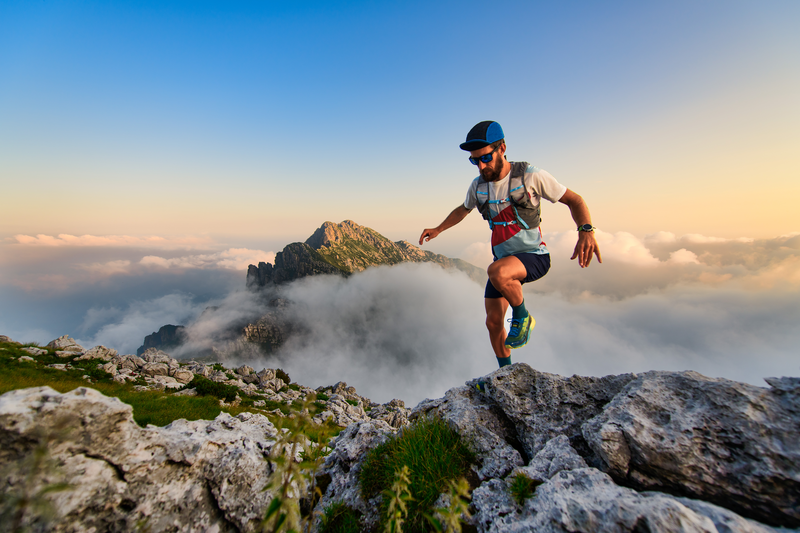
95% of researchers rate our articles as excellent or good
Learn more about the work of our research integrity team to safeguard the quality of each article we publish.
Find out more
ORIGINAL RESEARCH article
Front. Sustain. Food Syst. , 08 November 2024
Sec. Crop Biology and Sustainability
Volume 8 - 2024 | https://doi.org/10.3389/fsufs.2024.1480964
Bioactive compounds have been shown to promote healthy human development and protect against certain diseases including some cancers. Tropical pumpkins bred for consumption, such as calabaza (Cucurbita moschata), possess these compounds, though concentrations vary considerably between varieties. Bioactive compound quantification methods can be expensive, complex, and generate considerable hazardous waste. A predictive tool utilizing the relationship between extrinsic properties and tristimulus colorimetry values of calabaza would allow for significantly higher throughput with lower requirements for equipment, training, and laboratory consumables. Therefore, this study examined correlations between flesh color and bioactive compound quantities of several calabaza germplasm lines to assess the utility of a sustainable and portable predictive tool. CIEL* a* b* values of pumpkin flesh were assessed using a colorimeter and compared to bioactive compound concentrations determined analytically. Redness was found to correlate with increased ascorbic acid and total soluble solids content. Yellowness correlated with β-carotene equivalent carotenoids and ascorbic acid content. These results suggest that colorimetry may be a viable pre-screening technique for quantifying bioactive compounds in C. moschata.
Official methods of analysis of bioactive compounds are often reserved for “well-equipped and staffed” analytical laboratory settings (Nielsen, 2017). For example, the ideal standard for the characterization of carotenoids is typically high-performance liquid chromatography (HPLC) in conjunction with sophisticated detection (i.e., diode-array detector) to produce high sensitivity, reproducibility, and accuracy (Itle and Kabelka, 2009). Such methods require multistep sample preparation before sample injection and thus, the HPLC analysis is a time-consuming and expensive method (Itle and Kabelka, 2009). Alternative (secondary) methods of analysis of target compounds are more rapid, allow high throughput, are more cost-effective, and can reduce the use of organic solvents, which are often harmful. Secondary methods can be employed to quickly screen samples after sufficient calibration against primary official methods (Nielsen, 2017). These methods may be especially beneficial to scientists and farmers in countries where resources are limited (Avnee et al., 2023). Secondary methods of analysis may also promote the use of green chemistry. Green chemistry, as defined by the American Chemical Society, is governed by 12 principles, among them energy conservation, waste reduction, and limited use of hazardous substances for the benefit of the economy, people, and the planet (ACS, 2024). Compared to individual compound analysis methods like HPLC, colorimetry creates less waste and reduces the use of organic solvents (e.g. methanol and hexane) thereby exemplifying green chemistry principles (Demir et al., 2024).
Color is often used as an indication of micronutrient and bioactive compound status in plants; for example, orange-hued fruit flesh is associated with high concentrations of carotenoids such as β-carotene (Feder et al., 2019). Carotenoids are fat-soluble, natural pigments that contribute to the deep coloring of many yellow, orange, and red fruits and vegetables and green, leafy vegetables (Ninčević Grassino et al., 2023). Colorimetry has been used in the food industry as a nondestructive method to assess fruit maturity, senescence, pesticide residues, antioxidants, chlorophyll, and carotenoids (Dutta et al., 2023; Umapathi et al., 2021; Li et al., 2022). Fruit color can be precisely and objectively described using tristimulus colorimetry in which colors are expressed as x, y, and z coordinates that correspond to the three primary colors (red, green, and blue) (Simonne et al., 1993). Prior research findings indicate significant correlations between tristimulus colorimetric values and carotenoid content in other fruits and vegetables, such as sweet potatoes (Itle and Kabelka, 2009; Simonne et al., 1993). Therefore, tristimulus colorimetry could potentially be used as a lower-cost, greener method to estimate the concentrations of certain valuable bioactive compounds in yellow and orange-fleshed vegetables such as tropical pumpkin (Curcurbita moschata).
Previous studies support tropical pumpkin as an appropriate crop to examine the relationship between color and concentration of bioactive compounds. The primary carotenoids identified in C. moschata are β-carotene, zeaxanthin, and lutein (Kulczyński and Gramza-Michałowska, 2019). Tropical pumpkin, commonly referred to as winter squash, is a highly nutritious commodity, though it remains a minor agricultural crop in the contiguous United States and is marketed mostly to Latin populations. It is one of the most important commodities in Puerto Rico and is prominent in many Latin American countries (Maynard et al., 2002). A surge in ethnic populations in the U.S. has led to increased demand for tropical pumpkin (Erwin, 2022). Cucurbits (e.g. Cucurbita spp.) contain numerous bioactive compounds such as β-carotene and ascorbic acid that promote human health beyond nutritional requirements (Kulczyński and Gramza-Michałowska, 2019; USDA FDC, 2024). Cucurbits are also considered to be “excellent” sources of vitamin C, phosphorus, magnesium, and several B vitamins (USDA FDC, 2024). The Food and Drug Administration (FDA) defines an excellent source of an essential nutrient as any food providing ≥20 percent of the recommended daily amount in a typical single serving (FDA, 2024).
In addition to their health-promoting effects, bioactive compounds found in C. moschata, particularly antioxidants, are also important to the food industry given the frequency of oxidation-reduction reactions in foods, some of which can be deleterious. Oxidation-reduction reactions in foods can lead to the degradation of lipids, vitamins, and pigments and decrease nutritional value or catalyze the development of off-flavors (Lindsay, 2017). Food antioxidants, such as ascorbic acid, phenols, and β-carotene, either interrupt the free radical chain oxidation-reduction reaction, scavenge and quench singlet oxygen, or both. Mixed antioxidants in a food system may work synergistically to increase the overall efficiency of antioxidant capacity, but the mechanisms governing this synergy are not well understood (Lindsay, 2017).
Significant variability exists in the nutrient compositions among C. moschata cultivars (Kulczyński and Gramza-Michałowska, 2019). The nutrient variability found in Kulczyński and Gramza-Michalowska's research (Kulczyński and Gramza-Michałowska, 2019) supports the need for a low-cost, predictive tool to quickly and efficiently assess bioactive compounds in cucurbits. The utility of a predictive model requires strong correlations between measured and predicted parameters. Therefore, an exploration of the relationship between physical parameters and bioactive compound concentrations is warranted. In this study, moisture, soluble solids, carotenoids, ascorbic acid, and phenolics were determined in nine tropical pumpkin breeding lines and a commercially available butternut squash cultivar as a control using official methods of analysis. In the first part of this study, the concentrations of these analytes were correlated with tristimulus colorimetric values obtained from nonrandom samples (plugs, Figure 1) of tropical pumpkin flesh to analyze the strength of linear relationships between color and extrinsic properties. In the second part of this study, the extrinsic properties and tristimulus colorimetric values of homogenized flesh samples were assessed to evaluate the potential for bioactive content predictions in whole tropical pumpkin fruit. This study's objective was to assess the strength of the correlations between micronutrient and bioactive compound concentrations determined via official methods with those measured using colorimetry. A simple pre-screening tool to assess vitamin C, carotenoids, and phenolic compounds could benefit plant breeding efforts and allow for crop nutrient assessments performed in the field while increasing analysis efficiency, mitigating waste, and reducing hazardous solvent use.
Figure 1. Subsample plugs of flesh, each measured for color (“x”) on both sides of plug, in triplicate (skin removed) for a total of six colorimetric measures per plug. Each plug was designated for one of the following analyses: β-carotene equivalent carotenoids, ascorbic acid, soluble solids, and total phenolic content. An image of pumpkin flesh is included.
Aqueous 3% meta-phosphoric acid in acetic acid; hexane with added 0.001% butylated hydroxytoluene (BHT) (Fisher Chemical, Fair Lawn, NJ, USA); 2,6-dichloroindophenol (DCIP) sodium salt hydrate (Sigma-Aldrich, St. Louis, MO, USA); sodium bicarbonate (NaHCO3) (Fisher Chemical, Fair Lawn, NJ, USA); 95% aqueous methanol; gallic acid standard; 10% (v/v) Folin-Ciocâlteu (FC) reagent.
Tropical pumpkins were harvested 92 days after transplanting at the Plant Science Research and Education Unit in Citra, FL. Harvest indices of rind color and rind hardness (thumb prick test) were used to determine fruit maturity. Because “Waltham” butternut squash (Cucurbita moschata) is of the same species as tropical pumpkin and boasts a wealth of published nutrient composition data, it was used as a control in this study and was planted alongside the experimental breeding lines of tropical pumpkin as two-week-old transplants, as well. During the growing period, air temperatures (60 cm height) ranged from 14.1 to 30.0°C. Immediately following harvest, tropical pumpkin fruits were cleaned of dirt and debris, then weighed and measured. The fruits were then stored at 10°C with 60–65% relative humidity for ~2 weeks until they were processed for analysis.
A metal borer (21 mm diameter) was used to remove four plugs of flesh from the widest equatorial point of each sample tropical pumpkin fruit (Figure 1). Each plug was trimmed to approximately 4 mm in length and approximately 2 g in mass. The rind was removed from each plug and the color parameters of plugs were measured with a handheld colorimeter (Konica Minolta Chroma Meter CR-400, Ramsey, NJ). Employing diffuse illumination, a 0° viewing angle, illuminant C, and the CIEL* a* b* color space, L* a* b* values for the rind side of exposed flesh, and the seed side of the flesh subsample were measured three times, for a total of six L* a* b* measurements per plug subsample (Figure 2).
Figure 2. The CIEL* a* b* color space includes three axes where a* indicates redness to greenness, b* indicates yellowness to blueness, and L* indicates whiteness to blackness. As the hue* angle increases about the axis along the a*, b* plane, the hues change (e.g., red = 0°, yellow = 90°, green = 180°, blue = 270°). Chroma describes the saturation of color. (Adapted from Jacobolus (2007) via Wikimedia Commons, licensed under CC BY-SA 3.0).
Flesh plugs were wrapped in aluminum foil, vacuum-sealed, labeled for each analysis and frozen at −26°C until the analyses were performed after 1–6 months. Each remaining sample fruit was then cut in half from stem to blossom end opposite the point from which the plug was drawn. Rind and seeds were discarded and flesh from half the fruit was ground in a food processor until it was similar in size to coarse sea salt.
Ground subsamples were randomly apportioned by assay, wrapped in aluminum foil, and vacuum sealed (Figure 3). For moisture content analyses, 2 g of ground subsample were wrapped in foil and vacuum sealed while 20 g of ground subsample were reserved for both carotenoid and phenolic analyses. The remaining flesh was also wrapped in aluminum foil and vacuum sealed. The subsamples were held at −26°C until the analyses were performed, ~1–6 months following preservation.
Figure 3. Schematic illustrating triplicate colorimetric measurements (“x”) taken from the skin side of a plug of flesh subsample (skin removed). The opposite half of tropical pumpkin was reserved for moisture, soluble solids, β-carotene equivalent carotenoids, ascorbic acid, and total phenolic compound analyses.
Two subsamples of ground flesh from each pumpkin were reserved for ascorbic acid analysis. The subsamples were weighed (~2 g), and its mass was recorded before being submerged in 20 mL aqueous 3% meta-phosphoric acid/acetic acid held within conical plastic test tubes fitted with screw caps. The test tubes were then covered with aluminum foil and stored at −20°C until the analysis was performed. All frozen subsamples were brought to room temperature before being analyzed.
The moisture content of the tropical pumpkin breeding lines and the butternut squash was assessed using AOAC Official Method 930.15, Two-gram ground subsamples were apportioned into moisture tins and dried in a forced draft moisture oven at 135 ± 2°C for 2 h ± 5 min or until the mass of the subsamples plateaued (Carvalho de Gomes et al., 2012; AOAC International, 2012; FDA, 2021). Upon drying, covered moisture tins were placed in a desiccator to cool. Once cooled, the loss in weight on drying (LOD) was used to calculate the moisture content (fresh weight basis).
Ground tropical pumpkin and butternut squash subsamples were pressed through cheesecloth. The liquid fraction was applied to the sample well of a temperature-compensating refractometer (r2i300, Reichert Technologies, Depew, NY). Total soluble solids were measured in triplicate, averaged, and reported in °Brix for each tropical pumpkin sample. The total soluble solids content of each subsample plug was determined using the same method, except a mortar and pestle was used to expel the liquid fraction from the flesh plug.
A direct solvent extraction method based on the method used by Kimura et al. (2007) was used to extract carotenoids from the tropical pumpkin and butternut squash subsamples. Duplicate subsamples, weighing approximately 3 g each, were homogenized (PowerGen 700D, Fischer Scientific, Waltham, MA) in hexane with 0.001% butylated hydroxytoluene (BHT) (Fischer Chemical, Fair Lawn, NJ) for 2 min at 8,000 RPM. Homogenized subsamples were filtered into respective beakers through Whatman #2 filter paper-lined glass funnels until homogenate was free of flesh particulates. The homogenates were then filtered into new beakers through anhydrous sodium sulfate-filled Whatman #42 filter paper-lined glass funnels until solutions were no longer visibly turbid. Once clear, 200 μL of homogenate were transferred to a 96-well microplate. The absorbance of each subsample was measured at 450 nm at 30°C. Calibration curves were constructed using β-carotene standards (Sigma Aldrich, St. Louis, MO) in concentrations ranging from 0.6 μM to 3.0 mM. This method was repeated to determine the approximate β-carotene equivalent concentrations in each subsample plug.
This titrimetric method was adapted from AOAC Method 967.21, AOAC Official Methods of Analysis 45.1.14, and modified by Eitenmiller et al. (AOAC International, 2012; Ye et al., 2007). This analysis was performed in duplicate using subsamples from three tropical pumpkin fruits from each of the nine experimental breeding lines plus the butternut squash, totaling 60 subsamples. To extract ascorbic acid, 2 g ground tropical pumpkin flesh was homogenized with 35 mL of 3% meta-phosphoric acid in acetic acid (w/v) using a high shear homogenizer (PowerGen 700D, Fisher Scientific, Waltham, MA). The homogenate was filtered using fluted Whatman #2 filter paper.
The standard dye was prepared by dissolving 251 mg 2,6-dichloroindophenol (DCIP) sodium salt hydrate (Sigma-Aldrich, St. Louis, MO) in 250 mL of deionized water containing 211 mg sodium bicarbonate (NaHCO3) (Fisher Chemical, Fair Lawn, NJ). The DCIP solution was diluted to 1,000 mL with deionized water. The dye was filtered through fluted Whatman #2 filter paper and stored under refrigeration (4°C) in an amber glass bottle. To determine the equivalency factor of DCIP concentration to ascorbic acid concentration, 2 mL of ascorbic acid standard were added to 5 mL of the 3% meta-phosphoric acid extractant and titrated with DCIP solution until a light, rose pink color persisted for 5 s or longer. DCIP concentration was expressed as mg ascorbic acid equivalent per 1.0 mL dye solution. A blank was determined by titrating 7 mL of solvent containing deionized water equal to the average volume of dye required to titrate the ascorbic acid standard. This method was repeated for the determination of ascorbic acid content in subsamples homogenates.
This method was adapted from the Folin-Ciocâlteu assay of Singleton and Rossi (1965, as cited by Ainsworth and Gillespie) with modifications described by Ainsworth and Gillespie and Loayza Davila (Ainsworth and Gillespie, 2007; Loayza et al., 2020). This method was performed in duplicate using three fruits from each experimental breeding line plus the butternut squash, totaling 60 subsamples. Approximately 0.4 g of ground tropical pumpkin flesh was homogenized with ice-cold 95% aqueous methanol for 2 min using a high shear homogenizer (PowerGen 700D, Fisher Scientific, Waltham, MA). The homogenate was incubated at room temperature for 48 h in the dark. Homogenate was then centrifuged at 13,000 g for 5 min at room temperature. Then 100 μL each of sample supernatant, gallic acid standard solutions (ranging from 39 μM to 2.5 mM in 95% methanol), and 95% methanol (blank) were added to fresh microcentrifuge tubes. Next, 200 μL of 10% (v/v) Folin-Ciocâlteu reagent was added and the solution vortexed thoroughly. Following the addition of Folin-Ciocâlteu reagent, 800 μL of 700 mM sodium carbonate (Na2CO3) was added to each tube and left to incubate at room temperature for 2 h. After incubation, the microcentrifuge tubes were centrifuged for 5 min at 13,000 g to pelletize sodium carbonate particulates. A 200 μL aliquot of either sample, standard, or blank supernatant were transferred to a clear 96-well microplate, and the absorbance was read at 765 nm.
Gallic acid standards ranging from 39 μM to 2.5 mM in concentration were used to construct a calibration curve to calculate the total phenolic content of each sample, reported as gallic acid equivalents (GAE). This method was repeated to approximate phenolic compound concentrations using 2 g subsamples.
Linear Pearson correlation coefficients (r) were calculated using R. Pearson correlation coefficients are values ranging from −1 to 1 that describe the strength of a linear correlation between variables. Categories of r-value strengths referred to in this study are described by Akoglu (2018) where values ≥0.6 were strongly correlated, and values ≥0.4 were moderately correlated. Statistical hypothesis testing of each correlation was conducted in R (RStudio, Vienna, Austria) using Tukey's Honestly Significant Difference test and the multcomp package library. An alpha level of 0.05 was used.
Moisture content was calculated according to the following equation:
Chroma (C*) and hue* angle (ha, b) were calculated from the rendered tristimulus values using the following equations.
In this first part of this study, moisture, bioactive contents, and color of the nine breeding lines of tropical pumpkin were assessed and compared to the control, “Waltham” Butternut Squash (WBS) (Tables 1, 2). βCE analysis of tropical pumpkin yielded a range of 17.4 ± 9.78 to 73.5 ± 27.35 μg/g. The ascorbic acid analysis yielded a range of 58.2 ± 7.45 to 207.4 ± 71.60 μg/g. Analysis of total phenolics yielded results ranging from 168.42 ± 33.58 to 526.59 ± 84.44 μg/g. Results support previous research indicating large variations in bioactive compound concentrations among tropical pumpkin cultivars (Kulczyński and Gramza-Michałowska, 2019). Average CIEL* a* b* values differed by breeding line as expected. Lightness (L*) did not seem to correlate with physical attributes reliably. Redness-greenness (a*) appeared to correlate with carotenoid content, and to a lesser extent ascorbic acid. The lowest yellowness-blueness (b*) value corresponded to the lowest concentration of carotenoids. The correlations between CIEL* a* b* values (including the calculated derivatives C* and hue* angle) and the physical properties: β-carotene, ascorbic acid, total soluble solids (TSS), and total phenolics concentrations were then examined by generating Pearson coefficients (Table 3).
Table 1. Average β-carotene equivalent carotenoids (βCE), ascorbic acid, total phenolic content, and moisture content of raw tropical pumpkin samples from nine breeding lines and the control, butternut squash (WBS) measured on a wet basis.
Table 2. Physical appearance and average CIEL* a* b* values of raw tropical pumpkin samples from nine breeding lines and the control, butternut squash (WBS).
Table 3. Pearson coefficients denoting strength and direction of linear correlations between bioactive compounds of non-random tropical pumpkin plugs and each plug's colorimetric values.
Statistically significant positive correlations were found between a* and ascorbic acid, a* and total soluble solids, b* and β-carotene equivalent carotenoid concentrations, and b* and ascorbic acid. Statistically significant positive correlations were also found between chroma* and β-carotene equivalent carotenoid concentrations, chroma* and ascorbic acid content, hue* angle and ascorbic acid, and hue* angle and total soluble solids.
In the second phase of this study, the same methodology was applied to random samples of tropical pumpkin flesh to test the viability of colorimetry as an indicator of moisture, total soluble solids content, and bioactive compounds (Table 4). Similar results were observed when assessing color and bioactive compounds of random tropical pumpkin samples. The correlations remained statistically significant, albeit slightly weaker relationships in terms of Pearson coefficients. Moisture content was also found to correlate with a*, b*, chroma, and hue* angle.
Table 4. Pearson coefficients denoting strength and direction of linear correlations between target compounds and colorimetric values of random tropical pumpkin flesh samples.
Lastly, the relationships between each extrinsic property were analyzed (Table 5). Total soluble solids content was found to have a statistically significant positive correlation with ascorbic acid and total phenolics content. A statistically significant negative correlation with moisture content was observed. These results suggest that °Brix measurements may also be an indication of the content of ascorbic acid and total phenolic content within the tropical pumpkin varieties in addition to colorimetry. Total phenolic content was also found to strongly correlate positively with ascorbic acid concentration and negatively with moisture content.
Table 5. Pearson coefficients denoting strength and direction of linear correlations between moisture and bioactive compound concentrations of tropical pumpkin samples.
Increased saturation of the hue (measured by chroma*) of the flesh may indicate decreased moisture content; that is, dull-hued flesh is likely to have more moisture than clear, brightly-hued flesh. In contrast, increased saturation of hue may indicate increased concentrations of β-carotene equivalent concentrations, ascorbic acid, total soluble solids, and total phenolic compounds. Results show that an increased hue* angle may indicate increased moisture, which agrees with other findings of this study that correlate redness and yellowness (a* and b*) with decreased moisture. Results from Simonne et al. (1993) and Itle and Kabelka (2009) were also similar in correlation directions. In particular, Simonne et al. (1993) found that β-Carotene content was highly correlated (r = −0.99) with hue angle in sweet potato breeding lines with yellow to deep orange color. The difference in correlation strengths between this study and those of Simonne et al. (1993) and Itle and Kabelka (2009) may be attributed to the methods of analysis used in each study and, in the case of research conducted by Simonne et al., the use of a different color space (Hunter L, a, b vs. CIEL* a* b* used here). As others have shown, correlations can be used in a predictive manner (Dutta et al., 2023). The results overall make a case for the potential of colorimetry as a predictive tool for assessing bioactive compound content.
In this study, colorimetry was found to have some predictive potential for moisture content in tropical pumpkins. Using nonrandom plug samples, redness was correlated with ascorbic acid and total soluble solids content (r = 0.61, r = 0.65). Yellowness was found to indicate higher β-carotene equivalent carotenoid concentrations and greater amounts of ascorbic acid (r = 0.64, r = 0.59). The random blended flesh samples also displayed similar correlation strengths for colorimetry and β-carotene, ascorbic acid, and total soluble solids (r = 0.54, 0.44, 0.64 for redness, r = 0.56, 0.51, 0.60 for yellowness). In the first part of this study, chroma strongly correlated with β-carotene and ascorbic acid (r = 0.66, 0.61). In the second part, chroma also moderately correlated with all five extrinsic properties (moisture, carotene, ascorbic acid, total soluble solids, and total phenolics) (r = −0.57, 0.57, 0.51, 0.62, 0.41). The correlations remained significant for the random samples, though they were slightly weaker in some cases due to normal variation in both the color and nutritional composition of Cucurbita species (Kulczyński and Gramza-Michałowska, 2019). Total phenolic content was found to strongly correlate positively with ascorbic acid concentration and negatively with moisture content. This finding aligns with previous results that found a Pearson correlation between sinapic and ferulic phenolic acids and L-ascorbic acid in Chinese cabbage sprouts (Šola et al., 2020). Since chroma represents color saturation and correlations were found between various properties and redness, yellowness, or both, chroma may more accurately predict bioactive compound presence, particularly that of ascorbic acid, than redness or yellowness alone (Mouw, 2018). The negative correlation between moisture and chroma makes sense as fruit color generally becomes more saturated in color and bioactive compound concentrations increase as fruits lose moisture during ripening (Frenkel and Hartman, 2012).
A predictive tool utilizing the relationships between extrinsic properties and tristimulus colorimetry values of tropical pumpkins would allow for significantly higher throughput while reducing requirements for equipment, training, and laboratory consumables. Based on the results of this study, colorimetry can be an effective tool for assessing relative bioactive contents in tropical pumpkins. As displayed in Figure 4, the colorimeter identified color differences between tropical pumpkin flesh that are not discernable to the naked eye. Due to the moderate correlations found between some properties, utilizing several of the relationships explored (i.e., using both chroma and a* to predict β-carotene content) may improve a tool's accuracy and usefulness. Multiple factors could be integrated using a mathematical model. Several types of models should be explored in the future to optimize predictive capabilities. Such a predictive assessment tool could save hundreds of mL of organic solvents per analysis (Itle and Kabelka, 2009; Kimura et al., 2007), indicating that colorimetry could be a useful device to promote green chemistry.
Figure 4. Bioactive content of nine tropical pumpkin breeding lines alongside a control, butternut squash. Snapshots of each pumpkin's flesh color are included above its respective breeding line.
Overall, there were correlations between tristimulus colorimetry values and bioactive compounds such as ascorbic acid, β-carotene, and total phenolics content (Figure 4). The results of the study suggest that tristimulus colorimetry may be a viable pre-screening assessment tool to reduce laboratory consumables and waste. Such a tool could offer utility in plant breeding efforts and nutritional assessments of crops in resource-limited populations or work sites (e.g., pilot plants, rural farms). This concept may also apply to other commodities. Efforts toward simple, low-cost precision agriculture tools are key to maximizing the feasibility of implementation in low-income countries where specialized training and capital are limited. Future studies could further explore the correlations between soluble solids and bioactive compounds and evaluate the strength of correlations between color space values and bioactive compound concentrations by increasing sample sizes. Future research could also explore potentially nonlinear relationships (e.g., chroma with ascorbic acid) to better elucidate the viability of colorimetry as a predictive tool.
The raw data supporting the conclusions of this article will be made available by the authors, without undue reservation.
MK: Conceptualization, Data curation, Formal analysis, Investigation, Methodology, Project administration, Software, Validation, Visualization, Writing – original draft, Writing – review & editing. MS: Data curation, Visualization, Writing – original draft, Writing – review & editing. GM: Project administration, Resources, Writing – review & editing, Funding acquisition. CC: Project administration, Resources, Writing – review & editing, Funding acquisition. SS: Project administration, Resources, Writing – review & editing, Supervision. AS: Project administration, Resources, Writing – review & editing, Conceptualization, Formal analysis, Funding acquisition, Methodology, Supervision. AM: Conceptualization, Funding acquisition, Project administration, Resources, Software, Supervision, Writing – review & editing, Visualization.
The author(s) declare financial support was received for the research, authorship, and/or publication of this article. This material is based upon work that was supported by the National Institute of Food and Agriculture, U.S. Department of Agriculture, under award number 2020-38640-31521 through the Southern Sustainable Agriculture Research and Education program under subaward number LS21-360. USDA is an equal opportunity employer and service provider.
This study was supported by the University of Florida's Food Science and Human Nutrition and Horticultural Sciences Department. Thank you to the staff at the UF/IFAS Plant Science Research and Education Unit and Dr. Parmeshwor Aryal for crop management. Thank you to Dr. Ziynet Boz and Dr. Martin-Ryals for providing mentorship through the USDA Research and Extension Experience for Undergraduates. Thanks to Megan E. Kinsman for her substantial work on her thesis (Kinsman, 2023).
The authors declare that the research was conducted in the absence of any commercial or financial relationships that could be construed as a potential conflict of interest.
All claims expressed in this article are solely those of the authors and do not necessarily represent those of their affiliated organizations, or those of the publisher, the editors and the reviewers. Any product that may be evaluated in this article, or claim that may be made by its manufacturer, is not guaranteed or endorsed by the publisher.
ACS (2024). 12 Principles of Green Chemistry. Washington, D.C.: American Chemical Society. Available at: https://www.acs.org/greenchemistry/principles/12-principles-of-green-chemistry.html (accessed June 06, 2024).
Ainsworth, E. A., and Gillespie, K. M. (2007). Estimation of total phenolic content and other oxidation substrates in plant tissues using folin–ciocalteu reagent. Nat. Protoc. 2, 875–877. doi: 10.1038/nprot.2007.102
Akoglu, H. (2018). User's guide to correlation coefficients. Turk. J. Emerg. Med. 18, 91–93. doi: 10.1016/j.tjem.2018.08.001
AOAC International (2012). “Official method 930.15,” in AOAC International, Official Methods of Analysis, edition no. 19, ed. G. W. Latimer (Gaithersburg, MD: AOAC International).
Avnee, Sood, S., Chaudhary, D. R., Jhorar, P., and Rana, R. S. (2023). Biofortification: an approach to eradicate micronutrient deficiency. Front. Nutr. 10:1233070. doi: 10.3389/fnut.2023.1233070
Carvalho de Gomes, L. M. J., Godoy, P. B., Pacheco, R. L., do Monte, P. H. F., de Carvalho, J. L. V., Nutti, M. R., et al. (2012). Total carotenoid content, α-carotene and β-carotene, of landrace pumpkins (Cucurbita moschata Duch): a preliminary study. Food Res. Intern. 47, 337–340. doi: 10.1016/j.foodres.2011.07.040
Demir, I., Bulduk, I., Darwish, I. A., and Enginar, H. (2024). A green approach for metoclopramide quantification in pharmaceutical products: new HPLC and spectrophotometric methods. Sci. Rep. 14:8765. doi: 10.1038/s41598-024-59149-6
Dutta, K., Nath, R., Dutta, K., and Nath, R. (2023). Application of Colorimetry in Food Industries. London, UK: IntechOpen.
Erwin, J. (2022). For the Greater Gourd: Growing Calabaza in Florida to Fuel the Fall Season. Available at: https://blogs.ifas.ufl.edu/fshndept/2022/04/04/for-the-greater-gourd-growing-calabaza-in-florida-to-fuel-the-fall-season/ (accessed June 14, 2024).
FDA (2021). Food homogenization. Elemental Analysis Manual for Food and Related Products. Available at: https://www.fda.gov/media/89329/download (accessed June 11, 2024).
FDA (2024). 21 CFR Part 101 Subpart D – Specific Requirements for Nutrient Content Claims. Available at: https://www.ecfr.gov/current/title-21/part-101/subpart-D (accessed June 06, 2024).
Feder, A., Chayut, N., Gur, A., Freiman, Z., Tzuri, G., Meir, A., et al. (2019). The role of carotenogenic metabolic flux in carotenoid accumulation and chromoplast differentiation: lessons from the melon fruit. Front. Plant Sci. 10:1250. doi: 10.3389/fpls.2019.01250
Frenkel, C., and Hartman, T. G. (2012). Decrease in fruit moisture content heralds and might launch the onset of ripening processes. J. Food Sci. 77, S365–376. doi: 10.1111/j.1750-3841.2012.02910.x
Itle, R. A., and Kabelka, E. A. (2009). Correlation between L*a*b* color space values and carotenoid content in pumpkins and squash (Cucurbita spp.). HortScience 44, 633–637. doi: 10.21273/HORTSCI.44.3.633
Jacobolus (2007). Wikimedia Commons. Available at: https://commons.wikimedia.org/wiki/File:Munsell-system.svg (accessed October, 30, 2024).
Kimura, M., Kobori, C. N., Rodriguez-Amaya, D. B., and Nestel, P. (2007). Screening and HPLC methods for carotenoids in sweetpotato, cassava and maize for plant breeding trials. Food Chem. 100, 1734–1746. doi: 10.1016/j.foodchem.2005.10.020
Kinsman, M. E. (2023). Macronutrients and Bioactive Compounds Present in Calabaza (Tropical Pumpkin) Breeding Lines and Correlations with Mesocarp Color (Master's thesis). UF Digital Collections, University of Florida, Gainesville, FL, United States.
Kulczyński, B., and Gramza-Michałowska, A. (2019). The profile of secondary metabolites and other bioactive compounds in Cucurbita pepo L. and Cucurbita moschata pumpkin cultivars. Molecules 24:2945. doi: 10.3390/molecules24162945
Li, Y., Sun, X., Zhou, L., Tian, L., Zhong, K., Zhang, J., et al. (2022). Novel colorimetric and NIR fluorescent probe for bisulfite/sulfite detection in food and water samples and living cells based on the PET mechanism. J. Agric. Food Chem. 70, 10899–10906. doi: 10.1021/acs.jafc.2c04571
Loayza, F. E., Brecht, J. K., Simonne, A. H., Plotto, A., Baldwin, E. A., Bai, J., et al. (2020). Enhancement of the antioxidant capacity of ripe tomatoes by the application of a hot water treatment at the mature-green stage. Postharvest Biol. Technol. 161:111054. doi: 10.1016/j.postharvbio.2019.111054
Maynard, D. N., Elmstrom, G. W., Talcott, S. T., and Carle, R. B. (2002). ‘El Dorado' and ‘La Estrella': compact plant tropical pumpkin hybrids. HortScience 37, 831–833. doi: 10.21273/HORTSCI.37.5.831
Mouw, T. (2018). Tolerancing Part 3: Color Space vs. Color Tolerance. Available at: https://www.xrite.com/blog/tolerancing-part-3 (accessed June 14, 2024).
Nielsen, S, . (ed.). (2017). Food Analysis: Introduction to Food Analysis, 5th Edn. Cham: Springer. doi: 10.1007/978-3-319-45776-5_1
Ninčević Grassino, A., Rimac Brnčić, S., Badanjak Sabolović, M., Šic Žlabur, J., Marović, R., Brnčić, M., et al. (2023). Carotenoid content and profiles of pumpkin products and by-products. Molecules 28:858. doi: 10.3390/molecules28020858
Simonne, A. H., Kays, S. J., Koehler, P. E., and Eitenmiller, R. R. (1993). Assessment of β-carotene content in sweetpotato breeding lines in relation to dietary requirements. J. Food Comp. Analy. 6, 336–345. doi: 10.1006/jfca.1993.1037
Singleton, V. L., and Rossi, J. A. (1965). Colorimetry of total phenolics with phosphomolybdic-phosphotungstic acid reagents. Am. J. Enol. Vitic. 16, 144–158. doi: 10.5344/ajev.1965.16.3.144
Šola, I., Vujčić Bok, V., Dujmović, M., and Rusak, G. (2020). Developmentally-related changes in phenolic and l-ascorbic acid content and antioxidant capacity of Chinese Cabbage Sprouts. J. Food Sci. Technol. 57, 702–712. doi: 10.1007/s13197-019-04103-y
Umapathi, R., Sonwal, S., Lee, M. J., Mohana Rani, G., Lee, E. S., Jeon, T. J., et al. (2021). Colorimetric based on-site sensing strategies for the rapid detection of pesticides in agricultural foods: new horizons, perspectives, and challenges. Coord. Chem. Rev. 446:214061. doi: 10.1016/j.ccr.2021.214061
USDA FDC (2024). FoodData Central. Available at: https://fdc.nal.usda.gov/ (accessed June 06, 2024).
Keywords: Cucurbita moschata, tropical pumpkin, colorimetry, bioactive compounds, β-carotene, green chemistry
Citation: Kinsman ME, Serviss MT, Meru G, Chase CA, Sargent SA, Simonne A and MacIntosh AJ (2024) A greener approach to assess bioactive compounds in tropical pumpkin (Cucurbita moschata) using colorimetry. Front. Sustain. Food Syst. 8:1480964. doi: 10.3389/fsufs.2024.1480964
Received: 14 August 2024; Accepted: 21 October 2024;
Published: 08 November 2024.
Edited by:
Pushp Sheel Shukla, Centre for Cellular and Molecular Platforms, IndiaReviewed by:
Alan T. Critchley, The Verschuren Centre (VC), CanadaCopyright © 2024 Kinsman, Serviss, Meru, Chase, Sargent, Simonne and MacIntosh. This is an open-access article distributed under the terms of the Creative Commons Attribution License (CC BY). The use, distribution or reproduction in other forums is permitted, provided the original author(s) and the copyright owner(s) are credited and that the original publication in this journal is cited, in accordance with accepted academic practice. No use, distribution or reproduction is permitted which does not comply with these terms.
*Correspondence: Andrew J. MacIntosh, YW5kcmV3bWFjaW50b3NoQHVmbC5lZHU=
Disclaimer: All claims expressed in this article are solely those of the authors and do not necessarily represent those of their affiliated organizations, or those of the publisher, the editors and the reviewers. Any product that may be evaluated in this article or claim that may be made by its manufacturer is not guaranteed or endorsed by the publisher.
Research integrity at Frontiers
Learn more about the work of our research integrity team to safeguard the quality of each article we publish.