- 1College of Animal Science and Technology, Henan Agricultural University, Zhengzhou, China
- 2Henan PUAI Feed Co., Ltd., Zhoukou, China
- 3Henan Delin Biological Product Co., Ltd., Xinxiang, China
The purpose of the study was to demonstrate how soybean peptides (SBP) protect against H2O2 -induced injury in intestinal porcine epithelial cells (IPEC-J2). SBP were prepared by protease hydrolysis, in which the molecular weights of 95.76% SBP were smaller than 3 kDa. Cell experiment included four groups: Control group (IPEC-J2 cells were treated with HGDMEM), SBP group (100 μg/mL SBP incubation for 13 h), H2O2 treatment group (1 mM H2O2 treatment for 1 h), SBP + H2O2 group (100 μg/mL SBP pretreatment for 12 h followed by 1 mM H2O2 treatment for 1 h). This study showed that that treatment with single 1 mM H2O2 for 1 h significantly reduced cell viability to 52.99% (p < 0.05), up-regulated Bax and Caspase-3 gene expressions (p < 0.05), and down-regulated gene expressions of ZO-1, CAT, SOD1, HO-1 and Nrf2 (p < 0.05), compared with the control group. However, pretreatment with SBP followed by H2O2 inducement significantly increased cell viability to 72.99%, decreased cell apoptosis, increased SOD, CAT and GSH-Px activity (p < 0.05), down-regulated Bax and Caspase-3 gene expressions (p < 0.05), and up-regulated the gene expressions of ZO-1, Claudin-1, Occludin, catalase, glutathione GPX1, SOD1, HO-1, NQO1 and Nrf2, compared with the single H2O2–induced cells. According to the study, SBP pretreatment reduced H2O2-induced oxidative stress in cells and preserved the integrity of intestinal cells.
1 Introduction
A variety of stresses such as piglet weaning (Boudry et al., 2004), alcohol consumption (Das and Vasudevan, 2007), mycotoxins and discomfort environment conditions can exacerbate the generation of free radicals and thus force oxidative damage (Svobodová et al., 2006). In general, gastrointestinal epithelial tissues play an important role in nutrient absorption, digestion and metabolism as well as gut microbiota. The gastrointestinal epithelial cells often produce many kinds of free radicals, resulting in oxidative stress in the cells to cause a variety of gastrointestinal disorders or diseases. The redox homeostasis of cells is important for the health of the organism (Bhattacharyya et al., 2014; Xiang et al., 2020). Therefore, in-depth study of the efficacy of feed additives with antioxidant function in vivo is of great significance to alleviate the effects of oxidative stress on intestinal cells. Many kinds of protein hydrolysates and peptides from milk (Wang et al., 2016), egg white (Liu et al., 2014), cereals (Guo et al., 2014) and fish (Je et al., 2015) have been reported to have antioxidant properties. Therefore, selecting the optimal and economic peptide for decreasing cell oxidation stress becomes more and more important.
Soybean is known as an important protein resource in animal diets. The specific soybean protein hydrolysates and peptides possess many kinds of antioxidant qualities, including the ability to chelate metal ions, scavenge free radicals, and prevent lipid peroxidation (Moure et al., 2006; Peña-Ramos and Xiong, 2002; Zhang et al., 2010). Reactive oxygen species (ROS) and free radicals can be effectively scavenged by soy peptide, which inhibits oxidative stress in rats (Takenaka et al., 2003). Another report showed that the active peptides in tempeh increased catalase (CAT) and superoxide dismutase (SOD) activity in rat liver and glutathione peroxidase (GSH-Px) and SOD activities in the kidneys (Wang et al., 2008). The SOD and GSH-Px activities in the serum of juvenile halibut were increased by adding different levels of soybean peptides (Song et al., 2014). Despite SBP has potential application in animal diets, there are no further studies how SBP protects porcine intestinal epithelial cells.
Currently, IPEC-J2 is frequently utilized for probiotic bacteria screening (Brosnahan and Brown, 2012), intestinal immune and inflammatory research (Aperce et al., 2010; Liu et al., 2010), and mycotoxin studies (Diesing et al., 2011). It is non-transformed and non-tumorigenic (Geens and Niewold, 2011).
This research focused on preparing SBP and exploring its ability to alleviate cytotoxicity, antioxidant capacity, apoptosis and barrier function for H2O2-induced IPEC-J2 cells, in order to find out its acting mechanisms to reduce oxidative stress.
2 Materials and methods
2.1 Chemicals and reagents
Alkaline protease (200,000 U/g) was obtained from Nanning Pangbo Biological Engineering Co., Ltd. (Nanning, China). High glucose Dulbecco’s modified Eagle’s medium (DMEM), phosphate-buffered saline (PBS) and fetal bovine serum (FBS) were purchased from Biological Industries (Kibbutz Beit-Haemek, Israel). Cell counting kit 8 (CCK-8) was provided by Abbkine Scientific Co., Ltd. (Wuhan, China). The qRT-PCR kit was purchased from Takara Company (Dalian, China). H2O2 was purchased from Sigma-Aldrich (St. Louis, MO, USA). SOD, CAT and GSH assay kits were purchased from Nanjing Jian Cheng Bioengineering Institute (Nanjing, China). Goat anti-rabbit antibody such as β-actin, SOD1, heme oxygenase-1 (HO-1), zonulaoccluden-1 (ZO-1), claudin-1, keap1 and nuclear factor erythroid 2-related factor 2 (Nrf2) were purchased from Wuhan Service Bio-Technology Co., Ltd. (Wuhan, China).
2.2 Soybean peptide preparation
About 25 g soybean meals were dispersed in distilled water in a ratio of 1:4 (w/v). The mixture was hydrolyzed using alkaline protease (10,000 U/g soybean meal) under the reactive conditions of 55°C, pH = 9 and 4.5 h. After pH was adjusted to 5.0, the mixture was placed in boiling water for 30 min to inactivate the enzymes, centrifuged at 5000 g for 10 min, and the supernatant was collected. Then, active carbon with a ratio of 1:10 (w/v) was added for decoloration and debittering. The supernatants was obtained using a membrane of 0.45 μm, then quickly freeze-dried and stored at 4°C for backup.
2.3 Peptide identification by RPLC-MS/MS
Identification of peptides was performed by an Easy-nLC 1,000 UHPLC system (Thermo Fisher Scientific, San Jose, CA, USA), online with an Orbitrap Fusion mass spectrometer (Thermo Fisher Scientific, San Jose, CA, USA). Peptide separations were performed on Acclaim PepMap RSLC C18 threaded columns (Thermo Fisher Scientific, San Jose, CA, USA). The eluents of mobile phases A and B were 0.1% formic acid (FA) in water and 0.1% FA in acetonitrile, respectively. A gradient elution procedure was set: 0.0–3.0 min, 3% eluent B; 3.0–7.0 min, 3–8% eluent B; 7.0–46.0 min, 8–32% eluent B; 46.0–51.0 min, 32–44% eluent B. The polypeptide concentration was 10.0 mg/mL. The injection amount was 8.0 μL. The flow rate was 400 nL/min. The Orbitrap Fusion Mass Analyser collected all of the data throughout a 350–1,550 m/z scan range. Resequencing was used to analyze the spectra, and the UniProt database was used to look for matches in the MS/MS spectra.1 Sequence comparison of reported peptides was performed using the BIOPEP database2.
2.4 Cell culture and experimental design
IPEC-J2 cells were grown in High-glucose Dulbecco’s Modified Eagle Medium (HGDMEM) supplemented with 1% penicillin–streptomycin and 10% fetal bovine serum, respectively, at 37°C and 5% CO2 in the incubator. For the experiments, IPEC-J2 cells were seeded into 6-well plates at a density of 2.5 × 105 cells/mL for 2 mL or 96-well plates at a density of 1.0 × 105 cells/mL for 100 μL. Cells were allowed to adhere for 24 h-36 h until they reached confluency before treatment. Cell viability was assessed using CCK-8 solution (1%, v/v). The protocol was as follows: Each well received a 10 μL CCK8 solution, and was incubated for 2 hours. An enzyme-linked immunosorbent assay (ELISA) was used to determine optical density (OD) at 490 nm.
To determine a model of cellular oxidative stress, after IPEC-J2 cells were adhere for 24 h, different concentrations (0.1, 0.5, 1, 2 or 4 mM) of H2O2 were added to the wells, then incubated for 1 h. The incubation time was selected according to the previous reports (Cai et al., 2013; Paszti-Gere et al., 2012). The optimal H2O2 concentration for decreasing cell viability was obtained for the following research. For the optimal SBP concentration, after IPEC-J2 cells reached confluency, different concentrations (0, 25, 50, 100, 200, 400, 800, 1,000, 2000 μg/mL) of SBP were added and went on incubating for 12 h, then incubated in H2O2 for 1 h. H2O2 was completely removed by washing with PBS twice. The viability of cell viability was measured with CCK8 to obtain the optimal SBP concentration for alleviating cell damage induced by H2O2.
The cell experiment was divided into 4 groups: (1) Control group: Incubated the cells with HGDMEM for 12 h, replaced the medium with fresh HGDMEM once, and continued to incubate for 1 h; (2) SBP group: Incubated the cells with HGDMEM supplemented with 100 μg /mL SBP for 12 h, replaced the above solution with fresh HGDMEM containing 100 μg /mL SBP, and continued to incubate for 1 h; (3) H2O2 treatment group: Incubated the cells with HGDMEM containing 1 mM H2O2 for 1 h; (4) SBP + H2O2 group: Incubated the cells with HGDMEM supplemented with 100 μg /mL SBP for 12 h, followed by 1 mM H2O2 treatment for 1 h.
2.5 Quantitative real-time PCR
After four treatments (Control, H2O2, SBP, SBP + H2O2), Total cellular RNA was completely extracted using Trizol reagent (Invitrogen, Carlsbad, CA, USA) according to the instructions. The cDNA in a 10 μL reaction system were synthesized by using PrimeScript RT reagent Kit (TakaRa, Dalian, China). Real-time PCR in a 10 μL reaction system were performed by using the SYBR Premix Ex Taq (TakaRa, Dalian, China), and the cycle conditions of each step were 95°C for 300 s, followed by 38 cycles of 95°C for 20 s, 60°C for 30 s and 72°C for 30 s. The primers used in this research are all listed in Table 1 (Xun et al., 2021). To normalize the levels of gene expression, glyceraldehyde-3-phosphate dehydrogenase (GAPDH) was utilized as a reference gene. The 2-ΔΔCT method (Livak and Schmittgen, 2001) was utilized to analyze RT-PCR data and determine the relative fold changes of the target genes.
2.6 Western blotting analysis
After four groups of cell treatments, total proteins from treated cells were extracted with RIPA Lysis buffer (Service biotechnology, Wuhan, China), and the extracted protein concentrations were accurately measured by BCA protein assay kit (Nanjing Jiancheng Bioengineering Institute, Nanjing, China). Using sodium dodecyl sulfate polyacrylamide gel electrophoresis (SDS-PAGE), equal amounts of proteins was isolated, then electro-transferred onto the methanol-activated polyvinylidene ifluoride (PVDF) membranes. The transferred membrane was placed in an incubation tank with TBST, quickly swabbed once, then added with 5% milk, closed in a decolourising shaker for 2 h at room temperature, and incubated in a shaker overnight at 4°C with the prepared primary antibodies. After being washed for three times with TBST, the treated membranes were incubated with the diluted secondary antibodies for 2 h. Enhanced chemiluminescence was used to observe the protein bands, and ImageJ (National Institutes of Health, Maryland, USA) software was used for analysis. All sample protein expressions were normalized using β-actin.
2.7 Measurements of antioxidant parameters
Antioxidant parameters including SOD, GSH-Px and CAT were analysed by xanthine oxidase-xanthine reaction, reduced glutathione and ammonium molybdate method, respectively. The determination of these antioxidants was carried out according to the instructions of the kits (Nanjing Jiancheng Bioengineering Institute, Nanjing, China).
2.8 Statistical analyses
GraphPad Prism 7 (GraphPad Software, La Jolla, USA) was used to produce each graph. Statistical analysis was assessed using SPSS 20.0 one-way analysis of variance (ANOVA) (Sishu Software, Shanghai Co., Ltd. Shanghai, China). The replicates in each group were least 3 times. The Duncan test was used to perform multiple comparisons. The means ± standard deviations of the results were displayed, and differences were considered statistical significance at p < 0.05.
3 Results
3.1 Identification of SBP and its antioxidant capacity
About 2,314 kinds of peptides were obtained in this study. The analysis of molecular weights for SBP showed that 500–1,500, 1,500–3,000, 3,000–5,000 and 5,000–10,000 Da accounted for 57.13, 38.63, 3.98 and 0.26%, respectively (Figure 1). It indicated that the molecular weights of 95.76% SBP were smaller than 3 kDa. About 15 identified soybean peptides and their potential bioactivity were listed in Table 2. By using the BIOPEP database and the known biological activity, the sequences or structures in the listed polypeptides can be identified as having a variety of activities, such as angiotensin-I-converting enzyme (ACE) inhibitory, antioxidant, and dipeptidyl peptidase (DPP) IV inhibitory activities.
3.2 H2O2 cytotoxicity
Figure 2 revealed that the survivability of IPEC-J2 cells was significantly reduced after 1 h incubation when H2O2 concentration exceeded 1 mM (p < 0.05), therefore, 1 mM H2O2 was selected for the further research. Incubation time was selected as 1 h based to the previous report (Cai et al., 2013; Paszti-Gere et al., 2012).
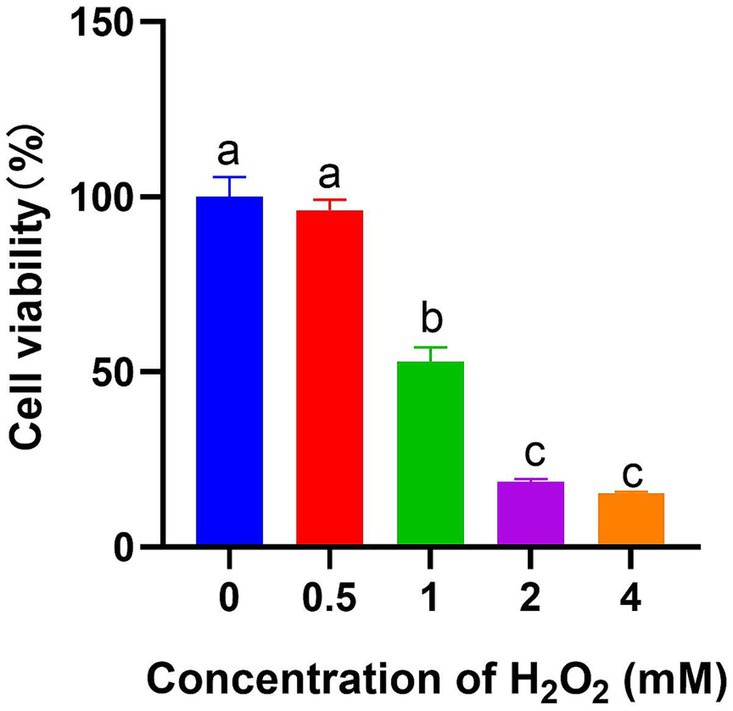
Figure 2. Effect of different H2O2 concentrations on IPEC-J2 cell viability after 1 h treatment (n = 6). The different lowercase letters on the bars are significantly different (p < 0.05), while the same lowercase letters on the bars are insignificantly different (p > 0.05).
3.3 Cell viability affected by SBP
Figure 3 showed that the cell viability had insignificant influence when SBP concentration was lower than 200 μg/mL during 12 h treatment (p > 0.05); however, it had insignificant influence when SBP concentration was lower than 80 μg/mL during 24 h treatment (p > 0.05), compared with the control group.
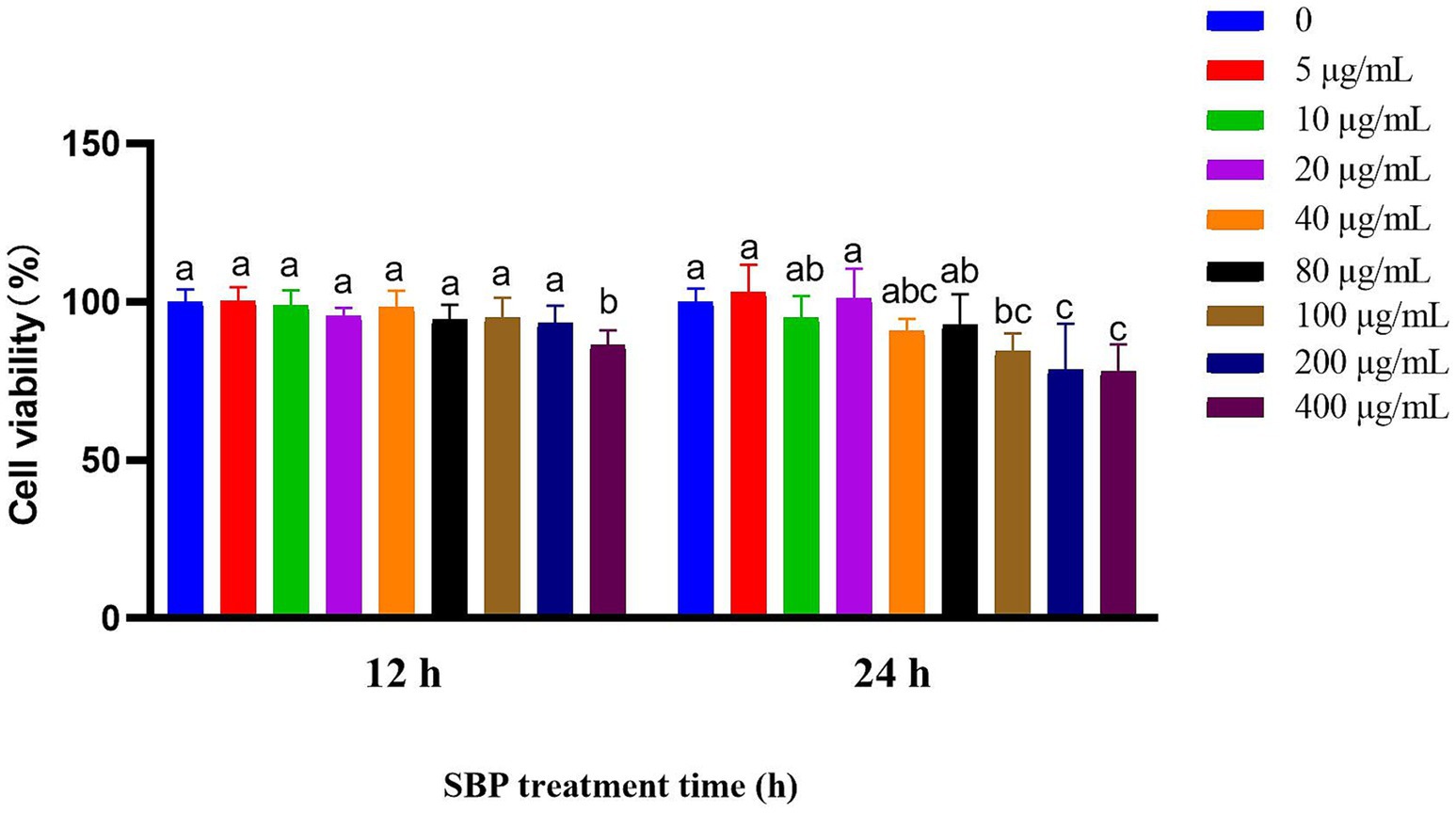
Figure 3. Effect of different SBP concentrations on IPEC-J2 cell viability at different incubation time (n = 6). The different lowercase letters on the bars are significantly different (p < 0.05), while the same lowercase letters on the bars are insignificantly different (p > 0.05).
3.4 SBP alleviating H2O2-induced damage
Under H2O2-induced condition, 100–800 μg/mL SBP could significantly alleviate H2O2-induced cell damages after 12 h or 24 h treatment (p < 0.05), compared with the single H2O2-induced group (Figure 4). Therefore, 100 μg/mL SBP was recommended for alleviating H2O2-induced cell damages in the further experiment.
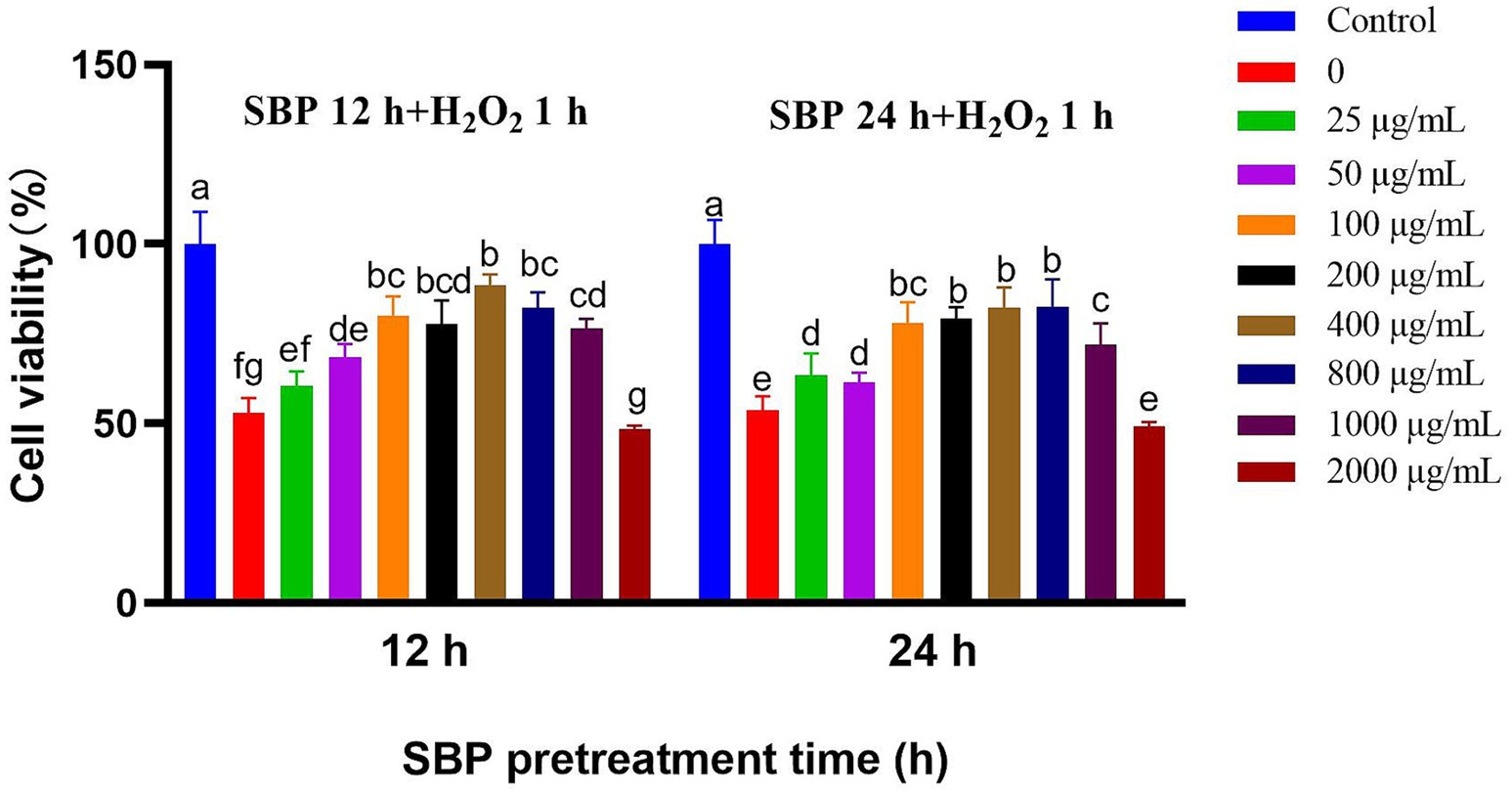
Figure 4. Effect of different SBP concentrations on IPEC-J2 cell viability induced by H2O2 after different SBP pretreatment time (n = 6). The different lowercase letters on the bars are significantly different (p < 0.05), while the same lowercase letters on the bars are insignificantly different (p > 0.05).
3.5 Tight junction protein expressions
Figure 5 showed that single H2O2 addition in IPEC-J2 cell incubation significantly reduced mRNA abundance as well as protein expression of ZO-1 (p < 0.05), while SBP addition significantly increased ZO-1 mRNA abundance. After the addition of SBP, Claudin-1 protein expression was significantly increased, and Claudin-1 and Occludin mRNA abundance were also increased, compared with single H2O2 group. It could be concluded that SBP pre-incubation increased intestinal compactness to safeguard intestinal cells from oxidative stress.
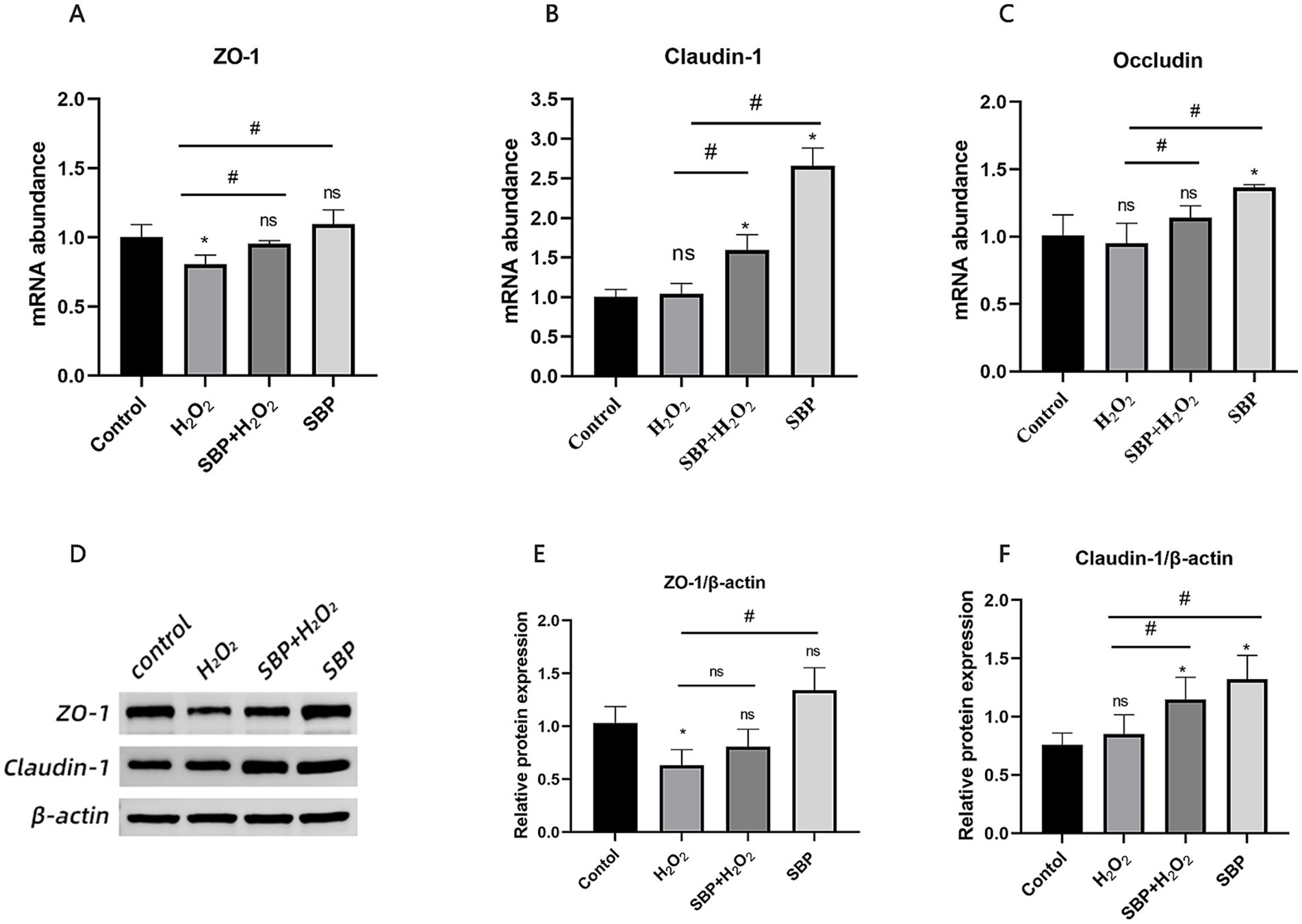
Figure 5. Effect of SBP on relative mRNA abundances and protein expressions of tight junction protein in H2O2-induced IPEC-J2 cells. (A–C) The mRNA abundances of ZO-1, Occludin and Claudin-1 (n = 5). (D) Western blot analysis of ZO-1 and Claudin-1 (n = 3). (E,F) The protein expressions of ZO-1 and Claudin-1 (n = 3). Control (IPEC-J2 cells were treated with HGDMEM), H2O2 (1 mM H2O2 treatment for 1 h), SBP + H2O2 (100 μg/mL SBP pretreatment for 12 h followed by 1 mM H2O2 treatment for 1 h), SBP (100 μg/mL SBP incubation for 13 h). Compared with the control group, “*” indicates p < 0.05; compared with single H2O2 group, “#” indicates p < 0.05; “ns” indicates p > 0.05.
3.6 Apoptosis gene expression
Figure 6 showed that H2O2 increased the mRNA abundance of Bax and cysteinyl aspartate-specific protease 3 (Caspase-3) (p < 0.05); instead, after SBP pre-incubation, they were down-regulated significantly (p < 0.05). In addition, H2O2 or SBP treatment had insignificant effect on B-cell lymphoma-2 (Bcl-2) mRNA abundance.
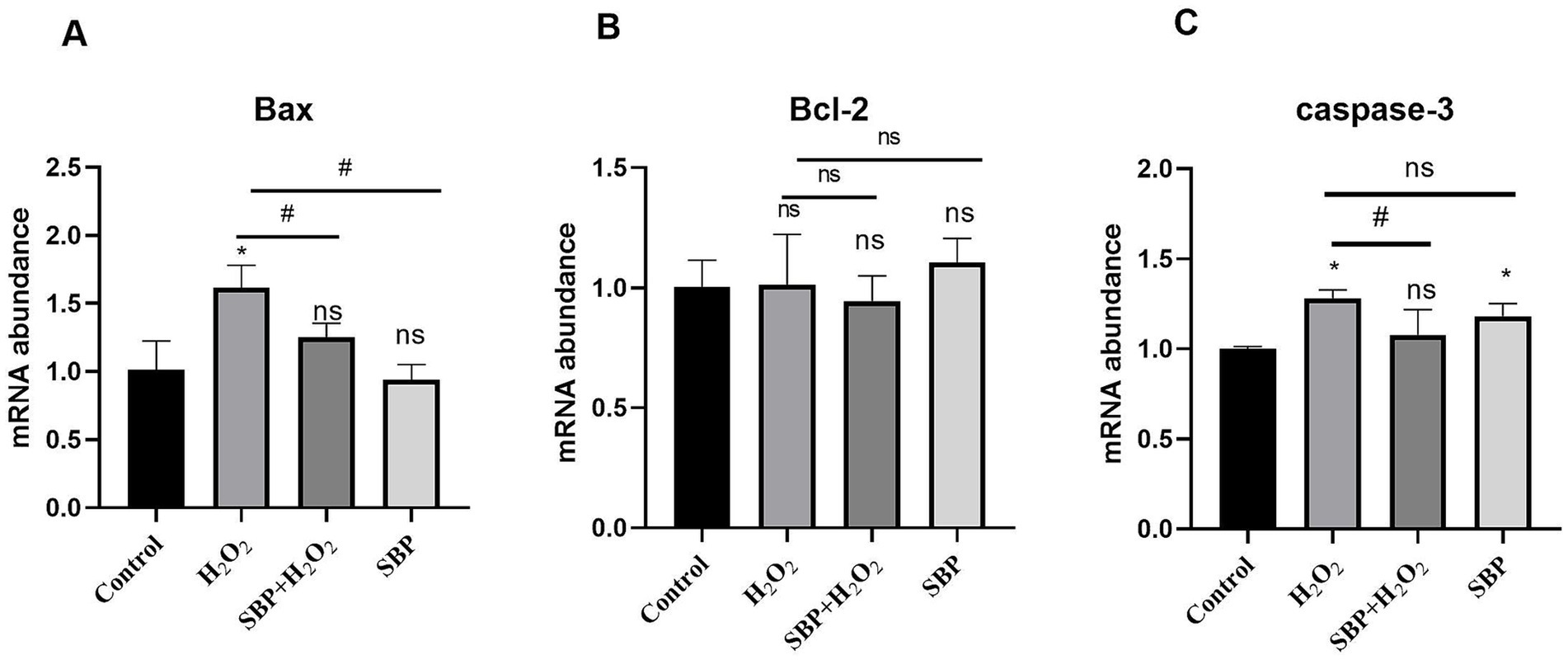
Figure 6. Effect of SBP on relative mRNA abundances of cell apoptotic genes in H2O2-induced IPEC-J2 cells (n = 5). (A) The mRNA abundances of Bax. (B) The mRNA abundances of Bcl-2. (C) The mRNA abundances of caspase-3. Control (IPEC-J2 cells were treated with HGDMEM), H2O2 (1 mM H2O2 treatment for 1 h), SBP + H2O2 (100 μg/mL SBP pretreatment for 12 h followed by 1 mM H2O2 treatment for 1 h), SBP (100 μg/mL SBP incubation for 13 h). Compared with the control group, “*” indicates p < 0.05; compared with single H2O2 group, “#” indicates p < 0.05; “ns” indicates p > 0.05.
3.7 mRNA and protein expressions of Nrf2-related genes
Figure 7 indicated that H2O2 significantly reduced the gene expressions of SOD1 (0.50 fold), CAT (0.78 fold), Nrf2 (0.78 fold) and HO-1 (0.74 fold) (p < 0.05), compared to the control group; however, SBP early addition before H2O2–induced cell incubation was able to upregulate the relative mRNA abundances of SOD1 (0.85 fold), CAT (1.15 fold), glutathione peroxidase1 (GPX1, 1.54 fold), Nrf2 (1.17 fold), HO-1 (1.05 fold) and quinone oxidoreductase1 (NQO1, 1.44 fold)(p < 0.05). Figure 7 also showed that H2O2 significantly declined the protein expressions of Nrf2, SOD1 and HO-1 (p < 0.05), however, SBP early addition during H2O2–induced cell incubation was able to increase the protein expressions of Nrf2, SOD1 and HO-1 (p < 0.05). Compared with the control group, the single SBP addition could upregulate the mRNA abundances of CAT (1.61 fold), GPX1 (1.45 fold), Nrf2 (1.21 fold) and NQO1 (1.92 fold) as well as protein expressions of Nrf2 and HO-1 (p < 0.05). Therefore, SBP was able to alleviate oxidative damage for H2O2-induced IPEC-J2 cells.
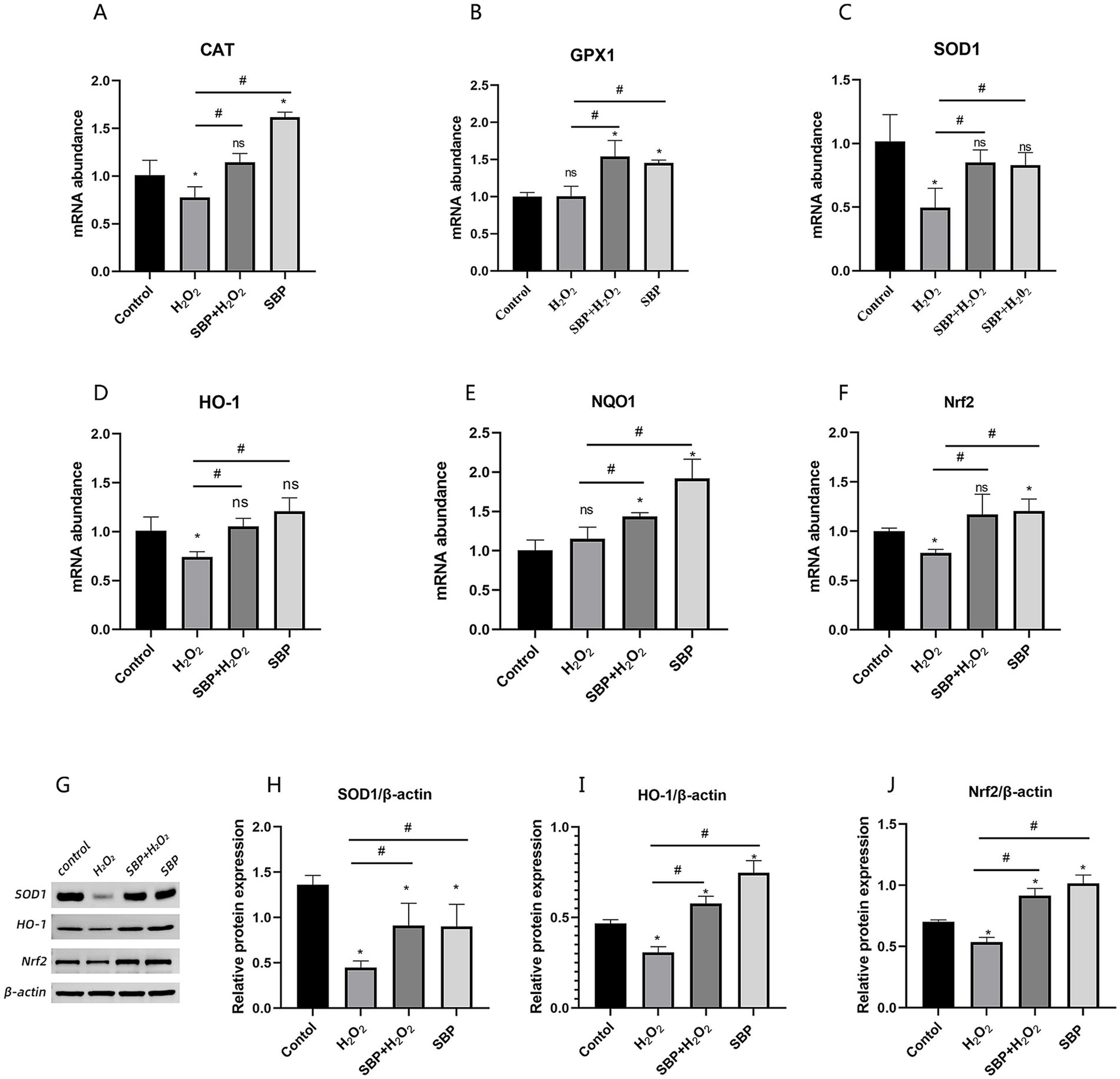
Figure 7. Effect of SBP on relative mRNA abundances and protein expressions of antioxidase and Nrf2-related genes in H2O2-induced IPEC-J2 cells. (A–C) The mRNA abundances of CAT, GPX1 and SOD1 (n = 5). (D–F) The mRNA abundances of HO-1, NQO1 and Nrf2 (n = 5). (G) Western blot analysis of SOD1, HO-1 and Nrf2 (n = 3). (H–J) The protein expressions of SOD1, HO-1 and Nrf2 (n = 3). Control (IPEC-J2 cells were treated with HGDMEM), H2O2 (1 mM H2O2 treatment for 1 h), SBP + H2O2 (100 μg/mL SBP pretreatment for 12 h followed by 1 mM H2O2 treatment for 1 h), SBP (100 μg/mL SBP incubation for 13 h). Compared with the control group, “*” indicates p < 0.05; compared with single H2O2 group, “#” indicates p < 0.05; “ns” indicates p > 0.05.
3.8 Antioxidant enzyme activity determination
Table 3 showed that the enzyme vitality value of CAT, GSH-Px and SOD were significantly declined with the addition of H2O2 (p < 0.05); but SBP could significantly increase their enzyme activity (p < 0.05), indicating that SBP could increase antioxidant enzyme activity in H2O2-induced IPEC-J2 cells to alleviate H2O2-induced oxidative stress.
4 Discussion
Oxidative stress or dysregulation of cellular redox can lead to a variety of animal diseases (Demmig-Adams and Adams, 2002). The degree of oxidative stress is determined by ROS concentration and the duration of exposure, the cell types, and the antioxidant capacity of the cell (Saberi et al., 2008). Numerous antioxidant characteristics of soybean hydrolysates such as scavenging free radicals and chelating metal ions as well as preventing lipid peroxidation have been demonstrated (Moure et al., 2006; Peña-Ramos and Xiong, 2002; Zhang et al., 2010). In order to obtain high yield of soybean peptides, alkaline proteases have been used (Arise et al., 2016; Shi et al., 2014). This study showed that more than two thousands kinds of soybean peptides were successfully achieved from soybean meal by hydrolysis of alkaline protease after modifying the enzymatic hydrolysis conditions, which provided the novel peptides for their future applications.
The biological function of peptides has been reported to be correlated with their molecular weights, and the peptides within 500–1,500 Da had a higher antioxidant capacity compared to other peptides (Sarmadi and Ismail, 2010; Yan et al., 2015). Another report indicated that the peptides with small molecular weight (< 3 kDa) inhibited oxidative stress and neuroinflammation well in LPS-induced cognitive impairment mice (Wen et al., 2022). This study showed that the molecular weights of 57.13% SBP were 500–1,500 Da, and 95.76% SBP were smaller than 3 kDa, indicating that SBP prepared in this study was able to alleviate oxidative stress effectively. The peptides mentioned in Table 2 had molecular weights ranging from 677.41 to 1296.70 Da, which may be linked to their antioxidant potential. Furthermore, peptides as one kind of antioxidant are correlated with their amino acid composition. Strong antioxidants have been found to be the peptides containing amino acid residues like leucine (Leu), phenylalanine (Phe), valine (Val), tyrosine (Tyr), alanine (Ala), proline (Pro), and methionine (Met) (Farvin et al., 2016; Sarmadi and Ismail, 2010; Yan et al., 2015). It is inferred that all the identified peptides containing more than one such amino acid residue in this study may have the anti-oxidative properties.
H2O2 is more stable in vivo than other types of ROS, so it is often used to study redox-regulated processes (Paszti-Gere et al., 2012). In order to create a model of SBP on alleviating oxidative stress, H2O2 was used in this study. This study indicated that H2O2 significantly decreased cell viability. This study also showed that high SBP concentrations and long-term treatment decreased cell viability. Previous study showed that high-level soybean peptides addition (more than 100 μg/mL) decreased cell viability (Zhang et al., 2020), corresponding with this study. The reason may be due to the interaction between cells and peptides. However, optimal SBP concentrations was found to have the best protective effect on H2O2-induced IPEC-J2 cells at concentrations of 100–800 μg/mL. The protective effect of these peptides may be partially attributed to the fact that their ability to neutralize intracellular ROS, inhibit lipid peroxidation, and enhance antioxidant enzymes.
Occludin, ZO-1 and Claudin-1, are part of a junctional multiprotein complex that maintains the intestinal epithelial barrier function (Ashida et al., 2012), which are essential part of the animal’s intestinal mucosal barrier, preventing bacteria, endotoxins and other harmful substances from crossing the epithelium (Ulluwishewa et al., 2011). According to this study, adding SBP increased the relative mRNA abundances of tight junction protein. Increased expression of ZO-1 and Occludin genes has been shown to reduce intestinal permeability due to tight junction disruption in weaned piglets (Hu et al., 2013; Zhang and Guo, 2009), this indicated that SBP may enhance the compactness of intestinal tight junction proteins, shielding the intestinal barrier from H2O2 damage.
Generally, cell apoptosis was promoted by the expressions of Bax and Caspase-3. In this study, the level of apoptotic genes of cell was significantly increased in the single H2O2 group, suggesting that H2O2 can lead to cell apoptosis, but mRNA abundances were down-regulated by SBP addition; therefore, it can be inferred that SBP could alleviate H2O2-induced apoptosis. The previous report indicated that SBP could significantly promote IEC-6 cell proliferation, alleviate LPS-induced intestinal inflammation (Wen et al., 2022), corresponding with this study.
Nrf2 signaling could be one of the possible ways that SBP reduced oxidative damage. In the normal state, Nrf2 and Keap1 form a complex in the cytoplasm. When Nrf2 encounters oxidative stress, it separates from Keap1 and moves into the nucleus. There, it constitutes a heterodimeric polymer with tiny Maf proteins, triggering the production of several antioxidant enzymes (Kaspar et al., 2009). In order to maintain a normal redox state and lessen cellular damage, Nrf2 has been demonstrated to influence the expression level of phase II metabolic enzyme genes (HO-1 and NQO1) and downstream antioxidant enzyme genes (SOD1, GPX1, and CAT) (Mine et al., 2015). In IPEC-J2, this study showed that H2O2-induced damage inhibited the expression of the Nrf2 protein and its downstream genes (SOD1, CAT, and HO-1), but with the addition of SBP, all of them were reversed. The results indicated that promoting the Nrf2 pathway can upregulate the expressions of related antioxidant enzymes of IPEC-J2 cells.
Intracellularly, H2O2 can be removed by GSH-Px and CAT (Valko et al., 2007). From the study, H2O2 inhibited the antioxidant ability of GSH-Px, SOD and CAT, leading to a decreased ability to detoxify ROS through antioxidant enzymes (Halici et al., 2012; Kaur et al., 2006). However, after oxidative damage, SBP can help the body to improve the expression of antioxidant enzymes and rebalance the redox state (Demmig-Adams and Adams, 2002).
5 Conclusion
Pre-incubation of IPEC-J2 cells with 100 mg/kg SBP for 12 h alleviated H2O2-induced oxidative damage in cells. SBP enhanced the gene expression level of tight junction proteins, reduced the expression of apoptotic genes, and increased the antioxidant capacity of the cells. This ability of SBP may be related to the activation of the Nrf2-related pathway. These results suggest that soybean peptides prepared by alkaline protease hydrolysis will be a good candidate to protect intestinal mucosa from oxidative stress for improving animal production performance.
Data availability statement
The datasets presented in this study can be found in online repositories. The names of the repository/repositories and accession number(s) can be found below: https://www.ncbi.nlm.nih.gov/, XM-004387206; https://www.uniprot.org/, P04405.
Ethics statement
Ethical approval was not required for the studies on animals in accordance with the local legislation and institutional requirements because only commercially available established cell lines were used.
Author contributions
FL: Data curation, Formal analysis, Investigation, Methodology, Writing – original draft. QY: Project administration, Supervision, Writing – review & editing. XW: Investigation, Methodology, Writing – review & editing. LX: Data curation, Formal analysis, Writing – review & editing. ML: Data curation, Methodology, Writing – review & editing. QiZ: Data curation, Formal analysis, Writing – review & editing. HS: Data curation, Methodology, Writing – review & editing. CL: Formal analysis, Software, Writing – review & editing. PW: Investigation, Methodology, Writing – review & editing. LW: Data curation, Formal analysis, Methodology, Writing – review & editing. SJ: Data curation, Formal analysis, Methodology, Writing – review & editing. XL: Data curation, Formal analysis, Methodology, Writing – review & editing. JC: Investigation, Methodology, Writing – review & editing. QuZ: Data curation, Methodology, Writing – review & editing.
Funding
The author(s) declare that financial support was received for the research, authorship, and/or publication of this article. The authors are grateful to the financial supports from Postgraduate Education Reform and Quality Improvement Project of Henan Province (YJS2023JD18), Xinxiang Key Scientific and Technological Projects (22ZD011), Henan Key Research and Development Project (241111113700).
Conflict of interest
FL, XW, LX were employed by Henan PUAI Feed Co., Ltd. QZ was employed by Henan Delin Biological Product Co., Ltd.
The remaining authors declare that the research was conducted in the absence of any commercial or financial relationships that could be construed as a potential conflict of interest.
Publisher’s note
All claims expressed in this article are solely those of the authors and do not necessarily represent those of their affiliated organizations, or those of the publisher, the editors and the reviewers. Any product that may be evaluated in this article, or claim that may be made by its manufacturer, is not guaranteed or endorsed by the publisher.
Supplementary material
The Supplementary material for this article can be found online at: https://www.frontiersin.org/articles/10.3389/fsufs.2024.1487774/full#supplementary-material
Footnotes
References
Aperce, C. C., Burkey, T. E., KuKanich, B., Crozier-Dodson, B. A., Dritz, S. S., and Minton, J. E. (2010). Interaction of Bacillus species and Salmonella enterica serovar typhimurium in immune or inflammatory signaling from swine intestinal epithelial cells1. J. Anim. Sci. 88, 1649–1656. doi: 10.2527/jas.2009-2263
Arise, A. K., Alashi, A. M., Nwachukwu, I. D., Ijabadeniyi, O. A., Aluko, R. E., and Amonsou, E. O. (2016). Antioxidant activities of bambara groundnut (vigna subterranea) protein hydrolysates and their membrane ultrafiltration fractions. Food Funct. 7, 2431–2437. doi: 10.1039/c6fo00057f
Ashida, H., Ogawa, M., Kim, M., Mimuro, H., and Sasakawa, C. (2012). Bacteria and host interactions in the gut epithelial barrier. Nat. Chem. Biol. 8, 36–45. doi: 10.1038/nchembio.741
Bhattacharyya, A., Chattopadhyay, R., Mitra, S., and Crowe, S. E. (2014). Oxidative stress: an essential factor in the pathogenesis of gastrointestinal mucosal diseases. Physiol. Rev. 94, 329–354. doi: 10.1152/physrev.00040.2012
Boudry, G., Péron, V., Le Huërou-Luron, I., Lallès, J. P., and Sève, B. (2004). Weaning induces both transient and long-lasting modifications of absorptive, secretory, and barrier properties of piglet intestine. J. Nutr. 134, 2256–2262. doi: 10.1093/jn/134.9.2256
Brosnahan, A. J., and Brown, D. R. (2012). Porcine IPEC-J2 intestinal epithelial cells in microbiological investigations. Vet. Microbiol. 156, 229–237. doi: 10.1016/j.vetmic.2011.10.017
Cai, X., Chen, X., Wang, X., Xu, C., Guo, Q., Zhu, L., et al. (2013). Pre-protective effect of lipoic acid on injury induced by H2O2 in IPEC-J2 cells. Mol. Cell. Biochem. 378, 73–81. doi: 10.1007/s11010-013-1595-9
Das, S. K., and Vasudevan, D. M. (2007). Alcohol-induced oxidative stress. Life Sci. 81, 177–187. doi: 10.1016/j.lfs.2007.05.005
Demmig-Adams, B., and Adams, W. W. (2002). Antioxidants in photosynthesis and human nutrition. Science 298, 2149–2153. doi: 10.1126/science.1078002
Diesing, A. K., Nossol, C., Dänicke, S., Walk, N., Post, A., Kahlert, S., et al. (2011). Vulnerability of polarised intestinal porcine epithelial cells to mycotoxin deoxynivalenol depends on the route of application. PLoS One 6:e17472. doi: 10.1371/journal.pone.0017472
Farvin, K. H. S., Andersen, L. L., Otte, J., Nielsen, H. H., Jessen, F., and Jacobsen, C. (2016). Antioxidant activity of cod (Gadus morhua) protein hydrolysates: fractionation and characterisation of peptide fractions. Food Chem. 204, 409–419. doi: 10.1016/j.foodchem.2016.02.145
Geens, M. M., and Niewold, T. A. (2011). Optimizing culture conditions of a porcine epithelial cell line IPEC-J2 through a histological and physiological characterization. Cytotechnology 63, 415–423. doi: 10.1007/s10616-011-9362-9
Guo, Y. J., Zhang, T., Jiang, B., Miao, M., and Mu, W. M. (2014). The effects of an antioxidative pentapeptide derived from chickpea protein hydrolysates on oxidative stress in Caco-2 and HT-29 cell lines. J. Funct. Foods 7, 719–726. doi: 10.1016/j.jff.2013.12.013
Halici, M., Imik, H., Koç, M., and Gümüs, R. (2012). Effects of α-lipoic acid, vitamins E and C upon the heat stress in japanese quails. Z. Tierphysiol. Tierernahr. Futtermittelkd. 96, 408–415. doi: 10.1111/j.1439-0396.2011.01156.x
Hu, C. H., Xiao, K., Luan, Z. S., and Song, J. (2013). Early weaning increases intestinal permeability, alters expression of cytokine and tight junction proteins, and activates mitogen-activated protein kinases in pigs. J. Anim. Sci. 91, 1094–1101. doi: 10.2527/jas.2012-5796
Je, J. Y., Park, S. Y., Hwang, J. Y., and Ahn, C. B. (2015). Amino acid composition and in vitro antioxidant and cytoprotective activity of abalone viscera hydrolysate. J. Funct. Foods 16, 94–103. doi: 10.1016/j.jff.2015.04.023
Kaspar, J. W., Niture, S. K., and Jaiswal, A. K. (2009). Nrf2:INrf2 (Keap1) signaling in oxidative stress. J. Free Radic. Biol. Med. 47, 1304–1309. doi: 10.1016/j.freeradbiomed.2009.07.035
Kaur, G., Alam, M. S., Jabbar, Z., Javed, K., and Athar, M. (2006). Evaluation of antioxidant activity of Cassia siamea flowers. J. Ethnopharmacol. 108, 340–348. doi: 10.1016/j.jep.2006.05.021
Liu, J. B., Chen, Z. F., He, J., Zhang, Y., Zhang, T., and Jiang, Y. Q. (2014). Anti-oxidative and anti-apoptosis effects of egg white peptide, trp-asn-trp-ala-asp, against H2O2-induced oxidative stress in human embryonic kidney 293 cells. Food Funct. 5, 3179–3188. doi: 10.1039/c4fo00665h
Liu, Y. Y., Fatheree, N. Y., Mangalat, N., and Rhoads, J. M. (2010). Human-derived probiotic strains differentially reduce intestinal inflammation. Am J Physiol-Gastr L. 299, G1087–G1096. doi: 10.1152/ajpgi.00124.2010
Livak, K. J., and Schmittgen, T. D. (2001). Analysis of relative gene expression data using real-time quantitative PCR and the 2−ΔΔCT method. Methods 25, 402–408. doi: 10.1006/meth.2001.1262
Mine, Y., Young, D., and Yang, C. B. (2015). Antioxidative stress effect of phosphoserine dimers is mediated via activation of the Nrf2 signaling pathway. Mol. Nutr. Food Res. 59, 303–314. doi: 10.1002/mnfr.201400381
Moure, A., Domínguez, H., and Parajó, J. C. (2006). Antioxidant properties of ultrafiltration-recovered soy protein fractions from industrial effluents and their hydrolysates. Process Biochem. 41, 447–456. doi: 10.1016/j.procbio.2005.07.014
Paszti-Gere, E., Csibrik-Nemeth, E., Szeker, K., Csizinszky, R., Jakab, C., and Galfi, P. (2012). Acute oxidative stress affects IL-8 and TNF-α expression in IPEC-J2 porcine epithelial cells. Inflammation 35, 994–1004. doi: 10.1007/s10753-011-9403-8
Peña-Ramos, E. A., and Xiong, Y. L. (2002). Antioxidant activity of soy protein hydrolysates in a liposomal system. J. Food Sci. 67, 2952–2956. doi: 10.1111/j.1365-2621.2002.tb08844.x
Saberi, B., Shinohara, M., Ybanez, M. D., Hanawa, N., Gaarde, W. A., Kaplowitz, N., et al. (2008). Regulation of H2O2-induced necrosis by pkc and amp-activated kinase signaling in primary cultured hepatocytes. Am. J. Physiol. Cell Physiol. 295, C50–C63. doi: 10.1152/ajpcell.90654.2007
Sarmadi, B. H., and Ismail, A. (2010). Antioxidative peptides from food proteins: a review. Peptides 31, 1949–1956. doi: 10.1016/j.peptides.2010.06.020
Shi, Y. N., Kovacs-Nolan, J., Jiang, B., Tsao, R., and Mine, Y. (2014). Antioxidant activity of enzymatic hydrolysates from eggshell membrane proteins and its protective capacity in human intestinal epithelial Caco-2 cells. J. Funct. Foods 10, 35–45. doi: 10.1016/j.jff.2014.05.004
Song, Z. D., Li, H. Y., Wang, J. Y., Li, P. Y., Sun, Y. Z., and Zhang, L. M. (2014). Effects of fishmeal replacement with soy protein hydrolysates on growth performance, blood biochemistry, gastrointestinal digestion and muscle composition of juvenile starry flounder (Platichthys stellatus). Aquaculture 426-427, 96–104. doi: 10.1016/j.aquaculture.2014.01.002
Svobodová, A., Walterová, D., and Psotová, J. (2006). Influence of silymarin and its flavonolignans on H2O2-induced oxidative stress in human keratinocytes and mouse fibroblasts. Burns 32, 973–979. doi: 10.1016/j.burns.2006.04.004
Takenaka, A., Annaka, H., Kimura, Y., Aoki, H., and Igarashi, K. (2003). Reduction of paraquat-induced oxidative stress in rats by dietary soy peptide. Biosci. Biotech. Bioch. 67, 278–283. doi: 10.1271/bbb.67.278
Ulluwishewa, D., Anderson, R. C., McNabb, W. C., Moughan, P. J., Wells, J. M., and Roy, N. C. (2011). Regulation of tight junction permeability by intestinal bacteria and dietary components. J. Nutr. 141, 769–776. doi: 10.3945/jn.110.135657
Valko, M., Leibfritz, D., Moncol, J., Cronin, M. T. D., Mazur, M., and Telser, J. (2007). Free radicals and antioxidants in normal physiological functions and human disease. Int. J. Biochem. Cell Biol. 39, 44–84. doi: 10.1016/j.biocel.2006.07.001
Wang, D., Wang, L. J., Zhu, F. X., Zhu, J. Y., Chen, X. D., Zou, L., et al. (2008). In vitro and in vivo studies on the antioxidant activities of the aqueous extracts of douchi (a traditional chinese salt-fermented soybean food). Food Chem. 107, 1421–1428. doi: 10.1016/j.foodchem.2007.09.072
Wang, B., Xie, N. N., and Li, B. (2016). Charge properties of peptides derived from casein affect their bioavailability and cytoprotection against H2O2-induced oxidative stress. J. Dairy Sci. 99, 2468–2479. doi: 10.3168/jds.2015-10029
Wen, L. R., Bi, H. M., Zhou, X. S., Jiang, Y. M., Zhu, H., Fu, X., et al. (2022). Structure characterization of soybean peptides and their protective activity against intestinal inflammation. Food Chem. 387:132868. doi: 10.1016/j.foodchem.2022.132868
Xiang, X. W., Zhang, Z. S., Zhou, Y. F., Zhou, T. Y., Sun, P. L., and Zheng, B. (2020). Protective effect of seleno-amino-oligosaccharide on oxidative damage of IPEC-1 cells by activating Keap1/Nrf2 signaling pathway. Int. J. Biol. Macromol. 155, 972–978. doi: 10.1016/j.ijbiomac.2019.11.057
Xun, W., Fu, Q., Shi, L., Cao, T., Jiang, H., and Ma, Z. (2021). Resveratrol protects intestinal integrity, alleviates intestinal inflammation and oxidative stress by modulating Ahr/Nrf2 pathways in weaned piglets challenged with diquat. Int. Immunopharmacol. 99:107989. doi: 10.1016/j.intimp.2021.107989
Yan, Q. J., Huang, L. H., Sun, Q., Jiang, Z. Q., and Wu, X. (2015). Isolation, identification and synthesis of four novel antioxidant peptides from rice residue protein hydrolyzed by multiple proteases. Food Chem. 179, 290–295. doi: 10.1016/j.foodchem.2015.01.137
Zhang, Y., Chen, S., Zong, X., Wang, C., Shi, C. Y., Wang, F. Q., et al. (2020). Peptides derived from fermented soybean meal suppresses intestinal inflammation and enhances epithelial barrier function in piglets. Food Agric. Immunol. 31, 120–135. doi: 10.1080/09540105.2019.1705766
Zhang, B. K., and Guo, Y. M. (2009). Supplemental zinc reduced intestinal permeability by enhancing occludin and zonula occludens protein-1 (ZO-1) expression in weaning piglets. Brit J Nutr. 102, 687–693. doi: 10.1017/S0007114509289033
Keywords: soybean peptides, oxidative stress, IPEC-J2 cells, antioxidant capacity, gene expressions
Citation: Lu F, Yin Q, Wang X, Xu L, Liu M, Zhang Q, Sheng H, Liu C, Wang P, Wang L, Jin S, Li X, Chang J and Zhu Q (2024) Protective effects of soybean peptides on H2O2-induced oxidative injury in IPEC-J2 cells. Front. Sustain. Food Syst. 8:1487774. doi: 10.3389/fsufs.2024.1487774
Edited by:
Baohua Wang, Nantong University, ChinaReviewed by:
Muthukumar Serva Peddha, Central Food Technological Research Institute (CSIR), IndiaXinjian Shi, Henan University, China
Copyright © 2024 Lu, Yin, Wang, Xu, Liu, Zhang, Sheng, Liu, Wang, Wang, Jin, Li, Chang and Zhu. This is an open-access article distributed under the terms of the Creative Commons Attribution License (CC BY). The use, distribution or reproduction in other forums is permitted, provided the original author(s) and the copyright owner(s) are credited and that the original publication in this journal is cited, in accordance with accepted academic practice. No use, distribution or reproduction is permitted which does not comply with these terms.
*Correspondence: Qingqiang Yin, cXF5MTk2NEBoZW5hdS5lZHUuY24=; Ping Wang, d2FuZ3Bpbmc4NTA1MTZAMTYzLmNvbQ==