- 1Fisheries Research Institute Ministry of Agriculture, Keelung, Taiwan
- 2Department of Food Science, Fu Jen Catholic University, New Taipei City, Taiwan
- 3Ph.D. Program in Nutrition and Food Science, Fu Jen Catholic University, New Taipei City, Taiwan
Grouper aquaculture is a key industry in Taiwan, yet its processing generates significant byproducts, leading to challenges in waste management and environmental sustainability. Recent research has focused on developing innovative methods to valorize these byproducts, with grouper bone hydrolysate (GBH) emerging as a potential candidate for applications in health promotion and exercise performance enhancement. In this study, we investigated the amino acid composition of grouper bone hydrolysate (GBH) and analyzed its peptide contents. We also investigated GBH supplementation in relation to body composition and exercise performance in mice. Male Institute of Cancer Research (ICR) mice were divided into 3 groups (n = 7 per group) and orally administered GBH once daily for 6 weeks at doses of 0 g/kg/day (vehicle), 103 mg/kg/day (GBH-1X), 205 mg/kg/day (GBH-2X), and 513 mg/kg/day (GBH-5X). The GBH was rich in branched-chain amino acids and bioactive peptides, and supplementation enhanced the exercise performance of the mice. GBH supplementation increased their exhaustive swimming time, forelimb grip strength, and tissue glycogen content while reducing fatigue markers such as lactate, ammonia, and creatine kinase. The results indicate that GBH contains dipeptides such as Leu-Ala, Glu-Asp., Met-Leu, Met-Ile, Phe-Pro, Trp-Asp., Leu- Val, and Leu-Cys, as well as tetrapeptides such as Pro-Ser-Met-Ala, Ser-Val-Pro- Ile, and Ala-Val-Pro-Trp. GBH supplementation could aid in overcoming fatigue during endurance exercise and decrease metabolic waste after acute exercise.
1 Introduction
The United Nations’ Sustainable Development Goals include a commitment to reducing food waste significantly. Using currently underexploited ingredients for food production could mitigate food loss and the waste of potential food sources in the supply chain. These food products and ingredients are called upcycled foods and embody the concept of “waste to value.” They originate from side streams and co-products and are resources for value-added surplus food that could be integral to enhancing supply-chain sustainability (Bhatt et al., 2018; Coderoni and Perito, 2020; Peschel and Aschemann-Witzel, 2020).
Agriculture in Taiwan is highly developed, but copious amounts of by-products are generated from agro-industrial processes and pose severe environmental challenges and increase production costs (Hsu, 2021). Strategic minimization of waste in these processes could markedly enhance the economic viability of by-products derived from fish processing, conserve resources, and promote sustainable agriculture (Aschemann-Witzel et al., 2023). Such practices support an environmentally friendly production ethos, which is essential for maintaining ecological balance and ensuring the sustainability of agricultural practices (Spratt et al., 2021).
Grouper aquaculture represents a significant sector within Taiwan’s economy (Huang et al., 2023). However, the processing of this valuable seafood resource generates substantial byproducts, posing challenges for waste management and environmental sustainability. To address this issue, research efforts should prioritize the development of innovative technologies aimed at converting these byproducts into value-added products, such as polyester fibers, fish oil, and collagen (Hou et al., 2022; Lin et al., 2023). This valorization strategy has the potential to enhance resource efficiency, minimize environmental impact, and improve the economic viability of the grouper aquaculture industry.
Integrating bioactive compounds like lycopene, beta-carotene, and ferrous sulfate from food by-products could add value to waste or transform it into valuable products (Kharel et al., 2021; Madia et al., 2021). Based on the principles of upcycling food, this investigation was done to add value to the skeletal remains of Taiwanese grouper fish, which are typically discarded during food processing, through their transformation into a useful product. The bones were used as a substrate and processed to generate a promising nutraceutical supplement that is tailored for athletic nutrition.
The supplement is mainly characterized by a heightened concentration of branched-chain amino acids (BCAAs). BCAAs can affect exercise fatigue, which can be partially attributed to the build-up of lactic acid, ammonia, and blood urea nitrogen (BUN) in the serum and muscular tissue (Nikolaidis et al., 2018). Sustained high-intensity contractions during exercise can also rapidly deplete muscle glycogen and precipitate muscle fatigue (Gonzalez et al., 2016; Shulman et al., 2001). The supplementation of BCAAs could potentially enhance the serum levels of metabolites associated with fatigue, glucose, and muscle recovery markers [including lactate dehydrogenase (LDH) and creatine kinase (CK) (Kim et al., 2013)].
2 Methods
2.1 Grouper bone hydrolysate preparation
Grouper fish bones were sourced from a processing factory in the Yong’an District of Kaohsiung, Taiwan. The bones were first defrosted and then boiled for 10 min in water to facilitate the separation of residual flesh and connective tissues. After boiling, debridement was carried out to ensure thorough removal of organic matter, after which the bones were weighed. An ultrafine bubble cleansing was then done for 5 min (Chen et al., 2020) for deodorization and sterilization.
The decontaminated bones were then dried with hot air, weighed again, and ground into a fine powder. This powder was preserved at-20°C for later use. The bone powder was later macerated for 12 h in a 0.25% citric acid solution with a solid-to-liquid ratio of 1:4 and continuous agitation. This was done to demineralize the bone matrix and prepare it for extraction. The material was extracted for 20 h at 65°C with a 1:1 ratio of distilled water under constant stirring to ensure optimal diffusion. After extraction, the resultant mixture was filtered, and a gelatinous sample was obtained.
Next, 0.5% FoodPro®30 L enzyme (International Flavors & Fragrances Inc., NY., United States) was added to the sample, which was then extracted again for 6 h at 50°C in distilled water. The enzymatic treatment was halted by thermal deactivation, in which the extract was held at 100°C for 30 min to ensure complete enzyme inactivation. Finally, centrifugation was done for 15 min at 10,000 × g at 4°C, and the clarified GBH supernatant was obtained.
2.2 Analysis of GBH amino acids, hydrolysis rate, and peptide contents
The amino acid components of the GBH were separated using high-performance liquid chromatography (HPLC) in a C18 column (Hypersil GOLD C18, 100 × 2.1-mm inner diameter, particle size of 1.9 μm). The mobile phase consisted of (A) sodium dihydrogen phosphate and (B) acetonitrile and methanol (1:1) with a flow rate of 0.5 mL/min. Gradient analysis was conducted using the method reported by previous (Innocente et al., 2007).
2.3 Analysis of possible peptide content
A comprehensive comparison of literature and databases was done to predict the possible types of each mass-to-charge ratio (m/z) and identify potential peptide sequences. The results of the mass spectrometry can be confirmed through several resources. The amino acid mass table provided by Biosyn was used for initial comparisons. The NIST Online Chemistry Molecular Weight Search Engine was used for identifying molecular weights. The proteomics database provided by NCBI was used to match and confirm the approximate peptide sequences.
2.4 Animals
Male Institute of Cancer Research (ICR) mice (6 weeks old; average weight 27 g) were purchased from BioLASCO (Taipei, Taiwan). The mice were given Laboratory Rodent Diet 5,001 and distilled water ad libitum. They were housed at room temperature (24 ± 2°C) under humidity-controlled conditions (60 ± 5%) and a regular 12-h light/dark cycle. The animal protocol (A11202) was reviewed and approved by the Institutional Animal Care and Use Committee (IACUC) of Fu Jen Catholic University, New Taipei City, Taiwan.
The recommended daily intake of GBH is approximately 200–1,000 mg per day per mouse based on the previous method (Nirmal et al., 2022). Thus, the mouse dose per kilogram of body weight as follows: 500/60 × 12.3 = 103 mg/kg (GBH-1X). Accordingly, the GBH-2X dose was 205 mg/kg, and the GBH-5X dose was 513 mg/kg.
2.5 Fatigue-associated biochemical indices
After day 26 of GBH supplementation, blood samples were collected at 15 min after the mice performed swimming exercises. Blood was centrifuged for 10 min (1,500 × g) at 4°C, and sera were collected for analysis. Lactate, ammonia, CK, glucose, BUN, and LDH levels were determined using an autoanalyzer (Hitachi 7,600; Hitachi Co. Ltd., Japan).
2.6 Exhaustive exercise test and forelimb grip strength
On day 27 of the supplementation period, 30 min after GBH supplementation, the forelimb grip strength of the mice was tested according to the method described by Chen et al. (2016). A low-force testing system (PicoScope 2000, Pico Technology Limited, Cambridgeshire, United Kingdom) was used to measure the forelimb absolute grip strength. Animals were acclimated, then gently held by the tail to allow only their forelimbs to grasp the bar. Once a firm grip was established, animals were pulled back until they released the bar, and peak force was recorded. Each animal underwent 3 trials with 1 min rest intervals, the highest recorded force (in grams) was used as an indicator of the grip strength.
A treadmill exhaustion test was conducted based on a previous study using a mouse treadmill with an incline that started at 10o (Bio-Cando Incorporation, Taipei, Taiwan). The test was performed with a 10% incline, and the speed and duration were gradually increased as follows: 10 m/min for 5 min, 16 m/min for 10 min, 21 m/min for 10 min, 26 m/min for 10 min, 31 m/min for 10 min, 36 m/min for 10 min, and 41 m/min for 10 min until exhaustion. The running time for each mouse was recorded.
2.7 Body composition and tissue glycogen analyses
At the end of the study on day 28, the fat-free mass (FFM) and fat mass of the mice were detected using time-domain nuclear magnetic resonance (TD-NMR) with an LF50 body composition analyzer (Minispec; Bruker, Germany). Briefly, animals were restrained in a 50-mm-diameter plastic cylinder and scanned for 2 min. The TD-NMR device measures radiofrequency signals from hydrogen nuclei in tissues and uses differences in relaxation times to estimate body composition. Glycogen content was analyzed using a chemical method based on literature protocols, with commercial glycogen (Sigma, United States) as the calibration standard. Tissue samples were homogenized with five volumes (w/v) of buffer using a Bullet Blender (Next Advance, MA, United States). Homogenates were centrifuged at 12,000 × g for 15 min at 4°C, and the supernatant was collected for direct glycogen quantification (Chen et al., 2020).
2.8 Histological tissue staining and blood assessments
On day 28, all mice were sacrificed using 95% CO2 asphyxiation. Different tissues were collected and fixed in 10% formalin. Hematoxylin and eosin staining was carried out as previously described. Blood was withdrawn through cardiac puncture, and serum was collected through centrifugation. The levels of aspartate aminotransferase (AST), alanine aminotransferase (ALT), LDH, CK, creatinine, total cholesterol (TC), triacylglycerol (TG), high-density lipoprotein (HDL), low-density lipoprotein (LDL), total protein (TP), albumin, and glucose were assessed using an autoanalyzer (Hitachi 7,600; Hitachi Co. Ltd., Japan).
2.9 Statistical analysis
Data were expressed as the mean ± standard deviation (SD). Significant differences between multiple groups were calculated using one-way analysis of variance (ANOVA) and Duncan’s post-hoc test. Values of p < 0.05 were considered significant. The Cochran–Armitage trend test was used to examine the dose-dependence effect.
3 Results
3.1 Resource identification initiative total BCAAs content, hydrolysis rate, and peptide contents
Table 1 presents the total BCAAs content in GBH. Following enzymatic hydrolysis, the bone material exhibited a high concentration of amino acids, and the contents of the BCAAs valine, isoleucine, and leucine were measured as 644 ± 64, 260 ± 26, and 603 ± 57 μg/mL, respectively. The substantial presence of BCAAs in GBH suggests that it has potential to stimulate protein synthesis in skeletal muscle. Thus, it may exert anabolic effects on protein metabolism, potentially enhance the rate of protein synthesis, and decrease protein degradation in resting human skeletal muscle. Table 2 shows the hydrolysis efficiency of GBH. The results indicate a hydrolysis rate of 29.95 ± 1.08%, and the peptide concentration in the GBH was 117.03 ± 2.94 mg/mL.
3.2 Possible peptide components
Table 3 displays the initial peptide test results of GBH, which indicate the potential presence of dipeptides or tetrapeptides. MALDI-TOF mass spectrometry analysis identified five peaks at retention times of 0.9, 1.3, 8.7, 11, and 11.9 min. It is important to note that these findings are preliminary, and due to the nature of grouper fish bones as a residual food resource, the consistency of the sample source cannot be guaranteed. Therefore, the identification of peptides is based on inference. These peptides included dipeptides Leu-Ala, Glu-Asp., Met-Leu, Met-Ile, Phe-Pro, Trp-Asp., Leu-Val, and Leu-Cys and tetrapeptides Pro-Ser-Met-Ala, Ser-Val-Pro-Ile, and Ala-Val-Pro-Trp.
The proposed peptide sequences were determined through a comparison of mass spectrometry data with the amino acid mass table, molecular weight databases, and proteomics databases. Additional validation is needed to confirm these peptide sequences. Leu: leucine, Ala: alanine, Glu: glutamic acid, Asp: aspartic acid, Met: methionine, Phe: phenylalanine, Pro: proline, Trp: tryptophan, Ser: serine, Val: valine, Cys: cysteine, Am: amide, His: histidine, Ile: isoleucine.
Effect of supplementation on tissue weight, body weight, and food and water intake.
Figure 1 illustrates the growth curves of mice supplemented with various doses of GBH (1X, 2X, and 5X) compared to a vehicle control group over 4 weeks. All groups exhibited a steady increase in body weight throughout the experimental period. However, no statistically significant differences in body weight were observed between the GBH-supplemented groups and the vehicle control group at any time point.
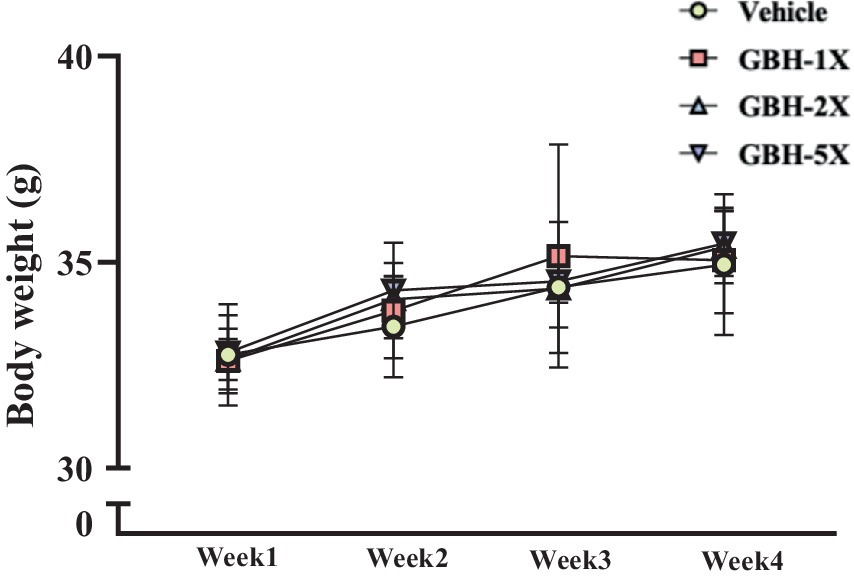
Figure 1. Effect of GBH supplementation on body weight in mice. Mice were supplemented with GBH at different doses or vehicle for 4 weeks. Body weight was measured weekly. Data are presented as mean ± SD (n = 7 per group).
Table 4 displays the data on body weight, tissue weights, and food and water consumption. There were no significant differences in any weights or consumption across groups, indicating that GBH supplementation at the tested doses did not significantly affect the overall body weight gain and diet of mice. Table 4 also shows the measured epididymal fat pad (EFP) weights. The weights of the vehicle, GBH-1X, GBH-2X, and GBH-5X groups were 0.50 ± 0.05 g, 0.39 ± 0.09 g, 0.36 ± 0.06 g, and 0.40 ± 0.13 g, respectively. Trend analysis revealed a significant dose-dependent decrease in EFP weight (p = 0.0297). The EFP weights were significantly lower in the GBH-1X (21.88%, p = 0.0287), GBH-2X (29.26%, p = 0.0047), and GBH-5X groups (19.89%, p = 0.0449) compared with the vehicle group.
The weights of muscle tissue from the vehicle, GBH-1X, GBH-2X, and GBH-5X groups were 0.36 ± 0.05, 0.40 ± 0.07, 0.46 ± 0.04, and 0.42 ± 0.03 g, respectively. The muscle weight of the GBH-2X and GBH-5X groups were 1.27 times (p = 0.0012) and 1.16 times (p = 0.0369) higher than that of the vehicle group. The EFP and muscle relative weights of GBH-treated groups were significantly lower than that of the vehicle group (p = 0.0197 and p = 0.0176, respectively).
There were no significant differences in the liver, kidney, and lung weights among groups. Additionally, the relative weights of the liver, kidneys, and lungs were not significantly different. Heart weight was significantly lower in the GBH-2X (p = 0.0107) and GBH-5X (p = 0.0143) groups compared to the vehicle group, but the relative weights of muscle were not significantly different [𝐹 (2, 27) = 2.63, 𝑝 = 0.0733].
Figure 2 presents the effects of GBH supplementation on body composition. There was a significant increase in FFM in the GBH-2X group (1.06 times, p = 0.0217) compared to the vehicle group (Figure 2A). Conversely, fat mass was significantly reduced in all GBH-supplemented groups compared to the vehicle group [GBH-1X: 19.70% (p < 0.0001), GBH-2X: 20.14% (p < 0.0001), GBH-5X: 27.77% (p < 0.0001; Figure 2B)].
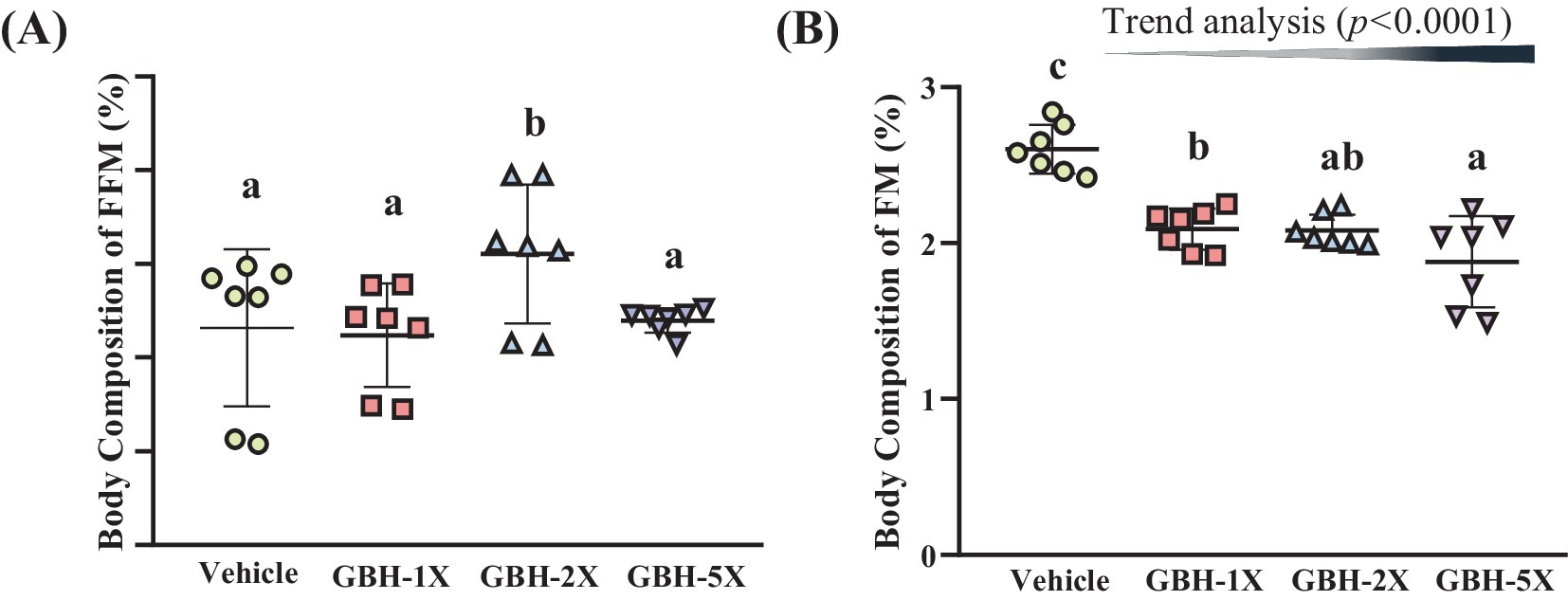
Figure 2. Effect of grouper bone hydrolysate (GBH) supplementation on body composition. (A) Free-fat mass (FFM) percentage and (B) fat mass (FM) percentage in mice following 4 weeks of GBH supplementation at different doses (1X, 2X, 5X) or vehicle control. Data are presented as mean ± SD, bars with different letters (a, b, c) indicate significant differences at p < 0.05 according to one-way ANOVA. Trend analysis (p < 0.05) indicates a significant dose-dependent effect of GBH on FM reduction.
3.3 Effect on forelimb grip strength and exhaustive treadmill test
The vehicle, GBH-1X, GBH-2X, and GBH-5X groups’ forelimb grip strengths were 126.0 ± 16.6, 142.0 ± 11.4, 149.6 ± 6.5, and 154.1 ± 16.2 g, respectively (Figure 3A). The GBH-2X and GBH-5X groups exhibited 1.19 times (p = 0.0146) and 1.22 times (p = 0.0133) higher grip strength than the vehicle group, respectively. Additionally, a significant dose-dependent effect on relative forelimb grip strength was observed (p < 0.0001).
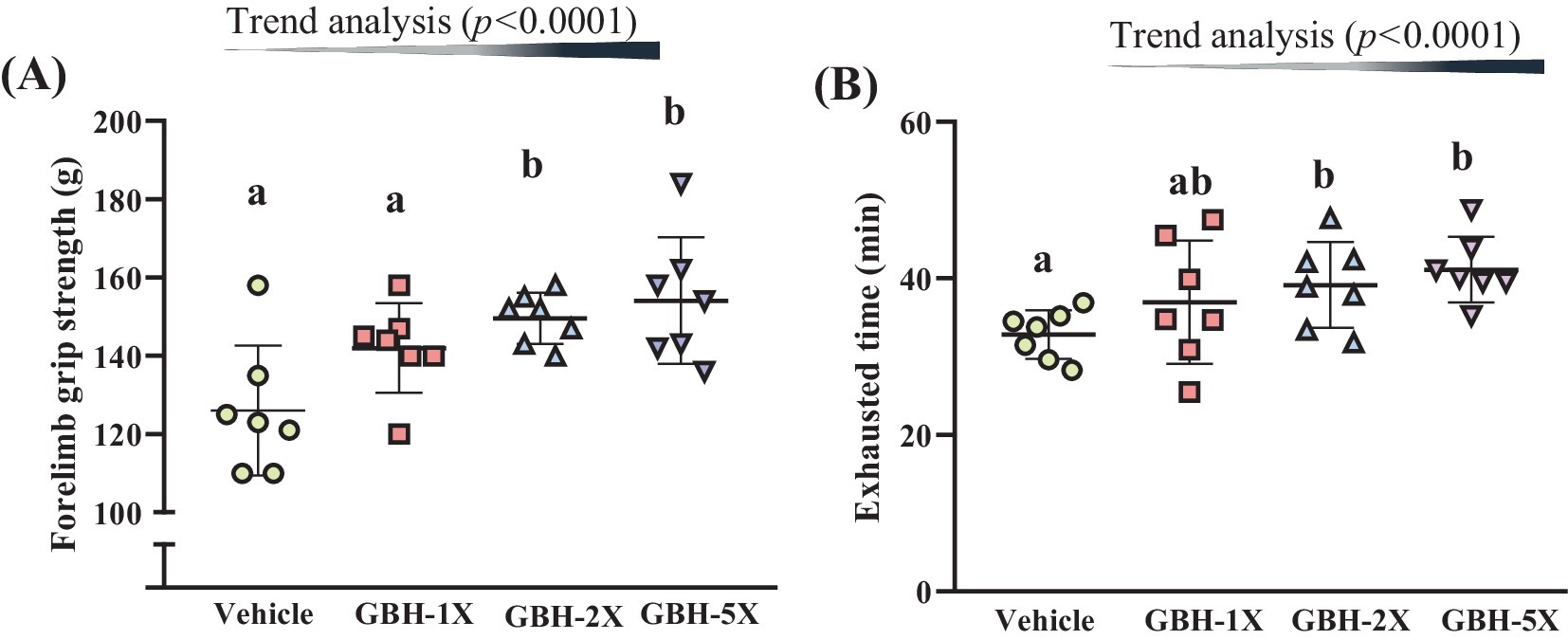
Figure 3. Effect of grouper bone hydrolysate (GBH) supplementation on (A) forelimb grip strength and (B) exhaustive treadmill test. Data are presented as mean ± SD (n = 7). Bars with different letters (a, b) indicate significant differences at p < 0.05 determined using one-way ANOVA. Trend analysis (p < 0.05) indicates a significant dose-dependent effect of GBH.
As illustrated in Figure 3B, the mean exhaustive treadmill test time of the GBH-2X group was 39.2 ± 5.5 min, which was 1.19 times longer than that of the vehicle group (p = 0.0402). The time of the GBH-5X group was significantly longer (1.25 times) at 41.1 ± 4.2 min compared to the vehicle group (p = 0.0091). Trend analysis revealed a statistically significant dose-dependent increase in the treadmill test time (p < 0.0001).
3.4 Effect on fatigue profile in swimming test
A 15-min swimming test was used to evaluate fatigue-related indicators (Figure 4A). Compared to the vehicle group (11.06 ± 1.58 mmol/L), serum lactate levels were significantly lower in the GBH-2X (7.79 ± 1.58 mmol/L) and GBH-5X (7.63 ± 1.39 mmol/L) groups, with reductions of 29.56% (p = 0.0007) and 30.96% (p = 0.0005), respectively. Furthermore, a significant dose-dependent decrease (p < 0.0001) in blood lactate levels was observed following the 15-min swimming test.
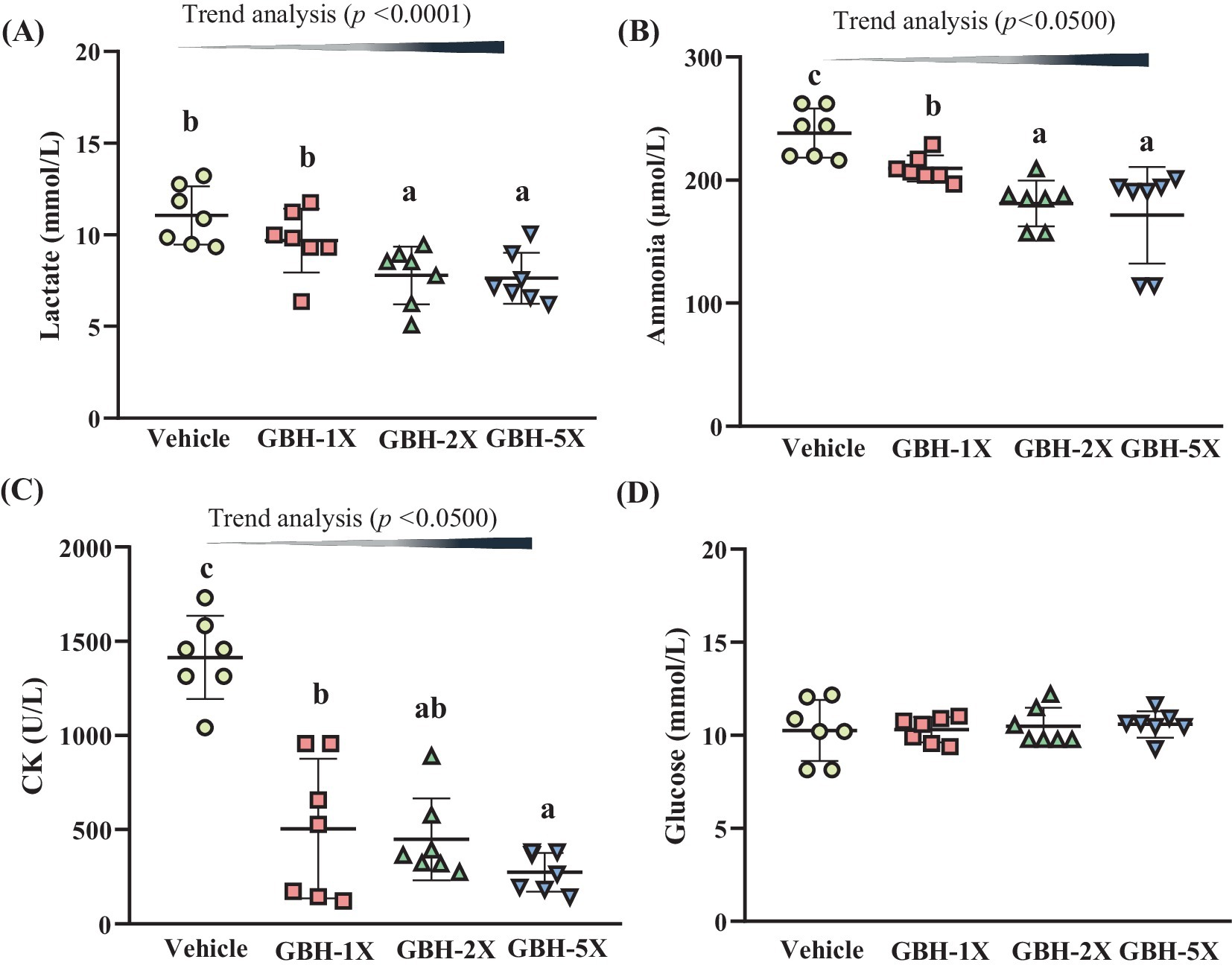
Figure 4. Effect of grouper bone hydrolysate (GBH) supplementation on the serum levels of (A) lactate, (B) ammonia, (C) creatine kinase (CK), and (D) glucose after a 15-min swimming test. Data are presented as mean ± SD (n = 7). Bars with different letters (a, b, c) indicate significant differences at p < 0.05 according to one-way ANOVA. Trend analysis (p < 0.05) indicates a significant dose-dependent effect of GBH.
The serum ammonia levels in the GBH-1X (210 ± 11 μmol/L), GBH-2X (181 ± 18 μmol/L), and GBH-5X (171 ± 39 μmol/L) groups were 12.05% (p = 0.0379), 23.99% (p = 0.0002), and 28.03% (p < 0.0001) lower than that in the vehicle group (238 ± 20 μmol/L), respectively. A significant dose-dependent effect on the serum ammonia levels was observed (p < 0.0500; Figure 4B). In the GBH-1X (506 ± 370 U/L), GBH-2X (449 ± 217 U/L), and GBH-5X (274 ± 103) groups, the serum CK levels were significantly lower (64.23%, p < 0.0001; 68.25%, p < 0.0001; and 80.66%, p < 0.0001) compared to the vehicle group (1,414 ± 220 U/L). A significant dose-dependent effect on the CK level was also observed (p < 0.0500; Figure 4C). In contrast, the glucose levels in the GBH group did not show a significant difference from the vehicle group (p = 0.9332; Figure 4D).
3.5 Effect on liver and muscular glycogen
As shown in Figure 5A, the liver glycogen content of the GBH-1X, GBH-2X, and GBH-5X groups exhibited a statistically significant elevation relative to the vehicle group. The fold-changes were 1.37 (p = 0.0482) for GBH-1X, 1.42 (p = 0.0582) for GBH-2X, and 1.80 (p = 0.0019) for GBH-5X, corresponding to respective values of 17.5 ± 0.9 mg/g liver, 18.2 ± 5.3 mg/g liver, and 23.0 ± 3.5 mg/g liver, respectively. Similarly, muscle glycogen levels were elevated in the GBH-1X, GBH-2X, and GBH-5X groups compared to the vehicle group, as shown in Figure 5B. The fold-changes were 1.74 (p = 0.0193) for GBH-1X, 1.71 (p = 0.0082) for GBH-2X, and 2.25 (p < 0.0001) for GBH-5X, with respective values of 1.5 ± 0.4 mg/g liver, 1.5 ± 0.7 mg/g liver, and 1.9 ± 0.8 mg/g liver, respectively. Notably, a clear and significant dose-dependent effect on both liver and muscle glycogen content was observed (p < 0.0001).
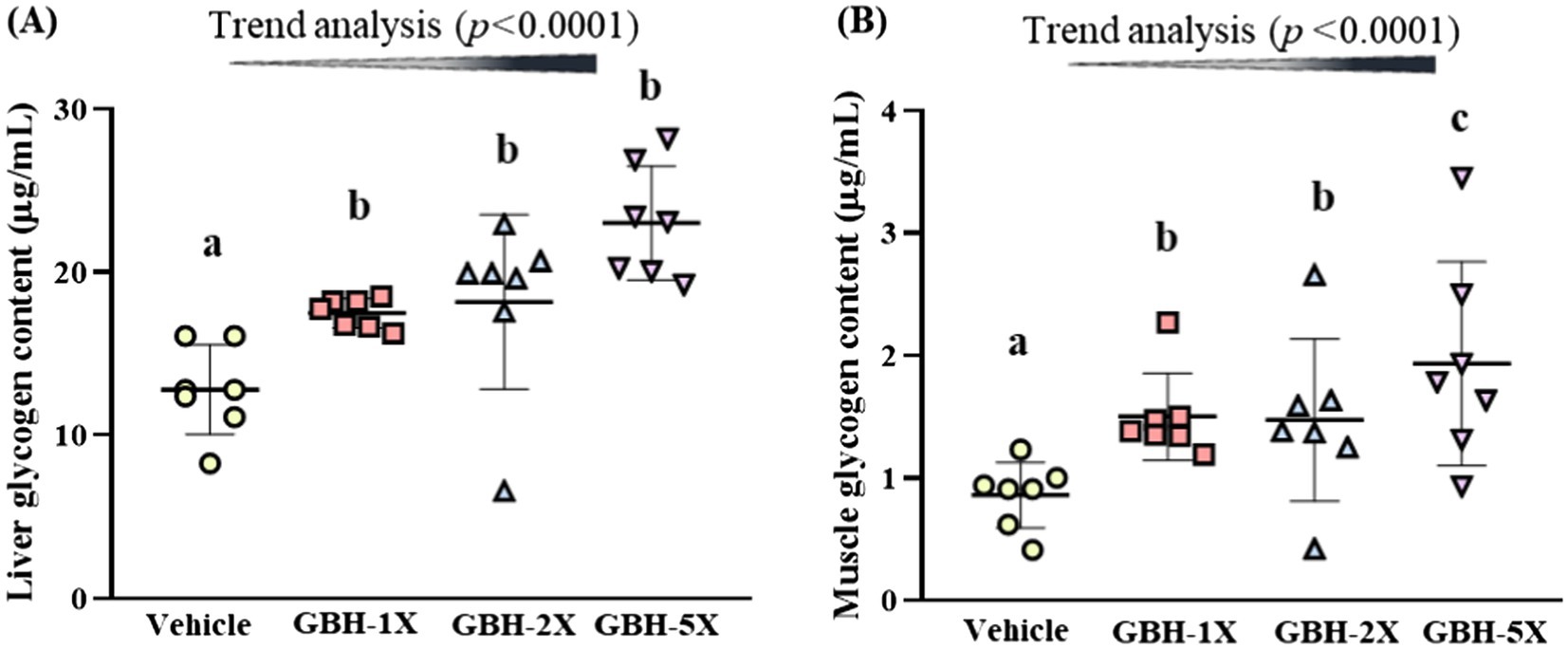
Figure 5. Effect of GBH supplementation on (A) liver glycogen and (B) muscle glycogen. Data are presented as mean ± SD (n = 7). Bars with different letters (a, b, c) indicate significant differences at p < 0.05 according to one-way ANOVA. Trend analysis (p < 0.05) indicates a significant dose-dependent effect of GBH.
3.6 Effect on tissue histopathology and biochemical variables
Representative histological sections from each excised tissue are presented in Figure 6, which shows the morphological and cellular characteristics that appeared following GBH supplementation. No significant differences were observed between GBH-supplemented groups and the vehicle control group in any of the examined tissues, indicating that 4 weeks of GBH supplementation did not induce any adverse effects on the evaluated organs.
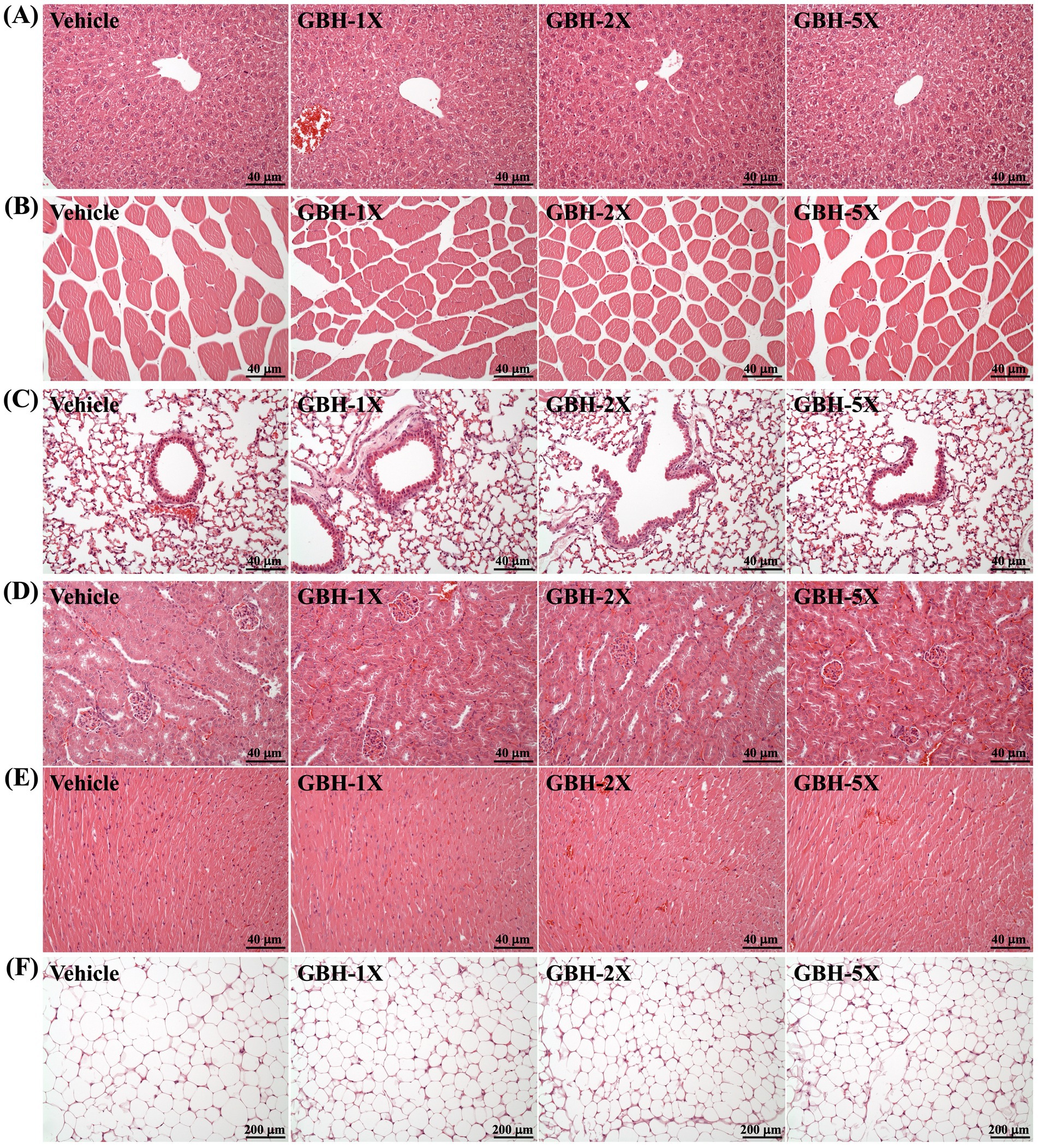
Figure 6. Representative histological sections showing the effect of GBH supplementation on (A) liver, (B) lungs, (C) muscle, (D) heart, (E) kidneys, and (F) epididymal fat pad (EFP). Specimens were observed using light microscopy. Hematoxylin and eosin stain, magnification: 200 × .
Table 5 shows that GBH supplementation did not induce significant changes in liver function markers (AST, ALT, and LDH) compared to the vehicle group (p > 0.05). However, a decreasing trend was observed in CK levels with increasing GBH dosage, suggesting a potential protective effect of GBH against muscle damage (p = 0.2170). GBH supplementation significantly reduced creatinine levels in a dose-dependent manner (p < 0.0001), indicating improved kidney function. No significant changes were observed in other parameters, including TC, TG, HDL, TP, albumin, and glucose levels (p > 0.05). These results suggest that GBH supplementation may have beneficial effects on kidney function and potentially muscle health and lipid metabolism, warranting further investigation.
4 Discussion
The food system continually faces a challenge of feeding the ever-growing global population, which is projected to reach 9.7 billion by 2050 (United Nations Department of Economic and Social Affairs, 2022). To help to address this challenge, there have been attempts to align consumption with the rate at which nature generates resources and neutralizes waste. However, after years of using natural resources, we are now encountering the limits of available resources and hazards posed by undesired emissions and accumulated waste (Giampietro, 2019). In Europe, approximately 40% of food waste occurs during production. Therefore, reducing food waste in the food industry is crucial (Aschemann-Witzel et al., 2023).
One strategy to manage waste in the food supply chain is to repurpose edible portions of food waste to produce food for human consumption. In this way, the food waste becomes a valuable resource for food production rather than being discarded. An example of this practice involves the reprocessing of stale bread or bread that is nearing or past its expiration date into beverages such as beer. Similarly, sunflower-seed pressings and spent grain from breweries could be processed into flour instead of being used as animal feed (Aschemann-Witzel et al., 2023; Grasso and Asioli, 2020).
Studies have investigated the potential benefits of combining protein hydrolysates with carbohydrates during endurance exercise (Vegge et al., 2012). Protein hydrolysates are derived from purified protein sources and composed of peptides of varying lengths and free amino acids. They exhibit distinct properties based on their amino acid composition. Substituting an equivalent caloric amount of soy protein with fish protein hydrolysates resulted in increased fatty acid oxidation and decreased adipose tissue mass in a rat model (Liaset et al., 2009).
GBH exhibits diverse properties based on its amino acid composition. For instance, GBH is rich in small-chain amino acids such as the dipeptides Leu-Ala, Glu-Asp., Met-Leu, Met-Ile, Phe-Pro, Trp-Asp., Leu-Val, and Leu-Cys, as well as tetrapeptide, which has demonstrated effects of enhanced digestion, absorption kinetics, and a heightened insulinemic response when consumed independently (Siegler et al., 2013). Peptides are short chains of amino acids with customizable physiological benefits. Furthermore, they have demonstrated bioactive properties, including antioxidant, antimicrobial, and anti-inflammatory activities, so they could contribute to human health and wellbeing (Martini and Tagliazucchi, 2023).
Substituting soy protein with fish protein hydrolysates such as GBH in mice led to increased fatty acid oxidation and reduced adipose tissue mass. Our data showed that GBH supplementation had positively influences on body composition, including increased lean body mass (GBH-2X) and decreased fat mass (all GBH treatments). Additional benefits include enhanced muscle strength and improved glucose uptake in liver and muscle tissue. These findings are consistent with previous research on marine-derived peptide supplements, which have demonstrated similar effects on body composition, muscle strength, glucose uptake, and exercise recovery (Jendricke et al., 2019; Kirmse et al., 2019; Roblet et al., 2014). Some studies have also reported accelerated recovery from exercise-induced muscle soreness (Clifford et al., 2019).
Another contributing factor to GBH’s efficacy is its high BCAAs content. BCAAs supplementation has been shown to enhance athletic performance, and studies have demonstrated improved endurance in runners (Gawedzka et al., 2020) and enhance exercise performance in untrained cyclists (Manaf et al., 2021). Beyond enhancing physical performance, BCAAs supplementation has been linked to increased muscle strength and mass (Ko et al., 2020), as well as mitigation of exercise-induced muscle damage (Fouré and Bendahan, 2017).
Leucine and isoleucine are key BCAAs that have been found in high concentrations in various fish species (Cruzat et al., 2018). Furthermore, leucine, isoleucine, and valine have been detected in a variety of marine products, such as tuna, mackerel, emperor fish, silky shark, lobster, and crab (Mohanty et al., 2014). These findings highlight the potential of marine-derived products as a valuable source for developing sports-nutrition supplements (Fouré and Bendahan, 2017). Recent studies have found that GBH decreases lactate, ammonia, and CK levels after acute exercise. Post-exercise muscle damage indicators such as CK are indicative of muscle damage, including muscular dystrophy and rhabdomyolysis (Chen et al., 2016). Reviews examining the effects of BCAAs on muscle damage markers and soreness have indicated a positive impact (Doma et al., 2021; Khemtong et al., 2021).
GBH’s attenuation of CK levels suggests a protective effect against exercise-induced muscle damage, which could potentially lead to enhanced performance through reduced accumulation of detrimental metabolites. The abundance of BCAAs and glutamine in GBH may explain its efficacy in reducing lactate, CK, and ammonia levels, thereby mitigating muscle soreness and reduce fatigue. A possible key contributor to this effect could be glutamine, which is a plentiful free amino acid in plasma and skeletal muscle that plays a crucial role in protein synthesis (Cruzat et al., 2018).
During exercise, energy is primarily derived from the oxidation of muscle and liver glycogen, which is supplemented by liver gluconeogenesis and exogenous carbohydrate intake. Notably, glycogen stores and glucose uptake in skeletal muscle are the predominant substrates used during prolonged exercise (Kimura et al., 2011). Glycogen content is a crucial determinant of fatigue, and GBH supplementation has been found to enhance glycogen accumulation, thereby improving aerobic exercise capacity. This increase in muscle glycogen may directly enhance exercise performance and mitigate physical fatigue.
Grouper Bone Hydrolysate (GBH) demonstrates potential in enhancing exercise performance through a range of biochemical mechanisms attributed to its high concentration of bioactive peptides, amino acids, and branched-chain amino acids (BCAAs). Evidence indicates that GBH increases fatty acid oxidation and reduces adipose tissue, optimizing energy utilization during prolonged exercise. Specific peptides within GBH, have been shown to improve digestion and enhance glucose uptake in muscle and liver tissues, facilitating rapid recovery and consistent energy availability. The BCAAs content in GBH supports protein synthesis, increases endurance, and mitigates muscle damage markers such as creatine kinase (CK), thereby reducing post-exercise metabolic waste. Additionally, elevated levels of BCAAs in GBH are linked to decreased lactate and ammonia accumulation, which contributes to delayed onset of fatigue and improved recovery. Furthermore, GBH supplementation has been associated with enhanced glycogen storage in muscle tissues, providing a sustained energy source that delays fatigue during endurance activities. Collectively, these mechanisms underscore the utility of GBH as a functional supplement in sports nutrition, offering benefits that support endurance exercise performance.
5 Conclusion
This study has investigated GBH as a food residue derived from hydrolysis of grouper bone, which is rich in BCAAs and potentially bioactive peptides. Enzymatic hydrolysis yielded GBH with a hydrolysis rate of 29.95% and peptide concentration of 117.03 mg/mL. Preliminary analysis suggested the presence of di-and tetrapeptides.
Four weeks of GBH supplementation in mice led to a decrease in fat mass and improved fatigue resistance, as evidenced by reduced fatigue-related serum markers. Additionally, GBH supplementation enhanced forelimb grip strength, treadmill exhaustion time, and glycogen content. These findings highlight the potential of GBH as an upcycled food product and nutritional supplement to enhance endurance exercise performance by mitigating fatigue indicators. Nevertheless, further research is needed to elucidate the mechanisms through which GBH affects energy homeostasis and its potential as a nutritional supplement.
Data availability statement
The original contributions presented in the study are included in the article/supplementary material, further inquiries can be directed to the corresponding author/s.
Ethics statement
The animal study was approved by Institutional Animal Care and Use Committee (IACUC) of Fu Jen Catholic University, New Taipei City, Taiwan. The study was conducted in accordance with the local legislation and institutional requirements.
Author contributions
Y-FK: Writing – original draft, Visualization, Validation, Supervision, Software, Resources, Project administration, Methodology, Investigation, Funding acquisition, Formal analysis, Data curation, Conceptualization. H-JC: Writing – original draft, Validation, Supervision, Resources, Project administration, Investigation, Funding acquisition, Formal analysis, Data curation. C-JT: Writing – original draft. T-YT: Writing – review & editing, Writing – original draft, Visualization, Validation, Supervision, Resources, Funding acquisition, Conceptualization. T-HL: Writing – review & editing, Writing – original draft. T-KY: Writing – original draft, Visualization, Validation, Project administration, Methodology, Investigation, Formal analysis, Data curation. Y-MC: Writing – review & editing, Writing – original draft, Visualization, Validation, Supervision, Software, Resources, Project administration, Methodology, Investigation, Funding acquisition, Formal analysis, Data curation, Conceptualization.
Funding
The author(s) declare that financial support was received for the research, authorship, and/or publication of this article. This work is partially financially supported by Fisheries Research Institute, Ministry of Agriculture, Taiwan (grant no. MOA 112AS-6.3.2-AI-A5), and the National Science and Technology Council of Taiwan (grant no. NSTC 112-2320-B-030-013).
Acknowledgments
The authors are grateful to Chien-Chao Chiu for his technical assistance in the histopathological examination.
Conflict of interest
The authors declare that the research was conducted in the absence of any commercial or financial relationships that could be construed as a potential conflict of interest.
Publisher’s note
All claims expressed in this article are solely those of the authors and do not necessarily represent those of their affiliated organizations, or those of the publisher, the editors and the reviewers. Any product that may be evaluated in this article, or claim that may be made by its manufacturer, is not guaranteed or endorsed by the publisher.
Abbreviations
GBH, grouper bone hydrolysate; EFP, epididymal fat pad; Asp, aspartic acid; Glu, glutamic acid; Ser, serine; His, histidine; Gly, glycine; Thr, threonine; Arg, arginine; Ala, alanine; Tyr, tyrosine; Cys, cysteine; Val, valine; Met, methionine; Phe, phenylalanine; Ile, isoleucine; Leu, leucine; Lys, lysine; Pro, proline; AST, aspartate aminotransferase; ALT, alanine aminotransferase; LDH, lactate dehydrogenase; CK, creatine kinase; TC, total cholesterol; TG, triacylglycerol; HDL, high-density lipoprotein; LDL, low-density lipoprotein; TP, total protein.
References
Aschemann-Witzel, J., Asioli, D., Banovic, M., Perito, M. A., Peschel, A. O., and Stancu, V. (2023). Defining upcycled food: the dual role of upcycling in reducing food loss and waste. Trends Food Sci. Technol. 132, 132–137. doi: 10.1016/j.tifs.2023.01.001
Bhatt, S., Lee, J., Deutsch, J., Ayaz, H., Fulton, B., and Suri, R. (2018). From food waste to value-added surplus products (VASP): consumer acceptance of a novel food product category. J. Consum. Behav. 17, 57–63. doi: 10.1002/cb.1689
Chen, Y. M., Chiu, W. C., Chiu, Y. S., Li, T., Sung, H. C., and Hsiao, C. Y. (2020). Supplementation of nano-bubble curcumin extract improves gut microbiota composition and exercise performance in mice. Food Funct. 11, 3574–3584. doi: 10.1039/C9FO02487E
Chen, Y. M., Lin, C. L., Wei, L., Hsu, Y. J., Chen, K. N., Huang, C. C., et al. (2016). Sake protein supplementation affects exercise performance and biochemical profiles in power-exercise-trained mice. Nutrients 8:106. doi: 10.3390/nu8020106
Clifford, T., Ventress, M., Allerton, D. M., Stansfield, S., Tang, J. C., Fraser, W. D., et al. (2019). The effects of collagen peptides on muscle damage, inflammation and bone turnover following exercise: a randomized, controlled trial. Amino Acids 51, 691–704. doi: 10.1007/s00726-019-02706-5
Coderoni, S., and Perito, M. A. (2020). Sustainable consumption in the circular economy. An analysis of consumers’ purchase intentions for waste-to-value food. J. Clean. Prod. 252:119870. doi: 10.1016/j.jclepro.2019.119870
Cruzat, V., Macedo Rogero, M., Noel Keane, K., Curi, R., and Newsholme, P. (2018). Glutamine: metabolism and immune function, supplementation and clinical translation. Nutrients 10:1564. doi: 10.3390/nu10111564
Doma, K., Singh, U., Boullosa, D., and Connor, J. D. (2021). The effect of branched-chain amino acid on muscle damage markers and performance following strenuous exercise: a systematic review and meta-analysis. Appl. Physiol. Nutr. Metab. 46, 1303–1313. doi: 10.1139/apnm-2021-0110
Fouré, A., and Bendahan, D. (2017). Is branched-chain amino acids supplementation an efficient nutritional strategy to alleviate skeletal muscle damage? A systematic review. Nutrients 9:1047. doi: 10.3390/nu9101047
Gawedzka, A., Grandys, M., Duda, K., Zapart-Bukowska, J., Zoladz, J. A., and Majerczak, J. (2020). Plasma BCAA concentrations during exercise of varied intensities in young healthy men—the impact of endurance training. PeerJ 8:e10491. doi: 10.7717/peerj.10491
Giampietro, M. (2019). On the circular bioeconomy and decoupling: implications for sustainable growth. Ecol. Econ. 162, 143–156. doi: 10.1016/j.ecolecon.2019.05.001
Gonzalez, J. T., Fuchs, C. J., Betts, J. A., and van Loon, L. J. (2016). Liver glycogen metabolism during and after prolonged endurance-type exercise. Am. J. Physiol. Endocrinol. Metab. 311, E543–E553. doi: 10.1152/ajpendo.00232.2016
Grasso, S., and Asioli, D. (2020). Consumer preferences for upcycled ingredients: a case study with biscuits. Food Qual. Prefer. 84:103951. doi: 10.1016/j.foodqual.2020.103951
Hou, E. J., Huang, C. S., Lee, Y. C., and Chu, H. T. (2022). Upcycled aquaculture waste as textile ingredient for promoting circular economy. Sustain. Mater. Technol. 31:e00336. doi: 10.1016/j.susmat.2021.e00336
Hsu, E. (2021). Cost-benefit analysis for recycling of agricultural wastes in Taiwan. Waste Manag. 120, 424–432. doi: 10.1016/j.wasman.2020.09.051
Huang, P. L., Afero, F., Chang, Y., Chen, B. Y., Lan, H. Y., Hou, Y. L., et al. (2023). The bioeconomic analysis of hybrid Giant grouper (Epinephelus fuscoguttatus× Epinephelus lanceolatus) and green grouper (Epinephelus malabaricus): a case study in Taiwan. Aust. Fish. 8:610. doi: 10.3390/fishes8120610
Innocente, N., Biasutti, M., Padovese, M., and Moret, S. (2007). Determination of biogenic amines in cheese using HPLC technique and direct derivatization of acid extract. Food Chem. 101, 1285–1289. doi: 10.1016/j.foodchem.2005.12.026
Jendricke, P., Centner, C., Zdzieblik, D., Gollhofer, A., and König, D. (2019). Specific collagen peptides in combination with resistance training improve body composition and regional muscle strength in premenopausal women: a randomized controlled trial. Nutrients 11:892. doi: 10.3390/nu11040892
Kharel, S., Gautam, A., Mahotra, M., Theniko, N. M., and Loo, S. C. J. (2021). Valorizing okara waste into nutritionally rich polysaccharide/protein-extracts for co-encapsulation of β-carotene and ferrous sulphate as a potential approach to tackle micronutrient malnutrition. J. Funct. Foods 87:104749. doi: 10.1016/j.jff.2021.104749
Khemtong, C., Kuo, C. H., Chen, C. Y., Jaime, S. J., and Condello, G. (2021). Does branched-chain amino acids (BCAAs) supplementation attenuate muscle damage markers and soreness after resistance exercise in trained males? A meta-analysis of randomized controlled trials. Nutrients 13:1880. doi: 10.3390/nu13061880
Kim, D. H., Kim, S. H., Jeong, W. S., and Lee, H. Y. (2013). Effect of BCAA intake during endurance exercises on fatigue substances, muscle damage substances, and energy metabolism substances. J. Exerc. Nutr. Biochem. 17, 169–180. doi: 10.5717/jenb.2013.17.4.169
Kimura, I., Inoue, D., Maeda, T., Hara, T., Ichimura, A., Miyauchi, S., et al. (2011). Short-chain fatty acids and ketones directly regulate sympathetic nervous system via G protein-coupled receptor 41 (GPR41). Proc. Natl. Acad. Sci. 108, 8030–8035. doi: 10.1073/pnas.1016088108
Kirmse, M., Oertzen-Hagemann, V., de Marées, M., Bloch, W., and Platen, P. (2019). Prolonged collagen peptide supplementation and resistance exercise training affects body composition in recreationally active men. Nutrients 11:1154. doi: 10.3390/nu11051154
Ko, C. H., Wu, S. J., Wang, S. T., Chang, Y. F., Chang, C. S., Kuan, T. S., et al. (2020). Effects of enriched branched-chain amino acid supplementation on sarcopenia. Aging 12, 15091–15103. doi: 10.18632/aging.103576
Liaset, B., Madsen, L., Hao, Q., Criales, G., Mellgren, G., Marschall, H. U., et al. (2009). Fish protein hydrolysate elevates plasma bile acids and reduces visceral adipose tissue mass in rats. Biochim. Biophys. Acta Mol. Cell Biol. Lipids 1791, 254–262. doi: 10.1016/j.bbalip.2009.01.016
Lin, C. H., Huang, Y. T., Ciou, J. Y., Cheng, C. M., Wang, G. T., You, C. M., et al. (2023). Circular economy and sustainable recovery of Taiwanese Tilapia (Oreochromis mossambicus) byproduct—the large-scale production of umami-rich seasoning material application. Food Secur. 12:1921. doi: 10.3390/foods12091921
Madia, V. N., De Vita, D., Ialongo, D., Tudino, V., De Leo, A., Scipione, L., et al. (2021). Recent advances in recovery of lycopene from tomato waste: a potent antioxidant with endless benefits. Molecules 26:4495. doi: 10.3390/molecules26154495
Manaf, F. A., Peiffer, J. J., Maker, G. L., and Fairchild, T. J. (2021). Branched-chain amino acid supplementation improves cycling performance in untrained cyclists. J. Sci. Med. Sport 24, 412–417. doi: 10.1016/j.jsams.2020.10.014
Martini, S., and Tagliazucchi, D. (2023). Bioactive peptides in human health and disease. J. Proteome 24:5837. doi: 10.3390/ijms24065837
Mohanty, B., Mahanty, A., Ganguly, S., Sankar, T., Chakraborty, K., Rangasamy, A., et al. (2014). Amino acid compositions of 27 food fishes and their importance in clinical nutrition. J. Amino Acids 2014:269797, 1–7. doi: 10.1155/2014/269797
Nikolaidis, S., Kosmidis, I., Sougioultzis, M., Kabasakalis, A., and Mougios, V. (2018). Diurnal variation and reliability of the urine lactate concentration after maximal exercise. Chronobiol. Int. 35, 24–34. doi: 10.1080/07420528.2017.1380037
Nirmal, N. P., Santivarangkna, C., Benjakul, S., and Maqsood, S. (2022). Fish protein hydrolysates as a health-promoting ingredient—recent update. Nutr. Rev. 80, 1013–1026. doi: 10.1093/nutrit/nuab065
Peschel, A. O., and Aschemann-Witzel, J. (2020). Sell more for less or less for more? The role of transparency in consumer response to upcycled food products. J. Clean. Prod. 273:122884. doi: 10.1016/j.jclepro.2020.122884
Roblet, C., Doyen, A., Amiot, J., Pilon, G., Marette, A., and Bazinet, L. (2014). Enhancement of glucose uptake in muscular cell by soybean charged peptides isolated by electrodialysis with ultrafiltration membranes (EDUF): activation of the AMPK pathway. Food Chem. 147, 124–130. doi: 10.1016/j.foodchem.2013.09.108
Shulman, R. G., Hyder, F., and Rothman, D. L. (2001). Cerebral energetics and the glycogen shunt: neurochemical basis of functional imaging. Proc. Natl. Acad. Sci. 98, 6417–6422. doi: 10.1073/pnas.101129298
Siegler, J. C., Page, R., Turner, M., Mitchell, N., and Midgely, A. W. (2013). The effect of carbohydrate and marine peptide hydrolysate co-ingestion on endurance exercise metabolism and performance. J. Int. Soc. Sports Nutr. 10:29. doi: 10.1186/1550-2783-10-29
Spratt, O., Suri, R., and Deutsch, J. (2021). Defining upcycled food products. J. Culinary Sci. Technol. 19, 485–496. doi: 10.1080/15428052.2020.1790074
United Nations Department of Economic and Social Affairs. (2022). World population prospects 2022: ten key messages. Population Division.
Keywords: sustainable food, upcycling foods, grouper bone hydrolysate, branched chain amino acids, endurance exercise
Citation: Kao Y-F, Chai H-J, Tsai C-J, Tsai T-Y, Liu T-H, Yi T-K and Chen Y-M (2024) Development of functional foods from grouper fish-bone residues to enhance muscle strength and exercise endurance in mice. Front. Sustain. Food Syst. 8:1483028. doi: 10.3389/fsufs.2024.1483028
Edited by:
Shalini Gaur Rudra, Indian Agricultural Research Institute, IndiaCopyright © 2024 Kao, Chai, Tsai, Tsai, Liu, Yi and Chen. This is an open-access article distributed under the terms of the Creative Commons Attribution License (CC BY). The use, distribution or reproduction in other forums is permitted, provided the original author(s) and the copyright owner(s) are credited and that the original publication in this journal is cited, in accordance with accepted academic practice. No use, distribution or reproduction is permitted which does not comply with these terms.
*Correspondence: Yi-Ming Chen, MTU1NDI5QG1haWwuZmp1LmVkdS50dw==