- 1Department of Biological Sciences Ålesund, Norwegian University of Science and Technology, Ålesund, Norway
- 2Møreforsking AS, Borgundvegen, Ålesund, Norway
- 3Department of Biotechnology and Food Science, Norwegian University of Science and Technology, Trondheim, Norway
To produce fish protein hydrolysates (FPH) from fish raw material and side streams, enzymatic hydrolysis is commonly used. To optimize this traditional method of recovery and improve the physicochemical and functional parameters of recovered FPH, advanced non-thermal techniques like ultrasonication (US) can be applied as a pre-treatment step. The present study focused on evaluating the impact of US pre-treatment on the physicochemical parameters of FPH derived from mackerel side streams. Four experimental samples of FPH were produced: one control (without US pre-treatment) and three US pre-treated at power levels of 300 W, 450 W, and 600 W at 20 kHz before enzymatic hydrolysis. The findings revealed that FPHs from US-treated mackerel mince were lighter, less reddish, and contained a lower proportion of hydrophobic free amino acids ascribed to potentially lower bitterness. Moreover, FPH samples obtained from US-treated fish mince exhibited higher protein solubility and a greater degree of hydrolysis than the control (untreated). These functional improvements are attributed to the reduction in peptide particle size, leading to an increased presence of very small (<200 Da) and small peptides (500–2,000 Da) in FPH samples. Thus, it was shown that US pre-treatment of fish side streams before enzymatic hydrolysis can significantly improve protein solubility, degree of hydrolysis, and color parameters of FPH, while potentially affecting their bitterness through the reduction of hydrophobic free amino acids.
1 Introduction
As the global population is expected to approach 10 billion by 2050, finding sustainable sources of high-quality protein is crucial (Henchion et al., 2017). Oceans, covering over 70% of the Earth’s surface, have a huge potential to support the nutritional needs of the continuously growing global population by providing seafood rich in high quality protein such as fish, invertebrates, vertebrates, microalgae (Bank et al., 2022). Moreover, since the nutritional value of the side streams generated during processing of fish is comparable to that of the edible parts, their further use can enhance the value of the product and support efficient resource utilization (Álvarez et al., 2018; Hosseini et al., 2020). Fish side streams are known to contain essential nutrients such as lipids rich in polyunsaturated fatty acids (omega-3), fat-soluble vitamins, minerals and proteins containing all essential amino acids, which can act as a potential source for recovery of valuable ingredients with nutraceutical, functional and health-promoting properties (Cropotova et al., 2024; Senadheera et al., 2023). This will not only reduce the environmental load of food production but will also ensure food security while promoting circularity and sustainability across the entire food system and the environment (Henchion et al., 2017). Contrary, if left unutilized, fish side streams or by-products would create additional waste disposal challenges and environmental issues (Álvarez et al., 2018).
Atlantic mackerel (Scomber scombrus) is a pelagic fish found widely in the North Atlantic Ocean. It is valued for its meat quality rich in high value protein containing all essential amino acids, as well as minerals (selenium, iodine, calcium, magnesium, iron, and zinc), vitamins (A, D and B12) and omega-3 eicosapentaenoic (EPA) and docosahexaenoic (DHA) fatty acids (Sveinsdóttir et al., 2021). Moreover, the protein content of mackerel side streams and by-products obtained after fish processing and constituting approximately 40% of the total fish weight, is comparable to that of the fillet, ranging from 14 to 16% (Ramakrishnan et al., 2013). Thus, underutilized mackerel side streams, including trimmings, heads, fins, bones, scales, and skin, along with other discarded fish components, could serve as a valuable source of high-quality protein compounds, including small bioactive peptides and hydrolysates (Hassoun et al., 2022). It was previously shown that fish hydrolysates recovered from mackerel side streams contain small bioactive peptides with techno-functional (e.g., water-holding, emulsifying, thickening and gelling) and health promoting (e.g., anticancer, anti-inflammatory, antimicrobial, antihypertensive, and antidiabetic) properties (Cropotova et al., 2024). However, at the present time, the fishing industry mainly utilizes fish side streams to produce low-value products such as fish meal and oil (Meidell et al., 2024; Raeesi et al., 2021). To efficiently utilize fish side streams for high value applications such as food grade oil or protein hydrolysates requires safe and effective methods of extraction. In the downstream processing of fish side streams, enzymatic hydrolysis is commonly used as a conventional method of extraction (Cropotova et al., 2024). As shown in the previous studies, nearly 80% of the total protein can be recovered in the form of peptides and free amino acids from mackerel side streams, yielding in a good outcome (Ramakrishnan et al., 2013). However, innovative technological solutions can assist conventional enzymatic hydrolysis in recovery of protein ingredients with better quality characteristics from underutilized fish side streams (Álvarez et al., 2018). The most commonly applied innovative solutions include the use of advanced non-thermal technologies such as pulsed electric field (PEF), high pressure processing (HPP), ultrasound (US) treatment, and microwave-assisted extraction (MAE) before enzymatic hydrolysis to increase the yield and improve the quality of extracted compounds (Cropotova et al., 2024; Hassoun et al., 2023). These technologies have several advantages including no or minimal solvent usage, fast extraction rate, and increased yield. In addition, they have widely been recognized as environmentally friendly technologies (Al Khawli et al., 2019; Siddiqui et al., 2023).
Ultrasonication (US) technology is officially accepted for use as a treatment in the food industry and has already shown positive effects in terms of the increased yield of protein recovery from mackerel (Álvarez et al., 2018) and trout by-products (Misir and Koral, 2019) when compared to conventional methods of extraction. Moreover, according to the recent study of Cropotova et al. (2024), it was revealed that US-treatment can significantly enhance quality parameters and health properties of fish hydrolysates recovered from Atlantic mackerel side streams. Ultrasound waves generate cavitation in the liquid media, which further produces macroturbulence and high-speed interparticle collisions. This improves the permeability of the food matrix resulting in a more efficient penetration of the target compounds and the solvent into the cell the matrix, allowing the enzyme to interact better with the fish raw material and enhance the extraction (Álvarez et al., 2018). Thus, the efficiency of extraction rising significantly by increasing the mass transfer and internal diffusion mechanisms (Vilkhu et al., 2011).
This study aims to enhance traditional enzymatic hydrolysis of mackerel side streams by incorporating ultrasound pre-treatment as an emerging non-thermal technology to improve quality characteristics of the extracted fish protein hydrolysates.
2 Materials and methods
2.1 Preparation of fish raw material for hydrolysis
Fresh fish side streams (heads, backbones, fins, tails and trimmings) obtained after the filleting of Atlantic mackerel (Scomber scombrus) were collected at a local fish processing facility (Fosnavåg, Norway) on the day of processing (October 2023) and delivered to NTNU (Ålesund, Norway) in insulated containers filled with ice. The mackerel side streams were then minced using a mincer with 4.5 mm hole size (Hobart A 200 N), divided into batches of 1 kg, and immediately frozen and stored at −80°C until enzymatic hydrolysis.
2.2 Ultrasound pre-treatment
The previously prepared mackerel mince samples were subjected to ultrasound pre-treatment before enzymatic hydrolysis at the ultrasonic power of 300 W, 450 W and 600 W with a 20 kHz probe (Sonics & Materials Inc., Danbury, CT., USA, model: VCX 1500) for 10 min. The probe has a vibrating titanium tip of 1.2 cm which was immersed into the mixture of mackerel side streams and water (ratio 1:1 w/w) followed by its irradiation with an ultrasonic wave directly from the horn tip. Samples were treated for 10 min with intervals of 5 s passive (rest) and active (treatment) phase each. Every 2 min during the passive intervals of ultrasonication, the blending of mackerel mince and water was mixed to ensure the homogeneous treatment of the material by the sonication waves.
2.3 Enzymatic hydrolysis
The enzymatic hydrolysis of the experimental (US-treated) and control (untreated) mackerel samples was conducted in a 4-litre closed glass bioreactor placed in a water bath at 52°C. Warm (50 ± 2°C) distilled water was added to the control fish mince in a 1:1 w/w ratio. The US-treated experimental mackerel samples were already added water in the ration of 1:1 w/w, and the temperature of the mixture was 50 ± 2°C right after the ultrasonication. The mixtures were stirred at 150 rpm with an overhead stirrer. When the temperature of the mixtures was 50°C, Alcalase® enzyme (Sigma-Aldrich, Germany) was added in all bioreactors at the level of 0.1% (w/w) of the raw material weight. After 60 min of hydrolysis, bones were removed by filtering the hydrolysate through a sieve before the enzymes were inactivated by heating up to 90°C for 10 min in a microwave oven. The mixture was cooled down up to 30°C before being transferred to 1 L centrifugation bottles and then centrifuged at 4,100 g at 4°C for 30 min. The liquid fractions (lipids and water-soluble proteins) were separated from the insoluble fraction (sludge) in the following way. The liquid fraction was placed in a separatory funnel and allowed to settle, and then separated into oil and water-soluble proteins. The water-soluble protein phase representing fish protein hydrolysate (FPH) was collected and dried in the laboratory vacuum freeze-dryer (Labconco Freezone Console 12 L Freeze Dry System).
2.4 Proximate composition analysis
The content of nitrogen (N) in the minced mackerel raw material and the obtained FPH were determined using a Vario-El-Cube CHNS Elemental Analyzer (Elementar, GmbH, Germany). Approximately 4 mg of dried samples were weighed out in tin capsules and oxidized at 1,150°C. The amount of protein in the samples was estimated using a nitrogen-to-protein conversion factor determined for fish raw material of 6,25 (ISO, 2009). Water content was determined gravimetrically after drying at 105°C for 24 h. Ash content was determined by incineration to constant weight at 550°C (AOAC, 2000). Lipid content in mackerel mince was determined by the Bligh & Dyer (B&D) method (Bligh and Dyer, 1959) using a binary mixture of chloroform and methanol diluted with distilled water as an extraction medium, as described by Cropotova et al. (2020). Lipid content in FPH was calculated mathematically due to a very low amount of fat in the samples through the deduction of total protein, ash and water content from 100.
2.5 Protein solubility of mackerel FPH
To determine protein solubility in FPH recovered from mackerel side streams, the extracts were prepared by dissolving 0.1 g of each FPH sample in 10 mL of distilled water. The solutions were homogenized and centrifuged. Water-soluble proteins were determined in triplicates by using the Lowry method (Lowry et al., 1951). Bovine serum albumin (BSA) was used to prepare a standard curve. The absorbance of the incubated standards and samples was determined using a SpectraMax ix3 microplate reader (Molecular Devices, USA) at a wavelength of 750 nm. The analyses were run in triplicate and the mean value ±SD was calculated.
Protein solubility was calculated from the following Equation 1:
2.6 Molecular weight distribution of FPH
Freeze-dried FPH was diluted with Milli-Q (MQ) water to a concentration of 10 mg/mL. Then, 100 μL of the diluted FPH solution was further diluted with 900 μL of 10% acetonitrile in MQ water in an HPLC vial. Analysis was performed on an AQUITY UPLC H-Class PLUS System (Waters Corporation, Milford, MA, USA) with an AQUITY BEH125 SEC 1.7u 4.6 mm × 150 mm column (Waters) and an AQUITY UPLC PDA Detector (Waters Corporation, Milford, MA, USA) set to 220 nm. Runs were isocratic, and a 100 mM phosphate buffer (pH 6.8) was used as the mobile phase with 0.5 mL/min of flow rate, an injection volume of 2 μL, and a total run time of 15 min. The column temperature was set to 30°C for analysis. Bovine serum albumin (66,000 Da), cytochrome C (12,327 Da), aprotinin (6,512 Da), insulin A (2,531 Da), Leu-enkephalin (555.6 Da), Met-enkepalin (573.7 Da) Val-Tyr-Val (379.5 Da), and Gly-Tyr (238.2 Da) were used as standards. All were purchased from Merck. Chromatograms were manually integrated and separated into intervals of <0.2, 0.2–0.5, 0.5–1, 1–2, 2–5, and > 5 kDa, expressed as percentages of the total area. All samples were analysed in triplicate.
2.7 Degree of hydrolysis
The degree of hydrolysis (DH) was analysed by formol titration as the proportion (%) of free amino groups with regard to the total nitrogen in the sample previously determined by the Kjeldahl method (ISO, 2009). A FPH sample of 1.5 g was weighed into a beaker and filled up to 50 g with distilled water. The pH was adjusted to 7.0 using 0.1 M NaOH and then 10 mL of 9% w/w formaldehyde with a pH of 8.5 was added. The beaker was covered with aluminium foil and stirred for 5 min. For the titration, a TITROLINE 7000 automatic titrator (SI Analytics, Xylem Analytics Germany Sales GmbH & Co. KG, Germany), was used. The titrator was rinsed 3 times before starting the titration. Furthermore, the titration was set to pH 8.5 with stopping automatically when reaching a pH of 8.5. The samples were titrated with 0.1 M NaOH and the used amount of NaOH was recorded. Degree of hydrolysis was further determined as described by Cropotova et al. (2024).
2.8 Total amino acid profile
About 50 mg of freeze-dried FPH was weighed into glass tubes, 1 mL 6 M HCl was added. The glass tubes placed into a heating cupboard for 24 h, at 105°C. Samples were diluted 50 times using distilled water before filtering through 0.22 μm.
For the derivatization, 200 μL of the sample were transferred to a glass tube, containing 600 μL 0.4 M borate buffer (pH 9). 400 μL FMOC (9-fluorenylmethoxycarbonyl chloride, 15 mM in acetonitrile) was added, vortexed for 1 min, and then allowed to stand at room temperature for 4 min. After amino acid derivatization with FMOC, 400 μL ADAM (60 mM in acetonitrile:water 2:1) was added.
Amino acids were analyzed using a Shimadzu Nexera XR HPLC system, equipped with a PDA detector (Shimadzu, USA). Separation of amino acids were carried out on a Restec ARC-18 column (10 mm x 2.1 mm) at 30°C. The mobile phase was 0.1% formic acid with 20 mM ammonium formate and 0.1% formic acid with 10 mM ammonium formate in 90:10 acetonitrile water in gradient mode, with a flowrate of 0.8 mL/min.
2.9 Free amino acid profile
Water extracts were made by adding 0.1 g of FPH in 10 mL of distilled water. One mL of water extract was mixed with 0.25 mL of sulfosalicylic acid (10%) and incubated at 4°C for 30 min. The samples were centrifuged at 10000G for 10 min. For the derivatization, 300 μL of the sample were transferred to a glass tube, containing 400 μL 0.4 M borate buffer (pH 9). 100 μL FMOC (9-fluorenylmethoxycarbonyl chloride, 15 mM in acetonitrile) was added, mixed on a vortex for 1 min, and then allowed to stand at room temperature for 4 min. After amino acid derivatization with FMOC, 300 μL ADAM (60 mM in acetonitrile:water 2:1) was added. Free amino acids were analyzed using a Shimadzu Nexera XR HPLC system, as described in chapter 2.8.
2.10 Thiol groups
Total thiol groups were determined according to Ellman (1959) and Kvangarsnes et al. (2023). To 0.1 mL of the water-soluble extract or distilled water (blank), 0.8 mL of 8 M urea and 0.1 mL of DTNB were added. Samples were mixed, incubated at room temperature for 30 min, and centrifuged for 3 min at 11000 g at room temperature. The absorbance was measured spectrophotometrically with Shimadzu UV-1800 UV/visible scanning spectrophotometer (Shimadzu Europa GmbH, Germany) at 412 nm with the blank as reference. The thiol content was calculated using a molar extinction coefficient of 14,290 M−1 cm−1. The results are expressed as nmol/mg protein.
2.11 Colour measurements
Colour parameters of the FPH obtained from mackerel side streams were determined using a Minolta Chromometer Model CR 400 (Konica Minolta, Japan) calibrated on a white reference plate before use. L* (lightness), a* (redness) and b* (yellowness) were measured on the protein hydrolysates in triplicate at a room temperature. The L*, a* and b* parameters of the CIELAB scale were measured according to the lab scale established by CIE (2001), and the average with standard deviation were calculated.
2.12 Statistical analysis
All results were expressed as the mean ± standard deviation (s.d.), where p-values <0,05 were considered to be significant. Statistical analyses were performed by one-way ANOVA followed by Tukey’s post-test (Statgraphics, USA).
3 Results and discussion
3.1 Proximate composition
The proximate composition of mackerel raw material (side stream mince) and FPH obtained from it, is displayed in Table 1.
According to Table 1, the ultrasound pre-treatment of mackerel raw material at the ultrasonic power of 300 W yielded FPH with significantly (p < 0,05) higher total protein content compared to control (untreated) sample. However, the US pre-treatment of mackerel mince at 450 W resulted in FPH with significantly lower protein content compared to the control. In addition, there was no significant difference in total protein content between the control and the FPH sample obtained after US pre-treatment at the highest ultrasonic power of 600 W. This phenomenon can be explained by the fact that at lower ultrasonic powers the US-treatment can improve the enzyme activity by disrupting molecular aggregates, which facilitates the enzyme’s access to the substrate (Ma et al., 2011) and increase the efficiency of enzymatic hydrolysis. However, higher ultrasonic powers of 450 W and 600 W could lead to a stronger cavitation effect, increasing the emulsification of proteins and yielding FPHs with lower protein contents compared to control sample. This hypothesis is supported by the fact that the mackerel side streams used as fish raw material for the hydrolysis were high in lipids (21,1%) and therefore more emulsified by the proteins (data not shown) released after the US-induced rupture of mackerel mince at higher ultrasonic powers. Taking into account the high lipid content in FPH samples US-treated at 450 W and 600 W compared to 300 W, we suggest that proteins were emulsified by the increased amount of phospholipids in sludge after the sonication (data not shown). This is confirmed by the fact that FPH obtained after ultrasonication of fish mince at 450 W and 600 W had higher lipid content that FPH after US-treatment at 300 W (Table 1). Low lipid content is a desired quality for protein hydrolysates to assure high oxidative stability during storage. Therefore, FPH obtained after US pre-treatment of mackerel mince at 300 W before enzymatic hydrolysis has shown best results in terms of lower lipid and higher protein content compared both to control and other US-treated samples. Regarding water content, all experimental FPH samples obtained after ultrasonication of mackerel mince, had significantly higher water content than control sample. However, there was no significant difference in ash content between the control and the experimental FPH samples obtained after US pre-treatment.
3.2 Protein solubility of mackerel FPH
The analyzed water-soluble protein content in the mackerel side stream mince before enzymatic hydrolysis was 6,61 ± 0,01%. After the enzymatic hydrolysis, fish proteins were broken down into FPH made up of water-soluble peptides and amino acids (Nguyen et al., 2022). In the food industry, the solubility of protein ingredients, including FPH, is one of the most important techno-functional parameters influencing their acceptability by both consumers and food professionals (Misir and Koral, 2019). According to the results shown in Figure 1, protein solubility increased in all FPH after US-treatment compared to control, however this increase was not significant (p < 0,05). This phenomenon can be explained by a better access of the enzyme to cutoff sites in mackerel raw material after the cavitation effect of ultrasonication. This process led to the enhanced enzymatic hydrolysis and generation of bigger number of small peptides with increased solubility, which is supported by a significant increase in degree of hydrolysis for the samples 300 W and 450 W (Figure 2) and molecular weight of small peptides (Figure 3) compared to control sample (Misir and Koral, 2019). At the same time, there were not significant changes in protein solubility between the FPH samples after US-treatment at 300 W and 600 W and the control sample.
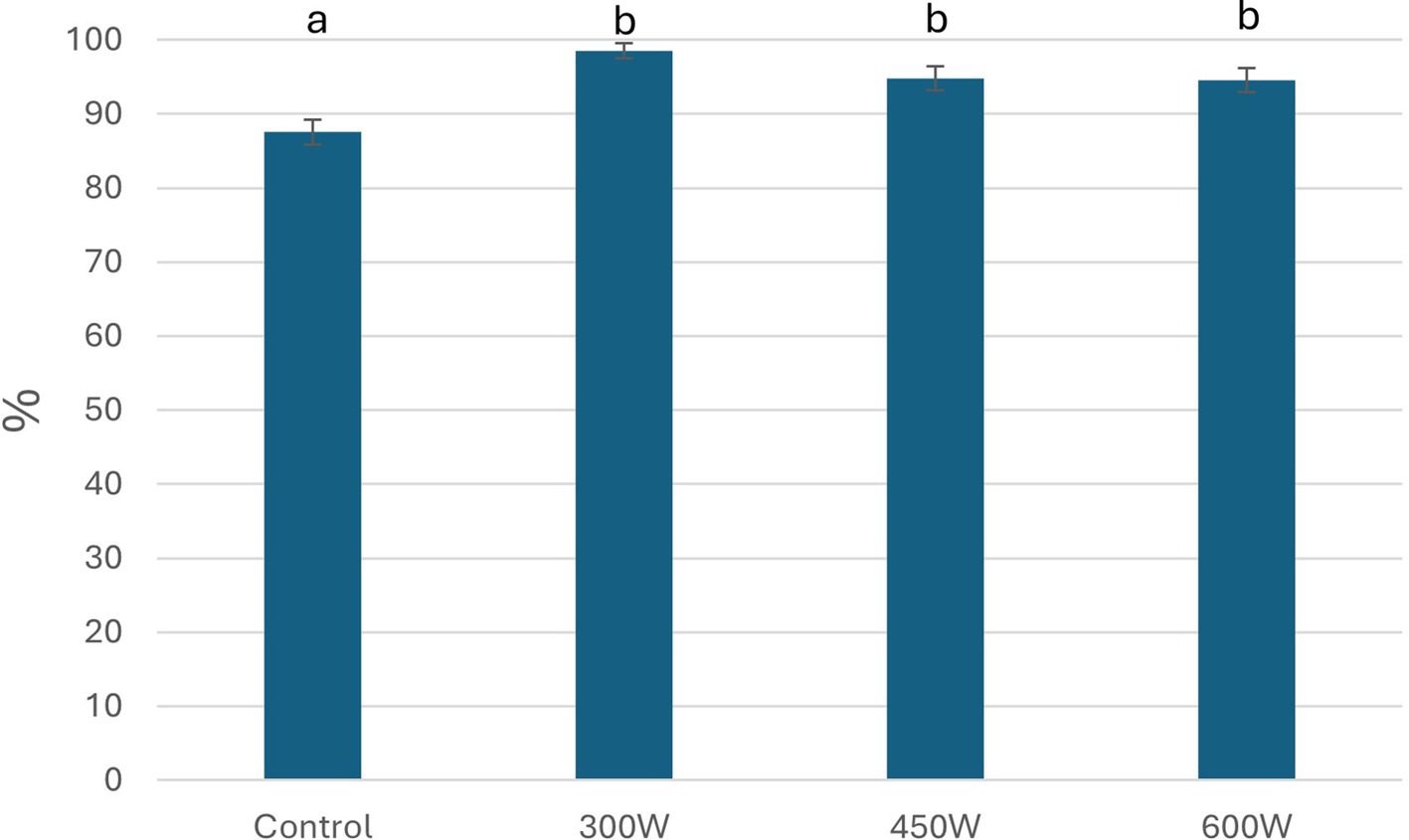
Figure 1. Protein solubility of FPH, %. Mean value and standard deviation are shown. Different letters indicate significant differences (p < 0.05).
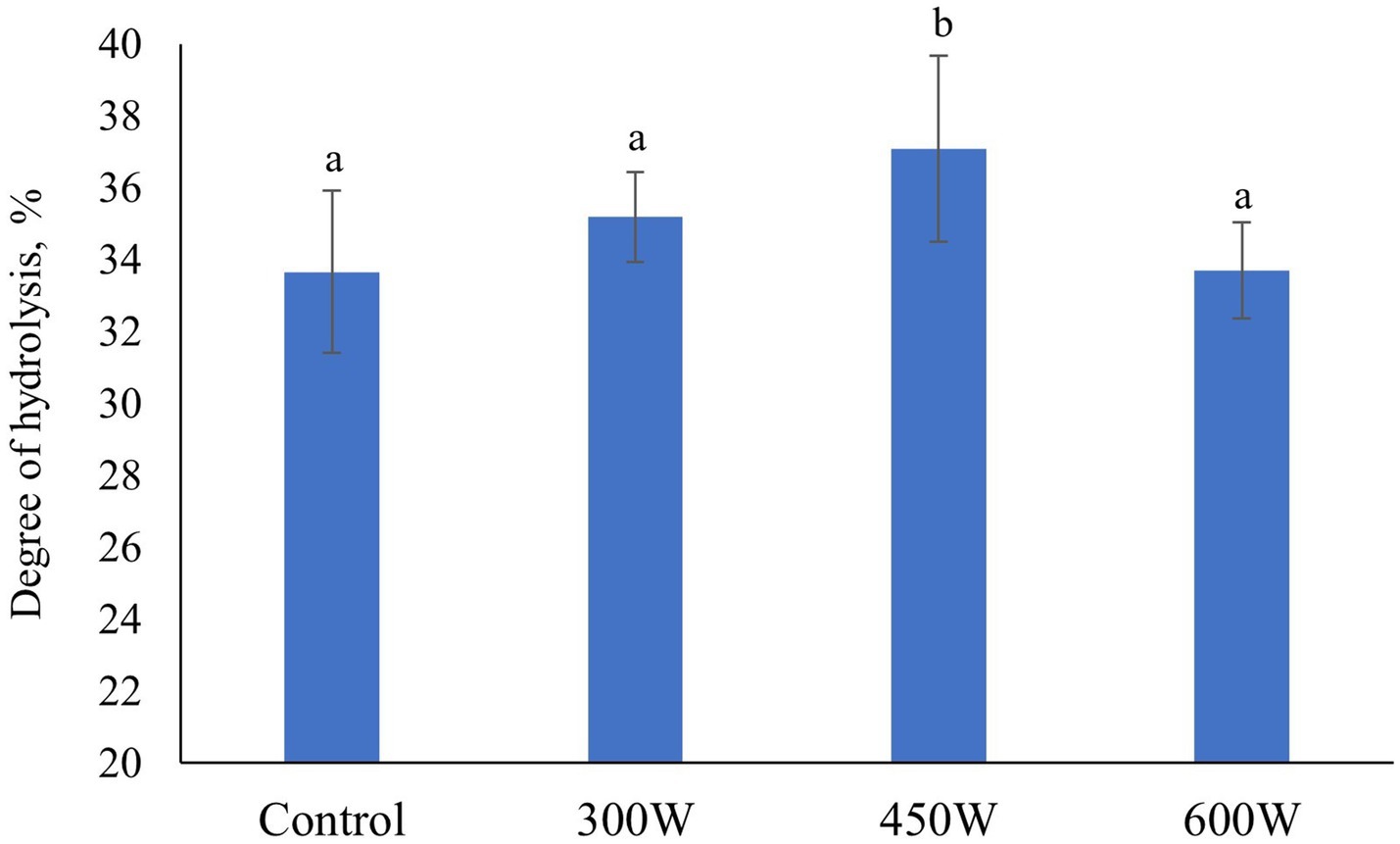
Figure 2. Degree of hydrolysis in herring FPH. Mean value and standard deviation are shown. Different letters indicate significant differences (p < 0.05).
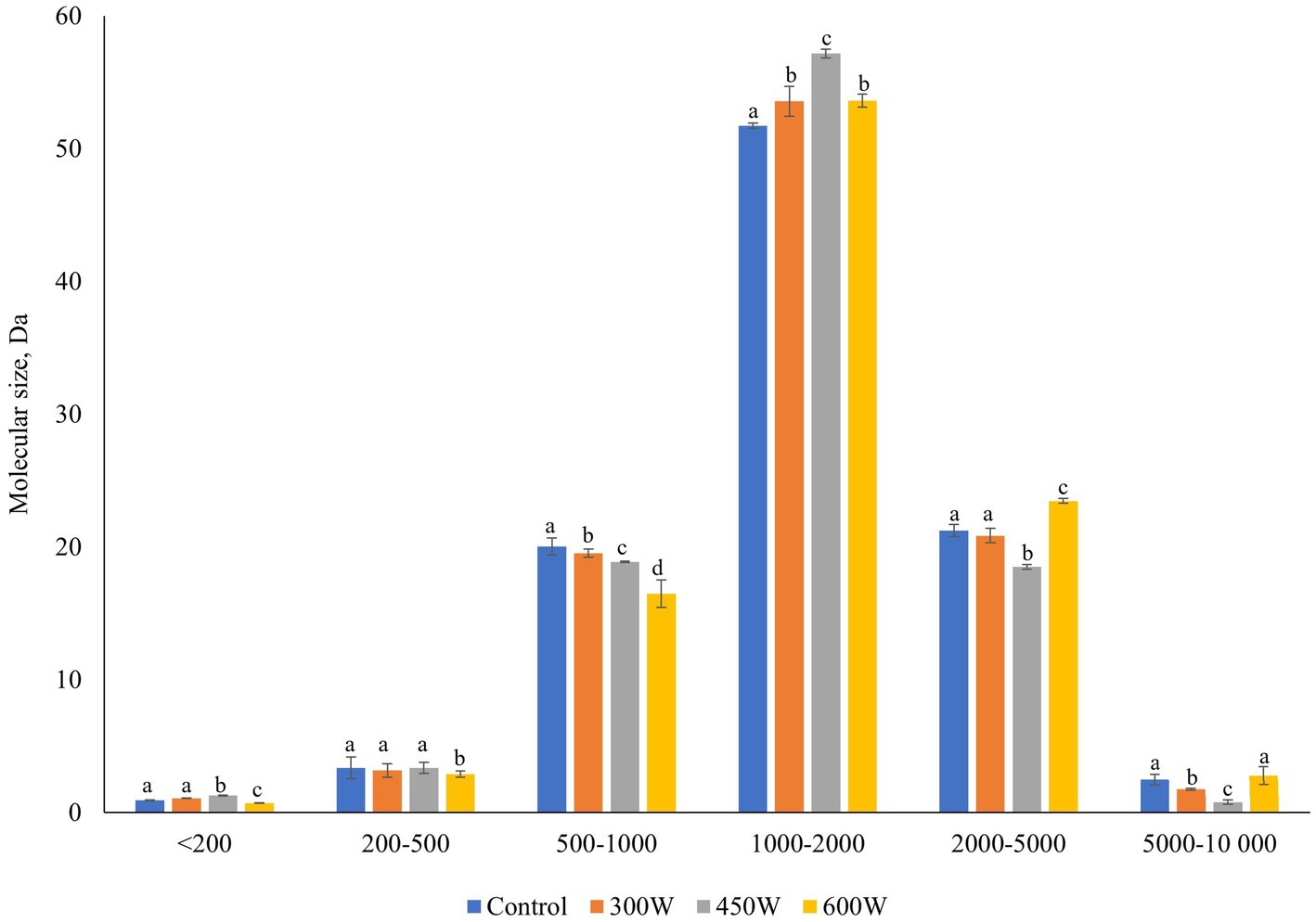
Figure 3. Molecular weight distribution in herring FPH. Mean value and standard deviation are shown. Different letters indicate significant differences (p < 0.05).
3.3 Degree of hydrolysis
Ultrasonication of mackerel raw material before enzymatic hydrolysis increased the degree of hydrolysis for all experimental samples of FPHs compared to control, however these changes were significant only for FPH sample treated at 450 W (Figure 2). As in the case of protein solubility, it was first a gradual increase in the degree of hydrolysis for the samples treated at 300 W and 450 W, followed by a drop in the degree of hydrolysis for FPH after ultrasonication at 600 W. This phenomenon can be explained by the cavitation effect of ultrasonication leading to protein unfolding which creates favorable cutoff sites for the enzyme to act during enzymatic hydrolysis (Ma et al., 2011) at lower ultrasonic powers of 300 W and 450 W. However, at the high ultrasonic power of 600 W, the combined cavitation and mechanical oscillation effect led to aggregation and denaturation of protein molecules altering both the substrate and the enzyme characteristics (Huang et al., 2017) thus reducing the degree of hydrolysis (Umego et al., 2021). In addition, a significant correlation between protein solubility and degree of hydrolysis between all FPH samples (p < 0,05; R2 = 0,84), was observed.
3.4 Molecular weight distribution (MWD)
The MWD of FPHs (Figure 3) has shown that ultrasonic pre-treatment of mackerel raw material before enzymatic hydrolysis resulted in a significant (p < 0,05) gradual linear decrease in the number of small peptides with particle size of 500–1,000 Da in experimental samples of FPHs compared to control. In addition, the amount of medium-size peptides with the MW of 1,000–2,000 Da increased significantly (p < 0,05) in all FPHs after US-treatment of mackerel mince compared to control, and the increase was linear for 300 W and 450 W. Similar trend of increase was observed for very small peptides with MWD < 200 Da. Our previous investigation studying the effect of US-treatment of mackerel FPHs on MWD distribution (Cropotova et al., 2024) displayed similar variation in MWD of very small (< 200 Da) and small peptides with particle size of 500–1,000 Da. In that study, it was shown that ultrasonication can significantly modify the particle size of mackerel peptide molecules through US-promoted denaturation and rearrangement of peptide chains by breaking hydrogen bonds (Cropotova et al., 2024). Thus, we suggest that the US-induced changes in the MWD distribution in FPH were caused by the partial denaturation of bigger peptides and disruption of hydrophobic interactions between the molecules. This phenomenon led to a reduction in particle size of FPHs and an increase in the amount of small and very small peptides which, as it was shown in our previous study (Cropotova et al., 2024), may possess strong bioactive and health-promoting activities. It is well known that the protein solubility is increasing together with an increase in the proportion of small peptides in protein hydrolysates (Režek et al., 2014). Moreover, in the previous studies it was shown that ultrasonication can increase protein solubility of FPH by ultrasound-induced denaturation effect affecting secondary and tertiary structure of peptides and generating more small peptides with increased solubility (Cropotova et al., 2024; Tian et al., 2020). However, in the present study, no significant difference in soluble proteins was observed for US-treated FPH samples compared to control (Figure 1), even though the proportion of small peptides with MWD 500–1,000 Da decreased significantly compared to control.
3.5 Total amino acid profile
The total amino acid profile of mackerel FPHs is displayed in Table 2.
According to the results displayed in Table 2, the proportion of essential amino acids increased in FPHs obtained from mackerel mince subjected to US pre-treatment at the ultrasonic power of 300 W and 450 W compared to control. However, there was no significant difference in the proportion of essential amino acids between the control sample and FPH sample obtained after ultrasonic pre-treatment of fish mince at 600 W. In terms of non-essential amino acids, ultrasonic pre-treatment of mackerel raw material before enzymatic hydrolysis increased significantly (p < 0,05) the proportion of Glutamic acid, Alanine, Cystine, while significantly reducing (p < 0,05) the proportion of Arginine, Glycine, Proline in all experimental FPH samples compared to control. The most predominant amino acid in all FPHs including the control sample was Glycine. We hypothesize that this phenomenon is attributed to the high content of fish skin, fins and tails in the mackerel side streams used as raw material in the study. Fish skin is rich in collagen containing high proportions of Glycine. The highest amount of this amino acid was found in the control sample of FPH (23,69%). It was shown that ultrasound pre-treatment of mackerel mince reduced significantly the proportion of this amino acid in all experimental FPHs compared to control (Table 2). This phenomenon can be explained by the nature of this amino acid. Glycine is a hydrophobic amino acid and according to Rajapakse et al. (2005) is highly soluble in lipids. Thus, during ultrasonication of mackerel mince due to a strong cavitation effect, it could get easier in touch with lipid radicals compared to neutral and hydrophilic amino acids. Similar results were obtained in our previous study on US post-treatment of mackerel hydrolysates (Cropotova et al., 2024).
There is a lot of scientific evidence showing the effect of hydrophobic/hydrophilic amino acid residues on protein solubility (Hou et al., 2018). However, in the present study, no correlation between the hydrophobic/hydrophilic amino acid profile and protein solubility was found.
3.6 Free amino acid profile
One of the major obstacles in the production and use of FPH is bitter taste generated during enzymatic hydrolysis, which is mainly ascribed to free hydrophobic amino acids and small hydrophobic peptides (Remme et al., 2023). Therefore, it is crucial to reduce bitterness for the successful utilization of FPH as a food ingredient.
The free amino acid profile displayed in Table 3 was analysed in the present study to evaluate the potential bitterness of FPH samples obtained from mackerel side streams subjected to ultrasound pre-treatment.
The free amino acids in the four FPH samples differed with the use of ultrasound. The free amino acids content is known to vary due to duration and degree of hydrolysis, as well as type and concentration of enzyme used (Novikov et al., 2018). In addition, the ultrasonication can generate heat, and change the conformation and structure of the protein. It is most likely that the liberation of free amino acids will be affected in different ways in our samples, and thereby the differences in free amino acid distribution (Qian et al., 2023). Histidine, Serine and Lysine has shown antioxidative effects (He et al., 2013), and our results showed that the FPHs obtained after US-treatment of fish raw material at 450 W had a significantly higher content of Histidine and Serine, and a significant lower content of Lysine compared to the other samples. The proportion of hydrophobic free amino acids such as Phenylalanine, Methionine, Valine, Leucine, Isoleucine, and Proline determine the perception of bitterness (Pérez-Santaescolástica et al., 2019). Thus, changes in the concentration of these amino acids in FPHs can significantly affect their bitter taste. In the present study, the use of ultrasonic pre-treatment of mackerel mince before enzymatic hydrolysis resulted in FPHs with significantly lower proportion of free amino acids compared to control sample (Table 3). Moreover, a gradual linear decrease in the proportion of free amino acids in FPHs was found along with an increase in ultrasonic power from 0 W (control) to 600 W (p < 0,05; R2 = 0,96). Thus, it was revealed that US-treatment of fish raw material prior to enzymatic hydrolysis can significantly reduce bitterness of FPHs. However, no sensory analysis was conducted in this study to support this statement.
3.7 Thiol groups
Compared to control (11.05 nmol/mg protein), all samples that were pre-treated with ultrasound had significant lower number of thiol groups, which indicate protein oxidation. The sample treated with ultrasound with a power of 450 W showed a thiol content of 9.6 nmol/mg protein. The lowest levels of thiols were found in the samples treated with ultrasound with a power of 300 W and 600 W with a thiol content of 7.8 and 7.2 nmol/mg protein respectively, and there were no significant differences between these two samples (Figure 4).
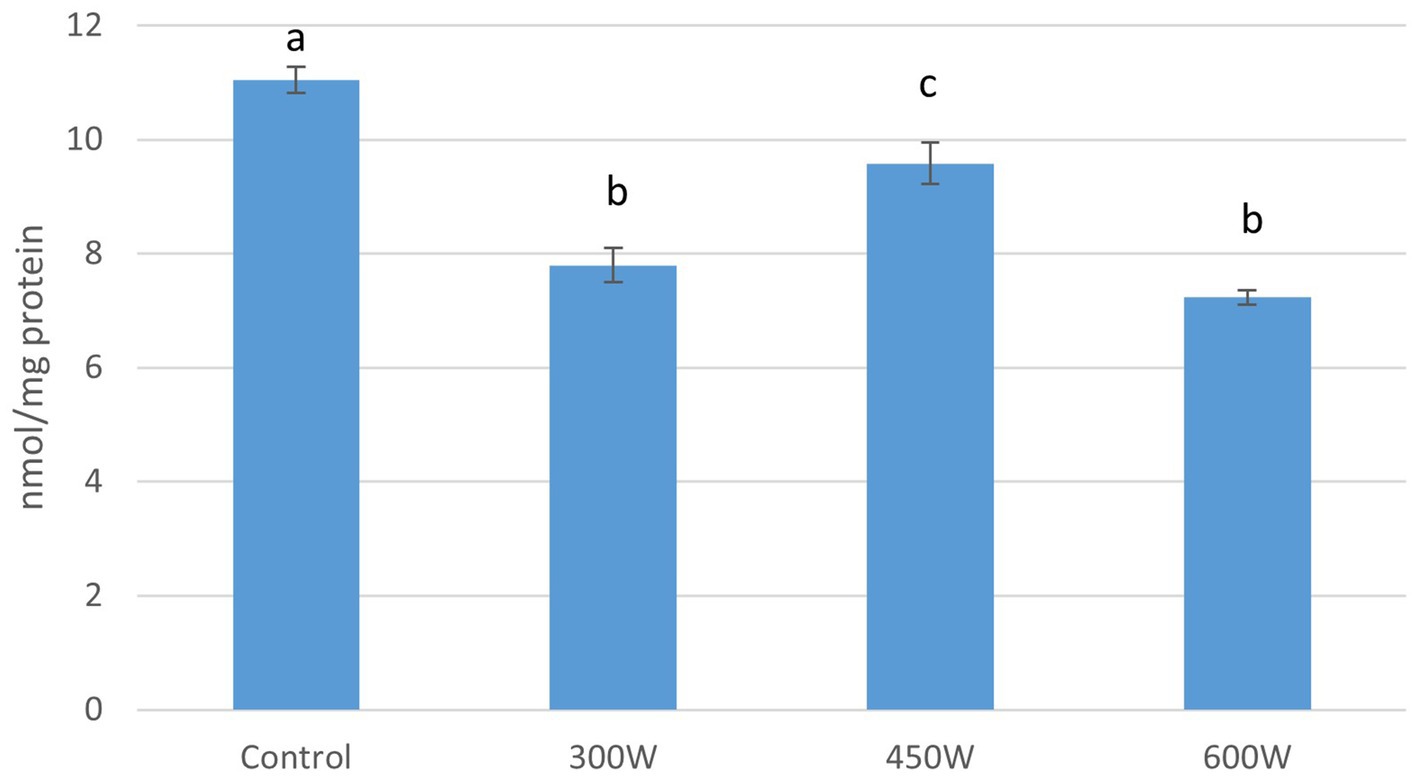
Figure 4. Thiol groups in FPH, nmol/mg protein. Mean value and standard deviation are shown Different letters indicates significant differences (p < 0.05).
Cysteine and methionine are amino acids containing thiol groups, and the loss in thiol groups in FPH can be reflected by the amino acid composition. When cysteine is oxidized, it can form cystine which consist of two molecules of cysteine bound by a disulfide bound (Anastassakis, 2022). Our results showed that the relative amount of cystine increased significantly by the use of ultrasound pre-treatment at 300 W. There were also observed an increase with ultrasound power of 450 W and 600 W, but this increase was not significant compared to control group. Regarding methionine, the relative amount decreased with power of ultrasound, with significant differences between 300 W, 450 W and 600 W. This reflects the proteins oxidation showed by the thiol groups with the use of ultrasound pre-treatment.
3.8 Color parameters
Color is one of the most important sensory parameters influencing consumer acceptability and commercialization of protein ingredients, including FPHs (Makri et al., 2021). It was previously shown that lighter FPHs are generally associated with freshness and thus are more preferred by consumers because are considered of higher quality compared to darker FPHs (Nguyen et al., 2022). The color parameters of mackerel FPHs are displayed in Table 4.
According to the results of color parameters, all experimental FPHs obtained after ultrasonication of mackerel mince before enzymatic hydrolysis, had significantly (p < 0.05) higher L* and significantly lower a*-values compared to control. Thus, the obtained results show that FPHs became much lighter and less reddish after ultrasonication of fish mince. This can be also seen in Figure 5 illustrating experimental samples of mackerel FPH. The significant decrease in redness is probably due to ultrasonic denaturation of myoglobin and blood-derived hemoglobin molecules in mackerel mince (Sajib et al., 2021), while increase in lightness is attributed to the changes in secondary structure of protein molecules due to cavitation effect of ultrasound treatment. Thus, the absorption peaks of light may shift to higher wavelengths, resulting in lightness increase of FPHs (Fadimu et al., 2021), which can positively influence consumer perception of the product.
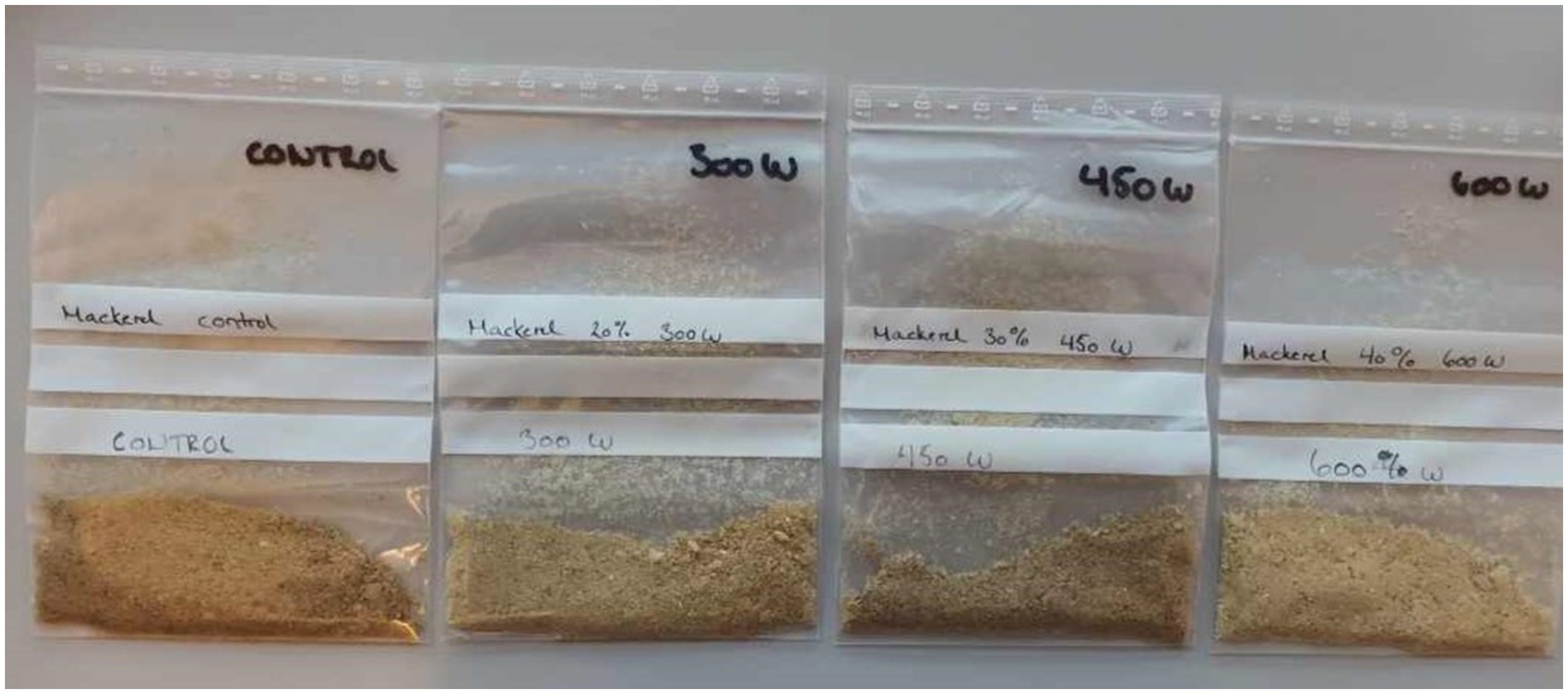
Figure 5. Illustration of FPH obtained from mackerel side streams with and without (control) ultrasound pre-treatments.
4 Conclusion
In conclusion, the study has demonstrated that ultrasonication pre-treatment may significantly enhance the physicochemical, functional and structural parameters of fish protein hydrolysates derived from mackerel side streams.
US pre-treatment at varying power levels (300 W, 450 W, and 600 W) before enzymatic hydrolysis resulted in FPH with improved physicochemical properties, including lighter color, reduced redness, and potentially lower bitterness due to a decrease in hydrophobic free amino acid proportion. Additionally, US-treated FPH exhibited slightly but insignificantly higher degree of hydrolysis and significantly higher protein solubility, attributed to the reduction in peptide particle size and the increased presence of very small (<200 Da) peptides. The FPH sample obtained after ultrasonication of fish raw material at 450 W had the best functional, nutritional and structural parameters. Thus, this sample had significantly higher protein solubility and degree of hydrolysis, together with significantly higher lightness and lower redness, as well as greater proportion of essential amino acids compared to control. In addition, the proportion of hydrophobic free amino acids was significantly lower in the sample 450 W compared to control.
These findings suggest that US pre-treatment of fish side streams at the ultrasonic power of 450 W before enzymatic hydrolysis is a promising method to produce fish protein hydrolysates with improved physicochemical, functional, nutritional and structural parameters compared to conventionally used method of recovery.
Data availability statement
The raw data supporting the conclusions of this article will be made available by the authors, without undue reservation.
Author contributions
JC: Conceptualization, Funding acquisition, Investigation, Methodology, Project administration, Resources, Supervision, Validation, Writing – original draft, Writing – review & editing. KK: Data curation, Investigation, Methodology, Writing – original draft. JS: Data curation, Investigation, Methodology, Writing – original draft. TR: Investigation, Methodology, Writing – review & editing.
Funding
The author(s) declare that financial support was received for the research, authorship, and/or publication of this article. The authors acknowledge the ERA-NET BlueBio Consortium for the support in funding the project “IMPRESSIVE” (grant No. 341732 of NRC).
Acknowledgments
The authors from Norway would like to acknowledge the local fish processing facility in Fosnavåg (Norway) for providing Atlantic mackerel for the research.
Conflict of interest
The authors declare that the research was conducted in the absence of any commercial or financial relationships that could be construed as a potential conflict of interest.
Publisher’s note
All claims expressed in this article are solely those of the authors and do not necessarily represent those of their affiliated organizations, or those of the publisher, the editors and the reviewers. Any product that may be evaluated in this article, or claim that may be made by its manufacturer, is not guaranteed or endorsed by the publisher.
References
Al Khawli, F., Ferrer, E., Berrada, H., Barba, F. J., Pateiro, M., Domínguez, R., et al. (2019). Innovative green Technologies of Intensification for valorization of seafood and their by-products. Mar. Drugs 17:689. doi: 10.3390/md17120689
Álvarez, C., Lélu, P., Lynch, S. A., and Tiwari, B. K. (2018). Optimised protein recovery from mackerel whole fish by using sequential acid/alkaline isoelectric solubilization precipitation (ISP) extraction assisted by ultrasound. LWT 88, 210–216. doi: 10.1016/j.lwt.2017.09.045
Anastassakis, K. (2022). “Cysteine and Cystine” in Androgenetic alopecia from a to Z. Eds. G. Zevelekakis (Cham: Springer).
AOAC (2000). “Official methods of analysis: official method for ash” in Method no. 936.03; Association of Official Analytical Chemists (Washington, DC: AOAC).
Bank, M. S., Duarte, C. M., and Sonne, C. (2022). Intergovernmental panel on blue foods in support of sustainable development and nutritional security. Environ. Sci. Technol. 56, 5302–5305. doi: 10.1021/acs.est.2c00119
Bligh, E. G., and Dyer, W. J. (1959). A rapid method of total lipid extraction and purification. Can. J. Biochem. Physiol. 37, 911–917. doi: 10.1139/o59-099
CIE (2001). Commission Internationale de l’Eclairage improvement to Industrial Color-Difference Evaluation. Wien: CIE.
Cropotova, J., Kvangarsnes, K., Rustad, T., Stangeland, J., Roda, G., Fanzaga, M., et al. (2024). Effect of ultrasound treatment on quality parameters and health promoting activity of fish protein hydrolysate (FPH) extracted from side streams of Atlantic mackerel (Scomber scombrus). Front. Nutr. 11:1446485. doi: 10.3389/fnut.2024.1446485
Cropotova, J., Mozuraityte, R., Standal, I. B., Ojha, S., Rustad, T., and Tiwari, B. (2020). Influence of high-pressure processing on quality attributes of haddock and mackerel minces during frozen storage, and fishcakes prepared thereof. Innovative Food Sci. Emerg. Technol. 59:102236. doi: 10.1016/j.ifset.2019.102236
Ellman, G. L. (1959). Tissue sulfhydryl groups. Arch. Biochem. Biophys. 82, 70–77. doi: 10.1016/0003-9861(59)90090-6
Fadimu, G. J., Gill, H., Farahnaky, A., and Truong, T. (2021). Investigating the impact of ultrasound pretreatment on the physicochemical, structural, and antioxidant properties of Lupin protein hydrolysates. Food Bioprocess Technol. 14, 2004–2019. doi: 10.1007/s11947-021-02700-4
Hassoun, A., Cropotova, J., Trollman, H., Jagtap, S., Garcia-Garcia, G., Parra-López, C., et al. (2023). Use of industry 4.0 technologies to reduce and valorize seafood waste and by-products: a narrative review on current knowledge. Current Res. Food Science 6:505. doi: 10.1016/j.crfs.2023.100505
Hassoun, A., Rustad, T., and Bekhit, A. (2022). Bioconversion of marine by-products into edible protein. Alternat. Proteins 31, 302–324.
He, T., Spelbrink, R. E., Witteman, B., and Giuseppin, M. L. (2013). Digestion kinetics of potato protein isolates in vitro and in vivo. Int J Food Sci Nutr. 64, 787–793. doi: 10.3109/09637486.2013.793300
Henchion, M., Hayes, M., Mullen, A. M., Fenelon, M., and Tiwari, B. (2017). Future protein supply and demand: strategies and factors influencing a sustainable equilibrium. Food Secur. 6:53. doi: 10.3390/foods6070053
Hosseini, S. F., Rezaei, M., and McClements, D. J. (2020). Bioactive functional ingredients from aquatic origin: a review of recent progress in marine-derived nutraceuticals. Crit. Rev. Food Sci. Nutr. 62, 1242–1269. doi: 10.1080/10408398.2020.1839855
Hou, Q., Bourgeas, R., Pucci, F., and Rooman, M. (2018). Computational analysis of the amino acid interactions that promote or decrease protein solubility. Sci. Rep. 8:14661. doi: 10.1038/s41598-018-32988-w
Huang, G., Chen, S., Dai, C., Sun, L., Sun, W., Tang, Y., et al. (2017). Effects of ultrasound on microbial growth and enzyme activity. Ultrason. Sonochem. 37, 144–149. doi: 10.1016/j.ultsonch.2016.12.018
ISO (2009). Determination of nitrogen content and calculation of crude protein content. Geneva: International Organization for Standardization.
Kvangarsnes, K., Dauksas, E., Tolstorebrov, I., Rustad, T., Bartolomei, M., Xu, R., et al. (2023). Physicochemical and functional properties of rainbow trout (Oncorhynchus mykiss) hydrolysate. Heliyon 9:e17979. doi: 10.1016/j.heliyon.2023.e17979
Lowry, O. H., Rosebrough, N. J., Lewis, F. A., and Randall, R. J. (1951). Protein measurement with the Folin phenol reagent. J. Biol. Chem. 193, 265–275. doi: 10.1016/S0021-9258(19)52451-6
Ma, H., Huang, L., Jia, J., He, R., Luo, L., and Zhu, W. (2011). Effect of energy-gathered ultrasound on Alcalase. Ultrason. Sonochem. 18, 419–424. doi: 10.1016/j.ultsonch.2010.07.014
Makri, V., Feidantsis, K., Papadopoulos, D., Lattos, A., Georgoulis, I., Michaelidis, B., et al. (2021). Natural-like pigmentation in cultured fish stocks, not only a matter of nutrition. A review of Salmonidae and Sparidae families, with a particular focus on the red porgy Pagrus pagrus. Aquac. Res. 52, 2942–2953. doi: 10.1111/are.15156
Meidell, L. S., Slizyte, R., Mozuraityte, R., Carvajal, A. K., Rustad, T., and Falch, E. (2024). Valorization of Saithe (Pollachius virens) residuals into protein hydrolysates—Silaging as preservation technology. Food Secur. 13:2133. doi: 10.3390/foods13132133
Misir, G. B., and Koral, S. (2019). Effects of ultrasound treatment on structural, chemical and functional properties of protein hydrolysate of rainbow trout (Oncorhynchus mykiss) by-products. Italian J. Food Sci. 31, 205–223. doi: 10.14674/IJFS-1218
Nguyen, H. T., Bao, H. N. D., Dang, H. T. T., Tómasson, T., Arason, S., and Gudjónsdóttir, M. (2022). Protein characteristics and bioactivity of fish protein hydrolysates from Tra catfish (Pangasius hypophthalmus) side stream isolates. Food Secur. 11:4102. doi: 10.3390/foods11244102
Novikov, V. Y., Derkach, S. R., Kuchina, Y. A., Shironina, A. Y., and Mukhin, V. A. (2018). Kinetics of enzymatic reactions in the production of fish protein hydrolysates. J. Dispers. Sci. Technol. 39, 1454–1461. doi: 10.1080/01932691.2017.1414612
Pérez-Santaescolástica, C., Carballo, J., Fulladosa, E., Munekata, P., Campagnol, P. B., Gómez, B., et al. (2019). Influence of high-pressure processing at different temperatures on free amino acid and volatile compound profiles of dry-cured ham. Food Res. Int. 116, 49–56. doi: 10.1016/j.foodres.2018.12.039
Qian, J., Chen, D., Zhang, Y., Gao, X., Xu, L., Guan, G., et al. (2023). Ultrasound-assisted enzymatic protein hydrolysis in food processing: mechanism and parameters. Food Secur. 12:4027. doi: 10.3390/foods12214027
Raeesi, R., Shabanpour, B., and Pourashouri, P. (2021). Quality evaluation of produced silage and extracted oil from rainbow trout (Oncorhynchus mykiss) wastes using acidic and fermentation methods. Waste Biomass Valoriz. 12, 4931–4942. doi: 10.1007/s12649-020-01331-8
Rajapakse, N., Mendis, E., Jung, W.-K., Je, J.-Y., and Kim, S.-K. (2005). Purification of a radical scavenging peptide from fermented mussel sauce and its antioxidant properties. Food Res. Int. 38, 175–182. doi: 10.1016/j.foodres.2004.10.002
Ramakrishnan, V. V., Ghaly, A. E., Brooks, M. S., and Budge, S. M. (2013). Extraction of proteins from mackerel fish processing waste using Alcalase enzyme. J. Bioprocess. Biotechniq. 3, 2–9. doi: 10.4172/2155-9821.1000130
Remme, J. F., Korsnes, S., Steen, S., Durand, R., Kvangarsnes, K., and Stangeland, J. (2023). The effects of enzymes, species, and storage of raw material on physicochemical properties of protein hydrolysates from whitefish heads. Mar. Drugs 21:587. doi: 10.3390/md21110587
Režek, J. A., Mason, T. J., Lelas, V., Paniwnyk, L., and Herceg, Z. (2014). Effect of ultrasound treatment on particle size and molecular weight of whey proteins. J. Food Eng. 121, 15–23. doi: 10.1016/j.jfoodeng.2013.08.012
Sajib, M., Wu, H., Fristedt, R., and Undeland, I. (2021). Hemoglobin-mediated lipid oxidation of herring filleting co-products during ensilaging and its inhibition by pre-incubation in antioxidant solutions. Sci. Rep. 11:19492. doi: 10.1038/s41598-021-98997-4
Senadheera, T. R. L., Hossain, A., and Shahidi, F. (2023). Marine bioactives and their application in the food industry: a review. Appl. Sci. 13:12088. doi: 10.3390/app132112088
Siddiqui, S. A., Schulte, H., Pleissner, D., Schönfelder, S., Kvangarsnes, K., Dauksas, E., et al. (2023). Transformation of seafood side-streams and residuals into valuable products. Food Secur. 12:422. doi: 10.3390/foods12020422
Sveinsdóttir, H. I., Sverrisdóttir, S. B., Karlsdóttir, M. G., Rustad, T., Arason, S., and Gudjónsdóttir, M. (2021). Effects of anatomical variation of muscle on composition and oxidation susceptibility of Atlantic mackerel (Scomber scombrus). LWT 146:111431. doi: 10.1016/j.lwt.2021.111431
Tian, R., Feng, J., Huang, G., Tian, B., Zhang, Y., Jiang, L., et al. (2020). Ultrasound driven conformational and physicochemical changes of soy protein hydrolysates. Ultrason. Sonochem. 68:105202. doi: 10.1016/j.ultsonch.2020.105202
Umego, E. C., He, R., Ren, W., Xu, H., and Ma, H. (2021). Ultrasonic-assisted Enzymolysis: principle and applications. Process Biochem. 100, 59–68. doi: 10.1016/j.procbio.2020.09.033
Keywords: Atlantic mackerel, enzymatic hydrolysis, ultrasound treatment, fish protein hydrolysates, quality characteristics
Citation: Cropotova J, Kvangarsnes K, Stangeland J and Rustad T (2024) Effect of ultrasound pre-treatment prior to enzymatic hydrolysis on physicochemical parameters of fish protein hydrolysates (FPH) extracted from side streams of Atlantic mackerel (Scomber scombrus). Front. Sustain. Food Syst. 8:1500359. doi: 10.3389/fsufs.2024.1500359
Edited by:
Roberto Anedda, Porto Conte Ricerche, Parco Scientifico e Tecnologico della Sardegna, ItalyReviewed by:
Ioannis A. Giantsis, Aristotle University of Thessaloniki, GreeceJen-Yi Huang, Purdue University, United States
Copyright © 2024 Cropotova, Kvangarsnes, Stangeland and Rustad. This is an open-access article distributed under the terms of the Creative Commons Attribution License (CC BY). The use, distribution or reproduction in other forums is permitted, provided the original author(s) and the copyright owner(s) are credited and that the original publication in this journal is cited, in accordance with accepted academic practice. No use, distribution or reproduction is permitted which does not comply with these terms.
*Correspondence: Janna Cropotova, amFubmEuY3JvcG90b3ZhQG50bnUubm8=