- 1Department of Food Science, Faculty of Life Sciences, Government College University, Faisalabad, Punjab, Pakistan
- 2Faculty of Natural and Applied Sciences, Institute of Food Science and Technology, Khwaja Fareed University of Engineering and Information Technology, Rahim Yar Khan, Pakistan
- 3University Institute of Food Science and Technology, The University of Lahore, Lahore, Pakistan
- 4College of Food Engineering and Nutritional Science, Shaanxi Normal University, Xi'an, China
- 5Department of Food Science & Nutrition, College of Food and Agricultural Sciences, King Saud University, Riyadh, Saudi Arabia
The demand for clean-label products continues to rise, as consumers increasingly prioritize natural and transparent ingredient lists. Natural substances are generally deemed safe for consumption by consumers. This study was focused on the development of clean-label ostrich meat patties with Chenopodium album extract and their storage stability. To prepare C. album extract, microwave-assisted extraction (MAE) and ultrasound-assisted extraction (UAE) were employed. Ostrich meat patties were prepared using diverse combinations of extract, including 1% UAE, 2% UAE, 1% MAE, 2% MAE, 0.5% UAE+0.5% MAE, and 1% UAE + 1% MAE. The highest pH was observed for MAE in T3 on the 14th day (6.19 ± 0.03). The L* value was observed between 39.12 ± 1.09 and 44.00 ± 1.1. As storage intervals passed, the a* and b* values of ostrich meat patties decreased. After the 14th day of storage, the best results were obtained from 2% UAE (T2), with the lowest TBRAS, Peroxide value (POV), and Total of volatile basic nitrogen (TVBN) readings recorded for T2 (0.74 ± 0.02 MDA/kg, 0.56 ± 0.01 meq peroxide/kg, and 6.28 ± 0.40 mg/100 mL, respectively). At the end of the storage study, the lowest Total Microbial Count (TMC) and coliform count were recorded for T2 (8.08 ± 0.03 and 4.97 ± 0.05 cfu/mL, respectively). At the end of the storage study, T2 exhibited the highest values for total phenolic content (TPC), diphenyl-1-picrylhydrazyl (DPPH), Ferric reducing antioxidant power (FRAP), and azino-bis-3-ethylbenzothiazoline-6-sulfonic acid (ABTS) assay, namely of T2 122 ± 0.28 mg GAE/100 g, 73 ± 0.27%, 5.9 ± 0.01 mmol FSE/100 g, and 83 ± 0.08 mmol/L, respectively. The current study concludes that the UAE extract of C. album incorporated into ostrich meat patties manifests improved safety, quality, and storage stability. The implementation of clean label strategies can facilitate food manufacturers to align with consumer preferences for product transparency and sustainability while ensuring product safety and quality.
Introduction
Modern human life has created a generation of fast-food eaters with terrible habits and unhealthy lifestyles. Due to poor nutrition and insufficient exercise, cardiovascular disease and cancer are becoming more common today and account for a significant portion of all deaths. People seek to eat high-biological value meat with a greater protein proportion and lower cholesterol and fat proportion, considering the trend toward making “healthy food” and leading a healthy lifestyle (Gastaldello et al., 2022). The ostrich flesh is the best remedy. The United States of America, Canada, Israel, Australia, China, and France have developed ostrich farming, but the extreme practice of raising these birds has not yet been achieved (Awada et al., 2021).
Lean meat makes up around 62.5% of the ostrich carcass, which is equal to the proportion of beef and chicken meat. In recent years, global-scale demand for ostrich meat has been consciously rising (Brassó et al., 2021). Because it is acknowledged as a dietetic food with low-fat levels (less than 2 mg/100 g), a reduced calorific value of 390 kJ/100 g compared to beef (517 kJ/100 g), and a significant percentage of polyunsaturated fatty acids (PUFA), ostrich meat is quite popular. For instance, the PUFA content of ostrich meat (24–38% total fatty acids) is higher than that of chicken meat (19%) and beef (4.8% total fatty acids) (Horbańczuk et al., 2019). Ostrich meat has become more popular in this context as a result of its outstanding leanness and advantageous nutritional structure (Manap and Serikkyzy, 2023).
There has been extensive research into new ingredients and processing techniques to meet the increasing demand for wholesome, clean-label, and sustainable food products in the global food industry (Kainat et al., 2022). Consumers increasingly prefer clean-label products, which feature natural, recognizable ingredients without synthetic additives or preservatives (Galanakis, 2022). Ostrich meat and Chenopodium album extracts coming together presents an intriguing path toward the development of new, healthy meat products. The commercialization of clean-label products has potential, but this field is still in the phase of infancy and requires research-based evidential support to fill in numerous gaps in the required information.
The term “Chenopodium” originated from the Greek words “Khen,” which means goose, and “Pous,” which means foot, because of the shape of the leaves of this plant. Goosefoot has 21 species widely spread throughout North America, Asia, Europe, and Africa. This plant requires nitrogen-rich soil for its growth (Arora et al., 2019). C. album leaves contain 0.94% total phenolic content (TPC) and 0.27% total flavonoid content (TFC) (Poonia, 2020).
It is essential to investigate and implement advanced extraction techniques to meet the high-performance requirements of commercial systems. There exist a number of extraction techniques, including ultrasound-, microwave-, supercritical fluid-, subcritical water-, enzyme-, and pulsed electric field-assisted extraction. Modern non-thermal food processing technologies, such as ultrasound, have gained popularity as alternatives to traditional processing methods due to their comparatively high efficacy, cheap production costs, and environmental friendliness (Chacha et al., 2021).
Ultrasound waves are high-frequency (>20 kHz) waves that are audible to humans, but not to other creatures. Modern food analysis places a greater emphasis on ultrasounds (US) because of how extensively they have been used in solid and liquid food media for centuries (Gouda et al., 2021). Considerable focus has been devoted to the utilization of Ultrasonic-Assisted Extraction (UAE) in procuring bioactive components from food sources, which typically require extended extraction durations when using established techniques (Yildiz et al., 2020). The use of microwaves for extracting phytoconstituents from complex herbal samples is the outcome of extensive research. The application of microwave energy effectively raises the temperature of the moisture within the innermost plant cells, causing it to evaporate and create internal pressure against the cell wall. The pressure generated within the cell weakens the cell wall, causing it to rupture. As a result, valuable components of the cell are released, enhancing the extraction efficiency and yield of phytoconstituents.
The present study was focused on the development and comparative assessment of clean-label ostrich meat patties with C. album extract, which was prepared using MAE and UAE. To confirm the storage stability of the product, a 14-day study was conducted, which included physicochemical analysis (pH and Hunter’s color), quality and stability tests (Thiobarbituric acid reactive substances value [TBARS], POV, TVBN), antioxidant potential (TPC, DPPH, ABTS, FRAP), and microbial test (Total Microbial Count [TMC], coliform count), and a sensory study was conducted at 0, 7, and 14 days of storage. This study will provide scientific evidential support for the future commercial use of C. album extract to produce healthy drinks and functional foods. However, the ethnomedicinal potential of the plant still needs to be explored to develop nutraceutical foods.
Materials and methods
Raw material procurement
The study was conducted by the Department of Food Science at Government College University Faisalabad (GCUF). The sample of ostrich meat was purchased from the Signature Meat Shop in Lahore, Pakistan, and the sample of C. album was procured from the local market in Faisalabad, Pakistan. All of the reagents and chemicals used in the study were sourced from Sigma Aldrich (Tokyo, Japan).
Preparation of Chenopodium album extract
Ultrasonic-assisted extraction
The extraction method for obtaining bioactive compounds from C. album, as outlined by Bimakr et al. (2017), involved the utilization of ultrasonic assistance. For extraction, a binary solvent containing 70% ethanol and 30% distilled water was used. To initiate the process, 10 grams of C. album powder was dissolved in 30 mL of the solvent, forming a solution. The extraction was carried out using a sonication apparatus (Model VCX 750, manufactured by Sonic and Materials, Inc., Newtown, CT, United States) at a temperature of 40°C, with an amplitude range of 70 to 90% and a sonication duration of 20 min. Subsequently, the solution was cooled at room temperature (25 ± 2°C) for a period of 20 min. The cooled solution underwent centrifugation at a low temperature of 10°C and a speed of 3,000 rpm. After centrifugation, the supernatants were collected and transferred to a vacuum rotary evaporator (STRIKE 100, WIGGENS CHINA) where the solvent was evaporated at a temperature of 50°C and 150 rpm. For further testing, extracts were freeze-dried and stored at 4°C in glass containers.
Microwave assisted extraction
The MAE of bioactive compounds from C. album was performed according to the protocol followed by Khan et al. (2021). A binary solvent comprising 70% ethanol and 30% distilled water was used for the preparation of plant leaf extract. 10 grams of powder sample of C. album leaves was added into the 30 mL of solvent for making a solution. The extraction was performed using the microwave apparatus HDG 236S (Homage, Korea) with an operating power of 80w. The sample was placed in a microwave chamber for 15 min to extract bioactive components. The slurry obtained from the extraction was filtered by using a simple filter assembly of Whatman No.1 filter paper. From the filtered sample, the solvent was evaporated at 50°C and 150 rpm. Samples were then frozen and stored at 4°C in glass jars.
Analysis design and treatments
A variety of treatments were created from the obtained meat samples. The C. album extract (MAE & UAE) in varying concentrations with the meat (Table 1). The treatment plan for ostrich meat patties treated with C. album extract is as mentioned below:
The treated meat was then used to prepare meat patties. The C. album extract powder was mixed into the ground ostrich meat as per the treatment plan mentioned above. The mixture of each treatment was then molded into patties of 30 g weight and 1.5 cm thickness. Control (T0) was prepared solely with 30 g of ostrich meat. T1 contained 0.3 g of UAE C. album extract and 29.7 g ostrich meat, T2 contained 0.6 g of UAE C. album extract and 29.4 g ostrich meat, T3 contained 0.3 g of MAE C. album extract and 29.7 g ostrich meat, and T4 contained 0.6 g of UAE C. album extract and 29.4 g ostrich meat. T5 and T6 contained C. album extract prepared using both UAE and MAE. T5 was prepared using 0.15 g UAE extract, 0.15 g MAE extract, and 29.7 g ostrich meat, whereas T6 was prepared using 0.3 g UAE extract, 0.3 g MAE extract, and 29.4 g ostrich meat (Bouarab-Chibane et al., 2017). Prepared patties were then individually placed into a polystyrene tray having dimensions 12 × 21 × 8 cm. The value-added product was then stored at 4°C for 2 weeks storage study. The proximate stability, physicochemical analysis, quality, and stability test, microbial quality, and sensory evaluation of all the samples were performed at different storage intervals, i.e., 0, 7, and 14 days.
Physicochemical analysis
pH
To determine the pH of the sample, a homogenate of the meat patties was prepared by mixing them with distilled water (1:10). A digital pH meter (Model PH-25; WANT Balance Instrument Co., Ltd., Changzhou, Jiangsu, China) was used for the test. The pH meter was calibrated with standard pH buffer solutions (pH 4.01, 7.00, and 10.01) at a temperature of 25°C. The calibration ensured the accuracy of the pH meter. A total of three readings were recorded and the mean values were considered as the final pH reading.
Hunter’s color
According to the methodology adapted by Khalid et al. (2021), surface shading estimation of the meat patties was carried out using a Hunter’s colorimeter. This refers to a white alignment plate with the coordinates L* = 89.2, a* = 0.921, and b* = 0.783. To obtain the shading Commission Internationale de l’Éclairage (CIE) L* “lightness,” CIE a* “redness,” and CIE b* “yellowness” values. Nine random readings on each sample surface were taken to increase the accuracy of the statistical results.
Stability and quality tests
Thiobarbituric acid reactive substances value
The extent of oxidation of lipids in the sample was determined using the 2-Tertiary butyl alcohol (TBA) reactive substances method, as defined by Ahn et al. (2000), with minor alterations. Initially, 5 grams of the sample remained assorted with 15 mL of deionized distilled water. The mixture was then homogenized for 30 s. To achieve this, 50 μL of butylated hydroxytoluene (7.2% ethanol) and 1 mL of the homogenized sample were added to a disposable test tube.
In a separate tube, 2 mL of a solution containing 20 mM 2-thiobarbituric acid (TAB) and trichloroacetic acid (TCA) at 15% concentration was prepared. This solution was then added to the test tube containing the sample and mixed thoroughly using vortexing. Then the sample was placed in a water bath for 15 min. The incubated sample was then cooled to room temperature by placing it in a cold-water bath for 10 min. The sample was then centrifuged for 15 min at 2500 rpm and the temperature was maintained at 4°C. The spectrophotometeric absorbance was recorded at 532 nm wavelength against the blank solution. The blank solution was prepared using a 1 mL TAB/TCA solution without the addition of a sample to it. The malonaldehyde concentration was determined using the formula mentioned below:
Peroxide value
The peroxide value (POV) of ostrich meat patties was determined using the method designed by the International Dairy Federation (IDF) and followed by Bashir et al. (2022) with minor modifications. 0.4 g of dehydrated barium chloride was added to 50 mL of water. The iron sulfate solution was prepared using 50 mL of water and FeSO4 7H2O (0.5 g). The iron chloride solution was mixed with the iron sulfate solution with continuous stirring. A total of 10 N of 2 mL HCl was added to the final solution. The filter was used to remove the precipitates. Barium sulfate from the mixture produced a clear iron (II) solution. 30 g of ammonium thiocyanate was mixed with water to reach a 100 mL volume. The 0.2 g sample was mixed in 40 mM, pH 6.8 cold phosphate (9.8 mL) buffer. For homogenization, the sample was kept in a homogenizer (Model D-500 Pro; WIGGENS, CHINA) (at 13000 rpm for 10 s). The sample was mixed with 7:3, V/V chloroform-methanol (9.8 mL), and kept in a vortex mixer for 4 h. After that, the solution was prepared with 50 μL of ammonium thiocyanate and a sample. The mixture then was vortexed for 4 s. 50 μL of iron (III) was added to the solution and mixed again on the vortex mixer for 4 s. The sample was incubated at room temperature for 5 min. The absorbance of the spectrophotometer (Model Biolab 310; Biolab Scientific Ltd., Canada) was recorded at 500 nm for both blank and sample readings. The peroxide value was calculated using the formula mentioned below:
Where,
As = absorbance of sample.
Ab = absorbance of blank.
m = slope of standard curve.
m0 = mass in grams of the sample.
55.84 = atomic weight of iron.
Total of volatile basic nitrogen
The total of volatile basic nitrogen (TVBN) was determined using the protocol defined by Qiao et al. (2017). A total of 5 g of ostrich meat patties samples were homogenized with 45 mL of distilled water for 30 s using a homogenizer (D-500 Pro, WIGGENS, China). The homogenized solution was then mixed with 10% trichloroacetic acid (TCA) (5 mL) in an equal volume. The TVBN value was calculated on the basis of the TCA extract obtained. The inner well of the Conway unit was filled with 1 mL TVBN reagent, while the outer well was filled with 1 mL TCA and sample, along with a 1 mL potassium carbonate solution. The Conway unit was tightly locked with the help of a ground glass plate to create an airtight seal. The assembly was then rotated clockwise and anticlockwise, followed by 3 h of incubation at room temperature. During incubation, the blue color was changed to pink. After the color transition, the TVBN reagent in the inner well was reverse-titrated against 0.02 N sulfuric acid. The TVBN value was then calculated with the formula mentioned below:
Where,
a = is ml of sulfuric acid.
b = molarity of sulfuric acid.
Antioxidant potential
Total phenolic content
The TPC of ostrich meat patties was determined by following the method designed by Aslam et al. (2020) using the Folin–Ciocalteu reagent. A 0.5 mL sample aliquot was mixed with 1 mL of Folin–Ciocalteu (10%). The sample was then incubated in the ark for 6 min. After the incubation period, 2 mL Na2CO3 (20%) was added to the mixture. The gallic acid standard solution was utilized to generate a calibration curve. The TPC was calculated as 1 g of gallic acid per 1 g of sample. Absorbance was determined at 765 nm against a blank sample.
2,2-diphenyl-1-picrylhydrazyl free radical scavenging activity
The DPPH value of meat patties was assessed following the method followed by Kang (2016) with slight modifications. 1 mg of the sample was thoroughly mixed with 0.0012 M DPPH. The mixture was then homogenized and the supernatant was collected. The collected sample was then incubated for 60 min at room temperature in a dark area. The spectrophotometric absorbance was then recorded at 517 nm wavelength using a spectrophotometer.
To calculate the percentage (%) of free radical inhibition by DPPH (2,2-diphenyl-1-picrylhydrazyl), the following equation can be used:
Ferric reducing antioxidant power assay
A FRAP assay was performed to calculate the ferric-reducing potential by following the protocol designed by Benzie and Strain (1996). 2,4,6-tripyridyl-s-triazine solution (10 mM), 1 volume of FeCl3, and 10 volumes of acetate buffer hexahydrate (300 mM, pH 3.6). Spectrophotometric absorbance was measured at 593 nm after 10 min of incubation at 25°C using 20 mL of sample mixture added to a fresh 1.5 mL FRAP reagent.
Where,
Abs = the solvent of the extract.
b = is the coefficient of the FSE.
2,2′-azino-bis-3-ethylbenzothiazoline-6-sulfonic acid assay
A 5 mmol of pure ABTS was dissolved in 5 mM of phosphate-buffered saline with a pH of 7.4 to create a final solution. The fluid was filtered by passing through the activated Manganese oxide (MnO) using Whatman No.1 filter paper. To facilitate an effective radical generation, the filtered solution was given a 12 h dark period. Then, 0.2 mm syringe filters were used to filter the incubated solution, which was then stored in a light-protected area. The average of two readings of blank samples was taken to generate a standard calibration curve. For each run, 50, 100, 150, 200, and 250 mmol/L of the standard antioxidant Trolox was used. In the 96-well microplate with 190 mL of ABTS and 10 mL of the prediluted sample, the absorbance was measured at 620 nm following the inhibition period of 20 min (Salman et al., 2013).
Microbial quality
The microbial quality of the ostrich meat patties was tested on the basis of total microbial and total coliform count following the protocols outlined by AOAC (2005).
Sample preparation
A 10 g ostrich meat patties from each treatment were collected under aseptic conditions within a stomacher pouch. A BagMixer 400 CC stomacher (Interscience, France) was used to homogenize the samples for 90 s. 90 mL of 0.85% saline solution (NaCl) was added to the stomacher. Serial dilutions of each homogenate were prepared, and each test was conducted in triplicate (Turan and Şimşek, 2021).
Total microbial count
In a clean and sterile petri dish, 1 mL sample from each dilution was pipetted. The nutrient agar medium (Merck, Darmstadt, Germany) was cooled to 45 ± 1°C, followed by the addition of 15 mL of media to each petri dish. Petri dishes were rotated both clockwise and anticlockwise to ensure uniform mixing of samples. They were left for solidification at room temperature. The petri dishes were then incubated at 35 ± 2°C for 24 h. After incubation, the TMC count was determined from the plates with pinpoint-sized colonies ranging from 25 to 250 colonies per dish (Tang et al., 2020).
Total coliforms count
From each dilution, 1 mL of sample was pipetted into sterile petri dishes using the pour plate method. After solidification plates were incubated at 35 ± 2°C for 24 h. After incubation, the total coliform count was determined from the plates, with pinpoint-sized colonies ranging from 25 to 250 colonies per dish (Shakhes et al., 2023).
Sensory evaluation
A trained panel of evaluators conducted a sensory assessment of all the treatments of ostrich meat patties treated with C. album, using a nine-point hedonic scale, following the recommended procedures outlined by Meilgaard et al. (2007). The scale ranged from 1 to 9, wherein 9 indicated “extremely liked” and 1 indicated “extremely disliked.” To ensure fair and impartial evaluations, all samples were coded. A total of 10 panelists were selected for their expertise in sensory evaluation, and they took part in the study.
Statistical analyses
The statistical package Statplus was used for analyzing the collected data for various parameters. An analysis of variance (ANOVA) was implemented to determine the level of significance (p ≤ 0.05) as per the method described by Steel and Torrie (2012). The least significant difference (LSD) test was used to compare the means. All parameters were repeated three times except for the sensory evaluation and Hunter’s color. Nine random readings were taken in Hunter’s color, and 10 panelists were selected for sensory evaluation.
Results
C. album is a rich source of antioxidants and antimicrobial compounds. There were two extraction techniques (MAE and UAE) for the extraction of bioactive compounds from C. album. After that the extract was added to ostrich meat and meat patties were prepared to check the effect of the extract on the meat patties. Tests were done at different storage intervals, namely 0, 7, and 14 days for measuring the antioxidant potential, physicochemical, and stability of meat patties.
Physicochemical analysis
pH
The pH of the meat patties ranged from 5.89 ± 0.02 to 6.22 ± 0.05 as shown in Figure 1. The value of the T6 sample was the lowest, while the value of the controlled sample was the highest. On day 1st day of storage, a minimal pH reading was observed. Our results exhibited that the pH reading of meat patties samples increased during the 14-day storage period. The lowest pH value of treated ostrich meat patties was found in the T6 pH values. Our research revealed that the pH value dropped significantly (p ≤ 0.05) as the C. album content was increased in ostrich meat patties. The decrease in pH was because of bioactive components present in C. album, i.e., protochachuic acid, gallic acid, and m-coumaric acid (Chamkhi et al., 2022). A similar study was conducted by Khan et al. (2020) on goat meat patties with Morifolium flower extract and observed a marked reduction in pH with an increase in plant extract concentration.
The lowest pH was observed in T2 (UAE 02% extract) on the 7th day, i.e., 5.88 ± 0.03. However, the highest pH was observed in T3 (MAE 01% extract) on the 14th day (6.19 ± 0.03). The trend observed for pH among the techniques was MAE > UAE. According to Glamoclija et al. (2015), a high pH value of meat is generally considered good, especially when it comes to the quality and tenderness of the meat.
Comparing the techniques, the highest results were obtained by (0.5% + 0.5) MAE and UAE in T5 on the 14th day, i.e., 6.18 ± 0.04. The lowest pH was recorded for (1% + 1) MAE and UAE in T6 at 0 days, i.e., 5.89 ± 0.02.
Hunter’s color
L* value
The L* (Lightness) value of ostrich meat patties varied from 39.12 ± 1.09 to 44.00 ± 1.11. The smallest L* value was determined after 0 days of storage. The highest L* value was established on the 14th day of storage. The control sample (T0) had the highest L* value and the lowest L* value of C. album-treated meat. As shown in Table 2, the L* value rises as the concentration of C. album increases.
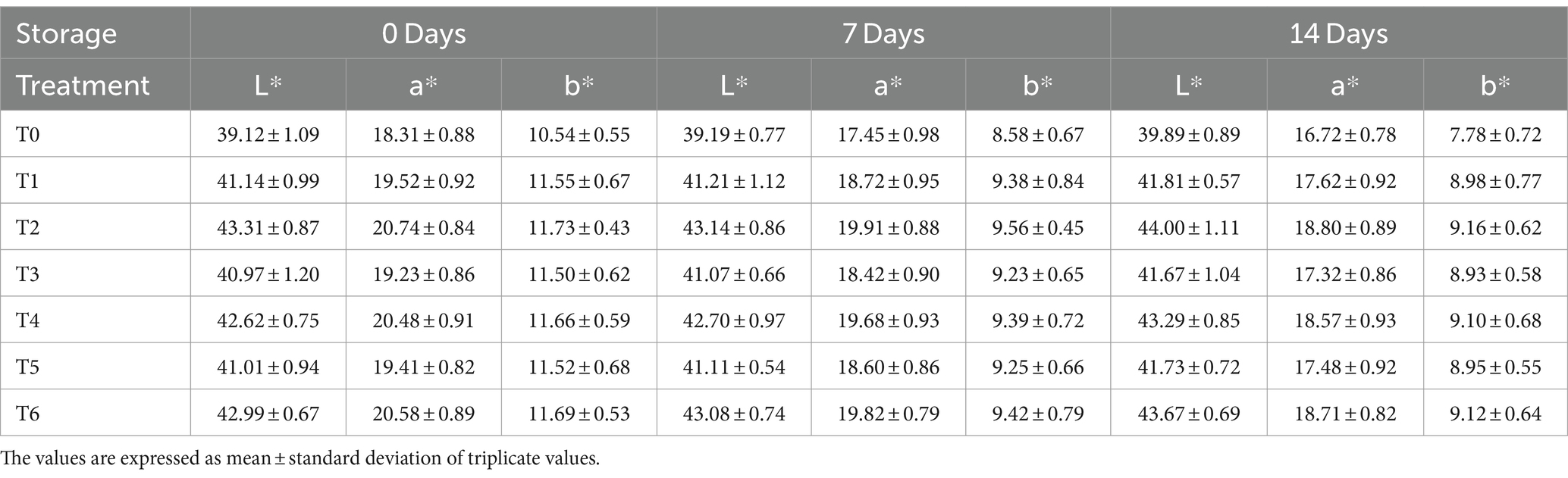
Table 2. Hunter’s color value of ostrich meat patties treated with C. album extract at different storage intervals.
The highest value of L* value was observed for T2 (UAE 02% extract) on the 14th day, which was 44.00 ± 1.11. However, the lowest value of L* value was observed for MAE T3 (01 extract) at 0 days (40.97 ± 1.20). The trend among the techniques was UAE > MAE. While comparing the techniques, the lowest results of the L* value obtained by (0.5 + 0.5%) MAE and UAE in T5 after 0 days were 41.01 ± 0.94. The highest L* value was recorded for (1% + 1%) MAE and UAE in T6 on the 14th day (43.67 ± 0.69). Similar results were achieved when the amount of kale powder extract was increased in research carried out by Khalid et al. (2021), and the meat sample’s lightness L* value significantly increased.
a* value
Different treatments of meat samples had a* (red/green) value in different ranges from 16.72 ± 0.78 to 20.74 ± 0.84. During the two-week storage period, a minimum a* value was observed on the 14th day. While the value of a* was highest at 0 days of storage, the a* value of ostrich meat patties samples declined as storage intervals passed, according to our findings. The lowest a* reading of C. album-treated meat was recorded in the control sample (T0), while the maximum a* value of C. album-treated meat was detected in T2 (2% UAE). According to the results of the current study, the a* value increased as the concentration of C. album extract increased.
The highest value of a* was observed for UAE T2 at 0 days (20.74 ± 0.84). However, the lowest value of a* was observed for MAE T3 on the 14th day, i.e., 17.32 ± 0.86. The trend among the techniques was UAE > MAE. Comparing the techniques, the lowest results of the a* value were obtained by (0.5% + 0.5) MAE and UAE in T5 on the 14th day, i.e., 17.48 ± 0.92. The highest a* value was recorded for (1% + 1) MAE and UAE in T6 after 0 days, i.e., 20.58 ± 0.89. The findings of the current study exhibited that extracts from the UAE exhibit a greater potential of a*.
The highest a* value was 20.74 ± 0.84, while the lowest a* value was 16.72 ± 0.78. The highest value came from the T2 (2% UAE) sample, while the lowest reading came from the control sample.
b* value
Ostrich meat patties had b* (Yellow) values varying from 7.78 ± 0.72 to 11.73 ± 0.43. At 0-day, the highest b* value was observed during the 14th day of storage. Outcomes of the study exhibited that the b* value of ostrich meat patties samples declined as the storage period progressed. The control sample (T0) had the lowest b* value of C. album-treated meat, while the highest b* value of C. album-treated meat patties was detected in T2 (2% UAE). According to our findings, the b* value increased as the concentration of C. album extract increased.
The highest value of b* value observed for UAE was T2 (02%) extract at 0 days, which was 11.73 ± 0.43. However, the lowest value of b* value was observed for MAE, i.e., T3 (01) extract on the 14th day (8.93 ± 0.58).
Comparing the techniques, the lowest results of b* value were obtained by (0.5 + 0.5%) MAE and UAE (T5) on the 14th day, i.e., 8.95 ± 0.55. The highest b* value recorded for (1% + 1%) MAE and UAE (T6) after 0 days was 11.69 ± 0.53. The results concluded that extracts from the UAE exhibit greater potential for b*.
The highest value of b* was 11.73 ± 0.43, whereas the lowest b* reading was 7.78 ± 0.72. The T2 (2% UAE) exhibited the highest value, while the T0 (control sample) showed the lowest value as demonstrated by Table 2. A similar study designed by Khalid et al. (2021) demonstrated that the increasing concentration of kale leaf powder in meat significantly increased the yellowness (b*) value of the meat sample, as observed in the current study. The trend among the techniques for L*, a*, and b* values was UAE > MAE.
Quality and stability test
TBARS
Ostrich meat patties samples had TBARS values ranging from 0.60 ± 0.03 MDA/kg to 0.84 ± 0.04 MDA/kg. The value of the control sample (T0) was the highest, whereas, the value of the T6 sample was the lowest. At 0, 7, and 14 days, the TBARS levels for the control meat patties samples were 0.69 ± 0.06 MDA/kg, 0.75 ± 0.05 MDA/kg, and 0.84 ± 0.04 MDA/kg, respectively. In T1, the TBARS levels were 0.64 ± 0.05 MDA/kg, 0.70 ± 0.02 MDA/kg, and 0.79 ± 0.01 MDA/kg, respectively, at 0, 7, and 14 days. Throughout the storage period, T2 exhibited the TBARS values, i.e., 0.59 ± 0.03 MDA/kg, 0.65 ± 0.04 MDA/kg, and 0.74 ± 0.02 MDA/kg, at 0, 7, and 14th day of storage, respectively. T3 showed the TBARS values, i.e., 0.66 ± 0.04 MDA/kg, 0.72 ± 0.03 MDA/kg, and 0.81 ± 0.02 MDA/kg, at 0, 7, and 14th day of storage, respectively. On the 0, 7th, and 14th day, the TBARS values of T4 were 0.61 ± 0.04 MDA/kg, 0.67 ± 0.02 MDA/kg, and 0.76 ± 0.03 MDA/kg, respectively. For T5 throughout the storage, TBARS values were 0.65 ± 0.02 MDA/kg, 0.71 ± 0.02 MDA/kg, and 0.80 ± 0.04 MDA/kg, respectively. On the 0, 7th, and 14th day, the TBARS values of T6 were 0.60 ± 0.03 MDA/kg, 0.66 ± 0.04 MDA/kg, and 0.75 ± 0.01 MDA/kg, respectively.
The highest TBARS value was recorded at the 14th day of storage, while the lowest reading was observed at the first day of storage period. According to our findings, the TBARS value of ostrich meat patties samples increased non-significantly as storage intervals passed. The highest TBARS value of C. album-treated meat was found in the control sample, while the lowest TBARS value of treated meat was found at T6. As per the outcomes of the current study, the TBARS value decreased significantly as the C. album concentration increased.
The lowest TBARS for UAE was observed T2 after 0 days, i.e., 0.59 ± 0.03 MDA/kg. However, the highest TBARS value was observed for MAE T3 (01% extract) on the 14th day, i.e., 0.81 ± 0.02 MDA/kg. The trend among the techniques was MAE > UAE. According to Khalid et al. (2021), TBARS values were higher in the presence of a higher level of oxidized lipids.
Comparing the techniques, the highest results were obtained by (0.5% + 0.5) MAE, and UAE (T5) on the 14th day was 0.80 ± 0.04 MDA/kg. The lowest TBARS value recorded for (1% + 1) MAE and UAE (T6) after 0 days was 0.60 ± 0.03 MDA/kg.
The highest TBARS value was 0.84 ± 0.04 MDA/kg, while the lowest TBARS value was 0.60 ± 0.03. The control sample (T0) exhibited the highest value, while the T6 treatment showed the lowest TBARS readings. On the first day of storage (0 days), the smallest value was recorded, and after 14 days, the highest values were observed. Khalid et al. (2021) found that the TBARS content of a kale-fortified meat-treated sample met the standard requirement for meat (Figure 2).
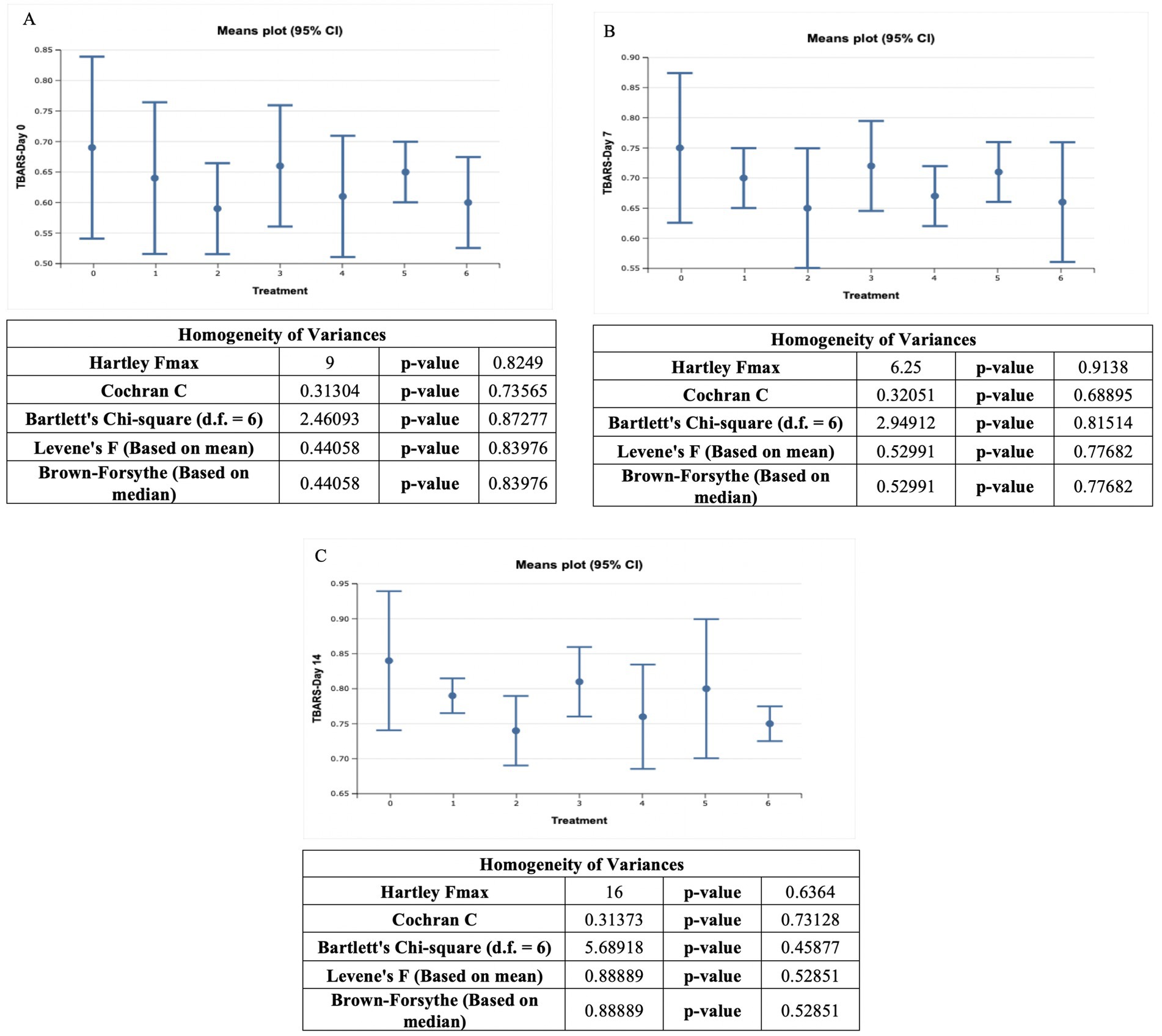
Figure 2. TBARS (MDA/kg) value of ostrich meat treated with C. album extract at different storage intervals, (A) Day 0 storage, (B) Day 7 storage, and (C) Day 14 storage.
POV
The POV content of ostrich meat patties ranged from 0.28 ± 0.01 meq peroxide/kg to 0.65 ± 0.04 meq peroxide/kg. The T0 (control sample) exhibited the highest value, while treatment T4 showed the lowest reading (Figure 3). The POV levels in the control sample (T0) were 0.40 ± 0.03 meq peroxide/kg, 0.54 ± 0.05 meq peroxide/kg, and 0.65 ± 0.042 meq peroxide/kg at 0, 7, and 14 days, respectively. At 0, 7, and 14 days, the POV levels in T1 were 0.36 ± 0.02 meq peroxide/kg, 0.48 ± 0.03 meq peroxide/kg, and 0.60 ± 0.03 meq peroxide/kg, respectively. The POV values were 0.30 ± 0.02 meq peroxide/kg, 0.44 ± 0.04 meq peroxide/kg, and 0.56 ± 0.01 meq peroxide/kg at 0, 7, and 14, in T2, respectively. The POV values were 0.34 ± 0.03 meq peroxide/kg, 0.50 ± 0.01 meq peroxide/kg, and 0.62 ± 0.02 meq peroxide/kg at 0, 7th, and 14th day in T3, respectively. The POV values in T4 samples were 0.28 ± 0.01 meq peroxide/kg, 0.46 ± 0.02 meq peroxide/kg, and 0.58 ± 0.02 meq peroxide/kg at 0, 7th, and 14th day, respectively. The POV values were 0.35 ± 0.02 meq peroxide/kg, 0.49 ± 0.02 meq peroxide/kg, and 0.61 ± 0.03 meq peroxide/kg at 0, 7th, and 14th day in T5, respectively. The POV values of T6 were 0.29 ± 0.01 meq peroxide/kg, 0.45 ± 0.03 meq peroxide/kg, and 0.57 ± 0.01 meq peroxide/kg at 0, 7th, and 14th day, respectively.
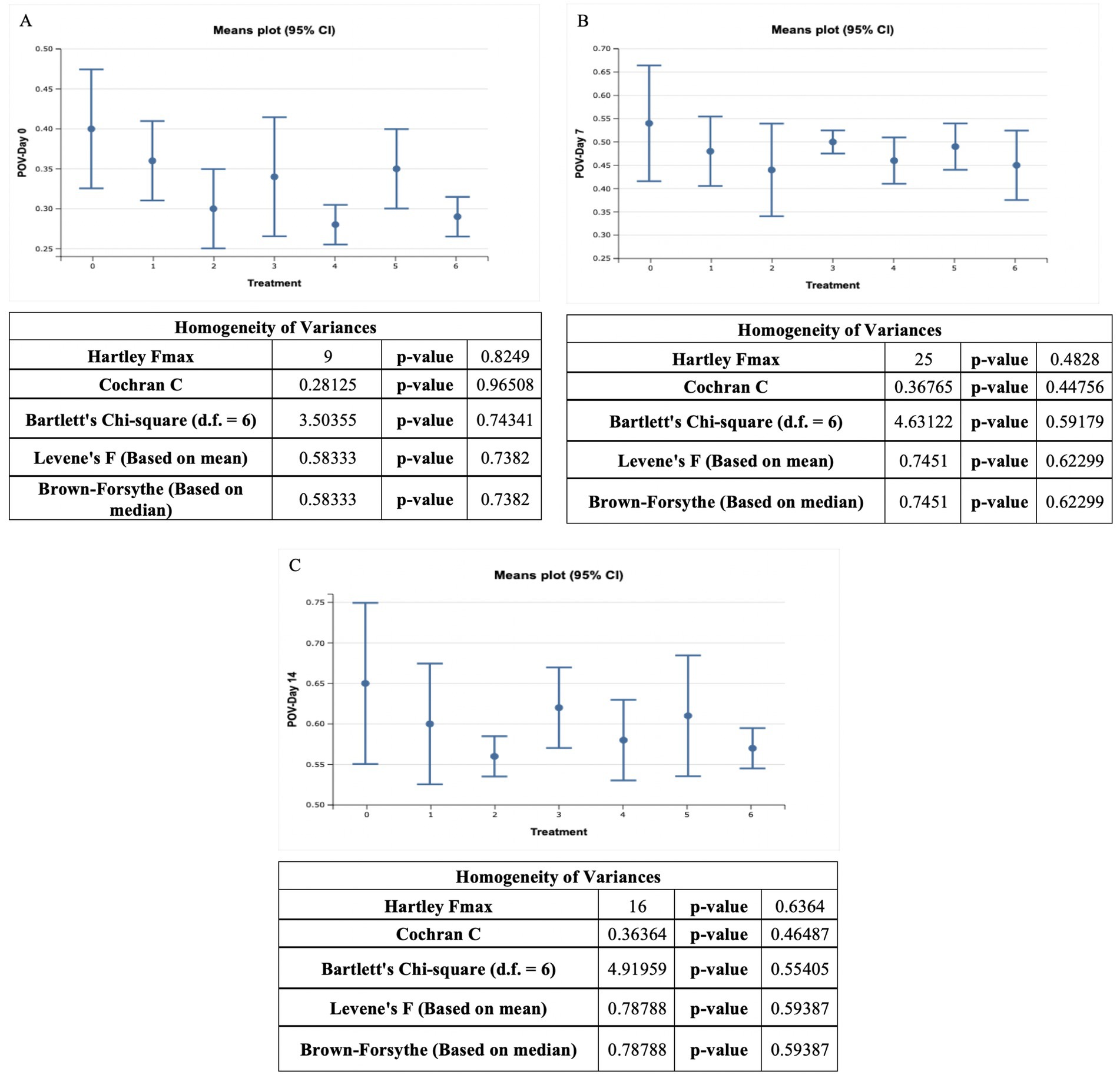
Figure 3. POV value (meq peroxide/kg) of ostrich meat treated with C. album extract at different storage intervals, (A) Day 0 storage, (B) Day 7 storage, and (C) Day 14 storage.
As per the current findings, the POV value of all-meat patties samples escalated non-significantly as the duration of storage proceeded. The control sample (T0) had the higher POV values among all treated meat samples, whereas, the T4 had the lowest POV value. The POV value decreased as the C. album content increased, according to our observations. The highest POV value was 0.65 ± 0.04 meq peroxide/kg, whereas, the lowest POV reading was 0.28 ± 0.01 meq peroxide/kg. The higher POV value was discovered after the 14th day of storage, the control sample (T0) yielded the highest result, whereas, the T2 sample yielded the lowest POV value 0.56 ± 0.01 meq peroxide/kg.
The trend among the techniques was MAE > UAE. According to Khalid et al. (2021), a high peroxide value (POV) in meat is generally considered bad and indicative of poor quality. The peroxide value measures the extent of lipid (fat) oxidation in a food product, including meat. High POV values suggest that the meat has undergone oxidative rancidity, which can lead to the development of off-flavors and odors and a deterioration of the meat’s overall quality.
TVBN
The TVBN content of ostrich meat patties samples ranged from 4.04 ± 0.36 mg/100 mL to 6.65 ± 0.41 mg/100 mL. The control sample (T0) had the lowest value on the 0-day, while the control ostrich meat patties samples had a greater value on the 14th day. The TVBN values for the control meat-treated sample were 4.04 ± 0.36 mg/100 mL, 5.44 ± 0.39 mg/100 mL, and 6.65 ± 0.41 mg/100 mL at 0, 7th, and 14th day, respectively. The TVBN levels were 4.27 ± 0.35 mg/100 mL, 5.28 ± 0.35 mg/100 mL, and 6.49 ± 0.39 mg/100 mL, respectively, at 0, 7th, and 14th day for T1. The TVBN values of T2 were 4.14 ± 0.33 mg/100 mL, 5.14 ± 0.36 mg/100 mL, and 6.28 ± 0.40 mg/100 mL, respectively, on 0, 7th, and 14th day. Furthermore, the TVBN values were determined to be 4.30 ± 0.38 mg/100 mL, 5.31 ± 0.33 mg/100 mL, and 6.53 ± 0.37 mg/100 mL at 0, 7th, and 14th day for T3. TVBN value for sample T4 was 4.20 ± 0.37 mg/100 mL, 5.18 ± 0.38 mg/100 mL, and 6.32 ± 0.40 mg/100 mL, respectively, at 0, 7th, and 14th day. The TVBN values were 4.28 ± 0.32 mg/100 mL, 5.30 ± 0.34 mg/100 mL, and 6.29 ± 0.38 mg/100 mL, respectively, at 0, 7th, and 14th days for T5. Furthermore, the TVBN values were determined to be 4.16 ± 0.34 mg/100 mL, 5.16 ± 0.37 mg/100 mL, and 6.30 ± 0.36 mg/100 mL at 0, 7th, and 14th day for T6.
On day 0 of the storage study, the highest TVBN value was recorded. On the 14th day of storage, the lowest TVBN value was observed. As per the outcomes of this study, the TVBN value of all meat samples decreased as storage intervals passed. The control sample (T0) had the lowest TVBN value on the first day, while the maximum TVBN value of ostrich meat patties was found in the control sample (T0) on the 14th day. The TVBN value decreased as the C. album concentration increased, according to our data. As per the findings of Bekhit et al. (2021), a high TVBN value in meat is generally considered bad and undesirable. TVBN is a measure of the freshness and spoilage of meat, particularly seafood and fish. It represents the amount of volatile nitrogenous compounds, such as ammonia and amines that are produced as a result of microbial and enzymatic degradation of proteins in the meat.
The lowest TVBN was observed for UAE T2 after 0 days, i.e., 4.14 ± 0.33 mg/100 mL. However, the highest TVBN value was observed for MAE T3 on the 14th day (6.53 ± 0.37 mg/100 mL). The trend among the techniques was MAE > UAE. Comparing the techniques, the highest results were obtained by (0.5 + 0.5%) MAE and UAE in T6 at 14 days, i.e., 6.30 ± 0.36 mg/100 mL. The lowest TVBN was recorded for (1 + 1%) MAE and UAE in T6 after 0 days, i.e., 4.16 ± 0.34 mg/100 mL. The greater value was detected after 14 days of storage and the lowest value at 0 days. The study by Khalid et al. (2021) on ostrich meat proved that TVBN was high on the 14th day and low on the first day of storage (Figure 4).
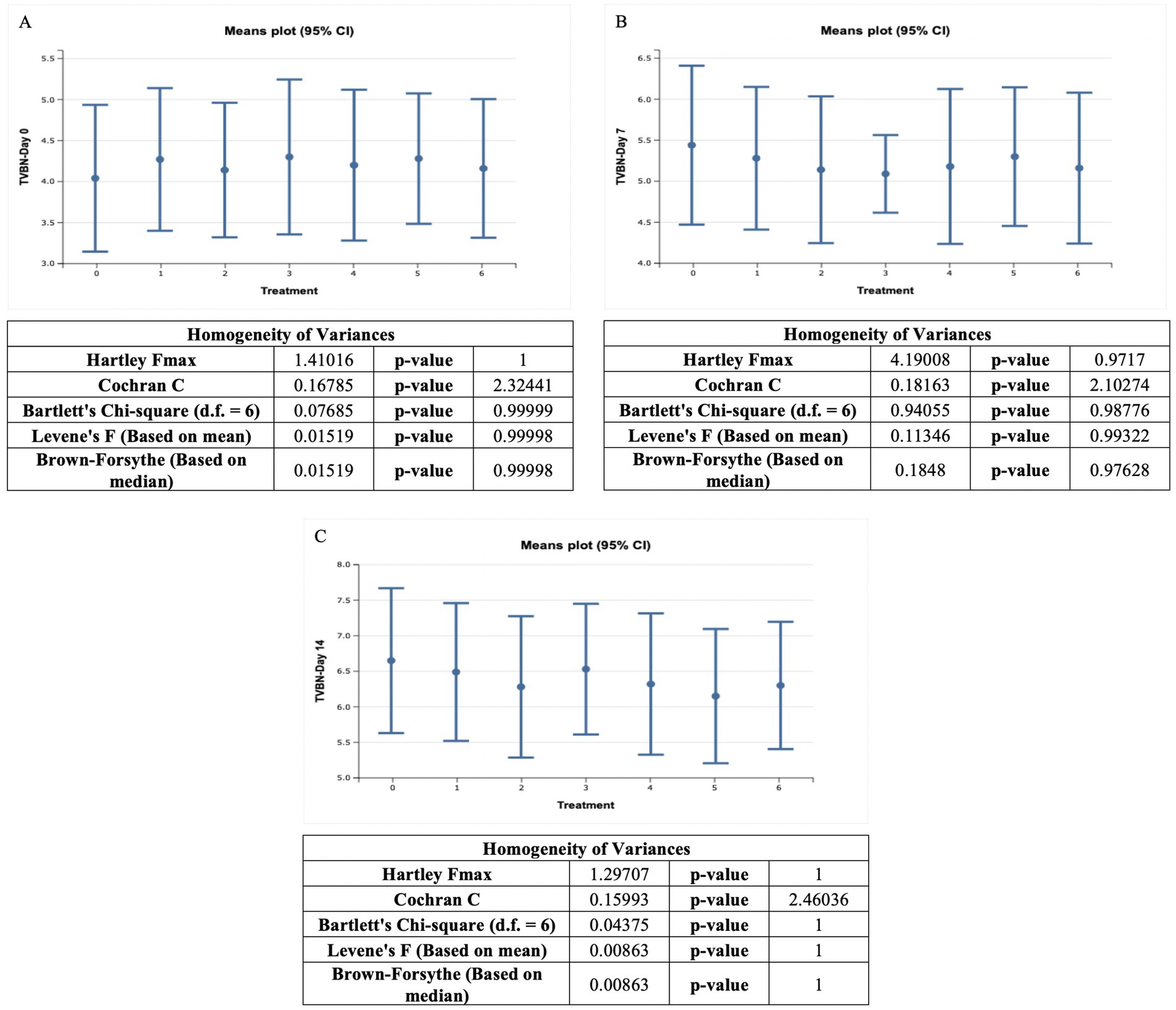
Figure 4. TVBN value (mg/100 mL) of ostrich meat treated with C. album extract at different storage intervals, (A) Day 0 storage, (B) Day 7 storage, and (C) Day 14 storage.
Antioxidant potential
Total phenolic content
The total phenolic content of ostrich meat patties ranged from 99 ± 0.29 mg GAE/ 100 g to 130 ± 0.07 mg GAE/100 g. The T2 sample had the highest value, while the control sample (T0) had the lowest value as shown in Figure 5. The TPC measured on the 14th day of storage was found to be the minimum. 0 days of storage exhibited the maximum TPC for all the samples. As per the outcomes, the TPC value of meat patties samples declined as storage intervals passed. The lowest TPC value was found in the control sample, whereas, the maximum TPC value of C. album-treated meat patties was found in T2 (2% UAE of C. album extract). As per the results of the current study, the TPC values rise as the concentration of C. album rises.
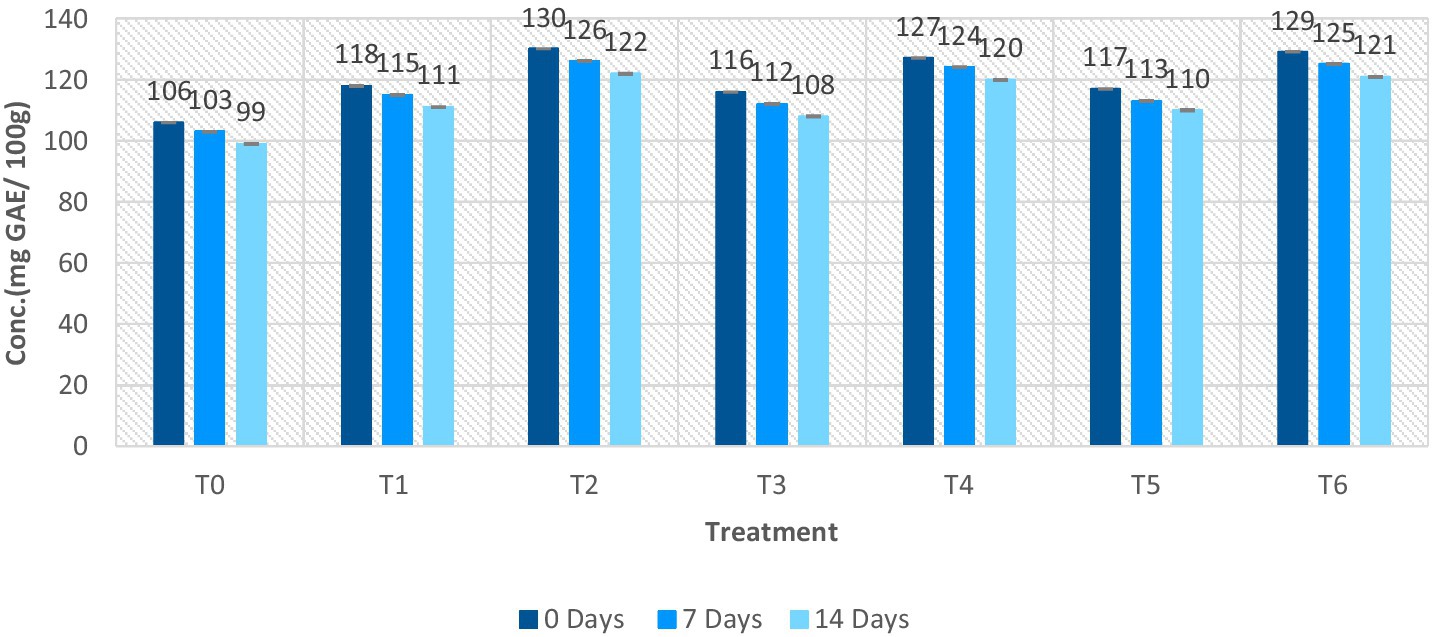
Figure 5. TPC value of ostrich meat treated with C. album extract at different storage intervals (mg GAE/100 g).
The highest value of TPC was observed for UAE in T2 after 0 days, i.e., 130 ± 0.07 mg GAE/100 g. However, the lowest value of TPC was observed for T3 (01% MAE extract) on the 14th day, i.e., 108 ± 0.27 mg GAE/100 g. Comparing the techniques, the lowest results of TPC were obtained by (0.5% + 0.5 MAE and UAE) T5 on the 14th day (110 ± 0.29 mg GAE/100 g). The highest TPC was recorded for (1% + 1 MAE and UAE) T6 after 0 days, i.e., 129 ± 0.13 mg GAE/100 g.
DPPH assay
DPPH free radical is a nanometric structure that impedes free radical generation by hydrogen donation (Mejía-Méndez et al., 2024). The DPPH range of different meat samples was found to be between 62 ± 0.22% to 79 ± 0.23% as shown in Figure 6. On the 14th day of storage, the lowest DPPH value was recorded. The highest DPPH assay reading was achieved on the first day of storage (0-day). The DPPH value of all-meat patties samples declined as storage intervals proceeded, as per current results. The control sample (T0) exhibited the lowest DPPH value, whereas, the highest DPPH value of C. album-treated meat patties was identified in T2 (2% UAE of C. album extract). As per the outcomes, the DPPH value elevated as C. album concentration increased.
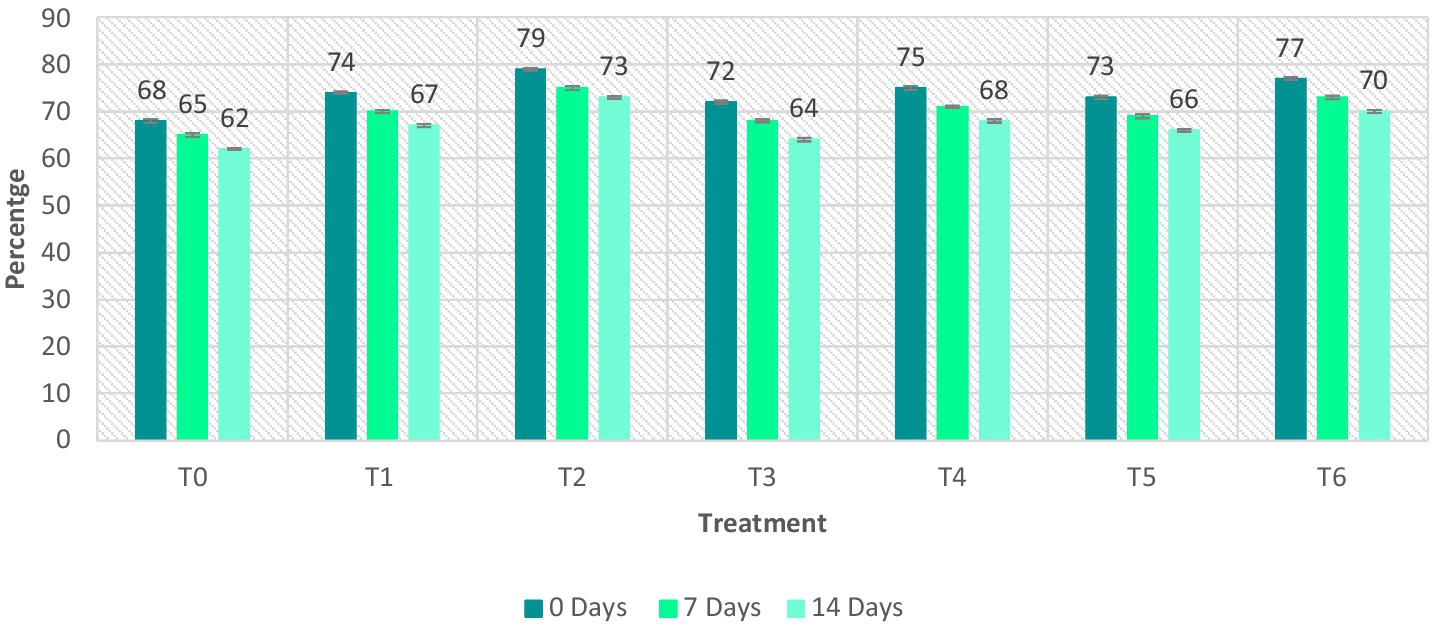
Figure 6. DPPH value of ostrich meat treated with C. album extract at different storage intervals (%).
The highest value of DPPH was observed for T2 after 0 days, i.e., 79 ± 0.23%. However, the lowest value of DPPH was observed for T3 (MAE 01 extract) on the 14th day (5.2 ± 0.03%). The trend among the techniques was UAE > MAE. Comparing the techniques, the lowest results of DPPH radical scavenging potential were obtained by T5 (0.5 + 0.5% MAE and UAE) on the 14th day, i.e., 66 ± 0.28%. The highest TPC was recorded for T6 (1% + 1% MAE and UAE) after 0 days, i.e., 77 ± 0.30%. They concluded that the extracts with UAE exhibit greater antioxidant potential. The highest DPPH was 79 ± 0.23%, whereas, the lowest DPPH was 62 ± 0.22%. The highest DPPH value was observed in the T2 (2% UAE), whereas, the lowest percent inhibition was observed in the control sample. The lowest value of DPPH value was recorded on the last day (day 14) of storage, whereas, the highest value was recorded on the first day of storage (0 days).
FRAP assay
The FRAP assay value was recorded between 5.0 ± 0.06 mmol FSE /100 g to 6.9 ± 0.04 mmol FSE/100 g as shown in Figure 7. During 2-week storage, the lowest FRAP assay readings were observed in T0 (control sample) while the highest FRAP values were observed on the first day (0-day), and the lowest FRAP levels were observed on the last day of storage (14-day). The results demonstrated that the FRAP values of meat patties samples displayed a declining trend over the 2-week storage period. However, a decrease in the FRAP values of the evaluated samples exhibited that the antioxidant potential decreased with the increase in storage time.
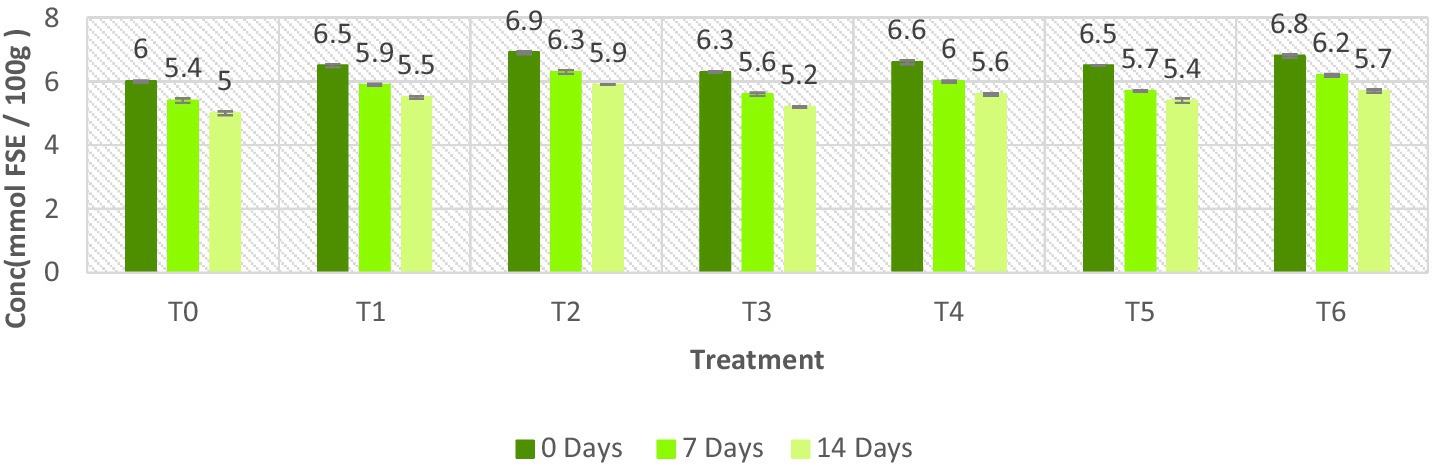
Figure 7. FRAP value of ostrich meat treated with C. album extract at different storage intervals (mmol FSE/100 g).
Among different treatments, the lowest FRAP values were found in T0 (the control sample), while the highest FRAP value of C. album-treated meat was detected in T2 (2% UAE). Our finding exhibited that the FRAP value increases with the intensification of the concentration of C. album extract. The higher FRAP value was 6.9 ± 0.04 mmol FSE /100 g, and the lower FRAP value was 5.0 ± 0.06 mmol FSE /100 g. The highest value of FRAP was observed for T2 at 0 days, i.e., 6.9 ± 0.04 mmol FSE/100 g. However, the lowest value of FRAP was observed for T0 at 14 days, i.e., 5.0 ± 0.06 mmol FSE/100 g.
The lowest results of FRAP were obtained by (0.5% + 0.5 MAE and UAE) T5 on the 14th day, i.e., 5.4 ± 0.07 mmol FSE /100 g. The highest FRAP value was recorded for T6 (1% + 1% MAE and UAE) at 0 days, i.e., 6.8 ± 0.05 mmol FSE /100 g. They concluded that extracts prepared using the UAE technique exhibit greater antioxidant potential. According to the findings of Khalid et al. (2020), the increase in the concentration of plant extract increases the antioxidant potential of the product.
ABTS assay
The ABTS value of meat patties was found to range from 72 ± 0.13 mmol/L to 91 ± 0.04 mmol/L. The control sample (T0) had the lowest value, and the T2 sample had the highest value, as shown in Figure 8. As per the outcomes of this study, the ABTS value of ostrich meat patties declined as storage intervals passed. The lowest ABTS value was found in the control sample, while the maximum ABTS value of C. album-treated meat patties was found in T2 (2% UAE). As per the outcomes, the ABTS value rises as the concentration of C. album extract increases. The highest value of ABTS was observed for T2 (02% UAE extract) after 0 days, i.e., 91 ± 0.04 mmol/L. Comparing the techniques, the lowest results of ABTS value were obtained by (0.5% + 0.5 MAE and UAE) T5 on the 14th day was 79 ± 0.05 mmol/L. The highest ABTS value was recorded for (1% + 1 MAE and UAE) T6 after 0 days, i.e., 90 ± 0.06 mmol/L.
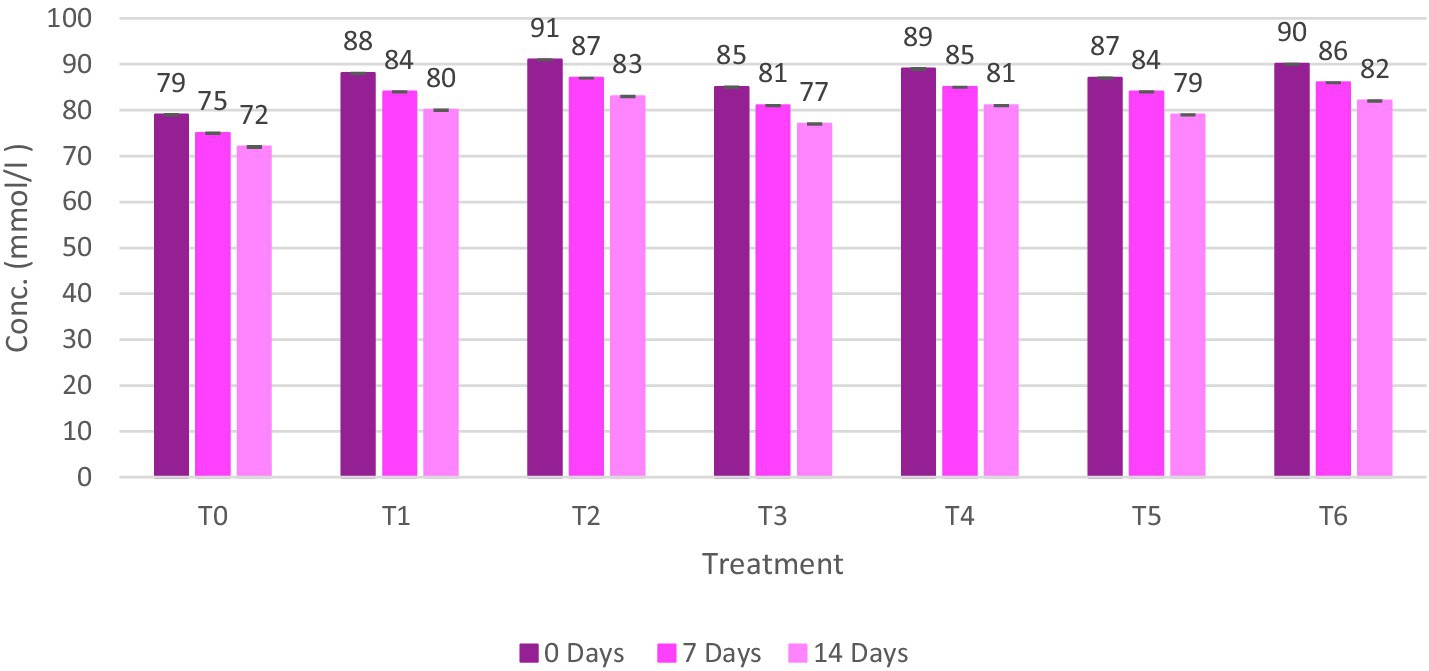
Figure 8. ABTS value (mmol/L) of ostrich meat treated with C. album extract at different storage intervals.
Total microbial count
Ostrich meat patties had TMC values ranging from 6.12 ± 0.08 cfu/mL to 9.12 ± 0.04 cfu/mL. The value of the control sample (T0) was the highest, while the value of the T2 sample was the lowest. During storage, the minimum TMC value was measured at 0 days. The maximum TMC value was determined on the 14th day of storage. The TMC value of all-meat patties samples increased non-significantly as storage intervals passed. According to our findings, the highest TMC value was observed in the control sample, while the lowest TMC value of C. album-treated meat was detected in T2. According to our findings, the TMC value decreased as the C. album concentration increased.
The highest TMC value was 9.12 ± 0.04 cfu/mL, whereas the lowest TMC value was 6.12 ± 0.08 cfu/mL. The lowest value came from the T2 sample, and the highest came from the control sample. The highest value was recorded on the 14th day of storage, whereas, the lowest value was observed on the 0-day. The given results are shown in Table 3. The trend among the techniques was MAE > UAE. According to Khalid et al. (2021), a high value of TMC in meat is generally considered bad and indicates poor quality.
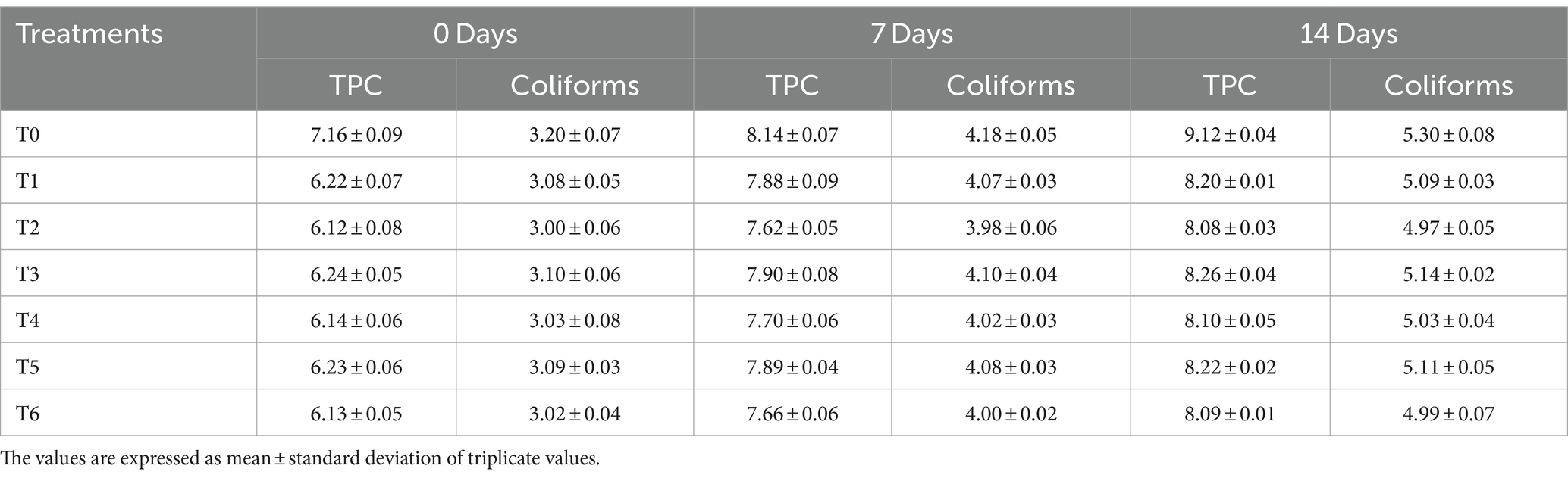
Table 3. Total microbial count and coliform count (cfu/mL) of ostrich meat treated with C. album extract at different storage intervals.
Our results of TMC of meat enriched with C. album extract agreed with a study carried out by Khalid et al. (2022), who reported that adding kale to ostrich meat significantly reduced the microbial load.
Coliform bacteria
The coliform value of various meat samples varies from 3.00 ± 0.06 cfu/mL to 5.30 ± 0.08 cfu/mL, with the control sample (T0) having a higher value and the T2 has a lower value as shown in Table 3. The value of coliform was recorded to be highest on the 14th day of storage. The smallest value of coliform was recorded at 0-day storage. The coliform count in ostrich meat patties increased as storage intervals passed. The control sample (T0) had the highest coliform count, while the lowest coliform count of C. album-treated meat was found in T2. The coliform count decreased as the concentration of C. album increased, as per the findings of the current study. The lowest coliform count was 3.00 ± 0.06 cfu/mL, while the highest coliform count was 5.30 ± 0.08 cfu/mL.
Sensory evaluation
Appearance
The appearance parameter in various meat samples ranged from 6.65 ± 0.4 to 7.72 ± 0.4, with the lowest value for the T4 (2% MAE) sample and the highest for the control sample. The lowest score for the appearance of the meat patties was achieved on the last day of storage (Day 14), while the best appearance score was for fresh meat patties at day 0. The appearance parameter value in ostrich meat patties decreases as storage intervals pass. The highest score of appearance was observed for T1 at 0 days (7.68 ± 0.3). However, the lowest value of appearance was observed for T4 on the 14th day of storage, i.e., 6.65 ± 0.4.
Taste
The taste values in various meat samples ranged from 6.29 ± 0.8 to 6.90 ± 0.9, with the control sample (T0) having the highest value and the T4 (2% MAE) sample having the lowest.
Texture
The texture values in meat patties samples ranged from 6.50 ± 0.6 to 8.19 ± 0.4, with the lowest value being T4 (2% MAE) and the highest being T0 (control sample). The texture value decreased as the concentration of C. album extract increased, according to our findings.
Flavor
The flavor value of various meat samples varies from 6.65 ± 0.7 to 7.50 ± 0.4, with the T4 sample having the lowest value and the control sample (T0) having the highest. According to our findings, the flavor values of all meat patties decreased as storage intervals passed. The control sample (T0) had the highest flavor score, whereas the C. album-treated meat patties T4 had the lowest flavor score. When comparing the techniques, the lowest results of flavor values were obtained by T6 (1 + 1% MAE and UAE) on the 14th day (6.68 ± 0.2). The highest flavor value was recorded for (0.5 + 0.5% MAE and UAE) T5 at 0 days (7.45 ± 0.3).
Overall acceptability
The score of overall acceptability of ostrich meat patties ranged from 6.23 ± 0.2 to 7.13 ± 0.5, with the best results achieved for the control sample (T0) and the lowest for the T4. Overall acceptability in meat patties samples decreased as the storage duration increased. The panel preferred control treatment the most, whereas, the C. album extract treated T4 (2% UAE) meat had the lowest overall acceptability. As per the outcomes of the current study, the overall acceptability value decreased as the concentration of C. album increased. Figure 9 shows the sensory evaluation of ostrich meat patties treated with C. album extract at various storage intervals.
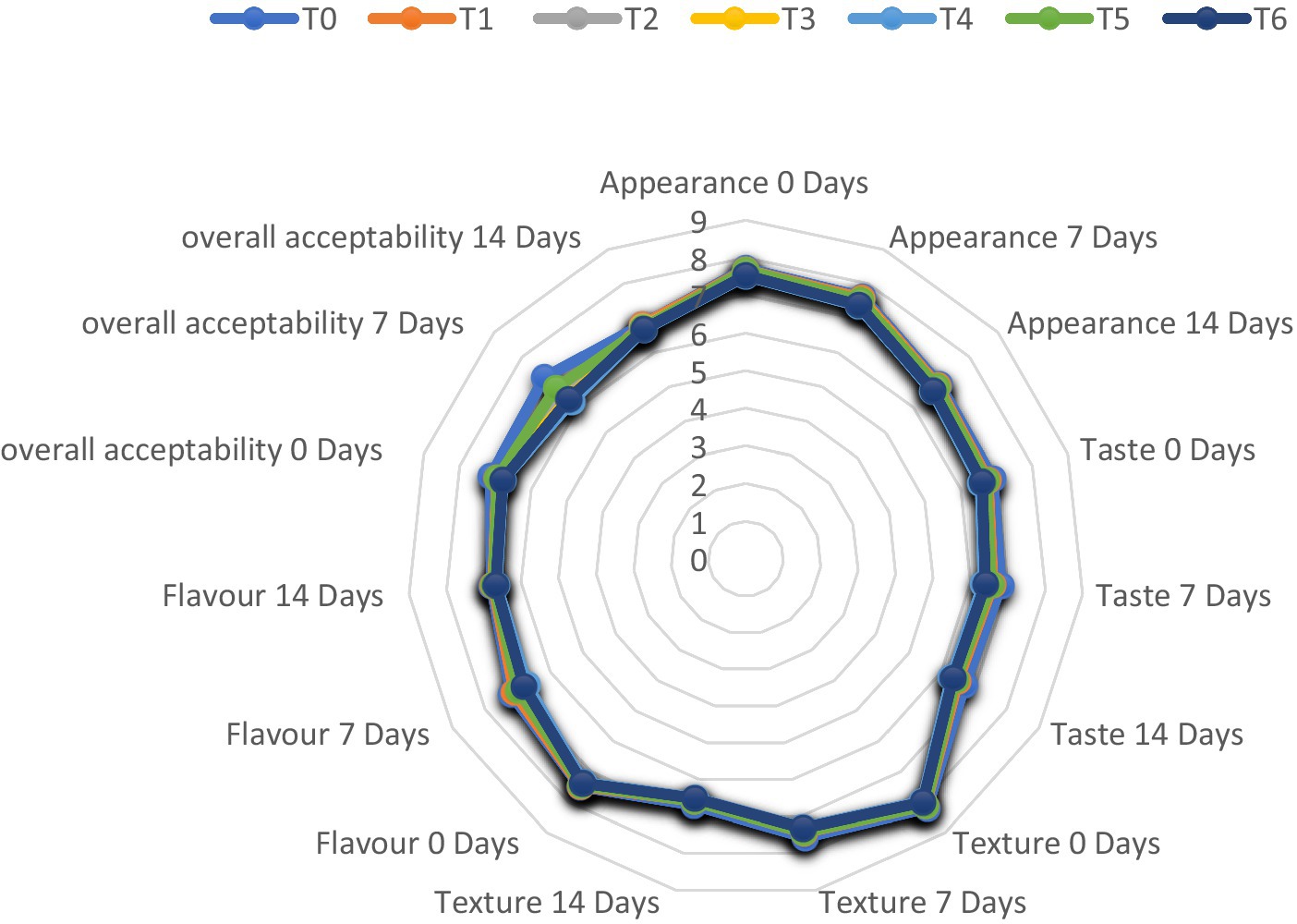
Figure 9. Sensory evaluation of ostrich meat patties treated with C. album extract at different storage intervals.
Discussion
For the recovery of phytochemicals, the selection of extraction techniques is crucial. To enhance extraction efficiency, it is necessary to consider the nature of bioactive components and plant material. Bioactive components of C. album have been identified as having medicinal and biological value. Protein content is often higher in the plant’s young leaves and sensitive shoots. The protein concentration can be in the range of 2 to 5% of the dry weight (Ford et al., 2023). Leucine, isoleucine, lycine, and vitamin C are the primary active ingredients in C. album, which contains a large number of amino acids (Singh et al., 2023). Healthy unsaturated fats, such as monounsaturated and polyunsaturated fats, make up the majority of the C. album fat content. In a balanced diet, it can offer a small quantity of beneficial fats even though it is not often regarded as a substantial source of dietary fat (Abedi and Sahari, 2014). 2.5% of the C. album seed weight contains fat, according to Khattak et al. (2011). Rutin and chlorogenic acid, two biological substances, remain discovered in the C. album ethyl acetate extract (Amodeo et al., 2019). The Turkish C. album methanolic extract has hesperidin as its primary flavonoid (Yılmaz et al., 2019). Additionally, identified compounds include protocatechuic acid, quercetin, apigetrin, astragalin, and chlorogenic acid. In the methanolic extract of C. album bioactive compounds like luteolin, apigetrin, and astragalin (11.28, 7.94, and 40.58 g/g, individually) were found.
UAE and MAE are novel extraction techniques for the isolation of plant bioactive components (Usman et al., 2022). These methods are environmentally friendly and can have a positive influence on the chemical and food sector development (Carpentieri et al., 2021). To achieve full compound retrieval within a very short time, these techniques are appropriate methods and characterized by better extraction efficiency, easy post-extraction handling, minimal solvent requirement, and remarkable product output (Reddy et al., 2020). To produce clean-label foods, a wide range of natural colorants, acids, antioxidants, and inorganic elements can be extracted from food matrices using advanced extraction techniques (Alvi et al., 2022). In an intriguing investigation conducted by Mir et al. (2019), they examined the impact of high-intensity ultrasound on C. album seed protein isolates. A significant improvement in whiteness index, solubility, foaming capacity, and stability was observed. In addition, greater exposure to ultrasound waves breaks the molecular bonds and imparts conformational and structural changes, resulting in a decrease in denaturation temperature. Choudhary et al. (2021) studied the effect of MAE on phytoconstituents of C. album, and their results exhibited that MAE escalated the secondary metabolite concentration significantly. In C. album extract, the TPC of the extract was found to be 2.94 ± 0.068 mg GAE/g.
Peroxide value, also known as POV, represents the peroxide content of fats and oils produced in the initial stages of oxidation (Xu et al., 2020). Lower peroxide concentration is caused by the potential of plant bioactive components by altering the fat structure and reducing the enzyme activity of fat synthase. The production of peroxides is among the main catalysts for oxidation process initiation (Pérez-Torres et al., 2021). In meat and meat products, the fat oxidation reaction is catalyzed by iron (Bu et al., 2023). Malondialdehyde is an aldehyde produced as a result of lipid oxidation in unsaturated fatty acids (Mas-Bargues et al., 2021). In meat, this aldehyde is of the utmost importance because even trace levels of malondialdehyde have the potential to induce rancidity and off aroma (Tateo et al., 2020). During the storage period of all treatments, an increase in TBA was observed because of continuous aldehyde production (Song et al., 2022; Al-Dalali et al., 2022; Khodanazary and Mohammadzadeh, 2023).
Several studies have examined the antiseptic action of C. album extracts and essential oil by means of diffusion, dilution, and colony counting examinations (Khomarlou et al., 2017; Balpetek Külcü et al., 2019; Saini et al., 2019; Umar et al., 2020). It was discovered that Gram-negative bacteria are typically less susceptible to C. album than Gram-positive bacteria. However, the results varied according to the plant part used, the sort of extract, the methods employed, the strains that were examined, and the extraction solvent. According to Lone et al. (2017), inhibition zone diameters ranging from 16 mm for Klebsiella pneumoniae to 28 mm for Staphylococcus aureus were observed. Furthermore, the Indian C. album leaf methanol extract revealed dose-dependent effectiveness (25–100%). The largest inhibitory zones against S. aureus, Bacillus subtilis, and Escherichia coli were obtained when 100% pure C. album extract was used, but similar effectiveness was observed with 75% concentration against Pseudomonas aeruginosa (Parkash and Patel, 2015). Singh et al. (2011) explored the antibacterial potential of Indian C. album extracts from leaves (methanol and water solvent) against human pathogenic microbes. In general, methanol extract surpassed aqueous extract against S. aureus, E. coli, Salmonella typhimurium, and P. aeruginosa, whereas aqueous extract generated a wider zone of effectiveness against Proteus vulgaris.
Conclusion
Overall best results were achieved in ostrich meat patties with 2% UAE extract of C. album, i.e., (TBARS, 0.74 ± 0.02 MDA/kg; POV, 0.56 ± 0.01 meq peroxide/kg; TVBN, 6.28 ± 0.40 mg/100 mL; TMC, 8.08 ± 0.03 cfu/mL, Total coliforms, 4.97 ± 0.05 cfu/mL; TPC, 122 ± 0.28 mg GAE/ 100 g; DPPH assay, 73 ± 0.27%; FRAP assay, 5.9 ± 0.01 mmol FSE / 100 g and ABTS assay, 83 ± 0.08 mmol/L) after 14 days of storage. The results proved that when MAE and UAE were compared in terms of the extraction of bioactive components, continued exposure to sound waves gave better results than microwave radiation. Ultrasound boosts mass transfer and disintegrates the cellular matrix, facilitating the release of bioactive components, without any damage. In conclusion, the sustainable clean label extraction of bioactive compounds from C. album using UAE, combined with the incorporation of ostrich meat, offers a truly innovative approach in the food industry. Ostrich meat is lean with low fat and a distinguished, unique flavor that pairs really well with the aromatic compounds of C. album. The results of the current study prove that this combination of plant extract and ostrich meat not only provides a clean-label food product but also provides an opportunity to produce an exceptionally flavorful and nutritious meat product. This approach caters to the consumer preference for sustainable, clean-label, and nutritionally enhanced food products. This path still requires exploration and development to redefine the production processes for functional meat products. The clean-label approach provides consumers with environmentally friendly and wholesome food choices. However, further research in this field holds great opportunities for developing sustainable and nutritious food options.
Data availability statement
The original contributions presented in the study are included in the article/supplementary material, further inquiries can be directed to the corresponding authors.
Author contributions
MK: Conceptualization, Methodology, Writing – original draft. HS: Investigation, Supervision, Writing – review & editing. MN: Project administration, Software, Validation, Writing – review & editing. KS: Formal analysis, Supervision, Writing – original draft. MA: Methodology, Writing – review & editing. WK: Formal analysis, Funding acquisition, Project administration, Writing – review & editing. HR: Conceptualization, Formal analysis, Writing – review & editing. MF: Resources, Visualization, Writing – original draft. TA: Investigation, Methodology, Writing – review & editing. AA: Resources, Software, Supervision, Writing – review & editing.
Funding
The author(s) declare that no financial support was received for the research, authorship, and/or publication of this article.
Acknowledgments
The authors acknowledge support from the Department of Food Science, GCUF, for carrying out all research activities. The authors are very thankful to the Researchers Supporting Project (Number RSPD2024R641) at King Saud University in Riyadh, Saudi Arabia for supporting this research.
Conflict of interest
The authors declare that the research was conducted in the absence of any commercial or financial relationships that could be construed as a potential conflict of interest.
Publisher’s note
All claims expressed in this article are solely those of the authors and do not necessarily represent those of their affiliated organizations, or those of the publisher, the editors and the reviewers. Any product that may be evaluated in this article, or claim that may be made by its manufacturer, is not guaranteed or endorsed by the publisher.
References
Abedi, E., and Sahari, M. A. (2014). Long‐chain polyunsaturated fatty acid sources and evaluation of their nutritional and functional properties. Food Sci. Nutr. 2, 443–463.
Ahn, D. U., Jo, C., Du, M., Olson, D. G., and Nam, K. C. (2000). Quality characteristics of pork patties irradiated and stored in different packaging and storage conditions. Meat Sci. 56, 203–209. doi: 10.1016/S0309-1740(00)00044-9
Al-Dalali, S., Li, C., and Xu, B. (2022). Effect of frozen storage on the lipid oxidation, protein oxidation, and flavor profile of marinated raw beef meat. Food Chem. 376:131881. doi: 10.1016/j.foodchem.2021.131881
Alvi, T., Asif, Z., and Khan, M. K. I. (2022). Clean label extraction of bioactive compounds from food waste through microwave-assisted extraction technique-a review. Food Biosci. 46:101580. doi: 10.1016/j.fbio.2022.101580
Amodeo, V., Marrelli, M., Pontieri, V., Cassano, R., Trombino, S., Conforti, F., et al. (2019). Chenopodium album L. and Sisymbrium officinale (L.) Scop.: phytochemical content and in vitro antioxidant and anti-inflammatory potential. Plants, 8:505.
AOAC. (2005). Official methods of analysis of the association of official analytical chemists. 8th Edition. Maryland, USA: Association of Official Analytical Chemists.
Arora, C., Sahua, D., Bhartib, D., Tamrakara, V., Sonia, S., and Sharma, S. (2019). Adsorption of hazardous dye crystal violet from industrial waste using low-cost adsorbent Chenopodium album. Desalin. Water Treat. 167, 324–332. doi: 10.5004/dwt.2019.24595
Aslam, M. N., Sohaib, M., Khan, A. U., Ali, S., Amjad, A., and Ahmed, S. (2020). Lipids oxidative stability and microbial shelf life quality of licorice (glycyrrhiza glabra L.) extract supplemented chicken patties. Braz. J. Poult. Sci. 22:eRBCA-2020. doi: 10.1590/1806-9061-2020-1316
Awada, L., Chalvet-Monfray, K., Tizzani, P., Caceres, P., and Ducrot, C. (2021). Global formal live poultry and hatching egg trade network (2004–2016): description and association with poultry disease reporting and presence. Poult. Sci. 100:101322. doi: 10.1016/j.psj.2021.101322
Balpetek Külcü, D., Gökışık, C. D., and Aydın, S. (2019). An investigation of antibacterial and antioxidant activity of nettle (Urtica dioica L.), mint (Mentha piperita), thyme (thyme serpyllum) and Chenopodium album L. plants from Yaylacık plateau, Giresun, Turkey. Turk. J. Agric. Food Sci. Technol. 7:73. doi: 10.24925/turjaf.v7i1.73-80.2123
Bashir, S., Arshad, M. S., Khalid, W., Nayik, G. A., Al Obaid, S., Ansari, M. J., et al. (2022). Effect of antimicrobial and antioxidant rich pomegranate peel based edible coatings on quality and functional properties of chicken nuggets. Molecules 27:4500. doi: 10.3390/molecules27144500
Bekhit, A. E. D. A., Holman, B. W., Giteru, S. G., and Hopkins, D. L. (2021). Total volatile basic nitrogen (TVB-N) and its role in meat spoilage: A review. Trends Food Sci. Technol. 109, 280–302.
Benzie, I. F., and Strain, J. J. (1996). The ferric reducing ability of plasma (FRAP) as a measure of “antioxidant power”: the FRAP assay. Anal. Biochem. 239, 70–76. doi: 10.1006/abio.1996.0292
Bimakr, M., Ganjloo, A., Zarringhalami, S., and Ansarian, E. (2017). Ultrasound-assisted extraction of bioactive compounds from Malva sylvestris leaves and its comparison with agitated bed extraction technique. Food Sci. Biotechnol. 26, 1481–1490. doi: 10.1007/s10068-017-0229-5
Bouarab-Chibane, L., Ouled-Bouhedda, B., Leonard, L., Gemelas, L., Bouajila, J., Ferhout, H., et al. (2017). Preservation of fresh ground beef patties using plant extracts combined with a modified atmosphere packaging. Eur. Food Res. Technol. 243, 1997–2009. doi: 10.1007/s00217-017-2905-3
Brassó, L. D., Szabó, V., Komlósi, I., Pusztahelyi, T., and Várszegi, Z. (2021). Preliminary study of slaughter value and meat characteristics of 18 months ostrich reared in Hungary. Agriculture 11:885. doi: 10.3390/agriculture11090885
Bu, X., Wang, H., Wang, Y., Ojangba, T., Nan, H., Zhang, L., et al. (2023). Effects of iron-catalyzed oxidation and methemoglobin oxidation systems on endogenous enzyme activity and myofibrillar protein degradation in yak meat. Food Chem. 404:134647. doi: 10.1016/j.foodchem.2022.134647
Carpentieri, S., Soltanipour, F., Ferrari, G., Pataro, G., and Donsì, F. (2021). Emerging green techniques for the extraction of antioxidants from Agri-food by-products as promising ingredients for the food industry. Antioxidants 10:1417. doi: 10.3390/antiox10091417
Chacha, J. S., Zhang, L., Ofoedu, C. E., Suleiman, R. A., Dotto, J. M., Roobab, U., et al. (2021). Revisiting non-thermal food processing and preservation methods—action mechanisms, pros and cons: a technological update (2016–2021). Food Secur. 10:1430. doi: 10.3390/foods10061430
Chamkhi, I., Charfi, S., El Hachlafi, N., Mechchate, H., Guaouguaou, F. E., El Omari, N., et al. (2022). Genetic diversity, antimicrobial, nutritional, and phytochemical properties of Chenopodium album: a comprehensive review. Food Res. Int. 154:110979. doi: 10.1016/j.foodres.2022.110979
Choudhary, N., Chatterjee, M., Kumar, S., Singh, G., and Suttee, A. (2021). Effect of conventional method and microwave assisted extraction on phytoconstituents of Chenopodium album. Mater. Today 45, 5362–5367. doi: 10.1016/j.matpr.2021.01.947
Ford, S., Ilgaz, F., Hawker, S., Cochrane, B., Hill, M., Ellerton, C., et al. (2023). Amino Acid Analyses of Plant Foods Used in the Dietary Management of Inherited Amino Acid Disorders. Nutr. 15:2387.
Gastaldello, A., Giampieri, F., De Giuseppe, R., Grosso, G., Baroni, L., and Battino, M. (2022). The rise of processed meat alternatives: a narrative review of the manufacturing, composition, nutritional profile and health effects of newer sources of protein, and their place in healthier diets. Trends Food Sci. Technol. 127, 263–271. doi: 10.1016/j.tifs.2022.07.005
Glamoclija, N., Starcevic, M., Janjic, J., Ivanovic, J., Boskovic, M., Djordjevic, J., et al. (2015). The effect of breed line and age on measurements of pH-value as meat quality parameter in breast muscles (m. pectoralis major) of broiler chickens. Procedia Food Sci. 5, 89–92.
Gouda, M., Bekhit, A. E. D., Tang, Y., Huang, Y., Huang, L., He, Y., et al. (2021). Recent innovations of ultrasound green technology in herbal phytochemistry: a review. Ultrason. Sonochem. 73:105538. doi: 10.1016/j.ultsonch.2021.105538
Horbańczuk, O. K., Moczkowska, M., Marchewka, J., Atanasov, A. G., and Kurek, M. A. (2019). The composition of fatty acids in ostrich meat influenced by the type of packaging and refrigerated storage. Molecules 24:4128. doi: 10.3390/molecules24224128
Kainat, S., Arshad, M. S., Khalid, W., Zubair Khalid, M., Koraqi, H., Afzal, M. F., et al. (2022). Sustainable novel extraction of bioactive compounds from fruits and vegetables waste for functional foods: a review. Int. J. Food Prop. 25, 2457–2476. doi: 10.1080/10942912.2022.2144884
Kang, S. N. (2016). Ethanol extracts from mistletoe (Viscum album L.) act as natural antioxidants and antimicrobial agents in uncooked pork patties during refrigerated storage. Asian Australas. J. Anim. Sci. 29:109. doi: 10.5713/ajas.15.0253
Khalid, W., Arshad, M. S., Yasin, M., Imran, A., and Ahmad, M. H. (2021). Quality characteristics of gamma irradiation and kale leaf powder treated ostrich and chicken meat during storage. Int. J. Food Prop. 24, 1335–1348. doi: 10.1080/10942912.2021.1963274
Khalid, W., Farhan, M., Arif, M. A., Rehan, M., Jabeen, A., Khalid, M. Z., et al. (2020). Chemical composition, antioxidants and phenolic contents of Aloe vera: using as a medicinal purpose against various diseases. Int. J. Biosci. 17, 143–151.
Khalid, W., Maqbool, Z., and Arshad, M. S. (2022). Recent advances and innovation in meat with reference to processing technologies. doi: 10.5772/intechopen.108620
Khan, M. K. I., Ghauri, Y. M., Alvi, T., Amin, U., Khan, M. I., Nazir, A., et al. (2021). Microwave assisted drying and extraction technique; kinetic modelling, energy consumption and influence on antioxidant compounds of fenugreek leaves. Food Sci. Technol. 42:e56020. doi: 10.1590/fst.56020
Khan, I. A., Xu, W., Wang, D., Yun, A., Khan, A., Zongshuai, Z., et al. (2020). Antioxidant potential of chrysanthemum morifolium flower extract on lipid and protein oxidation in goat meat patties during refrigerated storage. J. Food Sci. 85, 618–627. doi: 10.1111/1750-3841.15036
Khattak, K. F. (2011). Nutrient composition, phenolic content and free radical scavenging activity of some uncommon vegetables of Pakistan. Pak. J. Pharm. Sci. 24.
Khodanazary, A., and Mohammadzadeh, B. (2023). Effect of alginate-gallic acid coating on freshness and flavor properties of mackerel (Scomberomorus commerson) fillets under refrigerated storage. Int. J. Biol. Macromol. 249:125999. doi: 10.1016/j.ijbiomac.2023.125999
Khomarlou, N., Aberoomand-Azar, P., Lashgari, A. P., Hakakian, A., and Ayatollahi, S. A. (2017). Evaluation of antibacterial activity against multidrug-resistance (Mdr) bacteria and antioxidant effects of the ethanolic extract and fractions of Chenopodium Album (sub Sp striatum). Int. J. Pharm. Sci. Res. 8:14. doi: 10.13040/IJPSR.0975-8232.8(9).3696-08
Lone, B. A., Chishti, M. Z., Bhat, F. A., Tak, H., Bandh, S. A., and Khan, A. (2017). Evaluation of anthelmintic antimicrobial and antioxidant activity of Chenopodium album. Trop. Anim. Health Prod. 49, 1597–1605. doi: 10.1007/s11250-017-1364-y
Manap, K., and Serikkyzy, M. (2023). Production of ostrich meat pâtés: design of a food safety management system. Food Sci. Technol. Int. 29, 847–856. doi: 10.1177/10820132221124195
Mas-Bargues, C., Escriva, C., Dromant, M., Borras, C., and Vina, J. (2021). Lipid peroxidation as measured by chromatographic determination of malondialdehyde. Human plasma reference values in health and disease. Arch. Biochem. Biophys. 709:108941. doi: 10.1016/j.abb.2021.108941
Meilgaard, M., Civille, G. V., and Carr, B. T. (2007). Overall difference tests: does a sensory difference exist between samples. Sens. Eval. Tech. 4, 63–104. doi: 10.1201/b16452
Mejía-Méndez, J. L., Navarro-López, D. E., Sanchez-Martinez, A., Ceballos-Sanchez, O., Garcia-Amezquita, L. E., Tiwari, N., et al. (2024). Lanthanide-doped ZnO nanoparticles: Unraveling their role in cytotoxicity, antioxidant capacity, and nanotoxicology. Antioxidants 13:213. doi: 10.3390/antiox13020213
Mir, N. A., Riar, C. S., and Singh, S. (2019). Physicochemical, molecular and thermal properties of high-intensity ultrasound (HIUS) treated protein isolates from album (Chenopodium album) seed. Food Hydrocoll. 96, 433–441. doi: 10.1016/j.foodhyd.2019.05.052
Parkash, J., and Patel, K. R. (2015). Hepatoprotective activity of Chenopodium album leaves extract in CCl4 induced hepatotoxicity in rats. J. Drug Deliv. Ther. 5, 88–93. doi: 10.22270/jddt.v5i2.1063
Pérez-Torres, I., Castrejón-Téllez, V., Soto, M. E., Rubio-Ruiz, M. E., Manzano-Pech, L., and Guarner-Lans, V. (2021). Oxidative stress, plant natural antioxidants, and obesity. Int. J. Mol. Sci. 22:1786. doi: 10.3390/ijms22041786
Poonia, A. (2020). “Bioactive compounds of fat-hen (Chenopodium album L.)” in Bioactive compounds in underutilized vegetables and legumes. eds. H. N. Murphy and K. Y. Paek (Cham: Springer), 1–11.
Qiao, L., Tang, X., and Dong, J. (2017). A feasibility quantification study of total volatile basic nitrogen (TVB-N) content in duck meat for freshness evaluation. Food Chem. 237, 1179–1185. doi: 10.1016/j.foodchem.2017.06.031
Reddy, A. V. B., Moniruzzaman, M., Madhavi, V., and Jaafar, J. (2020). Recent improvements in the extraction, cleanup and quantification of bioactive flavonoids. Stud. Nat. Prod. Chem. 66, 197–223. doi: 10.1016/B978-0-12-817907-9.00008-8
Saini, R., Kumar, D., and Mittal, A. (2019). Antimicrobial and phytochemical potential of Chenopodium album Linn. Int. J. Sci. Technol. Res. 8, 877–880.
Salman, S., Dinse, D., Khol-Parisini, A., Schafft, H., Lahrssen-Wiederholt, M., Schreiner, M., et al. (2013). Colostrum and milk selenium, antioxidative capacity and immune status of dairy cows fed sodium selenite or selenium yeast. Arch. Anim. Nutr. 67, 48–61. doi: 10.1080/1745039X.2012.755327
Shakhes, S. A., Wasim, W. A., Nasiry, Z., and Tookhy, N. A. (2023). Coliform contamination of raw beef at the slaughterhouse and butchery levels in Herat City, Afghanistan. NUIJB 2, 137–144.
Singh, K. P., Kumar, A., and Dhak, G. (2011). Revaluation of antibacterial activities of chenopodium album L. Int. J. Applied Biolo. Pharm. Technol. 2, 398–401.
Singh, S., Singh, A., Hallan, S. S., Brangule, A., Kumar, B., and Bhatia, R. (2023). A compiled update on nutrition, phytochemicals, processing effects, analytical testing and health effects of Chenopodium album: a non-conventional edible plant (NCEP). Molecules 28:4902. doi: 10.3390/molecules28134902
Song, Z., Cao, Y., Zhang, Y., Zhang, Z., Shi, X., Zhang, W., et al. (2022). Effects of storage methods on the microbial community and quality of Sichuan smoked bacon. LWT 158:113115. doi: 10.1016/j.lwt.2022.113115
Steel, R., and Torrie, J. (2012). Principles and procedures of statistics: a biometrical approach. Revi. Veteri. 13:481. Toronto: MCGraw-Hill Book Company.
Tang, H., Darwish, W. S., El-Ghareeb, W. R., Al-Humam, N. A., Chen, L., Zhong, R. M., et al. (2020). Microbial quality and formation of biogenic amines in the meat and edible offal of camelus dromedaries with a protection trial using gingerol and nisin. Food Sci. Nutr. 8, 2094–2101. doi: 10.1002/fsn3.1503
Tateo, A., Maggiolino, A., Domínguez, R., Lorenzo, J. M., Dinardo, F. R., Ceci, E., et al. (2020). Volatile organic compounds, oxidative and sensory patterns of vacuum aged foal meat. Animals 10:1495. doi: 10.3390/ani10091495
Turan, E., and Şimşek, A. (2021). Effects of lyophilized black mulberry water extract on lipid oxidation, metmyoglobin formation, color stability, microbial quality and sensory properties of beef patties stored under aerobic and vacuum packaging conditions. Meat Sci. 178:108522. doi: 10.1016/j.meatsci.2021.108522
Umar, M. F., Ahmad, F., Saeed, H., Usmani, S. A., Owais, M., and Rafatullah, M. (2020). Bio-mediated synthesis of reduced graphene oxide nanoparticles from chenopodium album: their antimicrobial and anticancer activities. Nano 10:1096. doi: 10.3390/nano10061096
Usman, I., Hussain, M., Imran, A., Afzaal, M., Saeed, F., Javed, M., et al. (2022). Traditional and innovative approaches for the extraction of bioactive compounds. Int. J. Food Prop. 25, 1215–1233. doi: 10.1080/10942912.2022.2074030
Xu, Z., Ye, Z., Li, Y., Li, J., and Liu, Y. (2020). Comparative study of the oxidation stability of high oleic oils and palm oil during thermal treatment. J. Oleo Sci. 69, 573–584. doi: 10.5650/jos.ess19307
Yildiz, G., Izli, G., and Aadil, R. M. (2020). Comparison of chemical, physical, and ultrasound treatments on the shelf life of fresh-cut quince fruit (Cydonia oblonga mill.). J. Food Process. Preserv. 44:e14366. doi: 10.1111/jfpp.14366
Keywords: ultrasound-assisted extraction (UAE), Chenopodium album , clean-label, ostrich meat, microwave-assisted extraction (MAE)
Citation: Khalid MZ, Saima H, Nadeem MT, Saeed K, Arshad MS, Khalid W, Rafique H, Fatima M, Alsulami T and Alzahrani A (2024) Clean label extraction of bioactive compounds from Chenopodium album and their role in the characterization and stability of ostrich meat. Front. Sustain. Food Syst. 8:1472110. doi: 10.3389/fsufs.2024.1472110
Edited by:
Tahmeena Khan, Integral University, IndiaReviewed by:
Tabussam Tufail, Jiangsu University, ChinaSeydi Yıkmış, Namik Kemal University, Türkiye
Copyright © 2024 Khalid, Saima, Nadeem, Saeed, Arshad, Khalid, Rafique, Fatima, Alsulami and Alzahrani. This is an open-access article distributed under the terms of the Creative Commons Attribution License (CC BY). The use, distribution or reproduction in other forums is permitted, provided the original author(s) and the copyright owner(s) are credited and that the original publication in this journal is cited, in accordance with accepted academic practice. No use, distribution or reproduction is permitted which does not comply with these terms.
*Correspondence: Hafiza Saima, ZHJoYWZpemFzYWltYUBnY3VmLmVkdS5waw==; Waseem Khalid, d2FzZWVtLmtoYWxpZEB1aWZzdC51b2wuZWR1LnBr; Muhammad Zubair Khalid, enViYWlya2hhbGlkNzMwQGdtYWlsLmNvbQ==