- 1Laboratório de Etologia, Ecologia e Evolução de Insetos Sociais, Departamento de Psicologia Experimental, Instituto de Psicologia, Universidade de São Paulo, São Paulo, SP, Brazil
- 2Programa de pós-graduação em Psicologia Experimental, Instituto de Psicologia, Universidade de São Paulo, São Paulo, SP, Brazil
- 3Laboratoire d'Ethologie Expérimentale et Comparée (LEEC UR4443), Université Sorbonne Paris Nord, Villetaneuse, France
Since Tinbergen’s seminal contribution in 1963, ethology has blossomed as a multifaceted research field. Sixty years later, uncountable articles followed the four questions proposed as necessary for understanding animal behaviour, and they culminated in the segmentation of subareas which communicate little among themselves. Foraging in ants is one example where this division happened, despite the clear need to integrate results obtained from different approaches. We chose this research subject to revise the literature, relating the main results to the relevant level of explanation in Tinbergen’s four questions theoretical framework. Through such revision, we aim to foster the integration of different approaches and to bring to light how this can clarify how we understand foraging behaviour, sixty years after Tinbergen’s initial proposition.
1 Introduction
In his seminal paper ‘On Aims and Methods of Ethology’ (1963), Nikolaas Tinbergen defined ethology as the biological study of behaviour (Tinbergen, 1963). This paper is famously known for how Tinbergen clarifies the contours of ethology by proposing four different levels of analysis of animal behaviour: causes (mechanisms), ontogeny (development), evolution and function. Mechanisms and development are proximate levels of explanation of behaviour; that is, they target the role of organism development on the construction of the behavioural repertoire and what triggers behaviour expression here and now. Complementarily, functional and evolutionary explanations focus on evolutionary times over generations; they aim at unravelling the role of behaviour in evolutionary processes and how it has been selected over time by particular selection pressures (Tinbergen, 1963).
Tinbergen also discusses the need for “modern ethology” to study behaviour at all levels of biological integration, from molecules to supra-individual levels such as societies. As ants represent one of the best examples of superorganisms, they are a very adequate model to evaluate behaviour at different levels. That is, molecular constituents of ants can be evaluated, but also whole ants as individual organisms and ultimately the colony level. Sixty years after Tinbergen’s seminal article and fifty years after the Nobel Prize was awarded to three ethologists (Karl von Frisch, Nikolaas Tinbergen, and Konrad Lorenz), it is momentous to reflect upon the legacy of this highly integrative and multifaceted approach to the study of behaviour. We focus on ant foraging behaviours because it is a much, albeit still incompletely, studied subject (e.g., reviewed in Jaffe, 1984; Beckers et al., 1989; Traniello, 1989; Lanan, 2014; Reeves and Moreau, 2019).
Our revision aims to: 1) provide a rapid outlook of the conceptual framework proposed by Tinbergen for clarifying the levels of analysis of animal behaviour, and advocate that they are still valuable to integrate the most modern research advances, and 2) show how those levels of explanation proposed by Tinbergen can be studied in practice and the need for their integration using foraging behaviours in an incredibly diverse animal group such as ants. First, we define the central concepts of our review (behaviour, foraging, etc.). Then we summarise the main characteristics of foraging in ants to discuss afterwards the findings in the literature that permeate Tinbergen’s four questions. Finally, we propose some lines of thoughts on the directions future works could follow.
2 What is behaviour and how to study it?
2.1 What is behaviour?
Behaviour is a non-trivial concept that can admit a variety of definitions depending on the discipline, the theoretical approach and even the biological model of predilection (Levitis et al., 2009). Explicit and truly operational definitions are lacking even in the very ethological literature. Here we propose to define behaviour as observable movements and postures that allow individuals to act on their environment and therefore maintain an adequate internal state for survival, growth, and reproduction. When behaviour is often presented as the “motor output” triggered by the coordinated analysis of stimuli present in the environment (see for example Hogan, 2021), we believe that it is of utmost importance to stress the agency property of biological organisms (Gomez-Marin and Ghazanfar, 2019). From an operational point of view, agentivity is a crucial parameter to distinguish between behaviour, which is what an animal does, and any other movement or posture of the animal that is merely due to the action of the environment. We can agree with the definition proposed by Levitis et al. (2009): “behaviour is the internally coordinated responses (actions or inactions) of whole living organisms (individuals or groups) to internal and/or external stimuli, excluding responses more easily understood as developmental changes”. We however stress that the notion of “collective behaviour” is a metaphor referring to the emergence and maintenance of collective patterns structuring (generally by means of self-organised process) the behaviour of individuals within the group. We propose that the determinants of behaviour are rooted in the interaction between the individual organism and its environment.
Behaviour should not be seen as a mere reaction of organisms to pre-existing stimuli in the environment, but rather as the way an organism actively regulates its interactions with its environment at the organismic level, including how it controls its perception of relevant features of its surroundings (Pellis and Pellis, 2021). Then behaviour can be described as an emergent property of the functional loops linking action to perception (Varela et al., 1991; Thompson, 2010).
2.2 What are mechanisms, ontogeny, function and evolution?
If we endeavour to propose an integrated approach to animal behaviour, we must first define how we envisage the four questions in this review. The causes encompass all mechanisms responsible for the expression of behaviour here-and-now, i.e., the internal state and processes of the animal, and the environmental context that triggers, support and/or orientate this behavioural expression. This includes the sensory, neural, physiological and morphological constituents of behaviour within the organism (for example, the mechanisms approach can be taken by analysing how pheromones affect foraging behaviours; Ma et al., 2019). Some of these mechanisms, such as genetic and epigenetic factors, are of utmost importance since they can act as supports of inheritance for behavioural traits. Other processes are associated with potential modifications of the behavioural response, such as sensory modification, learning and experienced-based mechanisms, and motivational processes (Lehrman, 1953). This means that the internal state of the animal is also an object of study. Although not a behaviour per se, it is affected by the interactions of the animal with its environment. Reciprocally, it affects all aspects of behaviour since it influences the animal’s point of view on its surroundings (d’Ettorre et al., 2017; Hogan, 2021). This stand sharply contrasts with the “anti-subjective” stances of Tinbergen in 1963 (e.g., p.413), which must be properly replaced in its historical context. Nowadays most academic textbooks in Ethology contain sections dedicated to Cognitive ethology, or at least referring to the link between cognition and behaviour (e.g. Shettleworth, 2010; Bolhuis et al., 2022). This also stands for monographies on insects, especially for Hymenoptera (e.g. Wehner, 2020; Chittka, 2022). Noteworthy, we propose that after almost fifty years of cognitive ethology (Griffin, 1976; Allen and Bekoff, 1997; Andrews, 2015; Bolhuis et al., 2022) even subjective states of animals can nowadays be scientifically studied and should be considered in explanations of behaviour when possible (for recent reviews see Birch et al., 2020; Paul et al., 2020). For example the standard method of cognitive judgement bias test for assessing emotion-like processes in non-human animals, where the behavioural reaction of an animal confronted to an ambiguous situation depends on its affective state (for a review see Roelofs et al., 2016), can be adapted for insects (Perry and Baciadonna, 2017). How conscious such internal states can be obviously remains a hard and vividly discussed question (e.g. Baracchi et al., 2017). It will undoubtedly benefit from close collaboration between cognitive scientists and philosophers (e.g. Veit, 2023).
The second of Tinbergen’s proximal questions, the ontogeny of behaviour, is intimately linked to the mechanisms of behaviour. Following Tinbergen (1963), one needs to consider the historicity of all living organisms at three intermingled timescales: (i) how evolution shaped organisms and led to the inheritance of behavioural traits (including both genetic and non-genetic mechanisms; Bonduriansky and Day, 2018; Jablonka and Lamb, 2020), (ii) how contingent individual experiences of the organism influenced its development (Spirov et al., 2021) and (iii) the actual expression of behaviour here-and-now. In this review, we will refer to this question as development, since the focus of Tinbergen on ontogeny did not consider all aspects of behavioural development, but rather insisted on the changes that occurred in the “behavioural machinery” during the animal’s development. Nowadays, researchers on behaviour consider this subject to be broader, including how experience and learning deeply modify the expression of the behaviours of individuals even after they have developed to their mature expression (Hogan and Bolhuis, 2005). Development is thus seen as an interaction process between the individual and the environment (Lehrman, 1953). This allows us to understand at a proximal level the great flexibility of behaviour and the decision process associated with it. Examples of studies that analyse behaviour at the developmental level are those that evaluate the effect of age and experience on the performance of foragers (Beshers and Fewell, 2001; Franklin et al., 2012).
The two distal, or ultimate questions of Tinbergen (1963), are related to the function and evolution of behaviour. The study of function, or current utility of behaviour (Bateson and Laland, 2013), aims at understanding its adaptive value, i.e., its consequences on individual fitness. It posits that behaviour is under the effect of natural selection as soon as: (i) its expression varies among individuals of a given population, (ii) it impacts individuals’ fitness and (iii) it can be transmitted through one of the various inheritance supports (Bonduriansky and Day, 2018; Jablonka and Lamb, 2020). This can be done by analysing the costs and benefits linked to a particular foraging strategy (Fewell et al., 1996). The study of evolution seeks understanding from a historical point of view how the different behaviours came to be selected in species and populations. Phylogenetic reconstructions of how foraging strategies emerged and diverged in the Formicidae group are an example of this type of analysis (Reeves and Moreau, 2019). Although it looks at evolutionary and genetic/epigenetic hereditary processes, several authors advocate that cultural processes and phenomena such as niche construction should be included in this question (Laland et al., 2016). This also stresses the fact that behaviour is not only a result of evolutionary processes but also plays a key role in evolutionary mechanisms. This circular causality (as for the feedback loop we underlined between behaviour and the perception of the world) has been put forward in recent advances in evolutionary sciences, starting with the field of EvoDevo (Laland et al., 2013). It is thus clear that the question of the evolution of behaviour is not independent of the proximal questions addressed above since it is both a cause and a consequence of the observed behaviours.
From the 1960s onwards, ethology branched out, and ethologists no longer saw themselves as researchers who dealt with the same problem using different approaches (Griffiths, 2007). Depending on the kind of study, explanations may be limited to proximate (in fields of behavioural analysis, cellular and molecular approaches to behaviour) or ultimate levels (typical in behavioural ecology) (MacDougall-Shackleton, 2011). The behavioural studies also got used to not specifying which approach would be utilised in many cases, despite several authors having highlighted potential problems with these blurred distinctions (Hogan, 2015). Thus, there seems to be a mismatch between the theoretical foundation and the research in ethology.
3 Understanding ant’s foraging behaviour
3.1 Ants’ foraging behaviour
Foraging is a suite of behaviours that together allow an animal to obtain food resources. It is traditionally associated with the hunger motivational system, and it ultimately permits self-maintenance through the resources obtained. Foraging behaviours are not restricted to food consumption but also encompass all the aspects associated with the localization, exploitation, food processing and sometimes stocking of resources. Consequently, they include a wide diversity of behaviours that can be articulated in a variety of behavioural strategies. In social insects, which display a social division of labour, foraging is usually performed to provide food to individuals who do not forage by themselves. In this case, individual behaviours should then be explained both at the individual and collective levels (e.g., social regulations and auto-organising phenomena).
There are several reasons to study foraging, such as the obvious adaptive function of these behaviours, their enormous diversity (such as different foraging strategies) and complexity, and the ease of being analysed analytically and mathematically which generated numerous theories (Griffiths, 2007). Unsurprisingly, foraging is probably one of the most studied themes in ant species, whose behavioural repertoires are notably vast. Extremely remarkable phenomena can be observed in many ants, for example, the massive recruitment of thousands of workers to a resource (Reeves and Moreau, 2019). Also, ants occupy diverse ecological niches and present feeding habits that range from generalist predators and detritivores to fungi cultivator specialists (Dornhaus and Powell, 2010). Food acquisition, done by a small part of group members to feed the whole colony, requires the resolution of several problems that vary according to species, ecology, life history traits, and immediate context (Traniello, 1989). Generally, this activity consists in the discovery, retrieval, and transportation of the food resource to the colony, where most of the time, other individuals, mainly the immatures, consume it.
Depending on the studies, the same term “foraging behaviour” can refer to a variety of behavioural items, studied at distinct levels of organisation. We here focus on behaviours concerning three distinct moments of foraging activity: the decision to start foraging, searching behaviours, and food recovery strategies/behaviours. In ants, many variables can alter the internal state of individual foragers and trigger foraging initiation (Figure 1A). These variables can be internal (e.g., response threshold) or external (e.g., social environment). After that, individuals use a variety of search strategies, information sources and cues to find food. For example, desert ants (Cataglyphis sp.) use sun light, idiothetic and visual odometers, path integration, learning of visual scenes and other strategies based on multiple sensory modalities to retrieve food resources (Wehner, 2020). Finally, food items are rescued and brought to the nest in different ways. Individual or solitary foraging refers to a situation where no cooperation or communication occurs during the discovery, capture, and transporting of food resources. Each forager then leaves the nest, searches for food, and transports it back independently (Jaffe, 1984). In collective or group foraging, usually, one ant performs the scouting role and, after finding food, goes back to the nest to recruit nestmates, which involves some kind of communication (e.g., using pheromone or tandem communication; Beckers et al., 1989).
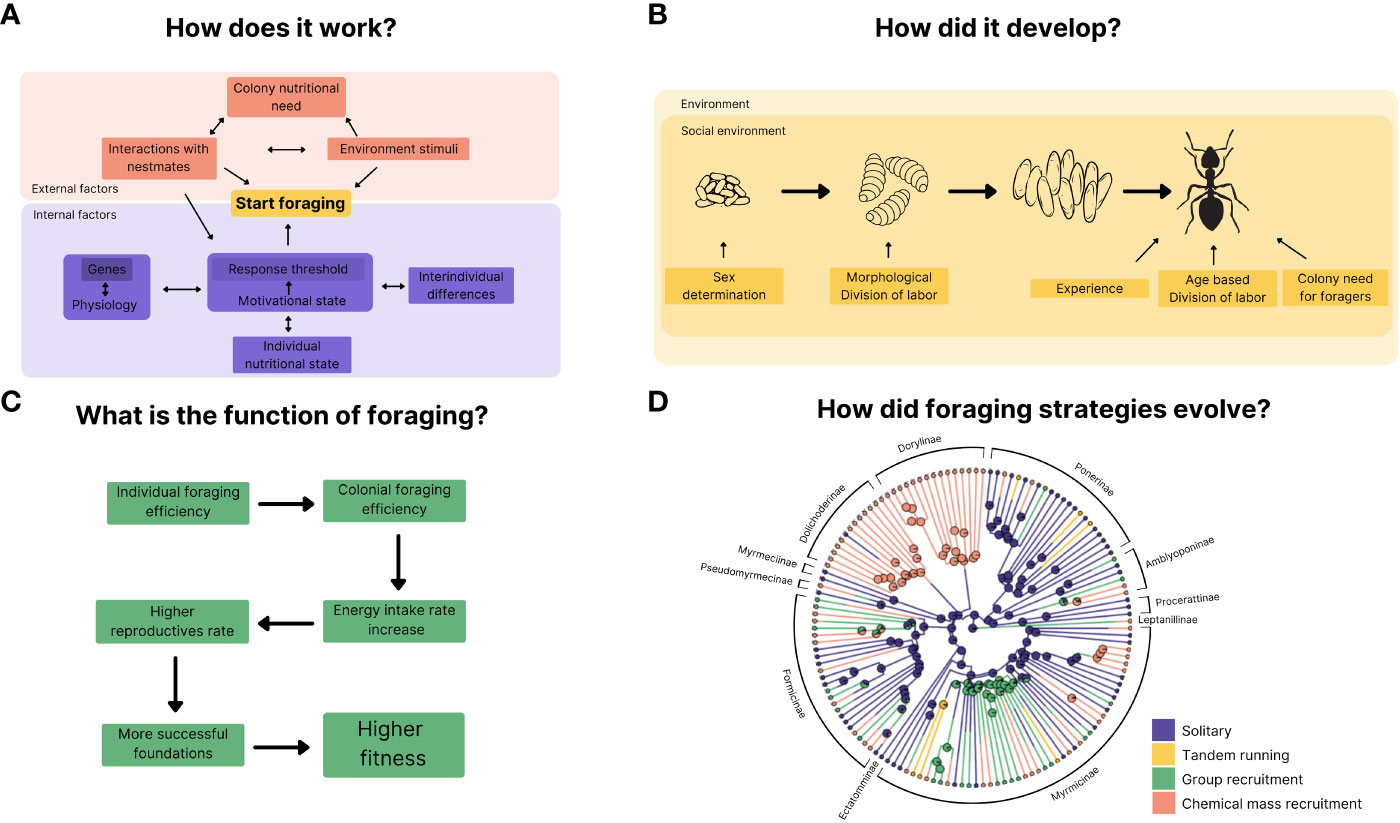
Figure 1 Tinbergen’s four questions applied to factors related to foraging in ants. (A) illustrates known mechanisms that start foraging. (B) deals with moments of individual development which allow or alter the occurrence of foraging. (C) exhibits how the efficient acquisition of food was selected and leads to fitness increase at individual and colonial level, and (D) is a cladogram of how the foraging strategies possibly diversified along the Formicidae genera. We present the different subfamilies to show how diverse the strategies our in them. The pie charts along the tree are the results of the reconstruction of ancestral states (modified from Reeves and Moreau, 2019).
The most common types of recruitment are tandem running, group and mass recruitment by pheromone trails (Jaffe, 1984; Beckers et al., 1989). Tandem running involves mechanical as well as chemical signals (Hölldobler et al., 1974). During a tandem run, one inexperienced ant (follower) follows an experienced ant (leader) closely, touching its gaster with its antennae. If this contact is interrupted, the ant leader stays in place and waits for the follower to catch up (Richardson et al., 2007). In group recruitment, one individual can recruit a small group of nestmates that keep physical contact with it (Lanan, 2014). Mass recruitment, instead, happens when pheromones are deposited from the nest to a target place; thus, several ants can be indirectly recruited to the destination (Lanan, 2014). Foraging by swarm or column happens in army ants without scouts and consists of many individuals going out together (forming a raid) in search of food (Lanan, 2014).
3.2 Mechanisms
The quest for understanding how behaviours operate is old and can already be found in Aristotle’s writings (Bateson and Laland, 2013). In modern ethology, however, the mechanism evaluation lost momentum by not being able to satisfactorily explain the adaptive value of behaviours as investigated by behavioural ecology (Griffiths, 2007). Here, we consider known and hypothesised causes in different moments, levels of biological organisation to present a broad approach with many examples of mechanisms that initiate, operate, and finish foraging behaviours in ants (Figure 1A). It is worth mentioning that, here, causal mechanisms are taken on separately from each other and from the three other levels of explanation (development, function and evolution) for the sake of clarity. However, foraging depends on a set of factors which present intersections, do not act like separate or exclusionary and has intertwinement with ultimate factors (view introduction session). We organise this section following biological organization levels from infra-organism molecules to supra-organism ecosystems.
3.2.1 Internal factors: cognition, internal states and physiological correlates
Using molecular and genetic tools to analyse the mechanisms behind behaviour expression began with the Drosophila flies. Initially, a gene responsible for foraging regulation in the larval stage was identified in this species and named the ‘for gene’ (Sokolowski, 1980). This gene regulates feeding and foraging indirectly by affecting metabolic response to stress (Dason et al., 2020). The for gene in ants has an influence on morphological and age-dependent division of labour. It also is involved in a cascade of effects at an internal level which has important consequences in the modulation of foraging behaviour and the way individuals interact with the environment (Lucas and Ben-Shahar, 2021).
Despite being the most studied gene in relation with foraging, other genes and modifications of genetic expression affect behaviour or cognition and, thus, food search. An indication of this comes from pioneering studies with allozymes (Robinson and Page, 1989) or artificial selection (Page et al., 1995), showing in polyandric species like the honeybee Apis mellifera (Linnaeus, 1758) that paternal lines have different probabilities for foraging after different resources. Foraging behaviours can be affected by a variety of environmental and physiological factors that modulate some genes expression in a more or less flexible way — for example, age and light (Yilmaz et al., 2016), pheromones (Ma et al., 2019), and chronotype (Das and de Bekker, 2022). This highlight the Omic shift for the scientific study of the molecular bases of behaviour where not only the sequence of genes but their expression and integration in the complex and diverse molecular network are envisaged (Ghoul et al., 2017).
New techniques for evaluating global changes in gene expression will undoubtedly enhance our knowledge of the genetic architecture related with the foraging behaviour’s variability and diversity. For example, Friedman et al. (2020) evaluate two different foragers’ variable traits, dopamine to serotonin brain ratio and sensitivity to humidity. Then, they show that these traits are associated with gene expression differences notably in neurotransmitter receptor signalling and metabolic functions. Most studies, however, focused more on the gene expression differences linked to transition to a foraging role (Glastad et al., 2020). In the latter study, an epigenetic factor linked to chromatin regulation (CoREST) is shown to influence transition of major workers of Camponotus floridanus (Buckley, 1866) to the forager role, and associated gene expression differences appear to be linked to JH and ecdysone signalling, both hormones known to influence division of labour in many species.
When genes act in the background of behavioural systems, hormones and neurotransmitters are the principal molecular components that can have direct and rapid effects on animal behaviour. Juvenile hormone and vitellogenin are responsible for radical changes in development, and they are associated with behavioural changes such as caste, reproduction, and task allocation (Guidugli et al., 2005; Norman and Hughes, 2016; Jeanson, 2019; Prato et al., 2021). In general, one identified category of circulating molecules that probably influences answers the most in insects, in a specific way or not, is neuroamines or neurohormones, such as octopamine, tyramine, dopamine, and serotonin (Roeder, 2005). These molecules can exert general or specific, central or peripheral effects, and can modulate social traits like reproductive division of labour and task allocation in social insects (Barron et al., 2002; Sasaki et al., 2021). In bees, that are closely related to ants, they have been involved in recruitment for food behaviours (Barron et al., 2007). They could also be involved in the motivation and/or evaluative processes that trigger and sustain foraging behaviours, maybe being at the base of some kind of emotion-like states (Bateson et al., 2011; Solvi et al., 2016; but see Baracchi et al., 2017). Despite the more detailed studies demonstrating the practical implication of these molecules, it can nevertheless be challenging to go beyond the correlation (but see some experimental approaches such as Friedman et al., 2018).
Neuroimaging and staining tools also allow the study of neural and physiological correlates of foraging behaviour. As an illustration, Fetter-Pruneda et al. (2021) show in Ooceraea biroi (Forel, 1907) the association between an increased level of inotocin, the insect orthologue of oxytocin/vasopressin related neuropeptides, and the forager role. They also show using pharmacological treatments a context and age dependent effect of this neuropeptide on foraging. Physiology can thus be seen as both a cause and a consequence of behaviour, illustrating once again the circular causality between physiology and behavioural expression. Nutritional state, for example, which is a direct consequence of foraging behaviour success, is a physiological variable that can directly affect the behavioural response threshold of an individual. It is also as such an important parameter to consider when looking at the motivational state of the individual, a subject that we will consider next.
Motivational models predict that positive and negative events can alter the individuals’ foraging behaviour and decisions, including immediate success, long-term experience, presence of pheromones, presence of predators and time looking for prey (Waage, 1979). Such events can lead to learn some characteristics of the environment that are correlated with food, therefore allowing them to trigger or direct the expression of future behaviours, be it directly or not (e.g., by altering motivation). For example, the theoretical hypothesis of a decremental mechanism postulates that finding food decreases motivation to explore and consequently results in a higher chance of leaving the food patch. This hypothesis is likely to occur in animals with central point foraging and has been empirically confirmed for Bombus genus bees and parasitoid wasps (Driessen and Bernstein, 1999; Lefebvre et al., 2007).
It is well-known that insects and especially ants can learn cues leading to food sources (Wehner, 2020). Behaviour is a goal-directed process and knowing (without postulating any conscious state here) that some food can be found could participate to trigger departure for foraging. Some kind of expectation processes, based on the representation of some food properties (qualitative or quantitative), can also influence motivation for foraging and even for food-consumption. In ants and bees, individuals who expect to find low-quality sources show high acceptance of medium-quality resources. Instead, those who previously found high-quality sources display low acceptance of medium-quality items (Bitterman, 1975; Wendt et al., 2019). Interestingly, Wendt et al. (2019) showed that such modulation of behaviour was associated with processes at the cognitive level rather than mere sensory adjustments. They also showed that expectation could be modified inside the nest through trophallactic interactions with returning foragers. Many other elements — spotting of predators (Ings and Chittka, 2009; Dawson and Chittka, 2014) or competitors (Tanner, 2009), the distance between resource and nest (Silva et al., 2021) — influence motivational states and individual cognitive assessment of the situation and, hence, foraging decisions. The individual nutritional state can for example affect foraging behaviour by increasing the foraging motivation and effort of the starving individuals. These mechanisms lessen the foragers’ response threshold to food-related cues, making individuals respond faster to stimuli related to foraging. In Temnothorax albipennis (Curtis, 1854), less fed individuals make significantly more foraging efforts (i.e., give rise to clustered bouts of activity) in response to increased demand (Robinson et al., 2009).
3.2.2 Foraging at the group level
A hallmark of insect societies is division of labour, also known as task allocation among colony members. One subset of the main models of work self-organisation in social insects — built on both theoretical and empirical studies — proposes that nestmates belonging to different behavioural groups (e.g., nurses or foragers) show different response thresholds when faced with tasks-related stimuli (e.g., larvae or food odours; Beshers and Fewell, 2001; Duarte et al., 2012). As a result, workers collectively exposed to a variety of stimuli will show varying probability to responding with a given behaviour (Bonabeau et al., 1998; Duarte et al., 2012; Leitner and Dornhaus, 2019). As an illustration, experimental studies have shown that foragers present low response thresholds and therefore respond first to stimuli related to food and external environment, like the response to sucrose or light (Ben-Shahar, 2005; Perez et al., 2013; Detrain and Prieur, 2014).
In social insects, behavioural differences can also be traced at a colonial level. Colonies can indeed differ consistently from others in task performances and activity regulation (Webster and Ward, 2011). Such variations of behavioural responses that are consistent across time and contexts are referred to as personality traits (Stamps, 2016). Gordon et al. (2011) for instance showed that, in Pogonomyrmex barbatus (Smith, 1858), colonies can vary in the scouts rate and the number of foragers leaving the nest. These inter-colony differences persist each year (Gordon et al., 2013), thus validating the characterization as colonial personality across time. Colonies of Pogonomyrmex occidentalis (Cresson, 1865) show consistent variation in the temporal pattern and thermal tolerance during foraging (Cole et al., 2010). Colonies of Temnothorax rugatulus (Emery, 1895) vary consistently in foraging efforts (Bengston and Dornhaus, 2014). In Lasius niger (Linnaeus, 1758), colonies with greater exploratory levels exploit sources faster (Pasquier and Grüter, 2016). Despite being able to quantify traits to the colonial level, these results arise from a complex interaction of factors, one of them being the characteristics of the individuals that make up the group. Therefore, one weakness of these studies comes from the lack of hypotheses and tests of the mechanisms associated with such consistent differences and their permanence in natural environments. Another flaw is that most analyse personality traits over time but not across different contexts.
Colonial success also leads to the selection of individuals with specific traits. In that regard, personality on an individual level is one of the elements responsible for characteristics at the group level. However, it is hard to predict and evaluate experimentally how such collective properties can emerge from the association of many individuals with different traits. Today, three hypotheses could explain how individual personality acts towards building colonial personality: 1) colonies differ consistently in the personality average of their workers; 2) workers’ personality distribution, not average, varies between colonies, and 3) colonial personality does not emerge from workers’ personality but from consistent external limitations (Pinter-Wollman, 2012). As an example, the ingested volume threshold in L. niger is specific for each individual, independent from its size, and stays constant even with successive trips to the food source. There are also differences in the propensity to deposit trail pheromone, with some foragers never laying trails (Mailleux et al., 2005). Learning ability can also be correlated with the exploratory behaviour of foragers. In Camponotus aethiops (Latreille, 1798), more active explorers were slower to learn an odour than less active ones when harnessed in a conditioning maxilla-labium-extension paradigm (MALEr, Udino et al., 2017).
All these variations in behavioural traits probably shape the colonial phenotype. For example, dividing foragers into scouts and recruits, trail followers or innovators, leaves the colony more flexible to respond to the environmental conditions. Little is known about the ontogeny and the mechanisms responsible for personality differences at an individual level (Horna-Lowell et al., 2021). These differences can hamper the flexibility of the colonies and thus explain the differences in group fitness that collectively show different responses to the environment, notably in foraging.
Individual foragers also base their decision to forage on the information received from nestmates (social information). Information transfer can occur directly, mostly through antennal interactions, or indirectly through pheromone trails (Kolay et al., 2020). In P. barbatus the combination of these two processes stimulates foraging (Greene et al., 2013). Ants interact with conspecifics through antennal contacts, during which they identify the nestmate’s chemical profile of cuticular hydrocarbons (Greene and Gordon, 2003). Encounters between foragers coming in and out of the nest convey information on the identity of the nestmate, the type of food found, and the food source abundance. The rate at which successful foragers return to the nest entrance reflects the food type available and the time it takes to find food (Schafer et al., 2006). Foragers’ returning rate is the local information use by workers to regulate their own foraging effort, without the need to get direct access to actual food availability.
Social facilitation has been observed in several ant species where the return of successful foragers in the nest triggers the initiation of foraging bouts by nestmates (Holcoponera moelleri (Forel, 1912), Cogni and Oliveira, 2004; Dinoponera quadriceps (Kempf, 1971), Nascimento et al., 2012; Odontomachus bauri (Emery, 1892), Oliveira and Hölldobler, 1989; P. barbatus, Schafer et al., 2006). Decision-making models reported that the odds of a forager going out for food depend on its interactions with returning foragers (Greene et al., 2013; Pinter-Wollman et al., 2013). Foragers inside the nest engage in a series of antennal contacts with returning foragers. When the frequency of these contacts is high, ants are more likely to go out of the nest to forage. This mechanism was modelled successfully with a stochastic accumulation of contact numbers (Davidson et al., 2016). In social insects’ colonies, individuals only have access to local information depending on their nest position and the presence of nestmates in the vicinity (Mersch, 2016). Foragers lack direct knowledge on the colony’s nutritional needs. Thus, social communication is critical to regulating foraging activity.
Among all colony members, larvae are the most energy-demanding individuals to fulfil their development, and they usually are the end consumers of the collected resources. They could therefore play a key role to stimulate foraging by adult nestmates (Ulrich et al., 2016). Larvae can indeed signal their hunger level to workers (Cassill and Tschinkel, 1995; Creemers et al., 2003). Both mechanical and chemical signalling have been evidenced in ant larvae (Schultner and Pulliainen, 2020). Larvae number, size, and nutritional needs influence the workers’ foraging activity. For instance, the frequency of trophallaxis — mouth-to-mouth food transfer between colony members — is correlated to the larvae/worker ants’ proportion (Cassill and Tschinkel, 1999). In addition, workers respond to larvae number by increasing foraging activity and inhibiting ovarian activation in a progressive manner, especially in smaller colonies (Ulrich et al., 2016).
3.2.3 The role of environment
To overcome the uncertainty of a changing environment, animals must continuously get information on their surroundings (Czaczkes and Heinze, 2015). Sensory information, physiologic state, and memory in animals are integrated by the brain and impact how the allocation of foraging effort will be done between food patches. In that regard, learning allows animals to acquire, remember, and use crucial environmental information (Shettleworth, 2010). In central-place foragers, a range of cognitive processes allow efficient resource exploitation by learning and use of reliable predictors of food location and quality in the environment (Perry et al., 2017).
When information on past routes is recovered from memory and utilised by the same individual to retrieve new food items, this information is considered private. Insects can use a set of navigational strategies to orient, especially combining path integration and the learning of familiar scenes (Collett and Graham, 2004). If the cognitive structure of spatial information use is still a subject of debate (e.g., Menzel, 2023; Wehner et al., 2023; see also Clément et al., 2023) it clearly leads to robust and flexible spatial exploitation of resources. For instance, in D. quadriceps, the heterogeneous distribution of resources requires a detailed search over a known area. The more experienced ants are more flexible and capable of incorporating new information rapidly in their routes (Azevedo et al., 2021). Besides, social information transmission occurs when one individual utilises information left by its nestmates to locate and retrieve food. This can happen through cues, like the mere presence of nestmates at the food source or collisions with them during foraging (Pinter-Wollman et al., 2013; Avarguès-Weber and Chittka, 2014), and/or through signals, like the dance of bees or chemical trail recruitment (Hölldobler and Wilson, 1990; von Frisch, 1993).
Ants prioritise one information over another based on its details, accuracy, and reliability. Once again, we refer to any mechanism that can functionally lead to selective use or differential weighting of potential information, without any assumption on the cognitive level of the mechanism. As the reliability of a cue decreases, individuals tend to change their information use (Czaczkes et al., 2019). In L. niger, ants significantly express more trail-laying behaviour when mistakes occur in private information use or when colonies have low exploratory activity (Czaczkes and Heinze, 2015; Pasquier and Grüter, 2016). In Paraponera clavata (Fabricius, 1775), the use of pheromone trails is prevalent in inexperienced ants. In contrast, the experienced ones use private information from visual cues and make the trip with greater speed (Harrison et al., 1989). Interestingly, in this case, the chemical trail is for individual use and allows for the navigation in complex environments in three dimensions.
Prey availability is a crucial biotic factor in the environment and it alters foraging behaviours in several ways. Foraging intensity is intimately related to the presence of prey, its quality and distribution (Dornhaus and Powell, 2010). Species with non-linear recruitment (such as through pheromone trails) usually reach a consensus in choosing the better food source. However, because of the non-linear response of foragers (strong trails are disproportionately preferred), pheromone trail recruitment can lead to mistakes and lack of flexibility in decision-making (Sumpter and Beekman, 2003). Thus, it can take time to switch from exploiting an existent food source to a newly discovered one, even when the latter is more valuable. Notwithstanding, in L. niger, changes in resource location influence pheromone deposition, where workers increase pheromone deposition to the new source. Individuals that made an error also deposit more pheromones, and uncertain ants that were likely to make an error decrease pheromone deposition en route to the food source. Such behavioural regulations allow ants to communicate and make decisions in a changing environment and probably permit the exploitation of several food sources simultaneously (Czaczkes and Heinze, 2015). Solitary foraging or linear recruitment (such as tandem running) also lead to efficient foragers’ allocation at the colonial level toward the best food source, while maintaining some monitoring of other sources. An increase in the resource value can rapidly lead to the colony redirecting its foraging effort (Shaffer et al., 2013). Such collective regulations are fine-tuned since the behaviour of scouts finding food sources depends on the resource evaluation — the probability to actively exploit it and recruit nestmates is higher for good or more valuable food sources (Shaffer et al., 2013; Silva et al., 2021).
The presence of intra and interspecific predators or competitors inhibits foraging and can cause behavioural changes such as modification of foraging period and even dietary changes (Glaser et al., 2021; revised in Adams, 2016). Reactions of individual foragers to distinct food sources and social interactions allow food patches selection at the colonial level with respects to food quality and predation risk (Nonacs and Dill, 1990, 1991). However, to inform others about the dangers of a path to the food source, the forager needs to be able to communicate about a non-shared experience (with a non-knowledgeable worker), which they probably do using chemical (Nonacs, 1990). Mortality risk on a path to the food source can vary over time (Nonacs and Dill, 1991). Therefore, foragers must continuously sample several routes to gauge the current conditions (Hölldobler and Wilson, 1990). Their responses indicate flexibility in balancing multiple objects and different kinds of risk (Lessig and Nonacs, 2021).
Many abiotic environmental variables impact foraging activity in ants. Climatic conditions (temperature, humidity, luminosity, rainfall) directly affect the physiology of ants, which are ectothermic animals and have limited autoregulation capacity (Dunn et al., 2007). Therefore, there is a tendency for most species to avoid foraging in high temperatures with direct luminosity and low humidity, conditions which cause foragers’ desiccation and death (Traniello, 1989; Gordon, 2013; Friedman et al., 2019; but see Wehner, 2020). Iridomyrmex purpureus (Smith, 1858), for example, adjusts its foraging time to the colder hours of the day, climbs the grass to lower its temperature and forages only in shaded areas (Andrew et al., 2013). Ants can perceive these factors by approaching the nest chambers next to the exit (Pinter-Wollman et al., 2013). Thermal preference and tolerance are crucial factors to explain the abundance and richness of ant communities (Lasmar et al., 2021; Roeder et al., 2021). It is interesting to mention that some subordinate species manage to thrive because of their higher tolerance to hot temperature, as is the case of species of the genus Cataglyphis (Wehner, 2020).
3.3 Ontogeny
Before Tinbergen, Julian Huxley had defined three aspects of research in biology: causation, adaptive value, and evolution (Huxley, 1923; Tinbergen, 1963). He and other researchers received criticism from developmental biologists (e.g., Lehrman, 1953), and from that, Tinbergen added a fourth why to the ones known in the literature: ontogeny. Ethologists and psychobiologists since then developed a vast literature on attachment, parental and sexual imprinting, and song development in birds, among other themes, explicitly addressing the question of how behaviour emerges during individual development (Bateson and Laland, 2013). In a broader sense ethologists consider that complete explanation of any behaviour includes to shed light on its historicity at the individual timescale.
Ants, as holometabolous insects, undergo a complete transformation during their development (egg, larva, pupa, and adult). Despite foraging behaviour being expressed only in adult life, it can be affected by pre-imaginal events. Here, we review how morphological and age modifications, as well as individual experiences, affect foraging behaviours in ants both at the individual and collective level (Figure 1B).
3.3.1 Task allocation of individuals
In ant colonies, each individual temporarily or permanently specialises in a specific behavioural repertoire related to the execution of a task. Division of labour can typically be predicted by age, when task allocation is age-dependent, or morphology, when the worker’s shape and size determine its behavioural caste (Hölldobler and Wilson, 1990; Beshers and Fewell, 2001). Theories on age-polyethism propose a centrifugal theory, where individuals gradually move form brood chambers to outside of the nest, taking in charge the tasks related to each location. Callow workers who recently hatched from the pupal stage will first stay in brood chambers and display brood care (Beshers and Fewell, 2001). As they grow older, these individuals move inside the nest and eventually leave it for short periods to perform hybrid tasks like cleaning and nest maintenance. At the end of their lives, they finally dedicate themselves to foraging (Beshers and Fewell, 2001; Richardson et al., 2021). In this case, colony members respond individually to the dynamic signals, which indicate the need to perform specific tasks (Ulrich et al., 2016). But no universal rule can be drawn, and dynamic task allocation depends both on species and task specificity (Leitner and Dornhaus, 2019).
In species displaying age-polyethism, each individual will thus sequentially take in charge all ergonomic tasks. On the contrary, caste-polyethism rely on morphological specialization of workers as a result of their larval development. Production of workers of different sizes and shapes takes place through differential larval feeding by nurses, according to the colony’s need for each caste-related function (Hölldobler and Wilson, 1990). When age-polyethism permits highly dynamic task allocation between workers, each of them being able to switch from one task to another, task allocation in a caste-polyethism system is slower since it relies on differential development of larvae. Despite both processes being known and well documented for many species, underlying mechanisms are not entirely known. In polymorphic species, age polyethism also influences behavioural maturation of workers, even though the sequence of behaviours differs between morphological castes.
3.3.2 The role of experience
As mentioned in section 2.3, insects adapt their behaviour through learning of the regularities in their environment. Different of internal states, experience can have not only short but also long-term effects. Those effects can arise from changes in the structure of the organisms, usually at the neuronal level (through memory consolidation mechanisms for example), or from persistent changes in the gene expression (even leading sometimes to epigenetic effects within the lineage). The concept of “individual experience” subsumes all those processes that affect behaviour at a long timescale.
Ants and bees, when finding a new food source, do not initially recruit and begin only after a few visits when information on the resource adds up (Grüter and Czaczkes, 2019). In ant species that forage solitarily, foragers display sector fidelity; namely, they tend to consistently leave the nest in the same direction (Neoponera apicalis (Latreille, 1802): Fresneau, 1985; Dinoponera genus: Fourcassié and Oliveira, 2002; Azevedo et al., 2014; Hanisch et al., 2023; Cataglyphis bicolor (Fabricius, 1793): Harkness and Maroudas, 1985; Formica sp.: Tanner, 2009; P. occidentalis: Fewell, 1990; Ectatomma opaciventre (Roger, 1861a): Pie, 2004). Some studies, following the first foraging paths of foragers, have shown that ants first display random searches and then tend to return in direction of places where they had positive experiences in the past (Tanner, 2009).
The experience of successful foraging paths can also influence the propensity to forage, and even to perform some specific recruitment behaviour. Experience is usually confounded with aging in natural conditions, but these variables can sometimes be experimentally distinguished. In O. biroi, the foraging tendency of individuals of the same age increases with success in past foraging experiences (Ravary et al., 2007). The number and quality of tandem runs performed by T. albipennis seem to depend on experience, not age (Franklin et al., 2012); the probability of becoming a tandem run leader also increases with experience (Franklin et al., 2012). Experience, through learning processes, can thus modulate the frequency of foraging behaviour, and finely tune its expression (both spatially and socially).
3.4 Function
Tinbergen (1963) praised Lorenz for stressing the role of behavioural patterns as adaptations, using his famous analogy with organs. Today, the functional aspect of behaviour is by far the most studied in ethology and has been the foundation for the rise and maintenance of all research in behavioural ecology (Bateson and Laland, 2013).
Behaviour is, by definition, the property of an organism, even if one can metaphorically speak of ‘collective behaviour’ to characterise group properties emerging from the interaction of individuals – like for a flock of geese or the structure of raid columns in army ants (Deneubourg et al., 1989; Gordon, 2019; Walsh et al., 2020). Survival and reproduction of fertile individuals are essential for each sterile worker’s inclusive fitness (Bourke, 2011), and the former depends on optimised functioning of the colony as a whole, which is frequently subsumed under the metaphor of the colony forming a superorganism (Hölldobler and Wilson, 2009; Figure 1C). Natural selection then act at both individual and group levels by selecting colonies that present individuals with specific advantageous characteristics (Hölldobler and Wilson, 1990).
A variety of foraging strategies, integrating individual behaviours within the social context of the colony, have been selected for. Foraging is a risky task for a social insect, since it means leaving the shelter of the nest and enduring many costs. Besides energy and time expenditure to search for food, foragers face an increased risk of death by predation, desiccation and pathogens or simply by getting lost (Hölldobler and Wilson, 1990; Nonacs and Dill, 1990; Friedman et al., 2019). The classical evolutionary calculation of the costs and benefits balance must be envisaged at the group level, for which typical task allocation system is a good illustration. As described in Section 3.3.1, foragers in species displaying age-polyethism are the oldest and less fertile members of the colony. One can estimate that losing an old nestmate is less prejudicial than losing a young worker, since the colony can benefit from the latter’s workforce for a longer time (Beshers and Fewell, 2001). Here again, combining ultimate (costs and benefits calculation at the colony level) with proximate (development of individuals) explanations of behaviours provide a deeper understanding of foraging in ants.
General economic theories have been proposed to evaluate gains and losses associated with foraging in animals. The main theory is optimal foraging, which builds on the principle that behavioural sequences of animals have been selected for because they maximise energy acquisition by time unit (MacArthur and Pianka, 1966). At a cognitive level, literature usually refers to (non-conscious) decision mechanisms as a basis for foraging behaviours (but see Wystrach, 2021). Those ‘decisions’ affect behavioural transitions, such as the time spent searching for food in a patch before moving on to the next, as well as the fine-tuning of behaviour, such as the type of food that is searched for.
Another core concept commonly applied to ants and other social insects is the theorem of central place foraging, where animals travel through an area searching for resources and return with them to the initial and central place, the nest (Orians and Pearson, 1979). One of the theorem’s predictions is that the farther from the central point the search occurs, the better the food quality must be, so there is real energetic net gain (Orians and Pearson, 1979). In Pogonomyrmex californicus (Buckley, 1867), several predictions of optimal foraging theory and central place foraging were tested (Holder Bailey and Polis, 1987). Ants selected seeds in function of both size and handling time and success, thus maximising the energy/time ratio. Selectivity was also influenced by the relative abundance of the less preferred food, another prediction of optimal foraging theory (Hughes, 1979). Last, in contrast with central place foraging theory, the energy intake did not differ according to the distance of the patch (Holder Bailey and Polis, 1987). P. clavata forage in the canopy for nectar sources and insect prey (Breed et al., 1987; Fewell et al., 1992), adapting their recruitment strategies to energy intake rate, that is recruiting more frequently to insect prey than to nectar sources. Fewell et al. (1996) performed a comparison of individual and colony energy intake and expenditure between P. clavata and the seed harvesting ant P. occidentalis. In the first species, the benefit to cost ratio was much smaller than in the latter one. This suggested that workers should flexibly adapt their foraging strategies, which they did, and that colony growth was much more constrained by energy intake. As a result, those constraints, mainly associated with ecological parameters, probably also influence reproductive strategies. P. occidentalis can stock large quantities of reserves, resulting in massive seasonal production of alates, while P. clavata is limited in its resources, and produce few alates in a continuous manner (Fewell et al., 1996).
It is often assumed that solitary strategies would be ‘less efficient’ than collective strategies, especially mass recruitment (Hölldobler and Wilson, 1990). This and other kinds of recruitment would help colonies find and monopolise food sources (Detrain and Deneubourg, 2008). However, recent studies, experiments, and models stress that no strategy can be said the most efficient for all species. Unsurprisingly, the efficiency of any foraging strategy is highly context-specific and varies according to the food sources characteristics (type, size, distribution and quality), colony size and ecology (Beckers et al., 1989; Lanan, 2014). Solitary foraging appears very efficient in environments with dispersed distribution of unpredictable food sources, as exemplified by scavenger desert ants (Wehner, 2020).
In a wide variety of contexts, independent search for food brings more energetic gain than recruitment since it speeds up food-collection: other foragers do not need to wait for the return of successful individuals to start the search (Dechaume-Moncharmont et al., 2005). Additionally, mass recruitment through pheromone trails is very costly and considered less efficient for small ant colonies (Beckers et al., 1989). Some empirically validated models also predict that species which mass recruit to exploit a resource could difficultly switch to a new and more valuable food source (Sumpter and Beekman, 2003; Detrain and Deneubourg, 2008). This happens because the positive feedback established surmounts any new trail initiated to the better source (but see Czaczkes, 2014).
Lastly, social foraging strategy emerges from the expression and articulation of individual behaviours, and can show some flexibility within a given species. As an example, Pachycondyla striata (Smith, 1858) workers can switch from solitary foraging to recruitment by tandem running depending on the nature and distance of food (Silva et al., 2021). In species that mass recruit, workers can nevertheless choose not to follow the chemical trail and search for new food sources (Czaczkes et al., 2011), as they do in L. niger. In these cases, individual experience plays a key role in the emergence of an adaptive response of colonies to environmental conditions.
3.5 Evolution
Mayr (1961), earlier than Tinbergen, defined ‘ultimate causes’ as evolutionary advantages attributed to biological characteristics. This definition, however, had two problems: first, it mixes adaptive function and evolutionary history of the trait in a single aspect, and second, it is confusing by the word ‘cause’, which was frequently used by researchers investigating mechanisms, a proximate approach (Bateson and Laland, 2013). Tinbergen’s (Tinbergen, 1963) contribution in this regard aimed to organise this level of analysis conceptually and temporally.
The phylogenetic reconstructions of the evolution of foraging strategies in ants are not easy, since this group presents more than fourteen thousand known species (Bolton, 2023; Figure 1D). With a huge diversity of diets, foraging behaviours, and foraging strategies, seldom do we find similarities, nor can we trace back to where and when some particular patterns, like recruitment, came to exist. Here, we summarise the literature that debates the evolution of foraging in ants. The fact that many species recruit for new nests but not for food raises the hypothesis that recruitment was first selected for emigration, and later co-opted for communicating the location of food sources (Glaser, 2022). A similar evolutionary path has been suggested for the bee dance (Beekman et al., 2008; l’Anson Price and Grüter, 2015). Nest-related behaviours could indeed play a paramount role in the evolution of the social behavioural repertoire in social insects (Bos et al., 2011; Robinson, 2014). The value of the nest is so high for ant colonies’ survival (except for some exceptions like army ants) that there could have been a strong selection enabling fast and precise relocation in case of nest damage, even for individuals that had no experience of the outside world (Franks et al., 2003; Dornhaus et al., 2004). Recruitment for food sources, on the other hand, could be under weaker selection pressures since foragers can uncover new sources for themselves, especially when they are abundant and evenly distributed (Dechaume-Moncharmont et al., 2005; Dornhaus et al., 2006; l’Anson Price et al., 2019; Goy et al., 2021). Note that recruitment nevertheless can bring a real advantage over competitors, namely in interference competition (e.g., Cordonnier et al., 2020).
It is believed that the evolution of foraging strategies has followed a succession model where, throughout evolutionary times, one strategy would replace the other (Glaser, 2022). Nowadays, two putative evolutionary paths are proposed. The first one considers solitary foraging as a basal strategy. Tandem running could have emerged in some groups, then evolving to group foraging and lastly, mass foraging as the most derived strategy (revised by Traniello, 1989). Recent phylogenetic analyses support this hypothesis, showing that the Formicidae group first displayed solitary foraging a basal state and then evolved ‘more complex’ strategies (Reeves and Moreau, 2019; Figure 1D). However, this study is flawed by analysing strategies at the genus level. The authors indeed attributed to each genus the strategy that was the most frequently observed between the congeneric species, therefore masking the diversity existing within each genus. This is all the more important that genera themselves varies tremendously in their diversity. For example Camponotus harbours 1504 species, Pheidole 1294 while Pachycondyla counts only 17 species (Bolton, 2023).
An alternative hypothesis considers that the first ants displayed medium-sized colonies of around few hundreds of individuals (Burchill and Moreau, 2016) and performed mass foraging (Glaser, 2022). As the group differentiated, there was recruitment loss in some taxa, leading to the emergence of group foraging and later of solitary foraging, which in this scenario is the most derived strategy (Glaser, 2022). This hypothesis considers foraging strategies at the species level but is flawed by recruitment being considered both for foraging and nest emigration. Besides, it is based on the reconstitution of the common ancestor made by Burchill and Moreau (2016), where information used is not sufficient to firmly infer the foraging strategy — since species with medium colony size can use different strategies and use solitary foraging. Last, it is not consistent with the previously cited reconstitution (Reeves and Moreau, 2019), using a different database and level of analysis.
It is essential to point out that actually there are not enough data to robustly support any of these hypotheses, mainly because the social organisation of foraging is still unknown or registered anecdotally for many ant species. Moreover, it is noticeable that recruitment evolved independently multiple times in different subfamilies, so a simple succession and substitution mechanism cannot explain the range of existing strategies and their distribution in the clade of living ants (Reeves and Moreau, 2019). For instance, the species Neoponera marginata (Roger, 1861b) belongs to the Ponerinae subfamily, originating from a generalist and solitary hunting ancestor, which is also the most frequent phenotype of extant Neoponera species. N. marginata nevertheless displays swarm foraging, like attack columns of army ants, and a termite-specialised diet, both characteristics considered derived in Formicidae (Leal and Oliveira, 1995). Last, one can also deplore a research bias towards ant species that perform group or mass recruitment, neglecting solitary foraging ant species.Few recent studies use comparative methods to study the diversification of foraging behaviour in ants and its relationship with environmental conditions, especially in groups that perform solitary foraging (Reeves and Moreau, 2019). There is also a need for studies that tackle intraspecific behavioural differences between different ecosystems or biomes, a very interesting tool to understand flexibility, plasticity and potential niche, “cultural” and/or genetic populational differentiation. Previous speculations on how foraging evolved and differentiated suggest no correlation between phylogeny and the foraging method (Jaffe, 1984; Urbani, 1989). However, these studies are based on insufficient data and outdated taxonomy.
3.6 Integrating Tinbergen’s four approaches
Ant behaviour is a complex subject that can be approached through various lenses. In the last sections, we brought examples of studies that address ant foraging behaviour focusing on each of Tinbergen’s four questions. Figure 1 serves as a visual synthesis, capturing these complementary approaches. The major known mechanisms, that triggers and shape the expression of behaviours associated with foraging in ants are diverse (Figure 1A). For the sake of clarity, we chose to present here a simplified version of the actual network of the factors involved in foraging behaviours. These factors can be internal or external to the individual and usually they interact with each other (not all interactions are shown, again for the sake of clarity). Thus, it can be challenging to isolate and measure their specific effect on foraging decision-making. We propose that the focus should rather be on the elucidation of the network of factors and their interactions, since the expression of foraging arises from the global functioning of this network. These factors and processes are modulated throughout the ants’ development and affect the propensity to forage, and the way behaviours associated with foraging are expressed (Figure 1B). Again, while some changes are generated by internal factors (genes, hormones), others are activated mainly by external variables, such as different feeding regimes in larval stages that lead genetically identical individuals to present different adult phenotypes, both for morphology and behaviour. Developmental influences are not confined to the immature stages; factors such as foraging experience in adulthood, age, and colony needs continually shape foraging activity across an individual’s lifespan.
Considering that evolution ultimately acts both at the individual and colonial level, Figure 1C illustrates how foraging optimization in foragers, a caste of sterile workers, leads to increased colonial reproductive success and increased individual and group fitness. Finally, the diversity of foraging strategies and how they have diversified within the ant clade cannot be explained using one or a few biological or ecological parameters (Figure 1D). Ants foraging diversity also allows little room for generalization about how foraging evolved in these insects. Within the same subfamily, there is a wide range of different foraging strategies (and consequently, behaviours associated with them). This wide variety is also observed within ants of the same genus and in notable cases, even within the same species, with ant species showing polymorphism in foraging strategies. Thus, although a general picture of the evolution of the different strategies is difficult to draw, the repeated evolution and reversion of the different strategies across many taxa allows us to study in detail these events. This also suggests that the mechanisms and the behavioural machinery associated with the expression of foraging associated behaviours may be similar across species, but that specific ecological and selective pressures may lead to small modifications, for example during development, that lead to drastic changes of the behaviour at the collective level.
One of the best examples of integrative research in behaviour is the investigation of foraging in Pogonomyrmex species, carried out mainly by Deborah Gordon’s group. For the last decades, researchers made a great effort to evaluate most of the aspects influencing the foraging of those seed-harvesting ants, making this group one of the most studied. Several studies were developed addressing the four different questions and those were integrated and discussed together. For this reason, we discuss how integrative efforts can be made using those articles as examples.
In seed-harvesting ants, many factors can simultaneously alter behavioural activity and gene expressions, such as the circadian rhythm (Ingram et al., 2016), seasonal climatic effects, and species (Das and Gordon, 2023). Desiccation is a powerful selection pressure in desert ants. Friedman and collaborators (Friedman et al., 2019) showed that individual workers adapted their foraging activity to both their hydration state and the environmental risk of water loss. They then investigated at a genomic level the individual variation in tolerance to desiccation (Friedman et al., 2020). Gene expression was linked to sensitivity of foraging activity to humidity and was associated with dopamine to serotonin brain ratio (Friedman et al., 2020). Dopamine titre plays a key role in the initiation of foraging bouts, especially in colonies that were the most sensitive to humidity (Friedman et al., 2018). On a collective level, several works pointed out the importance of interactions among workers at the nest entrance in individual foraging decisions. Foragers display distinct cuticular hydrocarbon profiles, and encountering these profiles motivated workers to start foraging (Greene et al., 2013). More specifically, the amount of interactions with returning workers dynamically and positively influenced foraging decisions. Besides, workers who interacted less also moved further away from the nest entrance, thus being less likely to be activated (Pinter-Wollman et al., 2013). Transitions from inside tasks to foraging showed ample variability, and outside workers could change tasks rapidly when the context changed (Gordon, 1989; Gordon et al., 2005).
Long-term studies were also conducted to stress distal behavioural questions, such as function and evolution. Through observations over 30 years in the same populations, sensitivity to humidity of workers proved to be an important characteristic positively affecting reproductive success of the colony (Gordon, 2013). In the competition context, colony age and colony size influence foraging strategies (Gordon, 1992). Adler and Gordon (2003) modelled the competitive interactions of Pogonomyrmex colonies using optimal foraging theory. The results showed that the adaptive response of individuals and colonies depended on the existence of competition with adjacent colonies. They suggested that individual optimization of foraging behaviour explained well empirical data, even if in the collective model colonies could allocate a larger than optimal number of foragers to boundaries with neighbours, suggesting that both levels probably influence collective response (Adler and Gordon, 2003). Even though seed-eating is common in myrmicine ants (genera Messor, Aphenogaster, Pheidole, etc.), seed-harvesting behaviour and preference for xeric environment is derived in the tribe Pogonomyrmicini (Ward et al., 2015). This makes comparing species extremely relevant to understand evolutionary ecological patterns (Johnson, 2000). Several Pogonomyrmex spp. Occur in sympatry in the Sonoran Desert. Their foraging behaviour is also determined by this co-occurrence of species with the same diet. Some species are dominant and defend food resources and territory while others, with smaller workforces, tend to avoid other species when foraging (Gordon, 1984).
In conclusion, the set of research carried out on seed-harvesting Pogonomyrmex gives an unprecedented understanding of mechanistic, ontogenetic, ecological and evolutionary aspects of foraging. We advocate that similar research efforts should be carried out on more species, in an attempt to cover the range of foraging strategies (from solitary to mass recruitment). The fact that this research spans over forty years shows that integrating all these aspects is a time-consuming process that needs perennial and experienced research teams. Unfortunately, most research groups can face limitations while trying to integrate proximate and ultimate causes. Not all ant species can be kept in laboratories or have a fixed population in the same region throughout the years. Laboratories usually have a higher rotation of members, who have short-duration projects and restricted budgets.
4 Final considerations and future directions
Despite Tinbergen’s four questions being the foundation of modern ethology and the foraging behaviour being primarily dealt with, it is unusual to find articles and researchers that work with a global understanding of the levels of analyses (see Shettleworth, 2010). Generally, ultimate causes received more attention than the proximate ones (Bateson and Laland, 2013). This is all the truer with studies of “foraging”, where behaviours of interest are identified for their role in providing food resources to the colony (their function) rather than their actual expression (precise description, underlying mechanisms and development). Nevertheless, explaining the determinants of how ants behave here-and-now is a necessary part of the explanation of the adaptative value and evolution of behaviours.
In ants, phylogenies that consider behavioural traits are rare, mainly because they are harder to obtain at a fine scale than morphological and biogeographical traits. Of all the cladistic analyses performed, it is estimated that only 5% consider behavioural characters (De Queiroz and Wimberger, 1993). This bias, most frequent in ecological, taxonomic and evolutionary research, is at odds with Tinbergen’s proposal of addressing all questions with the same intensity since there is no hierarchy among them (Tinbergen, 1963). Division among researchers and research centres investigating proximate or ultimate causes confirms Tinbergen’s fear of witnessing ethology being dismembered in subareas that communicate very little among themselves (Bateson and Laland, 2013).
In addition to the adaptive and evolutionary aspects we highlighted, there is a specific critical bias to be mentioned in ants: much research is done on some species and ant genus, whereas there is little to no behavioural information on others (Reeves and Moreau, 2019). For studies on foraging an even greater focus is given to species that perform mass recruitment and have large population size. In contrast, information on linear recruitments (i.e., tandem running, tandem carrying) and solitary foraging are partially neglected. Considering that both phylogenies and behavioural data are incomplete, phylogenetic studies across the Family are not actually that useful to understand specific trends in a more detailed way. Analysing precisely closely related taxa that are better documented in several genera or subfamily to understand specific patterns and their spread across the Formicidae could be more fruitful. A few studies already aimed to do that (For example, Yagound et al., 2017, for nestmate recognition). Joining such fine-grain analyses seem more promising than general analyses, especially in the case of foraging where numerous independent evolution of the different strategies, and also reversion, exist (Reeves and Moreau, 2019). Another hypothesis that could arise from the diversity of foraging behaviours between phylogenetically-close species is that quite distinct behaviours could emerge from very similar, or even conserved, underlying mechanisms at the cognitive and sensorimotor levels (similarly to what Wystrach – 2021 – proposed for navigation). The studies we suggest would be a way to tackle this question in an integrative way.
Despite the recent rise of research that evaluates mechanisms and developmental aspects of foraging behaviours, such as physiology and experience, these findings are ignored by a large gamut of research focusing on ultimate and ecological questions. Other variables have their effect questioned by part of the myrmecologists, like, for instance, the effect of motivational states that could even be associated with some kind of emotion-like subjective state (Baracchi et al., 2017), and the existence of personality in ants (Japyassú et al., 2021). Alternatively, research that evaluates ultimate questions focuses on the evolutionary history and adaptive function; these factors are neglected in some laboratory mechanistic studies. This is also a consequence of a mismatch in model species studied at both levels. However, research on ultimate causes often does not make explicit assumptions about the mechanisms behind these adaptive characteristics, which frequently can be complex and differ from one species to another, even if the observed functional motor response seems similar.
Recently a number of research groups have developed high throughput behavioural experiments, which are more or less automated and can be analysed with neural learning and other tools of artificial intelligence (Robinson et al., 2009; Davidson et al., 2016; Ulrich et al., 2016). Some studies also started virtual reality set-up to look into forager navigation in the laboratory (Dauzere-Peres and Wystrach, 2023). This opens the possibility of generating large amount of data across taxa. If laboratories collaborate to provide results from different species, this could further our knowledge about foraging behaviour. The research collaboration networks should however carefully consider the contribution of all participants, because cost associated constraints often prevent scientists from the areas where the ants actually originate (the tropics) to lead this kind of research. This actually slows down the collection of large multidisciplinary datasets, apart from lowering the recognition of less developed countries scientists (Ocampo-Ariza et al., 2023). Similar approaches have successfully been used in functional ecology to characterise thermal tolerance (Corley et al., 2023). However, this kind of studies are often limited because of size, number of individuals, and of costs. These studies also are limited in their reach when considering the four questions, and they may not transfer easily to the field (but see Haalck et al., 2023). One of the difficulties is to design standardised tests which are flexible enough to accommodate the different species foraging areas, colony size, worker size, and foraging strategies. It seems more reasonable to propose that specific questions about specific strategies could be tested in several taxa in parallel. This connects to our proposal to study related species across taxa to compare the convergent evolution of the different strategies. An important point is also to design a set of experiments that would document the proximate and ultimate aspects of foraging and a connection with ecological and social factors. Looking into mechanisms can shed light on the conserved nature of the machinery of behaviour or on the evolution of innovation in the expression of similar strategies. We also advocate that flexibility should be tested using a range of experimental treatments with varying biotic and abiotic factors, in the field and/or in the lab, to assess the potential of the species to cope with environmental changes and also to observe intraspecific variation in foraging behaviour. For example, several studies on different species of the genus Dinoponera (Fourcassié and Oliveira, 2002; Hanisch et al., 2023; Lima Vieira et al., 2024) that all species are flexible in their foraging strategies, and that nest density and prey availability are the main factors determining foraging characteristics. Comparative studies thus can evidence the relative influence of pre-determined and environmentally mediated factors in shaping individual foraging behaviours. This can in turn highlights the mechanisms and developmental elements of the expression of behaviour and their similarities across the Formicidae. This endeavour is crucial and it would put back ant behavioural research on the tracks proposed by Tinbergen (1963). Indeed, one of Tinbergen’s worries was that ethology would turn to uniquely functional studies, and he pointed out why this would weaken behavioural sciences. A frequent trend in ant behavioural sciences is that unusual behaviours often get the community attention, being published in high impact journals (for example: ant traps: Dejean et al., 2005; tandem running: Schultheiss et al., 2015; but see Gordon, 2013) and end up not being studied more carefully using the four questions. This is a bias that also should be looked at with attention. A prominent ant scientist also predicted in 1975 that ethology actually would become extinct and behavioural ecology would take its place, mechanistic studies becoming limited to neurosciences and physiology (Wilson, 1975). We disagree with that predictions and show in our review that the Tinbergen approach is certainly very important in its potential to generate knowledge and a better understanding of foraging behaviour. Ethology is still present and, whether through the collaboration of multidisciplinary groups or the continued research of individual groups, it should allow us to get a very detailed picture of ant behaviour in the future.
To provide more robust and complete explanations of behaviour, researchers focusing on proximate questions should have a profound understanding of the ultimate explanations of the studied aspects, and researchers of ultimate questions should have detailed knowledge of proximate mechanisms that modulate evolutionary processes. It seems also that an experience with the animals in the field, especially for foraging, is an important part of the process. Unfortunately, field studies often are not carried out using the necessary rigor of ethological studies and their report as natural history anecdotes often limits their relevance to the behavioural sciences. These methodological issues should be taken into account in future research. Future works would also gain much more impact by stating their level of analysis. Besides, research which aims to integrate proximate and ultimate questions should be performed more frequently. This involves the formulation of hypotheses which are not exclusive and recognising that there is more than one type of explanation for the same phenomenon. Priority should be given to studies on species whose behaviour is less described to contribute to building a broader knowledge of the Family Formicidae. This may avoid the sometimes-undue generalisations made about foraging in ants and allow highlighting the existence of a greater diversity of foraging strategies in ants.
Author contributions
MELV: Conceptualization, Methodology, Writing – original draft, Writing – review & editing, Project administration. SC: Conceptualization, Writing – review & editing. NC: Conceptualization, Writing – review & editing, Funding acquisition, Methodology, Project administration, Supervision, Writing – original draft.
Funding
The author(s) declare financial support was received for the research, authorship, and/or publication of this article. NC and MELV received funding grants from the Brazilian Science Ministry (Conselho Nacional de Desenvolvimento Cientı́fico e Tecnológico, CNPq) PQ 458736/2014, PQ 311790/2021-8 and from CAPES (Coordenação de Aperfeiçoamento de Pessoal de Nível Superior) process CAPES/PRInt 88887.915491/2023-00 and CAPES/PRInt 88887.916823/2023-00. This research was supported by FAPESP (Fundação de Amparo à Pesquisa do Estado de São Paulo), process FAPESP 2019/20357-7.
Acknowledgments
We thank Gilles Gheusi for his helpful comments, and two referees for their constructive comments.
Conflict of interest
The authors declare that the research was conducted in the absence of any commercial or financial relationships that could be construed as a potential conflict of interest.
The author NC declared that they were an editorial board member of Frontiers, at the time of submission. This had no impact on the peer review process and the final decision.
Publisher’s note
All claims expressed in this article are solely those of the authors and do not necessarily represent those of their affiliated organizations, or those of the publisher, the editors and the reviewers. Any product that may be evaluated in this article, or claim that may be made by its manufacturer, is not guaranteed or endorsed by the publisher.
References
Adams E. S. (2016). Territoriality in ants (Hymenoptera: Formicidae): a review. Myrmecol. News 23, 101-118.
Adler F. R., Gordon D. M. (2003). Optimization, conflict, and nonoverlapping foraging ranges in ants. Am. Nat. 162, 529–543. doi: 10.1086/378856
Allen C., Bekoff M. (1997). Species of mind: The philosophy and biology of cognitive ethology (Cambridge, MA: MIT Press).
Andrew N. R., Hart R. A., Jung M. P., Hemmings Z., Terblanche J. S. (2013). Can temperate insects take the heat? A case study of the physiological and behavioural responses in a common ant, Iridomyrmex purpureus (Formicidae), with potential climate change. J. Insect Physiol. 59, 870–880. doi: 10.1016/j.jinsphys.2013.06.003
Andrews K. (2015). The animal mind: An introduction to the philosophy of animal cognition (Abingdon, UK: Routledge).
Avarguès-Weber A., Chittka L. (2014). Local enhancement or stimulus enhancement? Bumblebee social learning results in a specific pattern of flower preference. Anim. Behav. 97, 185–191. doi: 10.1016/j.anbehav.2014.09.020
Azevedo D. L. O., Medeiros J. C., Araújo A. (2014). Adjustments in the time, distance and direction of foraging in dinoponera quadriceps workers. J. Insect Behav. 27, 177–191. doi: 10.1007/s10905-013-9412-6
Azevedo D. L. O., Medeiros J. C., Araújo A. (2021). Flexibility in the integration of environmental information by Dinoponera quadriceps Kempf during foraging. Rev. Bras. Entomol. 65, 1-7. doi: 10.1590/1806-9665-RBENT-2021-0084
Baracchi D., Lihoreau M., Giurfa M. (2017). Do insects have emotions? Some insights from bumble bees. Front. Behav. Neurosci. 11. doi: 10.3389/fnbeh.2017.00157
Barron A. B., Maleszka R., vander Meer R. K., Robinson G. E. (2007). Octopamine modulates honey bee dance behavior. Proc. Natl. Acad. Sci. U.S.A. 104, 1703–1707. doi: 10.1073/pnas.0610506104
Barron A. B., Schulz D. J., Robinson G. E. (2002). Octopamine modulates responsiveness to foraging-related stimuli in honey bees (Apis mellifera). J. Comp. Physiol. A: Neuroethol. Sensory Neural Behav. Physiol. 188, 603–610. doi: 10.1007/s00359-002-0335-5
Bateson M., Desire S., Gartside S. E., Wright G. A. (2011). Agitated honeybees exhibit pessimistic cognitive biases. Curr. Biol. 21, 1070–1073. doi: 10.1016/j.cub.2011.05.017
Bateson P., Laland K. N. (2013). Tinbergen’s four questions: An appreciation and an update. Trends Ecol. Evol. 28, 712–718. doi: 10.1016/j.tree.2013.09.013
Beckers R., Goss S., Deneubourg J. L., Pasteels J. M. (1989). Colony size, communication and ant foraging strategy. Psyche 96, 239–256. doi: 10.1155/1989/94279
Beekman M., Gloag R. S., Even N., Wattanachaiyingchareon W., Oldroyd B. P. (2008). Dance precision of Apis florea - Clues to the evolution of the honeybee dance language? Behav. Ecol. Sociobiol. 62, 1259–1265. doi: 10.1007/s00265-008-0554-z
Bengston S. E., Dornhaus A. (2014). Be meek or be bold? A colony-level behavioural syndrome in ants. Proc. R. Soc. B: Biol. Sci. 281, 1-9. doi: 10.1098/rspb.2014.0518
Ben-Shahar Y. (2005). The foraging gene, behavioral plasticity, and honeybee division of labor. J. Comp. Physiol. A: Neuroethol. Sensory Neural Behav. Physiol. 191, 987–994. doi: 10.1007/s00359-005-0025-1
Beshers S. N., Fewell J. H. (2001). Models of division of labor in social insects. Annu. Rev. Entomol. 46, 413–440. doi: 10.1146/annurev.ento.46.1.413
Birch J., Schnell A. K., Clayton N. S. (2020). Dimensions of animal consciousness. Trends Cogn. Sci. 24, 789–801. doi: 10.1016/j.tics.2020.07.007
Bitterman M. E. (1975). Incentive contrast in honey bees. Science 192, 380-382. doi: 10.1126/science.1257773
Bolhuis J. J., Giraldeau L. A., Hogan J. A. (2022). The behavior of animals: Mechanisms, function and evolution. 2nd ed. (Hoboken, NJ: John Wiley & Sons).
Bonabeau E., Theraulaz G., Deneubourg J. L. (1998). Fixed response thresholds and the regulation of division of labor in insect societies. Bull. Math. Biol. 60, 753–807. doi: 10.1006/bulm.1998.0041
Bonduriansky R., Day T. (2018). Extended heredity: A new understanding of inheritance and evolution (Princeton, NJ: Princeton University Press).
Bos N., Grinsted L., Holman L. (2011). Wax on, wax off: nest soil facilitates indirect transfer of recognition cues between ant nestmates. PloS One 6, e19435. doi: 10.1371/journal.pone.0019435
Breed M. D., Fewell J. H., Moore A. J., Williams K. R. (1987). Graded recruitment in a ponerine ant. Behav. Ecol. Sociobiol. 20, 407–411. doi: 10.1007/BF00302983
Buckley S. B. (1866). Descriptions of new species of North American Formicidae. Proc. Entomol. Soc Phila. 6, 152–172.
Buckley S. B. (1867). Descriptions of new species of North American Formicidae (continued from page 172.). Proc. Entomol. Soc. Philadelphia 6, 335–350.
Burchill A. T., Moreau C. S. (2016). Colony size evolution in ants: macroevolutionary trends. Insect. Sociaux 63, 291–298. doi: 10.1007/s00040-016-0465-3
Cassill D. L., Tschinkel W. R. (1995). Allocation of liquid food to larvae via trophallaxis in colonies of the fire ant, Solenopsis invicta. Anim. Behav. 50, 801–813. doi: 10.1016/0003-3472(95)80140-5
Cassill D. L., Tschinkel W. R. (1999). Effects of colony-level attributes on larval feeding in the fire ant. Solenopsis invicta. Insect. Sociaux 46, 261–266. doi: 10.1007/s000400050144
Clément L., Schwarz S., Wystrach A. (2023). An intrinsic oscillator underlies visual navigation in ants. Curr. Biol. 33, 411–422.e5. doi: 10.1016/j.cub.2022.11.059
Cogni R., Oliveira P. S. (2004). Patterns in foraging and nesting ecology in the neotropical ant, Gnamptogenys moelleri (Formicidae, Ponerinae). Insect. Sociaux 51, 123–130. doi: 10.1007/s00040-003-0711-3
Cole B. J., Smith A. A., Huber Z. J., Wiernasz D. C. (2010). The structure of foraging activity in colonies of the harvester ant, Pogonomyrmex occidentalis. Behav. Ecol. 21, 337–342. doi: 10.1093/beheco/arp193
Collett T. S., Graham P. (2004). Animal navigation: path integration, visual landmarks and cognitive maps. Curr. Biol. 14, R475–R477. doi: 10.1016/j.cub.2004.06.013
Cordonnier M., Blight O., Angulo E., Courchamp F. (2020). The native ant lasius Niger can limit the access to resources of the invasive argentine ant. Animals 10, 2451. doi: 10.3390/ani10122451
Corley R. B., Dawson W., Bishop T. R. (2023). A simple method to account for thermal boundary layers during the estimation of Ctmax in small ectotherms. J. Thermal Biol. 116, 103673. doi: 10.1016/j.jtherbio.2023.103673
Creemers B., Billen J., Gobin B. (2003). Larval begging behaviour in the ant Myrmica rubra. Ethol. Ecol. Evol. 15, 261–272. doi: 10.1080/08927014.2003.9522671
Cresson E. T. (1865). Catalogue of hymenoptera in the collection of the entomological society of Philadelphia, from Colorado territory. [concl.]. Proc. Entomol. Soc. Philadelphia 4, 426–488.
Curtis J. (1854). On the genus Myrmica and other indigenous ants. Trans. Linn. Soc. London 21, 211–220. doi: 10.1111/j.1096-3642.1852.tb00456.x
Czaczkes T. J. (2014). How to not get stuck-Negative feedback due to crowding maintains flexibility in ant foraging. J. Theor. Biol. 360, 172–180. doi: 10.1016/j.jtbi.2014.07.005
Czaczkes T. J., Beckwith J. J., Horsch A. L., Hartig F. (2019). The multi-dimensional nature of information drives prioritization of private over social information in ants. Proc. R. Soc. B: Biol. Sci. 286, 1-9. doi: 10.1098/rspb.2019.1136
Czaczkes T. J., Grüter C., Jones S. M., Ratnieks F. L. W. (2011). Synergy between social and private information increases foraging efficiency in ants. Biol. Lett. 7, 521–524. doi: 10.1098/rsbl.2011.0067
Czaczkes T. J., Heinze J. (2015). Ants adjust their pheromone deposition to a changing environment and their probability of making errors. Proc. R. Soc. B: Biol. Sci. 282, 1-6. doi: 10.1098/rspb.2015.0679
Das B., de Bekker C. (2022). Time-course RNASeq of Camponotus floridanus forager and nurse ant brains indicate links between plasticity in the biological clock and behavioral division of labor. BMC Genomics 23, 1-23. doi: 10.1186/s12864-021-08282-x
Das B., Gordon D. M. (2023). Biological rhythms and task allocation in ant colonies. Curr. Opin. Insect Sci. 101062, 1-8. doi: 10.1016/j.cois.2023.101062
Dason J. S., Cheung A., Anreiter I., Montemurri V. A., Allen A. M., Sokolowski M. B. (2020). Drosophila melanogaster foraging regulates a nociceptive-like escape behavior through a developmentally plastic sensory circuit. Proc. Natl. Acad. Sci. U.S.A. 117, 23286–23291. doi: 10.1073/pnas.1820840116
Dauzere-Peres O., Wystrach A. (2023). Ants integrate proprioception, visual context and efference copies to make robust predictions. bioRxiv, 2023–2003. doi: 10.1101/2023.03.29.534571
Davidson J. D., Arauco-Aliaga R. P., Crow S., Gordon D. M., Goldman M. S. (2016). Effect of interactions between harvester ants on forager decisions. Front. Ecol. Evol. 4. doi: 10.3389/fevo.2016.00115
Dawson E. H., Chittka L. (2014). Bumblebees (Bombus terrestris) use social information as an indicator of safety in dangerous environments. Proc. R. Soc. B: Biol. Sci. 281, 20133174. doi: 10.1098/rspb.2013.317410.1098/rspb.2013.3174
Dechaume-Moncharmont F. X., Dornhaus A., Houston A. I., McNamara J. M., Collins E. J., Franks N. R. (2005). The hidden cost of information in collective foraging. Proc. R. Soc. B: Biol. Sci. 272, 1689–1695. doi: 10.1098/rspb.2005.3137
Dejean A., Solano P. J., Ayroles J., Corbara B., Orivel J. (2005). Arboreal ants build traps to capture prey. Nature 434 (7036), 973–973.
Deneubourg J. L., Goss S., Franks N., Pasteels J. M. (1989). The blind leading the blind: modeling chemically mediated army ant raid patterns. J. Insect Behav. 2, 719–725. doi: 10.1007/BF01065789
De Queiroz A., Wimberger P. H. (1993). The usefulness of behavior for phylogeny estimation: levels of homoplasy in behavioral and morphological characters. Evolution 47, 46–60. doi: 10.1111/j.1558-5646.1993.tb01198.x
Detrain C., Deneubourg J. L. (2008). Collective decision-making and foraging patterns in ants and honeybees. Adv. Insect Physiol. 35, 123–173. doi: 10.1016/S0065-2806(08)00002-7
Detrain C., Prieur J. (2014). Sensitivity and feeding efficiency of the black garden ant Lasius Niger to sugar resources. J. Insect Physiol. 64, 74–80. doi: 10.1016/j.jinsphys.2014.03.010
d’Ettorre P., Carere C., Demora L., Le Quinquis P., Signorotti L., Bovet D. (2017). Individual differences in exploratory activity relate to cognitive judgement bias in carpenter ants. Behav. Process. 134, 63–69. doi: 10.1016/j.beproc.2016.09.008
Dornhaus A., Collins E. J., Dechaume-Moncharmont F. X., Houston A. I., Franks N. R., McNamara J. M. (2006). Paying for information: Partial loads in central place foragers. Behav. Ecol. Sociobiol. 61, 151–161. doi: 10.1007/s00265-006-0246-5
Dornhaus A., Franks N. R., Hawkins R. M., Shere H. N. S. (2004). Ants move to improve: Colonies of Leptothorax albipennis emigrate whenever they find a superior nest site. Anim. Behav. 67, 959–963. doi: 10.1016/j.anbehav.2003.09.004
Dornhaus A., Powell S. (2010). “Foraging and Defence Strategies,” in Ant Ecology (Oxford, UK: Oxford University Press). doi: 10.1093/acprof:oso/9780199544639.003.0012
Driessen G., Bernstein C. (1999). Patch departure mechanisms and optimal host exploitation in an insect parasitoid. J. Anim. Ecol. 68, 445–459. doi: 10.1046/j.1365-2656.1999.00296.x
Duarte A., Pen I., Keller L., Weissing F. J. (2012). Evolution of self-organized division of labor in a response threshold model. Behav. Ecol. Sociobiol. 66, 947–957. doi: 10.1007/s00265-012-1343-2
Dunn R. R., Parker C. R., Sanders N. J. (2007). Temporal patterns of diversity: Assessing the biotic and abiotic controls on ant assemblages. Biol. J. Linn. Soc. 91, 191–201. doi: 10.1111/j.1095-8312.2007.00783.x
Emery C. (1892). Voyage de M. Ch. Alluaud dans le territoire d'‘Assinie (Afrique occidentale) en juillet et août 1886. Formicides. Annales la Société Entomol. France 60, 553–574.
Emery C. (1895). Beiträge zur Kenntniss der nordamerikanischen Ameisenfauna. (Schluss). Zool. Jahrbücher. Abteilung Für Systematik Geogr. Und Biol. Der Tiere 8, 257–360.
Fabricius J. C. (1775). Systema entomologiae, sistens insectorum classes, ordines, genera, species adiectis synonymis, locis, descriptionibus, observationibus, Flensburgi et Lipsiae [= Flensburg and Leipzig]: Korte. Officina Libraria Kortii (Flensburg and Leipzig, DE).
Fabricius J. C. (1793). Entomologia systematica emendata et aucta. Secundum classes, ordines, genera, species, adjectis synonimis, locis observationibus, descriptionibus. Tome 2. (Copenhagen, DK), 519, Hafniae [= Copenhagen]: C. G. Proft.
Fetter-Pruneda I., Hart T., Ulrich Y., Gal A., Oxley P. R., Olivos-Cisneros L., et al. (2021). An oxytocin/vasopressin-related neuropeptide modulates social foraging behavior in the clonal raider ant. PloS Biol. 19, 1-23. doi: 10.1371/journal.pbio.3001305
Fewell J. H. (1990). Directional fidelity as a foraging constraint in the western harvester ant, Pogonomyrmex occidentalis. Oecologia 82, 45–51. doi: 10.1007/BF00318532
Fewell J. H., Harrison J. F., Lighton J. R. B., Breed M. D. (1996). Foraging energetics of the ant, Paraponera clavata. Oecologia 105, 419–427. doi: 10.1007/BF00330003
Fewell J. H., Harrison J. F., Stiller T. M., Breed M. D. (1992). Distance effects on resource profitability and recruitment in the giant tropical ant, Paraponera clavata. Oecologia 92, 542–547. doi: 10.1007/BF00317846
Forel A. (1907). Formicides du musée national hongrois. Annales Historico-Naturales Musei Nation. Hungarici 5, 1–42.
Fourcassié V., Oliveira P. S. (2002). Foraging ecology of the giant Amazonian ant Dinoponera gigantea (Hymenoptera, Formicidae, Ponerinae): Activity schedule, diet and spatial foraging patterns. J. Natural History 36, 2211–2227. doi: 10.1080/00222930110097149
Franklin E. L., Robinson E. J. H., Marshall J. A. R., Sendova-Franks A. B., Franks N. R. (2012). Do ants need to be old and experienced to teach? J. Exp. Biol. 215, 1287–1292. doi: 10.1242/jeb.064618
Franks N. R., Dornhaus A., Fitzsimmons J. P., Stevens M. (2003). Speed versus accuracy in collective decision making. Proc. R. Soc. B: Biol. Sci. 270, 2457–2463. doi: 10.1098/rspb.2003.2527
Fresneau D. (1985). Individual foraging and path fidelity in a ponerine ant. Insect. Sociaux 32, 109–116. doi: 10.1007/BF02224226
Friedman D. A., Greene M. J., Gordon D. M. (2019). The physiology of forager hydration and variation among harvester ant (Pogonomyrmex barbatus) colonies in collective foraging behavior. Sci. Rep. 9, 1-9. doi: 10.1038/s41598-019-41586-3
Friedman D. A., Pilko A., Skowronska-Krawczyk D., Krasinska K., Parker J. W., Hirsh J., et al. (2018). The role of dopamine in the collective regulation of foraging in harvester ants. Iscience 8, 283–294. doi: 10.1016/j.isci.2018.09.001
Friedman D. A., York R. A., Hilliard A. T., Gordon D. M. (2020). Gene expression variation in the brains of harvester ant foragers is associated with collective behavior. Commun. Biol. 3,, 1-9. doi: 10.1038/s42003-020-0813-8
Ghoul M., Andersen S. B., West S. A. (2017). Sociomics: using omic approaches to understand social evolution. Trends Genet. 33, 408–419. doi: 10.1016/j.tig.2017.03.009
Glaser S. M. (2022). The adaptive significance of social information in tandem running ants (Mainz, DE: Johannes Gutenberg-Universität Mainz). doi: 10.25358/OPENSCIENCE-6657
Glaser S. M., Feitosa R. M., Koch A., Goß N., do Nascimento F. S., Grüter C. (2021). Tandem communication improves ant foraging success in a highly competitive tropical habitat. Insect. Sociaux 68, 161–172. doi: 10.1007/s00040-021-00810-y
Glastad K. M., Graham R. J., Ju L., Roessler J., Brady C. M., Berger S. L. (2020). Epigenetic regulator CoREST controls social behavior in ants. Mol. Cell 77, 338–351. doi: 10.1016/j.molcel.2019.10.012
Gomez-Marin A., Ghazanfar A. A. (2019). The life of behavior. Neuron 104, 25–36. doi: 10.1016/j.neuron.2019.09.017
Gordon D. M. (1984). Species-specific patterns in the social activities of harvester ant colonies (Pogonomyrmex). Insect. sociaux 31, 74–86. doi: 10.1007/BF02223693
Gordon D. M. (1989). Dynamics of task switching in harvester ants. Anim. Behav. 38, 194–204. doi: 10.1016/S0003-3472(89)80082-X
Gordon D. M. (1992). How colony growth affects forager intrusion between neighboring harvester ant colonies. Behav. Ecol. sociobiol. 31, 417–427. doi: 10.1007/BF00170609
Gordon D. M. (2013). The rewards of restraint in the collective regulation of foraging by harvester ant colonies. Nature 498, 91–93. doi: 10.1038/nature12137
Gordon D. M. (2019). The ecology of collective behavior in ants. Annu. Rev. Entomol. 64, 35–50. doi: 10.1146/annurev-ento-011118-111923
Gordon D. M., Chu J., Lillie A., Tissot M., Pinter N. (2005). Variation in the transition from inside to outside work in the red harvester ant. Pogonomyrmex barbatus. Insect. Sociaux 52, 212–217. doi: 10.1007/s00040-004-0796-3
Gordon D. M., Dektar K. N., Pinter-Wollman N. (2013). Harvester ant colony variation in foraging activity and response to humidity. PloS One 8,1-6. doi: 10.1371/journal.pone.0063363
Gordon D. M., Guetz A., Greene M. J., Holmes S. (2011). Colony variation in the collective regulation of foraging by harvester ants. Behav. Ecol. 22, 429–435. doi: 10.1093/beheco/arq218
Goy N., Glaser S. M., Grüter C. (2021). The adaptive value of tandem communication in ants: insights from an agent-based model. J. Theorical Biol. 526, 1-11. doi: 10.1101/2020.09.14.296426
Greene M. J., Gordon D. M. (2003). Social insects: Cuticular hydrocarbons inform task decisions. Nature 423, 32. doi: 10.1038/423032a
Greene M. J., Pinter-Wollman N., Gordon D. M. (2013). Interactions with combined chemical cues inform harvester ant foragers’ Decisions to leave the nest in search of food. PloS One 8, 1–8. doi: 10.1371/journal.pone.0052219
Griffin D. R. (1976). The Question of Animal Awareness: Evolutionary Continuity of Mental Experience (New York, NY: The Rockfeller University Press).
Griffiths P. E. (2007). History of ethology comes of age. Biol. Philos. 23, 129–134. doi: 10.1007/s10539-007-9075-9
Grüter C., Czaczkes T. J. (2019). Communication in social insects and how it is shaped by individual experience. Anim. Behav. 151, 207–215. doi: 10.1016/j.anbehav.2019.01.027
Guidugli K. R., Nascimento A. M., Amdam G., Barchuk A. R., Omholt S., Simões Z. L. P., et al. (2005). Vitellogenin regulates hormonal dynamics in the worker caste of a eusocial insect. FEBS Lett. 579, 4961–4965. doi: 10.1016/j.febslet.2005.07.085
Haalck L., Mangan M., Wystrach A., Clement L., Webb B., Risse B. (2023). CATER: combined animal tracking & environment reconstruction. Sci. Adv. 9, eadg2094. doi: 10.1126/sciadv.adg2094
Hanisch P. E., Hanisch E. R., Blanco V., Tubaro P. L., Suarez A. V. (2023). Spatial fidelity and uniform exploration in the foraging behaviour of a giant predatory ant. Anim. Behav. 203, 63–73. doi: 10.1016/j.anbehav.2023.06.009
Harkness R. D., Maroudas N. G. (1985). Central place foraging by an ant (Cataglyphis bicolor Fab.): a model of searching. Anim. Behav. 33, 916–928. doi: 10.1016/S0003-3472(85)80026-9
Harrison J. F., Fewell J. H., Stiller T. M., Breed M. D. (1989). Effects of experience on use of orientation cues in the giant tropical ant. Anim. Behav. 37, 869–871. doi: 10.1016/0003-3472(89)90076-6
Hogan J. A. (2015). A framework for the study of behavior. Behav. Process. 117, 105–113. doi: 10.1016/j.beproc.2014.05.003
Hogan J. A. (2021). “Motivation and emotion,” in The behavior of animals, 2nd edition: mechanisms, function and evolution Hoboken, NJ: WIley, 48–77.
Hogan J., Bolhuis J. (2005). The development of behaviour: trends since Tinbergen, (1963). Anim. Biol. 55, 371–398. doi: 10.1163/157075605774840914
Holder Bailey K., Polis G. A. (1987). Optimal and central-place foraging theory applied to a desert harvester ant, Pogonomyrmex californicus. Oecologia 72, 440–448. doi: 10.1007/BF00377577
Hölldobler B., Möglich M., Maschwitz U. (1974). Communication by tandem running in the ant Camponotus sericeus. J. Comp. Physiol. 90 (2), 105–127.
Hölldobler B., Wilson E. O. (2009). The superorganism: The beauty, elegance, and strangeness of insect societies (New York, NY: W.W. Norton).
Horna-Lowell E., Neumann K. M., O’fallon S., Rubio A., Pinter-Wollman N. (2021). Personality of ant colonies (Hymenoptera: Formicidae) – underlying mechanisms and ecological consequences. Myrmecol. News 31, 47–59. doi: 10.25849/myrmecol.news_031:047
Hughes R. N. (1979). Optimal diets under the energy maximization premise: the effects of recognition time and learning. Am. Nat. 113, 209–221. doi: 10.1086/283380
Huxley J. S. (1923). Courtship Activities in the Red-throated Diver (Colymbus stellatus Pontopp.); together with a discussion of the Evolution of Courtship in Birds*. J. Linn. Soc. London Zool. 35, 253–292. doi: 10.1111/j.1096-3642.1923.tb00048.x
Ingram K. K., Gordon D. M., Friedman D. A., Greene M., Kahler J., Peteru S. (2016). Context-dependent expression of the foraging gene in field colonies of ants: the interacting roles of age, environment and task. Proc. R. Soc. B: Biol. Sci. 283, 20160841. doi: 10.1098/rspb.2016.0841
Ings T. C., Chittka L. (2009). Predator crypsis enhances behaviourally mediated indirect effects on plants by altering bumblebee foraging preferences. Proc. R. Soc. B: Biol. Sci. 276, 2031–2036. doi: 10.1098/rspb.2008.1748
Jablonka E., Lamb M. (2020). Inheritance systems and the extended evolutionary synthesis (Cambridge, UK: Cambridge University Press).
Jaffe K. (1984). Negentropy and the evolution of chemical recruitment in ants (Hymenoptera: Formicidae). J. Theor. Biol. 106, 587–604. doi: 10.1016/0022-5193(84)90009-2
Japyassú H. F., Neco L. C., Nunes-Neto N. (2021). Minimal organizational requirements for the ascription of animal personality to social groups. Front. Psychol. 11. doi: 10.3389/fpsyg.2020.601937
Jeanson R. (2019). Within-individual behavioural variability and division of labour in social insects. J. Exp. Biol. 222, 1-8. doi: 10.1242/jeb.190868
Johnson R. A. (2000). Seed-harvester ants (Hymenoptera: Formicidae) of North America: an overview of ecology and biogeography. Sociobiology 36, 89–122.
Kempf W. W. (1971). A preliminary review of the ponerine ant genus Dinoponera Roger (Hymenoptera: Formicidae). Studia Entomol. 14, 369–394. doi: 10.5281/zenodo.26042
Kolay S., Boulay R., d’Ettorre P. (2020). Regulation of ant foraging: A review of the role of information use and personality. Front. Psychol. 11. doi: 10.3389/fpsyg.2020.00734
Laland K., Matthews B., Feldman M. W. (2016). An introduction to niche construction theory. Evol. Ecol. 30, 191–202. doi: 10.1007/s10682-016-9821-z
Laland K. N., Odling-Smee J., Hoppitt W., Uller T. (2013). More on how and why: Cause and effect in biology revisited. Biol. Philos. 28, 719–745. doi: 10.1007/s10539-012-9335-1
Lanan M. (2014). Spatiotemporal resource distribution and foraging strategies of ants (Hymenoptera: Formicidae). Myrmecol. News 20, 53–70.
l’Anson Price R., Dulex N., Vial N., Vincent C., Grüter C. (2019). Honeybees forage more successfully without the “dance language” in challenging environments. Sci. Adv. 5, 1-9. doi: 10.1126/sciadv.aat0450
l’Anson Price R., Grüter C. (2015). Why, when and where did honey bee dance communication evolve? Front. Ecol. Evol. 3. doi: 10.3389/fevo.2015.00125
Lasmar C. J., Bishop T. R., Parr C. L., Queiroz A. C., Schmidt F. A., Ribas C. R. (2021). Geographical variation in ant foraging activity and resource use is driven by climate and net primary productivity. J. Biogeogr. 48, 1448–1459. doi: 10.1111/jbi.14089
Latreille P. A. (1802). Histoire naturelle des fourmis, et recueil de mémoires et d'observations sur les abeilles, les araignées, les faucheurs, et autres insectes (Paris: Impr. Crapelet (chez T. Barrois), 445.
Leal I. R., Oliveira P. S. (1995). Behavioral ecology of the neotropical termite-hunting ant Pachycondyla (=Termitopone) marginata: colony founding, group-raiding and migratory patterns. Behav. Ecol. Sociobiol. 37, 373–383. doi: 10.1007/BF00170584
Lefebvre D., Pierre J., Outreman Y., Pierre J. S. (2007). Patch departure rules in Bumblebees: Evidence of a decremental motivational mechanism. Behav. Ecol. Sociobiol. 61, 1707–1715. doi: 10.1007/s00265-007-0402-6
Lehrman D. S. (1953). A critique of Konrad Lorenz’s theory of instinctive behavior. Q. Rev. Biol. 28, 337–363. doi: 10.1086/399858
Leitner N., Dornhaus A. (2019). Dynamic task allocation: how and why do social insect workers take on new tasks? Anim. Behav. 158, 47–63. doi: 10.1016/j.anbehav.2019.09.021
Lessig E. K., Nonacs P. (2021). Ant foraging path use responds to different types of risk and their encounter probabilities. Insect. Sociaux 68, 173–180. doi: 10.1007/s00040-021-00811-x
Levitis D. A., Lidicker W. Z., Freund G. (2009). Behavioural biologists do not agree on what constitutes behaviour. Anim. Behav. 78, 103–110. doi: 10.1016/j.anbehav.2009.03.018
Lima Vieira M. E., Teseo S., Azevedo D. L. O., Châline N., Araújo A. (2024). Competition through ritualized aggressive interactions between sympatric colonies in solitary foraging neotropical ants. Sci. Nat. 111, 4. doi: 10.1007/s00114-024-01891-y
Linnaeus C. (1758). Systema naturae per regna tria naturae, secundum classes, ordines, genera, species, cum characteribus, differentiis, synonymis, locis. Tomus I. Editio decima, reformata, Stockholm, SW: L. Salvii.
Lucas C., Ben-Shahar Y. (2021). The foraging gene as a modulator of division of labour in social insects. J. Neurogene. 35, 168–178. doi: 10.1080/01677063.2021.1940173
Ma R., Rangel J., Grozinger C. M. (2019). Honey bee (Apis mellifera) larval pheromones may regulate gene expression related to foraging task specialization. BMC Genomics 20, 1-15. doi: 10.1186/s12864-019-5923-7
MacArthur R. H., Pianka E. R. (1966). On optimal use of a patchy environment. Am. Nat. 100, 603–609. doi: 10.1086/282454
MacDougall-Shackleton S. A. (2011). The levels of analysis revisited. Philos. Trans. R. Soc. B: Biol. Sci. 366 (1574), 2076–2085.
Mailleux A. C., Detrain C., Deneubourg J. L. (2005). Triggering and persistence of trail-laying in foragers of the ant Lasius Niger. J. Insect Physiol. 51, 297–304. doi: 10.1016/j.jinsphys.2004.12.001
Mayr E. (1961). Cause and effect in biology. Science 134, 1501–1506. doi: 10.1126/science.134.3489.1501
Menzel R. (2023). Navigation and dance communication in honeybees: A cognitive perspective. J. Comp. Physiol. A 209, 515–527. doi: 10.1007/s00359-023-01619-9
Mersch D. P. (2016). The social mirror for division of labor: what network topology and dynamics can teach us about organization of work in insect societies. Behav. Ecol. Sociobiol. 70, 1087–1099. doi: 10.1007/s00265-016-2104-4
Nascimento F. S., Souza D. I. S. A., Tannure-Nascimento I. C., Dantas J. O. (2012). Social facilitation and food partitioning in the queenless ant Dinoponera quadriceps (Hymenoptera: Formicidae). J. Natural History 46, 1959–1967. doi: 10.1080/00222933.2012.700333
Nonacs P. (1990). Death in the distance: mortality risk as information for foraging ants. Behaviour 112, 23–35. doi: 10.1163/156853990X00662
Nonacs P., Dill L. M. (1990). Mortality risk vs. food quality trade-offs in a common currency: Ant patch preferences. Ecology 71, 1886–1892. doi: 10.2307/1937596
Nonacs P., Dill L. M. (1991). Mortality risk versus food quality trade-offs in ants: patch use over time. Ecol. Entomol. 16, 73–80. doi: 10.1111/j.1365-2311.1991.tb00194.x
Norman V. C., Hughes W. O. H. (2016). Behavioural effects of juvenile hormone and their influence on division of labour in leaf-cutting ant societies. J. Exp. Biol. 219, 8–11. doi: 10.1242/jeb.132803
Ocampo-Ariza C., Toledo-Hernández M., Librán-Embid F., Armenteras D., Vansynghel J., Raveloaritiana E., et al. (2023). Global South leadership towards inclusive tropical ecology and conservation. Perspect. Ecol. Conserv. 21, 17–24. doi: 10.1016/j.pecon.2023.01.002
Oliveira P. S., Hölldobler B. (1989). Orientation and communication in the neotropical ant odontomachus bauri emery (Hymenoptera, formicidae, ponerinae). Ethology 83, 154–166. doi: 10.1111/j.1439-0310.1989.tb00525.x
Orians G. H., Pearson N. E. (1979). “On the theory of central place foraging,” in Analysis of Ecological Systems. Eds. Horn D. J., Mitchell R. D., Stairs G. R. (The Ohio State University Press, Columbus), 154–177.
Page R. E., Waddington K. D., Hunt G. J., Fondrk M. K. (1995). Genetic determinants of honey bee foraging behaviour. Anim. Behav. 50, 1617–1625. doi: 10.1016/0003-3472(95)80015-8
Pasquier G., Grüter C. (2016). Individual learning performance and exploratory activity are linked to colony foraging success in a mass-recruiting ant. Behav. Ecol. 27, arw079. doi: 10.1093/beheco/arw079
Paul E. S., Sher S., Tamietto M., Winkielman P., Mendl M. T. (2020). Towards a comparative science of emotion: Affect and consciousness in humans and animals. Neurosci. Biobehav. Rev. 108, 749–770. doi: 10.1016/j.neubiorev.2019.11.014
Pellis S., Pellis V. (2021). Understanding animal behaviour: What to measure and why (Cambridge, UK: Cambridge university press).
Perez M., Rolland U., Giurfa M., D’Ettorre P. (2013). Sucrose responsiveness, learning success, and task specialization in ants. Learn. Memory 20, 417–420. doi: 10.1101/lm.031427.113
Perry C. J., Baciadonna L. (2017). Studying emotion in invertebrates: what has been done, what can be measured and what they can provide. J. Exp. Biol. 220, 3856–3868. doi: 10.1242/jeb.151308
Perry C. J., Barron A. B., Chittka L. (2017). The frontiers of insect cognition. Curr. Opin. Behav. Sci. 16, 111–118. doi: 10.1016/j.cobeha.2017.05.011
Pie M. R. (2004). Foraging ecology and behaviour of the ponerine ant Ectatomma opaciventre Roger in a Brazilian savannah. J. Natural History 38, 717–729. doi: 10.1080/0022293021000041699
Pinter-Wollman N. (2012). Personality in social insects: How does worker personality determine colony personality? Curr. Zool. 58, 580–588. doi: 10.1093/czoolo/58.4.580
Pinter-Wollman N., Bala A., Merrell A., Queirolo J., Stumpe M. C., Holmes S., et al. (2013). Harvester ants use interactions to regulate forager activation and availability. Anim. Behav. 86, 197–207. doi: 10.1016/j.anbehav.2013.05.012
Prato A., da Silva R. C., Assis D. S., Mateus S., Hartfelder K., do Nascimento F. S. (2021). Juvenile hormone affects age polyethism, ovarian status and cuticular hydrocarbon profile in workers of the wasp Polybia occidentalis. J. Exp. Biol. 224, 1-11. doi: 10.1242/jeb.240200
Ravary F., Lecoutey E., Kaminski G., Châline N., Jaisson P. (2007). Individual experience alone can generate lasting division of labor in ants. Curr. Biol. 17, 1308–1312. doi: 10.1016/j.cub.2007.06.047
Reeves D. D., Moreau C. S. (2019). The evolution of foraging behavior in ants (Hymenoptera: Formicidae). Arthropod System. Phyl. 77, 351–363. doi: 10.26049/ASP77-2-2019-10
Richardson T. O., Kay T., Braunschweig R., Journeau O. A., Rüegg M., McGregor S., et al. (2021). Ant behavioral maturation is mediated by a stochastic transition between two fundamental states. Curr. Biol. 31, 2253–2260.e3. doi: 10.1016/j.cub.2020.05.038
Richardson T. O., Sleeman P. A., McNamara J. M., Houston A. I., Franks N. R. (2007). Teaching with evaluation in ants. Curr. Biol. 17 (17), 1520–1526.
Robinson E. J. (2014). Polydomy: the organisation and adaptive function of complex nest systems in ants. Curr. Opin. Insect Sci. 5, 37–43. doi: 10.1016/j.cois.2014.09.002
Robinson G. E., Page R. E. (1989). Behavioral Ecology and Sociobiology Genetic determination of nectar foraging, pollen foraging, and nest-site scouting in honey bee colonies. Behav. Ecol. Sociobiol. 24, 317-323. doi: 10.1007/BF00290908
Robinson E. J. H., Richardson T. O., Sendova-Franks A. B., Feinerman O., Franks N. R. (2009). Radio tagging reveals the roles of corpulence, experience and social information in ant decision making. Behav. Ecol. Sociobiol. 63, 627–636. doi: 10.1007/s00265-008-0696-z
Roeder T. (2005). Tyramine and octopamine: Ruling behavior and metabolism. Annu. Rev. Entomol. 50, 447–477. doi: 10.1146/annurev.ento.50.071803.130404
Roeder K. A., Roeder D. V., Bujan J. (2021). Ant thermal tolerance: a review of methods, hypotheses, and sources of variation. Ann. Entomol. Soc. America 114, 459–469. doi: 10.1093/aesa/saab018
Roelofs S., Boleij H., Nordquist R. E., Van Der Staay F. J. (2016). Making decisions under ambiguity: judgment bias tasks for assessing emotional state in animals. Front. Behav. Neurosci. 10. doi: 10.3389/fnbeh.2016.00119
Sasaki K., Okada Y., Shimoji H., Aonuma H., Miura T., Tsuji K. (2021). Social evolution with decoupling of multiple roles of biogenic amines into different phenotypes in hymenoptera. Front. Ecol. Evol. 9. doi: 10.3389/fevo.2021.659160
Schafer R. J., Holmes S., Gordon D. M. (2006). Forager activation and food availability in harvester ants. Anim. Behav. 71, 815–822. doi: 10.1016/j.anbehav.2005.05.024
Schultheiss P., Raderschall C. A., Narendra A. (2015). Follower ants in a tandem pair are not always naïve. Sci. Rep. 5 (1), 10747.
Schultner E., Pulliainen U. (2020). Brood recognition and discrimination in ants. Insect. Sociaux 67, 11–34. doi: 10.1007/s00040-019-00747-3
Shaffer Z., Sasaki T., Pratt S. C. (2013). Linear recruitment leads to allocation and flexibility in collective foraging by ants. Anim. Behav. 86, 967–975. doi: 10.1016/j.anbehav.2013.08.014
Shettleworth S. J. (2010). Cognition, Evolution, and Behavior. 2nd ed. (Oxford, UK: Oxford University Press).
Silva J. P., Valadares L., Lima Vieira M. E., Teseo S., Châline N. (2021). Tandem running by foraging Pachycondyla striata workers in field conditions vary in response to food type, food distance, and environmental conditions. Curr. Zool. 67, 541–549. doi: 10.1093/cz/zoab050
Smith F. (1858). Catalogue of Hymenopterous Insects in the Collection of the British Museum (Part VI) (London, UK: Formicidae).
Sokolowski M. B. (1980). Foraging strategies of drosophila melanogaster: A chromosomal analysis. Behav. Genet. 10, 291–302. doi: 10.1007/BF01067774
Solvi C., Baciadonna L., Chittka L. (2016). Unexpected rewards induce dopamine-dependent positive emotion–like state changes in bumblebees. Science 353, 1529–1531. doi: 10.1126/science.aaf4454
Spirov A. V., Levchenko V. F., Sabirov M. A. (2021). Concepts of canalization and genetic assimilation in developmental biology: current approaches and studies. J. Evol. Biochem. Physiol. 57, 1–15. doi: 10.1134/S0022093021010014
Stamps J. A. (2016). Individual differences in behavioural plasticities. Biol. Rev. 91, 534–567. doi: 10.1111/brv.12186
Sumpter D. J. T., Beekman M. (2003). From nonlinearity to optimality: Pheromone trail foraging by ants. Anim. Behav. 66, 273–280. doi: 10.1006/anbe.2003.2224
Tanner C. J. (2009). Individual experience-based foraging can generate community territorial structure for competing ant species. Behav. Ecol. Sociobiol. 63, 591–603. doi: 10.1007/s00265-008-0694-l
Thompson E. (2010). Mind in Life: Biology, Phenomenology, and the Sciences of Mind (Cambridge, MA: Belknap Press).
Tinbergen N. (1963). On aims and methods of Ethology. Z. Für Tierpsychol. 20, 410–433. doi: 10.1111/j.1439-0310.1963.tb01161.x
Traniello J. F. A. (1989). Foraging strategies of ants. Annu. Rev. Entomol. 34, 191–210. doi: 10.1146/annurev.en.34.010189.001203
Udino E., Perez M., Carere C., d’Ettorre P. (2017). Active explorers show low learning performance in a social insect. Curr. Zool. 63, 555–560. doi: 10.1093/cz/zow101
Ulrich Y., Burns D., Libbrecht R., Kronauer D. J. C. (2016). Ant larvae regulate worker foraging behavior and ovarian activity in a dose-dependent manner. Behav. Ecol. Sociobiol. 70, 1011–1018. doi: 10.1007/s00265-015-2046-2
Urbani C. B. (1989). Phylogeny and behavioural evolution in ants, with a discussion of the role of behaviour in evolutionary processes. Ethol. Ecol. Evol. 1, 137–168. doi: 10.1080/08927014.1989.9525520
Varela F. J., Thompson E., Rosch E. (1991). The embodied mind: Cognitive science and human experience (Cambridge, MA: MIT Press).
Veit W. (2023). A philosophy for the science of animal consciousness (First edition) (Abingdon, UK: Routledge).
von Frisch K. (1993). The Dance Language and Orientation of Bees (Cambridge, MA: Harvard University Press). doi: 10.4159/harvard.9780674418776
Waage J. K. (1979). Foraging for patchily-distributed hosts by the parasitoid, nemeritis canescens. J. Anim. Ecol. 48, 353–371. doi: 10.2307/4166
Walsh J. T., Garnier S., Linksvayer T. A. (2020). Ant collective behavior is heritable and shaped by selection. Am. Nat. 196, 541–554. doi: 10.1086/710709
Ward P. S., Brady S. G., Fisher B. L., Schultz T. R. (2015). The evolution of myrmicine ants: phylogeny and biogeography of a hyperdiverse ant clade (hymenoptera: Formicidae). System. Entomol. 40, 61–81. doi: 10.1111/syen.12090
Webster M. M., Ward A. J. W. (2011). Personality and social context. Biol. Rev. 86, 759–773. doi: 10.1111/j.1469-185X.2010.00169.x
Wehner R. (2020). Desert Navigator: The Journey of an Ant (Cambridge, MA: Belknap Press: An Imprint of Harvard University Press).
Wehner R., Hoinville T., Cruse H. (2023). On the ‘cognitive map debate’ in insect navigation. Studies in History and Philosophy of Science 102, 87–89. doi: 10.1016/j.shpsa.2023.08.004
Wendt S., Strunk K. S., Heinze J., Roider A., Czaczkes T. J. (2019). Positive and negative incentive contrasts lead to relative value perception in ants. ELife 8, e45450. doi: 10.7554/eLife.45450.001
Wystrach A. (2021). Movements, embodiment and the emergence of decisions. Insights from insect navigation. Biochem. Biophys. Res. Commun. 564, 70–77. doi: 10.1016/j.bbrc.2021.04.114
Yagound B., Crowet M., Leroy C., Poteaux C., Châline N. (2017). Interspecific variation in neighbour–stranger discrimination in ants of the Neoponera apicalis complex. Ecol. Entomol. 42, 125–136. doi: 10.1111/een.12363
Keywords: social insects, Ponerinae, mechanisms, ontogeny, development, evolution, function, integrative studies
Citation: Lima Vieira ME, Chameron S and Châline N (2024) The ethology of foraging in ants: revisiting Tinbergen’s four questions. Front. Ethol. 3:1341120. doi: 10.3389/fetho.2024.1341120
Received: 19 November 2023; Accepted: 06 February 2024;
Published: 05 March 2024.
Edited by:
Shizuko Hiryu, Doshisha University, JapanReviewed by:
Cintia Akemi Oi, University College London, United KingdomMatthew Lovern, Oklahoma State University, United States
Copyright © 2024 Lima Vieira, Chameron and Châline. This is an open-access article distributed under the terms of the Creative Commons Attribution License (CC BY). The use, distribution or reproduction in other forums is permitted, provided the original author(s) and the copyright owner(s) are credited and that the original publication in this journal is cited, in accordance with accepted academic practice. No use, distribution or reproduction is permitted which does not comply with these terms.
*Correspondence: Nicolas Châline, bmNoYWxpbmVAdXNwLmJy