposed to other behaviors such as flying (see below). Transmitters were programmed to collect accelerometer data in three burst types, dependent on battery voltage (solar recharge): 12 s at 5 Hz every 15 mins, 2 s at 30 Hz every 30 mins, or 3 s at 10 Hz every 30 mins. Transmitters were set to record GPS data every 30 mins during the night when there was sufficient solar recharge. Accelerometer data were linked with the GPS duty cycle. The duty cycles of the transmitters were monitored and changed remotely via Global System for Mobile (GSM) network using the web-panel depending upon the solar recharge of the batteries.
Data were collected via a GSM link from GPS trackers to 3G-enabled mobile phone towers that then reported the data to a File Transfer Protocol (FTP) server, accessed through the GPS data processing software package “NGA Analyzer” (Ecotone Telemetry, Poland).
The amount of time that the trackers produced usable data varied from 3 to 154 days (Mean = 78.4; Supplementary Table 1). Some of the collared individuals left the Adelaide region (defined as ≥75 km from the center of the Adelaide Botanic Garden roost) during our study (Supplementary Table 1). One female (FFOX05) left the Adelaide region before the transmitter began collecting data and hence her data were excluded from analyses herein. Another female (FFOX02) left the Adelaide region on 18 January 2020, and returned on 2 April 2020. Two males (FFOX07 and FFOX09) left the Adelaide region on 31 December 2019 and on 19 March 2020, respectively, and did not return to the region for the duration of the study.
We removed GPS fixes associated with travel outside of the Adelaide region (GPS fixes greater than 75 km from the colony). GPS fixes less than 500 m from the center of the colony were also removed to exclude resting time within the colony. As the boundary of the roost varies with the number of flying-foxes present, this conservative approach means that we are potentially excluding some nearby foraging fixes. For those individuals that left the Adelaide region, GPS fixes recorded on the day of departure or day of return were excluded as any food resources associated with these fixes were likely supporting the individual’s journey to or from another roost rather than their stay in Adelaide.
Identifying feeding fixes
Global positioning systems locations were designated “feeding fixes” if they were temporally aligned with moderate levels of activity, as determined by variance in the acceleration data. We applied a principal component analysis (PCA) using the “prcomp” function from the “stats” package in R to the three recorded axes of acceleration forces to maximize the amount of variation caused by movement that is expressed in a single vector (i.e., principal component 1). We did this also to account for variation in the spatial orientation of the transmitter between individuals, which could have influenced the distribution of acceleration forces caused by movement across the three axes. We plotted a frequency histogram of the standard deviation (SD) of the PC1 scores over each burst of acceleration data for each individual and used troughs between primary modes in the distribution of SD values as thresholds for designating among broad categories of acceleration intensity (Collins et al., 2015). Firstly, we identified a mode of greatest SD values, most likely associated with wing flapping during flight, and assigned data above a trough threshold defining the lower limit of this mode to high level activity (Supplementary Figure 1A). The remaining data were subject to another PCA. We identified two modes in the frequency distribution of SD values of the new PC1 vector: a lower mode clearly associated with inactivity (i.e., little, if any, and body movement), and an upper mode that included values of greater acceleration intensity, but excluded the highest values previously assigned to high level activity. We assigned data above the trough between these two modes as moderate activity that likely pertains to tree-based movements, including feeding (i.e., the behavior of eating a dietary component and any associated movement within a foraging tree; Supplementary Figure 1B).
Date-time stamps associated with moderate activity were rounded to the nearest second. We then aligned the closest activity date-time stamps to the GPS date-time stamp. To minimize the possibility of misinterpreting the level of activity at each GPS coordinate, we calculated the time discrepancy between each pair of activity and GPS data, using the “difftime” function from the “lubridate” package in R. We excluded the data to include only data pairs that were within a ±60 s discrepancy buffer. We used the “suncalc” package in R to calculate local sunrise and sunset times and define night (after sunset and before sunrise) and included GPS data for the moderate level of activity during the night only, which resulted in 489 GPS fixes associated with moderate activity during the night.
Land-use categories
To investigate which land-use categories P. poliocephalus used for feeding we used the South Australian Government’s “Generalized Land Use 2020” shapefile of the land-use categories of South Australia (Government of South Australia, 2020). Land-use polygons were grouped into 10 categories: “primary production” (i.e., areas that are used for agriculture, horticulture, forestry, and livestock), “residential” (i.e., urban and rural residential, hotel/motel accommodation, institutional accommodation, and orchards nestled within peri-urban residential areas), “reserve” (i.e., national park, median strips, and road reserves), “vacant” (i.e., urban and non-urban vacant land, and steep/rocky land), “utilities” (i.e., gas, electricity, water/sewage/waste disposal, public transportation, and telecommunications), “institution” (i.e., government, and education), “mining” (i.e., mines, open workings, wells, and quarries), “recreation” (i.e., ovals, golf courses, camping grounds, and stadiums), “commercial” (i.e., wholesale trade, retail, and finance), and “industrial” (i.e., food manufacture). The shapefile has gaps between polygons that pertain to linear landscape features including roads and watercourses and thus, we assigned these areas a new land-use category called “road/river.”
Land-use by foraging Pteropus poliocephalus
The land-use shapefile was clipped by a circle with a 75 km radius (Figure 1), and a geoBoundaries shapefile of Australia (Runfola et al., 2020), using the function “st_buffer” in the R package “st,” to obtain the total area where P. poliocephalus foraging could have occurred. A 75 km radius was selected since the maximum distance any of the tracked individuals traveled and returned to the Botanic Park colony was 70.8 km.
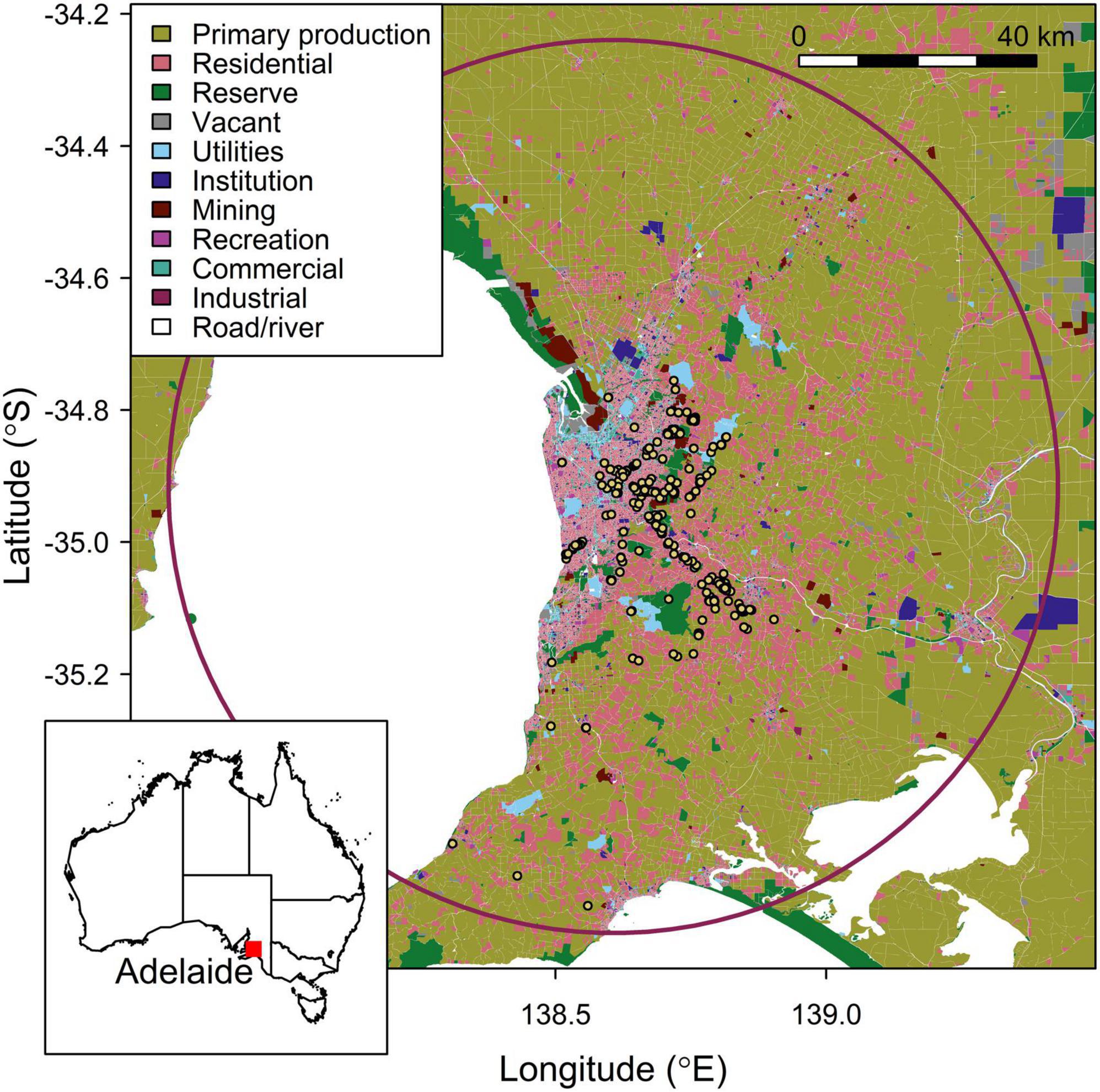
Figure 1. Study area; the Adelaide region, depicted by a maroon circle (75 km radius) on the main map, and a red marker on the inlaid map of Australia. Land-use categories are indicated in the key. Pteropus poliocephalus feeding fixes are represented by yellow markers. All feeding fixes are 500 m–75 km from the center of the Adelaide Botanic Park roost; –34.916, 138.607. Data (n = 489) were obtained from (n = 9) GPS tracked P. poliocephalus and were collected between December 2019 and May 2020.
Land-use categories were extracted for all feeding fixes in the study area (n = 489). The areal extent of each land-use category within the study area was calculated. To calculate the area pertaining to the “road/river” land-use category, the sum of the area of all the land-use categories was subtracted from the clipped study area.
To examine whether P. poliocephalus foraged at random across the landscape, we compared the proportion of feeding fixes in each land-use category to the proportion that would be expected based on the area of each land-use category in the study area, using a Chi-squared test for given probabilities.
Food plant species
To investigate the food plant species visited by foraging individuals, we first read fixes pertaining to moderate levels of activity at night into Google Earth Pro and confirmed that all likely feeding fixes were in trees. This resulted in 489 feeding tree locations.
A random sample (n = 322) of the total 489 feeding tree locations, were approached in person. Of these, some (n = 35) could not be visited due to dangerous terrain or formal access restrictions; of which 60% were in residential areas, 20% in reserves, 9% in agricultural areas, 6% in mining and road/river areas, and 3% in utilities (Supplementary Table 2). The frequency of inaccessible fixes in each land-use category was not significantly different to the observed frequency of feeding fixes in each land-use category overall (Pearson’s Chi-squared test n = 489; χ2 = 55, df = 50, p = 0.29), and thus, exclusion of these fixes from the dataset is unlikely to bias the results.
Each accessible feeding tree (n = 287) was photographed, and samples of bark, leaves, buds, flowers and fruit were taken, where possible, to aid in species identification. In addition, close-up photographs of plant characteristics using a telephoto zoom lens were captured, particularly where direct samples were unable to be obtained (see Figures 2A–F). Tree characteristics including details on the bark, leaves, buds, fruits and inflorescences were recorded for each tree following the EUCLID key, and an attempt at identifying the species was made using tree identification guides (Nicolle, 2013; Centre for Australian National Biodiversity Research [CANBR], 2015; Brooker and Kleinig, 2016; Lucid, 2016). Author DN provided subsequent validations on each food plant species from photographs and detailed field notes. Of the 287 food plants assessed, 22 lacked sufficient detail and thus could not be confidently identified. Therefore, a total of 265 individual food plants were identified.
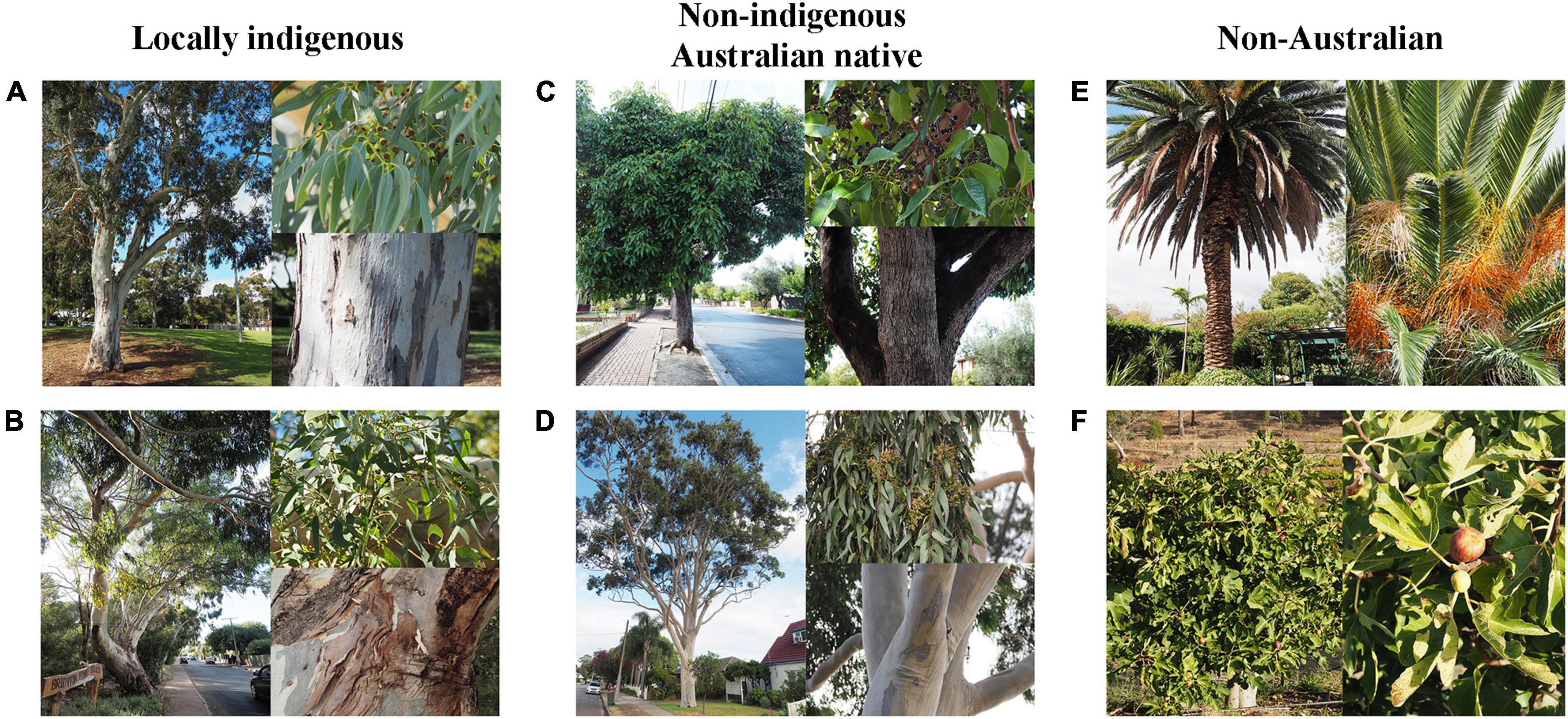
Figure 2. Example photographs of locally indigenous (to the Adelaide region), non-indigenous Australian native (not originally occurring in South Australia but indigenous to Australia), and non-Australian food plant species visited by nine GPS tracked Pteropus poliocephalus roosting in Adelaide Botanic Park (–34.916, 138.607) between December 2019 and May 2020. (A) Eucalyptus leucoxylon, (B) E. camaldulensis, (C) Lophostemon confertus, (D) Corymbia citriodora, (E) Phoenix canariensis, and (F) Ficus carica.
We used known distributions from plant identification guides (Nicolle, 2013; Centre for Australian National Biodiversity Research [CANBR], 2015; Brooker and Kleinig, 2016; Lucid, 2016) to classify each identified tree (n = 265) as “locally indigenous” to the study region, “non-indigenous Australian native,” or “non-Australian” species, this is referred to as their “geographic origin.” Where plants could not be identified to species level, their origin was conservatively classified as “unknown.”
Here, two Pearson’s Chi-squared tests were conducted, respectively, to examine (i) whether the relative number of unique species that occurred in each of the four “geographic origin” categories (i.e., locally indigenous, non-indigenous Australian native, non-Australian, and unknown) varied across land-use categories (Supplementary Table 3); and (ii) whether the relative number of visits (i.e., feeding fixes) to trees of varying “geographic origin” (i.e., locally indigenous, non-indigenous Australian native, non-Australian, and unknown) varied across the visited land-use categories (Supplementary Table 4).
Food plant species flowering and fruiting phenologies
Phenology tables were constructed by compiling flowering and fruiting data collected from a range of published articles, online databases, and apiary flowering records. Where data were available, the peak time of flowering/fruiting, duration of flowering/fruiting in months, and annual reliability of flowering/fruiting, was recorded and standardized. Where records from the Northern Hemisphere were used, flowering/fruiting phenology was standardized by stating in which season flowering was recorded.
Data filtering, compilation and analyses were performed in the R environment for statistical computing (version 4.1.2; R Core Team, 2017) interfaced with RStudio (version 2021.09.0; RStudio Team, 2015).
Results
Land-use by foraging Pteropus poliocephalus
The spatial distribution of P. poliocephalus feeding fixes (n = 489) across land-use categories was significantly different to the proportions that would be expected based on the areal extent of available land-use types overall (Chi-squared for given probabilities χ2 = 726.33, df = 10, p < 0.001), indicating that individuals visited certain land-use categories more than others irrespective of their areal availability (Figure 3).
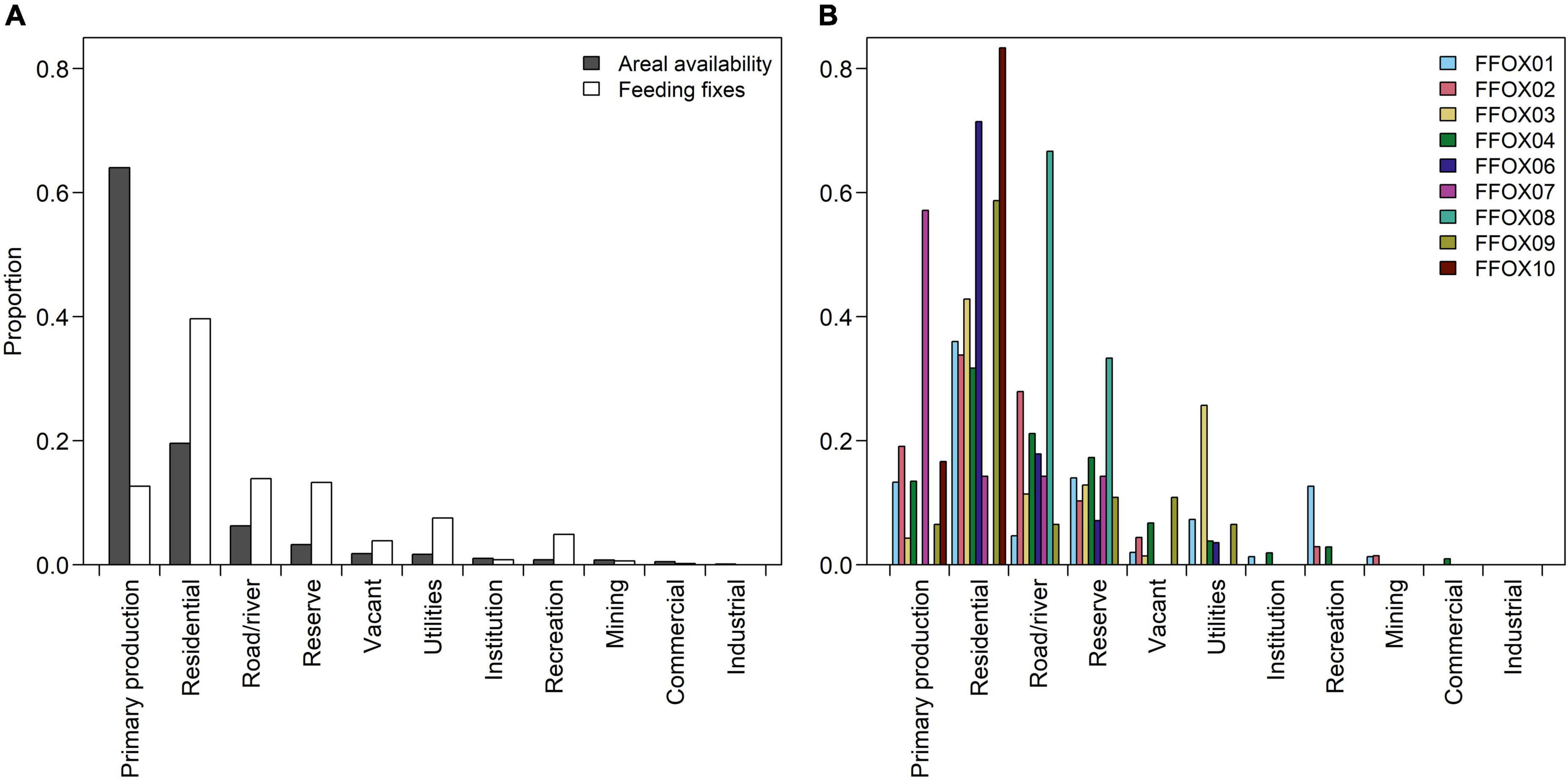
Figure 3. (A) the expected proportion of Pteropus poliocephalus feeding fixes in each land-use category based on areal availability (grey bars) and the observed proportion of feeding fixes in each land-use category overall (white bars), (B) the observed proportion of feeding fixes in each land-use category shown for each of the nine individuals (shown in different colors). Data (n = 489) were obtained from GPS tracked P. poliocephalus collected between December 2019 and May 2020.
The most visited land-use category, in terms of numbers of foraging fixes, was “residential” (n = 194, 39.7% of fixes), followed by “road/river” (n = 68, 13.9%, including roads: n = 66 fixes, and watercourses: n = 2 fixes; as the majority (97%) of the feeding fixes in this category pertained to road-side areas, we refer to the “road/river” category as “road-side areas” henceforth), “reserve” which includes national parks and natural vegetation (n = 65, 13.3%) and “primary production” (n = 62, 12.7%). However, of those most-visited categories, “reserves” were visited four times more frequently than expected from areal availability (n = 65, 13.3 vs 3.3% areal availability), followed by “residential” (n = 194, 39.7 vs 19.6%) and “road-side” (n = 68, 13.9 vs 6.3%) that were each visited approximately two times more frequently than expected; in contrast, the “primary production” category was visited approximately five times less frequently than expected (n = 62, 12.7 vs 64.0%).
The remaining visits to each land-use category made up less than 8% of fixes per category. In three of the less-visited land-use categories, visitation exceeded areal availability: “utilities” (n = 37; 7.6 vs 1.7% of areal availability), “recreation” (n = 24; 4.9 vs 0.8%), and “vacant” (n = 19; 3.8 vs 1.8%), and in the remaining less visited land-use categories visitation was lower than areal availability: “institution” (n = 16; 3.3 vs 1.0%), “mining” (n = 3; 0.6 vs 0.8%), “commercial” (n = 1; 0.2 vs 0.5%) and “industrial” (n = 0; 0.0 vs 0.1%) (Figure 3A). There was considerable variation within and between individuals in terms of land-use categories visited (Figure 3B).
Food plant species
The majority (63%) of the surveyed feeding locations (n = 265) comprised locally indigenous plant species (Figures 4A–J). However, 18% of visited food plants were identified as non-indigenous Australian natives, 16% non-Australian species, and 3% with unknown geographic origin (Figures 4A–J). The two most visited plants were Eucalyptus leucoxylon (35% of all visitations) and E. camaldulensis (16% of all visitations), both of which are locally indigenous to South Australia (Figures 4A–J and Table 1). The most visited non-Australian species was F. carica (n = 16; Figures 4A–J). The majority (81%) of F. carica feeding fixes occurred in residential areas (Figure 4B), although ten out of thirteen (76.9%) of these fixes were at a fig orchard.
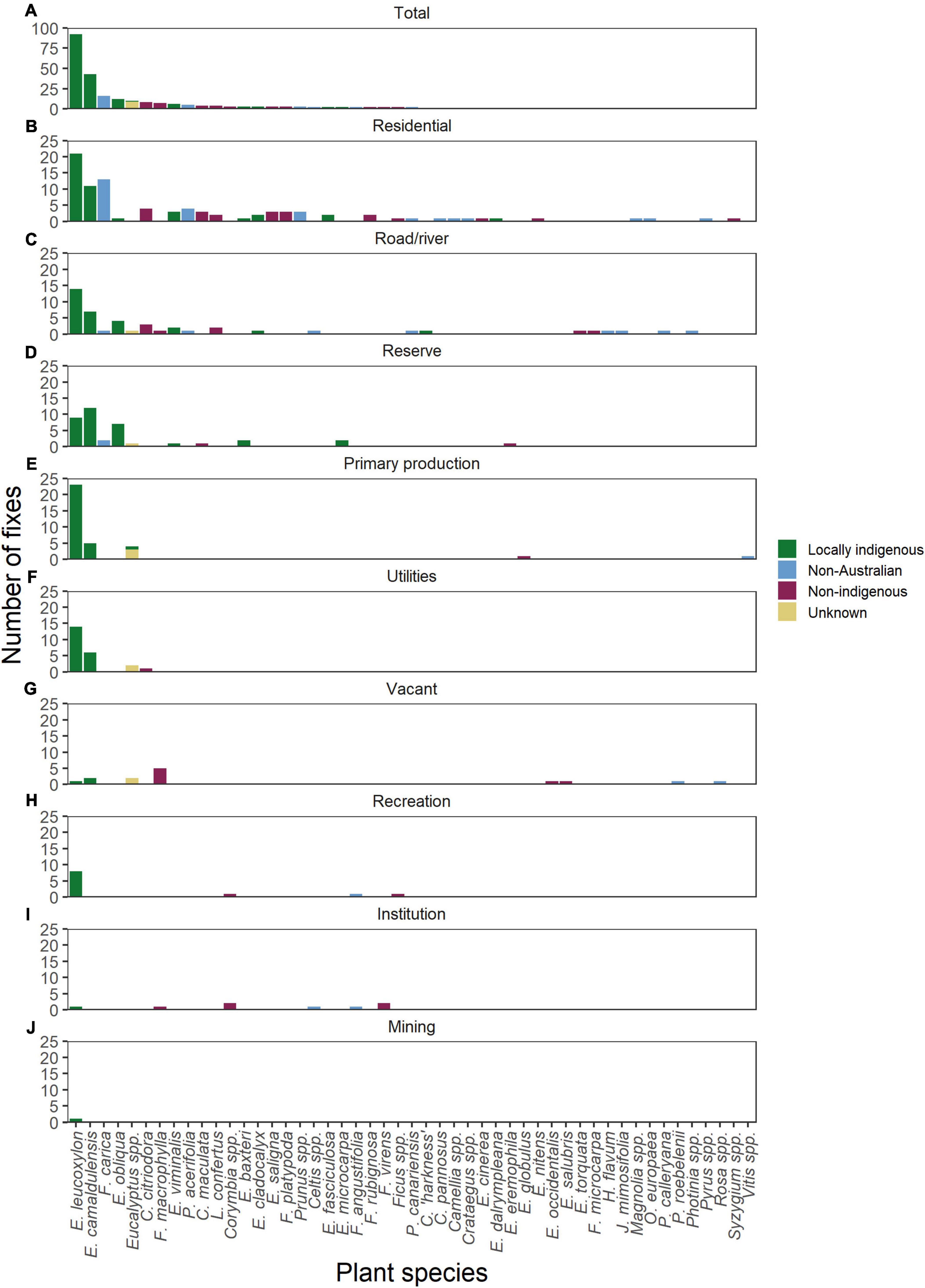
Figure 4. The number of plant species visitations (n = 265) by Pteropus poliocephalus. The top panel (A) shows the total number of plant species visitation across all land-use categories. The following panels show the number of visits in each land-use category separately: (B) residential (n = 90), (C) road/river (n = 46), (D) reserve (n = 38), (E) primary production (n = 34), (F) utilities (n = 23), (G) vacant (n = 14), (H) recreation (n = 11), (I) institution (n = 8), (J) mining (n = 1). Plant species are listed in order of most feeding visitations overall. Locally indigenous plant species are shown in green, non-indigenous Australian native species are shown in maroon, non-Australian species are shown in blue, and those with unknown geographic origin are shown in yellow. Data were obtained from nine GPS tracked P. poliocephalus roosting in the Adelaide region (–34.916, 138.607) from December 2019 to May 2020.
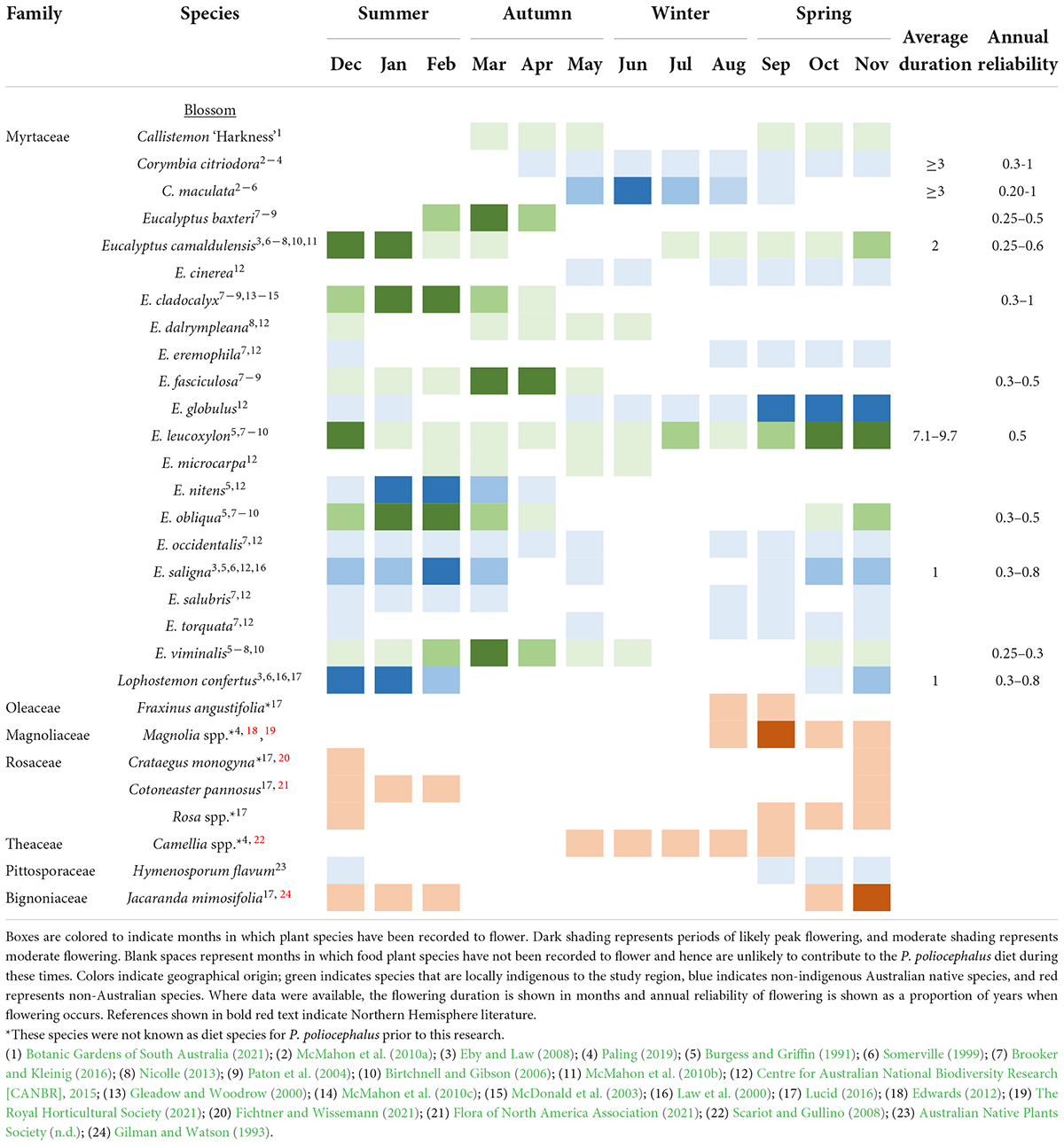
Table 1. The flowering phenology of the food plant species visited by (n = 9) GPS tracked Pteropus poliocephalus in the Adelaide region between Dec 2019 and May 2020.
Tracked P. poliocephalus were recorded visiting a total of 49 unique plant species during the study (Supplementary Table 5). Of these, 11 were classified as “locally indigenous” to the study region, 19 as “non-indigenous Australian natives,” and 19 as “non-Australian” species. Where plants could not be identified to species level so that their geographic origin could not be resolved, their geographic origin was conservatively classified as “unknown.” We found no difference in the relative number of unique species that were visited in each of the four “geographic origin” categories (i.e., locally indigenous, non-indigenous Australian native, non-Australian, and unknown) (Pearson’s Chi-squared χ2 = 19.415, df = 24, p = 0.730). However, we found that flying-foxes visited species from some geographic origins more frequently in some land-use categories than in others (Figures 4B–J; Pearson’s Chi-squared χ2 = 83.933, df = 24, p < 0.001). Flying-foxes visited locally indigenous species relatively more frequently in reserves (n = 33; 87% of fixes; Figure 4B), utilities (n = 20; 87% of fixes; Figure 4F), and areas used for primary production (n = 29; 85% of fixes; Figure 4E). Non-indigenous Australian native plant species were visited relatively more frequently in the institution (n = 5; 63% of fixes; Figure 4I), vacant (n = 7; 50% of fixes; Figure 4G), and residential (n = 21; 23% of fixes; Figure 4B) land-use categories than in the other land-use categories (Figure 4); while non-Australian plant species were visited relatively more frequently in residential (n = 27; 30% of fixes; Figure 4B), institution (n = 2; 25% of fixes; Figure 4I), road-side (n = 8; 17% of fixes; Figure 4C), and vacant land-use (n = 2; 14% of fixes; Figure 4G) land-use categories than in the other land-use categories (Figure 4).
Food plant species flowering and fruiting phenologies
Flowering and fruiting phenology records indicated that collectively, the plant species visited by the tracked individuals provide feeding resources throughout the year (Tables 1, 2). All of the locally indigenous food plant species visited were of the Myrtaceae family and known to contribute to the P. poliocephalus blossom diet (Table 1). The majority of these locally indigenous food plant species had been recorded to provide blossom during austral summer–autumn (Table 1). In addition, eleven of the twelve non-indigenous Australian native blossom food plant species were members of the Myrtaceae family and are known to contribute to the P. poliocephalus blossom diet (Table 1). While recurrent periods of natural food shortage during winter and spring have been recorded elsewhere in the P. poliocephalus range (Eby, 1991; Parry-Jones and Augee, 2001), tree species including C. citriodora and C. maculata (non-indigenous Australian natives) had been recorded to provide blossom during winter in the Adelaide region (Table 1). Though, like other Australian native species, Corymbias are unlikely to flower every year (Table 1). All of the fruiting food plant species visited by tracked P. poliocephalus were either non-Australian or non-indigenous Australian natives (Table 2). Non-indigenous Australian native food plants comprised fruiting Australian Ficus species including F. rubiginosa, F. platypoda, F. macrophylla, and F. microcarpa (Table 2). While many species of Ficus exhibit asynchronous fruiting and thus can produce fruits at any time of the year (Innis, 1989), ripening was often documented during summer-winter (Table 2). Several of the non-Australian fruiting food plant species have been recorded to provide food resources during winter and/or spring, and include Ficus carica (brown turkey fig), Cotoneaster pannosus (silverleaf cotoneaster), Crataegus monogyna (common hawthorn) Phoenix canariensis (Canary Island date palm), and Olea europaea (olive) (Table 2). One of the non-Australian food plant species visited (Platanus acerifolia) does not produce prominent flowers or fleshy fruit, and, although P. poliocephalus is known to consume the leaves and/or bark of some species including Populus spp. (Parry-Jones and Augee, 1991; Table 4.2), leaves and bark are not considered primary dietary components and are generally consumed during shortages of blossom and fruits (Eby and Law, 2008). These species are thus considered leave/bark food plant species and may provide minor supplementary resources year-round (Table 2).
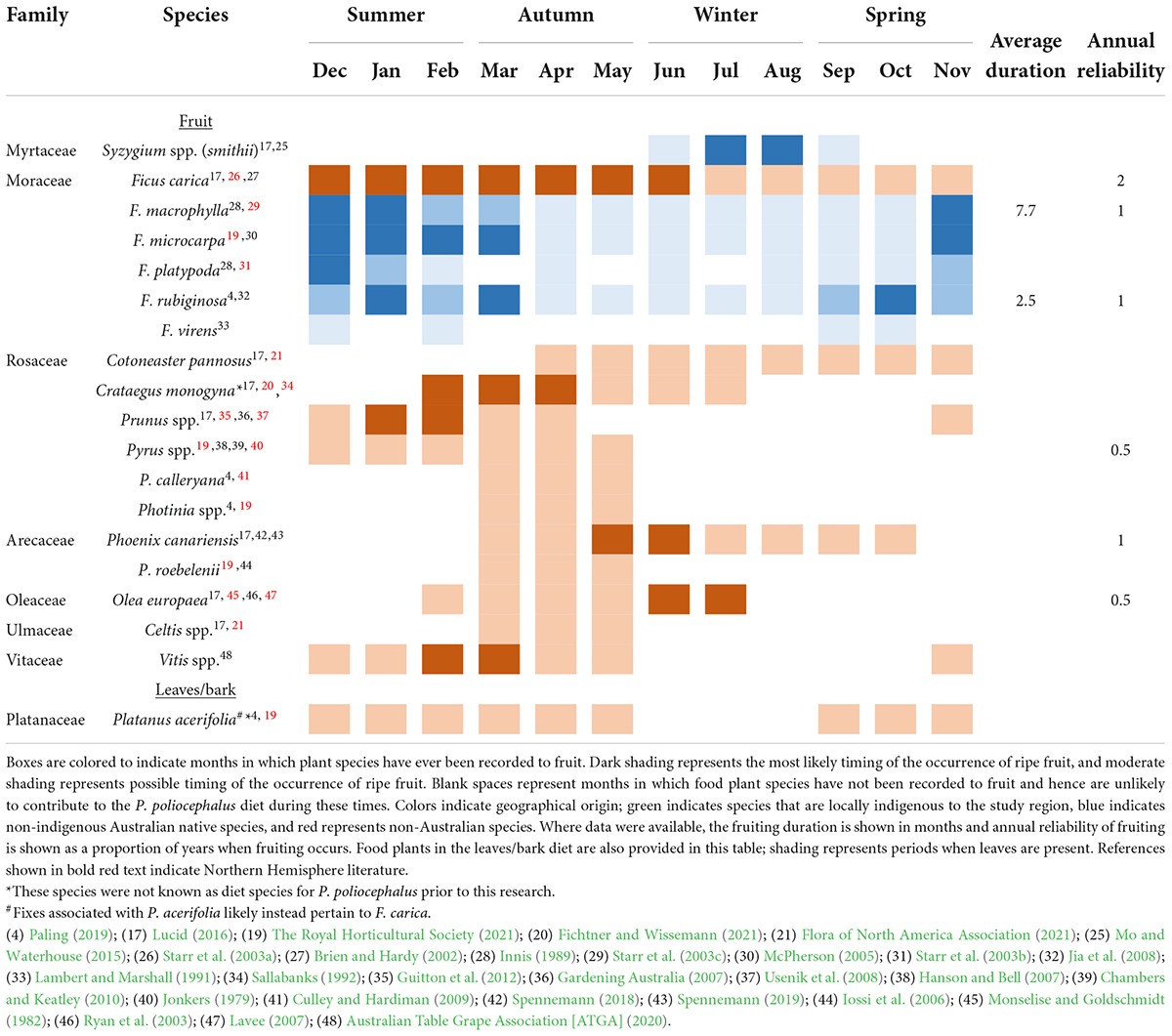
Table 2. The fruiting phenology of the food plant species visited by (n = 9) GPS tracked Pteropus poliocephalus in the Adelaide region between Dec 2019 and May 2020.
The annual reliability of food plant species was highly variable (Tables 1, 2). Where annual reliability data were available, the findings indicated that many locally indigenous and non-indigenous Australian native plant species contributing to the blossom diet do not flower every year but rather every 1–4 years (annual reliability of 0.25–1; Table 1). Most non-Australian, cultivated food plants fruit biennially; fruit either does not occur every other year, or in low abundance (Table 2; Monselise and Goldschmidt, 1982). However, some Ficus spp. including F. carica produce two crops per year; the main crop and the breba crop (Table 2; Stover et al., 2007). Thus, these non-Australian species exhibit higher annual reliability than many locally indigenous species (Tables 1, 2).
Discussion
Over recent decades, flying-foxes have become increasingly more common in urban areas, but the drivers of this were hitherto unknown. In this study, we used paired GPS and accelerometry data of nine P. poliocephalus tracked between December 2019 and May 2020 in urban Adelaide, South Australia, to investigate how individuals used the urban landscape mosaic for feeding. The four land-use categories used most for foraging were “residential” (40% of all feeding fixes), followed by “road-side,” “reserve,” and “primary-production” (13–14% each). However, of those most-visited categories, “reserves” were visited four times more frequently than expected from areal availability, followed by “residential” and “road-side” that were each visited approximately two times more frequently than expected; in contrast, the “primary production” category was visited approximately five times less frequently than expected (Figure 3). These results suggest that while residential areas are the most important land-use category for providing foraging resources supporting Adelaide’s flying-fox population, reserves provide foraging resources that are particularly attractive to the species. In contrast, the land used for primary production surrounding Adelaide was relatively less utilized, presumably because this land type is largely cleared of woody vegetation. Throughout the study, the tracked P. poliocephalus individuals foraged on an eclectic mixture of diet plants (n = 49 unique tree species), including locally indigenous species (63% of feeding fixes), non-indigenous Australian natives (18%), and non-Australian species (16%). However, in residential areas, non-indigenous Australian natives (23% of feeding fixes) and non-Australian species (30%) were visited relatively more frequently than in the other areas, whereas in reserves the overwhelming majority of plants visited were locally indigenous (87% of feeding fixes) (Figure 4). Data on the phenology of the plant species that were visited further indicated that the anthropogenic mixture of plants from different geographic origins provides greater temporal stability of foraging resources and access to food at times of the year when locally indigenous species tend not to flower or fruit (Tables 1, 2), particularly in residential areas. Thus, our findings highlight the common use of residential areas for foraging by urban-roosting P. poliocephalus and provide support for the hypothesis that the anthropogenic mixture of food resources enables the now near-permanent presence of the species in major urban areas. In addition, our findings emphasize the importance of conserving natural habitats for P. poliocephalus within urban landscape mosaics, and stress the need for accounting for wildlife responses to urban greening initiatives.
The majority of feeding visitations of tracked P. poliocephalus individuals were to locally indigenous food plant species, regardless of land-use category. The most visited locally indigenous food plant species were E. leucoxylon and E. camaldulensis. Other research has revealed similar results; that locally indigenous plant species are the most consumed food plants by fruit bat species in urban landscape mosaics (e.g., Lim et al., 2018). Our finding highlights the importance of locally indigenous species to the diet of urban-roosting P. poliocephalus during summer and autumn, this is reflected by the high rates of visitation to the reserve land-use category. All of the locally indigenous food plant species visited in our study belonged to the Myrtaceae family, a group often reported to exhibit irregular and intermittent flowering (Table 1; see also Law et al., 2000) and in particular limited flowering in winter (Table 1; see also Law et al., 2000), a reported time of natural food scarcity for Australian flying-foxes (Eby and Law, 2008). Urban landscapes are often warmer than surrounding natural areas (Magee et al., 1999), and are maintained by human intervention, and thus can have increased soil moisture and soil nutrient loads (Sukopp, 2004; Neil and Wu, 2006). These factors have been hypothesized to promote more regular and intense flowering for plants, including locally indigenous Australian natives, within urban landscapes (e.g., Davis et al., 2016), although given the key role of photoperiod in regulating plant reproduction (Jackson and Thomas, 1999; Jarillo et al., 2008; Abasolo et al., 2014) it is unlikely that the season of flowering would change even if conditions were favorable. Thus, while urban landscape characteristics may promote more reliable flowering and extended food availability of locally indigenous plant species, it is unlikely that these locally indigenous species alone could support P. poliocephalus in Adelaide year-round.
Tracked P. poliocephalus individuals visited a broad variety of food plants comprising a mixture of species from a range of geographic origins (Figure 4). Similar trends of urban diet composition have been recorded in P. alecto in southeast Queensland in Australia (Markus and Hall, 2004) and in other species of nectivorous and frugivorous bat in Singapore (e.g., Chan et al., 2021), Brazil (e.g., Nunes et al., 2017), and Malaysia (e.g., Lim et al., 2018). The flowering and fruiting phenology records presented in the current study show that several of the non-indigenous Australian native and non-Australian species visited provide nectar, pollen, and/or fruit during winter (e.g., C. citriodora, C. maculata, F. carica, O. europaea, and P. canariensis; Tables 1, 2) and so complement the temporal availability of foraging resources for flying-foxes, particularly in residential areas where more than 68% of the unique plant species visited were not locally indigenous. Although we did not track flying-foxes during winter, several of the known non-indigenous Australian native winter-flowering diet species were also used for foraging during our study period (e.g., C. citriodora and C. maculata; Eby and Law, 2008), and thus, we would expect individuals to feed on these species during winter if they were available. It is also possible that known native diet plants that only flower in winter and/or spring including Banksia integrifolia, E. albens, E. sideroxylon, and E. robusta (Eby and Law, 2008) may be present and support flying-foxes in urban Adelaide. Other research suggests that locally non-indigenous plants are important food resources during times of food scarcity, e.g., Rousettus aegyptiacus in central Cyprus exhibited a greater reliance on locally non-indigenous plant species during critical seasonal periods of food shortage (Luèan et al., 2016). Nevertheless, further research involving GPS tracking of P. poliocephalus and other flying-fox species throughout the calendar year will improve our understanding of how plants of different geographic origins support urban flying-fox populations year-round.
While there is little documented information on the history of urban plantings in Australia, the abundance and temporal stability of urban food resources have likely increased over the past few decades (Yau, 1982), and this is consistent with the recently observed urbanization of P. poliocephalus (Williams et al., 2006; Plowright et al., 2011; Timmiss et al., 2021). Non-Australian species have been planted in Australian cities and towns since European settlement in the late-18th century, replacing many Australian native species with those native to Europe (see Yau, 1982). These non-Australian species tended to be unsuitable as diet species for flying-foxes (planes, oaks, elms, ashes and poplars; Yau, 1982; Oldrey, 2003). However, native Australian trees (both locally indigenous and non-indigenous Australian natives), including Eucalyptus, Corymbia, Angophora, Lophostemon, and Ficus species gained popularity for street plantings in the late twentieth century (e.g., Yau, 1982; Oldrey, 2003), along with a new assemblage of non-Australian species including fruit-bearing apple and pear (Oldrey, 2003), thereby increasing the variety and abundance of floral and fruit resources available for flying-foxes in urban areas. Clearly, tracked P. poliocephalus were foraging on a variety of species that are relatively new additions to the composition of Adelaide’s plant assemblages (Figure 4; see also Oldrey, 2003), suggesting that these anthropogenic foraging resources have at least in part, led to the recent influx and permanent establishment of the species in urban Adelaide (since 2011). It has been suggested that recent flying-fox urbanization in Australia may also be a response to loss of natural habitat (e.g., Parry-Jones and Augee, 2001; van der Ree et al., 2006; Williams et al., 2006; Plowright et al., 2011; Páez et al., 2018) that has resulted from clearing of more than 40% of all forest and 80% of Eucalyptus-dominated areas on the continent (see Bradshaw, 2012). However, the increased urbanization of P. conspicillatus was not associated with loss or fragmentation of habitat immediately surrounding roost locations (Tait et al., 2014), potentially indicating that the increased urbanization of this species is a behavioral response to benefits offered by urban areas, albeit what these benefits are remained unclear (Tait et al., 2014). Like P. conspicillatus, P. poliocephalus is an extremely mobile species capable of tracking ephemeral foraging resources over vast spatial scales (Welbergen et al., 2020); therefore, at a local level, the abundance of the species can be expected to reflect the spatiotemporal variation in the carrying capacity of the environment (Welbergen et al., 2020). While the substantial loss of natural habitat has likely reduced the carrying capacity of Australia’s natural landscape for P. poliocephalus, and is clearly implicated in the decline of the species (Woinarski et al., 2014; Eby et al., 2021), it is unlikely to have resulted in an increased presence of the species in urban environments without a concomitant change in the carrying capacity of urban areas. However, to confirm that recent flying-fox urbanization is, at least in part, the result of increased spatiotemporal availability of urban food plant resources, detailed mapping of the historical changes in Australia’s urban vegetation is needed.
Our findings also highlight the importance of accounting for wildlife species’ responses when devising urban greening initiatives as the tree species chosen to revegetate urban areas may support wildlife species in unexpected ways. It is now clear that flying-foxes are attracted to the variety of food resources offered by urban areas, but feeding in these habitats can expose P. poliocephalus to human-wildlife conflict (Kung et al., 2015) and other anthropogenic threats including electrocution on powerlines, fruit tree netting entanglement, and vehicle collisions (Scheelings and Frith, 2015). The areas and food plant species visited by P. poliocephalus in our study provide potential targets for government-subsidized promotional activities and direct tree plantings to help support flying-foxes in urban areas. For example, our findings suggest that residential areas are currently the most important land-use category for providing foraging resources supporting Adelaide’s flying-fox population year-round, with the greatest number of feeding visits occurring in this land-use category, predominantly to species that were not locally indigenous. Thus, increasing the local uptake of species such as C. maculata, C. citriodora, and Ficus spp. via public awareness campaigns and/or promotion through local garden centers could benefit P. poliocephalus by further enhancing the abundance and temporal stability of food resources for flying-foxes in residential areas. Conversely, these same food plants highlighted here could also provide targets for removal to help minimize local exposure of P. poliocephalus to conflict with humans and other anthropogenic threats. Provided such management of foraging resources does not result in a net reduction in the foraging resource base for the species overall, this too can thus support the conservation of this vulnerable species in anthropogenic environments (Yabsley et al., 2021). Our findings also suggest that natural habitats, including reserves, nestled within urban landscape mosaics, provide locally indigenous foraging resources that are particularly attractive to the species. Thus, land managers could consider species such as E. leucoxylon and E. camaldulensis as good candidates for tree planting schemes in emerging and developing urban greenspaces, albeit they would not provide food resources for P. poliocephalus year-round. The non-locally indigenous species mentioned above could also be planted in non-human occupied areas including reserves to increase the availability of urban foraging resources in areas away from human residences. Further research combining long-term tracking (e.g., Welbergen et al., 2020), diet analysis (e.g., Bell et al., 2020), and comprehensive mapping of urban foraging resources for flying-foxes and other fauna is necessary to account for wildlife species’ responses to urban greening initiatives, and inform more effective approaches to urban wildlife management.
Data availability statement
The datasets presented in this study can be found in online repositories. The names of the repository/repositories and accession number(s) can be found below: Dryad: https://doi.org/10.5061/dryad.tx95x6b0t.
Ethics statement
The animal study was reviewed and approved by Western Sydney University, Animal Research Authority no. A12217.
Author contributions
SY led the writing of the manuscript. JW, CT, JMa, JMe, and SY were responsible for methodology and conceptualization. JMa, CT, WB, and MW caught bats and fitted transmitters. JMa programmed and remotely monitored transmitters. SY, TH, and WB visited feeding locations, collected plant samples, and photographed trees for identification. DN confirmed plant species identifications from photographs. MW and CT performed accelerometer analysis. SY and JMe performed statistical analyses. JW, JMe, CT, and JMa were responsible for funding acquisition. All authors worked to produce the study, contributed to drafts, and gave final approval for publication.
Funding
This research was supported by funding from the Australian Research Council (Discovery Grant DP170104272), the Hawkesbury Institute for the Environment (Western Sydney University), and the South Australian Department for Environment and Water.
Acknowledgments
We would like to thank volunteers for their assistance with capture of flying-foxes and the deployment of transmitters, and to Sonya Yabsley for her fieldwork assistance with plant species visitations. We thank Jason van Weenen (South Australian Department for Environment and Water, Environment, Heritage and Sustainability Division) for making GPS trackers available, for logistical support, and help with plant identification. We thank Ian Smith (Zoos SA) and Kathy Burbidge (University of Adelaide) for logistical support. We also thank the owner of Glen Ewin Estate for kindly allowing us access to their fig orchard and for providing information regarding the species planted on the property.
Conflict of interest
The authors declare that the research was conducted in the absence of any commercial or financial relationships that could be construed as a potential conflict of interest.
Publisher’s note
All claims expressed in this article are solely those of the authors and do not necessarily represent those of their affiliated organizations, or those of the publisher, the editors and the reviewers. Any product that may be evaluated in this article, or claim that may be made by its manufacturer, is not guaranteed or endorsed by the publisher.
Supplementary material
The Supplementary Material for this article can be found online at: https://www.frontiersin.org/articles/10.3389/fevo.2022.907966/full#supplementary-material
Footnotes
- ^ Walker, M. J. (unpublished). Controlled hyperthermia in a freeliving flying-fox exposed to extreme heat. Ph.D thesis. Penrith, NSW: Western Sydney University.
References
Abasolo, M., Lee, D. J., Brooks, L., Raymond, C., and Shepherd, M. (2014). Genetic control of flowering in spotted gum, Corymbia citriodora subsp. variegata and C. maculata. Aust. J. Bot. 62, 22–35. doi: 10.1071/BT13223
Aston, H. (1987). Influx of the grey-headed flying-fox Pteropus poliocephalus (Chiroptera: Pteropodidae) to the Melbourne area, Victoria, in 1986. Vic. Nat. 104, 9–13.
Australian Native Plants Society (n.d.). Hymenosporum Flavum. Available Online at: http://anpsa.org.au/h-fla.html (accessed Sept 25, 2021).
Australian Table Grape Association [ATGA] (2020). Australian Table Grape Association. Mildura VIC: Australian Table Grape Association.
Baruch-Mordo, S., Wilson, K. R., Lewis, D. L., Broderick, J., Mao, J. S., and Breck, S. W. (2014). Stochasticity in natural forage production affects use of urban areas by black bears: Implications to management of human-bear conflicts. PLoS One 9:e85122. doi: 10.1371/journal.pone.0085122
Bell, K. L., Batchelor, K. L., Bradford, M., McKeown, A., Macdonald, S. L., and Westcott, D. (2020). Optimisation of a pollen DNA metabarcoding method for diet analysis of flying-foxes (Pteropus spp.). Aust. J. Zool. 68, 273–284. doi: 10.1071/ZO20085
Birtchnell, M. J., and Gibson, M. (2006). Long-term flowering patterns of melliferous Eucalyptus (Myrtaceae) species. Aust. J. Bot. 54, 745–754. doi: 10.1071/BT05160
Boardman, W. S. J., Roshier, D., Reardon, T., Burbidge, K., McKeown, A., Westcott, D. A., et al. (2021). Spring foraging movements of an urban population of grey-headed flying foxes (Pteropus poliocephalus). J. Urban Ecol. 6, 1–10.
Botanic Gardens of South Australia (2021). Plant Selector. Adelaide: Botanic Gardens of South Australia.
Bradshaw, C. J. (2012). Little left to lose: Deforestation and forest degradation in Australia since European colonization. Plant Ecol. 5, 109–120. doi: 10.1093/jpe/rtr038
Brien, J., and Hardy, S. (2002). Fig growing in NSW. New South Wales Government Department of Primary Industries. Available Online at: https://www.dpi.nsw.gov.au/__data/assets/pdf_file/0017/119501/fig-growing-nsw.pdf (accessed Sept 16, 2021).
Broekhuis, F., Cushman, S. A., and Elliot, N. B. (2017). Identification of human–carnivore conflict hotspots to prioritize mitigation efforts. Ecol. Evol. 7, 10630–10639. doi: 10.1002/ece3.3565
Brooker, M. I. H., and Kleinig, D. A. (2016). Field Guide to Eucalypts: South-Western and Southern Australia. Melbourne: Bloomings Books Pty Ltd.
Burgess, I., and Griffin, A. R. (1991). Flowering Time Of Eucalyptus Species In Their Natural Habitat. Canberra: CSIRO, Division of Forestry.
Callaghan, C. T., Bino, G., Major, R. E., Martin, J. M., Lyons, M. B., and Kingsford, R. T. (2019). Heterogeneous urban green areas are bird diversity hotspots: Insights using continental-scale citizen science data. Landsc. Ecol. 34, 1231–1246. doi: 10.1007/s10980-019-00851-6
Centre for Australian National Biodiversity Research [CANBR] (2015). EUCLID: Eucalypts of Australia Edition 4. Available Online at: https://apps.lucidcentral.org/euclid/text/intro/index.html (accessed Sept 16, 2021).
Chace, J. F., and Walsh, J. J. (2006). Urban effects on native avifauna: A review. Landsc. Urban Plan. 74, 46–69. doi: 10.1016/j.landurbplan.2004.08.007
Chambers, L. E., and Keatley, M. R. (2010). Phenology and climate–early Australian botanical records. Aust. J. Bot. 58, 473–484. doi: 10.1071/BT10105
Chan, A. A. Q., Aziz, S. A., Clare, E. L., and Coleman, J. L. (2021). Diet, ecological role and potential ecosystem services of the fruit bat, Cynopterus brachyotis, in a tropical city. Urban Ecosyst. 24, 251–263. doi: 10.1007/s11252-020-01034-x
Collins, P. M., Green, J. A., Warwick-Evans, V., Dodd, S., Shaw, P. J., Arnould, J. P. Y., et al. (2015). Interpreting behaviors from accelerometry: A method combining simplicity and objectivity. Ecol. Evol. 5, 4642–4654. doi: 10.1002/ece3.1660
Concepción, E. D., Moretti, M., Altermatt, F., Nobis, M. P., and Obrist, M. K. (2015). Impacts of urbanisation on biodiversity: The role of species mobility, degree of specialisation and spatial scale. Oikos 124, 1571–1582. doi: 10.1111/oik.02166
Culley, T. M., and Hardiman, N. A. (2009). The role of intraspecific hybridization in the evolution of invasiveness: A case study of the ornamental pear tree Pyrus calleryana. Biol. Invasions 11, 1107–1119. doi: 10.1007/s10530-008-9386-z
Currey, K., Kendal, D., van der Ree, R., and Lentini, P. (2018). Land manager perspectives on conflict mitigation strategies for urban flying-fox camps. Diversity 10, 1–15. doi: 10.3390/d10020039
Davis, A., Major, R. E., and Taylor, C. E. (2016). Do trees flower longer in the city? A comparison of flowering eucalyptus trees in streets, remnants and continuous forest and their association with nectarivorous birds. Urban Ecosyst. 19, 735–747. doi: 10.1007/s11252-015-0515-z
de Souza Laurindo, R., and Vizentin-Bugoni, J. (2020). Diversity of fruits in Artibeus lituratus diet in urban and natural habitats in Brazil: A review. J. Trop. Ecol. 36, 65–71. S0266467419000373 doi: 10.1017/S0266467419000373
Delahay, R., Davison, J., Poole, D., Matthews, A., Wilson, C., Heydon, M., et al. (2009). Managing conflict between humans and wildlife: Trends in licensed operations to resolve problems with badgers Meles meles in England. Mamm. Rev. 39, 53–66. doi: 10.1111/j.1365-2907.2008.00135.x
Dwyer, J. F., and Mannan, R. W. (2007). Preventing raptor electrocutions in an urban environment. J. Raptor Res. 41, 259–267. doi: 10.3356/0892-1016(2007)41[259:PREIAU]2.0.CO;2
Eby, P. (1991). Seasonal movements of grey-headed flying-foxes, Pteropus poliocephalus (Chiroptera: Pteropodidae), from two maternity camps in northern New South Wales. Wildl. Res. 18, 547–559. doi: 10.1071/WR9910547
Eby, P., and Law, B. (2008). Ranking The Feeding Habitat Of Grey-Headed Flying Foxes For Conservation Management”. Canberra: Department of Environment and Climate Change (NSW).
Eby, P., Roberts, B., Pennay, M., and Welbergen, J. A. (2021). Pteropus poliocephalus. The IUCN red list of threatened species. e.T18751A22085511. doi: 10.2305/IUCN.UK.2021-3.RLTS.T18751A22085511.en
Edwards, R. A. (2012). Observations On Magnolias At Lincoln University. Available Online at: https://researcharchive.lincoln.ac.nz/bitstream/handle/10182/4460/Observations_on_Magnolias.pdf;sequence=1 (accessed Sept 25, 2021).
Evans, K. L., Newton, J., Gaston, K. J., Sharp, S. P., McGowan, A., and Hatchwell, B. J. (2012). Colonisation of urban environments is associated with reduced migratory behaviour, facilitating divergence from ancestral populations. Oikos 121, 634–640. doi: 10.1111/j.1600-0706.2011.19722.x
Fenoglio, M. S., Calviño, A., González, E., Salvo, A., and Videla, M. (2021). Urbanisation drivers and underlying mechanisms of terrestrial insect diversity loss in cities. Ecol. Entomol. 46, 757–771. doi: 10.1111/een.13041
Fernández-Gil, A., Naves, J., Ordiz, A., Quevedo, M., Revilla, E., and Delibes, M. (2016). Conflict misleads large carnivore management and conservation: Brown bears and wolves in Spain. PLoS One 11:e0151541. doi: 10.1371/journal.pone.0151541
Fichtner, A., and Wissemann, V. (2021). Biological flora of the British Isles: Crataegus monogyna. J. Ecol. 109, 541–571. doi: 10.1111/1365-2745.13554
Flora of North America Association (2021). Flora of North America. Available Online at: http://beta.floranorthamerica.org/w/index.php?search= (accessed Sept 24, 2021).
Gardening Australia (2007). Plant Finder. Available Online at: https://www.abc.net.au/gardening/plant-finder/prunus/9442090 (accessed Dec 26, 2021).
Gilman, E. F., and Watson, D. G. (1993). Jacaranda Mimosifolia. Gainesville: Environmental Horticulture Department.
Gleadow, R. M., and Woodrow, I. E. (2000). Temporal and spatial variation in cyanogenic glycosides in Eucalyptus cladocalyx. Tree Physiol. 20, 591–598. doi: 10.1093/treephys/20.9.591
Government of South Australia (2020). Generalised land use (2017-current). Barton: Attorney-General’s Department.
Grimm, N. B., Faeth, S. H., Golubiewski, N. E., Redman, C. L., Wu, J., Bai, X., et al. (2008). Global change and the ecology of cities. Science 319, 756–760. doi: 10.1126/science.1150195
Guitton, B., Kelner, J.-J., Velasco, R., Gardiner, S. E., Chagne, D., and Costes, E. (2012). Genetic control of biennial bearing in apple. J. Exp. Bot. 63, 131–149. doi: 10.1093/jxb/err261
Hall, L. S., and Richards, G. (2000). Flying Foxes: Fruit And Blossom Bats Of Australia. Sydney, NSW: University of New South Wales.
Hanson, J., and Bell, M. (2007). Harvest trails in Australia: Patterns of seasonal migration in the fruit and vegetable industry. J. Rural Stud. 23, 101–117. doi: 10.1016/j.jrurstud.2006.05.001
Innis, G. J. (1989). Feeding ecology of fruit pigeons in subtropical rainforests of south-eastern Queensland. Wildl. Res. 16, 365–394. doi: 10.1071/WR9890365
International Union for Conservation of Nature [IUCN] (2021). The IUCN Red List of Threatened Species. Gland: International Union for Conservation of Nature.
Iossi, E., Moro, F. V., and Sader, R. (2006). Seed anatomy and germination of Phoenix roebelenii O’Brien (Arecaceae). J. Seed Sci. 28, 121–128. doi: 10.1590/S0101-31222006000300018
Jackson, S. D., and Thomas, B. (1999). “The photoperiodic control of plant reproduction,” in Concepts In Photobiology: Photosynthesis And Photomorphogenesis, eds G. S. Singhal, G. Renger, S. K. Sopory, K. D. Irrgang, and Govindjee (Dordrecht: Springer), 868–896.
Jarillo, J. A., del Olmo, I., Gómez-Zambrano, A., Lázaro, A., López-González, L., Miguel, E., et al. (2008). Photoperiodic control of flowering time: A review. Span. J. Agric. Res. 6, 221–244. doi: 10.5424/sjar/200806S1-391
Jia, X. C., Yao, J. Y., Chen, Y. Z., Cook, J. M., and Crozier, R. H. (2008). The phenology and potential for self-pollination of two Australian monoecious fig species. Symbiosis 45, 91–96.
Jonkers, H. (1979). Biennial bearing in apple and pear: A literature survey. Sci. Hortic. 11, 303–317. doi: 10.1016/0304-4238(79)90015-3
Jung, K., and Threlfall, C. G. (2018). Trait-dependent tolerance of bats to urbanization: A global meta-analysis. Proc. R. Soc. B 285, 1–9. doi: 10.1098/rspb.2018.1222
Kendal, D., Williams, K. J., and Williams, N. S. (2012). Plant traits link people’s plant preferences to the composition of their gardens. Landsc. Urban Plan. 105, 34–42. doi: 10.1016/j.landurbplan.2011.11.023
Kung, N. Y., Field, H. E., McLaughlin, A., Edson, D., and Taylor, M. (2015). Flying-foxes in the Australian urban environment—community attitudes and opinions. One Health 1, 24–30. doi: 10.1016/j.onehlt.2015.07.002
Lambert, F. R., and Marshall, A. G. (1991). Keystone characteristics of bird-dispersed Ficus in a Malaysian lowland rain forest. J. Ecol. 79, 793–809. doi: 10.2307/2260668
Lavee, S. (2007). Biennial bearing in olive (Olea europaea). Ann. Ser. Hist. Nat. 17, 101–112. doi: 10.1016/j.scienta.2006.12.017
Law, B., Mackowski, C., Schoer, L., and Tweedie, T. (2000). Flowering phenology of myrtaceous trees and their relation to climatic, environmental and disturbance variables in northern New South Wales. Austral Ecol. 25, 160–178. doi: 10.1046/j.1442-9993.2000.01009.x
Lim, V.-C., Clare, E. L., Littlefair, J. E., Ramli, R., Bhassu, S., and Wilson, J.-J. (2018). Impact of urbanisation and agriculture on the diet of fruit bats. Urban Ecosyst. 21, 61–70. doi: 10.1007/s11252-017-0700-3
Lothian, N. (1980). Trees in botanic gardens - 7. Adelaide Botanic Gardens. Arboric. J. 4, 11–21. doi: 10.1080/03071375.1980.10590582
Luèan, R. K., Bartonièka, T., Jedlièka, P., Øeøucha, Š, Šálek, M., Èížek, M., et al. (2016). Spatial activity and feeding ecology of the endangered northern population of the Egyptian fruit bat (Rousettus aegyptiacus). J. Mammal. 97, 815–822. doi: 10.1093/jmammal/gyw014
Magee, N., Curtis, J., and Wendler, G. (1999). The urban heat island effect at Fairbanks, Alaska. Theor. Appl. Climatol. 64, 39–47. doi: 10.1007/s007040050109
Markus, N., and Hall, L. (2004). Foraging behaviour of the black flying-fox (Pteropus alecto) in the urban landscape of Brisbane, Queensland. Wildl. Res. 31, 345–355. doi: 10.1071/WR01117
Marzluff, J. M., and Ewing, K. (2001). Restoration of fragmented landscapes for the conservation of birds: a general framework and specific recommendations for urbanizing landscapes. Restor. Ecol. 9, 280–292. doi: 10.1046/j.1526-100x.2001.009003280.x
McDonald, M., Rawlings, M., Butcher, P., and Bell, J. (2003). Regional divergence and inbreeding in Eucalyptus cladocalyx (Myrtaceae). Aust. J. Bot. 51, 393–403. doi: 10.1071/BT02106
McDonald-Madden, E., Schreiber, E., Forsyth, D., Choquenot, D., and Clancy, T. (2005). Factors affecting grey-headed flying-fox (Pteropus poliocephalus: Pteropodidae) foraging in the Melbourne metropolitan area, Australia. Austral Ecol. 30, 600–608. doi: 10.1111/j.1442-9993.2005.01492.x
McKinney, M. L. (2002). Urbanization, biodiversity, and conservation. Bioscience 52, 883–890. doi: 10.1641/0006-3568(2002)052[0883:UBAC]2.0.CO;2
McKinney, M. L., and Lockwood, J. L. (1999). Biotic homogenization: A few winners replacing many losers in the next mass extinction. Trends Ecol. Evol. 14, 450–453. doi: 10.1016/S0169-5347(99)01679-1
McMahon, L., George, B., and Hean, R. (2010a). Corymbia Maculata, Corymbia Citriodora Subsp, Variegata And Corymbia Henryi. Sydney, NSW: New South Wales Government Industry and Investment.
McMahon, L., George, B., and Hean, R. (2010b). Eucalyptus Camaldulensis. Sydney, NSW: New South Wales Government Industry and Investment.
McMahon, L., George, B., and Hean, R. (2010c). Eucalyptus Cladocalyx. Sydney, NSW: New South Wales Government Industry and Investment.
McPherson, J. R. (2005). Phenology of six Ficus L., Moraceae, species and its effects on pollinator survival, in Brisbane, Queensland, Australia. Geogr. Res. 43, 297–305. doi: 10.1111/j.1745-5871.2005.00329.x
Meade, J., Martin, J. M., and Welbergen, J. A. (2021). Fast food in the city? Nomadic flying-foxes commute less and hang around for longer in urban areas. Behav. Ecol. 32, 1151–1162. doi: 10.1093/beheco/arab078
Milazzo, A., Giles, L., Zhang, Y., Koehler, A., Hiller, J., and Bi, P. (2016). The effect of temperature on different Salmonella serotypes during warm seasons in a Mediterranean climate city, Adelaide, Australia. Epidemiol. Infect. 144, 1231–1240. doi: 10.1017/S0950268815002587
Mo, M., and Waterhouse, D. R. (2015). Fruiting phenologies of rainforest plants in the Illawarra region, New South Wales, 1988-1992. Proc. Linn. Soc. N. S. W. 137, 17–27.
Mo, M., Roache, M., Haering, R., and Kwok, A. (2020). Using wildlife carer records to identify patterns in flying-fox rescues: A case study in New South Wales, Australia. Pac. Conserv. Biol. 27, 61–69. doi: 10.1071/PC20031
Monselise, S., and Goldschmidt, E. (1982). Alternate bearing in fruit trees. Hortic. Rev. 4, 128–173. doi: 10.1007/978-1-349-06519-6_5
Neil, K., and Wu, J. (2006). Effects of urbanization on plant flowering phenology: A review. Urban Ecosyst. 9, 243–257. doi: 10.1007/s11252-006-9354-2
Nelson, J. (1965). Movements of Australian flying foxes (Pteropodidae: Megachiroptera). Aust. J. Zool. 13, 53–73. doi: 10.1071/ZO9650053
Nunes, H., Rocha, F. L., and Cordeiro-Estrela, P. (2017). Bats in urban areas of Brazil: Roosts, food resources and parasites in disturbed environments. Urban Ecosyst. 20, 953–969. doi: 10.1007/s11252-016-0632-3
Öckinger, E., Schweiger, O., Crist, T. O., Debinski, D. M., Krauss, J., Kuussaari, M., et al. (2010). Life-history traits predict species responses to habitat area and isolation: A cross-continental synthesis. Ecol. Lett. 13, 969–979. doi: 10.1111/j.1461-0248.2010.01487.x
Oldrey, L. (2003). “Adelaide’s street trees: past, present and future,” in Proceedings Of The 4th National Street Tree Symposium, (Myrtle Bank: Treenet), 151.
Páez, D. J., Restif, O., Eby, P., and Plowright, R. K. (2018). Optimal foraging in seasonal environments: Implications for residency of Australian flying foxes in food-subsidized urban landscapes. Philos. Trans. R. Soc. B Biol. Sci. 373, 1–8. doi: 10.1098/rstb.2017.0097
Parry-Jones, K. A., and Augee, M. L. (2001). Factors affecting the occupation of a colony site in Sydney, New South Wales by the grey-headed Flying-fox Pteropus poliocephalus (Pteropodidae). Austral Ecol. 26, 47–55. doi: 10.1111/j.1442-9993.2001.01072.pp.x
Parry-Jones, K., and Augee, M. (1991). Food selection by grey-headed flying foxes (Pteropus poliocephalus) occupying a summer colony site near Gosford, New South Wales. Wildl. Res. 18, 111–124. doi: 10.1071/WR9910111
Parry-Jones, K., and Augee, M. (1992). Movements of grey-headed flying foxes (Pteropus poliocephalus) to and from colony site on the central coast of New South Wales. Wildl. Res. 19, 331–339. doi: 10.1071/WR9920331
Paton, D. C., Crossfield, E. L., Hurrell, B., and Rogers, D. J. (2004). Floral Resources Used By The South Australian Apiary Industry. Canberra: Rural Industries Research and Development Corporation, Australian Government.
Plowright, R. K., Foley, P., Field, H. E., Dobson, A. P., Foley, J. E., Eby, P., et al. (2011). Urban habituation, ecological connectivity and epidemic dampening: The emergence of Hendra virus from flying foxes (Pteropus spp.). Proc. R. Soc. B Biol. Sci. 278, 3703–3712. doi: 10.1098/rspb.2011.0522
R Core Team (2017). R: A Language And Environment For Statistical Computing. Vienna: R Foundation for Statistical Computing.
Richards, G. (2002). “The development of strategies for management of the flying-fox colony at the Royal Botanic Gardens, Sydney,” in Managing The Grey-Headed Flying-Fox As A Threatened Species In New South Wales, eds P. Eby and D. Lunney (Mosman, NSW: Royal Zoological Society of New South Wales(). doi: 10.7882/FS.2002.052
Roberts, B. J., Mo, M., Roache, M., and Eby, P. (2021). Review of dispersal attempts at flying-fox camps in Australia. Aust. J. Zool. 68, 254–272. doi: 10.1071/ZO20043
Runfola, D., Anderson, A., Baier, H., Crittenden, M., Dowker, E., Fuhrig, S., et al. (2020). GeoBoundaries: A global database of political administrative boundaries. PLoS One 15:e0231866. doi: 10.1371/journal.pone.0231866
Ryan, D., Prenzler, P. D., Lavee, S., Antolovich, M., and Robards, K. (2003). Quantitative changes in phenolic content during physiological development of the olive (Olea europaea) cultivar Hardy’s Mammoth. J. Agric. Food Chem. 51, 2532–2538. doi: 10.1021/jf0261351
Sallabanks, R. (1992). Fruit fate, frugivory, and fruit characteristics: A study of the hawthorn, Crataegus monogyna (Rosaceae). Oecologia 91, 296–304. doi: 10.1007/BF00317800
Scariot, V., and Gullino, P. (2008). Evaluation of flowering time and ornamental characteristics in autumn camellias. Acta Hortic. 885, 319–324. doi: 10.17660/ActaHortic.2010.885.44
Scheelings, T. F., and Frith, S. E. (2015). Anthropogenic factors are the major cause of hospital admission of a threatened species, the grey-headed flying fox (Pteropus poliocephalus), in Victoria, Australia. PLoS One 10:e0133638. doi: 10.1371/journal.pone.0133638
Somerville, D. (1999). Floral Resource Database For The NSW Apiary Industry. Goulburn, NSW: Rural Industries Research and Development Corporation.
Soulsbury, C. D., and White, P. C. L. (2015). Human-wildlife interactions in urban areas: A review of conflicts, benefits and opportunities. Wildl. Res. 42, 541–553. doi: 10.1071/WR14229
Spennemann, D. (2018). Observations on the consumption and dispersal of Phoenix canariensis drupes by the grey-headed flying-fox (Pteropus poliocephalus). Eur. J. Ecol. 4, 41–49. doi: 10.2478/eje-2018-0005
Spennemann, D. H. (2019). The contribution of the Canary Island date palm (Phoenix canariensis) to the winter diet of frugivores in novel ecosystems. Eur. J. Ecol. 5, 27–37. doi: 10.2478/eje-2019-0005
Starr, F., Starr, K., and Loope, L. (2003b). Ficus cf. Platypoda. Reston: United States Geological Survey.
Starr, F., Starr, K., and Loope, L. (2003c). Ficus Macrophylla. Reston: United States Geological Survey.
Stover, E., Aradhya, M., Ferguson, L., and Crisosto, C. H. (2007). The fig: Overview of an ancient fruit. HortScience 42, 1083–1087. doi: 10.21273/HORTSCI.42.5.1083
Sukopp, H. (2004). Human-caused impact on preserved vegetation. Landsc. Urban Plan. 68, 347–355. doi: 10.1016/S0169-2046(03)00152-X
Tait, C. J., Daniels, C. B., and Hill, R. S. (2005). Changes in species assemblages within the Adelaide metropolitan area, Australia, 1836–2002. Ecol. Appl. 15, 346–359. doi: 10.1890/04-0920
Tait, J., Perotto-Baldivieso, H. L., McKeown, A., and Westcott, D. A. (2014). Are flying-foxes coming to town? Urbanisation of the spectacled flying-fox (Pteropus conspicillatus) in Australia. PLoS One 9:e109810. doi: 10.1371/journal.pone.0109810
Taylor-Brown, A., Booth, R., Gillett, A., Mealy, E., Ogbourne, S. M., Polkinghorne, A., et al. (2019). The impact of human activities on Australian wildlife. PLoS One 14:e0206958. doi: 10.1371/journal.pone.0206958
The Royal Horticultural Society (2021). Find Gardening Advice. London: The Royal Horticultural Society.
Threatened Species Scientific Committee (2001). Commonwealth Listing Advice On Pteropus Poliocephalus (Grey-Headed Flying-Fox). Canberra: Department of the Environment and Energy.
Threatened Species Scientific Committee (2019). Conservation Advice: Pteropus Conspicillatus Spectacled Flying-Fox. Canberra: Department of the Environment and Energy.
Tillman, E. A., Van Doom, A., and Avery, M. L. (2000). “Bird damage to tropical fruit in south Florida,” in Wildlife Damage Management Conferences–Proceedings, eds M. C. Brittingham, J. Kays, and R. McPeake (State College, PA: College of Agricultural Sciences).
Timmiss, L. A., Martin, J. M., Murray, N. J., Welbergen, J. A., Westcott, D., McKeown, A., et al. (2021). Threatened but not conserved: Flying-fox roosting and foraging habitat in Australia. Aust. J. Zool. 68, 226–233. doi: 10.1071/ZO20086
Usenik, V., Kastelec, D., Veberiè, R., and Štampar, F. (2008). Quality changes during ripening of plums (Prunus domestica L.). Food Chem. 111, 830–836. doi: 10.1016/j.foodchem.2008.04.057
van der Ree, R., McDonnell, M. J., Temby, I., Nelson, J., and Whittingham, E. (2006). The establishment and dynamics of a recently established urban camp of flying foxes (Pteropus poliocephalus) outside their geographic range. J. Zool. 268, 177–185. doi: 10.1111/j.1469-7998.2005.00005.x
van Heezik, Y., Smyth, A., Adams, A., and Gordon, J. (2010). Do domestic cats impose an unsustainable harvest on urban bird populations? Biol. Conserv. 143, 121–130. doi: 10.1016/j.biocon.2009.09.013
Welbergen, J. A., Meade, J., Field, H. E., Edson, D., McMichael, L., Shoo, L. P., et al. (2020). Extreme mobility of the world’s largest flying mammals creates key challenges for management and conservation. BMC Biol. 18:101. doi: 10.1186/s12915-020-00829-w
Williams, N. S. G., McDonnell, M. J., Phelan, G. K., Keim, L. D., and van der Ree, R. (2006). Range expansion due to urbanization: Increased food resources attract grey-headed flying-foxes (Pteropus poliocephalus) to Melbourne. Austral Ecol. 31, 190–198. doi: 10.1111/j.1442-9993.2006.01590.x
Wilson, J. (2002). Flowering Ecology Of A Box-Ironbark Eucalyptus Community. Ph.D. thesis, Geelong: Deakin University.
Woinarski, J. C. Z., Burbidge, A. A., and Harrison, P. L. (2014). The action plan for Australian mammals 2012. Collingwood, VIC: CSIRO publishing. doi: 10.1071/9780643108745
Yabsley, S. H., Meade, J., Martin, J. M., and Welbergen, J. A. (2021). Human-modified landscapes provide key foraging areas for a threatened flying mammal: The grey-headed flying-fox. PLoS One 16:e0259395. doi: 10.1371/journal.pone.0259395
Yau, D. (1982). Street trees of Melbourne. Arboric. J. 6, 95–105. doi: 10.1080/03071375.1982.9746556
Keywords: bats (chiroptera), fruit bat, wildlife urbanization, feeding resource use, GPS tracking
Citation: Yabsley SH, Meade J, Hibburt TD, Martin JM, Boardman WSJ, Nicolle D, Walker MJ, Turbill C and Welbergen JA (2022) Variety is the spice of life: Flying-foxes exploit a variety of native and exotic food plants in an urban landscape mosaic. Front. Ecol. Evol. 10:907966. doi: 10.3389/fevo.2022.907966
Received: 30 March 2022; Accepted: 29 July 2022;
Published: 23 August 2022.
Edited by:
Ryan Jensen, Brigham Young University, United StatesReviewed by:
Tamika Lunn, University of Tasmania, AustraliaColleen T. Downs, University of KwaZulu-Natal, South Africa
Copyright © 2022 Yabsley, Meade, Hibburt, Martin, Boardman, Nicolle, Walker, Turbill and Welbergen. This is an open-access article distributed under the terms of the Creative Commons Attribution License (CC BY). The use, distribution or reproduction in other forums is permitted, provided the original author(s) and the copyright owner(s) are credited and that the original publication in this journal is cited, in accordance with accepted academic practice. No use, distribution or reproduction is permitted which does not comply with these terms.
*Correspondence: Samantha H. Yabsley, Uy5ZYWJzbGV5QHdlc3Rlcm5zeWRuZXkuZWR1LmF1