- 1G.B. Pant National Institute of Himalayan Environment, Kosi-Katarmal, Pantnagar, Uttarakhand, India
- 2Department of Vegetable Science, G.B. Pant University of Agriculture and Technology, Pantnagar, Uttarakhand, India
Climate change, coupled with the rising human population and increased demand for food, pose significant challenges for achieving the sustainable development goals (SDGs) of zero hunger, no poverty, and good health and well-being. These intertwined challenges demand urgent action to identify and promote un-popularized, underutilized, and unexplored climate-smart crops that can ensure food and nutritional security. The present study aimed to investigate the nutraceutical potential of Glycine soja, a wild ancestor of cultivated soybean that has been used traditionally as food and medicine in Indian Himalayas. Seed samples of wild and cultivated soybeans were collected from different locations in Uttarakhand, India, and screened for their phytochemical and biochemical contents using standard methods. The results of the study revealed that wild soybean contains a significant quantity of total phenols (27.44 ± 0.836 mg/g dw), flavonoids (3.319 ± 0.305 mg QE/g dw), and antioxidant activity (3.56 ± 1.090 mg AAE/g dw). Additionally, the species is a rich source of minerals such as zinc (4.68 ± 0.101 mg/100 g dw), vitamins, and amino acids such as histidine (0.95 ± 0.071 mg/100 g dw), isoleucine (1.74 ± 0.108 mg/100 g dw), leucine (2.94 ± 0.044 mg/100 g dw), lysine (2.13 ± 0.082 mg/100 g dw), methionine (0.53 ± 0.044 mg/100 g dw), threonine (0.67 ± 0.196 mg/100 g dw) etc. than the cultivated soybean. This study is the first to comprehensively compare phytochemicals, antioxidants, amino acids, vitamins, anthocyanins, and mineral content of wild and cultivated soybean seed quality traits. From the results of the present study, the inclusion of wild soybean in the cultivation system and daily diets might help to achieve livelihood, food and nutritional security.
1 Introduction
Soybean is the major commercial grain crop consumed worldwide due to its high protein and oil content and remains a vital component of global agriculture. The global market value of soybeans accounts for around USD 117 billion from 2020 to 2021 (Nuthalapati et al., 2024). Its center of origin is China, while the USA, Brazil, China, Argentina, and India are the major soybean producers globally (Pagano and Miransari, 2016). India is the fifth largest producer of soybean at the global level, contributing significantly to the world soybean market with an average national yield of around 1 t/ha. The crop accounted for 37% of the country’s total oilseed area from 2010 to 2012 (Sharma, 2016; Prashnani et al., 2024). In India, it is cultivated in many states including Uttarakhand, Himachal Pradesh, Madhya Pradesh, eastern Bengal, Maharashtra, Karnataka, Rajasthan, Chhattisgarh and Andra Pradesh (Agarwal et al., 2013). In Uttarakhand, it is a major Kharif crop cultivated entirely under rainfed conditions in an area of approximately 13.5 thousand hectares with a total production of 17.40 thousand tons (Bhartiya et al., 2017).
In Uttarakhand, two types of soybeans are cultivated and consumed: yellow soybean (Glycine max) and black soybean (Glycine max and Glycine soja). Although, both wild and black cultivated soybeans have black colored seeds but phenotypically they differ in terms of seed size, plant growth habit, leaf shape and pods (Saitoh et al., 2004; Chen and Nelson, 2004; Kofsky et al., 2018) (Figures 1, 2). In hilly areas of Uttarakhand, the total land area under yellow soybean cultivation is 8.12 thousand hectares, with a total production of 12.19 quintals per hectare (Qt/Ha). In comparison, the area under black soybean cultivation is 7.96 thousand hectares with a production of 10.51 Qt/ha, which is generally intermixed with wild soybeans (Department of Agriculture Uttarakhand, 2020). Yellow soybean is well known for its high oil content and extensive application in the food industry (Singh and Krishnaswamy, 2022). In Asia, soybeans have been consumed since antiquity in various forms such as tofu, soy milk, natto, tempeh, soy sauce, and miso (Chen et al., 2012). Additionally, it is a rich source of isoflavones, oxalate, phytic acid, and bioactive peptides, which all play beneficial roles in the human body, such as in the prevention of cardiovascular diseases, osteoporosis, prostate cancer as well as beneficial in reducing the aching symptoms experienced by postmenopausal women and exhibits antimutagenic, antioxidant, antimicrobial, and antineoplastic effects (Chen et al., 2012). Nevertheless, there is a high consumer preference for wild soybean compared to cultivated soybean (black and yellow soybeans) in hilly areas of the Himalayan states of India, especially Himachal Pradesh and Uttarakhand. G. soja is the wild ancestor of cultivated soybeans (G. max) that command higher market prices due to its limited cultivation area and higher preference over the cultivated soybean. Black soybean (wild and cultivated) is locally known as Bhat, Bhatmash, and kala Bhat in Garhwal and Kumaon regions of Uttarakhand west Himalaya and is an integral part of various traditional cuisines of Himalayan communities (Ojha et al., 2022; Kala and Nautiyal, 2023). Additionally, wild soybean is used in the treatment of fever, jaundice, insomnia, cold, headaches, chest problems and a potent source of anticancer compounds (Amaani and Dwira, 2018). However, there is still a vast gap between its demand and productivity compared to the cultivated black soybean, and limited research is available on the nutritional quality traits.
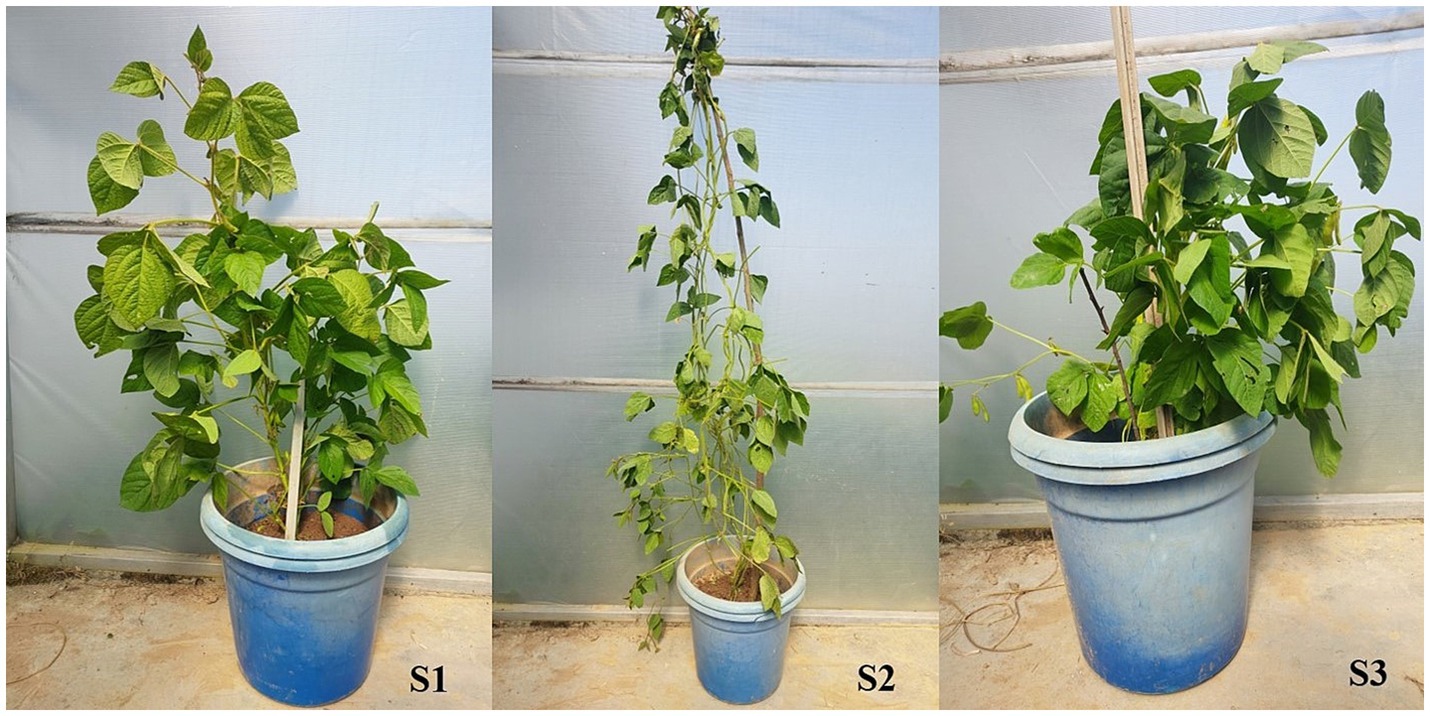
Figure 1. Illustration of phenotypic difference between wild and cultivated soybean. S1 – Black cultivated soybean, S2 – Wild soybean, S3 – Yellow cultivated soybean.
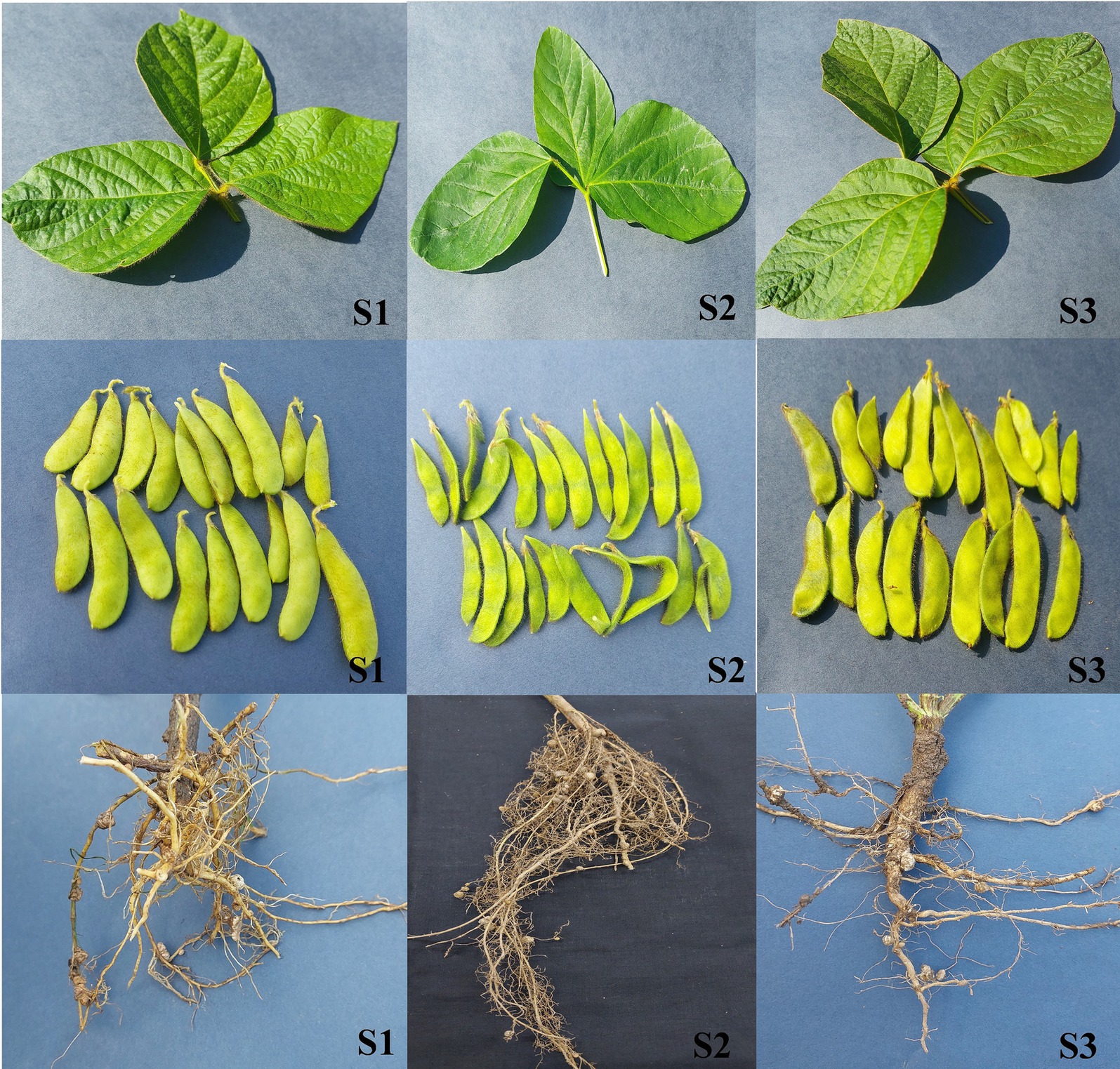
Figure 2. Phenotypic differences of different plant parts (leaves, pods and root nodules) of wild (S2) and cultivated soybean (S1 and S3). S1 – Black cultivated soybean, S2 – Wild soybean, S3 – Yellow cultivated soybean.
The rising human population, increased food demand, and continuous trends in climate change are putting stress on the global yield of domesticated crops, posing significant challenges to sustain global food security. Therefore, incorporating climate-resilient wild crop relatives into the agricultural system might be a promising strategy to ensure food security. The present study aims to assess and compare the phytochemicals and biochemicals of wild and cultivated soybeans to popularize the nutraceutical-rich traits of underutilized wild soybeans.
2 Materials and methods
2.1 Sample collection
500 g of seeds of Glycine max [Black (S1) and yellow (S3)] and Glycine soja (S2) were collected from different locations across Uttarakhand, West Himalaya, India (Figures 3, 4). The collected seeds were brought to the Rural Technology Complex of GB Pant National Institute of Himalayan Environment (NIHE), Kosi-Katarmal, Almora, situated in the Agro-climatic zone (NARP) Zone-1 with latitude: 29°37′46.9”N, longitude: 79°37′36.3″E, 1160 msL. The experimental site is characterized as maximum temperature ranges between 28 and 29.9°C and the minimum between 11.4 and 20°C with average rainfall of 197.41 mm. Collected genotypes were grown in the same location in the month of May with similar agronomic practices to reduce the effect of the environment on genotypes. Matured seeds were harvested in the second week of October and evaluated for various quality traits. Each seed sample was ground with a mixture grinder (Usha International LTD, India) and sieved to a particle size of 1 mm, which was then used for further analyses. All the chemicals and reagents used in the experimental work were of analytical grade and were brought from HiMedia, Laboratories, Pvt. Ltd., India.
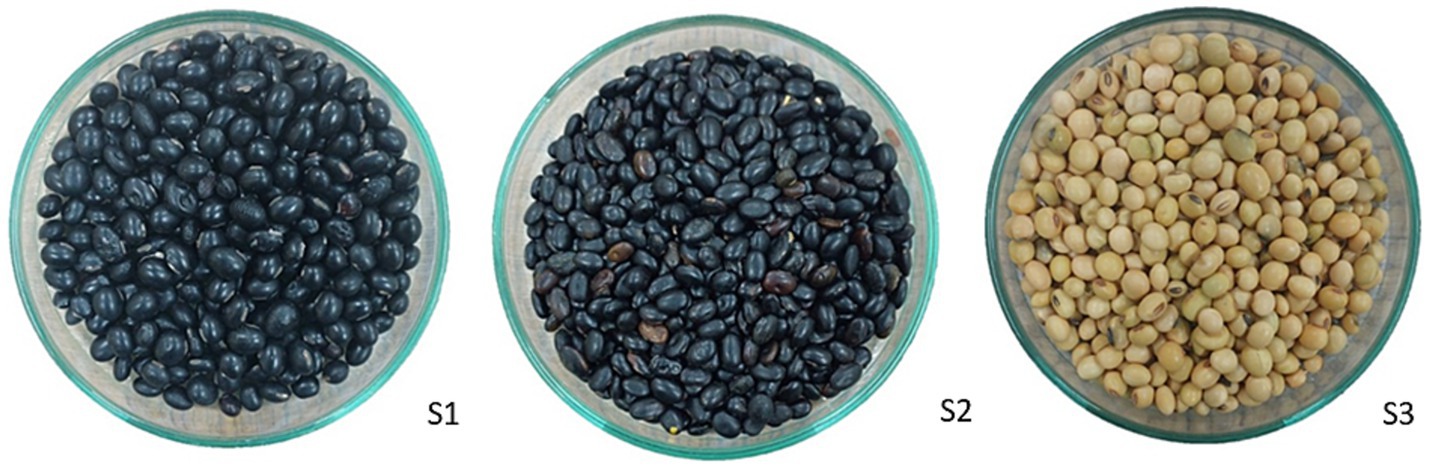
Figure 3. Soybean genotypes. S1 – Black cultivated soybean, S2 – Wild soybean, S3 – Yellow cultivated soybean.
2.2 Proximate analysis
Total ash and fat content were estimated using the Association of Official Analytical Chemists (AOAC) method following Abdulkadir et al. (2016). method. The ash content was calculated by incinerating 2 g of sample in pre-weighed crucibles in a muffle furnace (Macro Scientific Works, India) at 550°C for 5 h. The difference in weight to the initial sample weight was regarded as total ash content. Total fat content was estimated using the Soxhlet method by extracting 2 g of each sample in petroleum ether for 5 h. Carbohydrate and protein content were estimated using a UV–vis spectrophotometer (Hitachi U-2001, Tokyo, Japan) following the Anthrone method by Das et al. (2023) with slight modifications and by Lowry method using the Folin’s reagent (Waterborg, 2009) respectively.
2.3 ICP-MS analysis for macro and micro-nutrients
Sample preparation, digestion and analytical determination was done following the method of Kılıç Altun et al. (2017) with slight modifications. One (1 g) of each sample was weighed and transferred to digestion tubes, followed by adding a digestion mixture (2.5 mL HNO3 and 1 mL H2O2). The solution was digested in a closed microwave system (Multiwave Anton Par, 5,000 series) following the procedure: 25°C to 140°C in 15 min then hold for 5 min; 140 to 160°C in 10 min hold for 35 min; then cool to room temperature. After digestion, the solutions were diluted with ultrapure water to 50 mL and mixed. The blank was also prepared likewise. Standard stock solutions (Calcium, Iron, Magnesium, Phosphorus, Potassium, Sodium, and Zinc) were purchased from Merck Pvt. Ltd. India. Identification and quantification of the minerals were carried out by ICP-MS (Agilent, USA based, 7,000 series).
2.4 Estimation of vitamins
The stock standard solutions of vitamins were prepared by dissolving 10 mg of each standard vitamin purchased from Agilent, weighed, and transferred to a 10 mL volumetric flask. Dissolved and diluted to volume with water as mobile phase (pH adjusted to 2.1 with H2SO4). Thereafter, 5.0 g of well-grinded sample was soaked in 20 mL of water (pH 2.1) and kept in the dark for 24 h. The filtrate was then filtered through Whatman No. 1 filter paper, and the volume was adjusted using HPLC grade water. The sample solution was again filtered through a 0.45 μm syringe membrane filter and filled into an auto-sampler vial. After that, the analysis was performed on the HPLC 1100 series Agilent with a DAD detector following the method of Seal (2016). The mobile phase contains water (pH 2.1), the column (300SB-C84.6 × 250 mm, 5 μm pore size) was thermostatically controlled at 25°C, and the injection volume was kept at 20 μL. The total analysis time per sample was kept at 10 min. The flow rate was 1.0 mL/min. HPLC chromatograms of all vitamins were detected using an array UV detector at 210 nm.
2.5 Amino acid profile
Powdered sample (1 g) was extracted via microwave-assisted digestion (Multiwave Anton Par, 5,000 series) in 8 mL nitric acid and 1 mL hydrochloric acid. Then, the samples were diluted with 50% acetonitrile in 1:4 (v/v), and centrifuged at 6,000 RPM for 10 min. The extracts were filtered and dried, followed by the addition of derivatization reagents 0-phthalaldehyde (OPA) and 9-fluorenylmethyl chloroformate (FMOC) to identify total free amino acids. Each derivatization was conducted at 40°C for 10 min and filtered through a 0.45 μm filter before analysis. Amino acid identification was done by Agilent P/N 5061–3,339 high-performance liquid chromatography (Agilent Technologies, USA) following the Agilent method (Mengerink et al., 2002). The separation was performed using gradient elution. Mobile phase A contains 500 mL 20 mM sodium acetate with 2 mg EDTA and 0.018% triethylamine (v/v), and pH adjusted to 7.2 using acetic acid and 0.3% tetrahydrofuran. Mobile phase 2 comprised of 100 mL 20 mM sodium acetate adjusted to pH 7.2 with acetic acid, 200 mL methanol, and 200 mL acetonitrile. A constant flow rate was set at 0.45 mL/min, and the column temperature was set at 40°C. The fluorescence excitation and emission wavelength were set at 338 nm and 390 nm, respectively.
2.6 Determination of phytochemical, anthocyanin, and antioxidant activity
2.6.1 Sample preparation
Powdered sample (2 g each) was extracted in 30 mL of 80% methanol (MeOH) and kept in a conical flask covered with parafilm tape. Samples were macerated in the rotary shaker (Remi, India) at 160 rpm for 24 h. Filtration was done with Whatman filter paper 1 then the filtrates were dried, weighed, diluted with 30 mL 80% MeOH, and stored (15 days) at 4°C until analysis.
2.6.2 Phytochemicals determination
Extracts were used to estimate total phenolics, flavonoids, tannin, and total monomeric anthocyanin following standard methods (Hatami et al., 2014; Lee et al., 2005; Nwinuka et al., 2005; Quettier-Deleu et al., 2000) specifically. All the quantitative estimations were carried out using a UV–Vis spectrophotometer (Hitachi U-2001, Tokyo, Japan) at respective wavelengths according to the target compounds. Results were expressed in milligram equivalent per gram dry weight (mg equivalent/g dw).
2.6.3 Antioxidant activity by ferric reducing antioxidant power assay
The antioxidant activity was analyzed using Benzie and Strain (1996) method. The sample extract (0.1 mL) was mixed with 1.8 mL 2,4,6-Tripyridyl-S-triazine solution (10 mM/L acetate buffer: 10 Mm/L TPTZ: 20 Mm FeCl3 in the ratio of 10:1:1). The mixture was kept at 35°C for 10 min. The absorbance of the resultant mixture was measured at 593 nm using a UV–vis spectrophotometer (Hitachi U-2001, Tokyo, Japan). The results were expressed in milligram ascorbic acid equivalent (AAE) per gram extract dry weight (mg AAE/g dw).
2.7 Statistical analysis
All the parameters were performed in triplicate, and data are expressed as the mean ± standard deviation (SD). Differences within parameters (p < 0.05) were analyzed by the Duncan multiple range test (DMRT) using Statistical Package for the Social Sciences (SPSS) version 29.0.2.0 (20). The principal component analysis (PCA) and heat map analyses were also conducted to correlate phytochemicals, antioxidants, vitamins, minerals, and proximate activity performed in origin software (Origin 2023, Origin Lab, USA).
3 Results and discussion
3.1 Proximate composition
A well-balanced diet containing adequate amount of all the essential nutrients such as protein, carbohydrates, fat, vitamins and minerals are very essential for proper growth, development, maintenance, and regulation of certain metabolic function in the body (Chen et al., 2018). The proximate composition of soybean samples is shown in Table 1. There was a significant difference (p < 0.05) between the protein content of soybean genotypes. For instance, the highest protein content was recorded in wild soybean (40.98%) followed by black cultivated soybean (37.85%). The highest ash (5.27%) and fat content (22.20%) were observed in yellow cultivated soybean. According to a study by Ren et al. (2012), the ash (4.99%) and fat content (17.86%) was reported highest in yellow cultivated soybean compared to black cultivated soybean having 4.37% fat and 16.64% ash. Similarly, all the samples showed significant differences in carbohydrate content. The highest carbohydrate content was observed in black cultivated soybean (21.75%), followed by wild soybean (20.49%). Etiosa et al. (2017) reported 37.69% protein, 16.31% carbohydrate, and 4.08% ash in soybean seeds. Kim et al. (2023) reported 39.17 to 52.27% protein and 4.74 to 12.68% oil in wild soybean. The protein content in the present investigation was in the range of the reported results (Kim et al., 2023). However, the fat content in the present study was higher than the reported one, and this difference might be due to the difference in extraction solvent, climate, and the other agronomic conditions followed for the genotype’s cultivation. Legumes are mainly consumed because of their richness in protein content and from the results of the present investigation, wild soybean is an excellent source of protein compared to the other soybean genotypes thus, consumption of wild soybean might play an important role in growth, development of muscle mass, maintaining overall health and also in eradication of malnutrition.
3.2 Macro and micronutrients
Nutrients play a vital role in maintaining the biological structure, regulating metabolic reactions, and properly functioning the immune system (Munteanu and Schwartz, 2022). Soybean genotypes are rich sources of certain minerals, and among them, the highest zinc content was observed in wild soybean (4.68 mg/100 g). The presence of this trace element is effective in treating several chronic diseases such as diabetes, Alzheimer’s disease, Wilson’s disease, and also helps in boosting immunity (Chasapis et al., 2012). The highest magnesium (Mg) (273.00 mg/100 g) and phosphorus (P) (802.00 mg/100 g) content was reported in black cultivated soybean followed by wild soybean. Although black cultivated soybean is a rich source of these minerals, wild soybean also contains greater Mg and P when compared to yellow cultivated soybean. The significant presence of P in black soybeans helps to maintain bone health and Mg aids in DNA synthesis and repair (Hartwig, 2001; Takeda et al., 2012; Takeda et al., 2012). The highest Calcium (301.12 mg/100 g), iron (15.92 mg/100 g dw), and potassium (1785.67 mg/100 g) content was observed in yellow cultivated soybean (Table 1). Whereas, the highest sodium (2.98 mg/100 g) content was observed in black cultivated soybean. It was observed that potassium and phosphorus have statistically strong significant differences amongst the soybean genotypes. The sequence of minerals concentration in soybean genotypes is as follows: potassium>phosphorus>magnesium>calcium>iron>zinc>sodium. Rani et al. (2008) investigated the mineral content in nine varieties of soybean and reported the calcium content ranged from 230.2 to 255.2 mg/100 g, phosphorus in the range 496.1–514.2 mg/100 g, iron content (8.40–11.20 mg/100 g) and zinc content ranged from 7.16 to 7.89 mg/100 g. The results reported in the present study are consistent with the previously reported values, and the mineral content was notably higher than the previously reported values. Very limited data was available on the mineral content estimation in wild soybean. However, an earlier study from 1984 reported higher zinc content in wild soybean(59–83 mg/g) compared to cultivated soybean(38–67 mg/kg) (Raboy et al., 1984). These slight variations in mineral content values are attributable to several factors, including genetic factors, biotic and abiotic influences, genotype, maturity, growing season, geographic location, and agronomic practices (Bellaloui et al., 2015).
3.3 Vitamin content in soybean genotypes
Dietary vitamins are essential for human health, normal growth, maintenance, and development (Maqbool et al., 2017). The vitamin content in soybeans is present in very trace quantities (Vineet Kumar et al., 2010). Vitamins such as thiamine, riboflavin, niacin, folate, and pyridoxine were estimated for all three soybean genotypes (Table 2). No significant difference was observed between black cultivated soybean and wild soybean in terms of thiamine and niacin content. Similarly, the level of riboflavin does not show any significant difference between black and yellow cultivated soybean, and pyridoxine showed no significant difference among the soybean genotypes. In summary, there was no significant difference (p > 0.05) observed for vitamins among all the soybean genotypes except for folate. The highest folate (368.00 μg/100 g) content was observed in yellow cultivated soybean followed by wild soybean (356.00 μg/100 g). These vitamins were present in the following order of concentration in soybean genotypes niacin>thiamine>riboflavin>pyridoxine>folate. According to the Food and Nutrition Board, Institute of Medicine the recommended dietary allowance and adequate intake of vitamins for different age groups is presented in Table 2 (NIH, 2024). Based on the recommended dietary allowance, all the soybean genotypes apparently contain an appropriate amount of vitamins necessary for all the age groups. Soybean genotypes contain a significant amount of folate and its consumption is essential for biochemical functions such as DNA synthesis, repair and maintenance (Warzyszynska and Kim, 2014). Humans fail to synthesize folate; thus, it should be taken through dietary sources (Donnelly, 2001). Similarly, niacin is very effective in maintaining cardiovascular health; riboflavin exhibits anticancer, anti-inflammatory, antioxidant, anti-aging, and anti-nociceptive properties; thiamine acts as an important coenzyme for protein, carbohydrates, and pyridoxine acts as a strong anti-inflammatory agent (MacKay et al., 2012; Suwannasom et al., 2020; Mikkelsen et al., 2023; Mrowicka et al., 2023). This is the first study to perform the comparative vitamin estimation among wild and cultivated soybean genotypes.
3.4 Amino acids in soybean genotypes
Amino acids are the building blocks of protein structure and well known to play an important role in protein regulation (Yu and Fukagawa, 2020). A total of 17 amino acids (Figure 5) were analyzed in the soybean genotypes and recorded a significant difference (p < 0.05) amongst most of the amino acids (Supplementary Table S1). It was observed that wild soybean is an excellent source of all the essential and non-essential amino acids. Significant difference was observed in histidine, glutamic acid, glycine, aspartic acid, proline, and phenylalanine among all the samples. Based on the results of the present investigation, glutamic acid is the main component predominantly present in the soybean seeds, followed by aspartic acid and leucine. The concentration of amino acids in soybean genotypes was as follows: Glutamic acid>Aspartic acid>Leucine>Valine>Glycine>Lysine>Phenylalanine>Proline>Isoleucine>Alanine>Tyrosine>Histidine>Cystiene>Threonine>Methionine>Serine>Arginine. All soybean genotypes are rich in essential and non-essential amino acids, possessing various health benefits such as glycine and isoleucine preventing obesity, diabetes and liver diseases (Alves et al., 2019; Yu et al., 2021). Similarly, lysine is a key component of protein synthesis, and glutamic acid is a multifunctional amino acid that helps in promoting brain health. Similar findings of predominance of glutamic acid in soybean genotypes were reported by other researchers (Hong-Tae et al., 2005; Kim et al., 2023). According to a study by Chotekajorn et al. (2021) wild soybean is a great source of various amino acids such as alanine (0.051 mg/g dw), arginine (0.775 mg/g dw), asparagine (0.218 mg/g dw), aspartic acid (0.235 mg/g dw), glutamic acid (0.220 mg/g dw), glycine (0.014 mg/g dw), histidine (0.114 mg/g dw), isoleucine (0.028 mg/g dw), leucine (0.025 mg/g dw), lysine (0.030 mg/g dw), methionine (0.022 mg/g dw), phenylalanine (0.059 mg/g dw), proline (0.022 mg/g dw), etc. The noteworthy number of amino acids in G. soja benefits gastrointestinal development and integrity, enhances immune response potential, influences behavior, and supports sustainability (Meletis and Barker, 2005). Currently, there is a paucity of research focused on the amino acid’s estimation in wild soybean to conduct any comparative study highlighting a critical gap in understanding the amino acid composition of this species.
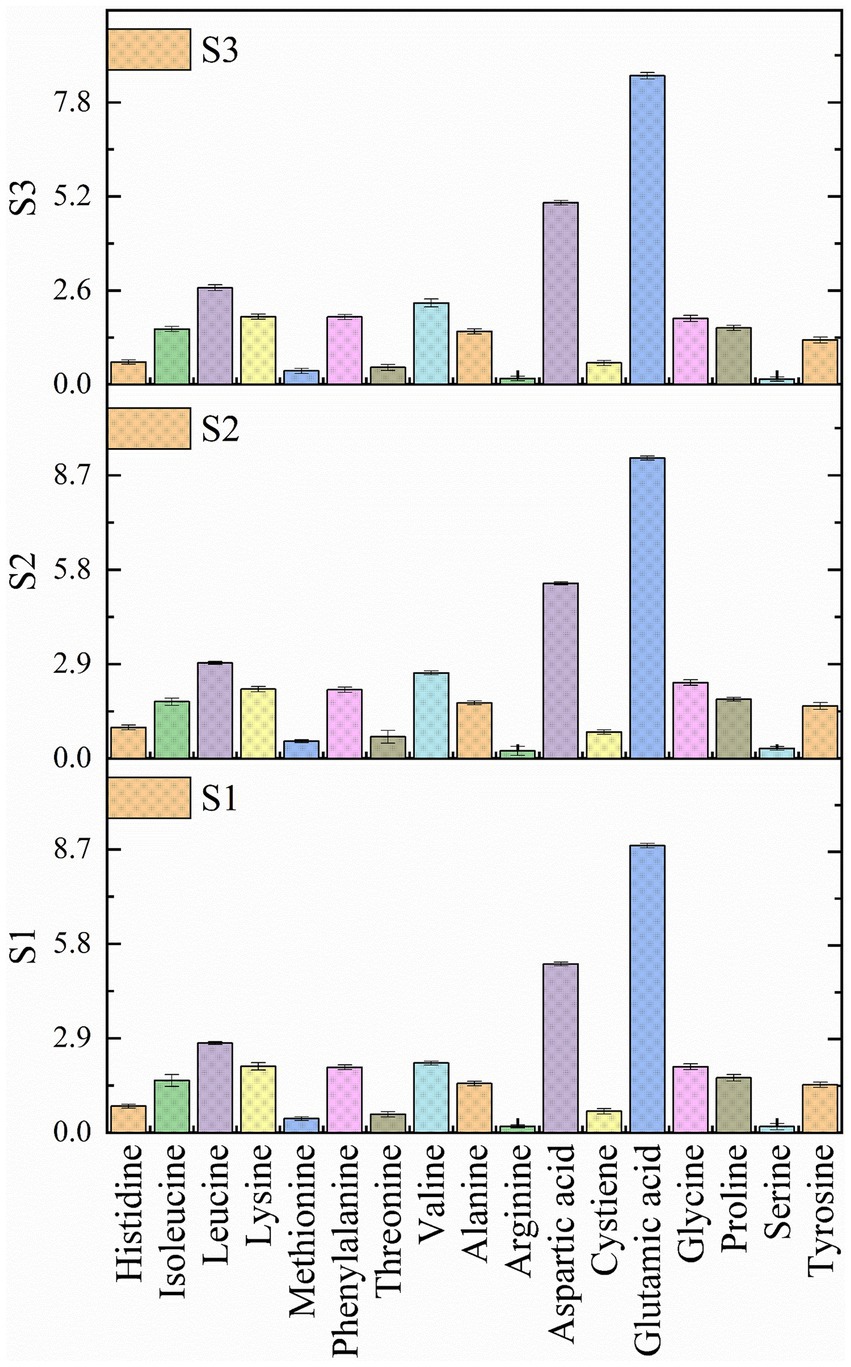
Figure 5. Amino acid profile of soybean genotypes. S1 – Black cultivated soybean, S2 – Wild soybean, S3 – Yellow cultivated soybean.
3.5 Phytochemicals, anthocyanin, and antioxidant activity
Consumption of food sources containing significant amount of phytochemicals and antioxidants are of great interest due to their beneficial effects on humans as they offer prevention and protection from certain chronic conditions (Zhang et al., 2015). In the sample context, soybean genotypes evaluated for phytochemical, anthocyanin and antioxidant property showed significant variation (p < 0.05) in all the parameters. Results revealed that the highest total phenol content (TPC) was observed in wild soybean (27.44 mg GAE/g dw) followed by black and yellow cultivated soybeans (15.18 mg GAE/g dw; 14.90 mg GAE/g dw) respectively as shown in Figure 6. The flavonoid content was observed highest in wild soybean (3.319 mg QE/g dw) followed by the other two-colored shades of cultivated soybean. Similar findings were reported by Chen et al. (2021), where the comparative phenol and flavonoid content between wild and cultivated soybeans revealed the highest content in wild soybeans, but the values of the present investigation were somewhat lower than those of the reported one. Additionally, results revealed that black soybeans have significantly higher phenol content when compared to yellow soybeans (Kumari et al., 2015). The highest tannin content was observed in black cultivated soybean (12.03 mg TAE/g dw). Sharma et al. (2013) investigated 20 soybean genotypes and reported the tannin content ranged from 11.1 to 18.8 mg/g dw. The total monomeric anthocyanin (TAC) was found to be highest in black soybeans. The highest anthocyanin content was found in wild soybean (9.150 mg CyE/g dw) followed by black cultivated soybeans (4.79 mg CyE/g /g dw). Yong-Ha et al. (2004) also investigated the total anthocyanin content among thirteen cultivated black soybean cultivars and reported varied anthocyanin ranges from 0.55–16.92 mg/g. Moreover, it was reported in an earlier study that anthocyanins from black soybeans act as a protective agent against oxidative damage to human glial cells (Kim et al., 2012). The antioxidative property of the samples showed that the highest ferric reducing antioxidant power (FRAP) activity was observed to be higher in wild soybean (3.56 mg AAE/g dw) followed by black cultivated soybean (2.62 mg AAE/g dw) and yellow cultivated soybean (1.95 mg AAE/g dw). Likewise, Choi et al. (2021) reported the highest FRAP value in soybeans as 1.58 mg AAE/g dw. The results of the present investigation were somewhat higher, which might be attributed to differences in extraction solvent and specific climatic and agronomic conditions under which the plants were cultivated. It has been previously reported that the genotype, growing environment, and their interaction can significantly impact the phytochemical and antioxidant activities of soybeans (Whent et al., 2009). Several studies have been done to investigate the comparative assessment of the different shades of cultivated soybean, but very limited studies have included wild soybean for the comparative analysis (Zhang et al., 2011; Oh et al., 2019; Choi et al., 2021). The present study has revealed a significant amount of phytochemicals and antioxidants in the wild species and inclusion of this species in the diet may help in the prevention of diabetes, obesity, aging, cancer, cardiovascular and neuro generative diseases (Muscolo et al., 2024).
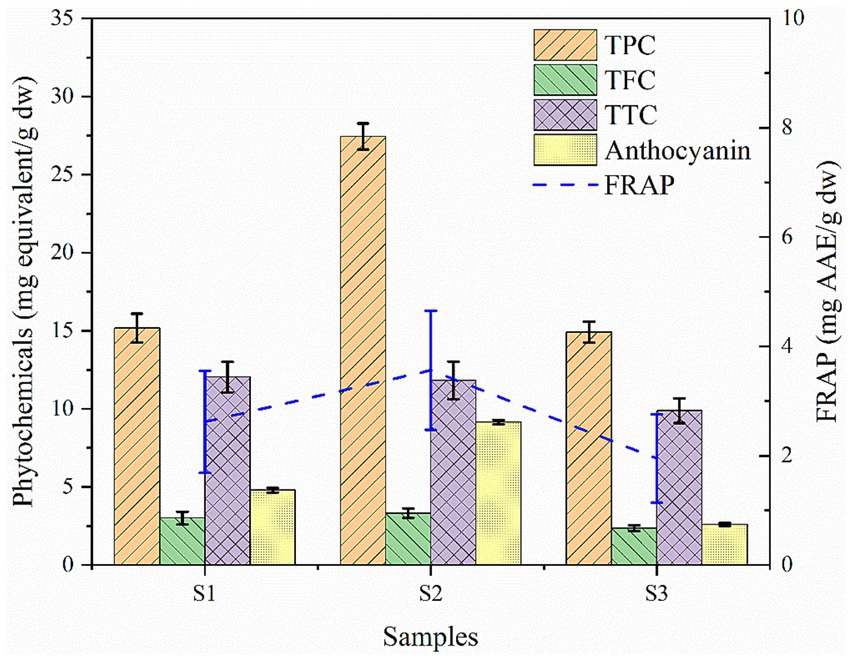
Figure 6. Phytochemical and antioxidant activity of soybean genotypes. S1 – Black cultivated soybean, S2 – Wild soybean, S3 – Yellow cultivated soybean, TPC-Total phenol content, TFC – Total flavonoid content, TTC-Total tannin content, FRAP – ferric reducing antioxidant power.
3.6 Correlation and principal component analysis
In this study, heat map analysis examined the correlation between the minerals and vitamins among the different soybean genotypes. It is evident from the heat map that vitamins have a strong positive correlation with minerals. Thiamine was correlated with minerals such as iron, and calcium; riboflavin with sodium; niacin with zinc; folate with potassium, and pyridoxine with iron, potassium, and calcium (Figure 7). Thus, improvement or enhancement of one mineral or vitamin may concurrently enhance the concentration of other minerals and vitamins. Additionally, most of the minerals analyzed showed a strong positive correlation with thiamine and pyridoxine (Figure 5). Similarly, principal component analysis (PCA) was performed to study the relationship between the phytochemicals, antioxidants, nutrients, and the soybean genotypes and between the genotypes themselves. The comparative analysis showed that the first principal component 1 (PC1 = 78.25%) and the second principal component 2 (PC2 = 21.75%) dimensions represent a total of 100% of variations among the parameters and genotypes (Figure 8). It is apparent from the biplot that wild soybean expressed a significant amount of protein, niacin, zinc, anthocyanin, flavonoids, phenol, and antioxidant activity. Black cultivated soybean contains a fair amount of phosphorus, magnesium, carbohydrates, tannin, and protein was found in moderate quantity. Yellow cultivated soybean showed a high amount of thiamine, folate, fat, potassium, sodium, and ash content. In summary, wild soybean and black cultivated soybean contains more Whereas, thiamine, fat, iron, sodium, and riboflavin were present in very less amount in all the three soybean genotypes. Wild soybeans with the highest protein content might be a suitable genetic material for domestication or breeding and, therefore, should be further investigated via advanced biotechnological techniques such as molecular markers for further validation. The biplot shows that fat, iron, calcium, thiamine, pyridoxine, and ash content are positively correlated. Likewise, phosphorus, magnesium, anthocyanin, carbohydrates, tannin, and protein are positively correlated. Phytochemicals, antioxidants, niacin, and protein also showed a strong positive correlation. On the other hand, traits significant in black cultivated soybean and wild soybean showed a negative correlation with the traits significant in yellow cultivated soybean. Additionally, it is also observed that phytochemicals (TPC, TFC, TTC) are negatively correlated with vitamins (Vit B1, Vit B2, and Vit B9) (Supplementary Table S2). This study highlights the advantage of improving traits that showed a positive correlation simultaneously. Whereas the traits that showed negative correlation could be improved independently. The correlation value r ≤ 0.35 characterize a weak correlation, whereas 0.36 to 0.67 signify moderate, and 0.68 to 1.00 shows a strong correlation (Taylor, 1990).
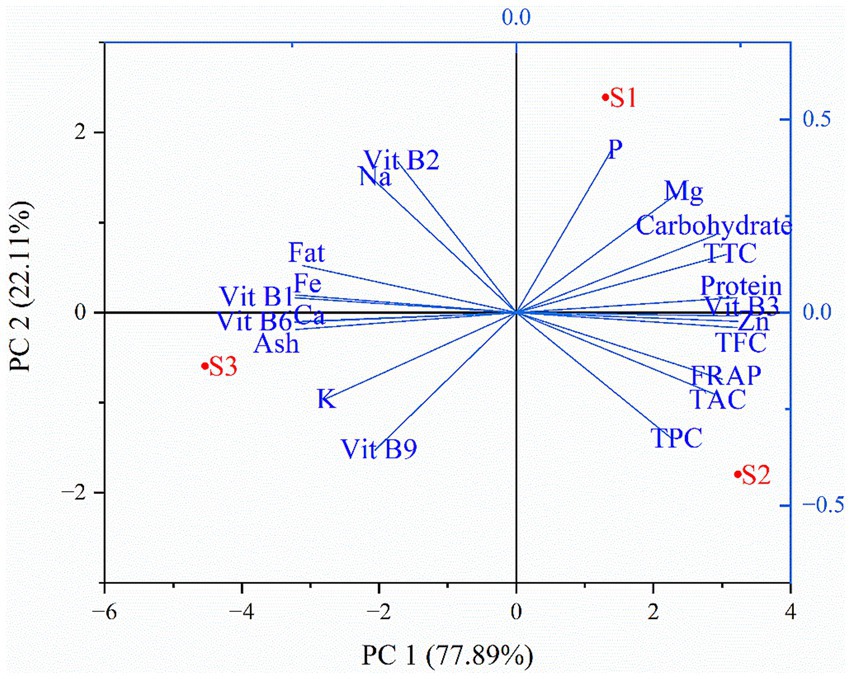
Figure 8. Biplot showing correlations between phytochemicals, antioxidants and nutrients contents of soybean genotypes. S1 – Black cultivated soybean, S2 – Wild soybean, S3 – Yellow cultivated soybean, P – Phosphorus, Mg – Magnesium, TTC – Total tannin content, Zn – Zinc, TFC – Total flavonoid content, FRAP – Ferric reducing antioxidant power, TAC – Total monomeric anthocyanin content, TPC – Total phenol content, Vit B1 – Thiamine, Vit B2 – Riboflavin, Vit B3 – Niacin, Vit B9 - Folate, Vit B6-Pyridoxine, K – Potassium, Ca – Calcium, Fe – Iron, Na – Sodium.
4 Conclusion
Among the genotypes analyzed wild soybean present a considerably rich diversity in terms of anthocyanin, protein, phytochemicals, antioxidants, some minerals, and amino acids specifically. The application of wild soybeans genes in enhancing stress tolerance and nutritional potential of cultivated soybeans is helping in maintaining genetic diversity despite continuous climatic fluctuations. Additionally, wild soybean also contains various bioactive compounds, i.e., isoflavones that are reported to cure various health conditions, making wild soybean a valuable genetic resource in agriculture as well as in cosmetic and pharmaceutical markets. However, the phytochemical and anthocyanin estimation were done quantitatively by spectrophotometer thus, further studies need to be done using advanced liquid chromatography methods to identify the specific compounds responsible that might be of use to the pharmaceutical industry. The exceptional amino acid profile or protein content comparable to cultivated soybean presents a valuable opportunity that should not be only limited to breeding approaches. The wild soybean can further be expanded to its bio-fortification and domestication to enhance the nutritional diversity as well as the conservation of wild crops. However, other cultivated soybean genotypes also showed promising traits for other factors like vitamins, minerals, ash, and fat content that enhance the overall nutritional profile. Further investigation, including studies involving the seed composition, biological properties, and advanced biotechnological tools, should be used to improve the agronomic traits of wild soybeans that limit their cultivation and production. In addition to this, extensive research should be done to evaluate the barriers that limit its adoption as a food source in many countries. Appropriate culinary practices should be highlighted that promote its easy acceptance and consumption. Moreover, mainstreaming nutritionally rich climate-smart wild crops could be of great importance in ensuring food and nutritional security.
Data availability statement
The original contributions presented in the study are included in the article/Supplementary material, further inquiries can be directed to the corresponding authors.
Author contributions
PO: Data curation, Investigation, Methodology, Software, Writing – original draft, Writing – review & editing. SP: Conceptualization, Formal analysis, Supervision, Writing – original draft, Writing – review & editing. ZW: Writing – review & editing. OA: Project administration, Validation, Writing – review & editing. IB: Writing – review & editing. DS: Writing – review & editing.
Funding
The author(s) declare that financial support was received for the research, authorship, and/or publication of this article. The present study was funded by Uttarakhand Council for Biotechnology, Haldi, Pantnagar, U.S. Nagar, Uttarakhand, India.
Acknowledgments
The authors are grateful to Prof. Sunil Nautiyal, Director, G.B. Pant National Institute of Himalayan Environment for extending the facility. The Director of Uttarakhand Council for Biotechnology, Haldi Uttarakhand is greatly acknowledged for financial support.
Conflict of interest
The authors declare that the research was conducted in the absence of any commercial or financial relationships that could be construed as a potential conflict of interest.
Publisher’s note
All claims expressed in this article are solely those of the authors and do not necessarily represent those of their affiliated organizations, or those of the publisher, the editors and the reviewers. Any product that may be evaluated in this article, or claim that may be made by its manufacturer, is not guaranteed or endorsed by the publisher.
Supplementary material
The Supplementary material for this article can be found online at: https://www.frontiersin.org/articles/10.3389/fsufs.2024.1495734/full#supplementary-material
References
Abdulkadir, A. R., Zawawi, D. D., and Jahan, M. S. (2016). Proximate and phytochemical screening of different parts of Moringa oleifera. Russ. Agric. Sci. 42, 34–36.
Agarwal, D. K., Billore, S. D., Sharma, A. N., Dupare, B. U., and Srivastava, S. K. (2013). Soybean: introduction, improvement, and utilization in India—problems and prospects. Agric. Res. 2, 293–300. doi: 10.1007/s40003-013-0088-0
Alves, A., Bassot, A., Bulteau, A.-L., Pirola, L., and Morio, B. (2019). Glycine metabolism and its alterations in obesity and metabolic diseases. Nutrients 11:1356. doi: 10.3390/nu11061356
Amaani, R., and Dwira, S. (2018). Phytochemical content an in vitro toxicity of Glycine soja ethanol extract on the A549 lung cancer line cell. J. Phys. Conf. Ser. 1073:032042. doi: 10.1088/1742-6596/1073/3/032042
Bellaloui, N., Bruns, H. A., Abbas, H. K., Mengistu, A., Fisher, D. K., and Reddy, K. N. (2015). Agricultural practices altered soybean seed protein, oil, fatty acids, sugars, and minerals in the Midsouth USA. Front. Plant Sci. 6:31. doi: 10.3389/fpls.2015.00031
Benzie, I. F. F., and Strain, J. J. (1996). The ferric reducing ability of plasma (FRAP) as a measure of “antioxidant power”: the FRAP assay. Anal. Biochem. 239, 70–76. doi: 10.1006/abio.1996.0292
Bhartiya, A., Chandra, N., Pal, R. S., Aditya, J., and Bajeli, J. (2017). Comparative yield gaps, economic impact and constraint analysis in front line demonstrations of soybean and black soybean in Uttarakhand hills. Indian. J. Agric. Res. 51:4715. doi: 10.18805/IJARe.A-4715
Chasapis, C. T., Loutsidou, A. C., Spiliopoulou, C. A., and Stefanidou, M. E. (2012). Zinc and human health: an update. Arch. Toxicol. 86, 521–534. doi: 10.1007/s00204-011-0775-1
Chen, K.-I., Erh, M.-H., Su, N.-W., Liu, W.-H., Chou, C.-C., and Cheng, K.-C. (2012). Soyfoods and soybean products: from traditional use to modern applications. Appl. Microbiol. Biotechnol. 96, 9–22. doi: 10.1007/s00253-012-4330-7
Chen, Y., Michalak, M., and Agellon, L. B. (2018). Focus: nutrition and food science: importance of nutrients and nutrient metabolism on human health. Yale J. Biol. Med. 91, 95–103
Chen, Y., and Nelson, R. L. (2004). Genetic variation and relationships among cultivated, wild, and semiwild soybean. Crop Sci. 44, 316–325. doi: 10.2135/cropsci2004.3160
Chen, Q., Wang, X., Yuan, X., Shi, J., Zhang, C., Yan, N., et al. (2021). Comparison of phenolic and flavonoid compound profiles and antioxidant and α-glucosidase inhibition properties of cultivated soybean (Glycine max) and wild soybean (Glycine soja). Plan. Theory 10:813. doi: 10.3390/plants10040813
Choi, Y.-M., Yoon, H., Shin, M.-J., Lee, Y., Hur, O. S., Lee, B. C., et al. (2021). Metabolite contents and antioxidant activities of soybean (Glycine max (L.) Merrill) seeds of different seed coat colors. Antioxidants 10:1210. doi: 10.3390/antiox10081210
Chotekajorn, A., Hashiguchi, T., Hashiguchi, M., Tanaka, H., and Akashi, R. (2021). Evaluation of seed amino acid content and its correlation network analysis in wild soybean (Glycine soja) germplasm in Japan. Plant Genetic Resour. 19, 35–43. doi: 10.1017/S1479262121000071
Das, R., Mahapatra, S. D., Das, M. T., and Nayak, R. K. (2023). Estimation of carbohydrate, protein and secondary metabolites of selected Curcuma species from the northern coastal region of Odisha, India. J. Pharm. Phytochem. 12, 25–29. doi: 10.22271/phyto.2023.v12.i4a.14689
Department of Agriculture Uttarakhand. (2020). Agriculture statistics data. Available at: http://agriculture.uk.gov.in/pages/display/217-home (Accessed March, 20 2023).
Etiosa, O. R., Chika, N. B., and Benedicta, A. (2017). Mineral and proximate composition of soya bean. Asian J. Phys. Chem. Sci. 38, 183–223. doi: 10.1080/20014091084209
Hartwig, A. (2001). Role of magnesium in genomic stability. Mutation Res. 475, 113–121. doi: 10.1016/S0027-5107(01)00074-4
Hatami, T., Emami, S. A., Miraghaee, S. S., and Mojarrab, M. (2014). Total phenolic contents and antioxidant activities of different extracts and fractions from the aerial parts of Artemisia biennis Willd. Iran. J. Pharm. Res. 13, 551–559
Hong-Tae, Y., Min-Jung, S., and Sun-Ok, A. (2005). Variation of seed component contents in wild soybean (Glycine soja Sieb. & Zucc.). Korean J. Crop Sci. 50, 108–111.
Kala, C. P., and Nautiyal, S. (2023). Traditional food knowledge of local people and its sustainability in mountains of Uttarakhand state of India. J. Soc. Econ. Dev. 25, 32–51. doi: 10.1007/s40847-022-00222-z
Kılıç Altun, S., Dinç, H., Paksoy, N., Temamoğulları, F. K., and Savrunlu, M. (2017). Analyses of mineral content and heavy metal of honey samples from south and east region of Turkey by using ICP-MS. Int. J. Analyt. Chem. 2017:6391454. doi: 10.1155/2017/6391454
Kim, W. J., Kang, B. H., Kang, S., Shin, S., Chowdhury, S., Jeong, S.-C., et al. (2023). A genome-wide association study of protein, oil, and amino acid content in wild soybean (Glycine soja). Plan. Theory 12:1665. doi: 10.3390/plants12081665
Kim, Y.-K., Yoon, H.-H., Lee, Y.-D., Youn, D.-Y., Ha, T.-J., Kim, H.-S., et al. (2012). Anthocyanin extracts from black soybean (Glycine max L.) protect human glial cells against oxygen-glucose deprivation by promoting autophagy. Biomol. Therap. 20, 68–74. doi: 10.4062/biomolther.2012.20.1.068
Kofsky, J., Zhang, H., and Song, B. H. (2018). The untapped genetic reservoir: the past, current, and future applications of the wild soybean (Glycine soja). Front. Plant Sci. 9:949. doi: 10.3389/fpls.2018.00949
Kumari, S., Krishnan, V., and Sachdev, A. (2015). Impact of soaking and germination durations on antioxidants and anti-nutrients of black and yellow soybean (Glycine max. L) Varieties. J. Plant Biochem. Biotechnol. 24, 355–358. doi: 10.1007/s13562-014-0282-6
Lee, J., Durst, R. W., Wrolstad, R. E., Eisele, T., Giusti, M. M., Hach, J., et al. (2005). Determination of Total monomeric anthocyanin pigment content of fruit juices, beverages, natural colorants, and wines by the pH differential method: collaborative study. J. AOAC Int. 88, 1269–1278. doi: 10.1093/jaoac/88.5.1269
MacKay, D., Hathcock, J., and Guarneri, E. (2012). Niacin: chemical forms, bioavailability, and health effects. Nutr. Rev. 70, 357–366. doi: 10.1111/j.1753-4887.2012.00479.x
Maqbool, M. A., Aslam, M., Akbar, W., and Iqbal, Z. (2017). Biological importance of vitamins for human health: a review. J. Agric. Basic Sci. 2, 50–58.
Meletis, C. D., and Barker, J. E. (2005). Therapeutic uses of amino acids. Altern. Complem. Therap. 11, 24–28. doi: 10.1089/act.2005.11.24
Mengerink, Y., Kutlán, D., Tóth, F., Csámpai, A., and Molnár-Perl, I. (2002). Advances in the evaluation of the stability and characteristics of the amino acid and amine derivatives obtained with the o-phthaldialdehyde/3-mercaptopropionic acid and o-phthaldialdehyde/N-acetyl-L-cysteine reagents: high-performance liquid chromatography–mass spectrometry study. J. Chromatogr. A 949, 99–124. doi: 10.1016/S0021-9673(01)01282-1
Mikkelsen, K., Dargahi, N., Fraser, S., and Apostolopoulos, V. (2023). High-dose vitamin B6 (pyridoxine) displays strong anti-inflammatory properties in lipopolysaccharide-stimulated monocytes. Biomedicines 11:2578. doi: 10.3390/biomedicines11092578
Mrowicka, M., Mrowicki, J., Dragan, G., and Majsterek, I. (2023). The importance of thiamine (vitamin B1) in humans. Biosci. Rep. 43:374. doi: 10.1042/BSR20230374
Munteanu, C., and Schwartz, B. (2022). The relationship between nutrition and the immune system. Front. Nutr. 9:1082500. doi: 10.3389/fnut.2022.1082500
Muscolo, A., Mariateresa, O., Giulio, T., and Mariateresa, R. (2024). Oxidative stress: the role of antioxidant phytochemicals in the prevention and treatment of diseases. Int. J. Mol. Sci. 25:3264. doi: 10.3390/ijms25063264
NIH (2024) Nutrient Recommendations and Databases. Available at: https://ods.od.nih.gov/HealthInformation/nutrientrecommendations.aspx (Accessed March, 20 2023).
Nuthalapati, C. S., Kumar, A., Birthal, P. S., and Sonkar, V. K. (2024). Demand-side and supply-side factors for accelerating varietal turnover in smallholder soybean farms. J. Clean. Prod. 447:141372. doi: 10.1016/j.jclepro.2024.141372
Nwinuka, N. M., Ibeh, G. O., and Ekeke, G. I. (2005). Proximate composition and levels of some toxicants in four commonly consumed spices. J. Appl. Sci. Environ. Manag. 9, 150–155.
Oh, S. D., Yeo, Y., Lee, S. Y., Suh, S. J., Moon, J. K., Park, S. K., et al. (2019). A comparison of the characteristic properties between soybean (Glycine max [L.] Merrill) seeds with different seed coat colors. Korean. J. Agric. Sci. 46, 971–980. doi: 10.7744/kjoas.20190079
Ojha, P., Pathak, G., Maharjan, S., Manandhar, U., Maharjan, S., and Karki, R. (2022). Quality and textural properties evaluation of gluten-free biscuit developed from maize, rice, buckwheat, and soybean. Sci. Study Res. 23, 295–305.
Pagano, M. C., and Miransari, M. (2016). “The importance of soybean production worldwide” in Abiotic and biotic stresses in soybean production. ed. M. Miransari (Elsevier), 1–26.
Prashnani, M., Dupare, B., Vadrevu, K. P., and Justice, C. (2024). Towards food security: exploring the spatio-temporal dynamics of soybean in India. PLoS One 19:e0292005. doi: 10.1371/journal.pone.0292005
Quettier-Deleu, C., Gressier, B., Vasseur, J., Dine, T., Brunet, C., Luyckx, M., et al. (2000). Phenolic compounds and antioxidant activities of buckwheat (Fagopyrum esculentum Moench) hulls and flour. J. Ethnopharmacol. 72, 35–42. doi: 10.1016/S0378-8741(00)00196-3
Raboy, V., Dickinson, D. B., and Below, F. E. (1984). Variation in seed Total phosphorus, Phytic acid, zinc, calcium, magnesium, and protein among lines of Glycine max and G. soja. Crop Sci. 24, 431–434. doi: 10.2135/cropsci1984.0011183X002400030001x
Rani, V., Grewal, R. B., and Khetarpaul, N. (2008). Physical characteristics, proximate and mineral composition of some new varieties of soybean (Glycine max L.). Legume Res. Int. J. 31, 31–35.
Ren, S. C., Liu, Z. L., and Wang, P. (2012). Proximate composition and flavonoids content and in vitro antioxidant activity of 10 varieties of legume seeds grown in China. J. Med. Plants Res. 6:1408. doi: 10.5897/JMPR11.1408
Saitoh, K., Nishimura, K., and Kuroda, T. (2004). Characteristics of flowering and pod set in wild and cultivated types of soybeans. Plant prod. Sci. 7, 172–177. doi: 10.1626/pps.7.172
Seal, T. (2016). Quantitative HPLC analysis of phenolic acids, flavonoids and ascorbic acid in four different solvent extracts of two wild edible leaves, Sonchus arvensis and Oenanthe linearis of north-eastern region in India. J. Appl. Pharm. Sci. 6, 157–166. doi: 10.7324/JAPS.2016.60225
Sharma, P. (2016). Dynamics of growth of soybean in India: role of income and risk. Agric. Situat. India 73, 37–46.
Sharma, S., Goyal, R., and Barwal, S. (2013). Domestic processing effects on physicochemical, nutritional and anti-nutritional attributes in soybean (Glycine max L. Merill). Int. Food Res. J. 20, 3203–3209.
Singh, P., and Krishnaswamy, K. (2022). Sustainable zero-waste processing system for soybeans and soy by-product valorization. Trends Food Sci. Technol. 128, 331–344. doi: 10.1016/j.tifs.2022.08.015
Suwannasom, N., Kao, I., Pruß, A., Georgieva, R., and Bäumler, H. (2020). Riboflavin: the health benefits of a forgotten natural vitamin. Int. J. Mol. Sci. 21:950. doi: 10.3390/ijms21030950
Takeda, E., Yamamoto, H., Yamanaka-Okumura, H., and Taketani, Y. (2012). Dietary phosphorus in bone health and quality of life. Nutr. Rev. 70, 311–321. doi: 10.1111/j.1753-4887.2012.00473.x
Taylor, R. (1990). Interpretation of the correlation coefficient: a basic review. J. Diagn. Med. Sonogr. 6, 35–39. doi: 10.1177/875647939000600106
Vineet Kumar, V. K., Anita Rani, A. R., and Chauhan, G. S. (2010). “Nutritional value of soybean” in The soybean: Botany, production and uses. ed. G. Singh (Wallingford UK: CABI), 375–403.
Warzyszynska, J. E., and Kim, Y. J. (2014). “Folate in human health and disease” in Encyclopedia of life sciences (Chichester, UK: John Wiley & Sons, Ltd), 1–14.
Whent, M., Hao, J., Slavin, M., Zhou, M., Song, J., Kenworthy, W., et al. (2009). Effect of genotype, environment, and their interaction on chemical composition and antioxidant properties of low-linolenic soybeans grown in Maryland. J. Agric. Food Chem. 57, 10163–10174. doi: 10.1021/jf902344h
Yong-Ha, J., Myoung-Gun, C., Seung-Gil, Y., and Kil-Woong, C. (2004). Variation of contents and color difference of anthocyanin by different cultivation year in black soybean seed. Korean J. Crop Sci. 49, 507–511.
Yu, Y. M., and Fukagawa, N. K. (2020). “Protein and amino acids” in Present knowledge in nutrition (Academic Press), 15–35.
Yu, D., Richardson, N. E., Green, C. L., Spicer, A. B., Murphy, M. E., Flores, V., et al. (2021). The adverse metabolic effects of branched-chain amino acids are mediated by isoleucine and valine. Cell Metab. 33, 905–922.e6. doi: 10.1016/j.cmet.2021.03.025
Zhang, Y. J., Gan, R. Y., Li, S., Zhou, Y., Li, A. N., Xu, D. P., et al. (2015). Antioxidant phytochemicals for the prevention and treatment of chronic diseases. Molecules 20, 21138–21156. doi: 10.3390/molecules201219753
Keywords: Glycine soja, black soybean, amino acids, nutritional security, phytochemicals
Citation: Oli P, Punetha S, Wani ZA, Arya OP, Bhatt ID and Singh DK (2024) Exploring the nutraceutical potential of soybean genotypes of Uttarakhand: implications for the mainstreaming of wild soybean. Front. Sustain. Food Syst. 8:1495734. doi: 10.3389/fsufs.2024.1495734
Edited by:
Tahmeena Khan, Integral University, IndiaReviewed by:
Wasiu Awoyale, Kwara State University, NigeriaWieslaw Oleszek, Institute of Soil Science and Plant Cultivation, Poland
Copyright © 2024 Oli, Punetha, Wani, Arya, Bhatt and Singh. This is an open-access article distributed under the terms of the Creative Commons Attribution License (CC BY). The use, distribution or reproduction in other forums is permitted, provided the original author(s) and the copyright owner(s) are credited and that the original publication in this journal is cited, in accordance with accepted academic practice. No use, distribution or reproduction is permitted which does not comply with these terms.
*Correspondence: Shailaja Punetha, c2hhaWx1cHVuZXRoYUBnbWFpbC5jb20=; Zishan Ahmad Wani, emlzaGFud2FuaTc4NkBnbWFpbC5jb20=