- 1Institute of Agricultural and Nutritional Sciences, Soil Biogeochemistry, Martin Luther University Halle-Wittenberg, Halle, Germany
- 2South Ethiopia Agricultural Research Institute, Jinka Agricultural Research Center, Jinka, Ethiopia
- 3Wondo Genet College of Forestry and Natural Resources, Hawassa University, Hawassa, Ethiopia
- 4Forest and Rangeland Biodiversity Directorate, Ethiopian Biodiversity Institute, Addis Ababa, Ethiopia
Introduction: Traditionally, some smallholder farmers in the South Ari District of southern Ethiopia retain natural forest remnant trees on their crop fields, and they manage them through pruning and pollarding techniques. Among these, the umbrella tree (Terminalia brownii) is one of the most preferred and dominant species, with numerous multipurpose uses. However, the effects of canopy management on microclimate and crop yield have not yet been investigated. Here, we present the effects of Terminalia brownii canopy management on microclimate and maize yield in agroforestry parkland.
Methods: Microclimate and maize yield data from three radial distances from the tree trunks to the open field: D1 (0–4.1 m), D2 (4.1–8.2 m), and D3 (>15 m outside the tree canopy cover) were collected. Three tree canopy management treatments (pollarding, pruning, and control) were considered and replicated three times in a randomized complete block design (RCBD) for applying canopy management treatments.
Results and discussion: Our results revealed that the canopy management of the tree affected the microclimate, which in turn influenced maize yield. In all treatments, there were statistically significant differences (p < 0.05) observed in maize yield and yield components under tree canopy and open fields of both canopy-managed trees and control. The mean highest grain yields were observed in plots with pruned (3,717 kg ha−1) and pollarded (3,718 kg ha−1) trees, while the lowest yield (2,146 kg ha−1) was obtained from the control plot. The observed differences in yield might be due to a higher accumulation of soil nutrients, modified microclimate under the tree canopy, and reduced shading effects via canopy management. Therefore, we conclude that either pruning or pollarding of Terminalia brownii enhances maize yield by modifying microclimate and improving soil nutrients. Pruning, in particular, offers a balance of shade and light, creating a stable microclimate with consistent seasonal light and reduced temperature fluctuations, which supports better maize growth in agroforestry systems.
1 Introduction
In rural areas of eastern Africa, agriculture provides food, employment, environmental services, and livelihoods for 80% of households (Salami et al., 2010; Neglo et al., 2021). However, issues with governance, economy, adoption of technology, and climate change all pose production challenges (Thornton et al., 2010). This is especially true in Ethiopia, where annual soil loss rates can reach 25 Mg ha−1 due to deforestation, population growth, and climate change resulting in crop yields below 1 Mg ha−1 for the past 50 years (Girmay et al., 2020; Buraka et al., 2022). Fortunately, agroforestry offers a promising approach to sustainable land management. It involves intentionally integrating economically viable tree species with crops and/or animals on the same area of land to boost income and provide both products and environmental benefits. Particularly, the agroforestry parkland system offers one solution by integrating selected scattered indigenous multipurpose trees and food crops in the same agricultural field positively influences soil fertility and modifies the soil microclimate, consequently leading to enhanced crop yields (Bekele, 2018; Gebrewahid et al., 2018).
In Ethiopia, smallholder farmers traditionally manage various tree species, including Acacia tortilis, Acacia seyal, Albizia gummifera, Vitex doniana, Balanites aegyptiaca, Cordia africana, Faidherbia albida, Terminalia brownii, and more, in agroforestry parkland systems (Bekele, 2018). Those versatile trees have proliferated on farmland, which is a defining feature of the Ethiopian agricultural landscape, and they are the predominant agroforestry trees in the country’s semi-arid and sub-humid regions (Tscharntke et al., 2011). In addition to improving soil physicochemical properties and increasing crop yield, trees also help mitigate the effects of climate change by preserving biodiversity and influencing microclimate like regulating soil moisture, and minimizing sunlight penetration (Gebrewahid et al., 2018).
Trees in crop fields can enhance the nutrient balance of soil in three main ways. Firstly, they minimize unproductive nutrient losses from erosion and leaching. Secondly, they augment nutrient inputs through nitrogen fixation. Finally, they enhance biological activities by supplying organic matter (OM) to the soil and creating a suitable microclimate (Oluseye et al., 2013). Some studies conducted on agroforestry parkland trees revealed a fertility gradient, with fertility decreasing from a tree’s base to the edge of its crown or beyond (Tolera et al., 2008). Thus, scattered tree canopies lead to improved yield and soil fertility. For example, sorghum grain yields increased by 14% under Cordia africana tree canopy compared to crops grown without trees (Boffa, 2000). Similarly, as a result of agroforestry, maize yields increased by 0.75% compared to tree/hedgerow-free monocultures (Baier et al., 2023).
The practice of integrating desirable tree species into the agricultural landscape has the significant effect of reducing environmental degradation, improving water infiltration through the addition of soil organic matter (SOM), and restoring soil fertility (Berhe, 2013; Tsegu, 2019). It also maximizes the efficient use of sunlight, moisture, and plant nutrients. Therefore, management practices of agroforestry parklands reduce the soil erosion risk and increase total productivity while also providing specialized socioeconomic services to individual farmers and their communities (Agena et al., 2014).
In Ethiopia, particularly in the south Omo Zone, south Ari district, some farmers maintain different tree species on their croplands for a long time for different objectives, especially for soil fertility improvements, thereby crop yield enhancement. According to Lemage and Anmaw (2022), the results confirmed that in the South Ari district; farmers deliberately retain trees on their crop fields from either natural regenerated or remnants of natural forest.
Among these trees, Terminalia brownii is one of the most dominant and preferred for improving soil fertility. However, the impact of canopy management of Terminalia brownii using pruning and pollarding practices on microclimate and maize crop yield has not been studied yet. Even the few studies on other trees have shown contradictory results. For instance, according to Abdella et al. (2020) and Haile et al. (2021), there was a significantly higher grain yield of maize and sorghum crops than in open fields due to a high fertility gradient under the tree canopy, while, according to Mohammed et al. (2018) and Belayneh et al. (2021), there was a significantly lower grain yield of maize, sorghum and wheat crops than an open field due to canopy shading effect under tree canopies. Even in the same study area, Lemage and Anmaw (2022) found that the highest maize yield occurred outside of Terminalia brownii tree canopy cover. As a result, they recommend further research to explore the impact of tree canopy management (such as pollarding and pruning) and microclimates on maize crop yields, as these factors were not taken into account in their study.
Besides this, in the study area, farmers have negative perceptions of how tree canopies affect crop yield and act as barriers for plowing, which has limited the management of Terminalia brownii. Some farmers attempt to manage these shading effects by pruning and pollarding different tree species, but there is no additional scientific knowledge to improve their traditional practices. According to Mamo and Asfaw (2017), integrating farmers’ experience with scientific knowledge is crucial for improving the effectiveness of tree canopy management on crop yield. Therefore, managing agroforestry parkland tree canopies in scientific field experiments is essential for optimizing tree management on crop fields under specific site conditions. Our study aimed to investigate the effects of canopy management of Terminalia brownii on microclimate and maize yield, both inside and outside managed and unmanaged canopies.
2 Materials and methods
2.1 Description of the study area
This study was conducted in the South Ari District of the South Omo Zone in southern Ethiopia (Figure 1). The study site is located at 05°41′5″–06°10′1” N latitude and 36°20′0″–36°45′0″ E longitude. The elevation of the site ranges between 1,213 m a.s.l. and 1,610 m a.s.l. It is located 770 km southwest of Addis Ababa, the capital city of Ethiopia.
The climate of the experimental site can be classified as warm semi-arid, with bimodal rainfall, with a shorter period of rainfall from March to May and a longer one from August to November. The total annual rainfall is 1,272 mm. The mean monthly minimum and maximum temperatures are 16.3°C and 27.7°C, respectively, as shown in Figure 2.
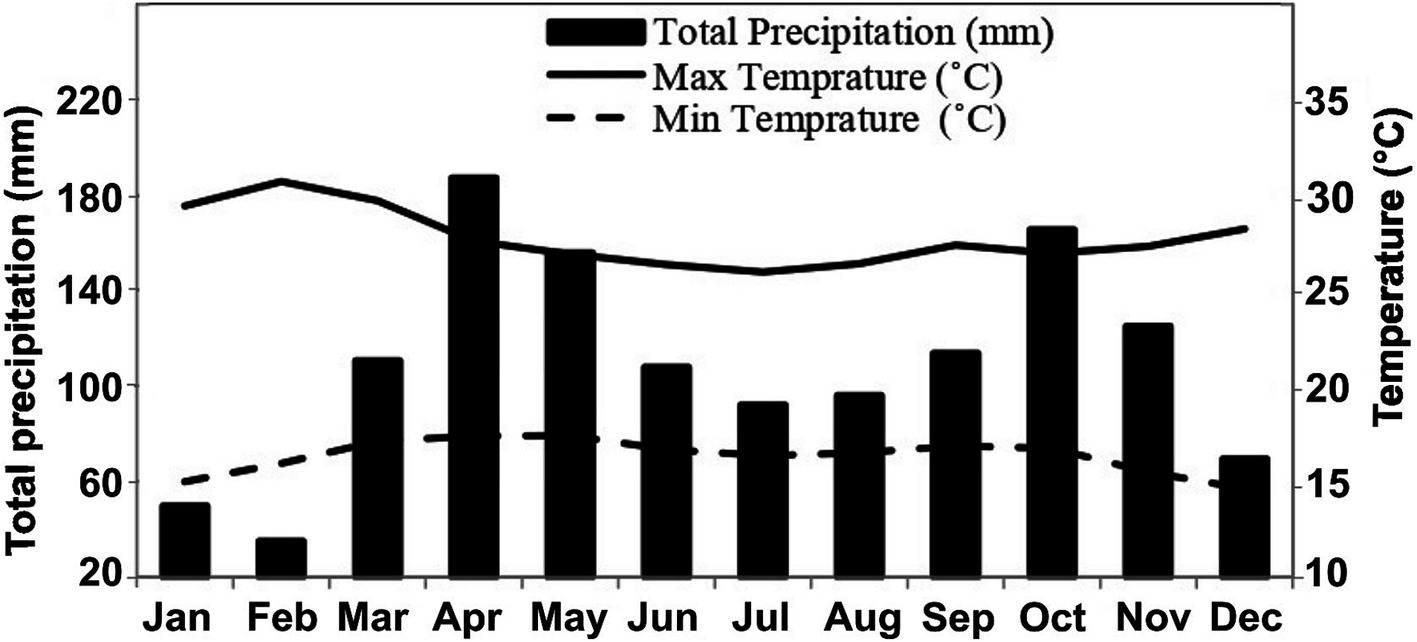
Figure 2. Mean monthly rainfall and temperature of the study area (Source: Jinka meteorology station).
The prevailing crop production systems in the study area are primarily rain-fed. The main crops grown are Triticum aestivum (wheat), Zea mays (maize), Eragrostis teff (teff), Phaseolus vulgaris (beans), Pisum sativum (peas), Sorghum bicolor (barley), and Ensete ventricosum (enset). Inorganic fertilizers have to be used for all crops except enset because the local organic fertilizers are not sufficiently available. Unfortunately, crop productivity is decreasing each year due to the gradual prevalence of droughts.
The livelihood activities of the households in the district involve mixed farming, which includes both crop production and livestock rearing. While staple crops such as maize, teff, and wheat are essential for local consumption, cash crops like beans and peas are cultivated for market sale. Additionally, livestock rearing plays a significant role in the local economy, providing households with milk, meat, and income from the sale of animals. Some households also engage in forestry activities and off-farm income-generating activities to diversify their income sources.
According to Mesfin et al. (2017), the study area has slightly to moderately acidic soils, low to medium soil organic carbon (SOC) and available phosphorus (P), fine to very fine textures (clayey to heavy clay textural class), and pH value ranging from 4.87 to 6.18. These soils are classified as Cambisols and are generally suitable for agricultural purposes.
2.2 Selection of sample trees on crop fields
Before the selection of sample trees on croplands, a survey was conducted to study effective management options for agroforestry parkland trees among smallholder farmers. The top ten individuals per Kebele (the smallest local administration unit), with extensive knowledge on agroforestry parkland tree management, were selected as key informants using the snowball sampling technique.
According to the key informant interviews, Terminalia brownii and Vitex doniana are the most preferred trees for improving soil fertility. While Terminalia brownii is the most common tree in the study area, its effect has not been studied as compared to Vitex doniana. Therefore, purposely, Terminalia brownii was chosen for this experiment. To ensure uniformity, Terminalia brownii trees were selected at the farmer household level based on similar diameter at breast height (DBH) class, canopy spread (crown diameter), maturity or estimated age, and height.
In addition to tree characteristics, the crop fields were carefully selected to control factors influencing maize response, soil moisture, and temperature dynamics. Fields were chosen based on similar cropping history, ensuring consistent practices over the years, and uniform management practices like plowing, planting, and fertilization. Soil type and texture were kept consistent to ensure comparable soil conditions across plots, while topography and slope were uniform to avoid variations in water runoff and erosion. The influence of neighboring trees was minimized to focus on the effects of Terminalia brownii. This systematic selection ensured control over key variables, allowing accurate assessment of Terminalia brownii’s impact on crop yields and soil dynamics, as listed in Table 1.
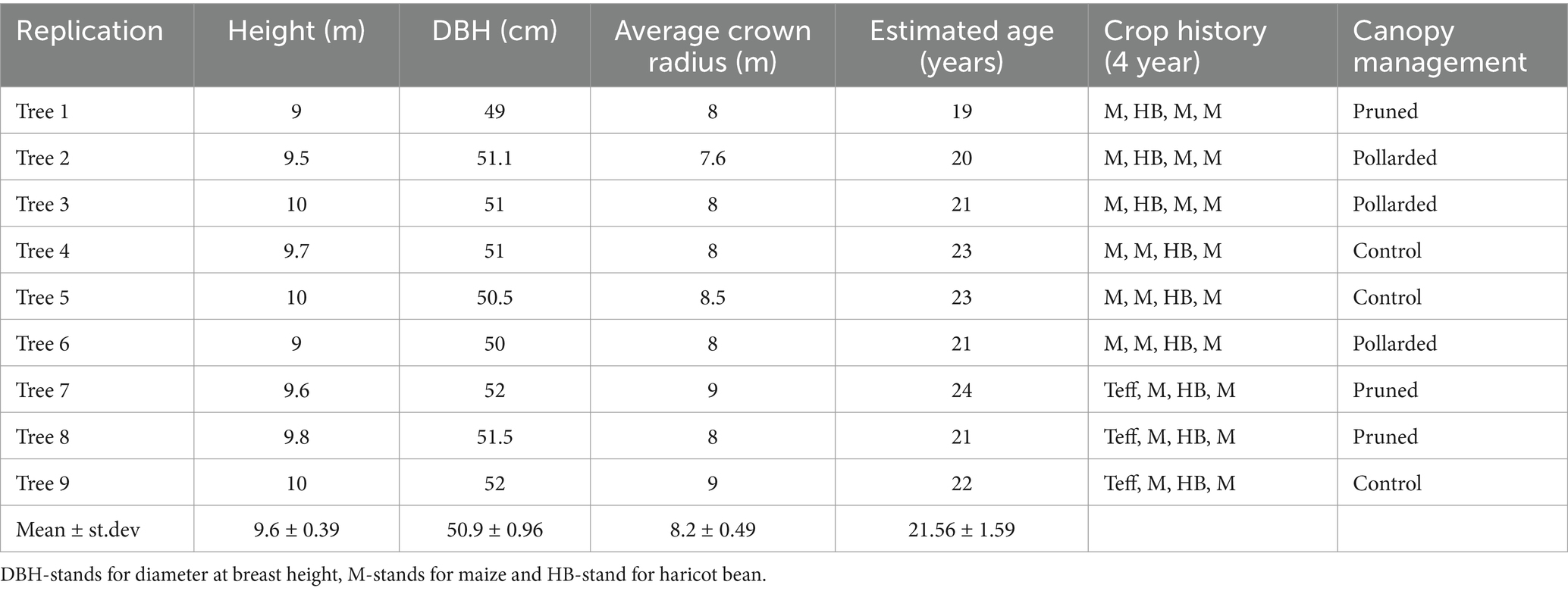
Table 1. Height, diameters of breast height (DBH), crown radius, age of Terminalia brownii trees and cropping history of the study crop field.
2.2.1 Treatments and experimental design
The selected trees underwent pollarding and pruning treatments, with the removal of pruning’s and litter falls from the base of the trees to ensure uniformity. Therefore, there were two factors.
2.2.1.1 Factor-I: tree management
Pollarded, pruned and control (neither pollarded nor pruned) trees with the integration of maize crops under the tree canopy.
Pollarding involves cutting back the upper branches of a tree to encourage the growth of a dense head of foliage. This method is used to reduce shading and improve the balance between light availability and tree canopy structure. While, pruning refers to the selective removal of specific branches or stems, usually from the sides, to shape the tree and improve light penetration. This technique retains the overall structure of the tree while allowing for better light distribution to the crops growing under the canopy (Harmer, 2004; Harris, 1994).
2.2.1.2 Factor-II: distances from tree trunk
Three transects were established to measure the effects of tree canopy on microclimate and crop performance (Figure 3). These transects extended from the tree trunk to different radial distances: 0–4.1 meters (D1), 4.1–8.2 meters (D2), and beyond 15 meters (D3). D1 represents half of the average crown radius, while D2 spans from half of the crown radius to the canopy edge. The third transect, D3 covers the open field outside the canopy’s influence.
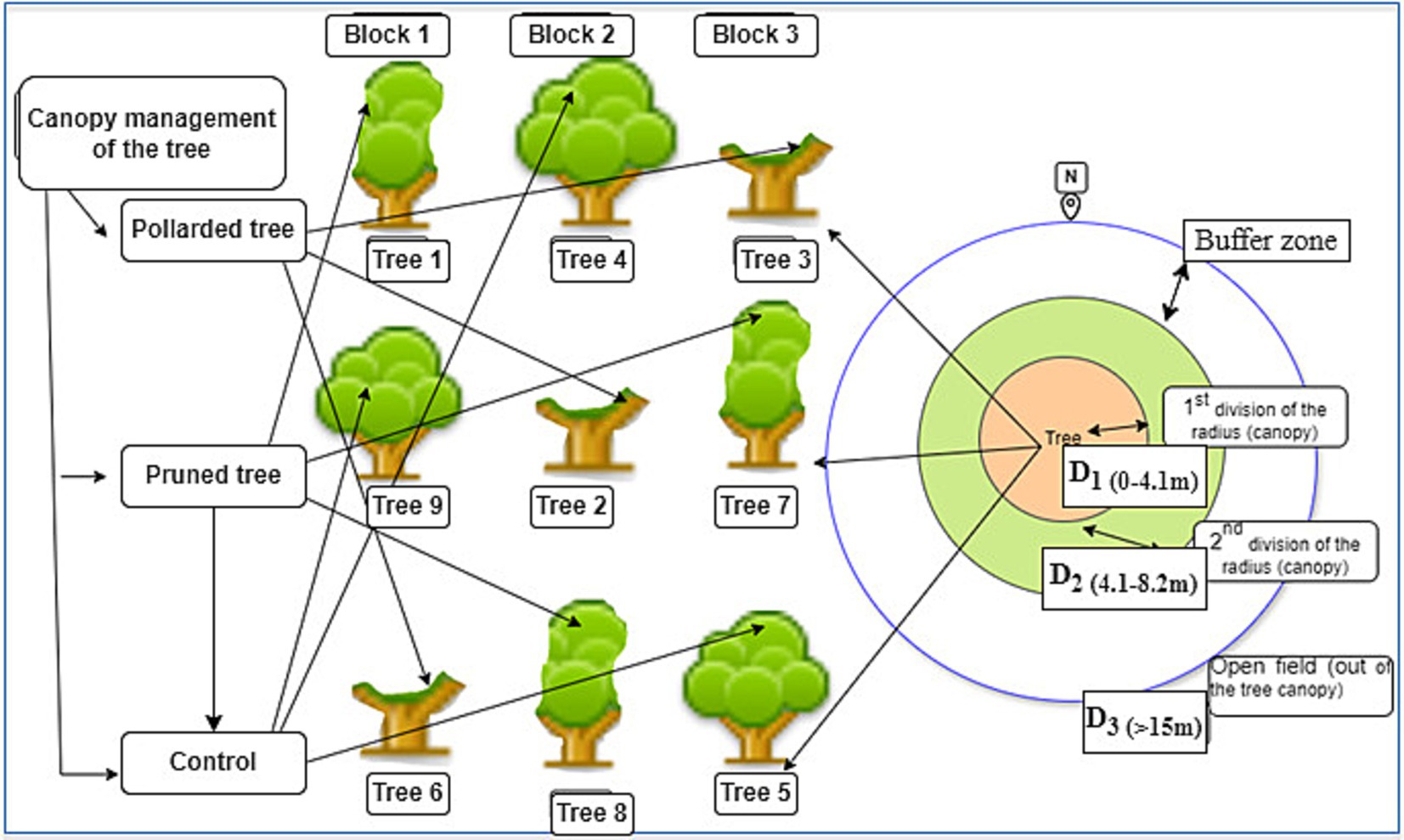
Figure 3. Schematic drawing of experimental design for sampling under the Terminalia brownii tree in crop fields.
Based on our field observations and discussions with local farmers D1 was determined as an average of half the crown radius for the selected trees. We noted that 0–4.1 meters typically represents the zone of significant influence from the tree canopy on microclimate and soil conditions. Beyond this distance, the effects of the tree on crop growth were less pronounced. By using these distances, we aimed to capture the gradient of tree influence on crop performance, from the tree trunk to the open field, allowing for a comprehensive analysis of canopy effects.
The study followed a factorial design with two factors: (1) the three canopy management practices (pollarding, pruning, and control) as factor one, arranged in a randomized complete block design (RCBD) for the canopy management treatments, and (2) the three radial distances from the tree trunks as factor two. The experiment was replicated across three farmer fields (blocks), with each field containing three tree replicates, resulting in 27 treatment combinations (3 canopy management practices × 3 radial distances), replicated three times. This approach allowed us to examine the interaction between canopy management practices and radial distances in a controlled and replicable manner.
2.2.2 Maize planting and management
During the field experiment, to test the impact of the selected trees on crop yield, agronomic practices were carefully implemented to ensure consistency and replicability across all plots. Maize was manually planted at a density of 44,444 plants per hectare, with a spacing of 75 cm between rows and 30 cm between plants. Planting took place 2 weeks after the onset of the rainy season. Pollarding and pruning were performed 4 weeks prior to maize planting, using machetes and following traditional methods. Weeding was conducted manually twice—three and 6 weeks after planting to prevent competition from weeds (Figure 4). The experiment used inorganic fertilizers, with blended nitrogen, phosphorus, sulfur, and boron (NPSB) fertilizer applied at a rate of 100 kg per hectare during planting and urea at 50 kg per hectare at the knee-height stage of maize growth, approximately 4–5 weeks after planting. Organic fertilizers were not used due to limited availability in the area. These practices ensured uniformity, allowing the experiment to be easily replicated in future studies.
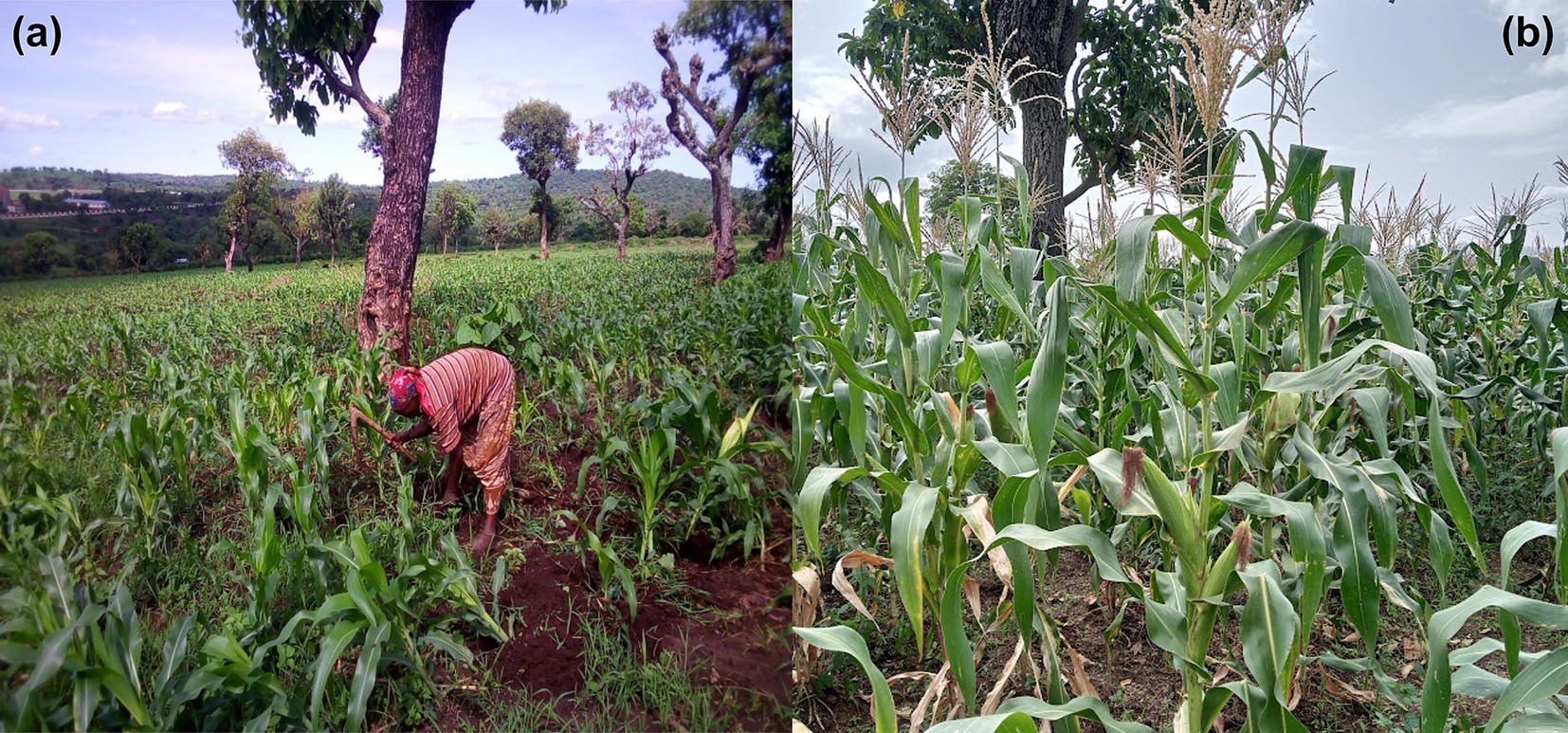
Figure 4. Growth of maize at initial weeding (A) and almost at crop maturity (B) as influenced by the shading effects of Terminalia brownii.
2.3 Data collection methods
2.3.1 Primary data collection
Through field experiments, we collected primary data on maize yield, yield components, microclimate, and soil physical and chemical attributes.
2.3.1.1 Soil sampling and laboratory analysis
The soil samples were collected from the same trees as in a previous study (Handiso et al., 2024 under review). Samples were taken before planting maize to investigate soil properties under Terminalia brownii trees. Samples were taken from nine trees at three radial distances from the tree trunk to the open field (D1, D2, and D3) in four directions and two soil depths (0–20 and 20–40 cm) (Figure 3). A composite sample of 150 g was made by combining samples from each direction within the same radial distances and soil depth. The composite samples were air-dried, grounded, sieved (particle size 2 mm diameter), and analyzed for various properties of the soil, including soil pH, total nitrogen (TN), soil organic carbon (SOC), plant available phosphorus (P), and potassium (K).
All soil samples were analyzed at the laboratory of the soil biogeochemistry department in Martin Luther University, Halle-Wittenberg, Germany. The pH of the soil was measured using a combination electrode in a 1:2.5 (volume/volume) soil-to-water suspension. The plant-available phosphorus was determined by analyzing sodium bicarbonate (NaHCO3)-extractable P or in an HCO3− extract as described by Olsen (1954). The Walkley and Black method was used to analyze SOC (Tscharntke et al., 2011), while TN was measured using the semi-micro Kjeldahl method (Bremner and Mulvaney, 2015).
2.3.1.2 Microclimate data
Soil moisture content was measured to assess the impact of Terminalia brownii tree management on microclimate (Figure 5). Soil samples were collected from each plot at two depths (0–20 cm and 20–40 cm) and three maize growing stages: maize sowing, after 65 days (crop development stage), and after 95 days (harvesting stage). This was done to increase the precision of rainfall variation and new shoot growth during these three months, as shown in Figure 6 (Agena et al., 2014). The percentage of water held in the soil (soil moisture content) was then calculated using the following formula, and the means were used for interpretation.
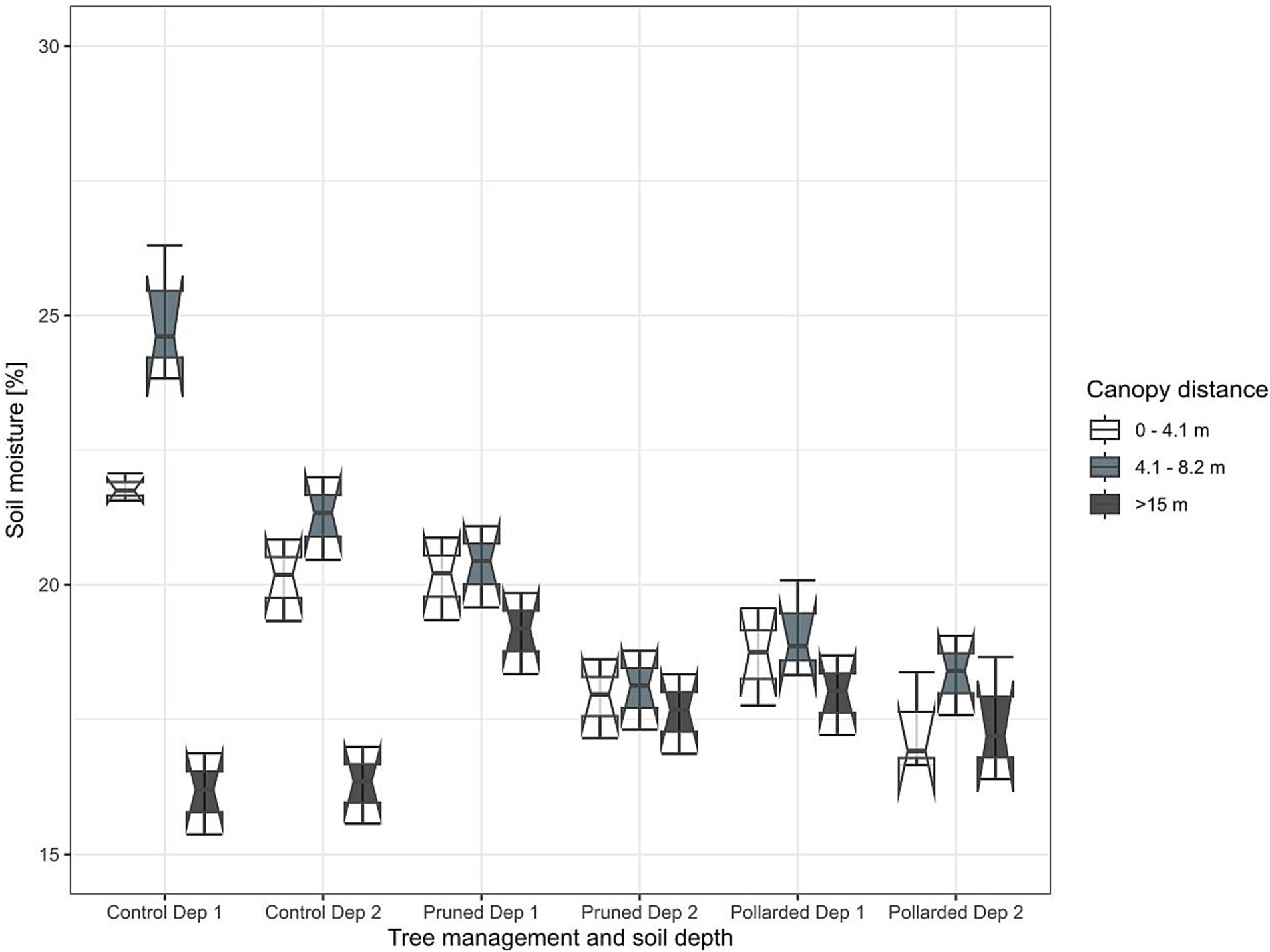
Figure 5. The figure presents the mean soil moisture content under the canopy of the Terminalia brownii tree, categorized by different tree management practices (Control, Pruned, Pollarded) and soil depths as Dep 1 (0–20 cm) and Dep 2 (20–40 cm). The data is further divided by canopy distance into three ranges: 0–4.1 m, 4.1–8.2 m, and > 15 m. Notched box plots provide a visual representation of data distribution, showing the median (represented by solid lines between the boxes) and the interquartile range (IQR), which encompasses the upper (75%) and lower (25%) quartiles. Additionally, the notches indicate the 95% confidence interval of the median, calculated as Median = median ± 5.57*ICR/n0.5. Where n is the sample size. The notches help to assess whether the medians of different groups are significantly different. Scattered points outside the whiskers represent outliers, highlighting values that deviate significantly from the rest of the data.
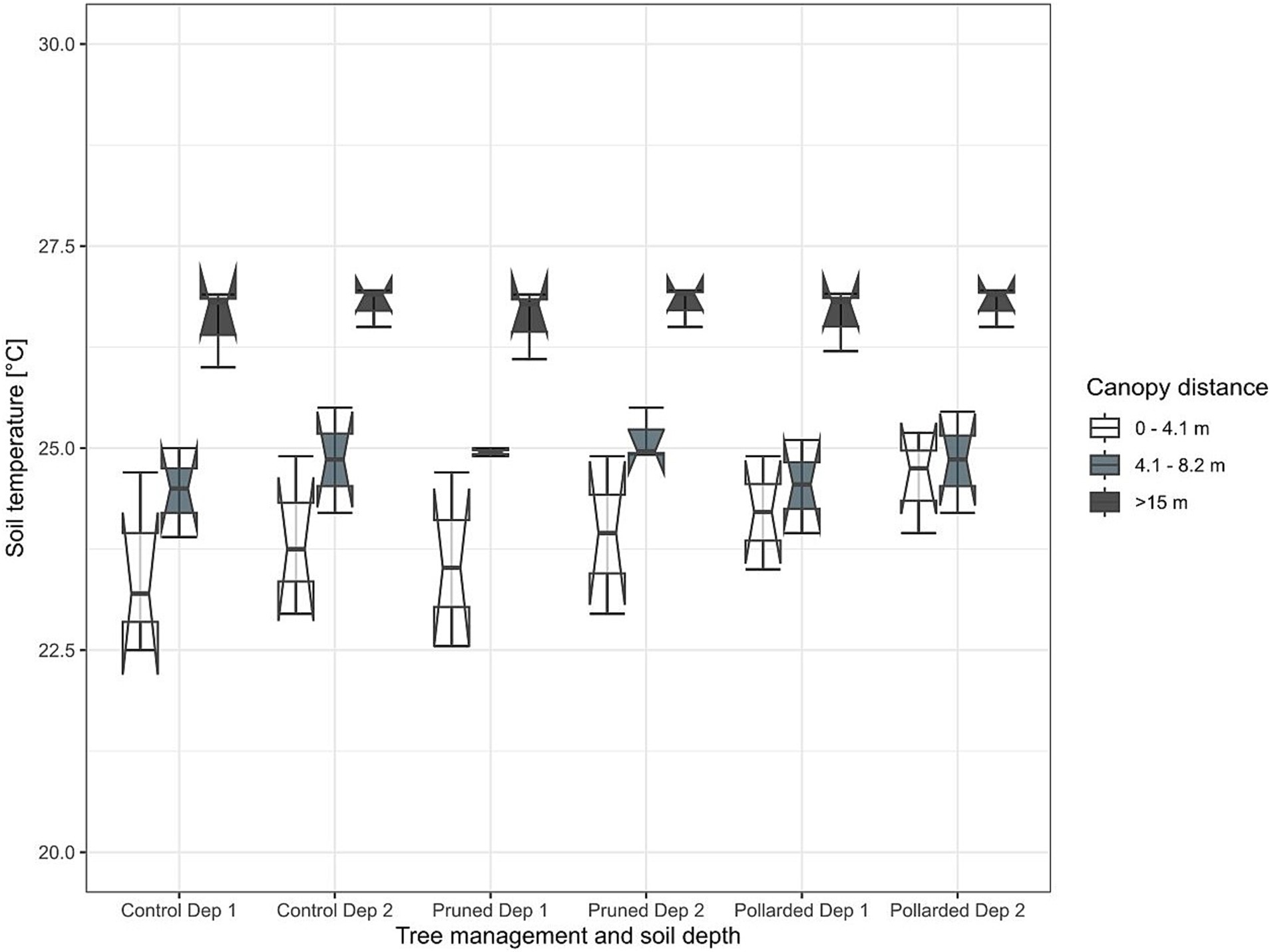
Figure 6. Mean soil temperature under the canopy of Terminalia brownii trees, categorized by different tree management practices (Control, Pruned, and Pollarded) and soil depths (Dep 1: 0–20 cm, Dep 2: 20–40 cm). The data is further divided by canopy distance into three ranges: 0–4.1 m, 4.1–8.2 m, and > 15 m. The notched box plots indicate the median (solid lines between the boxes), and interquartile range (IQR) with upper (75%) and lower (25%) quartiles as well as 95% confidence interval of the median = median ± 5.57*ICR/n0.5. The scattered points also indicate the outlier.
Where: SMC = Soil moisture content dry base (%), Ww = Weight of the wet soil (gm), and Wd = Weight of the dry soil (gm).
Soil temperature (°C) was measured at two depths (0–20 cm and 20–40 cm) using a soil thermometer. Air temperature (°C) was measured using a mercury thermometer, and relative illumination (%) was measured using a Lux meter. Readings for all microclimate parameters were taken from four compass directions at D1, D2, and D3 (in an open field) as described in Figure 3. The readings were taken three times with a 20-min interval, and the mean values were calculated for each radial distance separately. This method was described by Mohammed et al. (2018).
2.3.1.3 Agronomic data
We collected data for the total yield and yield components of maize for each plot, which included plant height, 100-seed weight, grain yield, and total biomass. Plant height was measured at the crop maturity stage by randomly selecting five plants from each net plot and recording the height from the base of the plant to the tip of the tassel using a measuring tape. For 100-seed weight, 100 sun-dried seeds were randomly taken from the seed lots of each plot using a seed counter machine and weighed with a precision balance.
Net plots were carefully delineated within each radial distance from the tree trunk to avoid border effects. A 1 m × 1 m sub-plot was used as the net plot, positioned adjacent to the soil sampling points to ensure consistency between agronomic and soil data collection. Four net plots were established at each radial distance (D1, D2, and D3) for each treatment, covering the four directions around the trees.
For grain yield and biomass, we used a 1 m × 1 m net plot at each radial distance (D1, D2, and D3), with four replicates in the four directions around each tree. Thus, every one-meter square net plot typically contained six maize plants (based on the planting scheme of 0.75 m by 0.30 m). By repeating the net plots four times around the tree, the total number of plants harvested for yield estimation per radial distance was almost 24 plants. This method allowed us to obtain a more representative yield estimate for each radial distance.
Biomass was measured at harvest by cutting all the above-ground plant material from the net plots. The fresh biomass was initially weighed, and then the plants were left in the open air to dry until constant weight was achieved. The dried biomass was then weighed, and the measurements were converted to tons per hectare for biomass. The grain yield was adjusted to a 12.5% moisture level, and the yield measurements were converted to kilograms per hectare.
2.4 Statistical analysis
The collected data from all factorial designs were analyzed using factorial analysis of variance (ANOVA) models. To separate mean differences at p-value <0.05 levels of significance, the least significant difference (LSD) was used with R software (version R-4.2.1-win).
ANOVA was performed to examine the effects of various independent variables on the dependent variables. The independent variables included tree management, canopy cover, soil depth, and their interactions, as well as the number of replications. The dependent variables included the grain yield, the yield component of the maize crop, and the indicator variables of the microclimate.
To evaluate the relationship between maize yield and other parameters such as tree management, the distance from the tree trunk, and microclimate, Pearson correlation, or product–moment correlation coefficient (r), was used. Finally, the results were presented in notched box-plots, bar graphs, and tables based on the properties of the data.
3 Results
3.1 Effects of Terminalia brownii tree management on microclimate
3.1.1 Soil moisture content
The results showed that different tree management practices (control, pruned, and pollarded) and soil depths significantly affect soil moisture content under the Terminalia brownii canopy. There were significant changes (p < 0.05) in the overall mean values of soil moisture content between soil depths and radial distances from the tree trunk (Figure 5). Generally, the higher average moisture content was found in the topsoil at 0–20 cm compared to 20–40 cm soil depth, indicating better moisture retention in the upper soil layers, which was true for all distances from the tree trunk. In addition, the soil moisture results revealed a decreasing trend with increasing radial distances and soil depth. The soil moisture is consistently highest within 4.1–8. 2 m of the tree canopy across all management practices, highlighting the significant impact of tree proximity on moisture retention (Figure 5). Proximity to the tree canopy enhances soil moisture retention, with pruning showing the most beneficial impact, followed by pollarding. These results underscore that trees provide shading and reduce soil water loss by evaporation and crop transpiration. The temperature regime is moderate along with more humidity in the modified microclimate beneath trees. The addition of organic matter through liter fall increases the soil water holding capacity.
3.1.2 Soil temperature
The results highlighted that different tree management practices (control, pruning, and pollarding), soil depths, and distances from the tree trunk significantly affect soil temperature under Terminalia brownii canopies (p < 0.05). Overall, soil temperature among radial distances from tree trunks was statistically significant (p < 0.05), (Figure 6). The maximum average soil temperature under and outside the trees showed that the surface soils’ (26.7°C) and subsurface soils’ (26.8°C) temperatures were lower under the trees than the surface soils’ (25.0°C) and subsurface soils’ (25.1°C) temperatures under pruned tree canopies. The lowest recorded soil temperature was consistently found under tree canopies compared to the open field.
Under control tree management, soil temperatures were lower near the tree trunk (0–4.1 m) and increased with distance (4.1–8.2 m, >15 m) at both depths, with lower temperatures observed in surface soil compared to subsurface soil. This indicates that the canopy provides a cooling effect, particularly effective in the upper soil layer (Dep 1).
Under pruned trees, soil temperature was relatively uniform across different distances but slightly higher compared to the control. This suggests that pruning may reduce the canopy’s cooling effect. Again, sub-surface soil layer (Dep 2) showed higher temperatures than Dep 1, indicating less effective cooling at deeper layers.
Under pollarded trees, the highest soil temperatures among the tree management practices were observed, indicating the least effective cooling. Dep 2 showed a similar trend with higher overall temperatures compared to Dep 1, suggesting that pollarding significantly reduces the cooling effect.
3.1.3 Relative illumination
The study found a significant difference in mean relative illumination values based on tree management and radial distances from tree trunks to open fields (Figure 7). Under control tree management, illumination decreased closer to the trunk but increased with distance, with the highest values observed at D3 across all months, indicating reduced shading effects closer to the tree trunk. In contrast, pruned trees maintained relatively high illumination levels across all distances, with slight seasonal variation, suggesting reduced canopy density enhances light penetration. Pollarded trees consistently showed near 100% illumination at all distances and months, indicating minimal canopy cover and maximum light penetration.
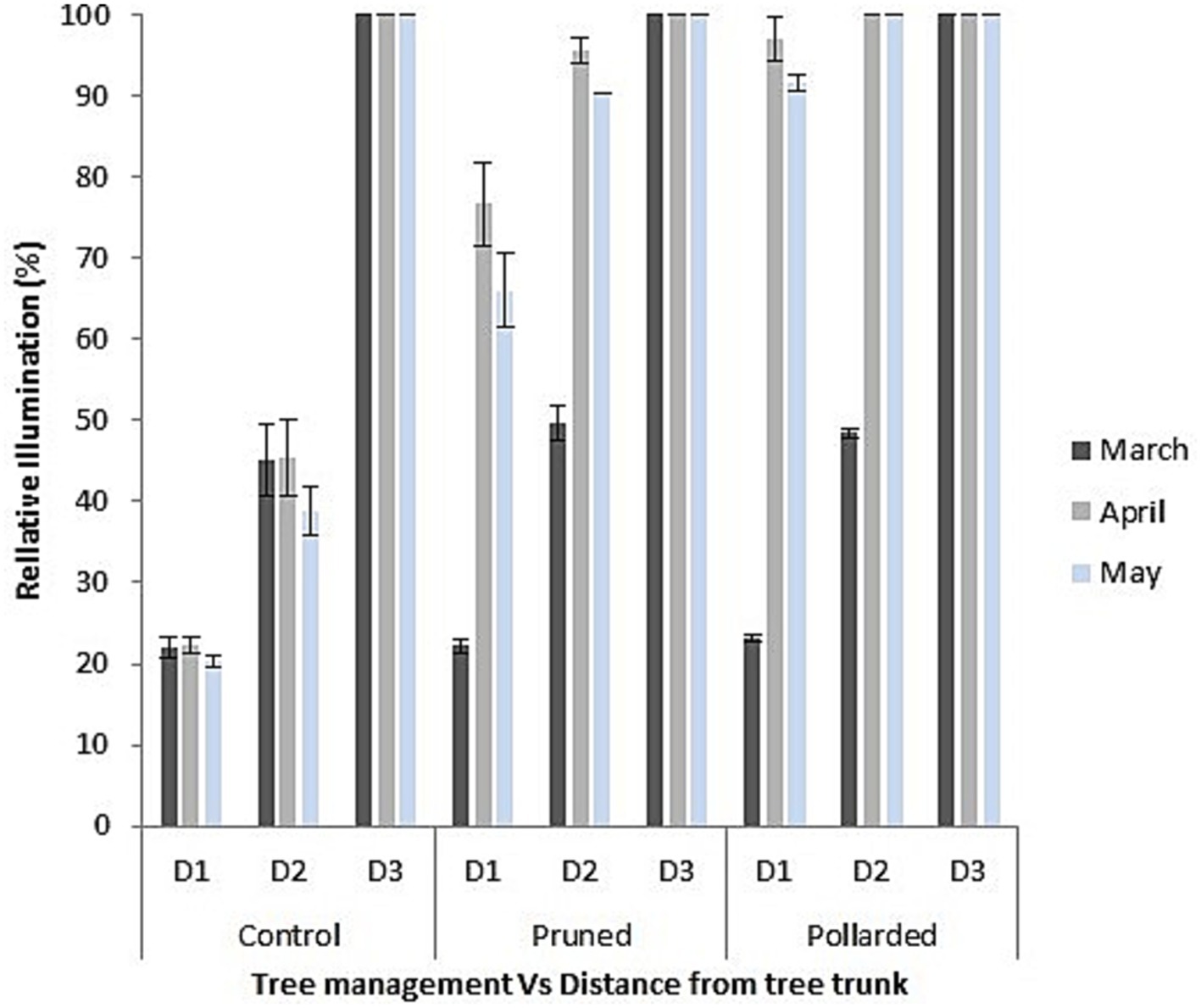
Figure 7. The figure depicts the relative illumination under the canopy of Terminalia brownii, segmented by different tree management practices (Control, Pruned, and Pollarded) and distances from the trunk D1, (0–4.1 m), D2 (4.1–8.2 m), and D3 (>15 m) for the months of March, April, and May.
Overall, the results illustrate how tree management practices influence canopy density and light penetration. Control trees maintain dense canopies, reducing light near the trunk. Pruning reduces this effect, increasing light availability, while pollarding minimizes canopy cover, maximizing illumination. Seasonal variations in March, April, and May reflect stable shading effects over time.
3.1.4 Below-canopy air temperature
The study found that different tree management practices (control, pruning, and pollarding) significantly influenced below-canopy air temperatures under Terminalia brownii. The air temperature near the tree trunk (0–4.1 m) under control tree management was lowering compared to greater distances from the trunk (Figure 8). This indicates that the dense canopy near the trunk had a cooling effect by reducing the amount of sunlight reaching the ground. As the distance from the tree trunk increased (4.1–8.2 m and > 15 m), the air temperature rose.
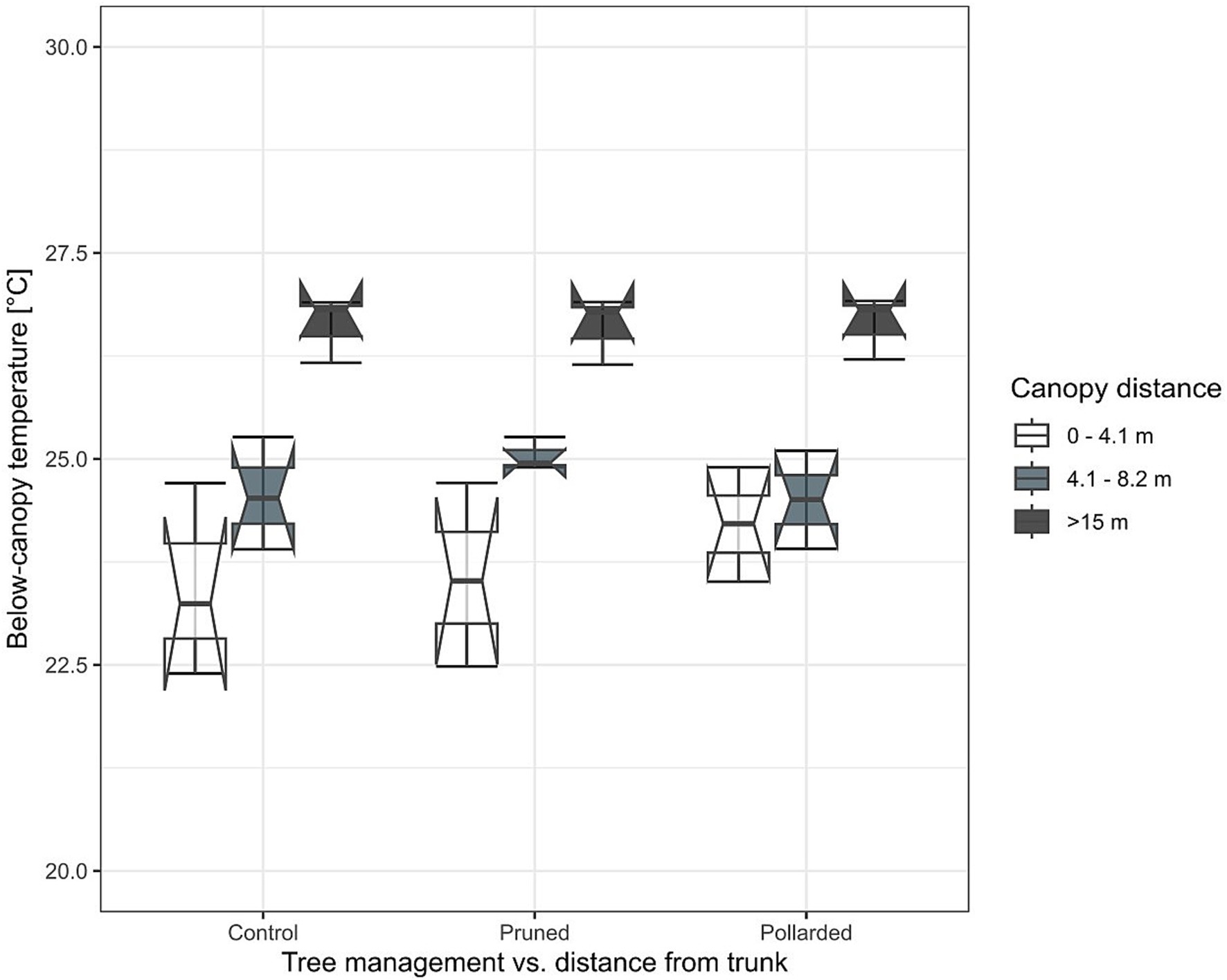
Figure 8. The figure depicts the mean below-canopy air temperature under the canopy of Terminalia brownii, segmented by different tree management practices (Control, Pruned, and Pollarded) and distances from the tree trunk (0–4.1 m, 4.1–8.2 m, and > 15 m). The notched box plots indicate the median (solid lines between the boxes), and interquartile range (IQR) with upper (75%) and lower (25%) quartiles as well as 95% confidence interval of the median = median ± 5.57*ICR/n0.5. The scattered points also indicate the outlier.
Under pruned tree management, air temperatures were more uniform across all distances from the tree trunk. Although the temperatures were slightly higher compared to those under the control tree managements, there was less variation between different distances from the trunk. This suggests that pruning reduces the canopy’s ability to cool the air underneath by allowing more sunlight to penetrate.
The highest below-canopy air temperatures were recorded under pollarded trees. This trend was observed at all distances, showing that the more extensive removal of canopy cover through pollarding resulted in the least effective cooling effect. The uniformity of high temperatures across all distances highlights that pollarding significantly reduces the ability of the canopy to lower air temperatures under the tree.
Overall, the results demonstrated that control trees with dense canopies provide the most substantial cooling effect, particularly near the tree trunk. Pruning reduces this effect, resulting in more uniform, yet higher, temperatures. Pollarding minimizes the cooling effect entirely, leading to the highest temperatures under the tree canopy.
3.2 Effects canopy management of Terminalia brownii on yield and yield components of maize
In Table 2, the mean values of yield and yield component of maize under all managed tree canopies, as well as the radial distances from tree trunks and their interactions, show significant differences (p < 0.05). The highest grain yields were recorded under pruned (4,947 Kg ha−1) and pollarded (4,968 Kg ha−1) trees, at D2, and the lowest grain yield (2,146 Kg ha−1) was recorded under control trees at D1. In terms of tree canopy management, the highest grain yields (3,717 Kg ha−1 and 3,719 Kg ha−1) were recorded on average under the pruned and pollarded trees, respectively, whereas the lowest grain yield (2,643 Kg ha−1) was recorded on average under control. Generally, the result showed that the grain yield and yield components of maize at the canopy zone, particularly at the edge of the tree canopy, were higher than in the open field, whereas, under pruned and pollarded trees, the grain yield and yield components of maize at the canopy zone were highly significant compared to the control.
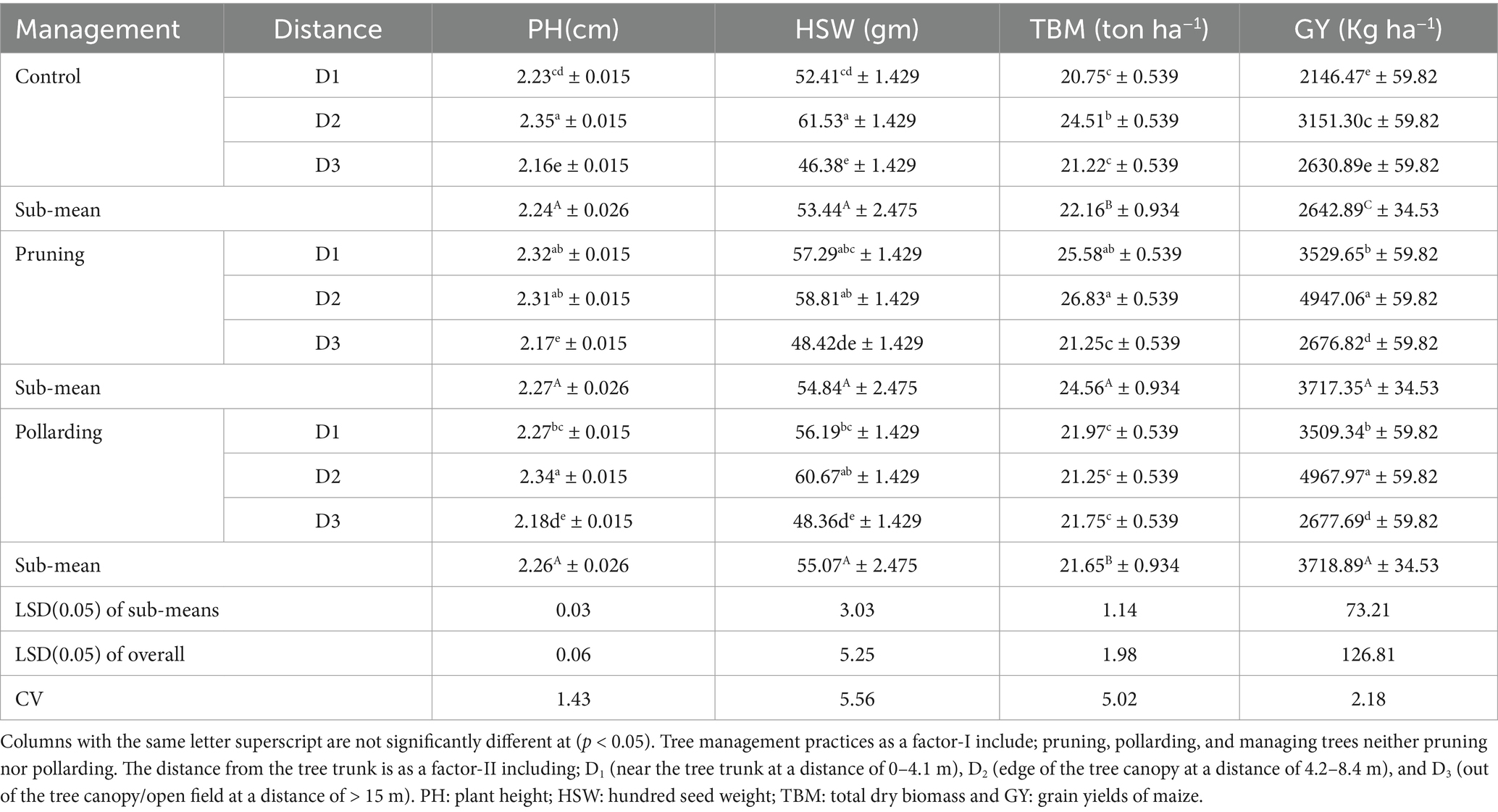
Table 2. Effect of Terminalia brownii tree canopy management on Maize yield and yield components, given as mean ± standard deviation of three times replicates.
3.3 Status of soils under the canopies of Terminalia brownii and outside
3.3.1 Soil pH
The soil pH values did not differ significantly at different distances from the trunks of Terminalia brownii trees. According to Table 3, the surface, subsurface, and overall mean soil pH values were not statistically significant (p < 0.05). However, there was a slight decrease in the mean soil pH values as the distance from the tree trunk increased towards the open field. The median soil pH values also showed significant differences between the area under the tree canopy and the open field at all radial distances.
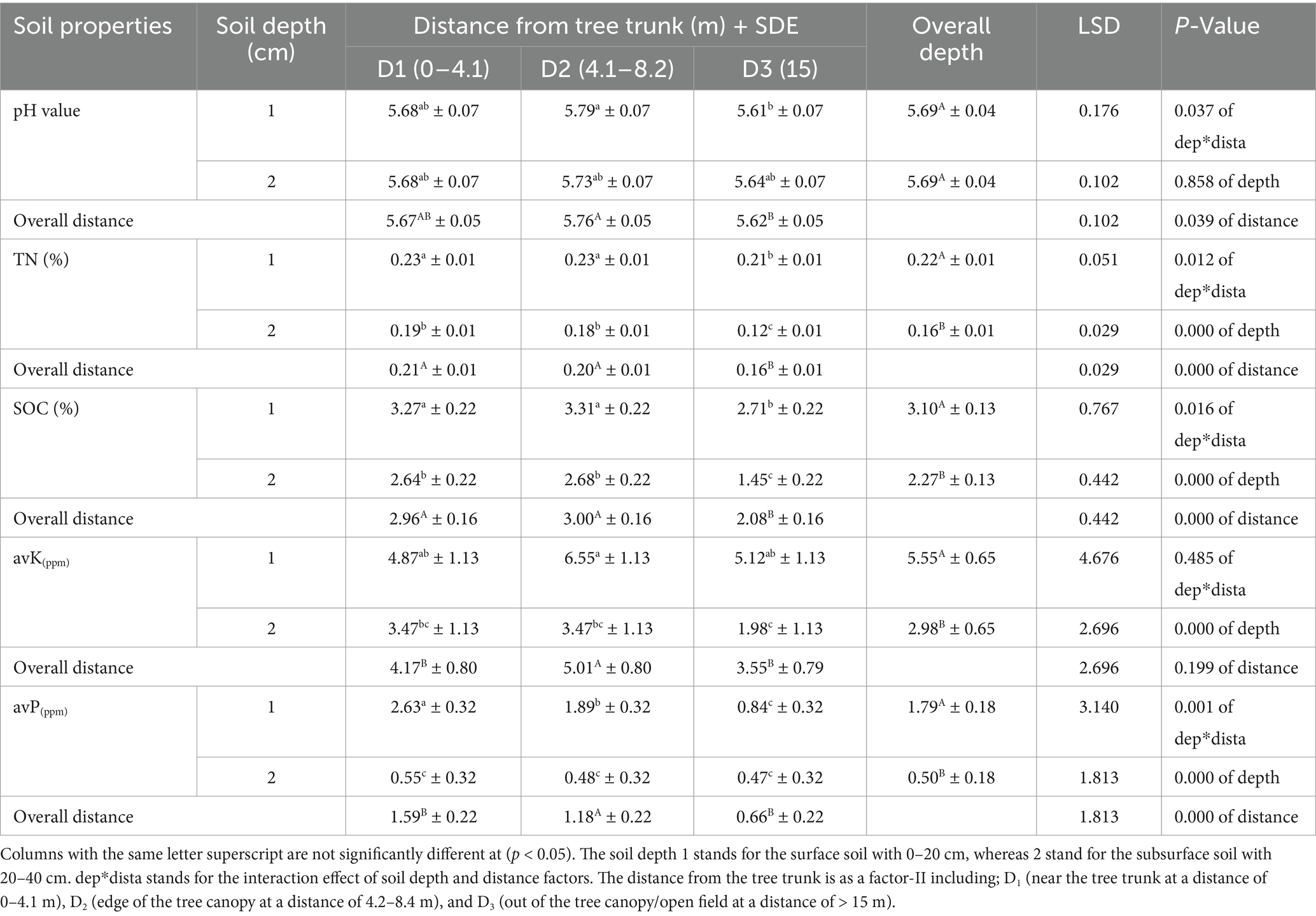
Table 3. Effect of distance from Terminalia brownii and soil depth on TN, SOC, avK, and avP, given as mean ± standard deviation of three times replicates.
3.3.2 Soil organic carbon (SOC) and total nitrogen (TN)
The data in Table 3 shows significant differences (p < 0.05) in SOC and TN levels among all radial distances and soil depths. Both surface and subsurface soil depths exhibit a declining trend in SOC and total nitrogen with increasing radial distances, likely due to high litter accumulation from above- and below-ground biomass.
3.3.3 Plant-available potassium (avK) and plant-available phosphorus (avP)
The mean values of avK depict a significant difference (p < 0.05) between both radial distances and soil depths, as described in Table 3. The avK on the soil surface and under the canopy of Terminalia brownii was higher as compared to subsurface soil and open fields. This may be due to a higher accumulation of above- and below-ground biomass both in the tree canopy and soil surface and high biological activities.
Plant-available phosphorus was significantly higher under the canopy of the Terminalia brownii than in the open fields and the surface soil than subsurface soil, as shown in Table 3. High organic matter accumulation under the tree canopy might lead to higher avP.
3.4 Relationship between maize grain yield and other parameters
The correlation analysis result showed that tree management (control, pollarded and pruned) and soil parameters such as pH, TN, SOC, avP, and avK were positively and significantly correlated to maize grain yield except the distance from tree trunk (r = −0.506), as shown in Table 4. This may be due to the increased grain yield with increasing distance from tree trunk as a result of minimized shading effect. However, the correlation analysis result under control trees showed that soil parameters such as pH, TN, SOC, avP, and avK were positively and significantly correlated to maize grain yield including the distance from tree trunk (i.e., inverse to the results under canopy managed trees), as shown in Table 5. This may be due to the decreasing maize yield with increasing distance from the tree trunk as a result of the shading effect.
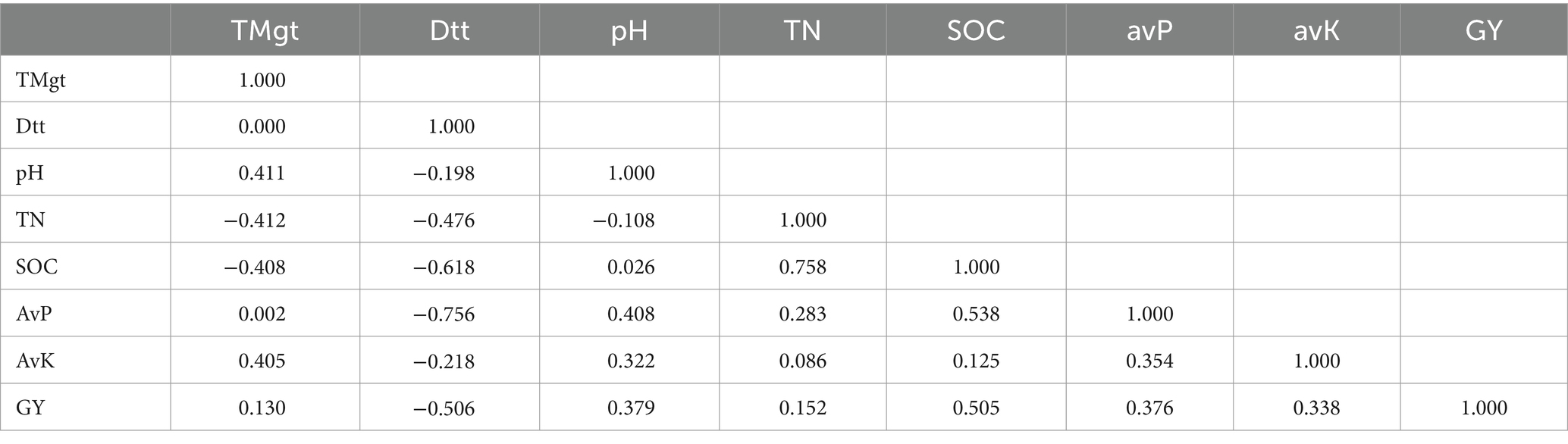
Table 4. Pearson correlation between maize grain yield and tree management, distance and soil parameters under canopy managed (pollarded and pruned) trees.
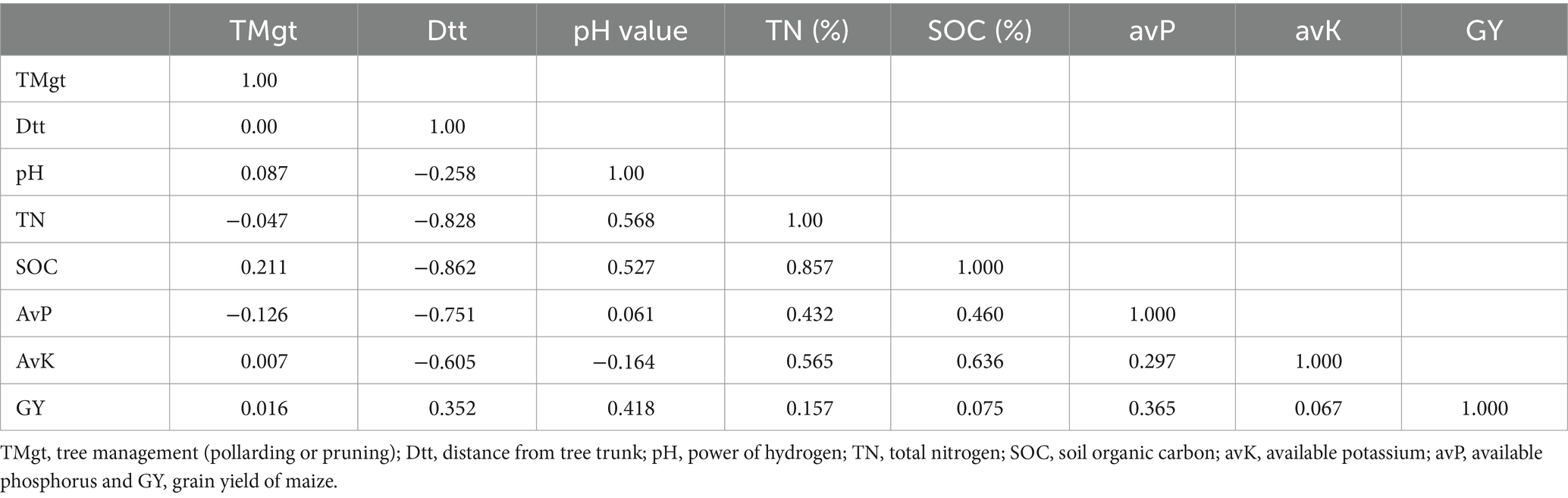
Table 5. Pearson correlation between maize grain yield and tree management, distance and other soil parameters under control (neither pruned nor pollarded) trees.
The result of the correlation analysis under canopy-managed (pollarded and pruned) trees showed that the distance from tree trunk and microclimate such as relative illumination, soil, and air temperatures were negatively and significantly correlated to maize grain yield except for soil moisture content (r = 0.042) and tree management(pollarding and pruning) (r = 0.130), as described in Table 6. This may be due to the increased grain yield with increasing distance from tree trunk as result of minimized shading effect. Lower illumination implies that removing the canopy of trees improves light penetration and thus crops yield. However, the result of correlation analysis under control (neither pruned nor pollarded) trees showed that tree management and microclimate parameters such as relative illumination, soil moisture content, and soil and air temperatures were positively and significantly correlated to maize grain yield except the distance from a tree trunk, as shown in Table 7. This may be due to the decreasing maize yield with increasing the distance from the tree trunk, even increased soil moisture content as a result of shading effect.
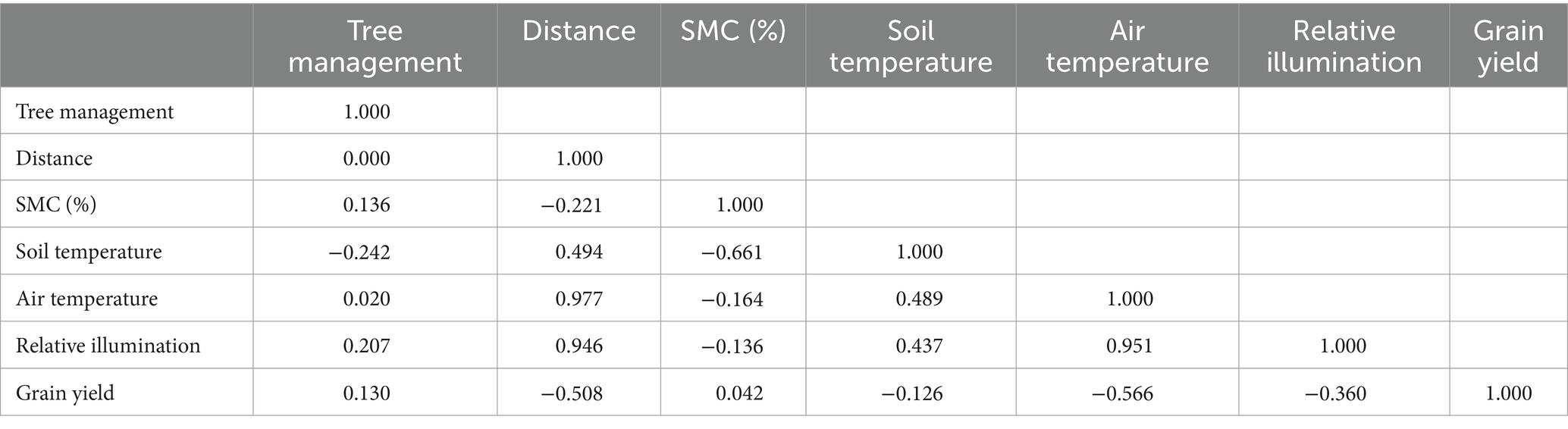
Table 6. Pearson correlation between maize grain yield and tree management, distance and microclimate parameters under canopy managed (pollarded and pruned) trees.
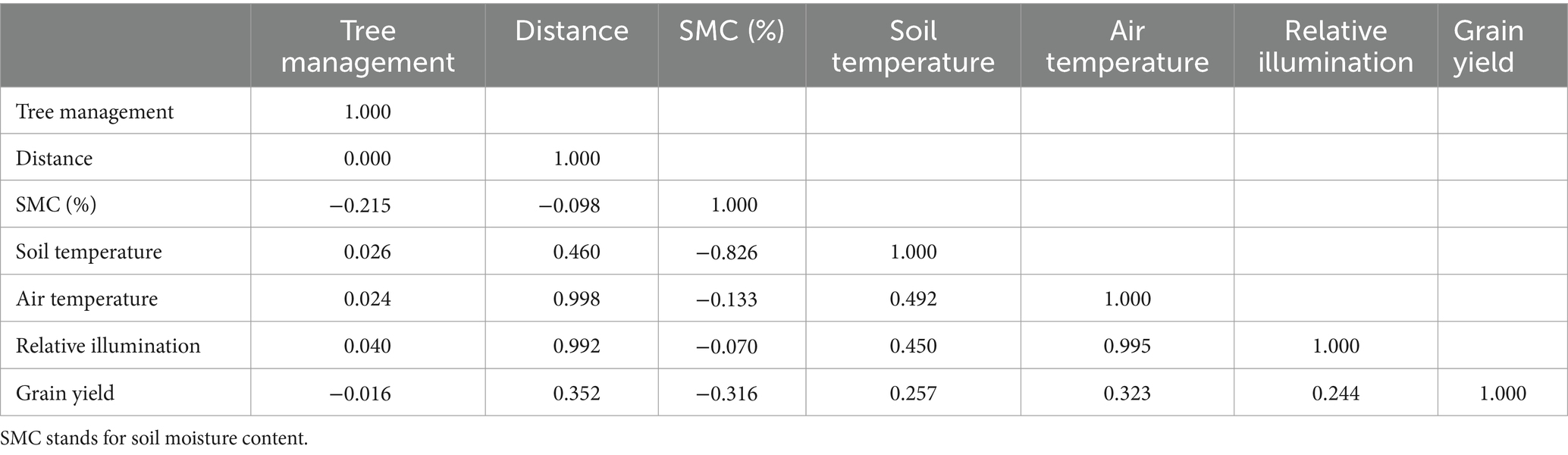
Table 7. Pearson correlation between maize grain yield and microclimate and tree management, distance and microclimate parameters under control (neither pruned nor pollarded) trees.
4 Discussion
4.1 Effects of Terminalia brownii tree management on microclimate
4.1.1 Soil moisture content
The findings of our study depict the significant role that canopy management plays in altering the microclimatic conditions in agroforestry parklands. The higher soil moisture observed in the control treatment aligns with the idea that intact tree canopies provide better shade and reduce evaporation from the soil surface, thereby conserving moisture. In contrast, the pruned and pollarded trees, which have reduced canopy cover, exhibit lower moisture content, especially in deeper soils and at greater canopy distances. These results are consistent with previous studies, which exhibit the soil moisture content was higher beneath the canopy zone (19.6%) on the surface and on the subsurface (10.9%) than on the surface (15.9%) and on the subsurface (8.9%) outside the canopy of the Millettia ferruginea tree (Hailu et al., 2000). Similar findings were observed for Acacia seyal in Guba Lafto district, North Wollo, Ethiopia by Belayneh et al. (2021), Cordia africana and Croton macrostachyus trees in Eastern Oromia, Ethiopia by Mohammed et al. (2018), and Balanties aegyptiaca, Acacia tortilis, and Acacia seyal in the central rift valley of Ethiopia by Agena et al. (2014).
The significant reduction in soil moisture under pollarded conditions, particularly in deeper soil layers, indicates that extensive canopy removal may compromise the trees’ ability to regulate moisture retention, which can have implications for crop growth, especially in water-limited environments. These findings are important in the context of agroforestry systems, where soil moisture conservation is crucial for crop yield, particularly in regions experiencing variable rainfall patterns due to climate change.
4.1.2 Soil temperature
Canopy management of Terminalia brownii trees significantly influences soil temperature, with the intact canopy (control treatment) providing the most effective cooling effect, especially near the tree trunk where the dense canopy shade reduces solar radiation reaching the soil. This shading results in lower surface soil temperatures compared to more open areas. The pruned treatment, though still cooler than exposed areas, allows moderately higher soil temperatures as reduced canopy cover diminishes the soil’s protection from direct sunlight, particularly further from the trunk. Pollarding, which removes substantial canopy cover, showed the least cooling effect, leading to the highest soil temperatures, especially near the surface (Dep 1) where exposure to sunlight is greatest, with even subsurface soils (Dep 2) experiencing increased warmth, albeit less intensively than at the surface.
The comparative analysis across different tree management practices underscores the vital role of canopy cover in regulating soil temperature. Dense canopies under control management provide the most substantial cooling effect, while pruning and pollarding reduce this benefit to varying degrees. These findings emphasize the importance of maintaining adequate canopy cover in agroforestry systems to optimize soil temperature regulation, which is crucial for moisture retention, soil health, and overall crop performance.
The cooling effect of tree canopies on soil temperature is well documented in agroforestry systems. For example, Akpo et al. (2009) found that soil temperatures under Acacia tortilis in a Senegal savanna were reduced by up to 6°C and similar reductions (5°C to 10°C) were observed under Faidherbia albida canopies. In the central rift valley of Ethiopia, Agena et al. (2014) also reported significantly reduced soil temperatures under Acacia tortilis and Balanties aegyptiaca compared to open fields. These findings are consistent with the results of the present study, reinforcing the role of tree canopies in moderating soil temperature by providing shade.
The cooling effect of tree canopies is particularly important in dryland agroforestry systems, where high soil temperatures can exacerbate moisture loss and negatively affect plant growth. High soil temperatures can exacerbate moisture loss and negatively impact plant growth. By regulating soil temperature, tree canopies help to create a more favorable microclimate for crops and enhance soil health. However, the extent of this effect depends on the level of canopy cover, as demonstrated by the differences between control, pruned, and pollarded tree management practices. Maintaining an optimal canopy structure is therefore crucial for achieving the benefits of agroforestry in terms of soil temperature regulation, moisture retention, and crop performance.
Recent studies have further highlighted the importance of tree canopy management in regulating soil temperature and microclimate (Yin et al., 2024). For instance, a systematic review on the cooling benefits of urban tree canopies found that tree canopies effectively alleviate the urban heat island effect through shading and evapotranspiration, regulating the urban thermal environment (Yin et al., 2024). Another study revealed that smaller canopy trees have a better cooling effect than larger canopy trees for the same level of coverage (Wang et al., 2015). Additionally, research on temperature patterns in tree canopies across diverse heights and types emphasized the relationship between canopy height and temperature regulation (Shaik et al., 2023).
4.1.3 Relative illumination
Our study showed that tree canopy management greatly affects light penetration under Terminalia brownii trees. In the control treatment, where the canopy remains dense, substantial shading occurs near the trunk, leading to lower light levels close to the tree and increasing at greater distances (D3) as shading diminishes. Pruning the canopy allows for higher light levels across all distances compared to the control, especially near the trunk, though some shading persists. Seasonal light variation is minimal under pruned canopies, indicating sustainable stable light availability year-round. Pollarding, which removes most of the canopy, allows nearly full light penetration across all distances and seasons, creating a consistent high-light environment due to the absence of shading effects.
This finding highlights the potential benefits of canopy management in agroforestry systems, especially in terms of optimizing light availability for understory crops. For example, pruning and pollarding can be strategically applied to enhance light penetration, thereby potentially improving crop yields by reducing the negative effects of shading. While dense canopies under unmanaged trees provide shade that may be beneficial in certain contexts (e.g., reducing soil temperature and moisture loss), reducing canopy cover through pruning or pollarding can be advantageous in systems where light availability is a limiting factor for crop growth.
Similar studies support these findings. For instance, Agena et al. (2014), reported that relative illumination under Balanties aegyptiaca was reduced by 86% in May, 87.9% in June, and 91.44% in July at 1/3 crown radius, compared to open fields. These results are consistent with the current study, showing the significant impact of canopy cover on light interception. Similarly, Mohammed et al. (2018) found a significant difference in mean relative illumination values at various radial distances from Cordia africana and Croton macrostachyus trees, with maximum light penetration (100%) at 15 m from the tree trunk and minimum values (40–44%) at 2.5 m, aligning with the observed patterns under Terminalia brownii in this study.
4.1.4 Below-canopy air temperature
Our study emphasizes the critical role of tree management practices in regulating below-canopy air temperatures. Control trees, with their dense canopies, create a significant cooling effect by intercepting sunlight and reducing heat transmission to the ground. This cooling effect is more pronounced near the tree trunk and decreases as the distance from the trunk increases. This gradient suggests that maintaining a dense canopy near the tree trunk is crucial for regulating temperatures, especially in agroforestry systems where microclimate management is important for enhancing agricultural productivity.
Pruned trees, by contrast, demonstrate a more uniform temperature distribution due to the thinning of the canopy. While this allows for greater sunlight penetration, which can be beneficial for crops requiring abundant light, it also reduces the cooling effect provided by the tree. The relatively higher temperatures observed under pruned trees indicate that although pruning improves light availability, it comes at the cost of reduced cooling, which may increase heat stress on crops.
Pollarded trees showed the highest air temperatures, indicating that the removal of a large portion of the canopy significantly reduces the tree’s ability to cool the area beneath it. The uniformity of these high temperatures across all distances from the tree trunk underscores the diminished role of the canopy in regulating temperatures. Pollarding may, therefore, be less suitable in agroforestry systems where microclimate regulation is a priority, as the cooling effect is minimized.
Dense canopies under control management provide a substantial cooling effect, which can help mitigate heat stress on crops and improve resilience in agricultural systems. On the other hand, while pruning and pollarding can enhance light penetration and potentially benefit crops that thrive in full sunlight, they also reduce the cooling capacity of the tree, which could negatively impact crop productivity in hotter climates. Previous studies, such as those on Balanties aegyptiaca, similarly demonstrated an increase in air temperature with distance from the tree trunk, supporting the idea that canopy density plays a critical role in microclimate regulation (Agena et al., 2014).
4.2 Effects canopy management of Terminalia brownii on yield and yield components of maize
Our finding indicated that the highest yields observed under pruned and pollarded trees, especially at the edge of the tree canopy, can be attributed to the moderated shading effect and improved soil conditions provided by these canopy management practices. The pruning and pollarding of trees likely reduce excessive shading, allowing more photosynthetically active radiation (PAR) to reach the crops, while still maintaining enough canopy cover to improve soil moisture retention and nutrient availability. This balance between shade and sunlight may enhance both above-ground and below-ground growing conditions for maize, resulting in higher yields.
In contrast, the lowest yield recorded under control trees, particularly near the trunk, can be explained by more intense shading, which reduces the amount of sunlight available for crop growth. Excessive shading may limit photosynthesis, reducing crop growth and yield. Additionally, competition for soil moisture and nutrients between the tree roots and maize plants is likely more intense near the tree trunk, further suppressing crop yields.
The current findings align with previous studies, which have also reported increased yields of maize and sorghum under the canopy of Faidherbia albida compared to outside the canopy (Abdella et al., 2020; Haile et al., 2021). These studies suggest that tree canopies, when managed appropriately, can create favorable microclimates and improve soil fertility, thereby enhancing crop performance. However, other studies, such as those by Haile et al. (2021) and Mohammed et al. (2018), report contrasting results where yields of maize and wheat were higher outside the canopy of Cordia africana, Faidherbia albida, and Acacia seyal, and sorghum yields were lower under the canopy of Croton macrostachyus. This variation in findings could be due to species-specific differences in canopy structure, shading effects, and root competition.
Sida et al. (2018) also observed a negative correlation between the presence of Croton macrostachyus and maize yield, with lower yields under the tree canopy (1,994 Kg ha−1) compared to the open field (3,415 Kg ha−1). This supports the idea that excessive shading and competition from certain tree species can reduce crop yields, highlighting the need for careful tree management in agroforestry systems to optimize both tree and crop productivity.
4.3 Status of soils under the canopies of Terminalia brownii and outside
4.3.1 Soil pH
The lack of significant variation in soil pH across different distances from the tree trunk suggests that Terminalia brownii trees have a relatively uniform influence on soil pH within their canopy zone. The slight decrease in pH values observed as the distance from the trunk increased may be attributed to a gradual reduction in the tree’s influence on soil conditions as one move further away from the trunk. This could result from a combination of organic matter deposition, root activity, and canopy-mediated effects being stronger closer to the trunk, but still minor overall.
These findings are consistent with previous studies. Belay et al. (2014) observed that soil pH under the canopy of scattered Faidherbia albida and Croton macrostachyus trees did not differ significantly from that of the open field, suggesting that the impact of tree canopies on soil pH may be minimal in agroforestry systems. Similarly, Aweto and Dikinya (2003) reported no significant differences in soil pH under the canopy of scattered Combretum apiculatum and Peltophorum africanum trees compared to open fields in semi-arid environments.
The slight decrease in soil pH with distance from the tree trunk might be linked to reduced organic matter inputs and biological activity further from the tree, but overall, the influence of Terminalia brownii on soil pH appears to be limited. This suggests that, while trees in agroforestry systems can modify other soil properties (e.g., organic matter, moisture content), their influence on soil pH may be relatively modest unless they are species known for more dramatic impacts on soil chemistry.
4.3.2 Soil organic carbon (SOC) and total nitrogen (TN)
The significant differences in SOC and TN levels between radial distances from the tree trunk highlight the role of Terminalia brownii canopies in influencing soil nutrient content. The higher levels of SOC and TN near the trunk can be attributed to the greater accumulation of leaf litter, root exudates, and other organic inputs close to the tree. As distance from the trunk increases, the influence of the tree canopy diminishes, resulting in lower levels of organic matter and nutrients in the soil.
This trend aligns with findings from previous studies, which have consistently reported higher SOC levels under tree canopies compared to open fields. For example, (Aweto and Dikinya, 2003) found that SOC was 47 and 55% higher under the canopies of Combretum apiculatum and Peltophorum africanum, respectively, compared to open fields in southeastern Botswana. Similarly, Cordia africana has been shown to significantly enhance SOC levels under its canopy compared to areas outside the canopy (Mohammed et al., 2018).
The enhanced SOC content under tree canopies is often a result of increased organic inputs such as leaf litter, root biomass, and microbial activity. Trees contribute organic matter through leaf fall and root decomposition, enriching the soil with carbon and nitrogen. Furthermore, the shade provided by tree canopies reduces evaporation and maintains soil moisture, which can promote microbial activity and nutrient cycling. This pattern is consistent across various tree species, including Faidherbia albida, Olea abyssinica, Dalbergia melanoxylon, Acacia seyal, Cordia africana, and Croton macrostachyus, all of which have been reported to significantly increase SOC and TN levels in the soil under their canopies (Gebrewahid et al., 2018; Mohammed et al., 2018; Tsegu, 2019; Abdella et al., 2020).
4.3.3 Plant-available potassium (avK) and plant-available phosphorus (avP)
The The elevated avK levels on the soil surface and under the canopy of Terminalia brownii suggest that tree canopies play an important role in nutrient accumulation. The higher avK content near the tree may be attributed to the accumulation of organic matter from above- and below-ground biomass, including leaf litter, root exudates, and microbial activity. This aligns with previous studies showing that trees such as Balanites aegyptiaca, Acacia tortilis, Acacia seyal, Faidherbia albida, Terminalia brownii, and Vitex doniana significantly increase potassium availability in the soil (Berhe, 2013; Agena et al., 2014; Lemage and Anmaw, 2022). These species enhance soil fertility by promoting biological activity and organic matter input, both of which contribute to the higher availability of potassium in the soil.
However, some studies, such as Kassa et al. (2010), did not observe significant differences in avK based on radial distances and soil depths for Balanites aegyptiaca. These discrepancies could be explained by factors such as species differences, tree age, and site-specific conditions, which influence nutrient dynamics and distribution.
The significantly higher avP levels observed under the canopy of Terminalia brownii compared to the open field suggest that tree canopies also enhance phosphorus availability. The increased avP concentration in the surface soil under tree canopies is likely a result of higher organic matter inputs, which facilitate the release of phosphorus into the soil. This is consistent with findings from previous studies on Cordia africana, Croton macrostachyus, Dalbergia melanoxylon, Faidherbia albida, Olea abyssinica, and Millettia ferruginea, where higher phosphorus availability was reported under tree canopies compared to open fields (Yadessa et al., 2009; Mohammed et al., 2018; Abdella et al., 2020).
4.4 Relationship between maize grain yield and other parameters
The results of the correlation analysis underscore the complex interactions between tree management, soil parameters, and microclimate in determining maize grain yield. In canopy-managed systems (pollarded and pruned), the negative correlation between distance from the tree trunk and maize yield suggests that minimizing shading effects through tree canopy management enhances light penetration and thus improves crop yield. This finding is consistent with the hypothesis that reduced shading increases the availability of photosynthetically active radiation (PAR), promoting crop growth, particularly as one move further away from the tree trunk.
On the other hand, in control systems where the tree canopy remains intact, maize yield appears to benefit from the microclimatic moderation provided by the tree canopy. The positive correlation between proximity to the tree trunk and grain yield suggests that the shade provided by the canopy may protect crops from excessive heat and evapotranspiration, leading to better crop performance. This result contrasts with canopy-managed systems, where increased light availability is more critical for improving yield.
The mixed correlation between soil parameters and maize yield also indicates the importance of nutrient availability in driving crop productivity. Higher levels of pH, TN, SOC, avP, and avK were all positively correlated with maize yield, highlighting the role of nutrient-rich soils in supporting crop growth under different tree management practices. This is in line with previous research by Lemage and Anmaw (2022), which reported both negative and positive correlations between maize yield and soil parameters, depending on the distance from the tree trunks of Terminalia brownii and Vitex doniana.
Similarly, Mohammed et al. (2018) found positive correlations between maize yield and microclimatic parameters such as relative illumination, soil temperature, and air temperature, except for soil moisture content. These findings are consistent with the current study, where increased light availability and moderated temperatures were linked to higher crop yields, especially under canopy-managed systems.
5 Conclusion
The present study illustrates the significant influence of Terminalia brownii canopy management on microclimate, maize yield, and soil properties in agroforestry parklands. The canopy management practices, including pruning and pollarding, play a crucial role in creating optimal conditions for crop growth by balancing shading and light penetration, soil moisture, temperature, and air conditions beneath the canopy. The soil moisture content was significantly higher under the Terminalia brownii tree canopy compared to outside the canopy, and it decreased with increasing distance from the tree trunk to the open field. Surface soil moisture was also significantly higher than subsurface soil moisture. Additionally, relative illumination, soil temperature, and air temperature were lower under the canopy of Terminalia brownii tree compared to the open field but higher under pruned and pollarded trees compared to the control unmanaged trees.
The grain yield and yield components of maize in the canopy zone, particularly at the edge of the tree canopy, were higher than in the open field. Similarly, under pruned and pollarded trees, the grain yield and yield components of maize in the canopy zone were significantly higher than the control. This may be due to above and/or below-ground competition, such as competition for soil moisture and nutrients between the tree roots and maize, the shading effect of trees on the crops, reduced growth due to intercepted photosynthetic active radiation, and soil moisture dynamics. Hence, the adoption of retaining Terminalia brownii trees on cropland through either pollarding or pruning is beneficial for farmers rather than removing them, as it enhances maize yield by reducing shading effect, modifying the microclimate, and improving soil fertility. Pruning, in particular, strikes a balance between maintaining some shading effect and allowing increased light penetration, creating a moderated microclimate that improves conditions for maize growth. This moderate microclimate from pruning provides stable seasonal light availability and limits excessive temperature fluctuations, contributing to improved crop yield in agroforestry systems. Further research should explore long-term impacts and the potential benefits of integrating other tree species in agroforestry systems.
Data availability statement
The raw data supporting the conclusions of this article will be made available by the authors, without undue reservation.
Author contributions
MAH: Conceptualization, Data curation, Formal analysis, Funding acquisition, Investigation, Methodology, Project administration, Resources, Software, Validation, Visualization, Writing – original draft, Writing – review & editing. ZA: Data curation, Methodology, Supervision, Writing – review & editing. BG: Formal analysis, Funding acquisition, Methodology, Resources, Software, Supervision, Writing – review & editing. TB: Formal analysis, Methodology, Resources, Writing – review & editing. AG: Writing – original draft, Writing – review & editing, Formal analysis, Methodology, Software. BL: Data curation, Formal analysis, Methodology, Resources, Software, Supervision, Writing – original draft, Writing – review & editing.
Funding
The author(s) declare that financial support was received for the research, authorship, and/or publication of this article. This study was funded by the German Academic Exchange Service (DAAD) and Martin Luther University Halle-Wittenberg.
Acknowledgments
We extend our heartfelt gratitude to the German Academic Exchange Service (DAAD) for awarding Mr. Mitiku Ayele Handiso a scholarship in collaboration with the Wondo Genet College of Forestry and Natural Resources, Hawassa University. Our thanks also go to Mr. Aaron Tinschert for his soil sample analysis and to Dr. Betelhem Mekonnen for her invaluable suggestions, comments, and support during Mr. Handiso’s stay in Germany. We are deeply grateful to the agricultural and rural development experts of South Ari District and the Alga Kebele administrations for their unwavering support during site selection and data collection. Additionally, we express our sincere appreciation to our close friends, especially Mr. Belayneh Lemage, for their assistance with data collection, and to the Jinka Agricultural Research Center for their collaboration. Finally, we sincerely appreciate the editor and reviewers for their valuable feedback, thoughtful revisions, and kind invitation to submit the revised version of our manuscript.
Conflict of interest
The authors declare that the research was conducted in the absence of any commercial or financial relationships that could be construed as a potential conflict of interest.
Publisher’s note
All claims expressed in this article are solely those of the authors and do not necessarily represent those of their affiliated organizations, or those of the publisher, the editors and the reviewers. Any product that may be evaluated in this article, or claim that may be made by its manufacturer, is not guaranteed or endorsed by the publisher.
References
Abdella, M., Nigatu, L., and Akuma, A. (2020). Impact of parkland trees (Faidherbia albida Delile and Cordia Africana lam) on selected soil properties and Sorghum yield in eastern Oromia, Ethiopia. Agric. For. Fish. 9:54. doi: 10.11648/j.aff.20200903.13
Agena, A. T., Tilahun, F. E., and Bekele, L. (2014). Effects of three tree species on microclimate and soil amelioration in the central rift valley of Ethiopia. J. Soil Sci. Environ. Manag. 5, 62–71. doi: 10.5897/JSSEM12.060
Akpo, L., Goudiaby, V., Grouzis, M., and Le Houerou, H. (2009). Tree shade effects on soils and environmental factors in a savanna of Senegal. West Afr. J. Appl. Ecol. 7, 41–52. doi: 10.4314/wajae.v7i1.45647
Aweto, A., and Dikinya, O. (2003). The beneficial effects of two tree species on soil properties in a semi-arid savanna rangeland in Botswana. Land Contamin. Reclam. 11, 339–344. doi: 10.2462/09670513.618
Baier, C., Gross, A., Thevs, N., and Glaser, B. (2023). Effects of agroforestry on grain yield of maize (Zea mays L.)—a global meta-analysis. Front. Sustain. Food Syst. 7:1167686. doi: 10.3389/fsufs.2023.1167686
Bekele, S. E. (2018). Parkland agroforestry of Ethiopia; key to production, productivity, biodiversity conservation and climate change mitigation: a review. Open J. For. 8:472. doi: 10.4236/ojf.2018.84030
Belay, M., Tesfaye, A., and Abdu, A. (2014). Effects of scattered F. albida (Del) and C. macrostachyus (lam) tree species on key soil physicochemical properties and grain yield of maize (Zea Mays): a case study at umbulo Wacho watershed, southernWudpecker. J. Agric. Res. 3, 063–073.
Belayneh, A. H., Gebremedhin, K. G., and Egziabher, Y. G. (2021). Role of Acacia seyal on selected soil properties and Sorghum growth and yield: a case study of Guba Lafto District, north Wollo, Ethiopia. Int. J. Agron. 2021, 1–8. doi: 10.1155/2021/6666674
Berhe, H. (2013). Evaluation of the effect of Ficus thonningii (blume) on soil physicochemical properties in Ahferom district of Tigray, Ethiopia. J. Soil Sci. Environ. Manag. 4, 35–45. doi: 10.5897/JSSEM13.0369
Boffa, J. M. (2000). West African agroforestry parklands: Keys to conservation and sustainable management. Unasylva. Rome, Italy: Food and Agriculture Organization (FAO), 11–17.
Buraka, T., Elias, E., Suryabhagavan, K. V., and Lelago, A. (2022). Assessment of soil erosion risks in response to land-use and land-cover changes in Coka watershed, southern Ethiopia. Geol. Ecol. Landsc. 3:12. doi: 10.1080/24749508.2022.2109825
Gebrewahid, Y., Gebre-Egziabhier, T. B., Teka, K., and Birhane, E. (2018). Carbon stock potential of scattered trees on farmland along an altitudinal gradient in Tigray Northern Ethiopia. Ecol. Process. 7, 1–8. doi: 10.1186/s13717-018-0152-6
Girmay, G., Moges, A., and Muluneh, A. (2020). Estimation of soil loss rate using the USLE model for Agewmariayam watershed, northern Ethiopia. Agric. Food Sec. 9, 1–12. doi: 10.1186/s40066-020-00262-w
Haile, G., Lemenih, M., Itanna, F., and Agegnehu, G. (2021). Comparative study on the effects of Acacia albida on yield and yield components of different cereal crops in southern Ethiopia. Acta Agric. Scand. Sect. B 71, 453–465. doi: 10.1080/09064710.2021.1918234
Hailu, T., Negash, L., and Olsson, M. (2000). Millettia ferruginea from southern Ethiopia: impacts on soil fertility and growth of maize. Agrofor. Syst. 48:762. doi: 10.1023/A:1006274912762
Harmer, R. (2004). Silviculture | coppice Silviculture practiced in temperate regions. Encycl. For. Sci. 1045–1052. doi: 10.1016/b0-12-145160-7/00230-1
Harris, R. (1994). Clarifying certain pruning terminology: thinning, heading, pollarding. Arboricult. Urban For. 20:10. doi: 10.48044/jauf.1994.010
Kassa, H., Gebrehiwet, K., and Yamoah, C. (2010). Balanites aegyptiaca, a potential tree for parkland agroforestry systems with sorghum in northern Ethiopia. J. Soil Sci. Environ. Manag. 1, 107–114.
Lemage, B., and Anmaw, Y. (2022). Effect of farmland trees Terminalia brownie and Vitex doniana on soil physicochemical properties and maize yield. J. Innov. Agric. 9, 1–9. doi: 10.37446/jinagri/rsa/9.4.2022.1-9
Mamo, D., and Asfaw, Z. (2017). Status of selected soil properties under Croton Macrostachyus tree at Gemechis District, west Hararghe zone, Oromia, Ethiopia. J. Biol. Agric. Healthcare 7, 36–43.
Mesfin, K., Tesfaye, S., Girma, K., Dejene, A., and Tsegaye, G. (2017). Description, characterization and classification of the major soils in Jinka agricultural research center, South Western Ethiopia. J. Soil Sci. Environ. Manag. 8, 61–69. doi: 10.5897/jssem2015.0498
Mohammed, M., Beyene, A., and Reshad, M. (2018). Influence of scattered Cordiaafricana and Crotonmacrostachyus trees on selected soil properties, microclimate and maize yield in eastern Oromia Ethiopia. Am. J. Agric. For. 6, 253–262. doi: 10.11648/j.ajaf.20180606.23
Neglo, K. A. W., Gebrekidan, T., and Lyu, K. (2021). The role of agriculture and non-farm economy in addressing food insecurity in Ethiopia: a review. Sustainability 13:3874. doi: 10.3390/su13073874
Olsen, S. R. (1954). Estimation of available phosphorus in soils by extraction with sodium bicarbonate. Washington, D.C., USA: US Department of Agriculture.
Oluseye, O. C., Fatoba, P. O., Awotoye, O. O., and Olorunmaiye, K. S. (2013). Root-shoot partitioning of copper, chromium and zinc in Lycopersicon esculentum and Amaranthus hybridus grown in cement-polluted soil. Environ. Exp. Biol. 11, 131–136.
Salami, A., Kamara, A. B., and Brixiova, Z. (2010). Smallholder agriculture in East Africa: trends, constraints and opportunities. Working paper no.105 African development Bank.
Shaik, R. U., Jallu, S. B., and Doctor, K. (2023). Unveiling temperature patterns in tree canopies across Diverse Heights and types. Remote Sens. 15, 1–19. doi: 10.3390/rs15082080
Sida, T. S., Baudron, F., Hadgu, K., Derero, A., and Giller, K. E. (2018). Crop vs. tree: can agronomic management reduce trade-offs in tree-crop interactions? Agric. Ecosyst. Environ. 260, 36–46. doi: 10.1016/j.agee.2018.03.011
Thornton, P. K., Jones, P. G., Alagarswamy, G., Andresen, J., and Herrero, M. (2010). Adapting to climate change: agricultural system and household impacts in East Africa. Agric. Syst. 103, 73–82. doi: 10.1016/j.agsy.2009.09.003
Tolera, M., Asfaw, Z., Lemenih, M., and Karltun, E. (2008). Woody species diversity in a changing landscape in the south-central highlands of Ethiopia. Agric. Ecosyst. Environ. 128, 52–58. doi: 10.1016/j.agee.2008.05.001
Tscharntke, T., Clough, Y., Bhagwat, S. A., Buchori, D., Faust, H., Hertel, D., et al. (2011). Multifunctional shade-tree management in tropical agroforestry landscapes - a review. J. Appl. Ecol. 48, 619–629. doi: 10.1111/j.1365-2664.2010.01939.x
Tsegu, E. (2019). The role of Faidherbia albida tree species in parkland agroforestry and its management in Ethiopia. J. Horticul. For. 11, 42–47. doi: 10.5897/JHF2018.0570
Wang, Y., Bakker, F., de Groot, R., Wortche, H., and Leemans, R. (2015). Effects of urban trees on local outdoor microclimate: synthesizing field measurements by numerical modelling. Urban Ecosyst. 18, 1305–1331. doi: 10.1007/s11252-015-0447-7
Yadessa, A., Itanna, F., and Olsson, M. (2009). Scattered trees as modifiers of agricultural landscapes: the role of waddeessa (Cordia africana lam.) trees in Bako area, Oromia, Ethiopia. Afr. J. Ecol. 47, 78–83. doi: 10.1111/j.1365-2028.2008.01053.x
Keywords: crop productivity, open field, pruning, pollarding, tree-crop interaction, shading effects
Citation: Handiso MA, Asfaw Z, Glaser B, Bromm T, Gross A and Lemma B (2024) Effects of canopy management of umbrella tree (Terminalia brownii Fres.) on microclimate and maize (Zea mays L.) yield in agroforestry parkland of South Ari District, southern Ethiopia. Front. Sustain. Food Syst. 8:1464609. doi: 10.3389/fsufs.2024.1464609
Edited by:
Alexandra Jacquelyn Gibbs, University of Nottingham, United KingdomReviewed by:
Kokou Adambounou Amouzou, African Plant Nutrition Institute (APNI), MoroccoNsharwasi Nabahungu, International Institute of Tropical Agriculture (IITA), Democratic Republic of Congo
Copyright © 2024 Handiso, Asfaw, Glaser, Bromm, Gross and Lemma. This is an open-access article distributed under the terms of the Creative Commons Attribution License (CC BY). The use, distribution or reproduction in other forums is permitted, provided the original author(s) and the copyright owner(s) are credited and that the original publication in this journal is cited, in accordance with accepted academic practice. No use, distribution or reproduction is permitted which does not comply with these terms.
*Correspondence: Mitiku Ayele Handiso, bWl0aWt1YXllbGU0M0BnbWFpbC5jb20=
†ORCID: Mitiku Ayele Handiso, https://orcid.org/0000-0003-2206-3419
Zebene Asfaw, https://orcid.org/0000-0002-9095-5032
Bruno Glaser, https://orcid.org/0000-0002-3057-3868
Tobias Bromm, https://orcid.org/0000-0001-5914-2917
Arthur Gross, https://orcid.org/0000-0002-4076-5118
Bruk Lemma, https://orcid.org/0000-0002-9943-2158