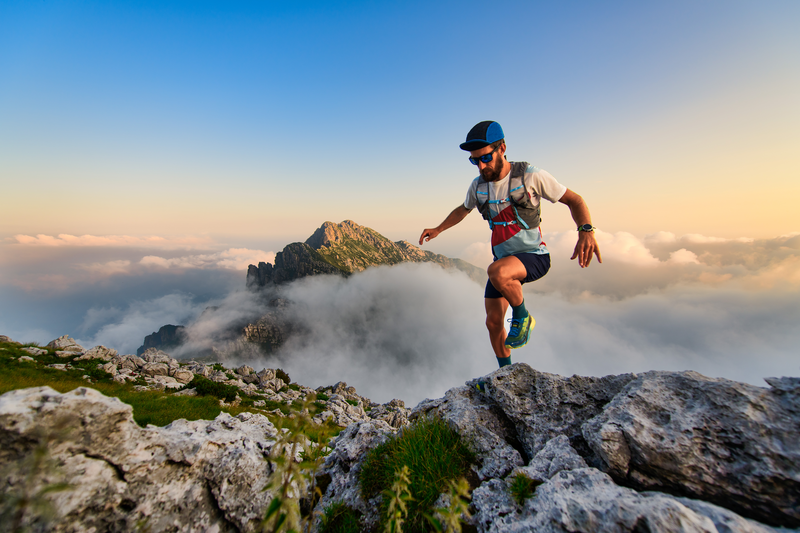
94% of researchers rate our articles as excellent or good
Learn more about the work of our research integrity team to safeguard the quality of each article we publish.
Find out more
REVIEW article
Front. Chem. , 10 December 2024
Sec. Green and Sustainable Chemistry
Volume 12 - 2024 | https://doi.org/10.3389/fchem.2024.1485354
This article is part of the Research Topic Renewable Chemistry View all 5 articles
Platform chemicals obtained from biomass will play an important role in chemical industry. Already existing compounds or not yet established chemicals are produced from this renewable feedstock. Using photochemical reactions as sustainable method for the conversion of matter furthermore permits to develop processes that are interesting from the ecological and economical point of view. Furans or levoglucosenone are thus obtained from carbohydrate containing biomass. Photochemical rearrangements, photooxygenation reactions or photocatalytic radical reactions can be carried out with such compounds. Also, sugars such pentoses or hexoses can be more easily transformed into heterocyclic target compounds when such photochemical reactions are used. Lignin is an important source for aromatic compounds such as vanillin. Photocycloaddition of these compounds with alkenes or the use light supported multicomponent reactions yield interesting target molecules. Dyes, surfactants or compounds possessing a high degree of molecular diversity and complexity have been synthesized with photochemical key steps. Alkenes as platform chemicals are also produced by fermentation processes, for example, with cyanobacteria using biological photosynthesis. Such alkenes as well as terpenes may further be transformed in photochemical reactions yielding, for example, precursors of jet fuels.
Sustainability play a key role for the development of mankind. In the case of chemical industry, this has been recognized very early. More than 100 years ago, G. Ciamician has published ideas about a non-polluting chemical industry based on photochemical and enzymatic reactions for the production of biomass as it is done by green plants (Ciamician, 1912; Ciamician, 1908). In fact biological organisms using photosynthesis constitute the biggest chemical industry with an annual production of 1.7 ∙ 1011 t per year (Lichtenthaler and Peters, 2004). Lignocellulose represents the major part of biomass (Shinde et al., 2020). It is mainly composed of carbohydrates (C6 sugar based material such as celluloses or starch and C5 sugar based material such as hemicelluloses) and on lignin which is an important source of aromatic compounds and an important source of platform chemicals. Various criteria for sustainable or green chemistry have been defined (Anastas and Kirchhoff, 2002). Approaching most closely the methods of chemical production to those used by nature is one of the strategies for a sustainable chemical industry. Another one is the optimization of already existing processes in view to reduce the environmental impact. This can be done, for example, by diminishing waste formation, simplifying the production processes by reducing the number of steps in multi-step syntheses or by using renewable feedstock.
In this regard, biomass as renewable feedstock play an important role. The molecular structure of biomass is different form corresponding fossil carbon compounds (Shinde et al., 2020; Wertz and Bédué, 2013; Barrault et al., 2018; Marion et al., 2017; Ravelli and Samorì, 2021; Gallezot P., 2012; Behr and Seidensticker, 2018). For this reason, transformations or production processes can be carried out and multi-step syntheses can be simplified which makes them more competitive form the economic and ecological point of view (Tietze, 1996; Groß et al., 2020). In the context of academic research, a lot of syntheses have been published with much more than 25 steps. Especially in the context of an industrial application and the environmental impact, these research approaches have been criticized (Tietze et al., 2000; Hudlicky, 1996; Tietze and Tietze, 2014). For example, when oxygen rich compounds are needed, they should preferentially be produced from carbohydrates because this renewable feedstock is oxygen rich (Lichtenthaler and Peters, 2004; Lichtenthaler et al., 2006) and the number of oxidation steps can be diminished (Levy and Fügedi, 2006). In some corresponding multi-step syntheses with fossil platform chemicals, complex chemo-, regio- or stereoselective oxidations are involved which makes them less competitive. In general, platform chemicals are key elements of the chemical industry as far as the production of bulk products or fine chemicals is concerned. The transformation of such compounds originating from biomass under sustainable conditions is therefore significant for the development of the chemical industry (Sheldon, 2014; Farmer et al., 2015; Shinde et al., 2020; Arias et al., 2020).
In the same context, organic photochemical reactions may be discussed. Using such reactions, compounds or compound families can be produced that are not or difficultly available by more conventional methods of organic synthesis (Turro and Schuster, 1975; Hoffmann, 2008; Bach and Hehn, 2011; Kärkäs et al., 2016a; Liu and Li, 2017; Zhu et al., 2024). This behavior is explained by the fact that photochemical excitation changes the electronic configuration of a molecule (Klán and Wirz, 2009). Many photochemical reactions are carried out without additional chemical reagents and activation of the starting compound occurs only by absorption of a photon. In this context, the photon is considered as a traceless reagent (Hoffmann, 2012; Oelgemöller et al., 2007) These reactions now gain in interest in the chemical industry (André et al., 1992; Braun et al., 1991; Bonfield et al., 2020; Moschetta et al., 2024). Recent activities in the domain of chemical engineering of photochemical reactions favor this interest (Elliott et al., 2014; Oelgemöller, 2014; Loubière et al., 2016; Noël, 2017; Zondag et al., 2023) In the context, of sustainable chemistry, it should also be mentioned that photochemical reactions can be carried out with sunlight as a renewable energy source (Oelgemöller, 2016). Some of such procedures are interesting in the context of an industrial application. Organic photochemistry has recently experienced a rebirth due to a wide range of work with different kinds of photocatalysis (Michelin and Hoffmann, 2018a; Michelin and Hoffmann, 2018b), especially photoredox catalysis applied to organic synthesis must be mentioned here (König, 2020; Stephenson et al., 2018; Marzo et al., 2018; Nicholls et al., 2016). Photochemical reactions are also studied in the context of depolymerization of biomass (Ouyang et al., 2022; Wu et al., 2020; Chen et al., 2021; Rao et al., 2021; Nwosu et al., 2021).
Both approaches, the transformations of biomass or biomass derived chemicals and the application of photochemical reactions significantly extend the space of chemical structures (Gómez Fernández and Hoffmann, 2023). The combination of these approaches also opens perspectives for a sustainable chemical industry. The present review deals with typical photochemical transformations of corresponding platform chemicals. The production of novel compounds is particularly focused.
Carbohydrates of carbohydrate based biopolymers are an important source of furans and many other compounds (Guigo et al., 2021; Mika et al., 2018). Furan compounds undergo easily photooxygenation involving singlet oxygen (Gollnick and Griesbeck, 1985; Montagnon et al., 2014; Montagnon et al., 2016). Among various methods (Nardello-Rataj et al., 2016), the photochemical production of singlet oxygen is a particularly attractive one (Ghogare and Greer, 2016; Bartoschek et al., 2005). In this case, the singlet species (Minaev, 2007; Schweitzer and Schmidt, 2003; Mittal et al., 2020) is generated form triplet oxygen by sensitization (Scheme 1). After photochemical excitation to the singlet state, the sensitizer (sens) undergoes intersystem crossing (isc) to the triplet state. Possessing the same spin multiplicity as oxygen at its ground state an interaction of both species is spin allowed. The sensitizer returns to its singlet ground state while the oxygen is excited to its singlet state. As the singlet energy of oxygen is relatively low (23 kcal mol−1), a large variety of sensitizers are used such as almost all kinds of dyes, organic and inorganic semiconductors, coordination compounds or nanoparticles with corresponding properties.
Furfural 1 is a furan derivative that is easily obtained from pentoses or hemicelluloses by dehydration (Zeitsch, 2000; Kabbour et al., 2020; Martel et al., 2010; Jaswal et al., 2022). The photooxygentation of this compound is very efficient and yields 5-hydroxy-2(5H)-furanone 2 (Scheme 2) (Schenck, 1953). The reaction starts with the addition of singlet oxygen leading to the endo peroxide 3 (Cottier et al., 1986). The reaction is often carried out with alcohols as solvent, in particular methanol or ethanol which attacks the endo peroxide intermediate 3 at the aldehyde function. Hydroxyfuranone 2 is generated by release of a corresponding formic ester 4.5-hydroxy-2(5H)-furanone 2 is also a platform chemical (Esser et al., 1994; Badovskaja et al., 2021; Palai et al., 2024). It is easily transformed into corresponding 5-alcoxy-2(5H)-furanones like 5 or acyclic compounds such as 6 or 7 that are flexible synthesis intermediates (Scharf and Janus, 1978). Hydroxyfuranone 2 was used, for example, in asymmetric synthesis (Marinković et al., 2004; Feringa and de Jong, 1992; Moradei and Paquette, 2003; Riguet, 2011). In this context, studies on chiral induction in reactions of such furanones at their excited state should be mentioned. Due to photochemical excitation, the structures changes which also modify steric hindrance and relevant stereoelectronic effects (Marinković et al., 2004; Hoffmann et al., 1994; Hoffmann and Scharf, 1991; Bertrand et al., 1998; Fréneau et al., 2016).
The photooxygenation of furfural can be carried out on large scale in the laboratory. Recently, an experimental procedure for the transformation of 100 g in 1.5 L of ethanol has been reported in detail (Desvals et al., 2022). Large scale transformations for 40-L-solutions have been carried out using sunlight (Esser et al., 1994). As a recent example of an application to organic synthesis, ethoxyfuranone 5 was transformed into a polymethine dye (Scheme 3) (Desvals et al., 2022). In the presence of bromine, the α-bromo derivative 8 is formed which leads to an increase of the oxidation state in this position. Thus hydrolysis yields the malondialdehyde intermediate 9 or its tautomer 10. Condensation with thiobarbituric acid derivatives such as 11 yields oxonol dyes 12. The present synthesis enabled a physico-chemical characterization of such dyes. These dyes play an important role in the photometric detection and quantification of enzyme activities (Unger, 1981; Nakashima et al., 1983) such as pectinlyase (Nedjma et al., 2001). In such tests, the intermediates 9 and 10 are generated from corresponding metabolites.
Scheme 3. Synthesis of polymethine dyes of oxonol type starting with ethoxyfuranone 5 obtained from hydroxyfuranone 2.
Further applications to the synthesis of biodegradable surfactants have been reported (Gassama et al., 2013; Gassama et al., 2009; Yue and Queneau, 2022). Furanone derivatives are interesting monomers for radical polymerization (Le Dot et al., 2024). Nevertheless, they undergo difficultly homo-polymerization. It was shown that copolymerization of alcoxyfuranones with electron rich monomers such as enolethers is very efficient (Poskonin et al., 1999; Lepage et al., 2023). The addition of photochemically generated radicals (Hoffmann, 1994; Bertrand et al., 2000a; Bertrand et al., 2000b; Harakat et al., 2006; Hoffmann et al., 2006) to furanones using ketones as sensitizer has been carried in continuous flow reactors (Yavorskyy et al., 2012; Yavorskyy et al., 2011) or microreactors (Shvydkiv et al., 2010). This reaction is suitable for the evaluation of different kinds of these reactors (Shvydkiv et al., 2011; Oelgemöller et al., 2014). Similar reactions have been carried out with inorganic semi-conductors as sensitizer (Marinković and Hoffmann, 2001; Marinković and Hoffmann, 2003; Marinković and Hoffmann, 2004).
As mentioned in previous paragraphs furans are obtained by dehydration of carbohydrates (Takkellapati et al., 2018). The efficiency of this process depends on the structure of the sugar precursors. A corresponding dehydration sequence is efficient when furanoses react, partly because these compounds contain the five membered ring of furans. The equilibrium between a pyranose and furanose form must be shifted to the furanose. Glucose is a major element of biomass and its transformation by dehydration into the corresponding hydroxymethylfurfural (HMF) and corresponding derivatives such as 2,5-furandicarboxylic acid or 2,5-diformylfuran is of high interest (for selected reviews see: Yue and Queneau, 2022; van Putten et al., 2013; Hou et al., 2021; Li, 2023; Post et al., 2023; de Vries, 2017; Al Ghatta and Hallett, 2023; Zhang S. et al., 2023; Velty et al., 2022; Shinde and Rode, 2020; Lewkowski, 2001). The selective dehydration of a furanose moiety in the presence of a pyranose structure has well been performed in the case of isomaltulose (Scheme 4) (Lichtenthaler et al., 1993). This disaccharide is produced by enzymatic isomerization of saccharose (Hagen and Lorenz, 1957). The dehydration of isomaltulose yields Glucosylmethylfurfural (GMF) 13. This compound is an interesting synthesis intermediate for the preparation of numerous bioinspired molecular structures (Tan et al., 2015). It should be pointed out that such α-annomeric derivatives of glucose are difficultly available by conventional synthesis techniques of carbohydrate chemistry (Levy and Fügedi, 2006). For different proposes, protecting groups can be introduced (14). In this case, the photooxygenation under conditions previously described for the transformation of furfural yields two epimers of hydroxyfuranone 15. After reduction, the two furanone derivatives 16 and 17 have been obtained (Jahjah et al., 2010). In the present case, a study on stereoelectronic effect in photochemically induced hydrogen atom transfer reactions (HAT) (Jahjah et al., 2010; Hoffmann, 2016; Hoffmann, 2015; Hoffmann, 2017) was carried out. The photooxydation conditions are compatible with the presence of a variety of functional groups. Thus 5-(azidomethyl)furfural was transformed with a similar reaction sequence into 5-aminolevolinic acid hydrochlorid that is a natural herbicide (Mascal and Dutta, 2011). Hydroxymethyl furanones also called hydroxymethyl butenolides are also valuable synthons for a broader application to organic synthesis (Flourat et al., 2020). Photooxygenation processes at the industrial scale are well known (Rojahn and Warnecke, 1980; Turconi et al., 2014; Wau et al., 2021).
Scheme 4. Selective dehydration of a furanosyl moiety in the presence of a pyranosyl group in isomaltulose. Photooxygenation of the furan substituent.
Sugars are considered as platform chemicals when they can be transformed in only few steps into interesting target molecules (Jäger and Minnaard, 2016). Due to the presence of numerous hydroxyl functions in these compounds, selective transformations often need a laborious strategy using protecting groups (Levy and Fügedi, 2006; Kocieński, 2005; Wuts, 2014). In this context, methods are required that enables a selective transformation, for example, of a single hydroxyl function into reactive a carbonyl group. A lot of enzymes enable such transformations. Thus galactose oxidase catalyzes the selective oxidation of the hydroxyl function in the position 6 of galactose into the corresponding aldehyde (Ito et al., 1991). Also artificial catalysts have been developed to imitate such enzyme activities in the context of biomimetic transformations (Pierre, 2000; Chaudhuri et al., 1999; Berkessel et al., 2005; Thomas, 2007; Lewis and Tolman, 2004; Mirica et al., 2004). Such an example is depicted in Scheme 5 (Gassama and Hoffmann, 2008). The galactose oxidase catalyzes the oxidation of compounds like 18 into the corresponding aldehydes 19. Such products are in equilibrium with their half acetale forms 20 which stabilizes these derivatives and consecutive transformations can be envisaged. In the present case, the oxidation has been carried out using Semmelhack reaction conditions (Semmelhack et al., 1984) with CuCl and TEMPO (2,2,6,6-Tetramethylpiperidinyloxyl) as catalysts and air as oxidant. These conditions are suitable for the oxidation of primary alcohols (Ryland and Stahl, 2014). Under the reported reaction conditions, the oxidation of the compounds such as 21 or 24 was inefficient. However, when carried out under irradiation with visible light, the reaction became efficient. A further improvement was achieved when the reaction mixture was subjected to a reductive amination. The resulting compounds 22 and 25 after deprotection and reductive amination yielded the azepane derivatives 23 and 26. Such compounds possess interesting pharmaceutical activities (Compain and Martin, 2007; Li et al., 2009; Désiré et al., 2014). As the examples show, this strategy for the synthesis of azepanes can be applied to a larger variety of hexoses with different relative and absolute configuration while a corresponding application of enzymes such as the galactose oxidase is limited to particular stereoisomers. The mechanism depicted in Scheme 6 has been suggested in which Cu(II) acts as the oxidant of the alcohol species (27) (Dijksman et al., 2003). The resulting Cu(I) is reoxidized to Cu(II) by addition of TEMPO (28) and the release of TEMPOH. The positive effect of irradiation with visible light can be explained by the fact that the Cu-O bond is weakened when such complexes are electronically excited via a ligand to metal charge transfer (LMCT). Thus ligand exchange steps in the mechanism are accelerated (Abderrazak et al., 2021).
Scheme 5. Synthesis of tetrahydroxyazepanes form glucose and mannose derivatives imitating galactose oxidase.
Recently levoglucosenone became an interesting platform chemical (Camp and Greatrex, 2022). It is obtained from cellulose by pyrolysis under acidic conditions (Scheme 7) (De bruyn et al., 2016; Halpern et al., 1973; He et al., 2017; Klepp et al., 2020). This compound is now produced on industrial scale as an intermediate in the production of Cyrene™, a biobased aprotic dipolar solvent (Sherwood et al., 2014; Citarella et al., 2022). A relatively high number of functional groups are located on a small enantiopure compound. Thus levoglucosenone is an interesting synthon for asymmetric synthesis (Comba et al., 2018; Awad et al., 2006; Sarotti et al., 2012; Tsai et al., 2018). It can also be transformed into other platform chemicals such as furanones (Diot-Néant et al., 2018; Bonneau et al., 2018). Also Cyrene™, is used as synthon in organic synthesis (Stini et al., 2022).
Hitherto, only few photochemical reactions with levoglucosenone have been reported. When levoglucosenone 29 is electronically excited by light absorption, a Norrish type I reaction occurs yielding the diradical intermediate 30 (Scheme 8) (Yamada and Matsumoto, 1992). Rearrangement yields the ketene 31 which is trapped by an alcohol leading to the corresponding ester 32. Trans-substituted alkenes 33 and 34 are also formed, most probably via sensitization. Similar steps are often observed in Norrish type I reactions (Bohne, 1995; Majhi, 2021). In this transformation, alkenes with an interesting substitution pattern are obtained.
Scheme 8. The photochemical reactivity of levoglucosenone is dominated by a Norrish type I reaction.
However, the complex bicyclic structure is destroyed and the chiral information is lost. In this context photocatalysis with light of longer wavelengths enables photochemical transformations. Under such reaction conditions, a catalytic system absorbs light while the substrate remains at its ground state. Tungstates such as tetrabutylammonium decatungstate (TBADT) are capable of generating radical species (Scheme 9) (Tanielian, 1998; Ravelli et al., 2016; Hong and Indurmuddam, 2024). Such intermediates can be generated by hydrogen atom transfer (HAT) in different ways. In the present context, two processes are often discussed: (a) The proton and the electron are transferred simultaneously or (b) the electron is transferred first and the proton follows (Hoffmann, 2016; Hoffmann, 2015). In a more general context, these processes are part of proton-coupled electron transfer (PCET) (Hoffmann, 2017; Miller et al., 2016; Murray et al., 2022; Tyburski et al., 2021). Also single electron transfer (SET) is observed with these catalysts. The reaction conditions are particularly mild so that also complex polyfunctional substrates such as morphine derivatives can be selectively transformed (Gorbachev et al., 2022).
Using TBADT as photocatalyst, a variety of radical species were generated and added to levoglucosenone 29 (Scheme 10) (Lefebvre et al., 2022). Thus adducts with alkanes (35a, 35b, 35c or 35d) have been obtained. The addition of formamidyl radicals (35e) was particularly efficient. Cyclic ethers (35f) have also been added. A large number of acyl radicals generated from corresponding aldehyde precursors have been added. Adducts with benzaldehyde derivatives (35g and 35h) have been synthesized. Also heterocyclic aldehydes (35i or 35j) and aliphatic aldehydes (35k) have been added. The photosensitization with TBADT of the radical addition is generally efficient in the transformation of aromatic aldehydes (Raviola et al., 2019; Qiao et al., 2022). Some recent works particularly deals with reactions of furfural (Nielsen et al., 2024). The radical addition occurred stereospecifically anti with respect to the (CH2-O)-bridge in levoglucosenone. An energy difference of the transition states for both diastereotopic attacks of the radical intermediates of 5 kcal∙mol−1 was calculated. The high stereoselectivity qualifies the reaction for application to asymmetric synthesis. The particular regioselectivity of the radical addition was observed in the case of cyclopentanone 36 (Scheme 11) (Lefebvre et al., 2022). One should expect the formation of a radical species in the α position of the cyclic ketone due to an increased stability by a mesomeric effect in the resulting intermediate. However, the reaction took place in the β position yielding adduct 35l. This observation has been explained by the fact that at the transition state (TS) of the hydrogen atom transfer (HAT) step, a positive partial charge is generated at the hydrogen donor partner. In the case of cyclopentanone 36 this is favorable for a reaction in the β position. Detailed investigations of the stereo- and regioelectronic effects in this step have been carried out (Okada et al., 2014; Yamada et al., 2017; Ravelli et al., 2018; Roberts, 1999). The regio and stereoselectivity of such reactions are very high. In the addition of cyrene™ 37, only two isomers of 32 possible products have been isolated. The hydrogen atom transfer from the β-position of 37, yields the highly symmetric adduct 35m. The reaction at the (CH2-O)-bridge of 37 yields the adduct 35n. The reaction mechanism for the addition of cyclopentanone is presented in Scheme 12. After excitation of the decatungstate, a hydrogen atom is transferred from the cyclopentanone to the photocatalyst yielding the radial intermediate 38. After addition of the latter to levoglucosenone 29, the electrophilic oxoallyl radical 39 is formed. In the final step, a hydrogen atom is transferred from the reduced photocatalyst species 40 to the intermediate 39 yielding the final product 35l. In this step, the photocatalyst is regenerated.
Scheme 11. Unusual regioselectivity in the reaction of cyclopentanone 36 to levoglucosenone 29. Addition of cyrene™ 37 to levoglucosenone 29.
Lignin is an important renewable source of aromatic compounds, especially of phenol derivatives (Farmer et al., 2015; Argyropoulos et al., 2023; Heitner et al., 2010; Zhang and Wang, 2022). However, depolymerization of this complex material for the production of aromatic compounds is challenging (Subbotina et al., 2021; Li et al., 2024; Zhang C. et al., 2023; Zhou et al., 2022). Among other methods, also photochemical, especially photocatalytic reactions are investigated in this context (Li et al., 2016; Zakzeski et al., 2010; Rinaldi et al., 2016; Kärkäs et al., 2016b; Magallanes et al., 2019; Zhang, 2018; Das and König, 2018; Niguyen et al., 2020). Very often, such reactions have been carried out with model compounds. Vanillin is one of the monomers which is currently produced from lignin on the industrial scale (Backa et al., 2012; Bjørsvik and Liguori, 2002; Fache et al., 2016; Araújo et al., 2010; Nayak et al., 2023).
Concerning photochemical transformations, the reactivity of electronically excited aromatic compounds is significantly different from their ground state reactivity. At the ground state, these compounds possess aromatic character. At the excited state (Franck Conton state) they are anti-aromatic (Rosenberg et al., 2014; Yan et al., 2023). Consequently, they become particularly reactive. In contrast to many ground state reactions, photochemical reactions are characterized by a high tendency to avoid the aromatic stabilization in the final products. This property is particularly interesting for application to organic synthesis since a high degree of molecular complexity is generated in such reactions (Hoffmann et al., 2016). The photochemical cycloadditions of electronically excited aromatic compounds with alkenes are typical examples (Hoffmann, 2012; Remy and Bochet, 2016; Hoffmann, 2004). Generally, three types of such reactions are observed with benzene derivatives (Scheme 13) (Cornelisse, 1993; Cornelisse et al., 2001). The [2+2] and the [2+3] photocycloaddition are often observed as competing reactions. The product ratios often depend on the substitution pattern or the redoxpotentials of the reaction partners (McCullough, 1987; Müller and Mattay, 1993; Desvals and Hoffmann, 2023). While the [2+3] was often applied to the synthesis of complex compounds (Hoffmann, 2012; Remy and Bochet, 2016; Desvals and Hoffmann, 2023; Wender et al., 1990; De Keukeleire and He, 1993; Streit and Bochet, 2011; Hoffmann et al., 2005; Zhang et al., 2020), the [2+2] photocycloaddition is only recently and in systematic way applied to organic synthesis (Gilbert and Bach, 2023; Proessdorf et al., 2022). In the case of the [2+3] photocycloaddition and its application to the synthesis of natural products, it was shown that the efficiency is improved when the reaction is carried out under continuous flow conditions, which open perspectives for large-scale transformations (Alshammari et al., 2024). The [2+4] cycloaddition is less frequently observed in such transformations.
Scheme 13. Three types of photocycloadditions of electronically excited benzene derivatives with alkenes.
In this context, intamolecular photocycloadditions of vanillin derivatives have been investigated. When compounds such as 41 – the aldehyde group was transformed into a nitrile function – are irradiated at λ = 300 nm, two types of products are formed (Scheme 14) (Desvals et al., 2021). The linear triquinane derivatives 43 and the corresponding angular derivatives 42 result from a [2+3] cycloaddition while tricyclic cyclobutene compounds 44 and 45 result from an initial [2+2] cycloaddition followed by thermal and photochemical rearrangements. Also, angular (44) and linear regioisomers (45) for this compound family are formed. The product ratio depends on the substitution pattern. In the case of the [2+3] adducts, the linear isomer 43 absorbs light at λ = 300 nm. Consequently, this compound is transformed into the angular isomer 42. It was also shown that the product ratio depends on the spin multiplicity of the electronically excited benzene moiety. Reactions depicted in Scheme 14 are singlet processes. Reactions at the triplet state are sensitized transformations in which triplet energy is transferred to the aromatic substrate. These reactions are less efficient and different isomers only resulting from initial [2+2] photocycloaddition followed by thermal and photochemical rearrangements are isolated. Reaction mechanisms of the singlet reactions of vanillin derivatives are depicted in Scheme 15. In the case of the main products resulting from a [2+3] cycloaddition, the alkene is added at the 1,3 positions of the photochemically excited benzene moiety and the intermediate 47 is generated. Due to the singlet multiplicity and the presence of polar substituents – the methoxy and the cyano group – this intermediate possesses zwitterionic character. Charge combination may occur in two ways. Path a yields the angular isomer 48 and path b generates the linear isomer 49. In the case of an initial [2+2] photocycloaddition in positions 1 and 2, the primary adduct 50 undergoes electrocyclic ring opening in a thermal disrotatory process involving 6 electrons and yielding the cyclooctatriene intermediate 51. A photochemical disrotarory involving 4 electrons yields the final product 52. In this case, only the angular isomer is generated. It must be pointed out that such pericyclic reactions steps are reversible. In the case of photochemical reactions, often photostationary equilibria are involved. For example, primary [2+2] adducts such as 50 also absorbs light and cycloreversion or retrocycloaddition may become efficient. In these cases, no photochemical conversion is observed under standard conditions. Primary photocycloadducts can be trapped, for example, by an acid catalyzed reaction and the photostationary equilibrium is displaced towards the product site and photoproducts can be isolated (Hoffmann and Pete, 1995; Hoffmann and Pete, 1997; Hoffmann et al., 2002; Hoffmann, 2002). Such conditions extend the scope of these reactions and further application to organic synthesis are envisaged. For example, rigidified dopamine analogues (Verrat et al., 2000; Verrat, 2000) or compounds possessing the of 5,5-dialkylcyclohexane-1,3-dione core structure of a herbicide family (Hoffmann and Pete, 2001) have been synthesized with this reactions as a key step.
Scheme 14. Intramolecular photocycloaddition of vanillin derivatives. Products result from an initial [2+3] or [2+2] photocycloaddition.
Scheme 15. Mechanisms for the formation of complex molecules resulting from initial [2+3] or [2+2] photocycloaddition. These reactions occur at the singlet state.
Photochemical reactions have been carried out also with hydrazones or oximes and related compounds derived from aromatic aldehydes (Latrache and Hoffmann, 2021). Thus oximes of vanillin and related compounds derived from lignin are transformed into corresponding oximes. Under photochemical conditions, they are transformed into nitriles (Ban et al., 2019; Joy et al., 2022). Condensation of vanillin and or syringaldehyde with Meldrum’s acid yields UV-A and blue light filters (Peyrot et al., 2020). Such compounds possessing a phenol moiety have also radical trapping properties. Therefore, they are particularly safe compared to established sunscreen compounds.
Aromatic aldehydes are suitable synthons for organic synthesis. An enormous number of syntheses with these compounds are reported, among them multi component reactions (Dömling et al., 2012; Nazeri et al., 2020). In a three component photocatalyzed reaction, vanillin 53 reacts with aniline 54 and tetrahydrofurane (THF) 55 yielding compound 56 (Scheme 16) (Pillitteri et al., 2021). As previously explained radical intermediates 57 are generated from THF 55 using photocatalysis with TBADT (Tanielian, 1998; Ravelli et al., 2016; Hong and Indurmuddam, 2024). Imine intermediates are formed by condensation of vanillin 53 with aniline 54. The alkyl radical 57 selectively adds to the imine 58 leading to the intermediate 59. The latter is reduced by hydrogen atom transfer from the photocatalyst. In this step, the final product 56 is formed and the catalyst is regenerated.
Scheme 16. Photocatalytic radical addition to an imine (58) as key step in a three component reaction with vanillin 53.
Most of the biomass contains oxygen rich compounds. On the other hand, traditionally chemical industry uses huge quantities of oxygen-free compounds such as alkenes as platform chemicals. Although several compounds, for example, terpenes or natural rubber are synthesized by plants, biotechnological processes are developed to produce alkenes on large scale (Lee et al., 2019; Wilson et al., 2018; van Leeuwen et al., 2012; Saaret et al., 2021). The transformation of oxygen rich biomass derived platform chemicals to alkenes is systematically studied (Nakagawa et al., 2023).
Recently, a process was developed for the production of C10 cycloalkanes which fulfill requirements of jet fuels (Rana et al., 2022). A photobiological transformation is followed by a photochemical reaction. Using photosynthesis, the cyanobacterium Synechocystis transforms CO2 into terpenes. In order to favor the production of isoprene, genetic modifications have been carried out (Figure 1). Such methods enable the non-farming production of biomass. Photosensitized dimerization of isoprene 60 yielded a variety of [2+2] 61, 62 and 63, [2+4] 64 and 65 and [4+4] cycloadducts 66 and 67 (Scheme 17) (Rana et al., 2022). The reaction was first carried out with benzophenone as sensitizer (Hammond et al., 1963). It was found that the product ratio depends on the triplet energy of the sensitizer (Liu et al., 1965). In the present study, the reaction was further optimized by using the dinaphthylketone 68 as sensitizer. Various other ketones were less efficient. Although, this ketone absorbs light close to the visible domain, its triplet energy is still high enough to excite isoprene 60 to the triplet state by energy transfer. Using a particular setup in which the mixture of isoprene 60 and the sensitizer 68 (0.1 mol%) was kept in a sealed fluorinated ethylene propylene tube, cooled to ∼10°C and irradiated at (λ = 365 nm) the mixture of dimers was obtained with 89% yield (120 ml scale, product quantum yield Φ = 0.91). The reaction was also carried out with solar irradiation or irradiation with a sunlight simulator. A detailed computational investigation of the reaction mechanism was carried out (Vajravel et al., 2023). In order to get the jet fuel compounds, the product mixture of the photoreaction was hydrogenated using Pd/C as catalyst. Similar reaction conditions have been studied for the photodimerization and cross dimerization of various terpenes (Cid Gomes et al., 2023). The [2+2] photocycloaddition as key step for the production of jet fuels was also studied with furfural derived compounds (Lebedeva et al., 2024) or with terpenes (Xie et al., 2019). It should be pointed out the present process as a combination of photobiological and a photochemical transformation perfectly corresponds to the requirement of a sustainable chemical industry as discussed by Ciamician (1912), Ciamician (1908) and others more than 100 years ago. This event can be considered as the beginning of green or sustainable chemistry (Albini and Fagnoni, 2004; Albini and Fagnoni, 2008).
Figure 1. Cyanobacterial terpenoid pathway (green) and genetic modifications favoring the production of isoprene (blue). CBB, Calvin–Benson–Bassham; TCA, tricarboxylic acid; Pyr, pyruvate; G3P, glyceraldehyde-3-phosphate; MEP, methylerythritol-4-phosphate; IPP, isopentenyl-pyrophosphate; DMAPP, dimethylallyl-pyrophosphate; CfDXS, 1-deoxy-d-xylulose-5-phosphate synthase from Coleus forskohlii; sIDI, IPP/DMAPP isomerase from Synechocystis sp. PCC 6803; EgIspS, isoprene synthase from Eucalyptus globulus [Adapted form ref. Rana et al. (2022)].
Scheme 17. Photosensitized dimerization of isoprene 60 using triplet sensitization with the dinaphthylketon 68.
Platform chemicals play a central role in chemical industry. In the context of sustainable chemistry, new concepts of their production and transformation are essential. In this context, photochemical reactions play an important role. Existing or new platforms can be produced from biomass as renewable feedstock. As biomass possesses particular structure elements that are less common in fossil feedstock based platform compounds, this material offers numerous accesses to new innovative starting compounds for many domains of chemical industry. Thus carbohydrate based, oxygen rich biomass is transformed into furans. These heterocyclic aromatic compounds are used as starting compounds in many syntheses. The photooxygenation of furans yields interesting synthesis intermediates that are themselves suitable platform chemicals. Furthermore, photooxygenation of furans can easily be carried out on the industustrial scale or on large scale using sunlight as renewable energy source. Recently, an efficient process for the industrial production of levoglucosenone by pyrolysis from cellulose containing biomass has been developed. This compound is an intermediate for the mass production of the agro-solvent cyrene™. Photochemical or photocatalytic transformations of this compound open new perspectives for the valorization of this compound in the chemical or pharmaceutical industry. Carbohydrates such as hexoses or pentoses can also more directly be transformed, for instance, into heterocyclic targets. Photochemical reactions play a key role in sustainable chemistry and in organic synthesis. They enable the access to compounds that are not or difficultly available with more conventional methods of organic synthesis. Many original transformations can be carried out without chemical activation and the photon is considered as a traceless reagent. A more consequent application of these reaction conditions to the transformation of biomass derived platform chemicals efficiently contributes to a sustainable chemical industry as it was described by G. Ciamician more than hundred years ago. In this regard recently, fermentation processes based on the photosynthesis have been developed for the industrial production of alkenes such as isoprene. Using photochemical reactions of these compounds or several other terpenes for the further production of targets represents a very innovative concept for a sustainable industry as it has been shown for the production of jet fuels.
NH: Writing–original draft, Writing–review and editing. MG: Writing–review and editing. AD: Writing–review and editing. CL: Writing–review and editing. CM: Writing–review and editing. ML: Writing–review and editing.
The author(s) declare that no financial support was received for the research, authorship, and/or publication of this article.
The authors declare that the research was conducted in the absence of any commercial or financial relationships that could be construed as a potential conflict of interest.
All claims expressed in this article are solely those of the authors and do not necessarily represent those of their affiliated organizations, or those of the publisher, the editors and the reviewers. Any product that may be evaluated in this article, or claim that may be made by its manufacturer, is not guaranteed or endorsed by the publisher.
Abderrazak, Y., Bhattacharyya, A., and Reiser, O. (2021). Visible-light-induced homolysis of earth-abundant metal-substrate complexes: a complementary activation strategy in photoredox catalysis. Angew. Chem. Int. Ed. 60, 21100–21115. doi:10.1002/anie.202100270
Al Ghatta, A., and Hallett, J. P. (2023). Bioderived furanic compounds as replacements for BTX in chemical intermediate applications. RSC Sustain 1, 698–745. doi:10.1039/D3SU00038A
Albini, A., and Fagnoni, M. (2004). Green chemistry and photochemistry were born at the same time. Green Chem. 6, 1–6. doi:10.1039/B309592D
Albini, A., and Fagnoni, M. (2008). 1908 Giacomo Ciamician and the Concept of Green Chemistry. ChemSusChem 1, 63–66. doi:10.1002/cssc.200700015
Alshammari, A. A. A., Boyd, J. W., Greaves, N., Kettle, J. G., McKendrick, J. E., Parker, L. G., et al. (2024). Effect of tether length on endo/exo stereoselectivity in alkene-arene meta-photocycloaddition reactions towards the aphidocolin/stemodin scaffold. Eur. J. Org. Chem. 2024, e202400463. doi:10.1002/ejoc.202400463
Anastas, P. T., and Kirchhoff, M. M. (2002). Origins, current status, and future challenges of green chemistry. Acc. Chem. Res. 35, 686–694. doi:10.1021/ar010065m
J. C. André, A. B. Vannes, and R. Planche (1992). Techniques d’utilisation des photons (Avon: DOPEE Diffusion).
Araújo, J. D. P., Grande, C. A., and Rodrigues, A. E. (2010). Vanillin production from lignin oxidation in a batch reactor. Chem. Eng. Res. Des. 88, 1024–1032. doi:10.1016/j.cherd.2010.01.021
Argyropoulos, D. D. S., Crestini, C., Dahlstrand, C., Furusjö, E., Gioia, C., Jedvert, K., et al. (2023). Kraft lignin: a valuable, sustainable resource, opportunities and challenges. ChemSusChem 16, e202300492. doi:10.1002/cssc.202300492
Arias, P. L., Cecilia, J. A., Gandarias, I., Iglesias, J., López Granados, M., Mariscal, R., et al. (2020). Oxidation of lignocellulosic platform molecules to value-added chemicals using heterogeneous catalytic technologies. Catal. Sci. Technol. 10, 2721–2757. doi:10.1039/D0CY00240B
Awad, L., Demange, R., Zhu, Y. H., and Vogel, P. (2006). The use of levoglucosenone and isolevoglucosenone as templates for the construction of C-linked disaccharides. Carbohydr. Res. 341, 1235–1252. doi:10.1016/j.carres.2006.04.008
Bach, T., and Hehn, J. P. (2011). Photochemical reactions as key steps in natural product synthesis. Angew. Chem. Int. Ed. 50, 1000–1045. doi:10.1002/anie.201002845
Backa, S., Andresen, M., and Rojahn, T. (2012). “Biorefineries using wood for production of specialty cellulose fibers, lignosulfonates, vanillin, bioethanol and biogas – the Borregaard Sarpsborg example,” in Biomass as energy source: resources, systems and applications. Editors J. Bundschuh,, and E. Dahlquist (Boca Raton: CRC Press), 141–150. doi:10.1201/b14513
Badovskaja, L. A., Poskonin, V. V., Tyukhteneva, Z. I., and Kozhina, N. D. (2021). 2(5H)-Furanone and 5-hydroxy-2(5H)-furanone: reactions and syntheses based on them. Russ. J. Gen. Chem. 91, 133–153. doi:10.1134/S1070363221020018
Ban, Y. L., Dai, J. L., Jin, X. L., Zhang, Q. B., and Liu, Q. (2019). Thiocyanate radical mediated dehydration of aldoximes with visible light and air. Chem. Commun. 55, 9701–9704. doi:10.1039/c9cc05354a
Barrault, J., Kervennal, J., and Isnard, P. (2018). Numéro thématique: La chimie durable: pour l’environement, l’économie notre socciété. Actual. Chim. N° 427-428, 15–116.
Bartoschek, A., El-Idressy, T. T., Griesbeck, A. G., Höinck, L. O., Lex, J., Miara, C., et al. (2005). A family of new 1,2,4-trioxanes by photooxygenation of allylic alcohols in sensitizer-doped polymers and secondary reactions. Synthesis, 2433–2444. doi:10.1055/s-2005-872103
Behr, A., and Seidensticker, T. (2018). Einführung in die Chemie nachwachsender Rohstoffe. Berlin: Springer Spektrum. doi:10.1007/978-3-662-55255-1
Berkessel, A., Dousset, M., Bulat, S., and Glaubitz, K. (2005). Combinatorial approaches to functional models for galactose oxidase. Biol. Chem. 386, 1035–1041. doi:10.1515/BC.2005.119
Bertrand, S., Hoffmann, N., Humbel, S., and Pete, J. P. (2000b). Diastereoselective tandem addition-cyclization reactions of unsaturated tertiary amines initiated by photochemical electron transfer (PET). J. Org. Chem. 65, 8690–8703. doi:10.1021/jo001166l
Bertrand, S., Hoffmann, N., and Pete, J. P. (1998). Photochemical [2+2] cycloaddition of cyclicenones to(5R)-5-menthyloxy-2[5H]-furanone. Tetrahedron 54, 4873–4888. doi:10.1016/S0040-4020(98)00171-9
Bertrand, S., Hoffmann, N., and Pete, J. P. (2000a). Highly efficient and stereoselective radical addition of tertiary amines to electron-deficient alkenes - application to the enantioselective synthesis of necine bases. Eur. J. Org. Chem. 2000, 2227–2238. doi:10.1002/1099-0690(200006)2000:12<2227::AID-EJOC2227>3.0.CO;2-8
Bjørsvik, H. R., and Liguori, L. (2002). Organic processes to pharmaceutical chemicals based on fine chemicals from lignosulfonates. Org. Proc. Res. Dev. 6, 279–290. doi:10.1021/op010087o
Bohne, C. (1995). “Norrish type I processes of ketones: basic concepts in: horspool WM, song PS,” in CRC handbook of organic photochemistry and photobiology. Boca Raton: CRC Press, 416–422.
Bonfield, H. E., Knauber, T., Lévesque, F., Moschetta, E. G., Susanne, F., and Edwards, L. J. (2020). Photons as a 21st century reagent. Nat. Commun. 11, 804. doi:10.1038/s41467-019-13988-4
Bonneau, G., Peru, A. A. M., Flourat, A. L., and Allais, F. (2018). Organic solvent- and catalyst-free Baeyer–Villiger oxidation of levoglucosenone and dihydrolevoglucosenone (Cyrene®): a sustainable route to (S)-γ-hydroxymethyl-α,β-butenolide and (S)-γ-hydroxymethyl-γ-butyrolactone. Green Chem. 20, 2455–2458. doi:10.1039/C8GC00553B
Braun, A. M., Maurette, A. T., and Oliveros, E. (1991). Photochemical Technology. Chichester: Wiley.
Camp, J. E., and Greatrex, B. W. (2022). Levoglucosenone: bio-based platform for drug discovery. Front. Chem. 10, 902239. doi:10.3389/fchem.2022.902239
Chaudhuri, P., Hess, M., Hildenbrand, K., Bill, E., Weyhermüller, T., Wieghardt, K., et al. (1999). Aerobic oxidation of primary alcohols (including methanol) by copper(II)− and zinc(II)−Phenoxyl radical catalysts. J. Am. Chem. Soc. 121, 9599–9610. doi:10.1021/ja991481t
Chen, H., Wan, K., Zheng, F., Zhang, Z., Zhang, H., Zhang, Y., et al. (2021). Recent advances in photocatalytic transformation of carbohydrates into valuable platform chemicals. Front. Chem. Eng. 3, 615309. doi:10.3389/fceng.2021.615309
Ciamician, G. (1912). The photochemistry of the future. Science 36, 385–394. doi:10.1126/science.36.926.385
Cid Gomes, L., Rana, A., Berglund, M., Wiklund, P., and Ottosson, H. (2023). Light-driven (cross-)dimerization of terpenes as a route to renewable C15-C30 crudes for fuel and lubricant oil applications. Sustain. Energy Fuels 7, 868–882. doi:10.1039/d2se01370c
Citarella, A., Amenta, A., Passarella, D., and Micale, N. (2022). Cyrene: a green solvent for the synthesis of bioactive molecules and functional biomaterials. Int. J. Mol. Sci. 23, 15960. doi:10.3390/ijms232415960
Comba, M. B., Tsai, Y., Sarotti, A. M., Mangione, M. I., Suárez, A. G., and Spanevello, R. A. (2018). Levoglucosenone and its new applications: valorization of cellulose residues. Eur. J. Org. Chem. 2018, 590–604. doi:10.1002/ejoc.201701227
P. Compain, and O. R. Martin (2007). Iminosugars (Chichester: Wiley and Sons). doi:10.1002/9780470517437
Cornelisse, J. (1993). The meta photocycloaddition of arenes to alkenes. Chem. Rev. 93, 615–669. doi:10.1021/cr00018a002
Cornelisse, J., and de Haan, R. (2001). “Ortho photocycloaddition of alkenes and alkynes to the benzene ring,” in Understanding and manipulating the excited-state processes. Editors V. Ramamurthy,, and K. S. Schanze (New York: Marcel Dekker), 1–126. doi:10.1201/9781482294637
Cottier, L., Descotes, G., Nigay, H., Parron, J. C., and Grégoire, V. (1986). Photo-oxygénation des dérivés de l'hydroxyméthyl-5 furfural-2. Bull. Soc. Chim. Fr., 844–850.
Das, A., and König, B. (2018). Transition metal- and photoredox-catalyzed valorisation of lignin subunits. Green Chem. 20, 4844–4852. doi:10.1039/C8GC02073F
De bruyn, M., Fan, J., Budarin, V. L., Macquarrie, D. J., Gomez, L. D., Simister, R., et al. (2016). A new perspective in bio-refining: levoglucosenone and cleaner lignin from waste biorefinery hydrolysis lignin by selective conversion of residual saccharides. Energy Environ. Sci. 9, 2571–2574. doi:10.1039/C6EE01352J
De Keukeleire, D., and He, S. H. (1993). Photochemical strategies for the construction of polycyclic molecules. Chem. Rev. 93, 359–380. doi:10.1021/cr00017a017
Désiré, J., Mondon, M., Fontelle, N., Nakagawa, S., Hirokami, Y., Adachi, I., et al. (2014). N- and C-alkylation of seven-membered iminosugars generates potent glucocerebrosidase inhibitors and F508del-CFTR correctors. Org. Biomol. Chem. 12, 8977–8996. doi:10.1039/C4OB00325J
Desvals, A., Baudron, S. A., Bulach, V., and Hoffmann, N. (2021). Photocycloadditions of arenes derived from lignin. J. Org. Chem. 86, 13310–13321. doi:10.1021/acs.joc.1c01361
Desvals, A., Fortino, M., Lefebvre, C., Rogier, J., Michelin, C., Alioui, S., et al. (2022). Synthesis and characterization of polymethine dyes carrying thiobarbituric and carboxylic acid moieties. New J. Chem. 46, 8971–8980. doi:10.1039/D2NJ00684G
Desvals, A., and Hoffmann, N. (2023). Photocycloadditions of benzene derivatives and their systematic application to organic synthesis. Aust. J. Chem. 76, 117–129. doi:10.1071/CH23029
de Vries, J. G. (2017). Green synthesis of heterocycles of industrial importance. 5-Hydroxymethylfurfural as a platform chemical. Adv. Heterocycl. Chem. 121, 1–47. doi:10.1016/bs.aihch.2016.09.001
Dijksman, A., Arends, IWCE, and Sheldon, R. A. (2003). Cu(ii)-nitroxyl radicals as catalytic galactose oxidase mimics. Org. Biomol. Chem. 1, 3232–3237. doi:10.1039/B305941C
Diot-Néant, F., Rastoder, E., Miller, S. A., and Allais, F. (2018). Chemo-enzymatic synthesis and free radical polymerization of renewable acrylate monomers from cellulose-based lactones. ACS Sustain. Chem. Eng. 6, 17284–17293. doi:10.1021/acssuschemeng.8b04707
Dömling, A., Wang, W., and Wang, K. (2012). Chemistry and biology of multicomponent reactions. Chem. Rev. 112, 3083–3135. doi:10.1021/cr100233r
Elliott, L. D., Knowles, J. P., Koovits, P. J., Maskill, K. G., Ralph, M. J., Lejeune, G., et al. (2014). Batch versus flow photochemistry: a revealing comparison of yield and productivity. Chem. Eur. J. 20, 15226–15232. doi:10.1002/chem.201404347
Esser, P., Pohlmann, B., and Scharf, H. D. (1994). The photochemical synthesis of fine chemicals with sunlight. Angew. Chem. Int. Ed. 33, 2009–2023. doi:10.1002/anie.199420091
Fache, M., Boutevin, B., and Caillol, S. (2016). Vanillin production from lignin and its use as a renewable chemical. ACS Sustainable Chem. Eng. 4, 35–46. doi:10.1021/acssuschemeng.5b01344
Farmer, T. J., and Mascal, M. (2015). “Platfom molecules,” in Introduction to chemicals from biomass. Editors J. Clark,, and F. Deswarte 2nd Edition (Chichester: Wiley), 89–152. doi:10.1002/9781118714478.ch4
Feringa, B. L., and de Jong, J. C. (1992). New strategies in asymmetric synthesis based on γ-alkoxybutenolides. Bull. Soc. Chim. Belg. 101, 627–640. doi:10.1002/bscb.19921010710
Flourat, A. L., Haudrechy, A., Allais, F., and Renauld, J. H. (2020). (S)-γ-Hydroxymethyl-α,β-butenolide, a valuable chiral synthon: syntheses, reactivity, and applications. Org. Process Res. Dev. 24, 615–636. doi:10.1021/acs.oprd.9b00468
Fréneau, M., de Sainte-Claire, P., Abe, M., and Hoffmann, N. (2016). The structure of electronically excited α,β-unsaturated lactones. J. Phys. Org. Chem. 29, 718–724. doi:10.1002/poc.3560
Gallezot P., (2012). Conversion of biomass to selected chemical products. Chem. Soc. Rev. 41, 1538–1558. doi:10.1039/C1CS15147A
Gassama, A., Ernenwein, C., and Hoffmann, N. (2009). Photochemical key steps in the synthesis of surfactants from furfural-derived intermediates. ChemSusChem 2 (2), 1130–1137. doi:10.1002/cssc.200900150
Gassama, A., Ernenwein, C., Youssef, A., Agach, M., Riguet, E., Marinković, S., et al. (2013). Sulfonated surfactants obtained from furfural. Green Chem. 15, 1558–1566. doi:10.1039/c3gc00062a
Gassama, A., and Hoffmann, N. (2008). Selective light supported oxidation of hexoses using air as oxidant – synthesis of tetrahydroxyazepanes. Adv. Synth. Catal. 350, 35–39. doi:10.1002/adsc.200700282
Ghogare, A. A., and Greer, A. (2016). Using singlet oxygen to synthesize natural products and drugs. Chem. Rev. 116, 9994–10034. doi:10.1021/acs.chemrev.5b00726
Gilbert, A., and Bach, T. (2023). The awakening of a sleeping beauty: the ortho photocycloaddition in the total synthesis of protoilludane- and prezizaene-type Sesquiterpenes. Synlett 34, 1343–1355. doi:10.1055/s-0042-1751354
Gollnick, K., and Griesbeck, A. (1985). Singlet oxygen photooxygenation of furans: isolation and reaction of (4+2)-cycloaddition products (unsaturated sec.-ozonides). Tetrahedron 41, 2057–2068. doi:10.1016/S0040-4020(01)96576-7
Gómez Fernández, M. A., and Hoffmann, N. (2023). Photocatalytic transformation of biomass and biomass derived compounds – application to organic synthesis. Molecules 28, 4746. doi:10.3390/molecules28124746
Gorbachev, D., Smith, E., Argent, S. P., Newton, G. N., and Lam, H. W. (2022). Synthesis of new morphinan opioids by TBADT-catalyzed photochemical functionalization at the carbon skeleton**. Chem. Eur. J. 28, e202201478. doi:10.1002/chem.202201478
Groß, J., Kühlborn, J., and Opatz, T. (2020). Applications of xylochemistry from laboratory to industrial scale. Green Chem. 22, 4411–4425. doi:10.1039/D0GC01484B
Guigo, N., Jérome, F., and Sousa, A. F. (2021). Biobased furanic derivatives for sustainable development. Green Chem. 23, 9721–9722. doi:10.1039/d1gc90124a
Hagen, W., and Lorenz, S. (1957). Ein neues bakterielles Umwandlungsprodukt der Saccharose. Angew. Chem. 69, 641. doi:10.1002/ange.19570692011
Halpern, Y., Ritter, R., and Broido, A. (1973). Levoglucosenone (1,6-anhydro-3,4-dideoxy-.DELTA.3-.beta.-D-pyranosen-2-one). Major product of the acid-catalyzed pyrolysis of cellulose and related carbohydrates. J. Org. Chem. 38, 204–209. doi:10.1021/jo00942a005
Hammond, G. S., Turro, N. J., and Liu, R. S. H. (1963). Mechanisms of photochemical reactions in solution. XVI.1 photosensitized dimerization of conjugated dienes. J. Org. Chem. 28, 3297–3303. doi:10.1021/jo01047a005
Harakat, D., Pesch, J., Marinković, S., and Hoffmann, N. (2006). Thiocarbonyl compounds as regulating reagent in the radical addition of tertiary amines with alkenes using photoelectron transfer conditions. Org. Biomol. Chem. 4, 1202–1205. doi:10.1039/B600220J
He, J., Liu, M., Huang, K., Walker, T. W., Maravelias, C. T., Dumesic, J. A., et al. (2017). Production of levoglucosenone and 5-hydroxymethylfurfural from cellulose in polar aprotic solvent–water mixtures. Green Chem. 19, 3642–3653. doi:10.1039/C7GC01688C
C. Heitner, D. R. Dimmel, and J. A. Schmidt (2010). Lignin and lignans (Boca Raton: CRC Press). doi:10.1201/EBK1574444865
Hoffmann, N. (1994). Highly diastereoselective addition of photochemically generated radicals to (5R)-(−)-menthyloxy-2[5H]-furanone - synthesis of (−)-terebic acid. Tetrahedron Asymm 5, 879–886. doi:10.1016/S0957-4166(00)86239-4
Hoffmann, N. (2002). New photochemical rearrangements and extrusion reactions of aromatic compounds induced by an intramolecular [2+2] photocycloaddition between a naphthalene and a resorcinol moiety. Tetrahedron 58, 7933–7941. doi:10.1016/S0040-4020(02)00902-X
Hoffmann, N. (2004). Photochemical cycloaddition between benzene derivatives and alkenes. Synthesis, 481–495. doi:10.1055/s-2004-815973
Hoffmann, N. (2005). “Ortho-, meta-, and para-photocycloadditions of arenes,” in Synthetic organic photochemistry. Editors A. G. Griesbeck,, and J. Mattay (New York: Marcel Dekker), 529–552. doi:10.1201/9780203997369
Hoffmann, N. (2008). Photochemical reactions as key steps in organic synthesis. Chem. Rev. 108, 1052–1103. doi:10.1021/cr0680336
Hoffmann, N. (2012). Photochemical reactions of aromatic compounds and the concept of the photon as a traceless reagent. Photochem. Photobiol. Sci. 11, 1613–1641. doi:10.1039/C2PP25074H
Hoffmann, N. (2015). Electron and hydrogen transfer in organic photochemical reactions. J. Phys. Org. Chem. 28, 121–136. doi:10.1002/poc.3370
Hoffmann, N. (2016). Photochemical electron and hydrogen transfer in organic synthesis: the control of selectivity. Synthesis 48, 1782–1802. doi:10.1055/s-0035-1561425
Hoffmann, N. (2017). Proton-coupled electron transfer in photoredox catalytic reactions. Eur. J. Org. Chem. 2017, 1982–1992. doi:10.1002/ejoc.201601445
Hoffmann, N., Bertrand, S., Marinković, S., and Pesch, J. (2006). Efficient radical addition of tertiary amines to alkenes using photochemical electron transfer. Pure Appl. Chem. 78, 2227–2246. doi:10.1351/pac200678122227
Hoffmann, N., Buschmann, H., Raabe, G., and Scharf, H. D. (1994). Chiral induction in photochemical reactions – 15.: detection of stereoelectronic effects by temperature dependent measurements of the diastereoselectivity in the photosensitized [2+2]-cycloaddition. Tetrahedron 50, 11167–11186. doi:10.1016/S0040-4020(01)89419-9
Hoffmann, N., and Pete, J. P. (1995). Intramolecular photochemical reactivity of O-Alk-3-enylsalicylic esters. Tetrahedron Lett. 36, 2623–2626. doi:10.1016/0040-4039(95)00352-D
Hoffmann, N., and Pete, J. P. (1997). Intramolecular photochemical reactions of bichromophoric 3-(alkenyloxy)phenols and 1-(Alkenyloxy)-3-(alkyloxy)benzene derivatives. Acid-catalyzed transformations of the primary cycloadducts. J. Org. Chem. 62, 6952–6960. doi:10.1021/jo970554t
Hoffmann, N., and Pete, J. P. (2001). Intramolecular [2+2] photocycloaddition of bichromophoric derivatives of 3,5-dihydroxybenzoic acid and 3,5-dihydroxybenzonitrile. Synthesis 112, 1236–1242. doi:10.1055/s-2001-15076
Hoffmann, N., Pete, J. P., Inoue, Y., and Mori, T. (2002). Novel [2+2] photocycloaddition-induced rearrangement of bichromophoric naphthalene-tethered resorcinol ethers. J. Org. Chem. 67, 2315–2322. doi:10.1021/jo011143m
Hoffmann, N., and Riguet, E. (2016). “Aromatic photochemical reactions,” in Arene chemistry. Editor J. Mortier (Hoboken: Wiley and Sons), 837–868. doi:10.1002/9781118754887.ch29
Hoffmann, N., and Scharf, H. D. (1991). Efficient and diastereoselective synthesis of (+)- and (-)-Grandisol and 2-([1R, 2S]-2-Isopropenylcyclobutyl)ethanol (demethylgrandisol) in high purity. Liebigs Ann. Chem. 1991, 1273–1277. doi:10.1002/jlac.1991199101219
Hong, B. C., and Indurmuddam, R. R. (2024). Tetrabutylammonium decatungstate (TBADT), a compelling and trailblazing catalyst for visible-light-induced organic photocatalysis. Org. Biomol. Chem. 22, 3799–3842. doi:10.1039/d4ob00171k
Hou, Q., Qi, X., Zhen, M., Qian, H., Nie, Y., Bai, C., et al. (2021). Biorefinery roadmap based on catalytic production and upgrading 5-hydroxymethylfurfural. Green Chem. 23, 119–231. doi:10.1039/d0gc02770g
Hudlicky, T. (1996). Design constraints in practical syntheses of complex molecules: current status, case studies with carbohydrates and alkaloids, and future perspectives. Chem. Rev. 96, 3–30. doi:10.1021/cr950012g
Ito, N., Phillips, S. E. V., Stevens, C., Ogel, Z. B., McPherson, M. J., Keen, J. N., et al. (1991). Novel thioether bond revealed by a 1.7 Å crystal structure of galactose oxidase. Nature 350, 87–90. doi:10.1038/350087a0
Jäger, M., and Minnaard, A. J. (2016). Regioselective modification of unprotected glycosides. Chem. Commun. 52, 656–664. doi:10.1039/C5CC08199H
Jahjah, R., Gassama, A., Bulach, V., Suzuki, C., Abe, M., Hoffmann, N., et al. (2010). Stereoselective triplet-sensitised radical reactions of furanone derivatives. Chem. Eur. J. 16, 3341–3354. doi:10.1002/chem.200903045
Jaswal, A., Singh, P. P., and Mondal, T. (2022). Furfural – a versatile, biomass-derived platform chemical for the production of renewable chemicals. Green Chem. 24, 510–551. doi:10.1039/d1gc03278j
Joy, F., Peter, F., Gokul, P. C., Nizam, A., and Chinnam, S. (2022). UV-promoted metal- and photocatalyst-free direct conversion of aromatic aldehydes to nitriles. Russ. J. Org. Chem. 58, 379–384. doi:10.1134/S1070428022030174
Kabbour, M., and Luque, R. (2020). “Furfural as a platform chemical: from production to applications,” in Recent advances in development of platform chemicals - biomass, biofuels, biochemicals. Editor A. Pandey (Amsterdam: Elsevier), 283–297. doi:10.1016/B978-0-444-64307-0.00010-X
Kärkäs, M. D., Matsuura, B. S., Monos, T. M., Magallanes, G., and Stephenson, C. R. J. (2016b). Transition-metal catalyzed valorization of lignin: the key to a sustainable carbon-neutral future. Org. Biomol. Chem. 14, 1853–1914. doi:10.1039/C5OB02212F
Kärkäs, M. D., Porco, J. A., and Stephenson, C. R. J. (2016a). Photochemical approaches to complex chemotypes: applications in natural product synthesis. Chem. Rev. 116, 9683–9747. doi:10.1021/acs.chemrev.5b00760
Klepp, J., Dillon, W., Lin, Y., Feng, P., Greatrex, B. W., Imamura, Y., et al. (2020). Preparation of (-)-Levoglucosenone from cellulose using sulfuric acid in polyethylene glycol. Org. Synth. 97, 38–53. doi:10.15227/orgsyn.097.0038
B. König (2020). Chemical photocatalysis. 2nd Edition (Berlin: De Gruyter). doi:10.1515/9783110576764
Latrache, M., and Hoffmann, N. (2021). Photochemical radical cyclization reactions with imines, hydrazones, oximes and related compounds. Chem. Soc. Rev. 50, 7418–7435. doi:10.1039/d1cs00196e
Lebedeva, D., Schick, L. W., Cracco, D., Sangsuwan, W., Castiella-Ona, G., Siva, D. O., et al. (2024). Sustainable aviation fuel from prehydrolysis liquors. Green Chem. 26, 7258–7267. doi:10.1039/D4GC01257G
Le Dot, M., Gomez Fernandez, M. A., Langovist, A., Charrière, B., Gérard, P., Dumur, F., et al. (2024). New monomers or co-monomers based on the alkoxyfuranone Scaffold: toward new alternatives to petroleum-based structures. Eur. Polym. J. 241, 113259. doi:10.1016/j.eurpolymj.2024.113259
Lee, S. Y., Kim, H. U., Chae, T. U., Cho, J. S., Kim, J. W., Shin, J. H., et al. (2019). A comprehensive metabolic map for production of bio-based chemicals. Nat. Catal. 2, 18–33. doi:10.1038/s41929-018-0212-4
Lefebvre, C., Van Gysel, T., Michelin, C., Rousset, E., Djiré, D., Allais, F., et al. (2022). Photocatalytic radical addition to levoglucosenone. Eur. J. Org. Chem. 2022, e202101298. doi:10.1002/ejoc.202101298
Lepage, M. L., Alachouzos, G., Hermens, G. H., Elders, N., van den Berg, K. J., and Feringa, B. L. (2023). Electron-poor butenolides: the missing link between acrylates and maleic anhydride in radical polymerization. J. Am. Chem. Soc. 145, 17211–17219. doi:10.1021/jacs.3c04314
Levy, D. E., and Fügedi, P. (2006). The organic chemistry of sugars. Boca Raton: CRC Taylor and Francis. doi:10.1201/9781420027952
Lewis, E. A., and Tolman, W. B. (2004). Reactivity of dioxygen-copper systems. Chem. Rev. 104, 1047–1076. doi:10.1021/cr020633r
Lewkowski, J. (2001). Synthesis, chemistry and applications of 5-hydroxymethyl-furfural and its derivatives. Arkivoc 2001, 17–54. doi:10.3998/ark.5550190.0002.102
Li, C., Jiang, D., Cheng, X., Li, H., He, S., Mu, M., et al. (2024). Elucidating reaction mechanisms in the oxidative depolymerization of sodium lignosulfonate for enhancing vanillin production: a Density functional Theory study. J. Anal. Appl. Pyrolysis. 179, 106499. doi:10.1016/j.jaap.2024.106499
Li, H., Marcelo, F., Bello, C., Vogel, P., Butters, T. D., Rauter, A. P., et al. (2009). Design and synthesis of acetamido tri- and tetra-hydroxyazepanes: potent and selective β-N-acetylhexosaminidase inhibitors. Bioorg. Med. Chem. 17, 5598–5604. doi:10.1016/j.bmc.2009.06.022
Li, S. H., Liu, S., Colmenares, J. C., and Xu, Y. J. (2016). A sustainable approach for lignin valorization by heterogeneous photocatalysis. Green Chem. 18, 594–607. doi:10.1039/C5GC02109J
Li, X. (2023). Current approaches of the functional and synergetic catalytic systems for converting renewable carbohydrates into 2, 5-diformylfuran. Mol. Catal. 551, 113629. doi:10.1016/j.mcat.2023.113629
Lichtenthaler, F. W. “Carbohydrate-based product lines: the key sugars of biomass: availability, present non-food users and potential future development lines,” in Kamm, B., Gruber, P. R., and Kamm, M. editors. Biorefineries – industrial processes and products Vol. 1. Weinheim: Wiley VCH. (2006). 2–59. doi:10.1002/9783527619849
Lichtenthaler, F. W., Martin, D., Weber, T., and Schiweck, H. (1993). Studies on ketoses, 7 – 5-(α-D-Glucosyloxymethyl)furfural: preparation from isomaltulose and exploration of its ensuing chemistry. Liebigs Ann. Chem. 1993, 967–974. doi:10.1002/jlac.1993199301154
Lichtenthaler, F. W., and Peters, S. (2004). Carbohydrates as green raw materials for the chemical industry. CR Chim. 7, 65–90. doi:10.1016/j.crci.2004.02.002
Liu, R. S. H., Turro, Jr N. J., and Hammond, G. S. (1965). Mechanisms of photochemical reactions in solution. XXXI. Activation and deactivation of conjugated dienes by energy transfer. J. Am. Chem. Soc. 87, 3406–3412. doi:10.1021/ja01093a021
Liu, W., and Li, C. J. (2017). Recent synthetic applications of catalyst-free photochemistry. Synlett 28, 2714–2754. doi:10.1055/s-0036-1590900
Loubière, K., Oelgemöller, M., Aillet, T., Dechy-Cabaret, O., and Prat, L. (2016). Continuous-flow photochemistry: a need for chemical engineering. Chem. Eng. Process. 104, 120–132. doi:10.1016/j.cep.2016.02.008
Magallanes, G., Kärkäs, M. D., Bosque, I., Lee, S., Maldonado, S., and Sephenson, C. R. J. (2019). Selective C–O bond cleavage of lignin systems and polymers enabled by sequential palladium-catalyzed aerobic oxidation and visible-light photoredox catalysis. ACS Catal. 9, 2252–2260. doi:10.1021/acscatal.8b04172
Majhi, S. (2021). Applications of Norrish type I and II reactions in the total synthesis of natural products: a review. Photochem. Photobiol. Sci. 20, 1357–1378. doi:10.1007/s43630-021-00100-3
Marinković, S., Brulé, C., Hoffmann, N., Prost, E., Nuzillard, J. M., and Bulach, V. (2004). Origin of chiral induction in radical reactions with the diastereoisomers (5R)- and (5S)-5-l-Menthyloxyfuran-2[5H]-one. J. Org. Chem. 69, 1646–1651. doi:10.1021/jo030292x
Marinković, S., and Hoffmann, N. (2001). Efficient radical addition of tertiary amines to electron-deficient alkenes using semiconductors as photochemical sensitisers. Chem. Commun., 1576–1577. doi:10.1039/B104387K
Marinković, S., and Hoffmann, N. (2003). Semiconductors as sensitisers for the radical addition of tertiary amines to electron deficient alkenes. Int. J. Photoenergy. 5, 175–182. doi:10.1155/S1110662X03000308
Marinković, S., and Hoffmann, N. (2004). Diastereoselective radical tandem addition-cyclization reactions of aromatic tertiary amines by semiconductor-sensitized photochemical electron transfer. Eur. J. Org. Chem. 2004, 3102–3107. doi:10.1002/ejoc.200400102
Marion, P., Bernela, B., Piccirilli, A., Estrine, B., Patoullard, N., Guilbot, J., et al. (2017). Sustainable chemistry: how to produce better and more from less? Green Chem. 1, 4973–4989. doi:10.1039/C7GC02006F
Martel, F., Estrine, B., Plantier-Royon, R., Hoffmann, N., and Portella, C. (2010). Development of agriculture left-overs: fine organic chemicals from wheat hemicellulose-derived pentoses. Top. Curr. Chem. 294, 79–115. doi:10.1007/128_2010_54
Marzo, L., Pagire, S. K., Reiser, O., and König, B. (2018). Visible-light photocatalysis: does it make a difference in organic synthesis? Angew. Chem. Int. Ed. 57, 10034–10072. doi:10.1002/anie.201709766
Mascal, M., and Dutta, S. (2011). Synthesis of the natural herbicide d-aminolevulinic acid from cellulose-derived 5-(chloromethyl) furfural. Green Chem. 13, 40–41. doi:10.1039/C0GC00548G
McCullough, J. J. (1987). Photoadditions of aromatic compounds. Chem. Rev. 87, 811–860. doi:10.1021/cr00080a008
Michelin, C., and Hoffmann, N. (2018a). Photosensitization and photocatalysis – perspectives in organic synthesis. ACS Catal. 8, 12046–12055. doi:10.1021/acscatal.8b03050
Michelin, C., and Hoffmann, N. (2018b). Photocatalysis applied to organic synthesis – a green chemistry approach. Curr. Opin. Green Sustain. Chem. 10, 40–45. doi:10.1016/j.cogsc.2018.02.009
Mika, L. T., Cséfalvay, E., and Németh, A. (2018). Catalytic conversion of carbohydrates to initial platform chemicals: chemistry and sustainability. Chem. Rev. 118, 505–613. doi:10.1021/acs.chemrev.7b00395
Miller, D. C., Tarantino, K. T., and Knowles, R. R. (2016). Proton-coupled electron transfer in organic synthesis: fundamentals, applications, and opportunities. Top. Curr. Chem. 374, 30. doi:10.1007/s41061-016-0030-6
Minaev, B. F. (2007). Electronic mechanisms of activation of molecular oxygen. Russ. Chem. Rev. 76, 1059–1083. doi:10.1070/rc2007v076n11abeh003720
Mirica, L. M., Ottenwaelder, X., and Stack, D. P. (2004). Structure and spectroscopy of copper-dioxygen complexes. Chem. Rev. 104, 1013–1046. doi:10.1021/cr020632z
Mittal, A., Pilath, H. M., and Johnson, D. K. (2020). Direct conversion of biomass carbohydrates to platform chemicals: 5-hydroxymethylfurfural (HMF) and furfural. Energy fuels. 34, 3284–3293. doi:10.1021/acs.energyfuels.9b04047
Montagnon, T., Kalaitzakis, D., Sofiadis, M., and Vassilikogiannakis, G. (2016). Chemoselective photooxygenations of furans bearing unprotected amines: their use in alkaloid synthesis. Org. Biomol. Chem. 14, 8636–8640. doi:10.1039/c6ob01689h
Montagnon, T., Kalaitzakis, D., Triantafyllakis, M., Stratakis, M., and Vassilikogiannakis, G. (2014). Furans and singlet oxygen – why there is more to come from this powerful partnership. Chem. Commun. 50, 15480–15498. doi:10.1039/c4cc02083a
Moradei, O. M., and Paquette, L. A. (2003). (5S)-(d-Menthyloxy)-2(5H)-furanone. Org. Synth. 80, 66–73. doi:10.15227/orgsyn.080.0066
Moschetta, E. G., Cook, G. C., Edwards, L. J., Ischay, M. A., Lei, Z., Buono, F., et al. (2024). Photochemistry in pharmaceutical development: a survey of strategies and approaches to industry-wide implementation. Org. Process Res. Dev. 28, 831–846. doi:10.1021/acs.oprd.3c00499
Müller, F., and Mattay, J. (1993). Photocycloadditions: control by energy and electron transfer. Chem. Rev. 93, 99–117. doi:10.1021/cr00017a006
Murray, P. R. D., Cox, J. H., Chiappini, N. D., Roos, C. B., McLoughlin, E. A., Hejna, B. G., et al. (2022). Photochemical and electrochemical applications of proton-coupled electron transfer in organic synthesis. Chem. Rev. 122, 2017–2291. doi:10.1021/acs.chemrev.1c00374
Nakagawa, Y., Yabushita, M., and Tomishige, K. (2023). A perspective on catalytic production of olefinic compounds from biomass. RSC Sustain 1, 814–837. doi:10.1039/D3SU00033H
Nakashima, K., Ando, T., Nakamura, K., and Akiyama, S. (1983). A convenient method for a sensitive colorimetric determination of lipoperoxides with 1,3-diphenyl-2-thiobarbituric acid. Chem. Pharm. Bull. 31, 2523–2525. doi:10.1248/cpb.31.2523
Nardello-Rataj, V., Alsters, P. L., and Aubry, J. M. (2016). “Industrial prospects for the chemical and photochemical singlet oxygenation of organic compounds,” in Liquid phase aerobic oxidation catalysis. Editors S. S. Stahl,, and P. L. Alsters (Weinheim: Wiley VCH), 369–395. doi:10.1002/9783527690121.ch22
Nayak, J., Basu, A., Dey, P., Kumar, R., Upadhaya, A., Ghosh, S., et al. (2023). Transformation of agro-biomass into vanillin through novel membrane integrated value-addition process: a state-of-art review. Biomass Conv. bioref. 13, 14317–14340. doi:10.1007/s13399-022-03283-6
Nazeri, M. T., Fahid, H., Mohammadian, R., and Shaabani, A. (2020). Cyclic imines in ugi and ugi-type reactions. ACS Comb. Sci. 22, 361–400. doi:10.1021/acscombsci.0c00046
Nedjma, M., Hoffmann, N., and Belarbi, A. (2001). Selective and sensitive detection of pectin lyase activity using a colorimetric test: application to the screening of microorganisms possessing pectin lyase activity. Anal. Biochem. 291, 290–296. doi:10.1006/abio.2001.5032
Nicholls, T. P., Leonori, D., and Bissember, A. C. (2016). Applications of visible light photoredox catalysis to the synthesis of natural products and related compounds. Nat. Prod. Rep. 33, 1248–1254. doi:10.1039/C6NP00070C
Nielsen, E. K., El-Chami, K., Mondrup de Lichtenberg, C., and Madsen, R. (2024). Decatungstate-catalyzed carbon-carbon bond formation between furfural and electron-deficient olefins. Eur. J. Org. Chem. 27, e202400109. doi:10.1002/ejoc.202400109
Niguyen, S. T., Murray, P. R. D., and Knowles, R. (2020). Light-Driven depolymerization of native lignin enabled by proton-coupled electron transfer. ACS Catal. 10, 800–805. doi:10.1021/acscatal.9b04813
Noël, T. (2017). A personal perspective on the future of flow photochemistry. J. Flow. Chem. 217 (7), 87–93. doi:10.1556/1846.2017.00022
Nwosu, U., Wang, A., Palma, B., Zhao, H., Khan, M. A., Kibria, M., et al. (2021). Selective biomass photoreforming for valuable chemicals and fuels: a critical review. Renew. Sustain. Energy Rev. 148, 111266. doi:10.1016/j.rser.2021.111266
Oelgemöller, M. (2014). Green photochemical processes and technologies for research and development, scale-up and chemical production. J. Chin. Chem. Soc. 61, 743–748. doi:10.1002/jccs.201400064
Oelgemöller, M. (2016). Solar photochemical synthesis: from the beginnings of organic photochemistry to the solar manufacturing of commodity chemicals. Chem. Rev. 116, 9664–9682. doi:10.1021/acs.chemrev.5b00720
Oelgemöller, M., Hoffmann, N., and Shvydkiv, O. (2014). From 'lab and light on a chip' to parallel microflow photochemistry. Aust. J. Chem. 67, 337–342. doi:10.1071/CH13591
Oelgemöller, M., Jung, C., and Mattay, J. (2007). Green photochemistry: production of fine chemicals with sunlight. Pure Appl. Chem. 79, 1939–1947. doi:10.1351/pac200779111939
Okada, M., Fukuyama, T., Yamada, K., Ryu, I., Ravelli, D., and Fagnoni, M. (2014). Sunlight photocatalyzed regioselective β-alkylation and acylation of cyclopentanones. Chem. Sci. 5, 2893–2898. doi:10.1039/C4SC01072H
Ouyang, D., Wang, F., Gao, D., Han, W., Hu, X., Qiao, D., et al. (2022). Light-driven lignocellulosic biomass conversion for production of energy and chemicals. iScience 25, 105221. doi:10.1016/j.isci.2022.105221
Palai, Y. N., Fukuoka, A., and Shrotri, A. (2024). Unlocking the potential of 5-hydroxy-2(5H)-furanone as a platform for bio-based four carbon chemicals. ACS Catal. 14, 2545–2551. doi:10.1021/acscatal.3c04872
Peyrot, C., Mention, M. M., Brunissen, F., Balaguer, P., and Allais, F. (2020). Innovative bio-based organic UV-A and blue light filters from Meldrum’s acid. Molecules 25, 2178. doi:10.3390/molecules25092178
Pierre, J. L. (2000). One electron at a time oxidations and enzymatic paradigms: from metallic to non-metallic redox centers. Chem. Soc. Rev. 29, 251–257. doi:10.1039/A909719H
Pillitteri, P., Ranjan, P., Voskressensky, L. G., Van der Eycken, E. V., and Sharma, U. K. (2021). Alkylation of in situ generated imines via photoactivation of strong aliphatic C-H bonds. Mol. Catal. 514, 111841. doi:10.1016/j.mcat.2021.111841
Poskonin, V. V., Yakovlev, D. N., Kovardakov, V. A., and Badovskaja, L. L. (1999). Studies on substituted butane- and butenolides: XIV. Synthesis of high-molecular butanolides on the basis of 4-Alkoxy-2-butenolides and vinyl monomers. Russ. Org. Chem. 35, 721–726.
Post, C., Maniar, D., Voet, V. S. D., Folkersma, R., and Loos, K. (2023). Biobased 2,5-Bis(hydroxymethyl)furan as a versatile building block for sustainable polymeric materials. ACS Omega 8, 8991–9003. doi:10.1021/acsomega.2c07629
Proessdorf, J., Jandl, C., Pickl, T., and Bach, T. (2022). Arene activation through iminium ions: product diversity from intramolecular photocycloaddition reactions. Angew. Chem. Int. Ed. 61, e202208329. doi:10.1002/anie.202208329
Qiao, L., Fu, X., Si, Y., Chen, X., Qu, L., and Yu, B. (2022). Switchable aroylation and diaroylation of allyl sulfones with aldehydes enabled by decatungstate photocatalysis. Green Chem. 24, 5614–5619. doi:10.1039/d2gc01381a
Rana, a, Cid Gomes, L., Rodrigues, J. S., Yacout, D. M. M., Arrou-Vignod, H., Sjölander, J., et al. (2022). A combined photobiological-photochemical route to C10 cycloalkane jet fuels from carbon dioxide via isoprene. Green Chem. 24, 9602–9619. doi:10.1039/D2GC03272D
Rao, V. N., Malu, T. J., Krishnan, C. K., Sakar, M., Pitchaimuthu, S., Rodríguez-González, V., et al. (2021). Light-driven transformation of biomass into chemicals using photocatalysts - vistas and challenges. J. Environ. Manage. 284, 111983. doi:10.1016/j.jenvman.2021.111983
Ravelli, D., Fagnoni, M., Fukuyama, T., Nishikawa, T., and Ryu, I. (2018). Site-selective C–H functionalization by decatungstate anion photocatalysis: synergistic control by polar and steric effects expands the reaction scope. ACS Catal. 8, 701–713. doi:10.1021/acscatal.7b03354
Ravelli, D., Protti, S., and Fagnoni, M. (2016). Decatungstate anion for photocatalyzed “window ledge” reactions. Acc. Chem. Res. 49, 2232–2242. doi:10.1021/acs.accounts.6b00339
Ravelli, D., and Samorì, C. (2021). Biomass valorisation. Weinheim: Wiley VCH. doi:10.1002/9783527825028
Raviola, C., Protti, S., Ravelli, D., and Fagnoni, M. (2019). Photogenerated acyl/alkoxycarbonyl/carbamoyl radicals for sustainable synthesis. Green Chem. 21, 748–764. doi:10.1039/C8GC03810D
Remy, R., and Bochet, C. G. (2016). Arene-alkene cycloaddition. Chem. Rev. 116, 9816–9849. doi:10.1021/acs.chemrev.6b00005
Riguet, E. (2011). Enantioselective organocatalytic friedel–crafts alkylation reaction of indoles with 5-hydroxyfuran-2(5H)-one: access to chiral γ-lactones and γ-lactams via a ugi 4-center 3-component reaction. J. Org. Chem. 76, 8143–8150. doi:10.1021/jo201184p
Rinaldi, R., Jastrzebski, R., Clough, M. T., Ralph, J., Kennema, M., Bruijnincx, P. C. A., et al. (2016). Paving the way for lignin valorisation: recent advances in bioengineering, biorefining and catalysis. Angew. Chem. Int. Ed. 55, 8164–8215. doi:10.1002/anie.201510351
Roberts, B. P. (1999). Polarity-reversal catalysis of hydrogen-atom abstraction reactions: concepts and applications in organic chemistry. Chem. Soc. Rev. 28, 25–35. doi:10.1039/A804291H
Rojahn, W., and Warnecke, H. U. (1980). Die photosensibilisierte Sauerstoffübertragung – eine Methode zur Herstellung hochwertiger Riechstoffe. Dragoco Report 27, 159–164.
Rosenberg, M., Dahlstrand, C., Kilså, K., and Ottosson, H. (2014). Excited state aromaticity and antiaromaticity: opportunities for photophysical and photochemical rationalizations. Chem. Rev. 114, 5379–5425. doi:10.1021/cr300471v
Ryland, B. L., and Stahl, S. S. (2014). Practical aerobic oxidations of alcohols and amines with homogeneous copper/TEMPO and related catalyst systems. Angew. Chem. Int. Ed. 53, 8824–8838. doi:10.1002/anie.201403110
Saaret, A., Villiers, B., Stricher, F., Anissimova, M., Cadillon, M., Spiess, R., et al. (2021). Directed evolution of prenylated FMN-dependent Fdc supports efficient in vivo isobutene production. Nat. Commun. 12, 5300. doi:10.1038/s41467-021-25598-0
Sarotti, A. M., Zanardi, M. M., Spanevello, R. A., and Suárez, A. G. (2012). Recent applications of levoglucosenone as chiral synthon. Curr. Org. Synth. 9, 439–459. doi:10.2174/157017912802651401
Scharf, H. D., and Janus, J. (1978). Zur Darstellung der Fumaraldehydsäure und einiger Derivate. Chem. Ber. 111, 2741–2744. doi:10.1002/cber.19781110733
Schenck, G. O. (1953). Photochemische Reaktionen II. Über die unsensibilisierte und photosensibilisierte Autoxydation von Furanen. Justus Liebigs Ann. Chem. 584, 156–176. doi:10.1002/jlac.19535840111
Schweitzer, C., and Schmidt, R. (2003). Physical mechanisms of generation and deactivation of singlet oxygen. Chem. Rev. 103, 1685–1758. doi:10.1021/cr010371d
Semmelhack, M. F., Schmid, C. R., Cortés, D. A., and Chou, C. S. (1984). Oxidation of alcohols to aldehydes with oxygen and cupric ion, mediated by nitrosonium ion. J. Am. Chem. Soc. 106, 3374–3376. doi:10.1021/ja00323a064
Sheldon, R. A. (2014). Green and sustainable manufacture of chemicals from biomass: state of the art. Green Chem. 16, 950–963. doi:10.1039/C3GC41935E
Sherwood, J., De bruyn, M., Constantinou, A., Moity, L., McElroy, C. R., Farmer, T. J., et al. (2014). Dihydrolevoglucosenone (Cyrene) as a bio-based alternative for dipolar aprotic solvents. Chem. Commun. 50, 9650–9652. doi:10.1039/C4CC04133J
Shinde, S. H., Hengne, A., and Rode, C. V. (2020). “Lignocellulose-derived platform molecules: an introduction,” in Recent advances in development of platform chemicals. Editors S. Saravanamurugan, A. Pandey, H. Li, and A. Riiasager (Amsterdam: Elsevier), 1–31. doi:10.1016/B978-0-444-64307-0.00001-9
Shinde, S. H., and Rode, C. V. (2020). “2,5-Diformylfuran – an oxidation product of 5-hydroxymethylfurfural,” in Recent advances in development of platform chemicals - biomass, biofuels, biochemicals. Editor A. Pandey (Amsterdam: Elsevier), 95–133. doi:10.1016/B978-0-444-64307-0.00004-4
Shvydkiv, O., Yavorskyy, A., Nolan, K., Youssef, A., Riguet, E., Hoffmann, N., et al. (2010). Photosensitized addition of isopropanol to furanones in a 365 nm UV-LED microchip. Photochem. Photobiol. Sci. 9, 1601–1603. doi:10.1039/c0pp00223b
Shvydkiv, O., Yavorskyy, A., Tan, S. B., Nolan, K., Hoffmann, N., Youssef, A., et al. (2011). Microphotochemistry: a reactor comparison study using the photosensitized addition of isopropanol to furanones as a model reaction. Photochem. Photobiol. Sci. 10, 1399–1404. doi:10.1039/c1pp05024a
C. R. J. Stephenson, T. P. Yoon, and D. W. C. MacMillan (2018). Visible light photocatalysis in organic synthesis (Weinheim: Wiley VCH). doi:10.1002/9783527674145
Stini, N. A., Gkizis, P. L., and Kokotos, C. G. (2022). Cyrene: a bio-based novel and sustainable solvent for organic synthesis. Green Chem. 24, 6435–6449. doi:10.1039/D2GC02332F
Streit, U., and Bochet, C. G. (2011). The arene-alkene photocycloaddition. Beilstein J. Org. Chem. 7, 525–542. doi:10.3762/bjoc.7.61
Subbotina, E., Rukkijakan, T., Marquez-Medina, M. D., Yu, X., Johnsson, M., and Samec, J. S. M. (2021). Oxidative cleavage of C-C bonds in lignin. Nat. Chem. 13, 1118–1125. doi:10.1038/s41557-021-00783-2
Takkellapati, S., Li, T., and Gonzalez, M. A. (2018). An overview of biorefinery-derived platform chemicals from a cellulose and hemicellulose biorefinery. Clean. Technol. Environ. Policy 20, 1615–1630. doi:10.1007/s10098-018-1568-5
Tan, J. N., Ahmar, M., and Queneau, Y. (2015). Glucosyloxymethylfurfural (GMF): a creative renewable scaffold towards bioinspired architectures. Pure Appl. Chem. 87, 827–839. doi:10.1515/pac-2015-0202
Tanielian, C. (1998). Decatungstate photocatalysis. Coord. Chem. Rev. 178-180, 1165–1181. doi:10.1016/S0010-8545(98)00160-X
Thomas, F. (2007). Ten years of a biomimetic approach to the copper(II) radical site of galactose oxidase. Eur. J. Inorg. Chem. 2007, 2379–2404. doi:10.1002/ejic.200601091
Tietze, L. F. (1996). Domino reactions in organic synthesis. Chem. Rev. 96, 115–136. doi:10.1021/cr950027e
Tietze, L. F. (2014). “Introduction,” in Domino reactions. Editor L. F. Tietze (Weinheim: Wiley VCH), 1–5. doi:10.1002/9783527671304.ch0
Tietze, L. F., and Haunert, F. (2000). “Domino reactions in organic synthesis. An approach to efficiency, elegance, ecological benefit, economic advantage and preservation of our resources,”. Editors Vögle F, J. F. Stoddart, and M. Shibasaki (Weinheim: Wiley VCH), 39–64. doi:10.1002/3527605746.ch4
Tsai, Y., Borini Etichetti, C. M., Di Benedetto, C., Girardini, J. E., Terra Martins, F., Spanevello, R. A., et al. (2018). Synthesis of triazole derivatives of levoglucosenone as promising anticancer agents: effective exploration of the chemical space through retro-aza-Michael//aza-Michael isomerizations. J. Org. Chem. 83, 3516–3528. doi:10.1021/acs.joc.7b03141
Turconi, J., Griolet, F., Guevel, R., Oddon, G., Villa, R., and Geatti, A. (2014). Semisynthetic Artemisinin, the Chemical Path to Industrial Production. Org. Process Res. Dev. 18, 417–422. doi:10.1021/op4003196
Turro, N. J., and Schuster, G. (1975). Photochemical reactions as a tool in organic syntheses. Science 187, 303–312. doi:10.1126/science.187.4174.303
Tyburski, R., Liu, T., Glover, S. D., and Hammarström, L. (2021). Proton-coupled electron transfer guidelines, fair and square. J. Am. Chem. Soc. 143, 560–576. doi:10.1021/jacs.0c09106
Unger, F. M. (1981). The chemistry and biological significance of 3-Deoxy-d-manno-2-Octulosonic acid (KDO). Adv. Carbohydr. Chem. Biochem. 38, 323–388. doi:10.1016/S0065-2318(08)60313-3
Vajravel, S., Cid Gomes, L., Rana, A., and Ottosson, H. (2023). Towards combined photobiological-photochemical formation of kerosene-type biofuels: which small 1,3-diene photodimerizes most efficiently? Photochem. Photobiol. Sci. 22, 1875–1888. doi:10.1007/s43630-023-00418-0
van Leeuwen, B. N. M., van der Wulp, A. M., Duijnstee, I., van Maris, J. A., and Straathof, J. J. (2012). Fermentative production of isobutene. App. Microbiol. Biotechnol. 93, 1377–1387. doi:10.1007/s00253-011-3853-7
van Putten, R. J., van der Waal, J. C., de Jong, E., Rasrendra, C. B., Heeres, H. J., and de Vries, J. G. (2013). Hydroxymethylfurfural, A versatile platform chemical made from renewable resources. Chem. Rev. 113, 1499–1597. doi:10.1021/cr300182k
Velty, A., Iborra, S., and Corma, A. (2022). Synthetic routes for designing furanic and non furanic biobased surfactants from 5-hydroxymethylfurfural. ChemSusChem 15, e202200181. doi:10.1002/cssc.202200181
Verrat, C. (2000). Photocycloadditions [2 + 2] intramoléculaires d’éthers de polyphénols: accès au squelette de produits naturels hétérocycliques. Université de Reims Champagne-Ardenne. PhD thesis.
Verrat, C., Hoffmann, N., and Pete, J. P. (2000). An easy access to benzo[f]isoquinoline derivatives using benzocyclobutenes derived from resorcinol. Synlett, 1166–1168. doi:10.1055/s-2000-6737
Wau, J. S., Robertson, M. J., and Olegemöller, M. (2021). Solar Photooxygenations for the Manufacturing of Fine Chemicals–Technologies and Applications. Molecules 26, 1685. doi:10.3390/molecules26061685
Wender, P. R., Ternansky, R., deLong, M., Singh, S., Olivero, A., and Rice, K. (1990). Arene-alkene cycloadditions and organic synthesis. Pure Appl. Chem. 62, 1597–1602. doi:10.1351/pac199062081597
Wilson, J., Gering, S., Pinard, J., Lucas, R., and Briggs, B. R. (2018). Bio-production of gaseous alkenes: ethylene, isoprene, isobutene. Biotechnol. Biofuels 11, 234. doi:10.1186/s13068-018-1230-9
Wu, X., Luo, N., Xie, S., Zhang, H., Zhang, Q., Wang, F., et al. (2020). Photocatalytic transformations of lignocellulosic biomass into chemicals. Chem. Soc. Rev. 49, 6198–6223. doi:10.1039/D0CS00314J
Wuts, P. G. M. (2014). Protective groups in organic synthesis. 5th edition. Hoboken: John Wiley and Sons.
Xie, J., Pan, L., Nie, G., Xie, J., Liu, Y., Ma, C., et al. (2019). Photoinduced cycloaddition of biomass derivatives to obtain high-performance spiro-fuel. Green Chem. 21, 5886–5895. doi:10.1039/C9GC02790D
Yamada, K., Fukuyama, T., Fujii, S., Ravelli, D., Fagnoni, M., and Ryu, I. (2017). Cooperative polar/steric strategy in achieving site-selective photocatalyzed C(sp3)−H functionalization. Chem. Eur. J. 23, 8615–8618. doi:10.1002/chem.201701865
Yamada, S., and Matsumoto, M. (1992). Photochemical α-cleavage reaction of levoglucosenone. Chem. Lett. 21, 2273–2276. doi:10.1246/cl.1992.2273
Yan, J., Slanina, T., Bergman, J., and Ottosson, H. (2023). Photochemistry driven by excited-state aromaticity gain or antiaromaticity relief. Chem. Eur. J. 29, e202203748. doi:10.1002/chem.202203748
Yavorskyy, A., Shvydkiv, O., Hoffmann, N., Nolan, K., and Oelgemöller, M. (2011). Photosensitized addition of isopropanol to furanones in a continuous-flow dual capillary microreactor. Tetrahedron Lett. 52, 278–280. doi:10.1016/j.tetlet.2010.11.018
Yavorskyy, A., Shvydkiv, O., Hoffmann, N., Nolan, K., and Oelgemöller, M. (2012). Parallel microflow photochemistry: process optimization, scale-up, and library synthesis. Org. Lett. 14, 4342–4345. doi:10.1021/ol301773r
Yue, X., and Queneau, Y. (2022). 5-Hydroxymethylfurfural and furfural chemistry toward biobased surfactants. ChemSusChem 15, e202102660. doi:10.1002/cssc.202102660
Zakzeski, J., Bruijnincx, P. C. A., Jongerius, A. L., and Weckhuysen, B. M. (2010). The catalytic valorization of lignin for the production of renewable chemicals. Chem. Rev. 110, 3552–3599. doi:10.1021/cr900354u
Zeitsch, K. J. (2000). The chemistry and Technology of furfural and its many by-products. Amsterdam: Elsevier.
Zhang, C., Shen, X., Jin, Y., Cheng, J., Cai, C., and Wang, F. (2023). Catalytic strategies and mechanism analysis orbiting the center of critical intermediates in lignin depolymerization. Chem. Rev. 123, 4510–4601. doi:10.1021/acs.chemrev.2c00664
Zhang, C., and Wang, F. (2022). Lignin conversion catalysis: transformation to aromatic chemicals. Weinheim: Wiley VCH. doi:10.1002/9783527835034
Zhang, J. (2018). Conversion of lignin models by photoredox catalysis. ChemSusChem 11, 3071–3080. doi:10.1002/cssc.201801370
Zhang, S., Chen, Z., G, J. F., Sang, W., Jiang, M., Li, S., et al. (2023). Recent progress in metal-catalyzed selective oxidation of 5-hydroxymethylfurfural into furan-based value-added chemicals. Chem. Rec. 23, e202300019. doi:10.1002/tcr.202300019
Zhang, Z., Zhou, Y. J., and Liang, X. W. (2020). Total synthesis of natural products using photocycloaddition reactions of arenes. Org. Biomol. Chem. 18, 5558–5566. doi:10.1039/d0ob01204a
Zhou, N., Thilakarathna, WPDW, He, Q. S., and Rupasinghe, H. P. V. (2022). A review: depolymerization of lignin to generate high-value bio-products: opportunities, challenges, and prospects. Front. Energy Res. 9, 758744. doi:10.3389/fenrg.2021.758744
Zhu, W. F., Empel, C., Pelliccia, S., Koenigs, R. M., Proschak, E., and Hernandez-Olmos, V. (2024). Photochemistry in medicinal chemistry and chemical biology. Chem. Biol. J. Med. Chem. 67, 4322–4345. doi:10.1021/acs.jmedchem.3c02109
Keywords: carbohydrates, furans, levoglucosenone, lignin, organic synthesis, photocatalysis, terpenes, vanillin
Citation: Hoffmann N, Gomez Fernandez MA, Desvals A, Lefebvre C, Michelin C and Latrache M (2024) Photochemical reactions of biomass derived platform chemicals. Front. Chem. 12:1485354. doi: 10.3389/fchem.2024.1485354
Received: 23 August 2024; Accepted: 21 November 2024;
Published: 10 December 2024.
Edited by:
James Clark, University of York, United KingdomReviewed by:
Saikat Dutta, National Institute of Technology, Karnataka, IndiaCopyright © 2024 Hoffmann, Gomez Fernandez, Desvals, Lefebvre, Michelin and Latrache. This is an open-access article distributed under the terms of the Creative Commons Attribution License (CC BY). The use, distribution or reproduction in other forums is permitted, provided the original author(s) and the copyright owner(s) are credited and that the original publication in this journal is cited, in accordance with accepted academic practice. No use, distribution or reproduction is permitted which does not comply with these terms.
*Correspondence: Norbert Hoffmann, bm9yYmVydC5ob2ZmbWFubkBpcGNtcy51bmlzdHJhLmZy
Disclaimer: All claims expressed in this article are solely those of the authors and do not necessarily represent those of their affiliated organizations, or those of the publisher, the editors and the reviewers. Any product that may be evaluated in this article or claim that may be made by its manufacturer is not guaranteed or endorsed by the publisher.
Research integrity at Frontiers
Learn more about the work of our research integrity team to safeguard the quality of each article we publish.