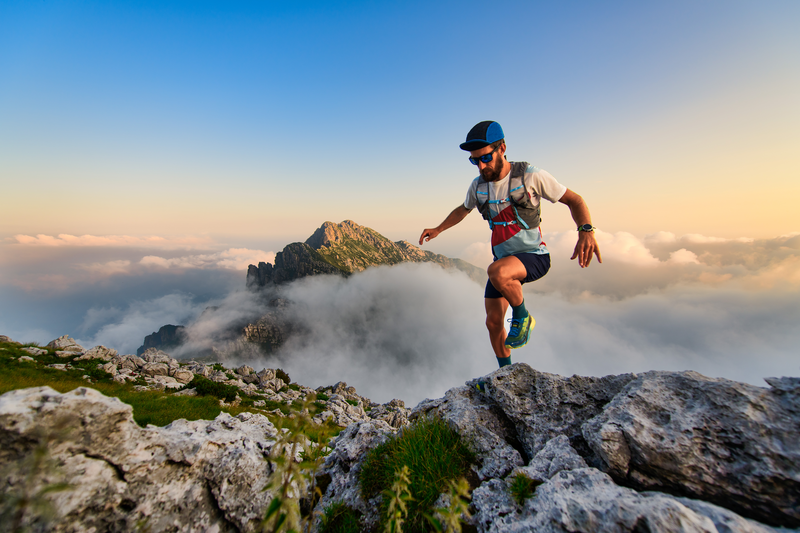
94% of researchers rate our articles as excellent or good
Learn more about the work of our research integrity team to safeguard the quality of each article we publish.
Find out more
MINI REVIEW article
Front. Allergy , 12 March 2024
Sec. Rhinology
Volume 5 - 2024 | https://doi.org/10.3389/falgy.2024.1374771
This article is part of the Research Topic Rhinitis and pollution View all 4 articles
Combined exposures to allergens and air pollutants emerged as a topic of concern in scientific circles by the 1980's, when it became clear that parallel increases in respiratory allergies and traffic-related air pollution had been occurring during the 20th century. Although historically there has been a tendency to treat exposure-related symptoms as either allergic or toxicologic in nature, cross-interactions have since been established between the two modalities. For example, exposure to selected air pollutants in concert with a given allergen can increase the likelihood that an individual will become sensitized to that allergen, strongly suggesting that the pollutant acted as an adjuvant. Although not a review of underlying mechanisms, the purpose of this mini-review is to highlight the potential significance of co-exposure to adjuvant chemicals in predicting allergic sensitization in the respiratory tract. The current discussion emphasizes the upper airway as a model for respiratory challenge studies, the results of which may be applicable—not only to allergic rhinitis—but also to conjunctivitis and asthma.
Allergy denotes an acquired (and maladaptive) physical reaction to an otherwise innocuous foreign substance. It occurs in two phases—sensitization and challenge (i.e., “triggering”). An acutely triggered response to an allergen to which an individual is already sensitized is referred to as an allergic reaction, with the terms “allergy” and “allergen” normally being reserved for [Gell-Coombs] Type I (“immediate”) hypersensitivity. A hallmark of allergic sensitization is the presence of allergen-specific IgE in tissues, secretions, or in the circulation (1, 2). Toxicologic irritation (e.g., of the mucous membranes or airways), on the other hand, refers to the nociceptive and physiologic response to noxious chemicals, regardless of prior exposure history (3). While some primary sensations (symptoms such as itching) are more common with allergy than irritation, most secondary (“reflex”) symptoms—including nasal congestion, rhinorrhea, chest tightness, cough and wheezing—can occur with either phenomenon alone. When evaluating an individual case (or an aggregate population), the clinician (or epidemiologist) aiming to attribute symptoms to a specific mechanism may—however inadvertently—overlook interactive effects (4). In fact, the underlying mechanisms of allergy and toxicology can—and do—affect one another. Toxicologic potentiation of allergy is the topic of this brief review.
The term “air pollutant” refers to a range of potentially deleterious chemical substances found in outdoor, residential, or work atmospheres due to anthropogenic or natural emissions. Anthropogenic outdoor sources are conventionally classified as “stationary” (e.g., power plants or smelters), or “mobile” (motor vehicles), with vehicular emissions being a major concern. “Criteria Air Pollutants” (NOx, SOx, O3, CO, Pb, and particulate matter) are mandated for regulation as Ambient Air Quality Standards (AAQS) in the US (https://www.epa.gov/criteria-air-pollutants), leading to the establishment of ambient air monitoring networks in the US and elsewhere. Both ambient and indoor air may also include so-called “Toxic Air Contaminants” (or “TACs”), as defined in state and local standards (https://ww2.arb.ca.gov/resources/documents/carb-identified-toxic-air-contaminants). TACs include potential carcinogens, reproductive, or organ system toxicants. Indoor atmospheres can be influenced by both outdoor air and indoor sources [e.g., combustion appliances or (formaldehyde-containing) building materials]. Workplace air may contain any or all of the above substances, plus a wide range of industrial chemicals which may or may not have applicable workplace airborne exposure standards (see: https://www.osha.gov/annotated-pels and https://series.publisso.de/pgseries/overview/mak). Combustion processes (e.g., tobacco or cannabis smoking and various types of fires) can produce complex chemical mixtures. Despite this multiplicity of potential chemical exposures, data regarding interaction with allergens– which we summarize here—are available for only a small subset of chemicals.
In vaccine biology, the term “adjuvant” refers to a chemical added to a vaccine to increase its immunogenicity (hence, its effectiveness). Since the mid-1980's the phenomenon of adjuvancy has also been recognized in air pollution toxicology (in this case, chemical potentiation of allergen sensitization) (Figure 1). Air contaminants containing polycyclic aromatic hydrocarbons (PAHs, products of incomplete combustion of carbonaceous materials)—initially diesel exhaust particles (DEP)—were the first to be studied for their apparent adjuvant activity. In fact, the earliest published in vivo studies (in laboratory animals) came from Japan, where rapid industrialization and the proliferation of diesel-powered vehicles in the mid-20th century coincided with a significant uptick in observed respiratory allergies to aeroallergens—particularly to Japanese Cedar (6). Subsequent studies have built on this foundation.
Figure 1. Potential mechanisms by which air pollutants may affect the response to airborne allergens. Air pollutants can interact physically and/or chemically with allergen particles, alter allergen penetration through the mucosa, interact with immune processes that elaborate allergen-specific immunoglobulin E (IgE), and/or carry out downstream effects of allergic triggering. PM, particulate matter; O3, NOx, ozone and nitrogen oxides; PALMs, pollen-associated lipid mediators; DAMPs, damage-associated molecular patterns; PRR, pattern recognition receptors; Th2, type 2 T helper cells (5).
Beginning this area of experimental study, Muranaka and colleagues examined the effect of diesel exhaust particles (DEP) on immunologic sensitization to an allergen in female BDF1 mice. Using both ovalbumin (OVA) and Japanese Cedar Pollen as allergens of interest, aluminum hydroxide—Al (OH)3—as the positive control adjuvant, and intraperitoneal injection (IP) as the exposure system, the group showed that DEP was effective in boosting the specific IgE antibody response to allergen (7). The same group subsequently published a follow-up study showing an adjuvant effect of DEP on OVA sensitization when the two were co-presented to mice via the intranasal route (8).
Inspired by the above work, air pollutant adjuvants began to be examined in vivo using human volunteers by researchers in several countries. At the University of California (Los Angeles), Diaz-Sanchez and colleagues initially studied a mixed group of non-atopics and seasonal allergic rhinitics (studied outside of their relevant pollen season). They first found that direct application of DEP alone to the nasal mucosa elicited an increase in total IgE-secreting cells in nasal lavage (NL) fluid (9). Studying a similar volunteer group, the investigators later found that nasal DEP administration alone enhanced pro-inflammatory cytokine expression in NL fluid (10). The same group subsequently documented both DEP-induced augmentation of ragweed-specific IgE after allergen challenge of sensitized individuals (11) and IgE isotype-switching in vivo (12).
Against this backdrop, a major breakthrough was achieved in 1999 by researchers pairing DEP exposure with exposure to an antigenic substance to which casual contact is unlikely in the general population—keyhole limpet hemocyanin or “KLH”—a substance found in the endolymph of a marine mollusk (13). Using KLH as the allergen, researchers studied seasonal allergic rhinitics by applying the substance topically in the nose with and without DEP pre-treatment (14). Among the 10 subjects exposed to the allergen alone, none had detectable anti-KLH IgE in NL fluid post-exposure. Among the subjects treated with both KLH and DEP, on the other hand, 9 of 15 showed detectable levels of KLH-specific IgE (14). Following this dramatic finding, respiratory adjuvant studies gained further momentum. Investigators in Japan, for example, using cultured human nasal epithelial cells, documented that DEP alone (unpaired with allergen) upregulates messenger RNA for histamine receptors, as well as increasing histamine-induced IL-8 production (15). Back at the University of California, the Diaz-Sanchez group studied basophils from allergic rhinitic and control subjects in vitro, showing that DEPs alone (without allergen) could release IL-4 and histamine from these cells regardless of the allergy status of their donors (16).
At this point another research thread developed, regarding the constituents of DEP (itself a complex mixture of ingredients). In 2000, the Diaz-Sanchez group pre-treated dust mite (Der p 1)—sensitive allergic rhinitics with either DEP or carbon black (pure carbon particles) and found that DEP—but not carbon black—augmented their symptoms, lowered their threshold for response, and increased IL-4 and histamine after allergen triggering (17). In 2002, researchers at the Pasteur Institute in Paris compared the adjuvant effect of traffic particulate matter (TPM) with that of pure carbon core particles, and found that only TPM boosted levels of birch pollen-specific IgE and eosinophils (18). The following year researchers in the Netherlands published a wide-ranging study in which both mice and rats were sensitized (to timothy grass and OVA, respectively), and five different particulate matter sources were used. The mouse / OVA system had a lower threshold for response and was able the rank the different PM sources by potency (19). In 2007 group in Germany incubated basophils from birch pollen-allergic donors in the presence of two different PAH compounds—benzo[a]pyrene (BaP) and phenanthrene (Phe)—then stimulated the cells with birch allergen (rBet v 1). They found that combining either of these DEP constituents with allergen significantly increased basophil activation over that of allergen alone (20).
Coincident with the above work, a parallel line of inquiry emerged concerning a different product of incomplete combustion—so-called “second-hand smoke” (“SHS”). Like DEP, SHS contains PAHs (i.e., known adjuvants). A link between childhood SHS exposure and persistent wheeze / asthma had been documented by the late 1980's, but differentiating between irritant and adjuvant effects remained ambiguous (21–23). It was only once biomarkers of both SHS exposure (cotinine) and allergic sensitization (specific IgE) were asayed from the same individuals that the likely role of SHS as an adjuvant was established (24, 25).
In 1997, Seymour and colleagues, from the University of California (Davis), sensitized mice to OVA by intraperitoneal injection (with and without pre-exposure to SHS), with subsequent OVA aerosol exposure. The mice who were pre-exposed to SHS displayed an augmented biochemical response to allergen provocation, including higher total IgE and OVA-specific IgG1 (26). In 2001, SHS was employed experimentally by the UCLA group to exhibit an OVA-specific IgE adjuvant effect via airborne exposure—along with OVA—in two strains of mice (27), a finding replicated in Belgium some 5 years later (28). 2006 would also see the publication of the first human study involving combined SHS + allergen exposures. Diaz-Sanchez and colleagues nasally challenged 19 nonsmoking, ragweed-sensitized allergic rhinitics with either ragweed pollen extract (containing Amb a 1) or placebo. These challenges occurred immediately after the subjects had spent 2 h in a chamber with either clean air (mean particulate concentration 46 μg/m3) or SHS (mean particulate concentration, 310 μg/m3). Significantly, the combined SHS-allergen exposure yielded a ∼16-fold greater increase in ragweed-specific IgE in NL fluid (compared to the clean air-allergen exposure) when subjects were sampled 4 days later. In addition, IL-4, IL-5 and IL-13 (all Th2-cytokines) and histamine were increased after SHS-allergen exposure, and IFN-γ (a Th1 cytokine) was suppressed (29).
In 2008 Samuelsen et al. in Norway, combining subdermal injection of OVA in Balb/cA mice with selected particles, compared the IgE-related adjuvant activity of particles from woodsmoke and road traffic with that of DEP. They found that woodsmoke and road traffic particles were roughly equipotent, although still less effective than DEP (30). A 2009 mouse study conducted at UCLA revealed that ultrafine PM (<0.15 μm) was a more potent adjuvant than was fine PM (<2.5 μm) (31). More recently, Castaneda and colleagues (2018) studied the adjuvant properties of atmospheric fine particulate matter (<2.5 μm) collected near a major highway in an asthma-prevalent area of California, finding that pairing house dust mite (HDM) inoculation with PM in a murine model substantially enhanced both pulmonary IgE elaboration and inflammation relative to HDM exposure alone (32). Most recently in Shanghai, a 2023 study characterized pulmonary lymph nodes in mice sensitized to HDM found that co-exposure to PM2.5 increased both IgE production and associated cellular inflammation (33).
As noted above in the discussion of DEP adjuvancy, de novo sensitization of atopic human subjects to KLH in the presence of DEP was not a universal outcome, given that 6 of 15 subjects receiving both allergen and adjuvant failed to show sensitization. The UCLA group revisited this anomaly initially in 2003 by comparing 18 ragweed-allergic volunteer subjects' responses to either DEP + Amb a 1 or Placebo + Amb a 1 in a randomized, counter-balanced, replicate provocation study. Examining [allergen-specific] IgE, IL-4 and IFN-γ in NL fluid, they found that the net response to allergen challenge was both highly variable between—and highly reproducible within—subjects. They concluded that susceptibility to the adjuvant effect of DEP was and “intrinsic trait” rather than a random phenomenon (34).
The following year the same group followed-up with another comparison of DEP vs. Placebo pre-treated [ragweed-sensitized] subjects, adding both histamine as an analyte and an assay for possible variants in their glutathione-S-transferase enzymes (i.e, GSTM1, GSTP1, and GSTT1 genotypes). They found that subjects that were GSTM1 null, as well as those homozygous for the GSTP1 I105 (wildtype) genotype, displayed a more robust IgE response (and greater histamine production) than their genetic counterparts. The authors concluded that the inter-individual differences previously documented in DEP adjuvant studies were—in large part—mediated by differences in enzymatic reduction of “reactive oxygen species,” with the more effective glutathione-S-transferase genotypes conferring a protective effect against DEP (35). Similar results were obtained when SHS was substituted for DEP in an equivalent study (36).
Successful interruption of DEP-mediated adjuvancy via administration of exogenous antioxidants has also lent credibility to the “oxidative stress” model of adjuvant action. Whitekus et al. screened six candidate antioxidants—two botanicals (silibinin and luteolin), two vitamins (C and E), and two sulfur-donors (Bucillamine [or BUC] and N-acetylcystine [or NAC])—for their ability to interrupt DEP-induced adjuvancy when co-administered with OVA (37). The last two (both sulfur-donors) were highly efficacious in that role. As an alternative to exogenous supplementation, induction of phase II metabolic enzymes has since been shown to exert an equivalent antioxidant role. For example, in 2007, Ritz et al. added sulforaphane (a natural compound derived from cruciferous vegetables) to cultured bronchial epithelial cells and observed inhibition of DEP-stimulated production of the proinflammatory cytokines IL-8, GMCSF, and IL-1β (38). In vivo, this effect was later replicated in a nutrition study in which broccoli sprout extract was administered to human subjects in mango juice on a daily basis (39).
In addition to PAH-containing combustion products (DEP, SHS and woodsmoke), other commonly encountered chemical compounds have been assayed with respect to their potential role as adjuvants. These include formaldehyde (a common indoor air pollutant), phthalates (found in plastics), the industrial chemicals styrene, chloroform, and 1,1-dichloroethylene, and the reaction products of ozone and limonene (an unsaturated terpene used in some cleaning products).
Studies of the potential effect of formaldehyde (CH2O) vapor on allergic sensitization date back at least to the mid-1990s. Riedel and colleagues pre-exposed Dunkin-Hartley guinea pigs to clean air, 0.13 ppm, or 0.25 ppm CH2O vapor on a daily basis for 5 days, followed by aerosolized OVA after pre-exposure and 2 weeks later. After another week's time the animals underwent venipuncture and bronchoprovocation with OVA. In the 0.25 ppm pre-exposed group 10/12 animals (vs. 3/12 in the control group) exhibited a positive provocation reaction to OVA. The median OVA-specific IgG1 level was also elevated in the 0.25 ppm group compared to the controls (specific IgE was, however, not assayed). The 0.13 ppm exposure group showed intermediate reactivity to OA on pulmonary function and also an intermediate distribution of IgG1 antibody titers (40).
Two additional studies on formaldehyde were published during the period 2009–2011 using CH2O exposures comparing two different Chinese workplace exposure standards (which had recently been reduced∼6-fold from 2,500 to 400 ppb). Qiao and colleagues found that CH2O at the higher concentration increased bronchial reactivity in rats, both alone (a possible irritant effect) and even more so with OVA sensitization (i.e., as an adjavant) (41). Similarly, Liu et al. found that co-exposure of mice to CH2O at the higher standard in concert with OVA sensitization greatly amplified bronchial reactivity vs. clean air or CH2O at the lower standard (42).
Similar to DEP, potential exposures to phthalate plasticizers increased dramatically in the 2nd half of the 20th century, with increased use of plastics in consumer products coinciding with the observed increase in the prevalence of airway allergies. As a result, this class of chemicals was also suspected to be an adjuvant for allergic sensitization. Since most phthalates have very limited volatility, the majority of the early phthalate assays involved either parenteral administration or gavage. Of these studies, some did indicate that phthalates could facilitate allergic sensitization (including elaboration of allergen-specific IgE) (43, 44). In a later set of studies, phthalates were co-administered with a test allergen (OVA) via the inhalation route. Two such studies looked at di-ethylhexyl phthalate (DEHP) and its chief metabolite, mon-ethylhexyl phthalate (MEHP), utilizing OVA as the allergen. Both phthalates boosted allergen-specific IgG1, but neither significantly affected allergen-specific IgE levels (45, 46).
Ban and colleagues studied the effect of co-exposure to three commonly used industrial chemicals:—1,1-dichloroethylene (DCE), chloroform (CF), and styrene (S)—during OVA sensitization in mice. The endpoints studied were all biochemical in nature, including OVA-specific IgE in blood, and cytokine production in lung-draining lymph nodes. The authors found that of the three, only chloroform and styrene co-exposures reliably potentiated the generation of allergen-specific IgE. Further, of these two, only styrene produced an expected boost in Th2 cytokine production (47).
Hansen et al. compared OVA-specific Ab's in BALB/cJ mice exposed to OVA alone (negative control), OVA with Al(OH)3 (positive control), OVA with O3, OVA with limonene (a cyclic terpene), and OVA with reaction products of O3 + limonene. Besides the positive control, only the combination of OVA with reaction products of O3 + limonene boosted allergen-specific IgE compared with the negative control. Of note, the authors stopped short of labeling this an “adjuvant effect,” since airway inflammation was not evident when bronchoalveolar lavage fluid was obtained from the animals' lungs ex vivo (48) Figure 2.
Figure 2. Activation (“triggering”) of a sensitized mast cell in the nasal mucosa, a prerequisite for which is the presence of allergen-specific IgE. Two reaction kinetics are delineated, “early” (minutes) and “late” (>4–6 h), with distinct biological markers associated with each. Differences in cellular / biochemical outcomes between otherwise similar studies may result from sampling time differences (49).
Over the last three decades, considerable attention has been devoted to studying the effects of air pollutants on respiratory allergies (particularly adjuvant effects). Candidate adjuvants have mostly been identified based on epidemiologic correlations. Specifically, in the 20th century an increasing prevalence of respiratory allergies was observed coincident with increases in: (1) traffic-related air pollutants (e.g., DEP), (2) exposure to “second-hand” tobacco smoke, (3) use of formaldehyde-releasing resins in building materials, and (4) use of phthalates in consumer products. For the first three examples (DEP, SHS and CH2O), combined inhalation exposures confirmed adjuvancy. For the fourth, effective adjuvant doses would require ingestion of the phthalate in concert with allergen inhalation.
Approaches to studying adjuvant effects have included in vitro (cell culture) and in vivo (animal and human) studies. The most impressive of these featured atopic human volunteers who were successfully sensitized to what at the time was termed a “neoallergen” (KLH). At the opposite end of the spectrum, a number of ostensible “adjuvant” studies have dealt with biochemical endpoints without allergen-specific IgE determination. These diverse results can be challenging to fit into a single mechanistic model, and after more than three decades of study, we are still struggling to put the “puzzle pieces” together.
In terms of completed studies, some methodologic issues are readily apparent (albeit not conspicuously highlighted in the published literature). Foremost among these is the fact that a subset of authors hold that—in addition to a candidate adjuvant chemical needing to facilitate formation of allergen-specific IgE—it would also need to produce elevated biochemical markers of tissue inflammation post-triggering. Since this criterion is inconsistently applied, establishing guidelines regarding this requirement would hopefully reduce the level of ambiguity in interpreting future study findings.
Another potential contemporaneous theme in adjuvant research would take its inspiration from early work in which the effects of DEP (vs. pure black carbon particles) were compared, finding that adjuvancy (increased elaboration of anti-OVA IgE + IgG) was confined to the former (17). Surprisingly, Granum and colleagues subsequently demonstrated an adjuvant effect when pairing allergen exposures with other pure “model” particles (composed of a variety of materials including polystyrene, amorphous silica, titanium dioxide, and tetrafluoroethylene or Teflon). Granum also highlighted the fact that “ROFA” (residual oil fly ash), which is produced under similar conditions as DEP, only exhibits adjuvancy when selected metals (i.e., nickel or vanadium—but not iron) are adsorbed thereto (50). Implicit in these findings is the fact that—mechanistically—the physical chemistry of a candidate adjuvant must be taken into account and reconciled with the prevailing model emphasizing oxidative stress as a common factor.
Numerous review articles deal with pollutant adjuvants, although in most cases in a wider context of chemically induced inflammation (5, 49, 51–55). Research into pollutant adjuvant effects would benefit from a more stringent definition of the phenomenon, as well as clearer boundaries with respect to related—but distinct—studies of xenobiotic-induced inflammation. Once the term “adjuvant” stands out as a distinct entity with well-defined borders, further progress will likely follow.
DS: Conceptualization, Visualization, Writing – original draft, Writing – review & editing.
The author declare that no financial support was received for the research, authorship, and/or publication of this article.
The author wishes to acknowledge feedback received from Kent Pinkerton on an initial draft of this manuscript.
The author declares that the research was conducted in the absence of any commercial or financial relationships that could be construed as a potential conflict of interest.
All claims expressed in this article are solely those of the authors and do not necessarily represent those of their affiliated organizations, or those of the publisher, the editors and the reviewers. Any product that may be evaluated in this article, or claim that may be made by its manufacturer, is not guaranteed or endorsed by the publisher.
1. Burks AW, Holgate ST, O'Hehir RE, Broide DH, Bacharier LB, Khurana Hershey GK, et al. Middleton’s allergy: Principles and practice (9th edition). NY: Elsevier (2020).
2. Wise SK, Lin SY, Taskala E, Orlandi RR, Akdis C, Alt JA, et al. International consensus statement on allergy and rhinology: allergic rhinitis. Int Forum Allergy Rhinol. (2018) 8(2):108–352. doi: 10.1002/alr.22073
3. Aleksumes LM, Eaton DL. Principles of toxicology. In: Klassen CD, editors. Casarett and Doull’s Toxicology. 9th edition, NY: McGraw Hill (2019). p. 25–64.
4. Von Mutius E. The rising trends in asthma and allergic disease. Clin Exp Allergy. (1998) 28(Suppl 5):45–9. doi: 10.1046/j.1365-2222.1998.028s5045.x
5. Reinmuth-Selzle K, Kampf CJ, Lucas K, Lang-Yona N, Fröhlich-Nowoisky J, Shiraiwa M, et al. Air pollution and climate change effects on allergies in the anthropocene: abundance, interaction, and modification of allergens and adjuvants. Environ Sci Technol. (2017) 51(8):4119–41. doi: 10.1021/acs.est.6b04908
6. Kaneko S, Shimada K, Horucki H. Nasal allergy and air pollution. Oto-Rhino_Laryngology Tokyo. (1980) 23(suppl 4):270.
7. Muranaka M, Suzuki S, Koizumi K, Takafuji S, Miyamoto T, Ikemori R, et al. Adjuvant activity of diesel-exhaust particulates for the production of IgE antibody in mice. J Allergy Clin Immunol. (1986) 77(4):616–23. doi: 10.1016/0091-6749(86)90355-6
8. Takafuji S, Suzuki S, Koizumi K, Tadokoro K, Miyamoto T, Ikemori R, et al. Diesel-exhaust particulates inoculated by the intranasal route have an adjuvant activity for IgE production in mice. J Allergy Clin Immunol. (1987) 79(4):639–45. doi: 10.1016/S0091-6749(87)80161-6
9. Diaz-Sanchez D, Dotson AR, Takenaka H, Saxon A. Diesel exhaust particles induce local IgE production in vivo and alter the pattern of IgE messenger RNA isoforms. J Clin Invest. (1994) 94(4):1417–25. doi: 10.1172/JCI117478
10. Diaz-Sanchez D, Tsien A, Casillas A, Dotson AR, Saxon A. Enhanced nasal cytokine production in human beings after in vivo challenge with diesel exhaust particles. J Allergy Clin Immunol. (1996) 98(1):114–23. doi: 10.1016/S0091-6749(96)70233-6
11. Diaz-Sanchez D, Tsien A, Fleming J, Saxon A. Combined diesel exhaust particulate and ragweed allergen challenge markedly enhances human in vivo nasal ragweed-specific IgE and skews cytokine production to a T helper cell 2-type pattern. J Immunol. (1997) 158(5):2406–13. doi: 10.4049/jimmunol.158.5.2406
12. Fujieda S, Diaz-Sanchez D, Saxon A. Combined nasal challenge with diesel exhaust particles and allergen induces in vivo IgE isotype switching. Am J Respir Cell Mol Biol. (1998) 19(3):507–12. doi: 10.1165/ajrcmb.19.3.3143
13. Saghari M, Jansen MAA, Grievink HW, Rissmann R, Moerland M. Characterization of KLH-driven immune responses in clinical studies: a systematic review. Front Drug Discovery. (2022) 2:992087. doi: 10.3389/fddsv.2022.992087
14. Diaz-Sanchez D, Garcia MP, Wang M, Jyrala M, Saxon A. Nasal challenge with diesel exhaust particles can induce sensitization to a neoallergen in the human mucosa. J Allergy Clin Immunol. (1999) 104(6):1183–8. doi: 10.1016/S0091-6749(99)70011-4
15. Terada N, Hamano N, Maesako KI, Hiruma K, Hohki G, Suzuki K, et al. Diesel exhaust particulates upregulate histamine receptor mRNA and increase histamine-induced IL-8 and GM-CSF production in nasal epithelial cells and endothelial cells. Clin Exp Allergy. (1999) 29(1):52–9. doi: 10.1046/j.1365-2222.1999.00406.x
16. Devouassoux G, Saxon A, Metcalfe DD, Prussin C, Colomb MG, Brambilla C, et al. Chemical constituents of diesel exhaust particles induce IL-4 production and histamine release by human basophils. J Allergy Clin Immunol. (2002) 109(5):847–53. doi: 10.1067/mai.2002.122843
17. Diaz-Sanchez D, Penichet-Garcia M, Saxon A. Diesel exhaust particles directly induce activated mast cells to degranulate and increase histamine levels and symptom severity. J Allergy Clin Immunol. (2000) 106(6):1140–6. doi: 10.1067/mai.2000.111144
18. Fernvik E, Peltre G, Sénéchal H, Vargaftig BB. Effects of birch pollen and traffic particulate matter on Th2 cytokines, immunoglobulin E levels and bronchial hyper-responsiveness in mice. Clin Exp Allergy. (2002) 32(4):602–11. doi: 10.1046/j.0954-7894.2002.01347.x
19. Steerenberg PA, Withagen CE, Dormans JA, van Dalen WJ, van Loveren H, Casee FR. Adjuvant activity of various diesel exhaust and ambient particles in two allergic models. J Toxicol Environ Health A. (2003) 66(15):1421–39. doi: 10.1080/15287390306415
20. Schober W, Lubitz S, Belloni B, Gebauer G, Lintelmann J, Matuschek G, et al. Environmental polycyclic aromatic hydrocarbons (PAHs) enhance allergic inflammation by acting on human basophils. Inhal Toxicol. (2007) 19(Suppl 1):151–6. doi: 10.1080/08958370701496046
21. Toyoshima K, Hayashida M, Yasunami J, Takamatsu I, Niwa H, Muraoka T. Factors influencing the prognosis of wheezy infants. J Asthma. (1987) 24(5):267–70. doi: 10.3109/02770908709070951
22. Geller-Bernstein G, Kenett R, Weisglass L, Tsur S, Lahav M, Levin S. Atopic babies with wheezy bronchitis. Follow-up study relating prognosis to sequential IgE values, type of early infant feeding, exposure to parental smoking and incidence of lower respiratory tract infections. Allergy. (1987) 42(2):85–91. doi: 10.1111/j.1398-9995.1987.tb02364.x
23. Atici A, Güneşer S, Alparslan N, Antmen B, Yilmaz M, Onenli N. Influence of smoke exposure on serum IgE levels of atopic patients. Acta Paediatr Jpn. (1994) 36(3):266–7. doi: 10.1111/j.1442-200X.1994.tb03176.x
24. Duff AL, Pomeranz ES, Gelber LE, Price GW, Farris H, Hayden FG, et al. Risk factors for acute wheezing in infants and children: viruses, passive smoke, and IgE antibodies to inhalant allergens. Pediatrics. (1993) 92(4):535–40. doi: 10.1542/peds.92.4.535
25. Wjst M, Heinrich J, Liu P, Dold S, Wassmer G, Merkel G, et al. Indoor factors and IgE levels in children. Allergy. (1994) 49(9):766–71. doi: 10.1111/j.1398-9995.1994.tb02100.x
26. Seymour BW, Pinkerton KE, Friebertshauser KE, Coffman RL, Gershwin LJ. Second-hand smoke is an adjuvant for T helper-2 responses in a murine model of allergy. J Immunol. (1997) 159(12):6169–75. doi: 10.4049/jimmunol.159.12.6169
27. Rumold R, Jyrala M, Diaz-Sanchez D. Secondhand smoke induces allergic sensitization in mice. J Immunol. (2001) 167(8):4765–70. doi: 10.4049/jimmunol.167.8.4765
28. Moerloose KB, Robays LJ, Maes T, Brusselle GG, Tournoy KG, Joos GF. Cigarette smoke exposure facilitates allergic sensitization in mice. Respir Res. (2006) 7(1):49. doi: 10.1186/1465-9921-7-49
29. Diaz-Sanchez D, Rumold R, Gong H Jr.. Challenge with environmental tobacco smoke exacerbates allergic airway disease in human beings. J Allergy Clin Immunol. (2006) 118(2):441–6. doi: 10.1016/j.jaci.2006.04.047
30. Samuelsen M, Nygaard UC, Lovik M. Allergy adjuvant effect of particles from wood smoke and road traffic. Toxicology. (2008) 246:124–31. doi: 10.1016/j.tox.2008.01.001
31. Li N, Wang M, Bramble LA, Schmitz DA, Schaur JJ, Sioutas C, et al. The adjuvant effect of ambient particulate matter is closely reflected by the particulate oxidant potential. Environ Health Perspect. (2009) 117(7):1116–23. doi: 10.1289/ehp.0800319
32. Castaneda AR, Vogel CFA, Bein KJ, Hughes HK, Smiley-Jewell S, Pinkerton KE. Ambient particulate matter enhances the pulmonary allergic immune response to house dust mite in a BALB/c mouse model by augmenting Th2- and Th17-immune responses. Physiol Rep. (2018) 6(18):e13827. doi: 10.14814/phy2.13827
33. Liu KY, Gao Y, Xiao W, Fu J, Wang S, Han X, et al. Multidimensional analysis of lung lymph nodes in a mouse model of allergic lung inflammation following PM2.5 and indeno[1,2,3-cd]pyrene exposure. Environ Health Perspect. (2023) 131(3):37014. doi: 10.1289/EHP11580
34. Bastain TM, Gilliland FD, Li YF, Saxon A, Diaz-Sanchez D. Intraindividual reproducibility of nasal allergic responses to diesel exhaust particles indicates a susceptible phenotype. Clin Immunol. (2003) 109(2):130–6. doi: 10.1016/S1521-6616(03)00168-2
35. Gilliland FD, Li YF, Saxon A, Diaz-Sanchez D. Effect of glutathione-S-transferase M1 and P1 genotypes on xenobiotic enhancement of allergic responses: randomised, placebo-controlled crossover study. Lancet. (2004) 363(9403):119–25. doi: 10.1016/S0140-6736(03)15262-2
36. Gilliland FD, Li YF, Gong H Jr, Diaz-Sanchez D. Glutathione s-transferases M1 and P1 prevent aggravation of allergic responses by secondhand smoke. Am J Respir Crit Care Med. (2006) 174(12):1335–41. doi: 10.1164/rccm.200509-1424OC
37. Whitekus MJ, Li N, Zhang M, Wang M, Horwitz MA, Nelson SK, et al. Thiol antioxidants inhibit the adjuvant effects of aerosolized diesel exhaust particles in a murine model for ovalbumin sensitization. J Immunol. (2002) 168(5):2560–7. doi: 10.4049/jimmunol.168.5.2560
38. Ritz SA, Wan J, Diaz-Sanchez D. Sulforaphane-stimulated phase II enzyme induction inhibits cytokine production by airway epithelial cells stimulated with diesel extract. Am J Physiol Lung Cell Mol Physiol. (2007) 292(1):L33–9. doi: 10.1152/ajplung.00170.2006
39. Heber D, Li Z, Garcia-Lloret M, Wong AM, Lee TY, Thames G, et al. Sulforaphane-rich broccoli sprout extract attenuates nasal allergic response to diesel exhaust particles. Food Funct. (2014) 5(1):35–41. doi: 10.1039/C3FO60277J
40. Riedel F, Hasenauer E, Barth PJ, Koziorowski A, Rieger CH. Formaldehyde exposure enhances inhalative allergic sensitization in the guinea pig. Allergy. (1996) 51(2):94–9. doi: 10.1111/j.1398-9995.1996.tb04564.x
41. Qiao Y, Li B, Yang G, Yao H, Yang J, Liu D, et al. Irritant and adjuvant effects of gaseous formaldehyde on the ovalbumin-induced hyperresponsiveness and inflammation in a rat model. Inhal Toxicol. (2009) 21(14):1200–7. doi: 10.3109/08958370902806159
42. Liu D, Zheng Y, Li B, Yao H, Li R, Zhang Y, et al. Adjuvant effects of gaseous formaldehyde on the hyper-responsiveness and inflammation in a mouse asthma model immunized by ovalbumin. J Immunotoxicol. (2011) 8(4):305–14. doi: 10.3109/1547691X.2011.600738
43. Larsen ST, Lund RMNGD, Thygesen P, Melchior Poulsen O. Di-(2-ethylhexyl) phthalate possesses an adjuvant effect in a subcutaneous injection model with BALB/c mice. Toxicol Lett. (2001) 125(1-3):11–8. doi: 10.1016/S0378-4274(01)00419-2
44. Larsen ST, Lund RM, Nielsen GD, Thygesen P, Poulsen OM. Adjuvant effect of di-n-butyl-, di-n-octyl-, di-iso-nonyl- and di-iso-decyl phthalate in a subcutaneous injection model using BALB/c mice. Pharmacol Toxicol. (2002) 91(5):264–72. doi: 10.1034/j.1600-0773.2002.910508.x
45. Larsen ST, Hansen JS, Hansen EW, Clausen PA, Nielsen GD. Airway inflammation and adjuvant effect after repeated airborne exposures to di-(2-ethylhexyl)phthalate and ovalbumin in BALB/c mice. Toxicology. (2007) 235(1-2):119–29. doi: 10.1016/j.tox.2007.03.010
46. Hansen JS, Larsen ST, Poulsen LK, Nielsen GD. Adjuvant effects of inhaled mono-2-ethylhexyl phthalate in BALB/cJ mice. Toxicology. (2007) 232(1-2):79–88. doi: 10.1016/j.tox.2006.12.011
47. Ban M, Langonné I, Huguet N, Pépin E, Morel G. Inhaled chemicals may enhance allergic airway inflammation in ovalbumin-sensitised mice. Toxicology. (2006) 226(2-3):161–71. doi: 10.1016/j.tox.2006.06.012
48. Hansen JS, Nielsen GD, Sørli JB, Clausen PA, Wolkoff P, Larsen ST. Adjuvant and inflammatory effects in mice after subchronic inhalation of allergen and ozone-initiated limonene reaction products. J Toxicol Environ Health A. (2013) 76(19):1085–95. doi: 10.1080/15287394.2013.838915
49. Nur Husna SM, Tan HT, Md Shukri N, Mohd Ashari NS, Wong KK. Allergic rhinitis: a clinical and pathophysiological overview. Front Med (Lausanne). (2022) 9:874114. doi: 10.3389/fmed.2022.874114
50. Granum B, Lovik M. The effect of particles on allergic immune responses. Tox Sci. (2002) 65:7–17. doi: 10.1093/toxsci/65.1.7
51. Nikasinovic L, Momas I, Just J. A review of experimental studies on diesel exhaust particles and nasal epithelium alterations. J Toxicol Environ Health B Crit Rev. (2004) 7(2):81–104. doi: 10.1080/10937400490241952
52. Saxon A, Diaz-Sanchez D. Air pollution and allergy: you are what you breathe. Nat Immunol. (2005) 6(3):223–6. doi: 10.1038/ni0305-223
53. Viera L, Chen K, Nel A, Lloret MG. The impact of air pollutants as an adjuvant for allergic sensitization and asthma. Curr Allergy Asthma Rep. (2009) 9:327–33. doi: 10.1007/s11882-009-0046-x
54. Li CH, Sayeau K, Ellis AK. Air pollution and allergic rhinitis: role in symptom exacerbation and strategies for management. J Asthma Allergy. (2020) 13:285–92. doi: 10.2147/JAA.S237758
Keywords: allergy, irritation, rhinitis, conjunctivitis, asthma, adjuvants
Citation: Shusterman D (2024) History of pollutant adjuvants in respiratory allergy. Front. Allergy 5:1374771. doi: 10.3389/falgy.2024.1374771
Received: 22 January 2024; Accepted: 4 March 2024;
Published: 12 March 2024.
Edited by:
R. Maximiliano Gomez, Catholic University of Salta, ArgentinaReviewed by:
Özlem Göksel, Ege University, Türkiye© 2024 Shusterman. This is an open-access article distributed under the terms of the Creative Commons Attribution License (CC BY). The use, distribution or reproduction in other forums is permitted, provided the original author(s) and the copyright owner(s) are credited and that the original publication in this journal is cited, in accordance with accepted academic practice. No use, distribution or reproduction is permitted which does not comply with these terms.
*Correspondence: Dennis Shusterman ZGVubmlzLnNodXN0ZXJtYW5AdWNzZi5lZHU=
Disclaimer: All claims expressed in this article are solely those of the authors and do not necessarily represent those of their affiliated organizations, or those of the publisher, the editors and the reviewers. Any product that may be evaluated in this article or claim that may be made by its manufacturer is not guaranteed or endorsed by the publisher.
Research integrity at Frontiers
Learn more about the work of our research integrity team to safeguard the quality of each article we publish.