- 1Institute of Evolutionary Ecology and Conservation Genomics, Ulm University, Ulm, Germany
- 2Department of Food, Environmental and Nutritional Sciences, University of Milan, Milan, Italy
- 3Organismic and Cellular Interactions, Biocenter, Ludwig-Maximilians-Universität München, Planegg, Germany
- 4Faculty of Biological and Environmental Sciences, Federal University of Grande Dourados, Dourados, Brazil
Bumble bees (Bombus terrestris) are important pollinators of wild and crop plants. Despite their importance in the process of fruit and seed production on crop sites, their activity may be impaired due to exposure to pesticides. This species has a yearly life cycle and colony success may rely on effective foraging of workers on ruderal plants late in summer when most crops are no longer flowering. In the current study, we investigated the effect of chronic exposure to Sulfoxaflor on aspects of the foraging behavior of bumble bees and whether Sulfoxaflor influences the body size of workers of B. terrestris in a crop landscape. We found that 2 weeks of continuous exposure to Sulfoxaflor influenced workers’ foraging dynamics and collection of resources. However, there was no evidence that the 5 ppb dose of the pesticide impacted the ability of bees to handle flowers with different traits. Workers from colonies exposed to Sulfoxaflor were smaller. The effect on worker size may be explained as a consequence of the reduced pollen income per unit of worker foraging. Thus, if the effects of Sulfoxaflor applied directly to crops had the same effect as that observed on commercial bumble bees after our chronic exposure, it might negatively impact colony success due to the impact on pollen collection and the reduction in the size of workers.
Introduction
The ecosystem services provided by bees are among the most important for the reproduction of wild plants (Ollerton et al., 2011) and crop production (Klein et al., 2007; Giannini et al., 2015; Sagwe et al., 2021). Nutritional resources that bees collect from flowers (e.g., nectar, pollen) during flower visits are usually consumed to supply their own metabolic demand or carried into the nest to feed the young and larvae. The nutrition of immatures is often based on a mixture of nectar and pollen (Donkersley et al., 2017). The latter is rich in protein, lipids, and carbohydrates (Kenđel and Zimmermann, 2020), important components for the development of the brood (Watrous et al., 2019).
The ability of a given bee to collect pollen from a flower may depend on the evolutionary process between bees and plants. Specialized bees, for example, may be adapted to collect resources only from a few floral types (Konzmann et al., 2020), while generalist species explore resources in a wide variety of flowers (Wilson et al., 2021). Among the generalist social bee species, such as Bombus terrestris, diet composition may depend on the environment including the presence of competitors (Balfour et al., 2015; Lihoreau et al., 2016). Some flowers can also present a set of floral traits (e.g., shape, color, and resources) that influence generalists’ foraging behavior (Spaethe et al., 2001; Wood et al., 2021). Social bees (e.g., honey bees) are organisms performing complex tasks (Nityananda and Chittka, 2021) that together with their social communication work synergistically to mitigate the negative effects of landscape (Nürnberger et al., 2019). However, it has been shown that unhealthy or stressed bees are less efficient at performing social tasks (Boff et al., 2018; Crall et al., 2018; Raine, 2018). Among environmental stressors, we highlight systemic insecticides. These agrochemicals (e.g., neonicotinoids, sulfoximines, butenolides) target nicotinic acetylcholine receptors (nAChRs) in insect nervous systems which, depending on concentration and class, can impair common bee activities, such as pollination (Stanley et al., 2015a; Whitehorn et al., 2017), social communication (Boff et al., 2018), antennal sensitivity (Straub et al., 2021), thermoregulation (Tong et al., 2019), and memory (Williamson and Wright, 2013; Stanley et al., 2015b; Tison et al., 2019) but see Siviter et al. (2019).
Workers of B. terrestris audax exposed to the neonicotinoid Thiamethoxan displayed a different choice for floral resource collection compared to control bees (Stanley and Raine, 2016). Moreover, the number of manipulated flowers increased significantly for pesticide exposed bumble bees, suggesting an impact on maximum foraging ability (Stanley and Raine, 2016). The ability of bumble bee workers to handle tomato flowers was also impacted when they were exposed to Thiamethoxan. According to Whitehorn et al. (2017), the bees were less able to remove pollen from flowers by sonication, averaging about 50% less pollen transportation than control bees. Similarly, Switzer and Combes (2016) found that chronic exposure to a dose of Imidacloprid, below LD50, significantly reduced the number of individuals performing sonication on tomato flowers. Additionally, workers exposed to the pesticide were less likely to perform additional sonication events (Switzer and Combes, 2016). Therefore, since colony development and individual health rely on diversified pollen feeding (Kaluza et al., 2018; Vaudo et al., 2018), impaired individual ability to collect pollen (Stanley et al., 2016; Switzer and Combes, 2016; Whitehorn et al., 2017) can have a negative impact on colony development and in the nutritional aspects of foragers and other members of the colony.
In addition to sonication on buzz pollination flowers, the sub-lethal effects of pesticides on bees include impairment of navigation (Fischer et al., 2014; Balbuena et al., 2015), flying, and homing (abilities to return to nest) (e.g., Stanley et al., 2016). For instance, acute (one time exposure) and chronic exposure to Thiamethoxam changed flight dynamics in honey bees (Tosi et al., 2017). While the acute dose increased flight distance, chronic exposure decreased flight duration, distance, and velocity. Similar impacts were recorded for bumble bee species exposed to a different Neonicotinoid. Bumble bee workers exposed to Imidacloprid were faster but achieved a shorter distance and reduced flight time in the flight cage experiment (Kenna et al., 2019). Recently, studies with a new acetylcholinesterase inhibitor pesticide (Sulfoxaflor) have shown that harmful effects on foraging are likely to vary with dosage and bee species (Siviter et al., 2018; Boff et al., 2021; Tamburini et al., 2021a,b). For example, in a previous study, flight dynamics of solitary mason bees were adversely affected when exposed to field-realistic doses of Sulfoxaflor (Boff et al., 2021). In this study, Sulfoxaflor had the effect of increasing the time necessary for a bee to land in a flower (Boff et al., 2021). Further studies, with honey bees (Tamburini et al., 2021b) and bumble bees (Tamburini et al., 2021a), showed that Sulfoxaflor may have species-specific effects, supporting previous reports of different bee species having different responses when exposed to the same pesticide (Cresswell et al., 2012; Arena and Sgolastra, 2014; Mayack and Boff, 2019; Azpiazu et al., 2021; Schmolke et al., 2021). A negative effect of Sulfoxaflor on non-commercial bumble bee colony development was suggested by the impact on reproductive output by lowering larvae (Linguadoca et al., 2021) and male production (Siviter et al., 2018), but did not appear to affect foraging and weight of pollen loads of workers (Siviter et al., 2018).
In the current study, we investigated the effect of a field-realistic dose of Sulfoxaflor on workers of commercial standard colonies of B. terrestris by recording (1) worker foraging activities, (2) the weight of their pollen loads, (3) and the plant species from which pollen grains were brought to the colonies. Foraging activities also included whether (4) workers exposed to Sulfoxaflor interacted with flowers with similar traits to those visited by unexposed workers; and finally, (5) whether Sulfoxaflor influenced the body size of workers. Given the results of previous studies, we expected impairment of reproduction to explain the amount of collected resources and changes in collected plant species composition. Since impairment at different levels on foragers across bee species (Boff et al., 2021; Tamburini et al., 2021a,b) has been associated with this insecticide, studying the effect of Sulfoxaflor in commercial bumblebees is an important approach to the safety of pollinators and pollination outcomes in conventional agricultural environments late in the summer.
Materials and methods
Study area
The study site where bumblebee nests were located corresponds to the orchard farm of the University of Milan, at Arcagna (45° 20′ 31″N, 9° 27′ 5.18″ E), Lodi province, at about 25 km away from the city center of Milan, Italy. The area is characterized by the presence of cultivars of different fruit species such as apple trees (1.5 ha), pear trees (1.0 ha), peach trees (5.0 ha), apricot trees (0.2 ha), plum trees (1.5 ha), cherry trees (0.15 ha) and other small fruits (0.15 ha). The study was performed in late summer (August 2019) when local fruit crop species were no longer blooming.
Pesticide preparation
In this study, we exposed commercial colonies of bumble bees B. terrestris to a field-realistic concentration of Sulfoxaflor according to the amount of the pesticide found in the nectar of cotton flowers, the United States Environmental Protection Agency (2016) and used in previous studies (e.g., Siviter et al., 2018; Boff et al., 2021). To reach a sucrose solution with a final dose of 5 ppb, 1.8 M sucrose solution (616.19 gr of sugar) was diluted in 1 L of distilled water. The stock solution of 1 mg of Sulfoxaflor was mixed with 100 ml of pure acetone (Medrzyck et al., 2021). The Sulfoxaflor solution of use (5 ppb, 5 × 10–6 gr Sulfoxaflor L–1) was prepared by diluting 500 μl of the stock solution in 1 L of the sucrose solution 1.8 M.
Colony treatment
Standard commercial colonies of B. terrestris (n = 8) type Natupol (Koppert) were delivered in a plastic cage set in a cardboard box. Two of the colonies were delivered approximately 2 weeks earlier than the rest. Bees from these two colonies were let free to forage at our experimental site. These two colonies (herein called old colonies) were brought back to the laboratory at night when the other six colonies were delivered. In the laboratory, on the following day, one of the old colonies was added to the pool of Sulfoxaflor exposed colonies, and the other four (one old and three new) were assigned to the control. Ad libitum supply of 1.8 M sucrose solution with 5 ppb of Sulfoxaflor was made available to the treated colonies (n = 4) attaching a bag with 1.5 L of the mixed solution to the feeder at the bottom of the nest box. Control colonies were allowed access to ad libitum 1.8 M sucrose solution without Sulfoxaflor, plus an equivalent concentration of the acetone added in the treatment group. Colonies were maintained closed in a laboratory shielded from direct light exposure with a temperature set at 25°C for the period of 12 days (considered week 1 and week 2 of the experiment). Additionally, 16 g of commercial dry honeybee collected pollen were provided daily to the colonies. At the end of the 12th day, the colonies were transferred and positioned at the study site and the feeder containing sucrose solution with or without Sulfoxaflor was removed. The colonies were set in a covered area near the crops site (∼10 m) circa 20 cm from the ground. Colonies exposed to Sulfoxaflor were set on one side of the covered area attached to a lateral wall with a 2 m distance between each colony. Control colonies were set on the other side of the covered area at a distance of circa 6 m from the closest Sulfoxaflor exposed colony (Supplementary Figure 1). The day after installing the colonies, in the morning, entrances were opened one by one in an interval of 30 min allowing bumblebees to forage. Once a week, the entrances of the colonies were closed at night. The following morning, before opening the colony entrances, the colonies were weighed. Weight of colonies did not differ across treatments throughout the experiment (LM, F = 2.78, df = 1, p = 0.10).
Flight activities
The number of individual foragers leaving each colony was counted twice a day (between 8 a.m. and 1 p.m.) for 5 min each time. The census was performed independently at each colony and repeated once a week for a total of 5 weeks, totaling 400 min of monitoring. Additionally, except for the first monitoring day (the third week of the experiment), the number of individuals returning to the nest and individuals returning with pollen were also recorded, totaling 320 min of observations.
Collection of pollen
After the census, between 2 and 4 p.m., workers returning to the hive with pollen loads were collected at the hive entrance, each week, during the 3rd, 4th, 5th, and 6th week, with the aid of tweezers. Captured workers were placed individually in a Petri dish (55 mm diameter) and maintained inside a styrofoam box with ice packs. Cooled immobilized workers (n = 76 for control and n = 76 for exposed colonies, ntotal = 152, see Table 1 for details of sample size) had their pollen loads removed with tweezers. The tweezers were washed with pure ethanol following heat sterilization after each pollen load removal. Pollen loads presented in the corbicula were placed in a sterile plastic tube, labeled, and transferred to a –80°C freezer in the laboratory. After the removal of pollen loads, workers were measured (see below), put back on the Petri dish, and released near their hive entrance. In the laboratory, pollen loads were lyophilized at –60°C and each pollen load from a given individual was individually weighed with a balance (precision 10–3g).

Table 1. Total and mean (± SD) number of individuals per colony from which pollen loads were analyzed.
Metabarcoding for identification of pollen grains
Metabarcoding followed strictly the strategy of Sickel et al. (2015) using the ITS2 region as suggested by Keller et al. (2015). Beside the biological samples (n = 152), negative PCR controls were added. Forward ITS-S2F (Chen et al., 2010) and reverse primers ITS4R (White et al., 1990) contained multiplex identifiers so each had a unique combination. Triplicate polymerase chain reactions were performed for each sample and marker using the following conditions: heat activation at 95°C for 3 min; 35 cycles of denaturation at 95°C for 30 s, annealing at 55°C for 30 s, and elongation at 72°C for 1 min; followed by a final extension at 72°C for 10 min, holding at 10°C until storage. Amplification success was checked on a 1% agarose gel, using 2 μl product and 4 μl dye per sample, and electrophoresed at 100 V for 45 min. Amplicons were pooled by the sample in 96-well plates and concentrations were normalized using the SequalPrep Normalization Plate Kit (Invitrogen Corporation, CA). Samples were further pooled by plate (hereafter, “libraries”) and quantified with a Qubit (Invitrogen Corporation, CA) fluorometer. The final pooled library was spiked with 5% PhiX control. The library was sequenced on an Illumina MiSeq v2, 2 × 250 bp spiked with custom index, Read1 and Read2 sequencing primers as detailed in the protocol.
Bioinformatics followed mostly the protocol as described in Campos et al. (2021): subsequent filtering and data processing steps were carried out with VSEARCH (Rognes et al., 2016). First, forward and reverse reads were joined. We removed reads of low quality (-fastq_maxee 1) and subsequently removed reads with < 200 bp or > 500 bp. Second, filtered reads of all samples were dereplicated, denoised, and assigned to amplicon sequence variants (ASVs). Sample-specific reads were then mapped to ASVs to generate count data for each sample and plant species. Subsequently, we classified the ASVs using global alignments and best hit selection (at least 97% identity), against pre-defined reference databases. For this, we used the BCdatabaser (Keller et al., 2020) to subset the NCBI database for the ITS2 marker and contain only reference sequences known to occur in the Milan wider region (see Bartolucci et al., 2018)1 ASVs that remained unclassified were mapped against a global reference database (Ankenbrand et al., 2015). Lastly, SYNTAX was used to obtain a taxonomy of remaining unclassified reads as deep as possible to a maximum of genus level. Using the R package phyloseq (McMurdie and Holmes, 2013), we imported the final read abundance and taxonomic tables, normalized the read abundance by sample such that each sample totaled 100 (relative read abundances), and filtered taxa that represented less than 1% per sample.
Floral diet diversity
The final list of plant species identified using metabarcoding was investigated with regard to their species richness (just number of species) and composition in control and treated colonies.
Floral traits and resource exploitation
The most common plant species (n = 40) identified in the pollen diet of broods from control and treated colonies were investigated regarding their floral traits. The description of the floral shape was performed based on Faegri and Van Der Pijl (1979) and Machado and Lopes (2004). Floral shape types were the followings: tube, gullet, dish, brush, flag, bell-funnel, chamber, and inconspicuous (< 4 mm) (Faegri and Van Der Pijl, 1979; Machado and Lopes, 2004). The resource types were classified as predominantly nectar flower, pollen flower, or flower offering both resources simultaneously (Faegri and Van Der Pijl, 1979). Flower colors were classified according to human perception and classified as greenish, purple, red, pink, white, and yellow.
Body size of workers
Workers returning from foraging with pollen loads were sized with a digital pachymeter by measuring the thorax size at the insertion point of wings. In the same manner, at the end of the experiment, workers were sized and remained frozen at –20°C.
Statistical analyses
Model fit to all General Linear Mixed Models (GLMM) was assessed with the package DHARMa (Hartig, 2020). All analyses were performed in R 1.4.1106 (R Core Team, 2018).
Flight activities
We used GLMM to test the effect of Sulfoxaflor exposure and its interaction with time (temporal effect, “weeks”) on the number of individuals leaving the nest, the number of individuals returning to the nest, and the proportion of individuals returning to the nest carrying pollen loads. In our models, colony identity was used as a random factor to account for multiple measurements from individuals from the same colony. A Poisson distribution of errors was used in the model for workers leaving and returning to the colonies. The proportion of individuals carrying pollen was arcsine square root transformed to avoid variance dependence (Gotelli and Ellison, 2013). For the proportion of individuals with pollen loads, we used a model with a negative binomial distribution of errors. Model selections were based on AIC comparisons. Models with delta AIC smaller than 2 were considered similar and selection was based on the lowest p-value.
Pollen collection
To test the effect of Sulfoxaflor exposure, temporal effects (weeks) and nest age on the weight of pollen loads sampled per worker, we used a GLMM with colony identity as a random factor to account for multiple measurements of multiple individuals from the same colony and a negative binomial distribution of errors.
Floral diet diversity
The number of plant species visited was compared among treatments and weeks of observation. The size of the workers and the dry weight of pollen grains were additionally considered dependent variables with nest identity and nest age as random factors in a model with a negative binomial distribution of errors.
Distance-based redundancy analysis (dbRDA), a constrained ordination method, was used to quantify the proportion of variation in community composition in a site by species matrix. The matrix can be explained by variables represented in a site by an environmental data matrix and a matrix of spatial variables (Legendre and Legendre, 2012). Bray-Curtis distances (capscale function from vegan R package, Oksanen et al., 2022) were used to ordinate workers by floral species composition (identified from worker’s pollen load) considering pesticide treatment, age of the nest, and period (weeks) from the 3rd to 6th week. Permutational ANOVA was used to test the proportion of variance explained by each of these variables and the variance in the floral species composition.
Floral traits and resource exploitation
We used the procedure of dbRDA with Bray-Curtis distances and permutational ANOVA to test the proportion of variance explained by flower shape, color, and resources, as well as the variance in flowers, with respect to the composition of specific floral traits.
Body size of workers
We used a GLMM to test whether exposure to Sulfoxaflor during the immature phase (workers at larvae-feeding phase during pesticide exposure) affected the size of workers. In this model, the average body size of workers was compared between treatments taking into consideration the temporal effect (week) and the age of nests with colony identity used as a random factor with Gaussian and a log link function. Workers measured in week 3 and week 4 were removed from the model to mitigate a potential inclusion of individuals that were already adults at the beginning of the experiment. Model selections were performed based on AIC comparison with the ANOVA function.
Results
Flight activities
Both week and treatment affected the number of individuals leaving the colonies. More individuals were observed leaving treated colonies (mean ± SD) 2.95 ± 2.41 5 min–1 relative to the control group 2.2 ± 1.98 5 min–1 (GLMM, χ2 = 3.89, df = 1, p = 0.04, Figure 1A). Overall, after week four, there was a reduction in the number of individuals leaving the colonies as the weeks progressed (GLMM, χ2 = 10.42, df = 4, p = 0.005, Figure 1A for Tukey HSD post hoc test). The number of returning workers to control colonies 2.47 ± 1.94 5 min–1 and to treated colonies 3.31 ± 3.04 5 min–1 were not statistically different (GLMM, χ2 = 2.0, df = 1, p = 0.157, Figure 1B). Yet, the number of individuals returning to the colonies significantly decreased as the weeks progressed (GLMM, χ2 = 48.73, df = 3, p < 0.001, Figure 1B for Tukey HSD post hoc test). No significant difference between the number of individuals returning with pollen (average for control colonies 1.18 ± 1.30 5 min–1, average for treated colonies 1.5 ± 2.2 5 min–1) was found between treatments (GLMM, χ2 = 0.52, df = 1, p = 0.468, Figure 1C); the number of pollen loads collected by workers decreased as the week progressed (GLMM, χ2 = 48.73, df = 3, p < 0.001, Figure 1C for Tukey HSD post hoc test).
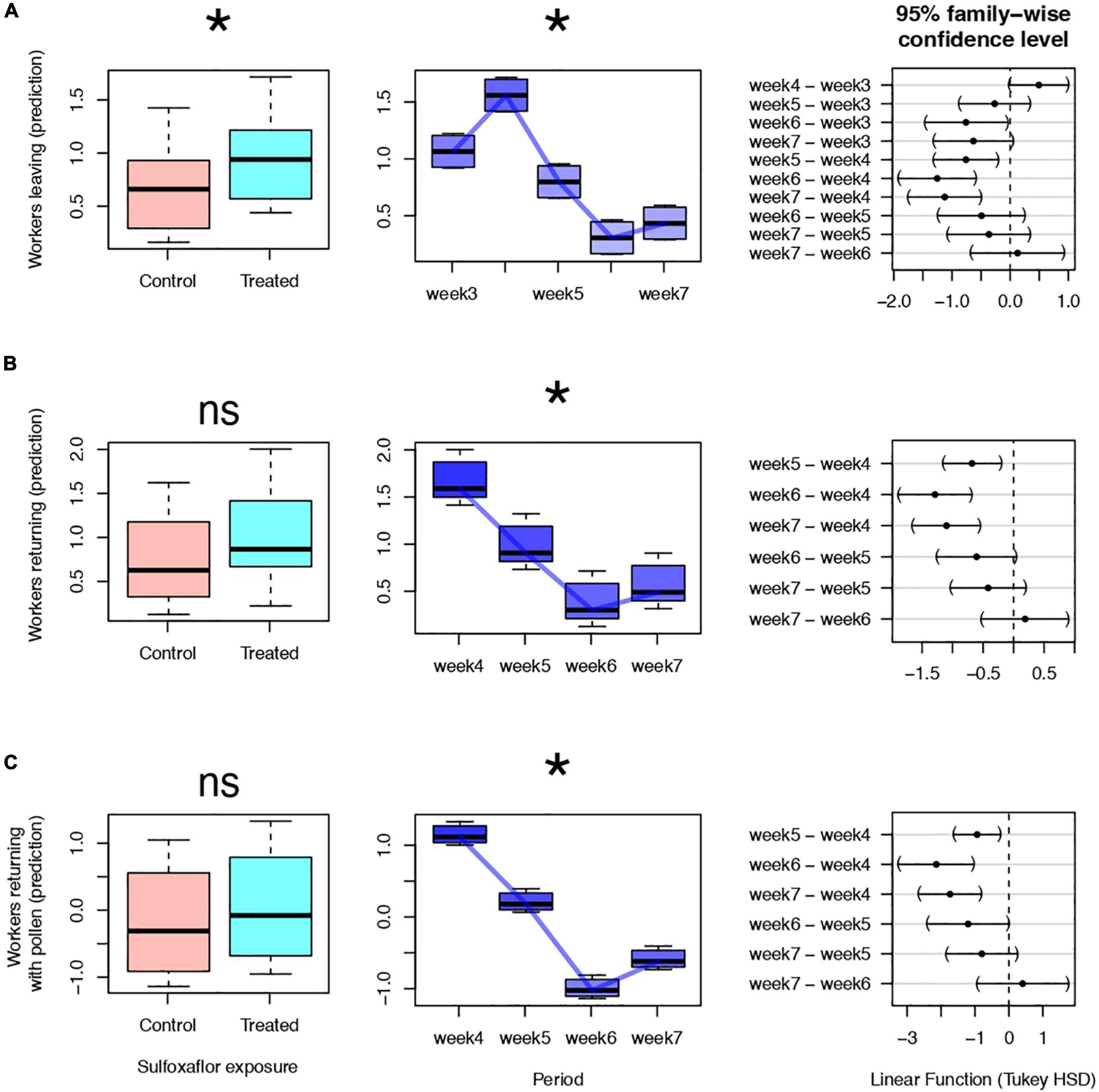
Figure 1. Flight activities of bumble bees measured in terms of individuals leaving (A), returning (B), and returning with pollen (C) to the nest (predictions from Generalized Linear Mixed Model, with Poisson distribution family, in which treatment and period of observations (weeks) were fixed and nest identity random factors). The Left and middle plots show the effects of treatment and weeks, respectively. The plots on the right show pairwise comparison (Tukey HSD test) between weeks (intervals apart from zero -dashed line- indicate significant differences). *Significant (p < 0.05) and ns: non-significant effect.
Pollen collection
Treatment and week affected the weight of pollen loads per forager brought back to the colonies. Bees brought heavier pollen loads back to the hive in the treated group 20.03 ± 13 mg, relative to the untreated group 16.3 ± 13 mg (GLMM, z = 1.991, p = 0.04). The age of the nest had no effect on the weight of pollen load carried by the workers (GLMM, χ2 = 2.83, df = 1, p = 0.09); the period (weeks) had a significant effect on decreasing pollen weight (see Supplementary Table 1, Tukey HSD post hoc test).
Floral diet diversity
Only treatment influenced the number of plant species found in pollen loads. The number of plant species found in the pollen loads removed from the corbiculae ranged from one to 20 species. Workers from treated colonies visited (mean ± SD) 5.95 ± 3.4 species per foraging event; while control bees visited (mean ± SD) 5.0 ± 3.8 species; thus, Sulfoxaflor influenced the average number of plant species (alpha diversity; GLMM, χ2 = 4.13, df = 1, p = 0.04) identified in the pollen loads of workers from treated in comparison to control colonies. However, there were no effects of weeks, bee size, or pollen load weight on alpha diversity (Table 2). Only week affected the composition of plant species (dbRDA, R2adj = 0.14, F = 8.03, df = 3, p = 0.001, Supplementary Figure 2). There was no effect of treatment (dbRDA, R2adj = 0, F = 0.53, df = 1, p = 0.76) or nest age (R2adj = 0.01, F = 1.99, df = 1, p = 0.07) on the composition of plant species.

Table 2. Variables affecting alpha diversity of ruderal plant species visited by Bombus terrestris in late summer.
Floral traits and resources exploitation
Only week and nest age (but not treatment) affected the type of flowers bees interacted with. The combined floral traits of plant species making up the diet of controls and treated colonies did not differ (dbRDA, R2adj = 0, F = 0.52, df = 1, p = 0.76). Floral traits differed between nests of different ages (dbRDA, R2adj = 0.01, F = 2.47, df = 1, p = 0.04) and over weeks (dbRDA, R2adj = 0.14, F = 8.06, df = 3, p = 0.001, Supplementary Figure 3).
The body size of workers
Treatment, age of the colony, and week affected the body size of workers. Sulfoxaflor exposure had an impact on the size of workers (GLMM, χ2 = 4.82, df = 1, p = 0.02). Workers exposed to Sulfoxaflor were significantly smaller (mean ± SD) 5.76 ± 1.27 mm than workers from control colonies (mean ± SD) 6.05 ± 1.26 mm (t = 2.185, p = 0.02, Figure 2). A reduction in size was observed in workers from older colonies (GLMM, t = 2.482, p = 0.013). We also found a temporal effect on the size of workers, with an increase in the size of the bees at the end of the experiment (GLMM, Tukey HSD post hoc test; 5th–6th weeks, z = 1.691, p = 0.202; 5th–7th weeks, z = 8.112, p < 0.001; 6th–7th weeks, z = 9.604, p < 0.001).
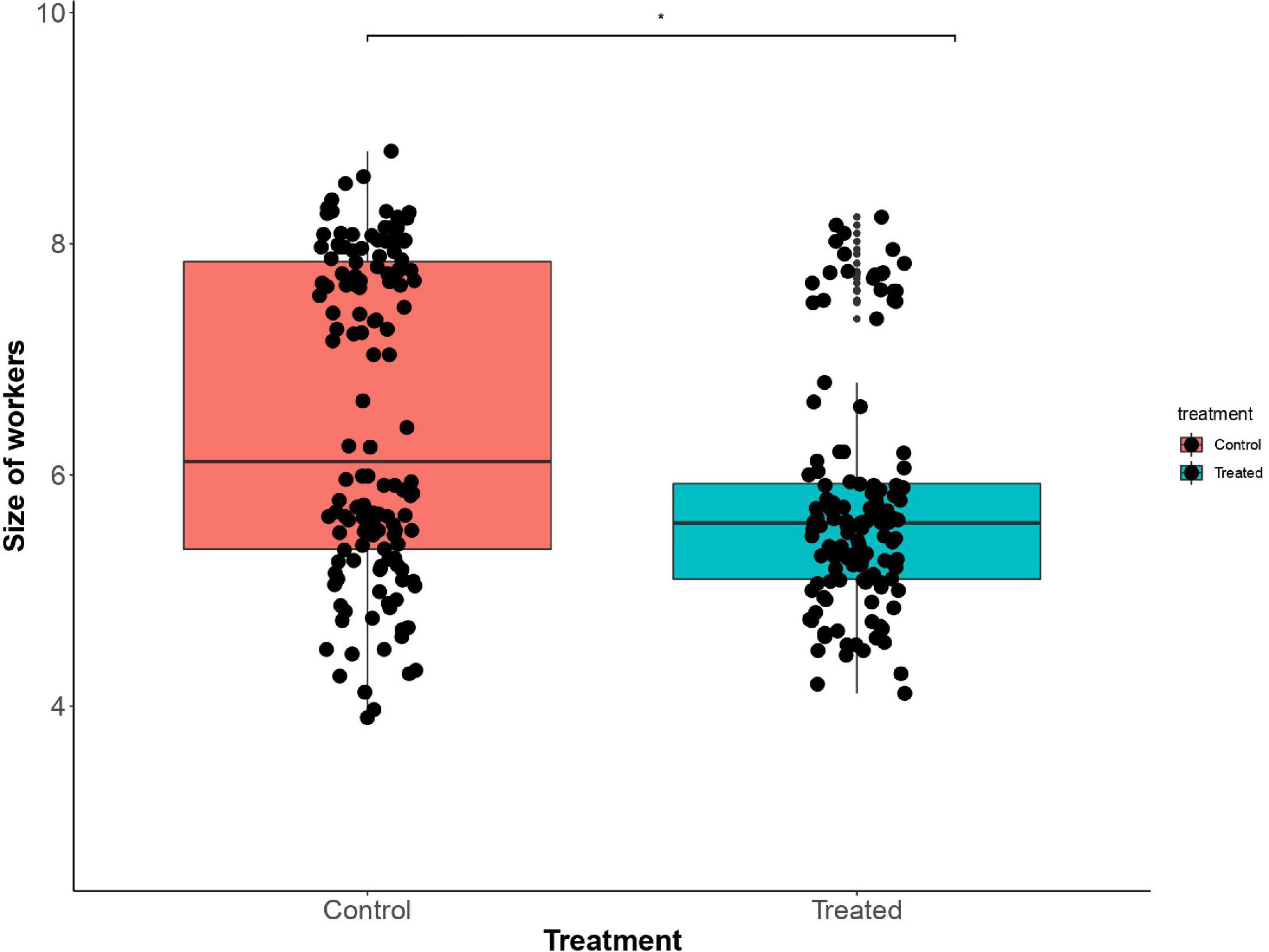
Figure 2. Bees exposed to Sulfoxaflor present a smaller size compared to bees not exposed to the insecticide (control). *p = 0.02.
Discussion
We tested whether a realistic dose of 5 ppb of Sulfoxaflor influenced flight dynamics, foraging strategies, or affected body sizes of commercial bumble bees, B. terrestris during late European summer. We found that a field-realistic dose of Sulfoxaflor affected foraging activities, leading to a reduction in the amount of pollen per forager brought back to the colony. The insecticide did not affect the plant composition bees foraged on. The sub-lethal dose studied here did not impair the ability of workers to handle flowers with different traits. We found a reduction in the size of workers exposed to Sulfoxaflor during the larval period.
Sub-lethal doses of insecticide neonicotinoids often affect the flight behavior of bumble bees (Gill and Raine, 2014; Kenna et al., 2019), the duration of foraging trips for bumble and honey bees (Stanley et al., 2016; Prado et al., 2019, respectively), and the amount of pollen collected by the bumble bees (Gill et al., 2012; Feltham et al., 2014). Sublethal effects of these pesticides impact flight motivation (Lämsä et al., 2018), foraging ability (Gill et al., 2012; Feltham et al., 2014), and foraging performance (Colin et al., 2019), leading to reduced pollination efficiency (Stanley et al., 2015a; Whitehorn et al., 2017). In addition to neonicotinoids, the sub-lethal doses of sulfoxamine-based pesticides were recently found to impair the survival of wild bees (Azpiazu et al., 2021; Boff et al., 2021). Moreover, mason bees exposed to Sulfoxaflor fly longer distances and for a longer time in relation to the control bees during foraging (Boff et al., 2021).
Sulfoxaflor belongs to a class of pesticides that has been shown to impact the reproduction of bumble bees (Siviter et al., 2018; Linguadoca et al., 2021). For instance, in a previous study, colonies of non-commercial bumble bees exposed to 5 ppb of Sulfoxaflor (the same dose as tested here) produced a smaller amount of males than control colonies (Siviter et al., 2018). In this study, Siviter et al. (2018) performed in an urbanized environment in England, exposure to Sulfoxaflor did not affect the foraging behavior of workers. In the conventional farming area where the current study was performed, we did find an effect of Sulfoxaflor on the foraging of bumble bees. Indeed, agricultural areas may impose an extra impairment on bumble bees due to potential reduced floral diversity compared to rich flower areas in urban sites (Theodorou et al., 2016). Here, the colonies were similar in weight, independent of treatment, suggesting a similar number of workers, yet the number of foragers leaving the colonies exposed to Sulfoxaflor differed from the control colonies. Interestingly, the frequency of workers returning with pollen did not differ between treatments, indicating that bees exposed to Sulfoxaflor may have experienced an impact on foraging. A similar finding was reported earlier when bumble bee workers were exposed to Imidacloprid (Gill et al., 2012). These results suggest that individuals exposed to Imidacloprid and Sulfoxaflor need more time to return from foraging events due to impairment over navigation, as observed in bumble bees exposed to Imidacloprid (Gill and Raine, 2014), and bumble bees (current study), mason bees (Boff et al., 2021), and honey bees (Barascou et al., 2022) exposed to Sulfoxaflor. Moreover, pollen loads collected by workers from Sulfoxaflor exposed colonies were on average heavier, indicating the successful foragers in a colony are probably working harder, collecting more pollen to offset the poorer foraging performance of their nest mates. Additionally, a higher proportion of foragers carrying pollen from control compared to Sulfoxaflor treated colonies, suggests that pesticide-exposed colonies had to send out more foragers to bring in the same amount of pollen in comparison with control colonies (see, Gill et al., 2012; Feltham et al., 2014).
Our current knowledge on the impact of Sulfoxaflor on bees is diverse, indicating these impairments may differ among species, the season, and the route of exposure; for example, Sulfoxaflor did not have an effect on the flight intensity of honey bees after administration of two different doses on cucumber flowers (Cheng et al., 2018). Similarly, no effect was found on honey bees foraging on Phacelia flowers exposed to Sulfoxaflor (Tamburini et al., 2021b). In a different study, the authors, using the same high recommended maximum rates of Sulfoxaflor (Tamburini et al., 2021b) found an impact on bumble bee flight activities (Tamburini et al., 2021a). The contrasting results between honey bees and bumble bees in sensitivity to the same pesticide, reinforce that sub-lethal effects of pesticides can be species-specific, as mentioned in previous studies on a variety of pesticides (Mayack and Boff, 2019; Azpiazu et al., 2021; Mundy-Heisz et al., 2022).
Bombus terrestris is a generalist flower visitor. Here, when set free to forage, the workers interacted with a wide diversity of ruderal flower types. According to previous studies, pesticides, such as Thiamethoxam and Imidacloprid, impact the foraging ability of bumble bees, likely reducing pollination of tomato flowers and collection of pollen (Switzer and Combes, 2016; Whitehorn et al., 2017). Sulfoxaflor at 5 ppb impaired the amount of pollen (in mg) brought to the colony in comparison to the control group; however, their ability to handle flowers of several traits might not have been impaired enough to be detected, as observed with bumble bees handling flowers with poricidal anthers (Whitehorn et al., 2017). We found that bees visited a similar set of plant species, but workers from Sulfoxaflor exposed colonies explored on average a greater pollen diversity. It would be interesting to test in the future whether these differences resulted from a lower capacity of competition for the best source of resources compared to control bees and whether workers from treated colonies are able to identify potential income from new food sources to balance the lack of primary sources due to reduction in competitiveness. Here, since workers became smaller, it might be that they added additional sources of food, probably becoming relatively more corpulent (Couvillon et al., 2011; Kelemen et al., 2020), independent of floral architecture.
The observed reduction in the size of workers from treated colonies may be explained by two non-exclusive hypotheses. First, despite workers from Sulfoxaflor exposed colonies collecting heavier pollen loads, the extra amount collected per returning worker might not have been sufficient to compensate for the loss of pollen income per unit of a worker leaving the colony. Yet, a substantial loss of adult foragers in pesticide exposed colonies may be neglected since colony weight remained stable over time. For instance, of 10 workers leaving control colonies to forage, 60% were returning with pollen loads. These percentages decreased to 49% in workers from Sulfoxaflor exposed colonies. This finding is also supported by the significant interaction factor between treatment and the period after exposure (weeks). For instance, while the number of individuals leaving the colony reduced over time, it was always higher for Sulfoxaflor exposed colonies. Moreover, there was an interesting trend showing that while the number of workers returning with pollen remained mostly constant over time in the control colonies, pollen income decreased sharply in the first 3 weeks of monitoring for Sulfoxaflor exposed colonies. Second, exposing queens to Sulfoxaflor might have led to a reduction in egg size leading to a reduction in workers’ size. Although the current study did not directly investigate effects on queen reproductive cells, egg plasticity in regard to size is known to occur in honey bees in response to environmental stressors (Amiri et al., 2020), therefore, it may possibly also be the case in bumble bees. For example, B. terrestris exposed chronically to Pyrethroid resulted in the production of smaller workers (Baron et al., 2014). Thus, in addition to impacting the reproductive output by reducing the production of males over time (Siviter et al., 2018), our study provides evidence of the impairment by Sulfoxaflor of reproductive potential by decreasing the size of workers but does not let us solve completely the mechanism driving the reduction in size. Nonetheless, bee size if correlated with flight distance ability, especially in the summer period when low local resources require significantly greater distances in foraging (Pope and Jha, 2018), reduced worker size may play an important role in colony success, especially in environments with low food quality (Osborne et al., 2008). Bees of smaller sizes patrol at smaller distances when compared to bigger bees and therefore they could be more susceptible to higher costs and competition due to local resource constraints (Osborne et al., 2008). Moreover, since workers from the 5th and 6th weeks were in general smaller than workers from the 7th week, it is possible that the pollen diet offered as dry pellets in the laboratory phase had a negative effect on growth. A full four factorial experiment with pesticide and variable food quality (Straub et al., 2022) may help us, in the future, to understand the mechanism through which food quality can offset the effects of pesticides on bees (Klaus et al., 2021).
Conclusion
Sulfoxaflor at the field-realistic dose of 5 ppb disrupted the foraging output of workers of B. terrestris in an area with ruderal plants when forced feeding was imposed continuously for a period of 12 days. A reduction in the proportion of returning workers with pollen per unit of a worker leaving the colony suggests a deficit in pollen income. Workers from Sulfoxaflor exposed colonies carried heavier pollen loads, possibly to compensate for the reduced pollen income in treated colonies. Workers from all colonies foraged on plant populations of similar composition and handled flowers of similar architecture and resources. Thus, 5 ppb of Sulfoxaflor seems to not impose a barrier for bee-plant interaction in the study environment. Our study is limited to comprehending whether the impact on non-reproductive workers occurs only due to reduced pollen income or might also be due to an impact on queen reproductive cells.
Data availability statement
The original contributions presented in this study are included in the article/Supplementary material, further inquiries can be directed to the corresponding author/s.
Author contributions
SB and DL designed the experiment and performed the data collection. SB, JR, and AK performed the data analysis. SB drafted the manuscript. SB, AK, JR, and DL contributed to the final version of the manuscript. All authors contributed to the article and approved the submitted version.
Acknowledgments
We thank Can Disbudak, Alp Kaan Kutay, and Emanuele Quattrini for field assistance, as well as Anna Friedel. We would also like to thank Matteo Zugno and Gudrum Grimmer for lab assistance, Maureen Labarussias for helping with editing the previous version of the manuscript, Koppert-Italy for providing commercial bumble bee colonies, DAAD (Deutscher Akademischer Austauschdienst), SCIAS (Siebold-Collegium, Institute for Advanced Studies, University of Würzburg) for the first author Award and Aurelia Stiftung for personal support, and Cariplo Foundation for its support through the project Api-Gis (id: 2019-4675).
Conflict of interest
The authors declare that the research was conducted in the absence of any commercial or financial relationships that could be construed as a potential conflict of interest.
Publisher’s note
All claims expressed in this article are solely those of the authors and do not necessarily represent those of their affiliated organizations, or those of the publisher, the editors and the reviewers. Any product that may be evaluated in this article, or claim that may be made by its manufacturer, is not guaranteed or endorsed by the publisher.
Supplementary material
The Supplementary Material for this article can be found online at: https://www.frontiersin.org/articles/10.3389/fevo.2022.842563/full#supplementary-material
Footnotes
References
Amiri, E., Le, K., Melendez, C. V., Strand, M. K., Tarpy, D. R., and Rueppell, O. (2020). Egg-size plasticity in Apis mellifera: Honey bee queens alter egg size in response to both genetic and environmental factors. J. Evol. Biol. 33, 534–543. doi: 10.1111/jeb.13589
Ankenbrand, M. J., Keller, A., Wolf, M., Schultz, J., and Förster, F. (2015). ITS2 Database V: Twice as Much. Mol. Biol. Evol. 32, 3030–3032. doi: 10.1093/molbev/msv174
Arena, M., and Sgolastra, F. (2014). A meta-analysis comparing the sensitivity of bees to pesticides. Ecotoxicology 23, 324–334. doi: 10.1007/s10646-014-1190-1
Azpiazu, C., Bosch, J., Bortolotti, L., Medrzycki, P., Teper, D., Molowny-Horas, R., et al. (2021). Toxicity of the insecticide sulfoxaflor alone and in combination with the fungicide fluxapyroxad in three bee species. Sci. Rep. 11:6821. doi: 10.1038/s41598-021-86036-1
Balbuena, M. S., Tison, L., Hahn, M. L., Greggers, U., Menzel, R., and Farina, W. M. (2015). Effects of sublethal doses of glyphosate on honeybee navigation. J. Exp. Biol. 218, 2799–2805. doi: 10.1242/jeb.117291
Balfour, N. J., Gandy, S., and Ratnieks, F. L. W. (2015). Exploitative competition alters bee foraging and flower choice. Behav. Ecol. Sociobiol. 69, 1731–1738. doi: 10.1007/s00265-015-1985-y
Barascou, L., Requier, F., Sené, D., Crauser, D., Le Conte, Y., and Alaux, C. (2022). Delayed effects of a single dose of a neurotoxic pesticide (sulfoxaflor) on honeybee foraging activity. Sci. Total Environ. 805:15035. doi: 10.1016/j.scitotenv.2021.150351
Baron, G. L., Raine, N. E., and Brown, M. J. F. (2014). Impact of chronic exposure to a pyrethroid pesticide on bumblebees and interactions with a trypanosome parasite. J. Appl. Ecol. 51, 460–469. doi: 10.1111/1365-2664.12205
Bartolucci, F., Peruzzi, L., Galasso, G., Albano, A., Alessandrini, A., Ardenghi, N. M. G., et al. (2018). An updated checklist of the vascular flora native to Italy. Plant Biosyst. 152, 179–303. doi: 10.1080/11263504.2017.1419996
Boff, S., Friedel, A., Mussury, R. M., Lenis, P. R., and Raizer, J. (2018). Changes in social behavior are induced by pesticide ingestion in a Neotropical stingless bee. Ecotoxicol. Environ. Saf. 164, 548–553. doi: 10.1016/j.ecoenv.2018.08.061
Boff, S., Scheiner, R., Raizer, J., and Lupi, D. (2021). Survival rate and changes in foraging performances of solitary bees exposed to a novel insecticide. Ecotoxicol. Environ. Saf. 211:111869. doi: 10.1016/j.ecoenv.2020.111869
Campos, M. G., Anjos, O., Chica, M., Campoy, P., Nozkova, J., Almaraz-Abarca, N., et al. (2021). Standard methods for pollen research. J. Apic. Res. 60, 1–109. doi: 10.1080/00218839.2021.1948240
Chen, S., Yao, H., Han, J., Liu, C., Song, J., Shi, L., et al. (2010). Validation of the ITS2 region as a novel DNA barcode for identifying medicinal plant species. PLoS One 5:e8613. doi: 10.1371/journal.pone.0008613
Cheng, Y., Bu, Y., Tan, L., Wu, W., Li, J., Zhou, J., et al. (2018). A semi-field study to evaluate effects of sulfoxaflor on honey bee (Apis mellifera). Bull. Insectology 71, 225–233.
Colin, T., Meikle, W. G., Wu, X., and Barron, A. B. (2019). Traces of a neonicotinoid induce precocious foraging and reduce foraging performance in honey bees. Environ. Sci. Technol. 53, 8252–8261. doi: 10.1021/acs.est.9b02452
Couvillon, M. J., Jandt, J. M., Bonds, J., Helm, B. R., and Dornhaus, A. (2011). Percent lipid is associated with body size but not task in the bumble bee Bombus impatiens. J. Comp. Physiol. A Neuroethol. Sens. Neural Behav. Physiol. 197, 1097–1104. doi: 10.1007/s00359-011-0670-5
Crall, J. D., Switzer, C. M., Oppenheimer, R. L., Ford Versypt, A. N., Dey, B., Brown, A., et al. (2018). Neonicotinoid exposure disrupts bumblebee nest behavior, social networks, and thermoregulation. Science 362, 683–686. doi: 10.1126/science.aat1598
Cresswell, J. E., Page, C. J., Uygun, M. B., Holmbergh, M., Li, Y., Wheeler, J. G., et al. (2012). Differential sensitivity of honey bees and bumble bees to a dietary insecticide (imidacloprid). Zoology 115, 365–371. doi: 10.1016/j.zool.2012.05.003
Donkersley, P., Rhodes, G., Pickup, R. W., Jones, K. C., Power, E. F., Wright, G. A., et al. (2017). Nutritional composition of honey bee food stores vary with floral composition. Oecologia 185, 749–761. doi: 10.1007/s00442-017-3968-3
Faegri, K., and Van Der Pijl, L. (1979). Principles of Pollination Ecology, 3rd Edn. Oxford: Pergamon Press.
Feltham, H., Park, K., and Goulson, D. (2014). Field realistic doses of pesticide imidacloprid reduce bumblebee pollen foraging efficiency. Ecotoxicology 23, 317–323. doi: 10.1007/s10646-014-1189-7
Fischer, J., Müller, T., Spatz, A. K., Greggers, U., Grünewald, B., and Menzel, R. (2014). Neonicotinoids interfere with specific components of navigation in honeybees. PLoS One 9:e91364. doi: 10.1371/journal.pone.0091364
Giannini, T. C., Boff, S., Cordeiro, G. D., Cartolano, E. A., Veiga, A. K., Imperatriz-Fonseca, V. L., et al. (2015). Crop pollinators in Brazil: A review of reported interactions. Apidologie 46, 209–223. doi: 10.1007/s13592-014-0316-z
Gill, R. J., and Raine, N. E. (2014). Chronic impairment of bumblebee natural foraging behaviour induced by sublethal pesticide exposure. Funct. Ecol. 28, 1459–1471. doi: 10.1111/1365-2435.12292
Gill, R. J., Ramos-Rodriguez, O., and Raine, N. E. (2012). Combined pesticide exposure severely affects individual-and colony-level traits in bees. Nature 491, 105–108. doi: 10.1038/nature11585
Gotelli, N., and Ellison, A. (2013). A Primer of Ecological Statistics. Sunderland: Harvard University.
Hartig, F. (2020). DHARMa: Residual Diagnostics for Hierarchical (Multi-Level/Mixed) Regression Models. R Package Version 0.3.3.0. Available online at: http://florianhartig.github.io/DHARMa/
Kaluza, B. F., Wallace, H. M., Heard, T. A., Minden, V., Klein, A., and Leonhardt, S. D. (2018). Social bees are fitter in more biodiverse environments. Sci. Rep. 8:12353. doi: 10.1038/s41598-018-30126-0
Kelemen, E. P., Davidowitz, G., and Dornhaus, A. (2020). Size variation does not act as insurance in bumble bees; instead, workers add weight in an unpredictable environment. Anim. Behav. 170, 99–109. doi: 10.1016/j.anbehav.2020.10.018
Keller, A., Danner, N., Grimmer, G., Ankenbrand, M., von der Ohe, K., von der Ohe, W., et al. (2015). Evaluating multiplexed next-generation sequencing as a method in palynology for mixed pollen samples. Plant Biol. 17, 558–566. doi: 10.1111/plb.12251
Keller, A., Hohlfeld, S., Kolter, A., Schultz, J., Gemeinholzer, B., and Ankenbrand, M. J. (2020). BCdatabaser: On-the-fly reference database creation for (meta-)barcoding. Bioinformatics 36, 2630–2631. doi: 10.1093/bioinformatics/btz960
Kenđel, A., and Zimmermann, B. (2020). Chemical analysis of pollen by FT-Raman and FTIR spectroscopies. Front. Plant Sci. 11:352. doi: 10.3389/fpls.2020.00352
Kenna, D., Cooley, H., Pretelli, I., Ramos Rodrigues, A., Gill, S. D., and Gill, R. J. (2019). Pesticide exposure affects flight dynamics and reduces flight endurance in bumblebees. Ecol. Evol. 9, 5637–5650. doi: 10.1002/ece3.5143
Klaus, F., Tscharntke, T., Bischoff, G., and Grass, I. (2021). Floral resource diversification promotes solitary bee reproduction and may offset insecticide effects – evidence from a semi-field experiment. Ecol. Lett. 24, 668–675. doi: 10.1111/ele.13683
Klein, A. M., Vaissière, B. E., Cane, J. H., Steffan-Dewenter, I., Cunningham, S. A., Kremen, C., et al. (2007). Importance of pollinators in changing landscapes for world crops. Proc. R. Soc. B Biol. Sci. 274, 303–313. doi: 10.1098/rspb.2006.3721
Konzmann, S., Kluth, M., Karadana, D., and Lunau, K. (2020). Pollinator effectiveness of a specialist bee exploiting a generalist plant—tracking pollen transfer by Heriades truncorum with quantum dots. Apidologie 51, 201–211. doi: 10.1007/s13592-019-00700-0
Lämsä, J., Kuusela, E., Tuomi, J., Juntunen, S., and Watts, P. C. (2018). Low dose of neonicotinoid insecticide reduces foraging motivation of bumblebees. Proc. R. Soc. B Biol. Sci. 285:20180506. doi: 10.1098/rspb.2018.0506
Lihoreau, M., Chittka, L., and Raine, N. E. (2016). Monitoring flower visitation networks and interactions between pairs of bumble bees in a large outdoor flight cage. PLoS One 11:e0150844. doi: 10.1371/journal.pone.0150844
Linguadoca, A., Rizzi, C., Villa, S., and Brown, M. J. F. (2021). Sulfoxaflor and nutritional deficiency synergistically reduce survival and fecundity in bumblebees. Sci. Total Environ. 795:148680. doi: 10.1016/j.scitotenv.2021.148680
Machado, I. C., and Lopes, A. V. (2004). Floral traits and pollination systems in the Caatinga, a brazilian tropical dry forest. Ann. Bot. 94, 365–376. doi: 10.1093/aob/mch152
Mayack, C., and Boff, S. (2019). LD50 values may be misleading predictors of neonicotinoid toxicity across different bee species. Uludag Aricilik Derg. 19, 19–33. doi: 10.31467/uluaricilik.568251
McMurdie, P. J., and Holmes, S. (2013). Phyloseq: An R package for reproducible interactive analysis and graphics of microbiome census data. PLoS One 8:e61217. doi: 10.1371/journal.pone.0061217
Medrzyck, P., Hellström, S., Straw, E., Linguadoca, A., Jürison, M., Alaux, C., et al. (2021). Improved protocols for testing agrochemicals in bees. Available at: Deliverable D3.2. EU Horizon 2020 PoshBee Project, Grant agreement No. 773921.
Mundy-Heisz, K. A., Prosser, R. S., and Raine, N. E. (2022). Acute oral toxicity and risks of four classes of systemic insecticide to the common eastern bumblebee (Bombus impatiens). Chemosphere 295:133771. doi: 10.1016/j.chemosphere.2022.133771
Nityananda, V., and Chittka, L. (2021). Different effects of reward value and saliency during bumblebee visual search for multiple rewarding targets. Anim. Cogn. 24, 803–814. doi: 10.1007/s10071-021-01479-3
Nürnberger, F., Keller, A., Härtel, S., and Steffan-Dewenter, I. (2019). Honey bee waggle dance communication increases diversity of pollen diets in intensively managed agricultural landscapes. Mol. Ecol. 28, 3602–3611. doi: 10.1111/mec.15156
Oksanen, J., Simpson, G. L., Blanchet, F. G., Kindt, R., Legendre, P., Minchin P. R., et al. (2022). vegan: Community Ecology Package. R Package Version 2.6-2. Available online at: https://cran.r-project.org/package=vegan
Ollerton, J., Winfree, R., and Tarrant, S. (2011). How many flowering plants are pollinated by animals?. Oikos 120, 321–326. doi: 10.1111/j.1600-0706.2010.18644.x
Osborne, J. L., Martin, A. P., Carreck, N. L., Swain, J. L., Knight, M. E., Goulson, D., et al. (2008). Bumblebee flight distances in relation to the forage landscape. J. Anim. Ecol. 77, 406–415. doi: 10.1111/j.1365-2656.2007.01333.x
Pope, N. S., and Jha, S. (2018). Seasonal food scarcity prompts long-distance foraging by a wild social bee. Am. Nat. 191, 45–57. doi: 10.1086/694843
Prado, A., Pioz, M., Vidau, C., Requier, F., Jury, M., Crauser, D., et al. (2019). Exposure to pollen-bound pesticide mixtures induces longer-lived but less efficient honey bees. Sci. Total Environ. 650, 1250–1260. doi: 10.1016/j.scitotenv.2018.09.102
R Core Team (2018). R: A Language and Environment for Statistical Computing. Vienna: R Foundation for Statistical Computing. Available online at: https://www.r-project.org/
Raine, N. E. (2018). Pesticide affects social behavior of bees. Science 362, 643–644. doi: 10.1126/science.aav5273
Rognes, T., Flouri, T., Nichols, B., Quince, C., and Mahé, F. (2016). VSEARCH: A versatile open source tool for metagenomics. PeerJ. 4:e2584. doi: 10.7717/peerj.2584
Sagwe, R. N., Peters, M. K., Dubois, T., Steffan-Dewenter, I., and Lattorff, H. M. G. (2021). Pollinator supplementation mitigates pollination deficits in smallholder avocado (Persea americana Mill.) production systems in Kenya. Basic Appl. Ecol. 56, 392–400. doi: 10.1016/j.baae.2021.08.013
Schmolke, A., Galic, N., Feken, M., Thompson, H., Sgolastra, F., Pitts-Singer, T., et al. (2021). Assessment of the Vulnerability to Pesticide Exposures Across Bee Species. Hoboken, NJ: John Wiley & Sons, Ltd. doi: 10.1002/etc.5150
Sickel, W., Ankenbrand, M. J., Grimmer, G., Holzschuh, A., Härtel, S., Lanzen, J., et al. (2015). Increased efficiency in identifying mixed pollen samples by meta-barcoding with a dual-indexing approach. BMC Ecol. 15:20. doi: 10.1186/s12898-015-0051-y
Siviter, H., Brown, M. J. F., and Leadbeater, E. (2018). Sulfoxaflor exposure reduces bumblebee reproductive success. Nature 561, 109–112. doi: 10.1038/s41586-018-0430-6
Siviter, H., Scott, A., Pasquier, G., Pull, C. D., Brown, M. J. F., and Leadbeater, E. (2019). No evidence for negative impacts of acute sulfoxaflor exposure on bee olfactory conditioning or working memory. PeerJ. 7:e7208. doi: 10.7717/peerj.7208
Spaethe, J., Tautz, J., and Chittka, L. (2001). Visual constraints in foraging bumblebees: Flower size and color affect search time and flight behavior. Proc. Natl. Acad. Sci. U. S. A. 98, 3898–3903. doi: 10.1073/pnas.071053098
Stanley, D. A., Garratt, M. P. D., Wickens, J. B., Wickens, V. J., Potts, S. G., and Raine, N. E. (2015a). Neonicotinoid pesticide exposure impairs crop pollination services provided by bumblebees. Nature 528, 548–550. doi: 10.1038/nature16167
Stanley, D. A., Smith, K. E., and Raine, N. E. (2015b). Bumblebee learning and memory is impaired by chronic exposure to a neonicotinoid pesticide. Sci. Rep. 5, 1–10. doi: 10.1038/srep16508
Stanley, D. A., and Raine, N. E. (2016). Chronic exposure to a neonicotinoid pesticide alters the interactions between bumblebees and wild plants. Funct. Ecol. 30, 1132–1139. doi: 10.1111/1365-2435.12644
Stanley, D. A., Russell, A. L., Morrison, S. J., Rogers, C., and Raine, N. E. (2016). Investigating the impacts of field-realistic exposure to a neonicotinoid pesticide on bumblebee foraging, homing ability and colony growth. J. Appl. Ecol. 53, 1440–1449. doi: 10.1111/1365-2664.12689
Straub, F., Orih, I. J., Kimmich, J., and Ayasse, M. (2021). Negative effects of the neonicotinoid Clothianidin on foraging behavior and antennal sensitivity in two common pollinator species, Osmia bicornis and Bombus terrestris. Front. Ecol. Evol. 9:697355. doi: 10.3389/fevo.2021.697355
Straub, L., Strobl, V., Bruckner, S., Camenzind, D. W., Van Oystaeyen, A., Wäckers, F., et al. (2022). Buffered fitness components: Antagonism between malnutrition and an insecticide in bumble bees. Sci. Total Environ. 833:155098. doi: 10.1016/j.scitotenv.2022.155098
Switzer, C. M., and Combes, S. A. (2016). The neonicotinoid pesticide, imidacloprid, affects Bombus impatiens (bumblebee) sonication behavior when consumed at doses below the LD50. Ecotoxicology 25, 1150–1159. doi: 10.1007/s10646-016-1669-z
Tamburini, G., Pereira-peixoto, M., Borth, J., Lotz, S., Wintermantel, D., Allan, M. J., et al. (2021a). Fungicide and insecticide exposure adversely impacts bumblebees and pollination services under semi-field conditions. Environ. Int. 157:106813. doi: 10.1016/j.envint.2021.106813
Tamburini, G., Wintermantel, D., Allan, M. J., Dean, R. R., Knauer, A., Albrecht, M., et al. (2021b). Sulfoxaflor insecticide and azoxystrobin fungicide have no major impact on honeybees in a realistic-exposure semi-field experiment. Sci. Total Environ. 778:146084. doi: 10.1016/j.scitotenv.2021.146084
Theodorou, P., Radzevičiūtė, R., Settele, J., Schweiger, O., Murray, T. E., and Paxton, R. J. (2016). Pollination services enhanced with urbanization despite increasing pollinator parasitism. Proc. R. Soc. B Biol. Sci. 283:20160561. doi: 10.1098/rspb.2016.0561
Tison, L., Rößner, A., Gerschewski, S., and Menzel, R. (2019). The neonicotinoid clothianidin impairs memory processing in honey bees. Ecotoxicol. Environ. Saf. 180, 139–145. doi: 10.1016/j.ecoenv.2019.05.007
Tong, L., Nieh, J. C., and Tosi, S. (2019). Combined nutritional stress and a new systemic pesticide (flupyradifurone, Sivanto®) reduce bee survival, food consumption, flight success, and thermoregulation. Chemosphere 237:124408. doi: 10.1016/j.chemosphere.2019.124408
Tosi, S., Burgio, G., and Nieh, J. C. (2017). A common neonicotinoid pesticide, thiamethoxam, impairs honey bee flight ability. Sci. Rep. 7:1201. doi: 10.1038/s41598-017-01361-8
United States Environmental Protection Agency. (2016). 2016 Addendum to the Environmental Fate and Ecological Risk Assessment for Sulfoxaflor Registration. Washington, DC: United States Environmental Protection Agency.
Vaudo, A. D., Farrell, L. M., Patch, H. M., Grozinger, C. M., and Tooker, J. F. (2018). Consistent pollen nutritional intake drives bumble bee (Bombus impatiens) colony growth and reproduction across different habitats. Ecol. Evol. 8, 5765–5776. doi: 10.1002/ece3.4115
Watrous, K. M., Duennes, M. A., and Woodard, S. H. (2019). Pollen diet composition impacts early nesting success in queen bumble bees Bombus impatiens Cresson (Hymenoptera: Apidae). Environ. Entomol. 48, 711–717. doi: 10.1093/ee/nvz043
White, T. J., Bruns, T., Lee, S., and Taylor, J. W. (1990). “Amplification and direct sequencing of fungal ribosomal RNA genes for phylogenetics,” in PCR Protocols: A Guide to Methods and Applications, eds M. A. Innis, D. H. Gelfand, J. J. Sninsky, and T. J. White (New York, NY: Academic Press), 315–322.
Whitehorn, P. R., Wallace, C., and Vallejo-Marin, M. (2017). Neonicotinoid pesticide limits improvement in buzz pollination by bumblebees. Sci. Rep. 7:15562. doi: 10.1038/s41598-017-14660-x
Williamson, S. M., and Wright, G. A. (2013). Exposure to multiple cholinergic pesticides impairs olfactory learning and memory in honeybees. J. Exp. Biol. 216, 1799–1807. doi: 10.1242/jeb.083931
Wilson, R. S., Keller, A., Shapcott, A., Leonhardt, S. D., Sickel, W., Hardwick, J. L., et al. (2021). Many small rather than few large sources identified in long-term bee pollen diets in agroecosystems. Agric. Ecosyst. Environ. 310:107296. doi: 10.1016/j.agee.2020.107296
Keywords: Bumble terrestris, flight dynamics, flower handling abilities, pollen metabarcoding, ruderal plants, bee size
Citation: Boff S, Keller A, Raizer J and Lupi D (2022) Decreased efficiency of pollen collection due to Sulfoxaflor exposure leads to a reduction in the size of bumble bee workers in late European summer. Front. Ecol. Evol. 10:842563. doi: 10.3389/fevo.2022.842563
Received: 23 December 2021; Accepted: 25 July 2022;
Published: 08 September 2022.
Edited by:
Michael Hrncir, University of São Paulo, BrazilReviewed by:
Nigel E. Raine, University of Guelph, CanadaJohanne Brunet, United States Department of Agriculture, United States
Copyright © 2022 Boff, Keller, Raizer and Lupi. This is an open-access article distributed under the terms of the Creative Commons Attribution License (CC BY). The use, distribution or reproduction in other forums is permitted, provided the original author(s) and the copyright owner(s) are credited and that the original publication in this journal is cited, in accordance with accepted academic practice. No use, distribution or reproduction is permitted which does not comply with these terms.
*Correspondence: Samuel Boff, samboff@gmail.com
†ORCID: Samuel Boff, orcid.org/0000-0003-2649-3619; Alexander Keller, orcid.org/0000-0001-5716-3634; Josué Raizer, orcid.org/0000-0002-3623-4437; Daniela Lupi, orcid.org/0000-0002-9467-2419