- 1Departamento de Entomologia, Universidade Federal de Viçosa, Viçosa, Brazil
- 2Departamento de Biologia Geral, Universidade Federal de Viçosa, Viçosa, Brazil
- 3Departamento de Biologia Animal, Universidade Federal de Viçosa, Viçosa, Brazil
The lack of consensus regarding pollinator decline in various parts of the planet has generated intense debates in different spheres. Consequently, much research has attempted to identify the leading causes of this decline, and a multifactorial synergism (i.e., different stressors acting together and mutually potentiating the harmful effects) seems to be the emerging consensus explaining this phenomenon. The emphasis on some stressor groups such as agrochemicals, and pollinators such as the honey bee Apis mellifera, can hide the real risk of anthropogenic stressors on pollinating insects. In the present study, we conducted a systematic review of the literature to identify general and temporal trends in publications, considering the different groups of pollinators and their exposure to agrochemicals over the last 76 years. Through an artificial intelligence (AI)-aided meta-analysis, we quantitatively assessed trends in publications on bee groups and agrochemicals. Using AI tools through machine learning enabled efficient evaluation of a large volume of published articles. Toxicological assessment of the impact of agrochemicals on insect pollinators is dominated by the order Hymenoptera, which includes honey bees. Although honey bees are well-explored, there is a lack of published articles exploring the toxicological assessment of agrochemicals for bumble bees, solitary bees, and stingless bees. The data gathered provide insights into the current scenario of the risk of pollinator decline imposed by agrochemicals and serve to guide further research in this area.
Systematic Review Registration: https://asreview.nl/.
Introduction
Insects are a major group of pollinators of flowering plants, which include flies (Diptera), moths (Lepidoptera), butterflies (Lepidoptera), beetles (Coleoptera), ants, wasps, and bees (Hymenoptera) (Ostiguy, 2011; Wardhaugh, 2015). Bees are the most important group of pollinating insects (Klein et al., 2006; Potts et al., 2016). Pollinator insects are vital for the maintenance of natural ecosystems, conservation of biodiversity, and food security. Insects, including bees, are undergoing a significant decline in their populations (Wagner et al., 2021). Approximately 40% of insect species are threatened, and the most affected orders include Lepidoptera, Hymenoptera, and Coleoptera (Sánchez-Bayo and Wyckhuys, 2019).
The decline of pollinators can impact 35% of global agricultural land, putting the world's major food crops at risk (FAO, 2018a). Climate change and anthropogenic factors, such as habitat loss and fragmentation, agricultural intensification and large-scale use of agrochemicals, environmental pollution, pathogens, and invasive alien species, have been identified as the main drivers of this decline in pollinators (IPBES, 2016).
Concerns regarding conservation of pollinators are unquestionable. Currently, there are global initiatives to protect pollination services in sustainable agriculture, such as monitoring the status of pollinator insects, implementation of risk assessments, systematization of information, and development of public conservation policies (IPBES, 2016; Potts et al., 2016; FAO, 2018a; EFSA, 2020). These initiatives provide valuable information for assessing the actual scale of risks to pollinators. Consequently, research can be directed toward filling the knowledge gaps (IPBES, 2016). This could potentially aid in designing management and conservation strategies for pollinators.
Bees comprise a large group of pollinators of flowering plants. Approximately 20,000 species of wild and managed bees contribute to the pollination of plants worldwide, exhibiting high efficiency in plant reproduction and improving crop yield (Garibaldi et al., 2013; Potts et al., 2016; FAO, 2018a). For instance, the genus Apis, which comprises 11 known species, has spread worldwide, and currently, different subspecies are pollinating on almost every continent (Crane, 2009). Some species of bees are managed, which are used as suppliers of pollination services to crops, and also provide other benefits or products of broad use, including honey, wax, propolis, and pollen (IPBES, 2016; Grüter, 2020).
Honey bee (Apis mellifera) is the most commonly managed and commercialized bee species in the world (Hung et al., 2018), followed to a lesser extent by some species of bumble bees (Bombus sp.), and some stingless bees and solitary bees (Grüter, 2020; Delaplane, 2021). Although there is growing evidence about the important role of wild pollinators in contributing to crop production and environmental health (Garibaldi et al., 2013), most studies have focused on honey bees and bumble bees because of their role in agriculture (Abati et al., 2021). In recent years, non-Apis species (excluding those of the Apini tribe) have also been prioritized in conservation policies because of their ecological importance, particularly in the Pan-Tropical region (Barbosa et al., 2015; IPBES, 2016; Pires et al., 2018; EFSA, 2020). However, information on the biology and management of non-Apis bees, compared to that on honeybees, is still lacking.
Approximately two million tons of agrochemicals are used globally per year, with almost 95% corresponding to herbicides (47.5%), insecticides (29.5%), and fungicides (17.5%) (Sharma et al., 2019); there are estimates that this use will increase to 3.5 million tons in a short period (Sarkar et al., 2021). Among these agrochemicals, insecticides, mainly neonicotinoids, are often considered one of the main factors driving the decline of bees (Hopwood et al., 2012; Abati et al., 2021; Siviter et al., 2021). Most studies have used honey bees as a suitable model for risk assessment given their controversial interaction with neonicotinoids, and this has resulted in a dearth of studies on other bee species and impacts of other agrochemical groups (Barbosa et al., 2015; Lima et al., 2016; Abati et al., 2021; Siviter et al., 2021).
Guidance for assessing the risks of agrochemicals in bees is based on the assessment of the toxic effects of these chemical compounds. Variables such as mortality (lethal effects) and behavioral changes (sublethal effects) are used as parameters to estimate the harmful effects of agrochemicals on colony health (EFSA, 2013; IPBES, 2016; Botina et al., 2020). Evidence indicates that non-Apis bees are more susceptible to insecticide exposure than honey bees (Arena and Sgolastra, 2014; Tomé et al., 2017), and that three main groups of agrochemicals (herbicides, insecticides, and fungicides) can have lethal and sublethal effects on bees (Tomé et al., 2017; Botina et al., 2020; Araújo et al., 2021).
There is a large volume of toxicological studies on pollinators. However, many of them are single-species studies, focusing on honey bees. Therefore, a meta-analysis can be a useful approach to identify knowledge gaps and direct future research efforts. Performing a meta-analysis can be a challenging task as it entails intensive efforts to systematically review published studies. However, with artificial intelligence (AI) tools, researchers can now interactively apply natural language processing and machine learning (ML) models, thereby reducing the effort involved in systematic review (Marshall and Wallace, 2019). In interactive ML procedures, the researcher interacts with an ML model in a loop and based on the researcher's decisions (training data set with relevant vs. irrelevant studies), the model updates its predictions for the remaining dataset, selecting the next study that is presented to the researcher. The researcher then screens this study and provides a label (i.e., relevant or irrelevant). The newly labeled study is added to the training dataset, and another round is iterated (Van de Schoot et al., 2021a). Thus, interactive ML minimizes the number of studies to be screened by the researcher by prioritizing articles that are most likely to be relevant.
In the present study, we conducted an AI-aided systematic review of the literature to recognize general and temporal trends in publications on different groups of insect pollinators and their agrochemical exposure over the last 76 years. Initially, we searched two databases to identify published studies with pollinating insects in general, and a qualitative analysis was performed to identify the main groups of pollinators and agrochemicals studied. Through a meta-analysis, we quantitatively assessed trends in publications on the main pollinator groups (bees from the tribes Apini, Bombini, and Meliponini) and agrochemicals (insecticides). This allowed us to systematically analyze the state of agrochemical risk assessments for bees and to detect any relevant knowledge gaps.
Methods
We followed the guidelines outlined in the “Preferred Reporting Items for Systematic Reviews and Meta-Analyses” (PRISMA) (Moher et al., 2009) for the systematic literature review, which included these stages: identification, screening, eligibility, and inclusion. The following subsections provide further details of the literature review and data analyses.
Identification
To identify published studies on toxicological assessment of agrochemicals in insect pollinators, we searched the databases of Scopus (Elsevier) and Web of Science (main collection, Clarivate Analytics) for the years 1945–2021. We screened the title, abstract, or keywords of the manuscript for the search words. The search heuristic was defined as: (pollinator* OR insect*) AND (pesticide* OR insecticide* OR agrochemical* OR “agricultural protectant*” OR “phytosanitary product*” OR biopesticide* OR bioinsecticide* OR “botanical pesticide*” OR “botanical insecticide*” OR gmo OR “bt crop*” OR “crop protection” OR “bt toxin*” OR “cry toxin*” OR “proteinase inhibitor*” OR “transgenic plant”). Studies that were found in duplicate from the databases were manually removed based on title matching.
Screening
We applied text classification based on interactive ML to title and abstract screening. Accordingly, we constructed a training dataset by labeling studies to build our model. The training dataset consisted of 86 irrelevant papers and 79 relevant papers. The title and abstracts of the articles were processed (converted to lowercase and no stop words were removed), and translated into matrices through the term frequency-inverse document frequency method (Ramos, 2003). We used the naïve Bayes algorithm, which is known to perform very well for text (Schneider, 2005), to compute the relevance scores of the published articles. We stopped reviewing after we found 150 non-relevant published articles in succession during the learning cycle, and the output of the process included 1,050 relevant published articles. These AI-based screening procedures were performed using the open-source tool ASReview (Van de Schoot et al., 2021b).
Eligibility and Inclusion
The full-text of the articles selected after the initial screening was assessed for eligibility. We selected peer-reviewed articles, written in English, reporting the possible effects of agrochemicals (active ingredient alone or formulations) on insect pollinators, describing the response variable evaluated, and delineating a rigorous study design with controls. Books, book chapters, conference papers, thesis, dissertations, and reviews were excluded. From the articles selected after evaluation of the full-text, we systematically extracted the information for qualitative analysis based on the defined topics (Supplementary Table S1 is available on http://rpubs.com/bernardesrc/851826). Finally, based on the qualitative analysis, we considered only those articles for the meta-analyses that assessed insect pollinators from the tribes Apini, Bombini, and Meliponini.
Statistical Analyses
We performed meta-analyses on studies that assessed the toxicological effects of insecticides as the main group of agrochemicals on bees (Apini, Bombini, and Meliponini) as the main group of pollinators. We considered randomized controlled bioassays (i.e., a single study assessing different insecticides or pollinator groups in independent trials) as a binary outcome. Therefore, the overall likelihood of studies covering some of the bee groups was determined through risk ratio (RR) and 95% confidence intervals (CIs). These estimates were based on mixed-effect models with inverse-variance and the DerSimonian–Laird method. We measured between-study heterogeneity (τ2), and performed a heterogeneity test based on total heterogeneity (I2; p < 0.05) (Schwarzer et al., 2015). Subgroup analyses were performed to explain the statistical heterogeneity, considering insecticide groups and type of response assessed as moderators. All analyses were performed using the packages meta (Balduzzi et al., 2019) and stats in R software (version 4.1.1) (R Core Team, 2021).
Results
Literature Review
We found 62,715 published articles in the databases, and after removing duplicates, we identified 62,560 articles (Figure 1). Finally, 784 papers were selected that included data from independent bioassays (Supplementary Table S2 is available on http://rpubs.com/bernardesrc/851826).
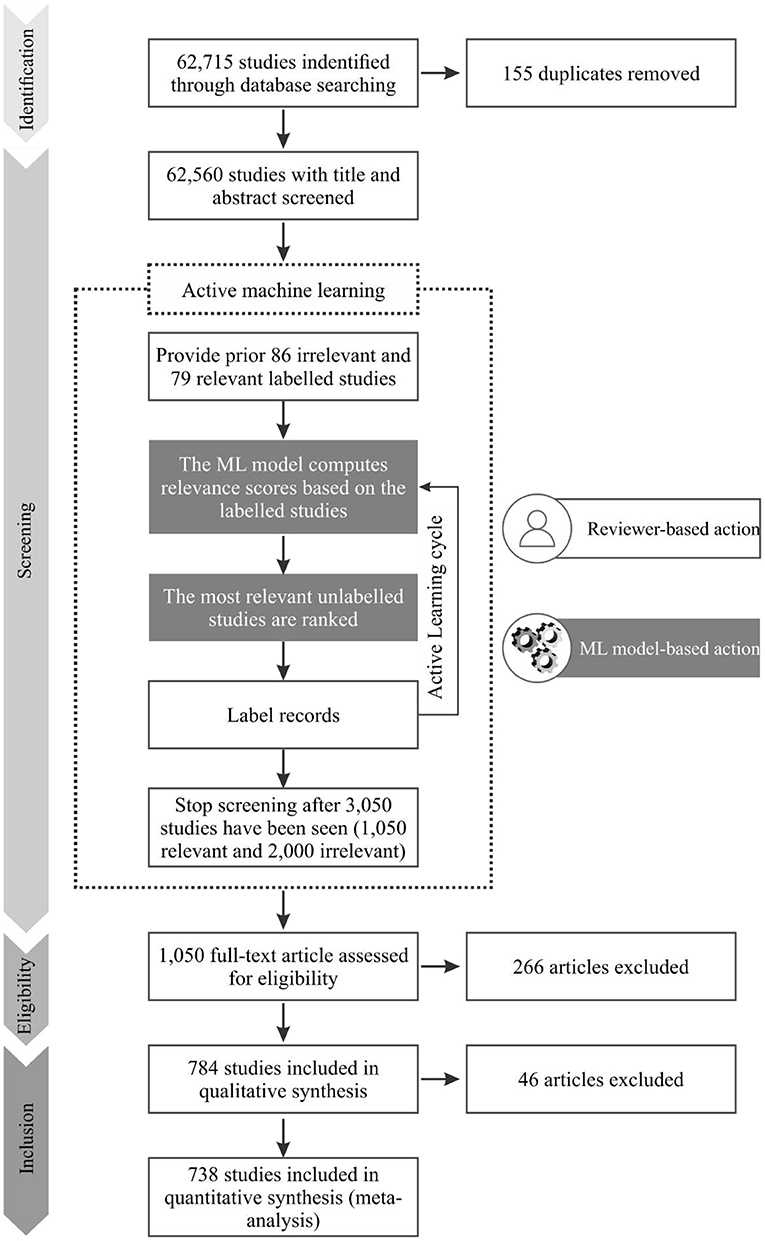
Figure 1. Flowchart depicting the stages (identification, screening, eligibility, and inclusion) of systematic literature review on the toxicological assessment of the impact of agrochemicals on insect pollinators. In the identification stage, duplicates were recognized through the paper titles. The screening of titles and abstracts was performed with active machine learning (ML), in which 165 labeled studies (86 irrelevant and 79 relevant) were included in the ML model. Then, several active learning cycles were performed until 150 irrelevant studies were found in sequence (i.e., 3,050 studies were detected and 1,050 of the most relevant studies were selected). In the eligibility stage, articles (n = 266) that did not report agrochemical tests with any insect pollinator and/or did not describe the response evaluated in pollinators were excluded. In the inclusion stage, articles that did not assess at least one of the main identified bee groups (honey bees, bumble bees, and stingless bees) were excluded.
Qualitative Results
Most of the studies focused on the order Hymenoptera (Figure 2A). There were only nine and five bioassays for Lepidoptera and Diptera, respectively, representing 0.6% of the studies. Within Hymenoptera (2,299 independent bioassays; 99.40%), the main family was Apidae and some representatives of Megachilidae (Figure 2A). The main pollinator species group covered was honey bees (Apini), followed by bumble bees (Bombini) and stingless bees (Meliponini), all of which belong to the Apidae family (Figure 2A). Therefore, these three groups of bees were included in subsequent meta-analyses.
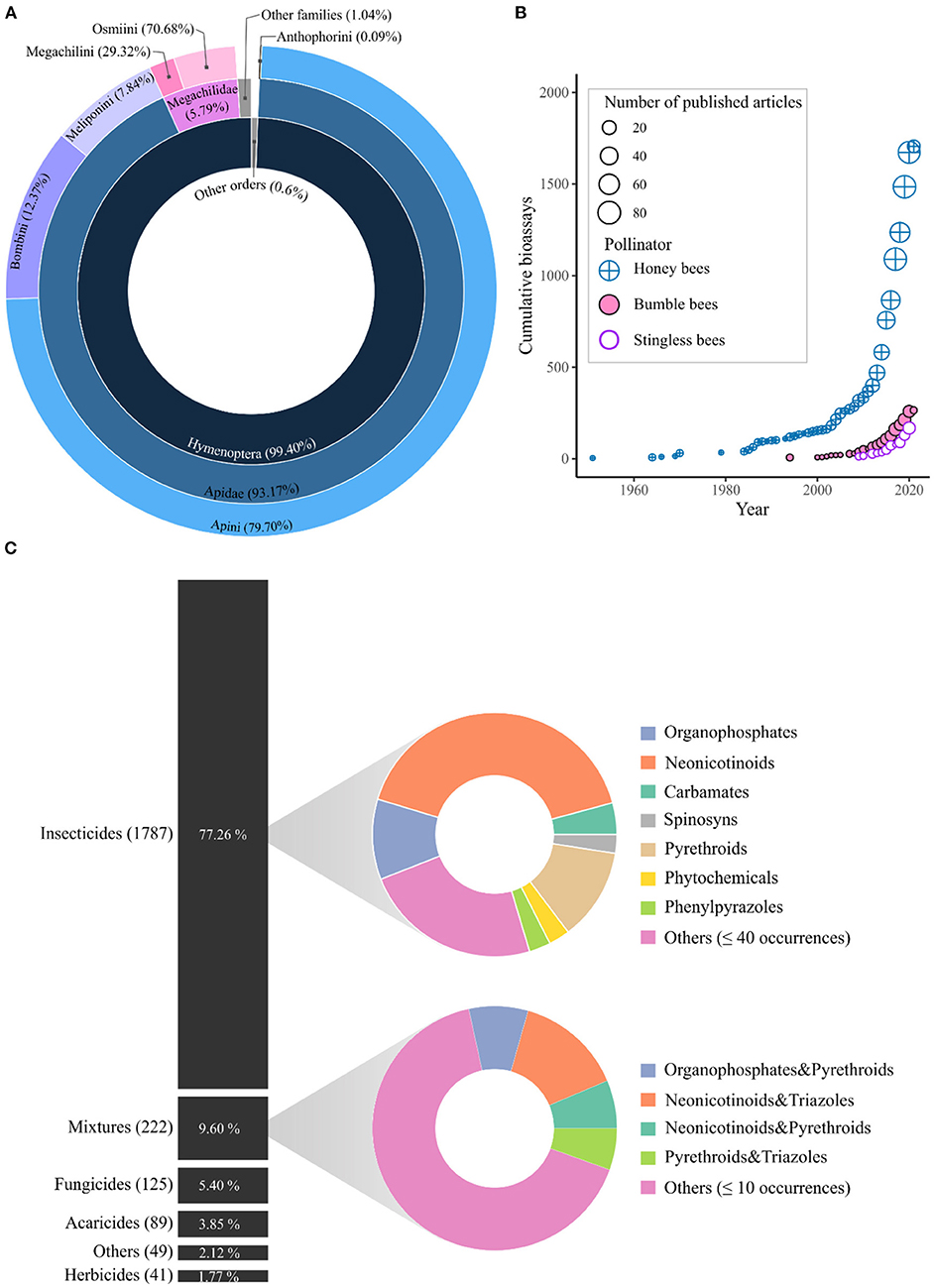
Figure 2. Overall trends in publications on toxicological assessment of agrochemicals for insect pollinators. (A) Distribution of bioassays according to pollinator groups; the circle in the center represents the root node, with the hierarchy moving outward from the center. (B) Cumulative independent bioassays (i.e., a single study assessing a different agrochemical stressor or pollinator in independent trials) over time exhibiting the number of published articles per year and the main groups of pollinators (bees). (C) Agrochemical groups evaluated in the published articles, detailing the insecticides and mixtures (i.e., mix of two or more agrochemical groups) comprising the main groups. Agrochemicals, insecticides, and mixtures with occurrences ≤ 40, ≤ 40, and ≤10 were grouped as “others,” respectively.
There was an impressive increase in research output after the 2000s, with 28.1 ± 5.6 (mean ± standard error) independent bioassays from 10 ± 2 articles published per year (Figure 2B). Insecticides were the most tested group among agrochemicals, accounting for 1,787 bioassays (77.26%) (Figure 2C). The most predominant insecticide group was neonicotinoids (41.13%), followed by pyrethroids (12.26%), organophosphates (10.63%), carbamates (4.2%), phenylpyrazoles (2.85%), phytochemicals (2.80%), and spinosyns (2.46%) (Figure 2C). Insecticide mixtures were tested in 9.60% of the trials, and mixtures of neonicotinoids and triazoles were the most tested (Figure 2C).
Of these studies that assessed the main groups of bees and insecticides, only 7.52% assessed the interaction with other stressors, and 6.37% of these studies were on honeybees, 1.07% on bumble bees, and 0.08% on stingless bees (Figure 3). Moreover, most of these studies tested the association with neonicotinoids (5.37%) (Figure 3).
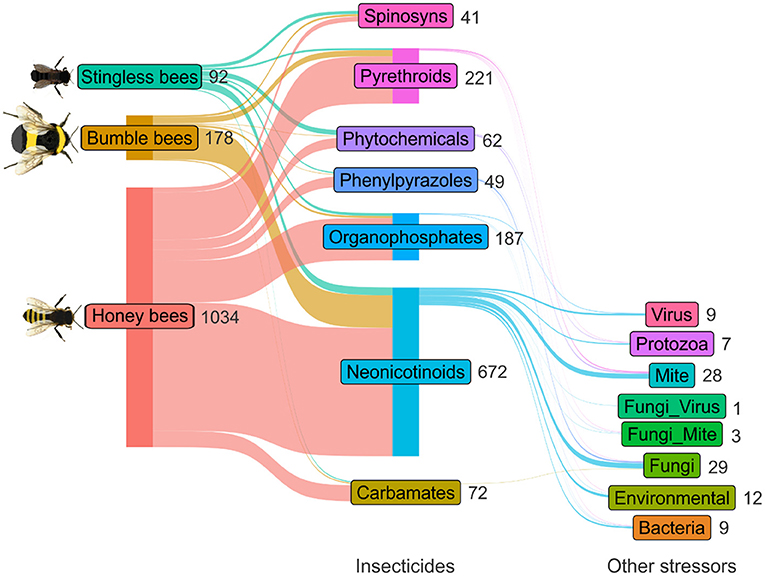
Figure 3. Interaction flows among insecticides and other stressors that were tested in the main groups of bees found in the literature review (n = 1,304) of toxicological assessment of the impact of agrochemicals on insect pollinators. The values on the right side of each node indicate the number of trials. The thickness of the nodes (rectangles) and arcs (links going from one node to another) is proportional to the frequency of interactions. Terms separated by dash indicate that both terms were assessed.
Most studies were conducted under laboratory conditions (77.33%) and involved oral application (58.53%). The studies conducted in the field (n = 266; 12.44%) were almost exclusively with honey bees (n = 211; 79.32%). In addition, acute exposure occurred in 64.38% and chronic exposure in 26.6% (Figure 4). In acute exposure, 49.7% were through oral application, 15.4% contact, and 14.5% topical application. In chronic exposure, 85.8% were through oral application, 9.3% contact, and 1.6% topical application (Figure 4).
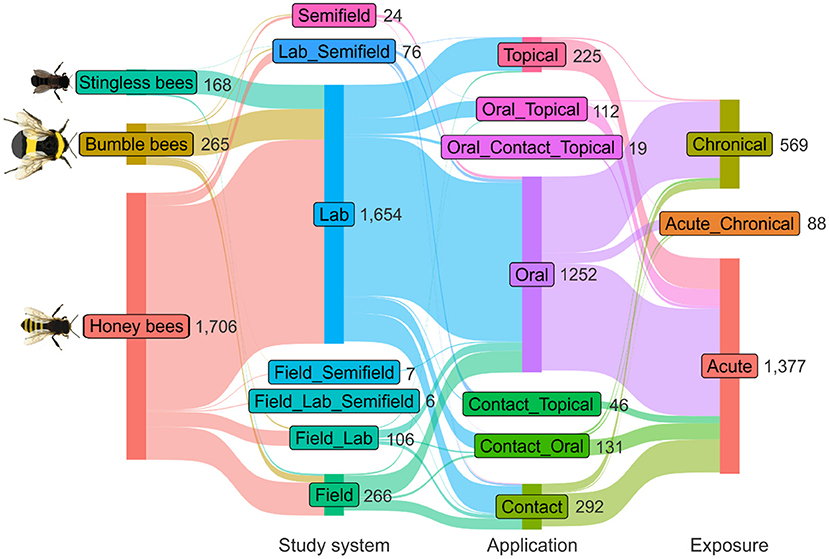
Figure 4. Interaction flows among the study systems, applications, and exposures that were assessed in the main groups of bees found in the literature review (n = 2,139) on toxicological assessment of agrochemicals for insect pollinators. The values on the right side of each node indicate the number of trials. The thickness of the nodes (rectangles) and arcs (links going from one node to another) is proportional to the frequency of interactions. Terms separated by dash indicate that both terms were assessed.
The studies mainly assessed adult workers. In addition, some studies assessed whole colonies (9.4%), and 78.1% of the colony studies included honey bees (Figure 5).
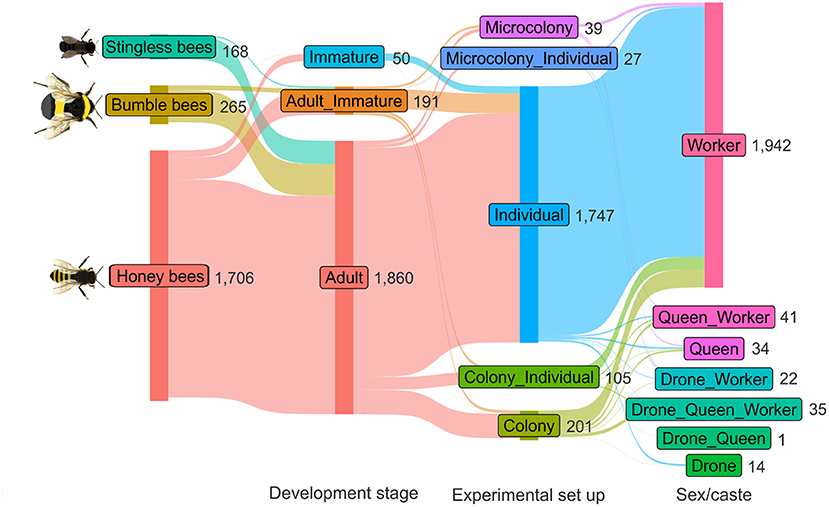
Figure 5. Interaction flows among the development stages, experimental set ups (the level of social organization of the pollinators in which the study was performed), and sex/castes that were assessed in the main groups of bees found in the literature review (n = 2,139) of toxicological assessment of the impact of agrochemicals on insect pollinators. The values on the right side of each node indicate the number of trials. The thickness of the nodes (rectangles) and arcs (links going from one node to another) is proportional to the frequency of interactions. Terms separated by a dash indicates that both terms were assessed. Microcolony: a queenless colony, initially established with few newly emerged workers.
Quantitative Results
Meta-analyses with the most studied insecticides showed a high likelihood of published articles covering honey bees, wherein the RR was significantly higher than that expected by the null hypothesis (RR = 3.81, 95% CI = 2.29–6.34, z = 5.15, p < 0.001). There was a nearly 10 times lower probability of published articles addressing bumble bees (RR = 0.10, 95% CI = 0.05–0.17, z = −7.69, p < 0.001) and stingless bees (RR = 0.11, 95% CI = 0.04–0.27, z = −4.77, p < 0.001) (Figure 6). Due to the high heterogeneity among insecticide groups for all bee groups (honey bees: I2 = 93%, τ2 = 0.42; bumble bees: I2 = 88%, τ2 = 0.48; stingless bees: I2 = 95%, τ2 = 1.4), insecticides were included as moderators in the mixed-effect models, and there was a significant difference among insecticide groups for all bees (honey bees: χ26 = 83.51, p < 0.001; bumble bees: χ26 = 48.23, p < 0.001; stingless bees: χ26 = 119.3, p < 0.001). In this scenario, synthetic insecticides (i.e., carbamates, neonicotinoids, organophosphates, phenylpyrazoles, and pyrethroids) were the most tested in honey bees and bumble bees, whereas biopesticides (e.g., phytochemicals and spinosyns) were the most tested in stingless bees (Figure 6).
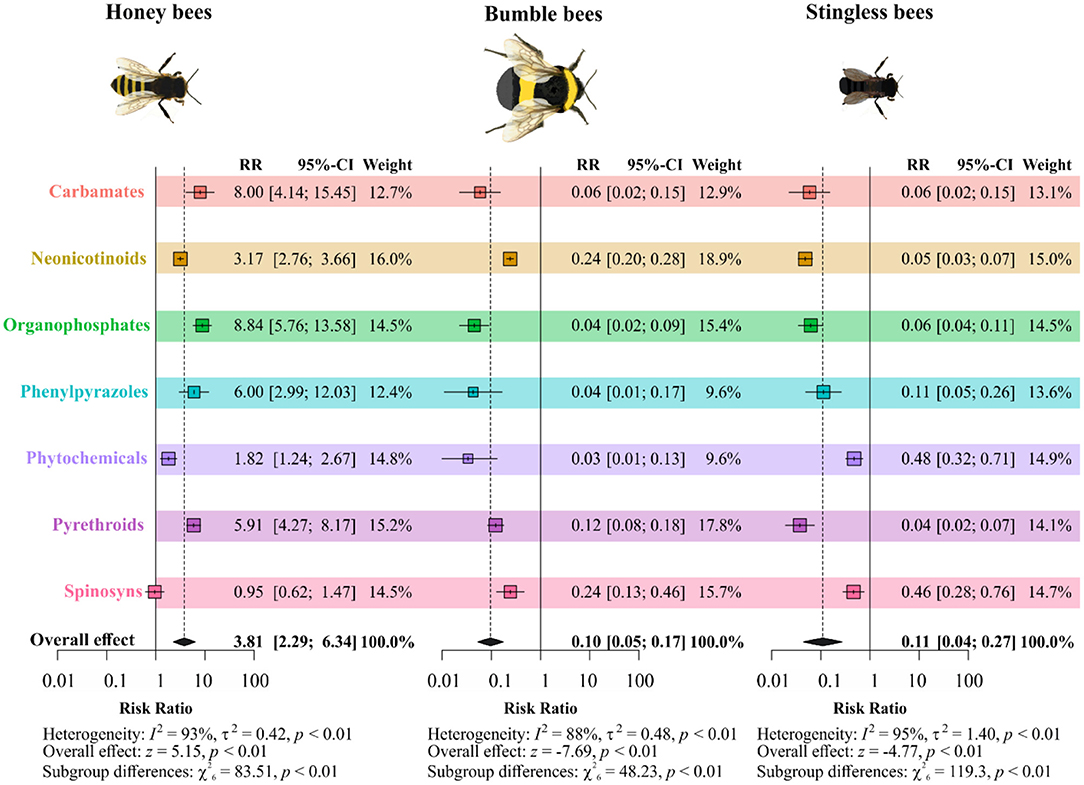
Figure 6. Estimated size effect in the meta-analysis of the occurrence of published articles on toxicological assessment of the impact of insecticides on bees from the main groups found in the literature review. The forest plots depict the estimated risk ratio (RR), 95% confidence interval (CI), and weight (inverse of the variance; larger squares indicate more precise results, i.e., small variances). The black diamond shape indicates the summary of RR ± 95% CI (overall effect for all insecticides). These estimates are based on mixed-effect model meta-analyses with inverse-variance and DerSimonian–Laird methods. RR ± 95% CI > 1 indicates a high likelihood of published articles covering the effects of agrochemicals in the bee group, while RR ± 95% CI < 1 indicates a low likelihood, and no bias is present when RR ± 95% CI includes 1.
The results of the meta-analyses considering the type of response assessed as moderators demonstrated that honey bees were well-explored (RR = 3.67, 95% CI = 2.48–5.43, z = 6.50, p < 0.001). In contrast, there is a lack of published articles exploring these responses for both bumble bees (RR = 0.16, 95% CI = 0.10–0.27, z = 7.27, p < 0.001) and stingless bees (RR = 0.07, 95% CI = 0.04–0.11, z = 11.97, p < 0.001) (Figure 7). Heterogeneity was high (honey bees: I2 = 97%, τ2 = 0.46; bumble bees: I2 = 97%, τ2 = 0.71; stingless bees: I2 = 90%, τ2 = 0.43), and the subgroup test showed a significant difference in the type of response assessed (honey bees: χ212 = 388.9, p < 0.001; bumble bees: χ212 = 392.9, p < 0.001; stingless bees: χ212 = 125.1, p < 0.001). Survival and behavioral responses were more prevalent in the research, as they presented the highest weights, indicating high representativeness and low variability. In contrast, the omics and microbiota responses presented the lowest weights, indicating little prevalence in the research. Likewise, gene expression in stingless bees also exhibited very little weight (Figure 7).
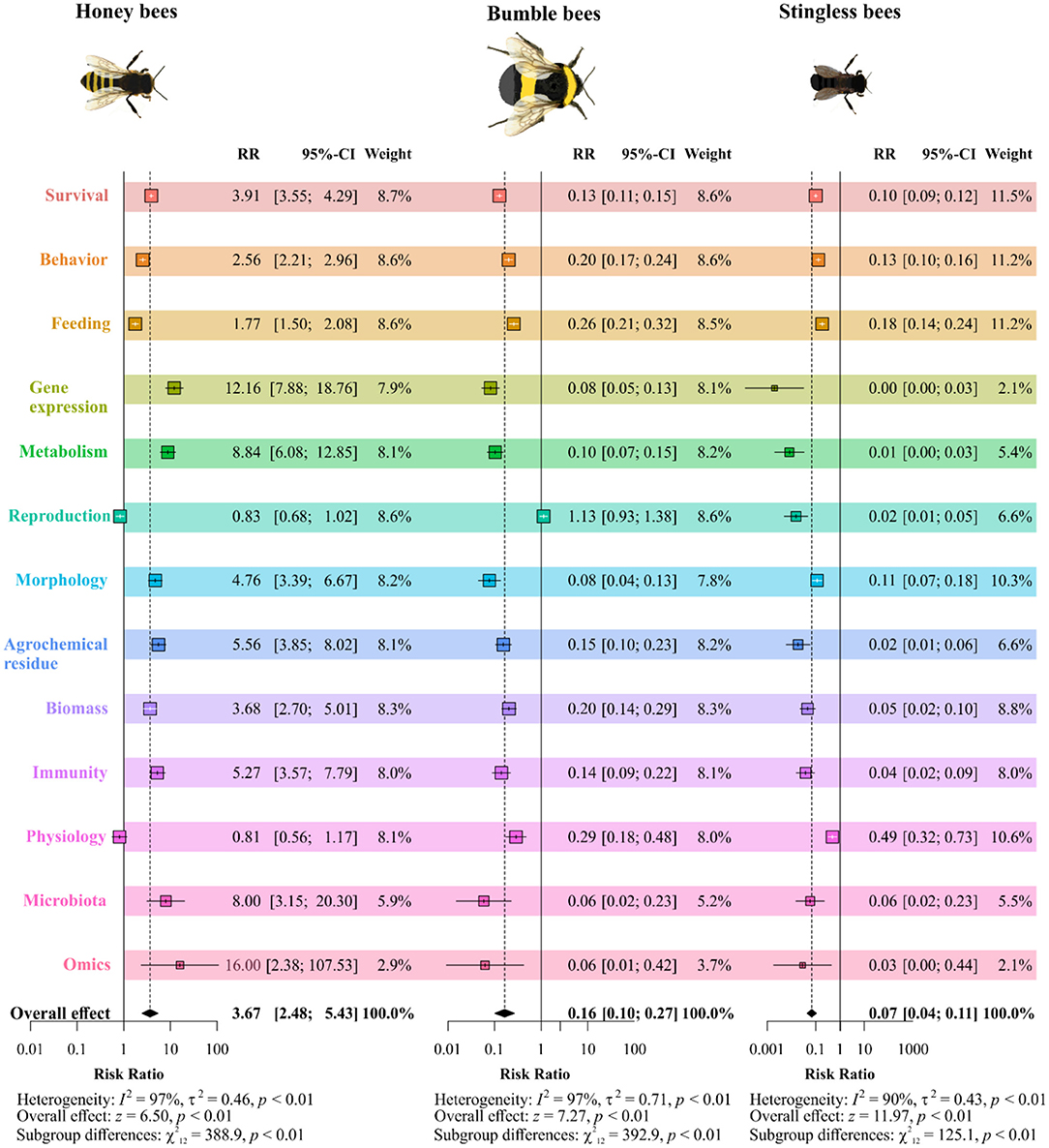
Figure 7. Estimated effect sizes in the meta-analysis of the occurrence of published articles covering the type of response assessed from the main groups found in the literature review of toxicological assessment of the impact of agrochemicals on bees. The forest plots depict the estimated risk ratio (RR), 95% confidence interval (CI), and weight (inverse of the variance; larger squares indicate more precise results, i.e., small variances). The black diamond shape indicates the summary of RR ± 95% CI (overall effect for all types of responses). These estimates are based on mixed-effect model meta-analyses with inverse-variance and DerSimonian–Laird methods. RR ± 95% CI > 1 indicates a high likelihood of published articles covering the effects in some response, RR ± 95% CI < 1 indicates a low likelihood, and no bias are present when RR ± 95% CI includes 1.
Discussion
Most toxicological assessments of the effects of agrochemicals on insect pollinators included the Hymenoptera clade, especially the family Apidae. After the first report of massive honey bee colony losses occurring due to the phenomenon called “Colony Collapse Disorder,” the scientific community joined efforts to identify and understand the factors that caused this phenomenon, which remain under investigation (VanEngelsdorp et al., 2009; IPBES, 2016). Not surprisingly, protocols for risk assessment in pollinators are focused on bees, mainly honey bees, and protocols for other groups of pollinators are uncommon (OECD, 1998a,b; EFSA, 2013). The testing guidelines provided by these agencies for the development of toxicity tests and risk analysis for agrochemical exposure are standardized for honey bees, and recently, some of them included guidelines for Bombus sp. and solitary bees (IPBES, 2016; EFSA, 2020). Among the pollinators, honey bees and bumble bees have a higher commercial value in crop pollination programs, as they have the advantage of being generalist pollinators (Goulson, 2010; Hung et al., 2018). Therefore, there is a greater investment in these studies due to the influence of farmers and beekeepers (IPBES, 2016; Abati et al., 2021). This is revealed by the number of publications on each group of non-Apis pollinators, which reflects the lack of data to assess the actual risks that these pollinators face when exposed to agrochemicals.
One of the main factors identified as causing (directly and indirectly) the decline of bee colonies is the exposure to agrochemicals, widely used in plant protection, during foraging (Johnson, 2015; Lima et al., 2016). Accordingly, it is not surprising that many pesticide toxicity studies, particularly those on insecticides, have focused on control-targeted and non-target insects, such as pollinators (Brittain and Potts, 2011). Concerns regarding the effects of insecticides on bees are evident in the high number of studies on these agrochemicals. These studies have demonstrated the high toxicity of insecticides to bees. Likewise, the few studies carried out with stingless bees showed higher susceptibility to insecticides than that of honey bees (Arena and Sgolastra, 2014; Tomé et al., 2017).
The number of papers on stingless bees has increased in the last 12 years. This group is predominant in the Neotropical region (77% of the species), followed by the Indo-Malay/Australasian region (16% of the species) and the Afrotropics (7% of the species). In countries such as Brazil, Colombia, Mexico, and Costa Rica, stingless bees represent ~50–70% of all flower visitations by bees (Grüter, 2020). Despite this, toxicological studies on stingless bees are incipient compared to those on honey bees, and there are reports that stingless bees may be more susceptible to exposure to agrochemicals than honey bees (Lima et al., 2016; Tomé et al., 2017). In addition, the Brazilian Institute of Environment and Renewable Natural Resources includes stingless bees in risk assessments, and some protocol proposals have been developed to diagnose the impact of agrochemicals on these bees and consequently on their ecosystem services, which are invaluable in both natural and agricultural ecosystems (Barbosa et al., 2015; Lima et al., 2016; Pires et al., 2018; Botina et al., 2020; Grüter, 2020). Our meta-analyses demonstrated that neonicotinoids, phytochemicals, and spinosyns were the agrochemicals most tested on stingless bees, and that the most assessed toxicological responses were survival, behavior, and feeding (Figures 6, 7). However, there are many gaps in our knowledge about the toxicological effects of agrochemicals on stingless bees, especially the effects of other synthetic insecticides and their impact on gene expression, omics, metabolism, and microbiota.
Omics and microbiota were the least studied parameters in honey bees and bumble bees. Considering that omics studies may reveal responsive biomarkers (i.e., genes, metabolites, and proteins) that potentially cause homeostatic changes (Goh et al., 2021) and that gut microbiota is associated with insect nutritional health, immunocompetence, and neutralization of damage caused by xenobiotics and pathogens (Nogrado et al., 2019; Giambò et al., 2021), further research including these two parameters could significantly contribute to our understanding of the metabolic changes behind the decline of bees.
As in the case of stingless bees, there are few published articles on the toxicological assessment of the effects of agrochemicals on solitary bees. Their role in pollination is being studied in specific crops in the United States and Europe. As a consequence, their role as important wild pollinators has been recognized in recent years (Peterson and Artz, 2013; Woodcock et al., 2013). In 2013, solitary bees were included in the risk assessment protocols proposed by the European Food Safety Authority (EFSA, 2013). Given this scenario, it is expected that studies will be carried out in other parts of the world and also include non-Apis species.
The results showed that neonicotinoid insecticides are the chemical group of pesticides receiving the most attention in toxicological assessments (Figure 6). Neonicotinoids are widely used globally. They are characterized by their rapid absorption and translocation within the plant and are found in both pollen and nectar, which are food for pollinators and a direct contamination source (Hopwood et al., 2012, 2018; Van Der Sluijs et al., 2015). Their exposure exhibits high lethality and triggers sublethal effects on bees (Lundin et al., 2015; Bernardes et al., 2022). However, it should be noted that exposure to a product with a relatively high toxicity, and thus hazard, does not necessarily result in a high risk if the level of exposure is low. In contrast, high exposure to agrochemicals with low toxicity may result in adverse effects on bees. This issue has been recognized by the Intergovernmental Science-Policy Platform on Biodiversity and Ecosystem Services. It provides an opportunity for governments, organizations, civil society, and concerned citizens everywhere to promote actions that will protect and enhance pollinators and their habitats, improve their abundance and diversity, and support the sustainable development of beekeeping (FAO, 2018b).
The most studied neonicotinoids were imidacloprid, thiamethoxam, and their metabolites, together with clothianidin (used alone, but also a thiamethoxan metabolite). This does not preclude the possibility that other agrochemicals could influence bee health, nor should it be presumed that the regulation of neonicotinoids alone is sufficient. Concern about the negative effects of these insecticides on bees should lead to research in assessing compounds in mixtures with other insecticides and in combination with other biotic stressors, such as viruses, fungi, and bacteria. In addition, our findings demonstrate that there are few reports on the insecticides phenylpyrazoles and phytochemicals in bumble bees and phenylpyrazoles and carbamates in honey bees and stingless bees.
The data obtained here indicate that, besides insecticides, other agrochemicals, including fungicides, acaricides, and herbicides, were also targets of attention and concern. Insects are not targets of these compounds, but there is evidence that bee exposure to these agrochemicals can lead to lethal and sublethal effects, potentially compromising colony success (Lima et al., 2016; Botina et al., 2020; Tomé et al., 2020). Therefore, it is crucial to include these compounds, and other newly developed chemicals aimed at crop protection, in risk assessments for honey bees as well as non-Apis bees.
Most published articles have conducted tests under laboratory conditions, rather than in the field. There are commonly accepted methods for assessing the toxicity of agrochemicals in bees under laboratory conditions (Medrzycki et al., 2013), and at least three key dosage factors (concentration, duration, and choice) are relevant to field conditions; however, these factors have been overestimated in many laboratory-based studies (Carreck and Ratnieks, 2014). Additionally, most of the published articles indicated assessment of individual adults, though most species of bees are social. Therefore, these findings highlight the need for future research to develop tests or experimental protocols capable of establishing new approaches that consider the sociability of bees and field conditions.
The meta-analysis detected a discrepancy in the number of articles published with adult individuals, mostly workers, oral application, and acute exposure in relation to those with immature individuals, other sex/castes, contact or topical application, and chronic exposure. It is evident that testing with adult workers is much easier than testing with immature individuals, males, or queens, because of their relative abundance and ease of collection. However, understanding the effects of agrochemicals on all sex/castes and stages of development is imperative to fully understand the implications of these compounds on colony life. Finally, methods with contact/topical applications and chronic exposure should receive special attention in bee agrochemical investigations.
Conclusions
Using AI tools through ML allowed us to efficiently assess a large volume of published articles, providing a more complete picture of the status of toxicological assessments of agrochemicals for insect pollinators. This systematic review recognized a primary focus on the order Hymenoptera, mainly bees of the family Apidae, highlighting gaps in other pollinating insects and even within the same family, such as bumble bees and stingless bees. The most studied agrochemical group comprised insecticides, especially neonicotinoids. These studies were primarily conducted in laboratory settings, focusing on acute oral exposure. Furthermore, the pattern of the quantified response variable in the studies was quite heterogeneous, with most studies quantifying survival and behavior as response variables. The data from this work gather valuable information on the current scenario of these toxicological analyses and can guide further research in this area.
Data Availability Statement
The datasets generated/analyzed for this study can be found in the rpubs repository (http://rpubs.com/bernardesrc/851826).
Author Contributions
RB: conceptualization, data curation, formal analysis, investigation, methodology, software, validation, visualization, writing—original draft, and writing—review and editing. LB and RA: conceptualization, data curation, investigation, methodology, writing—original draft, and writing—review and editing. RG and ML: conceptualization, supervision, and writing—review and editing. GM: conceptualization, funding, supervision, writing—original draft, and writing—review and editing. All authors contributed to the article and approved the submitted version.
Funding
This research was supported by Conselho Nacional de Desenvolvimento Científico e Tecnológico (CNPq, 142206/2017-2, and 301725/2019-5), Coordenação de Aperfeiçoamento de Pessoal de Nível Superior, Brasil (CAPES, Finance Code 001), and Fundação de Amparo à Pesquisa do Estado de Minas Gerais (FAPEMIG and CBB-APQ-00247-14).
Conflict of Interest
The authors declare that the research was conducted in the absence of any commercial or financial relationships that could be construed as a potential conflict of interest.
Publisher's Note
All claims expressed in this article are solely those of the authors and do not necessarily represent those of their affiliated organizations, or those of the publisher, the editors and the reviewers. Any product that may be evaluated in this article, or claim that may be made by its manufacturer, is not guaranteed or endorsed by the publisher.
Acknowledgments
We would like to thank Editage (www.editage.com) for English language editing.
References
Abati, R., Sampaio, A. R., Maciel, R. M. A., Colombo, F. C., Libardoni, G., Battisti, L., et al. (2021). Bees and pesticides: the research impact and scientometrics relations. Environ. Sci. Pollut. Res. 28, 32282–32298. doi: 10.1007/s11356-021-14224-7
Araújo, R., dos, S., Bernardes, R. C., and Martins, G. F. (2021). A mixture containing the herbicides Mesotrione and Atrazine imposes toxicological risks on workers of Partamona helleri. Sci. Total Environ. 763, 142980. doi: 10.1016/j.scitotenv.2020.142980
Arena, M., and Sgolastra, F. (2014). A meta-analysis comparing the sensitivity of bees to pesticides. Ecotoxicology 23, 324–334. doi: 10.1007/s10646-014-1190-1
Balduzzi, S., Rücker, G., and Schwarzer, G. (2019). How to perform a meta-analysis with R: a practical tutorial. Evid. Based Ment. Heal. 22, 153–160. doi: 10.1136/ebmental-2019-300117
Barbosa, W. F., Smagghe, G., and Guedes, R. N. C. (2015). Pesticides and reduced-risk insecticides, native bees and pantropical stingless bees: pitfalls and perspectives. Pest Manag Sci. 71:1049–1053. doi: 10.1002/ps.4025
Bernardes, R. C., Botina, L. L., da Silva, F. P., Fernandes, K. M., Lima, M. A. P., and Martins, G. F. (2022). Toxicological assessment of agrochemicals on bees using machine learning tools. J. Hazard. Mater. 424, 127344. doi: 10.1016/j.jhazmat.2021.127344
Botina, L. L., Bernardes, R. C., Barbosa, W. F., Lima, M. A. P., Guedes, R. N. C., and Martins, G. F. (2020). Toxicological assessments of agrochemical effects on stingless bees (Apidae, Meliponini). MethodsX 7, 100906. doi: 10.1016/j.mex.2020.100906
Brittain, C., and Potts, S. G. (2011). The potential impacts of insecticides on the life-history traits of bees and the consequences for pollination. Basic Appl. Ecol. 12, 321–331. doi: 10.1016/j.baae.2010.12.004
Carreck, N. L., and Ratnieks, F. L. W. (2014). The dose makes the poison: have “field realistic” rates of exposure of bees to neonicotinoid insecticides been overestimated in laboratory studies? J. Apic. Res. 53, 607–614. doi: 10.3896/IBRA.1.53.5.08
Crane, E. (2009). “Chapter 9 - apis species: (Honey Bees),” in Encyclopedia of Insects, 2nd Edn., eds V. H. Resh and R. T. Cardé (San Diego: Academic Press), 31–32. doi: 10.1016/B978-0-12-374144-8.00009-6
Delaplane, K. S. (2021). Crop Pollination by Bees, Volume 1: Evolution, Ecology, Conservation, and Management. Wallingford: CABI. doi: 10.1079/9781786393494.0001
EFSA (2013). Guidance on the risk assessment of plant protection products on bees (Apis mellifera, Bombus spp. and solitary bees). EFSAJ. 11, 3295. doi: 10.2903/j.efsa.2013.3295
EFSA (2020). Review of the evidence on bee background mortality. EFSA Support. Publ. 17, 1880E. doi: 10.2903/sp.efsa.2020.EN-1880
FAO (2018a). The Pollination of Cultivated Plants: A Compendium for Practitioners - Vol. 1. Available online at: www.fao.org/ (accessed October 8, 2021).
FAO (2018b). Why Bees Matter. #16. Available online at: http://www.fao.org/documents/card/en/c/i9527en (accessed October 8, 2021).
Garibaldi, L. A., Steffan-Dewenter, I., Winfree, R., Aizen, M. A., Bommarco, R., Cunningham, S. A., et al. (2013). Wild pollinators enhance fruit set of crops regardless of honey bee abundance. Science (80-.). 340, 1608–1611. doi: 10.1126/science.1230200
Giambò, F., Teodoro, M., Costa, C., and Fenga, C. (2021). Toxicology and microbiota: how do pesticides influence gut microbiota? A review. Int. J. Environ. Res. Public Health 18, 5510. doi: 10.3390/ijerph18115510
Goh, M. S., Lam, S. D., Yang, Y., Naqiuddin, M., Addis, S. N. K., Yong, W. T. L., et al. (2021). Omics technologies used in pesticide residue detection and mitigation in crop. J. Hazard. Mater. 420, 126624. doi: 10.1016/j.jhazmat.2021.126624
Goulson, D. (2010). Bumblebees : Behaviour, Ecology, and Conservation. Available online at: https://books.google.com/books/about/Bumblebees.html?hl=pt-BR&id=F6wVDAAAQBAJ (accessed November 23, 2021).
Grüter, C. (2020). Stingless Bees. Cham: Springer International Publishing. doi: 10.1007/978-3-030-60090-7
Hopwood, J., Code, A., Vaughan, M., Biddinger, D., and Sheperd, M. (2018). How Neonicotinoids Can Kill Bees. Available online at: www.xerces.org (accessed November 22, 2021).
Hopwood, J., Vaughan, M., Matthew, S., Biddinger, D., Eric, M., Hoffman, S., et al. (2012). Are Neonicotinoids Killing Bees? A Review of Research Into the Effects of Neonicotinoid Insecticides on Bees, With Recommendations for Action. Available online at: www.xerces.org (accessed December 3, 2021).
Hung, K. L. J., Kingston, J. M., Albrecht, M., Holway, D. A., and Kohn, J. R. (2018). The worldwide importance of honey bees as pollinators in natural habitats. Proc. R. Soc. B Biol. Sci. 285, 20172140. doi: 10.1098/rspb.2017.2140
IPBES (2016). Assessment report on pollinators, pollination and food production. UNEP/GRID Eur. 37, 556. doi: 10.5281/zenodo.3402856
Johnson, R. M. (2015). Honey bee toxicology. Annu. Rev. Entomol. 60, 415–434. doi: 10.1146/annurev-ento-011613-162005
Klein, A.-M., Vaissire, B. E., Cane, J. H., Steffan-Dewenter, I., Cunningham, S. A., Kremen, C., et al. (2006). Importance of pollinators in changing landscapes for world crops. Proc. R. Soc. B Biol. Sci. 274, 303–313. doi: 10.1098/rspb.2006.3721
Lima, M. A. P., Martins, G. F., Oliveira, E. E., and Guedes, R. N. C. (2016). Agrochemical-induced stress in stingless bees: peculiarities, underlying basis, and challenges. J. Comp. Physiol. A 202, 733–747. doi: 10.1007/s00359-016-1110-3
Lundin, O., Rundlöf, M., Smith, H. G., Fries, I., and Bommarco, R. (2015). Neonicotinoid insecticides and their impacts on bees: a systematic review of research approaches and identification of knowledge gaps. PLoS ONE 10, e0136928. doi: 10.1371/journal.pone.0136928
Marshall, I. J., and Wallace, B. C. (2019). Toward systematic review automation: a practical guide to using machine learning tools in research synthesis. Syst. Rev. 8, 163. doi: 10.1186/s13643-019-1074-9
Medrzycki, P., Giffard, H., Aupinel, P., Belzunces, L., Chauzat, M.-P., Classen, C., et al. (2013). Standard methods for toxicology research in Apis mellifera. J. Apic. Res. 52, 60. doi: 10.3896/IBRA.1.52.4.14
Moher, D., Liberati, A., Tetzlaff, J., Altman, D. G., Altman, D., Antes, G., et al. (2009). Preferred reporting items for systematic reviews and meta-analyses: the PRISMA statement. PLOS Med. 6, e1000097. doi: 10.1371/journal.pmed.1000097
Nogrado, K., Lee, S., Chon, K., and Lee, J.-H. (2019). Effect of transient exposure to carbaryl wettable powder on the gut microbial community of honey bees. Appl. Biol. Chem. 62, 6. doi: 10.1186/s13765-019-0415-7
OECD (1998a). Test no. 2014 : Honeybees, Acute Contact Toxicity Test. Organisation for Economic Co-operation and Development.
OECD (1998b). Test No. 213: Honeybees, Acute Oral Toxicity Test. OECD Guidelines for the Testing of Chemicals. Organisation for Economic Co-operation and Development.
Ostiguy, N. (2011). Pests and pollinators | learn science at scitable. Nat. Educ. Knowl. 3, 3. Available online at: https://www.nature.com/scitable/knowledge/library/pests-and-pollinators-23564436/
Peterson, S. S., and Artz, D. R. (2013). “Production of solitary bees for pollination in the United States,” in Mass Production of Beneficial Organisms: Invertebrates and Entomopathogens, eds J. A. Morales-Ramos, M. Guadalupe Rojas, and D. I. Shapiro-Ilan (Cambridge, MA: Academic Press), 653–681. doi: 10.1016/B978-0-12-391453-8.00019-4
Pires, C. S. S., Ribeiro de Sá Torezani, K., de Oliveira Cham, K., de Castro Viana-Silva, F. E., de Oliveira Borges, L., Tonelli, C. A. M., et al. (2018). Seleção de espécies de abelhas nativas para avaliação de risco de agrotóxicos. Available online at: https://www.ibama.gov.br/phocadownload/agrotoxicos/reavaliacao-ambiental/2018/Selecao_Especies_Abelhas_Nativas_para_Avaliacao_de_Risco_de_Agrotoxicos.pdf (accessed December 21, 2021).
Potts, S. G., Imperatriz-Fonseca, V., Ngo, H. T., Aizen, M. A., Biesmeijer, J. C., Breeze, T. D., et al. (2016). Safeguarding pollinators and their values to human well-being. Nature 540, 220–229. doi: 10.1038/nature20588
R Core Team (2021). R: A Language and Environment for Statistical Computing. Available online at: https://www.r-project.org/ (accessed December 21, 2021).
Ramos, J. (2003). “Using tf-idf to determine word relevance in document queries,” in Proceedings of the First Instructional Conference on Machine Learning (Banff, AB), 29–48.
Sánchez-Bayo, F., and Wyckhuys, K. A. G. (2019). Worldwide decline of the entomofauna: a review of its drivers. Biol. Conserv. 232, 8–27. doi: 10.1016/j.biocon.2019.01.020
Sarkar, S., Gil, J. D. B., Keeley, J., and Jansen, K. (2021). The Use of Pesticides in Developing Countries and Their Impact on Health and the Right to Food. European Union.
Schneider, K.-M. (2005). “Techniques for improving the performance of naive bayes for text classification,” in Lecture Notes in Computer Science, ed A. Gelbukh (Berlin; Heidelberg: Springer), 682–693. doi: 10.1007/978-3-540-30586-6_76
Schwarzer, G., Carpenter, J. R., and Rücker, G. (2015). Meta-Analysis With R. Cham: Springer International Publishing. doi: 10.1007/978-3-319-21416-0
Sharma, A., Kumar, V., Shahzad, B., Tanveer, M., Sidhu, G. P. S., Handa, N., et al. (2019). Worldwide pesticide usage and its impacts on ecosystem. SN Appl. Sci. 1, 1446. doi: 10.1007/s42452-019-1485-1
Siviter, H., Bailes, E. J., Martin, C. D., Oliver, T. R., Koricheva, J., Leadbeater, E., et al. (2021). Agrochemicals interact synergistically to increase bee mortality. Nature 596, 389–392. doi: 10.1038/s41586-021-03787-7
Tomé, H. V. V., Ramos, G. S., Araújo, M. F., Santana, W. C., Santos, G. R., Guedes, R. N. C., et al. (2017). Agrochemical synergism imposes higher risk to Neotropical bees than to honey bees. R. Soc. Open Sci. 4, 160866. doi: 10.1098/rsos.160866
Tomé, H. V. V., Schmehl, D. R., Wedde, A. E., Godoy, R. S. M., Ravaiano, S. V., Guedes, R. N. C., et al. (2020). Frequently encountered pesticides can cause multiple disorders in developing worker honey bees. Environ. Pollut. 256, 113420. doi: 10.1016/j.envpol.2019.113420
Van de Schoot, R., de Bruin, J., Schram, R., Zahedi, P., de Boer, J., Weijdema, F., et al. (2021a). An open source machine learning framework for efficient and transparent systematic reviews. Nat. Mach. Intell. 3, 125–133. doi: 10.1038/s42256-020-00287-7
Van de Schoot, R., De Bruin, J., Schram, R., Zahedi, P., De Boer, J., Weijdema, F., et al. (2021b). ASReview: Active Learning for Systematic Reviews. Utrecht University.
Van Der Sluijs, J. P., Amaral-Rogers, V., Belzunces, L. P., Bijleveld Van Lexmond, M. F., Bonmatin, J. M., Chagnon, M., et al. (2015). Conclusions of the worldwide integrated assessment on the risks of neonicotinoids and fipronil to biodiversity and ecosystem functioning. Environ. Sci. Pollut. Res. 22, 148–154. doi: 10.1007/s11356-014-3229-5
VanEngelsdorp, D., Evans, J. D., Saegerman, C., Mullin, C. A., Haubruge, E., Nguyen, B. K., et al. (2009). Colony collapse disorder: a descriptive study. PLoS ONE 4, e6481. doi: 10.1371/journal.pone.0006481
Wagner, D. L., Grames, E. M., Forister, M. L., Berenbaum, M. R., and Stopak, D. (2021). Insect decline in the Anthropocene: death by a thousand cuts. Proc. Natl. Acad. Sci. 118, e2023989118. doi: 10.1073/pnas.2023989118
Wardhaugh, C. W. (2015). How many species of arthropods visit flowers? Arthropod. Plant. Interact. 9, 547–565. doi: 10.1007/s11829-015-9398-4
Keywords: Apidae, ecotoxicology, Hymenoptera, insecticides, machine learning, pollinator decline, quantitative systematic review
Citation: Bernardes RC, Botina LL, Araújo RDS, Guedes RNC, Martins GF and Lima MAP (2022) Artificial Intelligence-Aided Meta-Analysis of Toxicological Assessment of Agrochemicals in Bees. Front. Ecol. Evol. 10:845608. doi: 10.3389/fevo.2022.845608
Received: 30 December 2021; Accepted: 14 April 2022;
Published: 19 May 2022.
Edited by:
Alberto Pozzebon, University of Padua, ItalyReviewed by:
May Berenbaum, University of Illinois at Urbana–Champaign, United StatesYahya Al Naggar, Martin Luther University of Halle-Wittenberg, Germany
Copyright © 2022 Bernardes, Botina, Araújo, Guedes, Martins and Lima. This is an open-access article distributed under the terms of the Creative Commons Attribution License (CC BY). The use, distribution or reproduction in other forums is permitted, provided the original author(s) and the copyright owner(s) are credited and that the original publication in this journal is cited, in accordance with accepted academic practice. No use, distribution or reproduction is permitted which does not comply with these terms.
*Correspondence: Renan dos Santos Araújo, cmVuYW5kb3NhbnRvc2FyYXVqb0BnbWFpbC5jb20=