- 1Rice Research and Training Center, Field Crops Research Institute, Kafrelsheikh, Egypt
- 2Nanotechnology and Advanced Materials Central Laboratory, Agricultural Research Center, Giza, Egypt
- 3Department of Crop Sciences, University of Illinois Urbana-Champaign, Urbana, IL, United States
- 4Plant Production Department, College of Food and Agriculture Sciences, King Saud University, Riyadh, Saudi Arabia
Researchers have repeatedly emphasized how urgently we have to decrease the massive nitrogen fertilizer consumption to support agricultural productivity and maintain a sustainable ecosystem. Using chitosan (CS) as a carrier for slow release is considered a potential tool for reducing synthetic fertilizer and improving crop productivity. Therefore, two field experiments were arranged in a randomized complete block design to investigate the effects of seven treatments including synthetic fertilizer and exogenous application of chitosan-based NPK nano-structure (Ch/NPs-NPK) on growth, productivity, and nutrient uptake traits of rice as a worldwide strategy crop during 2022 and 2023 growing seasons. The experimental treatments were: T1 = full recommended synthetic NPK (recommended urea, superphosphate, potassium sulfate; control treatment), T2 = 70% of T1 + Ch/NPs-NPK 100 ppm, T3 = 70% of T1+ Ch/NPs-NPK 200 ppm, T4 = 70% of T1+ Ch/NPs-NPK 300 ppm, T5 = 30% of T1+ Ch/NPs-NPK 100 ppm, T6 = 30% of T1+ Ch/NPs-NPK 200 ppm, and T7 = 30% of T1 + Ch/NPs-NPK 300 ppm. The results revealed that T4 (i.e., 70% of recommended NPK+ Ch/NPs-NPK 300 ppm) and T1 (full recommended synthetic NPK) resulted in the highest and most significant growth and yield traits of rice as well as nutrient grain contents compared to other treatments. Therefore, combining 70% of recommended NPK with Ch/NPs-NPK 300 ppm as an exogenous application can be a smart choice for reducing synthetic NPK fertilizers by 30% in paddy fields without producing a significant decline in terms of growth, yield characteristics, or nutrient grain contents when applying the full recommended synthetic NPK.
1 Introduction
Synthetic fertilizers play a fundamental role in guaranteeing food for a growing population (Seleiman et al., 2021). However, many countries overapply fertilizers, resulting in huge ecological problems including contamination of underground water, biodiversity loss, and decreased soil fertility (Seleiman et al., 2020; Zou et al., 2021; Dimkpa et al., 2023). Egypt is an example of a highly fertilizer-consumed country, where, fertilizer consumption increased from 136.2 kg/ha in 1971 up to 473.4 kg/ha in 2020 with an annual increasing rate of 2.98%. Moreover, massive amounts of synthetic fertilizers are lost via leaching, degradation, runoff, and volatilization which has adverse environmental and economic impacts (Vejan et al., 2021; Almutari, 2023). Therefore, deep attention has to be paid to reduce the intensive utilization of agrochemical fertilizers to reinforce sustainable agriculture production (Seleiman et al., 2017).
The main cereals such as wheat, barley, rice, and maize are a daily basis for human food supply and the largest agrochemicals consumers in the agriculture sector (Ladha et al., 2016; Hafez and Seleiman, 2017; Elshayb et al., 2022b; Alhammad et al., 2023; Haydar et al., 2024). Of the major cereals, rice is regarded as an essential crop that provides food for almost half of the global population (Carrijo et al., 2017; Badawy et al., 2021). Rice nutrient requirements are driven by balanced applications among nitrogen, phosphorus, and potassium (NPK) at different rates (Vijayakumar et al., 2024). The synthetic fertilizers of NPK have been the cornerstone of rice production because of the critical role of these nutrients in many physiological pathways such as the photosynthesis process, mitotic activities, root proliferation, tissue growth, and development (Seleiman et al., 2022). Although the growers mostly relied on synthetic fertilizers to increase rice production, the excessive amounts and unbalanced NPK ratios led to several issues with the soil (e.g., soil acidification, decrease in both soil organic matter and salt accumulation, and reduced cation exchange capacity) plus the negative effects on environmental integrity (Upadhyay et al., 2023). Therefore, environmentally friendly solutions that aim to reduce NPK inputs, preserve crop production outcomes, and overcome negative climate perturbations should be considered when considering fertilizer inputs, particularly NPK, in paddy fields.
In such a scenario, nano-fertilizers are one of the most advanced interventions with appropriate formulations with a smart mechanism to achieve optimal plant uptake and avoid nutrient losses (Badawy et al., 2021; Elshayb et al., 2022a; Verma et al., 2022; Devi et al., 2023; Kumar et al., 2023; Tomar et al., 2024). The application of nano-fertilizers to soil or sprayed over the vegetative system greatly enhances plant nutrition (Batool et al., 2024; Mukhtiar et al., 2024; Singh et al., 2024). With lower molecular weights, better bioavailability, longer half-lives, and a higher surface area-to-volume ratio, nanoparticles are more effective (Sarkar et al., 2022; Ferradj et al., 2024). Particularly biological nano fertilizers hold a lot of potential as an instrument for sustainable agriculture. In contrast to mineral fertilizers, these fertilizers may have a high nutrient use efficiency and a slow-release nutrient profile, which means they give plants the nutrients they require over a longer time (Sangwan et al., 2023). Notably, there are various types of nano fertilizers, including those based on macronutrients (N, K, and P) (Chakraborty et al., 2023). As a result of its wide surface area and capability, the macronutrients (N, P, and K) in nanocomposite fertilizer encourage the absorption use of nutrients by crops resulting in increasing plant height, dry matter, leaf area, chlorophyll synthesis, and photosynthesis rate (Sundararajan et al., 2024).
One of these smart nano-formulations, chitosan-based nanostructure (Ch/NPs) has gained more attention from scientists in the past few years owing to its biodegradable and biocompatible features (Abdel-Aziz et al., 2018; Ingle et al., 2022; Devi et al., 2023; Sangwan et al., 2023). It is worth mentioning that chitosan is a polycationic polymer that is isolated from chitin which is the second most abundant polysaccharide globally (a structural polymer in many different types of animals, mollusks, shrimp, fungi, and crabs) after it has been deacetylated (Ingle et al., 2022). One of the major advantages Ch/NPs typically degrade into non-toxic byproducts, reducing the risk of accumulation in the body or plant tissues and minimizing long-term side effects (Devi et al., 2023). In addition to their biocompatibility, Ch/NPs have been shown to stimulate a plant’s immune system and aid in biotic and abiotic stress management (Sangwan et al., 2023). As a non-toxic biopolymer, Ch/NPs can be organized and integrated strategically with fertilizer sources (i.e., urea, potassium sulfate, and calcium phosphate) to produce control-release fertilizer of NPK. Loading urea fertilizer into Ch/NPs has proven its efficiency in reducing urea fertilizer quantity and enhancing rice grain productivity as documented in a previous study by Elshayb et al. (2022b). As a result, Corradini et al. (2010) loading NPK fertilizer into Ch/NPs can be a benign approach for making a complex NPK fertilizer with slow-release properties which is known as chitosan-based NPK nano-structure (Ch/NPs-NPK). Prior studies demonstrated the outstanding merits of Ch/NPs-NPK in improving wheat productivity under different soils compared with classical NPK fertilizer (Abdel-Aziz et al., 2018), enhancing both growth and potato tuber (Elshamy et al., 2019), and improving both nutrient uptake and water absorption of French bean (Hasaneen et al., 2016). A prior study by Deshpande et al. (2017) declared that the foliar application of zinc amalgamated with Ch/NPs on two durum wheat cultivars caused a significant increase in zinc grain content by 27 and 42% compared to zinc-deficient control plants. However, the foliar spray of Ch/NPs significantly increased the levels of Fe, Zn, Mn, P, Ca, and Mg in finger millet plants in comparison of the control treatment (Sathiyabama and Manikandan, 2021). However, the application of Ch/NPs-NPK in paddy schedule fertilization and its effect on nutritional grain content has remained unexplored until now.
Looking at the above objectives, this study focused on evaluating the application of chitosan-based NPK nano-structure at a different rate with a combination of T-NPK aiming to determine the optimal dose for enhancing growth properties, minimize T-NPK inputs, and maximize both grain productivity and nutrients (N, P, and K) uptake.
2 Materials and methods
2.1 Site, soil, and climatological properties
In the Agriculture Research Station, Kar El-Sheikh, Egypt’s Experimental Farm of Sakha (31°05′17″N, 30°56′44″E), two field tests were carried out in 2022 and 2023 seasons.
The soil samples were taken from topsoil (depth of 0–20 cm), air-dried, and crushed (by passing through a 2-mm screen to remove big particles) before the setup of the experiments. The primary physiological properties were analyzed and recorded as described before (Ryan et al., 2001). Across the 2022 and 2023 seasons, the analysis data declared that soil type was clayey texture, organic matter (OM%; 6.40 and 7.12), pH (1:2.5 water suspension; 8.16 and 8.35), Ec (dS m−1; 3.08 and 2.90), available N (ppm; 585.4 and 592.3), available P (ppm; 5.68 and 6.00), available K (ppm; 1.44 and 1.65), available Zn+2 (ppm; 0.88 and 0.91), available Fe+2 (ppm; 4.97 and 5.00), and available Mn+2 (ppm; 3.14 and 3.82).
The weather databases were collected from a field observatory near the experimental site. The monthly meteorological particulars were codified annually from a field observatory and recorded in Figure 1.
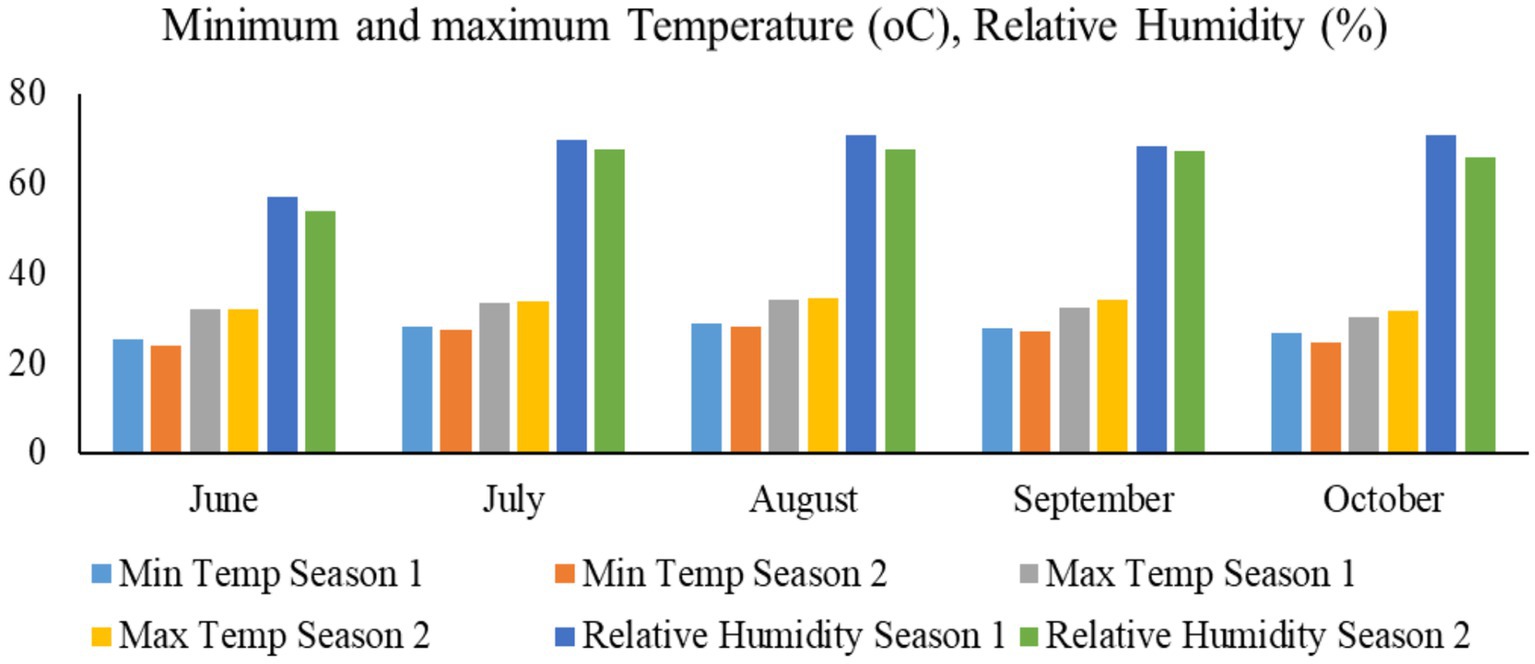
Figure 1. Minimum and maximum temperature and relative humidity during the growing seasons of the current experiment.
2.2 Preparation of chitosan-based NPK nano-structure
Chitosan-based NPK nano-structure (Ch/NPs-NPK) was prepared according to Corradini et al. (2010) with some modifications. Briefly, chitosan (Ch) (molecular weight 100,000–300,000 Da, and viscosity: 50 to 200 mPa·s, ACROS, China) aqueous solution (0.2% w/v) was prepared by dissolving Ch in acetic acid solution (1% v/v) (99–100%, Riedel-de Haën) at ambient temperature. The pH of the solutions was adjusted to 5.5 with 0.5 M sodium hydroxide pellets (≥95%, Sigma-Aldrich, US) solution. Subsequently, tripolyphosphate (TPP) (Technical grade, 85%, Sigma-Aldrich, US) as a cross-linking agent solution was dissolved in 10 mL deionized water to a final concentration of 0.6 mg/mL. After 30 min of vigorous magnetic stirring, the TPP solution was added dropwise (0.3 mL/min) to the chitosan solution in the Ch solution. Ch-NPK nano fertilizer was prepared by loading nitrogen (N), phosphorous (P), and potassium (K) into Ch/NPs by dissolving different amounts of NPK into 100 mL of nanoparticle solution under homogenizing at 18,000 rpm for 30 min in the presence of Tween 80 at 25°C. The resulting solution to incorporate NPK into the nanoparticles presents this final concentration: (i) 100, 200, and 400 ppm of N; (ii) 15, 30, and 60 ppm of P; (iii) 100, 200, and 400 ppm of K. The morphology of Ch/NPs-NPK was imaged by a High-Resolution Transmission Electron Microscope (HR-TEM) operating at an accelerating voltage of 200 kV (Tecnai G2, FEI, Netherlands). Diluted Ch-NPK NF solution was ultra-sonicated for 5 min to reduce the particle aggregation. Using a micropipette, three drops from the sonicated solution were deposited on a carbon-coated copper grid and left to dry at room temperature.HR-TEM images of the Ch/NPs-NPK that were deposited on the grid were captured for morphological evaluation. Dynamic light scattering (DLS) measurement of size and Zeta Potential was undertaken using a Nano-zeta sizer (Malvern, ZS Nano, UK). Synthesis and characterization of Ch/NPs-NPK were performed in Nanotechnology & Advanced Materials Central Laboratory (NAMCL), Agricultural Research Center (ARC), Giza, Egypt.
2.3 Plant material, and experimental details
During the dual seasons (2022 and 2023), the uniform size of rice grain (Oryza sativa L., Sakha super 300) was used in this study. After the nursery land was prepared, the rice grains at the rate of 120 kg/ha−1 were soaked (for 24 h), incubated (for 48 h) to accelerate the germination process, and then the pre-germinated seed broadcasted in the nursery on 20 and 22 of May in both seasons, respectively. The experiments were organized in a randomized complete block design (RCBD) including 3 replicates with 7 treatments. In the permanent field, the 21-day-old seedlings were transplanted in the experimental unit with a size of 12 m2 (4 m length × 3 m width). The transplanted seedlings (2–3 seedlings/hill) were pulled and organized in the respective units at a 20 × 20 cm distance between hills and rows. The studied treatments comprised factorial combinations among two fertilization levels (i.e., 70, and 30%) of traditional NPK fertilizer and three foliar doses of Ch/NPs-NPK (i.e., 100, 200, and 300 ppm) as well as the full recommended dose treatment (control treatment). The foliar application of Ch/NPs-NPK was applied twice (after transplanting at 20 and 40 D). The experimental treatment details are shown in Table 1.
According to the abovementioned amount of traditional soil application of NPK fertilizer, both potassium sulfate (48% K2O) and mono-superphosphate (15 %P2O5) were applied and integrated into the experimental soil before tillage. However, the amount of urea (CH4N2O) fertilizer was added as previously mentioned (twice at 2/3 as a basal application and 1/3 at panicle initiation). While observing the recommended doses of urea, mono-superphosphate, and potassium sulfate are 165 kg N/ha−1, 36 kg P2O5 /ha−1, and 60 kg K2O /ha−1, respectively. Before seedling transplanting and after puddling, Zinc sulfate was broadcasted and applied at the rate of 24 kg/ha−1. Six days after transplanting, 4.8 L/ha of the herbicide Saturn [S-(4-Chlorophenol methyl) diethyl carbamothioate] was sprayed to control weeds.
2.4 Data gathering
2.4.1 Physiological indicators
Photosynthetic pigments (i.e., chlorophyll a, chlorophyll b, and carotenoids) measured in fresh and fully expanded leaves (5 g) from the upper canopy and will be extracted in acetone (90% v/v) and then filtered to overact a final volume of 50 mL. Estimation of total chlorophyll (i.e., Ch. a and Ch. b) and carotenoid contents (C. content) was evaluated spectrophotometrically from the absorbance of the final extract as mg g−11FW−1 agreeing to the protocol given by Wellburn (1994) using Equations 1–3 as follows:
Where, A663.2, A646.8, and A470 represent the absorbance taken at 663.2 nm (Ch. a), 646.8 nm (Ch. b), and 470 nm (carotenoids) respectively.
At the heading stage, from a fixed point of each experimental unit, the rice leaf area index (LAIS) was estimated from 10 tagged leaves which were randomly sampled and calculated according to the following Equation 4:
concerning the evaluation of dry matter production per hill (DMP/hill), five hills from each plot were pulled out, air-dried, sealed into a kraft paper bag, and then exposed to oven-dried (70°C for 48 h) and stopped when a steady weight (g/hill) had been obtained according to the methodology proposed by Yoshida et al. (1971).
2.4.2 Yield causative components and yield outcomes
When the experimental field reached maturity, a certain area of the center of each experimental unit (10 m2) was harvested, well-dried, and mechanically threshed to evaluate the various studied characters. The estimation of plant height (cm2), number of panicles/m2 (No. Panicles/m2), panicle characters (length, weight, filled grains percentage “Equation 5”), grain weight (g), biological yield (BY), final grain yield (GY), and harvest index (HIs) percentage “Equation 6” were calculated. During 2 years, both BY and GY weights were converted to t/ha in the results databases noting the adjustment of paddy grains to 14% moisture content as mentioned by Yoshida (1981).
2.4.3 Nutrient uptake indices
The uptake of N (N-uptake) indices was calculated by using the Micro-Kjeldahl methodology. Samples of both grain and straw (5 g of each) in each experimental unit were exposed to oven-dried (70°C) to obtain a constant weight. Thereafter, samples were ground and digested using H2SO4-H2O2 as the standard given earlier by Jackson (2005). However, the uptake of P (P-uptake) indices was estimated as the methodology indicated by Watanabe and Olsen (1965). About the uptake of K nutrient, (K-uptake) indices were computed by a flame photometer (RHYS international LTD, India) using atomic absorption as described by Peterburgski (1968). Whereby, nutrient uptake in both grains and straw parts was calculated as kg/ha using Equation 7:
2.5 Statistical analysis
The obtained data was subjected to an analysis of variance (Gomez and Gomez, 1984). The means of different treatments were compared by Turkey’s test to clarify the significant difference at p ≤ 0.05 probability according to Hsu (1996). All statistical analyses were conducted utilizing the analysis of variance technique by the COSTATC computer software package.
3 Results
3.1 The characterization of chitosan-based NPK nano-structure
3.1.1 TEM analysis results
According to the findings displayed in Figure 2, Ch/NPs-NPK had a spherical surface, a uniform size distribution, was stable, and had a finely shaped exterior. The greatest surface area for loading NPK nutrients onto the surface of chitosan nanoparticles is found in their spherical shape, smooth surface, and an average size of about 25.7 nm.
3.1.2 Dynamic light scattering analysis
Hydrodynamic diameter was measured in the nanoscale range using the DLS technique. The zeta potential of Ch/NPs-NPK was +45.4 mV, and its size was 32.7 nm (Figure 3).
3.2 The effects of several rates of traditional NPK fertilizers and their combination with chitosan-based NPK nano-structure as a foliar application on physiological indicators
The physiological indicators of total photosynthetic pigments (Figure 4), LAIs, and DMP/hill were significantly (at p ≤ 0.05) influenced by several rates of traditional NPK fertilizers and their combination with Ch/NPs-NPK (Table 2). The higher values of total photosynthetic pigments (i.e., Ch. a, Ch. b, and C. content) showed in rice plots that fertilized with T1 (full recommended dosage of conventional NPK) which gave identical statistically with T4 (70% NPK + 300 mg NPK/l) in both seasons. Similar findings were noted for LAIs, where T4 and T3 (4.86 and 5.22) fertilization resulted in statistical conformity with T1 (5.48) in the 1st season. However, in the 2nd season, only T4 (5.37) recorded statistical similarity with T1. As for DMP/hill (g/hill), the highest weight of dry matter was rendered from the application of T1 with no significant difference between this treatment and T4 (81.9, 79.6, and 83.1, 80.3 g/hill respectively) in both cropping seasons. Whilst, the application of T5 gave the lowest values of total photosynthetic pigments (4.105 and 4.387 mg g−1FW−1), LAIs (4.12 and 4.32), and DMP/hill (63.8 and 65.2 g/hill) respectively in both sequential seasons.
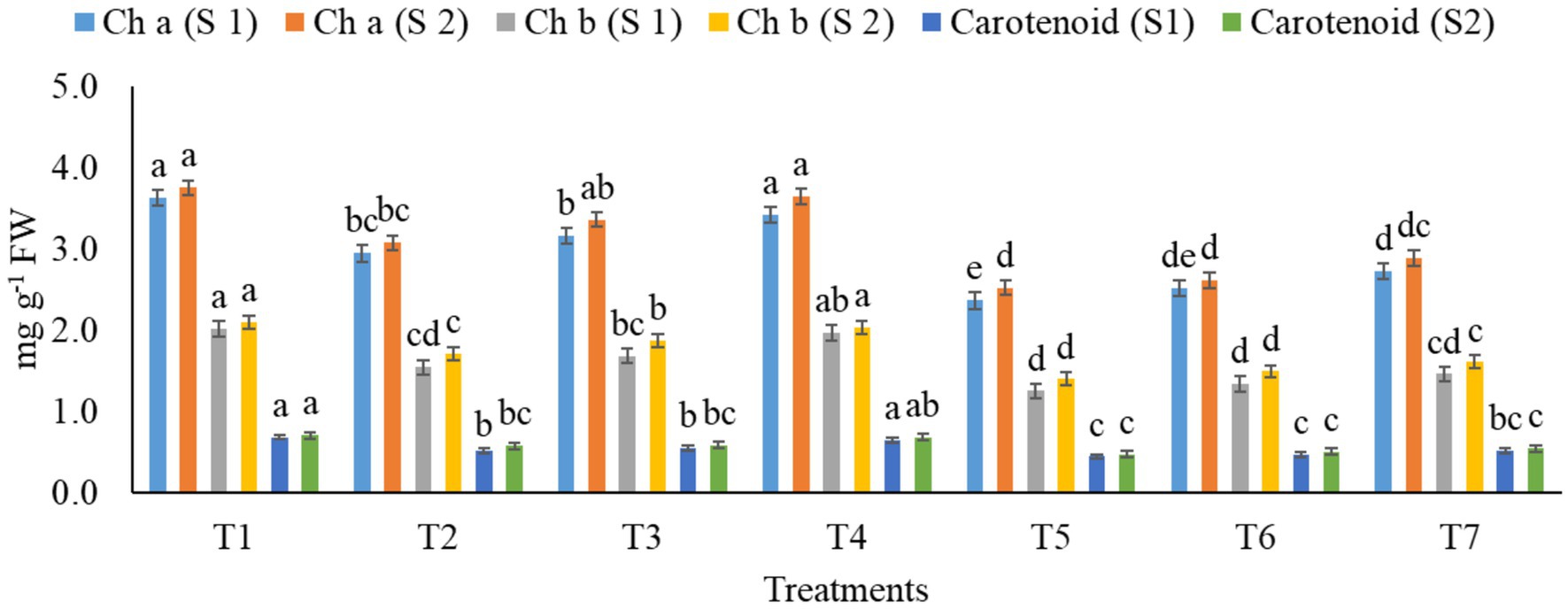
Figure 4. The effects of several rates of traditional NPK fertilizers and their combination with chitosan-based NPK nano-structure as a foliar application on total chlorophyll (i.e., Ch. a and Ch. b) and carotenoid contents (C. content). Various letters (a, b, c, d, etc.) denoted a statistically significant variation in the treatment means at p < 0.05. As for 366 Treatments treatments abbreviation (see Table 1).
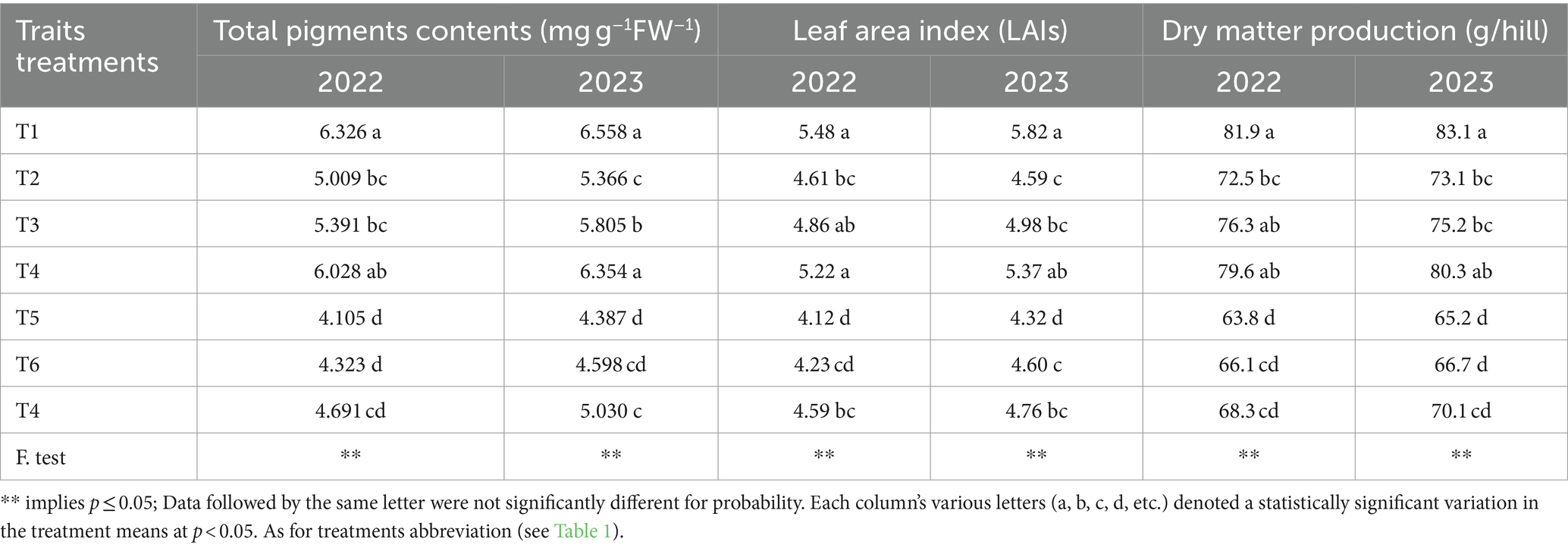
Table 2. Effects of synthetic NPK fertilizers and their combination with chitosan-based NPK nano-structure as a foliar application on total pigment contents, leaf area index, and dry matter production.
Various letters (a, b, c, d, etc.) denoted a statistically significant variation in the treatment means at p < 0.05. As for treatments abbreviation (see Table 1).
3.3 The effects of several rates of traditional NPK fertilizers and their combination with chitosan-based NPK nano-structure as a foliar application on yield causative components
The appraisal data in Table 3 imparted that a meaningful impact has been made by several treatments concerning plant height, No. panicles/m2, and panicle length in both seasons. The tallest plants (115.1 and 116.9 cm) were recorded under the T1 treatment without significant differences between this treatment (T1) and both T3 and T4 (115.1, 114.3, and 114.5 cm) treatments in the 2022 season. Whilst, in the 2023 season, T1 treatment gave identical statistically with T4 only. As for the result of counting panicles number /m2, T1 gave the best value (574.4 and 583.2 panicles/m2) which was statistically identical with T4 (556.4 panicles/m2) treatment in the 1st season and with both T3 and T4 (551.3 and 568.2 panicles/m2 respectively) treatments in the 2nd season. Likewise, T4 rendered a statistical match with T1 (22.8, and 24.1 cm, respectively in both seasons) concerning the length of the panicle. Again, the lowest values of these characters, i.e., plant height (103.3 and 104.3 cm), No. panicles/m2 (454.4 and 455.7), and panicle length (18.3, and 18.0 cm) respectively during both planting, seasons were witnessed under T5 treatment.
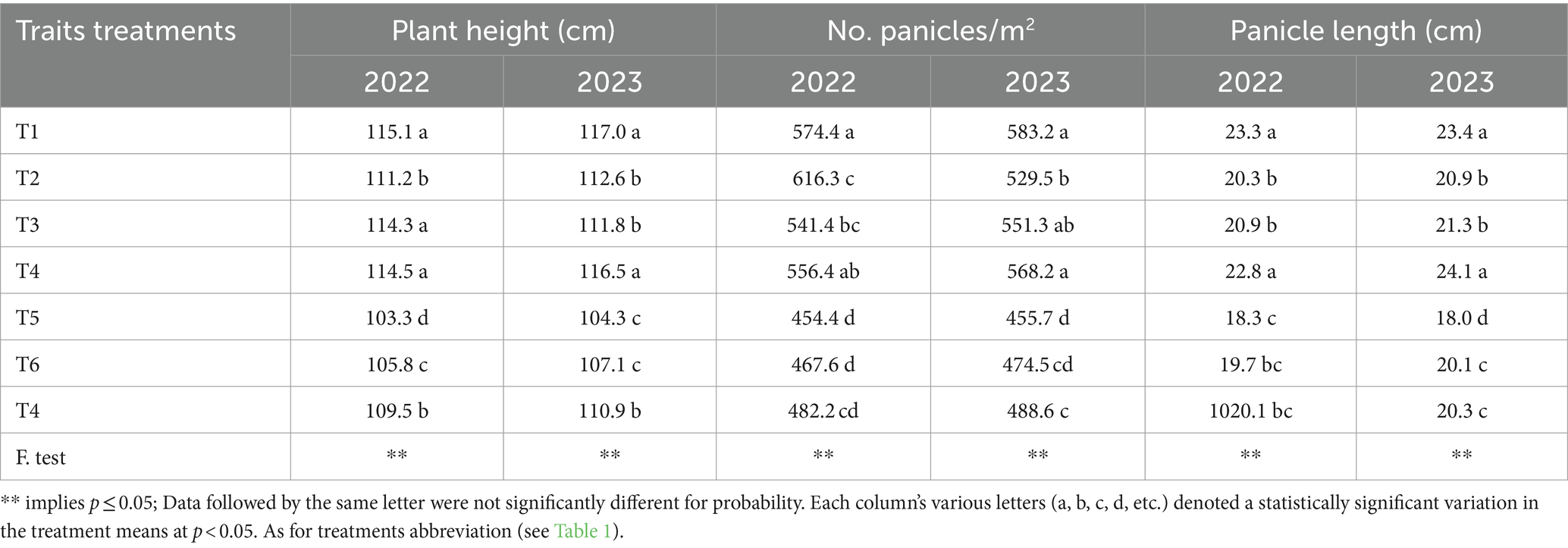
Table 3. Effects of synthetic NPK fertilizers and their combination with chitosan-based NPK nano-structure as a foliar application on some yield components traits.
A different and significant response on panicle weight, filled grains percentage, and 103-grain weight characters were observed under various treatments (Table 4). Compared to a traditional application of NPK (control treatment), both T3 and T4 (3.73 and 4.11 g respectively) gave statistical conformity with the T1 (4.12 g) concerning panicle weight in the 2022 season. However, T4 treatment only provided (4.30 g) a statistical match with T1 (4.13 g) in the 2023 season. Concerning the filled grains percentage character, there wasn’t a significant difference between T1 and T4 (89.6 and 92.2% respectively) in the 2022 season but in the 2023 season, T3 and T4 (88.6 and 92.6% respectively) treatments gave identical statistics to the application of T1. As regards 103-grain weight, the highest weight was observed in both treatments of T1 and T4 (26.93, 28.90, and 27.49, 28.16 g) respectively in the 2022 and 2023 seasons and without significant difference between them. Nevertheless, the lowest values of these characters, i.e., panicle weight (3.03 and 3.11 g), filled grain percentage (77.8 and 79.9%), and 103-grain weight (23.20 and 23.49 g) were noted under the treatment of T5.
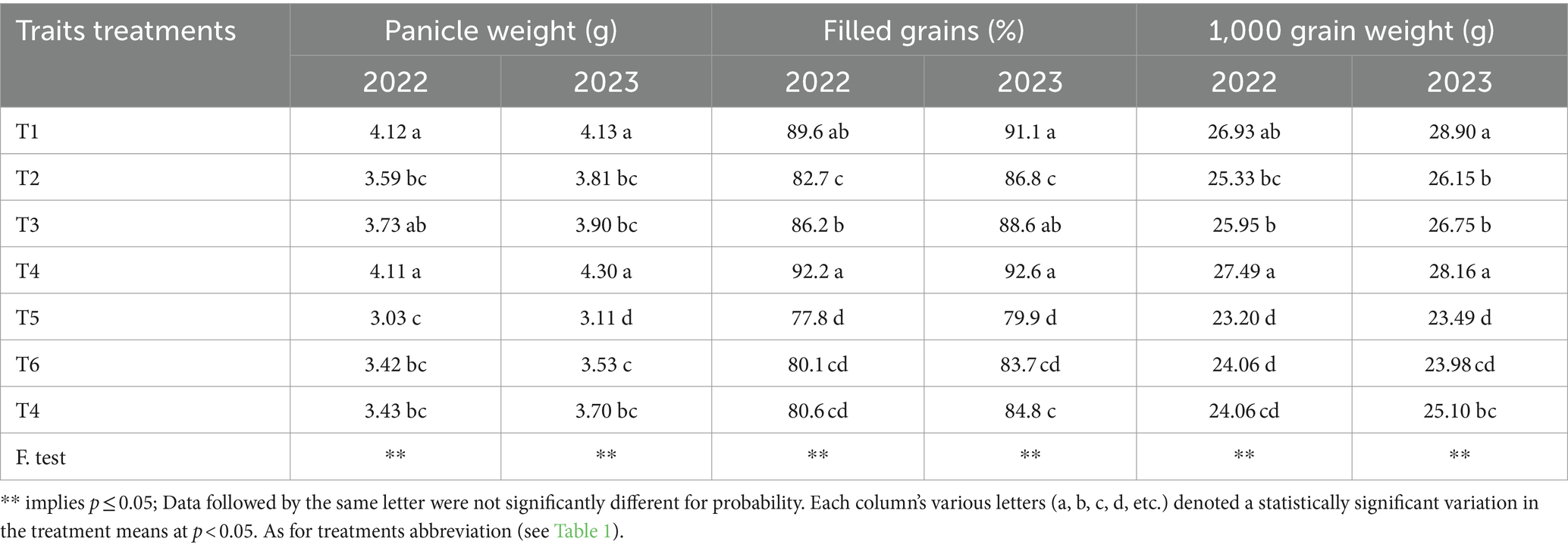
Table 4. Effects of synthetic NPK fertilizers and their combination with chitosan-based NPK nano-structure as a foliar application on some yield components traits.
3.4 The effects of several rates of traditional NPK fertilizers and their combination with chitosan-based NPK nano-structure as a foliar application on yield outcomes
The obtained data about yield outcomes (BY and GY) and HIs are recorded in Table 5. During both cultivated seasons, a significant influence (p ≤ 0.05) appeared among all treatments related to BY which recorded the highest value (24.16 and 24.93 t/ha−1) respectively from the T1 treatment and on par with the T4 treatment. Also, the same trend was revealed concerning GY which represented the highest value underlying T1 treatment (10.93 and 11.04 t/ha, respectively, over both seasons). Notably, the T1 and T4 treatments did not significantly differ in either of the two seasons. For HIs during both seasons, the implications exhibited that T1 and T4 contributed the highest HIs value (0.448, 0.451, and 0.447, 0.449 respectively) without significant difference between them. The lowest value of BY (20.83, and 21.27 t/ha), GY (8.35, and 8.56 t/ha), and HIs (0.403 and 0.410) is seen under the application of T5 in the 1st and 2nd seasons.
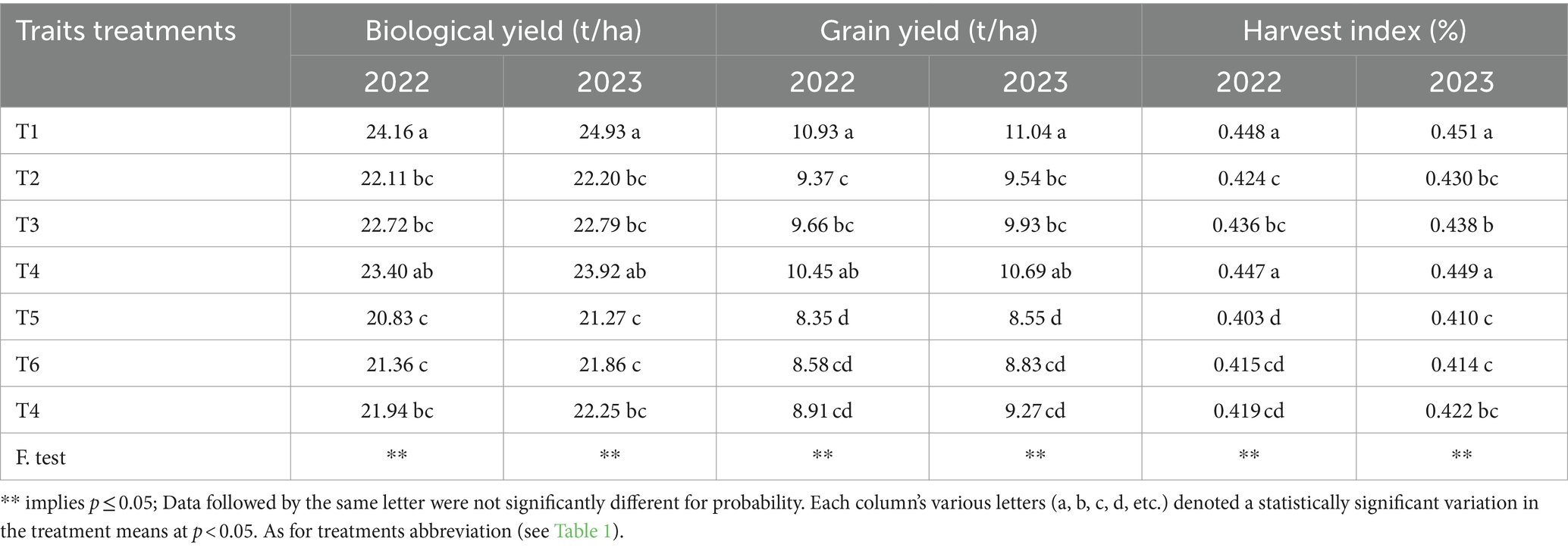
Table 5. Effects of synthetic NPK fertilizers and their combination with chitosan-based NPK nano-structure as a foliar application on yield outcomes.
3.5 The effects of several rates of traditional NPK fertilizers and their combination with chitosan-based NPK nano-structure as a foliar application on nutrient uptake indices
From the results set (Figure 5), a substantial change in nutrient uptake (i.e., N, P, and K nutrients) of rice grain was indicated by the effect of various applications during the 1st and 2nd seasons. Concerning N uptake indices by grains, the control treatment (T1) gave statistics similar to the T4 application in both seasons. Also, the same trend was observed concerning P uptake indices whereby the highest value was recorded by T1 without a significant difference between T1 and T4 in both sequential seasons. Whilst, a variable increase was noted concerning K uptake indices underlying rice plants treated with T3 and T4 applications and with no substantial difference between T1, T3, and T4 in both cropping seasons. Substantial difference between T1, T3, and T4 in both cropping seasons. The lowest values of N, P, and K elements uptake were presented from the plots that fertilized with T5 application in both of 2022 and 2023 seasons.
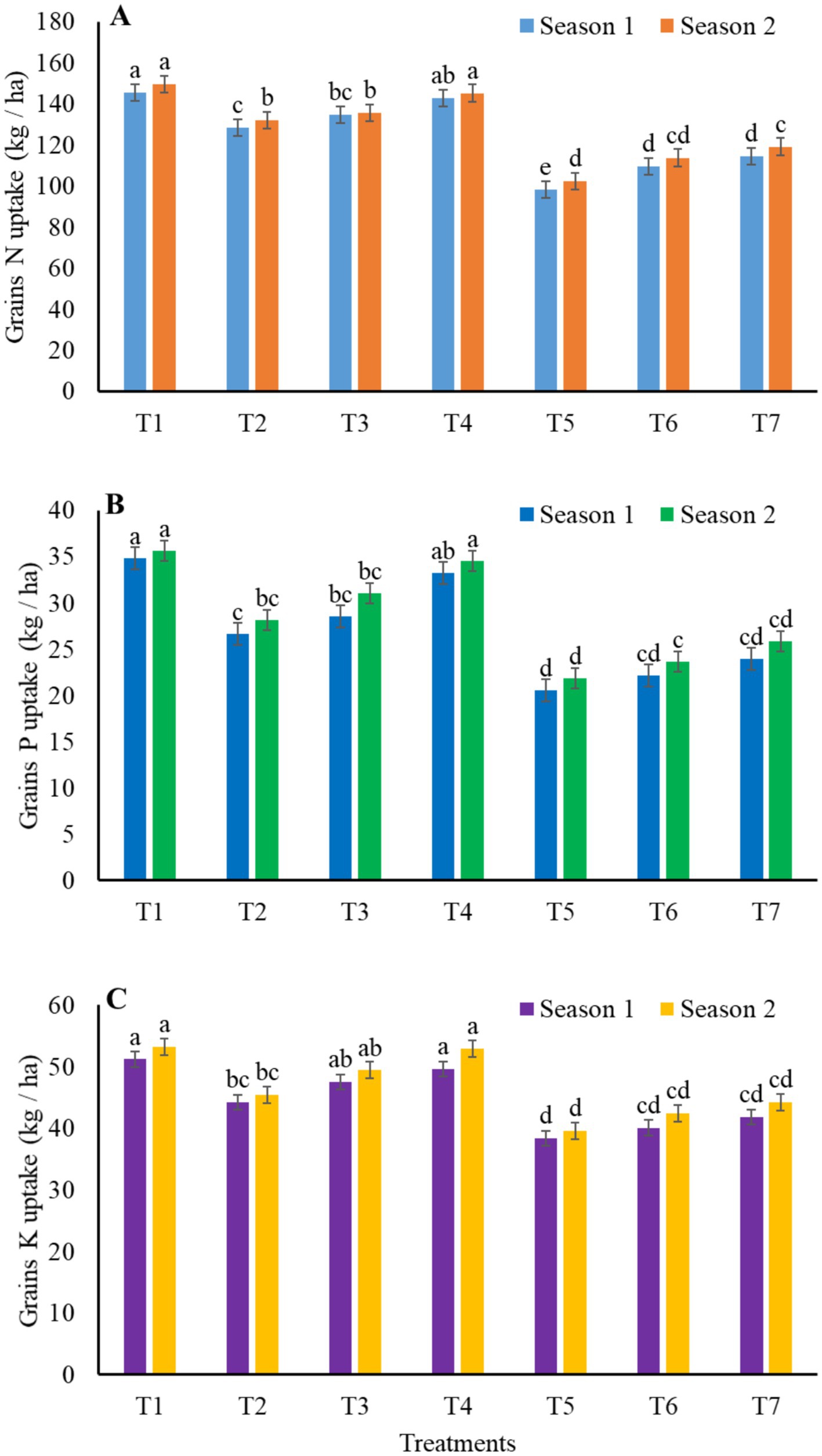
Figure 5. Effects of traditional NPK fertilizers and their combination with chitosan-based NPK nano-structure as a foliar application on (A) grain N uptake, grain P uptake (B), and grain K uptake (C) of rice. Various letters (a, b, c, d, etc.) indicate a statistically significant variation among treatment means at p < 0.05. As for treatments abbreviation (see Table 1).
Concerning nutrient uptake (i.e., N, P, and K nutrients) of rice straw parts, the resultant data set in Figure 6 illustrated a variable significant influence in these indices via the effect of various applications. The control treatment gave the highest rank concerning the indices of N uptake by rice straw without a significant difference between T1 and T4 treatments in both seasons. Once more, T1 reaffirmed superiority in the indices of P uptake by rice straw with statistical conformity with the application of T4 in the 1st and 2nd seasons. As for the indices of P uptake by rice straw, the treatment of T1, and T4 occupied the highest rank without significant difference among them in the 1st season. Whilst there was a statistical match between T1, T3, and T4 in the 2nd season. Across both seasons, the treatment of T5 submitted the lowest rank concerning all studied nutrient uptake in rice straw.
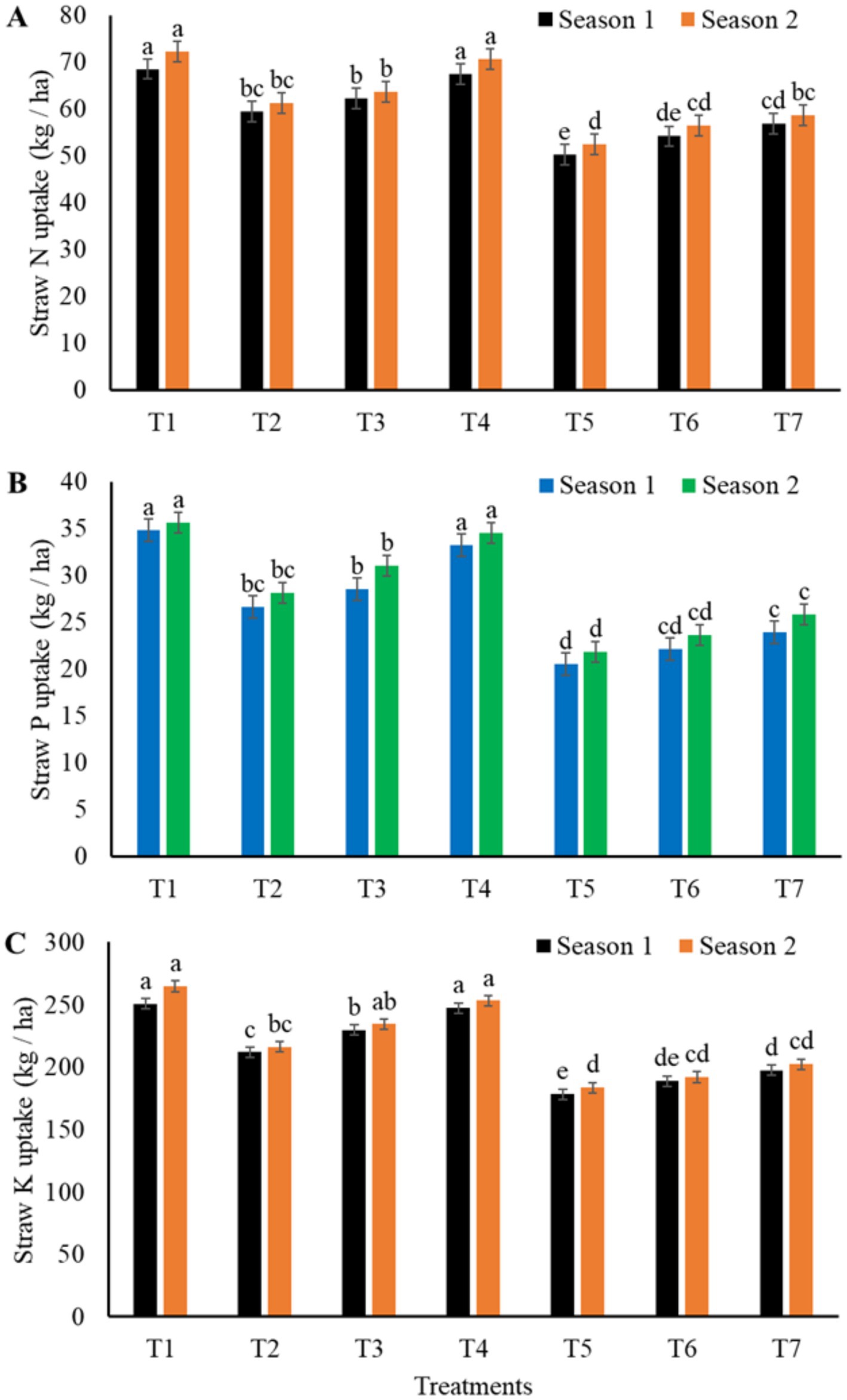
Figure 6. Effects of traditional NPK fertilizers and their combination with chitosan-based NPK nano-structure as a foliar application on (A) N uptake (B) P uptake, and (C) K uptake of rice straw. Various letters (a, b, c, d, etc.) indicate a statistically significant variation in the treatment means at p < 0.05. As for treatments abbreviation (see Table 1).
4 Discussion
Undoubtedly, crop agrarian productivity necessitates the usage of NPK-containing fertilizers because of their vital role in macronutrient (i.e., N, P, and K) homeostasis that determines biomass synthesis and dry matter production (Moe et al., 2019; Krasilnikov et al., 2022; Sarkar et al., 2022). More critically, the tiny particle size and wide surface area of nano fertilizers lead to an increase in particle number per unit of weight as well as high reactivity, which improves the fertilizer’s contact with the plant and boosts both fertilizer use efficacy and nutrient uptake (Abdel-Aziz et al., 2018; Abd-Elrahman et al., 2023; Devi et al., 2023; Elshayb et al., 2022a). Hence, modified NPK fertilizer with nanostructure and slow-release properties has a special potential to enhance the usage and uptake of nutrients.
Photosynthetic pigments have a cardinal role in light energy’s absorption, transmission, and transformation processes during photosynthesis (Moe et al., 2019). Numerous investigations have demonstrated that the regulation of photosynthetic pigment synthesis in crop leaves is significantly influenced by nitrogen nutrition (Vijayalakshmi et al., 2015; Peng et al., 2021). Also, an appositive correlation is well-documented between N-level supply and both Ch. content and C. content (Kumari et al., 2024; Srikanth et al., 2023). Therefore, the results in Figure 4 and Table 2 may be due to the different variations of N quantities supply which reflect on total photosynthetic pigments (i.e., chlorophyll a, chlorophyll b, and carotenoids) that were affected positively with several studied traits. The coprecipitation of chemical fertilizer of NPK and exogenous application of Ch/NPs-NPK 300 ppm underlying T4 may give plants adequate P nutrient addition that has a monumental role in several metabolic processes (i.e., photosynthesis, energy storage, and cell division) as a result of an integral constituent of phospholipids, nucleic acid, and co-enzymes that ultimately activate amino acid formation (Bechtaoui et al., 2021; Gao et al., 2023; Vance et al., 2003). On the other side, sufficient K supply plays an influential function in photosynthetic CO2 fixation and preservation of chloroplast status about enzyme activities, PH, and turgor (Dreyer et al., 2017). In this regard, Sheoran et al. (2021) found that wheat plants sprayed with nano-K utilized the exchangeable K in the sandy loam soil to the highest extent possible, resulting in the least amount of K leaching. This may be explained by the increased absorption of K and its related functions in cation-anion balance, energy transfer, stomatal movement, osmoregulation, photosynthesis, enzyme activation, protein synthesis, and phloem transport (Elshamy et al., 2019; Abd-Elrahman et al., 2023). Therefore, applying Ch/NPs-NPK 300 ppm can probably compensate for the missing quantity of chemical NPK fertilizer under the condition of T4 (when T-NPK decreases by up to 30%). Hence, compared to T1 (control), total pigment contents did not give a statistical difference with the T4 treatment (Table 2). Indeed, the higher efficacy of the photosynthesis process reflected positively on cell properties (e.g., elongation, division, and expansion) (Moe et al., 2019). It is obvious that the sole application of Ch/NPs (at the rate of 90 ppm) has been proven beneficial to wheat plants undergoing water stress conditions via enhancing Ch. Content (Behboudi et al., 2019). Another article given by Devi et al. (2023), indicated that Ch/NPs played a versatile growth promoter role by underpinning leaf area and total Ch. Content of rice plants. On the above basis, the higher contents of photosynthesis pigment led to higher values of LAIs which was witnessed clearly under the treatments of T1, and T4 (in both seasons). Consequently, the increase of LAIs values causes a benign utilization and capture of solar energy that leads to more photo-assimilated formation and ultimately generates higher DMP (Moe et al., 2019; Elshayb et al., 2022a; Elshayb et al., 2022b; He et al., 2024). Hereby, the perusal results (Table 2) deduced that the application of Ch/NPs-NPK 300 ppm concerning the treatment of T4 may be synchronized and underpinned with the plant’s nutrient needs despite the decrease of traditional NPK fertilizers till 30%.
After Ch/NPs-NPK is applied exogenously, it penetrates the epidermal of plant leaves, allowing for easy absorption through the plant’s organs. Due to the noncirculatory system of plant vascular systems, exogenous nanoscale material moves directly into the xylem (the shoot’s internal structure) and phloem (the root’s internal structure) (Gangwar et al., 2023). Probably, the foliar application of Ch/NPs-NPK (at the proper dose) supported and enhanced the translocation process from paddy shoots to their root. Moreover, a facility of molecule absorption (due to its tiny size) may be reflected favorably on enhancing the physiological processes that work on encouraging meristematic activity, cell division, and both stem and internode elongation Subsequently improving plant height. A previous study by Hasaneen et al. (2016), found that the Ch/NPs-NPK treatment had a significant favorable impact on the growth characteristics of the plants, and they attributed this to an enhanced nutritionally balanced state resulting from an increase in NPK flux within plant tissue.
Indeed, reproductive tiller per unit area is an important morpho-physiological characteristic of paddies and is considered an indicator of high N content (Banayo et al., 2021; Elshayb et al., 2022a; Tyagi et al., 2024). Moreover, the higher reproductive tillers under T4 treatment may be due to P sufficiency status in early stages that perhaps led to encouraging the formation of roots, tillers, and flowering because plants remobilize it within their tissues throughout the advanced growth stages (Miranda-Villagómez et al., 2019). These striking data concerning T1 and T4 treatments (Tables 3, 4) signified that sufficient and adequate absorption of N, P, and K nutrients before the panicle initiation stage perhaps occurred and caused a positive effect on panicle numbers per meter, panicle primordia formation, number of spikelet’s per panicle, and panicle branching (Yoshida, 1981). Thus, across both seasons, the increase in N, P, and K nutrients was more predominant with the application of T4. The increase in panicle length is related to the increase concurrently with N addition (Liu et al., 2020; He et al., 2024). This implies that utilization applications of T1 (applying the full recommended dose of NPK), and the T4 (applying 70% of NPK + 300 ppm Ch/NPs-NPK) treatments possibly gave the plants with optimum nitrogen dose. On the other side, chitosan promotes phytohormone formation, especially cytokinin which enhances cell proliferation in the filling stage resulting in the developing sink size (Behboudi et al., 2019; Sangwan et al., 2023). In this sense, the production and translocation of the pre-sorted assimilated output from enriched plant tissues can easily transfer from source to sink, indicating a notable improvement in yield-causative components including panicle qualities (i.e., length and weight), filled grains percentage, and 1,000-grain weights.
Overall, benign DMP is the infrastructure for producing a distinguished yield component and grain outcomes (Abdel-Aziz et al., 2018; Elshayb et al., 2022a; Seleiman et al., 2022; He et al., 2024). The photosynthetic synthesis of leaves (especially flag leaves) is responsible for 90% of rice grain output (Hai-Yan et al., 2009) Also, higher N intake during the vegetative period until panicle initiation is associated with benign carbohydrate buildup which could minimize tiller abortion, enhance reproductive tillers, and eventually maximize GY (Seleiman et al., 2022; Tyagi et al., 2024). Therefore, probably, T1 and T4 provided a benign content of N in rice tissues (Table 5). Previous research by Elshayb et al. (2022a), demonstrated that rice crops GY and BY were optimized and 40% less urea was required in paddy fields when urea chitosan nanoparticles were sprayed instead of the highly recommended traditional approach. On the other side, the presence of potassium may boost the GY magnitude underlying T1 and T4 treatment (Table 5) because of its vital role in converting photosynthetic products into starch and protein which are transported directly to grains (Al-Shahmani and Al-Juthery, 2021). An earlier investigation by Sirisena et al. (2012), declared that using potassium fertilizer in nanosizing form greatly improved GY outcomes. Also, Poudel et al. (2023), noted that the foliar application of P in the nanoscale form during tillering and panicle initiation stages enhanced some bioprocess that led to increasing rice grain and straw yields.
As for, nutrients (i.e., N, P, and K) uptake indices by grains, the obvious result (Figure 5) was the increase of nutrient uptake with increasing both Ch/NPs-NPK and T-NPK levels which perhaps produced sufficient nutrition for rice production. This study hypothesis was that Ch/NPs were grabbed by NPK nutrients which added a slow-release feature to the composite of Ch/NPs-NPK resulting in higher efficacy concerning nutrient uptake. As per Abdel-Aziz et al. (2018), using Ch/NPs-NPK in wheat fertilization has been able to lower N loss and increase N uptake’s effectiveness. Another investigation by Elshayb et al. (2022a), observed that utilizing urea-chitosan nanocomposite by 500 ppm led to optimizing N-uptake in both grain and straw rice yields. Another investigation by Abdel-Hakim et al. (2023) revealed that the leaf tissues of lettuce plants treated with NPK as a nano formulation had the largest accumulation of nutrients (N, P, K, Ca, Mg, Fe, Mn, and Zn). Recent studies pointed to the effective role of Ch/NPs in improving nutrient cycling and providing essential nutrients over an extended period, reducing the frequency of applications (Sarkar et al., 2022; Singh et al., 2024). Therefore, it is possible that the foliar application of Ch/NPs-NPK 300 ppm underlying T4 treatment was a good route to obtain the mechanism of uptake and transport of NPK nutrients into intact plant organs.
An analysis of the current findings showed that the nutrients (i.e., N, P, and K) uptake indices by straw (Figure 6) increased progressively by increasing both Ch/NPs-NPK and T-NPK levels. Meanwhile, T4 treatment gave a substantial increase in nutrient uptake by straw which may be due to the mass flow phenomenon via a pressure differential between the leaves and stems, which is what allows nanoparticles to travel from stomata and make their way to the xylem vessels and then move the phloem tissue where they were carried nutrients to reach all plant tissues (Hasaneen et al., 2016). A prior study by Corradini et al. (2010) pointed to the ability of endosomes (parts of the endocytic membrane transport pathway) to transport the nanoparticles to various sites within the cells. Notably the most important aspect of utilizing Ch/NPs associated with synthetic fertilizers contributes to reducing environmental pollution because it’s biodegradable (Sangwan et al., 2023). Consequently, even though T-NPK dropped by up to 30%, the application of T4 improved the status of nutrient (i.e., N, P, and K) absorption indices in grain and straw yields and can be considered a smart and eco-friendly application in paddy fields.
5 Conclusion
The findings of the current study reported that utilizing Ch/NPs-NPK with 300 ppm was considered a significant application to compensate synthetic NPK fertilizers by 30% without a significant reduction in growth, yield, and nutrient content of rice. Therefore, using Ch/NPs-NPK can be recommended as an exogenous application in the paddy fields to reduce the excessive use of synthetic NPK fertilizers and enhance physiological traits that lead to sustaining a satisfactory grain outcome. Accordingly, this approach not only can mitigate the environmental impact associated with excessive fertilizer use but also can enhance the physiological traits of the rice plants, contributing to sustainable agricultural practices. The biodegradable and slow-release feature of Ch/NPs makes it an alternative to smart-safe use in the agriculture sector. Ultimately, utilizing such modern and modified technologies to produce compensatory alternatives for balanced plant nutrition provides environmental and economic usefulness in the ecosystem. Moreover, there’s a dire demand to examine and explore the potential of Ch/NPs-NPK on different rice varieties, and other plant species for a deep and thorough evaluation of these materials.
Data availability statement
The raw data supporting the conclusions of this article will be made available by the authors, without undue reservation.
Author contributions
OE: Conceptualization, Funding acquisition, Methodology, Project administration, Resources, Software, Writing – original draft, Writing – review & editing. HG: Conceptualization, Data curation, Formal analysis, Funding acquisition, Methodology, Writing – original draft, Writing – review & editing. MW: Investigation, Methodology, Software, Supervision, Validation, Writing – original draft, Writing – review & editing. KF: Methodology, Software, Supervision, Validation, Visualization, Writing – original draft, Writing – review & editing. DW: Methodology, Software, Writing – original draft, Writing – review & editing. MS: Data curation, Funding acquisition, Investigation, Methodology, Project administration, Resources, Writing – original draft, Writing – review & editing.
Funding
The author(s) declare that financial support was received for the research, authorship, and/or publication of this article. This research was funded by Researchers Supporting Project (RSPD2024R751), King Saud University, Riyadh, Saudi Arabia.
Conflict of interest
The authors declare that the research was conducted in the absence of any commercial or financial relationships that could be construed as a potential conflict of interest.
Publisher’s note
All claims expressed in this article are solely those of the authors and do not necessarily represent those of their affiliated organizations, or those of the publisher, the editors and the reviewers. Any product that may be evaluated in this article, or claim that may be made by its manufacturer, is not guaranteed or endorsed by the publisher.
References
Abdel-Aziz, H. M. M., Hasaneen, M., and Omer, A. (2018). Foliar application of nano chitosan NPK fertilizer improves the yield of wheat plants grown on two different soils. J. Exp. Biol. 14, 63–72. doi: 10.5455/egyjebb.20180106032701
Abdel-Hakim, S. G., Shehata, A. S. A., Moghannem, S. A., Qadri, M., el-Ghany, M. F. A., Abdeldaym, E. A., et al. (2023). Nanoparticulate fertilizers increase nutrient absorption efficiency and agro-physiological properties of lettuce plant. Agronomy 13:691. doi: 10.3390/agronomy13030691
Abd-Elrahman, S. H., El-Gabry, Y. A., Hashem, F. A., Ibrahim, M. F., El-Hallous, E. I., Abbas, Z. K., et al. (2023). Influence of nano-chitosan loaded with potassium on potassium fractionation in sandy soil and strawberry productivity and quality. Agronomy 13:1126. doi: 10.3390/agronomy13041126
Alhammad, B. A., Ahmad, A., Seleiman, M. F., and Tola, E. (2023). Seed priming with nanoparticles and 24-epibrassinolide improved seed germination and enzymatic performance of Zea mays L. in salt-stressed soil. Plan. Theory 12:690. doi: 10.3390/plants12040690
Almutari, M. M. (2023). Synthesis and modification of slow-release fertilizers for sustainable agriculture and environment: a review. Arab. J. Geosci. 16:518. doi: 10.1007/s12517-023-11614-8
Al-Shahmani, A., and Al-Juthery, H. (2021). Response of rice (Oryza sativa L.) to silica fertilization and spraying with nano-potassium and calcium. IOP Conf. Ser. Earth Environ. Sci. 735:012068. doi: 10.1088/1755-1315/735/1/012068
Badawy, S. A., Zayed, B. A., Bassiouni, S. M., Mahdi, A. H., Majrashi, A., Ali, E. F., et al. (2021). Influence of nano silicon and nano selenium on root characters, growth, ion selectivity, yield, and yield components of rice (Oryza sativa L.) under salinity conditions. Plan. Theory 10:1657. doi: 10.3390/plants10081657
Banayo, N. P., Rahon, R. E., Sta. Cruz, P., and Kato, Y. (2021). Fertilizer responsiveness of high-yielding drought-tolerant rice in rainfed lowlands. Plant Product. Sci. 24, 279–286. doi: 10.1080/1343943X.2020.1847668
Batool, A., Azizullah, A., Ullah, K., Shad, S., Khan, F. U., Seleiman, M. F., et al. (2024). Green synthesis of Zn-doped TIO2 nanoparticles from Zanthoxylum armatum. BMC Plant Biol. 24:820 (2024). doi: 10.1186/s12870-024-05525-3
Bechtaoui, N., Rabiu, M. K., Raklami, A., Oufdou, K., Hafidi, M., and Jemo, M. (2021). Phosphate-dependent regulation of growth and stresses management in plants. Front. Plant Sci. 12:679916. doi: 10.3389/fpls.2021.679916
Behboudi, F., Tahmasebi-Sarvestani, Z., Kassaee, M. Z., Modarres-Sanavy, S. A. M., Sorooshzadeh, A., and Mokhtassi-Bidgoli, A. (2019). Evaluation of chitosan nanoparticles effects with two application methods on wheat under drought stress. J. Plant Nutr. 42, 1439–1451. doi: 10.1080/01904167.2019.1617308
Carrijo, D. R., Lundy, M. E., and Linquist, B. (2017). Rice yields and water use under alternate wetting and drying irrigation: a meta-analysis. Field Crop Res. 203, 173–180. doi: 10.1016/j.fcr.2016.12.002
Chakraborty, R., Mukhopadhyay, A., Paul, S., Sarkar, S., and Mukhopadhyay, R. (2023). Nanocomposite-based smart fertilizers: a boon to agricultural and environmental sustainability. Sci. Total Environ. 863:160859. doi: 10.1016/j.scitotenv.2022.160859
Corradini, E., De Moura, M., and Mattoso, L. (2010). A preliminary study of the incorparation of NPK fertilizer into chitosan nanoparticles. Express Polym Lett 4, 509–515. doi: 10.3144/expresspolymlett.2010.64
Deshpande, P., Dapkekar, A., Oak, M. D., Paknikar, K. M., and Rajwade, J. M. (2017). Zinc complexed chitosan/TPP nanoparticles: a promising micronutrient nanocarrier suited for foliar application. Carbohydr. Polym. 165, 394–401. doi: 10.1016/j.carbpol.2017.02.061
Devi, O. R., Ojha, N., Laishram, B., Dutta, S., and Kalita, P. (2023). Roles of nano-fertilizers in sustainable agriculture and biosafety. Environ. Ecol. 5:100044. doi: 10.1016/j.plana.2023.100044
Dimkpa, C., Adzawla, W., Pandey, R., Atakora, W. K., Kouame, A. K., Jemo, M., et al. (2023). Fertilizers for food and nutrition security in sub-Saharan Africa: an overview of soil health implications. Front. Soil Sci. 3:1123931. doi: 10.3389/fsoil.2023.1123931
Dreyer, I., Gomez-Porras, J., and Riedelsberger, J. (2017). The potassium battery: a mobile energy source for transport processes in plant vascular tissues. New Phytol. 216, 1049–1053. doi: 10.1111/nph.14667
Elshamy, M. T., El-Khallal, S. M., Husseiny, S. M., and Farroh, K. Y. (2019). Application of nano-chitosan NPK fertilizer on growth and productivity of potato plant. J. Sci. Res. Sci. 36, 424–441. doi: 10.21608/jsrs.2019.58522
Elshayb, O. M., Nada, A. M., Farroh, K. Y., Al-Huqail, A. A., Aljabri, M., Binothman, N., et al. (2022a). Utilizing urea–chitosan nanohybrid for minimizing synthetic urea application and maximizing Oryza sativa l. productivity and n uptake. Agriculture 12:944. doi: 10.3390/agriculture12070944
Elshayb, O. M., Nada, A. M., Sadek, A. H., Ismail, S. H., Shami, A., Alharbi, B. M., et al. (2022b). The integrative effects of biochar and ZnO nanoparticles for enhancing rice productivity and water use efficiency under irrigation deficit conditions. Plan. Theory 11:1416. doi: 10.3390/plants11111416
Ferradj, S., Yahoum, M. M., Rebiha, M., Nabi, I., Toumi, S., Lefnaoui, S., et al. (2024). Nanocurcumin-based sugar-free formulation: development and impact on diabetes and oxidative stress reduction. Nano 14:1105. doi: 10.3390/nano14131105
Gangwar, J., Kadanthottu Sebastian, J., Puthukulangara Jaison, J., and Kurian, J. (2023). Nano-technological interventions in crop production—a review. Physiol. Mol. Biol. Plants 29, 93–107. doi: 10.1007/s12298-022-01274-5
Gao, D., Cheng, R., Kun, D., Xiaolei, W., Yunhe, Z., Yanqiu, G., et al. (2023). Effect of phosphorus, iron, zinc, and their combined deficiencies on photosynthetic characteristics of rice (Oryza sativa L.) seedlings. Agronomy 13:1657. doi: 10.3390/agronomy13061657
Gomez, K. A., and Gomez, A. A. (1984). Statistical procedures for agricultural research. 2nd Edn. New York, NY: John Wiley & Sons. p. 680–696.
Hafez, E. H., and Seleiman, M. F. (2017). Response of barley quality traits, yield and antioxidant enzymes to water-stress and chemical inducers. Int. J. Plant Prod. 11, 477–490. doi: 10.22069/IJPP.2017.3712
Hai-Yan, W., Zhang, H.-C., Qun, M., Qi-Gen, D., Zhong-Yang, H., Ke, X., et al. (2009). Photosynthetic characteristics of flag leaf in rice genotypes with different nitrogen use efficiency. Acta Agron. Sin. 35, 2243–2251. doi: 10.1016/S1875-2780(08)60120-0
Hasaneen, M., Abdel-aziz, H. M. M., and Omer, A. M. J. (2016). Effect of foliar application of engineered nanomaterials: carbon nanotubes NPK and chitosan nanoparticles NPK fertilizer on the growth of French bean plant. Biochem. Biotechnol. Res. 4, 68–76.
Haydar, M. S., Ghosh, D., and Roy, S. (2024). Slow and controlled release nanofertilizers as an efficient tool for sustainable agriculture: recent understanding and concerns. Plant Nano Biol. 7:100058. doi: 10.1016/j.plana.2024.100058
He, X., Zhu, H., Shi, A., and Wang, X. (2024). Optimizing nitrogen fertilizer management enhances Rice yield, dry matter, and nitrogen use efficiency. Agronomy 14:919. doi: 10.3390/agronomy14050919
Ingle, P. U., Shende, S. S., Shingote, P. R., Mishra, S. S., Sarda, V., Wasule, D. L., et al. (2022). Chitosan nanoparticles (ChNPs): a versatile growth promoter in modern agricultural production. Heliyon 8:e11893. doi: 10.1016/j.heliyon.2022.e11893
Jackson, M. L. (2005). Soil chemical analysis: Advanced course: A manual of methods useful for instruction and research in soil chemistry, physical chemistry of soils, soil fertility, and soil genesis. Wisconsin, USA: UW-Madison Libraries Parallel Press.
Krasilnikov, P., Taboada, M., and Amanullah,. (2022). Fertilizer use, soil health and agricultural sustainability. Agriculture 12:462. doi: 10.3390/agriculture12040462
Kumar, N., Samota, S. R., Venkatesh, K., and Tripathi, S. (2023). Global trends in use of nano-fertilizers for crop production: advantages and constraints–a review. Soil Tillage Res. 228:105645. doi: 10.1016/j.still.2023.105645
Kumari, A., Sharma, H., Kumari, A., Sharma, P., Pathak, N., Singh, R., et al. (2024). “Movement and signaling of macronutrients in plant system” in Essential minerals in plant-soil systems" (Elsevier), 1–28.
Ladha, J., Tirol-Padre, A., Reddy, C., Cassman, K., Verma, S., Powlson, D., et al. (2016). Global nitrogen budgets in cereals: a 50-year assessment for maize, rice and wheat production systems. Sci. Rep. 6, 1–9. doi: 10.1038/srep19355
Liu, K., Li, T., Chen, Y., Huang, J., Qiu, Y., Li, S., et al. (2020). Effects of root morphology and physiology on the formation and regulation of large panicles in rice. Field Crop Res. 258:107946. doi: 10.1016/j.fcr.2020.107946
Miranda-Villagómez, E., Trejo-Téllez, L. I., Gómez-Merino, F. C., Sandoval-Villa, M., Sánchez-García, P., and Aguilar-Méndez, M. Á. (2019). Nanophosphorus fertilizer stimulates growth and photosynthetic activity and improves P status in rice. J. Nanomater. 2019, 1–11. doi: 10.1155/2019/5368027
Moe, K., Htwe, A. Z., Thu, T. T., Kajihara, Y., and Yamakawa, T. (2019). Effects on NPK status, growth, dry matter and yield of rice (Oryza sativa) by organic fertilizers applied in field condition. Agriculture 9:109. doi: 10.3390/agriculture9050109
Mukhtiar, A., Zia, M. A., Alawadi, H. F., Naqve, M., Seleiman, M. F., Mahmood, A., et al. (2024). Role of iron oxide nanoparticles in maize (Zea mays L.) to enhance salinity stress tolerance. Notulae Botanicae Horti Agrobotanici Cluj-Napoca 52:13695. doi: 10.15835/nbha52313695
Peng, J., Feng, Y., Wang, X., Li, J., Xu, G., Phonenasay, S., et al. (2021). Effects of nitrogen application rate on the photosynthetic pigment, leaf fluorescence characteristics, and yield of indica hybrid rice and their interrelations. Sci. Rep. 11:7485. doi: 10.1038/s41598-021-86858-z
Poudel, A., Singh, S. K., Jiménez-Ballesta, R., Jatav, S. S., Patra, A., and Pandey, A. (2023). Effect of nano-phosphorus formulation on growth, yield and nutritional quality of wheat under semi-arid climate. Agronomy 13:768. doi: 10.3390/agronomy13030768
Ryan, J., Estefan, G., and Rashid, A. (2001). Soil and plant analysis laboratory manual : ICARDA Available at: https://repo.mel.cgiar.org/items/149b8091-6d46-4a67-9c1e-733c899b5428.
Sangwan, S., Pankaj, S., Leela, W., and Sahil, M. (2023). “Effect of chitosan nanoparticles on growth and physiology of crop plants” in Engineered nanomaterials for sustainable agricultural production, soil improvement and stress management (Academic Press), 99–123.
Sarkar, M. R., Rashid, M. H., Rahman, A., Kafi, M. A., Hosen, M. I., Rahman, M. S., et al. (2022). Recent advances in nanomaterials based sustainable agriculture: an overview. Environ. Nanotechnol. Monit. Manag. 18:100687. doi: 10.1016/j.enmm.2022.100687
Sathiyabama, M., and Manikandan, A. (2021). Foliar application of chitosan nanoparticle improves yield, mineral content and boost innate immunity in finger millet plants. Carbohydr. Polym. 258:117691. doi: 10.1016/j.carbpol.2021.117691
Seleiman, M. F., Almutairi, K. F., Alotaibi, M., Shami, A., Alhammad, B. A., and Battaglia, M. L. (2021). Nano-fertilization as an emerging fertilization technique: why can modern agriculture benefit from its use? Plan. Theory 10:2. doi: 10.3390/plants10010002
Seleiman, M. F., Elshayb, O. M., Nada, A. M., El-leithy, S. A., Baz, L., Alhammad, B. A., et al. (2022). Azolla compost as an approach for enhancing growth, productivity and nutrient uptake of Oryza sativa L. Agronomy 12:416. doi: 10.3390/agronomy12020416
Seleiman, M. F., Santanen, A., and Mäkelä, P. (2020). Recycling sludge on cropland as fertilizer-advantages and risks. Resour. Conserv. Recycling 155:104647. doi: 10.1016/j.resconrec.2019.104647
Seleiman, M. F., Selim, S., Jaakkola, S., and Mäkelä, P. (2017). Chemical composition and in vitro digestibility of whole-crop maize fertilized with synthetic fertilizer or digestate and harvested at two maturity stages in boreal growing conditions. Agric. Food Sci. 26, 47–55. doi: 10.23986/afsci.60068
Sheoran, P., Goel, S., Boora, R., Kumari, S., Yashveer, S., and Grewal, S. (2021). Biogenic synthesis of potassium nanoparticles and their evaluation as a growth promoter in wheat. Plant Gene 27:100310. doi: 10.1016/j.plgene.2021.100310
Singh, A., Rajput, V. D., Varshney, A., Sharma, R., Ghazaryan, K., Minkina, T., et al. (2024). Revolutionizing crop production: nanoscale wonders-current applications, advances and future Frontiers. Egypt. J. Soil Sci. 64, 221–258. doi: 10.21608/ejss.2023.246354.1684
Sirisena, D., Dissanayake, D., Somaweera, K., Karunaratne, V., and Kottegoda, N. (2012). “Use of nano-K fertilizer as a source of potassium in rice cultivation” in First National Nanotechnology Conference, 98.
Srikanth, B., Subrahmanyam, D., Sanjeeva Rao, D., Narender Reddy, S., Supriya, K., Raghuveer Rao, P., et al. (2023). Promising physiological traits associated with nitrogen use efficiency in rice under reduced N application. Front. Plant Sci. 14:1268739. doi: 10.3389/fpls.2023.1268739
Sundararajan, N., Habeebsheriff, H. S., Dhanabalan, K., Cong, V. H., Wong, L. S., Rajamani, R., et al. (2024). Mitigating global challenges: harnessing green synthesized nanomaterials for sustainable crop production systems. Global Chall. 8:2300187. doi: 10.1002/gch2.202300187
Tomar, B., Tomar, S. S., Parihar, S. S., Patle, T., Patel, H., and Singh, P. (2024). “Advanced and intelligent nanofertilizer base soil management for sustainable agriculture” in Sustainable agriculture Nanotechnology and Biotechnology for Crop Production and Protection, vol. 17.
Tyagi, A., Chakraborty, N., and Raghuram, N. (2024). Morpho-physiological evaluation of Indica Rice genotypes with contrasting crop duration for nitrogen use efficiency under graded urea doses. J. Plant Growth Regul., 1–22. doi: 10.1007/s00344-024-11437-1
Upadhyay, P. K., Singh, V. K., Rajanna, G., Dwivedi, B. S., Dey, A., Singh, R. K., et al. (2023). Unveiling the combined effect of nano fertilizers and conventional fertilizers on crop productivity, profitability, and soil well-being. Front. Sustain. Food Syst. 7:1260178. doi: 10.3389/fsufs.2023.1260178
Vance, C. P., Uhde-Stone, C., and Allan, D. (2003). Phosphorus acquisition and use: critical adaptations by plants for securing a nonrenewable resource. New Phytol. 157, 423–447. doi: 10.1046/j.1469-8137.2003.00695.x
Vejan, P., Khadiran, T., Abdullah, R., and Ahmad, N. (2021). Controlled release fertilizer: a review on developments, applications and potential in agriculture. J. Control. Release 339, 321–334. doi: 10.1016/j.jconrel.2021.10.003
Verma, K. K., Song, X.-P., Joshi, A., Tian, D., Rajput, V. D., Singh, M., et al. (2022). Recent trends in nano-fertilizers for sustainable agriculture under climate change for global food security. Nano 12:173. doi: 10.3390/nano12010173
Vijayakumar, S., Kumar, D., Ramesh, K., Bussa, B., Kaje, V., and Shivay, Y. (2024). Effect of split application of potassium on nutrient recovery efficiency, soil nutrient balance, and system productivity under rice-wheat cropping system (RWCS). J. Plant Nutr. 47, 1546–1563. doi: 10.1080/01904167.2024.2315974
Vijayalakshmi, P., Vishnukiran, T., Kumari, B. R., Srikanth, B., Rao, I. S., Swamy, K., et al. (2015). Biochemical and physiological characterization for nitrogen use efficiency in aromatic rice genotypes. Field Crop Res. 179, 132–143. doi: 10.1016/j.fcr.2015.04.012
Watanabe, F., and Olsen, S. (1965). Test of an ascorbic acid method for determining phosphorus in water and NaHCO3 extracts from soil. Soil Sci. Soc. Am. J. 29, 677–678. doi: 10.2136/sssaj1965.03615995002900060025x
Wellburn, A. R. (1994). The spectral determination of chlorophylls a and b, as well as total carotenoids, using various solvents with spectrophotometers of different resolution. J. Plant Physiol. 144, 307–313. doi: 10.1016/S0176-1617(11)81192-2
Yoshida, S. (1981). Fundamentals of rice crop science. Los Baños, Philippines: International Rice Research Institute.
Yoshida, S., Forno, D. A., and Cock, J. H. (1971). Laboratory manual for physiological studies of rice. Los Baños, Philippines: International Rice Research Institute.
Keywords: chitosan-based NPK nanostructure, rice, productivity, sustainable agriculture, food security
Citation: Elshayb OM, Ghazy HA, Wissa MT, Farroh KY, Wasonga DO and Seleiman MF (2024) Chitosan-based NPK nanostructure for reducing synthetic NPK fertilizers and improving rice productivity and nutritional indices. Front. Sustain. Food Syst. 8:1464021. doi: 10.3389/fsufs.2024.1464021
Edited by:
Raffaella Balestrini, National Research Council (CNR), ItalyReviewed by:
Muthuraman Yuvaraj, Tamil Nadu Agricultural University, IndiaAnand Kumar Chaudhari, Government Girls’ P.G. College, India
Copyright © 2024 Elshayb, Ghazy, Wissa, Farroh, Wasonga and Seleiman. This is an open-access article distributed under the terms of the Creative Commons Attribution License (CC BY). The use, distribution or reproduction in other forums is permitted, provided the original author(s) and the copyright owner(s) are credited and that the original publication in this journal is cited, in accordance with accepted academic practice. No use, distribution or reproduction is permitted which does not comply with these terms.
*Correspondence: Mahmoud F. Seleiman, mseleiman@ksu.edu.sa