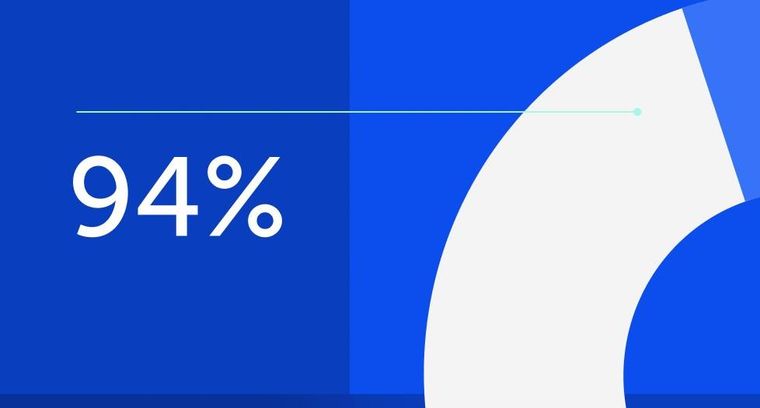
94% of researchers rate our articles as excellent or good
Learn more about the work of our research integrity team to safeguard the quality of each article we publish.
Find out more
HYPOTHESIS AND THEORY article
Front. Allergy, 27 March 2025
Sec. Infections and Microbiome
Volume 6 - 2025 | https://doi.org/10.3389/falgy.2025.1537467
The Acari Hypothesis proposes that vector-active acarians, i.e., mites and ticks, are the etiologic agents responsible for most, if not all, allergies. A corollary of The Hypothesis posits allergies are now more prevalent because contemporary hygienic practices remove from skin elements of sweat that otherwise deter acarians. Because the antimicrobial activity of sweat extends beyond acarians, disruption/removal of sweat on/from skin must enable aberrant microbial colonization, possibly potentiating comorbid conditions assignable to the aberrant microbial colonist(s). Allergy is strongly comorbid with metabolic syndrome. Available evidence links the principal features of metabolic syndrome to Staphylococcus aureus, an organism influenced significantly by constituents of sweat. Thus, the removal of sweat predisposes to both allergy and metabolic syndrome. Indeed, the “immune-compromised” state brought upon by contemporary hygienic practices likely accounts for the comorbidity of many contemporary medical conditions, examples of which are highlighted.
Scientists and clinicians alike have long suspected that the contemporary lifestyle disrupts human physiology in ways not yet fully understood or even imagined (1). This suspicion has been prompted by the increasing incidence of contemporary medical conditions, e.g., allergy, metabolic syndrome (MetS), Alzheimer disease, polycystic ovarian syndrome, etc. (2–5). Despite disparate pathophysiologies, many contemporary conditions are comorbid with allergy (6–9), hinting that the conditions share an underlying cause. The Acari Hypothesis and its corollaries presuppose that hygienic removal of eccrine gland secretions, i.e., sweat, is responsible for the modern-day allergy epidemic (10). Removal of sweat permits aberrant acarian—human interactions, enabling generation of IgE that is deleterious.
The Acari Hypothesis was formulated to account for the clinical and epidemiological features of allergic disease (10–15). Analysis of these features, when interpreted in the context of alpha-gal hypersensitivity and synanthropic acarians, implicates dust mites, e.g., Dermatophagoides farinae, as causative agents of allergy (10–15).
Although The Hypothesis focuses on acarians and their involvement in allergy, a host of pathogens and their associated conditions are influenced by immune effectors found in sweat (16, 17). It stands to reason, then, that hygienic practices that disrupt and/or remove sweat must render humans vulnerable not only to acarians and allergy, but also to the other pathogens and their associated conditions. As an example, MetS, which is comorbid with asthma and atopic dermatitis (18, 19), is a contemporary disorder prevalent in developed countries (20). The symptoms of MetS, i.e., obesity, insulin resistance, hypertension (HTN) and dyslipidemia [reduced high-density lipoproteins (HDL) and elevated triglycerides and low-density lipoproteins (LDL)], have all been linked to Staphylococcus aureus (15, 21–24), a pathogenic gram-positive bacterium that both colonizes human skin and is sensitive to sweat (25). In keeping with The Hypothesis, disruption of sweat by hygienic practices likely renders humans vulnerable to S. aureus and MetS. Although The Hypothesis focuses on hygiene as the primary determinant of sweat, other factors, such as physical activity and climate control, e.g., air-conditioning, no doubt further influence the secretory activity of eccrine glands and, consequently, sweat. Not unexpectedly, the manifestations of MetS are ameliorated by regular sauna use (26–29), a measure that induces sweat. Appreciation of both the immune function of sweat and the involvement of epidermal organisms in allergy provides valuable insight into the comorbidity of allergic disease and MetS.
Although a role for sweat in thermoregulation is already well-established, evidence for the involvement of sweat in innate immunity is only just developing. Five proteins constitute 94% of the protein of healthy human sweat, Table 1 (30). The two most abundant, dermcidin and clusterin, interact with the cell membrane of S. aureus (16, 17). Given a likely role for S. aureus in MetS, it is not surprising that serum levels of both dermcidin and clusterin are increased in MetS (31–33).
Table 1. Major proteins of eccrine gland secretions (30).
Dermcidin, the most abundant protein in sweat and the precursor of several antimicrobial peptides (30), is produced in dark cells of the secretory coils of eccrine glands. It is secreted constitutively onto epidermis (34). Once secreted, it is proteolyzed into cationic and anionic species that have broad antimicrobial activity, including activity against Mycobacteria, Pseudomonas and Staphylococcus (35). Anionic derivatives interact with negatively charged phospholipids of bacterial membranes, ultimately depolarizing the membranes and leading to cell death (36). Because dermcidin is the product of an orphan gene, DCD, unique to primates (37), there is no animal model with which to assess its loss-of-function. However, a recent family study reported the clinical consequences of dermcidin loss-of-function owing to mutated DCD (38). Affected family members experienced both staphylococcal overgrowth and recalcitrant hidradenitis suppurativa (HS) (38). HS is a dermatopathology linked to demodicosis. It is characterized by elevated levels of IgE, the antibody claimed central to human anti-acarian defense (39, 40). Importantly, dermcidin isoforms are elevated in sera from patients with insulin resistance and HTN (32, 33, 41), cardinal features of MetS. Such elevation is also linked to the formation of atheromatous lesions (42). Based on all of these, a role for S. aureus in MetS is likely.
Clusterin, or apolipoprotein J (apo J), is the second most abundant protein in the sweat of healthy individuals (30). This well-conserved mammalian glycoprotein is expressed ubiquitously in most tissues, and it is secreted abundantly in many biofluids (43, 44). It selectively binds to, and aggregates, pathogenic strains of S. aureus (17). This should come as no surprise because clusterin, like dermcidin, is secreted onto epidermis, the most obvious site of first contact with staphylococcal species. Clusterin has also been linked to MetS: firstly, the serum level of clusterin is elevated in persons who have insulin resistance (45); secondly, adipocytes of obese persons overexpress clusterin (46); and thirdly, advanced atheromatous lesions responsible for MetS-associated vascular disease have an abundance of platelet-derived clusterin (47). Inasmuch as clusterin likely serves an anti-staphylococcal function, it is entirely reasonable to suppose its increased expression in the serum and atheromatous lesions of persons with MetS occurs in response to increased staphylococcal burden. Further, at least some of that burden could relate to hygiene-enabled colonization of S. aureus on epithelial surfaces.
Individuals with MetS are at high risk for cardiovascular disease (48). The condition has been reviewed extensively by others and will not be reviewed here except to note that, like allergy, MetS was only first described in the post-hygiene era (49). Although others have linked S. aureus to isolated individual symptoms of MetS (50), none before has either related S. aureus to the condition directly or implicated a role for hygiene in the increasing incidence/prevalence of MetS.
Obesity has long been considered an immune-compromised state (51, 52). It confers an increased risk both for carriage of pathogenic staphylococci (53, 54) and for soft tissue infection (55, 56). Importantly, because the number of eccrine glands is fixed in early childhood (57), obesity necessarily decreases the surface density of eccrine glands, rendering it suboptimal.
As shown in Table 2, doubling the ideal body weight of an individual of average height, 175 cm, results in an estimated 60% increase in body surface area (BSA) (58). All else being equal, because over a lifetime the number of eccrine glands remains constant, the increase in BSA dilutes the two-dimensional concentration of immune effectors on the skin. Given such dilution, one might expect obese individuals to be especially vulnerable to any immune-compromising influence of hygiene, e.g., removal of sweat. There is evidence, however, that adipose tissue compensates by means of intrinsic immune activity. In mice, adipocyte proliferation and hypertrophy occur locally in direct response to epidermal inoculation of S. aureus (23). Adipocytes participate in anti-staphylococcal immunity via upregulation of the antimicrobial peptide, cathelicidin. They also upregulate production of clusterin (46). It is entirely plausible, then, that increased epithelial colonization by S. aureus promotes in humans local adipogenesis.
Table 2. BSA estimations by weight (58).
MetS is associated with visceral obesity, i.e., the disproportionate expansion of adipose tissue around the intra-abdominal organs (59). This expansion predicts risk of cardiovascular disease (60). If adipocyte proliferation and hypertrophy are consequences of local immune reactivity to S. aureus, then the relevant site of bacterial colonization may be intestinal epithelium, not skin. Indeed, much available evidence indicates the microbiomes of the skin and gut are interconnected/interdependent such that dysbiosis of one affects the operation of the other (61). Thus, aberrant colonization of skin by S. aureus may facilitate aberrant colonization of intestinal epithelium by the same organism. Relatedly, multiple studies have identified S. aureus in patient stools, confirming that the intestinal epithelium of at least some individuals harbors S. aureus routinely (62–64).
Insulin resistance is a consequence of a dysregulated insulin responsiveness. Such dysregulation may occur following disruption of any of several molecular pathways (65, 66). Insulin resistance affects primarily liver, muscle and fat, and raises glucose levels in blood and other body fluids, including ones secreted onto epithelial surfaces, e.g., sweat, tears, saliva and airway surface liquid (ASL) (67–70). Elevated surface glucose markedly influences the sterility of the epithelial interface (71). For example, elevated ASL glucose promotes S. aureus colonization of human airways (72). Indeed, S. aureus has an expansive repertoire of glucose transporter genes that allow the organism to thrive in glucose-rich environments (73). Those genes also enable S. aureus to flourish under the respiration-limiting conditions effected by the human immune system. Not only is S. aureus specially adapted to exist in a glucose-rich environment, but the bacterium can also induce a glucose-rich environment via generation of an insulin-resistant state. In mammals, this occurs by either of two means. According to one, the extracellular enzymatic domain of lipoteichoic acid synthase binds to insulin and blocks insulin-mediated glucose uptake (22). According to the other, glucose intolerance is prompted by chronic exposure to the S. aureus superantigen, toxic shock syndrome toxin-1 (TSST-1) (21). In summary, S. aureus can manipulate a mammalian host to induce an insulin-resistant state. That state then promotes an epithelial microenvironment supportive of S. aureus colonization. Inasmuch as insulin resistance may prove detrimental to a mammalian host, it is entirely reasonable to suppose mammals vulnerable to such resistance would have evolved means, e.g., sweat, to deter epithelial colonization by S. aureus.
HTN is defined by persistently elevated arterial blood pressure. Although the pathogenesis of HTN is still uncertain, much progress has been made in this regard. The renin—angiotensin system (RAS) appears paramount (74, 75). Briefly, renin from the kidney converts circulating liver-derived angiotensinogen to angiotensin I. Angiotensin-converting enzyme 1 (ACE1) then converts angiotensin I to angiotensin II. Subsequently, and by means of various biochemical pathways, angiotensin II increases arterial blood pressure. As evidenced by the ability of ACE1 inhibitors to abate HTN and prevent associated end-organ damage, dysregulation of the RAS appears responsible, at least in part, for MetS-associated HTN (76, 77).
To date, investigation of ACE1 expression has focused primarily on pulmonary and renal systems. ACE1, however, is also expressed abundantly both on cells lining blood vessels and on cells lining small intestine (78), the organ about which adipose tissue accumulates in persons with MetS. If the visceral obesity of MetS is a consequence of small intestinal colonization by S. aureus, then overexpression of ACE1 in the small intestine is also likely responsible for MetS-associated HTN.
Recent studies support a role for the RAS in innate immunity (24, 79, 80). In mice, expression of ACE1 on neutrophil membranes is upregulated following exposure of the cells to S. aureus (24). Overexpression of ACE1 enhances production of reactive oxygen species (ROS), thereby increasing both neutrophil bactericidal capability and survivability following bacterial phagocytosis (24). In comparison, neutrophils of either ACE1 knockout mice or mice treated with an ACE inhibitor have increased susceptibility to lysis by S. aureus (80). Not only neutrophils, but also ACE1-expressing enterocytes phagocytize pathogens (81–83). Although S. aureus has traditionally been considered an extracellular pathogen, accumulating evidence indicates the bacterium is a facultative intracellular one (84, 85). As examples, S. aureus exists intracellularly, both in individuals with recurrent rhinosinusitis and in individuals who are asymptomatic (86, 87). S. aureus can escape the phagosome of both professional and non-professional phagocytes, and it replicates intracellularly, remaining viable for up to 120 h (83). If hygiene increases exposure to, and phagocytosis of, S. aureus by ACE1-expressing enterocytes, then it seems likely upregulation of ACE1 by enterocytes in response to S. aureus contributes to systemic HTN.
Colonization of the intestine by S. aureus may be responsible for the association of dietary red meat with HTN, insulin resistance and heart disease (88–90). Although S. aureus generates fatty acids de novo, the bacterium routinely utilizes exogenous fatty acids, incorporating them into its membrane (91). Those fatty acids include scavenged ones from the host environment, especially during times of host infection (92, 93). Fatty acid content is a determinant of membrane fluidity (94), which, in turn, influences bacterial virulence. Evidence indicates that the SaeRS system, which controls the genes responsible for bacterial virulence, including TSST-1, is regulated by long branched-chain fatty acids (95, 96). In the modern diet, the primary sources of those fatty acids are red meat and dairy (97). From the foregoing, it stands to reason that a diet rich in red meat should predispose persons with intestinal S. aureus colonization to the symptoms of MetS.
Dyslipidemia is characterized by abnormal serum lipids, including diminished HDL and elevated triglycerides and LDL (48). Interestingly, individuals with seborrheic dermatitis (SD), a dermatopathology comorbid with MetS (98, 99), have the same lipid profile (100). Not only is SD comorbid with the dyslipidemia of MetS, but also its severity correlates positively with the degree of dyslipidemia (100). Clinical data also links SD to the events of acute coronary syndrome (101).
Although the pathophysiology of SD is still only poorly understood, epidermal Malassezia is believed essential to the condition. This is evidenced both by the presence of the fungus on affected skin and by the therapeutic response of SD to anti-fungal therapy (102, 103). Malassezia are lipid-dependent fungi that reside on the epidermis of most sebum-producing mammals (104). According to The Hypothesis and its corollaries, mutualism exists between Malassezia and mammals, with mammals having evolved sebaceous glands that enable malassezial colonization (15). In short, mammals provide Malassezia with lipid-rich secretions whilst the fungus protects mammals from parasitic acarians.
If colonization of epidermis by Malassezia is essential to mammalian health, then mammals likely evolved means to support the fungus during times of environmental stress. An obvious means of such support would be to increase sebum output. This could involve trafficking lipids—the raw materials of sebum—to epidermal surfaces. Consistent with this idea, sebaceous gland output is increased in persons with SD (105). As to the environmental stress eliciting such response, the epidermis of persons with SD is characterized by bacterial dysbiosis, with S. aureus predominating (106). Access to sebum benefits that bacterium, as evidenced by clustering of S. aureus around pilosebaceous units preferentially, and by colonization of S. aureus within the units themselves (107, 108). Like Malassezia, S. aureus produces lipases that digest sebum (109, 110). Given the nutritive requirement of Malassezia and the epidermal localization of S. aureus, the two organisms undoubtedly compete for sebum lipids. Should colonization of skin by S. aureus take hold by, for instance, the hygienic removal of sweat, the epidermal balance between the bacterium and fungus would favor the bacterium. Put simply, SD ensues from a specific derangement of the epidermal microbiome. Pathophysiological aspects of SD substantiate this derangement. For example, whilst the skin of SD patients reacts strongly to oleic acid, a fatty acid liberated from sebum triglycerides by malassezial lipases (111, 112), the skin of healthy persons does not. Importantly, oleic acid is deleterious to S. aureus: it disrupts the bacterial membrane, inhibits generation of biofilms and down-regulates virulence factors (113–115). This raises the possibility that the reactivity of the skin of persons with SD is not directed against oleic acid, but is instead directed against S. aureus-derived molecules expressed in response to an oleic acid challenge.
Clinical recognition of MetS is important because it enables early identification of persons at increased risk of atherosclerosis, the leading cause of death in developed countries (116–118). Much about atherosclerosis has already been elaborated. Lipoproteins, including chylomicrons, chylomicron remnants, LDL and very low-density lipoprotein (VLDL) are central to the disease process (119). Briefly, relevant lipoproteins generated in cells of the intestine and/or liver traffic within the arterial circulation where they nucleate and, subsequently, model plaques beneath the intima of larger arteries. Plaque lipids activate endothelial cells, which then recruit platelets and monocytes (120–123). Activated platelets release granules containing a variety of chemical mediators, many of which are pro-inflammatory (124). Plaque-associated monocytes differentiate into lipid-laden macrophages, i.e., foam cells (125, 126). Overaccumulation of lipids within foam cells leads to apoptosis and inflammation, and to plaque necrosis, instability and, ultimately, rupture (127). Ruptured plaques, which are exceedingly thrombogenic, impede blood flow and render tissues distal to them ischemic (128).
Plaque formation warrants further consideration. Oxidized lipoproteins are consumed by macrophages (129, 130). The site at which oxidation occurs is still open to debate (131). Chylomicrons originate within enterocytes, the epithelial cells lining the small intestine. Enterocytes are phagocytic. One strategy by which phagocytic cells manage intracellular pathogens, including S. aureus, is generation of ROS (132, 133). Given both the phagocytic activity of enterocytes and their membranous expression of ACE1, it seems likely those cells, when infected by S. aureus, generate ROS and upregulate ACE1. Lipoproteins within such enterocytes would then, by virtue of proximity, become oxidized before their release into circulation. Thus, just as the existence of S. aureus on intestinal epithelium accounts for the HTN and visceral adiposity of MetS, the existence of S. aureus within enterocytes accounts for oxidation of the lipids of MetS-associated atheromatous lesions.
Because S. aureus associates with host lipoproteins during infection (134), those lipoproteins may retain evidence of that association. In this regard, auto-inducing peptides (AIPs) unique to S. aureus and secreted in response to fluctuations in bacterial population density, i.e., quorum sensing, are ideally suited (135). AIPs accumulate outside the bacterium, eventually reaching a concentration at which the bacterial accessory gene regulator (agr) becomes activated. Once activated, the agr stimulates production of staphylococcal virulence factors, including TSST-1 (136). Recent studies indicate the B apolipoproteins, constituents of chylomicrons, chylomicron remnants, LDLs and VLDLs (137), participate not only in lipoprotein formation and trafficking, but also in defense against S. aureus (138). In the presence of oxidized lipoprotein lipids, the B apolipoproteins bind to, and sequester, AIPs (139). Thus, at least some of the building blocks of lipoproteins oxidized in response to intracellular S. aureus will have AIPs bound to them. Those AIPs could then amplify inflammation triggered by oxidized plaque lipids. The presence of such a staphylococcal “marker” on the oxidized lipids would also explain the deposition of clusterin, an anti-staphylococcal agent, by platelets infiltrating the plaque (47).
Persons with MetS are at increased risk of peripheral neuropathy (140, 141). If S. aureus is an etiological agent of MetS, then the bacterium may, in fact, drive the development of neuropathy. Importantly, intestinal colonization by S. aureus has already been linked to peripheral neuropathy: S. aureus in the duodenum of a patient sequestered dietary thiamine, thereby deranging systemic thiamine metabolism which yielded, in turn, debilitating polyneuritis (142).
In mammals, thiamine serves as a critical co-factor for enzymes involved in the metabolism of lipids, carbohydrates and branched-chain amino acids. Thiamine deprivation causes neuropathy in diet-dependent fashion, i.e., beriberi (143, 144). Furthermore, in humans, mutation in the thiamine transporter, solute carrier family 19 member 2 (SLC19A2), is characterized by hyperglycemia (145). Given these, S. aureus may disrupt thiamine metabolism, driving hyperglycemia and ultimately producing a glucose-rich epithelial surface that benefits the bacterium. Consistent with this proposal, there are substantial bodies of evidence that thiamine supplementation protects against the development of MetS and that persons with MetS have lower serum levels of thiamine analytes (146–149).
Just as allergic disease and MetS are comorbid, so, too, are other conditions comorbid with MetS, including, but not limited to, Alzheimer disease, Parkinson disease, polycystic ovary syndrome and thyroid disease (150–153). Although beyond the scope of this report, mechanistic understandings of these diseases would likely be accelerated if subject matter experts interpreted relevant data in the context of the human epithelial microbiome. In short, contemporary diseases comorbid with allergy and/or MetS undoubtedly involve acarians, S. aureus and/or other microbes influenced by eccrine gland secretions.
Many cancers, particularly ones derived from epithelium, e.g., adenocarcinoma of the colon, are comorbid with MetS (154). Interestingly, many of these cancers have been shown to dysregulate expression of clusterin and/or dermcidin (155–161). Such dysregulation not only renders tumor cells refractory to conventional chemo- and radiation therapies, but it also confers upon them metastatic potential (162, 163). Inasmuch as MetS is attributable to hygienic disruption of sweat and consequent epithelial colonization by S. aureus, it seems possible dysregulated clusterin and dermcidin in malignant epithelial cells is due to what the cells perceive as an unremitting staphylococcal challenge.
At the cellular level, clusterin plays an important role in stress responses and survival (164, 165). It has two isoforms, an intranuclear one and a secreted one, the latter of which operates both within the cytoplasm and extracellularly (166). Intranuclear clusterin is pro-apoptotic whilst the secreted isoform is anti-apoptotic (167). Secretory clusterin is an ATP-independent chaperone with very broad substrate specificity (165). As a chaperone, it is unusual in that it operates extracellularly (168). Because secretory clusterin regulates apoptosis, it seems intuitive that its dysregulation would yield a pro-oncotic state. That being the case, it is tempting to speculate that elimination of epithelial pathogens that dysregulate clusterin might not only prevent malignant transformation but might also provide therapy for some cancers.
Per The Hypothesis and its corollaries, many diseases of modern man are attributable to disruption of the human microbiome by direct and/or indirect means. As suggested above, this new understanding provides rationale for novel approaches to disease treatment. As examples, some diseases might be ameliorated either by limiting certain hygienic practices, e.g., showering daily, or by applying topical agents that mimic endogenous immune effectors, e.g., dermcidin. To better appreciate the full disease-related impact of hygiene, comparison should be made of the microbes colonizing epithelial surfaces of persons from industrialized societies with those colonizing epithelial surfaces of persons from primitive societies. Microbes colonizing the former but not the latter should be considered potential agents of modern disease(s).
The symptomatology and pathology of allergy are attributable to specious IgE-mediated adaptive responses. In persons not yet allergic, eliminating some hygienic practices should help prevent the development of allergy. Because synanthropic mites likely elicit most allergies, an evaluation of the environment of allergic persons should be undertaken to identify the causative acarian. In this regard, nucleic acid-based testing might prove useful, as mite infestation is ofttimes not apparent clinically.
Treatment of MetS is more subtle and involved. Certainly, increasing sweat would be important because sweat renders human epidermis inhospitable to S. aureus. Unfortunately, because MetS is associated with autonomic neuropathy (169, 170), the ability of colonized individuals to sweat may be compromised. Furthermore, bacterial colonization can extend beyond epidermis, to involve the mucosae of the airway and/or the gastrointestinal tract. More research is needed to identify immune effectors operating to deter S. aureus from all these surfaces.
Evidence in support of The Hypothesis might be obtained from autopsies of decedents who died from myocardial infarction and who had both clinical evidence of visceral adiposity and methicillin-resistant Staphylococcus aureus (MRSA) infection. Segments of the small bowel that display significant visceral adiposity could be analyzed for: (1) degree of epithelial ACE expression, and (2) presence of intracellular staphylococci. For comparative purpose, these same analyses could be performed using either less fatty segments of small intestine or segments of small intestine from healthy individuals. Although such analyses would not confirm causality, they would represent a preliminary step toward validating Koch's postulates.
Although oral and/or parenteral antibiotics could play a role in the treatment of MetS, their use might be problematic because the drugs do not discriminate between native organisms and invasive pathogens. Evidence indicates native flora protect mammals from microbial pathogens, including S. aureus. Commensal coagulase-negative staphylococci, e.g., S. epidermidis, produce bacteriocins and antimicrobial peptides (AMPs) that work synergistically with cathelicidin to inhibit epithelial colonization by S. aureus (171, 172). Indeed, reintroduction of coagulase-negative staphylococci onto skin of patients with atopic dermatitis limits S. aureus colonization (172).
Just as reintroducing coagulase-negative staphylococci onto the skin of patients with atopic dermatitis limits epidermal colonization by S. aureus, replenishing and/or supplementing gut microbiota with Bacillus subtilis limits S. aureus colonization of gut epithelium (173). B. subtilis, a gram-positive bacterium found routinely in the gastrointestinal tract of mammals, produces two lipopeptides bactericidal for S. aureus, surfactin and plipastatin (174). Once daily ingestion of a probiotic containing B. subtilis eliminates 97% of S. aureus from the gut and 65% of S. aureus from the nares (173). For these reasons, supplementation with relevant microbiota might benefit individuals with MetS.
Unfortunately, because most antibiotics do not discriminate between beneficial and pathogenic bacteria, pairing an antibiotic with hygienic disruption/removal of sweat could yield a dysbiosis that favors a pathogen. Indeed, studies have shown that antibiotic usage predisposes persons to MetS (175, 176). Because the native microbiota plays a role in human defense, its application to relevant epithelial surfaces may represent the most effective therapy for MetS, perhaps even a curative one.
Mammals other than humans are also subject to epithelial insult by S. aureus (177). That being the case, they, too, are likely to produce AMPs that selectively deter the bacterium. Indeed, epithelial secretions from mammalian sources may represent pharmaceutically useful agents. In this regard, breast milk seems especially promising: not only does it contain and support the growth of multiple microorganisms, but it also contains compounds that selectively inhibit the growth of S. aureus (178, 179). Furthermore, because mammalian mothers provide breast milk to their offspring, the microorganisms in that medium undoubtedly align with the intended health and fitness of the offspring.
The Acari Hypothesis provides rationale not only for allergy, but also for diseases of modernity comorbid with allergy. The authors hope this new understanding prompts serious consideration of both the epithelial microbiome and aerobiological elements in health and disease.
The original contributions presented in the study are included in the article/Supplementary Material, further inquiries can be directed to the corresponding author.
ACR: Conceptualization, Investigation, Writing – original draft, Writing – review & editing. GSR: Conceptualization, Funding acquisition, Resources, Writing – review & editing.
The author(s) declare that financial support was received for the research and/or publication of this article. This research was supported in part by funding to GSR from the Department of Pathology, Feinberg School of Medicine, Northwestern University.
ACR thanks GMR, DAR, and GSR for their inspiration.
The authors declare that the research was conducted in the absence of any commercial or financial relationships that could be construed as a potential conflict of interest.
The authors declare that no Generative AI was used in the creation of this manuscript.
All claims expressed in this article are solely those of the authors and do not necessarily represent those of their affiliated organizations, or those of the publisher, the editors and the reviewers. Any product that may be evaluated in this article, or claim that may be made by its manufacturer, is not guaranteed or endorsed by the publisher.
1. Corbett S, Courtiol A, Lummaa V, Moorad J, Stearns S. The transition to modernity and chronic disease: mismatch and natural selection. Nat Rev Genet. (2018) 19(7):419–30. doi: 10.1038/s41576-018-0012-3
2. Saklayen MG. The global epidemic of the metabolic syndrome. Curr Hypertens Rep. (2018) 20(2):12. doi: 10.1007/s11906-018-0812-z
3. Gutowska-Ślesik J, Samoliński B, Krzych-Fałta E. The increase in allergic conditions based on a review of literature. Postepy Dermatol Alergol. (2023) 40(1):1–7. doi: 10.5114/ada.2022.119009
4. Li X, Feng X, Sun X, Hou N, Han F, Liu Y. Global, regional, and national burden of Alzheimer’s disease and other dementias, 1990-2019. Front Aging Neurosci. (2022) 14:937486. doi: 10.3389/fnagi.2022.937486
5. Yu O, Christ JP, Schulze-Rath R, Covey J, Kelley A, Grafton J, et al. Incidence, prevalence, and trends in polycystic ovary syndrome diagnosis: a United States population-based study from 2006 to 2019. Am J Obstet Gynecol. (2023) 229(1):39.e1–39.e12. doi: 10.1016/j.ajog.2023.04.010
6. Scelo G, Torres-Duque CA, Maspero J, Tran TN, Murray R, Martin N, et al. Analysis of comorbidities and multimorbidity in adult patients in the international severe asthma registry. Ann Allergy Asthma Immunol. (2024) 132(1):42–53. doi: 10.1016/j.anai.2023.08.021
7. Song D, Liu D, Ning W, Chen Y, Yang J, Zhao C, et al. Incidence, prevalence and characteristics of multimorbidity in different age groups among urban hospitalized patients in China. Sci Rep. (2023) 13(1):18798. doi: 10.1038/s41598-023-46227-4
8. Xu Y, Zhou ZY, Pan JX, Huang HF. Associations between asthma and polycystic ovary syndrome: current perspectives. Front Endocrinol. (2022) 13:936948. doi: 10.3389/fendo.2022.936948
9. Joh HK, Kwon H, Son KY, Yun JM, Cho SH, Han K, et al. Allergic diseases and risk of incident dementia and Alzheimer’s disease. Ann Neurol. (2023) 93(2):384–97. doi: 10.1002/ana.26506
10. Retzinger AC, Retzinger GS. The Acari hypothesis, IV: revisiting the role of hygiene in allergy. Front Allergy. (2024) 5:1415124. doi: 10.3389/falgy.2024.1415124
11. Retzinger AC, Retzinger GS. Mites, ticks, anaphylaxis and allergy: the Acari hypothesis. Med Hypotheses. (2020) 144:110257. doi: 10.1016/j.mehy.2020.110257
12. Retzinger AC, Retzinger GS. The Acari hypothesis, II: interspecies operability of pattern recognition receptors. Pathogens. (2021) 10(9):1220. doi: 10.3390/pathogens10091220
13. Retzinger AC, Retzinger GS. The Acari hypothesis, III: atopic dermatitis. Pathogens. (2022) 11(10):1083. doi: 10.3390/pathogens11101083
14. Retzinger AC, Retzinger GS. The Acari hypothesis, V: deciphering allergenicity. Front Allergy. (2024) 5:1454292. doi: 10.3389/falgy.2024.1454292
15. Retzinger AC, Retzinger GS. The Acari hypothesis, VI: human sebum and the cutaneous microbiome in allergy and in lipid homeostasis. Front Allergy. (2024) 5:1478279. doi: 10.3389/falgy.2024.1478279
16. Schittek B, Hipfel R, Sauer B, Bauer J, Kalbacher H, Stevanovic S, et al. Dermcidin: a novel human antibiotic peptide secreted by sweat glands. Nat Immunol. (2001) 2(12):1133–7. doi: 10.1038/ni732
17. Partridge SR, Baker MS, Walker MJ, Wilson MR. Clusterin, a putative complement regulator, binds to the cell surface of Staphylococcus aureus clinical isolates. Infect Immun. (1996) 64(10):4324–9. doi: 10.1128/iai.64.10.4324-4329.1996
18. De Simoni E, Rizzetto G, Molinelli E, Lucarini G, Mattioli-Belmonte M, Capodaglio I, et al. Metabolic comorbidities in pediatric atopic dermatitis: a narrative review. Life. (2022) 13(1):2. doi: 10.3390/life13010002
19. Brumpton BM, Camargo CA Jr, Romundstad PR, Langhammer A, Chen Y, Mai XM. Metabolic syndrome and incidence of asthma in adults: the HUNT study. Eur Respir J. (2013) 42(6):1495–502. doi: 10.1183/09031936.00046013
20. Gupta N, Shah P, Nayyar S, Misra A. Childhood obesity and the metabolic syndrome in developing countries. Indian J Pediatr. (2013) 80(Suppl 1):S28–37. doi: 10.1007/s12098-012-0923-5
21. Vu BG, Stach CS, Kulhankova K, Salgado-Pabón W, Klingelhutz AJ, Schlievert PM. Chronic superantigen exposure induces systemic inflammation, elevated bloodstream endotoxin, and abnormal glucose tolerance in rabbits: possible role in diabetes. mBio. (2015) 6(2):e02554. doi: 10.1128/mBio.02554-14
22. Liu Y, Liu FJ, Guan ZC, Dong FT, Cheng JH, Gao YP, et al. The extracellular domain of Staphylococcus aureus LtaS binds insulin and induces insulin resistance during infection. Nat Microbiol. (2018) 3(5):622–31. doi: 10.1038/s41564-018-0146-2
23. Zhang LJ, Guerrero-Juarez CF, Hata T, Bapat SP, Ramos R, Plikus MV, et al. Innate immunity. Dermal adipocytes protect against invasive Staphylococcus aureus skin infection. Science. (2015) 347(6217):67–71. doi: 10.1126/science.1260972
24. Khan Z, Shen XZ, Bernstein EA, Giani JF, Eriguchi M, Zhao TV, et al. Angiotensin-converting enzyme enhances the oxidative response and bactericidal activity of neutrophils. Blood. (2017) 130(3):328–39. doi: 10.1182/blood-2016-11-752006
25. Senyürek I, Paulmann M, Sinnberg T, Kalbacher H, Deeg M, Gutsmann T, et al. Dermcidin-derived peptides show a different mode of action than the cathelicidin LL-37 against Staphylococcus aureus. Antimicrob Agents Chemother. (2009) 53(6):2499–509. doi: 10.1128/AAC.01679-08
26. Gryka D, Pilch W, Szarek M, Szygula Z, Tota Ł. The effect of sauna bathing on lipid profile in young, physically active, male subjects. Int J Occup Med Environ Health. (2014) 27(4):608–18. doi: 10.2478/s13382-014-0281-9
27. Zaccardi F, Laukkanen T, Willeit P, Kunutsor SK, Kauhanen J, Laukkanen JA. Sauna bathing and incident hypertension: a prospective cohort study. Am J Hypertens. (2017) 30(11):1120–5. doi: 10.1093/ajh/hpx102
28. Laukkanen T, Kunutsor SK, Khan H, Willeit P, Zaccardi F, Laukkanen JA. Sauna bathing is associated with reduced cardiovascular mortality and improves risk prediction in men and women: a prospective cohort study. BMC Med. (2018) 16(1):219. doi: 10.1186/s12916-018-1198-0
29. McCarty MF, Barroso-Aranda J, Contreras F. Regular thermal therapy may promote insulin sensitivity while boosting expression of endothelial nitric oxide synthase–effects comparable to those of exercise training. Med Hypotheses. (2009) 73(1):103–5. doi: 10.1016/j.mehy.2008.12.020
30. Csősz É, Emri G, Kalló G, Tsaprailis G, Tőzsér J. Highly abundant defense proteins in human sweat as revealed by targeted proteomics and label-free quantification mass spectrometry. J Eur Acad Dermatol Venereol. (2015) 29(10):2024–31. doi: 10.1111/jdv.13221
31. Won JC, Park CY, Oh SW, Lee ES, Youn BS, Kim MS. Plasma clusterin (ApoJ) levels are associated with adiposity and systemic inflammation. PLoS One. (2014) 9(7):e103351. doi: 10.1371/journal.pone.0103351
32. Bhattacharya S, Khan MM, Ghosh C, Bank S, Maiti S. The role of dermcidin isoform-2 in the occurrence and severity of diabetes. Sci Rep. (2017) 7(1):8252. doi: 10.1038/s41598-017-07958-3
33. Ghosh R, Bank S, Bhattacharya R, Khan NN, Sinha AK. Neutralization by insulin of the hypertensive effect of dermcidin isoform 2: an environmentally induced diabetogenic and hypertensive protein. Cardiol Res Pract. (2014) 2014:412815. doi: 10.1155/2014/412815
34. Rieg S, Garbe C, Sauer B, Kalbacher H, Schittek B. Dermcidin is constitutively produced by eccrine sweat glands and is not induced in epidermal cells under inflammatory skin conditions. Br J Dermatol. (2004) 151(3):534–9. doi: 10.1111/j.1365-2133.2004.06081.x
35. Steffen H, Rieg S, Wiedemann I, Kalbacher H, Deeg M, Sahl HG, et al. Naturally processed dermcidin-derived peptides do not permeabilize bacterial membranes and kill microorganisms irrespective of their charge. Antimicrob Agents Chemother. (2006) 50(8):2608–20. doi: 10.1128/AAC.00181-06
36. Paulmann M, Arnold T, Linke D, Özdirekcan S, Kopp A, Gutsmann T, et al. Structure-activity analysis of the dermcidin-derived peptide DCD-1l, an anionic antimicrobial peptide present in human sweat. J Biol Chem. (2012) 287(11):8434–43. doi: 10.1074/jbc.M111.332270
37. Schittek B. The multiple facets of dermcidin in cell survival and host defense. J Innate Immun. (2012) 4(4):349–60. doi: 10.1159/000336844
38. Tricarico PM, Gratton R, Dos Santos-Silva CA, de Moura RR, Ura B, Sommella E, et al. A rare loss-of-function genetic mutation suggest a role of dermcidin deficiency in hidradenitis suppurativa pathogenesis. Front Immunol. (2022) 13:1060547. doi: 10.3389/fimmu.2022.1060547
39. Ünal E, Güvendi Akçınar U, Arduç A. Hidradenitis suppurativa, metabolic syndrome, and demodex spp. Infestation. Turkiye Parazitol Derg. (2018) 42(2):171–4. doi: 10.5152/tpd.2018.5330
40. Pascual JC, García-Martínez FJ, Martorell A, González I, Hispan P. Increased total serum IgE levels in moderate-to-severe hidradenitis suppurativa. Br J Dermatol. (2016) 175(5):1101–2. doi: 10.1111/bjd.14870
41. Akan OY, Bilgir O. The relation of dermcidin with insulin resistance and inflammation in women with polycystic ovary syndrome. Rev Assoc Med Bras. (2022) 68(6):820–6. doi: 10.1590/1806-9282.20211215
42. Ghosh R, Maji UK, Bhattacharya R, Sinha AK. The role of dermcidin isoform 2: a two-faceted atherosclerotic risk factor for coronary artery disease and the effect of acetyl salicylic acid on it. Thrombosis. (2012) 2012:987932. doi: 10.1155/2012/987932
43. Jones SE, Jomary C. Clusterin. Int J Biochem Cell Biol. (2002) 34(5):427–31. doi: 10.1016/s1357-2725(01)00155-8
44. de Silva HV, Harmony JA, Stuart WD, Gil CM, Robbins J. Apolipoprotein J: structure and tissue distribution. Biochemistry. (1990) 29(22):5380–9. doi: 10.1021/bi00474a025
45. Seo JA, Kang MC, Ciaraldi TP, Kim SS, Park KS, Choe C, et al. Circulating ApoJ is closely associated with insulin resistance in human subjects. Metab Clin Exp. (2018) 78:155–66. doi: 10.1016/j.metabol.2017.09.014
46. Wittwer J, Bradley D. Clusterin and its role in insulin resistance and the cardiometabolic syndrome. Front Immunol. (2021) 12:612496. doi: 10.3389/fimmu.2021.612496
47. Witte DP, Aronow BJ, Stauderman ML, Stuart WD, Clay MA, Gruppo RA, et al. Platelet activation releases megakaryocyte-synthesized apolipoprotein J, a highly abundant protein in atheromatous lesions. Am J Pathol. (1993) 143(3):763–73.8362975
48. Fahed G, Aoun L, Bou Zerdan M, Allam S, Bou Zerdan M, Bouferraa Y, et al. Metabolic syndrome: updates on pathophysiology and management in 2021. Int J Mol Sci. (2022) 23(2):786. doi: 10.3390/ijms23020786
49. Oda E. Historical perspectives of the metabolic syndrome. Clin Dermatol. (2018) 36(1):3–8. doi: 10.1016/j.clindermatol.2017.09.002
50. Schlievert PM, Salgado-Pabón W, Klingelhutz AJ. Does Staphylococcus aureus have a role in the development of type 2 diabetes mellitus? Future Microbiol. (2015) 10(10):1549–52. doi: 10.2217/fmb.15.95
51. Milner JJ, Beck MA. The impact of obesity on the immune response to infection. Proc Nutr Soc. (2012) 71(2):298–306. doi: 10.1017/S0029665112000158
52. Muscogiuri G, Pugliese G, Laudisio D, Castellucci B, Barrea L, Savastano S, et al. The impact of obesity on immune response to infection: plausible mechanisms and outcomes. Obes Rev. (2021) 22(6):e13216. doi: 10.1111/obr.13216
53. Olsen K, Danielsen K, Wilsgaard T, Sangvik M, Sollid JU, Thune I, et al. Obesity and Staphylococcus aureus nasal colonization among women and men in a general population. PLoS One. (2013) 8(5):e63716. doi: 10.1371/journal.pone.0063716
54. Befus M, Lowy FD, Miko BA, Mukherjee DV, Herzig CT, Larson EL. Obesity as a determinant of Staphylococcus aureus colonization among inmates in Maximum-security prisons in New York state. Am J Epidemiol. (2015) 182(6):494–502. doi: 10.1093/aje/kwv062
55. Ghilotti F, Bellocco R, Ye W, Adami HO, Trolle Lagerros Y. Obesity and risk of infections: results from men and women in the Swedish national march cohort. Int J Epidemiol. (2019) 48(6):1783–94. doi: 10.1093/ije/dyz129; Erratum in: Int J Epidemiol. 2020 April 1;49(2):708. doi: 10.1093/ije/dyaa02531292615
56. Hu H, Mei J, Lin M, Wu X, Lin H, Chen G. The causal relationship between obesity and skin and soft tissue infections: a two-sample Mendelian randomization study. Front Endocrinol. (2022) 13:996863. doi: 10.3389/fendo.2022.996863
57. Wells TR, Landing BH, Sandhu M, Lipsey AI. Microdissection study of the incidence of branched eccrine sweat glands and the number of eccrine glands per unit area of infants’ and childrens’ skin. Pediatr Pathol. (1986) 6(2-3):301–7. doi: 10.3109/15513818609037720
58. Livingston EH, Lee S. Body surface area prediction in normal-weight and obese patients. Am J Physiol Endocrinol Metab. (2001) 281(3):E586–91. doi: 10.1152/ajpendo.2001.281.3.E586
59. Shuster A, Patlas M, Pinthus JH, Mourtzakis M. The clinical importance of visceral adiposity: a critical review of methods for visceral adipose tissue analysis. Br J Radiol. (2012) 85(1009):1–10. doi: 10.1259/bjr/38447238
60. Gruzdeva O, Borodkina D, Uchasova E, Dyleva Y, Barbarash O. Localization of fat depots and cardiovascular risk. Lipids Health Dis. (2018) 17(1):218. doi: 10.1186/s12944-018-0856-8
61. De Pessemier B, Grine L, Debaere M, Maes A, Paetzold B, Callewaert C. Gut-skin axis: current knowledge of the interrelationship between microbial dysbiosis and skin conditions. Microorganisms. (2021) 9(2):353. doi: 10.3390/microorganisms9020353
62. Gagnaire J, Verhoeven PO, Grattard F, Rigaill J, Lucht F, Pozzetto B, et al. Epidemiology and clinical relevance of Staphylococcus aureus intestinal carriage: a systematic review and meta-analysis. Expert Rev Anti Infect Ther. (2017) 15(8):767–85. doi: 10.1080/14787210.2017.1358611
63. Acton DS, Plat-Sinnige MJ, van Wamel W, de Groot N, van Belkum A. Intestinal carriage of Staphylococcus aureus: how does its frequency compare with that of nasal carriage and what is its clinical impact? Eur J Clin Microbiol Infect Dis. (2009) 28(2):115–27. doi: 10.1007/s10096-008-0602-7
64. Kates AE, Thapaliya D, Smith TC, Chorazy ML. Prevalence and molecular characterization of Staphylococcus aureus from human stool samples. Antimicrob Resist Infect Control. (2018) 7:42. doi: 10.1186/s13756-018-0331-3
65. Petersen MC, Shulman GI. Mechanisms of insulin action and insulin resistance. Physiol Rev. (2018) 98(4):2133–223. doi: 10.1152/physrev.00063.2017
66. Lee SH, Park SY, Choi CS. Insulin resistance: from mechanisms to therapeutic strategies. Diabetes Metab J. (2022) 46(1):15–37. doi: 10.4093/dmj.2021.0280
67. Moyer J, Wilson D, Finkelshtein I, Wong B, Potts R. Correlation between sweat glucose and blood glucose in subjects with diabetes. Diabetes Technol Ther. (2012) 14(5):398–402. doi: 10.1089/dia.2011.0262
68. Aihara M, Kubota N, Minami T, Shirakawa R, Sakurai Y, Hayashi T, et al. Association between tear and blood glucose concentrations: random intercept model adjusted with confounders in tear samples negative for occult blood. J Diabetes Investig. (2021) 12(2):266–76. doi: 10.1111/jdi.13344
69. Baker EH, Baines DL. Airway glucose homeostasis: a new target in the prevention and treatment of pulmonary infection. Chest. (2018) 153(2):507–14. doi: 10.1016/j.chest.2017.05.031
70. Mishra N, Trivedi A, Gajdhar SK, Bhagwat H, Khutwad GK, Mall PE, et al. Correlation of blood glucose levels, salivary glucose levels and oral colony forming units of Candida albicans in type 2 diabetes Mellitus patients. J Contemp Dent Pract. (2019) 20(4):494–8. doi: 10.5005/jp-journals-10024-2545
71. Pezzulo AA, Gutiérrez J, Duschner KS, McConnell KS, Taft PJ, Ernst SE, et al. Glucose depletion in the airway surface liquid is essential for sterility of the airways. PLoS One. (2011) 6(1):e16166. doi: 10.1371/journal.pone.0016166
72. Garnett JP, Baker EH, Naik S, Lindsay JA, Knight GM, Gill S, et al. Metformin reduces airway glucose permeability and hyperglycaemia-induced Staphylococcus aureus load independently of effects on blood glucose. Thorax. (2013) 68(9):835–45. doi: 10.1136/thoraxjnl-2012-203178
73. Vitko NP, Grosser MR, Khatri D, Lance TR, Richardson AR. Expanded glucose import capability affords Staphylococcus aureus optimized glycolytic flux during infection. mBio. (2016) 7(3):e00296–16. doi: 10.1128/mBio.00296-16
74. Muñoz-Durango N, Fuentes CA, Castillo AE, González-Gómez LM, Vecchiola A, Fardella CE, et al. Role of the renin-angiotensin-aldosterone system beyond blood pressure regulation: molecular and cellular mechanisms involved in end-organ damage during arterial hypertension. Int J Mol Sci. (2016) 17(7):797. doi: 10.3390/ijms17070797
75. Allikmets K, Parik T, Viigimaa M. The renin-angiotensin system in essential hypertension: associations with cardiovascular risk. Blood Press. (1999) 8(2):70–8. doi: 10.1080/080370599438239
76. Nehme A, Zibara K. Efficiency and specificity of RAAS inhibitors in cardiovascular diseases: how to achieve better end-organ protection? Hypertens Res. (2017) 40(11):903–9. doi: 10.1038/hr.2017.65
77. Takimoto-Ohnishi E, Murakami K. Renin-angiotensin system research: from molecules to the whole body. J Physiol Sci. (2019) 69(4):581–7. doi: 10.1007/s12576-019-00679-4
78. Bruneval P, Hinglais N, Alhenc-Gelas F, Tricottet V, Corvol P, Menard J, et al. Angiotensin I converting enzyme in human intestine and kidney. Ultrastructural immunohistochemical localization. Histochemistry. (1986) 85(1):73–80. doi: 10.1007/BF00508656
79. Cao DY, Saito S, Veiras LC, Okwan-Duodu D, Bernstein EA, Giani JF, et al. Role of angiotensin-converting enzyme in myeloid cell immune responses. Cell Mol Biol Lett. (2020) 25:31. doi: 10.1186/s11658-020-00225-w
80. Cao DY, Giani JF, Veiras LC, Bernstein EA, Okwan-Duodu D, Ahmed F, et al. An ACE inhibitor reduces bactericidal activity of human neutrophils in vitro and impairs mouse neutrophil activity in vivo. Sci Transl Med. (2021) 13(604):eabj2138. doi: 10.1126/scitranslmed.abj2138
81. Neal MD, Leaphart C, Levy R, Prince J, Billiar TR, Watkins S, et al. Enterocyte TLR4 mediates phagocytosis and translocation of bacteria across the intestinal barrier. J Immunol. (2006) 176(5):3070–9. doi: 10.4049/jimmunol.176.5.3070
82. Dean P, Quitard S, Bulmer DM, Roe AJ, Kenny B. Cultured enterocytes internalise bacteria across their basolateral surface for, pathogen-inhibitable, trafficking to the apical compartment. Sci Rep. (2015) 5:17359. doi: 10.1038/srep17359
83. Hess DJ, Henry-Stanley MJ, Erickson EA, Wells CL. Intracellular survival of Staphylococcus aureus within cultured enterocytes. J Surg Res. (2003) 114(1):42–9. doi: 10.1016/s0022-4804(03)00314-7
84. Hommes JW, Surewaard BGJ. Intracellular habitation of Staphylococcus aureus: molecular mechanisms and prospects for antimicrobial therapy. Biomedicines. (2022) 10(8):1804. doi: 10.3390/biomedicines10081804
85. Fraunholz M, Sinha B. Intracellular Staphylococcus aureus: live-in and let die. Front Cell Infect Microbiol. (2012) 2:43. doi: 10.3389/fcimb.2012.00043
86. Clement S, Vaudaux P, Francois P, Schrenzel J, Huggler E, Kampf S, et al. Evidence of an intracellular reservoir in the nasal mucosa of patients with recurrent Staphylococcus aureus rhinosinusitis. J Infect Dis. (2005) 192(6):1023–8. doi: 10.1086/432735
87. Hanssen AM, Kindlund B, Stenklev NC, Furberg AS, Fismen S, Olsen RS, et al. Localization of Staphylococcus aureus in tissue from the nasal vestibule in healthy carriers. BMC Microbiol. (2017) 17(1):89. doi: 10.1186/s12866-017-0997-3
88. Gu X, Drouin-Chartier JP, Sacks FM, Hu FB, Rosner B, Willett WC. Red meat intake and risk of type 2 diabetes in a prospective cohort study of United States females and males. Am J Clin Nutr. (2023) 118(6):1153–63. doi: 10.1016/j.ajcnut.2023.08.021
89. Shi W, Huang X, Schooling CM, Zhao JV. Red meat consumption, cardiovascular diseases, and diabetes: a systematic review and meta-analysis. Eur Heart J. (2023) 44(28):2626–35. doi: 10.1093/eurheartj/ehad336
90. Allen TS, Bhatia HS, Wood AC, Momin SR, Allison MA. State-of-the-art review: evidence on red meat consumption and hypertension outcomes. Am J Hypertens. (2022) 35(8):679–87. doi: 10.1093/ajh/hpac064
91. DeMars Z, Singh VK, Bose JL. Exogenous fatty acids remodel Staphylococcus aureus lipid composition through fatty acid kinase. J Bacteriol. (2020) 202(14):e00128–20. doi: 10.1128/JB.00128-20
92. Teoh WP, Chen X, Laczkovich I, Alonzo F 3rd. Staphylococcus aureus adapts to the host nutritional landscape to overcome tissue-specific branched-chain fatty acid requirement. Proc Natl Acad Sci U S A. (2021) 118(13):e2022720118. doi: 10.1073/pnas.2022720118
93. Frank MW, Yao J, Batte JL, Gullett JM, Subramanian C, Rosch JW, et al. Host fatty acid utilization by Staphylococcus aureus at the infection site. mBio. (2020) 11(3):e00920–20. doi: 10.1128/mBio.00920-20
94. Braungardt H, Singh VK. Impact of deficiencies in branched-chain fatty acids and staphyloxanthin in Staphylococcus aureus. Biomed Res Int. (2019) 2019:2603435. doi: 10.1155/2019/2603435
95. Pendleton A, Yeo WS, Alqahtani S, DiMaggio DA Jr, Stone CJ, Li Z, et al. Regulation of the sae two-component system by branched-chain fatty acids in Staphylococcus aureus. mBio. (2022) 13(5):e0147222. doi: 10.1128/mbio.01472-22
96. Baroja ML, Herfst CA, Kasper KJ, Xu SX, Gillett DA, Li J, et al. The SaeRS two-component system is a direct and dominant transcriptional activator of toxic shock syndrome toxin 1 in Staphylococcus aureus. J Bacteriol. (2016) 198(19):2732–42. doi: 10.1128/JB.00425-16
97. Ran-Ressler RR, Bae S, Lawrence P, Wang DH, Brenna JT. Branched-chain fatty acid content of foods and estimated intake in the USA. Br J Nutr. (2014) 112(4):565–72. doi: 10.1017/S0007114514001081
98. Imamoglu B, Hayta SB, Guner R, Akyol M, Ozcelik S. Metabolic syndrome may be an important comorbidity in patients with seborrheic dermatitis. Arch Med Sci Atheroscler Dis. (2016) 1(1):e158–61. doi: 10.5114/amsad.2016.65075
99. Akbaş A, Kılınç F, Şener S, Hayran Y. Investigation of the relationship between seborrheic dermatitis and metabolic syndrome parameters. J Cosmet Dermatol. (2022) 21(11):6079–85. doi: 10.1111/jocd.15121
100. Abdulrahman BB, Elethawi AMD, Abdullah HM. Assessment of lipid profile among patients with seborrheic dermatitis. Bali Medical Journal. (2020) 9(3):952–8. doi: 10.15562/bmj.v9i3.1959
101. Tager A, Berlin C, Schen RJ. Seborrheic dermatitis in acute cardiac disease. Br J Dermatol. (1964) 76:367–9. doi: 10.1111/j.1365-2133.1964.tb14544.x
102. Li J, Feng Y, Liu C, Yang Z, de Hoog S, Qu Y, et al. Presence of malassezia hyphae is correlated with pathogenesis of seborrheic dermatitis. Microbiol Spectr. (2022) 10(1):e0116921. doi: 10.1128/spectrum.01169-21
103. Okokon EO, Verbeek JH, Ruotsalainen JH, Ojo OA, Bakhoya VN. Topical antifungals for seborrhoeic dermatitis. Cochrane Database Syst Rev. (2015):CD008138. doi: 10.1002/14651858.CD008138.pub3
104. Gaitanis G, Magiatis P, Hantschke M, Bassukas ID, Velegraki A. The Malassezia genus in skin and systemic diseases. Clin Microbiol Rev. (2012) 25(1):106–41. doi: 10.1128/CMR.00021-11
105. el-Mofty AM, Ismail AA, Abdel-Hay A, Kamel G, Nada M, Talaat M. Skin surface lipid excretion and blood lipids in seborrhoeic disorders. J Egypt Med Assoc. (1967) 50(6):375–80.4232860
106. Tamer F, Yuksel ME, Sarifakioglu E, Karabag Y. Staphylococcus aureus is the most common bacterial agent of the skin flora of patients with seborrheic dermatitis. Dermatol Pract Concept. (2018) 8(2):80–4. doi: 10.5826/dpc.0802a04
107. Ten Broeke-Smits NJ, Kummer JA, Bleys RL, Fluit AC, Boel CH. Hair follicles as a niche of Staphylococcus aureus in the nose; is a more effective decolonisation strategy needed? J Hosp Infect. (2010) 76(3):211–4. doi: 10.1016/j.jhin.2010.07.011
108. Lone AG, Atci E, Renslow R, Beyenal H, Noh S, Fransson B, et al. Colonization of epidermal tissue by Staphylococcus aureus produces localized hypoxia and stimulates secretion of antioxidant and caspase-14 proteins. Infect Immun. (2015) 83(8):3026–34. doi: 10.1128/IAI.00175-15
109. Cadieux B, Vijayakumaran V, Bernards MA, McGavin MJ, Heinrichs DE. Role of lipase from community-associated methicillin-resistant Staphylococcus aureus strain USA300 in hydrolyzing triglycerides into growth-inhibitory free fatty acids. J Bacteriol. (2014) 196(23):4044–56. doi: 10.1128/JB.02044-14
110. Nakamura K, Williams MR, Kwiecinski JM, Horswill AR, Gallo RL. Staphylococcus aureus enters hair follicles using triacylglycerol lipases preserved through the genus Staphylococcus. J Invest Dermatol. (2021) 141(8):2094–7. doi: 10.1016/j.jid.2021.02.009; Invest Dermatol. 2021, 141(8), 2094-2097. doi: 10.1016/j.jid.2021.02.009.33705795
111. Ro BI, Dawson TL. The role of sebaceous gland activity and scalp microfloral metabolism in the etiology of seborrheic dermatitis and dandruff. J Investig Dermatol Symp Proc. (2005) 10(3):194–7. doi: 10.1111/j.1087-0024.2005.10104.x
112. DeAngelis YM, Gemmer CM, Kaczvinsky JR, Kenneally DC, Schwartz JR, Dawson TL Jr. Three etiologic facets of dandruff and seborrheic dermatitis: Malassezia fungi, sebaceous lipids, and individual sensitivity. J Investig Dermatol Symp Proc. (2005) 10(3):295–7. doi: 10.1111/j.1087-0024.2005.10119.x
113. Stenz L, François P, Fischer A, Huyghe A, Tangomo M, Hernandez D, et al. Impact of oleic acid (cis-9-octadecenoic acid) on bacterial viability and biofilm production in Staphylococcus aureus. FEMS Microbiol Lett. (2008) 287(2):149–55. doi: 10.1111/j.1574-6968.2008.01316.x
114. Parsons JB, Yao J, Frank MW, Jackson P, Rock CO. Membrane disruption by antimicrobial fatty acids releases low-molecular-weight proteins from Staphylococcus aureus. J Bacteriol. (2012) 194(19):5294–304. doi: 10.1128/JB.00743-12
115. Lee JH, Kim YG, Lee J. Inhibition of Staphylococcus aureus biofilm formation and virulence factor production by petroselinic acid and other unsaturated C18 fatty acids. Microbiol Spectr. (2022) 10(3):e0133022. doi: 10.1128/spectrum.01330-22
116. Guembe MJ, Fernandez-Lazaro CI, Sayon-Orea C, Toledo E, Moreno-Iribas C; RIVANA Study Investigators. Risk for cardiovascular disease associated with metabolic syndrome and its components: a 13-year prospective study in the RIVANA cohort. Cardiovasc Diabetol. (2020) 19(1):195. doi: 10.1186/s12933-020-01166-6
117. Mathieu P, Pibarot P, Després JP. Metabolic syndrome: the danger signal in atherosclerosis. Vasc Health Risk Manag. (2006) 2(3):285–302. doi: 10.2147/vhrm.2006.2.3.285
118. Malik S, Wong ND, Franklin SS, Kamath TV, L'Italien GJ, Pio JR, et al. Impact of the metabolic syndrome on mortality from coronary heart disease, cardiovascular disease, and all causes in United States adults. Circulation. (2004) 110(10):1245–50. doi: 10.1161/01.CIR.0000140677.20606.0E
119. Tomkin GH, Owens D. The chylomicron: relationship to atherosclerosis. Int J Vasc Med. (2012) 2012:784536. doi: 10.1155/2012/784536
120. Jiang H, Zhou Y, Nabavi SM, Sahebkar A, Little PJ, Xu S, et al. Mechanisms of oxidized LDL-mediated endothelial dysfunction and its consequences for the development of atherosclerosis. Front Cardiovasc Med. (2022) 9:925923. doi: 10.3389/fcvm.2022.925923
121. Kang H, Li X, Xiong K, Song Z, Tian J, Wen Y, et al. The entry and egress of monocytes in atherosclerosis: a biochemical and biomechanical driven process. Cardiovasc Ther. (2021) 2021:6642927. doi: 10.1155/2021/6642927
122. Kaplan ZS, Jackson SP. The role of platelets in atherothrombosis. Hematology Am Soc Hematol Educ Program. (2011) 2011:51–61. doi: 10.1182/asheducation-2011.1.51
123. Huilcaman R, Venturini W, Fuenzalida L, Cayo A, Segovia R, Valenzuela C, et al. Platelets, a key cell in inflammation and atherosclerosis progression. Cells. (2022) 11(6):1014. doi: 10.3390/cells11061014
124. Huo Y, Ley KF. Role of platelets in the development of atherosclerosis. Trends Cardiovasc Med. (2004) 14(1):18–22. doi: 10.1016/j.tcm.2003.09.007
125. Yu XH, Fu YC, Zhang DW, Yin K, Tang CK. Foam cells in atherosclerosis. Clin Chim Acta. (2013) 424:245–52. doi: 10.1016/j.cca.2013.06.006
126. Gui Y, Zheng H, Cao RY. Foam cells in atherosclerosis: novel insights into its origins, consequences, and molecular mechanisms. Front Cardiovasc Med. (2022) 9:845942. doi: 10.3389/fcvm.2022.845942
127. Bentzon JF, Otsuka F, Virmani R, Falk E. Mechanisms of plaque formation and rupture. Circ Res. (2014) 114(12):1852–66. doi: 10.1161/CIRCRESAHA.114.302721
128. Palasubramaniam J, Wang X, Peter K. Myocardial infarction-from atherosclerosis to thrombosis. Arterioscler Thromb Vasc Biol. (2019) 39(8):e176–85. doi: 10.1161/ATVBAHA.119.312578
129. Mushenkova NV, Bezsonov EE, Orekhova VA, Popkova TV, Starodubova AV, Orekhov AN. Recognition of oxidized lipids by macrophages and its role in atherosclerosis development. Biomedicines. (2021) 9(8):915. doi: 10.3390/biomedicines9080915
130. Quinn MT, Parthasarathy S, Fong LG, Steinberg D. Oxidatively modified low density lipoproteins: a potential role in recruitment and retention of monocyte/macrophages during atherogenesis. Proc Natl Acad Sci U S A. (1987) 84(9):2995–8. doi: 10.1073/pnas.84.9.2995
131. Jessup W, Kritharides L, Stocker R. Lipid oxidation in atherogenesis: an overview. Biochem Soc Trans. (2004) 32(Pt 1):134–8. doi: 10.1042/bst0320134
132. Herb M, Schramm M. Functions of ROS in macrophages and antimicrobial immunity. Antioxidants. (2021) 10(2):313. doi: 10.3390/antiox10020313
133. Pidwill GR, Gibson JF, Cole J, Renshaw SA, Foster SJ. The role of macrophages in Staphylococcus aureus infection. Front Immunol. (2021) 11:620339. doi: 10.3389/fimmu.2020.620339
134. Delekta PC, Shook JC, Lydic TA, Mulks MH, Hammer ND. Staphylococcus aureus utilizes host-derived lipoprotein particles as sources of fatty acids. J Bacteriol. (2018) 200(11):e00728–17. doi: 10.1128/JB.00728-17
135. Wang B, Muir TW. Regulation of virulence in Staphylococcus aureus: molecular mechanisms and remaining puzzles. Cell Chem Biol. (2016) 23(2):214–24. doi: 10.1016/j.chembiol.2016.01.004
136. Kong C, Neoh HM, Nathan S. Targeting Staphylococcus aureus toxins: a potential form of anti-virulence therapy. Toxins. (2016) 8(3):72. doi: 10.3390/toxins8030072
137. Shapiro MD, Fazio S. Apolipoprotein B-containing lipoproteins and atherosclerotic cardiovascular disease. F1000Res. (2017) 6:134. doi: 10.12688/f1000research.9845.1
138. Elmore BO, Triplett KD, Hall PR. Apolipoprotein B48, the structural component of chylomicrons, is sufficient to antagonize Staphylococcus aureus quorum-sensing. PLoS One. (2015) 10(5):e0125027. doi: 10.1371/journal.pone.0125027
139. Hall PR, Elmore BO, Spang CH, Alexander SM, Manifold-Wheeler BC, Castleman MJ, et al. Nox2 modification of LDL is essential for optimal apolipoprotein B-mediated control of agr type III Staphylococcus aureus quorum-sensing. PLoS Pathog. (2013) 9(2):e1003166. doi: 10.1371/journal.ppat.1003166
140. Eid SA, Rumora AE, Beirowski B, Bennett DL, Hur J, Savelieff MG, et al. New perspectives in diabetic neuropathy. Neuron. (2023) 111(17):2623–41. doi: 10.1016/j.neuron.2023.05.003
141. Kazamel M, Stino AM, Smith AG. Metabolic syndrome and peripheral neuropathy. Muscle Nerve. (2021) 63(3):285–93. doi: 10.1002/mus.27086
142. Citron KM, Knox R. The uptake of thiamine by a strain of Staphylococcus aureus from the duodenum of a case of polyneuritis. J Gen Microbiol. (1954) 10(3):482–90. doi: 10.1099/00221287-10-3-482
143. Nisar S, Yousuf Wani I, Altaf U, Muzaffer U, Kareem O, Tanvir M, et al. Thiamine deficiency-related neuropathy: a reversible entity from an endemic area. Eur J Neurol. (2024) 31(3):e16155. doi: 10.1111/ene.16155
144. Smith TJ, Johnson CR, Koshy R, Hess SY, Qureshi UA, Mynak ML, et al. Thiamine deficiency disorders: a clinical perspective. Ann N Y Acad Sci. (2021) 1498(1):9–28. doi: 10.1111/nyas.14536
145. Marcé-Grau A, Martí-Sánchez L, Baide-Mairena H, Ortigoza-Escobar JD, Pérez-Dueñas B. Genetic defects of thiamine transport and metabolism: a review of clinical phenotypes, genetics, and functional studies. J Inherit Metab Dis. (2019) 42(4):581–97. doi: 10.1002/jimd.12125
146. Nguyen HD, Oh H, Kim MS. An increased intake of thiamine diminishes the risk of metabolic syndrome in the Korean population with various comorbidities. Diabetes Metab Syndr. (2022) 16(3):102443. doi: 10.1016/j.dsx.2022.102443
147. Ziegler D, Reiners K, Strom A, Obeid R. Association between diabetes and thiamine status - a systematic review and meta-analysis. Metab Clin Exp. (2023) 144:155565. doi: 10.1016/j.metabol.2023.155565
148. Beltramo E, Berrone E, Tarallo S, Porta M. Effects of thiamine and benfotiamine on intracellular glucose metabolism and relevance in the prevention of diabetic complications. Acta Diabetol. (2008) 45(3):131–41. doi: 10.1007/s00592-008-0042-y
149. Beltramo E, Mazzeo A, Porta M. Thiamine and diabetes: back to the future? Acta Diabetol. (2021) 58(11):1433–9. doi: 10.1007/s00592-021-01752-4
150. Chen W, Pang Y. Metabolic syndrome and PCOS: pathogenesis and the role of metabolites. Metabolites. (2021) 11(12):869. doi: 10.3390/metabo11120869
151. Ezkurdia A, Ramírez MJ, Solas M. Metabolic syndrome as a risk factor for Alzheimer’s disease: a focus on insulin resistance. Int J Mol Sci. (2023) 24(5):4354. doi: 10.3390/ijms24054354
152. Khatiwada S, Sah SK, Kc R, Baral N, Lamsal M. Thyroid dysfunction in metabolic syndrome patients and its relationship with components of metabolic syndrome. Clin Diabetes Endocrinol. (2016) 2:3. doi: 10.1186/s40842-016-0021-0
153. Nam GE, Kim SM, Han K, Kim NH, Chung HS, Kim JW, et al. Metabolic syndrome and risk of Parkinson disease: a nationwide cohort study. PLoS Med. (2018) 15(8):e1002640. doi: 10.1371/journal.pmed.1002640
154. Esposito K, Chiodini P, Colao A, Lenzi A, Giugliano D. Metabolic syndrome and risk of cancer: a systematic review and meta-analysis. Diabetes Care. (2012) 35(11):2402–11. doi: 10.2337/dc12-0336
155. Brauer HA, D'Arcy M, Libby TE, Thompson HJ, Yasui YY, Hamajima N, et al. Dermcidin expression is associated with disease progression and survival among breast cancer patients. Breast Cancer Res Treat. (2014) 144(2):299–306. doi: 10.1007/s10549-014-2880-3
156. Qiu F, Qiu F, Liu L, Liu J, Xu J, Huang X. The role of dermcidin in the diagnosis and staging of hepatocellular carcinoma. Genet Test Mol Biomarkers. (2018) 22(4):218–23. doi: 10.1089/gtmb.2017.0230
157. Chang WC, Huang MS, Yang CJ, Wang WY, Lai TC, Hsiao M, et al. Dermcidin identification from exhaled air for lung cancer diagnosis. Eur Respir J. (2010) 35(5):1182–5. doi: 10.1183/09031936.00169509
158. Zhang J, Ding W, Kuai X, Ji Y, Zhu Z, Mao Z, et al. Dermcidin as a novel binding protein of lncRNA STCAT3 and its effect on prognosis in gastric cancer. Oncol Rep. (2018) 40(5):2854–63. doi: 10.3892/or.2018.6673
159. Rizzi F, Bettuzzi S. The clusterin paradigm in prostate and breast carcinogenesis. Endocr Relat Cancer. (2010) 17(1):R1–17. doi: 10.1677/ERC-09-0140
160. Fu Y, Du Q, Cui T, Lu Y, Niu G. A pan-cancer analysis reveals role of clusterin (CLU) in carcinogenesis and prognosis of human tumors. Front Genet. (2023) 13:1056184. doi: 10.3389/fgene.2022.1056184
161. Andersen CL, Schepeler T, Thorsen K, Birkenkamp-Demtröder K, Mansilla F, Aaltonen LA, et al. Clusterin expression in normal mucosa and colorectal cancer. Mol Cell Proteomics. (2007) 6(6):1039–48. doi: 10.1074/mcp.M600261-MCP200
162. Peng M, Deng J, Zhou S, Tao T, Su Q, Yang X, et al. The role of clusterin in cancer metastasis. Cancer Manag Res. (2019) 11:2405–14. doi: 10.2147/CMAR.S196273
163. Qiu F, Long H, Zhang L, Liu J, Yang Z, Huang X. Dermcidin enhances the migration, invasion, and metastasis of hepatocellular carcinoma cells in vitro and in vivo. J Clin Transl Hepatol. (2022) 10(3):429–38. doi: 10.14218/JCTH.2021.00108
164. Humphreys DT, Carver JA, Easterbrook-Smith SB, Wilson MR. Clusterin has chaperone-like activity similar to that of small heat shock proteins. J Biol Chem. (1999) 274(11):6875–81. doi: 10.1074/jbc.274.11.6875
165. Poon S, Easterbrook-Smith SB, Rybchyn MS, Carver JA, Wilson MR. Clusterin is an ATP-independent chaperone with very broad substrate specificity that stabilizes stressed proteins in a folding-competent state. Biochemistry. (2000) 39(51):15953–60. doi: 10.1021/bi002189x; Biochemistry. 2000, 39(51), 15953-15960. doi: 10.1021/bi002189x.11123922
166. Park S, Mathis KW, Lee IK. The physiological roles of apolipoprotein J/clusterin in metabolic and cardiovascular diseases. Rev Endocr Metab Disord. (2014) 15(1):45–53. doi: 10.1007/s11154-013-9275-3
167. Rodríguez-Rivera C, Garcia MM, Molina-Álvarez M, González-Martín C, Goicoechea C. Clusterin: always protecting. Synthesis, function and potential issues. Biomed Pharmacother. (2021) 134:111174. doi: 10.1016/j.biopha.2020.111174
168. Poon S, Treweek TM, Wilson MR, Easterbrook-Smith SB, Carver JA. Clusterin is an extracellular chaperone that specifically interacts with slowly aggregating proteins on their off-folding pathway. FEBS Lett. (2002) 513(2-3):259–66. doi: 10.1016/s0014-5793(02)02326-8
169. Tentolouris N, Argyrakopoulou G, Katsilambros N. Perturbed autonomic nervous system function in metabolic syndrome. Neuromolecular Med. (2008) 10(3):169–78. doi: 10.1007/s12017-008-8022-5
170. Stino AM, Smith AG. Peripheral neuropathy in prediabetes and the metabolic syndrome. J Diabetes Investig. (2017) 8(5):646–55. doi: 10.1111/jdi.12650
171. Iwase T, Uehara Y, Shinji H, Tajima A, Seo H, Takada K, et al. Staphylococcus epidermidis esp inhibits Staphylococcus aureus biofilm formation and nasal colonization. Nature. (2010) 465(7296):346–9. doi: 10.1038/nature09074
172. Nakatsuji T, Chen TH, Narala S, Chun KA, Two AM, Yun T, et al. Antimicrobials from human skin commensal bacteria protect against Staphylococcus aureus and are deficient in atopic dermatitis. Sci Transl Med. (2017) 9(378):eaah4680. doi: 10.1126/scitranslmed.aah4680
173. Piewngam P, Khongthong S, Roekngam N, Theapparat Y, Sunpaweravong S, Faroongsarng D, et al. Probiotic for pathogen-specific Staphylococcus aureus decolonisation in Thailand: a phase 2, double-blind, randomised, placebo-controlled trial. Lancet Microbe. (2023) 4(2):e75–83. doi: 10.1016/S2666-5247(22)00322-6
174. Gonzalez DJ, Haste NM, Hollands A, Fleming TC, Hamby M, Pogliano K, et al. Microbial competition between Bacillus subtilis and Staphylococcus aureus monitored by imaging mass spectrometry. Microbiology. (2011) 157(Pt 9):2485–92. doi: 10.1099/mic.0.048736-0
175. Bejaoui S, Poulsen M. The impact of early life antibiotic use on atopic and metabolic disorders: meta-analyses of recent insights. Evol Med Public Health. (2020) 2020(1):279–89. doi: 10.1093/emph/eoaa039
176. Park SY, Ustulin M, Park S, Han KD, Kim JY, Shin DW, et al. Systemic antibiotics and obesity: analyses from a population-based cohort. J Clin Med. (2021) 10(12):2601. doi: 10.3390/jcm10122601
177. Haag AF, Fitzgerald JR, Penadés JR. Staphylococcus aureus in animals. Microbiol Spectr. (2019) 7(3). doi: 10.1128/microbiolspec.GPP3-0060-2019
178. Notarbartolo V, Giuffrè M, Montante C, Corsello G, Carta M. Composition of human breast milk microbiota and its role in children’s health. Pediatr Gastroenterol Hepatol Nutr. (2022) 25(3):194–210. doi: 10.5223/pghn.2022.25.3.194
Keywords: the Acari Hypothesis, eccrine glands, Malassezia, Staphylococcus aureus, metabolic syndrome, obesity, dyslipidemia, insulin resistance
Citation: Retzinger AC and Retzinger GS (2025) The Acari Hypothesis, VII: accounting for the comorbidity of allergy with other contemporary medical conditions, especially metabolic syndrome. Front. Allergy 6:1537467. doi: 10.3389/falgy.2025.1537467
Received: 30 November 2024; Accepted: 17 March 2025;
Published: 27 March 2025.
Edited by:
Elham Hossny, Ain Shams University, EgyptReviewed by:
Leticia Gomes De Pontes, Universidade de Minas Gerais, BrazilCopyright: © 2025 Retzinger and Retzinger. This is an open-access article distributed under the terms of the Creative Commons Attribution License (CC BY). The use, distribution or reproduction in other forums is permitted, provided the original author(s) and the copyright owner(s) are credited and that the original publication in this journal is cited, in accordance with accepted academic practice. No use, distribution or reproduction is permitted which does not comply with these terms.
*Correspondence: Andrew C. Retzinger, YW5kcmV3LnJldHppbmdlckBnbWFpbC5jb20=
Disclaimer: All claims expressed in this article are solely those of the authors and do not necessarily represent those of their affiliated organizations, or those of the publisher, the editors and the reviewers. Any product that may be evaluated in this article or claim that may be made by its manufacturer is not guaranteed or endorsed by the publisher.
Research integrity at Frontiers
Learn more about the work of our research integrity team to safeguard the quality of each article we publish.