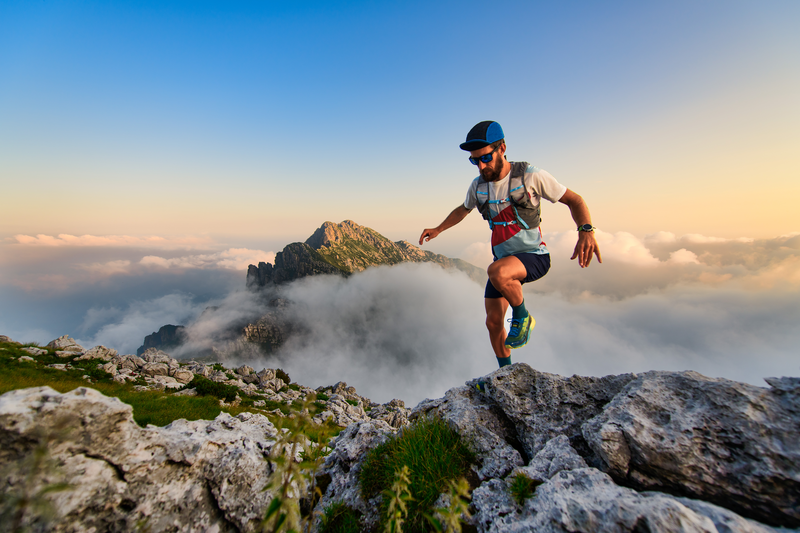
94% of researchers rate our articles as excellent or good
Learn more about the work of our research integrity team to safeguard the quality of each article we publish.
Find out more
ORIGINAL RESEARCH article
Front. Sustain. Food Syst. , 05 February 2025
Sec. Agroecology and Ecosystem Services
Volume 9 - 2025 | https://doi.org/10.3389/fsufs.2025.1489291
This article is part of the Research Topic Conservation Agriculture for Sustainable Food Production Systems View all 20 articles
Tahiti lime is one of the most exported fruits in Brazil, and the state of São Paulo accounts for more than half of the country’s fruit production, and drought in the region is a growing concern as rainfall distribution has become increasingly unpredictable. Therefore, identifying the best varieties and management strategies for citrus groves has become a subject of increasing research. This experiment was conducted at the “Sylvio Moreira” Citrus Center, Brazil; the experiment focused on different planting and mulching practices and scion-rootstock combinations. Two planting/management types were tested: (i) conventional tillage – CT (no mulch in the citrus row) and no-tillage – NT (mulch maintained in the citrus row), using Urochloa ruziziensis as an intercrop. Six grafts x rootstocks were tested: Tahiti acid IACC 5, IAC 10, and BRS IAC EECB Ponta Firme grafted onto two dwarf rootstocks: trifoliate orange IAC 718 Flying Dragon and citrandarin IAC 3152 Itajobi. Over three harvests (2022–2024), this study evaluated foliar nutrition, fruit quality, root development, water potential, gas exchange, water deficit biomarkers, and overall yield. No-tillage significantly improved vital performance indicators compared to conventional tillage. Plants managed with NT exhibited superior root development, greater water uptake, and greater leaf water potential. They also showed greater photosynthetic efficiency, leading to higher yields and improved fruit quality, particularly in juice yield and soluble solids/acidity ratio. The IAC 3152 Itajobi rootstock demonstrated remarkable resilience to drought stress, outperforming Flying Dragon in root development and physiological metrics. NT promoted healthier root systems and more robust plant growth. In contrast, conventional tillage led to poorer root growth, lower nutrient uptake, and increased disease susceptibility due to soil exposure and reduced water infiltration. Despite these challenges, 3,152 rootstock maintained active physiological responses such as gas exchange and/or water potential, especially with the Firm Tip graft. In conclusion, the integration of dwarf rootstocks with NT practices offers a viable strategy to increase the sustainability and productivity. This study highlights the importance of selecting appropriate scion-rootstock combinations and adopting sustainable management practices to improve resilience to environmental stress and optimize fruit yield and quality.
Citrus fruit plays an essential role in the global economy, highlighting Brazil’s position as one of the world’s largest producers of this genus, especially sweet oranges [Citrus sinensis (L.) Osbeck]. However, Brazil’s production of limes and lemons has grown, with more than 1.6 million tons of fruit in the 2022 harvest, and Tahiti acid lime (Citrus latifolia Yu. Tanaka) was responsible for 90% of this harvest. Furthermore, the state of São Paulo represented more than 55% of Brazilian exports of Tahiti acid lime and produced approximately 1.2 million tons of fruit in 2022 (IBGE, 2023). Due to their perennial nature, citrus groves have an estimated lifespan of 15–20 years (Talon et al., 2020), thus there is high exposure to adverse environmental conditions (Shafqat et al., 2021a). Which are of concern about, particularly increasing temperature and decreasing in rainfall (Abobatta, 2023; Lyra et al., 2018).
Flowering of citrus starts in September and October, in tropical regions and is considered a critical period for fruit production (Reuther et al., 1968). If an increase in temperature and drought occurs after pollination, fertilization of the ovule is inhibited, which reduces fruit set, stimulates fruit drop, and reduces the final yield (Shafqat et al., 2021a). The developmental stages of fruit can also be affected by drought events, reducing their growth (García-Sánchez et al., 2007). Water deficit reduces stomatal conductance, leaf transpiration rate, and carbon dioxide (CO2) assimilation in citrus (Miranda et al., 2021), which can promote oxidative stress in the plant, leading to dissociation of its cell membranes due to free radical accumulation (De Sousa et al., 2022). In addition, only 36.6% of the entire area planted with citrus in the Brazilian citrus belt is irrigated; thus, water management used both to stimulate development and productivity and to mitigate unfavorable environmental conditions is still limited (Fundecitrus, 2024). This makes it impossible to rely on water supplementation on a large scale, making it necessary to use varieties more tolerant of the climatic conditions and soil management methods that help trees acclimatize, guaranteeing high productivity.
Knowledge of species tolerant to these conditions can boost yield in citrus orchards. Therefore, grafting through budding, which combines the desirable characteristic of the scion (bud) and the rootstock (nucellar seed), is an important technique commercially used in Citrus production. The scion, the upper part of the plant, is selected based on its physicochemical fruit qualities (such as mass, juice yield, peel color, acidity, and soluble solids), while rootstocks are chosen based on their vigor, plant architecture, resistance to specific diseases, tolerance to climatic conditions, precocity, and productivity, in addition to compatibility with the scion (De Sousa et al., 2022). Using citrus rootstocks is essential, as they guarantee benefits to the scion, such as ease of management and, critically, tolerance to climatic factors (Mattiuzi et al., 2019).
In addition to selecting rootstocks that are tolerant of adversity factors, it is also helpful for increasing orchard planting density. Some rootstocks induce smaller sizes in the scion variety by reducing vigor (Donadio et al., 2019). Dwarf rootstocks have been sought, enabling higher orchard density and increasing the number of trees per cultivated area, consequently increasing the total fruit harvested (Bowman and Joubert, 2020). In Brazil, the trifoliate orange Flying Dragon (Poncirus trifoliata var. monstrosa (T. Itô) Swingle) is a dwarf rootstock widely used due to its dwarfing capacity and early production (De Azevedo et al., 2020). However, it has disadvantages, such as low drought tolerance and incompatibility with some commercial scions. Consequently, new dwarf and productive rootstocks are needed for greater diversification given the new demands for more sustainable production systems (Pereira Costa et al., 2021). A viable alternative is to use trifoliata hybrids with mandarins (citrandarins). These Citrus sunki × Poncirus trifoliata cv. Rubidoux hybrids result in smaller sizes recognized as dwarf or semi-dwarf, good productivity, and water deficit tolerance in the scion variety (Schinor et al., 2015).
On a global scale, most soil management in citrus groves is still not sustainable, keeping the soil exposed and associated with the intensive use of herbicides (Niu et al., 2021). This conventional soil management in orchards leads to soil disturbance and the use of machinery to incorporate the surface layer with cultural remains. However, this leads to soil compaction, reduced water infiltration, increased surface runoff, and greater sediment production (Cárceles Rodríguez et al., 2022). In addition, it exposes the roots to disease, such as gummosis, and to the high temperatures of the exposed soil (De Azevedo et al., 2020; Martinelli et al., 2022). Finally, conventional soil management contributes to the significant loss of organic carbon from the soil, which goes against the current demands for more sustainable production (Visconti et al., 2024).
One alternative of conservation citriculture is no-tillage which is the use of natural mulching, in which the inter-rows are covered with grass species, such as Urochloa spp., and this system is managed with ecological mower, which projects all cover crop shoot residue under the citrus plants (De Azevedo et al., 2020, Martinelli et al., 2017; Melo et al., 2023), moderating soil temperatures and providing a better water content due to the soil covering that inhibits evapotranspiration (de Almeida et al., 2018). There are also improvements as greater porosity, better infiltration, less erosion, and even decompaction. Additionally, it aids in weed management, lowering herbicide costs and overall expenses and thus promoting sustainable production (Busi et al., 2020).
A fast and efficient method to assess the impact of climate change is based on the physiological responses of plants, which directly influence their growth and productivity (Hayat et al., 2022a). Based on this premise, this study aimed to analyze the physiological and productive responses of six scion-rootstock combinations using different planting systems and cultural practices, considering the use of Urochloa as intercropping, and the consequent production and transfer (no-tillage), or not (conventional tillage) of biomass from the inter-rows to the row of citrus planting, since the citrus planting, in rainfed conditions. So, the main hypothesis of this research is that integrating dwarfing rootstocks with sustainable management practices, such as no-tillage planting with mulch, improves the sustainability and productivity of Tahiti acid lime. This is due to the potential of these practices to promote root development, increase photosynthetic efficiency, and improve water retention and fruit quality, especially under water deficit conditions.
The experiment was established in March 2020 at the “Sylvio Moreira” Citriculture Center of the Agronomic Institute (IAC) in Cordeirópolis, São Paulo state, Brazil. Before planting, Urochloa ruziziensis was established as a permanent cover crop in the inter-rows of the orchard. Twelve-month-old seedlings of Tahiti acid were planted in seven rows with a 6.0 m (inter-rows) × 2.5 m (inter-trees) spacing (769 tree ha−1). The trial was set up in a randomized block design, in a split plot scheme, with four replications. The two types of planting/management were allocated to the plots:
i. Conventional tillage (CT) – citrus planting: with disc plow and disc harrow to open furrows; the soil in the inter-rows with U. ruziziensis intercropped; cultural practices: conventional mowing in the inter-rows, maintaining the biomass in the inter-rows of the citrus orchard, without mulch in the row and manual weeding in the rows, three times a year (October to March).
ii. No-tillage (NT) – citrus planting: without disc plow or disc harrow; opening furrows in the mulch of U. ruziziensis intercropped; cultural practices: include ecological mowing in the inter-rows, U. ruziziensis intercropped, moving biomass to rows under the citrus tree, and manual weeding in the rows, three times per year (October to March), see Figure 1.
Figure 1. Aerial view of part of the experimental area, highlighting the two planting systems (Cordeirópolis, São Paulo State, Brazil).
In the split plots, six scions × rootstock combinations were evaluated: (i) Tahiti acid lime IAC 5 (5) grafted onto trifoliata IAC 718 Flying Dragon (FD); (ii), Tahiti acid lime IAC 10 (10) grafted onto FD; (iii) Tahiti acid lime BRS IAC EECB Ponta Firme (PF) grafted onto FD; (iv) 5 grafted onto citrandarin IAC 3152 Itajobi (152); (v) 10 grafted onto 152; (vi) PF grafted onto FD. Both roostscks are dwarf. Each split plot consisted of five trees of each scion–rootstock combination in the row and two inter-rows (U. ruziziensis intercropped) for each proposed management strategy.
Mature leaf samples were collected from the middle third of fruit-bearing branches during the summer (February 2023) to determine the total macronutrient (nitrogen, N; phosphorus, P; potassium, K; calcium, Ca; magnesium, Mg; sulfur, S; measured in g kg−1) and micronutrient concentrations (boron, B; copper, Cu; iron, Fe; manganese, Mn; zinc, Zn; measured in mg kg−1) following digestion of the plant material (Bataglia et al., 1983). The third leaf was collected from the fruit branches, with four trees sampled per plot and five leaves per plant.
Five fruits were sampled per plot (n = 15) in the summer (February 2024) for quality physicochemical measurements. The total fruit mass was obtained using an electronic scale with a maximum capacity of 15 kg. The juice content was determined after juicing fruit, calculated using the juice weight/fruit weight ratio, and expressed as a percentage. The acidity (g 100 g−1 in 0.3125 N NaOH solution) was measured using phenolphthalein as an indicator, and the acidity concentration was expressed as a percentage. The soluble solids content (°Brix), was determined by direct reading in a refractometer (B’S model RFM 330); the soluble solids (SS)/titratable acidity (TA) ratio was calculated, indicating the maturate stage. The contents of ascorbic acid (vitamin C) were determined through pipetting, using 2 mL of juice with 50 mL of 1% oxalic acid solution. The solution was titrated using the DCPIP solution (2,6-dichlorophenolindophenol) until the pink color persisted for 15 s; the result was expressed in mm.100 g−1 of juice using Tillman’s solution.
Acrylic tubes (minirhizotron) were installed 40 cm from the trunk of the plants in June 2021. Using a CI-600 root scanner (MODEL CI-600-TA66), images were taken from inside the access tube at depths of 0–20 cm in the summer (January 2022, 2023, and 2024) to monitor annual growth spurts. The images were analyzed using RootSnap® software (CID BioScience Inc., Camas, WA, USA) to obtain the observed area’s root length (mm).
Data were obtained during the winter from August 2022 to August 2024, corresponding to the dry season in Cordeirópolis, São Paulo state, Brazil. Undamaged Tahiti acid lime leaves were collected from different rootstocks/treatments in the middle third of the plant before sunrise, following the recommendations of Machado et al. (2002). A Scholander-type pressure chamber was used with a PMS Instrument (model 1,000 Corvallis, USA) to assess the water potential of the leaves, and the values were expressed in MPa.
The evaluations were performed during the summer (January 2024) and winter (August 2024) periods. The stomatal conductance to water vapor (gs), transpiration (E), photosynthesis (A), and intercellular CO2 concentration (Ci) were measured in vivo for all plants. The analyses were performed in the youngest, fully expanded leaf using a portable infrared gas analyzer (LCpro, ADC, Hoddesdon, UK). Evaluations were performed between 8 and 10 a.m. under ambient temperature, partial carbon dioxide pressure, and air-water vapor pressure. Photosynthetic active radiation (PAR) of 1,000 μmol m−2 s−1 was supplied by a light unit mounted on top of the leaf chamber.
Lipoperoxides were determined during summer (January 2024) and winter (August 2024). Undamaged Tahiti acid lime leaves were collected from the different rootstocks/treatments in the middle third of the plant, free of pests and disease symptoms, free of flowers, or free of fruits. A 0.3-g leaf tissue sample was homogenized with 0.5 mL of 0.1% TCA (trichloroacetic acid), which was then centrifuged for 10 min (15,000 × g, 4.0°C). Subsequently, 1 mL of the supernatant was collected and mixed with 2 mL of 0.25% TBA (2-thiobarbituric acid) diluted in 10% TCA in a test tube. The mixture was then incubated in a water bath at 95°C for 25 min, and the absorbance was finally measured at 532 and 600 nm in a spectrophotometer (Multiskan, Thermo Fisher Scientific, MA, USA). The data were calculated and expressed in mmol of lipoperoxides per gram of fresh tissue.
At the same time as lipoperoxide analysis, the proline content in Tahiti lime leaves was measured by homogenizing 0.5 g of fresh leaf tissue in 10 mL of 3% sulfosalicylic acid, followed by shaking the tubes every 15 min for 1 h. The samples were then centrifuged for 10 min (10,000 × g), and a 2-mL aliquot of the supernatant was collected for absorbance reading at 520 nm in a spectrophotometer (Multiskan, Thermo Fisher Scientific, MA, USA). The data were calculated and expressed in μmol of proline per gram of fresh tissue.
From 2022 to 2024, monthly evaluations were conducted following the appearance of ripe fruit in the orchard. The harvest and weight of all fruit and the cumulative productivity of each year, expressed as yield (t ha−1), were determined. All evaluations were performed in four central trees per plot with four replications.
Data were subjected to analysis of variance (ANOVA), and differences between the means were evaluated using Tukey’s test at a 5% probability level with the statistical software R (version 2023.12.1. Foundation for Statistical Computing, Vienna, Austria). Normality tests were previously performed. Multivariate analyses using principal component analysis (PCA) were carried out based on a correlation matrix of root length, water potential, CO2 assimilation, lipoperoxides, proline, and plant yield obtained in 2024 to analyze the interaction of the physiological responses in the productivity with different citrus combinations and two types of planting/management. PCA data were normalized and displayed using a biplot of principal component distances from their mean values. The data were standardized for hierarchical clustering of rootstock PCA, and Euclidean distance was used to measure similarity. The average method was employed to assess linkage (Hair et al., 2010). Cluster analysis was calculated using the “NbClust” package in R 3.6.1 (Charrad et al., 2014). PCA was performed using the FactoMineR package in R (Le et al., 2008). Only components with eigenvalues equal to or greater than 1 were considered (Kaiser, 1958).
The data obtained from the leaf nutritional analysis did not show significant interaction between planting types and scion x rootstock combinations (data not shown); however, there was a difference between no-tillage (NT) and conventional tillage (CT), where all nutrient contents evaluated, except Ca, were significantly higher under NT than those obtained from the leaves of plants in the CT treatment (Table 1). Except Ca and S, all other nutrients were influenced by the scion x rootstock combination. The N values, especially that for BRS IAC EECB Ponta Firme grafted onto trifoliate orange IAC 718 Flying Dragon (PF_FD), were lower than the recommendations for Tahiti acid lime (Mattos et al., 2020). The Mg content recommended for the crop is below. The micronutrient Fe was adequate in all combinations, except with scion IAC 10 on rootstocks 152 (10_152) and FD (10_FD). Mn reached the recommended values only in combinations 5_152, PF_152, and 5_FD. Adequate Zn contents for Tahiti acid lime (35–50 mg kg−1) were only reached in combination with PF_152 (Table 1).
Table 1. Leaf nutrient contents in different scion × rootstock combinations of Tahiti acid lime IAC 10 (10); IAC 5 (5) and BRS IAC EECB Ponta Firme (PF) grafted onto citrandarin IAC 3152 Itajobi (152) and trifoliate orange IAC 718 Flying Dragon (FD) under different types of planting: conventional (CT) and no-tillage (NT).
The combinations 10_152 and PF_152 provided heavier fruit in the NT treatment. Scion × rootstock combinations 5_152, PF_152, and PF_FD maintained under CT showed greater fruit mass than the others, while the fruit of combination PF_152 maintained in no-tillage showed a more significant average mass than 10_FD and 5_FD in the same conditions (Figure 2A). The combinations PF with 152 and 10, 5, and PF with FD showed lower yields with CT compared to the same combinations with NT. Furthermore, 10_FD showed the lowest juice yield in CT, while 5_FD and PF_FD were statistically inferior to combination PF_152. Through NT, combination PF_152 stood out and was superior to 5_152, 10_FD, and 5_FD (Figure 2B).
Figure 2. Physical quality: (A) Mass (g), (B) Juice yield [% (m m-1)], of fruits of the combinations scions x rootstocks: Tahiti acid lime IAC 10 (10); IAC 5 (5) and BRS IAC EECB Ponta Firme (PF) grafted onto citrandarin IAC 3152 Itajobi (152) and trifoliate orange IAC 718 Flying Dragon (FD) under conventional (CT) and no-tillage (NT). Summer 2024. *Averages followed by the same lowercase letters for each type of planting/management (tillage) within each different scion × rootstock combination, uppercase for scion on the same rootstock with the same types of planting/management, do not differ by Tukey’s test (p < 0.05). Bars indicate the least significant difference.
The values of ratio obtained from all combinations under NT were higher than those under CT. Moreover, when analyzing the types of planting/management individually, the PF_152 combination was significantly higher than 10_152, 5_FD, and PF_FD managed CT, demonstrating its better capacity for fruit development and ripening, even in CT. Bellow NT, the superiority of all scions grafted onto 152 rootstock was evident compared to the 10_FD and 5_FD combinations, reflecting better development and faster ripening of the fruits from this rootstock (Figure 3A). The average ascorbic acid concentration showed a significant interaction between types of planting/management and scion × rootstock combinations, with increased concentrations in all plants int. the CT treatment. Under this unprotected management, 10_152, 10_FD, and 5_FD had the highest levels of ascorbic acid in their fruit juice. Under NT, 10_152 showed higher values than 10_FD and 5_FD (Figure 3B).
Figure 3. Chemical quality: (A) Ratio (g), (B) Ascorbic acid (mg 100 mL−1), of the juice of the fruits of the combinations scion × rootstocks: Tahiti acid lime IAC 10 (10); IAC 5 (5) and BRS IAC EECB Ponta Firme (PF) grafted onto citrandarin IAC 3152 Itajobi (152) and trifoliate orange IAC 718 Flying Dragon (FD) under tconventional (CT) and no-tillage (NT). Summer 2024. *Averages followed by the same lowercase letters for each types of planting/management (tillage) within each scion × rootstock combination, uppercase for scion on the same rootstock with the same types of planting/management, do not differ by Tukey’s test (p < 0.05). Bars indicate the least significant difference.
In all scions × rootstock combinations, a greater root length was observed under NT. In CT, 10_152 and PF_152 had the best root development, while all canopy combinations grafted on FD had the lowest averages. In the NT treatment, PF_152 showed the highest average root length (mm) compared to the 10, 5, and PF canopies on FD (Figure 4A). In 2023, all combinations under NT were statistically superior to the average obtained in the CT treatment. For CT, the 10, 5, and PF scions on 152 rootstocks were superior to 10_FD and 5_FD. Under NT, PF_152 had the highest root length average, and 10_FD and 5_FD had the lowest (Figure 4B). In January 2024, NT stimulated better root development. Under CT, 10_FD and 5_FD presented the shortest root lengths. In addition, the PF_152 combination presented the most significant root development for two consecutive years (Figure 4C).
Figure 4. Root length (mm): (A) 2022 summer, (B) 2023 summer, and (C) 2024 summer, of the trees of the combinations scion × rootstocks: Tahiti acid lime IAC 10 (10); IAC 5 (5) and BRS IAC EECB Ponta Firme (PF) grafted onto citrandarin IAC 3152 Itajobi (152) and trifoliate orange IAC 718 Flying Dragon (FD) under conventional (CT) and no-tillage (NT). *Averages followed by the same lowercase letters for each types of planting/management (tillage) within each scion × rootstock combination, uppercase for scion on the same rootstock with the same types of planting/management, do not differ by Tukey’s test (p < 0.05). Bars indicate the least significant difference.
The data obtained in the winter of 2022 and 2023 showed an interaction between types of planting/management and scion × rootstock (Figures 5A,B). When comparing the effect of the types of planting/management on the scion × rootstock combinations, only 10_FD did not show significant differences between tillage types. In contrast, all other combinations had a higher water potential when managed in NT (with mulch in the row). Within CT, the three combinations with FD—10_FD, 5_FD, and PF_FD had the lowest values in MPa. Under NT, the same trend was observed, but PF_FD had less damage (Figure 5A). In 2023, in addition to 10_FD, the 5_152 combination did not show differences between the types of planting/management. However, the other plants had a higher water potential when subjected to management with mulch deposition. In CT, 5_FD and PF_FD had the lowest water potentials, while under NT, 10_152 was superior to 5_FD and PF_FD (Figure 5B). The analyses conducted in winter 2024 showed differences between the two management practices applied to the combinations, except for 10_152, which was superior to 5_152 and all combinations (10, 5, and PF) on rootstock FD under CT. In the NT treatment, the 152 rootstock with all scions provided higher water potential values, with averages below −1 MPa (Figure 5C).
Figure 5. Water potential (MPa): (A) 2022 winter, (B) 2023 winter, and (C) 2024 winter, in the trees of the combinations scion × rootstocks: Tahiti acid lime IAC 10 (10); IAC 5 (5) and BRS IAC EECB Ponta Firme (PF) grafted onto citrandarin IAC 3152 Itajobi (152) and trifoliate orange IAC 718 Flying Dragon (FD) conventional (CT) and no-tillage (NT). *Averages followed by the same lowercase letters for each type of planting/management (tillage) within each scion × rootstock combination, uppercase for scion on the same rootstock with the same types of planting/management strategy, do not differ by Tukey’s test (p < 0.05). Bars indicate the least significant difference.
The data obtained in the summer of 2024 did not show significant differences in the average intercellular CO2 concentration (Ci). However, there was a significant interaction between the types of planting/management and scion × rootstock combinations for average stomatal conductance. Conventional tillage provided more significant stomatal opening in plants of the 10_152 combination compared to PF_152, 10_FD, 5_FD, and PF_FD. However, under NT, 10_152 presented higher values than all scions (10, 5, and PF) on the FD rootstock (Figure 6A). The average transpiration (E) measured during the summer was higher in almost all combinations under NT, except for the 5_FD combination. In the CT treatment, 10_152 and PF_152 had higher transpiration rates than 10_FD. Through NT, these same combinations had higher averages than the others (Figure 6B). The CO2 assimilation indicated that NT was superior in almost all combinations, except for 10_FD. However, under CT, all scions (10, 5, and PF) on the 152 rootstock showed higher CO2 assimilation compared to the means evaluated in the same scions grafted onto FD (Figure 6C).
Figure 6. Gas exchange and stomatal conductance (gs, mol m−2 s−1) in (A) summer and (D) winter. Transpiration (E, mmol m−2 s−1) in (B) summer and (E) winter. CO2 assimilation (A), (μmol m−2 s−1) in (C) summer and (F) winter of 2024, in the trees of different scion × rootstock combinations: Tahiti acid lime IAC 10 (10); IAC 5 (5), and BRS IAC EECB Ponta Firme (PF) grafted onto citrandarin IAC 3152 Itajobi (152) and trifoliate orange IAC 718 Flying Dragon (FD) under conventional (CT) and no-tillage (NT). *Averages followed by the same lowercase letters for each type of planting/management (tillage) within each scion × rootstock combination, uppercase for scion on the same rootstock with the same types of planting/management (tillage), do not differ by Tukey’s test (p < 0.05). Bars indicate the least significant difference.
During winter 2024, no significant interactions were observed in the Ci data. However, the types of planting/management averages showed a statistical difference, with NT outperforming CT, with 298 and 289 mol m−2 s−1, respectively. During the same period, stomatal conductance values exhibited a double factorial interaction, with a 37.9% reduction in the average compared to that obtained in the summer. Additionally, combinations 10_152, 5_152, and PF_FD showed greater stomatal opening when maintained under NT. Within CT, all scions (10, 5, and PF) on the 152 rootstocks were superior to 10_FD and 5_FD, which were inferior to the other combinations managed in NT (Figure 6D). Transpiration in winter decreased by 27.7% compared to the average in summer.
Moreover, CT stimulated lower foliar transpiration in all combinations, with 5_152 standing out compared to the others. Bellow NT, 5_152, and 10_FD had higher transpiration averages than 5_FD (Figure 6E). CO2 assimilation evaluated in winter showed a 21.8% reduction in the average compared to summer. Only combinations with Flying Dragon showed lower assimilation under CT, with no distinctions between the management methods in combinations with Itajobi. Within both CT and NT, 5_FD had the lowest average among the plants in the experiment (Figure 6F).
During the summer of 2024, CT stimulated a higher lipoperoxide concentration in fresh tissue of all combinations with 152 rootstock and 5_FD. In addition, under this management without mulching (CT), the 10_152 and PF_FD combinations had higher concentrations than the other combinations and were superior to the 5_152 and 5_FD combinations under NT (Figure 7A). The proline concentrations in fresh tissue recorded during the same period showed higher averages under CT in almost all combinations, except 5_FD and PF_FD. When analyzing CT separately, 5_152 and PF_152 were superior to all combinations (10, 5, and PF) with the FD rootstock. In the NT treatment, 5_152 had the highest average of determined amino acids (Figure 7B).
Figure 7. Water deficit biomarkers. Lipoperoxides (mmol. g−1) on (A) summer and (C) winter. Proline (μmol. g−1) on (B) summer and (D) winter of 2024, in the trees of the combinations scion × rootstocks: Tahiti acid lime IAC 10 (10); IAC 5 (5) and BRS IAC EECB Ponta Firme (PF) grafted onto citrandarin IAC 3152 Itajobi (152) and trifoliate orange IAC 718 Flying Dragon (FD) under conventional (CT) and no-tillage (NT). *Averages followed by the same lowercase letters for each type of planting/management (tillage) within each scion × rootstock combination, uppercase for scion on the same rootstock with the same types of planting/management, do not differ by Tukey’s test (p < 0.05). Bars indicate the least significant difference.
In the winter of the same year, the average lipoperoxide content in the leaves doubled; this time, only the 5_152, 10_FD, and 5_FD combinations had lower averages under NT. In the CT treatment, the 10_152 and PF_152 combinations had the lowest averages; under NT, they were lower than 10_FD and PF_FD (Figure 7C). Combinations 10_152, PF_152, and PF_FD showed significant differences in the proline concentration between treatments, with conventional tillage stimulating a higher proline concentration in fresh tissue than those under NT. Within CT, 10_152 and PF_152 had the highest proline concentrations (Figure 6D) and were inversely proportional to the low lipoperoxide concentration in these two combinations (Figure 7C). Bellow NT, there was an increase of 24.9% compared to summer, and the 5_152 combination showed the highest proline concentration in the leaf tissues (Figure 7D).
All combinations, except for 10_FD, showed higher productivity in NT in the 2022 harvest (Figure 8A). In CT, the BRS IAC EECB Ponta Firme scion showed lower productivity on both rootstocks than 10_152, 10_FD, and 5_FD. Within NT, 10_FD had the lowest average compared to the other combinations (Figure 8A). The 2023 harvest, also showed higher productivity in all combinations under NT. In CT, the 10_FD and 5_FD combinations were the least productive. In the NT treatment, 10_152 and PF_152 showed the highest averages in tons per hectare (Figure 8B). The 2024 harvest showed higher productivity under NT in almost all combinations, except for 5_FD. In the CT treatment, 10_FD had the lowest value; under NT, 10_FD and 5_FD were the least productive (Figure 8C).
Figure 8. Yields (t ha−1). (A) 2022 harvest, (B) 2023 harvest, and (C) 2024 harvest, in the trees of the combinations scion × rootstocks: Tahiti acid lime IAC 10 (10); IAC 5 (5) and BRS IAC EECB Ponta Firme (PF) grafted onto citrandarin IAC 3152 Itajobi (152) and trifoliata IAC 718 Flying Dragon (FD) under conventional (CT) and no-tillage (NT). *Averages followed by the same lowercase letters for each type of planting/management (tillage) for each scion × rootstock combination, uppercase for scion on the same rootstock with the same types of planting/management, do not differ by Tukey’s test (p < 0.05). Bars indicate the least significant difference.
The PCA results provided good discrimination for all samples, indicating the presence of two groups (Figure 9). PC1 separated samples according to the experimental group (yield, root length, CO2 assimilation, water potential, and proline accumulation), explaining 64.94% of the variation. PC2 captured 19.86% of the variation among samples from the second group (lipoperoxides accumulation). The first group showed a significant correlation with root length, yield, water potential, CO2 assimilation, and proline content, where 10_152 and PF_152 in CT were associated with a higher amino acid content in leaves. Combinations 10_152, 5_152, PF_152, and PF_FD through NT were more closely associated with root development, water content in leaf tissues, photosynthetic activity, and yield. The second group showed the most significant correlation with lipoperoxide content in 5_152, 10_FD, 5_FD, and PF_FD under CT and 10_FD and 5_FD in NT, which had the highest concentration of free radicals.
Figure 9. Principal components analysis (PCA), where R: Root length (mm); WP: Water Potential (MPa); A: CO2 assimilation (μmol.m−2 s−1); L: Lipoperoxides (mmol.g−1); Proline (μmol.g−1) and Yields (T ha−1), which means are from the combinations of Tahiti acid lime IAC 10 (10); IAC 5 (5) and BRS IAC EECB Ponta Firme (PF) with the citrandarin IAC 3152 Itajobi (152) and trifoliate orange IAC 718 Flying Dragon (FD) under conventional (C) and no-tillage (E).
Based on the presented result, no-tillage provides more efficient physiological and productive responses to the different combinations of Tahiti acid lime, than conventional tillage. The findings demonstrate that NT management, characterized by using mulch, promotes improved water retention, nutrient absorption, and root development, contributing to higher gas exchange and overall plant health. The higher nutrient contents in citrus leaves observed in NT compared to CT (Table 1) can be attributed to the mulch of Urochloa ruziziensis, present in the rows under the citrus plants in the NT treatment, improving nutrient cycling in this system (De Azevedo et al., 2020).
Urochloa spp., such as the ruzizienis species used in this research, are perennial grasses with vigorous and deep roots that enhance conservation agriculture by improving soil health and nutrient efficiency. Their biomass protects soil, stabilizes organic matter, and promotes nutrient cycling. Root exudates inhibit biological nitrification, reduce nitrogen losses, and mobilize recalcitrant phosphorus, increasing nutrient availability. Deep roots recover nutrients beyond other crops’ root zones, enhancing nutrient use efficiency and supporting sustainable tropical farming systems (Baptistella et al., 2020), justifying the increase in nutrient content in citrus leaves in the non-tillage treatment with mulch of U. ruziziensis.
A previous study carried out in a grapefruit (Citrus paradisi Macfd.) orchard to evaluate different mulches for soil quality showed that rice straw and legume cover crops led to an increase in Ca and K nutrients in the second year of the orchard’s evaluation, similar that observed in the present study (Van Dung et al., 2022). The benefits of this approach in management practices are due to better water retention in the soil, as the mulch provides physical protection from direct sunlight, reducing soil temperature and preventing desiccation through evaporation (de Almeida et al., 2018). This advantage improves the soil structure, benefits the microbiota, and contributes organic matter from pruning residues (Melo et al., 2023). In addition, as shown in Supplementary Table S2, the soil’s organic matter content was higher under the NT than under the CT, which may help explain the contribution to better nutrient absorption.
The semidwarf 152 rootstock consistently outperformed the dwarf FD rootstock, particularly under water-deficit conditions. Although dwarfing rootstocks’ ability to reduce the plant’s overall size and allow for orchard densification is highly advantageous, there is also a downside. This size reduction leads to a corresponding decrease in the plant’s root system, negatively impacting its ability to absorb nutrients and water stored in the deeper soil layers (Hayat et al., 2019). The less extensive root system compromises the exploration for and absorption of nutrients available in the soil and the smaller interaction area with beneficial microorganisms, such as mycorrhizal fungi, which facilitate micronutrient absorption (Hayat et al., 2022b). Since the FD is a dwarf rootstock, unlike the 152, which is a semi-dwarf, this may explain the lower nitrogen concentration in the leaf tissues of PF_FD compared to the other combinations with a better capacity to absorb nutrients (Donadio et al., 2019; Schinor et al., 2015). The Mg and K contents in soil (Supplementary Table S1) are under the recommended concentrations for Tahiti acid lime production; however, the low Mg and Fe contents in 10_FD may be explained by the fact that plants covered with mulch ensure higher K availability in the soil for absorption. However, this can decrease magnesium, as potassium is absorbed more quickly due to facilitated diffusion, which competes with and suppresses magnesium uptake. This effect has been observed in studies involving mulch combined with herbicides on Tahiti acid lime plants grafted onto FD rootstock (De Azevedo et al., 2020).
Acidic soils comprise nearly 30% of the world’s land area, mainly in tropical and subtropical regions, such as Brazil, where this study was conducted (von Uexküll and Mutert, 1995). The soil used in the experiment has the characteristics of a red latosol, which is characterized by a pronounced red coloration due to the higher levels and nature of the iron oxides present in the original material, which leads to the soil’s with higher acidity (Nolla et al., 2020), and may explain the adequate values of Fe in Tahiti acid lime leaves. Therefore, selecting rootstocks tolerant of soil acidity and any damage caused by their characteristics is essential for sound production. Moreover, the plant’s nutritional status is influenced by its nutrient absorption efficiency, which can be significantly affected by the characteristics of the rootstock (Valverdi et al., 2019). For instance, deficiencies in Mn and Zn may occur due to the selected rootstock, as seen in Troyer citrange, compared to Volkamerian and Swingle citrumelo (Yilmaz et al., 2018). Among the observed combinations, only three reached the minimum Mn concentration, two of which were formed with the 152 rootstock (5_152 and PF_152). Regarding the minimum Zn concentrations, only PF_152 met the required level. This supports the idea that citrandarin presents better micronutrient absorption than FD, resulting in better micronutrient absorption.
No-tillage is recognized for its numerous benefits, such as weed control, better water infiltration, and organic matter content; however, one of the significant aspects is the enhancement of soil microbiota (de Almeida et al., 2018). The mulching layer provides the necessary shading, an ideal temperature, and moisture to maintain balance within the soil profile’s microbial ecosystem. This proliferation of beneficial microorganisms improves the physicochemical quality of the soil structure and prevents compaction and erosion (Cerdà et al., 2018). Conversely, CT does not provide all of these benefits in terms of soil architecture and quality; thus, roots are less developed in scion and rootstock combinations compared to the same varieties subjected to soil management with mulch. In a well-structured environment (NT), the rootstock has optimal conditions for root development, resulting in higher percentages of root length over three years of evaluation than CT.
Additionally, dwarf rootstocks are widely believed to have a poorly developed root system since the characteristic low vigor influences not only the scion architecture but also the root architecture (Donadio et al., 2019). This may explain the lower root length in combination with FD since it is a dwarf rootstock, while 152 is a semi-dwarf rootstock. Roots play an essential role in absorbing water and nutrients. When there is low water availability, plant performance can be boosted according to the carbon reserves in the root system. This distribution varies among rootstocks, with those more tolerant to water stress typically accumulating higher concentrations of photoassimilates, enabling them to explore deeper soil layers for water (Silva et al., 2021). This corroborates the water potential data, where 152 showed more positive means than FD.
Water potential values before dawn should be below −1.3 MPa to avoid adverse effects on citrus production (García-Tejero et al., 2010). The combinations of acid lime IAC 10, IAC 5, and PF on FD rootstock under CT reached values above the required minimum, which could be detrimental to the long-term cultivation of this variety. Conventional tillage lacks mulch at the base of the citrus trees due to conventional mowers, leaving the plant rows more exposed to sunlight. This leads to faster evaporation and, consequently, more negative leaf water potential values (−2 MPa) (De Azevedo et al., 2020). The CT exposed the citrus plants to increased oxidative stress, reduced root growth, and decreased physiological performance due to the absence of mulch, which led to greater water loss and soil degradation.
On Valencia orange grafted onto Rangpur lime and Swingle citrumelo under water restriction conditions, Pedroso et al. (2014) demonstrated a reduction in water potential and partial stomatal closure in mature and young leaves, regardless of the rootstock. However, Rangpur lime showed more efficient results than Swingle due to its greater drought tolerance. A reduction in leaf water potential, even before dawn, is expected under rainfed conditions during the dry season (winter); however, species with greater drought tolerance, such as the Rangpur lime rootstock, have a better capacity to retain water within their tissues, allowing for normal development (Miranda et al., 2021). Therefore, although a decrease in water potential is expected under low water availability, 152 rootstock can maintain more positive values in such situations. Additionally, keeping the plants under NT ensures better soil moisture retention, enables a higher stomatal conductance and increases water potential for the cultivated plants.
Under water deficit stress conditions, the plant’s initial response reduces its conductance by closing the stomata to minimize water loss through transpiration (Borim de Souza et al., 2023). This response was mainly observed under CT, which recorded the lowest stomatal conductance values compared to plants subjected to NT. The benefit of vegetation cover is maintaining a cooler soil temperature, which helps reduce evapotranspiration, thereby ensuring greater plant water availability and better stomatal opening, as observed under NT. Therefore, even under dry conditions, mulching creates more favorable conditions and tends to be less detrimental to the plant’s initial physiological responses than CT (Cárceles Rodríguez et al., 2022).
During the summer of 2024, intercellular CO2 concentration (Ci) did not show differences between treatments. This lack of variation can be seen as a positive sign, as it indicates no obstruction of the meristems responsible for capturing CO2 for photosynthetic activity, suggesting the entire functioning of the photosystems in all combinations under both cultural tillage practices (Borim de Souza et al., 2023). However, as early as the winter of 2024, an increase in Ci was observed, and it was associated with a rise in stomatal conductance values, which were mainly observed under NT, as there was a tendency to increase the CO2 concentration in the substomatal cavity simultaneously. This plant mechanism can enhance photosynthetic activity (Cechin et al., 2008). With greater stomatal conductance and higher CO2 concentrations in the substomatal cavity of all Tahiti lime varieties’ leaves on both rootstocks managed under NT, there was a noticeable impact on the photosynthetic activity of most combinations, resulting in higher CO2 assimilation compared to CT. The stomatal opening and closing process is driven by the water content available in the soil and plant leaves (Borim de Souza et al., 2023).
A previous study on drought induction in citrus showed decreased stomatal conductance as the soil water content decreased (Zhou et al., 2021). Consequently, stomatal closure, which prevents water loss through transpiration, also reduces the CO2 intake, limiting the essential gas for photosynthetic activity (Giordano et al., 2021). Therefore, choosing the best types of planting/management for a citrus orchard can demonstrate benefits within a few years, as evidenced by in vivo gas exchange analysis. Another factor to consider is the comparison of scions on both rootstocks. CO2 assimilation analyses showed that all varieties performed better when grafted onto 152, especially under CT. This suggests that selecting rootstocks with higher tolerance to unfavorable conditions, such as 152, is advantageous, given the current environmental challenges imposed by CT.
Environmental stressors, such as drought and extreme temperatures, lead to reactive oxygen species (ROS) accumulation in plant cells due to disruptions in the electron transport system and metabolic processes occurring in chloroplasts and mitochondria (Gupta et al., 2020). These ROS are detrimental to various cellular structures of the photosynthetic apparatus, inhibiting metabolic and enzymatic activities, oxidizing nucleic acids, and leading to cell death (Anderegg et al., 2019). Lipid peroxides are part of this group of ROS, and their increased concentrations indicate higher oxidative damage, reflecting the severity of stress encountered by plants (Farooq et al., 2009; Kaur and Asthir, 2017). Previous research on sour oranges revealed higher H2O2 levels in leaves and roots, showcasing the species’ greater susceptibility to stress and the lower efficiency of the antioxidant mechanisms in some citrus varieties (Zandalinas et al., 2017). No-tillage leads to lower concentrations of these free radicals within trees, likely due to improved soil moisture through mulching and vegetative cover.
Under dehydration, a defense mechanism of stress-tolerant plants includes the reduction of stomatal conductance or accumulation of osmoprotective solutes, such as proline, with antioxidant potential to contain these ROS, due to an unpaired electron, which associates with reactive oxygen species (e.g., lipid peroxides), it effectively neutralizes them, preventing oxidative damage (Vives-Peris et al., 2017). As a result of oxidative stress, the consequent increase in proline content acts as an adaptive response, as it acts as a compatible osmolyte to buffer cytosolic pH, as well as balancing the cell’s redox state and being a potential eliminator of ROS (Gholami Zali and Ehsanzadeh, 2018). Previous work with sweet oranges has shown an accumulation of leaf proline in scions grafted onto different rootstocks: Orlando tangelo (Citrus paradisi Macfad. × Citrus tangerine hort. Ex Tanaka), Indio and Riverside citrandarins [Citrus sunki (Hayata) hort. ex Tanaka × Poncirus trifoliata (L.) Raf.], and Sunki of Florida (C. sunki) mandarin (TSKFL) × C13 citrange (C. sinensis × P. trifoliata) (CTC13) - 012, when submitted to natural drought stress (de Carvalho et al., 2021).
When subjected to NT, all scion × rootstock combinations had higher yields. However, even under CT, the 152 combinations stand out, especially IAC 10 and BRS IAC EECB Ponta Firme. Previous work has demonstrated the better productivity of specific rootstocks compared to others; for example, the quantity of fruit produced by Mandared (C. clementina × C. sinensis) on Troyer citrange (C. sinenesis × Poncirus trifoliata) was significantly lower than the same scion variety on C57 citrange (C. sinensis × P. trifoliata) (Caruso et al., 2020). Late mandarin grafted onto Carrizo citrange [C. sinensis (L.) Osb. × P. trifoliata (L). Raf.] produced more than 77 kg of fruit per tree compared to Cleopatra mandarin (C. reshni Hort. ex Tan.) (Maciá-Vázquez et al., 2024). However, in the case of NT, IAC 10 and PF grafted on 152 and PF grafted on FD demonstrated the high productive capacity of the combination under favorable conditions promoted by soil management. On the other hand, 152 rootstock shows a better capacity to tolerate adverse conditions promoted by CT.
The improved moisture retention in soil profiles promoted by mulching in NT stimulated a higher juice content in almost all combinations. This is also reflected in the ratio, where fruit from trees under NT reached maturation faster than that under CT, demonstrating a better ability to maintain the chemical quality of the juice. The increase in ascorbic acid in fruit maintained under CT can be attributed to the compound’s inherent characteristics, as ascorbic acid is widely recognized as an essential antioxidant that helps eliminate and balance ROS production, thereby preventing damage caused by oxidative stress (Zheng et al., 2022). Consequently, its role has been observed in plant responses to various stressors, such as drought, salinity, extreme temperatures, heavy metals, and ozone (Celi et al., 2023). Therefore, the treatments found solutions to mitigate these effects in unprotected soil with greater susceptibility to environmental stress. This means that genotypes tolerant to climatic adversities tend to have higher endogenous ascorbic acid levels to avoid the oxidative stress promoted by adverse environmental conditions (Zheng et al., 2022).
Biochemically, plants under NT exhibited lower concentrations of lipoperoxides, indicating reduced oxidative damage, while CT stimulated higher levels of proline, a biochemical marker of stress response. Plants with the highest accumulation of lipoperoxides in their leaf tissues exhibited an inverse correlation with yield. The photooxidation caused by increased reactive oxygen species (ROS) triggers uncontrolled oxidation of photosynthetic components, thereby reducing the photosynthetic rate and limiting the availability of photoassimilates for the plant (Lourkisti et al., 2022). This makes full development, growth, and reproduction impossible (Osmolovskaya et al., 2018). We noted the proximity of proline to lipoperoxides and its association with the 5_152 and PF_FD combinations under CT. The plant accumulates this amino acid to combat ROS (Vives-Peris et al., 2017). An example of this was seen in a study of sour oranges subjected to different levels of drought and their ability to tolerate water deficits due to the lower H2O2 accumulation and higher proline accumulation (Shafqat et al., 2021b).
The PCA showed that combinations 10_152 and PF_152 were positively correlated with CO2 assimilation, water potential, root development, and yield. This indicates that even under less favorable conditions, the scions associated with the Itajobi rootstock are more likely to tolerate stress and maintain an active metabolism. Moreover, developing strategies to enhance drought tolerance is crucial in selecting rootstock that maintains productivity. Rootstocks with higher root growth rates, improved water potential, increased antioxidant accumulation, and better CO2 assimilation have a greater capacity to withstand drought conditions while maintaining high growth levels and biomass accumulation (Shafqat et al., 2021a). Therefore, selecting rootstocks that effectively mitigate stress and enhance water absorption by increasing root density or deepening the root system will ensure a more efficient orchard. Additionally, the orchard will become more profitable and productive, as proper management practices promote optimal conditions.
In the second half of 2024, we observed wood pocket symptoms with greater severity in IAC 10 plants, few symptoms in PF, and no symptoms in IAC 5. Wood pocket is a physiological disorder or physiopathy caused by genetic and abiotic factors, such as high temperatures causing sectoral death in Tahiti, also called Persian or bear trees, leading to total extermination (ICA, 2011). This information is essential for citrus breeding programs and may contribute to a better choice of varieties on the market.
Significant correlations were observed between soil conservation practices and improved fruit quality and productivity. In addition, the induction of physiological responses can better predict plant behavior in the face of adverse environmental conditions (Borim de Souza et al., 2023), thus facilitating the selection of the best candidates to be planted in the Tahiti acid lime orchard and which management practices should be adopted. By integrating NT practices with appropriate rootstock and scion combinations, such as IAC 3152 Itajobi, it is possible to enhance drought tolerance, optimize resource use, and maintain productivity under challenging environmental conditions. This study highlights the potential of combining sustainable management practices with advanced genetic selections to address the demands of modern agriculture and climate resilience.
This study demonstrated that no-tillage (NT) management with mulch significantly enhances the productivity, physiological responses and fruit quality of ‘Tahiti’ acid lime grafted onto dwarf rootstocks. The results show that citrandarin IAC 3152 Itajobi rootstock, under the best productivity, promotes better root development, improved water potential, and higher photosynthetic efficiency. Specifically, plants grafted onto this rootstock under NT exhibited greater root length, higher fruit yield, and improved physiological responses, such as water retention and CO2 assimilation, than those under CT. Although the productivity in the CT treatment was not as high as NT, it is still possible to highlight that even under this form of management without mulching, citrandarin Itajobi presented tolerance and a higher yield for a dwarf rootstock. These findings underscore the importance of selecting rootstocks and management practices that enhance plants’ resilience to water stress and overall production efficiency in citriculture. These findings provide valuable insights into optimizing citrus production by combining dwarf rootstocks and sustainable practices, addressing environmental challenges, and meeting the demand for sustainable citriculture.
The original contributions presented in the study are included in the article/Supplementary material, further inquiries can be directed to the corresponding author.
AS: Conceptualization, Data curation, Formal analysis, Investigation, Methodology, Project administration, Software, Visualization, Writing – original draft, Writing – review & editing, Funding acquisition, Supervision. MC-Y: Funding acquisition, Resources, Supervision, Validation, Writing – review & editing. PC: Validation, Visualization, Writing – review & editing. FD: Methodology, Visualization, Writing – review & editing. MB: Validation, Visualization, Writing – review & editing. PR: Methodology, Visualization, Writing – review & editing. PP: Methodology, Visualization, Writing – review & editing. FA: Funding acquisition, Resources, Supervision, Validation, Visualization, Writing – review & editing.
The author(s) declare that financial support was received for the research, authorship, and/or publication of this article. This study was financed in part by Fundação de Amparo à Pesquisa do Estado de São Paulo FAPESP (2021/10.123-9 and 2020/07045-3) and the Coordenação de Aperfeiçoamento de Pessoal de Nível Superior - Brasil (CAPES) - Finance Code 001.
AS thanks FAPESP 2021/10.123-9. MC-Y thanks FAPESP 2020/07045-3.
The authors declare that the research was conducted without commercial or financial relationships that could create a conflict of interest.
All claims expressed in this article are solely those of the authors and do not necessarily represent those of their affiliated organizations, or those of the publisher, the editors and the reviewers. Any product that may be evaluated in this article, or claim that may be made by its manufacturer, is not guaranteed or endorsed by the publisher.
The Supplementary material for this article can be found online at: https://www.frontiersin.org/articles/10.3389/fsufs.2025.1489291/full#supplementary-material
Abobatta, W. F. (2023). Citrus production in climate change era. Cultivat. Clim. Change Resilience 2, 68–93. doi: 10.1201/9781003351153-5
Anderegg, W. R. L., Anderegg, L. D. L., Kerr, K. L., and Trugman, A. T. (2019). Widespread drought-induced tree mortality at dry range edges indicates that climate stress exceeds species’ compensating mechanisms. Glob. Chang. Biol. 25, 3793–3802. doi: 10.1111/gcb.14771
Baptistella, J. L. C., de Andrade, S. A. L., Favarin, J. L., and Mazzafera, P. (2020). Urochloa in tropical agroecosystems. Front. Sustain. Food Syst. 4:543983. doi: 10.3389/FSUFS.2020.00119/BIBTEX
Bataglia, O. C., Furlani, A. M. C., Teixeira, J. P. F., Furlani, P. R., and Gallo, J. R. (1983). Metodos de analise quimica de plantas. Boletim Tecnico 78:78.
Borim de Souza, A. J., Ocampos, F. M. M., Catoia Pulgrossi, R., Dokkedal, A. L., Colnago, L. A., Cechin, I., et al. (2023). NMR-based metabolomics reveals effects of water stress in the primary and specialized metabolisms of Bauhinia ungulata L. (Fabaceae). Metabolites 13:381. doi: 10.3390/metabo13030381
Bowman, K. D., and Joubert, J. (2020). Citrus rootstocks. Genus Citrus, 105–127. doi: 10.1016/b978-0-12-812163-4.00006-1
Cárceles Rodríguez, B., Durán-Zuazo, V. H., Soriano Rodríguez, M., García-Tejero, I. F., Gálvez Ruiz, B., and Cuadros Tavira, S. (2022). Conservation agriculture as a sustainable system for soil health: a review. Soil Syst. 6:87. doi: 10.3390/SOILSYSTEMS6040087
Caruso, M., Continella, A., Modica, G., Pannitteri, C., Russo, R., Salonia, F., et al. (2020). Rootstocks influence yield precocity, productivity, and pre-harvest fruit drop of Mandared pigmented mandarin. Agronomy 10:1305. doi: 10.3390/AGRONOMY10091305
Cechin, I., Corniani, N., de Fátima Fumis, T., and Cataneo, A. C. (2008). Ultraviolet-B and water stress effects on growth, gas exchange and oxidative stress in sunflower plants. Radiat. Environ. Biophys. 47, 405–413. doi: 10.1007/s00411-008-0167-y
Celi, G. E. A., Gratão, P. L., Lanza, M. G. D. B., and Dos Reis, A. R. (2023). Physiological and biochemical roles of ascorbic acid on mitigation of abiotic stresses in plants. Plant Physiol. Biochem. 202:107970. doi: 10.1016/J.PLAPHY.2023.107970
Cerdà, A., Rodrigo-Comino, J., Giménez-Morera, A., and Keesstra, S. D. (2018). Hydrological and erosional impact and farmer’s perception on catch crops and weeds in citrus organic farming in Canyoles river watershed, Eastern Spain. Agric. Ecosyst. Environ. 258, 49–58. doi: 10.1016/j.agee.2018.02.015
Charrad, M., Ghazzali, N., Boiteau, V., and Niknafs, A. (2014). NbClust: an R package for determining the relevant number of clusters in a data set. J. Stat. Softw. 61, 1–36. doi: 10.18637/JSS.V061.I06
de Almeida, W. S., Panachuki, E., de Oliveira, P. T. S., da Silva Menezes, R., Sobrinho, T. A., and de Carvalho, D. F. (2018). Effect of soil tillage and vegetal cover on soil water infiltration. Soil Tillage Res. 175, 130–138. doi: 10.1016/j.still.2017.07.009
De Azevedo, F. A., De Almeida, R. F., Martinelli, R., Próspero, A. G., Licerre, R., Da Conceição, P. M., et al. (2020). No-tillage and high-density planting for Tahiti acid lime grafted onto flying dragon trifoliate Orange. Front. Sustain. Food Syst. 4:108. doi: 10.3389/fsufs.2020.00108
de Carvalho, L. M., de Araújo, S. B., de Carvalho, H. W. L., Girardi, E. A., and Filho, W. D. S. S. (2021). Leaf proline accumulation and fruit yield of ‘Pera’ sweet orange trees under natural water stress. Bragantia 80:e1121. doi: 10.1590/1678-4499.20200349
De Sousa, A. R. O., Silva, E. M. D. A., Filho, M. A. C., Costa, M. G. C., Filho, W. D. S. S., Micheli, F., et al. (2022). Metabolic responses to drought stress and rehydration in leaves and roots of three Citrus scion/rootstock combinations. Sci. Hortic. 292:110490. doi: 10.1016/J.SCIENTA.2021.110490
Donadio, L. C., Lederman, I. E., Roberto, S. R., and Stucchi, E. S. (2019). Dwarfing-canopy and rootstock cultivars for fruit trees. Rev. Bras. Frutic. 41:9997. doi: 10.1590/0100-29452019997
Farooq, M., Wahid, A., Kobayashi, N., Fujita, D., and Basra, S. M. A. (2009). “Plant drought stress: effects, mechanisms and management” in Sustainable Agriculture (Dordrecht: Springer Netherlands), 153–188.
García-Sánchez, F., Syvertsen, J. P., Gimeno, V., Botía, P., and Perez-Perez, J. G. (2007). Responses to flooding and drought stress by two citrus rootstock seedlings with different water-use efficiency. Physiol. Plant. 130, 532–542. doi: 10.1111/j.1399-3054.2007.00925.x
García-Tejero, I., Romero-Vicente, R., Jiménez-Bocanegra, J. A., Martínez-García, G., Durán-Zuazo, V. H., and Muriel-Fernández, J. L. (2010). Response of citrus trees to deficit irrigation during different phenological periods in relation to yield, fruit quality, and water productivity. Agric. Water Manag. 97, 689–699. doi: 10.1016/J.AGWAT.2009.12.012
Gholami Zali, A., and Ehsanzadeh, P. (2018). Exogenous proline improves osmoregulation, physiological functions, essential oil, and seed yield of fennel. Ind. Crop. Prod. 111, 133–140. doi: 10.1016/j.indcrop.2017.10.020
Giordano, M., Petropoulos, S. A., Cirillo, C., and Rouphael, Y. (2021). Biochemical, physiological, and molecular aspects of ornamental plants adaptation to deficit irrigation. Horticulturae 7:107. doi: 10.3390/HORTICULTURAE7050107
Gupta, A., Rico-Medina, A., and Caño-Delgado, A. I. (2020). The physiology of plant responses to drought. Science 368, 266–269. doi: 10.1126/SCIENCE.AAZ7614
Hair, J. F., Black, W. C., Babin, B. J., and Anderson, R. E. (2010). Logistic regression: regression with a binary dependent variable. Multivariate Data Analysis, 313–340.
Hayat, F., Li, J., Iqbal, S., Peng, Y., Hong, L., Balal, R. M., et al. (2022). A Mini review of Citrus rootstocks and their role in high-density orchards. Plan. Theory 11:2876. doi: 10.3390/PLANTS11212876
Hayat, F., Qiu, C., Xu, X., Wang, Y., Wu, T., Zhang, X., et al. (2019). Rootstocks influence morphological and biochemical changes in young “red Fuji” apple plants. Intl. J. Agric. Biol 21, 1097–1105. doi: 10.17957/IJAB/15.0999
Kaiser, H. F. (1958). The varimax criterion for analytic rotation in factor analysis. Psychometrika 23, 187–200. doi: 10.1007/BF02289233
Kaur, G., and Asthir, B. (2017). Molecular responses to drought stress in plants. Biol. Plant. 61, 201–209. doi: 10.1007/s10535-016-0700-9
Lourkisti, R., Froelicher, Y., Morillon, R., Berti, L., and Santini, J. (2022). Enhanced photosynthetic capacity, osmotic adjustment and antioxidant defenses contribute to improve tolerance to moderate water deficit and recovery of triploid Citrus genotypes. Antioxidants 11:562. doi: 10.3390/ANTIOX11030562
Maciá-Vázquez, A. A., Martínez-Nicolás, J. J., Núñez-Gómez, D., Melgarejo, P., and Legua, P. (2024). Influence of rootstock on yield, morphological, biochemical and sensory characteristics of “Afourer” variety mandarins. Sci. Hortic. 325:112644. doi: 10.1016/J.SCIENTA.2023.112644
Martinelli, R., Rufino, L. R., de Melo, A. C., Alcántara-de la Cruz, R., da Silva, M. F., da Silva, J. R., et al. (2022). Glyphosate excessive use chronically disrupts the shikimate pathway and can affect photosynthesis and yield in citrus trees. Chemosphere 308:136468. doi: 10.1016/J.CHEMOSPHERE.2022.136468
Mattiuzi, C. D. P., Marques, G. F., and Medellín-Azuara, J. (2019). Reassessing water allocation strategies and conjunctive use to reduce water scarcity and scarcity costs for irrigated agriculture in southern Brazil. Water 11:1140. doi: 10.3390/w11061140
Melo, L. B. B., Benevenute, P. A. N., Barbosa, S. M., Chiarini, T. P. A., Oliveira, G. C., Lima, J. M., et al. (2023). Spatial and temporal electrical resistivity dynamics in a dense Ultisol under deep tillage and different citrus root-stocks. Soil Tillage Res. 228:105629. doi: 10.1016/J.STILL.2022.105629
Miranda, M. T., Da Silva, S. F., Silveira, N. M., Pereira, L., Machado, E. C., and Ribeiro, R. V. (2021). Root osmotic adjustment and stomatal control of Leaf gas exchange are dependent on Citrus rootstocks under water deficit. J. Plant Growth Regul. 40, 11–19. doi: 10.1007/s00344-020-10069-5
Niu, Y. H., Wang, L., Wan, X. G., Peng, Q. Z., Huang, Q., and Shi, Z. H. (2021). A systematic review of soil erosion in citrus orchards worldwide. Catena 206:105558. doi: 10.1016/J.CATENA.2021.105558
Nolla, A., Oliveira, E., Alves, S., Garcez Da Silva, T., and Bordin, A. V. (2020). Correction of soil acidity and availability of phosphorus and potassium in an oxisol submited to surfaced and incorporated liming. Brazilian J. Anim. Environ. Res. 3, 2478–2487. doi: 10.34188/BJAERV3N3-156
Osmolovskaya, N., Shumilina, J., Kim, A., Didio, A., Grishina, T., Bilova, T., et al. (2018). Methodology of drought stress research: experimental setup and physiological characterization. Int. J. Mol. Sci. 19:4089. doi: 10.3390/IJMS19124089
Pedroso, F. K. J. V., Prudente, D. A., Bueno, A. C. R., Machado, E. C., and Ribeiro, R. V. (2014). Drought tolerance in citrus trees is enhanced by rootstock-dependent changes in root growth and carbohydrate availability. Environ. Exp. Bot. 101, 26–35. doi: 10.1016/J.ENVEXPBOT.2013.12.024
Pereira Costa, D., Sanches Stuchi, E., Girardi, E. A., Moreira, A. S., da Silva Gesteira, A., Coelho Filho, M. A., et al. (2021). Less is more: a hard way to get potential dwarfing hybrid rootstocks for Valencia sweet Orange. Agriculture 11:354. doi: 10.3390/agriculture11040354
Reuther, W., Batchelor, L., and Webber, H. (1968). The Citrus industry. Vol. II. Anatomy, physiology, genetics, and reproduction. Available online at: https://www.cabidigitallibrary.org/doi/full/10.5555/19690307355 (Accessed September 10, 2024).
Schinor, E. H., Nascimento, A. L., De Barros, V. L. N. P., Bastianel, M., De Azevedo, F. A., and Cristofani-Yaly, M. (2015). Fruit traits and vegetative growth of citrandarins rootstocks in the nursery. Citrus Res. Technol. 36, 27–35. doi: 10.5935/2236-3122.20150004
Shafqat, W., Jaskani, M. J., Maqbool, R., Chattha, W. S., Ali, Z., Naqvi, S. A., et al. (2021a). Heat shock protein and aquaporin expression enhance water conserving behavior of citrus under water deficits and high temperature conditions. Environ. Exp. Bot. 181:104270. doi: 10.1016/J.ENVEXPBOT.2020.104270
Shafqat, W., Mazrou, Y. S. A., Sami-ur-Rehman, Y., Nehela, Y., Ikram, S., Bibi, S., et al. (2021b). Effect of three water regimes on the physiological and anatomical structure of stem and leaves of different Citrus rootstocks with distinct degrees of tolerance to drought stress. Horticulturae 7:554. doi: 10.3390/HORTICULTURAE7120554
Silva, S. F., Miranda, M. T., Costa, V. E., Machado, E. C., and Ribeiro, R. V. (2021). Sink strength of citrus rootstocks under water deficit. Tree Physiol. 41, 1372–1383. doi: 10.1093/TREEPHYS/TPAB008
Valverdi, N. A., Cheng, L., and Kalcsits, L. (2019). Apple Scion and rootstock contribute to nutrient uptake and partitioning under different belowground environments. Agronomy 9:415. doi: 10.3390/AGRONOMY9080415
Van Dung, T., Ngoc, N. P., Van Dang, L., and Hung, N. N. (2022). Impact of cover crop and mulching on soil physical properties and soil nutrients in a citrus orchard. PeerJ 10:e14170. doi: 10.7717/peerj.14170
Visconti, F., Peiró, E., Pesce, S., Balugani, E., Baixauli, C., and de Paz, J. M. (2024). Straw mulching increases soil health in the inter-row of citrus orchards from Mediterranean flat lands. Eur. J. Agron. 155:127115. doi: 10.1016/J.EJA.2024.127115
Vives-Peris, V., Gómez-Cadenas, A., and Pérez-Clemente, R. M. (2017). Citrus plants exude proline and phytohormones under abiotic stress conditions. Plant Cell Rep. 36, 1971–1984. doi: 10.1007/s00299-017-2214-0
von Uexküll, H. R., and Mutert, E. (1995). Global extent, development and economic impact of acid soils. Plant Soil 171, 1–15. doi: 10.1007/BF00009558
Yilmaz, B., Cimen, B., Incesu, M., Uysal Kamiloglu, M., and Yesiloglu, T. (2018). Rootstock influences on seasonal changes in leaf physiology and fruit quality of rio red grapefruit variety. Appl. Ecol. Environ. Res. 16, 4065–4080. doi: 10.15666/AEER/1604_40654080
Zandalinas, S. I., Sales, C., Beltrán, J., Gómez-Cadenas, A., and Arbona, V. (2017). Activation of secondary metabolism in Citrus plants is associated to sensitivity to combined drought and high temperatures. Front. Plant Sci. 7:1954. doi: 10.3389/fpls.2016.01954
Zheng, X., Gong, M., Zhang, Q., Tan, H., Li, L., Tang, Y., et al. (2022). Metabolism and regulation of ascorbic acid in fruits. Plan. Theory 11:1602. doi: 10.3390/PLANTS11121602
Keywords: Citrus spp., citrus physiology, drought tolerance, cultural management, tillage
Citation: de Souza AJB, Cristofani-Yaly M, da Conceição PM, Devite FT, Bastianel M, Romero PVS, Padilha PHB and de Azevedo FA (2025) Physiological and productivity responses of Tahiti acid lime grafted onto dwarfing rootstocks under different planting and mulching practices. Front. Sustain. Food Syst. 9:1489291. doi: 10.3389/fsufs.2025.1489291
Received: 31 August 2024; Accepted: 21 January 2025;
Published: 05 February 2025.
Edited by:
Jennifer Blesh, University of Michigan, United StatesReviewed by:
Juan Fernando Hirzel, Agricultural Research Institute (Chile), ChileCopyright © 2025 de Souza, Cristofani-Yaly, da Conceição, Devite, Bastianel, Romero, Padilha and de Azevedo. This is an open-access article distributed under the terms of the Creative Commons Attribution License (CC BY). The use, distribution or reproduction in other forums is permitted, provided the original author(s) and the copyright owner(s) are credited and that the original publication in this journal is cited, in accordance with accepted academic practice. No use, distribution or reproduction is permitted which does not comply with these terms.
*Correspondence: Ana Júlia Borim de Souza, YW5hanVsaWFib3JpbUBnbWFpbC5jb20=
Disclaimer: All claims expressed in this article are solely those of the authors and do not necessarily represent those of their affiliated organizations, or those of the publisher, the editors and the reviewers. Any product that may be evaluated in this article or claim that may be made by its manufacturer is not guaranteed or endorsed by the publisher.
Research integrity at Frontiers
Learn more about the work of our research integrity team to safeguard the quality of each article we publish.