- 1Public Health Department, School of Public Health, Jilin Medical University, Jilin City, China
- 2Health Sciences Department, Louisiana State University Health Sciences Center, New Orleans, LA, United States
The widespread use of plastics in beverage packaging has led to the accumulation of microplastics (MPs) and nanoplastics (NPs) in beverages, which poses significant environmental and health hazards. This review explores the sources, entry pathways, and risk factors for MPs and NPs in beverages, emphasizing their toxicological profiles and harmful effects on human health and the environment. Methods for detecting MPs and NPs in beverages are discussed, highlighting the need for standardized testing protocols. Furthermore, future solution strategies, challenges, and preventive measures for mitigating MP and NP contamination in beverages are proposed, including advanced filtration systems, the development of alternative packaging materials, and the strengthening of regulatory standards. Collaborative efforts among industry stakeholders, scientific institutions, and policymakers are essential to address this complex issue and ensure the safety and purity of beverages for consumers worldwide.
1 Introduction
With the progress of science and technology and the rapid development of industrialization, plastic products have gradually replaced traditional materials and become an indispensable part of people's lives (Geyer et al., 2017; De la Torre, 2020). Plastic is a popular raw material because of its waterproof and sealing properties (Correia et al., 2022). They can be molded into diverse shapes and is capable of withstanding high temperatures, making it a versatile material for the preservation, transportation, and distribution of food. The global production of plastics grew by 4% to over 390 million tons in 2022, demonstrating the strong and sustained demand for plastics across a wide range of industries (Plastics Europe, 2022). However, the widespread use of plastics has also led to a series of environmental and health problems. In the food and beverage sector, plastics are widely used in packaging, transportation, storage, processing, and other operations (Kutralam Muniasamy et al., 2023). Beverages are a major component of the diet of the global population, especially in the adolescent population (Bi et al., 2023). The increase in beverage trade has resulted in a greater amount of single-use plastic waste during production and consumption. The extensive use of plastic products directly leads to the emission of large quantities of plastic waste. On the one hand, plastic waste generated by the food industry can cause serious environmental pollution. It can take hundreds of years for plastic packaging to decompose, and more than 8 million tons of plastic waste enter the ocean every year, causing severe damage to the marine ecosystem (Landrigan et al., 2023). Moreover, a large amount of plastic waste is either buried on land as solid basic plastics or floating in the ocean (Khant and Kim, 2022). On the other hand, in the food industry, plastic products can leach chemicals or polymer plastic fragments into food and beverages (Huang et al., 2022). As plastic materials continue to be broken down into plastic debris, large amounts of plastic products are broken down into microplastics (MPs) and nanoplastics (NPs), which are widely distributed in the environment (Oleksiuk et al., 2022). Depending on the size of the plastic debris, particles and fragments with a diameter of < 5 millimeters are categorized as MPs (Khant and Kim, 2022), and NPs are tiny plastic particles with sizes between 1 and 100 nanometers (Sewwandi et al., 2023). In food and beverages, they are generated mainly by excessive packaging, prolonged exposure during processing, long-term mass storage, and accumulation within the food chain. They can be either be intentionally manufactured at the nanoscale for various purposes or result from the degradation of larger plastic materials (Tan et al., 2024). They can be categorized into primary and secondary MPs and NPs (Lett et al., 2021). Primary plastics are made from primary feedstocks, which are natural gas and crude oil (Geyer, 2020). Examples of plastic products commonly used in food processing and storage include plastic films, plastic bottles, plastic fillers, and dryer packages. Secondary plastics result from the degradation of larger plastic wastes through physical, biological, and chemical processes like photodegradation. Both types of plastics are resistant to degradation, leading to persistent environmental and health issues (Akanyange et al., 2022). MPs and NPs are frequently detected in food and beverages, particularly from packaging materials like disposable tableware and bottle caps, and have been identified in products such as meat, carbonated, and alcoholic beverages (Chen J. et al., 2023; Karačonji et al., 2017; Chen Y. et al., 2023). The MPs and NPs in food and beverages indirectly enter our body through our diet, and the resulting health hazards, food chain accumulation, and environmental pollution are becoming increasingly serious. Understanding contaminants in beverages and proposing prevention strategies are urgent issues (Mao X. et al., 2022). Therefore, this review sheds light on the contamination and hazards of MPs and NPs in beverages. This review also explores various detection methods, prevention strategies, and future trends.
2 Sources and entry pathways of MPs and NPs in beverages
As of 2023, the sales volume of the global beverage industry continues to grow annually, with high consumption of soft drinks, carbonated beverages, and sparkling water, particularly in densely populated middle-income countries (Altunışık, 2023). The revenue of the global retail beverage market reached $868.077 billion by 2023. Carbonated beverages are the largest contributor to sales volume (Vandevijvere et al., 2019), accounting for 40.2% to 86.0% of sales (Bandy et al., 2023). The rapid growth of the beverage industry has resulted in its expanding market share globally (Liu J. et al., 2020).
2.1 Source pathways of food and beverages receiving MP and NP contamination
MPs and NPs are emerging pollutants released into beverages from plastic packaging and bottle caps by dissolution, temperature variation, and mechanical stress (Sohail et al., 2023). Common beverages include solid brewed beverages, liquid carbonated beverages, energy drinks, coffee, milk, soda, fruit and vegetable juices, protein, syrup, concentrate, and flavored beverages (Figure 1A). The commonly consumed beverages include alcoholic beverages such as brewed wine, distilled spirits, beer, and preproduce beverages (sold in fast-food stores) (Xing et al., 2023). The sources and entry routes of MPs and NPs can be categorized from several perspectives, such as liquid-based methods and different types of water, including beverages, drinking water, groundwater, and seawater. Solid-based examples include food packaging, straws, beverage bottles, tea bags, and paper scraps (Figure 1B). Moreover, most microplastics in beverages are liquid-based (Ramachandraiah et al., 2022). Single-use plastic bottles, cans, glass bottles, paper cups and cartons are widely used in the beverage industry because of their portability, long storage time, variety and other characteristics (Vega Herrera et al., 2023). However, these characteristics can also lead to the dissolution of large amounts of plastic debris into beverages. Paper cups have a hydrophobic film laminated to the inside of the cup, which is made of plastic or copolymers (Ranjan et al., 2020). These films degrade when exposed to hot water, and as the films deteriorate, ions such as fluoride, chloride, sulfate and nitrate are released into the water in the paper cup. The regular and consequent ingestion of microplastics, ions and heavy metals during the daily consumption of hot beverages such as tea and coffee exposes us to potential health risks in the future. The amount of dissolved plastic particles in a beverage is closely related to the duration of storage, and the presence or absence of substances such as high sugar content and carbonation in the beverage directly contributes to the increase in the number of plastic particles (Chen Y. et al., 2023). Distorted shapes and aggregated NPs were detected in heated polyethylene (PE) and polyethylene terephthalate (PET) paper cups by scanning electron microscopy (Wang et al., 2023). The greatest levels of MPs released into the containing water were found at 70–95°C in disposable cups; acidic carbonated beverages promoted the release of MPs more than plain water did, and that PE-coated paper cups were the most sensitive to the type of beverage in the test cup (Chen H. et al., 2023). One study detected MPs (9.66 particles/liter) and NPs (0.73 × 107 particles/liter) in beverages packaged in refrigerated polypropylene (PP) bottles (Chen Y. et al., 2023). Huang et al. (2022) found that spherical NP particles were detected in bottled water, suggesting that this was due to their release into PET bottles containing water. The chance of human MP ingestion increases with the frequent use of the same single-use plastic bottle through the bottleneck cap system (Winkler et al., 2019). MPs and phthalate esters can be released into tea beverages through nylon cloth-wrapped tea bags (Kashfi et al., 2023). The polyvinyl chloride (PVC) content of sugar-sweetened beverages was found to be significantly greater than that of unsweetened beverages in the analysis of six bottled beverages (Fernandez-Arribas et al., 2023). Schymanski et al. (2018) reported varying levels of plastic particles in commercially available single-use plastic bottles and glass bottles. The beverage industry requires a large amount of fresh water (Mortensen et al., 2021). Not only do instant solid beverages require fresh water as a solvent, but commercial bottled beverages also require fresh water as the main ingredient (Panno et al., 2019). The production of beverages requires large amounts of water and large amounts of MPs and NPs. This requires beverage factories to use natural water sources that have been filtered and sterilized. However, current tests of water sources in factories do not consider plastic particles, which does not guarantee safety in beverages (Arijeniwa et al., 2024). During this process, water can carry most nutrients and micronutrients and acts as a good solvent, which leads to the transfer of MPs and NPs from natural water to beverages (Moodie et al., 2013). A study revealed that microplastics in ice cubes can be spread to humans by adding them to beverages (Shruti et al., 2023). In addition, different processes, such as washing of fruits, filtration, sterilization, and canning, can induce microplastic contamination (Liu et al., 2022) (Figure 1C). The presence of MPs was detected in the edible parts of common vegetables and fruits in the study by Aydin et al. (2023), and the highest microplastic intake was in tomatoes (398,520 particles individual-1 year-1 for estimated annual intake). Disposable plastic packaging is the most significant source used in the production of food and beverages (Phelan et al., 2022; Kaur et al., 2024). Kalanyan et al. reported that PET released seven times more particles into hot beverages than into plastic bottles at room temperature (Zangmeister et al., 2022). However, it is important to note that the production of beverages indispensably requires autoclaving. PE sealing is commonly used to inhibit microbial growth during the production and processing of dairy products, but this has resulted in dairy products accessing PE during the sealing process, with MPs and NPs detected in milk and yogurt (Diaz-Basantes et al., 2020; Tristan et al., 2023). MP contamination is more severe when hot beverages are served in disposable paper cups, and the high surface area and volume ratio of microplastics increase their susceptibility to contamination with adsorbed pathogens or toxins (Joseph et al., 2023). Therefore, beverage outer packaging materials, transportation contact materials, prolonged storage and the use of bottles and caps can also be sources of MP and NP contamination.
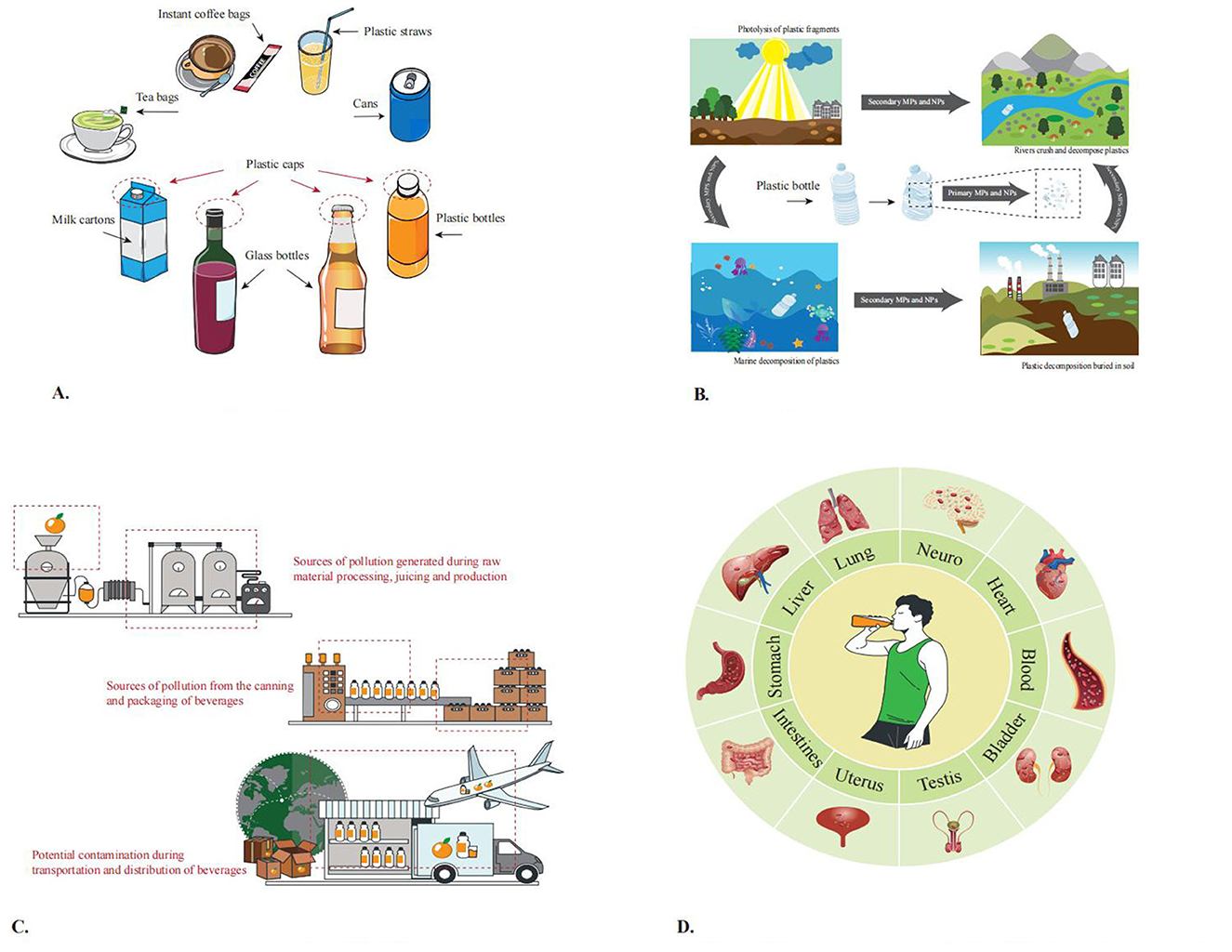
Figure 1. Sources and entry pathway of MPs and NPs in beverages. (A) Currently different types of beverage commodities. Including plastic bottles, plastic straws, cans, glass wine bottles, beer bottles, milk cartons, instant coffee bags, tea bags, etc. (B) Sources of MPs and NPs in beverages. The illustrative lists the pathways into beverages through different categories, photodegradable plastic fragments in the air, liquid-based including plastics broken down by crushed river debris, soil-buried solids including food packaging, straws, beverage bottles, tea bags and paper scraps, fragments of plastic bottles flowing into the oceans, and plastic bag waste. (C) Sources and entry pathway of MPs and NPs in beverages. Pathways of contamination that can occur in the packaging and transportation of beverages. Factors in the production and processing of beverages that may contribute to the accumulation of MPs and NPs include fruit residues, canning, and the transportation chain. (D) Distribution of MPs and NPs entering human organs in beverages. Plastic particles ingested through beverages accumulate in different organs of the body, including the nervous system, heart, blood system, kidneys, lungs, liver, stomach, intestines, uterus, testes and other organs.
2.2 Environmental impacts
Most plastics are made up of synthetic polymers, which ultimately determine their MP and NP compositions. Plastic packaging typically includes materials such as PE, PET, PP, PVC, and polystyrene (PS). PET is the most commonly used material for beverage bottles; PP is the main material for the caps and seals of beverage bottles; PVC is commonly used for disposable plastic cups and plastic food containers; PS is commonly used for fast-food containers and beverage cartons; and PE is mainly used for food packaging bags, preservation bags, milk bottles, and yogurt bottles (Yozukmaz, 2022). Liquids such as beverages are directly exposed and in contact with PET and other plastic materials, and studies have shown that almost all bottled beverages contain small amounts of dissolved MPs and NPs (Muhib et al., 2023). We summarize the characteristics (MatWeb, 2024; KIT, 2024), concentrations and applications of common microplastics in beverage applications in Table 1. Schymanski et al. (2018) detected trace amounts of MPs and NPs in retail beverages packaged in beverage cartons and glass bottles. The production and consumption of bottled beverages, which constitute a significant source of plastics in the pathway, are increasing every year. Heating causes plastic cups, beverage bottles, and food packaging to be more susceptible to plastic particles (Joseph et al., 2023), and studies have shown that hot water immersion leads to the release of 1 million submicron and particle-sized particles per milliliter of leachate from plastic materials (Liu et al., 2022). Plastic debris, through various pathways, such as runoff, atmospheric deposition, and improper waste management, can contaminate water sources and ultimately find their way into beverages. The source of the water determines the amount of microplastics in beverages. Moreover, large quantities of water are also used in cleaning beverage containers and assembly lines, and the amount of water used is much greater than the actual amount of water used for packaging and transportation (Keerthana Devi et al., 2022). This results in large amounts of MPs and NPs from the outer packaging of food and beverages being released into the natural environment through decomposition and metabolism. Moreover, MPs and NPs in the natural environment enter beverages through various pathways, predominantly in liquids, resulting in a vicious cycle of their continuous accumulation.
2.3 The way in which MPs and NPs enter the human body
There are several ways for plastics to enter the human body (Figure 1D). First, when plastic bottles or containers are used to hold beverages, particles of MPs and NPs may enter the beverage from the surface of the container. There is much evidence that beverages or bottled water contain varying degrees of plastic particles. In a study of 20 popular brands of bottled water, samples were detected to contain PP, PE, and PET, in addition to 28 plastic additives (Vega Herrera et al., 2023). Beverages packaged in plastic bottles are a nonnegligible route of exposure to MPs and NPs, and a reminder that what we consume through beverages not only contains water or nutrients that our bodies need but also contains harmful substances. Second, when we drink beverages containing MPs and NPs, these particles may be ingested through the digestive tract. Once in the body, MPs and NPs may pose potential health risks (Lee et al., 2022). PET, PE, and PS have been found to varying degrees in human blood investigations, suggesting that plastic pellets are bioavailable and can be absorbed into human blood (Leslie et al., 2022). Studies have shown that these particles may accumulate in the intestinal tract and further enter other tissues and organs, such as the liver, lungs, and lymphatic system. In addition, MPs and NPs may affect the immune system, endocrine system, and nervous system, which may lead to inflammatory responses, immune disorders, and other health problems (Osman et al., 2023). MPs and NPs are immeasurably harmful to human health. On the one hand, plastic pellets carry toxic chemicals into the ecosystem and thus act as a transport medium; on the other hand, they are polymers of hazardous chemicals that are voluntarily added as additives during the production process to improve the properties of the polymer and extend its life.
3 Risk factors of MPs and NPs in beverages
There is growing evidence that MPs and NPs are found in every corner of the planet (Muhib et al., 2023). Humans need to focus more attention on the safety and toxicity issues associated with MPs and NPs in the food and beverage industry. MPs and NPs have substantial toxic effects on human health and even on animals, plants and the environment. From the perspective of food safety, humans are at the top of the food chain, and plastic fragments continue to accumulate in plants and animals and will eventually be ingested by humans. Moreover, dissolved plastic fragments in beverages also contribute to the accumulation of MPs and NPs in the human body, all of which contribute to their varying degrees of toxicity and harm to human health (Stapleton, 2021).
3.1 Harmful effects of ingesting MPs and NPs derived from the beverage industry
Plastics in beverages are degraded when they enter the environment and are also ingested by organisms, affecting various physiological functions. The consumption of microplastic fragments can lead to a decrease in appetite, which reduces food intake and results in inadequate nutrient absorption, energy deficits, and growth restrictions (Deng et al., 2023). MPs and NPs can mimic the properties of native plastics and may therefore decompose more slowly in the environment. This may not only disrupt the balance of natural ecosystems but also have direct or indirect effects on human health. Studies have shown that invertebrates can accidentally ingest microplastics, which can cause damage to the animal's digestive tract, gastrointestinal obstruction, etc. MPs and NPs are extremely harmful to biota and may themselves cause sublethal effects (Battistin et al., 2023).
Maternal lung exposure to NPs results in the translocation of plastic particles to the placenta and fetal tissues and renders the fetal placental unit susceptible to adverse effects (Fournier et al., 2020). Induced molecular epigenetic changes can have transgenerational effects on multiple organ systems (Kumar, 2018). Furthermore, potential pathogens may be adsorbed to plastic debris and transported through plastic debris to various parts of the body (Keswani et al., 2016). The consumption of heated beverages results in the release of one million submicron and particle-sized particles per milliliter of leachate from the plastic material, and prolonged immersion or increased abrasion intensity increases the number of particles released (Liu et al., 2022). However, this is related to the outer packaging of the beverage; hot water immersion changes the chemical composition of PE plastic packaging but has a weaker effect on PP and PS. These findings suggest that plastic materials pose an unknown risk of human ingestion if they are regularly used to hold hot food or beverages.
In recent years, plastic fragments have been detected in various tissues of the human body. For example, blood (Leslie et al., 2022; Geppner et al., 2023), testis, semen (Zhao et al., 2023), lung (Amato-Lourenço et al., 2021), liver (Lin S. et al., 2022), kidney, colon (Ibrahim et al., 2020), intestine, integumentary system (Yan et al., 2021), cerebral cells (Prüst et al., 2020), heart and cardiovascular system (Yang Y. et al., 2023), bronchoalveolar lavage fluid (Fournier et al., 2020), feces, placenta, and breast milk (Liu et al., 2023b) can be used. We summarize the types and sizes of MPs and NPs in the tissues of different organs in the human body and how they are detected in Table 2. The nano- and subnanostructures in beverages are very small and may penetrate body tissues and organs (Liu L. et al., 2021). Upon the transfer of plastic fragments to body organs, especially the intestinal system, paracellular persorption and endocytosis may occur. This phenomenon may have wide-ranging implications for intracellular homeostasis and membrane toxicity (Danopoulos et al., 2020). The number of plastic particles found in the feces of patients with inflammatory bowel disease is significantly greater than that in the normal population because the consumption of beverages, bottled water, and dietary habits, as well as work and living conditions, can have a considerable effect on their number and composition (Angnunavuri et al., 2023). Considering the wide range of reactions that these MPs and NPs can induce in the human body, a human health risk assessment of granular plastics is necessary. However, there is a lack of consistency and standardization of current methods for the detection and identification of plastics. Further research is needed on exposure to MPs and NPs in the diet and beverages to reduce their accumulation and risk in human organs, as well as to provide systematic evidence and clear validation.
3.2 Toxicological profiles of MP and NP exposure
MPs and NPs in soil affect crop growth. Microplastics in the environment affect crop growth metabolically, microbiologically, and in the soil (Qiu et al., 2023). Furthermore, other stressors, such as extreme temperature changes, organic pollution, heavy metal pollution, nano-oxidized pollution, and greenhouse gases, can act synergistically with these factors to reduce their toxicity to agricultural crops. In general, MPs and NPs are characterized by relatively large surface areas and volumes and may leach into different processes through decomposition processes. More importantly, plastic particles can pass through the environment into aquatic biological systems. By studying aquatic systems and groundwater, Abduro Ogo et al. (2022) reported that MPs can enter aquatic organisms through the aquatic environment. The combined toxic effects of microplastics and lead on submerged macrophytes inhibit their physiological functions and destroy the entire flora and fauna of aquatic ecosystems. The presence of microplastics can potentially exacerbate the toxicity of heavy metals in aquatic plants, fishes, and shrimp (Gerhardt et al., 2020).
In animal experiments, microplastics not only severely disrupt aspects of the gut microbiota but also significantly disturb short-chain fatty acid levels (Tu et al., 2023). MPs have been found to have potential neurotoxic effects and harmful effects on development and growth in animal studies (Kauts et al., 2023). Kauts et al. reported that feeding causes MPs to accumulate in Drosophila, with larger doses having a greater effect on Drosophila motor coordination. Prolonged exposure to high levels of PET also resulted in slow development and a decreased survival rate in Drosophila. In studies of the cryptic rod nematode hidradenitis elegans, microplastic ingestion was found to inhibit acetylcholinesterase function and alter neurotransmitter levels, leading to behavioral abnormalities (Prüst et al., 2020). In addition, the addition of preservatives and additives to beverages for prolonged storage and transportation increases the toxicological effects of MPs and NPs (Curlej et al., 2023). MPs and NPs can adsorb and carry metals and are likely to be carriers of toxic heavy metals such as Cd, Pb, Bi or Hg, causing the accumulation of multiple toxins in organisms (Deng et al., 2023). The adsorption of heavy metals to MPs and NPs involves three steps: ionic mass transfer to the particle surface, internal transfer, and binding to adsorption sites (Li Y. et al., 2023). According to toxicological observations, heavy metal adsorption by plastic particles after the production of synergistic effects and MPs and NPs in nature can be exacerbated by increasing the bioavailability of different binding poisons to aggravate their toxicity; at the same time, poison and MP and NP desorption can be absorbed by organisms. In studies on plankton, polystyrene NPs have been shown to cause the overproduction of reactive oxygen species (ROS) and the activation of downstream pathways that inhibit growth, development, and reproduction (Liu Z. et al., 2020). Studies on human lymphocytes have shown that prolonged exposure to PVC MPs induces cellular toxicity, leading to oxidative stress and organelle damage (Salimi et al., 2022). Prolonged exposure causes polystyrene NPs to induce endothelial cell leakage via calcineurin dimers and may disrupt the mammalian blood–brain barrier. NPs can damage neuronal cells via lattice proteins. Binding to α-synuclein protofibrils exacerbates the spread of α-synuclein pathology in interconnected, vulnerable brain regions, leading to lysosomal damage, which can lead to Parkinson's disease and dementia (Liu Z. et al., 2023).
Exposure to plastic nanoparticles alters the microbiota, intestinal barrier permeability, oxidative stress, inflammation, neurotoxicity, and behavioral disorders, which in turn affects the digestive and nervous systems. Drinks and alcoholic beverages may contain MPs and NPs, which enter the human digestive tract through consumption and are distributed to various organs via the bloodstream or vascular epithelial cells. Studies have shown that ingested MPs can reach the lungs through the respiratory tract, leading to oxidative stress and inflammation. They may also be absorbed through gastrointestinal mucous membranes and are strongly associated with an increased risk of cardiovascular disease-related death (Vethaak and Legler, 2021). MPs can produce cytotoxic and inflammatory effects on lung epithelial cells by inducing the formation of ROS. Long-term exposure to MPs increases the risk of chronic obstructive pulmonary disease (Dong et al., 2020). MPs and NPs can enter the human circulatory system, including Peyer's patches and M cells, through endocytosis or paracellular diffusion. The ingestion and transport of these substances pose significant health risks, as adsorbed substances may be harmful. The accumulation and translocation of MPs and NPs after ingestion can result in serious consequences, including membrane damage, oxidative stress, genotoxicity, cancer, acute inflammation, and immune responses triggered by inhalation, ingestion, or dermal contact. In vivo modeling has shown that in the presence of microplastics, intestinal oxidative and inflammatory homeostasis was altered due to direct interactions of epithelial particles, which disrupted the intestinal microbiota, immune cytotoxicity, nutrient uptake, and impaired intestinal functions (Stapleton, 2021). The immune system is unable to routinely remove microplastic particles, thus increasing the risk of chronic inflammation and tumors after exposure.
3.3 Ecological toxicity of MPs and NPs in beverages and their effects
The pervasive use of plastics in modern society has led to their widespread distribution in the environment (Chae and An, 2017). PET packaging accounts for 44.7% of disposable beverage packaging in the U.S. and 12% of global solid waste (Baldridge et al., 2019). The presence of MPs and NPs in beverages not only poses risks to human health but also has broader environmental implications. Plastics used in the production and processing of beverages enter the environment (Menon et al., 2023). Owing to the slow disintegration process of synthetic plastic pollution, these particles remain in the aquatic environment for a longer period and become available to aquatic organisms. Plastic pollution in water can have long-term socioeconomic impacts, as it can alter water quality for future generations (Ravanbakhsh et al., 2022). Discarded beverage bottles are often found on the ground or in informal dumpsites and enter aquatic systems (Dias et al., 2020). Once plastic bottles are no longer reused, they are often discarded on beaches. When plastic debris reaches the open ocean, it will remain there for a long time and will be difficult to break down (Sohail et al., 2023). Wastewater generated during the washing and recycling of beverage bottles also contains trace amounts of plastic components, and this wastewater also ends up in the marine system, causing cumulative ecological harm (Dey et al., 2021). Plastic particles dissolved in the ocean can disrupt food webs and ecosystem dynamics by accumulating in organisms at different trophic levels, from zooplankton to top predators (Liu et al., 2023a). Moreover, the ingestion of microplastics by marine and terrestrial fauna can lead to physical injuries, reduced feeding efficiency, and impaired reproduction, thereby impacting population dynamics and biodiversity (Ullah et al., 2022). Additionally, the sorption of persistent organic pollutants (POPs) onto MPs and NPs can facilitate their transfer through the food chain, increasing the bioaccumulation and biomagnification of toxic compounds (Landrigan et al., 2020; Popli et al., 2022; Camacho Jimenez et al., 2023). POPs are chemical substances that are persistent and bioaccumulative (Ighalo et al., 2022). They have a combination of physical and chemical properties that make them capable of long-range transport, resistant to degradation and bioaccumulation, and are a serious environmental hazard worldwide. Furthermore, their physical and chemical properties may interfere with reproductive processes, behavior, and immune function in wildlife, ultimately impacting ecosystem stability and biodiversity (Kiran et al., 2022). The persistence of these plastics in the environment further exacerbates this issue, creating a cycle of contamination that is challenging to mitigate (Shruti et al., 2021). The highest levels of the polymer PP were found in the tidal flat sediments of Hangzhou Bay, where PP is the most important component of the outer packaging of beverages (Cai et al., 2023). This has led to the detection of MPs and NPs in large quantities of seafood and aquatic products and, for long periods of time, as invaders in the food chain (Osman et al., 2023).
Moreover, plastics in food and beverages contribute to the long-term persistence and bioaccumulation of MPs and NPs in the food web. Drinking bottles, plastic water cups, are the most significant type of marine plastic debris, and prolonged immersion and photodegradation lead to the dissolution of large quantities of MPs and NPs into seawater. In addition, they enter the food chain of aquatic organisms and bioaccumulate in their tissues, gradually rising to trophic levels as they are consumed by zooplankton, small fish, large fish and other organisms (Osman et al., 2023). For example, in aquatic invertebrates, MPs reduce feeding behavior and fecundity, slow larval growth and development, increase oxygen consumption, and stimulate the production of reactive oxygen species (Zolotova et al., 2022). MPs may cause structural damage to the gut, liver, gills, and brain in fish. In addition, MPs and NPs have the potential to directly affect human health, as they can enter the human food chain through the consumption of contaminated microplastics or other aquatic organisms. They accumulate in plankton, which constitute the lowest level of the food chain, and eventually migrate to higher predators (Lee et al., 2023). Furthermore, plastic is widely used in most beverage bottles, bottle caps, and plastic cups in our daily lives. Sooner or later, the entire food chain is contaminated with plastic (Wang et al., 2020). Microplastic contamination of beverages poses a multifaceted hazard, particularly in terms of interactions with POPs (Camacho Jimenez et al., 2023). When these plastics leach into beverages, they become vectors for the transportation and concentration of POPs, amplifying their detrimental effects on human health and the environment (Allan et al., 2021). Furthermore, the persistence and mobility of microplastics in the environment exacerbates the long-term consequences of POP contamination (Ormsby et al., 2024). Once released into the environment, these plastics can act as reservoirs for POPs, facilitating their transport over long distances and persisting in ecosystems for decades. This continuous cycle of contamination perpetuates the bioaccumulation of POPs in organisms and amplifies their impact on both terrestrial and aquatic ecosystems.
4 Methods for detecting MPs and NPs in beverages
Analytical techniques for detecting MP in beverages will become an issue of global consensus. The control of MPs in the food chain and drinking water is of particular concern because of the wide range of particle sizes, down to the nanometer scale, and the impact these particles can have on human health. In addition, larger MPs raise concerns about their related sources, occurrence, and fate (Bauer et al., 2022). The ability to detect the content and structure of MPs and NPs in beverages accurately and rapidly is highly important for regulating and combating plastic pollution.
There are many methods for the detection and identification of MPs and NPs. These methods include optical methods through optical microscopy (Wayman and Niemann, 2021), stereo microscopy (Panagiotidis et al., 2023; Kaushik et al., 2024), fluorescence microscopy (Monteleone et al., 2020; Giardino et al., 2023), scanning electron microscopy (SEM) (Gniadek and Dabrowska, 2019; Schmidt et al., 2021), quantum cascade laser microscopy (Primpke et al., 2020), atomic force microscopy (AFM) (Dazzi et al., 2015), spectroscopic methods through Raman spectroscopy (Anger et al., 2018; Guo et al., 2022; Yang H. et al., 2023), surface-enhanced Raman (Lee and Fang, 2022; Lin P. Y. et al., 2022), micro-Raman (Schymanski et al., 2021), laser direct infrared imaging (LDIR) (Nizamali et al., 2023), Fourier transform infrared spectroscopy (FTIR) (Jenner et al., 2022), hyperspectral imaging (Huang et al., 2021; Xu et al., 2021), terahertz spectroscopy (Park and Ahn, 2022), and thermal analysis methods through pyrolysis gas chromatography–mass spectrometry.
We present several detection methods that have been widely used thus far and summarize their characteristics, advantages, and limitations in Table 3. Optical detection methods are commonly used because they are inexpensive and intuitive; however, bulky and time-consuming instruments have limitations. Microscopic examination is virtually cost free, but it is time-consuming, requires more complex sample preparation, and is prone to missed detections when testing beverages. The accuracy and sensitivity of the test are low, and it is suitable for primary testing in the food industry. However, some of the sensitivity can be improved by fluorescence staining (Nile Red staining), but this method still takes a long time. Scanning electron microscopy (SEM) detection and atomic force microscopy can greatly improve accuracy, but the detection equipment requires specialized technicians and is less feasible for industrial use (Dazzi et al., 2015). Spectroscopy is currently the most used method for detecting plastics in food and beverages. One-time detection of 5,000 particles can be realized via Raman spectroscopy (Qian et al., 2024), which is a very efficient method. It has the advantages of high sensitivity, a high recovery rate and is nondestructive, but its high cost is a disadvantage. Laser direct infrared imaging (LDIM) can also realize the efficient detection of MPs and NPs, which can be applied to large-scale screening in the food industry. Liquid chromatography–mass spectrometry (LC–MS) has ultrahigh sensitivity and accuracy and can be customized to the width range of the particles; however, detection is time-consuming, requires large instruments and professional technicians, and is expensive, so it is only used for accurate detection of trace amounts (Elseblani et al., 2023). Thermal analysis relies on large instruments and requires specialized personnel to operate and interpret them, but it remains one of the most accurate methods of detection. Detection through a combination of sensor technology (microfluidics) is a portable and rapid detection method. It is dedicated to screening a wide range of food and beverage or primary screening for food quality engineering. Although the sensitivity and accuracy of these methods are average, but because of their low price and simple operation advantages, the future is hopeful for becoming a new trend in the detection of plastics in food and beverages. The ability to quickly and accurately detect MPs and NPs can facilitate environmental management and health regulation. How to reduce costs properly and minimize false-positive and false-negative results will be an area of focus for future research. Furthermore, there is a need to address and summarize the harmless disposal of samples and secondary contamination.
5 Prevention and challenges of MP and NP contamination in beverages
Food and beverages are inseparable from plastic packaging and have become convenient because of plastic products (Huang et al., 2021). In an era where beverage consumption is on the rise, ensuring the safety and purity of these products is paramount (Sohail et al., 2023). With the emergence of MPs and NPs as potential contaminants in beverages, it becomes imperative to address future solution strategies, anticipate challenges, and implement preventive measures to safeguard public health. First, investing in advanced filtration systems capable of efficiently removing MPs and NPs from beverages can significantly mitigate contamination risks (Dey et al., 2023). Innovative filtration methods such as nanofiltration, reverse osmosis (RO), and forward osmosis (FO) show promise in effectively eliminating microscopic plastic particles. Owing to the small size of MPs, most MPs can be filtered out via microfiltration, ultrafiltration, and nanofiltration systems. Nanofiltration is considered one of the advanced and promising wastewater treatment methods commonly used today because it can effectively remove inorganic salts, organics, bacteria, and large particulate matter from wastewater and ensure the safety of the effluent (Lin et al., 2024). RO technology is widely used in water treatment systems in the food and pharmaceutical industries (Choi et al., 2024). Water purification uses nonporous or nanofiltration membranes to remove heavy metals, plastic particles, and other impurities from the water. This method can remove MPs and NPs from a liquid to a large extent by applying high pressure to the concentrated liquid and forcing the liquid through a semipermeable membrane, leaving all other substances in the more concentrated aqueous solution. The FO system uses osmotic pressure differentials to drive water from the feed solution across the FO membrane to the extraction solution without the use of hydraulics (Golgoli et al., 2023). This method can separate plastic particles from water in large quantities; however, the performance of FO membranes and materials largely affects the technical feasibility and practical application of the process. However, the main obstacles to the widespread use of both approaches are membrane clogging and membrane fouling. If this drawback can be solved, reducing MPs and NPs will be the best way. Second, collaborating with material scientists to develop packaging materials that are less prone to leaching microplastics into beverages can be instrumental. Biodegradable and compostable packaging alternatives can help reduce the environmental burden of plastic pollution while ensuring beverage safety (Satti and Shah, 2020). In the current scenario, there is a need for the development of plastic materials that are biodegradable, biocompatible, sustainable, and eco-friendly with low/nontoxic degradation products. Traditional plastics are widely used because of their durability and barrier properties; however, they are not biodegradable and can be disposed of only by live incineration in landfills, which still places considerable pressure on the environment. Despite the advantages of biodegradable plastics, they face high prices and low production volumes and are difficult to scale up, and there is still a need for continued research to address the challenges and limitations associated with these plastics to develop a sustainable solution. In addition, educating consumers about the risks associated with microplastic contamination in beverages and encouraging responsible consumption habits can increase the demand for cleaner, safer products. Transparent labeling practices that disclose information about packaging materials and quality assurance measures can empower consumers to make informed choices. Finally, implementing rigorous quality control measures throughout the beverage production process, from sourcing raw materials to packaging, can help detect and mitigate potential contamination (Igalavithana et al., 2022). Regular testing for MPs and NPs via sensitive analytical techniques such as spectroscopy and microscopy can aid in maintaining product integrity.
5.1 Strengthening the supervision of beverage product safety
Based on the above strategies, several challenges remain. First, most plastic products pose a serious threat to the environment and deplete natural resources, limiting their use. Currently, there is a lack of stringent testing standards and regulatory systems for MP and NP contents in beverages (Kudzin et al., 2023). While some regions, such as the EU, are advancing precautionary policies with initiatives like the European Green Deal, formal limits for MPs and NPs in beverages are still under development. In the US, federal regulations are lacking, though state-level efforts and EPA research are paving the way for future standards. Countries like China and Australia are focusing on reducing plastic waste and researching MPs and NPs contamination, but comprehensive regulations for beverages are still emerging. To better understand the regulatory landscape, Table 4 provides a summary of key regulations, guidelines, and ongoing research initiatives concerning MPs and NPs in beverages across different regions. MPS and NPs contamination in beverages varies across regions due to differences in industrial practices, regulatory frameworks, and waste management policies. In Europe, particularly in bottled water, higher concentrations of MPs have been detected compared to North America, likely due to differences in water sourcing and processing techniques. The European Union has taken a precautionary approach, with ongoing research led by the European Food Safety Authority (EFSA) to establish regulatory standards. The levels of contamination in different types of beverages in different regions are summarized in Table 5, showing the levels of contamination in each region, thus reinforcing the need for global and region-specific regulatory frameworks to address MPs and NPs in beverages. Research on plastic debris in beverages should be multifaceted to include biological and chemical loading, as well as the role of microbial hitchhikers in mitigating the problem through biodegradation or exacerbating it through increased biofilm binding (Keswani et al., 2016). Attempts are also being made to establish a more accurate risk assessment of plastic debris, which could also help by considering the impact of potentially harmful plastic-associated microbial and chemical copolymers. One study, which collected statistics on whether consumers would choose sustainable food packaging, reported that they had limited knowledge of the practical implementation of recyclability, biodegradability, reusability and other environmental impacts (Otto et al., 2021). The sustainable purchasing behavior of consumers can potentially be supported by clearly printed product and packaging information on food and beverage packaging, as well as by awareness training to encourage sustainable behavior. The World Health Organization noted the inconsistency of data currently available in the literature and the lack of standardization of testing methods and data reporting and recommended continued protection of source waters against the possible presence of particulate plastics and other contaminants of concern in drinking water (World Health Organization, 2019). In 2000 (European Commission, 2000), the European Commission issued a Green Paper on PVC wastes, which addresses the management of PVC wastes and assesses various environmental and health aspects and the possibilities for reducing their environmental impact. The World Health Assembly in 2023 called on Member States to support the WHO in expanding its work on plastics and health and to contribute to the work of the Intergovernmental Negotiating Committee to develop an internationally binding document on plastic pollution (World Health Organization, 2023). In May 2024, the European Union issued Commission Authorization Resolution (EU) 2024/1441, which establishes a methodology for measuring microplastics in water intended for human consumption. The regulation requires that particles and fibers in drinking water be collected using a filter cascade, followed by optical microscopy or chemically mapped imaging to determine particle size and shape. Vibrational microspectroscopy is then used to identify the composition of these particles. This comprehensive approach ensures accurate detection and classification of microplastics, reflecting the EU's commitment to addressing their impact on drinking water. The regulation marks a significant breakthrough in the international detection of MPs and NPs. To better understand microplastic exposure, there is a need for quality assurance methodologies, harmonized reporting standards, and improved identification of microplastic concentration, shape, size, and composition throughout the human drinking water supply chain (European Commission, 2024). Therefore, more systematic quantification of the occurrence of NP and MP particles is strongly recommended, and national and international standards need to be established to limit the number of MPs and NPs in beverages (World Health Organization, 2019). In the future, countries around the world should specify standards for the detection and production of MPs and NPs in the beverage industry based on the World Health Organization's industry standards, which will greatly control the sources and emissions of microplastics.
5.2 Development of new energy sources or more environmentally friendly alternatives
The development of relevant food contaminant technologies and legislation is also critical (Vivekanand et al., 2021). It will take generations and is an enormous challenge to implement worldwide. Using biodegradable plastics, such as natural cellulose and its derivatives, polylactic acid (Kudzin et al., 2023) and nanofiber materials (Jiang et al., 2024) have been developed. However, they are also broken down into tiny plastic fragments that end up in the ocean if not treated thoroughly. The use of plastics in the beverage and food markets continues to develop on a large scale, so microplastic pollution remains a long-term problem (Zhang et al., 2022). Furthermore, long-term studies of seawater and extensive research are needed to determine the exact impact of plastics in beverages on the environment and the health of living organisms (Shen et al., 2020). Only then will we be able to obtain accurate impact data and make changes and actions accordingly. The use of recyclable metals or recyclable pulp as an alternative to beverage packaging can also reduce the production and distribution of plastic products but still has some disadvantages, such as a short shelf life, difficulty storing, perishability, and high cost (Vivekanand et al., 2021). If new materials can be developed or improved based on plastic as a substitute for plastic and to solve the problems of production costs, it will be the best way to address MP and NP pollution. Encouraging collaboration among industry stakeholders, scientific institutions, and policymakers fosters a collective approach to addressing the complex issue of microplastic contamination in beverages. Sharing knowledge, best practices, and resources can accelerate progress toward sustainable solutions. Moreover, methods for testing microplastics in government and other functional organizations need to be ordered and prescribed (Sorensen et al., 2022). In addition, society should be educated about some of the long-term effects of plastic products, which continue to produce MPs and NPs on the impact of new products on the environment, ecology, and human health.
6 Future perspectives and conclusions
The safety issues associated with MPs and NPs released from food and beverage contact materials have raised significant concerns about their potential impact on human health. This review summarizes the various aspects related to plastic particles released from beverage packaging and their potential harm to human health and the environment. First, MPs and NPs come mainly from the outer packaging of food and beverages. Beverage bottles, bottle caps, plastic paper, paper cups, etc., may become important sources of plastic particles through dissolution, temperature variation, and mechanical stress. Most of the polymers that make up beverage packaging also determine the composition of the MPs and NPs in the environment, such as PE, PET, PP, PVC, and PS. They can be released into beverages and food, leading to human exposure. There is substantial evidence that humans are exposed to MPs and NPs when they touch the mouths of beverage bottles and when they drink beverages. MPs and NPs are immeasurably harmful to human health, as they can be transported through the bloodstream to various organs and systems of the body and are difficult to metabolize or eliminate from the body. In addition to the harm to human health caused by the plastic pellets themselves, they can also synergize with disease-causing factors such as heavy metals and pathogens, which can seriously affect human health. More evidence has shown that MPs and NPs are also present in large quantities in ecological environments, especially in marine and aquatic environments. This has also led to the disruption of food webs and food chains.
By outlining the toxic effects of MPs and NPs from beverages and their derived industries in the ecosystem and in the health of organisms, several aspects have been characterized. First, the large number of plastic bottles generated by the beverage industry results in a large amount of plastic waste, and the ecological impacts of these plastic particles result from natural accumulation through physical decomposition over a long period of time, which is significant for the oceans and aquatic fauna and accumulates along the food chain. Second, humans ingest large amounts of NPs through beverage bottles, bottle caps, paper cups, etc., and these accumulate in the human body in large numbers. Finally, MPSs and NPs in beverages are difficult to detect and breakdown, making them susceptible to a vicious cycle in humans and nature.
Stricter regulatory and legal controls could be crucial in addressing the issue of excessive MPs and NPs contamination in beverages at its source. Effective detection methods, allowing for rapid and accurate identification of MPs and NPs, are key to improving both environmental management and health regulation. From a food safety perspective, enhancing the regulatory oversight of beverage packaging could mitigate the risks posed by MPs and NPs. Implementing rigorous national or international standards, promoting recycling, and ensuring the responsible disposal of packaging materials could significantly reduce plastic contamination. However, the effectiveness of these strategies will depend on global cooperation and consistent enforcement across regions, particularly in countries with varying levels of regulatory development. Analyzed from a new energy perspective, developing new environmentally friendly alternative sources of energy is a better way to address the root of the problem. If experts can develop new materials that can replace plastics, are airtight, malleable, easily accessible, biophilic, and easily degradable, they will be a better choice. Although some new materials, such as nanofibers, can replace plastic bottle packaging, they are expensive and cannot be mass produced. If the problem of high cost can be solved, it will be promising for future development and use in the food and beverage industry. In the future, further examination of safety issues surrounding the release of MPs and NPs from beverage contact materials, the need to raise awareness and take measures to reduce potential health risks, and the protection of food and beverages and people's health through improved and safer beverage packaging will be necessary.
Author contributions
CM: Conceptualization, Data curation, Investigation, Methodology, Validation, Writing – original draft. KR: Conceptualization, Funding acquisition, Methodology, Supervision, Visualization, Writing – review & editing. GJ: Funding acquisition, Investigation, Methodology, Supervision, Visualization, Writing – review & editing.
Funding
The author(s) declare financial support was received for the research, authorship, and/or publication of this article. This study was supported by Innovation and Entrepreneurship Training Program for University Students (S202413706034 and 2023CXXL027) Jilin Province, China and Foundation of the Education Department of Jilin Province, China (No. JJKH20230539KJ).
Conflict of interest
The authors declare that the research was conducted in the absence of any commercial or financial relationships that could be construed as a potential conflict of interest.
Publisher's note
All claims expressed in this article are solely those of the authors and do not necessarily represent those of their affiliated organizations, or those of the publisher, the editors and the reviewers. Any product that may be evaluated in this article, or claim that may be made by its manufacturer, is not guaranteed or endorsed by the publisher.
References
Abbasi, S., Razeghi, N., Yousefi, M. R., Podkoscielna, B., and Oleszczuk, P. (2023). Microplastics identification in water by TGA-DSC method: Maharloo Lake, Iran. Environm. Sci. Pollut. Res. 30, 67008–67018. doi: 10.1007/s11356-023-27214-8
Abduro Ogo, H., Tang, N., Li, X., Gao, X., and Xing, W. (2022). Combined toxicity of microplastic and lead on submerged macrophytes. Chemosphere 295:133956. doi: 10.1016/j.chemosphere.2022.133956
Akanyange, S. N., Zhang, Y., Zhao, X., Adom-Asamoah, G., Ature, A.-R. A., Anning, C., et al. (2022). A holistic assessment of microplastic ubiquitousness: pathway for source identification in the environment. Sustain. Prod. Consump. 33, 113–145. doi: 10.1016/j.spc.2022.06.020
Allan, J., Belz, S., Hoeveler, A., Hugas, M., Okuda, H., Patri, A., et al. (2021). Regulatory landscape of nanotechnology and nanoplastics from a global perspective. Regulat, Toxicol. Pharmacol. 122:104885. doi: 10.1016/j.yrtph.2021.104885
Altunışık, A. (2023). Prevalence of microplastics in commercially sold soft drinks and human risk assessment. J. Environm. Managem. 336:117720. doi: 10.1016/j.jenvman.2023.117720
Amato-Lourenço, L. F., Carvalho Oliveira, R., Júnior, G. R., dos Santos Galvão, L., Ando, R. A., and Mauad, T. (2021). Presence of airborne microplastics in human lung tissue. J. Hazardous Mater. 416:126124. doi: 10.1016/j.jhazmat.2021.126124
Anderson, L., and Gbor, N. (2024). Plastic Waste in Australia and the Recycling Greenwash. The Australia Institute Research that Matters. Available at: https://australiainstitute.org.au/wp-content/uploads/2024/01/P1482-Plastic-Waste-in-Australia-Web.pdf
Andjelkovic, T., Bogdanovic, D., Kostic, I., Kocic, G., Nikolic, G., and Pavlovic, R. (2021). Phthalates leaching from plastic food and pharmaceutical contact materials by FTIR and GC-MS. Environm. Sci. Pollut. Res. 28, 31380–31390. doi: 10.1007/s11356-021-12724-0
Anger, P. M., von der Esch, E., Baumann, T., Elsner, M., Niessner, R., and Ivleva, N. P. (2018). Raman microspectroscopy as a tool for microplastic particle analysis. Trends Analyt. Chem. 109, 214–226. doi: 10.1016/j.trac.2018.10.010
Angnunavuri, P. N., Attiogbe, F., and Mensah, B. (2023). Particulate plastics in drinking water and potential human health effects: current knowledge for management of freshwater plastic materials in Africa. Environm. Pollut. 316:120714. doi: 10.1016/j.envpol.2022.120714
Arijeniwa, V. F., Akinsemolu, A. A., Chukwugozie, D. C., Onawo, U. G., Ochulor, C. E., Nwauzoma, U. M., et al. (2024). Closing the loop: a framework for tackling single-use plastic waste in the food and beverage industry through circular economy- a review. J. Environm. Managem. 359:120816. doi: 10.1016/j.jenvman.2024.120816
Australian Government (2019). National Waste Policy Action Plan 2019. Available at: https://www.environment.gov.au/protection/waste-resource-recovery/national-waste-policy (accessed 2 October, 2024).
Aydin, R. B., Yozukmaz, A., Sener, I., Temiz, F., and Giannetto, D. (2023). Occurrence of microplastics in most consumed fruits and vegetables from turkey and public risk assessment for consumers. Life 13:1686. doi: 10.3390/life13081686
Baeza-Martínez, C., Olmos, S., González Pleiter, M., López Castellanos, J., García Pachón, E., Masiá Canuto, M., et al. (2022). First evidence of microplastics isolated in European citizens' lower airway. J. Hazard. Mater. 438:129439. doi: 10.1016/j.jhazmat.2022.129439
Baldridge, A. S., Huffman, M. D., Taylor, F., Xavier, D., Bright, B., Van Horn, L. V., et al. (2019). The healthfulness of the US packaged food and beverage supply: a cross-sectional study. Nutrients 11, 2–15. doi: 10.3390/nu11081704
Bandy, L., Jewell, J., Luick, M., Rayner, M., Li, Y., Shats, K., et al. (2023). The development of a method for the global health community to assess the proportion of food and beverage companies' sales that are derived from unhealthy foods. Globaliz. Health 19, 2–10. doi: 10.1186/s12992-023-00992-z
Battistin, G., Latella, L., and Iannilli, V. (2023). Microplastic pollution in the food web: observation of ingestion by the talitrid amphipod Cryptorchestia garbinii on the shores of Lake Garda. Eur. Zool. J. 90, 73–82. doi: 10.1080/24750263.2022.2160019
Bauer, J., Miclea, P. T., Braun, U., Altmann, K., Turek, M., and Hagendorf, C. (2022). “Microplastic detection and analysis in water samples,” in Sustainable Energy Water Environment Nexus in Deserts, 111–113. doi: 10.1007/978-3-030-76081-6_12
Bi, X., Davis, B. J. K., Barraj, L. M., Srinivasan, D., Mahadev, P., Mathew, P., et al. (2023). Beverage consumption patterns among U.S. adolescents and adults from a new 24-h beverage recall survey compared to the national health and nutrition examination survey (NHANES) 2017–2018. Nutrients 15:16. doi: 10.3390/nu15163561
Cai, X., Chen, H., Cheng, J., Huang, B., Jin, B., and Lu, J. (2023). Coupling of microplastic contamination in organisms and the environment: evidence from the tidal flat ecosystem of Hangzhou Bay, China. J. Hazardous Mater. 457:131838. doi: 10.1016/j.jhazmat.2023.131838
Camacho Jimenez, L., Gonzalez Ruiz, R., and Yepiz Plascencia, G. (2023). Persistent organic pollutants (POPs) in marine crustaceans: Bioaccumulation, physiological and cellular responses. Marine Environm. Res. 192:106184. doi: 10.1016/j.marenvres.2023.106184
Canadian Environmental Protection Act (CEPA) (2021). Plastics as a Toxic Substance. Available at: https://www.canada.ca/en/environment-climate-change/services/canadian-environmental-protection-act-registry/plastics-toxic-substance.html (accessed 2 October, 2024).
Chae, Y., and An, Y.-J. (2017). Effects of micro- and nanoplastics on aquatic ecosystems: current research trends and perspectives. Marine Pollut. Bullet. 124, 624–632. doi: 10.1016/j.marpolbul.2017.01.070
Chen, H., Xu, L., Yu, K., Wei, F., and Zhang, M. (2023). Release of microplastics from disposable cups in daily use. Sci. Total Environm. 854:158606. doi: 10.1016/j.scitotenv.2022.158606
Chen, J., Chen, G., Peng, H., Qi, L., Zhang, D., Nie, Q., et al. (2023). Microplastic exposure induces muscle growth but reduces meat quality and muscle physiological function in chickens. Sci. Total Environm. 882:163305. doi: 10.1016/j.scitotenv.2023.163305
Chen, Y., Xu, H., Luo, Y., Ding, Y., Huang, J., Wu, H., et al. (2023). Plastic bottles for chilled carbonated beverages as a source of microplastics and nanoplastics. Water Res. 242:120243. doi: 10.1016/j.watres.2023.120243
Choi, S. J., Osman, A., Kim, S., Kang, S., and Hwang, D. S. (2024). Adsorptive chito-beads for control of membrane fouling. Carbohyd. Polymers 327. doi: 10.1016/j.carbpol.2023.121642
Circular Economy Action Plan (2020). Available at: https://commission.europa.eu/strategy-and-policy/priorities-2019-2024/european-green-deal_en
Corrêa, T. R., Gaylarde, C. C., Baptista Neto, J. A., Delgado, J. d. F., Lima, L. d. S., Cunha, D. L., et al. (2023). The impact of microplastics on global food production: a brief overview of this complex sector. Microplastics 2, 371–388. doi: 10.3390/microplastics2040028
Correia, P. J., da Costa, J., Lopes, I., Duarte, A., and Santos, T. (2022). Environmental exposure to microplastics: An overview on possible human health. Sci. Total Environm. 702:134455. doi: 10.1016/j.scitotenv.2019.134455
Curlej, J., Zajac, P., Capla, J., and Hleba, L. (2023). Safety issues of microplastics released from food contact materials. J. Microbiol. Biotechnol. Food Sci. 13:e10317. doi: 10.55251/jmbfs.10317
Danopoulos, E., Twiddy, M., and Rotchell, J. M. (2020). Microplastic contamination of drinking water: a systematic review. PLoS ONE 15:e0236838. doi: 10.1371/journal.pone.0236838
Dazzi, A., Saunier, J., Kjoller, K., and Yagoubi, N. (2015). Resonance enhanced AFM-IR: a new powerful way to characterize blooming on polymers used in medical devices. Int. J. Pharmaceut. 484, 109–114. doi: 10.1016/j.ijpharm.2015.02.046
De la Torre, G. E. (2020). Microplastics: an emerging threat to food security and human health. J. Food Sci. Technol. 57, 1601–1608. doi: 10.1007/s13197-019-04138-1
Deng, Y., Wu, J., Chen, J., and Kang, K. (2023). Overview of microplastic pollution and its influence on the health of organisms. J. Environm. Sci. Health 58, 412–422. doi: 10.1080/10934529.2023.2190715
Dey, A., Dhumal, C. V., Sengupta, P., Kumar, A., Pramanik, N. K., and Alam, T. (2021). Challenges and possible solutions to mitigate the problems of single-use plastics used for packaging food items: a review. J. Food Sci. Technol. 58, 3251–3269. doi: 10.1007/s13197-020-04885-6
Dey, T. K., Rasel, M., Roy, T., Uddin, M. E., Pramanik, B. K., and Jamal, M. (2023). Post-pandemic micro/nanoplastic pollution: toward a sustainable management. Sci. Total Environm. 867:161390. doi: 10.1016/j.scitotenv.2023.161390
Di Bello, D., Junker, T., Zambianchi, E., Monticelli, D., and Ugoccioni, G. (2021). Microplastics in fruit juices: a preliminary study on their occurrence and potential exposure risks. J. Hazard. Mater. 415:125585.
Dias, J. M., Duncan, E. M., Davies, A., Brooks, A., Chowdhury, G. W., Godley, B. J., et al. (2020). Message in a bottle: open source technology to track the movement of plastic pollution. PLoS ONE 15:e0242459. doi: 10.1371/journal.pone.0242459
Diaz-Basantes, M. F., Conesa, J. A., and Fullana, A. (2020). Microplastics in honey, beer, milk and refreshments in ecuador as emerging contaminants. Sustainability 12:5514. doi: 10.3390/su12145514
Dong, C. D., Chen, C. W., Chen, Y. C., Chen, H. H., Lee, J. S., and Lin, C. H. (2020). Polystyrene microplastic particles: In vitro pulmonary toxicity assessment. J. Hazard. Mater. 385:121575. doi: 10.1016/j.jhazmat.2019.121575
Ece, E., Haciosmanoglu, N., and Inci, F. (2023). Microfluidics as a ray of hope for microplastic pollution. Biosensors 13, 322–342. doi: 10.3390/bios13030332
Elseblani, R., Cobo Golpe, M., Godin, S., Jimenez-Lamana, J., Fakhri, M., Rodriguez, I., et al. (2023). Study of metal and organic contaminants transported by microplastics in the Lebanese coastal environment using ICP MS, GC-MS, and LC-MS. Sci. Total Environm. 887:164111. doi: 10.1016/j.scitotenv.2023.164111
Environmental Protection Agency (EPA) (2022). Trash-Free Waters Program: Microplastics. Available at: https://www.epa.gov/trash-free-waters (accessed 2 October 2024).
European Commission (2000). Green Paper - Environmental Issues of PVC. Available at: https://op.europa.eu/en/publication-detail/-/publication/6d404501-bda4-4d6d-a38c-68a29e3170a9/language-en (accessed 26 July, 2000).
European Commission (2020). A new Circular Economy Action Plan: For a cleaner and more competitive Europe. Available at: https://ec.europa.eu/environment/circular-economy/ (accessed 2 October 2024).
European Commission (2024). Supplementing Directive (EU) 2020/2184 of the European Parliament and of the Council by Laying Down a Methodology to Measure Microplastics in Water Intended for Human Consumption (Brussels Member States: (EU) 2024/1441).
European Food Safety Authority (2021). EFSA Scientific Colloquium 25-A coordinated approach to assess the human health risks of micro- and nanoplastics in food. EFSA Support. Publ. 18:6815E. doi: 10.2903/sp.efsa.2021.EN-6815
Fernandez-Arribas, J., Moreno, T., and Eljarrat, E. (2023). Human exposure to organophosphate esters in water and packed beverages. Environm. Int. 175:107936. doi: 10.1016/j.envint.2023.107936
Food Safety Commission of Japan (FSCJ) (2022). Evaluation of the Health Risks of Microplastics in Food. Available at: https://www.fsc.go.jp (accessed 2 October, 2024).
Fournier, S. B., D'Errico, J. N., Adler, D. S., Kollontzi, S., Goedken, M. J., Fabris, L., et al. (2020). Nanopolystyrene translocation and fetal deposition after acute lung exposure during late-stage pregnancy. Particle Fibre Toxicol. 17, 55–74. doi: 10.1186/s12989-020-00385-9
Geppner, L., Ramer, G., Tomasetig, D., Grundhöfer, L., Küss, J., Kaup, M., et al. (2023). A novel enzymatic method for isolation of plastic particles from human blood. Environm. Toxicol. Pharmacol. 104:104318. doi: 10.1016/j.etap.2023.104318
Gerhardt, A., Schaefer, M., Blum, T., and Honnen, W. (2020). Toxicity of microplastic particles with and without adsorbed tributyltin (TBT) in Gammarus fossarum (Koch, 1835). Fundam. Appl. Limnol. 194, 57–65. doi: 10.1127/fal/2019/1114
Geyer, R. (2020). “A brief history of plastics,” in Mare Plasticum The Plastic Sea.), 31–47. doi: 10.1007/978-3-030-38945-1_2
Geyer, R., Jambeck, J. R., and Law, K. L. (2017). Production, use, and fate of all plastics ever made. Sci. Adv. 19:e1700782.
Giardino, M., Balestra, V., Janner, D., and Bellopede, R. (2023). Automated method for routine microplastic detection and quantification. Sci. Total Environm. 859:160036. doi: 10.1016/j.scitotenv.2022.160036
Gniadek, M., and Dabrowska, A. (2019). The marine nano- and microplastics characterisation by SEM-EDX: The potential of the method in comparison with various physical and chemical approaches. Marine Pollut. Bullet. 148, 210–216. doi: 10.1016/j.marpolbul.2019.07.067
Golgoli, M., Farahbakhsh, J., Asif, A. H., Khiadani, M., Razmjou, A., Johns, M. L., et al. (2023). Harnessing the power of metal-organic frameworks to develop microplastic fouling resistant forward osmosis membranes. J. Membrane Sci. 682:121766. doi: 10.1016/j.memsci.2023.121766
Guo, X., Lin, H., Xu, S., and He, L. (2022). Recent advances in spectroscopic techniques for the analysis of microplastics in food. J. Agricult. Food Chem. 70, 1410–1422. doi: 10.1021/acs.jafc.1c06085
Health Canada (2023). Guidelines for Microplastic Monitoring in Drinking Water. Available at: https://www.canada.ca/en/health-canada/services/environmental-workplace-health/water-quality.html (accessed 2 October, 2024).
Huang, H., Qureshi, J. U., Liu, S., Sun, Z., Zhang, C., and Wang, H. (2021). Hyperspectral imaging as a potential online detection method of microplastics. Bull. Environ. Contam. Toxicol. 107, 754–763. doi: 10.1007/s00128-020-02902-0
Huang, Y., Wong, K. K., Li, W., Zhao, H., Wang, T., Stanescu, S., et al. (2022). Characteristics of nano-plastics in bottled drinking water. J. Hazard. Mater. 424:127404. doi: 10.1016/j.jhazmat.2021.127404
Ibrahim, Y. S., Tuan Anuar, S., Azmi, A. A., Wan Mohd Khalik, W. M. A., Lehata, S., Hamzah, S. R., et al. (2020). Detection of microplastics in human colectomy specimens. An open access. J. Gastroenterol. Hepatol. 5, 116–121. doi: 10.1002/jgh3.12457
Igalavithana, A. D., Yuan, X., Attanayake, C. P., Wang, S., You, S., Tsang, D. C. W., et al. (2022). Sustainable management of plastic wastes in COVID-19 pandemic: the biochar solution. Environm. Res. 212:113495. doi: 10.1016/j.envres.2022.113495
Ighalo, J. O., Yap, P. S., Iwuozor, K. O., Aniagor, C. O., Liu, T., Dulta, K., et al. (2022). Adsorption of persistent organic pollutants (POPs) from the aqueous environment by nano-adsorbents: a review. Environm. Res. 212:113123. doi: 10.1016/j.envres.2022.113123
Janani, R., Bhuvana, S., Geethalakshmi, V., Jeyachitra, R., Sathishkumar, K., Balu, R., et al. (2024). Micro and nano plastics in food: a review on the strategies for identification, isolation, and mitigation through photocatalysis, and health risk assessment. Environ. Res. 241:117666. doi: 10.1016/j.envres.2023.117666
Jenner, L. C., Rotchell, J. M., Bennett, R. T., Cowen, M., Tentzeris, V., et al. (2022). Detection of microplastics in human lung tissue using muFTIR spectroscopy. Sci. Total Environm. 831:154907. doi: 10.1016/j.scitotenv.2022.154907
Jiang, G., Ramachandraiah, K., and Zhao, C. (2024). Advances in the development and applications of nanofibers in meat products. Food Hydrocolloids 146:109210. doi: 10.1016/j.foodhyd.2023.109210
Joseph, A., Parveen, N., Ranjan, V. P., and Goel, S. (2023). Drinking hot beverages from paper cups: lifetime intake of microplastics. Chemosphere 317:137844. doi: 10.1016/j.chemosphere.2023.137844
Karačonji, I. B., Jurica, S. A., Lasić, D., and Jurica, K. (2017). Facts about phthalate toxicity in humans and their occurrence in alcoholic beverages. Arch. Indust. Hygiene Toxicol. 68, 81–92. doi: 10.1515/aiht-2017-68-2951
Kashfi, F. S., Mohammadi, A., Rostami, F., Savari, A., De-la-Torre, G. E., Spitz, J., et al. (2023). Microplastics and phthalate esters release from teabags into tea drink: occurrence, human exposure, and health risks. Environm. Sci. Pollut. Res. 30, 104209–104222. doi: 10.1007/s11356-023-29726-9
Kaur, R., Shekhar, S., and Prasad, K. (2024). Functional beverages: recent trends and prospects as potential meal replacers. Food Mater. Res. 4:41. doi: 10.48130/fmr-0023-0041
Kaushik, A., Gupta, P., Kumar, A., Saha, M., Varghese, E., Shukla, G., et al. (2024). Identification and physico-chemical characterization of microplastics in marine aerosols over the northeast Arabian Sea. Sci. Total Environm. 912:168705. doi: 10.1016/j.scitotenv.2023.168705
Kauts, S., Mishra, Y., Yousuf, S., Bhardwaj, R., Singh, S. K., Alshabrmi, F. M., et al. (2023). Toxicological profile of polyethylene terephthalate (PET) microplastic in ingested drosophila melanogaster (Oregon R+) and Its adverse effect on behavior and development. Toxics 11:782. doi: 10.3390/toxics11090782
Keerthana Devi, M., Karmegam, N., Manikandan, S., Subbaiya, R., Song, H., Kwon, E. E., et al. (2022). Removal of nanoplastics in water treatment processes: a review. Sci. Total Environm. 845:157168. doi: 10.1016/j.scitotenv.2022.157168
Keswani, A., Oliver, D. M., Gutierrez, T., and Quilliam, R. S. (2016). Microbial hitchhikers on marine plastic debris: Human exposure risks at bathing waters and beach environments. Marine Environm. Res. 118, 10–19. doi: 10.1016/j.marenvres.2016.04.006
Khant, N. A., and Kim, H. (2022). Review of current issues and management strategies of microplastics in groundwater environments. Water 14, 1020–1036. doi: 10.3390/w14071020
Kiran, B. R., Kopperi, H., and Venkata Mohan, S. (2022). Micro/nano-plastics occurrence, identification, risk analysis and mitigation: challenges and perspectives. Rev. Environm. Sci. Bio-Technol. 21, 169–203. doi: 10.1007/s11157-021-09609-6
KIT (2024). Matmatch 2.0: we bring FAIR Material Data for All. Available at: https://matmatch.com/ (accessed May 14, 2024).
Kosuth, M., Mason, S. A., and Wattenberg, E. V. (2018). Anthropogenic contamination of tap water, beer, and sea salt. PLOS ONE 13:e0194970. doi: 10.1371/journal.pone.0194970
Kudzin, M. H., Piwowarska, D., Festinger, N., and Chruściel, J. J. (2023). Risks associated with the presence of polyvinyl chloride in the environment and methods for its disposal and utilization. Materials 17, 173–204. doi: 10.3390/ma17010173
Kumar, P. (2018). Role of plastics on human health. Indian J. Pediat. 85, 384–389. doi: 10.1007/s12098-017-2595-7
Kutralam Muniasamy, G., Shruti, V. C., Perez Guevara, F., and Roy, P. D. (2023). Microplastic diagnostics in humans: “The 3Ps” Progress, problems, and prospects. Sci. Total Environm. 856:159164. doi: 10.1016/j.scitotenv.2022.159164
La Porta, E., Exacoustos, O., Lugani, F., Angeletti, A., Chiarenza, D. S., Bigatti, C., et al. (2023). Microplastics and kidneys: an update on the evidence for deposition of plastic microparticles in human organs, tissues and fluids and renal toxicity concern. Int. J. Mol. Sci. 24:14391. doi: 10.3390/ijms241814391
Landrigan, P. J., Raps, H., Cropper, M., Bald, C., Brunner, M., Canonizado, E. M., et al. (2023). The minderoo-monaco commission on plastics and human health. Ann. Global Health 89, 1–215. doi: 10.5334/aogh.4083
Landrigan, P. J., Stegeman, J. J., Fleming, L. E., Allemand, D., Anderson, D. M., Backer, L. C., et al. (2020). Human health and ocean pollution. Ann. Global Health 86, 151–215. doi: 10.5334/aogh.2831
Lee, C. H., and Fang, J. K. (2022). The onset of surface-enhanced Raman scattering for single-particle detection of submicroplastics. J. Environm. Sci. 121, 58–64. doi: 10.1016/j.jes.2021.08.044
Lee, M., Kim, H., Ryu, H. S., Moon, J., Khant, N. A., Yu, C., et al. (2022). Review on invasion of microplastic in our ecosystem and implications. Sci. Prog. 105, 1–23. doi: 10.1177/00368504221140766
Lee, Y., Cho, J., Sohn, J., and Kim, C. (2023). Health effects of microplastic exposures: current issues and perspectives in South Korea. Yonsei Med. J. 64, 301–308. doi: 10.3349/ymj.2023.0048
Leslie, H. A., van Velzen, M. J. M., Brandsma, S. H., Vethaak, A. D., Garcia-Vallejo, J. J., and Lamoree, M. H. (2022). Discovery and quantification of plastic particle pollution in human blood. Environm. Int. 163:107199. doi: 10.1016/j.envint.2022.107199
Lett, Z., Hall, A., Skidmore, S., and Alves, N. J. (2021). Environmental microplastic and nanoplastic: Exposure routes and effects on coagulation and the cardiovascular system. Environm. Pollut. 291, 118190. doi: 10.1016/j.envpol.2021.118190
Li, J., Huang, F., Zhang, G., Zhang, Z., and Zhang, X. (2023). Separation and flow cytometry analysis of microplastics and nanoplastics. Front. Chem. 11:1201734. doi: 10.3389/fchem.2023.1201734
Li, Y., Li, Y., Li, J., Song, Z., Zhang, C., and Guan, B. (2023). Toxicity of polystyrene nanoplastics to human embryonic kidney cells and human normal liver cells: Effect of particle size and Pb2+ enrichment. Chemosphere 328:138545. doi: 10.1016/j.chemosphere.2023.138545
Lin, D., Lai, C., Wang, X., Wang, Z., Kuang, K., Wang, Z., et al. (2024). Enhanced membrane fouling by microplastics during nanofiltration of secondary effluent considering secretion, interaction and deposition of extracellular polymeric substances. Sci. Total Environm. 906:167110. doi: 10.1016/j.scitotenv.2023.167110
Lin, P. Y., Wu, I. H., Tsai, C. Y., Kirankumar, R., and Hsieh, S. (2022). Detecting the release of plastic particles in packaged drinking water under simulated light irradiation using surface-enhanced Raman spectroscopy. Analytica Chimica Acta 1198:339516. doi: 10.1016/j.aca.2022.339516
Lin, S., Zhang, H., Wang, C., Su, X. L., Song, Y., Wu, P., et al. (2022). Metabolomics reveal nanoplastic-induced mitochondrial damage in human liver and lung cells. Environ. Sci. Technol. 56, 12483–12493. doi: 10.1021/acs.est.2c03980
Liu, G., Wang, J., Wang, M., Ying, R., Li, X., Hu, Z., et al. (2022). Disposable plastic materials release microplastics and harmful substances in hot water. Sci. Total Environ. 818:151685. doi: 10.1016/j.scitotenv.2021.151685
Liu, J., Zhang, T., Piché-Choquette, S., Wang, G., and Li, J. (2020). Microplastic pollution in China, an invisible threat exacerbated by food delivery services. Bullet. Environm. Contaminat. Toxicol. 107, 778–785. doi: 10.1007/s00128-020-03018-1
Liu, L., Xu, K., Zhang, B., Ye, Y., Zhang, Q., and Jiang, W. (2021). Cellular internalization and release of polystyrene microplastics and nanoplastics. Sci. Total Environ. 779:146523. doi: 10.1016/j.scitotenv.2021.146523
Liu, P., Wang, H., Sun, Y., Liu, H., Zheng, C., Li, T., et al. (2021). Detection of microplastics in carbonated beverages from Chinese supermarkets. Environm. Sci. Pollut. Res. 28, 35393−35402.
Liu, S., Ding, H., Song, Y., Xue, Y., Bi, M., Wu, M., et al. (2023a). The potential risks posed by micro-nanoplastics to the safety of disinfected drinking water. J. Hazard. Mater. 450:131089. doi: 10.1016/j.jhazmat.2023.131089
Liu, S., Guo, J., Liu, X., Yang, R., Wang, H., Sun, Y., et al. (2023b). Detection of various microplastics in placentas, meconium, infant feces, breastmilk and infant formula: a pilot prospective study. Sci. Total Environ. 854:158699. doi: 10.1016/j.scitotenv.2022.158699
Liu, Z., Huang, Y., Jiao, Y., Chen, Q., Wu, D., Yu, P., et al. (2020). Polystyrene nanoplastic induces ROS production and affects the MAPK-HIF-1/NFkB-mediated antioxidant system in Daphnia pulex. Aquatic Toxicology 220:105420. doi: 10.1016/j.aquatox.2020.105420
Liu, Z., Sokratian, A., Duda, A. M., Xu, E., Stanhope, C., Fu, A., et al. (2023). Anionic nanoplastic contaminants promote Parkinson's disease-associated α-synuclein aggregation. Sci. Adv. 9, 3–20. doi: 10.1126/sciadv.adi8716
Mao, J., Wang, S., and Lin, Y. (2022). Risk assessment of microplastics in beverages: China's perspective. Food Control 140:109069.
Mao, X., Xu, Y., Cheng, Z., Yang, Y., Guan, Z., Jiang, L., et al. (2022). The impact of microplastic pollution on ecological environment: a review. Front. Biosci. Landmark 27, 46–53. doi: 10.31083/j.fbl2702046
Marx, A., Piehl, S., and Knepper, T. P. (2023). Microplastics in the environment and food: Assessing impacts and policy responses in the United States. J. Environm. Sci. 116, 50−65.
Mason, S. A., Welch, V. G., and Neratko, J. (2018). Synthetic polymer contamination in bottled water. Front. Chem. 6:407. doi: 10.3389/fchem.2018.00407
MatWeb (2024). MatWeb, Your Source for Materials Information. Available at: https://matweb.com/ (accessed May 14, 2024).
Menon, V., Sharma, S., Gupta, S., Ghosal, A., Nadda, A. K., Jose, R., et al. (2023). Prevalence and implications of microplastics in potable water system: an update. Chemosphere 317:137848. doi: 10.1016/j.chemosphere.2023.137848
Ministry of Ecology and Environment (MEE) China (2021). Plastic Pollution Control Action Plan (2021–2025). Beijing: Government of China.
Ministry of the Environment Japan (MoE) (2021). Act on Promotion of Resource Circulation for Plastics. Tokyo: Government of Japan.
Monteleone, A., Brandau, L., Schary, W., and Wenzel, F. (2020). Using autofluorescence for microplastic detection-heat treatment increases the autofluorescence of microplastics1. Clini. Hemorheol. Microcircul. 76, 473–493. doi: 10.3233/CH-209223
Moodie, R., Stuckler, D., Monteiro, C., Sheron, N., Neal, B., Thamarangsi, T., et al. (2013). Profits and pandemics: prevention of harmful effects of tobacco, alcohol, and ultra-processed food and drink industries. Lancet 381, 670–679. doi: 10.1016/S0140-6736(12)62089-3
Mortensen, N. P., Fennell, T. R., and Johnson, L. M. (2021). Unintended human ingestion of nanoplastics and small microplastics through drinking water, beverages, and food sources. NanoImpact 21:100302. doi: 10.1016/j.impact.2021.100302
Muhib, M. I., Uddin, M. K., Rahman, M. M., and Malafaia, G. (2023). Occurrence of microplastics in tap and bottled water, and food packaging: a narrative review on current knowledge. Sci. Total Environ. 865:161274. doi: 10.1016/j.scitotenv.2022.161274
Nakajima, H., Shimizu, Y., and Fujita, S. (2023). The role of regulatory frameworks in addressing microplastic contamination in Japan. Environm. Policy Govern. 33, 203–213.
National Waste Policy Action Plan (2019). The National Waste Policy Action Plan 2019 Was Prepared by the Australian Government, State and Territory Governments and the Australian Local Government Association. Available at: https://www.agriculture.gov.au/sites/default/files/documents/national-waste-policy-action-plan-2019.pdf
Nizamali, J., Mintenig, S. M., and Koelmans, A. A. (2023). Assessing microplastic characteristics in bottled drinking water and air deposition samples using laser direct infrared imaging. J. Hazard. Mater. 441:129942. doi: 10.1016/j.jhazmat.2022.129942
Oleksiuk, K., Krupa Kotara, K., Wypych-Slusarska, A., Glogowska Ligus, J., Spychala, A., and Slowinski, J. (2022). Microplastic in food and water: current knowledge and awareness of consumers. Nutrients 14:4857. doi: 10.3390/nu14224857
Ormsby, M. J., Woodford, L., and Quilliam, R. S. (2024). Can plastic pollution drive the emergence and dissemination of novel zoonotic diseases? Environm. Res. 246:118172. doi: 10.1016/j.envres.2024.118172
Osman, A. I., Hosny, M., Eltaweil, A. S., Omar, S., Elgarahy, A. M., Farghali, M., et al. (2023). Microplastic sources, formation, toxicity and remediation: a review. Environm. Chem. Lett. 4, 1–41. doi: 10.1007/s10311-023-01593-3
Otto, S., Strenger, M., Maier-Nöth, A., and Schmid, M. (2021). Food packaging and sustainability – Consumer perception vs. correlated scientific facts: a review. J. Cleaner Product. 298:126733. doi: 10.1016/j.jclepro.2021.126733
Panagiotidis, K., Engelmann, B., Krauss, M., Rolle-Kampczyk, U. E., Altenburger, R., Rochfort, K. D., et al. (2023). The impact of amine and carboxyl functionalised microplastics on the physiology of daphnids. J. Hazard. Mater. 458:132023. doi: 10.1016/j.jhazmat.2023.132023
Panno, S. V., Kelly, W. R., Scott, J., Zheng, W., McNeish, R. E., Holm, N., et al. (2019). Microplastic contamination in karst groundwater systems. Groundwater 57, 189–196. doi: 10.1111/gwat.12862
Park, S. J., and Ahn, Y. H. (2022). Detection of polystyrene microplastic particles in water using surface-functionalized terahertz microfluidic metamaterials. Applied Sciences 12:7102. doi: 10.3390/app12147102
Phelan, A., Meissner, K., Humphrey, J., and Ross, H. (2022). Plastic pollution and packaging: Corporate commitments and actions from the food and beverage sector. J. Cleaner Prod. 331:129827. doi: 10.1016/j.jclepro.2021.129827
Pivokonsky, M., Cermakova, L., Novotna, K., Peer, P., Cajthaml, T., and Janda, V. (2018). Occurrence of microplastics in raw and treated drinking water. Sci. Total Environ. 643, 1644–1651. doi: 10.1016/j.scitotenv.2018.08.102
Plastics Europe (2022). Plastics-the Facts 2022. An Analysis of European Plastics Production, Demand, Conversion and End-of-Life Management.
Popli, S., Badgujar, P. C., Agarwal, T., Bhushan, B., and Mishra, V. (2022). Persistent organic pollutants in foods, their interplay with gut microbiota and resultant toxicity. Sci. Total Environ. 832:155084. doi: 10.1016/j.scitotenv.2022.155084
Primpke, S., Godejohann, M., and Gerdts, G. (2020). Rapid identification and quantification of microplastics in the environment by quantum cascade laser-based hyperspectral infrared chemical imaging. Environm. Sci. Ecotechnol. 54, 15893–15903. doi: 10.1021/acs.est.0c05722
Prüst, M., Meijer, J., and Westerink, R. H. S. (2020). The plastic brain: neurotoxicity of micro- and nanoplastics. Particle Fibre Toxicol. 17, 2–16. doi: 10.1186/s12989-020-00358-y
Qian, N., Gao, X., Lang, X., Deng, H., Bratu, T. M., Chen, Q., et al. (2024). Rapid single-particle chemical imaging of nanoplastics by SRS microscopy. Proc. Nat. Acad. Sci. United States Am. 121:e2300582121. doi: 10.1073/pnas.2300582121
Qiu, G., Wang, Q., Wang, T., Zhang, S., Song, N., Yang, X., et al. (2023). Microplastic risk assessment and toxicity in plants: a review. Environm. Chem. Letters 4, 1–18. doi: 10.1007/s10311-023-01665-4
Ramachandraiah, K., Ameer, K., Jiang, G., and Hong, G.-P. (2022). Micro- and nanoplastic contamination in livestock production: entry pathways, potential effects and analytical challenges. Sci. Total Environ. 844:157234. doi: 10.1016/j.scitotenv.2022.157234
Ranjan, V. P., and Joseph, A. S G. (2020). Microplastics and other harmful substances released from disposable paper cups. J. Hazard. Mater. 15:124118. doi: 10.1016/j.jhazmat.2020.124118
Ravanbakhsh, M., Ravanbakhsh, M., Jamali, H. A., Ranjbaran, M., Shahsavari, S., and Jaafarzadeh Haghighi Fard, N. (2022). The effects of storage time and sunlight on microplastic pollution in bottled mineral water. Water Environm. J. 37, 206–217. doi: 10.1111/wej.12829
Saha, S. C., and Saha, G. (2024). Effect of microplastics deposition on human lung airways: A review with computational benefits and challenges. Heliyon 10:e24355. doi: 10.1016/j.heliyon.2024.e24355
Salimi, A., Alavehzadeh, A., Ramezani, M., and Pourahmad, J. (2022). Differences in sensitivity of human lymphocytes and fish lymphocytes to polyvinyl chloride microplastic toxicity. Toxicology and Industrial Health 38, 100–111. doi: 10.1177/07482337211065832
Satti, S. M., and Shah, A. A. (2020). Polyester-based biodegradable plastics: an approach towards sustainable development. Lett. Appl. Microbiol. 70, 413–430. doi: 10.1111/lam.13287
Schirinzi, G. F., Llorca, M., Sero, R., Moyano, E., Barcelo, D., Abad, E., et al. (2019). Trace analysis of polystyrene microplastics in natural waters. Chemosphere 236, 124321. doi: 10.1016/j.chemosphere.2019.07.052
Schmidt, R., Nachtnebel, M., Dienstleder, M., Mertschnigg, S., Schroettner, H., Zankel, A., et al. (2021). Correlative SEM-Raman microscopy to reveal nanoplastics in complex environments. Micron 144:103034. doi: 10.1016/j.micron.2021.103034
Schymanski, D., Goldbeck, C., Humpf, H.-U., and Fürst, P. (2018). Analysis of microplastics in water by micro-Raman spectroscopy: release of plastic particles from different packaging into mineral water. Water Res. 129, 154–162. doi: 10.1016/j.watres.2017.11.011
Schymanski, D., Oßmann, B. E., Benismail, N., Boukerma, K., Dallmann, G., von der Esch, E., et al. (2021). Analysis of microplastics in drinking water and other clean water samples with micro-Raman and micro-infrared spectroscopy: minimum requirements and best practice guidelines. Analyt. Bioanalyt. Chem. 413, 5969–5994. doi: 10.1007/s00216-021-03498-y
Sewwandi, M., Wijesekara, H., Rajapaksha, A. U., Soysa, S., and Vithanage, M. (2023). Microplastics and plastics-associated contaminants in food and beverages; Global trends, concentrations, and human exposure. Environm. Pollut. 317:120747. doi: 10.1016/j.envpol.2022.120747
Sharma, S., Chatterjee, S., and Nandan, R. (2021). Microplastics in food: focus on bottled water and milk products. J. Water Health 19, 381−392.
Shen, M., Song, B., Zhu, Y., Zeng, G., Zhang, Y., Yang, Y., et al. (2020). Removal of microplastics via drinking water treatment: Current knowledge and future directions. Chemosphere 251:126612. doi: 10.1016/j.chemosphere.2020.126612
Shruti, V. C., Kutralam-Muniasamy, G., Pérez-Guevara, F., Roy, P. D., and Elizalde-Martínez, I. (2023). First evidence of microplastic contamination in ready-to-use packaged food ice cubes. Environm. Pollut. 318:120905. doi: 10.1016/j.envpol.2022.120905
Shruti, V. C., Perez Guevara, F., Elizalde-Martinez, I., and Kutralam Muniasamy, G. (2021). Toward a unified framework for investigating micro(nano)plastics in packaged beverages intended for human consumption. Environm. Pollut. 268:115811. doi: 10.1016/j.envpol.2020.115811
Sohail, M., Urooj, Z., Noreen, S., Baig, M., Zhang, X., and Li, B. (2023). Micro- and nanoplastics: contamination routes of food products and critical interpretation of detection strategies. Sci. Total Environ. 891:164596. doi: 10.1016/j.scitotenv.2023.164596
Sorensen, R. M., Kanwar, R. S., and Jovanovi, B. (2022). Past, present, and possible future policies on plastic use in the United States, particularly microplastics and nanoplastics: a review. Integrat. Environm. Assessm. Managem. 19, 474–488. doi: 10.1002/ieam.4678
Stapleton, P. A. (2021). Microplastic and nanoplastic transfer, accumulation, and toxicity in humans. Current Opini. Toxicol. 28, 62–69. doi: 10.1016/j.cotox.2021.10.001
Tan, H., Mong, G. R., Wong, S. L., Wong, K. Y., Sheng, D., Nyakuma, B. B., et al. (2024). Airborne microplastic/nanoplastic research: a comprehensive Web of Science (WoS) data-driven bibliometric analysis. Environm. Sci. Pollut. Res. 31, 109–126. doi: 10.1007/s11356-023-31228-7
Tristan, G., Tay, K. H., and Wong, S. Y. (2023). Near-infrared probe as a quality control tool for milk powder blending processes. Food Mater. Res. 3, 1–8. doi: 10.48130/FMR-2023-0003
Tsochatzis, E. D., Alberto Lopes, J., Hoekstra, E., and Emons, H. (2020). Development and validation of a multi-analyte GC-MS method for the determination of 84 substances from plastic food contact materials. Analyt. Bioanalyt. Chem. 412, 5419–5434. doi: 10.1007/s00216-020-02758-7
Tsochatzis, E. D., Lopes, J. A., Gika, H., Dalsgaard, T. K., and Theodoridis, G. (2021). A fast SALLE GC-MS/MS multi-analyte method for the determination of 75 food packaging substances in food simulants. Food Chem. 361:129998. doi: 10.1016/j.foodchem.2021.129998
Tu, P., Xue, J., Niu, H., Tang, Q., Mo, Z., Zheng, X., et al. (2023). Deciphering gut microbiome responses upon microplastic exposure via integrating metagenomics and activity-based metabolomics. Metabolites 13, 2–16. doi: 10.3390/metabo13040530
U.S. Food and Drug Administration (FDA) (2020). Microplastics in Food and Water Systems. Available at: https://www.fda.gov/food/foodborne-pathogens/microplastics (accessed 2 October, 2024).
Ullah, R., Tsui, M. T., Chow, A., Chen, H., Williams, C., and Ligaba-Osena, A. (2022). Micro(nano)plastic pollution in terrestrial ecosystem: emphasis on impacts of polystyrene on soil biota, plants, animals, and humans. Environm. Monitor. Assessm. 195:252. doi: 10.1007/s10661-022-10769-3
Vandevijvere, S., Jaacks, L. M., Monteiro, C. A., Moubarac, J. C., Girling-Butcher, M., Lee, A. C., et al. (2019). Global trends in ultraprocessed food and drink product sales and their association with adult body mass index trajectories. Obesity Rev. 20, 10–19. doi: 10.1111/obr.12860
Vega Herrera, A., Garcia Torné, M., Borrell Diaz, X., Abad, E., Llorca, M., Villanueva, C. M., et al. (2023). Exposure to micro(nano)plastics polymers in water stored in single-use plastic bottles. Chemosphere 343:140106. doi: 10.1016/j.chemosphere.2023.140106
Vethaak, A. D., and Legler, J. (2021). Microplastics and human health. Science 371, 672–674. doi: 10.1126/science.abe5041
Vivekanand, A. C., Mohapatra, S., and Tyagi, V. K. (2021). Microplastics in aquatic environment: challenges and perspectives. Chemosphere 282:131151. doi: 10.1016/j.chemosphere.2021.131151
Wang, Y., Wang, Z., Lu, X., Zhang, H., and Jia, Z. (2023). Simulation and characterization of nanoplastic dissolution under different food consumption scenarios. Toxics 11:7. doi: 10.3390/toxics11070550
Wang, Y. L., Lee, Y. H., Chiu, I. J., Lin, Y. F., and Chiu, H. W. (2020). Potent impact of plastic nanomaterials and micromaterials on the food chain and human health. Int. J. Mol. Sci. 21:1727. doi: 10.3390/ijms21051727
Wang, Y. L., Lee, Y. H., Hsu, Y. H., Chiu, I. J., Huang, C. C. Y., Huang, C. C., et al. (2021). The kidney-related effects of polystyrene microplastics on human kidney proximal tubular epithelial cells HK-2 and male C57BL/6 mice. Environ. Health Perspect. 129:57003. doi: 10.1289/ehp7612
Wayman, C., and Niemann, H. (2021). The fate of plastic in the ocean environment - a minireview. Environm. Sci. 23, 198–212. doi: 10.1039/D0EM00446D
Winkler, A., Santo, N., Ortenzi, M. A., Bolzoni, E., Bacchetta, R., and Tremolada, P. (2019). Does mechanical stress cause microplastic release from plastic water bottles? Water Res. 166:115082. doi: 10.1016/j.watres.2019.115082
World Health Organization (2019). Microplastics in Drinking-Water. Geneva: World Health Organization.
World Health Organization (2023). WHO Health & Plastics Dialogues the Impact of Plastics on Health is an Increasing Concern. European Union; World Health Organization. Available at: https://www.who.int/news/item/14-09-2023-who-health—plastics-dialogues
Xing, D., Hu, Y., Sun, B., Song, F., Pan, Y., Liu, S., et al. (2023). Behavior, characteristics and sources of microplastics in Tea. Horticulturae 9:174. doi: 10.3390/horticulturae9020174
Xu, J. L., Lin, X., Hugelier, S., Herrero-Langreo, A., and Gowen, A. A. (2021). Spectral imaging for characterization and detection of plastic substances in branded teabags. J. Hazard. Mater. 418:126328. doi: 10.1016/j.jhazmat.2021.126328
Xu, Y., Chan, F. K. S., He, J., Johnson, M., Gibbins, C., Kay, P., et al. (2020). A critical review of microplastic pollution in urban freshwater environments and legislative progress in China: recommendations and insights. Crit. Rev. Environ. Sci. Technol. 51, 2637–2680. doi: 10.1080/10643389.2020.1801308
Yan, Z., Liu, Y., Zhang, T., Zhang, F., Ren, H., and Zhang, Y. (2021). Analysis of microplastics in human feces reveals a correlation between fecal microplastics and inflammatory bowel disease status. Environ. Sci. Technol. 56, 414–421. doi: 10.1021/acs.est.1c03924
Yang, H., Xue, Q., Lu, F., Dong, Y., Wang, F., and Li, Q. (2023). Detection of microplastic samples based on spatial heterodyne microscopic differential Raman spectroscopy. J. Raman Spectrosc. 54, 1090–1098. doi: 10.1002/jrs.6563
Yang, Y., Xie, E., Du, Z., Peng, Z., Han Zhongyi, L. L., Zhao, R., et al. (2023). Detection of various microplastics in patients undergoing cardiac surgery. Environ. Sci. Technol. 57, 10911–10918. doi: 10.1021/acs.est.2c07179
Yozukmaz, A. J. E. (2022). Detection and public health risk assessment of microplastics in disposable (PET) bottled water produced and sold locally in the Aegean Region. J. Fisher. Aquatic Sci. 39, 275–283. doi: 10.12714/egejfas.39.4.02
Zangmeister, C. D., Radney, J. G., Benkstein, K. D., and Kalanyan, B. (2022). Common single-use consumer plastic products release trillions of sub-100 nm nanoparticles per liter into water during normal use. Environ. Sci. Technol. 56, 5448–5455. doi: 10.1021/acs.est.1c06768
Zhang, Y., Wu, H., Xu, L., Liu, H., and An, L. (2022). Promising indicators for monitoring microplastic pollution. Marine Pollut. Bullet. 182:113952. doi: 10.1016/j.marpolbul.2022.113952
Zhao, Q., Zhu, L., Weng, J., Jin, Z., Cao, Y., Jiang, H., et al. (2023). Detection and characterization of microplastics in the human testis and semen. Sci. Total Environ. 877:162713. doi: 10.1016/j.scitotenv.2023.162713
Keywords: microplastics, nanoplastics, toxicity, plastic pollution, food safety, public health
Citation: Ma C, Ramachandraiah K and Jiang G (2024) Micro and nano plastics: contaminants in beverages and prevention strategies. Front. Sustain. Food Syst. 8:1491290. doi: 10.3389/fsufs.2024.1491290
Received: 04 September 2024; Accepted: 05 November 2024;
Published: 25 November 2024.
Edited by:
Shugo Watabe, Kitasato University, JapanReviewed by:
Maria Simona Chis, University of Agricultural Sciences and Veterinary Medicine of Cluj-Napoca, RomaniaTaozhu Sun, Virginia Tech, United States
Zhonghe Tian, The University of Tokyo, Japan
Copyright © 2024 Ma, Ramachandraiah and Jiang. This is an open-access article distributed under the terms of the Creative Commons Attribution License (CC BY). The use, distribution or reproduction in other forums is permitted, provided the original author(s) and the copyright owner(s) are credited and that the original publication in this journal is cited, in accordance with accepted academic practice. No use, distribution or reproduction is permitted which does not comply with these terms.
*Correspondence: Guihun Jiang, amlhbmdndWlodW5AMTYzLmNvbQ==