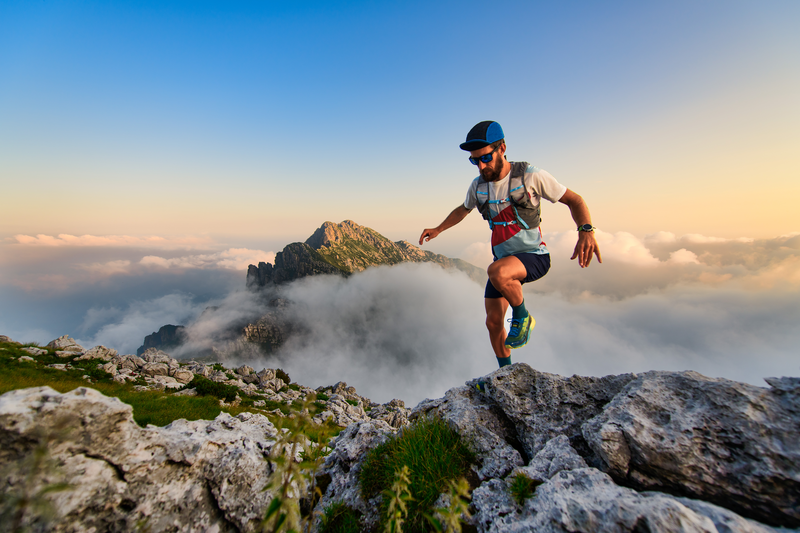
94% of researchers rate our articles as excellent or good
Learn more about the work of our research integrity team to safeguard the quality of each article we publish.
Find out more
ORIGINAL RESEARCH article
Front. Sustain. Food Syst. , 12 February 2025
Sec. Crop Biology and Sustainability
Volume 9 - 2025 | https://doi.org/10.3389/fsufs.2025.1513324
The issue of ongoing cropping barriers is getting worse as China’s melon planting area steadily grows, and the melon industry’s sustainable growth is being negatively impacted by the steadily diminishing yield and quality of the fruit. Trichoderma is a probiotic that can enhance the physiological traits of crops, encourage their growth, and raise their yield and quality. It is yet unknown, though, how Trichoderma influences the growth, physiological traits, and yield of melon grown on soils continuously cropped to melon. Trichoderma viride kf57 agents at 1.0 × 104, 8.0 × 104, 6.4 × 105, and 5.12 × 106 CFU/g and no Trichoderma viride agents (CK) were utilized as treatments. At the seedling and fruiting stages, a pot experiment and a bedding experiment were conducted to study the physiological properties and yield of melon under varying concentration of Trichoderma viride kf57 agents. As a result of the application of T. viride agents, the indexes of melon seedlings were all significantly improved. The treatment of 6.4 × 105 CFU/g had the best promoting effect on the morphology of melon seedlings, and the plant height, stem diameter, leaf area, fresh weight of whole plant, dry weight of whole plant, root shoot ratio, and strong seedling index of melon seedlings increased by 90.39, 46.30, 37.55, 81.35, 100.62, 51.47, and 240.00%, respectively, compared with CK. The results showed that different amounts of T. viride agents could improve physiological and biochemical indices of melon leaves during the fruiting stage; the treatment of 6.4 × 105 CFU/g was the most effective; chlorophyll content, nitrate nitrogen content, sucrose content, reducing sugar content, free proline content, nitrate reductase (NR) activity, peroxidase (POD) activity, and superoxide dismutase (SOD) activity of melon leaves increased at 30 days after melon pollination. Melon quality and yield was also enhanced by the use of T. viride agents, with the treatment of 6.4 × 105 CFU/g T. viride agents having the best boosting effects. The melon fruit’s transverse diameter, vertical diameter, single fruit weight, and yield all increased. The amount of soluble solids, vitamin C, soluble protein, soluble sugar, and sugar acid ratio also increased. In conclusion, by promoting the morphology of melon grown on soils continuously cropped to melon seedlings, T. viride agents can improve the physiological characteristics of melon grown on soils continuously cropped to melon and improve the production and quality qualities of melon. When using T. viride kf57 agents, 6.4 × 105 CFU/g is the highest effective dosage. The study revealed that T. viride agents had significant potential as biological agents as they showed good results in melon yield and quality formation, as well as in enhancing seedling quality.
Melon (Cucumis melo L.) is ranked among the top 10 fruit vegetables in the world. Melon’s rich flavor and crispy texture make it a popular choice among customers. It has a lengthy history of cultivation and is commonly cultivated worldwide (Grumet et al., 2021). China is an important producer and consumer of cantaloupe in the world (Zhang J. et al., 2019). According to data from the Food and Agriculture Organization of the United Nations (FAO), in 2021, the planting area of cantaloupe in China was 387,000 hectares, with a yield of 1.41 × 106 kg, accounting for 35.9 and 49.2% of the world’s cantaloupe cultivation area and yield, respectively. The unit area yield of sweet melons in China has increased from 3.57 × 108 kg/hm2 in 2020 to 3.64 × 108 kg /hm2 in 2021. This indicates that China’s melon farming has significant competitive advantages, growth potential, and great economic value. China’s melon production and planting area have consistently ranked top in the world in recent years (Cai et al., 2023) due to the ongoing advancements in melon growing technologies. Simultaneously, persistent cropping barriers in the industrialization and intensive melon cultivation process are getting more serious, which eventually results in a decline in melon quality and yield. This has become a significant factor limiting farmers’ income and adversely affecting the long-term growth of China’s melon industry (Ku et al., 2022). The demand for superior cantaloupe is rising in tandem with the expansion of the Chinese economy and the ongoing improvement in the standard of living of the people. In the present melon production cycle, improving melon quality and yield has emerged as a key research area (Cui et al., 2022). In actual production, different agricultural management techniques such as soil treatment, sensible rotation, soil sterilization, and artificial introduction of beneficial microorganisms have become the main means of improving melon yield and quality (Tang et al., 2023) due to the diversity and uneven quality of melon varieties, as well as differences in storage and transportation and disease resistance. One of the primary methods of improving soil microbial environment and growth of plant is thhe application of Trichoderma spp.
Trichoderma is one of the most researched and utilized of all the biocontrol fungi (Srivastava et al., 2012). Trichoderma has a positive regulatory effect on the morphology, physiology, nutrient dissolution and absorption, yield growth, and induced disease resistance of plants by speeding up their growth and development, increasing their absorption of nutrients, improving their tolerance to biotic and abiotic stress, and improving the rhizosphere environment. Disease resistance is induced, stress resistance is reinforced, and plant development is promoted (Sood et al., 2020).
There are currently just a few studies on the use of Trichoderma in melon, with the majority of the studies concentrating on the plant antioxidant defense system, soil microbial amount, soil enzyme activity, and the influence of certain agronomic parameters as well as the biological control effect of melon wilt. For instance, four Trichoderma species (T.viride, T. harzianum, T. helicum, and T. erinaceum) were investigated by Boughalleb-M’Hamdi et al. (2018), who also assessed the species’ efficacy in controlling soilborne fungi in melon and watermelon. According to the findings, Trichoderma not only lessened the severity of blight but also enhanced the melon and watermelon’s growth metrics and the rate at which their shoot and root dry weights developed. According to Bernal-Vicente et al. (2015), melon plants’ antioxidant defense system was regulated by inoculation with T. harzianum T-78, which also increased the activity of peroxidase and the ascorbic acid cycle enzymes. These findings suggest that T. harzianum may boost melon plant yield. T. harzianum treatment of melon seeds enhanced soil microbial biomass and enzyme activity during the flowering stage, leading to increase in root length, indicating a positive influence on melon root development, according to Galletti et al. (2015).
Nonetheless, more studies on Trichoderma on different plants, as those conducted by Ahmad et al. (2015), have demonstrated that Trichoderma is a significant plant growth-promoting rhizosphere fungus (PGPF) that has the ability to stimulate plant growth. For instance, Zhao et al. (2021) discovered that T. afroharzianum TM2-4 culture filtrate can create bioactive compounds and aid in tomato seed germination. They also discovered that tomato hypocotyl length, root length, and vitality index increased after 100 times dilution. According to Yani et al. (2019), Trichoderma spp. treatment raised the plant height, root length, and tuber weight of garlic plants. Trichoderma viride and Arbuscular mycorrhizal fungi were shown by Metwally and Al-Amri (2020) to be able to increase the fresh weight and dry weight of onions as well as morphological indices including leaf area, stem length, and root length as well as the pigment content of onions. Numerous studies have demonstrated that Trichoderma can enhance the physiological and biochemical traits of plants, which results in an increase in production and an improvement in quality. As an illustration, Li et al. (2022) discovered that Trichoderma considerably raised Mongholicus Astragalus’s root biomass, root length, nitrate reductase, and soil urease activity. Trichoderma agents were discovered by Kuang et al. (2024) to boost the activity of SOD (superoxide dismutase), POD (peroxidase), CAT (catalase), PPO (polyphenoloxidase), and PAL (Phenylalanineammonialyase) in chrysanthemum leaves. The fresh weight above ground, fresh weight below ground, root to shoot ratio, root vitality, and plant height all experienced increases. Pro (Proline), MDA (malondialdehyd), and total phenolic content of rice seeds treated with Trichoderma asperelloides and Trichoderma brevicompactum were shown to be significantly different from those of untreated plants by Quazi et al. (2024). According to Vultaggio et al. (2024), inoculating with T. atroviride considerably enhanced the commercial production of strawberries, total sugar, and anthocyanin content when compared to untreated plants. According to Lian et al. (2023), a formulation of Trichoderma harzianum agents containing 106 cfu/g also enhanced the quality index and yield production of cucumbers. In contrast to CK1 (104 cfu/g Fusarium oxysporum powder only), the fruit of cucumbers had higher levels of soluble sugar, vitamin C, soluble protein, and soluble solids and an increase in yield.
Trichoderma products have been used all around the world for more than 60 years. Trichoderma can produce mycelium, conidia, and chlamydospores during the course of its life cycle. At present, there are more than 50 kinds of commercial Trichoderma preparations at home and abroad (Woo et al., 2006), such as Trichoderma harzianum T22 strain in the United States and Trichoderma harzianum T39 strain in Israel; Trichoderma preparations Trichodry and Trichoflow in New Zealand; Trichoderma preparations Myc01 in Russia; Trichoderma YC458 from South Korea; and the mixed biocontrol agents TUSAL of Trichoderma harzianum and Trichoderma viridis in Spain (Sanchez et al., 2019). Therefore, creating stable Trichoderma conidium preparations is crucial for producing plants with high yields and good quality. In this study, the Trichoderma viride kf57 conidium agents was used to systematically investigate its physiological effects on the seedling morphogenesis, yield, and quality formation of melon grown on soils continuously cropped to melon with the aim of providing technical assistance for promoting the quality and secure production of melon and providing a theoretical foundation development and promotion of Trichoderma agents.
The Trichoderma research team from the Institute of Plant Protection, Chinese Academy of Agricultural Sciences, Beijing, China, contributed the tested strain of Trichoderma viride kf57.
From the rhizosphere soil of pine trees in Heilongjiang, China, strain T. viride kf57 was isolated, identified, and stored at the Trichoderma Research Group of the Institute of Plant Protection, Chinese Academy of Agricultural Sciences. HY1-22 was the strain number at first. The results of the plate confrontation test shown that the strain both stimulates the growth of melon seedlings and significantly inhibits the growth of the Fusarium oxysporum melon specialized type. T. viride kf57 strain is considered safe and does not require strain safety testing because it is derived from natural soil.
The soil used in the seedling-phase experiment came from a greenhouse where melons were continuously planted for 3 years. The soil is suitable for usage after it has been filtered using a 1 mm sieve. The basic agrochemical characteristics of the soil used for seedling testing were are shown in Table 1. Melons were grown continuously for 3 years in greenhouses at the fruit-phase experiment.
Table 1. Fundamental agrochemical characteristics of soils that have been grown for 3 years with melon.
Trichoderma viride was cultured on PDA culture material for 3 days at 28°C in the dark as an activation culture. To make the Trichoderma spore solution, five samples from the margins of each colony, each measuring 5 mm in diameter, were collected. After that, these samples were put into PDA culture media and kept at 28°C in the dark for 7 days. Then, distilled water was used to cleanse the spores before they were extracted. After diluting it with a sterile water gradient, it is coated over the Trichoderma selective medium and inverted for a couple of days at 25–28°C. By counting the colonies, determine the number of Trichoderma conidia. For a full night, clean, room-temperature water was used to soak barley grains, sterilizing them. One kilogram of fresh-keeping bags were then filled with the remaining mixture after the water was removed. After chilling, suspensions of Trichoderma viride were added, and they were cultivated for 2–3 weeks at 25°C. When the spore has gotten too big, rinse with sterile water and filter the grain away. After adding 10% talcum powder, the filtrate was crushed, filtered, and dried to produce Trichoderma viride conidium powder. With 1.1 × 1010 CFU/g of Trichoderma viride conidiomyces, the administered dose was calculated in compliance with the test requirements.
At seedling test, the melon cultivar Longtian No. 1 from Xiangnong Seed Corporation Ltd., Haerbin, Heilongjiang, China, was used in this investigation. The Heilongjiang Bayi Agricultural University’s teaching facility in China provided the plastic greenhouse where this experiment was conducted. The soil from the greenhouse where melons were continuously planted for 3 years for seedlings was then placed in plastic seedling culture plates (30.5 cm × 20.5 cm × 8.6 cm), each of which contains 5.25 kg of soil along with varying concentrations of Trichoderma viride conidia agents. Following germination treatment, 140 melon seeds were added to each plate, and 100 seedlings of a similar size were saved. After sowing, melons were given 1,000 mL of water every 2 days to maintain a normal growth stage.
Each of the five treatments in this study contained 100 seedlings spread across five dishes. Four times the experiment was run, with a different set of seedlings being chosen at random each time.
The following were the treatment groups:
(1) 1.0 × 104 cfu/g Trichoderma viride conidia agents (T1); (2) 8.0 × 104 cfu/g Trichoderma viride conidia agents (T2); (3) 6.4 × 105 cfu/g Trichoderma viride conidia agents (T3); (4) 5.12 × 106 cfu/g Trichoderma viride conidia agents (T4); (5) No Trichoderma viride agents (CK) were applied.
The root-shoot ratio and strong seedling index, as well as seedling morphological indices and material accumulation indices for melon seedlings, were calculated for 40 plants from each treatment at 30 days after sowing (10 plants per replication) high furrow cultivation.
At fruiting stage test, the soil at the plastic greenhouse was fertilized with 225 kg of diammonium phosphate, 300 kg of potassium sulfate, and 375 kg of organic fertilizer per acre. The ground was completely prepared for beds. Choose healthy seedlings that have steady growth and place them in a plastic greenhouse where melons were grown continuously for 3 years on April 25, 2022, when the melon seedlings have reached the size of three leaves and one heart. Bedding cultivation is used in plastic greenhouses with a randomized block arrangement. The experimental design is the same as in 1.3.1, with four replicates. Place a blank space between each bed. The bed measures 6 meters in length, 1.0 meters in width, 1.2 meters in width, 1:100 in slope, and 10 cm in depth. The cultivation of melon in the top beds uses double row planting, with 15 plants placed in each bed with a plant spacing of 40 cm and a row spacing of 50 cm.
After a delayed seedling, the melon was selected on the fourth leaf, grown on two vines, and watered every 3 days. When the seventh leaf was extended, the plants started to hang. Double vines are used to prune the melon plant, and after about 2–3 knots, the fruit vines begin to grow continually. Select 3–4 uniform and sturdy fruits from each melon plant when the fruit’s longitudinal diameter reaches 2–3 cm, discard the other fruits, and pluck the heart from the vine’s 20–25 leaves.
Female flowers from the same node and blossoming time were used to label 75 plants on the day of pollination. Sampling started on the tenth day following pollination, and it was done five times in total, once every 5 days. In the adult plant stage, 4–5 leaves on the fruit vine were used as the aim source from 9:00 to 10:00 a.m. to determine the physiological and biochemical indices. At 30 days following pollination, 16 fruits (4 fruits per replication) were randomly chosen from each treatment, and the quality indices were assessed using mixed fruit samples. In addition, the plot yield and hectare yield were determined while 16 randomly chosen fruits (4 fruits per replication) from each treatment were measured for fruit transverse diameter, longitudinal diameter, and single melon weight.
The distance between the stem base and its growth point on each seedling was measured with a ruler to calculate the plant height (Shoam et al., 2022). By measuring the diameter of the stem 1 cm below the cotyledon with a vernier caliper, the diameter of the stem was determined (Shoam et al., 2022).
By using the weighing method, the leaf area was calculated (Wang et al., 2021). A leaf with a particular area (A1) was first gathered and layered with other leaves. Next, round sheets were created using a 1-cm puncher pin. The weights of the round sheets and the remaining leaves were then measured, and from there, the weight of the leaf with a particular area (W1) and the weights of the other leaves (W2) were computed. The formula A = [A1(W1 + W2)]/W1 was used to calculate the leaf total area (A) based on these measurements.
The plants were periodically cleaned with clear water before being dried on absorbent paper. The fresh weight of the plants was computed based on their above- and below-ground parts. After that, the fresh samples were baked to a consistent weight at 70°C after being dried at 105°C for 15 min. The above- and below-ground components’ dry weights were calculated using an electronic scale with a resolution of 1/1,000 (Jang et al., 2021).
Using Gou et al. (2022) method, the root-shoot ratio was calculated using the following formula:
Root-shoot ratio = fresh weight of underground part/fresh weight of above-ground part.
The strong seedling index was calculated using the following formula in accordance with Liu X. J. et al. (2022) methodology:
Strong seedling index = (Stem diameter/Plant height + underground dry weight/above ground dry weight) × dry weight of whole plant.
The ethanol technique was used to determine the content of chlorophyll (Ahmad et al., 2022). For nitrate nitrogen content, the phenolic disulfonic acid technique was utilized (Zheng et al., 2018). The anthrone colorimetric method was used to estimate the content of sucrose (Song et al., 2011). The 3, 5-dinitrosalicylic acid technique was used to determine the content of reducing sugar (Xu et al., 2022). By using sulfosalicylic acid in an acid ninhydrin extraction, the content of free proline (Pro) was discovered (Chong and Jun, 2005). In vivo spectrophotometry was used to evaluate nitrate reductase (NR) activity (Rong et al., 2018). The guaiacol technique was used to assess the peroxidase (POD) activity (Zhang Q. et al., 2019). The nitrogen blue tetrazole photochemical reduction technique was used to examine the activity of superoxide dismutase (SOD) (Hussain et al., 2017).
The content of soluble solids was calculated using an Abel refractometer. The anthrone colorimetric method was used to quantify the content of soluble sugar (Song et al., 2011). The Coomassie Brilliant Blue G-250 staining procedure was used to quantify the content of soluble protein (Zheng et al., 2018). UV spectrophotometry was used to assess the vitamin C content (Kampfenkel et al., 1995). Using the 0.1 mol/L sodium hydroxide standard solution technique, the total acid content was titrated.
Vernier calipers were used to measure the transverse and longitudinal diameters of melon fruit. A single melon fruit was weighed using an analytical balance (TB-4002, BeiXiaogan Matguang Medical Electronics Co., Ltd., Xiaogan, Hubei, China), with an accuracy of 0.01 g.
The DPS 7.05 program was used for data statistics and variance analysis. Duncan’s distinct challenging range technique was employed to assess multiple comparisons across treatments (p < 0.05). Origin 2019 software was used for mapping.
The root-shoot ratio and strong seedling index were calculated on day 30 after sowing using the morphological indices of plant height, stem diameter, leaf area, and material accumulation indices of fresh weight and dry weight in the aboveground and underground parts of melon grown on soils continuously cropped to melon seedlings treated with various Trichoderma viride agents. The results are shown in Table 2.
Table 2. Mophological parameters of melon seedlings under various T. viride agents application rates.
Melon seedlings treated with 1.0 × 104, 8.0 × 104, 6.4 × 105 and 5.12 × 106 cfu/g Trichoderma viride agents had considerably greater morphogenetic indices and material accumulation indices than those treated with CK(no Trichoderma viride agents were used). The best effects on plant height, stem diameter, leaf area, fresh weight, dry weight, root-shoot ratio, and strong seedling index were seen with T3 (6.4 × 105 cfu/g Trichoderma viride agents). It grew by 90.39, 46.30, 37.55, 81.35, 100.62, 51.47, and 240.00% when compared to CK, respectively. These findings suggested that Trichoderma viride at the right concentration could encourage melon grown on soils continuously cropped to melon seedling morphology.
The chlorophyll and nitrate nitrogen content of melon leaves treated with T. viride agents during the fruiting stage exhibited a trend of first increasing and then decreasing with the passage of pollination time, with peaks occurring 25 days after pollination, as seen in Figures 1A,B. At 10, 15, 20, 25, and 30 days after pollination, the chlorophyll and nitrate nitrogen content of melon leaves showed an increasing trend followed by a decreasing trend with the increase of T. viride agents treatment concentration. T3 melon leaves had the highest levels of both chlorophyll and nitrate nitrogen. Melons treated with T3 had the maximum chlorophyll content at 10, 15, 20, 25, and 30 days following pollination, as seen in Figure 1A. The chlorophyll content of melon leaves rose by 39.30, 38.43, 41.94, 35.49, and 34.68%, respectively, when treated with T3 as opposed to CK. According to Figure 1B, the T3 treatment had the highest nitrate nitrogen content in melon leaves at 10, 15, 20, 25, and 30 days following melon pollination, which was noticeably higher than that of the other treatments. The nitrate nitrogen concentration of melon leaves rose by 34.97, 46.91, 75.86, 117.03, and 115.05% during T3 treatment as opposed to CK.
Figure 1. Chlorophyll content (A) and nitrate nitrogen content (B) under different ratios of T. viride agents in leaves during melon fruiting stage. Different lowercase letters indicate significant differences among the 5 treatments from 10 to 30 days after pollination at the 0.05 level (p < 0.05). CK, No T. viride agents were applied; T1, 1.0 × 104 cfu/g T. viride conidia agents; T2, 8.0 × 104 cfu/g T. viride conidia agents; T3, 6.4 × 105 cfu/g T. viride conidia agents; T4, 5.12 × 106 cfu/g T. viride conidia agents.
Melons treated with T. viride agents during the fruiting stage exhibited a trend of initially increasing and then declining with the passage of pollination time, with peaks occurring 25 days following pollination, as illustrated in Figures 2A,B. The sucrose and reducing sugar content in melon leaves indicated a trend of initially rising and then falling with the increase in T. viride agents treatment concentration at 10, 15, 20, 25, and 30 days after melon pollination. In terms of sucrose and decreasing sugar content, T3 melon leaves were the greatest. Figures 2A illustrates that at 10, 15, 20, 25, and 30 days following melon pollination, T3 treatment produced the highest sucrose content in melon leaves, increasing the sucrose content by 35.73, 38.23, 34.71, 31.27, and 33.47%, respectively, in comparison to CK. Figures 2B displays the highest content of reducing sugars in melon leaves at 10, 15, 20, 25, and 30 days following melon pollination, increasing the reducing sugar content in melon leaves by 33.38, 28.49, 25.42, 42.45, and 27.33%, respectively.
Figure 2. Sucrose content (A) and reducing sugar content (B) under different ratios of T. viride agents in leaves during melon fruiting stage. Different lowercase letters indicate significant differences among the 5 treatments from 10 to 30 days after pollination at the 0.05 level (p < 0.05). CK, No T. viride agents were applied; T1, 1.0 × 104 cfu/g T. viride conidia agents; T2, 8.0 × 104 cfu/g T. viride conidia agents; T3, 6.4 × 105 cfu/g T. viride conidia agents; T4, 5.12 × 106 cfu/g T. viride conidia agents.
The amount of free proline in melon leaves treated with T. viride agents during the fruiting stage exhibited a trend of steadily rising levels as pollination time passed, as illustrated in Figures 3A,B. During the fruiting phase treated with T. viride agents, the NR activity of melon leaves exhibited a tendency of first rising and then falling with the passage of pollination time, peaking 25 days after pollination. The amount of free proline and NR activity in melon leaves at 10, 15, 20, 25, and 30 days after pollination exhibited a trend of initially rising and then falling as the concentration of T. viride agents treated increased. The highest levels of free proline and nitrate reductase activity were seen in T3 melon leaves. The T3 treatment had the maximum amount of free proline in the melon leaves at 10, 15, 20, 25, and 30 days following pollination, as seen in Figure 3A. T3 treatment raised the amount of free proline in melon leaves by 51.95, 26.46, 26.63, 50.98, and 26.44%, respectively, in comparison to CK. The NR activity of T3 melon leaves was the highest and considerably higher than that of other treatments at 10, 15, 20, 25, and 30 days after melon pollination, as illustrated in Figure 3B. Melon leaves’ NR activity rose by 56.21, 53.91, 48.48, 39.92, and 37.84% with T3 treatment, respectively, in comparison to CK.
Figure 3. Free proline content (A) and nitrate reductase activity (B) under different ratios of T. viride agents in leaves during melon fruiting stage. Different lowercase letters indicate significant differences among the 5 treatments from 10 to 30 days after pollination at the 0.05 level (p < 0.05). CK, No T. viride agents were applied; T1, 1.0 × 104 cfu/g T. viride conidia agents; T2, 8.0 × 104 cfu/g T. viride conidia agents; T3, 6.4 × 105 cfu/g T. viride conidia agents; T4, 5.12 × 106 cfu/g T. viride conidia agents.
The POD and SOD activities of melon leaves treated with T. viride agents during the fruiting stage exhibited a trend of first rising and then falling with the passage of pollination time, with peaks occurring 25 days after pollination, as seen in Figures 4A,B. The POD and SOD activities of melon leaves exhibited a tendency of initially rising and then falling with the increase in T. viride agent treatment concentration at 10, 15, 20, 25, and 30 days following melon pollination. T3 melon leaves had the highest POD and SOD activity. Figures 4A illustrates that the POD activity of T3 melon leaves was the highest and substantially higher than that of other treatments at 10, 15, 20, 25, and 30 days following melon pollination. Melon leaves’ POD activity rose by 54.22, 48.29, 35.05, 73.97, and 61.20% with T3 treatment, respectively, in comparison to CK. Figures 4B illustrates that the SOD activity of T3 melon leaves peaked 10, 15, 20, 25, and 30 days after melon pollination. Melon leaves’ SOD activity rose by 78.20, 50.01, 28.33, 18.62, and 22.60% with T3 treatment, respectively, in comparison to CK.
Figure 4. Peroxidase activity (A) and superoxide dismutase activity (B) under different ratios of T. viride agents in leaves during melon fruiting stage. Different lowercase letters indicate significant differences among the 5 treatments from 10 to 30 days after pollination at the 0.05 level (p < 0.05). CK, No T. viride agents were applied; T1, 1.0 × 104 cfu/g T. viride conidia agents; T2, 8.0 × 104 cfu/g T. viride conidia agents; T3, 6.4 × 105 cfu/g T. viride conidia agents; T4, 5.12 × 106 cfu/g T. viride conidia agents.
After 30 days of pollination, melon fruit weight, transverse diameter, and longitudinal diameter were measured under various T.viride agents treatments, and melon yield was computed, as shown in Table 3. As the concentration of T. viride agent treatment increased, the transverse diameter, longitudinal diameter, single fruit weight, and yield of melon fruits treated with the agent exhibited a trend of initially increasing and then dropping. With transverse diameter of 8.621 cm, longitudinal diameter of 13.106 cm, single fruit weight of 521.726 g, and yield of 26321.254 kg/hm2, T3 was the largest. In comparison to CK, T3 rose by 27.87, 39.81, 62.23, and 93.99%, respectively.
A variety of melon quality indices were measured 30 days after pollination using various T. viride agents treatments. The results are displayed in Table 4. As the concentration of T. viride agent treatment increased, the soluble solids content, vitamin C content, soluble protein content, soluble sugar content, and sugar acid ratio of melon fruit treated with T. viride agents showed an increasing trend, followed by a decreasing trend. The T3 fruit exhibited the highest levels of soluble solids, vitamin C, soluble protein, soluble sugar, and sugar acid ratio, with respective contents of 12.064%, 266.381 mg/100 g, 1294.746 mg/kg, 64.095 mg/g, and 15.493. Comparing T3 to CK, the increases were 50.16, 58.07, 85.56, 50.03, and 90.82%, respectively. Compared to CK, T3 fruit had the lowest total acid content (4.137 g/kg), which was 27.19% lower.
Melon’s root vitality is impeded and its weight and growth of roots and leaves are greatly reduced as a result of long-term continuous cropping (Gava and Pinto, 2016). This leads to a significant degradation of the soil’s physical and chemical properties, reduced organic matter content, and an inbalanced soil nutrient (Xu et al., 2024). Simultaneously, the build-up of autotoxic chemicals in soil alters the soil microenvironment, impacts physiological functions as crop photosynthesis and enzyme activity, and prevents crop growth (Du et al., 2024). Trichoderma, a popular biological control agent, can successfully reduce ongoing cropping barriers in the production of melon (Ruangwong et al., 2021).
Trichoderma can spread quickly in soil, persist for a long period on the surface of plant roots, and release a number of chemicals (Raj and Sharma, 2009) that can aid in the growth of plants. This study’s findings demonstrated that T. viride agents treatment might stimulate the morphogenesis of melon seedlings, with T3 (6.4 × 105 cfu/g) having the most favorable results. Trichoderma viride Tv 911 was shown by Zaw and Matsumoto (2020) to promote the growth of Japanese mustard, tomato, and radish. As a result, the plant height of mustard and tomato increased by 16.22 and 50.26%, respectively, and the fresh branch and root weight of radish increased by 23.83 and 58.86%, respectively. This is so because Trichoderma regulates the physiological and biochemical metabolism processes of plants (Vinale et al., 2008), which in turn promotes plant growth (Elkelish et al., 2020).
Plants’ physiological metabolism can be enhanced by Trichoderma, in addition to its growth-promoting effects (Harman et al., 2004). The content of physiological chemicals such as MDA and Pro is impacted by the secondary metabolites, such as CAT, POD, APX, SOD, and others, that Trichoderma secretes during plant-microbe contact. Plants may become resistant to these chemicals on a systemic level (Gajera et al., 2016). According to the study’s findings, application of the T. viride agents improved the physiological properties of melons, with application of T3 having the greatest impact. According to Yu et al. (2021), T. asperellum spore solution raised the amount of soluble protein and soluble sugar in leaves, enhanced the activity of nitrate reductase and catalase, and boosted the plant height and stem thickness of tomato seedlings. According to Ahmad et al. (2022) research, T. harzianum Th23 treatment could boost tomato growth, increase the total chlorophyll content of leaves, and boost the activities of protective enzymes like PPO, CAT, and SOD (Abdelkhalek et al., 2022). According to Cao et al. (2022) the growth of continuous cropping cucumber was enhanced by the treatment of both biochar and Trichoderma in combination. There was a notable increase in root activity as well as the activities of POD, CAT, and SOD in leaves, along with a decrease in the amount of MDA. This is due to the fact that Trichoderma is a biological control pathogen that also stimulates plant development. In addition, its secondary metabolites have the ability to create hormone analogs and plant growth regulators, both of which stimulate plant growth (Vinale et al., 2012). This is in line with research conducted by Martínez-Medina et al. (2014) on the impact of four distinct strains of Trichoderma on the biological control activity of melon wilt disease and the promotion of plant growth. The outcomes demonstrated a substantial association between Trichoderma induction of auxin and stimulation of plant development, as well as an increase in auxin content that stimulated plant growth and a decrease in cytokinin and abscisic acid content.
The findings of this study demonstrated that T.viride agents treatment enhanced the physiological properties of melons and encouraged the development of melon yield and quality, with T3 treatment having the most favorable results. The results of a study by Liu L. et al. (2022) on the effects of T. harzianum biofilter on the growth, yield, and quality of bupleurum as well as the microbial reaction revealed that T. harzianum biofilter encouraged bupleurum growth and increased its yield and quality. Bupleurum had an increase in plant height, stem diameter, root diameter, and total plant weight compared to the control, respectively, of 20.52, 21.82, 38.36, and 126.70%. Saikosaponins A, C, and D levels in Bupleurum were also significantly higher thanks to T.harzianum bio-fertilizer, increasing by 8.06, 47.73, and 9.23%, respectively, in comparison to the control. According to Zhang et al. (2023), T. viride T23 enhanced the microbial community’s biomass and diversity, enhanced the physical and chemical characteristics of the soil, boosted the synthesis of soil-active substances, removed a barrier to melon continuous cropping, and encouraged the formation of melon yield. All of these support Fernando et al. (2018) finding that the two isolates of Trichoderma can boost melon production by promoting melon seed germination and seedling growth.
Trichoderma is a common antagonistic microorganism found in nature that can help plants absorb nutrients, enhance the soil environment, speed up the breakdown of organic matter in the soil, and boost crop quality and yield (Rudresh et al., 2005; Wonglom et al., 2020; Harman, 2006). Similar to the findings of Lu et al. (2022), the results of this study showed that T. viride applied in varying amounts can promote the formation of melon yield and quality, with T3 (6.4 × 105 cfu/g) treatment having the best promoting effect. The yield and quality also decreased as the application amount increased. According to Lu et al., applying the Trichoderma microbial agents might boost the output of Lycium barbarum, and the A4 treatment (74.25 kg•hm−2) produced the highest results. Trichoderma microbial agents were used to increase the amounts of soluble solids, lycium barbarum polysaccharide, betaine, total flavonoids, and carotenoids in Lycium barbarum fruits; the A3 (59.40 kg•hm−2) treatment had the greatest impact. In contrast to traditional substrate treatment, Wang et al. (2025) discovered that Trichoderma mixes at varying concentrations can considerably accelerate the growth of watermelon seedlings. The botanical characteristics and material accumulation of watermelon seedlings exhibit a trend of initially growing and then decreasing as the concentration of Trichoderma mixes rise. In comparison to the other treatments, the T3 (106 CFU/g) treatment resulted in higher plant height, stem diameter, fresh mass above ground, dry mass above ground, root length, fresh mass below ground, dry mass below ground, root surface area, number of tips, and root shoot ratio. The results of this study showed that the best concentration for melon growth was 6.4 × 105 CFU/g, which may be attributed to the necessity to maintain a specific degree of diversity and balance in the soil microbial community. Even helpful bacteria, excessive development can squeeze the living space of other microorganisms, resulting to a decline in soil ecosystem variety, which is not conducive to soil health and crop growth (Masquelier et al., 2022). Therefore, it is essential to regulate the volume of application while using various Trichoderma microbial agents. When Trichoderma microbial agents were applied more frequently, plant production and quality rose as well. However, it would seem that this effect is a threshold phenomenon. In other words, too much Trichoderma microbial agents were used, which would have reduced plant quality and productivity. Excessive application levels will also result in higher expenses; if promoted widely, Trichoderma microbial agents will be wasted (Li et al., 2018; Lian et al., 2021).
Numerous researchers have found that Trichoderma has a strong capacity to colonize soil (Zhao et al., 2023), enhance soil physicochemical conditions (Zhu et al., 2022), boost soil fertility (Fu et al., 2019), encourage the formation and upkeep of beneficial microbial communities in soil (Zhang et al., 2018), and increase crop development and yield. The effects of T. viride kf57conidia agents on the soil environment of melon cultivated on soils that have been constantly cropped to melon will be further investigated in future studies.
Overall, our research showed that different T. viride kf57 agent application rates improve the root shoot ratio and seedling strength index of melon seedlings, improve the morphological development at the seedling stage and the physiological and biochemical characteristics at the fruiting stage, and increase the yield and quality of melon grown on soils that are continuously cropped to melon. The most effective of these is the application of 6.4 × 105 cfu/g T. viride kf57 agent. The findings of the study offer fresh concepts for overcoming the challenges associated with melons’ continuous production and encouraging the melon industry’s sustainable growth. Additionally, they offer physiological mechanisms for the quick study and development of Trichoderma agents as well as a foundation for research to guarantee the high-quality and high-yield production of melons grown on soils that are consistently cropped with Trichoderma agents.
The original contributions presented in the study are included in the article/Supplementary material, further inquiries can be directed to the corresponding authors.
JLiu: Conceptualization, Data curation, Investigation, Software, Validation, Visualization, Writing – original draft, Writing – review & editing. HL: Conceptualization, Methodology, Project administration, Supervision, Writing – review & editing. JD: Data curation, Investigation, Writing – review & editing. JLi: Data curation, Investigation, Resources, Writing – original draft. GZ: Investigation, Resources, Writing – review & editing. JW: Conceptualization, Data curation, Investigation, Writing – original draft. GM: Conceptualization, Methodology, Project administration, Supervision, Writing – review & editing. ML: Funding acquisition, Project administration, Supervision, Writing – review & editing.
The author(s) declare that financial support was received for the research, authorship, and/or publication of this article. This work was supported by the National Key Research and Development (R&D) Program Project (2024YFD1400804).
The authors declare that the research was conducted in the absence of any commercial or financial relationships that could be construed as a potential conflict of interest.
All claims expressed in this article are solely those of the authors and do not necessarily represent those of their affiliated organizations, or those of the publisher, the editors and the reviewers. Any product that may be evaluated in this article, or claim that may be made by its manufacturer, is not guaranteed or endorsed by the publisher.
The Supplementary material for this article can be found online at: https://www.frontiersin.org/articles/10.3389/fsufs.2025.1513324/full#supplementary-material
Abdelkhalek, A., Al-Askar, A. A., Arishi, A. A., and Behiry, S. I. (2022). Trichoderma hamatum strain Th23 promotes tomato growth and induces systemic resistance against tobacco mosaic virus. J Fungi 8:228. doi: 10.3390/jof8030228
Ahmad, P., Hashem, A., Abd-Allah, E. F., Alqarawi, A. A., John, R., Egamberdieva, D., et al. (2015). Role of Trichoderma harzianum in mitigating NaCl stress in Indian mustard (Brassica juncea L) through antioxidative defense system. Front. Plant Sci. 6:868. doi: 10.3389/fpls.2015.00868
Ahmad, S., Wang, G. Y., Muhammad, I., Zeeshan, M., and Zhou, X. B. (2022). Melatonin and KNO3 application improves growth, physiological and biochemical characteristics of maize seedlings under waterlogging stress conditions. Biology 11:99. doi: 10.3390/biology11010099
Bernal-Vicente, A., Pascual, J. A., Tittarelli, F., Hernández, J. A., and Diaz-Vivancos, P. (2015). Trichoderma harzianum T-78 supplementation of compost stimulates the antioxidant defence system in melon plants. J. Sci. Food Agric. 95, 2208–2214. doi: 10.1002/jsfa.6936
Boughalleb-M’Hamdi, N., Salem, I. B., and M’Hamdi, M. (2018). Evaluation of the efficiency of Trichoderma, Penicillium, and aspergillus species as biological control agents against four soil-borne fungi of melon and watermelon. Egypt. J. Biol. Pest Control 28:25. doi: 10.1186/s41938-017-0010-3
Cai, Z. L., Bai, J. M., Li, R., He, D. W., Du, R. C., Li, D. Y., et al. (2023). Water and nitrogen management scheme of melon based on yield−quality−efficiency matching perspective under CO2 enrichment. Agric. Water Manag. 285:108379. doi: 10.1016/j.agwat.2023.108379
Cao, X., Zhang, N., Zeng, X. Y., Lan, Q. L., Ma, N., and Wu, C. C. (2022). Effects of biochar and Trichoderma on bacterial community diversity in continuous cropping soil. Hortic. Environ. Biotechnol. 63, 1–12. doi: 10.1007/s13580-021-00373-8
Chong, I. G., and Jun, C. H. (2005). Performance of some variable selection methods when multicollinearity is present. Chemom. Intell. Lab. Syst. 78, 103–112. doi: 10.1016/j.chemolab.2004.12.011
Cui, L., Siskos, L., Wang, C., Schouten, H. J., Visser, R. G. F., and Bai, Y. L. (2022). Breeding melon(Cucumis melo) with resistance to powdery mildew and downy mildew. Horticult. Plant J. 8, 545–561. doi: 10.1016/j.hpj.2022.07.006
Du, P. H., Cao, Y., Liu, H. T., Ji, J. H., Sun, W., Zhang, X. Y., et al. (2024). Dopamine improves apple replant disease resistance by regulating physiological resilience and rhizosphere microbial community structure. J. Integr. Agric. 23, 3025–3044. doi: 10.1016/j.jia.2024.07.011
Elkelish, A. A., Alhaithloul, H. A. S., Qari, S. H., Soliman, M. H., and Hasanuzzaman, M. (2020). Pretreatment with Trichoderma harzianum alleviates waterlogging-induced growth alterations in tomato seedlings by modulating physiological, biochemical, and molecular mechanisms. Environ. Exp. Bot. 171:103946. doi: 10.1016/j.envexpbot.2019.103946
Fernando, D., Milagrosa, S., Francisco, C., and Francisco, M. (2018). Biostimulant activity of Trichoderma saturnisporum in melon (Cucumis melo). Am. Soc. Horticult. Sci. 53, 810–815. doi: 10.21273/HORTSCI13006-18
Fu, J., Xiao, Y., Wang, Y. F., Liu, Z. H., and Yang, K. J. (2019). Trichoderma affects the physiochemical characteristics and bacterial community composition of saline-alkaline maize rhizosphere soils in the cold region of Heilongjiang province. Plant Soil 436, 211–227. doi: 10.1007/s11104-018-03916-8
Gajera, H. P., Zinkal, A., Katakpara, S. V., and Patel, B. A. (2016). Golakiya. Antioxidant defense response induced by Trichoderma viride against Aspergillus niger Van Tieghem causing collar rot in groundnut (Arachis hypogaea L.). Microb. Pathog. 91, 26–34. doi: 10.1016/j.micpath.2015.11.010
Galletti, S., Fornasier, F., Cianchetta, S., and Lazzeri, L. (2015). Soil incorporation of brassica materials and seed treatment with Trichoderma harzianum: effects on melon growth and soil microbial activity. Ind. Crop. Prod. 75, 73–78. doi: 10.1016/j.indcrop.2015.04.030
Gava, C. A. T., and Pinto, J. M. (2016). Biocontrol of melon wilt caused by Fusarium oxysporum Schlect f. sp. melonis using seed treatment with Trichoderma spp. and liquid compost. Biol. Control 97, 13–20. doi: 10.1016/j.biocontrol.2016.02.010
Gou, Q. Q., Song, B., Li, Y. D., Xi, L. L., and Wang, G. H. (2022). Effects of drought stress on annual herbaceous plants under different mixed growth conditions in desert oasis transition zone of the hexi corridor. Sustain. For. 14:14956. doi: 10.3390/su142214956
Grumet, R., McCreight, J. D., McGregor, C., Weng, Y. Q., Mazourek, M., Reitsma, K., et al. (2021). Genetic resources and vulnerabilities of major cucurbit crops. Genes 12:1222. doi: 10.3390/genes12081222
Harman, G. E. (2006). Overview of marhanisms and uses of Trichoderma spp. Phytopathology 96, 190–194. doi: 10.1094/PHYTO-96-0190
Harman, G. E., Howell, C. R., Viterbo, A., Chet, I., and Lorito, M. (2004). Trichoderma species-opportunistic, avirulent plant symbionts. Nat. Rev. Microbiol. 2, 43–56. doi: 10.1038/nrmicro797
Hussain, R. M., Razak, Z. N. R. A., Saad, W. M. M., and Mustakim, M. (2017). Mechanism of antagonistic effects of Andrographis paniculata methanolic extract against Staphylococcus aureus. Asian Pac J Trop Med 10, 685–695. doi: 10.1016/j.apjtm.2017.07.009
Jang, S. J., Park, H. H., and Kuk, Y. I. (2021). Application of various extracts enhances the growth and yield of cucumber (Cucumis sativus L.) without compromising the biochemical content. Agronomy 11:505. doi: 10.3390/agronomy11030505
Kampfenkel, K., Vanmontagu, M., and Inze, D. (1995). Extraction and determination of ascorbate and dehydroascorbate from plant tissue. Anal. Biochem. 225, 165–167. doi: 10.1006/abio.1995.1127
Ku, Y. L., Li, W. Q., Mei, X. L., Yang, X. N., Cao, C. L., Zhang, H. M., et al. (2022). Biological control of melon continuous cropping obstacles: weakening the negative effects of the vicious cycle in continuous cropping soil. Microbiol. Spectr. 10:e0177622. doi: 10.1128/spectrum.01776-22
Kuang, A., Xiaoyun, F., Liu, Z., Chen, Q., Jin, R., and Mao, H. (2024). Biocontrol effect of the complex inoculants of Trichoderma and Bacillus amyloliquefaciens on chrysanthemum white rust. Biocatal. Agric. Biotechnol. 56:103010. doi: 10.1016/j.bcab.2023.103010
Li, M., Ren, Y. F., He, C., Yao, J., Wei, M., and He, X. (2022). Complementary effects of dark septate endophytes and Trichoderma strains on growth and active ingredient accumulation of Astragalus mongholicus under drought stress. J. Fungi 8:920. doi: 10.3390/jof8090920
Li, P. C., Su, X. D., Wang, J. J., Guo, S. J., Li, M., Yuan, Y., et al. (2018). Effects of humic acid fertilizer and biological fertilizer on soil properties and grape yield and quality. Soil Fert. Sci. China 1, 121–126. doi: 10.11838/sfsc.20180120
Lian, H., Li, R. Z., Ma, G. S., Zhao, Z. H., Zhang, T., and Li, M. (2023). The effect of Trichoderma harzianum agents on physiological-biochemical characteristics of cucumber and the control effect against Fusarium wilt. Sci. Rep. 13:17606. doi: 10.1038/s41598-023-44296-z
Lian, H., Ma, G. S., Li, M., Zhang, F., and Zhang, J. M. (2021). Effects of Trichoderma Asperellum agents on physiological characteristics, yield and quality of cucumber. J. China Agric. Univ. 26, 42–52. doi: 10.11841/j.issn.1007-4333
Liu, X. J., Shi, R., Gao, M. F., He, R., Li, Y. M., and Liu, H. C. (2022). Effects of LED light quality on the growth of pepper (Capsicum spp.) seedlings and the development after transplanting. Agronomy 12:2269. doi: 10.3390/agronomy12102269
Liu, L., Xu, Y. S., Cao, H. L., Fan, Y., Du, K., Bu, X., et al. (2022). Effects of Trichoderma harzianum biofertilizer on growth, yield, and quality of Bupleurum chinense. Plant Direct 6:e461. doi: 10.1002/pld3.461
Lu, L. Y., Duan, G. Z., Su, C. F., Guo, H., and Fan, G. H. (2022). Effects of microbial agents on growth and soil properties of Lycium barbarum L. J. Shenyang Agric. Univ. 53, 476–482. doi: 10.3969/j.issn.1000-1700.2022.04.011
Martínez-Medina, A., Del Mar, M., Alguacil, J. A., Pascual, S. C. M., and Wees, V. (2014). Phytohormone profiles induced by Trichoderma isolates correspond with their biocontrol and plant growth-promoting activity on melon plants. J. Chem. Ecol. 40, 804–815. doi: 10.1007/s10886-014-0478-1
Masquelier, S., Sozzi, T., Bouvet, J. C., Bésiers, J., and Deogratias, J.-M. (2022). Conception and development of recycled raw materials (coconut fiber and bagasse)-based substrates enriched with soil microorganisms (Arbuscular Mycorrhizal Fungi, Trichoderma spp. and Pseudomonas spp.) for the soilless cultivation of tomato (S. lycopersicum). Agronomy 12:767. doi: 10.3390/agronomy12040767
Metwally, R. A., and Al-Amri, S. M. (2020). Individual and interactive role of Trichoderma viride and arbuscular mycorrhizal fungi on growth and pigment content of onion plants. Lett. Appl. Microbiol. 70, 79–86. doi: 10.1111/lam.13246
Quazi, S. A. J., Ferdous, J., Shozib, H. B., Khaton, A., and Zaidi, N. W. (2024). Role of Trichoderma asperelloides and Trichoderma brevicompactum in improving drought tolerance in rice. Plant Stress 12:100457. doi: 10.1016/j.stress.2024.100457
Raj, H., and Sharma, S. D. (2009). Integration of soil solarization and chemical sterilization with beneficial microorganisms for the control of white root rot and growth of nursery apple. Sci. Hortic. 119, 126–131. doi: 10.1016/j.scienta.2008.07.025
Rong, Q. L., Li, R. N., Huang, S. W., Tang, J. W., Zhang, Y. C., and Wang, L. Y. (2018). Soil microbial characteristics and yield response to partial substitution of chemical fertilizer with organic amendments in greenhouse vegetable production. J. Integr. Agric. 17, 1432–1444. doi: 10.1016/S2095-3119(18)61946-X
Ruangwong, O.-U., Wonglom, P., Phoka, N., Suwannarach, N., Lumyong, S., Ito, S.-i., et al. (2021). Biological control activity of Trichoderma asperelloides PSU-P1 against gummy stem blight in muskmelon (Cucumis melo). Physiol. Mol. Plant Pathol. 115:101663. doi: 10.1016/j.pmpp.2021.101663
Rudresh, D. L., Shivaprakash, M. K., and Prasad, R. D. (2005). Effect of combined application of Rhizobium, phosphate solubilizing bacterium and Trichoderma spp. on growth, nutrient uptake and yield of chickpea (Cicer aritenium L.). Appl. Soil Ecol. 28, 139–146. doi: 10.1016/j.apsoil.2004.07.005
Sanchez, A. D., Ousset, M. J., and Sosa, M. C. (2019). Biological control of phytophthora collar rot of pear using regional Trichoderma strains with multiple mechanisms. Biol. Control 135, 124–134. doi: 10.1016/j.biocontrol.2019.05.012
Shoam, I., Calderon, C. E., and Levy, M. (2022). Pseudozyma aphidis enhances cucumber and tomato plant growth and yield. Agronomy 12:383. doi: 10.3390/agronomy12020383
Song, S. W., Yi, L. Y., Liu, H. C., Sun, G. W., and Chen, R. Y. (2011). Effect of ammonium and nitrate ratio on nutritional quality of flowering Chinese cabbage. Appl. Mech. Mater. 142, 188–192. doi: 10.4028/www.scientific.net/AMM.142.188
Sood, M., Kapoor, D., Kumar, V., Sheteiwy, M. S., Ramakrishnan, M., Landi, M., et al. (2020). Trichoderma: the “secrets”of a multitalented biocontrol agent. Plan. Theory 9:762. doi: 10.3390/plants9060762
Srivastava, R. K., Singh, R. K., and Prasad, R. D. (2012). Relative antogonistic effect of different isolates of Trichoderma viridi and Trichoderma harzanium against Rhizoctonia solani. Natl. Acad. Sci. Lett. 35, 49–52. doi: 10.1007/s40009-011-0008-4
Tang, S. S., Zhang, Z. R., Liu, X. D., and Gao, Z. G. (2023). Study on screening and degradation effect of autotoxin-degrading bacteria in muskmelon. Agronomy 13:1334. doi: 10.3390/agronomy13051334
Vinale, F., Sivasithamparam, K., Ghisalberti, E. L., Marra, R., Barbetti, M. J., Li, H., et al. (2008). A novel role for Trichoderma secondary metabolites in the interactions with plants. Physiol. Mol. Plant Pathol. 72, 80–86. doi: 10.1016/j.pmpp.2008.05.005
Vinale, F., Sivasithamparam, K., Ghisalberti, E. L., Ruocco, M., Woo, S., and Lorito, M. (2012). Trichoderma secondary metabolites that affect plant metabolism. Nat. Prod. Commun. 7, 1545–1550. doi: 10.1177/1934578X1200701133
Vultaggio, L., Allevato, E., Consentino, B. B., Bellitto, P., Napoli, S., Cannata, C., et al. (2024). Joint action of Trichoderma atroviride and a vegetal derived-protein hydrolysate improves performances of woodland strawberry in Italy. Horticulturae 10:459. doi: 10.3390/horticulturae10050459
Wang, Y. S., Guo, P. Y., Zhang, J. L., Xie, Q. L., Shen, H., Hu, Z. L., et al. (2021). Overexpression of the MADS-box gene SlMBP21 alters leaf morphology and affects reproductive development in tomato. J. Integr. Agric. 20, 3170–3185. doi: 10.1016/S2095-3119(21)63638-9
Wang, B., Huang, Z. Y., Li, W. M., Ma, M., Chen, L. L., Liu, Q. Y., et al. (2025). Effects of Trichoderma mixtures with different concentrations on seedling rearing of watermelon. China Cucurb. Veget. 38, 1–8. doi: 10.16861/j.cnki.zggc.2024.0330
Wonglom, P., Ito, S.-i., and Sunpapao, A. (2020). Volatile organic compounds emitted from endophytic fungus Trichoderma asperellum T1 mediate antifungal activity, defense response and promote plant growth in lettuce (Lactuca sativa). Fungal Ecol. 43:100867. doi: 10.1016/j.funeco.2019.100867
Woo, S. L., Scala, F., Ruocco, M., and Lorito, M. (2006). The molecular biology of the interactions between Trichoderma spp., phytopathogenic fungi, and plants. Phytopathology 96, 181–185. doi: 10.1094/PHYTO-96-0181
Xu, W. W., Li, H. B., Ma, Q. Q., Mu, S. M., Zhao, Z. J., Gao, J. X., et al. (2024). Exploring the mitigation effect of microbial inoculants on the continuous cropping obstacle of capsicum. Sci. Hortic. 338:113507. doi: 10.1016/j.scienta.2024.113507
Xu, T. F., Yang, X., Zhang, M., Guo, S. H., Fu, W. J., Zhou, B. J., et al. (2022). The use of widely targeted metabolite profiling to reveal the senescence changes in postharvest 'Red Globe'(Vitis vinifera) grape berries. J. Integr. Agric. 21, 1028–1043. doi: 10.1016/S2095-3119(21)63725-5
Yani, S., Opik, T., Belinda, N., and Epa, P. (2019). Role of biological agents Trichoderma sp. at growth three garlic varieties (Allium sativum). J. Phys. Conf. Ser. 1402:033043. doi: 10.1088/1742-6596/1402/3/033043
Yu, Z. Y., Wang, Z. Y., Zhang, Y. Z., Wang, Y. C., and Liu, Z. H. (2021). Biocontrol and growth-promoting effect of Trichoderma asperellum TaspHu1 isolate from Juglans mandshurica rhizosphere soil. Microbiol. Res. 242:126596. doi: 10.1016/j.micres.2020.126596
Zaw, M., and Matsumoto, M. (2020). Plant growth promotion of Trichoderma virens, Tv911 on some vegetables and its antagonistic effect on Fusarium wilt of tomato. Environ. Contr. Biol. 58, 7–14. doi: 10.2525/ecb.58.7
Zhang, F. G., Huo, Y. Q., Xu, X. X., Hu, J., Sun, X., Xiao, Y., et al. (2018). Trichoderma improves the growth of Leymus chinensis. Biol. Fertil. Soils 54, 685–696. doi: 10.1007/s00374-018-1292-7
Zhang, Q., Li, Y., Sun, X., Xing, S. Y., Cong, W., and Liu, X. J. (2019). Study on dormancy mechanism and breaking dormancy method of Viburnum sargentii seeds. Am. J. Plant Sci. 10, 65–78. doi: 10.4236/ajps.2019.101007
Zhang, Z. R., Tang, S. S., Liu, X. D., Ren, X. L., Wang, S. N., and Gao, Z. G. (2023). The effects of Trichoderma viride T23 on rhizosphere soil microbial communities and the metabolomics of muskmelon under continuous cropping. Agronomy 13:1092. doi: 10.3390/agronomy13041092
Zhang, J., Tian, H. M., Wang, P. C., Xiao, Q. Q., Zhu, S. J., and Jiang, H. K. (2019). Variations in pH significantly affect cadmium uptake in grafted muskmelon (Cucumis melo L.) plants and drive the diversity of bacterial communities in a seedling substrate. Plant Physiol. Biochem. 139, 132–140. doi: 10.1016/j.plaphy.2019.03.013
Zhao, J., Liu, T., Liu, W. C., Zhang, D. P., Dong, D., Wu, H. L., et al. (2021). Transcriptomic insights into growth promotion effect of Trichoderma afroharzianum TM2-4 microbial agent on tomato plants. J. Integr. Agric. 20, 1266–1276. doi: 10.1016/S2095-3119(20)63415-3
Zhao, X., Wang, Y. F., Wang, J. J., Wang, P. Y., Wang, G. D., Zhu, L. X., et al. (2023). Trichoderma affects crop growth and soil ecological environment. J. Agric. Sci. Technol. 25, 166–172. doi: 10.13304/j.nykjdb.2022.0456
Zheng, Y. J., Zhang, Y. T., Liu, H. C., Li, Y. M., Liu, Y. L., Hao, Y. W., et al. (2018). Supplemental blue light increases growth and quality of greenhouse pak choi depending on cultivar and supplemental light intensity. J. Integr. Agric. 17, 2245–2256. doi: 10.1016/S2095-3119(18)62064-7
Keywords: continuously cropped, melon, physiological and biochemical indices, quality, Trichoderma viride agents, yield
Citation: Liu J, Lian H, Dou J, Li J, Zhu G, Wang J, Ma G and Li M (2025) Physiological impact of Trichoderma viride agents on the quality and production of melon that is grown on soils continuously cropped to melon. Front. Sustain. Food Syst. 9:1513324. doi: 10.3389/fsufs.2025.1513324
Received: 18 October 2024; Accepted: 31 January 2025;
Published: 12 February 2025.
Edited by:
Mohamed Ait-El-Mokhtar, University of Hassan II Casablanca, MoroccoReviewed by:
Theophilus Kwabla Tengey, CSIR-Savanna Agricultural Research Institute, GhanaCopyright © 2025 Liu, Lian, Dou, Li, Zhu, Wang, Ma and Li. This is an open-access article distributed under the terms of the Creative Commons Attribution License (CC BY). The use, distribution or reproduction in other forums is permitted, provided the original author(s) and the copyright owner(s) are credited and that the original publication in this journal is cited, in accordance with accepted academic practice. No use, distribution or reproduction is permitted which does not comply with these terms.
*Correspondence: Hua Lian, eXk2ODE5MTg0QDEyNi5jb20=; Mei Li, bGltZWlAY2Fhcy5jbg==; Guangshu Ma, bWdzX2xoQDE2My5jb20=
Disclaimer: All claims expressed in this article are solely those of the authors and do not necessarily represent those of their affiliated organizations, or those of the publisher, the editors and the reviewers. Any product that may be evaluated in this article or claim that may be made by its manufacturer is not guaranteed or endorsed by the publisher.
Research integrity at Frontiers
Learn more about the work of our research integrity team to safeguard the quality of each article we publish.