- 1Department of Food Science and Technology, Faculty of Agriculture, Urmia University, Urmia, Iran
- 2Department of Biology, Central Tehran Branch, Islamic Azad University, Tehran, Iran
- 3Helsinki Institute of Sustainability Science (HELSUS), University of Helsinki, Helsinki, Finland
- 4Department of Food and Nutrition, University of Helsinki, Helsinki, Finland
- 5HAMK Bio Research Unit, Häme University of Applied Sciences, Hämeenlinna, Finland
- 6Department of Microbiology, University of Helsinki, Helsinki, Finland
Biosynthesizing conjugated linoleic acid (CLA) using food processing by-products offers a practical and efficient method for producing this valuable compound, delivering health benefits, promoting sustainability, and providing economic advantages. Here, we optimized the fermentation conditions for in situ biosynthesis of CLA using Bacillus coagulans IBRC-M 10807, with grapeseed oil by-product providing the linoleic acid precursor, focusing on temperature, pH, incubation time, concentrations of grapeseed oil and yeast extract. The total CLA was measured using a UV–Vis spectrophotometer at 233 nm, and the concentration was evaluated using a standard curve of conjugated linoleic acid. To analyze the isomers and identify the functional groups of biosynthesized CLA, gas chromatography (GC) and Fourier-transform infrared spectroscopy (FTIR) were used, respectively. The results indicated that the maximum amount of CLA production was 225.37 ± 0.74 μg/mL, obtained under optimal conditions: an initial pH of 6.8, a temperature of 44.8°C, an incubation time of 48 h, a grapeseed oil concentration of 1,000 μg/mL, and a yeast extract concentration of 3.88%. Characterization of the produced CLA revealed two main isomers—CLA 1: c-9, t-11 C18:2, and CLA 2: t-10, c-12 C18:2 methyl esters. These results suggest that B. coagulans has high potential to produce CLA as a bioactive compound, offering an excellent opportunity for use in various industries, including nutraceuticals, foods, pharmaceuticals, cosmetics, and animal feeds.
1 Introduction
Cardiovascular disease is a major global health concern and a leading cause of mortality, but it can be prevented through a healthy and nutritious diet (Sikand et al., 2015; Adefegha, 2018). Recently, functional foods have gained popularity for their high nutritional value and health benefits, including protection against cardiovascular disease (Maqsood et al., 2020; Silva et al., 2020). These foods either naturally contain beneficial compounds or are enriched with various bioactive substances (Ribeiro et al., 2019), making them rich in nutrients like calcium, vitamins, zinc, omega-3 fatty acids, and conjugated linoleic acid (CLA). CLA, with its main isomers cis-9, trans-11 (c-9, t-11) and trans-10, cis-12 (t-10, c-12) in a roughly 3:1 ratio, is recognized as a functional ingredient due to its health-promoting effects (Koronowicz and Banks, 2018; Słowikowski et al., 2020). CLA is a heterogeneous unsaturated fatty acid with conjugated double bonds in various regions of its carbon chain (Trigueros and Sendra, 2015; Basak and Duttaroy, 2020). It has been generally recognized as safe—GRAS (Razmjooei et al., 2020), and exhibits anti-cancer, anti-atherogenic, anti-obesity and anti-inflammatory properties, potentially preventing chronic conditions such as cancer, liver steatosis, and non-alcoholic fatty liver disease. Recent studies suggest CLA can reduce tumor cell growth and inhibit cancer, although its mechanism of action remains unclear (Koronowicz and Banks, 2018). Due to its specific health benefits and biological functions, CLA is used as a functional ingredient (Jalilian et al., 2021). It is predominantly found in animal fats, with CLA content ranging from 0.12 to 0.68% in red meat and from 0.34 to 1.07% in dairy products, relative to total fat (Razmjooei et al., 2020). CLA in these products results from the isomerization of linoleic acid to conjugated linoleic acid by gram-positive bacteria in the gastrointestinal tract of ruminants such as Ruminococcus albus, Butyrivibrio fibrisolvens, and subspecies of Eubacterium (Liu et al., 2017). However, the CLA content in meat and dairy products is too low to confer significant health benefits, making bacterial biosynthesis of CLA an attractive method for commercial production (Razmjooei et al., 2020).
Bacillus coagulans, known for its spore-producing ability, has gained attention for its probiotic potential (Drago and De Vecchi, 2009). The U.S. Food and Drug Administration (FDA) has confirmed its safety for probiotic use, and it is also recognized as a safe species by the European Union Food Safety Authority. Research has demonstrated the safety and health advantages of various B. coagulans strains (Endres et al., 2009). Among these, B. coagulans IBRC-M 10807 is noted for its significant production of exopolysaccharide with antioxidant properties, marking it as a beneficial probiotic (Farajinejad et al., 2023). However, research on utilizing B. coagulans IBRC-M 10807 to enhance the co-production of bioactive compounds such as CLA remains scarce.
Due to the increasing amount of agro-industrial waste, a critical challenge in food bioprocessing, it is essential to explore methods to transform the food industry’s by-products into novel food products and ingredients with enhanced safety, functionality, and consumer appeal. Grapeseed oil, a by-product of the grape juice concentrate industry in West Azerbaijan province of Iran, contains a high percentage of linoleic acid, which is the primary precursor of CLA (Rajabi et al., 2023). Grapeseed oil contains 72–76% (w/w) linoleic acid, significantly higher than other common oils like safflower oil (70–72%), sunflower oil (60–62%), and corn oil (52%) (Cao and Ito, 2003). This high linoleic acid content makes grapeseed oil an excellent candidate for producing conjugated linoleic acid (CLA), particularly through bioconversion processes using bacteria. Despite its potential, this application of grapeseed oil remains largely unexplored. The high availability of linoleic acid in grapeseed oil could lead to efficient and cost-effective CLA production, offering substantial benefits for nutraceutical and functional food industries. Exploring this bioconversion pathway could enhance the economic value of grapeseed oil, a byproduct of the wine and juice industry, and contribute to the development of health-promoting food ingredients. In this study, we optimized CLA production with B. coagulans IBRC-M 10807 using grapeseed oil, extracted from grape seeds, as a pre-source of linoleic acid. Various conditions were tested based on a statistical design to identify the optimal parameters for maximizing CLA yield. By leveraging the high linoleic acid content of grapeseed oil, we aimed to enhance the efficiency of the bioconversion process.
The study aimed to optimize the fermentation condition for in situ biosynthesis of CLA using B. coagulans IBRC-M 10807, with grapeseed oil by-product providing the linoleic acid precursor, focusing on temperature, pH, incubation time, concentrations of grapeseed oil, and yeast extract. Although, there are a lot of study in the literature that already highlight the conversion rate of LA to CLA by different probiotic strains, it is the first study using Sardasht black grape seeds oil as a pre-source for CLA biosynthesize with B. coagulans IBRC-M 10807 as a spore forming probiotic bacterium.
2 Materials and methods
2.1 Materials and microorganisms
Sardasht black grape seeds were obtained from the Pakdis juice factory (Urmia, Iran). The kernels were dried for 2 h at 50°C to reach a moisture content of 6–7% and then stored in airtight packaging for further use. B. coagulans IBRC-M 10807 was obtained in freeze-dried form from the Iranian Biological Research Center (Tehran, Iran). Peptone from casein, glucose monohydrate, sodium chloride, yeast extract, magnesium chloride, calcium chloride, manganese chloride, Tween 80, hexane, chloroform, methanol, sodium sulfate, and isopropanol were purchased from Merck (Darmstadt, Germany). All chemicals are of analytical grade. Conjugated linoleic acid with 99% purity obtained from Sigma-Aldrich (St. Louis, Missouri, USA) was used as standard for determining CLA production.
Bacillus coagulans IBRC-M 10807 was grown for 37 h at 37°C, utilizing Nutrient Yeast Extract Salt Medium (NYSM) including glucose monohydrate (10 g/L), peptone from casein (5 g/L), sodium chloride (5 g/L), yeast extract (2–4%), magnesium chloride (0.2 g/L), calcium chloride (0.1 g/L), and manganese chloride (0.01 g/L), with 1% w/v Tween 80. After cultivation, the cultures were stored at 4°C and underwent three successive sub-culturing cycles within the same medium before each experiment.
2.2 Extraction of grapeseed oil
The Soxhlet method and hexane solvent were used to extract oil from the dried kernels following Baydar et al. (2007). Briefly, dried seeds were ground in a grinder for 2 min, with 15-s intervals to prevent overheating the sample. Four grams of the powdered grape seeds were extracted using a Soxhlet extractor (Büchi Universal Extraction System B-811, Germany) for 6 h with 150 mL of hexane at 60°C.
2.3 Preparation of inoculum
Bacillus coagulans IBRC-M 10807 was cultured in NYSM broth under sterile conditions, and incubated for 24 h at 37°C. The culture medium was centrifuged at 5000 × g for 20 min and washed twice in 0.85% w/v sodium chloride solution. The final pellet containing the bacterial cells was added to sterile saline solution to reach a suspension with 1010 CFU/mL B. coagulans IBRC-M 10807 (Amiri et al., 2020).
2.4 Experimental design to optimize the CLA productions and statistical analysis
NYSM broth was used to produce CLA by B. coagulans IBRC-M 10807. The pH of the culture medium was first adjusted using 1 N hydrochloric acid according to the statistical design, and the media was then sterilized at 121°C for 15 min. Next, grapeseed oil was added to the mixture with a syringe filter (pore size = 0.45 μm). Finally, 1 mL of the suspension containing 1010 CFU/mL of B. coagulans IBRC-M 10807 bacteria was added to 100 mL of NYSM broth and incubated according to the factorial experimental design order to determine the most effective factors of CLA production (Sekhavatizadeh et al., 2019; Amiri et al., 2021a).
For this purpose, five independent variables were evaluated: initial pH (5, 6 and 7), temperature (35, 40 and 45°C), incubation time (18, 33 and 48 h), grapeseed oil concentration (0, 500 and 1,000 μg/mL), and yeast extract concentration (0, 2 and 4%, w/w), as shown in Table 1. Analysis of variance was used to analyze the significance of the differences among variables at α ≥ 0.05. Numerical optimization was used to predict the optimal conditions based on the desirability function. The experimental design, statistical analysis, and numerical optimization were conducted with Design-Expert software version 12 (State-Ease Minneapolis, MN, USA).
2.5 Determination of CLA concentration
Briefly, 10 mL of culture medium was centrifuged at 7,500 × g for 5 min at 4°C. Then, 6 mL of isopropanol (Merck, Darmstadt, Germany) was added to 3 mL of the supernatant and vortexed for 3 min. Next, 5 mL of hexane was added and vortexed for another 3 min. The mixture was centrifuged at 2,000 × g for 5 min at 4°C, and the total CLA was measured using a UV–Vis spectrophotometer (80–2088-64, Pharmacia LKB Biochrom, Cambridge, UK) at 233 nm against hexane as a blank. The CLA concentration was evaluated using a standard curve of conjugated linoleic acid at concentration of 0–30 mg/mL (Ribeiro et al., 2018).
2.6 Determination of produced CLA structure
Further characterization analyses were done on optimized sample to show the properties of bio produced CLA.
2.6.1 Isomer profile of produced CLA
Gas chromatography was used to analyze CLA isomers. Fatty acids of CLA were extracted using chloroform and methanol (2:1). The upper phase was washed with 1 mL of normal saline (0.85% w/v) and dried with sodium sulfate. It was then mixed with 1 mL of HCl methanolic solution (10% v/v) and heated at 50°C for 20 min. After cooling to room temperature, methyl esters of fatty acids were extracted with 1 mL of hexane. The sample was kept under nitrogen to prevent oxidation before chromatography. The gas chromatography model GC-2010 (Shimadzu, Kyoto, Japan) with column (Rtx-225, Restek, Bellefonte, PA, USA), crossbond 50% cyanopropylmethyl polysiloxane, 60 m × 0.25 mm/D, 0.25 μm depth fraction, analyzed the CLA isomers. Operating conditions were: starting temperature 70°C, increasing to 220°C at 4°C/min, helium carrier gas flow rate 1 mL/min, and detector and injector temperatures at 260°C (AOAC, 1990; Badee and Hidaka, 2014; Amiri et al., 2021b).
2.6.2 Functional groups of CLA
The Fourier-transform infrared (FTIR) spectrometer model TENSOR 27 (Billerica, Massachusetts, USA) was used to identify the functional groups of biosynthesized CLA. For this, 1 μL of CLA was added to a 100 mg KBr tablet, and the tablet was scanned from 3,500 to 500 cm−1 at a resolution of 4 cm−1 (Ji and Guo, 2018).
3 Results and discussion
3.1 CLA bio-production
Accordingly, the results of this study also showed that initial pH, temperature, incubation time, as well as grapeseed oil and yeast extract concentrations had a significant effect on CLA production by B. coagulans IBRC-M 10807. As such, the total content of CLA was significantly increased by increasing all of the above factors (p < 0.05). The R2 (coefficient of determination) and Adj-R2 (adjusted coefficient of determination) values for Equation 1 were 0.977 and 0.959, respectively.
Where it is final equation in terms of coded factors: A is Initial pH, B is Temperature (°C), C is Incubation Time (h), D is Linoleic acid (%), and E is Yeast Extract (%).
Previous research has shown that fermentation conditions such as pH, temperature, time and the LA content of the medium affect the in vitro production of CLA (Terán et al., 2015; Hussain et al., 2016; Renes et al., 2017).
According to Figure 1A, the CLA production increased significantly by increasing the initial pH (p < 0.05). This finding is in line with the results of Özer et al. (2016), who showed that the CLA production by Lactobacillus plantarum at pH 6 was more than that produced at pH 5.5 and 5.7. Ye et al. (2013) indicated that near-neutral pH levels have a direct impact on cell growth and nutritional metabolism, which enhances the strain’s isomerizing capability, thereby increasing the conversion of linoleic acid to CLA. Previous research has highlighted that pH is a critical factor influencing the activity of the linoleate isomerase enzyme and the molecular synthesis of CLA, potentially serving as a limiting factor in CLA production (Dahiya and Puniya, 2018; Gorissen et al., 2010; Salsinha et al., 2018).
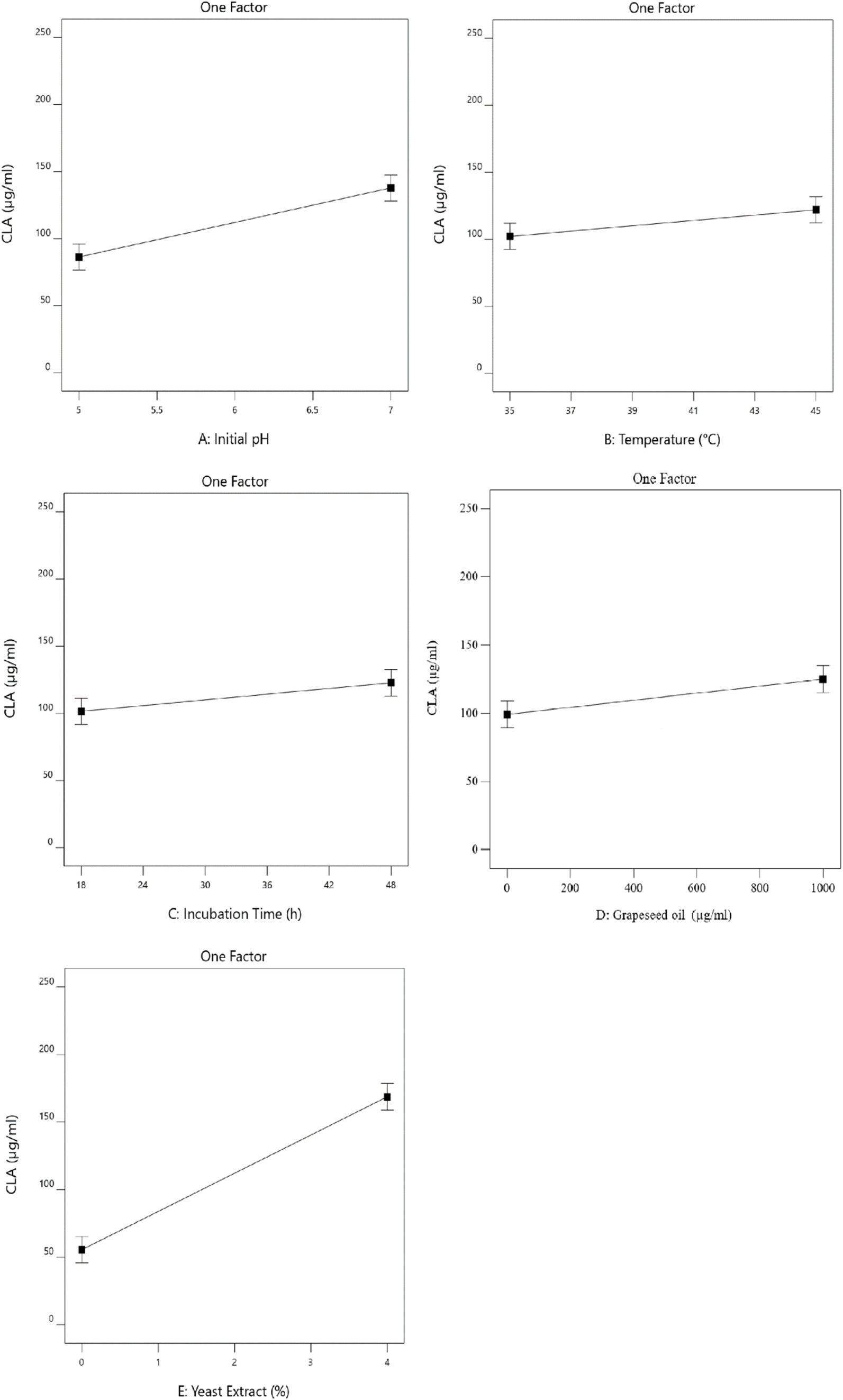
Figure 1. The effect of initial pH (A), temperature (B), incubation time (C), grapeseed oil concentration (D) and yeast extract concentration (E) on CLA production by B. coagulans.
In addition to pH, temperature is also one of the most important parameters for enzymatic reactions. In this study, it was shown that increasing the temperature caused an increase in the amount of CLA (Figure 1B). This is in agreement with the research of He et al. (2015), who reported that an increase in the reaction temperature from 20°C to 37°C improved the yield of CLA. However, a further increase in reaction temperature led to a decline in CLA production, which aligns with findings from previous similar studies. These studies suggested that higher fermentation temperatures can reduce linoleate isomerase enzyme activity and inhibit bacterial growth (Gorissen et al., 2010; Kishino et al., 2010).
Incubation time had a significant effect on CLA yield (Figure 1C), as there was a positive correlation (p < 0.05) between incubation time and amount of CLA produced. Hosseini et al. (2015) also showed that CLA1 (cis-9, trans-11) production increased during incubation for up to 72 h, but then decreased after 96 h; the CLA2 (trans-10, cis-12) ratio increased with prolonged time. In addition, Özer and Kiliç (2020) reported that the amount of CLA increased by increasing fermentation time up to 73–79 h for L. plantarum, but similar to others’ findings, a further increase in the fermentation time caused a decrease in CLA production. This might be explained by the fact that high CLA concentration may have a toxic effect and thus inhibit further bacterial growth (Gorissen et al., 2015; Özer and Kiliç, 2020). Substrate concentration of the medium is another important factor that influences CLA yield. As shown in Figure 1D, the CLA production by B. coagulans IBRC-M 10807 increased by increasing the grapeseed oil concentration as the source of LA. This increase could be due to the use of grape seed oil. Although increasing free LA can have an antimicrobial effect, grape seed oil is not a completely pure precursor of free LA. So, it is a suitable precursor that does not have an antimicrobial effect by increasing the concentration to a certain extent. According to the literature, LA up to 1,000 μg/mL has no antimicrobial effect of probiotics (Amiri et al., 2020). On the other hand, grapeseed oil contains about 72–76% of LA, therefore, at the maximum concentration used, it is less than the antimicrobial limit of LA (Cao and Ito, 2003; Amiri et al., 2020). Similarly, Khosravi et al. (2015) found that CLA production significantly increased with the addition of 1–3 mg/mL of substrate to the medium. Wang et al. (2022) reported that the conversion rate of LA to CLA by L. acidophilus gently decreased as LA increased from 0.5 mg/mL to 2.0 mg/mL. In addition, Zhao et al. (2011) stated that the maximum amount of CLA (275.7 μg/mL) was obtained in the mixture containing 2 mg/mL linoleic acid; with the addition of more substrate, CLA production decreased. This may be related to the change in enzyme structure, which affects its function or because of antimicrobial effect of free LA in high concentrations. Yeast extract is rich in nutrients such as peptides and amino acids, therefore it is commonly used as a source of nitrogen to improve bacterial growth and metabolite production (Dahiya and Puniya, 2018). As shown in Figure 1E, the CLA yield increased with increasing concentration of yeast extract. Khosravi et al. (2015) also concluded that the total CLA formation increased at higher concentrations of yeast extract. Furthermore, similar results reported by Hennessy et al. (2009) indicated that the increase in yeast extract caused an increase in bacterial growth and CLA production.
3.2 Numerical optimization
In this study, the conditions of CLA production by B. coagulans were optimized using desirability function. The optimal conditions for CLA production were found to be initial pH of 6.8, temperature of 44.8°C, incubation time of 48 h, grapeseed oil concentration of 1,000 μg/mL, and yeast extract concentration of 3.88% with the desirability value equal to 1.00. Next, five samples were cultivated under these optimized conditions. There was no significant difference (p > 0.05) between actual amount (225.37 ± 0.74 μg/mL) and predicted values (219.48 μg/mL) of total CLA (Figure 2). The transformation efficiency of converting LA into CLA by B. coagulans IBRC-M 10807 was 33.78%. Iorizzo et al. (2024) reported that the transformation efficiency of converting LA into CLA by Lactiplantibacillus plantarum NCUL005 was 26.67%, and Salsinha et al. (2018) reported that the rate of conversion of LA to CLA ranged from 17.00 to 35.90% in MRS broth containing 200 μg/mL LA which are in agreement with the result of present study.
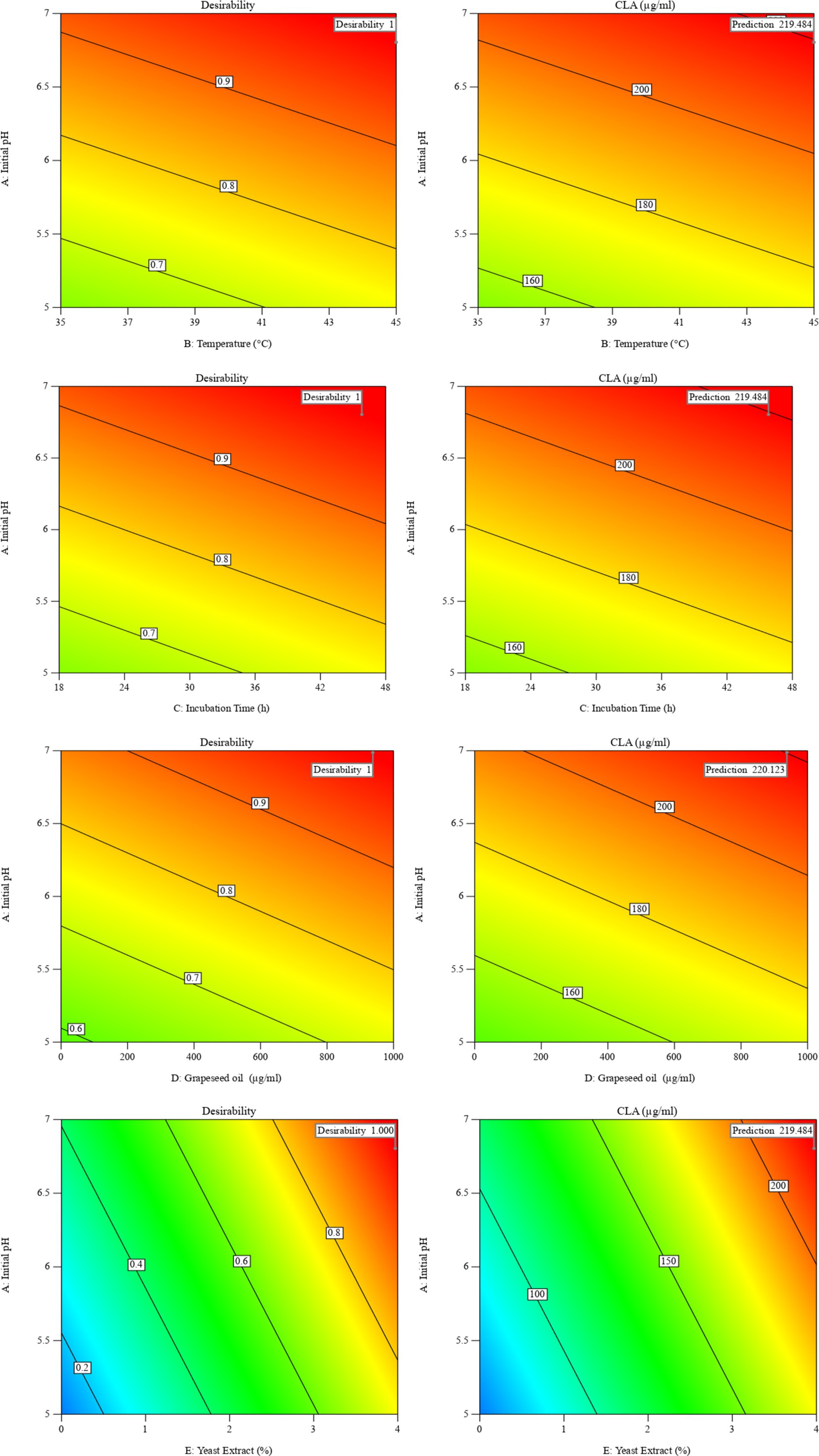
Figure 2. The interaction of temperature and initial pH on CLA production, and their desirability in optimal conditions.
3.3 GC analysis
Gas chromatography analysis of total fatty acid methyl esters was used to identify the composition of CLA produced by B. coagulans IBRC-M10807. Two main CLA isomers were detected – CLA 1: c-9, t-11 C18:2, and CLA 2: t-10, c-12 C18:2 methyl esters. The main representative CLA isomers are 9c,11 t-18:2 and 10 t,12c-18:2. CLA has been shown to exercise diverse potent health effects such as antiobese, anticarcinogenic, antihypertensive and antidiabetic properties. The health effects of CLA depend on its isomers, for example the 10 t,12c isomer is anticarcinogenic, antiobese and antidiabetic, whereas the 9c,11 t isomer is mainly anticarcinogenic. These results are in accordance with several previous studies (Amiri et al., 2020; Razmjooei et al., 2020). As shown in Figure 3, the amount of CLA 1 is higher than CLA 2. According to prior research, CLA 1 has a stronger anti-inflammatory effect, however CLA 2 is known as an anti-obesity product (Razmjooei et al., 2020).
3.4 FTIR analysis
An organic molecule is a set of almost independent functional groups whose properties determine the physical and chemical properties of the compound, therefore the analysis of the functional group of organic compounds is important in identifying the compound. In order to determine the functional groups of CLA produced by B. coagulans, Fourier-transform infrared spectroscopy was used (Figure 4). The absorption at 3258.19 cm−1 is due to a hydroxyl group (Costa et al., 2015), while the peak at 2934.53 cm−1 indicates hydrocarbon chain asymmetric fatty acids (Amiri et al., 2020). The produced CLA has a sharp peak at 1896.94 cm−1, which is related to carbonyl ester stretch (He et al., 2016), and the peak at 1084.91 cm−1 indicates the tensile strength of a symmetric C–O ester (Salas-Valerio et al., 2022). The absorption at 1100 cm−1 to 1,300 cm−1 is related to the stretch of the C=O and C=C bonds, and the peak at 946.24 cm−1 is a feature of the cis-trans conjugated bonds. Furthermore, the peak at 778.46 cm−1 is due to methylene vibrations, which indicates high-chain fatty acids in CLA produced by B. coagulans (Amiri et al., 2020). Also, the peaks at 772.97 cm−1 and 772.25 cm−1 are detected in the generated CLA by different probiotic bacteria. These peaks correspond to methylene vibrations, and signify a characteristic feature of long-chain fatty acids, as described by Roach et al. (2002). The obtained results are consistent with the results of Amiri et al. (2020) and indicate the bio-production of CLA isomers with health-giving properties by probiotic B. coagulans IBRC-M 10807 from grape seed oil as a precursor.
4 Conclusion
This study determined the effects of fermentation conditions on CLA production using B. coagulans IBRC-M 10807. The results showed that all evaluated factors including temperature, initial pH, incubation time, as well as grapeseed oil and yeast extract concentration significantly affected CLA formation (p < 0.05) and enhanced the production efficiency. This is the first report on the optimal requirements of CLA production containing two main isomers (CLA 1 and CLA 2) using B. coagulans IBRC-M 10807. The findings of this research are promising for the usage of B. coagulans IBRC-M 10807 in producing CLA with high utility in several industrial processes. Moreover, the industrial applications of CLA extend beyond the food sector. CLA is utilized in the production of nutritional supplements, pharmaceuticals, and cosmetics, owing to its purported health properties. By harnessing the efficiency of B. coagulans IBRC-M 10807 in CLA production, these industries can streamline their manufacturing processes, ensuring the availability of high-quality CLA for diverse applications.
Data availability statement
The raw data supporting the conclusions of this article will be made available by the authors, without undue reservation.
Author contributions
MR: Formal analysis, Investigation, Methodology, Writing – original draft, Writing – review & editing. SA: Conceptualization, Data curation, Formal analysis, Funding acquisition, Investigation, Methodology, Project administration, Resources, Software, Supervision, Validation, Visualization, Writing – original draft, Writing – review & editing. AA: Formal analysis, Methodology, Writing – original draft, Writing – review & editing. AK: Writing – review & editing. TH: Conceptualization, Visualization, Writing – review & editing. PS: Conceptualization, Visualization, Writing – review & editing. AY: Conceptualization, Visualization, Writing – review & editing.
Funding
The author(s) declare that financial support was received for the research, authorship, and/or publication of this article. Open access is funded by the Helsinki University Library.
Acknowledgments
We thank Dr. Mohammad Alizadeh Khaledabad from Urmia University who provided insight and expertise that greatly assisted the research.
Conflict of interest
The authors declare that the research was conducted in the absence of any commercial or financial relationships that could be construed as a potential conflict of interest.
The author(s) declared that they were an editorial board member of Frontiers, at the time of submission. This had no impact on the peer review process and the final decision.
Publisher’s note
All claims expressed in this article are solely those of the authors and do not necessarily represent those of their affiliated organizations, or those of the publisher, the editors and the reviewers. Any product that may be evaluated in this article, or claim that may be made by its manufacturer, is not guaranteed or endorsed by the publisher.
References
Adefegha, S. A. (2018). Functional foods and nutraceuticals as dietary intervention in chronic diseases; novel perspectives for health promotion and disease prevention. J. Diet. Suppl. 15, 977–1009. doi: 10.1080/19390211.2017.1401573
Amiri, S., Mokarram, R. R., Khiabani, M. S., Bari, M. R., and Alizadeh, M. (2021a). Optimization of food-grade medium for co-production of bioactive substances by Lactobacillus acidophilus LA-5 for explaining pharmabiotic mechanisms of probiotic. J. Food Sci. Technol. 58, 1–12. doi: 10.1007/s13197-020-04894-5
Amiri, S., Mokarram, R. R., Khiabani, M. S., Bari, M. R., and Khaledabad, M. A. (2020). In situ production of conjugated linoleic acid by Bifidobacterium lactis BB12 and Lactobacillus acidophilus LA5 in milk model medium. LWT Food Sci. Technol. 132:109933. doi: 10.1016/j.lwt.2020.109933
Amiri, S., Rezazadeh-Bari, M., Alizadeh-Khaledabad, M., Rezaei-Mokarram, R., and Sowti-Khiabani, M. (2021b). Fermentation optimization for co-production of postbiotics by Bifidobacterium lactis BB12 in cheese whey. Waste Biom. Valori. 12, 5869–5884. doi: 10.1007/s12649-021-01429-7
AOAC (1990). Official methods of analysis. 15th Edn. Washington DC: Association of Official Analytical Chemists.
Badee, G., and Hidaka, S. (2014). Growth performance, carcass characteristics, fatty acid composition and CLA concentrations of lambs fed diets supplemented with different oil sources. Anim. Sci. J. 85, 118–126. doi: 10.1111/asj.12094
Basak, S., and Duttaroy, A. K. (2020). Conjugated linoleic acid and its beneficial effects in obesity, cardiovascular disease, and cancer. Nutrients 12:1913. doi: 10.3390/nu12071913
Baydar, N. G., Özkan, G., and Çetin, E. S. (2007). Characterization of grape seed and pomace oil extracts. Grasas Aceites 58, 29–33. doi: 10.3989/gya.2007.v58.i1.5
Cao, X., and Ito, Y. (2003). Supercritical fluid extraction of grape seed oil and subsequent separation of free fatty acids by high-speed counter-current chromatography. J. Chromatogr. 1021, 117–124. doi: 10.1016/j.chroma.2003.09.001
Costa, A. M., Nunes, J., Lima, B., Pedrosa, C., Calado, V., Torres, A., et al. (2015). Effective stabilization of CLA by microencapsulation in pea protein. Food Chem. 168, 157–166. doi: 10.1016/j.foodchem.2014.07.016
Dahiya, D. K., and Puniya, A. K. (2018). Optimisation of fermentation variables for conjugated linoleic acid bioconversion by Lactobacillus fermentum DDHI 27 in modified skim milk. Int. J. Dairy Technol. 71, 46–55. doi: 10.1111/1471-0307.12375
Drago, L., and De Vecchi, E. (2009). Should Lactobacillus sporogenes and Bacillus coagulans have a future? J. Chemother. 21, 371–377. doi: 10.1179/joc.2009.21.4.371
Endres, J. R., Clewell, A., Jade, K. A., Farber, T., Hauswirth, J., and Schauss, A. G. (2009). Safety assessment of a proprietary preparation of a novel probiotic, Bacillus coagulans, as a food ingredient. FCT 47, 1231–1238. doi: 10.1016/j.fct.2009.02.018
Farajinejad, Z., Mohtarami, F., Pirouzifard, M., Amiri, S., and Hamishehkar, H. (2023). In situ produced exopolysaccharides by Bacillus coagulans IBRC-M 10807 and its effects on properties of whole wheat sourdough. Food Sci. Nutr. 11, 7000–7012. doi: 10.1002/fsn3.3624
Gorissen, L., Leroy, F., de Vuyst, L., de Smet, S., and Raes, K. (2015). Bacterial production of conjugated linoleic and linolenic acid in foods: a technological challenge. Crit. Rev. Food Sci. Nutr. 55, 1561–1574. doi: 10.1080/10408398.2012.706243
Gorissen, L., Raes, K., Weckx, S., Dannenberger, D., Leroy, F., De Vuyst, L., et al. (2010). Production of conjugated linoleic acid and conjugated linolenic acid isomers by Bifidobacterium species. Appl. Microbiol. Biotechnol. 87, 2257–2266. doi: 10.1007/s00253-010-2713-1
He, H., Hong, Y., Gu, Z., Liu, G., Cheng, L., and Li, Z. (2016). Improved stability and controlled release of CLA with spray-dried microcapsules of OSA-modified starch and xanthan gum. Carbohydr. Polym. 147, 243–250. doi: 10.1016/j.carbpol.2016.03.078
He, X., Shang, J., Li, F., and Liu, H. (2015). Yeast cell surface display of linoleic acid isomerase from Propionibacterium acnes and its application for the production of trans-10, cis-12 conjugated linoleic acid. Biotechnol. Appl. Biochem. 62, 1–8. doi: 10.1002/bab.1249
Hennessy, A., Ross, R., Devery, R., and Stanton, C. (2009). Optimization of a reconstituted skim milk based medium for enhanced CLA production by bifidobacteria. J. Appl. Microbiol. 106, 1315–1327. doi: 10.1111/j.1365-2672.2008.04098.x
Hosseini, E. S., Kermanshahi, R. K., Hosseinkhani, S., Shojaosadati, S. A., and Nazari, M. (2015). Conjugated linoleic acid production from various substrates by probiotic Lactobacillus plantarum. Ann. Microbiol. 65, 27–32. doi: 10.1007/s13213-014-0832-0
Hussain, S. A., Srivastava, A., Tyagi, A., Shandilya, U. K., Kumar, A., Kumar, S., et al. (2016). Characterization of CLA-producing Butyrivibrio spp. reveals strain-specific variations. 3 Biotech 6, 90–11. doi: 10.1007/s13205-016-0401-2
Iorizzo, M., Di Martino, C., Letizia, F., Crawford, T. W. Jr., and Paventi, G. (2024). Production of conjugated linoleic acid (CLA) by Lactiplantibacillus plantarum: a review with emphasis on fermented foods. Foods 13:975. doi: 10.3390/foods13070975
Jalilian, A., Golmohammadi, T., Meshkani, R., Koushki, M., Eivazi, N., Khorzoughi, R. B., et al. (2021). Evaluating the effect of a mixture of two main conjugated linoleic acid isomers on hepatic steatosis in hepg2 cellular model. Mol. Biol. Rep. 48, 1359–1370. doi: 10.1007/s11033-021-06203-5
Ji, X., and Guo, M. (2018). Preparation and properties of a chitosan-lignin wood adhesive. Int. J. Adhes. Adhes. 82, 8–13. doi: 10.1016/j.ijadhadh.2017.12.005
Khosravi, A., Safari, M., Khodaiyan, F., and Gharibzahedi, S. M. T. (2015). Bioconversion enhancement of conjugated linoleic acid by Lactobacillus plantarum using the culture media manipulation and numerical optimization. J. Food Sci. Technol. 52, 5781–5789. doi: 10.1007/s13197-014-1699-6
Kishino, S., Ogawa, J., Ando, A., Yokozeki, K., and Shimizu, S. (2010). Microbial production of conjugated gamma-linolenic acid from gamma-linolenic acid by Lactobacillus plantarum AKU 1009a. J. Appl. Microbiol. 108, 2012–2018. doi: 10.1111/j.1365-2672.2009.04609.x
Koronowicz, A. A., and Banks, P. (2018). Antitumor properties of CLA-enriched food products. Nutr. Cancer 70, 529–545. doi: 10.1080/01635581.2018.1460684
Liu, S., Wang, Z., Xu, X., Ding, Y., and Guo, Z. (2017). Production of conjugated linoleic acid-rich cottonseed oil by supported Ru catalyzed isomerization. Ind. Crop. Prod. 97, 10–20. doi: 10.1016/j.indcrop.2016.12.004
Maqsood, S., Adiamo, O., Ahmad, M., and Mudgil, P. (2020). Bioactive compounds from date fruit and seed as potential nutraceutical and functional food ingredients. Food Chem. 308:125522. doi: 10.1016/j.foodchem.2019.125522
Özer, C. O., and Kiliç, B. (2020). Utilization of optimized processing conditions for high yield synthesis of conjugated linoleic acid by L. plantarum AB20–961 and L. plantarum DSM2601 in semi-dry fermented sausage. Meat Sci. 169:108218. doi: 10.1016/j.meatsci.2020.108218
Özer, C. O., Kiliç, B., and Kiliç, G. B. (2016). In-vitro microbial production of conjugated linoleic acid by probiotic L. plantarum strains: utilization as a functional starter culture in sucuk fermentation. Meat Sci. 114, 24–31. doi: 10.1016/j.meatsci.2015.12.005
Rajabi, M., Amiri, S., and Rezazadeh-Bari, M. (2023). Optimization of hesperidin extraction using hot methanol method assisted with ultrasound waves from the peel wastes of bitter orange (Citrus aurantium) and Persian orange (Citrus reticulata). J. Food Measur. Charact. 17, 5582–5593. doi: 10.1007/s11694-023-02070-9
Razmjooei, M., Shad, E., Nejadmansouri, M., Safdarianghomsheh, R., Delvigne, F., and Khalesi, M. (2020). Effect of metal support and different carbon sources on CLA production using Lactobacillus plantarum. Biochem. Eng. J. 162:107715. doi: 10.1016/j.bej.2020.107715
Renes, E., Linares, D., González, L., Fresno, J., Tornadijo, M., and Stanton, C. (2017). Production of conjugated linoleic acid and gamma-aminobutyric acid by autochthonous lactic acid bacteria and detection of the genes involved. J. Funct. Foods 34, 340–346. doi: 10.1016/j.jff.2017.05.014
Ribeiro, A. R., Altintzoglou, T., Mendes, J., Nunes, M. L., Dinis, M. T., and Dias, J. (2019). Farmed fish as a functional food: perception of fish fortification and the influence of origin–insights from Portugal. Aquaculture 501, 22–31. doi: 10.1016/j.aquaculture.2018.11.002
Ribeiro, S. C., Stanton, C., Yang, B., Ross, R. P., and Silva, C. C. (2018). Conjugated linoleic acid production and probiotic assessment of Lactobacillus plantarum isolated from Pico cheese. LWT 90, 403–411. doi: 10.1016/j.lwt.2017.12.065
Roach, J. A., Mossoba, M. M., Yurawecz, M. P., and Kramer, J. K. (2002). Chromatographic separation and identification of conjugated linoleic acid isomers. Anal. Chim. Acta 465, 207–226. doi: 10.1016/S0003-2670(02)00193-9
Salas-Valerio, W. F., Aykas, D. P., Sakoda, B. A. H., Ludena-Urquizo, F. E., Ball, C., Plans, M., et al. (2022). In-field screening of trans-fat levels using mid-and near-infrared spectrometers for butters and margarines commercialized in the Peruvian market. LWT 157:113074. doi: 10.1016/j.lwt.2022.113074
Salsinha, A. S., Pimentel, L. L., Fontes, A. L., Gomes, A. M., and Rodriguez-Alcala, L. M. (2018). Microbial production of conjugated linoleic acid and conjugated linolenic acid relies on a multienzymatic system. Microbiol. Mol. Biol. Rev. 82, e00019–e00018. doi: 10.1128/MMBR.00019-18
Sekhavatizadeh, S. S., Aminlari, M., Gheisari, H. R., Shekarforoush, S. S., and Golmakani, M. T. (2019). Physicochemical and microbiological properties of probiotic kashk containing Bacillus coagulans. J. Consum. Prot. Food Sci. 14, 377–387. doi: 10.1007/s00003-019-01226-4
Sikand, G., Kris-Etherton, P., and Boulos, N. M. (2015). Impact of functional foods on prevention of cardiovascular disease and diabetes. Curr. Cardiol. Rep. 17, 1–16. doi: 10.1007/s11886-015-0593-9
Silva, M. A., Albuquerque, T. G., Alves, R. C., Oliveira, M. B. P., and Costa, H. S. (2020). Melon (Cucumis melo L.) by-products: potential food ingredients for novel functional foods? Trends Food Sci. Technol. 98, 181–189. doi: 10.1016/j.tifs.2018.07.005
Słowikowski, B. K., Drzewiecka, H., Malesza, M., Mądry, I., Sterzyńska, K., and Jagodziński, P. P. (2020). The influence of conjugated linoleic acid on the expression of peroxisome proliferator-activated receptor-γ and selected apoptotic genes in non-small cell lung cancer. Mol. Cell. Biochem. 466, 65–82. doi: 10.1007/s11010-020-03689-8
Terán, V., Pizarro, P. L., Zacarías, M. F., Vinderola, G., Medina, R., and Van Nieuwenhove, C. (2015). Production of conjugated dienoic and trienoic fatty acids by lactic acid bacteria and bifidobacteria. J. Funct. Foods 19, 417–425. doi: 10.1016/j.jff.2015.09.046
Trigueros, L., and Sendra, E. (2015). Fatty acid and conjugated linoleic acid (CLA) content in fermented milks as assessed by direct methylation. LWT Food Sci. Technol. 60, 315–319. doi: 10.1016/j.lwt.2014.09.053
Wang, J., Li, H., Meng, X., Tong, P., and Liu, X. (2022). Biosynthesis of c9, t11-conjugated linoleic acid and the effect on characteristics in fermented soy milk. Food Chem. 368:130866. doi: 10.1016/j.foodchem.2021.130866
Ye, S. H., Yu, T., Yang, H., Li, L., Wang, H., Xiao, S., et al. (2013). Optimal culture conditions for producing conjugated linoleic acid in skim-milk by co-culture of different Lactobacillus strains. Ann. Microbiol. 63, 707–717. doi: 10.1007/s13213-012-0523-7
Keywords: by-product valorization, spore-forming probiotics, lactic acid bacterium, conjugated linoleic acid, grapeseed oil
Citation: Rezavand Hesari M, Amiri S, Asianezhad A, Khalili A, Ho TM, Saris PEJ and Yousefvand A (2024) Optimized bioconversion of grapeseed oil-based bioactive conjugated linoleic acid by Bacillus coagulans IBRC-M 10807. Front. Sustain. Food Syst. 8:1487005. doi: 10.3389/fsufs.2024.1487005
Edited by:
Pedro Ferreira Santos, University of Minho, PortugalReviewed by:
Maria Simona Chis, University of Agricultural Sciences and Veterinary Medicine of Cluj-Napoca, RomaniaHaixing Li, Nanchang University, China
Copyright © 2024 Rezavand Hesari, Amiri, Asianezhad, Khalili, Ho, Saris and Yousefvand. This is an open-access article distributed under the terms of the Creative Commons Attribution License (CC BY). The use, distribution or reproduction in other forums is permitted, provided the original author(s) and the copyright owner(s) are credited and that the original publication in this journal is cited, in accordance with accepted academic practice. No use, distribution or reproduction is permitted which does not comply with these terms.
*Correspondence: Amin Yousefvand, amin.yousefvand@helsinki.fi; Saber Amiri, sa.amiri@urmia.ac.ir