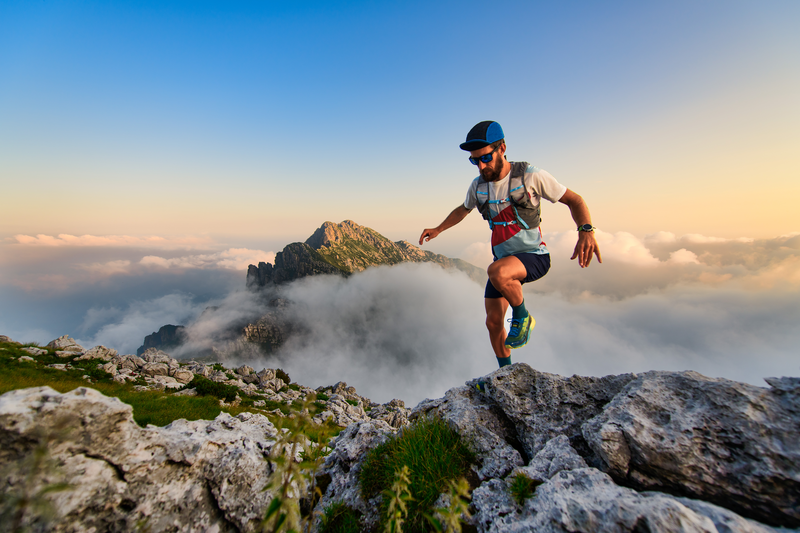
95% of researchers rate our articles as excellent or good
Learn more about the work of our research integrity team to safeguard the quality of each article we publish.
Find out more
ORIGINAL RESEARCH article
Front. Sustain. Food Syst. , 14 March 2025
Sec. Agroecology and Ecosystem Services
Volume 9 - 2025 | https://doi.org/10.3389/fsufs.2025.1470188
This article is part of the Research Topic Conservation Agriculture for Sustainable Food Production Systems View all 20 articles
Contemporary crop management practices are labor, water, and energy inefficient. Identifying a sustainable, productive, and resource-efficient alternate crop production system to the present crop management system is crucial. A field experiment was conducted to assess the effects of conservation agriculture (CA) on crop growth, productivity, and resource use efficiency under the maize-wheat-greengram system during 9th (2018–19) and 10th (2019–20) years of the study. CA-based bed planting methods such as permanent narrow, broad and flat beds with and without retention of crops residues and 75% and 100% of the recommended dose of nitrogen (N) were compared with conventional tillage (CT) treatment. The permanent broad bed with residue with 100% recommended dose of N (PBB + R + 100N) resulted in 56.0%, 60.0% and 26.5% higher root length density in maize, wheat, and greengram crops, respectively. The PBB + R + 100N registered 31.1% higher system productivity than CT. The partial factor productivity (PFP) of N, P, and K were higher under PBB + R + 75N. The CA-based broad-bed practices registered 10.2 and 18.1% savings of irrigation water application than narrow-bed and flat-bed with residue retention, respectively. The CA-based practices without residue retention obtained significantly higher energy productivity, net energy return, and energy ratio than CT. Adopting the CA practice involving a permanent broad bed with residue using 100% N (PBB + R + 100N) in a maize-wheat-greengram system would be more productive and efficient for nutrients, water, and energy. This study focuses on the potential of CA to improve nutrient, water, and energy security in the maize-wheat-greengram system in South Asia.
The changes in world scenarios such as extreme weather events, urbanization, and a burgeoning population, escalating the demand for essential resources (viz., land, water, energy, and nutrients) have resulted in more conflicts within the global food–energy–water nexus (Tian et al., 2021). Rice-wheat cropping system occupying ~10.5 million hectares areas in a populous country like India to supports its food and nutritional needs (Kakraliya et al., 2022). The conventional rice-wheat cropping system is labor-, water-, and energy-intensive; water and labor scarcity will make the system less profitable for farmers (Chauhan et al., 2012; Kumar et al., 2013). The maize-wheat and maize-wheat-greengram cropping system is being promoted as an alternative to existing rice-based cropping systems of the northwestern Indo-Gangetic Plains (IGP) in order to overcome challenges such as energy and nutritional scarcity, residue burning, reduction in biomass productivity, and water table decline (Parihar et al., 2017). The maize-wheat cropping system is currently practiced on ~1.66 million hectares (m ha) (Singh et al., 2023) and contributes significantly to national food production. From 2001 to 2018, the area under maize cultivation in India increased by 36%, while productivity rose by 96% (Dutta et al., 2023). Maize is a more water- and labor-efficient crop than both rice and wheat, and maize-based cropping systems serve as a viable alternative to rice-based cropping systems across much of northern India (Aulakh and Grant, 2008). The production potential of maize-based cropping systems is higher than that of the conventional rice-wheat system. To address issues of the conventional rice-wheat system, CA-based sustainable cropland intensification encompassing no/minimum tillage, crop residue retention, and efficient crop rotation is extremely important. CA-based management techniques have shown promise in halting land degradation, increasing the efficiency of resources, enhancing diversity, soil health, and agricultural profitability, and adapting and mitigating the effects of climate change to varying degrees in a range of crop production systems (Sharma et al., 2012; Ghosh et al., 2019). Numerous studies have shown that a CA-based sustainable intensification of the maize-wheat system can increase crop productivity, profitability, use efficiency of water and energy, and the accumulation of organic carbon with more sequestration potential (Das et al., 2013; Nath et al., 2017; Das et al., 2018; Jat et al., 2018; Parihar et al., 2018; Ghosh et al., 2021, 2022a). There is an opportunity to boost cropping intensity in a double cereal-based maize-wheat rotation under CA by including a legume crop such as greengram, which will not only increase system productivity and economic returns but also improve soil health (Gathala et al., 2013; Ghosh et al., 2022b). The carbon negative high food-producing technologies must be adopted to address the multiple challenges caused by conventional agricultural practices (Babu et al., 2023). Optimum N fertilization in CA can reduce N2O emissions, minimize N leaching losses, boost crop productivity, and enhance nutrient use efficiency (Yadav et al., 2017). Retaining crop residues and incorporating legumes into crop diversification decreases N inputs and can enhance food security, irrespective of their impact on mitigating climate change (Powlson et al., 2016). In the present context, N management in cereal-based cropping systems is crucial due to its higher greenhouse gas footprint (Cheng et al., 2015). According to Schoenau and Campbell (1996), there is a higher demand for N during the initial years of certain conservation tillage systems. The requirement for N fertilizer in CA will likely decline over time due to the accumulation of organic matter (Riley et al., 1994). The higher immobilization of nutrients in reduced and zero tillage (ZT) systems can improve long-term soil and fertilizer N conservation (Xiao-Bin et al., 2006). Application of ZT in permanent beds with crop residue input would be a superior management choice for soil N enhancement, as the management technique can promote soil aggregation with larger buildup of total soil N inside macro-aggregates (Bhattacharyya et al., 2013). CA practices affect nutrient transformation and distribution in soil by increasing their concentration and availability near the soil surface, indicating the positive effects of CA on resource use efficiency, environmental quality, and system sustainability (Das et al., 2020, 2021). CA practices increase fertilizer N use efficiency and have the potential to reduce greenhouse gases (GHGs) emissions (Jat et al., 2012). Improvement in aggregate stability, combined with residue retention in CA systems, has also been shown to significantly influence soil water storage. These improvements in soil water storage are typically driven by increased infiltration rates and reduced soil water evaporation (Li et al., 2019; Page et al., 2020). Zero-tillage is said to conserve 20%−35% of the irrigation water in the wheat crop, lowering water use by roughly 10 cm ha−1 or by 1 million l ha−1 in comparison with CT (Gupta and Seth, 2007; Erenstein and Laxmi, 2008). When comparing ZT and permanent beds to CT, the water productivity of maize increased by 13%−28% and 7%−30%, respectively, while the amount of water needed for irrigation dropped by 40–65 and 60–98 ha-mm, respectively (Parihar et al., 2016). Similarly, compared to a CT system, implementing a permanent broad bed with residue in a CA-based maize-wheat cropping system may yield 57% and 19% higher water productivity in maize and wheat, respectively (Das et al., 2018). An efficient cultivation technique that uses little energy and has a low potential to cause global warming is urgently needed (Chaudhary et al., 2017; Ghosh et al., 2022c). The growing reliance of agriculture on energy, particularly in activities like tillage and pumping, is a cause for concern (Parihar et al., 2022). An energy analysis in cultivation practice is necessary to find a resource-saving, energy-efficient technology with minimal environmental impact. Several researchers have reported the effects of different tillage, residue management, and cropping sequences on the efficiency of energy use (Saad et al., 2016; Parihar et al., 2017, 2018; Jat et al., 2020; Raj et al., 2022). An average of 36 l ha−1, or 81% savings across IGP, has been reported as the seasonal savings in diesel for land preparation with ZT (Erenstein and Laxmi, 2008). Parihar et al. (2017) discovered that CA-based management combined with sustainable intensification of maize production systems (maize-wheat-greengram and maize-mustard-greengram) resulted in significant improvements in system productivity and energy use efficiency compared to CT (Saad et al., 2016) observed that ZT with a raised bed with crop residue retention in a maize-wheat-greengram cropping system used 8% lower energy than CT with a flatbed. Adoption of CA principles with recommended efficient farming techniques could result in sustainable intensification of the maize-wheat-greengram cropping system in northwestern India (Parihar et al., 2018; Raj et al., 2023; Ghosh et al., 2023).
The present study aimed to meet the rising food and nutritional demands of the Indian population while ensuring water and energy security. The cultivation of maize and wheat in the experiment was focused to address food needs, while the inclusion of greengram as a legume aimed to meet the protein requirements of malnourished people. It also sought to inform policies for sustainable agriculture by assessing the impact of CA technologies (ZT alone, ZT with residue retention, and ZT with residue retention plus bed planting with N management) on the maize-wheat-greengram system in northwestern IGP, India. The study hypothesized that CA-based permanent broad-bed planting with a judicious combination of N would enhance crop growth, productivity, and efficiency in nutrient use, water, and energy.
The field experiment was conducted at the Division of Agronomy, ICAR-Indian Agricultural Research Institute, New Delhi (28°35′N latitude, 77°12′E longitude, and at an altitude of 228.6 m above mean sea level) during the rainy, winter, and summer seasons of 2018–19 and 2019–20. The study site had a semi-arid and subtropical climate. The mean annual precipitation was around 710 mm during the study period. The soil of the experimental site was clayey loam with a pH of 8.2, 0.60% organic C, 285 kg ha−1 available N, 18 kg ha−1 available P, and 329 kg ha−1 available K.
The experiment was conducted for two consecutive years, 2018–19 and 2019–20, in a randomized complete block design (RCBD) with three replications. It was conducted in a maize-wheat-greengram system. There were 10 CA/CT treatments initiated under a long-term CA system in 2010. Different CA-based practices such as zero till (ZT) permanent narrow, broad, and flatbeds with and without retention of maize, wheat, and greengram crops residues and 75% and 100% of the recommended dose of N were compared with CT practice (Table 1). The treatments were comprised of one CT practice [conventional tillage without residue with 100% N (CT)] and nine CA practices such as permanent narrow bed without residue with 100% N (PNB), permanent narrow bed with residue with 75% N (PNB + R + 75N), permanent narrow bed with residue with 100% N (PNB + R + 100N), permanent broad bed without residue with 100% N (PBB), permanent broad bed with residue with 75% N (PBB + R + 75N), permanent broad bed with residue with 100% N (PBB + R + 100N), flatbed without residue with 100% N (FB), flatbed with residue with 75% N (FB + R + 75N) and flatbed with residue with 100% N (FB + R + 100N). The dimension of narrow bed was 40 cm bed width and 30 cm furrow, whereas, broad bed was 110 cm bed width and 30 cm furrow width (Table 1). The same treatments were followed for each maize, wheat, and greengram crop. For residue removal and CT plots, maize, wheat, and greengram crops were harvested at ~3 cm above the soil surface. About 40% of maize stover and full stover of greengram were retained as anchored residue in all the residue retention plots (PNB + R, PBB + R, and FB + R). To quantify the residue load of each plot, an area of 1.0 m2 was selected in each plot, and residue samples from that area were weighed after oven drying. This dry weight was then equated with the total biomass obtained in respective plots to quantify the amount of residue retained in every treatment. The sowing of the wheat was done in anchored maize residue plots, those having residue treatment. In a similar way, 40% of the residue of wheat was retained where the greengram crop was sown, whereas the whole greengram crop residue was retained where maize was sown. The total amount of residue added in each residue retention plot was estimated as the sum of crop residues retained above ground and root biomass added in the soil. During 2018–19 a total of 11.22, 11.30, 11.25, 11.47, 11.26 and 11.43 t ha−1 crop residue of all three crops were added in PNB + R + 75N, PNB + R + 100N, PBB + R + 75N, PBB + R + 100N, FB + R + 75N and FB + R + 100N treatment respectively. Similarly, a total of 11.31, 11.48, 11.47, 11.60, 11.44 and 11.59 t ha−1 crop residue were added in PNB + R + 75N, PNB + R + 100N, PBB + R + 75N, PBB + R + 100N, FB + R + 75N and FB + R + 100N treatment respectively during 2019–20 (Supplementary Table 1).
The CT plot was prepared with two passes of tractor-drawn disc plow and thereafter two passes of cultivator followed by planking. There was no plowing in CA-based treatments (Table 1).
Maize variety “PMH 1,” wheat variety “HDCSW 18,” and greengram variety “SML 832” were sown during the rainy, winter, and summer seasons, respectively. Maize, wheat, and greengram crops were sown at a seed rate of 20, 100, and 20 kg ha−1, respectively. Row spacing of 70, 20, and 20 cm was maintained for maize, wheat, and greengram crops, respectively. In CT, the sowing of maize, wheat, and greengram crops was conducted by a tractor-drawn seed-cum-fertilizer drill. In PNB plots, sowing was done by a bed planter, whereas in PBB and FB plots, it was done by a turbo-happy seeder.
A common dose of 150 kg N, 26.2 kg P, and 33.1 kg K ha−1 was given to maize and wheat crops, whereas in greengram, 18 kg N and 20.1 kg P ha−1 was applied through 100 kg di-ammonium phosphate (DAP). The recommended dose of 150 kg N was given to maize and wheat under the 100% N treatments, irrespective of CA and CT plots. In CA-based plots with 75% N, 112.5 kg N was applied. At the time of sowing, the full doses of P and K and the half dose of N were applied to both maize and wheat. At 30 and 60 days after sowing (DAS) in maize, and following the first and second irrigations in wheat, the remaining N was top-dressed in two equal splits. Fertilizers were applied during top dressing, with caution, to leave the furrows intact, and fertilizers were applied along the crop rows on permanent narrow and broad beds. When seeding greengram, the entire amount of fertilizer was applied as basal.
Root samples were collected at 60 DAS in maize and wheat and at 45 DAS in greengram. In bed planting treatments with residue retained and residue removal plots, root samples were collected from each of the three rows on a given bed. Root samples were obtained from three consecutive rows for flatbed treatments and CT. The root samples were collected using the root auger, which had a diameter of 7 cm and a soil depth of 0–15 cm. Root samples were collected in plastic bags. Then, a preliminary separation of roots from the soil was done in a plastic bucket containing water. The root sample was soaked in water for 30 minutes, and the debris and the soil particles were washed off. Then, the liquid was poured via a bigger sieve (5 mm) and a smaller sieve (2 mm), respectively. The trapped roots collected in each sieve were washed with running tap water and then placed into containers. Then, the root samples were investigated using WinRHIZO software (Himmelbauer et al., 2004; Guan et al., 2014). This software was utilized to scan the root samples and analyze the obtained images. Total root length, surface area, volume, and average diameter were recorded. The root samples were then dried in an oven at 65°C to a constant weight for 24 hours, and the oven-dried weight was recorded using an electronic balance. Then root length density (RLD), root mass density (RMD), and root volume density (RVD) were computed by dividing the length, mass, and volume of the root by the volume of soil collected.
To estimate grain/cob and straw/stover yield, maize and wheat crops from the net plot area of 10 m2 were harvested and sun-dried. After drying, maize grains were separated from the cobs using a maize sheller and sun-dried. The grain yield was measured at 12% moisture. Manual threshing was done to obtain grain from spikes in wheat. Similarly, in greengram, matured pods were hand-picked from the net plot of 10 m2 and sun-dried. Dried pods from each treatment plot were manually weighed and threshed to obtain the grain. The weight of the greengram grain was recorded from a net plot basis, and then it was changed into tons per hectare (t ha−1). The stover yield was measured after picking pods from plants. Grain and straw/stover yield of all the crops were obtained from each treatment and were expressed in t ha−1.
N in plant samples was determined using the Kjeldahl method, P was measured by the vanadomolybdophosphoric yellow color method, and K was estimated using flame photometry. Nutrient uptake was calculated by using the following expression:
Nutrient uptake (kg ha−1) in grain or straw = [% Nutrient content in grain or straw x grain or straw yield (kg ha−1)]/100.
Total uptake of a particular nutrient (kg ha−1) = Nutrient uptake in grain (kg ha−1) + Nutrient uptake in straw (kg ha−1).
Partial factor productivity (PFP) of nutrients (N, P and K) in maize, wheat and greengram was estimated by dividing the yield (kg ha−1) with total amount of NPK applied through fertilizers (kg ha−1) in the respective crops.
Water productivity (kg grain ha−1 mm−1 of water) was calculated as given by Raj et al. (2017) and Das et al. (2018):
Water productivity (kg grain ha-mm−1 of water) = [Grain yield (kg ha−1)/ Total water applied (mm)].
Total water application involved both effective rainfall and irrigation water. The effective rainfall was determined using standard methods (provided by the FAO). FAO's effective rainfall estimation method calculates the portion of total rainfall available for crop use after accounting for losses like runoff, deep percolation, and evaporation, using either water balance approach (Mohammad et al., 2018). The Time Domain Reflectometer was used to measure the periodic soil moisture content before every irrigation to estimate the frequency and quantity of water. Crops were irrigated if the available soil moisture at the root zone decreased below 50%. The amount of irrigation water was measured with a star flow meter and from the wetted region of the field channel (Das et al., 2018). This method was used to measure the irrigation water depth in each treatment plot during every application period.
Energy-use indices were measured using the formula as suggested by Mittal and Dhawan (1988), Singh et al. (1997), Saad et al. (2016) and Parihar et al. (2018).
Total input energy was estimated by adding energy equivalents of all inputs. Operation-wise energy was estimated based on input energy consumed in field preparation, sowing, fertilizer application, irrigation, intercultural operation, plant protection, harvesting, and threshing to determine the energy consumed in the above crop management practices under CT and CA-based treatments. Although manual harvesting of grain and straw samples from the net areas was done, mechanized harvesting and threshing were assumed to calculate energy on a per-hectare basis.
Using the corresponding energy coefficients provided in Supplementary Table 2, the energy produced from the grain and straw/stover yields of maize, wheat, and greengram crops and their equivalent yields were converted in terms of energy (MJ ha−1). The total energy equivalents of the grain and straw/stover yields were added up to determine the output energy.
Net energy was calculated as the difference of total output energy (Eo) and total input energy (Ei). It was expressed in MJ ha−1.
Net energy (MJ ha−1) = Output energy (MJ ha−1) – Input energy (MJ ha−1).
Energy ratio was calculated by diving output energy (MJ ha−1) of total biomass to input energy (MJ ha−1).
Energy ratio = Output energy (MJ ha−1)/ Input energy (MJ ha−1).
The calculation of energy productivity involved dividing the grain yield (kg ha−1) to total input energy (MJ ha−1).
Energy productivity (kg MJ−1) = Grain yield (kg ha−1)/total input energy (MJ ha−1).
The statistical significance of treatment effects on studied variables like root growth parameters, crop productivity, partial factor productivity of nutrients, water productivity, and energy use efficiencies was analyzed using analysis of variance (ANOVA) techniques for a randomized complete block design. The PROC GLM procedure was followed to analyze the data in SAS 9.3 software (SAS Institute Inc., Cary, NC, USA). The contrast analysis was carried out for system productivity and water productivity (Supplementary Table 3).
A comparison of treatment means was done employing Fisher's least significant difference test. The treatment significance was decided at p = 0.05.
Root growth parameters (root length density, root mass density, and root volume density) of maize, wheat, and greengram were significantly influenced by growing seasons. All growth parameters in maize, wheat, and greengram were higher in 2018–19 than in crops grown during 2019–20. CA practices had a greater influence on crop root growth than CT across the crops (Tables 2–4). Residue retention as surface mulch and the inclusion of greengram into the maize-wheat system resulted in better root growth parameters. The residue removal treatments were not found beneficial as compared to treatments with residue retention in this regard. The significantly higher root growth parameters, such as root length, mass, and volume densities in maize, wheat, and greengram crops, were observed under PBB + R + 100N. The treatment FB + R + 100N was also observed to be comparable in this regard. In maize, the CA-based practices resulted in 16.9%−56.0% higher root length density, 5.9%−27.8% higher root mass density, and 3.8%−25.7% higher root volume density than CT. The higher values of root length, mass, and volume densities of maize in CA-based practices validated better root growth conditions than CT. Residue retention, along with 100% N application, resulted in increased belowground biomass growth in maize. The treatment PBB + R + 100N was proved to be significantly superior in improving the root growth of wheat. This treatment showed significantly higher root length density (2.84 cm cm−3), root mass density (8.69 × 10−4 g cm−3), and root volume density (6.71 × 10−3 cm3 cm−3) of wheat. Similarly, the CA-based practices registered 8.9%−26.5% higher root length density, 8.8%−40.5% higher root mass density, and 2.5%−15.1% higher root volume density of greengram as compared to CT. The treatments PBB + R + 100N and FB + R + 100N showed significantly higher root length, mass density, and volume density.
The grain yield of maize, wheat, and greengram and the system productivity (wheat grain equivalent yield) were significantly influenced by growing years and CA and CT practices (Table 5). Maize grain yield and system productivity during 2018–19 were significantly higher than in 2019–20, whereas wheat and greengram grain yield were similar during both the studied years. CA-based PBB + R + 100N treatment registered significantly higher yield of maize, wheat, and greengram and system productivity than CT and other CA-based treatments, except CA-based practices with residue retention with 100% N (FB + R + 100N and PNB + R + 100N), where, this treatment resulted in a similar yield of maize, wheat and greengram and system productivity with the former treatment. Treatment PBB + R + 100N resulted in 22.0%, 27.1%, 55.6%, and 31.1% higher maize, wheat, and greengram grain yield and system productivity, respectively, than CT. It also led to 6.2 and 2.1% higher system productivity than PNB + R + 100N and FB + R + 100N, respectively. The CA-based practices with residue retention with 75% N performed better than CA-based practices with no residue application. The triple zero-till system in bed planting practices and the application of maize, wheat, and greengram residues resulted in higher system productivity and proved superior to CT practice.
Similarly, the contrast analysis for system productivity showed significant variation between CA vs. CT, residue vs. no-residue, PNB vs. PBB and PNB vs. FB, except 100 vs. 75 N and PBB vs. FB (Table 6). The system productivity was 18.9% higher in CA than CT, 7.5% higher in residue retained treatment over no-residue, and 4.3% higher in PBB than PNB. The contrast analysis between 100% N and 75% N applications showed that the application of 100% N resulted in similar system productivity to that of the 75% N application. Similarly, PBB resulted in similar system productivity to that obtained in FB treatment.
Table 6. Contrasts on system productivity (t ha−1) and total water productivity (kg ha-mm−1) between pairs of treatments.
The nutrients such as N, phosphorus (P), and potassium (K) uptake by maize and N uptake by wheat and greengram were not significantly varied during the crop growing season of 2018–19 and 2019–20, whereas, P and K uptake by wheat and P uptake by greengram were significantly higher during 2018–19 than 2019–20 (Figures 1–3). The N, P, and K uptake by maize, wheat, and greengram were substantially affected due to differential tillage, residue, crop establishment, and N management practices (Figures 1–3). The treatments with residue retention had significantly higher nutrient uptake than residue removal plots. Also, the residue retained plots along with 100% N application recorded higher values of nutrient uptake in comparison to treatments with 75% N application. Results showed that the treatment PBB + R + 100N led to significantly higher total N, P, and K uptake by maize grain and stover.
Figure 1. Total nitrogen (N), phosphorus (P) and potassium (K) uptake by maize grain and stover across treatments. Treatment means followed by same lowercase letters on top of bar do not differ by Fisher's least significant difference (LSD) test at p = 0.05.
Figure 2. Total nitrogen (N), phosphorus (P) and potassium (K) uptake by wheat grain and stover across treatments. Treatment means followed by same lowercase letters on top of bar do not differ by Fisher's least significant difference (LSD) test at p = 0.05.
Figure 3. Total nitrogen (N) and phosphorus (P) uptake by greengram grain and stover across treatments. Treatment means followed by same lowercase letters on top of bar do not differ by Fisher's least significant difference (LSD) test at p = 0.05.
Similarly, the total N uptake by wheat grain and straw was recorded under PBB + R + 100N. It registered an 87.0% increase in N uptake than CT. The same trend was observed in the uptake of P and K. The treatment PBB + R + 100N also resulted in a significantly higher uptake of total N (105.2 kg ha−1) by greengram grain and stover and was statistically at par with FB + R + 100N and PNB + R + 100N. It resulted in 98.5% higher N uptake than the CT system (53 kg ha−1). The highest P uptake by greengram grain and stover was also observed under PBB + R + 100N.
The nutrient use efficiencies were evaluated in terms of partial factor productivity (PFP) of N, P, and K. The PFP of nutrients in the maize-wheat-greengram system was estimated in terms of wheat grain equivalent yield per unit application of each nutrient (N, P, and K). The crop-growing seasons had a significant effect on the PFP of N, P, and K (Figure 4). The crops grown during 2018–19 observed significantly higher PFP of N, P, and K than crops growing in the season of 2019–20. The tillage, residue, crop establishment, and N management also significantly influenced the PFP of all primary nutrients in the maize-wheat-greengram system (Figure 4). All CA-based treatments led to higher PFP of N, P, and K than CT treatment. Among CA-based practices, PBB + R + 75N and FB + R + 75N showed significantly higher PFP of N over CT and other CA-based treatments. Treatment PBB + R + 75N registered 59.4%, 46.7%, 44.4%, 44.1%, 28.9%, 23.9%, 21.4%, 5.5% and 1.3% higher PFP of N than CT, PNB, FB, PBB, PNB + R + 100N, FB + R + 100N, PBB + R + 100N, PNB + R + 75N and FB + R + 75N, respectively. The CA-based residue retained treatments with 75% N application outperformed 100% N applied treatments in registering higher PFP of N. Concerning PFP of P and K, CA-based treatments, namely, PBB + R + 100N and FB + R + 100N resulted in significantly higher PFP of both the nutrients over rest of the treatments. Treatment FB + R + 100N increased PFP of P by 31.1, 20.7, 18.9, 18.4, 13.6, 9.0, 7.8, 6.2 and 2.1% than CT, PNB, FB, PBB, PNB + R + 75N, FB + R + 75N, PBB + R + 75N, PNB + R + 100N and FB + R + 100N, respectively. Similarly, the PFP of K was highest in the PBB + R + 100N, closely followed by FB + R + 100N. However, the remaining treatments were inferior in this regard.
Figure 4. Partial factor productivity of nutrients (N, P and K) in maize-wheat-greengram system across treatments. Treatment means followed by same lowercase letters on top of bar do not differ by Fisher's least significant difference (LSD) test at p = 0.05.
The quantity of irrigation water and total water use varied across the years; due to that, irrigation water productivity and total water productivity were significantly influenced under the maize-wheat-greengram system (Table 7). The system's irrigation water productivity and total water productivity were 2.1 and 12.7% higher during 2018–19 than in 2019–20, respectively. The tillage, residues, crop establishment, and N management significantly influenced the amount of irrigation and total water use and irrigation, as well as the total water productivity of the system. The highest amount of irrigation water and total water consumption was observed in CT treatment. The CT observed 908 mm of irrigation water and 1,602 mm of total water consumption during the study period. Among CA-based practices, the treatments with residue retention retained more water, which resulted in less irrigation requirement than treatments with no residue application. Among CA-based practices with residue retention, the broad-bed practices resulted in more water savings than the narrow beds and flat beds with residue retention. The treatment PBB + R registered an average of 685.5 mm of irrigation water use. Broad-bed practices registered 10.2% and 18.1% of irrigation water application savings compared to narrow-bed and flat-bed practices. The treatment PBB + R + 100N registered significantly higher irrigation water productivity (24.7 kg ha-mm−1) and total water productivity (13.1 kg ha-mm−1) than the rest of the treatments. The other efficient treatments were observed to be PBB + R + 75N and PNB + R + 100N. The broad-bed practices with residue retention with 100% N (PBB + R + 100N) gained an average of 18.2% and 24.4% higher irrigation water productivity than narrow-bed with residue retention with 100% N (PNB + R + 100N) and flat-bed with residue retention with 100% N (FB + R + 100N), respectively. The results demonstrated that broad-bed practices with residue retention outperformed other CA-based practices regarding water conservation and irrigation water productivity.
Similarly, the contrast analysis for total water productivity showed significant variation between CA vs. CT, residue vs. no-residue, PNB vs. PBB and PBB vs. FB, except 100N vs. 75N and PNB vs. FB (Table 6). The total water productivity was 38.2% higher in CA than CT, 9.6% higher in residue retained treatment over no-residue, 10.1% higher in PBB than PNB, and 12.4% higher in PBB than FB. The contrast analysis between 100% N and 75% N applications showed that the application of 100% N resulted in a similar total water productivity to that of 75% N applications. Similarly, PNB resulted in total water productivity similar to that obtained in FB treatment.
Total output and net energy return did not differ, whereas energy productivity and energy ratio were significantly impacted due to the crop growing seasons/years (Table 8). Tillage, residue, crop establishment, and N management practices significantly influenced the energy relationship among various treatments in the maize-wheat-greengram system. The CA-based practices without residue retention had the lowest input energy compared to CT and CA-based practices with residue retention. The treatment PNB registered 18.9% lower input energy than CT. CA-based practices with residue retention incurred higher input energy due to energy involved in residue retention. However, the CA-based practices with residue retention outperformed CT as well as CA-based residue removal treatments in terms of registering higher output energy. The treatment PBB + R + 100N registered significantly higher output energy than the rest of the practices and remained at par with FB + R + 100N. It resulted in 22.5% higher output energy than CT treatment.
Table 8. Total input energy, output energy, net energy, energy productivity and energy ratio in maize-wheat-greengram system across treatments.
On the contrary, the CA-based residue removal practices obtained significantly higher energy productivity, net energy return, and energy ratio than the practices with residue retention and CT due to energy savings in no residue application. Among these practices, the treatment PBB significantly registered higher energy productivity (0.333 kg/MJ), and it was found to be comparable with other CA-based residue removal practices (PNB and FB). The same trend was observed in registering net energy return as well as energy ratio. The CA-based residue retained treatments with 75% and 100% N applications were found to be comparable in terms of energy productivity and energy ratio.
In the present study, practices like crop establishment, ZT, crop residue retention, and crop rotation for an extended period (10 years) had a significant direct and indirect impact on better root growth and higher grain yield and system productivity. The root system plays a pivotal role in plant growth and development. The CA practices provide a favorable soil environment (i.e., lesser compaction and lower bulk density) and moderate soil-air-water retention and hydrothermal regimes, leading to better root growth (Kumar et al., 2018; Mondal et al., 2019). CA-based broad-bed planting practices with residue retention led to improved crop root development compared to narrow-bed and flat-bed planting practices. Furthermore, using 100% N in PBB + R might assist in decomposing previous crop residues, resulting in better soil physical conditions for crop root development. The results of root growth parameters confirmed better crop root development in CA than in CT. It corroborated the findings of Choudhary and Behera (2020). According to Blanco-Canqui and Lal (2007), the better root growth observed under CA than in CT might be linked to decreased compaction. Adopting CA-based practices, in conjunction with appropriate N management, may be beneficial for improving root growth characteristics in maize, wheat, and greengram crops grown in a maize-wheat-greengram system.
The contrast analysis on system productivity revealed that the CA-based practices caused a significant increase in system productivity compared to CT practices in both years. The increased system productivity resulted from the beneficial effects of residue retention and improved crop-establishment practices on maize, wheat, and greengram yields. There was a 31.1% increase in yield under PBB + R + 100N as compared to CT, confirming the beneficial effects of residue retention under CA-based practices. Das et al. (2018) also reported that in the maize-wheat system, PBB + R plots resulted in a 10% increase in system productivity and 12% higher net returns compared to CT plots. Among CA-based bed planting practices, PBB + R retained more residues than PNB + R due to a more uniform distribution of residues on top of the broad beds. The increased yield under PBB + R treatment might be attributed to favorable mulching effects of crop residues. Kulagowski et al. (2021) studied the effects of conservation agriculture maize-based cropping systems on crop performance and soil health in New Caledonia and documented that cropping system management had both direct and indirect effects on crop productivity leading to a 1.3-times higher maize yield under CA than under CT.
Residue retention resulted in greater infiltration, higher soil moisture conservation on beds, reduced run-off and erosion, better temperature moderation, inhibition of weed proliferation and more soil microbial activity resulting in biological tillage under PBB + R with comparison to PNB + R (Swift and Sanchez, 1984; Sayre and Hobbs, 2004; Chauhan et al., 2007; Thomas et al., 2007; Bhattacharyya et al., 2013; Das et al., 2018; Baghel et al., 2020; Jat et al., 2020). The above factors contributed to increased crop yield under PBB + R + 100N. Greengram integration and residue retention under ZT enhanced soil characteristics, resulting in increased maize and wheat yields compared to the CT system without greengram residue (Hazra et al., 2018).
CA practices improve the soil's organic carbon and can enormously benefit plant nutrient availability and distribution in the soil. In the present investigation, the treatment PBB + R + 100N significantly improved nutrient (N, P, and K) uptake by both grain and stover/straw in maize, wheat, and greengram crops. The increased plant nutrient content in maize, wheat, greengram grain, and stover/straw under CA might be attributed to improved root growth, which raised nutrient concentration in these crops owing to growing forage area for nutrient removal under permanent beds with residue, resulting in increased nutrient absorption (Parihar et al., 2018).
The partial factor productivity (PFP) of nutrients (N, P, and K) in maize, wheat, and greengram crops differed significantly among the treatments due to different tillage, residue, crop establishment, and N management practices. Because CA-based practices produced more grain than CT practices, they had a significantly higher PFP of nutrients. Residue retention practices significantly improved the PFP of nutrients compared to residue removal practices. The treatment PBB + R + 75N significantly recorded higher PFP (N) in maize and wheat crops. The application of 75% N increased N use efficiency, and the yield gains of 100% N were found to be par. These treatments with 75% N saved 25% N and ultimately increased N use efficiency. The N fertilizer requirement under CA may be expected to decrease over time as a result of organic matter accumulation (Riley et al., 1994) and reduced erosion losses (Schoenau and Campbell, 1996). The present study revealed that significantly higher PFP of P and K were recorded under PBB + R + 100N. However, the CA-based residue retained treatments with 75% N application outperformed 100% N applied treatments in registering higher PFP of total nutrients (N, P, and K). The higher system productivity (wheat grain equivalent yield), along with 75% N application, resulted in the highest PFP of nutrients under PBB + R + 75N. N management is crucial due to its higher greenhouse gas footprint (Cheng et al., 2015). Limon-Ortega et al. (2000) and Fahong et al. (2004) reported that bed planting practices, along with appropriate management strategies, boosted N use efficiency compared with conventional planting after a few initial years. Singh et al. (2008) observed that increased agronomic efficiency of N in wheat under rice residue retention was linked with either a lower rate of fertilizer N or a rise in grain yield, which outweighed any yield gain from mulching in the absence of fertilizer.
Crops produced using ZT with partial or complete residue retention would increase crop and cropping system yields while decreasing crop water demand and boosting crop water productivity (Islam et al., 2019). Several researchers have observed that resource-saving technologies such as ZT can be effective in increasing field-level irrigation efficiency through savings of irrigation water (Humphreys et al., 2005; Jehangir et al., 2007; Erenstein and Laxmi, 2008; Das et al., 2018). ZT can improve soil structure and enable crop residue buildup, which is associated with greater water retention, improved infiltration, and lower total water usage (Erenstein, 2003). The results of the contrast analysis on total water productivity in maize, wheat, and greengram crops of the current study showed that CA-based practices significantly increased total water productivity compared to CT practices. The highest amount of irrigation water consumption was found in CT. It recorded an average of 908 mm of irrigation water and 1,602 mm of total water consumption during the study period under the maize-wheat-greengram system. Compared to CT, the CA-based practices resulted in 4.4%−24.5% savings in irrigation water use in the maize-wheat-greengram system. Among CA-based practices, the treatments with residue retention retained more water, which resulted in less irrigation requirement than treatments with no residue application. Among CA-based practices with residue retention, the broad-bed practices resulted in more water savings than the narrow beds and flatbeds with residue retention. The broad-bed practices registered an average of 10.2% and 18.1% savings in irrigation water application compared to narrow-bed and flatbed with residue retention, respectively. The numbers of beds and furrows per plot in broad bed practices were 6 and 7, respectively, but in narrow bed practices, the numbers of beds and furrows per plot were 12 and 13, respectively.
With furrow dimensions equal, the total amount of water applied in a given plot area was greater in narrow beds than in broad beds. The treatment PBB + R + 100N registered 36.6% and 37.2% higher total water productivity in maize as compared to CT in the first and second years of study. In wheat, plots under PBB + R + 100N increased irrigation water productivity by 54.4% and 58.5% compared to CT during 2018–19 and 2019–20, respectively. In greengram, the same treatment registered significantly higher irrigation water productivity due to lesser water use and higher crop productivity. PBB + R + 100N gained an average of 18.2% and 24.4% higher irrigation water productivity than PNB + R + 100N and FB + R + 100N, respectively, during the study period under the maize-wheat-greengram system. The subsequent best treatment was found to be PNB + R + 75N for registering higher irrigation as well as total water productivity. Improved crop establishment practice combined with decreased irrigation water use resulted in considerably improved irrigation and total water productivity under PBB + R + 100N. It could be due to increased root length, mass, and volume density under this treatment, leading to more soil water extraction and less reliance on irrigation water. In addition, permanent beds move water quicker, resulting in irrigation water savings (Das et al., 2014; Mohammad et al., 2018). Economic water productivity was also estimated to be greater under PBB + R + 100N because of better soil moisture storage and decreased irrigation water consumption. Previous studies also observed similar results (Das et al., 2014, 2018; Parihar et al., 2016, 2018).
Resources conservation practices such as PBB, PNB, and FB without residue had the lowest input energy among all CA and CT practices. The plots under CT registered lower input energy than the CA-based practices with residue retention. The CA-based practices with residue retention incurred higher input energy due to the energy involved in residue retention. Crop residue emitted the highest input energy in CA-based residue retention practices. However, total input energy was found to be lower in CT and PBB, PNB, and FB without residue due to input energy savings from residue application. The PBB, PNB, and FB without residue were observed to be superior in terms of generating lower input energy when compared to CT, owing to input energy savings due to field preparation as well as irrigation water savings. Among N management practices, the practices with 100%N recorded higher input energy than those with 75% N application. However, the CA-based practices with residue retention outperformed CT and CA-based residue removal treatments in terms of registering higher output energy. The treatment PBB + R + 100N registered significantly higher output energy. Under CA-based residue retention practices, grain and total biomass yield were recorded as higher than the rest. Surface retained residues had a mulching effect under ZT, resulting in improved soil physical, chemical, and biological properties, C-sequestration (Karunakaran and Behera, 2013; Bhattacharyya et al., 2015), abiotic stress moderation (Saad et al., 2015), reduced surface crust and weed population (Susha et al., 2018). As a result, increased crop or system productivity was observed (Das et al., 2016). Crop and system-wise output energy followed the trends of crop biomass yields and were significantly higher under PBB + R + 100N. The energy consumed in land preparation and sowing was much lower in PBB + R + 100N, while crop residue retention led to higher input energy in this treatment. Permanent bed planting practices were observed to be superior to flatbed planting practices owing to irrigation water savings in bed planting practices since the furrow system in permanent beds required less irrigation water (Parihar et al., 2018).
Even though CA-based residue retention practices resulted in greater output energy and net energy return, the energy ratio and energy productivity were observed to be much higher under CA-based practices without residue owing to energy savings from no residue application. Because a large quantity of energy was invested through crop residues, the net energy return, energy ratio, and energy productivity did not follow the trend of output energy in CA-based residue retention practices. Saad et al. (2016) and Parihar et al. (2018) reported similar findings. According to Saad et al. (2016), energy in-flow by residue application in crop production did not enhance much outflow energy since crop residues predominantly improved soil quality when returned to soils and were not reflected in the output energy. Erenstein and Laxmi (2008) estimated seasonal savings in diesel for land preparation using ZT in the range of 15–60 l ha−1, with an average of 36 l ha−1, or 81% savings over IGP. Crop residues, a renewable energy resource, contribute the highest energy input under CA (Saad et al., 2016). However, crop residue retention has various advantages, including increased crop yield, enhanced soil health, and improved environmental quality. Furthermore, crop residues may be used to replenish plant nutrients while having no negative impact on crop productivity (Prasad et al., 1999; Das et al., 2013; Saad et al., 2016; Bhattacharyya et al., 2013, 2019).
The conventional maize-wheat system is highly tillage-intensive and inefficient in resource use, including water, energy, labor, and nutrients. Transitioning to a maize-wheat-greengram cropping system under conservation agriculture (CA) can significantly enhance soil health and sustainability through crop diversification. This system improves soil organic matter, boosts microbial activity, and enhances nutrient cycling. Adopting CA principles in the maize-wheat-greengram system can also improve water-use efficiency and strengthen climate resilience. Additionally, it increases farm profitability by utilizing fallow land for greengram cultivation, generating additional income, and enhancing soil fertility through nitrogen fixation. The inclusion of pulses in the cropping system contributes to dietary protein intake, addressing nutritional security concerns, while also aiding in regional self-sufficiency in pulse production.
Moreover, the long-term adoption of this CA-based system can substantially improve soil physical properties, such as aggregation, bulk density, penetration resistance, water infiltration, and saturated hydraulic conductivity, thereby mitigating land degradation in the Indo-Gangetic Plains (IGP). Findings from this study indicate that intensifying the maize-wheat system with a short-duration pulse crop like greengram under permanent broad bed with residue retention can enhance farm productivity by 31% compared to conventional farmer practices. This, in turn, supports India's food and nutritional security. Furthermore, the study's findings align with India's commitments to COP-27 and contribute to achieving multiple Sustainable Development Goals (SDGs). To facilitate widespread adoption and scaling up of this technology in the IGP, concerted efforts from farmers, researchers, and policymakers are essential, alongside targeted policy interventions and market linkages. Governments can also incentivize the transition by introducing carbon credit programs for farmers adopting CA-based diversified systems.
The conventional tilled maize-wheat system faces challenges for sustainability, including low economic returns, soil physical and chemical health degradation, accelerated oxidation of soil organic matter, and negative environmental impacts. Adopting CA practices, such as minimizing soil disturbance, retaining residues, and diversifying crops, can enhance soil properties, improve system productivity and profitability, and contribute to climate change mitigation. The results of current study corroborate these ideas, confirming our hypotheses. The CA-based practice involving zero-tillage permanent broad bed (PBB) + residue retention (R) + recommended dose of N (100N) led to significant improvement in root growth parameters, grain yield, system productivity, as well as nutrient, energy, and water productivity of the maize-wheat-greengram cropping system compared to CT (farmers' practice) system. The PBB + R + 100N also bring about ~31% higher system productivity and 24.5% saving in irrigation water as compared to CT. The study also included horizontal crop intensification with a summer greengram, which could benefit the farmers of IGP of India. The CA-based PBB + R + 100N system in maize-wheat-greengram rotation offers a viable alternative to the dominant rice-wheat cropping system, which faces challenges like yield stagnation and low input-use efficiency. The amalgamation of these CA technologies in the maize-wheat-greengram system can also maintain the long-term sustainability of cereal-based cropping systems in IGP. The future research should explore the long-term impacts of CA on soil health, greenhouse gas emissions, and biodiversity conservation. Additionally, the economic feasibility and adoption potential of CA-based systems across different agro-ecological zones should be evaluated.
The original contributions presented in the study are included in the article/Supplementary material, further inquiries can be directed to the corresponding authors.
SonG: Data curation, Investigation, Writing – original draft. TD: Conceptualization, Writing – review & editing. RR: Validation, Writing – review & editing. SS: Formal analysis, Validation, Writing – review & editing. AM: Methodology, Software, Writing – review & editing. DB: Methodology, Visualization, Writing – original draft. KB: Methodology, Visualization, Writing – original draft. SouG: Formal analysis, Validation, Writing – review & editing. VS: Methodology, Software, Writing – review & editing. AR: Writing – original draft. GA: Formal analysis, Methodology, Writing – original draft. PS: Formal analysis, Methodology, Writing – original draft. TS: Methodology, Software, Writing – review & editing.
The author(s) declare that no financial support was received for the research and/or publication of this article.
The authors are grateful to ICAR-Indian Agricultural Research Institute, New Delhi for providing the necessary services and supplies during the investigation.
The authors declare that the research was conducted in the absence of any commercial or financial relationships that could be construed as a potential conflict of interest.
All claims expressed in this article are solely those of the authors and do not necessarily represent those of their affiliated organizations, or those of the publisher, the editors and the reviewers. Any product that may be evaluated in this article, or claim that may be made by its manufacturer, is not guaranteed or endorsed by the publisher.
The Supplementary Material for this article can be found online at: https://www.frontiersin.org/articles/10.3389/fsufs.2025.1470188/full#supplementary-material
Aulakh, M., and Grant, C. A. (2008). Integrated Nutrient Management for Sustainable Crop Production. Boca Raton, FL: CRC Press, 622. doi: 10.1201/9780367803216
Babu, S., Singh, R., Kumar, S., Rathore, S. S., Yadav, D., Yadav, S. K., et al. (2023). Biochar implications in cleaner agricultural production and environmental sustainability. Env. Sci. Adv. 2, 1042–1059. doi: 10.1039/D2VA00324D
Baghel, J. K., Das, T. K., Mukherjee, I., Nath, C. P., Bhattacharyya, R., Ghosh, S., et al. (2020). Impacts of conservation agriculture and herbicides on weeds, nematodes, herbicide residue and productivity in direct-seeded rice. Soil Tillage Res. 201:104634. doi: 10.1016/j.still.2020.104634
Bhattacharyya, R., Das, T. K., Das, S., Dey, A., Patra, A. K., Agnihotri, R., et al. (2019). Four years of conservation agriculture affects topsoil aggregate-associated 15nitrogen but not the 15nitrogen use efficiency by wheat in a semi-arid climate. Geoderma 337, 333–340. doi: 10.1016/j.geoderma.2018.09.036
Bhattacharyya, R., Das, T. K., Pramanik, P., Ganeshan, V., Saad, A. A., Sharma, A. R., et al. (2013). Impacts of conservation agriculture on soil aggregation and aggregate-associated N under an irrigated agroecosystem of the Indo-Gangetic Plains. Nutr. Cycl. Agroecosyst. 96, 185–202. doi: 10.1007/s10705-013-9585-6
Bhattacharyya, R., Das, T. K., Sudhishri, S., Dudwal, B., Sharma, A. R., Bhatia, A., et al. (2015). Conservation agriculture effects on soil organic carbon accumulation and crop productivity under a rice–wheat cropping system in the western Indo-Gangetic Plains. Eur. J. Agron. 70, 11–21. doi: 10.1016/j.eja.2015.06.006
Blanco-Canqui, H., and Lal, R. (2007). Impact of long-term wheat straw management on soil hydraulic properties under no-tillage. Soil Sci. Soc. Am. J. 71, 1166–1173. doi: 10.2136/sssaj2006.0411
Chaudhary, V. P., Singh, K. K., Pratibha, G., Bhattacharyya, R., Shamim, M., Srinivas, I., et al. (2017). Energy conservation and greenhouse gas mitigation under different production systems in rice cultivation. Energy 130, 307–317. doi: 10.1016/j.energy.2017.04.131
Chauhan, B. S., Gill, G. S., and Preston, C. (2007). Effect of seeding systems and dinitroaniline herbicides on emergence and control of rigid ryegrass (Lolium rigidum) in wheat. Weed Technol. 21, 53–58. doi: 10.1614/WT-06-016.1
Chauhan, B. S., Mahajan, G., Sardana, V., Timsina, J., and Jat, M. L. (2012). Productivity and sustainability of the rice–wheat cropping system in the Indo-Gangetic Plains of the Indian subcontinent: problems, opportunities, and strategies. Adv. Agron. 117, 315–369. doi: 10.1016/B978-0-12-394278-4.00006-4
Cheng, K., Yan, M., Nayak, D., Pan, G. X., Smith, P., Zheng, J. F., et al. (2015). Carbon footprint of crop production in China: an analysis of national statistics data. J. Agric. Sci. 153, 422–431. doi: 10.1017/S0021859614000665
Choudhary, R. L., and Behera, U. K. (2020). Effect of conservation agriculture and nitrogen management on root architecture and soil properties in maize–wheat cropping system. J. Soil Water Conserv. 19, 261–270. doi: 10.5958/2455-7145.2020.00035.1
Das, T. K., Bandyopadhyay, K. K., Bhattacharyya, R., Sudhishri, S., Sharma, A. R., Behera, U. K., et al. (2016). Effects of conservation agriculture on crop productivity and water-use efficiency under an irrigated pigeonpea–wheat cropping system in the western Indo-Gangetic Plains. J. Agric. Sci. 154, 1327–1342. doi: 10.1017/S0021859615001264
Das, T. K., Bhattacharyya, R., Sharma, A. R., Das, S., Saad, A. A., Pathak, H., et al. (2013). Impacts of conservation agriculture on total soil organic carbon retention potential under an irrigated agro-ecosystem of the western Indo-Gangetic Plains. Europ. J. Agron. 51, 34–42. doi: 10.1016/j.eja.2013.07.003
Das, T. K., Bhattacharyya, R., Sudhishri, S., Sharma, A. R., Saharawat, Y. S., Bandyopadhyay, K. K., et al. (2014). Conservation agriculture in an irrigated cotton–wheat system of the western Indo-Gangetic Plains: crop and water productivity and economic profitability. Field Crops Res. 158, 24–33. doi: 10.1016/j.fcr.2013.12.017
Das, T. K., Ghosh, S., Das, A., Sen, S., Datta, D., Ghosh, S., et al. (2021). Conservation agriculture impacts on productivity, resource–use efficiency and environmental sustainability: a holistic review. Indian J. Agron. 66, S111–S127.
Das, T. K., Nath, C. P., Das, S., Biswas, S., Bhattacharyya, R., Sudhishri, S., et al. (2020). Conservation agriculture in rice-mustard cropping system for five years: impacts on crop productivity, profitability, water-use efficiency, and soil properties. Field Crops Res. 250:107781. doi: 10.1016/j.fcr.2020.107781
Das, T. K., Saharawat, Y. S., Bhattacharyya, R., Sudhishri, S., Bandyopadhyay, K. K., Sharma, A. R., et al. (2018). Conservation agriculture effects on crop and water productivity, profitability and soil organic carbon accumulation under a maize-wheat cropping system in the North-western Indo-Gangetic Plains. Field Crops Res. 215, 222–231. doi: 10.1016/j.fcr.2017.10.021
Dutta, S. K., Laing, A., Kumar, S., Shambhavi, S., Kumar, S., Kumar, B., et al. (2023). Sustainability, productivity, profitability and nutritional diversity of six cropping systems under conservation agriculture: a long term study in eastern India. Agric. Syst. 207:103641. doi: 10.1016/j.agsy.2023.103641
Erenstein, O. (2003). Smallholder conservation farming in the tropics and sub-tropics: a guide to the development and dissemination of mulching with crop residues and cover crops. Agric. Ecosyst. Environ. 100, 17–37. doi: 10.1016/S0167-8809(03)00150-6
Erenstein, O., and Laxmi, V. (2008). Zero tillage impacts in India's rice–wheat systems: a review. Soil Tillage Res. 100, 1–14. doi: 10.1016/j.still.2008.05.001
Fahong, W., Xuqing, W., and Sayre, K. (2004). Comparison of conventional, flood irrigated, flat planting with furrow irrigated, raised bed planting for winter wheat in China. Field Crops Res. 87, 35–42. doi: 10.1016/j.fcr.2003.09.003
Gathala, M. K., Kumar, V., Sharma, P. C., Saharawat, Y. S., Jat, H. S., Singh, M., et al. (2013). Optimizing intensive cereal-based cropping systems addressing current and future drivers of agricultural change in the northwestern Indo-Gangetic Plains of India. Agric. Ecosyst. Environ. 177, 85–97. doi: 10.1016/j.agee.2013.06.002
Ghosh, S., Das, T. K., Nath, C. P., Bhatia, A., Biswas, D. R., Bandyopadhyay, K. K., et al. (2023). Weed seedbank, above-ground weed community and crop yields under conventional and conservation agriculture practices in maize–wheat–mungbean rotation. Weed Res. 63, 270–281. doi: 10.1111/wre.12589
Ghosh, S., Das, T. K., Sharma, D., and Gupta, K. (2019). Potential of conservation agriculture for ecosystem services: a review. Indian J. Agric. Sci. 89, 1572–1579. doi: 10.56093/ijas.v89i10.94578
Ghosh, S., Das, T. K., Shivay, Y. S., Bandyopadhyay, K. K., Bhatia, A., Yeasin, M., et al. (2022a). Weed interference and wheat productivity in a conservation agriculture-based maize-wheat-mungbean system. J. Crop Weed 18, 111–119. doi: 10.22271/09746315.2022.v18.i1.1540
Ghosh, S., Das, T. K., Shivay, Y. S., Bandyopadhyay, K. K., Sudhishri, S., Bhatia, A., et al. (2022b). Weeds response and control efficiency, greengram productivity and resource-use efficiency under a conservation agriculture-based maize-wheat-greengram system. Indian J. Weed Sci. 54, 157–164. doi: 10.5958/0974-8164.2022.00030.2
Ghosh, S., Das, T. K., Shivay, Y. S., Bhatia, A., Biswas, D. R., Bandyopadhyay, K. K., et al. (2021). Conservation agriculture effects on weed dynamics and maize productivity in maize-wheat-greengram system in north-western Indo-Gangetic Plains of India. Indian J. Weed Sci. 53, 244–251. doi: 10.5958/0974-8164.2021.00045.9
Ghosh, S., Das, T. K., Shivay, Y. S., Bhatia, A., Sudhishri, S., Yeasin, M., et al. (2022c). Impact of conservation agriculture on wheat growth, productivity and nutrient uptake in maize–wheat–mungbean system. Int. J. Bio-resource Stress Manag. 13, 422–429. doi: 10.23910/1.2022.2806
Guan, D., Al-Kaisi, M. M., Zhang, Y., Duan, L., Tan, W., Zhang, M., et al. (2014). Tillage practices affect biomass and grain yield through regulating root growth, root-bleeding sap and nutrients uptake in summer maize. Field Crops Res. 157, 89–97. doi: 10.1016/j.fcr.2013.12.015
Gupta, R., and Seth, A. (2007). A review of resource conserving technologies for sustainable management of the rice–wheat cropping systems of the Indo-Gangetic plains (IGP). Crop Protect. 26, 436–447. doi: 10.1016/j.cropro.2006.04.030
Hazra, K. K., Ghosh, P. K., Venkatesh, M. S., Nath, C. P., Kumar, N., Singh, M., et al. (2018). Improving soil organic carbon pools through inclusion of summer mungbean in cereal-cereal cropping systems in Indo-Gangetic plain. Arch. Agron. Soil Sci. 64, 1690–1704. doi: 10.1080/03650340.2018.1451638
Himmelbauer, M., Loiskandl, W., and Kastanek, F. (2004). Estimating length, average diameter and surface area of roots using two different image analyses systems. Plant Soil 260, 111–120. doi: 10.1023/B:PLSO.0000030171.28821.55
Humphreys, E., Meisner, C., Gupta, R., Timsina, J., Beecher, H. G., Lu, T. Y., et al. (2005). Water saving in rice-wheat systems. Plant Prod. Sci. 8, 242–258. doi: 10.1626/pps.8.242
Islam, S., Gathala, M. K., Tiwari, T. P., Timsina, J., Laing, A. M., Maharjan, S., et al. (2019). Conservation agriculture based sustainable intensification: increasing yields and water productivity for smallholders of the Eastern Gangetic Plains. Field Crops Res. 238, 1–17. doi: 10.1016/j.fcr.2019.04.005
Jat, H. S., Choudhary, K. M., Nandal, D. P., Yadav, A. K., Poonia, T., Singh, Y., et al. (2020). Conservation agriculture-based sustainable intensification of cereal systems leads to energy conservation, higher productivity and farm profitability. Environ. Manage. 65, 774–786. doi: 10.1007/s00267-020-01273-w
Jat, R. A., Wani, S. P., and Sahrawat, K. L. (2012). Conservation agriculture in the semi-arid tropics: prospects and problems. Adv. Agron. 117, 191–273. doi: 10.1016/B978-0-12-394278-4.00004-0
Jat, R. D., Jat, H. S., Nanwal, R. K., Yadav, A. K., Bana, A., Choudhary, K. M., et al. (2018). Conservation agriculture and precision nutrient management practices in maize-wheat system: effects on crop and water productivity and economic profitability. Field Crops Res. 222, 111–120. doi: 10.1016/j.fcr.2018.03.025
Jehangir, W. A., Masih, I., Ahmed, S., Gill, M. A., Ahmad, M., Mann, R. A., et al. (2007). “Sustaining crop water productivity in rice-wheat systems of South Asia: a case study from Punjab Pakistan,” in IWMI Working Paper 115 (Colombo: International Water Management Institute).
Kakraliya, S. K., Jat, H. S., Singh, I., Gora, M. K., Kakraliya, M., Bijarniya, D., et al. (2022). Energy and economic efficiency of climate-smart agriculture practices in a rice–wheat cropping system of India. Sci. Rep. 12:8731. doi: 10.1038/s41598-022-12686-4
Karunakaran, V., and Behera, U. K. (2013). Effect of tillage, residue management and crop establishment techniques on energetics, water use efficiency and economics in soybean (Glycine max)–wheat (Triticum aestivum) cropping system. Indian J. Agron. 58, 42–47. doi: 10.59797/ija.v58i1.4165
Kulagowski, R., Thoumazeau, A., Leopold, A., Lienhard, P., Boulakia, S., Metay, A., et al. (2021). Effects of conservation agriculture maize-based cropping systems on soil health and crop performance in New Caledonia. Soil Till. Res. 212:105079. doi: 10.1016/j.still.2021.105079
Kumar, V., Kumar, M., Singh, S. K., and Jat, R. K. (2018). Impact of conservation agriculture on soil physical properties in rice-wheat system of eastern Indo-Gangetic plains. J. Anim. Plant Sci. 28, 1432–1440.
Kumar, V., Singh, S., Chhokar, R. S., Malik, R. K., Brainard, D. C., Ladha, J. K., et al. (2013). Weed management strategies to reduce herbicide use in zero-till rice–wheat cropping systems of the Indo-Gangetic Plains. Weed Technol. 27, 241–254. doi: 10.1614/WT-D-12-00069.1
Li, Y., Li, Z., Cui, S., Jagadamma, S., and Zhang, Q. (2019). Residue retention and minimum tillage improve physical environment of the soil in croplands: a global meta-analysis. Soil Tillage Res. 194:104292. doi: 10.1016/j.still.2019.06.009
Limon-Ortega, A., Sayre, K. D., and Francis, C. A. (2000). Wheat nitrogen use efficiency in a bed planting system in northwest Mexico. Agron. J. 92, 303–308. doi: 10.2134/agronj2000.922303x
Mittal, J. P., and Dhawan, K. C. (1988). Research Manual on Energy Requirements in Agricultural Sector. New Delhi: ICAR, 20–23.
Mohammad, A., Sudhishri, S., Das, T. K., Singh, M., Bhattacharyya, R., Dass, A., et al. (2018). Water balance in direct-seeded rice under conservation agriculture in north-western Indo-Gangetic plains of India. Irrig. Sci. 36, 381–393. doi: 10.1007/s00271-018-0590-z
Mondal, S., Chakraborty, D., Das, T. K., Shrivastava, M., Mishra, A. K., Bandyopadhyay, K. K., et al. (2019). Conservation agriculture had a strong impact on the sub-surface soil strength and root growth in wheat after a 7-year transition period. Soil Tillage Res. 195:104385. doi: 10.1016/j.still.2019.104385
Nath, C. P., Das, T. K., Rana, K. S., Bhattacharyya, R., Pathak, H., Paul, S., et al. (2017). Greenhouse gases emission, soil organic carbon and wheat yield as affected by tillage systems and nitrogen management practices. Arch. Agron. Soil Sci. 63, 1644–1660. doi: 10.1080/03650340.2017.1300657
Page, K. L., Dang, Y. P., and Dalal, R. C. (2020). The ability of conservation agriculture to conserve soil organic carbon and the subsequent impact on soil physical, chemical, and biological properties and yield. Front. Sustain. Food Syst. 4:31. doi: 10.3389/fsufs.2020.00031
Parihar, C. M., Jat, S. L., Singh, A. K., Kumar, B., Pradhan, S., Pooniya, V., et al. (2016). Conservation agriculture in irrigated intensive maize-based systems of north-western India: effects on crop yields, water productivity and economic profitability. Field Crops Res. 193, 104–116. doi: 10.1016/j.fcr.2016.03.013
Parihar, C. M., Jat, S. L., Singh, A. K., Kumar, B., Rathore, N. S., Jat, M. L., et al. (2018). Energy auditing of long-term conservation agriculture based irrigated intensive maize systems in semi-arid tropics of India. Energy 142, 289–302. doi: 10.1016/j.energy.2017.10.015
Parihar, C. M., Jat, S. L., Singh, A. K., Majumdar, K., Jat, M. L., Saharawat, Y. S., et al. (2017). Bio-energy, water-use efficiency and economics of maize-wheat-mungbean system under precision-conservation agriculture in semi-arid agro-ecosystem. Energy 119, 245–256. doi: 10.1016/j.energy.2016.12.068
Parihar, C. M., Meena, B. R., Nayak, H. S., Patra, K., Sena, D. R., Singh, R., et al. (2022). Co-implementation of precision nutrient management in long-term conservation agriculture-based systems: a step towards sustainable energy-water-food nexus. Energy 254:124243. doi: 10.1016/j.energy.2022.124243
Powlson, D. S., Stirling, C. M., Thierfelder, C., White, R. P., and Jat, M. L. (2016). Does conservation agriculture deliver climate change mitigation through soil carbon sequestration in tropical agro-ecosystems? Agric. Ecosyst. Environ. 220, 164–174. doi: 10.1016/j.agee.2016.01.005
Prasad, R., Gangaiah, B., and Aipe, K. C. (1999). Effect of crop residue management in a rice–wheat cropping system on growth and yield of crops and on soil fertility. Exp. Agric. 35, 427–435. doi: 10.1017/S001447979935403X
Raj, R., Das, T. K., Banerjee, T., Ghosh, A., Bhattacharyya, R., Chakraborty, D., et al. (2022). Co-implementation of conservation tillage and herbicides reduces weed and nematode infestation and enhances the productivity of direct-seeded rice in North-western Indo-Gangetic Plains. Front Sustain. Food Syst. 6:1017013. doi: 10.3389/fsufs.2022.1017013
Raj, R., Das, T. K., Chakraborty, D., Bhattacharyya, R., Babu, S., Govindasamy, P., et al. (2023). Soil physical environment and active carbon pool in rice–wheat system of South Asia: impact of long-term conservation agriculture practices. Environ. Technol. Innov. 29:102966. doi: 10.1016/j.eti.2022.102966
Raj, R., Kumar, A., Solanki, I. S., Dhar, S., Dass, A., Gupta, A. K., et al. (2017). Influence of crop establishment methods on yield, economics and water productivity of rice cultivars under upland and lowland production ecologies of Eastern Indo-Gangetic Plains. Paddy Water Environ. 15, 861–877. doi: 10.1007/s10333-017-0598-7
Riley, H., Borresen, T., Ekeberg, E., and Rydberg, T. (1994). “Trends in reduced tillage research and practice in Scandinavia,” in Conservation Tillage in Temperate Agroecosystems, ed. M. R. Carter (Boca Raton, FL: Lewis Publisher), 23–45. doi: 10.4324/9781315150529-2
Saad, A. A., Das, T. K., Rana, D. S., and Sharma, A. R. (2015). Productivity, resource-use efficiency and economics of maize (Zea mays)–wheat (Triticum aestivum)–greengram (Vigna radiata) cropping system under conservation agriculture in irrigated north-western Indo-Gangetic plains. Indian J. Agron. 60, 502–510. doi: 10.59797/ija.v60i4.4508
Saad, A. A., Das, T. K., Rana, D. S., Sharma, A. R., Bhattacharyya, R., Lal, K., et al. (2016). Energy auditing of a maize–wheat–greengram cropping system under conventional and conservation agriculture in irrigated north-western Indo-Gangetic Plains. Energy 116, 293–305. doi: 10.1016/j.energy.2016.09.115
Sayre, K. D., and Hobbs, P. R. (2004). “The raised-bed system of cultivation for irrigated production conditions,” in Sustainable Agriculture and the Rice–Wheat System, eds. R. Lal, P. Hobbs, N. Uphoff, and D. O. Hansen (Columbus: Ohio State University), 337–355. doi: 10.1201/9780203026472.ch20
Schoenau, J. J., and Campbell, C. A. (1996). Impact of crop residues on nutrient availability in conservation tillage systems. Can. J. Plant Sci. 76, 621–626. doi: 10.4141/cjps96-111
Sharma, A. R., Jat, M. L., Saharawat, Y. S., Singh, V. P., and Singh, R. (2012). Conservation agriculture for improving productivity and resource-use efficiency: prospects and research needs in Indian context. Indian J. Agron. 57(3s), 131–140.
Singh, B., Shan, Y. H., Johnson-Beebout, S. E., Singh, Y., and Buresh, R. J. (2008). Crop residue management for lowland rice-based cropping systems in Asia. Adv. Agron. 98, 117–199. doi: 10.1016/S0065-2113(08)00203-4
Singh, M. K., Pal, S. K., Thakur, R., and Verma, U. N. (1997). Energy input-output relationship of cropping systems. Indian J. Agric. Sci. 67, 262–264.
Singh, R., Ravishankar, N., Ramamurthy, V., Ansari, M. A., Raghavendra, K. J., Shamim, M., et al. (2023). Atlas of Cropping Systems in India. ICAR- Modipuram: Indian Institute of Farming Systems Research, 320.
Susha, V. S., Das, T. K., Nath, C. P., Pandey, R., Paul, S., Ghosh, S., et al. (2018). Impacts of tillage and herbicide mixture on weed interference, agronomic productivity and profitability of a maize–wheat system in the North-western Indo-Gangetic Plains. Field Crops Res. 219, 180–191. doi: 10.1016/j.fcr.2018.02.003
Swift, M. J., and Sanchez, P. A. (1984). Biological management of tropical soil fertility for sustainable productivity. Nat. Resour. 20, 1–10.
Thomas, G. A., Dalal, R. C., and Standley, J. (2007). No-till effects on organic matter, pH, cation exchange capacity and nutrient distribution in a Luvisol in the semi-arid subtropics. Soil Tillage Res. 94, 295–304. doi: 10.1016/j.still.2006.08.005
Tian, Z., Wang, J. W., Li, J., and Han, B. (2021). Designing future crops: challenges and strategies for sustainable agriculture. The Plant J. 105, 1165–1178. doi: 10.1111/tpj.15107
Xiao-Bin, W., Dian-Xiong, C. A. I., Hoogmoed, W. B., Oenema, O., and Perdok, U. D. (2006). Potential effect of conservation tillage on sustainable land use: a review of global long-term studies. Pedosphere 16, 587–595. doi: 10.1016/S1002-0160(06)60092-1
Keywords: broad-bed planting, irrigation water use, nutrients uptake, root length, tillage
Citation: Ghosh S, Das TK, Raj R, Sudhishri S, Mishra AK, Biswas DR, Bandyopadhyay KK, Ghosh S, Susha VS, Roy A, Alekhya G, Saha P and Sharma T (2025) Long-term conservation agriculture improves water-nutrient-energy nexus in maize-wheat-greengram system of South Asia. Front. Sustain. Food Syst. 9:1470188. doi: 10.3389/fsufs.2025.1470188
Received: 25 July 2024; Accepted: 17 February 2025;
Published: 14 March 2025.
Edited by:
Pankaj Kumar Arora, M. J. P. Rohilkhand University, IndiaReviewed by:
Khuong Nguyen Quoc, Can Tho University, VietnamCopyright © 2025 Ghosh, Das, Raj, Sudhishri, Mishra, Biswas, Bandyopadhyay, Ghosh, Susha, Roy, Alekhya, Saha and Sharma. This is an open-access article distributed under the terms of the Creative Commons Attribution License (CC BY). The use, distribution or reproduction in other forums is permitted, provided the original author(s) and the copyright owner(s) are credited and that the original publication in this journal is cited, in accordance with accepted academic practice. No use, distribution or reproduction is permitted which does not comply with these terms.
*Correspondence: T. K. Das, dGtkYXM2NEBnbWFpbC5jb20=; Rishi Raj, cmlzaGlyYWppYXJpQGdtYWlsLmNvbQ==
†Present address: Sonaka Ghosh, ICAR Research Complex for Eastern Region, Patna, India
Disclaimer: All claims expressed in this article are solely those of the authors and do not necessarily represent those of their affiliated organizations, or those of the publisher, the editors and the reviewers. Any product that may be evaluated in this article or claim that may be made by its manufacturer is not guaranteed or endorsed by the publisher.
Research integrity at Frontiers
Learn more about the work of our research integrity team to safeguard the quality of each article we publish.