- 1Laboratory of Quality and Safety Risk Assessment for Agro-Products, Ministry of Agriculture and Rural Affairs, School of Food and Pharmaceutical Engineering, Zhaoqing University, Zhaoqing, China
- 2School of Agricultural Engineering, Jiangsu University, Zhenjiang, Jiangsu Province, China
- 3Zhaoqing Branch Center of Guangdong Laboratory for Lingnan Modern Agricultural Science and Technology, Zhaoqing, Guangdong, China
- 4Key Laboratory of Tropical Fruit and Vegetable Cold-Chain of Hainan Province, Institute of Agro-products of Processing and Design, Hainan Academy of Agricultural Sciences, Haikou, China
- 5Fujian Provincial Key Laboratory of Food Microbiology and Enzyme Engineering, Xiamen, China
Sample pretreatment is an essential procedure in pesticide analysis, as the matrix effect can significantly influence the results. In this study, a covalent organic framework (COF) was synthesized using 1,2,4,5-tetrakis-(4-formylphenyl)benzene (TFPB) and benzidine (BD) to mitigate the matrix effect in vegetable and fruit samples. This COF was then used to develop a solid-phase extraction (CSPE) method. In addition, the COF was used to create a magnetic COF (MCOF) for use in magnetic solid-phase extraction (MSPE). The synthesized COF and MCOF were thoroughly characterized using scanning electron microscopy (SEM) for morphological analysis, Fourier-transform infrared spectroscopy (FT-IR) for chemical bond identification, and N2 adsorption–desorption measurements for porosity and surface area assessment. Key pretreatment parameters such as buffers, dilution rate, sorbent dosage, extraction time, elution solvent, and reuse number were optimized. The developed CSPE and MSPE showed excellent purification ability for the matrix of vegetable and fruit samples. The reuse test demonstrated that the synthesized COF and MCOF can be reused up to 15 times. Moreover, the developed CSPE and MSPE showed acceptable recoveries in spiked recovery tests, suggesting that these pretreatment methods were feasible for sample purification in pesticide analysis.
Introduction
Pesticides are widely used in agricultural production to control pests and diseases, making them the backbone of the agri-food sector in its endeavor to secure food production (Chen Z. Y. et al., 2023; Li H. et al., 2022; Ma et al., 2023). However, pesticides are typically used in large quantities, so they pose potential risks to organisms across various environments and demonstrate toxicity toward human beings (Meng et al., 2022; Zhang et al., 2023). Moreover, high levels of pesticide residue in food may cause chronic poisoning and even cancer (Pedroso et al., 2022; Yang et al., 2018). Therefore, to protect consumers from the hazards of pesticides, it is necessary to improve the monitoring of pesticide residues in foods.
Instrumental methods are commonly used for the detection of pesticide residues in foods due to their high accuracy, precision, reproducibility, and selectivity. Several instrumental methods for pesticide analysis have been reported, including HPLC-MS/MS (Dong et al., 2023), GC–MS/MS (Harischandra et al., 2021), AChE sensor (Dai et al., 2024), immunoassays (Guo et al., 2024), and electrochemical sensors (Ding et al., 2024). In non-targeted food safety analysis, it is crucial to ensure that the sample pretreatment eliminates matrix effects and retains chemical contaminants (Wang et al., 2021). Previous studies have reported advanced materials for cleaning up the extracts from vegetable or fruit samples, including carbon nitride materials (Pan et al., 2022), molecularly imprinted polymers (Abdulhussein et al., 2021), metal–organic frameworks (MOFs) (Liu et al., 2022), and covalent organic frameworks (COFs) (Wang et al., 2021). COFs possess metal-free π-conjugated backbone structures connected by covalent bonds and feature large specific surface areas, good thermal stability, and abundant pores (Dong et al., 2024; Liu et al., 2024; Zhang et al., 2024). Hence, COFs can effectively adsorb organic compounds and eliminate the interference of macromolecular impurities in the matrix by the size exclusion effect (Lin et al., 2020), making them suitable materials for sample pretreatment.
In this study, a novel COF was synthesized using 1,2,4,5-tetrakis-(4-formylphenyl)benzene (TFPB) and benzidine (BD) to remove the matrix effect of vegetable and fruit samples (Scheme 1). The COF was used to prepare a solid-phase extraction (CSPE) method. It was further used to prepare a magnetic COF (MCOF) for the development of magnetic solid-phase extraction (MSPE). Moreover, key pretreatment parameters including buffers, dilution rate, dosage of sorbent, time for extraction, elution solvent, and number of reuses were optimized. Both pretreatment methods were used for pesticide analysis, and the results of the recovery test were verified using HPLC-MS/MS to evaluate the accuracy, reliability, and practicability of the two developed pretreatment methods.
Materials and methods
Materials and reagents
Pesticide standards were purchased from Tanmo Technology Ltd. (Beijing, China). 1,2,4,5-Tetrakis-(4-formylphenyl)benzene (TFPB), benzidine (BD), N-2-hydroxyethylpiperazine-N-ethane-sulfonic acid (Hepes), Tris-(hydroxymethyl)-aminomethane (Tris), and 1,4-dioxane were purchased from Aladdin Chemical Technology Co., Ltd. (Shanghai, China). Amino magnetic beads (5 mg/mL) were supplied by Beaver Biomedical Co., Ltd. (Suzhou, China). Boric acid, acetonitrile, acetone, N,N-dimethylformamide (DMF), methanol, and ethanol were supplied by Damao Chemical Technology Co., Ltd. (Tianjin, China).
Instruments
Absorbance was measured using an HBS-Scan Y microplate reader (DeTie Biotechnology Co., Ltd., China). Centrifuge (TGL-15B) was purchased from Anting Scientific Instrument Factory (Shanghai, China). HPLC-MS/MS analysis was performed using a 1,290 Infinity-6495 system (Agilent Technologies, Inc., CA, USA) using a liquid chromatography column (Welch, 100 × 2.1 mm, 3.5 μm).
Preparation of COF
The synthesis of COF was according to the method reported by Li et al. (2023). Briefly, 19.8 mg of TFPB and 14.7 mg of BD were dissolved in 4 mL of 1,4-dioxane, respectively. Afterward, the two solutions were mixed, and 1 mL of 6 mol/L acetic acid was added subsequently. The mixture was rested at room temperature for 72 h in the dark. The product was washed with ethanol three times and dried at 60°C. Then, 10 mg of COF was added into a 3-mL SPE column to prepare CSPE.
Preparation of MCOF
First, 19.8 of mg TFPB and 14.7 mg of BD were separately dissolved in 4 mL of 1,4-dioxane each. Afterward, 1.0 mL of amino magnetic beads was added to the BD solution before adding the TFPB solution. Then, 6 M acetic acid was added and mixed. The mixture was rested at room temperature for 72 h in the dark. The product (MCOF) was washed with ethanol three times and dried at 60°C. The MCOF powder was used to develop MSPE.
Optimization of CSPE and MSPE
To develop CSPE and MSPE, several parameters were optimized, including types of diluents, dilution rate, types of eluents, and addition amount for COF and MCOF. The removal percentage of the matrix (R%) and elution efficiency (E%) were calculated using the following equations:
Here, AS, AP, and AE are the absorption values at 666 nm for sample extract, purified solution, and eluent, respectively. The conditions for single-factor optimization are summarized in Supplementary Tables S1, S2.
Procedures of CSPE and MSPE
For the purification of CSPE, 3 mL of acetonitrile was added to the column for activation. Then, 1 mL of sample extract was loaded into the CSPE, and the flow speed was kept at 0.5 mL/min. After purification, the CSPE was renewed by washing with 5 mL of acetone.
For the purification of MSPE, 5 mg of MCOF was added to a 1.5 mL centrifuge tube. Afterward, 1 mL of acetonitrile was added for activation with the assistance of a vortex mixer for 30 s. Acetonitrile was removed after magnetic separation. The sample extract (1 mL) was subsequently loaded for 10-s vortex mixing. The pure extract was obtained after magnetic separation. The MCOF was renewed by washing with 3 mL of acetone after purification.
Sample purification
The sample purification step was performed according to the China National Food Safety Standard (GB 2763–2021, 2021). First, 10 g of samples (cucumber, Chinese cabbage, green grape, and pomelo) was homogenized and mixed with 10 mL of acetonitrile in a 50 mL polypropylene centrifuge tube. Then, 4 g of MgSO4, 1 g of NaCl, 1 g of sodium citrate, 0.5 g of sodium dihydrogen citrate, and a ceramic homogenizer were added and the tube was shaken vigorously for 1 min, followed by centrifuging for 5 min at 4200 r/min. Next, 1 mL of the upper layer was purified using a CSPE column or MSPE, respectively. The purified solution was filtered using a 0.22-μm membrane and analyzed using HPLC-MS/MS. The HPLC-MS/MS parameters are summarized in the Supplementary Tables S3, S4.
Results and discussion
Identification of COF and MCOF
This study synthesized SCOF and MCOF for sample purification owing to their large surface areas for matrix absorption. As shown in Figure 1, scanning electron microscopy (SEM) images revealed surface microstructures of COF and MCOF, displaying porous morphology. The irregular particle morphology of COF was observed in Figure 1A, and its dense porous morphology was clearly shown in Figure 1B, demonstrating the large surface area of COF. Based on the synthesized COF, the MCOF was further prepared. The SEM micrograph (Figure 1C) of the MCOF distinctly exhibited a spherical morphology, notably different from that of the COF. Moreover, the porous nanoflower morphology is clearly illustrated in Figure 1D, which can be attributed to the uniform growth of COF on the surface of amino magnetic beads.
To further analyze the structural characteristics of MCOF, a transmission electron microscope (TEM) was used. The TEM image of MCOF clearly showed that the Fe3O4 core was covered by the nanoflower structure of COF (Figures 2A,B). The element mapping graph showed the presence of Fe and O elements in the MCOF (Figures 2C,D), which can be attributed to the Fe3O4 core. Moreover, the Fe3O4 core was encapsulated by a nanoflower layer of C and N elements, with their presence being attributed to the formation of the C=N group (Figures 2E,F). The energy-dispersive spectrum of MCOF also verified the existence of Fe, O, C, and N elements (Figure 2G).
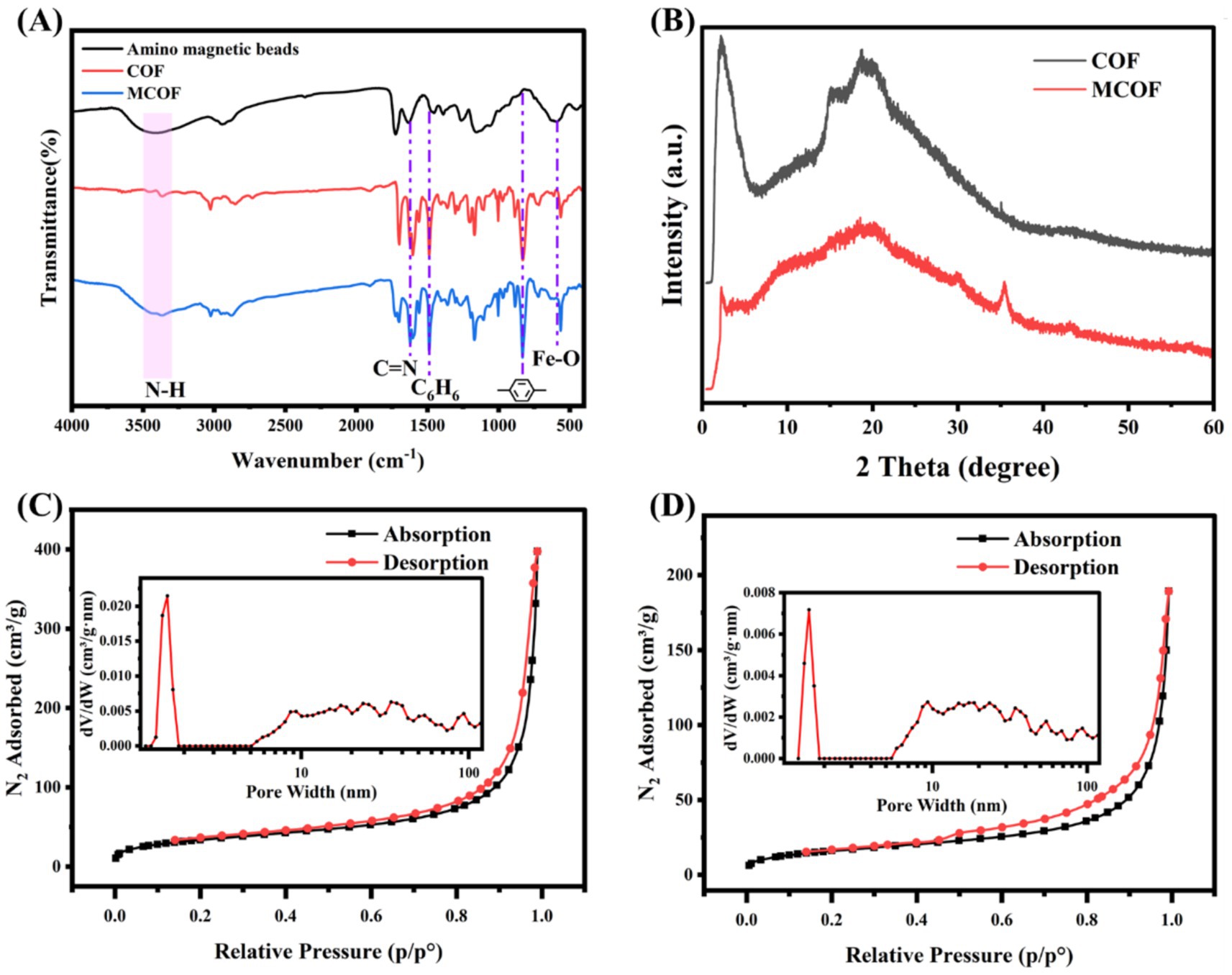
Figure 2. (A) The TEM image of MCOF; (B) the high-angle annular dark field (HAADF) of MCOF; the elemental mapping for MCOF: (C) C, (D) O, (E) N, and (F) Fe; and (G) the energy-dispersive spectrometer spectrum of MCOF.
Fourier-transform infrared (FT-IR) spectroscopy was further carried out to verify the conversion of COF and MCOF (Figure 3A). The broad bands located at 3500 cm−1 to 3,300 cm−1 belong to the N-H vibrations of amidogen (Chen Z. J. et al., 2023). The peak at 1620 cm−1 for COF and MCOF can be ascribed to the stretching vibration of the C=N group (Li W. et al., 2022). For COF and MCOF, the peaks ranging from 1,520 cm−1 to 1,430 cm−1 are indicative of the benzene ring (Wang et al., 2024), whereas the peaks between 860 cm−1 and 800 cm−1 correspond to the para-substitution of the benzene ring. These characteristics can be attributed to the structural elements of BD and TFPB. The weak stretching vibration of the Fe-O bond was observed at 587 cm−1 for MCOF (Li and Row, 2018). All the FT-IR spectra confirmed the successful synthesis of both COF and MCOF.
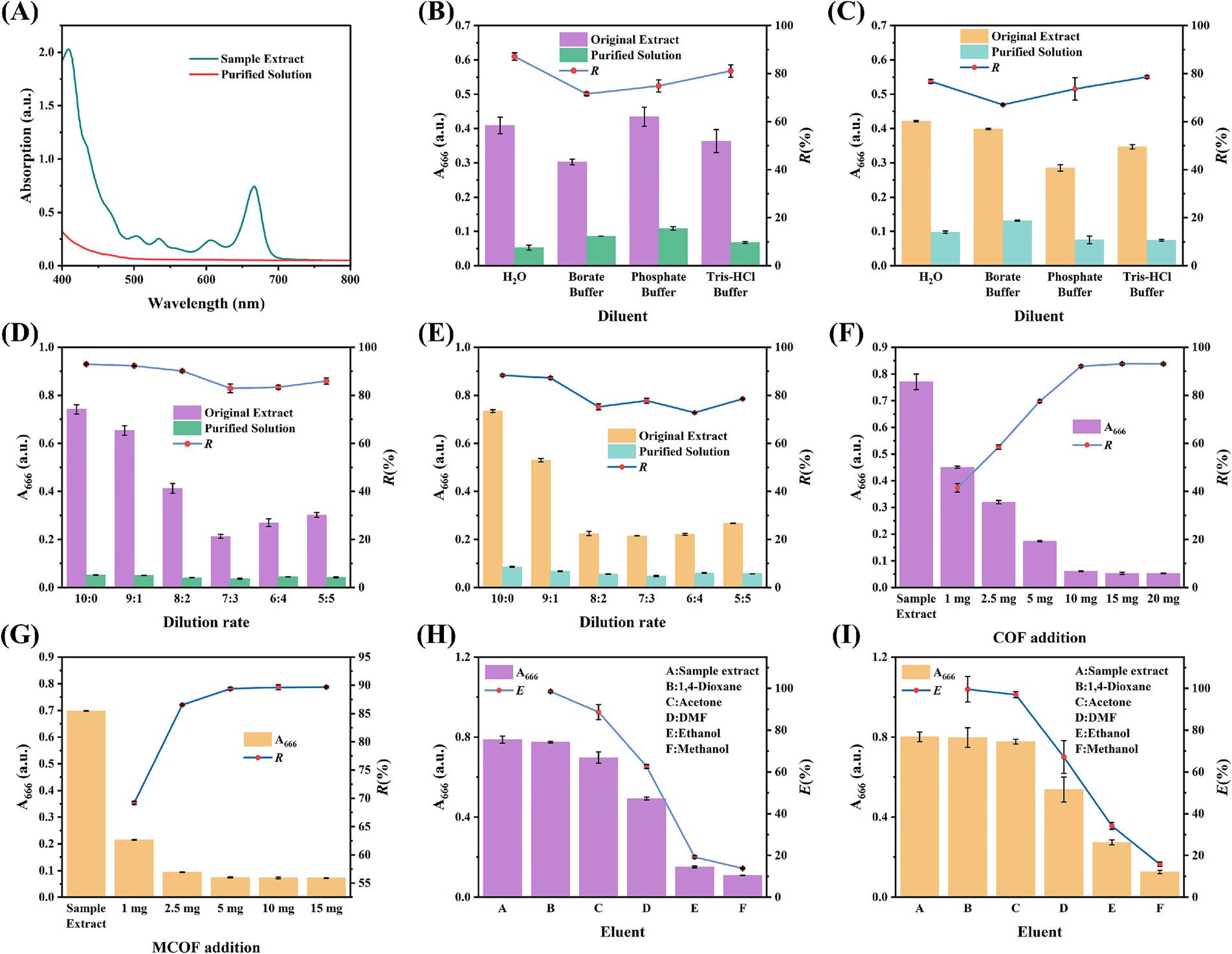
Figure 3. (A) The FT-IR spectrum for amino magnetic beads, COF, and MCOF; (B) the XRD of COD and MCOF; and the N2 adsorption–desorption isotherms of (B) COF and (C) MCOF.
The XRD patterns of COF and MCOF are shown in Figure 3B. Both COF and MCOF showed a strong diffraction peak at 2.22° (2θ), which was assigned to (100) diffractions (Li et al., 2023). Moreover, the broad diffraction band over the range of 15–25° indicates partial crystallinity within the two-dimensional COF and MCOF (Akyuz, 2020; Koonani and Ghiasvand, 2024). For MCOF, the strong diffraction peak at 35.5° and weak diffraction peaks at 30.1°, 43.1°, and 57.0° belonged to (311), (220), (400), and (511) diffractions for Fe3O4, respectively (Sajid et al., 2023). The above results indicated that the crystalline structure of COF and MCOF was well-retained. Moreover, the magnetic property of MCOF was investigated using a vibrating sample magnetometer (VSM). The hysteresis curve (Supplementary Figure S3) showed superparamagnetic behavior, with a coercivity of 36.4 Oe and a magnetization of 11.3 emu/g, indicating that MCOF can be used for efficient magnetic separation.
To further analyze the porosity of COF and MCOF, N2 gas adsorption/desorption isotherm was used. As shown in Figures 3C,D, the adsorption/desorption isotherm exhibited hysteresis loops with characteristic IV-type patterns, indicative of the concurrent presence of both micropores and mesopores within the COF and MCOF (An et al., 2023; Wu et al., 2024). The BJH pore distribution pattern of COF and MCOF (Figures 3C,D inset) revealed that the pore size was approximately 1.59 nm. The BET-specific surface area of COF was 122.0 m2/g. However, due to the core of the amino magnetic bead, the BET-specific surface area of MCOF only reached up to 58.3 m2/g. Hence, COF with high specific surface area and micropores can be effectively used for the absorption of matrix from food samples.
Development of CSPE and MSPE purification
Lettuce was used as a vegetable sample to optimize the parameters for the purification capacity of CSPE and MSPE. The UV–Vis spectrum of the lettuce sample extract exhibited a distinct absorption peak at 666 nm, whereas no prominent peaks were discernible within the range of 500 nm to 800 nm (Figure 4A). Therefore, the characteristic absorption peak at 666 nm was used as an indicator to evaluate the optimization parameters. For MCOF preparation, the usage of amino magnetic beads was optimized. As the amount of amino magnetic beads increased, the R% also increased accordingly (Supplementary Figure S1). Hence, the MCOF prepared using 1 mL of amino magnetic beads was used for subsequent optimization and MSPE development. For the diluent optimization, a parallel trend in R% was observed, and water demonstrated the highest R% for both CSPE and MSPE. Consequently, water was determined to be the optimal diluent (Figures 4B,C). Based on the optimized diluent, the effect of dilution rate on R% was further studied. For CSPE, as the dilution rate increased, the R% showed a decreasing trend, while the highest R% was observed for sample extract without dilution and MSPE (Figures 4D,E). Thus, it was inferred that the sample extract can be directly purified using CSPR or MSPE without any diluent, which is simple, convenient, and low-cost. For MSPE, the vortex time was investigated, and the results indicated that the matrix was removed after 10 s of vertexing, thereby demonstrating the remarkable purification ability of MCOF (Supplementary Figure S2).
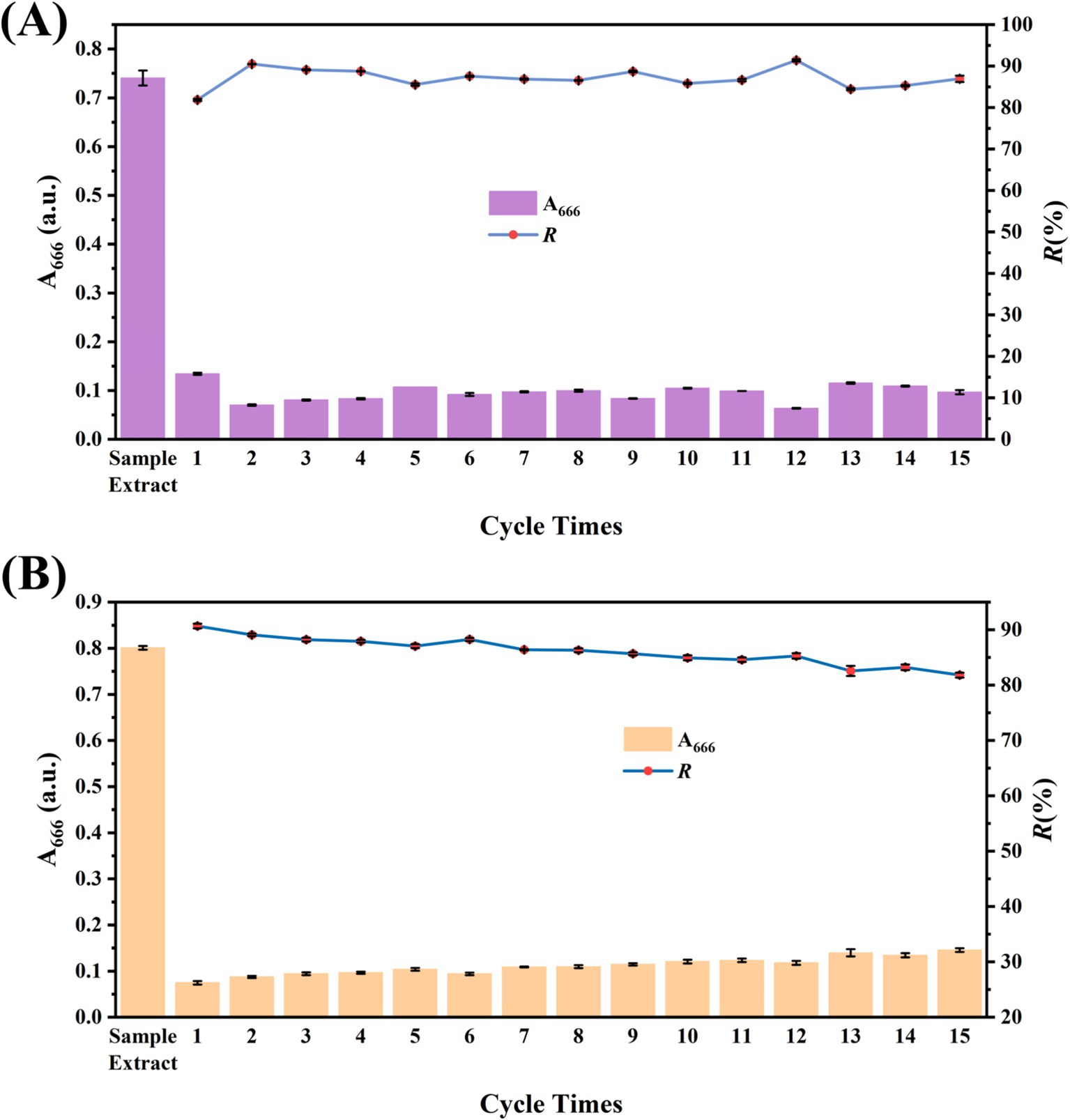
Figure 4. (A) The UV–Vis spectrum of sample extract and purified solution; the diluent optimization for (B) CSPE and (C) MSPE; the dilution rate diluent optimization for (D) CSPE and (E) MSPE; the optimization of COF and MCOF addition for (F) CSPE and (G) MSPE, respectively; and the eluent optimization for (H) CSPE and (I) MSPE.
The addition amounts of COF and MCOF for CSPE and MSPE were further optimized, respectively. As the amount of COF increased, the R% for both CSPE and MSPE also increased (Figures 4F,G). The CSPE and MSPE achieved the highest R% with the addition of 10 mg of COF and 5 mg of MCOF, respectively, which were chosen as the optimal addition amounts. The eluent was further studied to investigate the feasibility of the reuse of COF and MCOF. As shown in Figures 4H,I, 1,4-dioxane exhibited remarkable elution capacity and achieved the highest E%. In contrast, alcohols (ethanol and methanol) showed poor elution ability. Therefore, 1,4-dioxane was chosen as the optimal eluent. Under the optimized conditions, the developed CSPE showed stable purification capacity during 15 reuse cycles, while the purification capacity of MSPE decreased slightly. However, the R% remained above 80% (Figures 5A,B). These results indicated that the synthesized COF and MCOF were suitable materials for low-cost pretreatment of vegetable and fruit samples.
Recovery test
To assess the accuracy of the developed CSPE and MSPE, the recovery test was carried out. Samples (cucumber, Chinese cabbage, green grape, and pomelo) were spiked with 10 pesticides, respectively. Then, they were purified using CSPE and MSPE and analyzed using HPLC-MS/MS. The results are summarized in Tables 1, 2. The recoveries of CSPE and MSPE were 76–92% and 77.3–95.1%, respectively, with the coefficient of variances (CVs) of 1.75–9.55% and 1.63–12.9%, respectively. The results indicated acceptable recoveries, which can fulfill the pesticide maximum residue limits (MRLs) set by the Chinese government (GB 2763–2021, 2021), the European Union (EU Plant Pesticides Database, n.d.), the USA (States Environmental Protection Agency (EPA), n.d.), and the Codex Alimentarius Commission (Codex Alimentarius Commission Pesticide Index, n.d.). These findings demonstrated the accuracy and practicability of the developed CSPE and MSPE. Moreover, the developed MSPE required less usage and more convenient steps compared to CSPE. However, the CSPE showed potential for online HPLC or GC analysis. Therefore, these two formats of purification can both be used for monitoring, depending on the purification step that is required.
Conclusion
This study synthesized a COF and further prepared an MCOF to develop CSPE and MSPE, respectively. The synthesized materials were characterized using SEM and FT-IR analyses. The results indicated the successful synthesis of COF and MCOF. The parameter optimization test suggested that the sample extract can be directly purified by CSPE and MSPE without dilution, which is simple, convenient, and efficient. Furthermore, the synthesized COF and MCOF can be regenerated and reused up to 15 times, which reduces the cost of sample purification. The recovery test showed acceptable recoveries for vegetable and fruit samples, which fulfill the MRLs set by most countries and organizations. In summary, the developed CSPE and MSPE are ideal purification methods and show potential for pesticide analysis in vegetable and fruit samples.
Data availability statement
The original contributions presented in the study are included in the article/Supplementary material, further inquiries can be directed to the corresponding authors.
Author contributions
A-JH: Writing – original draft. X-XD: Data curation, Methodology, Writing – review & editing. ST: Data curation, Methodology, Writing – original draft. KC: Methodology, Writing – original draft. MZ: Methodology, Writing – original draft. BL: Methodology, Writing – original draft. HD: Methodology, Project administration, Writing – original draft. FH: Methodology, Project administration, Writing – review & editing. HN: Methodology, Project administration, Writing – review & editing. HW: Supervision, Writing – review & editing. Z-JC: Supervision, Writing – review & editing.
Funding
The author(s) declare that financial support was received for the research, authorship, and/or publication of this article. This study was supported by the 2023 Natural Science Youth Project of Zhaoqing University (QN202335), the Guangdong Basic and Applied Basic Research Foundation (2021A1515110513), the Science Project of the Department of Education of Guangdong Province (2020ZDZX2045), the Key Laboratory of Tropical Fruit and Vegetable Cold-Chain of Hainan Province (KF202402), the Fujian Provincial Key Laboratory of Food Microbiology and Enzyme Engineering (Z823280-10), the Priority Academic Program Development of Jiangsu Higher Education Institutions (No. PAPD-2023-87), and the Science and Technology Innovation Project of Zhaoqing City (2023040308004).
Conflict of interest
The authors declare that the research was conducted in the absence of any commercial or financial relationships that could be construed as a potential conflict of interest.
The author(s) declared that they were an editorial board member of Frontiers, at the time of submission. This had no impact on the peer review process and the final decision.
Publisher’s note
All claims expressed in this article are solely those of the authors and do not necessarily represent those of their affiliated organizations, or those of the publisher, the editors and the reviewers. Any product that may be evaluated in this article, or claim that may be made by its manufacturer, is not guaranteed or endorsed by the publisher.
Supplementary material
The Supplementary material for this article can be found online at: https://www.frontiersin.org/articles/10.3389/fsufs.2024.1472174/full#supplementary-material
References
Abdulhussein, A. Q., Jamil, A. K. M., and Bakar, N. K. A. (2021). Magnetic molecularly imprinted polymer nanoparticles for the extraction and clean-up of thiamethoxam and thiacloprid in light and dark honey. Food Chem. 359:129936. doi: 10.1016/j.foodchem.2021.129936
Akyuz, L. (2020). An imine based COF as a smart carrier for targeted drug delivery: from synthesis to computational studies. Micropor. Mesopor. Mat. 294:109850. doi: 10.1016/j.micromeso.2019.109850
An, Y. F., Zhang, H., Geng, D. X., Fu, Z. J., Liu, Z. M., He, J., et al. (2023). Double redox-active polyimide-based covalent organic framework induced by lithium ion for boosting high-performance aqueous Zn2+ storage. Chem. Eng. J. 477:147275. doi: 10.1016/j.cej.2023.147275
Chen, Z. J., Huang, A. J., Dong, X. X., Zhang, Y. F., Zhu, L., Luo, L., et al. (2023). A simple and sensitive fluoroimmunoassay based on the nanobody-alkaline phosphatase fusion protein for the rapid detection of fenitrothion. Front. Sustainable Food Syst. 7:1320931. doi: 10.3389/fsufs.2023.1320931
Chen, Z. Y., Sun, Y., Shi, J. Y., Zhang, W., Zhang, X. N., Hang, X. W., et al. (2023). Convenient self-assembled PDADMAC/PSS/au@ag NRs filter paper for swift SERS evaluate of non-systemic pesticides on fruit and vegetable surfaces. Food Chem. 424:136232. doi: 10.1016/j.foodchem.2023.136232
Codex Alimentarius Commission Pesticide Index. (n.d.) Pesticide Index. Available online at: http://www.fao.org/fao-who-codexalimentarius/codex-texts/dbs/pestres/pesticides/en/ (Accessed October 21, 2024).
Dai, Y., Xu, W., Wen, X. Y., Fan, H. Z., Zhang, Q., Zhang, J., et al. (2024). Smartphone-assisted hydrogel platform based on BSA-CeO2 nanoclusters for dual-mode determination of acetylcholinesterase and organophosphorus pesticides. Microchim. Acta 191:185. doi: 10.1007/s00604-024-06268-6
Ding, L. H., Guo, J. W., Chen, S., and Wang, Y. W. (2024). Electrochemical sensing mechanisms of neonicotinoid pesticides and recent progress in utilizing functional materials for electrochemical detection platforms. Talanta 273:125937. doi: 10.1016/j.talanta.2024.125937
Dong, M., Pan, Q. Q., Meng, F. F., Yao, X. H., You, S. Q., Shan, G. G., et al. (2024). Trinuclear cu-based covalent organic framework: π-conjugated framework regulating electron delocalization to promote photoreduction CO2. J. Colloid Interf. Sci. 662, 807–813. doi: 10.1016/j.jcis.2024.02.129
Dong, Y. B., Yao, X. L., Zhang, W. P., and Wu, X. M. (2023). Development of simultaneous determination method of pesticide high toxic metabolite 3,4-Dichloroaniline and 3,5 Dichloroaniline in chives using HPLC-MS/MS. Food Secur. 12:2875. doi: 10.3390/foods12152875
EU Plant Pesticides Database. (n.d.) Pesticide Residues. Available online at: https://ec.europa.eu/food/plant/pesticides/eu-pesticides-database/start/screen/mrls (Accessed October 21, 2024).
GB 2763–2021. (2021). China national food safety standard—maximum residue limits for pesticides in food.
Guo, M. W., Pang, J. R., Wang, Y., Bi, C. H., Xu, Z. L., Shen, Y. D., et al. (2024). Nanobodies-based colloidal gold immunochromatographic assay for specific detection of parathion. Anal. Chim. Acta 1310:342717. doi: 10.1016/j.aca.2024.342717
Harischandra, N. R., Pallavi, M. S., Bheemanna, M., PavanKumar, K., Chandra Sekhara Reddy, V., Udaykumar, N. R., et al. (2021). Simultaneous determination of 79 pesticides in pigeonpea grains using GC-MS/MS and LC-MS/MS. Food Chem. 347:128986. doi: 10.1016/j.foodchem.2020.128986
Koonani, S., and Ghiasvand, A. (2024). A highly porous fiber coating based on a Zn-MOF/COF hybrid material for solid-phase microextraction of PAHs in soil. Talanta 267:125236. doi: 10.1016/j.talanta.2023.125236
Li, H., Hassan, M. M., He, Z., Haruna, S. A., Chen, Q., and Ding, Z. (2022). A sensitive silver nanoflower-based SERS sensor coupled novel chemometric models for simultaneous detection of chlorpyrifos and carbendazim in food. LWT-Food Sci. Technol. 167:113804. doi: 10.1016/j.lwt.2022.113804
Li, G., and Row, K. H. (2018). Ternary deep eutectic solvent magnetic molecularly imprinted polymers for the dispersive magnetic solid-phase microextraction of green tea. J. Sep. Sci. 41, 3424–3431. doi: 10.1002/jssc.201800222
Li, W., Wang, R., Jiang, H. X., Chen, Y., Tang, A. N., and Kong, D. M. (2022). Controllable synthesis of uniform large-sized spherical covalent organic frameworks for facile sample pretreatment and as naked-eye indicator. Talanta 236:122829. doi: 10.1016/j.talanta.2021.122829
Li, W., Wang, X. H., Liu, J. Q., Jiang, H. X., Cao, D. X., Tang, A. N., et al. (2023). Efficient food safety analysis for vegetables by a heteropore covalent organic framework derived silicone tube with flow-through purification. Talanta 265:124880. doi: 10.1016/j.talanta.2023.124880
Lin, X. P., Wang, X. Q., Wang, J., Yuan, Y. W., Di, S. S., Wang, Z. W., et al. (2020). Magnetic covalent organic framework as a solid-phase extraction absorbent for sensitive determination of trace organophosphorus pesticides in fatty milk. J. Chromatogr. A 1627:461387. doi: 10.1016/j.chroma.2020.461387
Liu, J. L., Lin, M. X., Huang, J., Zhang, C. R., Qi, J. X., Cai, Y. J., et al. (2024). sp2-c linked cu-based metal-covalent organic framework for chemical and photocatalysis synergistic reduction of uranium. Chem. Eng. J. 491:151982. doi: 10.1016/j.cej.2024.151982
Liu, G. Y., Zhang, X., Lu, M., Tian, M. S., Liu, Y., Wang, J., et al. (2022). Adsorption and removal of organophosphorus pesticides from Chinese cabbages and green onions by using metal organic frameworks based on the mussel-inspired adhesive interface. Food Chem. 393:133337. doi: 10.1016/j.foodchem.2022.133337
Ma, L., Han, E., Yin, L., Xu, Q., Zou, C., Bai, J., et al. (2023). Simultaneous detection of mixed pesticide residues based on portable Raman spectrometer and au@ag nanoparticles SERS substrate. Food Control 153:109951. doi: 10.1016/j.foodcont.2023.109951
Meng, Z., Cui, J., Li, R., Sun, W., Bao, X., Wang, J., et al. (2022). Systematic evaluation of chiral pesticides at the enantiomeric level: a new strategy for the development of highly effective and less harmful pesticides. Sci. Total Environ. 846:157294. doi: 10.1016/j.scitotenv.2022.157294
Pan, Y. C., Liu, X., Liu, J., Wang, J. P., Liu, J. X., Gao, Y. X., et al. (2022). Determination of organophosphorus in dairy products by graphitic carbon nitride combined molecularly imprinted microspheres with ultra performance liquid chromatography. Food Chem X 15:100424. doi: 10.1016/j.fochx.2022.100424
Pedroso, T. M. A., Benvindo-Souza, M., de Araújo Nascimento, F., Woch, J., Dos Reis, F. G., de Melo, E., et al. (2022). Cancer and occupational exposure to pesticides: a bibliometric study of the past 10 years. Environ. Sci. Pollut. R. 29, 17464–17475. doi: 10.1007/s11356-021-17031-2
Sajid, M., Shuja, S., Rong, H., and Zhang, J. (2023). Size-controlled synthesis of Fe3O4 and Fe3O4@SiO2 nanoparticles and their superparamagnetic properties tailoring. Progress in Natural Science: Materials International 33, 116–119. doi: 10.1016/j.pnsc.2022.08.003
States Environmental Protection Agency (EPA). (n.d.) Code of Federal Regulations (40 CFR Part 180). Available online at: https://www.govinfo.gov/content/pkg/CFR-2014-title40-vol24/xml/CFR-2014-title40-vol24-part180.xml
Wang, X. H., Li, W., Jiang, H. X., Chen, Y., Gao, R. Z., Tang, A. N., et al. (2021). Heteropore covalent organic framework-based composite membrane prepared by in situ growth on non-woven fabric for sample pretreatment of food non-targeted analysis. Microchim. Acta 188:235. doi: 10.1007/s00604-021-04889-9
Wang, S. L., Liang, N. N., Hu, X. T., Li, W. T., Guo, Z. A., Zhang, X. A., et al. (2024). Carbon dots and covalent organic frameworks based FRET immunosensor for sensitive detection of Escherichia coli O157:H7. Food Chem. 447:138663. doi: 10.1016/j.foodchem.2024.138663
Wu, S. P., Shi, W. J., Cui, L. J., and Xu, C. (2024). Enhancing contaminant rejection efficiency with ZIF-8 molecular sieving in sustainable mixed matrix membranes. Chem. Eng. J. 482:148954. doi: 10.1016/j.cej.2024.148954
Yang, N., Wang, P., Xue, C. Y., Sun, J., Mao, H. P., and Oppong, P. K. (2018). A portable detection method for organophosphorus and carbamates pesticide residues based on multilayer paper chip. J. Food Process Eng. 41:e12867. doi: 10.1111/jfpe.12867
Zhang, X., Zhou, Y., Huang, X., Hu, X., Huang, X., Yin, L., et al. (2023). Switchable aptamer-fueled colorimetric sensing toward agricultural fipronil exposure sensitized with affiliative metal-organic framework. Food Chem. 407:135115. doi: 10.1016/j.foodchem.2022.135115
Keywords: pesticide, covalent organic framework, pretreatment, solid phase extraction, magnetic solid-phase extraction
Citation: Huang A-J, Dong X-X, Tan S, Chen K, Zhang M, Li B, Deng H, He F, Ni H, Wang H and Chen Z-J (2024) A covalent organic framework-derived pretreatment for pesticides in vegetables and fruits. Front. Sustain. Food Syst. 8:1472174. doi: 10.3389/fsufs.2024.1472174
Edited by:
Xiao-Hua Zhang, Hunan Institute of Science and Technology, ChinaReviewed by:
Rui Chen, Chinese Academy of Agricultural Sciences (CAAS), ChinaXiao-Li Yin, Yangtze University, China
Copyright © 2024 Huang, Dong, Tan, Chen, Zhang, Li, Deng, He, Ni, Wang and Chen. This is an open-access article distributed under the terms of the Creative Commons Attribution License (CC BY). The use, distribution or reproduction in other forums is permitted, provided the original author(s) and the copyright owner(s) are credited and that the original publication in this journal is cited, in accordance with accepted academic practice. No use, distribution or reproduction is permitted which does not comply with these terms.
*Correspondence: Hongwu Wang, aHd3YW5nQHpxdS5lZHUuY24=; Zi-Jian Chen, Y2hlbnppamlhbkB6cXUuZWR1LmNu