- 1Department of Food Science, College of Agriculture and Veterinary Medicine, United Arab Emirates University, Al Ain, United Arab Emirates
- 2Zayed Centre of Health Science, United Arab Emirates University, Al Ain, United Arab Emirates
There is a growing demand for plant-based protein ingredients with improved functionality for use in diverse food and nutraceutical applications. In line with this, the current study aimed to investigate the effect of plant protein-prebiotic (polydextrose) conjugation on the techno-functional properties (emulsification, solubility, fat absorption and foaming) of pea proteins through wet heating Maillard reaction. Pea protein (PeP) was conjugated with polydextrose by incubating the mixture at various process conditions (pea protein: polydextrose mass ratios, temperature, and time). Response surface methodology coupled with Box–Behnken design was used to optimize multiple responses, including conjugation efficiency (CE), emulsifying activity (EAI), and foaming capacity (FC). The pea protein conjugate (optimized value) showed improved solubility throughout a wide pH (2–10) range and higher emulsification activity than pea protein alone. The development of conjugates (PeC) was validated through ultraviolet–visible spectroscopy, FTIR, and o-Phthaldialdehyde (OPA) assay. Browning index, FT-IR spectra, thermal properties, and scanning electron microscopy (SEM) micrographs were analyzed for the conjugate (PeC) obtained at optimized values. The FTIR spectra of the conjugates showed new peaks at 3100–3480 cm−1 and 1,000–1,166 cm−1 indicating conjugation. The Maillard conjugation increased the proportion of β-turn, random coil, accompanied by a decrease in α-helix, and β-sheet. These conformational changes were associated to the improved techno-functional properties of the pea protein upon conjugation, offering potential applications in the formulation of plant-based foods and beverages.
1 Introduction
Due to the diminishing natural resources and the rising world population, it is hard to satisfy the consumer’s demand for animal proteins using solely traditional techniques. A systematic approach involves exploring several alternative protein sources to guarantee a steady supply. Moreover, environmental problems regarding methane emission from livestock are also pushing the demand for more sustainable protein alternatives with similar techno-functional characteristics, and a lower carbon footprint (Kumar et al., 2022). With this growing scientific and commercial interest, plant proteins have recently gained the attention as a source of alternative proteins to partially substitute animal proteins (Bryant and Sanctorum, 2021). Numerous studies have emphasized the health advantages of consuming plant protein, which is typically thought to have hypoallergenic proteins (Estell et al., 2021). Plant proteins have various functional properties, such as water, and oil holding capacity, solubility, emulsifying, foaming, and gelling properties, and health benefits like antihypertensive, antioxidant, and modulating intestinal bacteria activities (Grossmann and Weiss, 2021). From a consumer perspective, plant-based products are more ethical, environmentally friendly, and nutritious than animal proteins.
Pea protein has gained much interest from the agro-food market as pea is cheap and environmentally sustainable. Pea (Pisum sativum L.) is widely grown worldwide in over 85 countries as a cool-season vegetable crop (Zha et al., 2019; Zha et al., 2021). Peas are used in various food-based applications as protein isolates, produced from pea powder by following specific processing steps such as solubilization and concentration (Kutzli et al., 2020).
Pea protein contains high levels of lysine, threonine, and tryptophan along with other beneficial characteristics like high digestibility, no transgenic origin, and low allergenicity. Irrespective of many advantages pea proteins have some limitations, such as their low solubility, low surface charge, and an abundance of hydrophobic residues on the surface of the molecules, these characteristics contribute to their poor functional properties, specifically in terms of solubility and emulsifying properties (Shanthakumar et al., 2022; Schneider et al., 2023). Consequently, the applications of pea protein isolates in the food industry are challenging and influence their market share (Zha et al., 2021; Ke and Li, 2023). However, different modification procedures have been implemented to improve the plant protein isolates’ functionality (Saatchi et al., 2019; Sim et al., 2021; Ke and Li, 2023). Modification procedures include physical, chemical, enzymatic, and combination treatments to enhance their techno-functionality for increasing their application in different food systems (Saatchi et al., 2019). Among the listed approaches, the Maillard reaction is the most promising and non-enzymatic approach for protein conjugation. The Maillard reaction is a natural chemical process that occurs at high temperatures, involving the condensation of free α- and ε-amino groups of proteins with carbonyl groups of carbohydrates, resulting in the formation of glycoconjugates. This reaction takes place without adding any additives and plays an important role in the formation of color and flavor in the food. Recent studies on the functional characteristics of pea protein isolates and Gum Arabic conjugates produced through the Maillard reaction have shown improvement in techno functional properties of pea proteins (Zha et al., 2019; Zha et al., 2021).
Plant proteins have been intensively explored for diverse applications and utilizations in the food system. Recent research has shown that protein-polysaccharide conjugation can play an important role in enhancing the technological functionality of underused plant proteins (Dai et al., 2017; Zha et al., 2019). In this context, using prebiotics-based molecules in conjugation with the plant-derived proteins, can effectively modify the structural conformation of the proteins and enhance their biological and technological functionality together (Van den Abbeele et al., 2013; Zha et al., 2019).
Prebiotics are mainly oligosaccharide-based molecules known to have multiple health-promoting benefits including positive effect on stool quality, low risk of gastroenteritis, and lowincidence of allergy symptoms such as atopic eczema (Paganini et al., 2017; Urango et al., 2024). A recent study on pea protein conjugation with inulin via Maillard reaction has greatly enhanced the emulsification (Jiang et al., 2022). The study showed that pea protein-inulin conjugates were even found to have better antioxidant properties than proteins alone. Similar results were also reported for whey protein hydrolysates conjugated with inulin, where significant improvements in emulsification and antioxidant activities were observed after inulin grafting with whey protein isolates (Wang et al., 2020).
Litesse® polydextrose is a carbohydrate known for its low-calorie and low-glycemic properties as well as prebiotic characteristics (Tiihonen et al., 2008). To the best of our knowledge, no such study exist on the impact of polydextrose conjugation with proteins on their techno functional properties. Therefore, this study aimed to explore the effects of conjugating prebiotics (Litesse® polydextrose) with plant protein (Pea Protein), via the Maillard reaction, a combination not extensively explored before to develop new protein-prebiotic conjugates with improved functional properties through safe and cost-effective methods. Unlike previous studies, it focuses on enhancing both the techno-functional properties and health benefits of pea proteins, offering a novel, eco-friendly solution for developing superior plant-based protein products.
2 Materials and methods
Pea protein isolates used in this study were procured from ET-proteins headquartered in Suzhou, China. The prebiotic carbohydrate (Litesse® polydextrose) used in this study was procured from International Flavors & Fragrances Inc. (IFF, Dubai). All other analytical chemicals used such as sodium dodecyl sulfate (SDS), sodium borate, o-Phthaldialdehyde (OPA), methanol, β-mercaptoethanol and others were all procured from Sigma Aldrich (MO, United States). The protein concentration in the pea protein isolate sample (on a dry basis) was 86.0%.
2.1 Optimization of the pea protein isolates and polydextrose conjugates
For the optimization of pea protein (PeP) and polydextrose conjugation, the response surface methodology (RSM) model was developed based on the Box–Behnken design (BBD). The treatment temperature (X1) (60, 80, and 100°C), time (X2) (1, 2, and 3 days), and pea protein polydextrose ratio (X3) 0.5, 1, and 2 w/w were selected as the independent factors, meanwhile, conjugation efficiency (CE), emulsifying activity index (EAI) and foaming capacity (FC) of conjugates were the response factors. The experimental data were analyzed using Design-Expert software (version 13.0), and 15 sets of experimental design data were generated for Pea protein: polydextrose conjugate (PeC), as shown in Table 1. Actual and predicted values for foaming capacity and emulsion activity are also framed in Table 2. The proposed second-order polynomial model for the response surface analysis is presented in Equation 1:
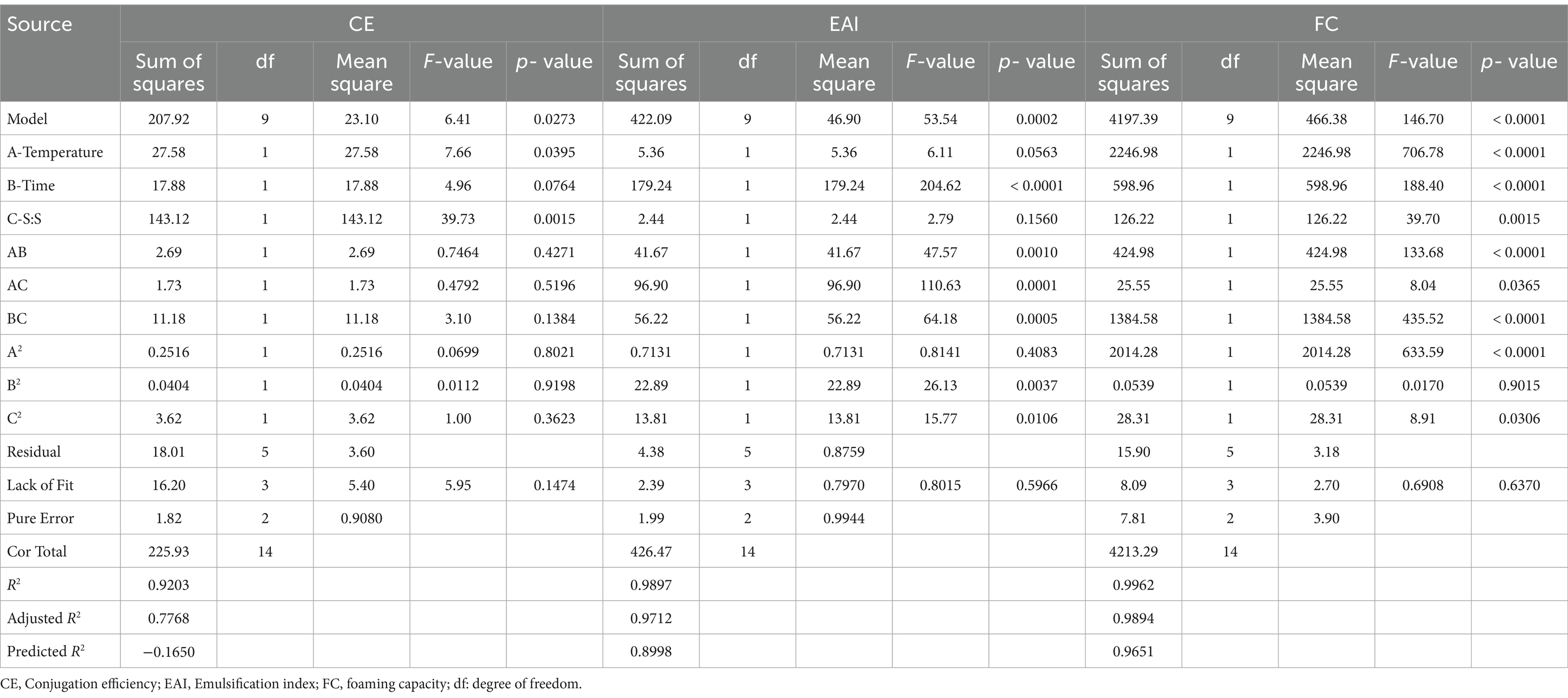
Table 2. Quadratic model and Response foaming for pea protein- polydextrose conjugates as analyzed by ANOVA.
In this context, Y represents the predicted responses, where β0 is the model constant, βi, βii, and βij denote the linear, squared, and 2-way interaction effects, respectively. Meanwhile, Xi and Xj stand for the independent factors in the model.
2.2 Preparation of pea protein-polydextrose conjugates
The wet heating Maillard reaction (MR) method as described by Wang et al. (2020) was used to prepare conjugates based on pea protein isolate and polydextrose with some modifications. Initially, pea proteins were dissolved by adjusting the pH to 10.0 using 1 M NaOH and stirred at room temperature for 4 h, following which the pH was adjusted to 7.0 using 1 M HCl. Further, PeP and polydextrose were mixed with a mass ratio (0.5–2.0 w/w) using a homogenizer at 3200 rpm for 10 min. To attain a pH of 8.0 in the reaction mixture, a 1 M NaOH solution was utilized. The final reaction mixtures were subjected to heating in a water bath for varying lengths of time (1–3 days) and at various temperatures (60–100°C). The Maillard reaction was stopped by shifting the solutions to the ice to let the temperature to drop to 25°C. All the samples were then lyophilized for further analysis.
2.3 Conjugation efficiency (CE)
Conjugation efficiency was measured using the method as described by Zhong et al. (2021). For this, o-Phthaldialdehyde (OPA) assay, which estimates total free amino groups in the conjugates after the Maillard reactions, was used to calculate the conjugation efficiency. Further, 20 μL of the samples were seeded onto 96-well microplates and mixed with 200 μL of OPA reagent. The absorbance (Abs) was measured at 340 nm using the SkanIt™ Software (Multiskan SkyHigh microplate reader, Thermo Fisher Scientific, United States). CE % was measured according to Equation 2 as follows:
2.4 Emulsifying activity index
EAI was calculated for the pea protein (PeP) and pea protein-polydextrose conjugates (PeC) samples using a method validated in our laboratory and reported by Al-Shamsi et al. (2018). A 2 mL of PeC solution (5% w/v) at different pH ranging from 2 to 10 was mixed with a similar amount of sunflower oil in a 10 mL glass tube. The mixtures were homogenized for 1 min at 20,600 rpm using an ultra-turrax homogenizer (Janke & Kunkel, Ultra-Turrax T25) and carefully 50 μL of the emulsion was removed from the bottom and combined with 5 mL of a 0.1% sodium dodecyl sulfate (SDS) solution. 300 μL of this solution was then transferred to 96 well microplate and the absorbance of the samples was read at 500 nm in a Multiskan SkyHigh Microplate Spectrophotometer (ThermoFisher Scientific, Richmond, United States). EAI values were then calculated using the formula described by (Pearce and Kinsella, 1978) as follows in Equation 3:
2.5 Foaming capacity
The analysis of foaming capacity was conducted following the method previously described by Baba et al. (2024) For this, the pH of the solutions was first adjusted to 2, 4, 6, 8, and 10 using a digital pH meter (Ohaus starter ST3100-B, NJ, USA) using 1 M HCl or 1 M NaOH. The solutions thus obtained were homogenized in a homogenizer for 3 min at a speed of 13,400 rpm in a measuring cylinder after which foaming capacity was determined using the Equation as follows in Equation 4:
2.6 Fat absorption capacity
The fat absorption capacity of PeP and PeC was determined as per the protocol reported by Maqsood et al. (2019). Briefly, 1 mL of sunflower oil and 100 mg of powdered samples were mixed and vortexed vigorously for a few minutes. Thereafter, free oil was removed by centrifugation at 13,600 x g for 30 min at 25°C. Afterward, the oil was emptied from the tubes at a 45-degree angle. Samples’ capacities for absorbing fat were determined using the formula shown in Equation 5:
2.7 Protein solubility in water
To find out the influence of pea protein and polydextrose conjugation on plant protein solubility method described earlier by Al-Shamsi et al. (2018), was used. For this, 200 mg of each sample, i.e., PeP as control and PeC, were dissolved in deionized water (20 mL) by vortexing and then centrifuged at 7500 g, 25°C, for 15 min. The protein content in the supernatants was measured using bicinchoninic acid assay as described by Nongonierma et al. (2019), and analyzed as milligrams of protein per gram of sample.
2.8 Browning intensity
The Browning intensity of the samples was investigated, as reported by Chailangka et al. (2022) with some modifications. A UV-1800 spectrophotometer (Shimadzu UV-1601 PC, Shimadzu Corp., Kyoto, Japan) was used to measure the absorbance of the samples at 294 and 420 nm. In order to determine the Browning intensity of the samples, a modified version of the procedure described by Capar and Yalcin, (2021) was used.
2.9 Differential scanning calorimeter
DSC was used to examine the thermal behavior of PeP and PeC (TA Instruments, DSC Q100, United States). All dried samples (2–3 mg) were fed into the apparatus chamber at room temperature in hermetically sealed aluminum pans. To create a controlled atmosphere around the sample, a flow of nitrogen gas at a rate of 40 mL/min was used in the purge line. The temperature gradually increased from 60°C to 200°C at a rate of 10°C/min. The DSC instrument’s universal analysis software was used to examine the data and Tg Peak of gelatinization was measured as characteristic temperatures.
2.10 Fourier transform infrared spectroscopy
FTIR spectra were recorded in Attenuated-total-reflectance (ATR) mode using a Spectrum-2 PerkinElmer FT-IR Spectrophotometer (model number 103146, United Kingdom). Samples were placed on the diamond detector and scans were then taken of each sample at a resolution of 4 cm−1 and 32 scans in the 500–4,000 cm−1 range. The spectral data were analyzed using Spectrum-2 Peak Analyzer software. Baseline corrections were carried out using the zeroes method of the second derivative to locate anchor points and identify the baseline. The second derivative method was used to find hidden peaks, and then the Savitsky-Golay function with 10 points and a polynomial order of 2 was used to smooth the data. All these procedures were performed using OriginPro 8.5 software.
2.11 Scanning electron microscopy
Microstructural features and particle morphology of spray-dried pea proteins and their polydextrose conjugates were observed using a Scanning Electron Microscope (JEOL JSM-6010LA) (SEM, Akishima, Tokyo, Japan). The samples were finely sprinkled on aluminum stubs containing double-sided carbon tape, sputtered with approximately 10 nm conductive layer of gold, and then scanned under vacuum by NeoScope JCM-5000 benchtop SEM from JEOL Ltd. in Tokyo, Japan. Micrographs of the samples were taken at different appropriate magnifications to find the best micrograph and 650x was selected and presented in the results.
2.12 Statistical analysis
All the conjugates (pea protein and polydextrose) were prepared in 3 batches and further analysis was done in triplicate. Mean differences were estimated using Tukey’s multiple range test via one-way ANOVA by SPSS 28.0 (IBM, United States) Software. Data is presented as the mean and standard deviation (n = 3) of three independent replicates.
3 Results and discussion
3.1 Optimization of Maillard reaction-based conjugation preparation
This study has investigated the most important factors affecting the Maillard reaction, i.e., incubation time, temperature, and protein & prebiotic ratio. The range for all three independent parameters, i.e., time, temperature, and solid-to-solid ratio, was selected based on a one-factor-at-a-time (OFAT) experiment performed in our lab (data not shown here). RSM was used to design the 15 experimental sets using three indented factors, as shown in Table 3. All the responses were assessed in relation to the quadratic response and interaction terms of the independent variables, which included plant protein and prebiotic ratio (S:S), temperature, and time. Regression analysis and ANOVA were employed to fit the model and examine the statistical significance of the terms. Table 2 displays the coefficients of determination (R2) for each dependent variable and the regression coefficients for the quadratic polynomial models. The R2 values for the conjugation efficiency, foaming, and emulsifying activities were 0.92, 0.99, and 0.98, respectively. The effect of solid-to-solid ratio, temperature, and time on the formation of plant protein and polydextrose conjugates is statistically analyzed in Table 2. The F value of the selected model for CE, EAI, and FC was found to be 6.41, 53.54, and 146.70, respectively. The probability of the F value for the model is found to be less than 0.05. The ANOVA results indicate that the selected model is a suitable fit for the experimental data in practical terms. The probability of the selected quadratic model was found to be significant for each response. The effect of coded independent process variables on CE, EAI, and FC are shown in Figure 1, respectively. For plant protein-based conjugates, the independent variables such as time, temperature, and solid: solid ratio directly affect the dependent responses, including CE, EAI, and FC. According to the desirability framework, utilizing 0.50 protein-to-prebiotic ratios and heating at 100°C for 1.0 day would result in the greatest desirability level of 0.78 on a scale of 0 to 1. The anticipated values for CE, FC, and EAI at these derived conditions were 31.26, 67.25, and 20.92%, respectively. When the experiment was conducted, the observed values were 30.24, 66.61, and 20.49% for CE, FC, and EAI, respectively.
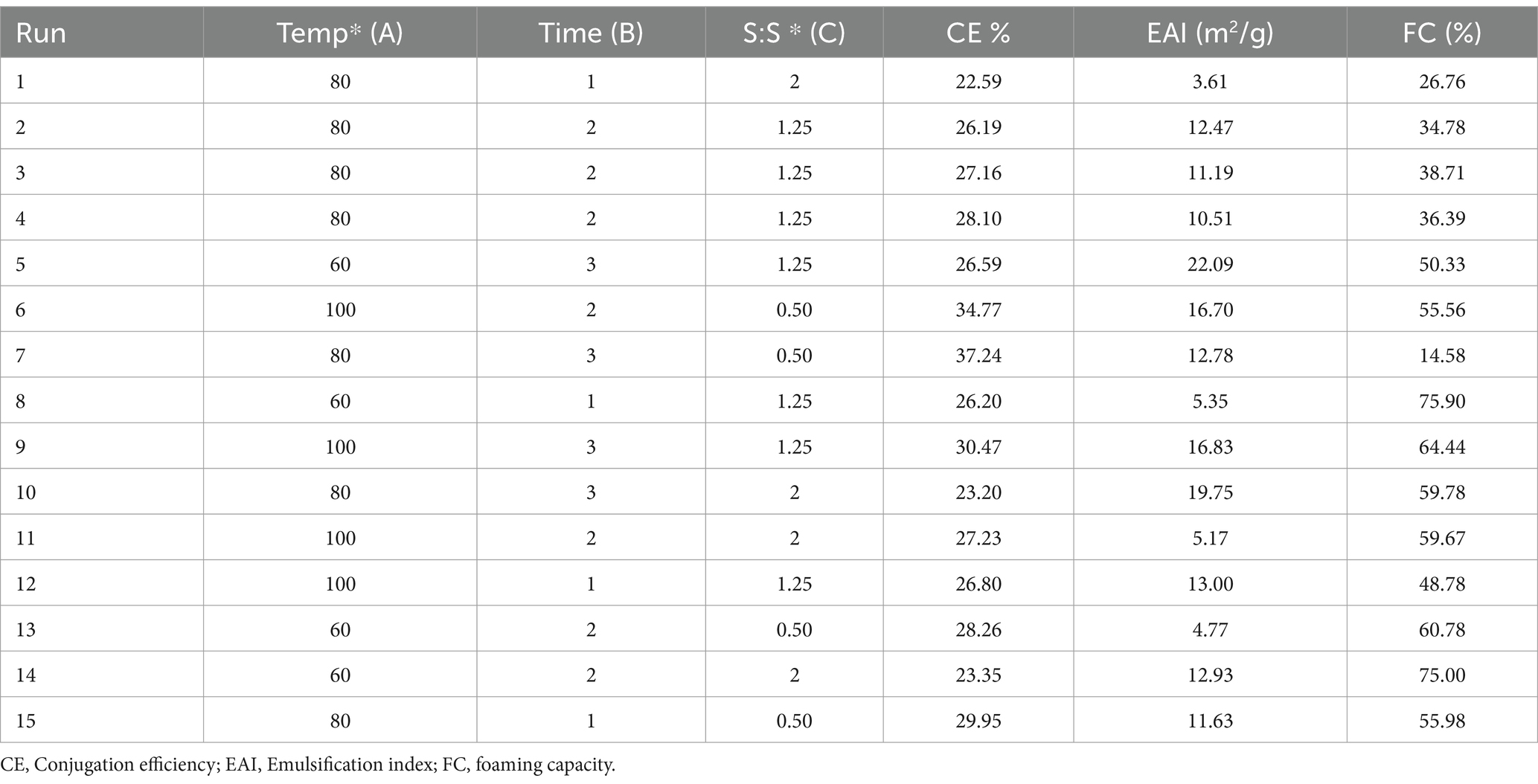
Table 3. Box–Behnken design matrix with experimental results and predicted values of CE, EAI and FC.
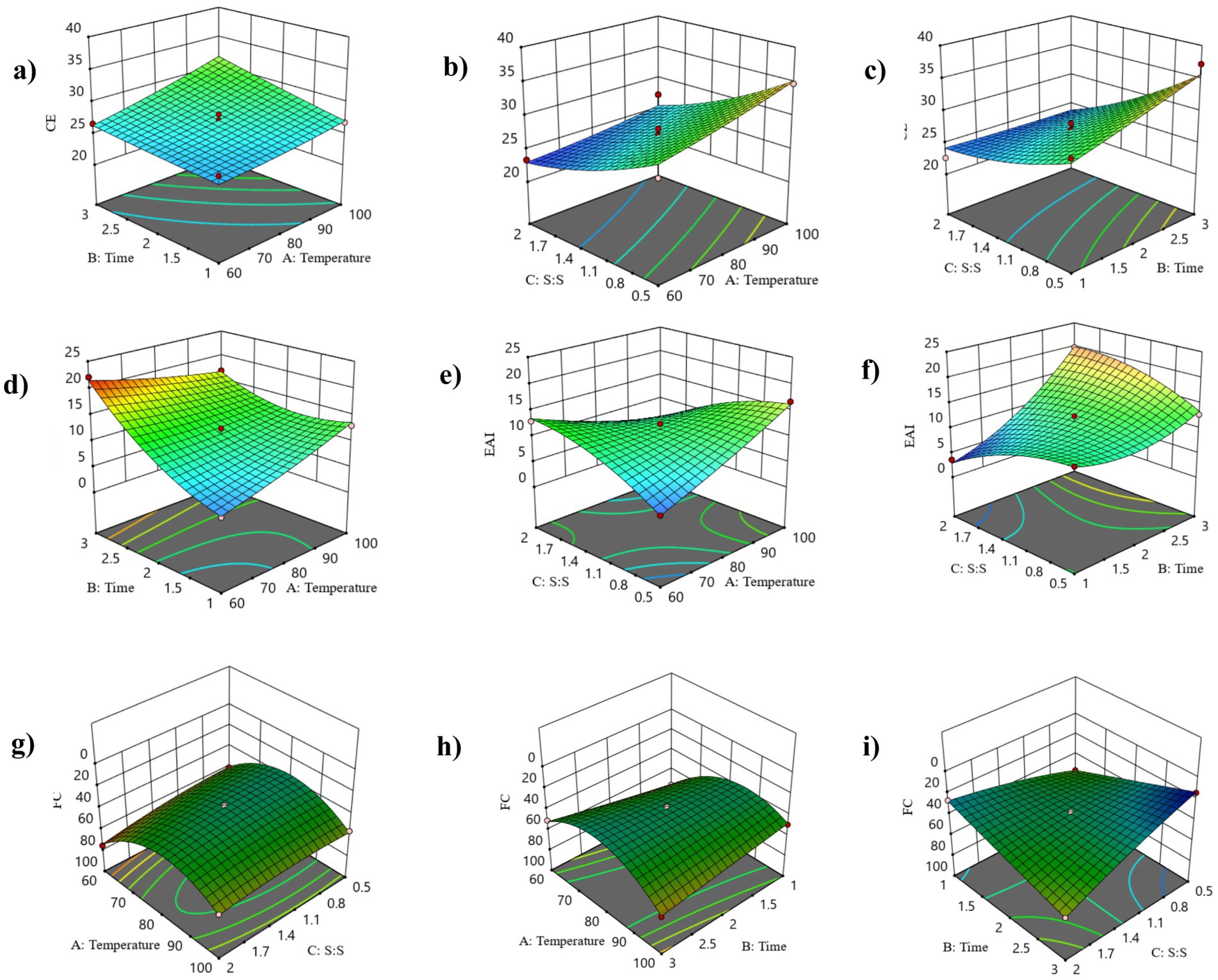
Figure 1. The 3D response curves for conjugation efficiency (CE), emulsification activity index (EAI), and foaming capacity (FC) are represented as functions of time and temperature (A, D, G), protein-prebiotic ratio and temperature (B, E, H), and protein-prebiotic ratio and time (C, F, I), respectively.
3.1.1 Conjugation efficiency
An OPA assay was conducted using 15 experimental data sets to measure the Conjugation efficiency (CE). The CE was reflected in reduced free amino groups per gram of protein throughout the Maillard reaction (Capar and Yalcin, 2021). The CE values for all the conjugates ranged between 22.59 and 37.24%. Significant factors (p < 0.05) in the model equation were temperature and protein-prebiotic ratio. In Table 2, the model shows 92.03% accuracy for CE. The maximum CE (37.24%.) was recorded with the 1:1 protein-prebiotic ratio at 80°C temperature for 3 days. Visual analysis was conducted to explore how the interaction between two design variables influences the response variables when one design variable is held constant at its central value. Changes in the CE values with respect to time, temperature, and protein-prebiotic ratio are depicted in Figures 1a–c. Throughout all design variable interactions, the interaction between temperature and time has the most significant effect on CE. There is a noticeable increase in CE with increasing temperature and time. The most notable influence on CE is shown in the relationship between temperature and protein-prebiotic ratios, where a decrease in protein-prebiotic ratios and an increase in temperature have a substantial additive effect on CE. The interaction between time and protein-prebiotic ratios has the most significant impact on CE. Increasing the time while decreasing protein-prebiotic ratios resulted in a substantial improvement in CE.
The Maillard reaction occurs between reducing carbonyl groups in sugars and the amino groups in proteins or peptides through covalent bonding, which leads to the production of the Schiff base (Capar and Yalcin, 2021). Consequently, the reduction of free amino groups can be related to protein engagement during the glycosylation reaction. These authors also reported an improvement in the functional properties of proteins while conjugating between protein -polysaccharides conjugation through Maillard reactions in an aqueous media. Using optimization via Response Surface Methodology (RSM), the most effective conjugation was achieved for faba bean protein and Carrageenan under specific conditions. This included a protein-polysaccharide ratio of 1:2, a reaction temperature of 90°C, and a reaction time of 60 min, which resulted in the best outcome.
3.1.2 Emulsification activity index
A range of 3.61 to 22.09 m2/g was observed for the EAI values of the samples after the Maillard reaction. The model equation indicated that the protein-prebiotic ratio was a significant factor (p < 0.05). The model expresses the EAI with 98.97% accuracy can be seen in Table 2. At 60°C for 3 days, the maximum EAI (22.09 m2/g) was recorded with a protein-prebiotic ratio of 1.25. Figures 1d–f shows the changes in the EAI values depending on the time, temperature, and protein-prebiotic ratio. Among all design variable interactions, the combination of temperature and time has the most significant impact on EAI. Lowering the temperature while increasing the time results in a notable enhancement of EAI. It is apparent that the interaction between temperature and protein-prebiotic ratios has exhibited a minimal effect on EAI. The most important influence on EAI is seen by the connection between temperature and protein-prebiotic ratios, where increasing protein-prebiotic ratios and temperature significantly enhanced EAI.
The expanded secondary structure of the molecules may be primarily responsible for improving the EAI of conjugated proteins. The molecules’ altered shape, which has more extended secondary structures, could help them adhere to the oil–water interface more quickly, which would improve the protein’s ability to emulsify. Moreover, it is expected that heating proteins might have altered the secondary structures of proteins, causing additional rearrangement of modified proteins at the oil droplet interfaces. It is well-known that glycoproteins possess excellent interfacial stability, primarily attributed to their superior interfacial arrangement during emulsification. In glycoproteins, the hydrophobic protein fractions are embedded within the oil phase, whereas the amphiphilic carbohydrate fractions have an affinity for the water phase, leading to the creation of a dense layer at the oil–water interface (Li et al., 2023).
3.1.3 Foaming capacity
The challenges of natural proteins in food industry applications, such as cosmetics and foam food, can be resolved by improving the foaming capabilities of proteins. The FC value ranged between 14.58 and 75.9% in all the experimental data sets. Notably, the model equation identified time, temperature, and protein-prebiotic ratio as significant factors (p < 0.05) in predicting the FC value. The maximum FC (75.9%) was recorded with the 1.25 protein-prebiotic ratio at 60°C for 1 day. Figures 1g–i illustrates the variations in the FC value resulting from differences in time, temperature, and protein-prebiotic ratio. Among all design variable interactions, the combination of temperature and time has the most significant impact on FC. Reducing both temperature and time leads to a significant enhancement in FC. The interaction between temperature and protein-prebiotic ratios exhibits the most significant effect on FC, with increasing protein-prebiotic ratios and decreasing temperature exhibiting a significant enhancement effect on FC. The interaction between time and protein-prebiotic ratios exhibited a minimal effect on FC.
The most effective explanatory models for CE, EAI, and FC were identified through regression analysis and are presented in Equations 6–8, respectively.
Where, CE, Conjugation efficiency; EAI, Emulsification index; FC, Foaming capacity; A, Temperature; B, Time; and C, solid to solid ratio
3.2 Effect of pH on emulsification activity index (m2/g)
Additionally, the emulsifying activity of pea proteins before and after conjugation with polydextrose was investigated against the various pH (2–10) using the optimized value as shown in Figure 2A. Overall, at all pH levels, it is evident that the emulsifying activities of the PeC samples were much better than those of pea protein (PeP) alone. The lowest EAI (m2/g) for all the samples was detected at pH 4.0, the isoelectric range of protein, and is attributed to the reduction of solubility of proteins at this pH. Overall, these improved emulsification activities at the neutral pH and alkaline ranges are like those obtained by other researchers. For instance, Karbasi and Askari (2021), also signified improved EAI (m2/g) activity of whey proteins when conjugated with various mono-oligo and polysaccharides by the Maillard reaction. The higher level of emulsification activity among conjugates might be ascribed to the possible covalent coupling between polyols groups of prebiotic carbohydrates that played a significant role in changing the hydrophobic nature of plant proteins to more amphiphilic making them more surface active and absorbable at the O/W interface (Maqsood et al., 2019).
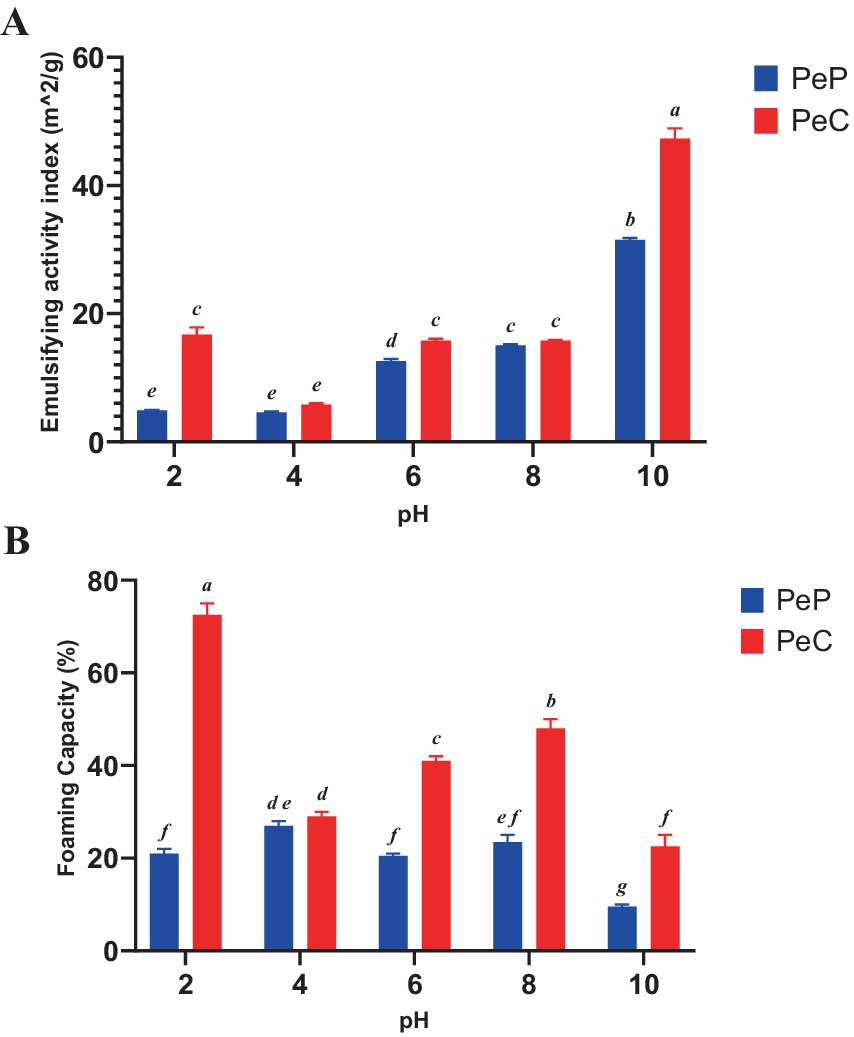
Figure 2. Functional properties of pea protein and pea protein conjugate as shown by (A) emulsifying activity index (EAI) and (B) Foaming capacity (FC) with different pH 2, 4, 6, 8, and 10, for 24 h. Different letters indicate significant differences (p < 0.05).
3.3 Effect of pH on foaming capacity
Additionally, FC of pea proteins and conjugation with polydextrose was investigated against the various pH (2–10) using the optimized value as displayed in Figure 2B. Many factors, including protein unfolding, pH, protein concentration, temperature, mixing duration, and foaming technique, have an impact on the foaming properties of proteins. The changes in the foaming capacity of protein upon conjugation with carbohydrates are ascribed to the possible development of new functional groups on conjugation. The decline in foaming capacity at pH 4.0 is usually due to their isoelectric point of the protein. Proteins exhibit less solubility at an isoelectric point and foam is usually formed by soluble proteins; hence at the isoelectric point where soluble proteins are in less number leads to lower foaming capacity (Maqsood et al., 2019). It is also noteworthy to notice that there was a possible shift in the isoelectric point of protein conjugates. As for native proteins, no decline in foaming capacity was observed as the foaming properties of proteins are influenced by numerous parameters, including concentration of proteins, method of isolation, pH, temperature, speed, and time of mixing. Analogous findings were also reported by Wang et al. (2020), who found that conjugating whey proteins with inulin enhanced forming capacity.
3.4 Fat absorption capacity: analysis and measurement
The potential of proteins to bind fats is essential for food applications, primarily because it improves mouthfeel and flavor retention (Wang and Arntfield, 2017). The fat absorption capacity percentage of pea protein and pea protein-polydextrose conjugate (optimized value) are shown in Figure 3A. The results did not display any significant difference between all samples before and after conjugation (p > 0.05). FAC of approximately 19% was observed for the PeP sample which did not show any significant improvement after conjugation and reached a value of only 20%. It has been stated that the FAC is higher in protein powders with lower density, as smaller particles allow for a greater entrapment of oil compared to protein powders with higher density (Maqsood et al., 2019). The number of non-polar sites, protein-lipid-carbohydrate interactions, and protein concentration can influence FAC. Proteins that are insoluble and hydrophobic possess a strong ability to bind with oils (Nakai, 1983; Chan et al., 2024).
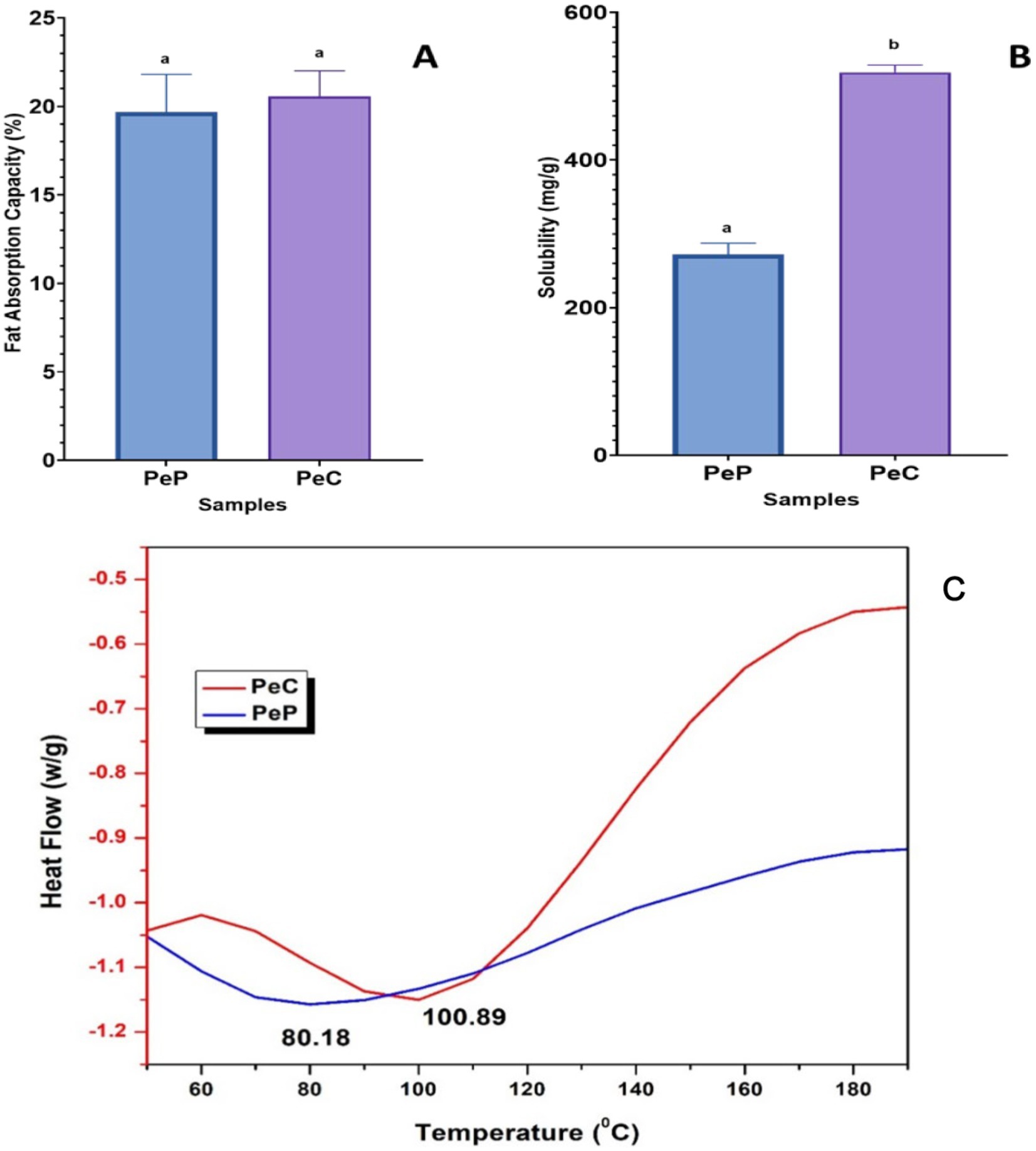
Figure 3. (A) The fat absorption capacity of the native protein and their conjugates (B) Protein solubility (C) DSC thermogram of pea proteins and pea protein conjugates at pH 7.0, prepared by conjugation with polydextrose Different letters indicate significantly different (p < 0.05).
3.5 Protein solubility in water
Protein solubility is one of the most crucial characteristics of proteins, which impacts their the texture, color, and sensory qualities of products (Boonlao et al., 2023). The composition of proteins has a direct impact on their utilization in food formulations and is closely linked to their ability to exhibit desirable physical, chemical, and functional properties, such as water retention, emulsification, and gelling (Kutzli et al., 2021). The outcomes showed that protein conjugation dramatically altered the samples’ solubility pattern. As seen in Figure 3B, the conjugated pea protein’s (PeC) (optimized value) solubility (460.17 mg/g) was more than that of pea protein alone (PeP), which was only 271.73 mg/g. These findings showed that while conjugation might significantly increase the protein’s solubility, pea proteins were weakly soluble in water. Comparable results were also observed by Boonlao et al. (2023), where the conjugation of soy proteins with maltodextrin led to enhanced protein solubility and foaming properties through conjugates. The increased solubility of protein upon conjugation could be attributed to exposed hydrophilic hydroxyl groups that may be aligned on the surface of conjugated proteins, thus enhancing the interaction of the protein with water (Zha et al., 2021).
3.6 Browning intensity
The browning intensity was also used to analyze the extent of the Maillard reaction. Additionally, UV–visible absorption measurements were performed at 294 and 420 nm on pea protein and optimized value of conjugate to check the possible formation of Amadori, Heyns, and melanoidin compounds during both the initial and final stages of the Maillard reaction process. Amadori and Heyns compounds might be formed at the early stage of MR and are not supposed to show a brown color at 294 nm. However, the presence of melanoidin compounds was confirmed by measuring the absorption at 420 nm during the final stage of Maillard reaction (Chailangka et al., 2022). The brown color development could be visually observed at different stages of the Maillard reaction process. After being heated for 3 days, the browning index of PeC conjugate was recorded as significantly higher than PeP (p < 0.05). The browning index for PeP was recorded at 0.147, whereas the index for the conjugate was found to be 0.546. As per the previous studies, it is crucial to maintain a low browning index because a high index indicates the degradation of Amadori products, which in turn can be headed to the dissociation of the protein–polysaccharides conjugates (Chailangka et al., 2022).
3.7 Differential scanning calorimetry
The thermal transition of the PeP and PeC sample (optimized value) was investigated by DSC and analyzed thermographs are depicted in Figure 3C. The denaturation temperature (Td) of pea protein isolates was reported to be 82.1°C (Liang and Tang, 2013). As depicted in Figure 3C, the denaturation peak for pea protein conjugate was observed to be higher at 100.89°C, in contrast to the pea protein isolates which exhibited a peak at approximately 80.18°C. The denaturation temperature is directly associated with the thermal stability of proteins and reflects the breakdown of hydrogen bonds that uphold the tertiary and quaternary structures of proteins, principally tertiary ones (Ladjal-Ettoumi et al., 2016). Pea protein conjugate showed significant improvement in thermal stability through the Maillard reaction, which might be more likely due to glycosylation restricting the unfolding of the proteins by means of steric effects (Boye et al., 2010; Zhang et al., 2024).
3.8 Fourier transform infrared spectroscopy
FTIR analysis captured the structural changes during the conjugation between the pea protein and polydextrose, as shown in Figure 4A. The protein composition is characterized by the presence of peptide bonds, which contribute to the amide I, II, and III spectral regions, which are assigned to C = O stretching, N–H deformations, and C–N stretching & N-H bending vibrations, respectively (Xu et al., 2019). The FTIR graph of the PeC (optimized value) displayed a broad band in the amide A region (N-H stretching and O-H stretching) at around 3,276 cm−1. The Maillard reaction modified the absorption band within the 3,200–3,700 cm−1 range induced by the stretching vibration of OH, which was more substantial in PeC compared to PeP. A clear peak at around 2,923 cm−1 (C-H stretching) in PeC has been observed. The signature bonds of amide I, II, and III in the PeP spectrum appeared at 1658 cm−1, 1,562 cm−1, and 1,398 & 1,248 cm−1, respectively. The partially shifted bands with increased intensities were observed for amide I, II, and III in PeC’s FTIR spectra. The intensity of absorption peaks around 1,050–1,150 cm−1 represents C=O stretching and OH deformation vibration, which has increased in PeC, as can be seen in Figure 4A (Xu et al., 2019). The use of several functional groups, including NH2, especially from lysine, that may be lost during the reaction could cause quite a few modifications in the FTIR spectrum due to the chemical changes that occur during the Maillard reaction in PeC during conjugation. Other than that, the aforementioned events were attributed to the Maillard reaction, shown in several earlier publications (Xu et al., 2019). Similar results were earlier demonstrated by Zhang et al. (2022), the Maillard reaction, which induces conjugation between pea proteins and maltodextrins also causes similar spectral changes in the FTIR spectrum.
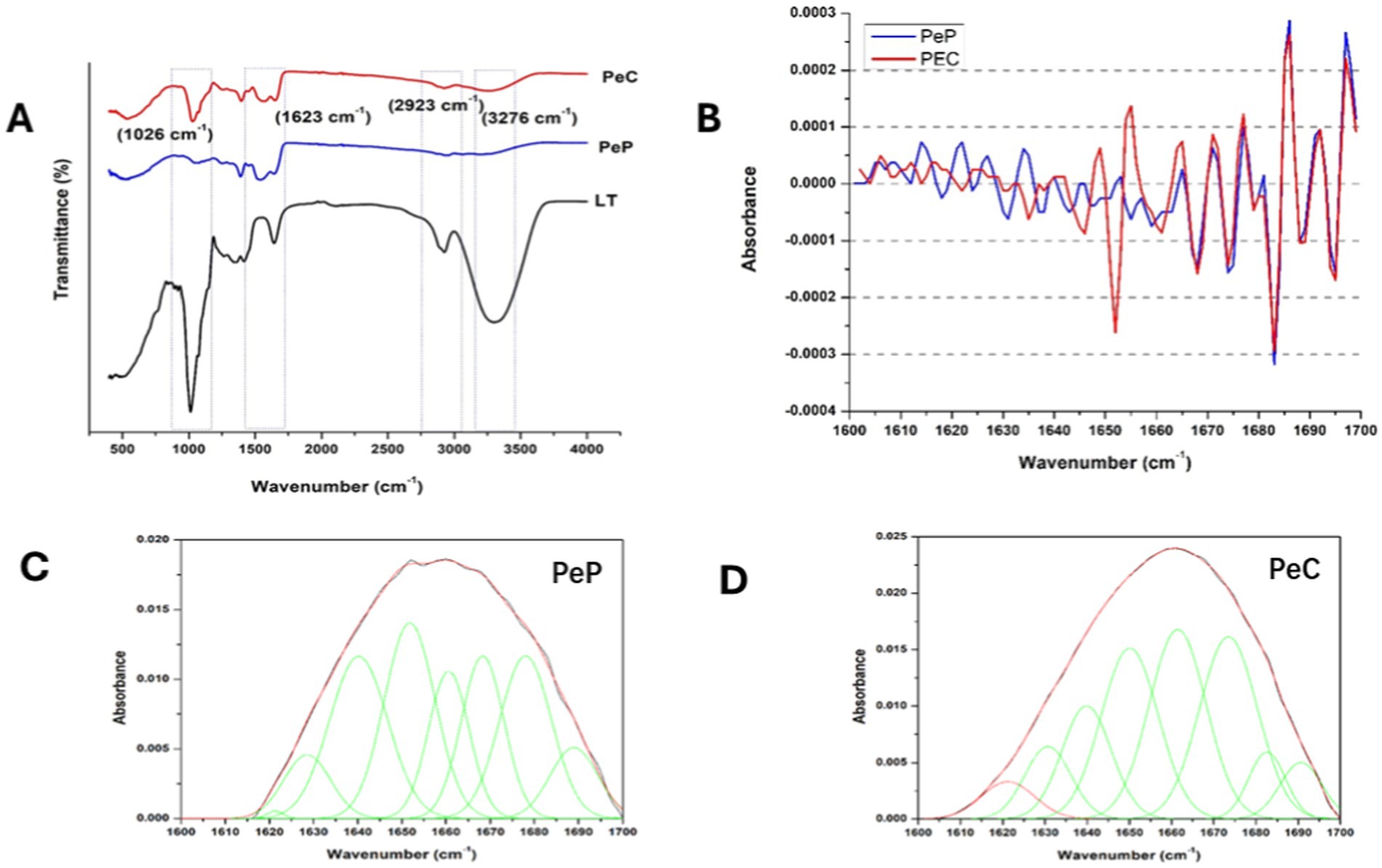
Figure 4. (A) FTIR spectrum (B) Second derivative (C,D) Curve fitted amide I region (1600–1700 cm −1) of native pea protein and its conjugates as obtained by wet heating via Maillard reaction.
Within the protein backbone, the amide I and amide II bands are the most prominent, with the amide I band (1700–1,600 cm 1) being particularly sensitive to the secondary structural components of the protein. The C=O stretch vibrations of the peptide bonds, which make up around 80% of the amide I band’s structure, are the main cause of this sensitivity. In Table 4, the relative secondary structure content of PeP is shown, indicating approximately 31.96% α-helix, 21.14% β-sheet, 32.10% β-turns, and 11.91% random coil. The pea conjugate structure differed from PeP in that it contained more unordered secondary structures, such as β-turn and random coil, and less ordered secondary structures, such as α-helix and β-sheet. This indicates that the glycosylation process can potentially affect the intermolecular forces within protein molecules, leading to changes in their overall structure (Wen et al., 2020).
3.9 Scanning Electron microscopy
SEM was used to examine the morphological features and changes in the protein, PeP, and their conjugation with polydextrose, PeC (optimized value). The changes in particle morphology during conjugation are visible in the micrographs, as seen in Figure 5. Cleary pea protein particles were seen as circular in shape but were somehow irregular in their morphology upon conjugation with polydextrose. The clear interaction of protein and prebiotic molecules was demonstrated through a dense network of protein and prebiotics. Compared to the pea protein sample (Figures 5A,B), PeC conjugate (Figures 5C,D) displayed distinct morphological characteristics and transitioned from smooth spherical particles to larger particle structures with wrinkled morphology. Zha et al. (2019), has reported similar morphological observations after the conjugation between pea protein and gum Arabic.
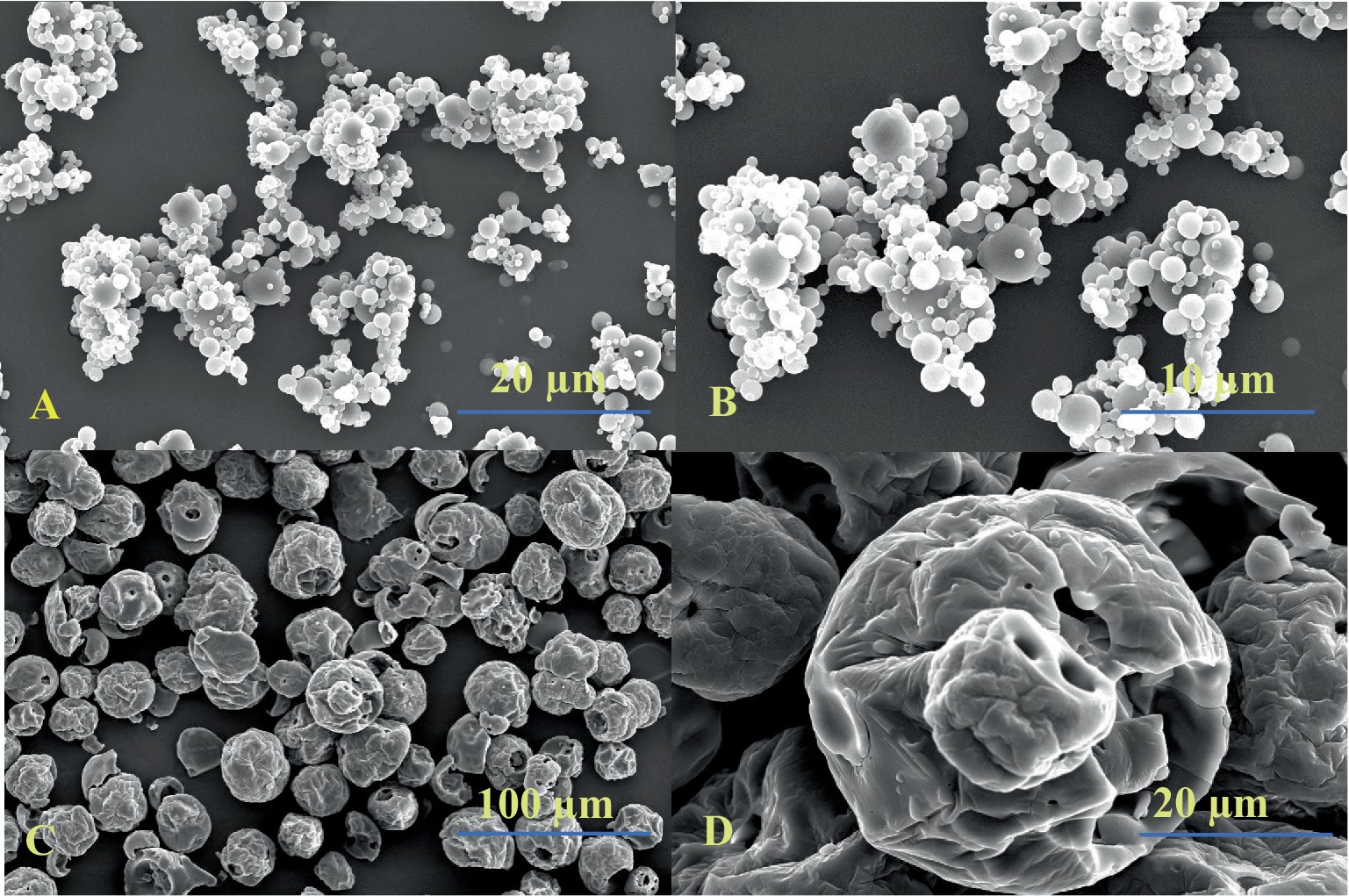
Figure 5. SEM micrographs of native pea protein (A,B) and their conjugate (C,D) as obtained by wet heating via Maillard reaction.
4 Conclusion
This study demonstrates that pea protein and polydextrose conjugates can be efficiently produced through the wet heating Maillard reaction, which is a cost-effective and straightforward approach for creating food-grade functional ingredients from underutilized plant proteins. The wet-heating conjugation process notably enhanced the functional properties of the conjugates, including solubility, emulsifying, and foaming activities, with the solid-to-solid ratio, temperature, and time identified as key factors influencing these improvements. The optimized conditions, specifically a 0.50 protein-polydextrose ratio and heating at 100°C for 1 day, yielded the maximum conjugation efficiency, foaming capacity, and emulsifying activity index. The findings of this research study contribute significantly to the area of food science by showcasing how underutilized plant proteins can be transformed into functional ingredients through controlled Maillard reactions. This advancement not only addresses the need for sustainable and plant-based food solutions but also opens avenues for further exploration in optimizing the conjugation process for various protein-prebiotic combinations. Future research could explore the application of these conjugates in different food systems, as well as their potential health benefits as functional food ingredients.
Data availability statement
The raw data supporting the conclusions of this article will be made available by the authors, without undue reservation.
Author contributions
HK: Formal analysis, Investigation, Methodology, Validation, Writing – original draft, Writing – review & editing. PM: Conceptualization, Formal analysis, Investigation, Methodology, Supervision, Visualization, Writing – original draft, Writing – review & editing. SA: Investigation, Writing – original draft. YR: Data curation, Investigation, Methodology, Writing – original draft. SM: Conceptualization, Funding acquisition, Supervision, Visualization, Writing – original draft, Writing – review & editing.
Funding
The author(s) declare that financial support was received for the research, authorship, and/or publication of this article. The authors express their appreciation to the United Arab Emirates University for funding this study through the SURE plus grant (2023) G00004484 awarded to SM.
Conflict of interest
The authors declare that the research was conducted in the absence of any commercial or financial relationships that could be construed as a potential conflict of interest.
The author(s) declared that they were an editorial board member of Frontiers, at the time of submission. This had no impact on the peer review process and the final decision.
Publisher’s note
All claims expressed in this article are solely those of the authors and do not necessarily represent those of their affiliated organizations, or those of the publisher, the editors and the reviewers. Any product that may be evaluated in this article, or claim that may be made by its manufacturer, is not guaranteed or endorsed by the publisher.
References
Al-Shamsi, K. A., Mudgil, P., Hassan, H. M., and Maqsood, S. (2018). Camel milk protein hydrolysates with improved technofunctional properties and enhanced antioxidant potential in in vitro and in food model systems. J. Dairy Sci. 101, 47–60. doi: 10.3168/jds.2017-13194
Baba, W. N., Mudgil, P., Mac Regenstein, J., and Maqsood, S. (2024). Impact of quercetin conjugation using alkaline and free radical methods with tandem ultrasonication on the functional properties of camel whey and its hydrolysates. Food Res. Int. 190:114562. doi: 10.1016/j.foodres.2024.114562
Boonlao, N., Ruktanonchai, U. R., and Anal, A. K. (2023). Glycation of soy protein isolate with maltodextrin through Maillard reaction via dry and wet treatments and compare their techno-functional properties. Polym. Bull. 80, 8603–8626. doi: 10.1007/s00289-022-04473-y
Boye, J., Aksay, S., Roufik, S., Ribéreau, S., Mondor, M., Farnworth, E., et al. (2010). Comparison of the functional properties of pea, chickpea and lentil protein concentrates processed using ultrafiltration and isoelectric precipitation techniques. Food Res. Int. 43, 537–546. doi: 10.1016/j.foodres.2009.07.021
Bryant, C., and Sanctorum, H. (2021). Alternative proteins, evolving attitudes: comparing consumer attitudes to plant-based and cultured meat in Belgium in two consecutive years. Appetite 161:105161. doi: 10.1016/j.appet.2021.105161
Capar, T. D., and Yalcin, H. (2021). Protein/polysaccharide conjugation via Maillard reactions in an aqueous media: impact of protein type, reaction time and temperature. Lwt 152:112252. doi: 10.1016/j.lwt.2021.112252
Chailangka, A., Seesuriyachan, P., Wangtueai, S., Ruksiriwanich, W., Jantanasakulwong, K., Rachtanapun, P., et al. (2022). Cricket protein conjugated with different degrees of polymerization saccharides by Maillard reaction as a novel functional ingredient. Food Chem. 395:133594. doi: 10.1016/j.foodchem.2022.133594
Chan, E., Rodas-Gonzalez, A., Tulbek, M., and Koksel, F. (2024). Effects of protein formula and extrusion cooking conditions on the techno-functional properties of texturised pea proteins. Int. J. Food Sci. Technol. 59, 584–595. doi: 10.1111/ijfs.16593
Dai, T., Yan, X., Li, Q., Li, T., Liu, C., Mcclements, D. J., et al. (2017). Characterization of binding interaction between rice glutelin and gallic acid: multi-spectroscopic analyses and computational docking simulation. Food Res. Int. 102, 274–281. doi: 10.1016/j.foodres.2017.09.020
Estell, M., Hughes, J., and Grafenauer, S. (2021). Plant protein and plant-based meat alternatives: consumer and nutrition professional attitudes and perceptions. Sustain. For. 13:1478. doi: 10.3390/su13031478
Grossmann, L., and Weiss, J. (2021). Alternative protein sources as technofunctional food ingredients. Annu. Rev. Food Sci. Technol. 12, 93–117. doi: 10.1146/annurev-food-062520-093642
Jiang, W., Zhang, Y., Julian Mcclements, D., Liu, F., and Liu, X. (2022). Impact of pea protein-inulin conjugates prepared via the Maillard reaction using a combination of ultrasound and pH-shift treatments on physical and oxidative stability of algae oil emulsions. Food Research International. 156 111161.
Karbasi, M., and Askari, G. (2021). Modification of whey protein microgel particles with mono-oligo-and polysaccharides through the Maillard reaction: effects on structural and techno-functional properties. Food Struct. 28:100184. doi: 10.1016/j.foostr.2021.100184
Ke, C., and Li, L. (2023). Influence mechanism of polysaccharides induced Maillard reaction on plant proteins structure and functional properties: a review. Carbohydr. Polym. 302:120430. doi: 10.1016/j.carbpol.2022.120430
Kumar, P., Mehta, N., Abubakar, A. A., Verma, A. K., Kaka, U., Sharma, N., et al. (2022). Potential alternatives of animal proteins for sustainability in the food sector. Food Rev. Intl. 1, 1–26. doi: 10.1080/87559129.2022.2094403
Kutzli, I., Griener, D., Gibis, M., Schmid, C., Dawid, C., Baier, S. K., et al. (2020). Influence of Maillard reaction conditions on the formation and solubility of pea protein isolate-maltodextrin conjugates in electrospun fibers. Food Hydrocoll. 101:105535. doi: 10.1016/j.foodhyd.2019.105535
Kutzli, I., Weiss, J., and Gibis, M. (2021). Glycation of plant proteins via maillard reaction: reaction chemistry, technofunctional properties, and potential food application. Food Secur. 10:376. doi: 10.3390/foods10020376
Ladjal-Ettoumi, Y., Boudries, H., Chibane, M., and Romero, A. (2016). Pea, chickpea and lentil protein isolates: physicochemical characterization and emulsifying properties. Food Biophys. 11, 43–51. doi: 10.1007/s11483-015-9411-6
Li, J., Huang, G., Qian, H., and Pi, F. (2023). Fabrication of soy protein isolate-high methoxyl pectin composite emulsions for improving the stability and bioavailability of carotenoids. Food Biosci. 53:102738. doi: 10.1016/j.fbio.2023.102738
Liang, H.-N., and Tang, C.-H. (2013). pH-dependent emulsifying properties of pea [Pisum sativum (L.)] proteins. Food Hydrocoll. 33, 309–319. doi: 10.1016/j.foodhyd.2013.04.005
Maqsood, S., Al-Dowaila, A., Mudgil, P., Kamal, H., Jobe, B., and Hassan, H. M. (2019). Comparative characterization of protein and lipid fractions from camel and cow milk, their functionality, antioxidant and antihypertensive properties upon simulated gastro-intestinal digestion. Food Chem. 279, 328–338. doi: 10.1016/j.foodchem.2018.12.011
Nakai, S. (1983). Structure-function relationships of food proteins: with an emphasis on the importance of protein hydrophobicity. J. Agric. Food Chem. 31, 676–683. doi: 10.1021/jf00118a001
Nongonierma, A. B., Cadamuro, C., Le Gouic, A., Mudgil, P., Maqsood, S., and Fitzgerald, R. J. (2019). Dipeptidyl peptidase IV (DPP-IV) inhibitory properties of a camel whey protein enriched hydrolysate preparation. Food Chem. 279, 70–79. doi: 10.1016/j.foodchem.2018.11.142
Paganini, D., Uyoga, M. A., Kortman, G. A., Cercamondi, C. I., Moretti, D., Barth-Jaeggi, T., et al. (2017). Prebiotic galacto-oligosaccharides mitigate the adverse effects of iron fortification on the gut microbiome: a randomised controlled study in Kenyan infants. Gut 66, 1956–1967. doi: 10.1136/gutjnl-2017-314418
Pearce, KN, and Kinsella, JE. (1978). Emulsifying properties of proteins: evaluation of a turbidimetric technique. Journal of agricultural and food chemistry. 26:716–23.
Saatchi, A., Kiani, H., and Labbafi, M. (2019). A new functional protein-polysaccharide conjugate based on protein concentrate from sesame processing by-products: functional and physico-chemical properties. Int. J. Biol. Macromol. 122, 659–666. doi: 10.1016/j.ijbiomac.2018.10.122
Schneider, A. A., Bu, F., and Ismail, B. P. (2023). Enhancement of pea protein solubility and thermal stability for acidic beverage applications via endogenous Maillard-induced glycation and chromatography purification. Curr. Res. Food Sci. 6:100452. doi: 10.1016/j.crfs.2023.100452
Shanthakumar, P., Klepacka, J., Bains, A., Chawla, P., Dhull, S. B., and Najda, A. (2022). The current situation of pea protein and its application in the food industry. Molecules 27:354. doi: 10.3390/molecules27165354
Sim, S. Y. J., Srv, A., Chiang, J. H., and Henry, C. J. (2021). Plant proteins for future foods: a roadmap. Food Secur. 10:1967. doi: 10.3390/foods10081967
Tiihonen, K., Suomalainen, T., Tynkkynen, S., and Rautonen, N. (2008). Effect of prebiotic supplementation on a probiotic bacteria mixture: comparison between a rat model and clinical trials. British journal of nutrition 99, 826–831.
Urango, A. C. M., Meireles, M. A. A., and Silva, E. K. (2024). Maillard conjugates produced from proteins and prebiotic dietary fibers: technological properties, health benefits and challenges. Trends Food Sci. Technol. 147:104438. doi: 10.1016/j.tifs.2024.104438
Van Den Abbeele, P., Verstraete, W., El Aidy, S., Geirnaert, A., and Van De Wiele, T. (2013). Prebiotics, faecal transplants and microbial network units to stimulate biodiversity of the human gut microbiome. Microb. Biotechnol. 6, 335–340. doi: 10.1111/1751-7915.12049
Wang, K., and Arntfield, S. D. (2017). Effect of protein-flavour binding on flavour delivery and protein functional properties: a special emphasis on plant-based proteins. Flavour Fragr. J. 32, 92–101. doi: 10.1002/ffj.3365
Wang, W.-D., Li, C., Bin, Z., Huang, Q., You, L.-J., Chen, C., et al. (2020). Physicochemical properties and bioactivity of whey protein isolate-inulin conjugates obtained by Maillard reaction. Int. J. Biol. Macromol. 150, 326–335. doi: 10.1016/j.ijbiomac.2020.02.086
Wen, C., Zhang, J., Qin, W., Gu, J., Zhang, H., Duan, Y., et al. (2020). Structure and functional properties of soy protein isolate-lentinan conjugates obtained in Maillard reaction by slit divergent ultrasonic assisted wet heating and the stability of oil-in-water emulsions. Food Chem. 331:127374. doi: 10.1016/j.foodchem.2020.127374
Xu, Z.-Z., Huang, G.-Q., Xu, T.-C., Liu, L.-N., and Xiao, J.-X. (2019). Comparative study on the Maillard reaction of chitosan oligosaccharide and glucose with soybean protein isolate. Int. J. Biol. Macromol. 131, 601–607. doi: 10.1016/j.ijbiomac.2019.03.101
Zha, F., Dong, S., Rao, J., and Chen, B. (2019). The structural modification of pea protein concentrate with gum Arabic by controlled Maillard reaction enhances its functional properties and flavor attributes. Food Hydrocoll. 92, 30–40. doi: 10.1016/j.foodhyd.2019.01.046
Zha, F., Gao, K., Rao, J., and Chen, B. (2021). Maillard-driven chemistry to tune the functionality of pea protein: structure characterization, site-specificity, and aromatic profile. Trends Food Sci. Technol. 114, 658–671. doi: 10.1016/j.tifs.2021.06.029
Zhang, Z., Wang, B., and Adhikari, B. (2022). Maillard reaction between pea protein isolate and maltodextrin via wet-heating route for emulsion stabilisation. Future Foods 6:100193. doi: 10.1016/j.fufo.2022.100193
Zhang, Y., Wu, C., Shen, X., Mcclements, D. J., Liu, X., and Liu, F. (2024). Effects of combined hot alkaline and pH-shift treatments on structure and functionality of legume protein-EGCG conjugates: soybean-, pea-, and chickpea protein-EGCG systems. Food Hydrocoll. 158:110424. doi: 10.1016/j.foodhyd.2024.110424
Zhong, S.-R., Li, M.-F., Zhang, Z.-H., Zong, M.-H., Wu, X.-L., and Lou, W.-Y. (2021). Novel antioxidative wall materials for Lactobacillus casei microencapsulation via the maillard reaction between the soy protein isolate and prebiotic oligosaccharides. J. Agric. Food Chem. 69, 13744–13753. doi: 10.1021/acs.jafc.1c02907
Keywords: pea proteins, polydextrose, conjugation, Maillard reaction, response surface methodology, techno-functional properties
Citation: Khan H, Mudgil P, Alkaabi SAS, AlRashdi YHS and Maqsood S (2024) Maillard reaction-based conjugation of pea protein and prebiotic (polydextrose): optimization, characterization, and functional properties enhancement. Front. Sustain. Food Syst. 8:1463058. doi: 10.3389/fsufs.2024.1463058
Edited by:
Anwar Ali, Hong Kong Polytechnic University, Hong Kong SAR, ChinaReviewed by:
Sipper Khan, University of Hohenheim, GermanySayantani Dutta, Universidad de Salamanca, Spain
Harsh Panwar, Guru Angad Dev Veterinary and Animal Sciences University, India
Copyright © 2024 Khan, Mudgil, Alkaabi, AlRashdi and Maqsood. This is an open-access article distributed under the terms of the Creative Commons Attribution License (CC BY). The use, distribution or reproduction in other forums is permitted, provided the original author(s) and the copyright owner(s) are credited and that the original publication in this journal is cited, in accordance with accepted academic practice. No use, distribution or reproduction is permitted which does not comply with these terms.
*Correspondence: Sajid Maqsood, c2FqaWQubUB1YWV1LmFjLmFl
†These authors have contributed equally to this work