- 1Department of Agronomy, N. M. College of Agriculture, Navsari Agricultural University, Navsari, India
- 2Department of Soil Science and Agricultural Chemistry, N. M. College of Agriculture, Navsari Agricultural University, Navsari, India
- 3Division of Genetics and Plant Breeding, ICAR-Indian Agricultural Research Institute, New Delhi, India
- 4Division of Agricultural Engineering, ICAR-Indian Agricultural Research Institute, New Delhi, India
- 5Krishi Vigyan Kendra, ICAR-Central Institute of Agricultural Engineering, Bhopal, India
- 6Department of Biology, Faculty of Science and Arts, King Khalid University, Abha, Saudi Arabia
- 7Soil Microbiology Research Department, Soils, Water, and Environment Research Institute (SWERI), Agriculture Research Center (ARC), Giza, Egypt
- 8Civil Engineering Department, Faculty of Engineering, Minia University, Minya, Egypt
- 9Structural Diagnostics and Analysis Research Group, Faculty of Engineering and Information Technology, University of Pécs, Pécs, Hungary
- 10Agricultural Engineering Department, Faculty of Agriculture, Mansoura University, Mansoura, Egypt
- 11Environmental Science Department, Faculty of Science, Damietta University, Damietta, Egypt
In a field trial at the College Farm, NAU, Navsari (Gujarat), evaluated four main plot treatments (T1: Green gram, T2: Cowpea, T3: Dhaincha, and T4: Fallow), which were grown during the summer with three replications following a randomized block design (RCBD). Green gram and cowpea were incorporated into the soil after harvest, but dhaincha was incorporated at 50% flowering. During Kharif, each main plot was split into six smaller plots with different treatments: W1 was 100% RDF, W2 was 75% RDF, W3 was 50% RDF, W4 was 75% RDF + 25% N from FYM, W5 was 50% RDF + 50% N from FYM, and W6 was no fertilizer. The results of all 24 treatment combinations were repeated three times in a split-plot design. The analysis showed significant growth, yield attributes, grain yield, and straw yield of rice in dhaincha-incorporated plots (T3), fb greengram (T1), and cowpea (T2) plots using 100% RDF, while fallow (T4) with no fertilizer application recorded significantly lower values. SPAD meter readings of rice were higher in W4 (75% RDF + 25% N from FYM), which was at par with W1 in dhaincha-incorporated plots (75% RDF + 25% N from FYM) (W4). However, applying no fertilizer (W6) resulted in lower values. The total uptake of nitrogen and phosphorus in rice was highest when it was grown in dhaincha incorporated (T3), followed by green gram (T1), cowpea (T2) incorporated plots with the usage of 100% RDF (W1) and 75% RDF + 25% N from FYM (W4), and lower values were recorded in fallow + no fertilizer treatment (T4W6). Our study revealed that incorporating summer legumes before planting rice significantly increased plant height, tillers, grain and straw yield, and total uptake. With dhaincha, inclusion has shown a greater advantage.
1 Introduction
Rice (Oryza sativa L.) is a fundamental dietary staple for over half of the global populus (Irshad et al., 2022; Yang et al., 2021). Given the current global population of 7.3 billion, projections indicate an increase to 8.5 and 9.7 billion between 2030 and 2050, respectively, with a stabilization at 11.2 billion by the end of the 21st century (UNDESA, 2015; Shen et al., 2024). This demographic shift necessitates a substantial increase in food grain production often at the expense of already scarce land and water resources (Xiao et al., 2024). Achieving this heightened demand for food and nutrition for an additional 3–4 billion people may require a doubling of energy and fertilizer usage within intensive agricultural practices (Sulieman and Tran, 2015; Huang et al., 2019; Wang et al., 2020). Unlike conventional green manures, grain legumes offer protein-rich seeds that augment income and combat protein deficiency while simultaneously reducing the need for mineral nitrogen in rice-based cropping systems (Radhakumari and Srinivasulureddy, 2010). Cropping systems integrate legumes to improve soil fertility, supply animal feed, and act as a direct source of human food (Meena et al., 2015). Mathematical modeling studies have indicated that legume based crop rotations can deliver environmental and economic benefits (Reckling et al., 2016). Depending on the crop’s age, nitrogen available in the soil, cropping strategy, and crop species, legumes can supply up to 73% of the nitrogen needed by cereals (Thilakarathna et al., 2016; Reilly et al., 2022). In order to achieve long-term release, intercropping intermediate wheatgrass (IWG) with perennial legumes can decrease nitrogen inhibition and improve the soil’s total nitrogen pool while avoiding issues associated with fertilizer use (Ashworth et al., 2015). The legume-cereal intercropping system can also improve soil carbon retention and nitrogen usage efficiency, which can lower nitrate leaching and have a positive impact on the environment. Modern agriculture faces two pressing sustainability challenges: soil degradation and the depletion of organic matter. Furthermore, the decreasing productivity of many tropical soils prevents crops from reaching their full genetic potential (Zhang et al., 2023; Qiu et al., 2023; Ban et al., 2024). In this context, the importance of organic manure is resurging. However, the substantial quantities of organic materials required to meet crop nutrient demands may pose financial challenges for small or low-income farmers. As an alternative approach, farmers can utilize crop residues from previous harvests to address soil fertility issues and it can be favorable practice (Dai et al., 2023). For instance, India generates substantial amounts of crop residues annually, totaling 500 million metric tons, albeit with considerable regional variation (Anonymous, 2016). Effective crop residue management can promote increased microbial populations, their activities, and subsequent nutrient transformations, in addition to modifying the soil environment (Singh et al., 2005). Nawaz et al. (2019) found incorporation of crop residues strengthens the soil, helps in nutrient recycling and improves the soil health by increasing the soil organic matter. Improved crop residue management enhances soil nutrient availability, minimizes erosion, enhances soil structure, and augments soil water retention capacity through elevated soil organic matter content, thereby directly elevating crop yields (Singh et al., 2005). Singh et al. (2018) reported that incorporating crop residues into the soil led to increased 1,000 grain weight, grain yield, straw yield, harvest index, and benefit–cost ratio in subsequent rice crop. Similarly, Reshma et al. (2019) found that residual effects from summer-grown fodder cowpea enhanced the yield of the succeeding rice crop. Efficient resource utilization, which includes water, fertilizers, and other reserves within a cropping system, depends on effective crop residue management. Given the escalating demand for fertilizers to meet nutrient requirements, it is advisable to concurrently apply chemical and organic fertilizers to fulfill rice’s nutritional needs while preserving soil health (Amgain et al., 2022a) and optimizing production. To find out if legumes can help increase rice production and to learn more about the role of crop residues in systems that use legumes to grow cereals, we oversaw a test to see how addition of summer legume residues into the soil and applying specific fertilizer regimes will improve rice growth, increase yield, and enhance nutrient uptake compared to using only synthetic fertilizers or no residue incorporation in Kharif rice.
2 Materials and methods
2.1 Conduct of experiment
During the summer and kharif seasons of 2021 and 2022, a field trial was superintended at the College Farm, NAU, Navsari (Gujarat), where the soil of the investigated field exhibited a clayey texture, moderate organic carbon content, low readily available nitrogen, moderate P2O5, and high readily available K2O. The soil reaction was slightly alkaline. The experiment comprised four main plot treatments: T1 (Green gram), T2 (Cowpea), T3 (Dhaincha), and T4 (Fallow), which were drilled in the summer season and replicated threefold in a randomized block design. Green gram (T1) and cowpea (T2) leftovers from summer legume crops were added to the plots after harvest, while dhaincha (T3) was added to the soil of the respective plot when it had flowered 50%. A main plot in the kharif season was divided into six sub-plot treatments representing disparate levels of the specified fertilizer dose for kharif rice: W1 (100% RDF: 100 N + 30 P2O5 + 00 K2O kg/ha), W2 (75% RDF), W3 (50% RDF), W4 (75% RDF + 25% N from FYM), W5 (50% RDF + 50% N from FYM), and W6 (no fertilizer application). This resulted in 24 treatment composites replicated threefold in a split-plot design to determine whether they had an whether they had an impact on the rice crop or not. The meteorological and soil conditions observed during the experiment, as well as the requirements for rice production, were identical. The crop is also free from major pests and diseases during the crop growth period, and the variety chosen was GNR-3. Regular biometric observations were conducted at specified intervals by randomly selecting five plants from each treatment. At maturity, the crop was harvested, and growth parameters along with yield traits were inscribed in the net plots.
2.2 Source of fertilizers used in the study
The source of nitrogen (N) is urea. A single superphosphate is the source of phosphorus. Potassium was not applied because the recommended dose for this region is zero. The farmyard manure (FYM) employed in this experiment was a combination of cattle FYM, cattle urine, and an assortment of other organic materials used as livestock bedding, as well as straw and plant stalk residue provided to the animals. The recovered FYM was allowed to decay entirely for 5 months. It was then applied to the targeted plots via broadcasting 15 days before transplanting. The chemical makeup of the FYM utilized in the experiment is shown in Table 1. Table 2 shows the nutrient status after harvest of summer legumes (just at the time of incorporation) and before sowing kharif rice.
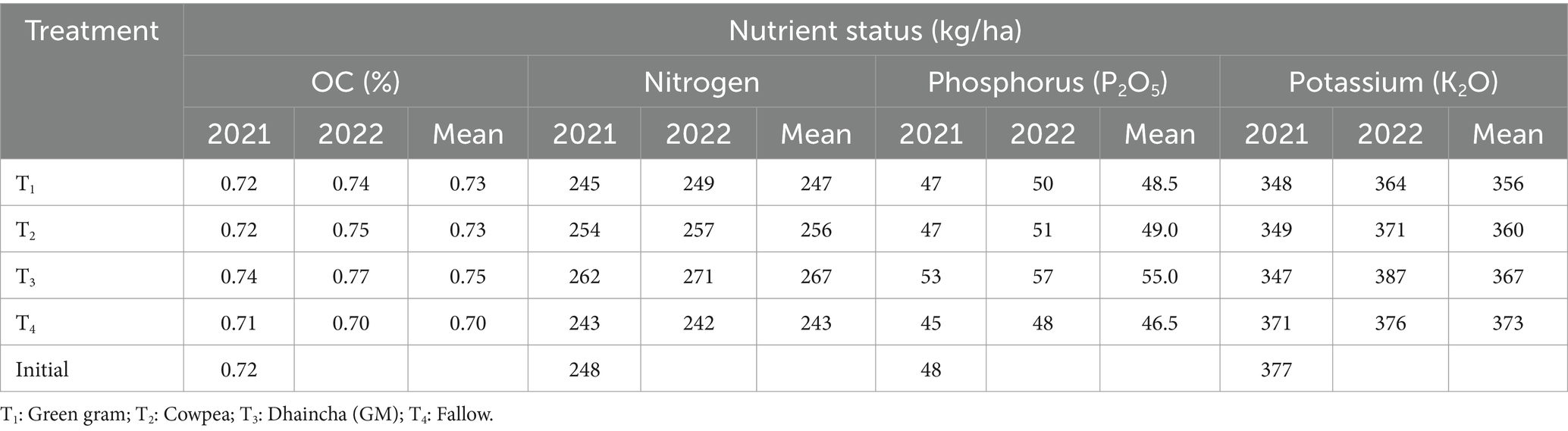
Table 2. Nutrient status after harvest of summer legumes (just at the time of incorporation) and before sowing kharif rice.
2.3 Phenotypic observations
2.3.1 Plant height
For each of the five labeled hills, the plant height (cm) was determined in centimeters from the base to the tip of the topmost fully opened leaf at harvest. The results were averaged per hill and expressed in centimeters.
2.3.2 Plant dry matter
Five successive plants were sampled, and to attain a consistent weight, these samples were first dried in the shade and then dried in a hot-air oven at 65°C for 24 h. In each treatment, the mean dry matter/hill was calculated by adding the recorded sample dry weights (g/plant).
2.3.3 SPAD meters readings (chlorophyll content)
The IRRI in the Philippines developed SPAD (the Soil Plant Analysis Development Chlorophyll Meter), a plant analysis method and diagnostic tool for measuring rice crop nitrogen status. SPAD readings were taken at 30, 60, and 90 days after transplantation (DAT), and at harvest, SPAD from the first completely developed leaf at the top of the plant was determined by inserting the central region of the leaf into the slit of the SPAD meter.
2.3.4 Productive tillers
The sum of tillers was assessed within the net plot area using a 1 m2 quadrat at harvest, expressed as tillers per square meter. Additionally, we enumerated, aggregated, and averaged the ear-bearing tiller density (productive tillers) from all five designated hills to determine the mean number of productive tillers per hill.
2.3.5 Yield
Harvesting rice began shortly after physiological maturity. The grain was manually threshed, cleaned, and sun-dried to a consistent weight before the ultimate yield was recorded. The yields of grain and straw from the designated hills were included in the net plot yield and reported in kg/ha.
2.4 Chemical analysis
Rice plant samples were collected during harvest from every plot and crushed in a Willey mill so that they could pass through a 40-mesh screen. To estimate P, plant materials were digested in a diacid HNO3:HCLO4 (9:4) mixture (Jackson, 1973). The ground material was collected in butter paper bags, and Jackson’s standard procedures were utilized to estimate the nitrogen content using Modified Kjeldahl’s method and the phosphorus content for grain and straw using the Vanadomolybdo phosphoric acid yellow color method (1973). Total nutrient intake is determined by adding the amounts of nitrogen and phosphorus absorbed from grain and straw, expressed in kg/ha.
The nutrient (NPK) uptake of rice (kg/ha) was worked out by using the following formula:
2.5 Statistical analysis
A statistical analysis was performed on the findings related to growth, yield, and yield components at harvest. Utilizing the statistical techniques outlined by Panse and Sukhatme (1967), the data on various factors were evaluated. The “F” test was used to compare how each treatment affected each of the characters under investigation. If disparateness between treatments was determined to be significant in the “F” test, the critical difference (CD) at 5% was calculated; otherwise, only the standard error of the mean was studied. The “F” statistic is then compared to a critical value from the F-distribution table, based on the degrees of freedom and the desired level of significance (e.g., 0.05). If the “F” value is greater than the critical value, it indicates that there are statistically significant differences between the group means. To ascertain precision, the coefficient of variation was studied for each character. Pooled analysis of the summer legumes and succeeding kharif rice was conducted for 2 years as per the procedure delineated by Cochran and Cox (1962). To probe the homogeneity of variation caused by error, Bartlett’s test was used. The variation resulting from the components of season X treatment was equated to the conjunct estimate of error variance. The statistically significant disparities between treatments and treatment means were found using ANOVA and the Duncans Multiple Range Test (DMRT), respectively. p ≤ 0.05 was the level of significance for all analyses.
3 Results
3.1 Growth attributes
3.1.1 Plant height and dry matter at harvest
At the time of harvest, we observed significantly greater plant height and dry matter production per hill in the rice crop when dhaincha was incorporated with green gram and cowpea. Compared with other nutrient levels, the application of 100% RDF (W1) produced better plant and dry matter, which was consistent with 75% RDF + 25% N from FYM.
In terms of dry matter production per hill, T3W1 outperformed T3W4, T3W5, and all other treatments. This treatment proved to be notably superior to T1, T2, and T4. Conversely, the lowest rice dry matter and plant height per hill were observed in plots left fallow during the summer season with no fertilizer application (Table 3).
3.1.2 Number of tillers at harvest
A higher tiller density per square meter was observed in plots where dhaincha was incorporated (Table 3). Conversely, a significantly lower number of tillers per square meter was noted at harvest in the T4 treatment. Use of the 100% recommended dose of fertilizer (W1) led to a substantial increment in the number of tillers per square meter. This performance was on par with the combination of a 75% recommended dose of fertilizer and 25% nitrogen from farmyard manure (W4), surpassing the control group, which received no fertilizer (W6). Furthermore, when considering the specific treatments, the highest tiller density per square meter was found in T3W1 (dhaincha +100% RDF), which exhibited a significant superiority over T4W6 (fallow + no fertilizer). Notably, the tiller density of T3W1 was comparable to that of T3W4, T1W1, and T2W1 (Figure 1; Table 3).
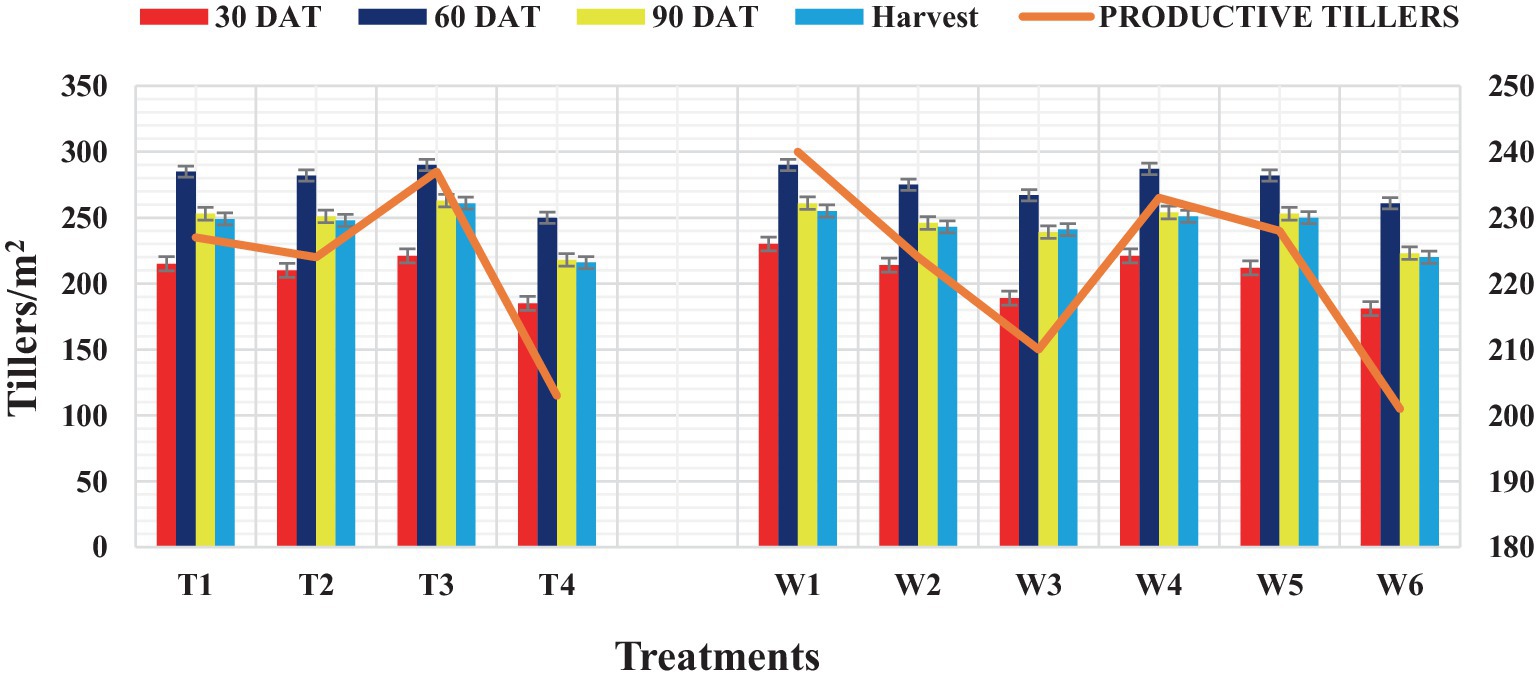
Figure 1. Number of tillers and productive tillers of Kharif rice as impacted by different treatments. Bar graphs represent the number of tillers at different intervals of transplanting, and the trendline across bar graphs represents the productive tillers at the respective intervals in each treatment. Error bars in bar graphs represent the standard error at the 5% level of significance.
3.1.3 Productive tillers
A greater number of productive tillers per m2 were observed in Dhaincha (T3) and W1 (100% RDF), which was noticeably superior to T4 (fallow) and W6 (no fertilizer) among the main and sub-plots, respectively. However, T3W1 was found to be on par with T3W4 and T1W1 and T2W1 (Table 3). A significantly lower number of productive tillers per m2 was found in T4W6 in the pooled study. There was no significant interaction effect with year in the pooled study (Table 3).
3.1.4 SPAD meter readings
Significantly higher SPAD meter readings in the leaves of rice were recorded in dhaincha (T3) incorporated plots than in T1, T2, and T4. However, the green gram (T1) incorporated plots were on par with cowpea (T2), and the significantly lower SPAD readings were recorded in rice grown in fallow plots (T4) at 30 DAT and 60 DAT of kharif rice (Figure 2). SPAD readings decreased at 90 DAT and at harvest. At 90 DAT, the SPAD readings were significantly greater in rice grown in dhaincha-incorporated (T3) plots, which remained at par with T1 treatment during the year 2021. Significantly lower SPAD readings were recorded in fallow treatment (T4) during both years and in a pooled study (Figure 2). At harvest, there was no significant difference in SPAD readings during both years of study. However, higher readings were recorded in dhaincha-incorporated plots (T3) than in green gram (T1) and cowpea (T2) incorporated plots. Lesser readings were recorded in the fallow treatment (T4). In pooled data, significant SPAD readings were recorded in dhaincha (T3), which remained statistically similar to treatment T1. Significantly lower readings were recorded in rice grown in fallow treatment (T4).
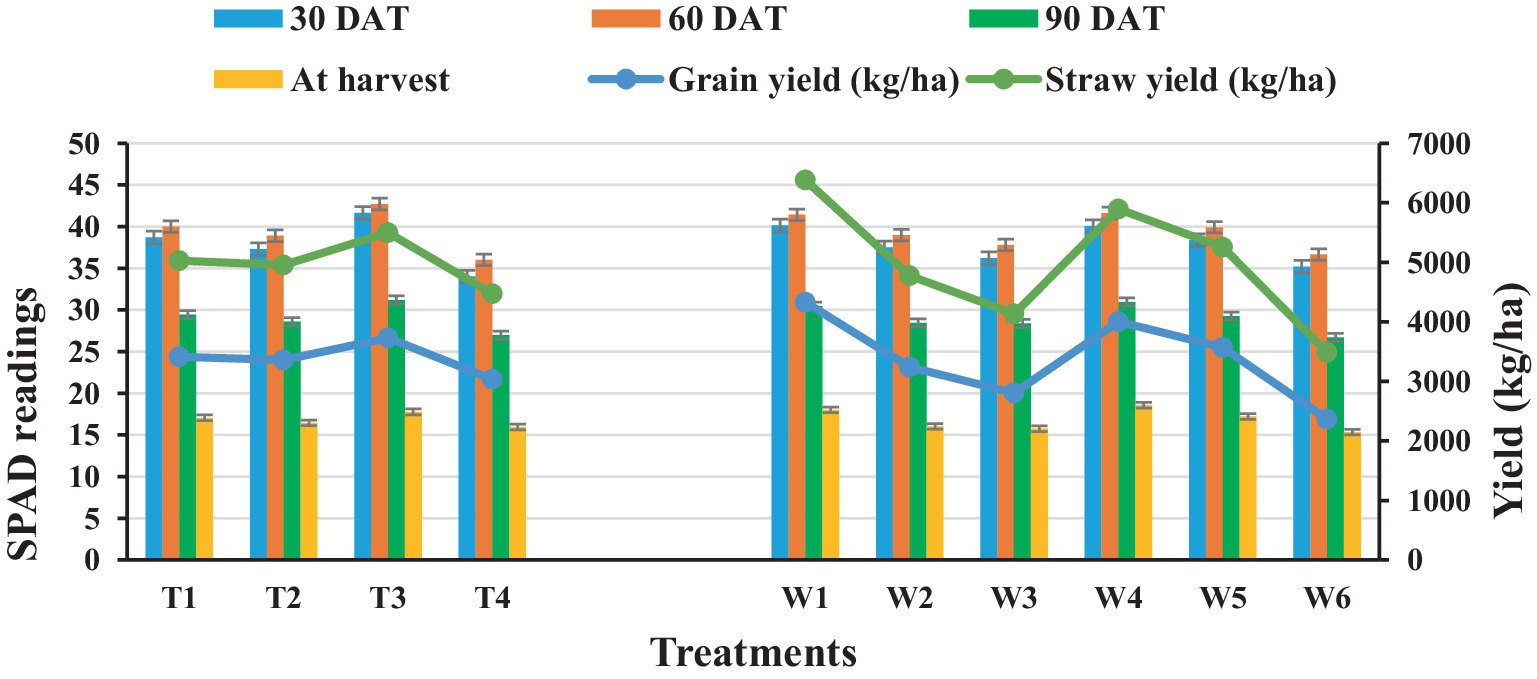
Figure 2. SPAD readings at different stages and their correlation to yield as influenced by different treatments. Bar graphs represent the grain yield (blue) and straw yield (light green) at different intervals of transplanting; the trendline across bar graphs represents the grain yield (blue) and straw yield (light green) at the respective intervals in each treatment. Error bars in bar graphs represent the standard error at the 5% level of significance.
The SPAD readings in the leaves exhibited a substantial increase when 75% RDF and 25% N from FYM (W4) were applied, not only at the 30, 60, and 90 DAT intervals but also at the time of harvest. This performance was on par with that of the 100% RDF (W1) treatment and was followed closely by the 50% RDF + 50% N from FYM (W5) in the combined dataset. Notably, during 2021, at both 30 and 60 DAT, treatment W5 also demonstrated SPAD readings that were comparable to those of treatment W4. However, at 90 DAT and during the harvest, the SPAD meter readings exhibited a decline compared to the readings at 60 DAT. Notably, the SPAD readings were significantly lower in the no-fertilizer treatment (W6) than in other treatments when the data were pooled for analysis (Figure 2).
3.2 Yield and yield attributes
3.2.1 Grains/panicle
Grains/panicle (T3W1, 123) were noticed more in rice grown in dhaincha (T3) incorporation plots with the use of 100% RDF (W1), followed by T3W4, T1W1, and the rest of the treatments. The lowest was recorded in T4W6 (72).
3.2.2 Filled grains per panicle
A substantially greater number of filled grains per panicle was observed in T3W1, followed by T3W4, T3W5, and other treatments. A lower number of filled grains per panicle was noticed in rice grown under fallow conditions with no fertilizer application (Table 4).
3.2.3 Grain yield
Kharif rice had a peak grain output of 3,732 kg/ha when it was planted after dhaincha (T3) incorporation, which was significantly superior to rice grown after the incorporation of other summer legumes, i.e., green gram (T1), cowpea (T2), and fallow plots (T4). The lowest grain yield of 3,036 kg/ha was noted in rice grown in fallow (T4) plots (Figure 3). Significantly substantial grain output of 4,329 kg/ha was witnessed with 100% RDF (W1), followed by 75% RDF + 25% N from FYM (W4), and 50% RDF + 50% N from FYM (W5) treatment. The lowest grain yield of 2,365 kg/ha was obtained in the no-fertilizer treatment (W6) (Figure 4). Finally, peak grain yield (4,688 kg/ha) was recorded in dhaincha incorporation with 100% RDF application (T3W1), followed by T1W1 and T2W1, and the lowest grain yield was recorded in fallow-no fertilizer treatment (2,167 kg/ha) (Figure 3).
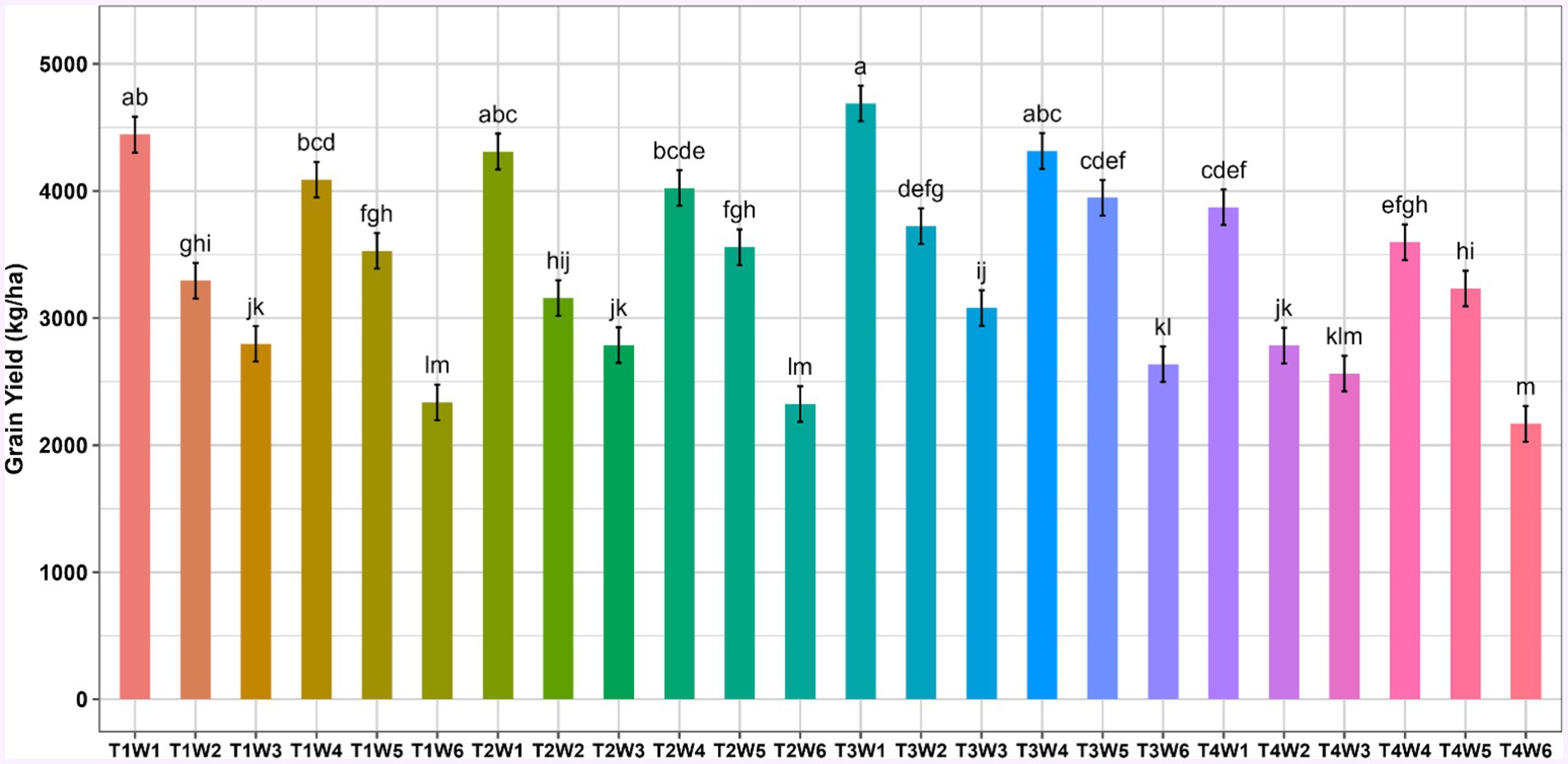
Figure 3. Grain yield of kharif rice as impacted by distinct treatments. Small alphabets in bar graphs represent the statistical distinctiveness among the treatments compared using DMRT at p ≤ 0.05.
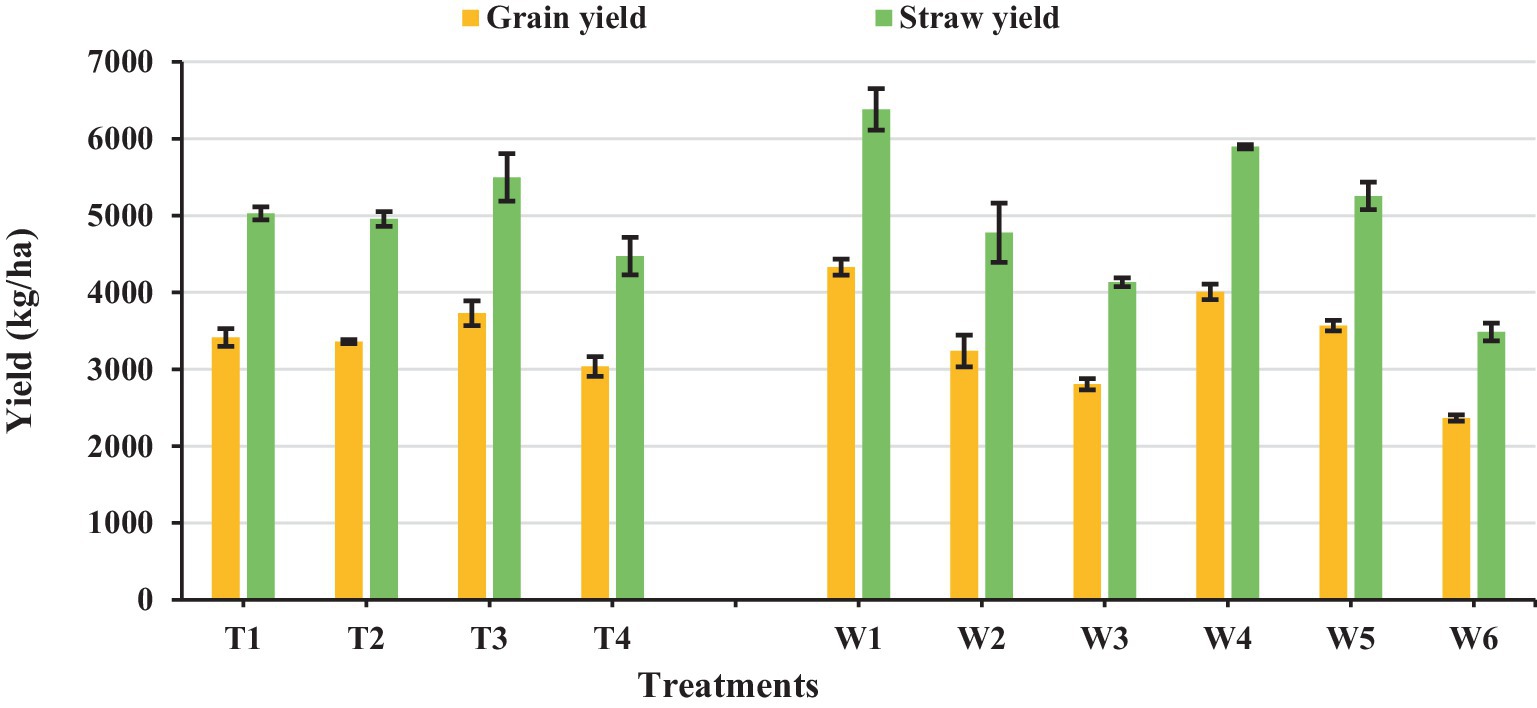
Figure 4. Grain and straw yield of Kharif rice as impacted by distinct treatments. Error bars define the standard error at 5% level of significance.
3.2.4 Straw yield
Significantly maximum straw yield (5,497 kg/ha) was recorded when crop was sown after incorporation of dhaincha (T3), which was significantly superior to green gram (T1, 5,029 kg/ha), cowpea (T2, 4,956 kg/ha), and summer fallow (T4, 4,472 kg/ha). Similarly, the straw yield obtained after the incorporation of green gram (T1) and cowpea (T2) was on par with each other, and both significantly exceeded the seeding of rice after the summer fallow (T4). Significantly lower straw yield was recorded in rice grown in fallow plots (T4, 4,472 kg/ha). Application of 100% RDF (W1) recorded a higher straw yield (6,383 kg/ha), which was found to be substantially better than other treatments and no-fertilizer application (W6) (3,487 kg/ha). The treatments differed significantly from each other and followed the trend of W1 > W4 > W5 > W2 > W3 > W6 (Figure 4). The incorporation of dhaincha (T3W1) produced a straw yield of 6,910 kg/ha, which was at par with T1W1 (6,544 kg/ha), and it was followed by T2W1, T3W4, and other treatments (Table 4). The lowest was noted in fallow treatment in the summer season and with no fertilizer application in kharif rice, i.e., T4W6, with 3,179 kg/ha (Table 4).
3.2.5 Total nutrient uptake of nitrogen and phosphorus
In our comprehensive study, we observed a noteworthy disparity in total nitrogen uptake among various treatments. The peak nitrogen uptake of 109.29 kg/ha was recorded in T3W1 (dhaincha +100%RDF), followed by treatments T1W1, T3W4, and T2W1, which also demonstrated substantial nitrogen uptake with no statistically significant differences among them. On the other hand, T4W6, representing fallow land with no fertilizer application, exhibited markedly lower total nitrogen uptake at just 36.55 kg/ha (Figure 5).
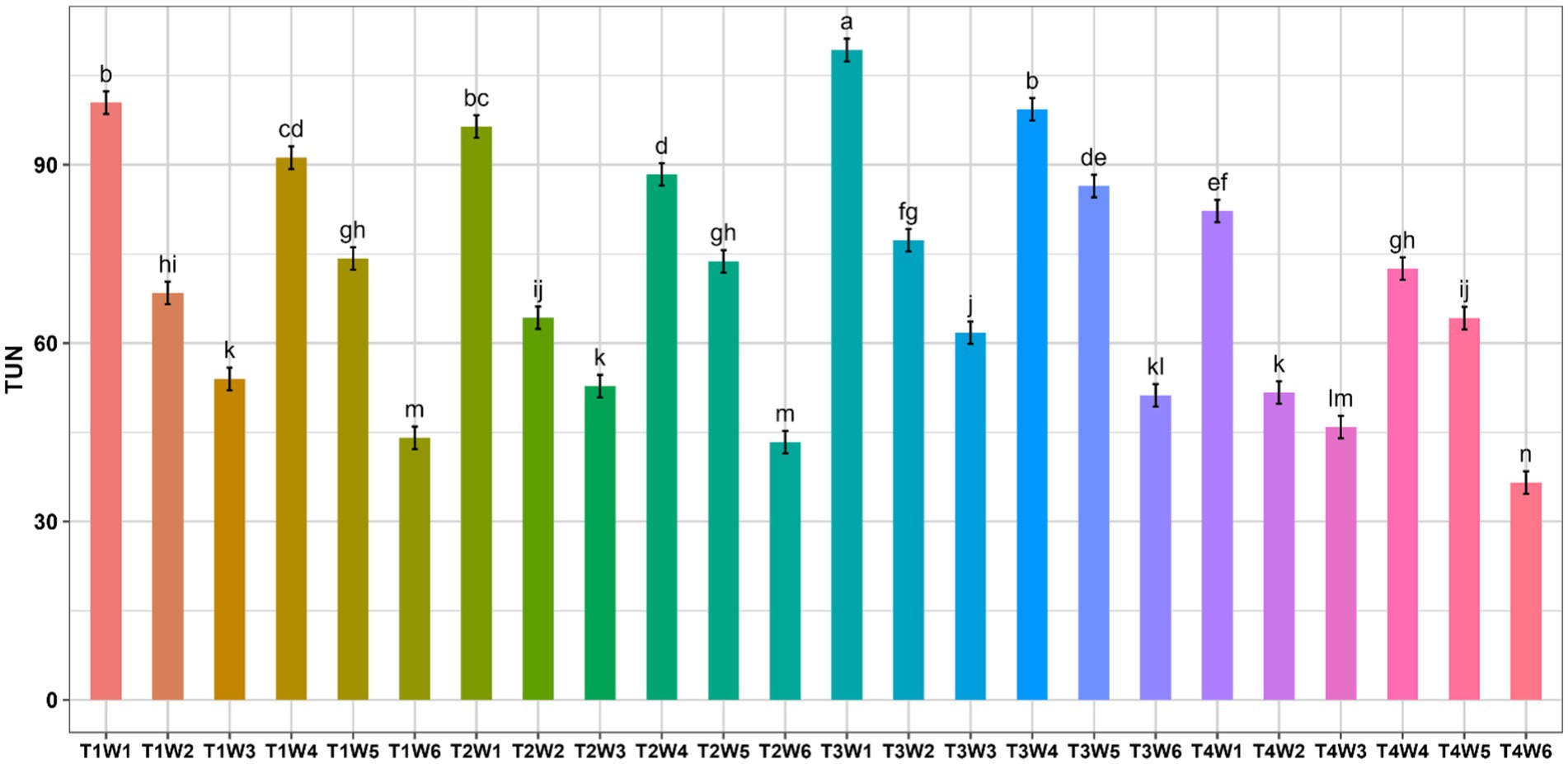
Figure 5. Total nitrogen uptake of kharif rice as impacted by different treatments. Small alphabets in bar graphs define the statistical distinctiveness among the treatments compared using DMRT at p ≤ 0.05.
We observed a distinct trend in the uptake of phosphorus. The treatment T3W1, once again, outperformed other treatments in terms of total phosphorus uptake, with a substantial quantity of 24.03 kg/ha. In comparison, T1W1, T2W1, and T3W4 also exhibited commendable phosphorus uptake, with no significant distinctions among them. In contrast, T4W6 displayed the lowest phosphorus uptake, totaling a mere 5.18 kg/ha. This outcome can be attributed to its status as fallow land with no fertilizer application (Figure 6).
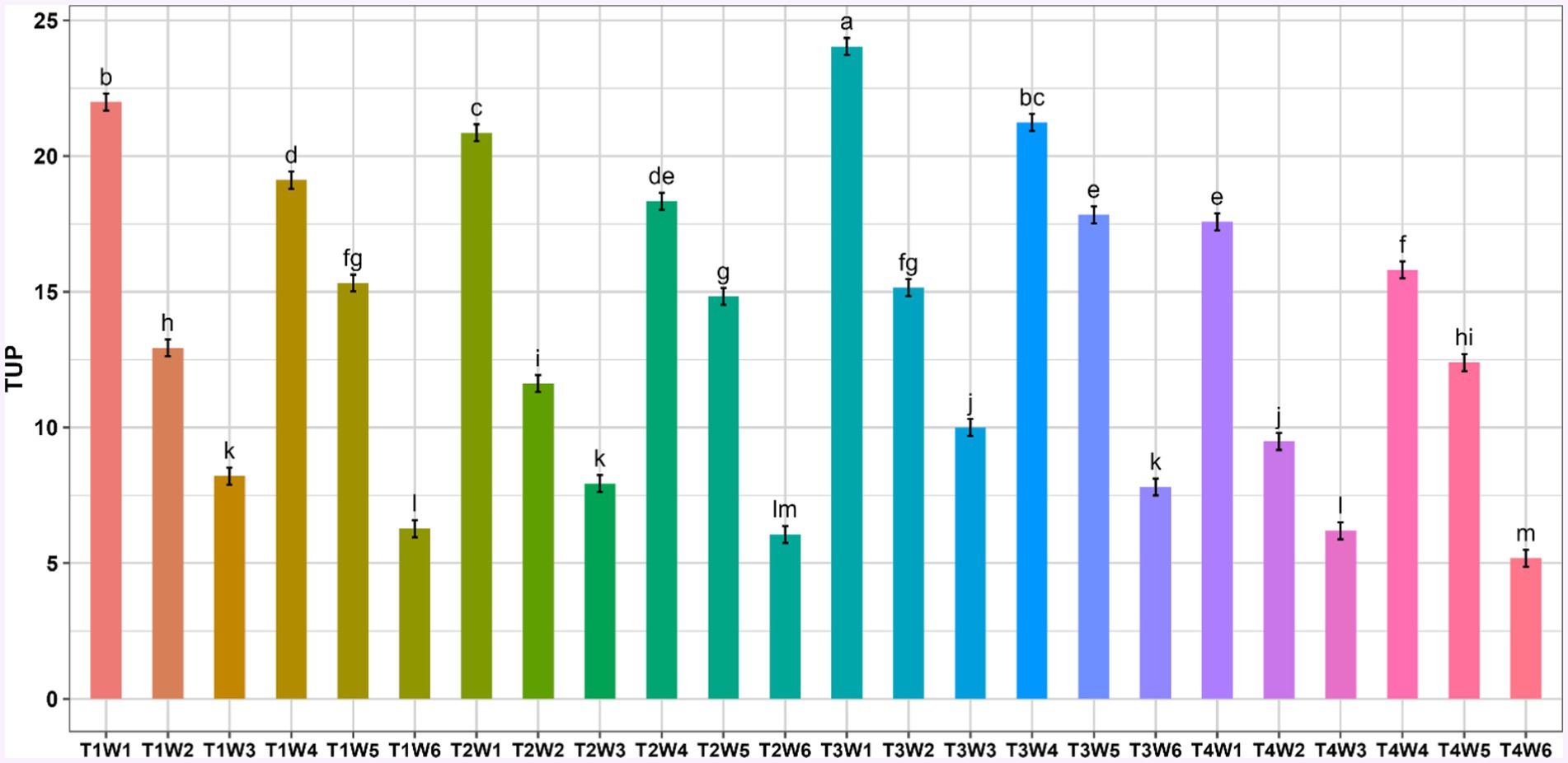
Figure 6. Total phosphorus uptake of kharif rice as impacted by distinct treatments. Small alphabets in bar graphs define the statistical distinctiveness among the treatments compared using DMRT at p ≤ 0.05.
4 Discussion
The study looked at what happened when leguminous green manure crops like green gram, cowpea, and dhaincha were planted on rice along with different amounts of inorganic fertilizers. It looked at how the growth, yield, and total uptake of nitrogen and phosphorus were affected. The aim was to preserve soil fertility and diminish the dependency on synthetic fertilizers. Arable cropping systems with legumes had 18% less nitrous oxide emission, 24% less N fertilizer use, no increase in nitrate leaching and no decrease in gross margin leading to sustainable production (Reckling et al., 2016).
4.1 Growth attributes
Compared to green gram and cowpea, dhaincha exhibited a remarkable capacity for biomass and residue production, showing synchronized decomposition rates. This feature likely facilitated the retention of substantial soil nutrients, meeting the requirements of Kharif rice. The incorporation of Farmyard Manure (FYM) further enriched both the biological and nutritional aspects of the soil, fostering an environment conducive to enhanced nutrient uptake by plants. Prathibha Sree et al. (2016) observed that this led to healthier plant growth and increased plant height. The subsequent rice crop also appeared to benefit from the nutrients released during the breakdown of these summer legumes, outcomes taller plants, as reported by Kumar et al. (2012). The heightened plant stature can be imputed to the pivotal role of nitrogen in the synthesis of essential components such as chlorophyll, proteins, RNA, DNA, and protoplasm. This could have led to increased cell division, elongation, and the sustained elevation of auxin levels at the meristematic region, essential for achieving greater plant height (Islam et al., 2014). Moreover, the increase in dry matter at the harvest of kharif rice following the incorporation of dhaincha (T3) compared to fallow (T4) was noteworthy, with a 28.22% improvement. Researchers Ramachandra et al. (2008) attribute this positive effect to the availability of soil nutrients during the decomposition of crop residues. Similarly, our study showed a substantial 74.51% uptick in dry matter at harvest of kharif rice with the use of W1 (100% RDF) as opposed to W6 (Fallow). This outcome supports the hypothesis put forth by Premalatha (2017), suggesting that favorable vegetative development can be attributed to the robust architectural support provided to the plants through a combination of organic and inorganic inputs.
The increase in the density of tillers observed in the FYM combination may be attributed to the rapid availability of nitrogen from chemical fertilizers, which is readily utilized by the crop initially, noted by Anitha and Mathew (2011). Subsequently, mineralization in the soil occurs due to the addition of FYM, a gradual and consistent process, as highlighted by Srivastava et al. (2013). This gradual release of nutrients is then harnessed by the crops, promoting vegetative growth and resulting in a higher number of tiller density per square meter. As a result, the application of 100% RDF (W1) demonstrated an equivalent effect to the combination of 75% RDF and 25% nitrogen from FYM (W4). However, a decline in the number of tillers density in rice was observed after 60 days after transplanting (DAT), specifically at 90 DAT and at the time of harvest. The allocation of increased resources and energy toward panicle and grain production could account for this decline. Sujatha et al. (2016) elucidated that farmers typically dry out inefficient tillers to redirect energy toward grain development and maximize seed production. Additionally, straw incorporation decreases the un-productive component of evapotranspiration and creates favorable root environment by improving soil structure, which provides a clean, uniform seedbed that facilitates crop establishment. The increased density of productive tillers per m2 may be due to the prolonged accessibility of nutrients through crop residue and FYM, owing to their slow-release nature. This effect led to rice crops achieving a similar number of productive tillers per square meter as with inorganic fertilizer application, despite the latter’s faster nutrient supply rate and the action of microorganisms in decomposing crop residue. The chlorophyll content exhibited an increase with plant age up to 60–70 DAT (Figure 2), followed by a decline irrespective of the treatment applied. Integration of summer legumes may have served as a nitrogen substitute and provided other essential plant nutrients, enabling rice to maintain higher chlorophyll levels. This observation aligns with the findings by Islam et al. (2019). There is a reduction in chlorophyll concentration after 70–75 days of transplantation, likely due to nutrient translocation from the green leaves to the developing grains. This phenomenon becomes more apparent during harvest, as photosynthates are redirected toward grain growth rather than leaf maintenance. As the rice crop matures, natural senescence sets in, resulting in the degradation of chlorophyll molecules in the leaves and, consequently, reduced SPAD meter readings at the time of harvest. The same aftereffect was reported by Xie et al. (2017). The observed maximum growth attributes are likely due to the improved availability of major and micronutrients in plots where biomass was incorporated and complemented with higher, balanced doses of inorganic fertilizers during the vegetative phase (Rajeshwari et al., 2007). The incorporation and decomposition of biomass likely facilitated the mobilization of nutrients from unavailable forms to available forms, as indicated by Balai et al. (2011). Another reason for the observed maximum growth attributes could be the increased number of leaves per plant in these treatments. This enhancement in leaf area, which measures the size of the plant’s assimilatory system and results from the combination of leaf length and width, is crucial for the effective accumulation and distribution of photosynthates to the plant’s economic parts (Sharan Kumar et al., 2017).
4.2 Yield and yield attributes
The higher grain count per panicle could be attributed to improved nutrient availability through the integration of organic and chemical fertilizers during panicle initiation and development. This support may have facilitated longer panicles with increased spikelet numbers, aligning with findings reported by Dalvi et al. (2017). Piccoli et al. (2020) established that, as a source of organic matter, incorporation of crop residues resulted in 12 and 16% higher yield in maize and sugar beet, respectively, than other sources. The incorporation of summer legumes enhanced the number of filled grains per panicle analogized to the control, indicating the necessity to improve nutrient translocation to the grain for greater filling (Neelima et al., 2007). Summer legumes applied plots’ continued access to nutrients to crop during subsequent stages may have enhanced photosynthesis of crop and greater transfer of photosynthates to grain for outshine in grain filling (Deshpande and Devasenapathy, 2011). Studies found that adding summer legume residues boosted grain filling and the amount of grains per panicle (Singh et al., 2009; Srivastava et al., 2013). When maize was rotated with legumes, maize productivity increased, suggesting improved soil water and fertility conditions (Chimonyo et al., 2019). The increased yield was mostly attributable to an increase in the number of panicles per unit area and the quantity of grains per panicle (Hu et al., 2023). Rice is particularly green in its early stages, and an appropriate food supply boosts photosynthetic rate, nutrient uptake, and, eventually, grain output (Naher and Paul, 2017). Nutrient concentration in fresh and dry matter accumulation was higher in the dhaincha crop than in green gram and cowpea (Shah et al., 2011). More dry matter incorporation increases nutrient availability in the soil, resulting in greater rice crop growth and yield in the dhaincha incorporation treatment when compared to other summer legumes (Sujatha et al., 2017). Higher yield with increasing levels of fertilizers might be due to higher amount of nutrients added to soil (Yadav and Meena, 2014). In our study, the percentage increase in kharif rice yield following dhaincha-100% RDF was 83.04% compared to fallow-no fertilizer treatment plots. The response to increased inorganic fertilizer levels could be due to the faster release of available nutrients from inorganic sources, and rice, being an exhaustive feeder, could use this nutrient to increase plant physiological processes, resulting in higher grain yields. Following 100% RDF, FYM treatments were applied, as FYM supplies NPK and other nutrients slowly, allowing rice crops to absorb nutrients at later phases of the growth cycle for improved development. The favorable effect of integrated nutrient management through both inorganic fertilizers and organic manures on higher crop growth and yield was also reported by Ahmed et al. (2014). The highest grain production may be attributed to improvements in yield attributing characteristics (Table 3), such as the number of productive tiller density, test weight, and number of filled grains per panicle, which were reported with this treatment compared to other treatments. Residue-incorporated plots produced better grain yields than fallow plots at the same fertilizer level. This can be attributed to crop residues providing favorable physical and chemical properties during the decomposition process, but the time of release of nutrients as per crop demand may not be the same, whereas nutrients from inorganic fertilizers may have been released at a faster rate, which the crop may have used for growth. Ehsan et al. (2014) investigated the residual effects of green manures on wheat and found that incorporating green manure, specifically Sesbania aculeata, led to significant increase in growth and yield attributes. Additionally, it enhanced the root density of wheat grown after rice.
The Dhaincha (T3) incorporation treatment produced 12.45% more straw than the fallow (T4) treatment. Summer legume integration may be superior due to faster decomposition, a narrower C:N ratio, higher biological nitrogen fixation in the soil, slow mineralization, and improved soil health, which results in a higher yield. This phenomenon may arise from enhanced vegetative growth, characterized by increased plant height, greater tiller numbers, and dry matter accumulation. These improvements stem from prolonged and adequate availability of nitrogen and phosphorus in the soil, coupled with ameliorated soil conditions and improved root penetration (Bai et al., 2024), thereby facilitating superior absorption of moisture and nutrients. In our study, we found that the 100% RDF (W1) treatment yielded 83.05 percent more straw than the no-fertilizer application (W6) control treatment. The uptick in straw production might be attributed to higher dry matter buildup as a result of the combined influence of preceding summer legumes, residue assimilation, and varied quantities of nutrients provided and their release from the soil. Overall, the escalated straw yield with these treatments could be attributed to ameliorated growth as measured by plant height, dry matter accumulation, tillering, and progressively increased total NPK uptake. These findings are harmonious with those of Kumar et al. (2017). Due to the direct relationship between nutrient removal and dry matter content, increased yields of grain and straw, accompanied by higher concentrations of potassium, nitrogen, and phosphorus, led to a greater overall removal of these nutrients. Although there is some evidence to the contrary, integrating grain legumes as intercrop components or in rotation with maize may improve yield stability (Soltani and Sinclair, 2012; Droppelmann et al., 2017; Raseduzzaman and Jensen, 2017).
4.3 Total nutrient uptake of nitrogen and phosphorus
Total nutrient uptake is influenced by the combined processes of dry matter accumulation and yield, and the production of dry matter is intricately linked to the availability of nutrients. Results state that incorporation of residues into soil add back the fertilizer ultimately providing more nutrients for a long run (Amgain et al., 2022b). The ample supply of nutrients fostered robust and vigorous growth in summer legumes, consequently leading to substantial dry matter production. Additionally, the increased nutrient content in the rice plants likely contributed to heightened nutrient assimilation. When comparing the N, P, and K uptake in rice crops sown after the incorporation of green gram, cowpea, and summer fallow, significant variations were observed among them. Furthermore, in the case of rice crops sown after dhaincha incorporation, notably higher total N and P uptake was recorded compared to crops sown after green gram, cowpea, and summer fallow incorporation. The incorporation of dhaincha also resulted in taller rice plants, increased leafiness, and accelerated root growth, potentially leading to greater dry matter production and more significant incorporation of biomass into the soil. This, in turn, could have resulted in higher N and P uptakes. It is worth noting that dhaincha was incorporated immediately after reaching 50% flowering, a stage characterized by high nutrient accumulation in plant tissues. In contrast, green gram and cowpea were incorporated after the economic yield harvest, at a point where most of the nutrients had already been allocated to the maintenance of reproductive and vegetative plant structures. Additionally, the decomposition of green plants released both macro and micronutrients from the organic and native pools, making them available for subsequent plant uptake. The increase in N, P, and K uptake due to incorporation of summer legumes are the results of improved environment in soil which encouraged proliferation of roots and results into more absorption of water and nutrients from large area of soil. These results also get supported by Talathi et al. (2009), Saraswat et al. (2010), Islam et al. (2014), and Islam et al. (2019). Total uptake of NPK by rice crop increased significantly with integration of inorganic fertilizer and organic manures over No-fertilizer application (W6), however maximum total N uptake was observed with the application of 100% RDF followed by 75% RDF through chemical fertilizer +25% N from FYM and 50% RDF through chemical fertilizer +50% N from FYM. Nearly similar trend was observed in case of phosphorus and potassium uptake by rice during both the years and in pooled analysis. This might be due to in general, the trend of nutrient uptake was very well resembled with dry matter accumulation and per hectare yield data of various treatments. The enhanced uptake of these nutrients in the corresponding treatments could be due to the increased and sustained availability of nutrients through organic and inorganic fertilizers. The increased uptake by rice might be due to improvement in soil physical, chemical, and biological health through application of organic and inorganic fertilizers under integrated nutrient management. These results are supported by Sunitha et al. (2010) and Kumari et al. (2013). Increasing a proportion of legume crops in a rotation will decrease the amount of inorganic fertilizer used, increase the amount of N that is derived from renewable resources in global nutrient cycles, and maybe even reduce the amount of reactive N that is lost from the environment (Foley et al., 2011; Seufert et al., 2012). The inclusion of summer mungbean in cereal-cereal cropping systems could be a long-term strategy to enrich soil organic C and to ensure sustainability of cereal-cereal cropping systems (Hazra et al., 2018).
5 Conclusion and future scope
Our study highlighted that incorporation of summer legumes before planting rice significantly augmented plant height, number of tillers, yield indices, and rice grain and straw yield. While the magnitude of growth was greater in the plot that received dhaincha inclusion. The crop grown after dhaincha inclusion at 100% RDF yielded considerably more grain than the crop sown after summer fallow with no fertilizer application. In most cases, use of 100% RDF and 75% RDF + 25% N from FYM fates in equivalent plant height, yield indices, and grain yield of rice. During the experiment, the treatment T3W1 (which involved using dhaincha and 100% recommended fertilizer) had the highest amounts of nitrogen and phosphorus uptake, both in the whole rice plant and specifically in the grain and straw. Conversely, the T4W6 (fallow field without fertilizer) showed the lowest values.
Future scope
Future research should look into alternative cropping systems, such as oil seeds and cereals, to see how they affect soil health and nutrient dynamics when incorporated into the soil. Furthermore, studying how summer legume inclusion affects soil microbial populations, nutrient cycling, and carbon profiling will provide more insight. Evaluating the cost-effectiveness and practicality of various crop sequences will be critical in building sustainable and economically viable farming practices.
Data availability statement
The original contributions presented in the study are included in the article/supplementary material, further inquiries can be directed to the corresponding authors.
Author contributions
TS: Data curation, Formal analysis, Software, Writing – original draft. HV: Conceptualization, Data curation, Formal analysis, Project administration, Software, Supervision, Writing – original draft. KP: Data curation, Formal analysis, Project administration, Software, Supervision, Writing – original draft. SR: Software, Supervision, Writing – original draft. MC: Methodology, Validation, Writing – review & editing. PK: Methodology, Validation, Writing – review & editing. ME: Funding acquisition, Investigation, Resources, Writing – review & editing. AO: Funding acquisition, Investigation, Resources, Writing – review & editing. AS: Funding acquisition, Investigation, Resources, Writing – review & editing. AE: Funding acquisition, Investigation, Resources, Writing – review & editing. DE-S: Funding acquisition, Investigation, Resources, Writing – review & editing.
Funding
The author(s) declare financial support was received for the research, authorship, and/or publication of this article. The authors extend their appreciation to the Deanship of Research and Graduate Studies at King Khalid University for funding this work through the Large Research Project under grant number RGP2/118/45.
Acknowledgments
We express my heartfelt gratitude to Head and Professor, Dr. H. M. Virdia (major advisor), as well as to my advisory committee, for their invaluable counsel in the course my academic journey. I acknowledge with deep appreciation the support provided under the “Scheme for Developing High-Quality Research, Education Department- SHODH” by the state government of Gujarat for the current research project.
Conflict of interest
The authors declare that the research was conducted in the absence of any commercial or financial relationships that could be construed as a potential conflict of interest.
Publisher’s note
All claims expressed in this article are solely those of the authors and do not necessarily represent those of their affiliated organizations, or those of the publisher, the editors and the reviewers. Any product that may be evaluated in this article, or claim that may be made by its manufacturer, is not guaranteed or endorsed by the publisher.
References
Ahmed, S., Basumatary, A., Das, K. N., Medhi, B. K., and Srivastava, A. K. (2014). Effect of integrated nutrient management on yield, nutrient uptake and soil fertility in autumn rice on Inceptisol of Assam. Ann. Plant Soil Res. 16, 192–197.
Amgain, N. R., Fan, Y., VanWeelden, M. T., Rabbany, A., and Bhadha, J. H. (2022b). From ground to grain: tracing phosphorus and potassium in flooded Rice cultivar grown on Histosols. Agriculture 12:1250. doi: 10.3390/agriculture12081250
Amgain, N. R., Xu, N., Rabbany, A., Fan, Y., and Bhadha, J. H. (2022a). Developing soil health scoring indices based on a comprehensive database under different land management practices in Florida. Agrosyst. Geosci. Environ. 5:e20304. doi: 10.1002/agg2.20304
Anitha, S., and Mathew, J. (2011). Effect of concurrent growing of Sesbania aculeate (wild) on the nitrogen mineralization pattern and soil nutrient status in wet seeded rice. Oryza 48, 329–334.
Anonymous (2016). Annual report Ministry of new and Renewable Energy, New Delhi 2020. Available at: http://mnre.gov.in
Ashworth, A. J., West, C. P., Allen, F. L., Keyser, P. D., Weiss, S. A., Tyler, D. D., et al. (2015). Biologically fixed nitrogen in legume intercropped systems: comparison of nitrogen-difference and Nitrogen-15 enrichment techniques. Agron. J. 107, 2419–2430. doi: 10.2134/agronj14.0639
Bai, X., Smidt, S. J., Fan, Y., Brophy, T., Her, Y. G., Manirakiza, N., et al. (2024). Farming shallow soils: impacts of soil depth on crop growth in the Everglades agricultural area of Florida, USA. Field Crop Res. 316:109523. doi: 10.1016/j.fcr.2024.109523
Balai, M. L., Verma, A., Nepalia, V., and Kanthaliya, P. C. (2011). Productivity and quality of maize (Zea mays) as influenced by integrated nutrient management under continuous cropping and fertilization. Indian J. Agric. Sci. 81, 374–376.
Ban, Z., Niu, C., Li, L., Gao, Y., Liu, L., Lu, J., et al. (2024). Exogenous brassinolides and calcium chloride synergically maintain quality attributes of jujube fruit (Ziziphus jujuba mill.). Postharvest Biol. Technol. 216:113039. doi: 10.1016/j.postharvbio.2024.113039
Chimonyo, V. G. P., Snapp, S. S., and Chikowo, R. (2019). Grain legumes increase yield stability in maize based cropping systems. Crop Sci. 59, 1222–1235. doi: 10.2135/cropsci2018.09.0532
Dai, W., Fan, Y., Cao, L., Cao, L., and Sha, Z. (2023). Soil organic carbon dynamics shift by incorporating wheat straw in paddy soil in China. JEQ 52, 960–971. doi: 10.1002/jeq2.20496
Dalvi, A. S., Chavan, L. S., and Mahadkar, U. V. (2017). Effect of different sources of nutrient on productivity and profitability of rice (Oryza sativa L.) - cowpea (Vigna unguiculata L.) cropping system. J. Ind. Soc. Coast. Agril. Res. 35, 8–14.
Deshpande, H. H., and Devasenapathy, P. (2011). Effect of different organic sources of nutrients and green manure on growth and yield parameters of rice (Oryza sativa L.) grown under lowland condition. Crop. Res. 41, 1–5.
Droppelmann, K. J., Snapp, S. S., and Waddington, S. R. (2017). Sustainable intensification options for smallholder maize-based farming systems in sub-Saharan Africa. Food Secur. 9, 133–150. doi: 10.1007/s12571-016-0636-0
Ehsan, S., Niaz, A., Saleem, I., and Mehmood, K. (2014). Substitution of major nutrient requirement of rice-wheat cropping system through Sesbania green manuring. Sci. Agric. 8, 99–102.
Foley, J. A., Ramankutty, N., Brauman, K. A., Cassidy, E. S., Gerber, J. S., Johnston, M., et al. (2011). Solutions for a cultivated planet. Nature 478, 337–342. doi: 10.1038/nature10452
Hazra, K. K., Ghosh, P. K., Venkatesh, M. S., Nath, C. P., Kumar, N., Singh, M., et al. (2018). Improving soil organic carbon pools through inclusion of summer mungbean in cereal-cereal cropping systems in indo-Gangetic plain. Arch. Agron. Soil Sci. 64, 1690–1704. doi: 10.1080/03650340.2018.1451638
Hu, Y., Yang, S., Qian, X., Li, Z., Fan, Y., Manevski, K., et al. (2023). Bibliometric network analysis of crop yield gap research over the past three decades. Agriculture 13:2105. doi: 10.3390/agriculture13112105
Huang, J., Ma, H., Sedano, F., Lewis, P., Liang, S., Wu, Q., et al. (2019). Evaluation of regional estimates of winter wheat yield by assimilating three remotely sensed reflectance datasets into the coupled WOFOST–PROSAIL model. Eur. J. Agron. 102, 1–13. doi: 10.1016/j.eja.2018.10.008
Irshad, M. K., Ibrahim, M., Noman, A., Shang, J. Y., Mahmood, A., Mubashir, M., et al. (2022). Elucidating the impact of goethite-modified biochar on arsenic mobility, bioaccumulation in paddy rice (Oryza sativa L.) along with soil enzyme activities. Process. Saf. Environ. Prot. 160, 958–967. doi: 10.1016/j.psep.2022.02.069
Islam, M. R., Hossain, M. B., Siddique, A. B., Rahman, M. T., and Malika, M. (2014). Contribution of green manure incorporation in combination with nitrogen fertilizer in rice production. SAARC J. Agricult. 12, 134–142. doi: 10.3329/sja.v12i2.21925
Islam, M., Tahmina, A., Sohel, R., Saiful, A., and Moynul, H. M. (2019). Green manuring effects on crop morpho-physiological characters, rice yield and soil properties. Physiol. Mol. Bio. Plants 25, 303–312. doi: 10.1007/s12298-018-0624-2
Jackson, M. L. (1973). Soil and Chemical Analysis. New Delhi: Prentice Hall of India Private Limited.
Kumar, N., Kumar, S., Sravan, S. U., and Singh, S. P. (2017). Growth and yield performance of aromatic rice (Oryza sativa L.) as influenced by bio-organics and fertility levels. J. Pharmacogn. Phytochem. 6, 2131–2136.
Kumar, V., Tripathi, H. C., and Mishra, S. K. (2012). Influence of integrated nutrient management on yield, economics, and nutrient uptake of hybrid rice (Oryza sativa L.). New Agriculturist 23, 117–121.
Kumari, N., Pal, S. K., and Barla, S. (2013). Effect of organic nutrient management on productivity and economics of scented rice. Oryza 50, 249–252.
Meena, V. S., Maurya, B. R., and Meena, R. S. (2015). Residual impact of well-grow formulation and NPK on growth and yield of wheat (Triticum aestivum L.). Bangladesh J. Bot. 44, 143–146. doi: 10.3329/bjb.v44i1.22738
Naher, M. S., and Paul, A. K. (2017). Effect of integrated nutrient management on nutrient uptake and sustainable grain yield in transplanted aman rice. SAARC J. of Ag. 15, 43–55. doi: 10.3329/sja.v15i1.33149
Nawaz, A., Farooq, M., Nadeem, F., Siddique, K. H. M., and Lal, R. (2019). Rice-wheat cropping systems in South Asia: issues, options and opportunities. Crop and Pasture Sci. 70, 395–427. doi: 10.1071/CP18383
Neelima, T. L., Bhanu Murthy, V. B., and Ramanjaneyulu, A. V. (2007). Response of rice to N fertilizer and sunhemp green manuring. Res. Crops 8, 533–536.
Panse, V. G., and Sukhatme, P. V. (1967). Statistical methods for agricultural workers. New Delhi: Indian Council of Agricultural Research, 145–152.
Piccoli, I., Sartori, F., Polese, R., and Berti, A. (2020). Crop yield after 5 decades of contrasting residue management. Nutr. Cycl. Agroecosyst. 117, 231–241. [CrossRef]. doi: 10.1007/s10705-020-10067-9
Prathibha Sree, S., Veera Raghavaiah, R., Subbaiah, G., Ashoka Rani, Y., and Sreenivasa Rao, V. (2016). Growth, yield attributes, yield and nutrient uptake of rice as influenced by organic manures and zinc supplementation at different nitrogen levels. Andhra Agric. J. 63, 34–39.
Premalatha, B. R. (2017). Use of leaf colour chart for nitrogen management as a tool in bridging the yield gap in rainfed rice (Oryza sativa L.) production. Advan. Res. J. Crop Improv. 8, 36–44. doi: 10.15740/HAS/ARJCI/8.1/36-44
Qiu, S., Yang, H., Zhang, S., Huang, S., Zhao, S., Xu, X., et al. (2023). Carbon storage in an arable soil combining field measurements, aggregate turnover modeling and climate scenarios. Catena 220:106708. doi: 10.1016/j.catena.2022.106708
Radhakumari, C., and Srinivasulureddy, D. (2010). Residue management and N dynamics in legume-rice (Oryza sativa)-groundnut (Arachis hypogaea) cropping system for sustainable crop production. J. Oilseeds Res. 27, 130–135.
Rajeshwari, R. S., Hebsur, N. S., Pradeep, H. M., and Bharamagoudar, T. D. (2007). Effect of integrated nutrient management on growth and yield of maize. Karnataka J. Agricult. Sci. 20, 399–400.
Ramachandra, C., Shivanna, M., Janardhan, G., and Shivakumar, K. V. (2008). Growth and productivity of kharif rice (Oryza sativa L.) as influenced by cropping sequences and fertility levels in hill zone of Karnataka. Crop. Res. 36, 12–15.
Raseduzzaman, M., and Jensen, E. S. (2017). Does intercropping enhance yield stability in arable crop production? A meta-analysis. Eur. J. Agron. 91, 25–33. doi: 10.1016/j.eja.2017.09.009
Reckling, M., Hecker, J.-M., Bergkvist, G., Watson, C., Zander, P., Stoddard, F., et al. (2016). A cropping system assessment framework—evaluating effects of introducing legumes into crop rotations. Eur. J. Agron. 76, 186–197. doi: 10.1016/j.eja.2015.11.005
Reilly, E. C., Gutknecht, J. L., Tautges, N. E., Sheaffer, C. C., and Jungers, J. M. (2022). Nitrogen transfer and yield effects of legumes intercropped with the perennial grain crop intermediate wheatgrass. Field Crop Res. 286:108627. [CrossRef]. doi: 10.1016/j.fcr.2022.108627
Reshma, M. R., Pillai, P. S., John, J., and Thampatti, K. C. M. (2019). Effect of crop diversification and fish integration in productivity enhancement of rice. Int. J. Curr. Microbiol. Appl. Sci. 8, 382–387. doi: 10.20546/ijcmas.2019.811.048
Saraswat, P. K., Kumar, K., Tiwari, R. C., and Singh, V. K. (2010). Influence of different summer green manures on rice–wheat yield, nutrient uptake, and soil characteristics. J. Hill Agricult. 1, 23–29.
Seufert, V., Ramankutty, N., and Foley, J. A. (2012). Comparing the yields of organic and conventional agriculture. Nature 485, 229–232. doi: 10.1038/nature11069
Shah, Z., Ahmad, S. R., and Rahman, H. U. (2011). Effect of green manure legumes on rice yield and soil quality. Pak. J. Bot. 43, 1569–1574.
Sharan Kumar, S., Prasad, P., and Rajak, D. (2017). Effect of integrated nutrient management on productivity and profitability of maize. Int. J. Curr. Microbiol. App. Sci. 6, 3878–3882. doi: 10.20546/ijcmas.2017.612.448
Shen, X., Bai, X., Zhao, C., Tan, Q., Luo, G., Li, C., et al. (2024). Global response of soil biodiversity to climate and land use changes. J. Clean. Prod. 471:143381. doi: 10.1016/j.jclepro.2024.143381
Singh, G., Bhattacharyya, R., Das, T. K., Sharma, A. R., Ghosh, A., Das, S., et al. (2018). Crop rotation and residue management effects on soil enzyme activities, glomalin and aggregate stability under zero tillage in the indo-gangetic plains. Soil Tillage Res. 184, 291–300. doi: 10.1016/j.still.2018.08.006
Singh, R. P., Singh, P. K., and Singh, A. K. (2009). Effect of green manuring on physico-chemical properties of soil and productivity of rice (Oryza sativa). Oryza 46, 120–123.
Singh, Y., Singh, B., and Timsina, J. (2005). Crop residue management for nutrient recycling and improving soil productivity in rice–based cropping systems in the tropics. Adv. Agron. 85, 269–407. doi: 10.1016/S0065-2113(04)85006-5
Soltani, A., and Sinclair, T. R. (2012). Optimizing chickpea phenology to available water under current and future climates. Eur. J. Agron. 38, 22–31. doi: 10.1016/j.eja.2011.11.010
Srivastava, V. K., Bohra, J. S., and Singh, J. K. (2013). Effect of integration of NPK levels and organic sources on growth, yield and economics of rice. Adv. Res. J. Crop Improv. 2, 113–117.
Sujatha, D. V., Kavitha, P., and Naidu, M. V. S. (2017). Influence of green manure and potassium nutrition on soil potassium fractions and yield of rice crop. Int. J. Curr. Microbiol. Appl. Sci. 6, 13–23. doi: 10.20546/ijcmas.2017.611.002
Sujatha, D. V., Kavitha, P., Naidu, M. V. S., and Maheswari, U. (2016). Influence of green manure and potassium on growth, yield and economics of rice (Oryza sativa L.). J. Res. ANGRAU 44, 43–49.
Sulieman, S., and Tran, L. S. P. (2015). Legume nitrogen fixation in a changing environment. Cham: Springer International Publishing, 35.
Sunitha, B. P., Prakasha, H. C., and Gurumurthy, K. T. (2010). Effect of INM approach on soil physical properties, available nutrient status, concentration, and their uptake by rice crop in bhadra command, Karnataka. Mysore J. Agric. Sci. 44, 905–910.
Talathi, M. S., Pinjari, S. S., Ranshur, N. J., Bhondave, T. S., and Suryawanshi, J. S. (2009). Response of hybrid rice (Oryza sativa L.) to green leaf manure, FYM and chemical fertilizers. Int. J. Agric. Sci. 5, 501–506.
Thilakarathna, M. S., McElroy, M. S., Chapagain, T., Papadopoulos, Y. A., and Raizada, M. N. (2016). Belowground nitrogen transfer from legumes to non-legumes under managed herbaceous cropping systems. A review. Agron. Sustain. Dev. 36:58. [CrossRef]. doi: 10.1007/s13593-016-0396-4
UNDESA (2015). “United Nations, Department of Economic and Social Affairs, population division (UN DESA), world population prospects: the 2015 revision, key findings and advance tables” in Working paper no. ESA/P/WP.241 (New York: United Nations, Department of Economics and Social Affairs).
Wang, X., Huang, J., Feng, Q., and Yin, D. (2020). Winter wheat yield prediction at county level and uncertainty analysis in Main wheat-producing regions of China with deep learning approaches. Remote Sens. 12:1744. doi: 10.3390/rs12111744
Xiao, D., Liu, D. L., Zhang, X., and Fan, Y. (2024). Agricultural production in a warmer world: challenges and sustainable development strategies. Front. Earth Sci. 12:1368149. doi: 10.3389/feart.2024.1368149
Xie, Z., He, Y., Tu, S., Xu, C., Liu, G., Wang, H., et al. (2017). Chinese milk vetch improves plant growth, development, and recovery in the rice-based rotation system of South China. Sci. Rep. 7, 35–77. doi: 10.1038/s41598-017-03919-y
Yadav, L., and Meena, N. (2014). Performance of aromatic rice (Oryza sativa) genotype as influenced by integrated nitrogen management. Ind. J. Agron. 59, 51–255.
Yang, X., Wang, J., Xia, X., Zhang, Z., He, J., Nong, B., et al. (2021). OsTTG1, a WD40 repeat gene, regulates anthocyanin biosynthesis in rice. Plant J. 107, 198–214. doi: 10.1111/tpj.15285
Keywords: summer legumes, rice, yield, growth attributes, nitrogen, phosphorus
Citation: Sunil Kumar T, Virdia HM, Patel KG, Ragi S, Chowdhury M, Kumar P, Elbagory M, Omara AE-D, Salem A, Elbeltagi A and El-Shinawy DM (2024) Effect of summer legume residue incorporation and fertilizer regimes on rice growth, yield, and nutrient uptake. Front. Sustain. Food Syst. 8:1467201. doi: 10.3389/fsufs.2024.1467201
Edited by:
Mohamed Ait-El-Mokhtar, University of Hassan II Casablanca, MoroccoReviewed by:
Vesna Vasić, Educons University, SerbiaYuchuan Fan, University of Florida, United States
Copyright © 2024 Sunil Kumar, Virdia, Patel, Ragi, Chowdhury, Kumar, Elbagory, Omara, Salem, Elbeltagi and El-Shinawy. This is an open-access article distributed under the terms of the Creative Commons Attribution License (CC BY). The use, distribution or reproduction in other forums is permitted, provided the original author(s) and the copyright owner(s) are credited and that the original publication in this journal is cited, in accordance with accepted academic practice. No use, distribution or reproduction is permitted which does not comply with these terms.
*Correspondence: T. Sunil Kumar, c3VuaWx0YW1taTcwODJAZ21haWwuY29t; Manojit Chowdhury, bWFub2ppdGNob3dkaHVyeTEzQGdtYWlsLmNvbQ==; Ali Salem, c2FsZW0uYWxpQG1pay5wdGUuaHU=