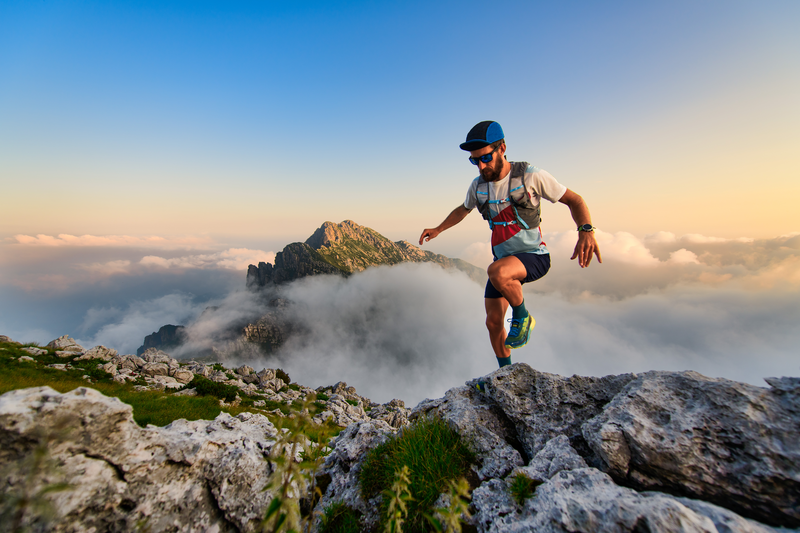
95% of researchers rate our articles as excellent or good
Learn more about the work of our research integrity team to safeguard the quality of each article we publish.
Find out more
ORIGINAL RESEARCH article
Front. Sustain. Food Syst. , 19 February 2025
Sec. Nutrition and Sustainable Diets
Volume 9 - 2025 | https://doi.org/10.3389/fsufs.2025.1520875
This article is part of the Research Topic Mushrooms as Functional Foods and Nutraceuticals View all 5 articles
Pholiota nameko (P. nameko) was identified as an edible mushroom known for its abundance of bioactive compounds. Previous studies suggested that extracts from this mushroom possess antioxidant properties attributed to the presence of phenolic acids, such as 4-hydroxybenzoic acid (4-HBA) and cinnamic acid (CA). These compounds show promise in potentially ameliorating insulin resistance. In this study, the highest levels of 4-HBA and CA were selected from six P. nameko extracts using ultra-high-pressure liquid chromatography (UHPLC) to investigate whether they could improve the glucose uptake resistance induced by palmitate in C2C12 myotubes. Additionally, the protein expression of GLUT4 was examined. The results indicated that P. nameko hot water extract (PN-HW) exhibited the highest total polyphenol content (9.34 ± 0.31 mg GAE/g) and demonstrates strong free radical scavenging ability (77.4 ± 1.1%). Furthermore, PN-HW contains notable amounts of 4-HBA (4.02 ± 0.17 mg/g DW) and CA (2.01 ± 0.09 mg/g DW). Importantly, the cell viability reveals that PN-HW extracts did not have a toxic effect on C2C12 myotubes. PN-HW significantly enhances glucose uptake at doses of 25 μg/mL. Hence, it was suggested that PN-HW might contain elevated levels of phenolic acids, potentially mitigating impaired glucose uptake caused by high-fat content and consequently enhancing the glucose uptake capacity of C2C12 cells.
Type 2 diabetes mellitus (T2DM) is a chronic metabolic disorder characterized by continuously elevated blood glucose levels majorly contributed by insulin resistance (IR) (Westman, 2021). Pathophysiology of IR is generally initiated through the free fatty acids, oxidative stress and pro-inflammatory cytokines and thus it contributed to a global prevalence of 10.5% (536.6 million people) in 2021 (Yan et al., 2022). According to the International Diabetes Federation Diabetes Atlas (IDFDA), the prevalence of T2DM is projected to reach its highest point by 2045, affecting approximately 10.90% of the global population, which corresponds to around 700 million individuals (Saeedi et al., 2019). Consequently, 60% of people with diabetes live in Asia, in which Taiwan has a diabetes prevalence rate of 9.82% (Nanditha et al., 2016; Lin C. L. et al., 2022). Currently, diabetes management primarily relies on oral medications and insulin therapy (Widyahening et al., 2022), which may have limitations and side effects. Therefore, there is increasing interest in investigating plant-based natural products to control blood glucose levels and reduce the risk of diabetes-related complications (Usai et al., 2022).
“One meat, one vegetable, one mushroom” has become a new trend in health eating, leading to a steady increase in global mushroom production and cultivation area (Zhao, 2023). Pholiota nameko (T.Itô) S.Ito & S.Imai is an edible mushroom extensively cultivated in Asian countries, including China and Japan (Lin H. et al., 2022). P. nameko, commonly referred to as the ‘nameko mushroom,’ is generally categorized as a macrofungus. In addition to its delicious taste and nutritional value, P. nameko is believed to have various pharmacological activities, including antioxidant activities of polysaccharides, anti-inflammatory, and hypoglycemic effects (Chou et al., 2019). Emerging evidence from several studies indicates that certain types of edible mushrooms, particularly P. nameko, hold promise as natural remedies for both the prevention and treatment of diabetes. These findings highlight the potential of edible mushrooms as a valuable resource on T2DM treatment (Das et al., 2022). A study by Sung et al. revealed P. nameko antioxidant effects in H2O2-induced L929 cells, derived from mouse embryonic fibroblasts, implying its potential impact on wound healing through antioxidative properties (Sung et al., 2020). Moreover, significant antioxidant and antidiabetic properties of mushrooms may be primarily attributed to phenolic acids, such as 4-hydroxybenzoic acid (4-HBA) and cinnamic acid (CA) (Nowacka et al., 2014). Moreover, P. nameko contains the tumor-suppressive protein PNAP, inducing apoptosis via caspase-9 and caspase-3 pathways (Zhang et al., 2014). Additionally, P. nameko phosphorylated polysaccharides offer antioxidant and anti-inflammatory properties, suitable for incorporation into functional foods and pharmaceuticals (Zhang et al., 2022).
However, it is important to note that early research did not report the impact of P. nameko polyphenols on high-fat-induced glucose resistance in muscle cells (Das et al., 2022). Kuo et al. (2016) indicated that the primary active component, antcin K, a type of triterpenoid, in Antrodia camphorata exhibits antidiabetic effects primarily through the activation of the AMP-activated protein kinase (AMPK) pathway, leading to an increase in glucose transporter 4 (GLUT4) protein expression in C2C12 cells. GLUT4 is primarily expressed in skeletal muscle either through the AMP-activated protein kinase (AMPK) signaling pathway or by facilitating insulin-stimulated glucose uptake via the phosphoinositide-3-kinase/ protein kinase B (PI3K/Akt) cascade (Takazawa et al., 2008; Wong et al., 2020). Therefore, this study utilized alcohol and hot water extraction methods to screen for P. nameko extracts with high polyphenol content and strong free radical scavenging properties, which were then used as interventions in a cellular model of palmitate-induced insulin resistance in C2C12 cells to investigate their mechanisms in relation to GLUT4 protein expression and improvement of glucose utilization.
P. nameko edible mushrooms were purchased from Fengnian Farm in Nantou County, Taiwan during October 2021. According to supplier, these edible mushrooms were cultivated at room temperature with 85% humidity. The mushroom strain originated from the bioresource collection and research center (BCRC) in Hsinchu, with the identification number MU30518.
The extraction of P. nameko was carried out using two approaches: (1) alcohol extraction and (2) hot water extraction. The alcohol extraction method was performed based on our previous study (Shyur et al., 2021) with minor modifications. Briefly, P. nameko mushroom (10 g) was subjected to liquid nitrogen treatment to facilitate the powder formation process. The powdered sample was then mixed with 300 mL of ethanol and incubated for 12 h. The reaction mixture was subsequently centrifuged under reduced pressure to obtain a total volume of 30 mL. The resulting mixture was freeze-dried and labelled as P. nameko ethanol extract (PN-EE). In second step, a bilayer extraction process was carried out by dissolving freeze dried PN-EE with 10 mL of ethyl acetate. The upper layer was separated and labelled as P. nameko ethyl acetate extract (PN-EA), while the bottom layer was labelled as P. nameko water layer (PN-EW). Additionally, all the samples were freeze dried to measure the final yield, which was found to be 14% for PN-EE, 2.8% for PN-EA, and 5.1% for PN-EW.
The hot water extraction of P. nameko was based on a modified method based on a published previously (Yang et al., 2020). The process involves weighing 10 g of dry P. nameko and adding it to 300 mL of deionized water, homogenizing, and then stirring at 80°C for 8 h. After cooling to room temperature and centrifugation, the P. nameko hot water extract (PN-HW) is obtained. Four times the volume of anhydrous ethanol is added to PN-HW, and the mixture is stirred at 4°C for 24 h to obtain the alcohol-soluble layer (P. nameko alcohol soluble, PN-HWA) and the alcohol-insoluble substance (P. nameko non-alcohol soluble, PN-HWNA). PN-HWNA is then washed three times with 80% ethanol solution, redissolved in deionized water, and proteins are removed using Sevag reagent (chloroform: n-butanol (v/v) = 4:1). The mixture is then dialyzed using a 3,500 Da dialysis bag and freeze-dried for use. The yields are 20.8% for PN-HW, 14.4% for PN-HWA, and 2.5% for PN-HWNA. The preparation of extracts from 6 different P. nameko samples is shown in Figure 1.
Total phenolic content of six different PN extracts were determined according to Truong et al. (2007) with modification. Gallic acid was employed as a reference standard (20 to 100 mg/L). A sample (0.50 mL) was mixed with 0.5 mL of Folin–Ciocalteu reagent and 50 μL of 10% sodium bicarbonate. The mixture was incubated at room temperature for 1 h, after which optical density (OD) values were measured at 735 nm using a plate reader. The results were expressed as milligrams of gallic acid equivalent (GAE) per gram of dry weight (mg GAE/g DW). Gallic acid was used as a reference standard (20 to 100 mg/L).
Candidate phenolic compounds were identified via ultra-performance liquid chromatography (UPLC), following Jeong et al. (2020) protocol with minor adaptations. An UPLC system (Waters Corp., Milford, MA, United States) was utilized to analyze the six P. nameko extracts. Standard curves for 4-HBA and CA were generated, spanning concentrations from 100 to 0.8 μg/mL for 4-HBA and 25 to 0.2 μg/mL for CA, and stored at 20°C for subsequent use. Chromatographic separation was conducted on a BEH C18 column (2.1 × 100 mm, 1.7 μm), maintained at 40°C. The mobile phase comprised Solvent A (deionized water) and Solvent B (acetonitrile with 0.1% formic acid). The flow rate was set at 0.4 mL/min. The chromatographic process commenced with a 99% Solvent A gradient at 0 min. Between 0 and 5 min, the gradient transitioned from 99 to 70% Solvent A. From 5 to 6 min, it shifted from 70 to 40% Solvent A. Subsequently, between 6 and 7 min, the gradient decreased from 40 to 1% Solvent A. From 7 to 8.5 min, it increased from 1 to 99% Solvent A, concluding at 10 min by returning to the initial 99% Solvent A gradient. Absorption spectra were recorded using a PDA detector set at 280 nm. The peaks for 4-HBA and CA were identified based on their retention times and UV absorption spectral characteristics, which were compared with those of the standards and verified against the established standard curves.
The free radical scavenging ability was determined following the methodology published previously (Shyur et al., 2021). Briefly, 200 μL of DPPH (0.5 mM in methanol) solution were added to the sample. The mixture was vigorously shaken and left to stand for 30 min at room temperature in the dark. Also, standard was implemented using ascorbic acid at a concentration of 0.078–10 mg/mL. The absorbance of sample was determined at 517 nm using a plate reader. The inhibition percentage of DPPH discoloration was calculated using the following scavenging effect (%) = (1 − ASample (517 nm)/AControl (517 nm)) × 100.
Following Xiong et al.'s experimental method (Amine et al., 2021) with adjustments, a 10 mM sodium palmitate solution (Sigma Aldrich, St. Louis, USA) was prepared using deionized water (Sawada et al., 2012). The solution (10 mL) was dissolved by agitation in a 70°C water bath for 1 h. Subsequently, 10 mL of BSA (Sigma Aldrich, St. Louis, USA) containing 150 mM NaCl was gradually added and thoroughly mixed. This yielded the palmitic solution, which was filtered through a 0.45 μm sterile membrane and stored in brown Eppendorf tubes at −20°C for use within 2 weeks.
C2C12 cells were cultured in DMEM (Sigma Aldrich, St. Louis, USA) supplemented with 10% FBS, 100 units/mL penicillin, and 100 μg/mL streptomycin, maintaining the culture medium pH at 7.2–7.4. The cells were incubated in a CO2 incubator (NUAIRE, MN, USA) at 37°C with 5% CO2. To induce differentiation into myotubes, cells at 80% confluence were switched to high-glucose DMEM supplemented with 2% horse serum (Sigma Aldrich, St. Louis, USA) and 2 mmol/L glutamine. Following complete differentiation, cells were treated with or without 1 mM palmitate, 500 nM insulin, and varying concentrations of PN-HW extract (6.25–400 μg/mL) for cellular interventions.
MTT assays were conducted with C2C12 myoblasts in a 96-well plate at a density of 10,000 cells/mL. PN-HW extracts (6.25 to 400 μg/mL) were added to fresh medium, and the cells were incubated for 24 h at 37°C. Subsequently, 20 μL of MTT solution (5 mg/mL) was added per well and further incubated for 3 h at 37°C. Afterward, 100 μL of DMSO was added, and the absorbance was measured at 570 nm.
For the glucose uptake assay, intracellular glucose uptake was assessed after 24 h of intervention using a 96-well plate and the Glucose Assay Kit-WST (Dojindo, Kumamoto, Japan), following the method described by Ishii et al. (2022). Cell lysates from C2C12 cells, obtained following various concentrations of PN-HW treatment with or without insulin supplementation, were prepared using 0.1% Triton-X-100 (Sigma Aldrich, St. Louis, United States) (50 μL per well). These lysates were mixed with an equal volume of glucose working solution. Glucose served as the standard within a concentration range of 0.5–0.00785 mmol/L, with concentrations determined by measuring absorbance at 450 nm using an ELISA reader (VERSA Max, Molecular Devices, USA).
After 24 h of intervention, C2C12 cells were homogenized in a modified RIPA buffer (0.5 M Tris–HCl at pH 7.4, 1.5 M sodium chloride, 2.5% deoxycholic acid, 10% NP-40, and 10 mM EDTA) and 10% protease and phosphatase inhibitor cocktail. The homogenates were centrifuged at 10,000 x g at 4°C for 15 min, and the supernatants was measured to perform BCA protein quantification. Finally, the extracted protein was adjusted to a concentration of 2 mg/mL for further analysis. To perform the western blot analysis, 40 μg of protein were combined with 5 x SDS-PAGE loading buffer, which contained 200 mM Tris–Cl (pH 6.8), 400 mM DTT, 7.5% SDS, 0.4% bromophenol blue, and 40% glycerol. The mixture was then heated in water for 10 min to denature the samples. The denatured samples were subjected to 10% sodium dodecyl sulfate–polyacrylamide gel electrophoresis and subsequently transferred onto a polyvinylidene difluoride transfer membrane. To block the membrane, it was incubated with 1% BSA in Tris-buffered saline with 0.1% Tween® 20 Detergent (TBST) (Sigma Aldrich, St. Louis, USA) for 1 h, followed by three washes with TBST. The membrane was incubated overnight at 4°C with diluted primary antibodies against GLUT4 (1:1000, EMD Millipore Corp, United States) and β-actin (1:5000, Proteintech, USA). β-actin was used as a loading control to ensure equal protein amounts in each lane. Following three washes with TBST, the membrane was incubated with goat anti-rabbit IgG or rabbit anti-goat IgG secondary antibodies for 1 h at room temperature. Following three times additional washes (10 min each), the binding of antibodies was detected using BCIP®/NBT tablet dissolved in deionized water. The band density was quantified using Bio-Rad Laboratories, Inc.’s Quantity One 1-D analysis software (Hercules, CA, United States).
Each experiment was conducted three times, and the results are presented as mean ± standard deviation (SD). Statistical analysis was performed using one-way ANOVA, followed by Tukey’s post-hoc test (version 19; SPSS Inc., Chicago, IL, USA). Differences were considered significant at a probability level of p < 0.05.
Among the extracts, PN-HW exhibited the highest scavenging activity at a concentration of 50 μg/mL, with a value of 77.4 ± 1.1% (Figure 2). Following PN-HW, PN-HWA, PN-EW, PN-HWNA, and PN-EE demonstrated scavenging activities of 59.9 ± 2.1%, 58.8 ± 1.5%, 54.7 ± 2.7%, and 47.2 ± 1.5%, respectively. The total polyphenol content was found to be highest in the hot water extract PN-HW (9.34 ± 0.31 mg/g GAE), followed by PN-EW (8.59 ± 0.3 mg/g GAE), PN-HWNA (7.22 ± 0.12 mg/g GAE), PN-HWA (7.2 ± 0.17 g/mg GAE), PN-EE (5 ± 0.17 g/mg GAE), and lowest in PN-EA (0.07 ± 0.002 g/mg GAE).
Figure 2. Relationship between radical-scavenging activity (%) and total phenolic content of different P. nameko extracts. Data are presented as the mean ± standard deviation (SD) of three independent determinations. PN-EE; P. nameko ethanol extract, PN-EA; P. nameko acetate extract; PN-EW; P. nameko water extract, PN-HW; P. nameko hot water extract, PN-HWA; P. nameko alcohol soluble, PN-HWNA; P. nameko non-alcohol soluble, GAE; gallic acid equivalent, DW; dry weight.
WSP-EA displayed the lowest scavenging activity, measuring 17.0 ± 0.8%. Notably, the scavenging activity of PN-HW was approximately more than 4.5 times higher than that of PNEA illustrates the correlation between the total phenolic content and the free radical scavenging activity. The data reveals a significant positive correlation (R2 = 0.933, p < 0.001), indicating that an increase in the total phenolic content corresponds to a higher free radical scavenging activity.
The UPLC analysis results utilizing 4-HBA and CA as candidates indicated that PN-EE possessed the highest 4-HBA content among the various extracts (Table 1). In contrast, the CA content was notably higher in the PN-HW extract. Consequently, when evaluating the combined phenolic acid content, encompassing both 4-HBA and CA, PN-HW demonstrated superiority compared to the other PN extracts. The phenolic content of 4-HBA and CA in PNHW was determined to be 6.03 ± 0.26 mg/100 g DW.
Table 1. Polyphenolic compound (4-HBA and CA) content of P. nameko extractsa.
The MTT assay was employed to evaluate the viability of C2C12 myoblasts after being exposed to various treatments using different concentrations of PN-HW extract for 24 and 48 h (Figure 3). The findings indicated insignificant difference (p > 0.05) in myotubes among the different treatment groups. This suggests that the PN-HW extract exhibited no toxicity even at a concentration of 400 μg/mL when tested on C2C12 muscle cells. There were no morphological changes in the microscopic images of cells before MTT measurement (data not shown).
Figure 3. C2C12 myotube viability assessed via the MTT assay following exposure to PN-HW 511 extract concentrations ranging from 6.25 to 400 μg/mL for 24 and 48 h at 37°C. Results are presented as mean ± standard deviation from ≥3 independent replicates, with error bars 513 indicating the standard deviation from the mean.
Our findings reveal that when C2C12 myotubes were treated with 1 mM palmitate for 24 h, it did not result in cell death (Figure 4A, No. 3–8), but significantly reduced glucose uptake (Figure 4B, No. 3). Furthermore, under conditions with palmitate but without insulin, the addition of different concentrations of PN-HW to C2C12 myotube cultures for 24 h did not increase intracellular glucose levels (data shown in Supplementary file 1). Interestingly, in the presence of insulin, PN-HW doses of 25 μg/mL significantly enhanced intracellular glucose content (Figure 4B, No. 5 and 6). However, higher concentrations of PN-HW resulted in a relative decrease in glucose uptake by C2C12 myotubes (Figure 4B, No. 7 and 8).
Figure 4. (A) C2C12 myotube morphology at 100X magnification observed with an inverted 528 microscope under different treatments. (B) Glucose uptake in C2C12 myotubes exposed to 529 varying PN-HW extract concentrations. Initially, C2C12 cells were differentiated into 530 myotubes in high-glucose DMEM with 2% horse serum. Insulin resistance was induced by a 531 24-h treatment with 1 mM palmitate. Results are presented as mean ± standard deviation 532 from >3 independent replicates, with error bars indicating standard deviations. Different 533 lowercase letters (a and b) above each bar denote significant differences (p < 0.05).
In the protein expression analysis, we investigated whether PN-HW at different concentrations (ranging from 6.25 to 400 μg/mL) could enhance glucose uptake by inducing the expression of GLUT4 protein (Figure 5A). Our observations revealed that PN-HW doses of 25 μg/mL resulted in a slight increase in total GLUT4 protein expression in the presence of palmitate and insulin (Figure 5B, No. 6), consistent with the findings obtained from the glucose uptake assay (Figure 4B, No 6). However, they did not affect the phosphorylation of p-AMPK protein expression (Figure 5C). Additionally, we also observed that higher concentrations of PN-HW led to a reduction in GLUT4 protein expression in C2C12 myotubes, consistent with the results of the glucose uptake assay (Figure 4B, No. 7 and 8).
Figure 5. The effects of P. nameko hot water extract (PN-HW) on insulin-stimulated GLUT4 expression and p-AMPK phosphorylation. (A) shows the Western blot analysis of GLUT4 and p-AMPK protein expression in C2C12 myotubes under different treatment conditions, including protein bands for total GLUT4, p-AMPK, and β-actin (as the loading control). (B) presents the quantitative analysis of GLUT4 protein expression normalized to β-actin (% of control), with statistically significant differences among treatment groups indicated by different lowercase letters (a, b, c), showing significant variation in GLUT4 levels (p < 0.05). (C) depicts the quantitative analysis of p-AMPK protein expression normalized to β-actin (% of control), demonstrating no significant differences in p-AMPK levels across treatment groups.
In this study, we explored the potential antidiabetic effects of polyphenols derived from edible P. nameko mushrooms utilizing C2C12 myotubes. Our findings revealed that the PNHW extract exhibited the highest total phenolic content, particularly with respect to 4-HBA and CA, as well as superior free radical scavenging activity compared to the other five extracts. Additionally, it mitigated insulin resistance induced by palmitate by upregulating the expression of GLUT4 protein and enhancing glucose uptake in C2C12 cells in the presence of insulin.
In our study, we performed alcohol and hot water extractions to separate the alcohol- and water-soluble polyphenols (PN-EE, PN-EA, PN-EW, PN-HW, PN-HWA, and PN-HWNA). Among the six extracts, PN-HW demonstrated the highest yield of 20.8%. This finding suggests promising opportunities for cost-effective commercial applications, which aligns with the similar findings reported in previous research (Fogarasi et al., 2021).
In 2009, Barros, Dueñas et al. published a study analyzing wild mushrooms in Portugal, yielding results similar to ours, i.e., the present of HBA and CA polyphenolic compounds. The authors examined 16 species of wild mushrooms, conducting HPLC analysis on ten of them, which revealed the presence of 4-HBA. The content of 4-HBA ranged from 14.00 ± 0.77 to 238.7 ± 12.4 mg/kg DW among the different species. In contrast, only seven species were analyzed for CA, with content ranging from 8.72 ± 0.71 to 68.37 ± 11.32 mg/kg DW. Our findings align with this literature, demonstrating that the 4-HBA and CA contents of P. nameko mushrooms fall within the concentration range reported in their study (Barros et al., 2009). Extract from Cucurbita ficifolia, specifically 4-Hydroxybenzoic acid, has been shown to ameliorate hyperglycemia induced by STZ in mice and stimulate the expression of GLUT4 mRNA in C2C12 cells (Rosiles-Alanis et al., 2022). Cinnamon polyphenol polymers may function as antioxidants, augment insulin action, and hold potential benefits in the management of glucose intolerance and diabetes (Anderson et al., 2004). Tian et al. revealed that tea polyphenols and ferulic acid improve gut health and alleviate high-fat diet-induced obesity and metabolic inflammation. Tea polyphenols enhance short-chain fatty acid production, strengthen the intestinal barrier, and suppress inflammation, while ferulic acid increases beneficial bacteria, promotes tight junction protein expression, and reduces endotoxins and inflammatory cytokines, demonstrating potential for gut health and obesity management (Tian et al., 2024; Tian et al., 2022).
Based on these findings, subsequent experiments were conducted utilizing PN-HW extracts and C2C12 myotubes. C2C12 cells, being muscle cells, play a pivotal role in insulin stimulated glucose uptake after differentiation, a process regulated by the coordinated actions of GLUT4 and the AMPK phosphorylation cascade (Kuo et al., 2016). The glucose uptake assay conclusively demonstrates that PN-HW extract, administered at a concentration of 25 μg/mL, effectively enhances intracellular glucose utilization after PA-induced insulin resistance. In the presence of insulin, PN-HW can reverse this resistance phenomenon, leading to an increase in intracellular glucose uptake. These observations suggest that PN-HW’s bioactive constituents have the potential to ameliorate PA-induced impedance. However, it is noteworthy that PN-HW fails to enhance glucose absorption in muscle cells at higher doses (Figure 4, No. 7 and 8). This might be attributed to glucose feedback inhibition, which reduces glucose absorption as a regulatory mechanism to prevent excessive energy production or other undesirable biochemical reactions. This phenomenon is also reflected in the immunoblotting of GLUT4, and we can conclude that there is a consistent trend between glucose uptake (Figure 4, No. 5 to 8) and GLUT4 protein expression (Figure 5, No. 5 to 8).
The AMPK phosphorylation signaling pathway plays a pivotal role in regulating cellular glucose uptake in muscle cells (Kurth-Kraczek et al., 1999). Moreover, insulin can also augment glucose absorption by activating the PI3K/Akt pathway. A study by Kuo et al. (2016) demonstrated that triterpenoids in Antrodia camphorata can enhance the expression of GLUT4 and p-AMPK proteins in C2C12 myotube cells. The research findings by Yang et al. (2020) also demonstrated that the hypoglycemic and hypolipidemic effects of Armillariella tabescens mycelia are associated with the amelioration of insulin resistance through the inhibition of the TXNIP/NLRP3 inflammasome pathway and the activation of the AMPK pathway. Our protein immunoblotting results showed that exposure to PN-HW at concentrations of 6.25 and 25 μg/mL significantly increased the total GLUT4 protein expression by 37 and 53%, respectively, compared to the control group without the extract. However, there was no difference in the expression of phosphorylated p-AMPK protein. This suggests that the increased expression of GLU4 protein is more likely related to the PI3K/Akt signaling pathway.
Although our study has uncovered novel findings, we must acknowledge some limitations. Firstly, since we used UPLC to screen for polyphenolic compounds that alleviate insulin resistance, it’s possible that PN-HW contains other phenolic compounds or polysaccharides besides CA and 4-HBA, which may have anti-insulin activities. Additionally, not using pure 4-HBA and CA is a limitation. Future research will include comparative studies with purified compounds to further validate their functions. Secondly, we did not conduct follow-up experiments to verify the phosphorylation of insulin receptor substrates (IRS) or the PI3K/Akt signaling pathways. Lastly, considering the inconsistencies in p-AMPK results compared to published cell experiments, which could be due to different strains of edible mushrooms used, future research should consider using diabetic animal models or human trials with PN-HW to ascertain whether PN-HW truly has the potential to improve insulin resistance and regulate blood sugar.
Based on our investigation, the edible mushroom P. nameko emerges as a valuable source of polyphenols, including 4-HBA and CA, with the potential to mitigate palmitate-induced insulin resistance in C2C12 myotubes. Notably, the mushroom extract demonstrated both antioxidant properties and non-toxic characteristics. Furthermore, at doses of 25 μg/mL, it displayed potential efficacy in enhancing cellular glucose uptake. Although our cytological study did not observe any dose-dependent changes in p-AMPK protein expression with PN-HW extract in C2C12 myotubes, it can be inferred that PN-HW mushroom extract may enhance glucose absorption in muscle cells by ameliorating insulin resistance or improving insulin sensitivity. In summary, we can conclude that PN-HW extract yields favorable results in samples and exerts beneficial effects on insulin resistance in vitro, offering potential applications in diabetes management.
The original contributions presented in the study are included in the article/supplementary material, further inquiries can be directed to the corresponding author.
S-KV: Formal analysis, Methodology, Visualization, Writing – original draft. C-MC: Resources, Validation, Writing – review & editing, Software. AD: Writing – original draft, Conceptualization. L-FS: Funding acquisition, Resources, Writing – review & editing. Y-MJ: Funding acquisition, Resources, Writing – review & editing. H-MH: Resources, Writing – review & editing. S-HY: Funding acquisition, Writing – review & editing. VV: Methodology, Writing – review & editing. S-CL: Investigation, Methodology, Writing – original draft, Writing – review & editing, Funding acquisition, Supervision.
The author(s) declare that financial support was received for the research, authorship, and/or publication of this article. This study was supported by the Council of Agriculture, Taiwan (grant number 111AS-11.1.2-CI-C1) and the National Science and Technology Council, Taiwan (grant number 1132635-H-158-001-SSS).
We thank Ms. Yu-Ching Wu and the Small Molecule Metabolomics Core Facility at the Institute of Plant and Microbial Biology, Academia Sinica, for technical assistance. Mass spectrometry analysis was supported by the Metabolomics Core Facility at the Agricultural Biotechnology Research Center under the Academia Sinica Core Facility and Innovative Instrument Project (AS-CFII-111-218).
The authors declare that the research was conducted in the absence of any commercial or financial relationships that could be construed as a potential conflict of interest.
The author(s) declared that they were an editorial board member of Frontiers, at the time of submission. This had no impact on the peer review process and the final decision.
The author(s) declare that no Gen AI was used in the creation of this manuscript.
All claims expressed in this article are solely those of the authors and do not necessarily represent those of their affiliated organizations, or those of the publisher, the editors and the reviewers. Any product that may be evaluated in this article, or claim that may be made by its manufacturer, is not guaranteed or endorsed by the publisher.
Amine, H., Benomar, Y., and Taouis, M. (2021). Palmitic acid promotes resistin-induced insulin resistance and inflammation in SH-SY5Y human neuroblastoma. Sci. Rep. 11:5427. doi: 10.1038/s41598-021-85018-7
Anderson, R. A., Broadhurst, C. L., Polansky, M. M., Schmidt, W. F., Khan, A., Flanagan, V. P., et al. (2004). Isolation and characterization of polyphenol type-a polymers from cinnamon with insulin-like biological activity. J. Agric. Food Chem. 52, 65–70. doi: 10.1021/jf034916b
Barros, L., Duenas, M., Ferreira, I. C., Baptista, P., and Santos-Buelga, C. (2009). Phenolic acids determination by HPLC-DAD-ESI/MS in sixteen different Portuguese wild mushrooms species. Food Chem. Toxicol. 47, 1076–1079. doi: 10.1016/j.fct.2009.01.039
Chou, C. H., Sung, T. J., Hu, Y. N., Lu, H. Y., Yang, L. C., Cheng, K. C., et al. (2019). Chemical analysis, moisture-preserving, and antioxidant activities of polysaccharides from Pholiota nameko by fractional precipitation. Int. J. Biol. Macromol. 131, 1021–1031. doi: 10.1016/j.ijbiomac.2019.03.154
Das, A., Chen, C. M., Mu, S. C., Yang, S. H., Ju, Y. M., and Li, S. C. (2022). Medicinal components in edible mushrooms on diabetes mellitus treatment. Pharmaceutics 14:117. doi: 10.3390/pharmaceutics14020436
Fogarasi, M., Socaciu, M.-I., Sălăgean, C. D., Ranga, F., Fărcaș, A. C., Socaci, S. A., et al. (2021). Comparison of different extraction solvents for characterization of antioxidant potential and polyphenolic composition in boletus edulis and Cantharellus cibarius mushrooms from Romania. Molecules 26:7508. doi: 10.3390/molecules26247508
Ishii, M., Ikeda, N., Miyata, H., Takahashi, M., and Nishimura, M. (2022). Purple sweet potato leaf extracts suppress adipogenic differentiation of human bone marrow-derived mesenchymal stem cells. J. Food Biochem. 46:e14057. doi: 10.1111/jfbc.14057
Jeong, W. T., Bang, J. H., Han, S., Hyun, T. K., Cho, H., Lim, H. B., et al. (2020). Establishment of a UPLC-PDA/ESI-Q-TOF/MS-based approach for the simultaneous analysis of multiple phenolic compounds in amaranth (a. cruentus and A. tricolor). Molecules 25:5674. doi: 10.3390/molecules25235674
Kuo, Y. H., Lin, C. H., Shih, C. C., and Yang, C. S. (2016). Antcin K, a triterpenoid compound from Antrodia camphorata, displays antidiabetic and antihyperlipidemic effects via glucose transporter 4 and AMP-activated protein kinase phosphorylation in muscles. Evid. Based Complement. Alternat. Med. 2016:4867092. doi: 10.1155/2016/4867092
Kurth-Kraczek, E. J., Hirshman, M. F., Goodyear, L. J., and Winder, W. W. (1999). 5' AMPactivated protein kinase activation causes GLUT4 translocation in skeletal muscle. Diabetes 48, 1667–1671. doi: 10.2337/diabetes.48.8.1667
Lin, C. L., Chang, Y. T., Liu, W. C., Huang, L. C., Tsao, S. Y., Chen, Y. H., et al. (2022). Exploring and developing a new culturally-appropriate diabetes distress scale in Taiwan. Front. Public Health 10:838661. doi: 10.3389/fpubh.2022.838661
Lin, H., Cheng, K. C., Lin, J. A., Hsieh, L. P., Chou, C. H., Wang, Y. Y., et al. (2022). Pholiota nameko polysaccharides protect against ultraviolet a-induced photoaging by regulating matrix metalloproteinases in human dermal fibroblasts. Antioxidants 11:739. doi: 10.3390/antiox11040739
Nanditha, A., Ma, R. C., Ramachandran, A., Snehalatha, C., Chan, J. C., Chia, K. S., et al. (2016). Diabetes in Asia and the Pacific: implications for the global epidemic. Diabetes Care 39, 472–485. doi: 10.2337/dc15-1536
Nowacka, N., Nowak, R., Drozd, M., Olech, M., Los, R., and Malm, A. (2014). Analysis of phenolic constituents, antiradical and antimicrobial activity of edible mushrooms growing wild in Poland. Food Sci. Technol. 59, 689–694. doi: 10.1016/j.lwt.2014.05.041
Rosiles-Alanis, W., Zamilpa, A., García-Macedo, R., Zavala-Sánchez, M. A., HidalgoFigueroa, S., Mora-Ramiro, B., et al. (2022). 4-Hydroxybenzoic acid and β-sitosterol from Cucurbita ficifolia act as insulin secretagogues, peroxisome proliferator-activated receptor-gamma agonists, and liver glycogen storage promoters: in vivo, in vitro, and in silico studies. J. Med. Food 25, 588–596. doi: 10.1089/jmf.2021.0071
Saeedi, P., Petersohn, I., Salpea, P., Malanda, B., Karuranga, S., Unwin, N., et al. (2019). Global and regional diabetes prevalence estimates for 2019 and projections for 2030 and 2045: results from the international diabetes federation diabetes atlas, 9th edition. Diabetes Res. Clin. Pract. 157:107843. doi: 10.1016/j.diabres.2019.107843
Sawada, K., Kawabata, K., Yamashita, T., Kawasaki, K., Yamamoto, N., and Ashida, H. (2012). Ameliorative effects of polyunsaturated fatty acids against palmitic acid-induced insulin resistance in L6 skeletal muscle cells. Lipids Health Dis. 11:36. doi: 10.1186/1476-511X-11-36
Shyur, L. F., Varga, V., Chen, C. M., Mu, S. C., Chang, Y. C., and Li, S. C. (2021). Extract of white sweet potato tuber against TNF-α-induced insulin resistance by activating the PI3K/Akt pathway in C2C12 myotubes. Bot. Stud. 62:7. doi: 10.1186/s40529-021-00315-8
Sung, T. J., Wang, Y. Y., Liu, K. L., Chou, C. H., Lai, P. S., and Hsieh, C. W. (2020). Pholiota nameko polysaccharides promotes cell proliferation and migration and reduces ros content in H2O2-induced L929 cells. Antioxidants 9:65. doi: 10.3390/antiox9010065
Takazawa, K., Noguchi, T., Hosooka, T., Yoshioka, T., Tobimatsu, K., and Kasuga, M. (2008). Insulin-induced GLUT4 movements in C2C12 myoblasts: evidence against a role of conventional kinesin motor proteins. Kobe J. Med. Sci. 54, E14–E22
Tian, B., Geng, Y., Wang, P., Cai, M., Neng, J., Hu, J., et al. (2022). Ferulic acid improves intestinal barrier function through altering gut microbiota composition in high-fat diet-induced mice. Eur. J. Nutr. 61, 3767–3783. doi: 10.1007/s00394-022-02927-7
Tian, B., Huang, P., Pan, Y., Gu, H., Yang, K., Wei, Z., et al. (2024). Tea polyphenols reduced obesity by modulating gut microbiota-SCFAs-barrier and inflammation in high-fat diet-induced mice. Mol. Nutr. Food Res. 68:e2400685. doi: 10.1002/mnfr.202400685
Truong, V. D., McFeeters, R. F., Thompson, R. T., Dean, L. L., and Shofran, B. (2007). Phenolic acid content and composition in leaves and roots of common commercial sweetpotato (Ipomea batatas L.) cultivars in the United States. J. Food Sci. 72, C343–C349. doi: 10.1111/j.1750-3841.2007.00415.x
Usai, R., Majoni, S., and Rwere, F. (2022). Natural products for the treatment and management of diabetes mellitus in Zimbabwe-a review. Front. Pharmacol. 13:980819. doi: 10.3389/fphar.2022.980819
Westman, E. C. (2021). Type 2 diabetes mellitus: a pathophysiologic perspective. Front. Nutr. 8:707371. doi: 10.3389/fnut.2021.707371
Widyahening, I. S., Khunti, K., Vos, R. C., and Chew, B. H. (2022). Editorial: achieving effective management and treatment of diabetes mellitus in future primary care. Front. Endocrinol. 13:854244. doi: 10.3389/fendo.2022.854244
Wong, C. Y., Al-Salami, H., and Dass, C. R. (2020). C2C12 cell model: its role in understanding of insulin resistance at the molecular level and pharmaceutical development at the preclinical stage. J. Pharm. Pharmacol. 72, 1667–1693. doi: 10.1111/jphp.13359
Yan, Y., Wu, T., Zhang, M., Li, C., Liu, Q., and Li, F. (2022). Prevalence, awareness and control of type 2 diabetes mellitus and risk factors in Chinese elderly population. BMC Public Health 22:1382. doi: 10.1186/s12889-022-13759-9
Yang, R., Li, Y., Cai, J., Ji, J., Wang, Y., Zhang, W., et al. (2020). Polysaccharides from Armillariella tabescens mycelia ameliorate insulin resistance in type 2 diabetic mice. Food Funct. 11, 9675–9685. doi: 10.1039/D0FO00728E
Zhang, Y., Liu, Z., Ng, T. B., Chen, Z., Qiao, W., and Liu, F. (2014). Purification and characterization of a novel antitumor protein with antioxidant and deoxyribonuclease activity from edible mushroom Pholiota nameko. Biochimie 99, 28–37. doi: 10.1016/j.biochi.2013.10.016
Zhang, X., Liu, T., Wang, X., Zhou, L., Qi, J., and An, S. (2022). Structural characterization, antioxidant activity and anti-inflammatory of the phosphorylated polysaccharide from Pholiota nameko. Front. Nutr. 9:976552. doi: 10.3389/fnut.2022.976552
Keywords: Pholiota nameko water extract, insulin resistance, phenolic acids, cinnamic acid, 4-hydroxybenzoic acid
Citation: Vong S-K, Chen C-M, Das A, Shyur L-F, Ju Y-M, Hsieh H-M, Yang S-H, Varga V and Li S-C (2025) Stimulation of glucose uptake by edible Pholiota nameko (T.Itô) S.Ito & S.Imai extract counteracts palmitate-induced insulin resistance in C2C12 myotubes. Front. Sustain. Food Syst. 9:1520875. doi: 10.3389/fsufs.2025.1520875
Received: 31 October 2024; Accepted: 30 January 2025;
Published: 19 February 2025.
Edited by:
Dibya Jyoti Hazarika, AAU-Zonal Research Station, IndiaReviewed by:
Baoming Tian, Zhejiang University of Technology, ChinaCopyright © 2025 Vong, Chen, Das, Shyur, Ju, Hsieh, Yang, Varga and Li. This is an open-access article distributed under the terms of the Creative Commons Attribution License (CC BY). The use, distribution or reproduction in other forums is permitted, provided the original author(s) and the copyright owner(s) are credited and that the original publication in this journal is cited, in accordance with accepted academic practice. No use, distribution or reproduction is permitted which does not comply with these terms.
*Correspondence: Sing-Chung Li, c2luY2h1bmdAdG11LmVkdS50dw==
†These authors have contributed equally to this work
Disclaimer: All claims expressed in this article are solely those of the authors and do not necessarily represent those of their affiliated organizations, or those of the publisher, the editors and the reviewers. Any product that may be evaluated in this article or claim that may be made by its manufacturer is not guaranteed or endorsed by the publisher.
Research integrity at Frontiers
Learn more about the work of our research integrity team to safeguard the quality of each article we publish.