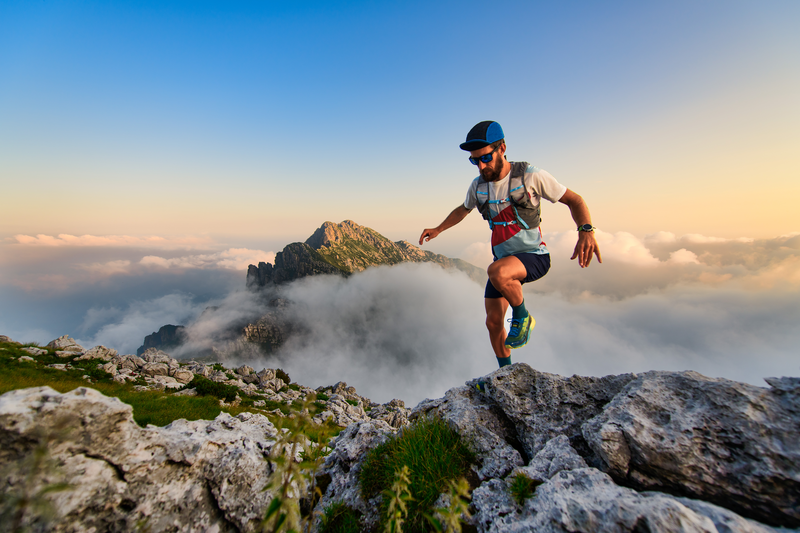
95% of researchers rate our articles as excellent or good
Learn more about the work of our research integrity team to safeguard the quality of each article we publish.
Find out more
ORIGINAL RESEARCH article
Front. Sustain. Food Syst. , 19 March 2025
Sec. Crop Biology and Sustainability
Volume 9 - 2025 | https://doi.org/10.3389/fsufs.2025.1551584
This article is part of the Research Topic Advancements in Post-Harvest Physiology and Technology of Fruits and Vegetables for Sustainable Food Production View all articles
Introduction: Milk thistle (Silybum marianum L.) is a wild plant commonly employed in traditional medicine, particularly for its recognized applications in treating liver diseases. However, there are limited data available on the phytochemical analysis and biological activity of the different parts of milk thistle cultivated in Morocco.
Material and methods: This study aims to examine and compare the phytochemical composition, the antioxidant activity and antibacterial activity of seeds, leaves and stems of milk thistle. The antioxidant activity has been carried out using 2,2-diphenyl-1- picrylhydrazyl (DPPH) and reducing power (RP) assays. The antibacterial activity was tested against seven bacteria, furthermore, we assessed the synergistic effects by evaluating the combination of these plant parts. High-Performance Liquid Chromatography with Ultraviolet Detection and Mass Spectrometry (HPLC-UV-MS) and Gas Chromatography–Mass Spectrometry (GC–MS) have been used to identify particular phytoconstituents in each component.
Results and discussion: Seeds and leaves showed comparable phenolic content However, leaves showed the highest flavonoid content with the highest antioxidant activity in both tests and no DPPH scavenging activity and reducing power activity detected in stems. Seeds showed the highest inhibition zone against S. aureus with an inhibition zone of 8 mm. The stems did not exhibit any inhibition zones against the tested bacteria except for K. pneumoniae where the inhibition zone was 6.60 mm. The combination of different parts did not show an increase in antibacterial activity. The extracts revealing a diverse array of bioactive compounds that enhance the antioxidant potential of milk thistle, emphasizing the distinct properties of each plant part. The closeness in phenolic content between seeds and leaves suggests that both parts could be valuable sources of antioxidants. However, despite the abundant phenolic content, the limited antibacterial activity indicates that the milk thistle’s extracts may be more pronounced in its antioxidant properties rather than its antibacterial effects.
Research on the antimicrobial activity of medicinal plants becomes important because phenolic compounds, which are commonly found in plant extracts, have strong antibacterial properties. This study is extremely noteworthy as microbial diseases continue to pose major global public health concerns. There is a growing interest in exploring natural alternatives to artificial preservatives in food, particularly plant-derived phenolic compounds (Savoia, 2012; Farhadi et al., 2019). Plant secondary metabolites are recognized as strong free radical scavengers and to offer diverse benefits across various biological pathways. They are present in almost all part of the plant, including the roots, fruits, seeds, leaves, and bark (Elshafie et al., 2023). Terpenoids, phenolic chemicals, and compounds containing sulfur or nitrogen are the three primary classes into which they can be divided. These secondary metabolites greatly enhance the capacity of the plant to defend itself against infections, herbivores, and other environmental threats (Elshafie et al., 2023; Jomova et al., 2023). Among these secondary metabolites, flavonoids and similar plant-derived compounds, known for their hydroxyl group-rich structures, are highly regarded for their remarkable antioxidant properties, making them exceptional in their ability to capture and neutralize detrimental free radicals. This remarkable characteristic positions them as strong contenders for protecting against diseases associated with an overabundance of free radicals. Through their active role in counteracting free radicals by donating hydrogen atoms, polyphenols offer a promising approach to reduce cellular damage and address the underlying oxidative stress (Panche et al., 2016; Rasouli et al., 2017). Many plant extracts have been proven to be more effective antioxidants than the synthetic antioxidant. This shows the potential of natural sources as antioxidants, which may provide better health advantages than synthetic alternatives. For example, Pelargonium endlicherianum extract has been demonstrated to be twice as effective as the synthetic antioxidant BHT (butylated hydroxytoluene) (Krishnaiah et al., 2011).
Extensive research has been conducted on the antimicrobial properties of polyphenols present in vegetable foods and medicinal plants, particularly focusing on flavan-3-ols, flavonols, and tannins. These polyphenols have gained significant interest due to their broad spectrum and superior antimicrobial activity compared to other polyphenolic compounds (Daglia, 2012; Manso et al., 2021). However, the specific way in which polyphenols act against bacteria is not fully understood and warrants further investigation. The susceptibility of microorganisms to polyphenols varies depending on species and strain along with the molecular structure of the phenolic compounds. An additional important factor is the composition of the extract and its concentration (Efenberger-Szmechtyk et al., 2021). Numerous research investigated the antibacterial properties of plant extracts and essential oils against both Gram-positive and Gram-negative bacteria. These studies used several solvents and plant organs for extraction, which enhanced our understanding of their efficacy in managing bacterial infections and have been found to be effective in preventing bacterial development. There is rising interest in investigating the potential synergistic effects of combining different polyphenols or combining extracts with conventional antibiotics to enhance antimicrobial activity while minimizing the risk of resistance development (Silva and Fernandes Júnior, 2010; Efenberger-Szmechtyk et al., 2021).
One notable plant that is rich in these valuable compounds is milk thistle, known for its liver-protecting properties due to silymarin, a mixture of flavonolignans (silybin A, silybin B, isosilybin A, isosilybin B, silychristin, isosilychristin, and silydianin) and the flavonoid taxifolin. Silybin is the most abundant and active component (Begum et al., 2010; Biedermann et al., 2014). Historically, milk thistle fruits were roasted and used as a coffee substitute. Its flower heads are prepared like artichokes, and its leaves are used in salads or as a spinach alternative (Abenavoli et al., 2018). In European folk medicine, the roots, bark, leaves, and immature fruits are employed to treat gastroenteritis, diarrhea, and dysentery. The leaves are utilized for soothing sores and relieving hemorrhoid discomfort. In Morocco, the plant is known as “Sûk ej-jmel” or “sûk el-hmîr” and its flowers and roots are employed as a remedy for fever in Moroccan traditional medicine (Marmouzi et al., 2021). Beyond its hepatoprotective properties, milk thistle has shown anticancer effects by inhibiting the growth of various cancer cells, inducing apoptosis in cancer cells, and possessing anti-inflammatory and immunomodulatory activities. Additionally, it has demonstrated neuroprotective and cardioprotective effects, antidiabetic properties, and skin protective benefits (Koltai and Fliegel, 2022). Milk thistle seeds are a valuable source of antioxidants and food preservatives due to their remarkable free radical scavenging ability and capacity to prevent lipid peroxidation (Rezk et al., 2024). Studies have shown that incorporating milk thistle extracts, and pure silymarin into food can slow the oxidation process, preserving nutritional quality and extending shelf life (Marceddu et al., 2022). It has been shown that milk thistle enhances antioxidant activity, improves nutritional content, and supports the viability of yogurt starter bacteria, contributing to the stability and shelf life of functional yogurt (Jaffar et al., 2024). Silymarin has shown promise in preliminary studies as an antibacterial agent against gram-positive bacteria (Bacillus subtilis, Bacillus cereus, and Staphylococcus aureus) and gram-negative bacteria (Escherichia coli, Pseudomonas aeruginosa) (SAA, 2017). It was found that silybin, when combined with oxacillin or ampicillin, showed enhanced synergistic activity against Methicillin-resistant Staphylococcus aureus (MRSA) (Kang et al., 2011). Silibinin reduced the synthesis of macromolecules, including RNA and proteins, in Gram-positive bacteria (Lee et al., 2003).
This study aimed to investigate and compare the phytochemical composition, antioxidant activity, and antibacterial properties of the seeds, leaves, and stems of milk thistle as research has predominantly concentrated on milk thistle seeds, although extracts from other parts of the plant also demonstrate significant antioxidant activity (Nowak et al., 2021). Additionally, to our knowledge, the phytochemical composition and its biological activity in various parts of wild Silybum marianum in Morocco have not been explored. Moreover, this study will examine the antibacterial properties of individual plant parts and explore potential combined effects. It provides new insights that contribute to a broader understanding of the milk thistle’s extracts properties and their potential applications. Additionally, a Gas Chromatography–Mass Spectrometry (GC–MS) and High-Performance Liquid Chromatography with Ultraviolet Detection and Mass Spectrometry HPLC-UV-MS analysis has been conducted to identify specific phytoconstituents in each part.
Milk thistle (Silybum Marianum L.) samples were carefully collected from the Rabat–Sale–Kenitra region, Morocco 34.1728° N, 6.2376° W during May 2023. The collection process involved gathering leaves and stems during the mid-flowering stage and seeds were harvested at maturity. These plant parts were then left to naturally dry at room temperature for 20 days. Subsequently, all the collected material underwent a grinding process, resulting in a finely powdered form. This powder will be used for further comprehensive investigations and analyses.
Six grams of plant material, including seeds, leaves, stems, and the combined parts were individually subjected to ethanol 96% extraction using the ultrasound (J.P. Selecta, Barcelona, Spain). This extraction procedure, following the approach of Nowak et al. with some modifications, was conducted at 45°C for 60 min at a frequency of 50 kHz. The solvent was removed under reduced pressure at 50°C using a rotary vacuum evaporator. The extracts were then placed in refrigeration until further analysis (Nowak et al., 2021).
Ethanol was deliberately selected as solvent due to its polar characteristics, which allowed it to efficiently dissolve a broad spectrum of compounds present in the plant material. Additionally, the decision to use ethanol was influenced by its favorable safety profile and its environmentally friendly attributes when compared to other polar solvents.
The yield of various extracts was determined using the subsequent Equation 1:
The Folin–Ciocalteu method was employed to assess the total phenolic content in the extracts. This followed the process described by Mansouri et al. (2022). The ability of phenolic compounds to reduce the reagent in an alkaline medium and produce a detectable blue complex is measured using the Folin–Ciocalteu method (Mansouri et al., 2022). In brief, 100 μL of each sample were added to test tubes. 400 mL of 7.5% sodium carbonate and 500 μL of Folin–Ciocalteu’s reagent 10% were then added to each tube. Following a good mixing, the tubes were incubated for 60 min, and the absorbance was measured at 765 nm. After that, the amount of total phenolic content was measured and expressed as milligrams of gallic acid equivalents (GAE) per gram of dry extract (mg GAE/g DE). The equation used to calculate the phenolic content of the plant extracts was established from the calibration range of gallic acid, y = 0.0096x + 0.0024, and R2 = 0.999.
In this study, the AlCl3 method was explored to quantify the flavonoid content in the extracts, following the protocol outlined by Ali et al. (2020). 1 mL of 2% methanolic AlCl3, 6H2O was mixed with 1 mL of the extracts. The tubes were incubated for 10 min. This method leverages the unique reactivity of flavonoids with aluminum chloride, resulting in the formation of a distinctive complex with a measurable absorbance at 430 nm. The utilization of quercetin equivalents (QE) as a unit of measurement allows for a standardized representation of the flavonoid content, expressed in milligrams of quercetin equivalent per grams of the dry extract (mg QE/g DE). The equation used to calculate the flavonoid content of the plant extracts was established from the calibration range of quercetin y = 0.0376x – 0.0245, and R2 = 0.9965.
Two common and easy assays for measuring antioxidant activity were the 2,2-diphenyl-1-picrylhydrazyl (DPPH) and reducing power (RP). These two methods are renowned for being simple, quick, and cost-effective.
The antioxidant activity was evaluated by DPPH using the protocol outlined by Zirari et al. (2024). The DPPH solution was first prepared at a concentration of 0.04 mg/mL, which is equivalent to 101.5 μM. Then, 2 mL of the DPPH solution is combined with 1 mL of milk thistle extracts at various concentrations ranging from 0 to 1,000 μg/mL. A control sample containing the DPPH solution and ethanol 96% used for extract solubilization is prepared. The mixtures were then incubated in darkness at room temperature for 30 min. After the incubation period, the absorbance of each solution is measured at 517 nm using a UV-2005 spectrophotometer (UV-2005, Selecta, Barcelona, Spain), with ascorbic acid serving as a positive control for reference (Zirari et al., 2024).
The percentage inhibition of DPPH was calculated using the formula (Equation 2):
Finally, the IC50 value, indicating the concentration of the sample necessary to inhibit 50% of the free radical DPPH was determined. Lower IC50 values indicate stronger antioxidant potential in the extracts.
The ferric ion reducing power of the extracts was assessed following the protocol outlined by Aouji et al. (2023). This method is based on the reduction of ferric ions (Fe3+) by antioxidants present in the extracts, providing a quantitative measure of the reducing capacity of these compounds. The procedure involved mixing 1 mL of milk thistle extracts at different concentrations (ranging from 0 to 1,000 μg/mL) with 2.5 mL of phosphate-buffered solution (0.2 M at pH 6.6) and 2.5 mL of potassium ferricyanide solution [K3Fe(CN)6] at 1% w/v. The mixture was then incubated at 50°C for 20 min. To stop the reaction, trichloroacetic acid (10% w/v) was added, followed by 2.5 mL of distilled water and 0.5 mL of 0.1% w/v FeCl3. The antioxidants in the extracts reduce ferric ions (Fe3+) to ferrous ions (Fe2+). The reduction is aided by the action of potassium ferricyanide, and the presence of ferrous ions is detected by the addition of ferric chloride. The absorbance of the resulting solution was measured at 700 nm, utilizing a blank that contained all the reagents and ethanol 96%.
The concentration of the extracts required for a 50% reduction in ferric ions (IC₅₀) serves as a quantitative measure of the antioxidant potential. In this assay, ascorbic acid is employed as a positive control for comparison (Aouji et al., 2023).
Antibacterial tests should be conducted using young cultures in the exponential growth phase. The strains are reactivated by inoculating the bacterial species into a liquid medium. After incubation for 24 h at 37°C, a second subculture is carried out on Petri dishes containing nutrient agar (NA) for bacteria.
From the young cultures on nutrient agar (NA), 3-to-5 well isolated and perfectly identical colonies are picked and placed into 5 mL of sterile physiological saline solution at 0.9% NaCl. The mixture is vortexed for a few seconds. The standardization of the suspension to 106 CFU/mL is performed using a spectrophotometer set at 620 nm (Candan et al., 2003).
The efficacy of the extracts in inhibiting microbial growth was evaluated using the disk diffusion technique on Muller Hinton Agar (MHA) medium, following the protocol outlined by Oubihi et al. (2020). The extracts were tested against seven pathogenic bacterial strains to assess their effectiveness, namely Staphylococcus aureus, Staphylococcus epidermidis, Escherichia coli, Acinetobacter baumannii, Enterobacter cloacae, Klebsiella pneumoniae, and MRSA, chosen for their pathogenic nature and potential to contaminate food sources and isolated from a clinical sample. To initiate the analysis, bacterial colonies were isolated and transferred to tubes with sterile water. Afterward, the MHA plates were inoculated with the microbial suspension. Small sterile discs, 6 mm in diameter, were loaded with 10 μL of the extract, diluted in dimethyl sulfoxide (DMSO) to a concentration of 30 mg/mL as per the protocol. These discs were then carefully positioned on the surfaces of the MHA plates. Incubation of the plates occurred at 37°C for 24 h, with triplicate experimental tests conducted.
The extent of inhibition zone diameters, measured in millimeters, acted as indicators of the antimicrobial efficacy of the extracts, norfloxacin and amoxicillin serving as reference standard (Oubihi et al., 2020).
The minimum inhibitory concentration (MIC) is determined using the agar dilution technique (Imane et al., 2024). Serial dilutions are prepared at 100 μL/mL, 40 μL/mL, 20 μL/mL, 10 μL/mL, 5 μL/mL, 3.3 μL/mL, and 2 μL/mL in an agar solution. In test tubes containing 13.5 mL of solid Mueller-Hinton medium, 1.5 mL of each dilution is aseptically added to obtain final concentrations of 10 μL/mL, 4 μL/mL, 2 μL/mL, 1 μL/mL, 0.5 μL/mL, 0.33 μL/mL, and 0.2 μL/mL. The contents of each tube are immediately poured into sterile Petri dishes. Control plates containing only the culture medium are also prepared. Inoculation is performed using a calibrated platinum loop to ensure a consistent volume of inoculum. The plates are then incubated at 37°C for 24 h, and each experiment is repeated three times. The MBC is determined the following day after reading the MIC by streaking samples from each plate with no visible growth onto Mueller-Hinton agar. The inoculated plates are then incubated at 37°C for 24 h.
Analysis of the extracts was carried out after derivatization of the extracts; acetylation was employed as the chosen reaction method for GC–MS sample preparation. To achieve this, 5 mg of the extract was dissolved in 1 mL of acetic anhydride, and 4 drops of pyridine, serving as a catalyst, were added. The mixture underwent a 20 min heating period in a water bath at 50°C. Subsequently, the mixture was allowed to cool overnight undergoing hydrolysis with 6 mL of cold water, utilizing magnetic stirring for 2 h in an ice water bath. The resulting products underwent extraction with chloroform, and the organic phase was neutralized using a saturated solution of sodium bicarbonate (NaHCO3). After drying with anhydrous sodium sulfate (Na2SO4), the solution was evaporated.
The experimental setup involved the utilization of the GC Trace 1,300 coupled with the TSQ 8000 Evo mass spectrometer. The capillary column used was a Thermo TR-5 (30 m × 0.25 mm × 0.25 μm). The column temperature was first kept at 60°C for ten minutes. After that, it ramped up by 10°C per minute to 80°C, which it then maintained for two minutes. Finally, it increased gradually by 5°C per minute to 300°C, which it sustained for five minutes. The ion source temperature was kept at 250°C while the injector was adjusted to 280°C. The carrier gas, helium, was injected at a volume of 1 μL and flowed at a rate of 1 mL/min. At 70 eV of electron energy, mass spectra were obtained (Haida et al., 2022).
The HPLC-UV-MS analysis was carried out according to Wallace et al. (2003). The following standard polyphenolic compounds were used: Ascorbic acid, Quercetin, Vanillin, Gallic acid, Glucose. A C18 column (150 mm × 4.6 mm, 5 μm) maintained at 40°C. Solvent A consisted of a 20:80 methanol-to-water ratio, while Solvent B contained 80:20 methanol-to-water. The injection volume was set at 10 μL, and the mobile phase was delivered at a flow rate of 0.75 mL/min. The initial composition of the mobile phase was 85% Solvent A and 15% Solvent B, maintained for 5 min. A linear gradient was then applied to adjust the ratio to 45% Solvent A and 55% Solvent B over 15 min. This composition was held constant for 20 min before being linearly returned to the initial 85:15 ratio over the next 10 min.
The mean and standard deviation (SD) were utilized to present the results of the analyses. The data collected during the experiments were performed in three repetitions (n = 3). Subsequently, a one-way analysis of variance (ANOVA) was employed to examine the acquired data, followed by Tukey’s multiple distribution test. A significance level of α = 5% was considered.
The yield percentages for seeds (7%), leaves (20%), and stems (6.67%) (Figure 1) provide a comprehensive overview of the plant’s resource distribution. As an interesting side note, the actual yields from the first 6 g vary: 0.42 g from seeds, 1.2 g from leaves, and 0.4 g from stems. Notably, there is no statistically significant variance in yield between seeds and stems. In contrast, Javeed et al. in the methanolic milk thistle extracts from Pakistan reported seeds having the highest yield 5.01% followed by leaves 3.47% and stems with the lowest yield 2.19% (Javeed et al., 2022). Ethanol demonstrated superior efficiency in extracting bioactive compounds from leaves (1.2 g) compared to other plant parts. While achieving a high extraction yield is important for efficiency, it does not necessarily mean that the concentration of bioactive components will be high. This is because certain bioactive compounds are sensitive to oxygen and heat. The differences in yield between the plant parts is influenced by several factors such as variations in the bioactive compound in each part affected by the growth stage and environmental conditions. Certain plant parts may have a higher concentration of bioactive compounds than others. Additionally, certain extraction methods and solvent could be more suitable for a specific plant part based on their chemical composition and physical characteristic (Aspé and Fernández, 2011; Yang et al., 2012).
Figure 1. Extraction yields of different plant parts of milk thistle. The significant difference (p ≤ 0.05) is illustrated by the letters a, b and c.
This study aimed to elucidate the phytochemical profiling of different parts of milk thistle. Antioxidants serve an important role in countering oxidative stress, and understanding their distribution in various plant tissues is critical for determining the full range of potential health benefits. Our findings, as shown in the table, highlight distinct variations in the total phenolics and flavonoids content among the different plant parts. According to the data presented in (Table 1) and (Figure 2), the phenolic content in both seeds and leaves appears relatively close or comparable 74.79 ± 2.65 mg GAE/g and 73.5 ± 2.94 mg GAE/g, respectively. However, leaves exhibit a significantly higher flavonoid content of 24.28 ± 0.08 mg QE/g compared to the seeds 6.50 ± 0.08 mg QE/g. Turning attention to stems, the analysis reveals relatively lower amounts of bioactive compounds compared to seeds and leaves. Stems exhibit a total phenolic content of 11.57 ± 3.46 mg GAE/g and a flavonoid content of 8.48 ± 0.05 mg QE/g. This indicates a comparatively modest presence of these bioactive compounds in stems. While our study only indicated a slight difference in phenolic content between leaves and seeds, the overarching pattern is consistent with the findings of Javeed et al. of the methanolic Silybum marianum extracts cultivated from Pakistan, who found that leaves demonstrated the highest concentration of phenolic and flavonoid content 21.79 ± 0.18 mg GAE/g and 129.66 ± 0.65 mg GAE/g of total extract, respectively. However, seeds as observed in their study, demonstrated the lowest content, with a negligible phenolic content of (1.70 ± 0.03 GAE/g DE). The various plant organs studied, revealed the presence of several phytochemicals, including alkaloids, glycosides, flavonoids, terpenoids, steroids, and catecholic tannins. However, phenols were absent in the seeds, and saponins and gallic tannins were not detected in any of the organs (Javeed et al., 2022). In contrast, in their investigation into Iraqi Silybum marianum, Eldalawy et al. found that the seeds exhibited the highest concentration of phenolic and flavonoid content among the different plant parts 67.03 ± 0.56 μg GAE /mg dry plant and 12.32 ± 0.45 μg QE/mg dry plant, respectively, followed by flowers and leaves. Stems, on the other hand, contained the lowest amount with 11.03 ± 0.45 μg GAE /mg dry plant and 7.5 ± 0.2 μg QE/mg dry plant while elevated tannin levels were noted in both leaves and stems 74 ± 0.63 and 66.34 ± 0.35 μg tannic acid /mg dry plant, respectively (Eldalawy et al., 2021). Sun et al., in their investigation of various plant components from China, including the pappus and roots, reported that the pappus exhibited the highest concentration of both phenolic and flavonoid contents compared to the other plant parts 48.97 ± 0.41 mg GAE/g of dry plant material and 17.10 ± 0.56 mg rutin/g of dry plant material, respectively, followed by fruit receptacle, root, and leaf. They suggested that the pappus and fruit receptacle could serve as potential sources for flavonoid extraction. The stems exhibited the lowest content of phenolic and flavonoid 9.80 ± 0.13 mg GAE/g of dry plant material and 6.64 ± 0.43 mg rutin/g of dry plant material (Sun et al., 2016). Mhamdi et al. reported that methanolic extracts of the Tunisian milk thistle seeds contained 29 mg GAE/g DW of phenolic compounds, 3.39 mg EC/g of flavonoids, and 1.8 mg EC/g of condensed tannins (Mhamdi et al., 2016). Aziz et al. reported that the total phenolic content (TPC) and total flavonoid content (TFC) of the methanolic milk thistle seeds extracts varied among different varieties and locations in Pakistan. For the blue variety, TPC ranged from 26.90 ± 1.34 mg GAE/g to 35.07 ± 1.75 mg GAE/g while TFC ranged from 17.61 ± 0.88 mg QE/g to 29.09 ± 1.45 mg QE/g. In the white variety, TPC ranged from 24.17 to 32.60 mg GAE/g, and TFC ranged from 16.01 to 27.12 mg QE/g (Aziz et al., 2021).
Figure 2. Phenolic and flavonoid contents of different parts of milk thistle. The significant difference (p ≤ 0.05) is illustrated by the letters a, b and c.
In a recent study conducted by Maaloul et al., they examined the various parts of two Silybum species, Silybum marianum and Silybum eburneum, harvested in Tunisia, which included both mature and immature seeds. Their findings revealed that the mature seeds of both species displayed the greatest levels of phenolic and flavonoid content with Silybum marianum exhibiting a higher level of 161.4 ± 13.9 mg GAE/g DE and 41.9 ± 0.4 mg QE/g DE followed by immature seeds 55.9 ± 8.3 mg GAE/g DE and 23.9 ± 1.1 mg QE/g DE. In their study, the leaves demonstrated a phenolic content of 1.7 ± 0.1 mg GAE/g DE and a flavonoid content of 7.4 ± 1.0 mg QE/g DE. The stems displayed the lowest content compared to other parts, with 0.7 ± 0.1 mg GAE/g DE for phenolics and 2.9 ± 0.3 mg QE/g DE for flavonoids (Maaloul et al., 2024). Guemari et al., in their investigation into the various parts of Silybum marianum from Algeria, reported that seeds displayed the highest phenolic content 127.39 ± 1.41 mg GAE/g DW followed by flowers 42.22 ± 2.07 mg GAE/g DW and leaves 22.25 ± 1.11 mg GAE/g DW, whereas the highest content of flavonoid was found in flowers 34.06 ± 0.45 mg EQ/g D followed by leaves 19.41 ± 0.44 mg EQ/g DW and seeds 18.33 ± 0.70 mg EQ/g DW, with leaves containing the highest content of flavanol followed by flowers and seeds. Twigs, however, were reported to have the lowest phenolic and flavonoid contents of 9.05 ± 0.04 mg GAE/g DW and 2.74 ± 0.03 mg EQ/g DW (Guemari et al., 2020).
It is noteworthy that the literature presents a diversity of findings regarding the primary source of natural antioxidants in Silybum marianum. However, it is commonly observed in the mentioned studies that the stems of the milk thistle plant tend to have fewer phenolic and flavonoid compounds compared to other parts. The concentration of phenolic compounds and flavonoids is subject to variations influenced by the time of harvest, geographical factors, extraction methods, and the solvent employed in the extraction process. The interplay of these elements significantly impacts the final composition and concentration of phenolic compounds within the extracted sample.
The methodology involved assessing antioxidant activity through established assays, such as free radical DPPH scavenging activity and reducing power (RP) assays. The findings (in Table 1 and Figure 3) ascorbic acid used as a reference, showed the highest antioxidant activity in both DPPH and RP assays with IC50 = 81.16 ± 5.55 μg /mL and IC50 = 92.04 ± 2.77 μg /mL, respectively. The findings revealed a robust positive association between the total phenolic and flavonoid content and the observed antioxidant activity, showcasing variations across the tested samples. Notably, leaves demonstrated the highest antioxidant activity in both DPPH and RP assays IC50 = 388.16 ± 2.47 μg/mL and 181.36 ± 4.76 μg/mL, respectively. Seeds, in contrast, showed no detectable DPPH scavenging activity in our investigation, while a significant RP value (447.76 ± 10.08 μg/mL) highlighted the potent reducing power of the seed extract. Our findings align with those of Nowak et al., who employed the same solvent and extraction technique, and similarly to their results, our study did not detect any antioxidant activity in seeds when assessed using the DPPH assay (Nowak et al., 2021). This can be explained by variations in the chemical composition of the extracts in different parts, as not all antioxidants may react with DPPH. Some antioxidants might have specific reactivity with other radicals or exhibit their antioxidant activity through different mechanisms that are not captured by the DPPH assay. The reaction between DPPH and antioxidants is largely influenced by the antioxidants’ ability to donate hydrogen, which is in turn determined by their structural characteristics (Tabart et al., 2009). Flavonoids with high radical-scavenging activity typically possess ortho-dihydroxyl groups (catechol structure) in the B-ring or A-ring, a hydroxyl group at position 3 of the C-ring, and a C2-C3 double bond conjugated with a 4-oxo group in the C-ring. These structural features enhance their ability to donate hydrogen atoms, making them more effective in neutralizing free radicals. Additionally, the presence of hydroxyl groups at positions 3, 5, and 7 further strengthens their antioxidant capacity (Ammar et al., 2009; Olszowy, 2019).
Figure 3. Antioxidant activity of different parts of milk thistle with ascorbic acid. The significant difference (p ≤ 0.05) is illustrated by the letters a, b and c.
The chemical composition can differ not only between plant species but also within different plant parts. Thus, the variability in antioxidant activity observed in different plant extracts can be attributed to differences in flavonoid composition and structural features, which directly influence their reactivity with DPPH (Ammar et al., 2009). Although seeds and leaves exhibit comparable phenolic content, the differences in antioxidant activity can be attributed not only to phenolic compounds but also to other non-phenolic antioxidant molecules. In the DPPH assay, the reagent mainly interacts with free radicals through hydrogen donation, while in the RP assay, it evaluates the antioxidant’s ability to donate electrons (Asif, 2015). Both assays do not exclusively measure phenolic compounds but also react with non-phenolic substances like ascorbic acid, saccharides, and proteins, which can contribute to the overall antioxidant activity (Tabart et al., 2009). It is crucial to recognize that each method has its own limitations and may interact differently with various antioxidant compounds. For instance, Thiol groups do not exhibit optimal or swift reactivity in the RP assay, leading to their minimal contribution to the overall RP value. Each method employed does not capture the entirety of antioxidants present in a mixture. Factors such as the chemical nature of antioxidants and the complexity of the sample matrix can influence the outcomes. Therefore, a comprehensive understanding of the reactivity and mechanisms involved in each assay is essential for an accurate interpretation and meaningful comparisons across studies (Benzie and Choi, 2014). The choice of solvent and extraction method significantly influences the extraction efficiency of specific molecules (Benzie and Choi, 2014).
The stems showed non-detectable values for DPPH and RP assays, suggesting a limited concentration of antioxidants within this plant part, aligning with the observed lower content. It is important to note that, in our study, the antioxidant assessment was conducted at a concentration of 1,000 μg/mL, at which no detectable DPPH scavenging or RP activity was observed in stems. This concentration choice emphasizes the specific conditions under which the antioxidant assays were performed and highlights the absence of measurable activity at the given concentration. In their investigation, Javeed et al. (2022) revealed that seeds exhibited the highest DPPH radical activity, whereas the stems displayed the maximum reducing activity. Notably, despite containing the highest level of phenolic and flavonoid content, the leaves’ extracts demonstrated the lowest DPPH radical activity and reducing power activity (Javeed et al., 2022). Guemari et al. (2020), in their study on Algerian Silybum marianum, conducted three assays, total antioxidant activity, DPPH radical scavenging activity, and ferric reducing power activity. They identified seeds as having the highest antioxidant activity among all three assays. However, twigs exhibited the lowest antioxidant activity in both the DPPH and RP assays, while leaves showed the lowest total antioxidant activity (Guemari et al., 2020). In contrast, Maaloul et al. (2024) in their investigation of antioxidant of Tunisian Silybum marianum reported that flowers exhibited the highest total antioxidant activity, followed by mature seeds and immature seeds. However, the strongest DPPH radical scavenging activity was seen in immature seeds followed by mature seeds. Moreover, mature seeds exhibited the highest reducing power activity. Stems, however, exhibited the lowest antioxidant activity among the three assays (Maaloul et al., 2024). Ahmed et al. investigated two varieties of Silybum marianum, purple flowering and white flowering, and found that the seeds and roots of both varieties showed the greatest ability to scavenge DPPH free radicals than other plant parts, making them a natural source of antioxidants and food supplements. Sun et al. similarly found in their study that stems displayed the lowest DPPH radical scavenging activity and reducing power activity. The pappi demonstrated the highest antioxidant activity in both tests, followed by the roots. They suggested that these two parts could serve as valuable sources of natural antioxidants (Ahmad et al., 2013). Mhamdi et al. (2016) found that the methanolic extract of milk thistle seeds exhibited strong DPPH radical scavenging activity with an IC50 of 39 μg/mL, while the reducing power was moderate, around 1,000 μg/mL (Mhamdi et al., 2016).
The findings of antibacterial activity clearly indicate a variance in effectiveness among the different parts of milk thistle (seeds, leaves, and stems) and the tested antibiotic norfloxacin and amoxicillin (Table 2). The seeds displayed the highest inhibitory effect, with ZI values ranging from 6.17 to 8.00 mm. This suggests a moderate antibacterial activity against a wide range of bacteria, such as S. aureus, S. epidermidis, K. pneumoniae, E. coli, A. baumannii, and MRSA. Notably, the leaves showed a more moderate inhibitory effect, particularly against S. epidermidis, K. pneumoniae, and E. coli, with ZI values ranging from 6.20 to 6.53 mm. The stems, however, displayed a specific inhibitory effect against K. pneumoniae, with an ZI value of 6.60 mm. In contrast to the extracts, the antibiotic norfloxacin and amoxicillin displays varying efficacy. Norfloxacin demonstrated inhibitory effects against all the tested bacteria, with ZI values ranging from 15 to 20 mm, except for K. pneumoniae, which showed any inhibitory effect. Amoxicillin has no inhibitory effect against any of the tested bacteria, highlighting potential limitations in its effectiveness against the selected strains. Interestingly, E. cloacae displayed no inhibitory effect for all milk thistle extracts, suggesting potential bacterial resistance or insensitivity to the compounds present in the extracts.
In our investigation, after examining the antibacterial activity of different parts, the investigation aimed to explore their combined effects and whether their interaction could influence and increase the antibacterial activity. The findings demonstrate that the mixture did not show any increased effectiveness. Notably, even when the parts are combined, E. cloacae does not show any inhibitory effect. While seeds showed an inhibitory effect against A. baumannii, this effect was not observed in the mixture of plant parts. The inhibition zone against S. aureus was greater in seeds than in the combination. S. epidermidis, K. pneumoniae, E. coli, MRSA showed moderate inhibition zones. Collectively, these results suggest that milk thistle seeds, in particular, demonstrated the highest antibacterial activity compared to other plant parts and the mixture.
In our investigation, despite the abundance of polyphenols and flavonoids in our extracts, the apparent lower antibacterial activity in comparison to the reference antibiotic Norfloxacin may be linked to restricted solubility, especially for silymarin with low water solubility, and this challenge was addressed by directly blending the extract with agar medium, ensuring even distribution and optimal interaction with bacteria according toAbed et al. (2015) who employed two methods and found that mixing the extract with agar medium ensures uniform distribution and effective contact with bacteria for enhanced efficacy (Abed et al., 2015). Shah et al. (2014) reported in their investigation that the silymarin extract from the blue and white capitulum seeds of milk thistle exhibited no inhibitory effect against Gram-negative bacteria but demonstrated significant activity against Gram-positive bacteria. The antibacterial activity varies depending on the plant part. We noticed that Gram-positive bacteria (S. aureus, S. epidermidis, and MRSA) exhibited larger inhibition zones (7.50–8.00 mm) compared to Gram-negative bacteria (K. pneumoniae, E. coli, and A. baumannii), which showed smaller inhibition zones (6.17–6.50 mm), with E. cloacae showing no inhibition. This suggests that milk thistle seed extract has a stronger effect on Gram-positive bacteria which can be attributed to silymarin with its major component silybin. A study showed that silybin, the major component of silymarin, is about 30 times more effective than silymarin against B. subtilis and S. epidermidis. Its antibacterial effect is likely due to the inhibition of RNA and protein synthesis rather than disruption of the bacterial membrane (Lee and Liu, 2003).
Stems were largely ineffective, showing no inhibition against most bacterial strains. This could be attributed to a lower concentration of bioactive compounds in the stems compared to seeds and leaves, which may have limited antibacterial potential.
Abdul Kareem and Dhahir conducted a study on both oil and ethanol extract from milk thistle seeds, testing them against various bacteria. The results indicated a lack of antibacterial activity against all tested bacteria, with the oil demonstrating only a mild effect against E. ludwigii, K. pneumonia, and P. gaviniae (Abdul et al., 2019). Yılmaz et al., in their study, similarly found no antibacterial activity present in the extract of Silybum marianum in the tested bacteria including S. aureus, B. cereus, E. coli, and S. typhimurium (Yılmaz et al., 2022). Guemari et al. observed that the methanolic extract from leaves exhibited no inhibition zone against Escherichia coli, while inhibition zones of 15 mm, 13 mm, and 10 mm were, respectively, noted for flowers, twigs, and seeds (Guemari et al., 2020). Bajwa et al. (2016) found that dimethylformamide extract of the aerial part of Silybum marianum was the most active against MRSA with an inhibition zone of 7.6 mm; the other extracts such as isopropyl alcohol extract, methanol, and dichloromethane showed no inhibition zone (Bajwa et al., 2016).
Table 3 presents the Minimal Inhibitory Concentration MIC and Minimum Bactericidal Concentration MBC of milk thistle seeds extract determined using the serial dilution method. The extract showed a minimum inhibitory effect against Staphylococcus epidermidis at 10 μL/mL, with the same concentration also exhibiting a bactericidal effect. In contrast, Staphylococcus aureus and MRSA had MIC values above 10 μL/mL, and no bactericidal effect was observed. These findings indicate that S. epidermidis was more sensitive, while S. aureus and MRSA were more resistant.
Table 3. Minimal method inhibitory concentration MIC and minimum bactericidal concentration MBC of milk thistle seeds extract.
The diverse composition of bacterial cell walls, varying across species, plays a role in influencing how effectively bioactive compounds can permeate and disrupt cellular functions. The nature of bacterial cell walls, varying among species, can influence the ability of bioactive compounds to penetrate and disrupt cellular functions. Additionally, the specific composition of our extracts, including the type of phenolic structures present, may interact differently with distinct bacterial strains. The type of phenolic structure holds greater significance than concentration. This emphasizes the need for understanding structure–activity correlations within the phenolic compounds. Further exploration is warranted, especially through additional studies involving diverse solvents and extraction methods.
The GC–MS analysis of the plant extracts from different parts of milk thistle provided detailed insights into their respective phytochemical profiles (Table 3). The extracts exhibit diverse chemical compositions. The occurrence of several common compounds across all three plant sections is notable. Diethyl phthalate has been found in seeds, leaves, and stems, showing that it is present throughout the plant. Phthalates are esters of phthalic acid (Roy, 2020). In fact, compounds of the phthalate ester are commonly known as plasticizers. Were once thought to be solely industrial contaminants. However, research now shows that these compounds are produced by various organisms, including plants, bacteria, fungi, and animals. Currently, 52 phthalate derivatives have been identified from natural sources (Roy, 2020). Diethyl phthalate, along with hydroxy-4-methoxybenzoic acid and oleic acid, was identified as a major bioactive compound in the potent antibacterial fraction of A. officinalis through GC–MS analysis, highlighting its key role in the antibacterial activity (Valentin Bhimba et al., 2010). Tariqul Islam et al. isolated three compounds from hairy vetch shoots: bis(2-ethylhexyl) phthalate, diethyl phthalate, and p-hydroxybenzoic acid, all of which demonstrated potential antibacterial activity (Islam et al., 2013). Diethyl phthalate and phthalic acid esters were identified as the primary constituents in extracts from the ethanolic stem of Mallotus philippensis (Lam.) var. philippensis and in the essential oil of Leea indica (Burm.) and found to have a potent antibacterial activity (Srinivasan et al., 2009; Velanganni et al., 2011).
Glycerol 1,2-diacetate is found in seeds and stems, indicating a similar chemical feature between these plant components. Glycerol-1,2-diacetate was detected in the methanolic extract of S. anquetilia and Ziziphus mauritiana leaves, as well as Penicillium and in the ethanolic leaves of Hanguana Malayana (Nabi et al., 2022; El-Sayed et al., 2023). Glycerol 1,2-diacetate is employed as a flavoring agent and as an additive in food products (Adebayo et al., 2018). It have been showed to have antimicrobial activity by inhibiting DNA gyrase and interacting with essential amino acids like Arg78, Glu79, and Thr167 (El-Sayed et al., 2023). Triacetin shows significant presence in seeds, and 2-Acetyl-1-tetralone dominates in leaves. 1-Pentadecyne is exclusively found in leaves, whereas 5-Acetoxymethyl-2-furaldehyde is notably present in leaves as well. Naphthalene, 2-methoxy-, stands out in stems, along with Hexanedioic acid, bis(2-ethylhexyl), and Ester Bis(2-ethylhexyl) phthalate. A minimal amount of 2,6-bis(1-methylpropyl) phenol was found in the leaves while an unidentified compound was detected in the stems. 1-Pentadecyne was found in the methanolic extract of Momordica charantia (Ritu et al., 2012) and in the hexane leaves of Hyptis verticillata and is found as a major component among other components in the stem essential oil of Leptoderris micrantha (Oloyede et al., 2022). It has been reported to have antibacterial activity (Kumar et al., 2011) and several other pharmacological activity (Tongkasee et al., 2023). In a study conducted by Hin Chow et al. it was found that 5-Acetoxymethyl-2-furaldehyde specifically decrease cell migration in cell lines that exhibit high levels of aquaporin-1 (Chow et al., 2020). Hexanedioic acid, bis(2-ethylhexyl) ester was reported to be the predominant compound in the methanolic flower extract of Bergenia ciliata (Ferdosi et al., 2021). It has been shown to exert antidiabetic, anti-inflammatory, anticancer and diuretic potentials (Ihegboro et al., 2024). The compounds identified in the Silybum marianum extracts differ from those found by Javeed et al. in their study on the methanolic Silybum marianum from Pakistan. However, the phthalate esters has been also identified in seeds, leaves and stems (Javeed et al., 2022).
The varying quantities of individual chemicals across different plant parts contribute to unique chemical profiles. In seeds, 2-Acetyl-1-tetralone is predominantly detected, while leaves are rich in 5-Acetoxymethyl-2-furaldehyde. However, the key compound in stems remains unknown. These differences in chemical composition among different parts of the plant highlight the unique metabolic characteristics of seeds, leaves, and stems, showcasing the diversity present in each component (Table 4).
The HPLC analysis identified several key bioactive compounds. By analyzing the mass spectra in both negative and positive modes, we were able to identify a range of molecules based on the observed molecular peaks, as well as the findings reported by (Wallace et al., 2003, 2007). The profiles revealed that the seeds contained the highest concentration of silymarin, including its major isomers silybin A and silybin B, along with isosilybin A, isosilybin B, silydianin, silychristin A, silychristin B, and taxifolin (Table 5 and Figure 4). These compounds are well-known for their antioxidant and hepatoprotective properties. In contrast, the extracts exhibited a variety of phenolic compounds. Pyrogallol and glucose were detected in all parts of the plant, while vanillin was present in both seeds and leaves. Gallic acid was identified in seeds and stems, with stems containing it as the major component, whereas it was not present in Tunisian milk thistle (Maaloul et al., 2024). Additionally, apigenin and Piceatannol were detected in the leaves and stems. Caffeic acid was exclusively found in the stems, while eriodyctiol was detected only in the seeds.
Milk thistle seeds, leaves, and stems shows interesting variations in phytochemical profiles and antioxidant activity. Notably, during the mid-flowering stage, the leaves displayed the greatest abundance of both phenolic and flavonoid compounds along with antioxidant activity. In contrast, the stems showed the lowest content of phenolic compounds, and the mature seeds exhibited the lowest content of flavonoids, with no observable antioxidant activity in both tests. The closeness in phenolic content between seeds and leaves suggests that both parts could be valuable sources of these beneficial compounds. The extracts displayed moderate antibacterial activity and no synergistic effect or enhanced activity observed when the different parts were combined. Among the different parts, seeds showed the highest inhibition zone. Notably E. cloacae did not exhibit any inhibitory effect against any of the extracts or the combined mixture. The stems showed inhibition only against K. pneumoniae. The GC–MS and HPLC-UV-MS analyses revealed the presence of various bioactive compounds, in the extracts. These compounds are known for their antioxidant and pharmacological properties, suggesting their potential contribution to the biological activities of the plant. Additional investigations are essential to elucidate the antibacterial properties of diverse plant parts and their combined effect, employing a variety of solvents and extraction methods. This comprehensive approach will contribute valuable insights into optimizing the extraction process for enhancing antibacterial efficacy.
The original contributions presented in the study are included in the article/supplementary material, further inquiries can be directed to the corresponding author/s.
OI: Conceptualization, Data curation, Formal analysis, Methodology, Writing – original draft. MA: Conceptualization, Formal analysis, Writing – review & editing. AO: Methodology, Writing – review & editing. HI: Data curation, Formal analysis, Methodology, Writing – review & editing. ON: Formal analysis, Resources, Writing – review & editing. RM: Formal analysis, Resources, Writing – review & editing. MT: Data curation, Resources, Writing – review & editing. YT: Formal analysis, Writing – review & editing. AH: Conceptualization, Supervision, Writing – review & editing.
The author(s) declare that financial support was received for the research and/or publication of this article. This study was funded by the King Saud University, researchers supporting project number (RSP2025R119), King Saud University, Riyadh, Saudi Arabia.
The authors extend their appreciation to the King Saud University, Researchers Supporting Project number (RSP2025R119), King Saud University, Riyadh, Saudi Arabia.
The authors declare that the research was conducted in the absence of any commercial or financial relationships that could be construed as a potential conflict of interest.
The authors declare that no Gen AI was used in the creation of this manuscript.
All claims expressed in this article are solely those of the authors and do not necessarily represent those of their affiliated organizations, or those of the publisher, the editors and the reviewers. Any product that may be evaluated in this article, or claim that may be made by its manufacturer, is not guaranteed or endorsed by the publisher.
Abdul, K., Dhahir, W., and Abid Ali, W. (2019). In -vitro evaluation of antibacterial activity of Silybum marianum seeds oil, ethanolic extract and antibiotics against isolated bacteria. BEST: International Journal of Humanities, Arts, Medicine and Sciences.
Abed, I. J., Al-Moula, R., and Abdulhasan, G. A. (2015). Antibacterial effect of flavonoids extracted from seeds of Silybum marianum against common pathogenic Bacteria. World J Exp Biosci 3, 36–39. doi: 10.13140/RG.2.1.4632.6240
Abenavoli, L., Izzo, A. A., Milić, N., Cicala, C., Santini, A., and Capasso, R. (2018). Milk thistle (): A concise overview on its chemistry, pharmacological, and nutraceutical uses in liver diseases. Phytother. Res. 32, 2202–2213. doi: 10.1002/ptr.6171
Adebayo, I. A., Arsad, H., and Samian, M. R. (2018). Total Phenolics, Total flavonoids, antioxidant capacities, and volatile compounds gas chromatography-mass spectrometry profiling of Moringa oleifera ripe seed polar fractions. Pharmacogn. Mag. 14, 191–194. doi: 10.4103/pm.pm_212_17
Ahmad, N., Fazal, H., Abbasi, B. H., Anwar, S., and Basir, A. (2013). DPPH free radical scavenging activity and phenotypic difference in hepatoprotective plant (Silybum marianum L.). Toxicol. Ind. Health 29, 460–467. doi: 10.1177/0748233712436637
Ali, B., Elsayed, A., Doheem, M., Eita, A., and Omar, A. (2020). Effect of Milk thistle (Silybum marianum (L.) Gaertn) seed extract on bacterial activities and growth of human liver Cancer cells. J. Biotechnol. Res., 27–33. doi: 10.32861/jbr.65.27.33
Ammar, R. B., Bhouri, W., Sghaier, M. B., Boubaker, J., Skandrani, I., Neffati, A., et al. (2009). Antioxidant and free radical-scavenging properties of three flavonoids isolated from the leaves of Rhamnus alaternus L. (Rhamnaceae): A structure-activity relationship study. Food Chem. 116, 258–264. doi: 10.1016/j.foodchem.2009.02.043
Aouji, M., Imtara, H., Rkhaila, A., Bouhaddioui, B., Alahdab, A., Parvez, M. K., et al. (2023). Nutritional composition, fatty acids profile, mineral content, antioxidant activity and acute toxicity of the flesh of Helix aspesra Müller. Molecules 28:6323. doi: 10.3390/molecules28176323
Asif, M. (2015). Chemistry and antioxidant activity of plants containing some phenolic compounds. Vol 1. p. 35–52. Available at: https://www.semanticscholar.org/paper/Chemistry-and-antioxidant-activity-of-plants-some-Asif/38b1b5f39ff0d2775acff1c9133a6bf9fd1f5888 (Accessed February 6, 2025).
Aspé, E., and Fernández, K. (2011). The effect of different extraction techniques on extraction yield, total phenolic, and anti-radical capacity of extracts from Pinus radiata bark. Ind. Crop. Prod. 34, 838–844. doi: 10.1016/j.indcrop.2011.02.002
Aziz, M., Saeed, F., Ahmad, N., Ahmad, A., Afzaal, M., Hussain, S., et al. (2021). Biochemical profile of milk thistle (Silybum Marianum L.) with special reference to silymarin content. Food Sci. Nutr. 9, 244–250. doi: 10.1002/fsn3.1990
Bajwa, A., Tariq, S., Yuchi, A., Hafeez, R., Arshad, A., Zaman, M., et al. (2016). Evaluation of anti-bacterial activity of Silybum marianum against pathogenic and resistant Bacteria. Eur. J. Med Plants 13, 1–7. doi: 10.9734/EJMP/2016/24732
Begum, S. A., Sahai, M., Ray, A. B., Gössinger, E., Luzhetska, M., and Härle, J. (2010). “Non-conventional Lignans: Coumarinolignans, Flavonolignans, and Stilbenolignans” in Fortschritte der Chemie organischer Naturstoffe / Progress in the chemistry of organic natural products. eds. M. Sahai, E. Gössinger, M. Luzhetska, J. Härle, S. A. Begum, and A. B. Ray, et al. vol. 93 (Vienna: Springer), 1–70.
Benzie, I. F. F., and Choi, S.-W. (2014). Antioxidants in food: content, measurement, significance, action, cautions, caveats, and research needs. Adv. Food Nutr. Res. 71, 1–53. doi: 10.1016/B978-0-12-800270-4.00001-8
Biedermann, D., Vavříková, E., Cvak, L., and Křen, V. (2014). Chemistry of silybin. Nat. Prod. Rep. 31, 1138–1157. doi: 10.1039/C3NP70122K
Candan, F., Unlu, M., Tepe, B., Daferera, D., Polissiou, M., Sökmen, A., et al. (2003). Antioxidant and antimicrobial activity of the essential oil and methanol extracts of Achillea millefolium subsp. millefolium Afan. (Asteraceae). J. Ethnopharmacol. 87, 215–220. doi: 10.1016/s0378-8741(03)00149-1
Chow, P. H., Kourghi, M., Pei, J. V., Nourmohammadi, S., and Yool, A. J. (2020). 5-Hydroxymethyl-furfural and structurally related compounds block the ion conductance in human Aquaporin-1 channels and slow Cancer cell migration and invasion. Mol. Pharmacol. 98, 38–48. doi: 10.1124/mol.119.119172
Daglia, M. (2012). Polyphenols as antimicrobial agents. Curr. Opin. Biotechnol. 23, 174–181. doi: 10.1016/j.copbio.2011.08.007
Efenberger-Szmechtyk, M., Nowak, A., and Czyzowska, A. (2021). Plant extracts rich in polyphenols: antibacterial agents and natural preservatives for meat and meat products. Crit. Rev. Food Sci. Nutr. 61, 149–178. doi: 10.1080/10408398.2020.1722060
Eldalawy, R., Al-Ani, W. M. K., and Abdul Kareem, W. (2021). Phenotypic, anatomical and phytochemical investigation of Iraqi Silybum marianum. J. Phys. Conf. Ser. 1879:022029. doi: 10.1088/1742-6596/1879/2/022029
El-Sayed, H., Hamada, M. A., Elhenawy, A. A., Sonbol, H., and Abdelsalam, A. (2023). Acetylcholine esterase inhibitory effect, antimicrobial, antioxidant, Metabolomic profiling, and an in silico study of non-polar extract of the halotolerant marine fungus Penicillium chrysogenum MZ945518. Microorganisms 11:769. doi: 10.3390/microorganisms11030769
Elshafie, H. S., Camele, I., and Mohamed, A. A. (2023). A comprehensive review on the biological, agricultural and pharmaceutical properties of secondary metabolites based-plant origin. Int. J. Mol. Sci. 24:3266. doi: 10.3390/ijms24043266
Farhadi, F., Khameneh, B., Iranshahi, M., and Iranshahy, M. (2019). Antibacterial activity of flavonoids and their structure-activity relationship: an update review. Phytother. Res. 33, 13–40. doi: 10.1002/ptr.6208
Ferdosi, M. F. H., Khan, I. H., Javaid, A., Saeed, H. M., Butt, I., and Munir, A. (2021). GC-MS analysis profile and bioactive components of flowers of bergenia ciliata, a weed of rock crevices. J. Weed Sci. Res. 27, 527–535. doi: 10.28941/pjwsr.v27i4.1012
Guemari, F., Laouini, S. E., Rebiai, A., and Bouafia, A. (2020). Phytochemical screening and identification of polyphenols, Evaluation of antioxidant activity and study of biological properties of extract Silybum marianum (L.). Asia. J. Rese. Chem. 13:190. doi: 10.5958/0974-4150.2020.00037.1
Haida, S., Kribii, A., Daoud, N. A., Belakhmima, R. A., and Kribii, A. (2022). Antioxidant activity of Haloxylon scoparium alkaloid extracts from Figuig region (southeastern of Morocco). Braz. J. Pharm. Sci. 58:e19494. doi: 10.1590/s2175-97902022e19494
Ihegboro, G. O., Ononamadu, C. J., Owolarafe, T. A., Onifade, O., Udeh, J. J., Saliu, A. O., et al. (2024). In vitro investigation and GC-MS analysis of the chemical constituents in the fraction of hexane leaf extract of Tapinanthus bangwensis (Engl. and K. Krause) Loranthaceae. Tropical J. Phytochemistry Pharm. Sci. 3, 143–152. doi: 10.26538/tjpps/v3i1.5
Imane, O., Oubihi, A., Elbouzidi, A., Taibi, M., Jaber, H., Haida, S., et al. (2024). Phytochemical components, antioxidant properties, antimicrobial effects, and insecticidal prospects against the black bean aphid (Aphis fabae Scop.) of Olea europaea L. leaves extracts from Morocco. Journal of. Ecol. Eng. 25, 64–74. doi: 10.12911/22998993/177639
Islam, M. T., Ahn, S.-Y., Cho, S. M., and Yun, H. K. (2013). Isolation of antibacterial compounds from hairy vetch (Vicia villosa) against grapevine crown gall pathogen. Hortic. Environ. Biotechnol. 54, 338–345. doi: 10.1007/s13580-013-0028-8
Jaffar, H. M., Ambreen, S., Al-Asmari, F., Hussain, I., Rahim, M. A., Fawzy Ramadan, M., et al. (2024). Antioxidant activity, microbial viability, and sensory attributes of traditional-yogurt enriched with silymarin. Cogent Food Agriculture 10:2417838. doi: 10.1080/23311932.2024.2417838
Javeed, A., Ahmed, M., Sajid, A. R., Sikandar, A., Aslam, M., Hassan, T. U., et al. (2022). Comparative assessment of Phytoconstituents, antioxidant activity and chemical analysis of different parts of Milk thistle Silybum marianum L. Molecules 27:2641. doi: 10.3390/molecules27092641
Jomova, K., Raptova, R., Alomar, S. Y., Alwasel, S. H., Nepovimova, E., Kuca, K., et al. (2023). Reactive oxygen species, toxicity, oxidative stress, and antioxidants: chronic diseases and aging. Arch. Toxicol. 97, 2499–2574. doi: 10.1007/s00204-023-03562-9
Kang, H.-K., Kim, H.-Y., and Cha, J.-D. (2011). Synergistic effects between silibinin and antibiotics on Methicillin-resistant Staphylococcus aureus isolated from clinical specimens. Biotechnol. J. 6, 1397–1408. doi: 10.1002/biot.201000422
Koltai, T., and Fliegel, L. (2022). Role of Silymarin in Cancer treatment: facts, hypotheses, and questions. J Evid Based Integr Med 27:2515690X211068826. doi: 10.1177/2515690X211068826
Krishnaiah, D., Sarbatly, R., and Nithyanandam, R. (2011). A review of the antioxidant potential of medicinal plant species. Food Bioprod. Process. 89, 217–233. doi: 10.1016/j.fbp.2010.04.008
Kumar, V., Bhatnagar, A., and Srivastava, J. (2011). Antibacterial activity of crude extracts of Spirulina platensis and its structural elucidation of bioactive compound. J. Med. Plants Res. 5, 7043–7048. doi: 10.5897/JMPR11.1175
Lee, D. G., Kim, H. K., Park, Y., Park, S.-C., Woo, E.-R., Jeong, H. G., et al. (2003). Gram-positive bacteria specific properties of silybin derived Fromsilybum marianum. Arch. Pharm. Res. 26, 597–600. doi: 10.1007/BF02976707
Lee, D. Y.-W., and Liu, Y. (2003). Molecular structure and stereochemistry of silybin A, silybin B, isosilybin A, and isosilybin B, isolated from Silybum marianum (milk thistle). J. Nat. Prod. 66, 1171–1174. doi: 10.1021/np030163b
Maaloul, S., Mahmoudi, M., Mighri, H., Ghzaiel, I., Bouhamda, T., Boughalleb, F., et al. (2024). Tunisian Silybum species: important sources of polyphenols, organic acids, minerals, and proteins across various plant organs. Plan. Theory 13:989. doi: 10.3390/plants13070989
Manso, T., Lores, M., and Miguel, T.De. (2021). Antimicrobial activity of polyphenols and natural polyphenolic extracts on clinical isolates. Antibiotics 11:46. doi: 10.3390/antibiotics11010046.
Mansouri, F. E., Silva, J. C. G. E., Cacciola, F., Asraoui, F., Tayeq, H., Ben Amar, Y. M., et al. (2022). Evaluation of different extraction methods on the phenolic profile and the antioxidant potential of Ceratonia siliqua L. Pods Extracts. Molecules 27:6163. doi: 10.3390/molecules27196163
Marceddu, R., Dinolfo, L., Carrubba, A., Sarno, M., and Di Miceli, G. (2022). Milk thistle (Silybum Marianum L.) as a novel multipurpose crop for agriculture in marginal environments: A review. Agronomy 12:729. doi: 10.3390/agronomy12030729
Marmouzi, I., Bouyahya, A., Ezzat, S. M., El Jemli, M., and Kharbach, M. (2021). The food plant Silybum marianum (L.) Gaertn.: Phytochemistry, Ethnopharmacology and clinical evidence. J. Ethnopharmacol. 265:113303. doi: 10.1016/j.jep.2020.113303
Mhamdi, B., Abbassi, F., Smaoui, A., Abdelly, C., and Marzouk, B. (2016). Fatty acids, essential oil and phenolics composition of Silybum marianum seeds and their antioxidant activities. Pak. J. Pharm. Sci. 29, 953–959
Nabi, M., Zargar, M. I., Tabassum, N., Ganai, B. A., Wani, S. U. D., Alshehri, S., et al. (2022). Phytochemical profiling and antibacterial activity of methanol leaf extract of Skimmia anquetilia. Plan. Theory 11:1667. doi: 10.3390/plants11131667
Nowak, A., Florkowska, K., Zielonka-Brzezicka, J., Duchnik, W., Muzykiewicz, A., and Klimowicz, A. (2021). The effects of extraction techniques on the antioxidant potential of extracts of different parts of milk thistle (Silybum marianum L.). Acta Sci. Pol. Technol. Aliment. 20, 37–46. doi: 10.17306/J.AFS.0817
Oloyede, G., Ibok, M., Ojo, T., Leptoderris, D., and Dunn, M. (2022). Chemical constituents, antimicrobial and antioxidant activities of Leptoderris brachyptera (Benth.). Dunn Leptoderris Micrantha Dunn Essential Oils 5, 13–23. doi: 10.30495/tpr.2021.680481
Olszowy, M. (2019). What is responsible for antioxidant properties of polyphenolic compounds from plants? Plant Physiol. Biochem. 144, 135–143. doi: 10.1016/j.plaphy.2019.09.039
Oubihi, A., Ouryemchi, I., Nounah, I., Tarfaoui, K., Harhar, H., Ouhssine, M., et al. (2020). Chemical composition, antibacterial and antifungal activities of Thymus leptobotrys Murb essential oil. Adv. Tradit. Med. 20, 673–679. doi: 10.1007/s13596-020-00488-w
Panche, A. N., Diwan, A. D., and Chandra, S. R. (2016). Flavonoids: an overview. J. Nutr. Sci. 5:e47. doi: 10.1017/jns.2016.41
Rasouli, H., Farzaei, M. H., and Khodarahmi, R. (2017). Polyphenols and their benefits: A review. Int. J. Food Prop. 20, 1–42. doi: 10.1080/10942912.2017.1354017
Rezk, D. M., Enas, M. M., and Nadia, Y. E. (2024). Determination of physiochemical properties, amino acids, and other bioactive compounds of Silybum Marianum seeds (Silybum Marianum L.). Scient J. Agricult. Sci. 6, 205–216. doi: 10.21608/sjas.2024.269937.1391
Ritu, S., Kumar, A., Giri, D., and Pandey, K. (2012). Gas Chromatogrphy- mass spectrometry analysis and phytochemical screening of Methanolic fruit extract of Momordica charantia. J. Recent Adv. Agricult. 1, 122–127.
Roy, R. N. (2020). Bioactive natural derivatives of phthalate ester. Crit. Rev. Biotechnol. 40, 913–929. doi: 10.1080/07388551.2020.1789838
SAA (2017). Effect of Silymarin as natural antioxidants and antimicrobial activity. Nutr. Food Sci. Int. J. 2, 1–6. doi: 10.19080/NFSIJ.2017.02.555589
Savoia, D. (2012). Plant-derived antimicrobial compounds: alternatives to antibiotics. Future Microbiol. 7, 979–990. doi: 10.2217/fmb.12.68
Shah, S., Shah, M., Khan, F., Khan, S., and Muhammad, H.Shah, et al. (2014). Evaluation of Phytochemicals and Antimicrobial Activity of White and Blue Capitulum and Whole Plant of Silybum Marianum. World Appl Sci J. 12, 1139–1144.
Silva, N. C. C., and Fernandes Júnior, A. (2010). Biological properties of medicinal plants: a review of their antimicrobial activity. J. Venom. Anim. Toxins incl. Trop. Dis 16, 402–413. doi: 10.1590/S1678-91992010000300006
Srinivasan, G. V., Sharanappa, P., Leela, N., Sadashiva, C. T., and Vijayan, K. (2009). Chemical composition and antimicrobial activity of the essential oil of Leea indica (Burm. F.) Merr. Flowers. Vol 5. p. 488–493. Available at: https://www.semanticscholar.org/paper/Chemical-composition-and-antimicrobial-activity-of-Srinivasan-Sharanappa/6bb327d4bf65978e989b6836ed0c53617228f026 (Accessed February 6, 2025).
Sun, J., Li, X. H., and Yu, X. L. (2016). Antioxidant activities, Total flavonoids and Phenolics content in different parts of Silybum Marianum L. Plants. Chem. Eng. Transact. 55, 37–42. doi: 10.3303/CET1655007
Tabart, J., Kevers, C., Pincemail, J., Defraigne, J.-O., and Dommes, J. (2009). Comparative antioxidant capacities of phenolic compounds measured by various tests. Food Chem. 113, 1226–1233. doi: 10.1016/j.foodchem.2008.08.013
Tongkasee, P., Srithat, D., Sriyasak, P., Jitcharerntham, A., Piwgern, T., Khoontawad, J., et al. (2023). Formulation and phytochemical profile of a product prototype infused with Cannabis sativa leaves. Trends Sci. 20:5835. doi: 10.48048/tis.2023.5835
Valentin Bhimba, B., Meenupriya, J., Joel, E. L., Naveena, D. E., Kumar, S., and Thangaraj, M. (2010). Antibacterial activity and characterization of secondary metabolites isolated from mangrove plant Avicennia officinalis. Asian Pac J Trop Med 3, 544–546. doi: 10.1016/S1995-7645(10)60131-9
Velanganni, J., Kadamban, D., and Chanemougame, T. (2011). Phytochemical screening and antimicrobial activity of the stem of mallotus philippensis (lam.) Muell. Arg. Var. philippensis (Euphorbiaceae). Int J Pharm Pharm Sci 3, 160–163.
Wallace, S., Carrier, D. J., Beitle, R. R., Clausen, E. C., and Griffis, C. L. (2003). HPLC-UV and LC-MS-MS characterization of Silymarin in Milk thistle seeds and corresponding products. J. Nutraceuticals Functional Medical Foods 4, 37–48. doi: 10.1300/J133v04n02_05
Wallace, S. N., Raible, J., Carrier, D. J., Vaughn, K. L., Griffis, C. L., Clausen, E. C., et al. (2007). Pressurized water versus ethanol as a Silybum marianum extraction solvent for inhibition of low-density lipoprotein oxidation mediated by copper and J774 macrophage cellsThis article is one of a selection of papers published in this special issue (part 1 of 2) on the safety and efficacy of natural health products. Can. J. Physiol. Pharmacol. 85, 894–902. doi: 10.1139/Y07-058
Yang, C.-H., Li, R.-X., and Chuang, L.-Y. (2012). Antioxidant activity of various parts of Cinnamomum cassia extracted with different extraction methods. Molecules 17, 7294–7304. doi: 10.3390/molecules17067294
Yılmaz, R., Çalık, H., Ay, H. F., Erci, F., and Koç, R. Ç. (2022). Anticancer, antibacterial and antioxidant activity of Urtica dioica, Silybum marianum and Cynara scolymus extracts. Tüseb Dergisi 5, 51–62. doi: 10.54537/tusebdergisi.1089792
Keywords: milk thistle, antioxidant activity, GC–MS, antibacterial activity, HPLC-MS-UV
Citation: Iraqi O, Aouji M, Oubihi A, Imtara H, Noman OM, Mothana RA, Tarayrah M, Taboz Y and Habsaoui A (2025) Exploring the chemical composition, antioxidant, and antibacterial properties of milk thistle (Silybum marianum L.). Front. Sustain. Food Syst. 9:1551584. doi: 10.3389/fsufs.2025.1551584
Received: 25 December 2024; Accepted: 26 February 2025;
Published: 19 March 2025.
Edited by:
Anka Trajkovska Petkoska, University St. Clement of Ohrid, North MacedoniaReviewed by:
Hassan Laaroussi, Sidi Mohamed Ben Ab-dellah University, MoroccoCopyright © 2025 Iraqi, Aouji, Oubihi, Imtara, Noman, Mothana, Tarayrah, Taboz and Habsaoui. This is an open-access article distributed under the terms of the Creative Commons Attribution License (CC BY). The use, distribution or reproduction in other forums is permitted, provided the original author(s) and the copyright owner(s) are credited and that the original publication in this journal is cited, in accordance with accepted academic practice. No use, distribution or reproduction is permitted which does not comply with these terms.
*Correspondence: Hamada Imtara, aGFtYWRhLnRhcmF5cmFoQGdtYWlsLmNvbQ==
Disclaimer: All claims expressed in this article are solely those of the authors and do not necessarily represent those of their affiliated organizations, or those of the publisher, the editors and the reviewers. Any product that may be evaluated in this article or claim that may be made by its manufacturer is not guaranteed or endorsed by the publisher.
Research integrity at Frontiers
Learn more about the work of our research integrity team to safeguard the quality of each article we publish.