- 1Department of Plant Production, Soil Science and Agricultural Engineering, University of Limpopo, Polokwane, South Africa
- 2Limpopo Agro-Food Technology Station, University of Limpopo, Polokwane, South Africa
Recalcitrance to root of kiwifruit (Actinidia spp.) stem cuttings (SCs) is a restriction for its commercial production. Exogenous hormone is the key factor to regulate root formation, but the research on exogenous hormone stimulatory effects on Actinidia spp. is still lagging. Kiwifruit plants had been mostly propagated by seeds. However, vegetative propagation offers several advantages over sexual propagation, among them being crop homogeneity, practicability, and simplicity of the technique. A study was therefore conducted to investigate the potential responses of kiwifruit SCs from Actinidia deliciosa rootstock and Actinidia arguta male scion SCs treated with indole-3-butyric acid (IBA) concentrations at low (10, 100 ppm), medium (1,000, 10,000 ppm) and high (100,000 ppm) levels. Parallel experiments for A. deliciosa and A. arguta’s treatments were arranged in a randomized complete block design, with 12 replications. Treatments comprised different IBA concentrations, namely, 0 (control), 10, 100, 1,000, 10,000 and 100,000 ppm. At 103 days, rooting percentage, number of roots, root length, dry root mass, size of callus formation and callus percentage were determined. In A. deliciosa SCs, treatments had high significant (p ≤ 0.05) effects in rooting percentage, number of roots, root length, size of callus formation and callus percentage, except for dry root mass. In A. arguta male SCs, treatments also, showed high significant (p ≤ 0.05) effects in the number of roots, root length and dry root mass, except for the rooting percentage. No callus formation was observed in A. arguta male SCs. Relative to the control, in A. deliciosa SCs, the highest (42%) rooting percentage and lengthy (0.3 cm) roots, both were observed at the medium (10,000 ppm) IBA concentration, whereas the most (0.29) number of roots were produced at the highest (100,000 ppm) IBA concentration. Calli percentage (94%) was highest at low (100 ppm) IBA, while the biggest (2.8) size of callus formation was at the highest IBA concentration of 100,000 ppm, when compared to the control. In A. arguta male SCs, the greatest number of roots (1.08) and root mass (0.07 g) were attained at the medium IBA concentration of 10,000 ppm. The longest (0.94 cm) root length was achieved at the low IBA concentration of 100 ppm. The SCs of A. arguta male scion required low to medium (100 to 10,000 ppm) concentrations of IBA for proper roots stimulation and development, whereas in SCs of A. deliciosa, highest rooting percentage paired with callus formation, was achieved at medium to higher (10,000 to 100,000 ppm) IBA concentrations. Principal component analysis (PCA) revealed that PC1 and PC2 accounted for 99% of the entire variation. While PC1 accounted for 94% of the total variation, PC2 accounted for 5% of the total variation in A. deliciosa. However, in A. arguta PCA revealed that PC1 and PC2 accounted for 93% of the entire variation. While PC1 accounted for 72% of the total variation, PC2 accounted for 21% of the total variation. The use of exogenous application of IBA was effective for adventitious root formation in SCs of A. deliciosa rootstock and A. arguta male scion. IBA showed its effectiveness in stimulating root development at 10000 ppm IBA.
1 Introduction
Worldwide, the genus Actinidia, with over 76 species and commonly known as kiwifruit plants, is difficult to propagate asexually by stem cuttings (SCs) without the use of plant growth regulators (PGRs) (Huang, 2016; Rana and Kumar, 2021). Propagation methods frequently used for the cultivation of kiwifruit (Actinidia spp.), included the use of cuttings, budding, grafting (Li et al., 2021; Sourati et al., 2022; Dutta et al., 2023) and sexual propagation (Sekhukhune et al., 2018). When clonal propagation is preferred, sexual propagation is avoided due to the plant’s dioecious nature as the sex of the fruit-tree is unknown until flower development stage (Arfaei et al., 2021). Although seedling rootstocks are preferred in the kiwifruit industry for various reasons (Ozcan and Erisgin, 2000), seed germination and the development of seedlings for use as rootstock material are slow and erratic (Windauer et al., 2016).
In general, kiwifruit seeds are relatively small in size and at harvest seeds are prone to embryo dormancy, with strict after-ripening attributes (Dutta et al., 2023). Embryo dormancy, also known as physiological dormancy, inhibits kiwifruit seeds from germination unless treated with gibberellic acid (GA3) or a lengthy after-ripening period for successful germination (Sekhukhune et al., 2018). Notably, kiwifruit plants are dioecious, with female and male interplants being necessary for successful cross-pollination and fruit development (Halder et al., 2019). However, the plants take approximately 7 years before sex determination or flowering, which necessitates male and female genetic study to differentiate the sex of the plants at seedling stage (Sekhukhune, 2023). Most of these challenges could be overcome through clonal propagation techniques.
In order to trap the genetic gain, propagation by SCs is the most convenient and cost effective technique of clonal regeneration for horticultural crops such as fruits, ornamentals and nuts (Davies et al., 2018). Thus, this method of propagation can be beneficial in the commercialisation of kiwifruit in meeting the significantly increasing demand for planting material. However, kiwifruit SCs are difficult to root, except when other techniques are adopted to enhance the protrusion of roots. The rooting potential of kiwifruit SCs depend on various factors such as the physiological stage of the mother plant, the season which the SCs are taken, the concentration and the type of the plant growth regulators (PGRs) used and the type of SC (hard-, semi- and soft-wood) (Thakur and Rana, 2011; Dutta et al., 2023).
A cutting is any vegetative plant part retrieved from the parent plant in order to regenerate new plants and lacking one of its physical and physiological support systems, the roots. The process of adventitious root induction is composed of several biochemical, physiological and genetic variables (El-banna et al., 2023). Adventitious root formation is the primary step for many plant species asexually propagated by SCs (Pant et al., 2022). There are two types of genesis of adventitious roots, indirect genesis, in which root primordia are produced in a callus previously formed by cells of the primary explant; and direct genesis, in which root primordia develop directly from cells of the primary explant, mainly those in close proximity to the vascular system. In both types of rhizogenesis/induction phase, a root develops from a meristemoid (Li et al., 2022). For the successful induction of adventitious roots in many difficult to root plants, exogenous PGR are widely used (Hobbie et al., 2000; Ercisli et al., 2003). Naturally, plants have the ability to produce their own endogenous PGRs. However, the concentration of endogenous PGRs within the plant changes with time, season, and during its developmental stage (Swarts et al., 2018).
The improvement of the rooting ability and the development of quality root systems through the use of PGRs such as auxins is well documented in several species (Verdi et al., 2020; Bettoni et al., 2023; Loconsole et al., 2022), including the Actinidia species (Ercisli et al., 2003). The PGR indole-3-acetic acid (IAA) is a key regulator in the initiation and proliferation of adventitious roots. Its exogenous application consistently affects the development of roots (Ilczuk and Jacygrad, 2016). Based on their effectiveness in stimulating adventitious roots, IAA and indole-3-butyric acid (IBA) have been utilized commercially to enhance rooting of cuttings in many plant species (Hobbie et al., 2000; Ercisli et al., 2003), with Actinidia species included (Ercisli et al., 2003). In terms of stability, IBA was found to be more stable than IAA and persists longer in the plant tissues (da Costa et al., 2013). Other synthetic auxins commonly used include α-naphthaleneacetic acid (NAA), which is another important auxin suitable for the rooting of cuttings, and most plant species respond positively to its action (Kaewchangwat et al., 2020; Saeed et al., 2021).
In other Actinidia species studies, inconsistent results were reported due to the use of PGRs, which included a complete lack of response (Ercisli et al., 2002; Ono et al., 2000; Erturk et al., 2010) due to unexplained factors. Ercisli et al. (2002) reported that the used 2000 ppm IBA was inefficient for rooting SCs of A. deliciosa kiwifruit cultivar. Similarly, Erturk et al. (2010) also observed poor rooting performance when A. deliciosa SCs were treated with 2000 ppm IBA. Working with A. chinensis kiwifruit, Ono et al. (2000) reported poor rooting ability in SCs treated with 3,000 ppm IBA. Based on their (Ercisli et al., 2002; Ono et al., 2000; Erturk et al., 2010) results, among other factors, the authors hypothesize that the PGR-concentrations used were too low for the stimulation, initiation and development of quality roots in the genus Actinidia. Therefore, the objective of this study was to investigate the potential responses of semi-hardwood SCs of A. deliciosa rootstock and A. arguta male scion plant treated at concentrations straddling low, medium, and high PGR(s).
2 Materials and methods
2.1 Description of the study site
Parallel experiments for A. deliciosa rootstock and A. arguta male scion semi-hardwood SCs were conducted at the Politsi, Westfalia Nursery (23°65′36” S, 30°21′67″ E), Tzaneen, Limpopo Province of South Africa (SA), under greenhouse conditions from winter (May–July) to spring (August–October), 2021. The temperature at the nursery location varies between 25°C (minimum night temperature) and 27°C (maximum day temperature) during summer (November–January) under natural photoperiod (12/12 h, day/night), with annual rainfall averaging 881 mm. Irrigation in the greenhouse comprised a mist irrigation system, installed to supply water in the form of mist at every 8 min for 10–15 s on a hot day, which could be reduced on cool days.
2.2 Preparation of plant material
Semi-hardwood SCs measuring 10–16 cm long, 0.1–0.5 cm diameter, with 3 − 4 nodal buds were excised at sunrise from 1-year-old canes developed from 14-years-old mature A. deliciosa rootstock and A. arguta male scion dormant plants using sterile secateurs. For sterilization process, secateurs were surface sterilized for 5 min in 70% commercial bleach (JIK®) solution containing 2.45% active sodium hypochlorite (NaOCl) and rinsed a few times with distilled water (dH2O), followed by 70% ethanol for 1 min prior to excising each SC to maintain sterility and avoiding contamination. The 14-years-old mature A. deliciosa rootstock and A. arguta male scion kiwifruit plants under commercial production are cultivated under field conditions at Nooyenskopje farm situated at Magoebaskloof (23°53′13” S, 29°56′13″ E), Tzaneen, in Limpopo Province of SA. The excised SCs were classified as A. deliciosa rootstock and A. arguta male scion, and each cultivar were placed in 200 L plastic beakers filled with dH2O to avoid dehydration and transported to the University of Limpopo. Upon arrival, all the SCs were removed from the plastic beaker, wrapped with a wet paper towels, and then sealed in zip-lock transparent plastic bags and stored in a refrigerator for a 10-day-cold stratification pre-treatment period prior to planting.
2.3 Preparation of plant growth regulators
Indole-3-butyric acid was acquired from Sigma-Aldrich (Merck Life Science Pty Ltd., SA). The required stock solutions were prepared according to Atak and Yalçın (2015). To prepare a 1 mg/mL stock solution, a 100 mg of IBA was added to a 100 mL volumetric flask. Thereafter, a 3–5 mL of 70% ethanol was added to the volumetric flask to dissolve the PGR powder. Once completely dissolved, the solution was brought to volume with dH2O through continuous stirring to keep the material in solution. Thereafter, the stock solution was used as required.
2.4 Treatment of stem cuttings and planting
After cold stratification period, the SCs from A. deliciosa rootstock and A. arguta male scion plants were disinfected at the base (5 cm) using 25% commercial bleach (JIK®) solution that contained 0.875% active NaOCl with 2 drops of Tween-20 for 10–15 min. The disinfected SCs were later rinsed 3 times with dH2O to remove traces of the sterilant solution. Prior to PGR-SCs treatment, the apical region were slant-cut toward the last apical nodal bud, whereas the basal part of the SCs were cut-horizontally just below the last bottom nodal bud, as described by Davies et al. (2018), to obtain SCs of 2–3 nodal buds. The basal 5 cm portion of all the tested Actinidia species SCs were immersed for 5 s in the prepared IBA concentrations (0–100,000 ppm). The treated SCs were then placed in a shade for few minutes to ensure sufficient absorption of root growth regulator before planting in a 24-hole-seedling trays (200 SQ), filled with a mixture of pine bark, vermiculite and perlite at a 2:1:1 (v/v) ratio to a depth of 5 cm. The cuttings in the control were treated with dH2O. Seedling trays with SCs were then irrigated to field capacity and later placed on a suspended mesh-wire to avoid interference of roots with the ground and minimize contamination. The SCs were maintained under natural photoperiods for 103 days. The glasshouse day and night ambient temperatures averaged 26°C and 16°C, respectively, with maximum temperatures controlled by opening the roof of the glasshouse. Relative humidity was maintained between 70 − 80%. 2 weeks after the SCs had established, each tray was fertilized with Multifeed P Efekto® fertilizer (Nulandies, Johannesburg, SA) to provide a total of 0.70 mg N, 0.64 mg K, 0.64 mg P, 1.21 mg Mg, 1.5 mg Fe, 0.15 mg Cu, 0.7 mg Zn, 2 mg B, 6 mg Mn and 0.14 mg Mo per ml water.
2.5 Treatments and research design
The treatments in each experiment comprised IBA at concentrations of 0, 10, 100, 1,000, 10,000 and 100,000 ppm, where zero (0) ppm constituted the untreated SCs (control). In both the A. deliciosa rootstock and A. arguta male scion SCs, treatments were arranged in a randomized complete block design (RCBD), with 12 replications (n = 72). Blocking was done for shading by the greenhouse walls in the morning. Each treatment contained 4 SCs of each tested plant species.
2.6 Data collection
After 103 days, plant variables, namely, rooting percentage, number of roots, root length (cm), dry root mass (g), size of callus formation and percentage, were assessed. Survived SCs from both experiments were gently uprooted from the seedling trays without damaging the roots, then rinsed under low running tap water to remove excess media and blotted dry with a paper towel. The number of roots was manually counted and the root length (cm) was measured using a meter stick. Thereafter, roots were severed from the SCs and fresh root mass (g) was determined. Measured roots were then oven-dried at 90C until a constant mass is reached, then the dry mass (g) of roots was determined using an analytical balance (Sartorius). Size of callus formation was assessed as follows: 1 = none, 2 = low, 3 = medium, 4 = extensive, 5 = very extensive.
The rooting and callus percentage were calculated using the formula by Atak and Yalçın (2015):
Rooting percentage
Callus percentage
2.7 Data analysis
Prior to analysis of variance (ANOVA) of the data using SAS software (SAS Institute Inc, 2010), treatments (0, 10, 100, 1,000, 10,000 and 100,000 ppm IBA) in both A. deliciosa and A. arguta experiments were expressed as exponentials [100, 101, 102, 103, 104, 105 and 106 (%)] and transformed using log1010x, where log1010x = 1 (Gomez, 2012), resulting in x-axis of 0, 1, 2, 3, 4, 5 and 6 (Causton, 1977). Transformation was done to homogenize the interval between the concentrations. Significant (p ≤ 0.05) treatment means were separated using Fisher’s Least Significant Difference test and then subjected to lines of the best fit. Generally, when the variables versus increasing treatment levels exhibited positive quadratic relations, the relationships were modeled through the regression curve estimates from the quadratic equation (Y = b2x2 + b1x + c) (Mamphiswana et al., 2010) to derive the optimum values using the relation x = −b1/2b2 (Gomez, 2012). Unless otherwise stated, results were discussed at the probability level of 5%. The IBA concentrations and the root growth variables of Actinidia plant species were analyzed through the Principal Component Analysis.
(PCA).
3 Results
The established kiwifruit SCs from A. deliciosa rootstock and A. arguta male scion plants, developed well and produced roots, some with calli depending on the applied IBA (ppm).
3.1 Actinidia deliciosa rootstock stem cuttings performance
Plant growth regulator IBA concentrations had high significant (p ≤ 0.05) effects on rooting percentage, number of roots and root length (cm), except for dry root mass (g), which was not significant (p ≥ 0.05).
Treatments contributed 26, 28, 20 and 11% to the total treatment variation (TTV) in rooting percentage, number of roots, root length (cm) and dry root mass (g), respectively (Table 1). Significant effects were also observed on calli percentage and size in A. deliciosa rootstock SCs. The treatments contributed 29 and 5% to the TTV in calli percentage and size, respectively (Table 2).
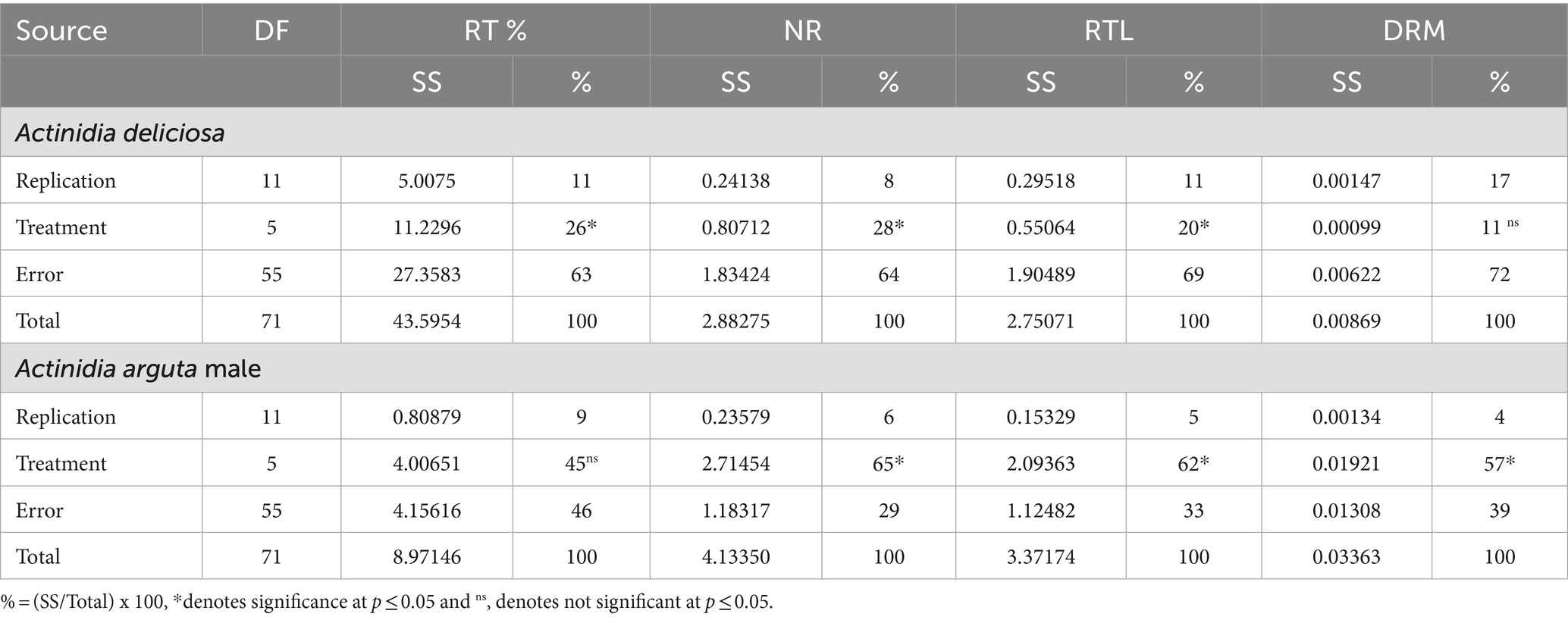
Table 1. Mean sum of squares for rooting percentage (RT%), number of formed roots (NR), root length (RTL) and dry root mass (DRM) stem cuttings of Actinidia deliciosa rootstock plants and Actinidia arguta male scion plants.
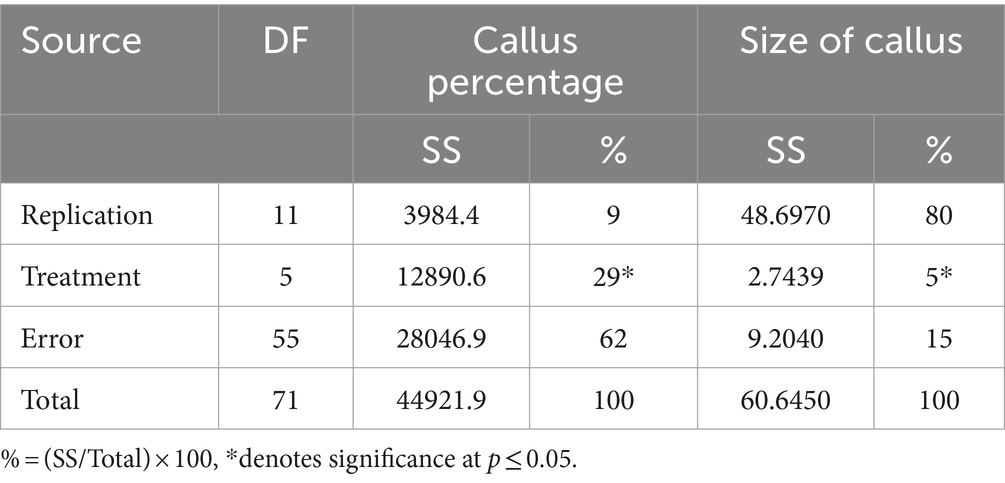
Table 2. Mean sum of squares for callus percentage and size of callus formation of stem cuttings of Actinidia deliciosa rootstock plants to IBA concentrations in vivo.
Relative to the untreated control (0 ppm IBA), IBA concentrations of 10, 100, 1,000, 10,000 and 100,000 ppm, significantly increased rooting percentage by 10, 6, 23, 42 and 31%, respectively, but the effects of the respective IBA at a medium concentration of 1,000 and 10,000 ppm as well as at a higher concentration of 100,000 ppm did not differ from each other (Table 3). Similarly, treatments at low concentration of 10, 100 and 0 ppm did not differ significantly from each other. Highest rooting percentage (42%) was achieved at the medium concentration of 10,000 ppm IBA (Figures 1A, 2E).
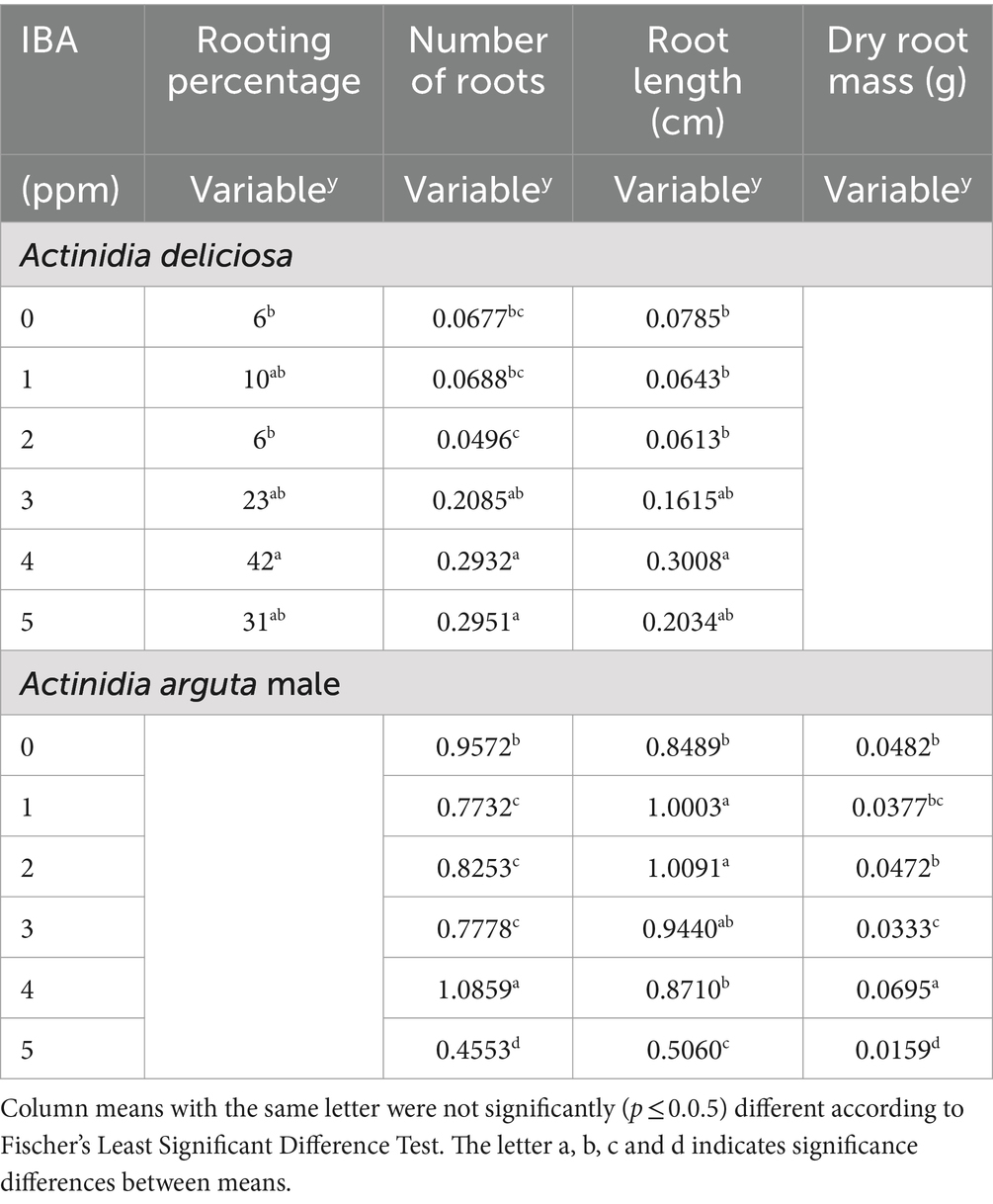
Table 3. Responses of rooting percentage, number of formed roots, root length and dry root mass of stem cuttings of Actinidia deliciosa rootstock plants and Actinidia arguta male plants to IBA concentrations.
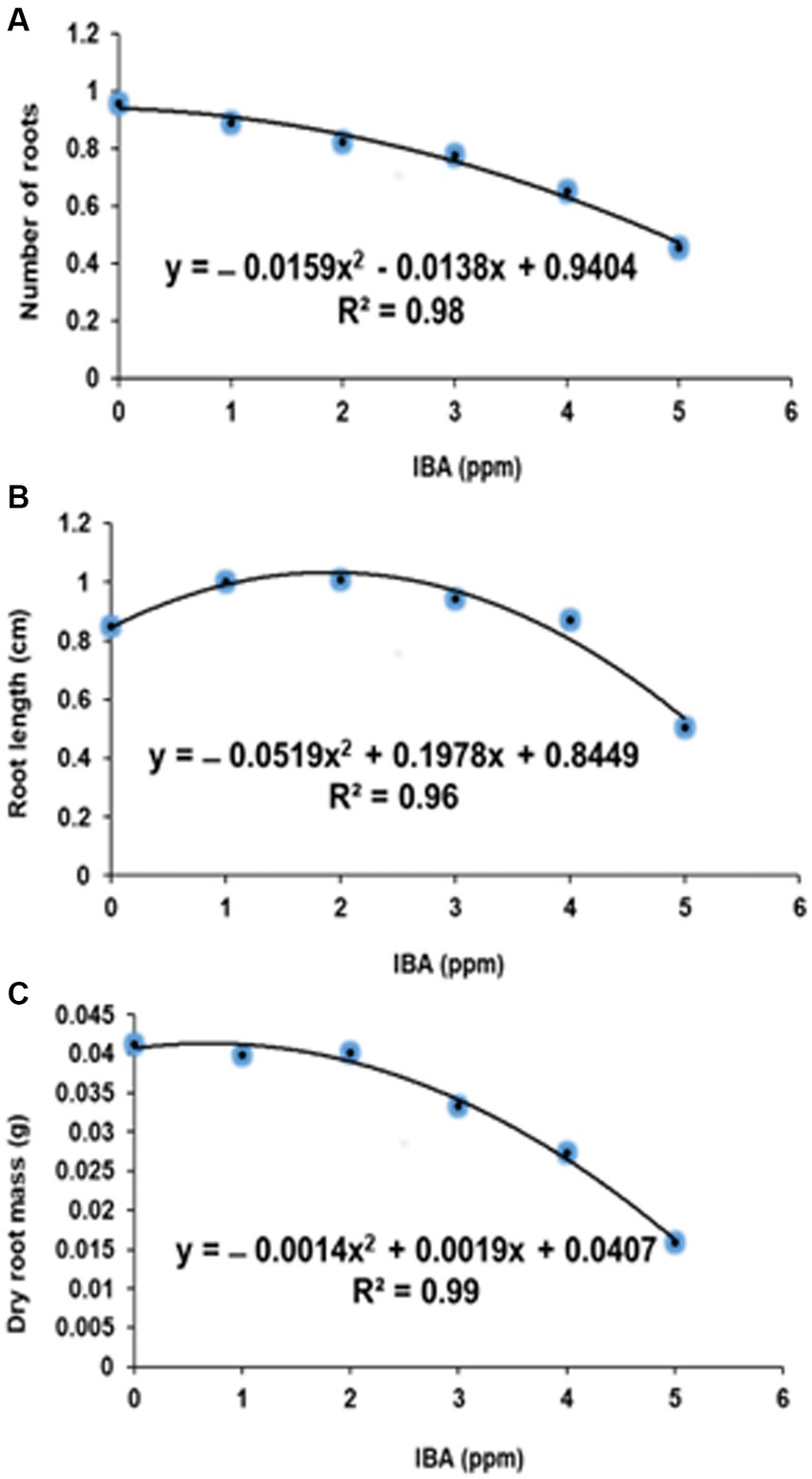
Figure 1. Quadratic relationship in rooting percentage (A), number of roots (B) and root length (C) against IBA treated stem cuttings of Actinidia deliciosa.
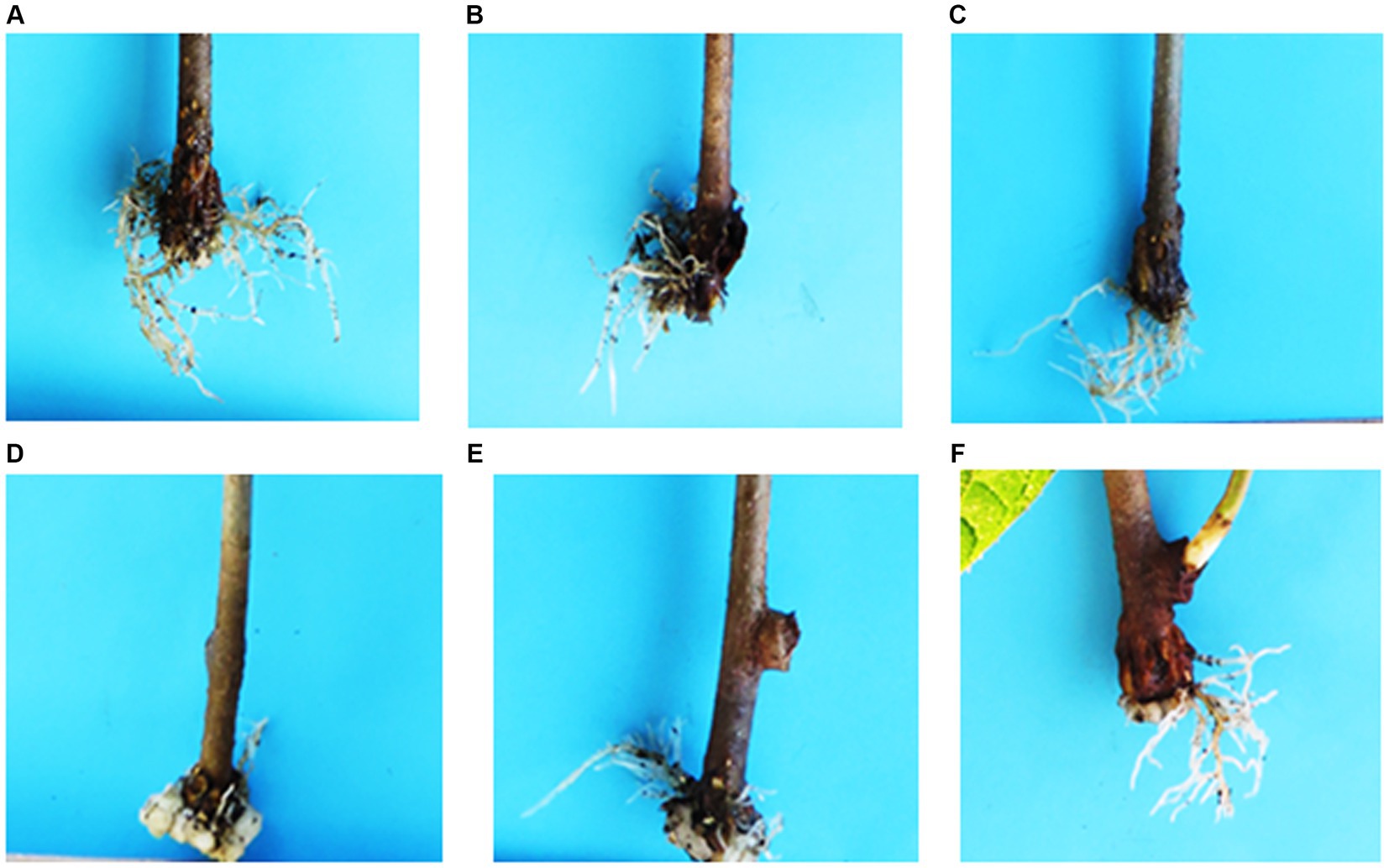
Figure 2. Representation of roots formation in Actinidia deliciosa stems cuttings in response to different IBA concentrations: (A) 0 ppm; (B) 10 ppm; (C) 100 ppm; (D) 1,000 ppm; (E) 10,000 ppm and (F) 100,000 ppm.
The number of roots increased by 0.208, 0.293 and 0.295%, at 1000, 10000 and 100,000 ppm IBA concentrations, respectively, when compared to the number of roots (0.067) at the control (0 ppm IBA).
However, the effects of the respective IBA at a medium concentration of 1,000 and 10,000 ppm as well as at a higher concentration of 100,000 ppm did not differ from each other (Table 3). At the lowest concentration of 10 ppm IBA, a slight (0.068) increase in the number of roots was also observed.
Contrarily, the number of roots had a reduction (0.049) at 100 ppm (Table 3). Again, at the lower concentrations of 10, 100 and 0 ppm, treatments did not differ from each other. Treatment of 100,000 ppm IBA resulted in the most number of roots (0.295) produced (Table 3).
When compared to the control (0 ppm IBA), at the lower IBA of 10 and 100 ppm, root length (cm) of A. deliciosa was the lowest by 0.064 and 0.061 cm, respectively (Table 3). At medium (1,000 ppm) and highest (10,000 and 100,000 ppm) IBA, longer root length of 0.161, 0.301 and 0.203 cm, respectively, when compared to control (0 ppm IBA) (Table 3). The longest root length (0.301 cm) was measured at the medium IBA of 10,000 ppm (Figures 1C, 2E).
The callus percentage ranged from 58 to 94% depending on the IBA concentrations applied (Table 4).
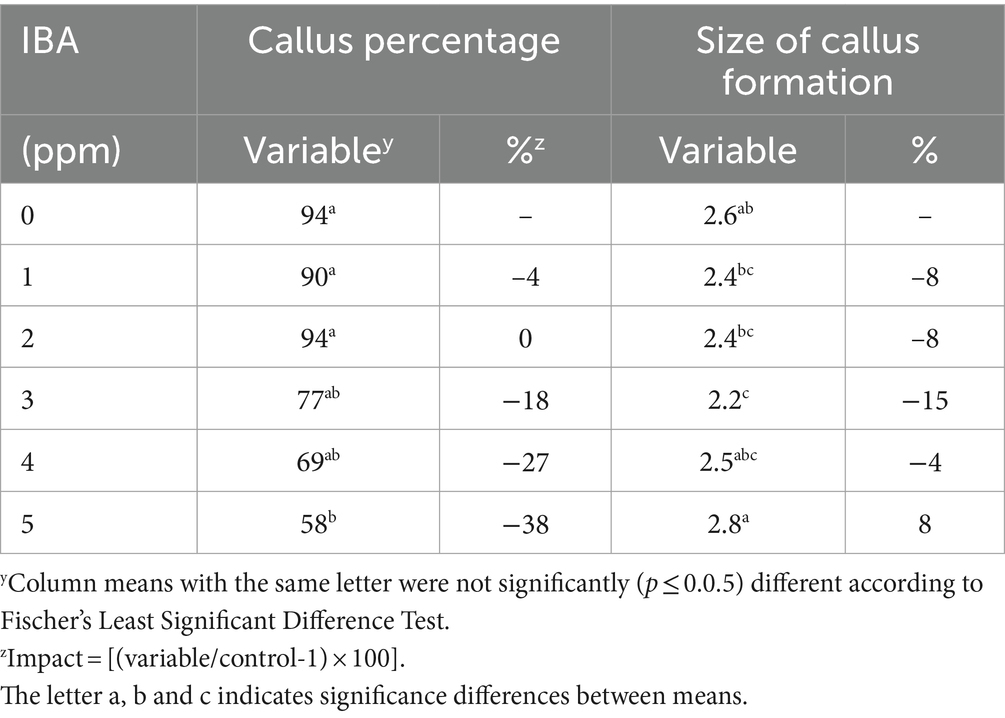
Table 4. Responses of callus percentage and size of callus formation of stem cuttings of Actinidia deliciosa rootstock plants to IBA concentrations in vivo.
The highest calli percentage (94%) was observed at a low IBA concentration of 100 ppm, but it was not different from calli percentage at 10 ppm (90%), 1,000 ppm (77%) and 10,000 ppm (69%) of IBA, when compared to the control (Table 4). The lowest calli (58%) percentage was observed at the highest
IBA concentration of 100,000 ppm (Table 4; Figure 3).
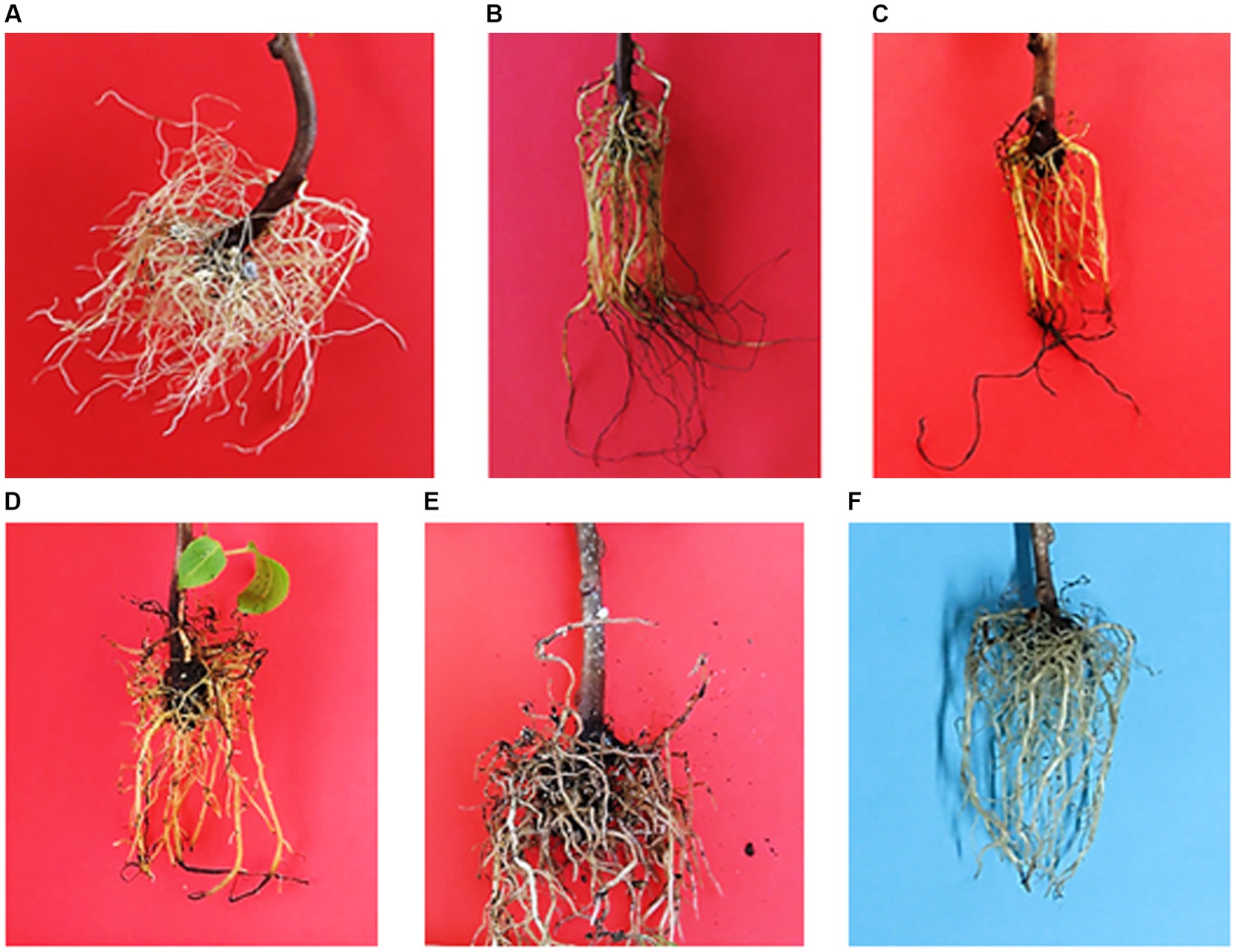
Figure 3. Representation of callus formation of stem cuttings of Actinidia deliciosa rootstock plants to IBA concentrations: (A) 0 ppm; (B) 10 ppm; (C) 100 ppm; (D) 1,000 ppm; (E) 10,000 ppm and (F) 100,000 ppm.
In terms of the size of callus formed, the medium-sized (2.8) calli was observed at the highest IBA concentration of 100,000 ppm, but it was not different from calli (2.5) at 10000 ppm as well as the control (Table 4). The lowest-sized (2.2) calli was observed at the IBA concentration of 1,000 ppm (Table 4). However, there were no differences observed in the sizes of calli formation at treatments 10 (2.4), 100 (2.4) and 10,000 (2.5) ppm IBA (Figure 2). Relative to the control (0 ppm IBA), the callus formation decreased by (2.4), (2.4), (2.2) and (2.5) at IBA treatments of 10, 100, 1,000 and 10,000 ppm, which was followed by an increase of (2.8) at the highest IBA concentration of 100,000 ppm, respectively (Figure 3).
Principal Component Analysis revealed that PC1 and PC2 accounted for 99% of the entire variation. While PC1 accounted for 94% of the total variation, PC2 accounted for 5% of the total variation. The contribution of IBA concentrations and root growth variables to the PCA indicated that rooting percentage were dominant in SCs treated with medium (1,000 and 10,000 ppm) IBA concentrations. Similarly, number of roots, root length (cm) and dry root mass (g) were dominant in SCs treated with medium (1,000 and 10,000 ppm) IBA concentrations. In contrast, the results indicated that callus percentage was dominant in SCs treated at the control (0 ppm) and those treated with low (10 and 100 ppm) IBA concentrations (Figure 4). Within PCA1, rooting percentage, number of roots, root length (cm) and dry root mass (g) showed positive correlation whereas callus percentage showed a negative correlation. Within PCA2, number of roots, showed a positive correlation. Whereas rooting percentage, root length (cm) and dry root mass (g) showed a negative correlation of PCA2 (Figure 4).
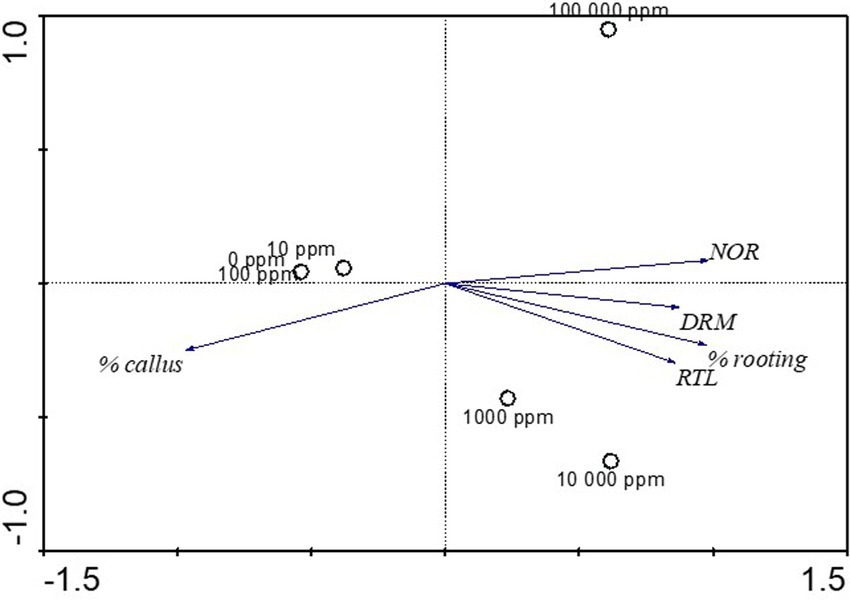
Figure 4. Biplot of Principal Component Analysis (PCA) of the IBA concentrations on root growth variables in Actinidia deliciosa rootstock stem cuttings.
3.2 Actinidia arguta male stem cuttings performances
High significant (p ≤ 0.05) effects were observed on number of roots, root length (cm), and dry root mass (g), except for rooting percentage. The treatments contributed 45, 65, 62, and 57% to the TTV in rooting percentage, number of roots, root length (cm), and dry root mass (g), respectively (Table 1).
The number of roots recorded 0.773, 0.825, 0.778, and 0.455 at 10, 100, 1,000 and 100,000 ppm IBA, respectively, when compared to the control. However, the effects of the respective IBA concentration at low (10 and 100 ppm) and medium (1,000 ppm) did not differ from each other (Table 3). In contrast, the number of roots (1.086) had an increase at medium concentration of 10,000 ppm IBA (Table 3; Figures 5A, 6E). Thus, treating SCs with 10,000 ppm IBA resulted in the more number of roots produced (Figures 5A, 6).
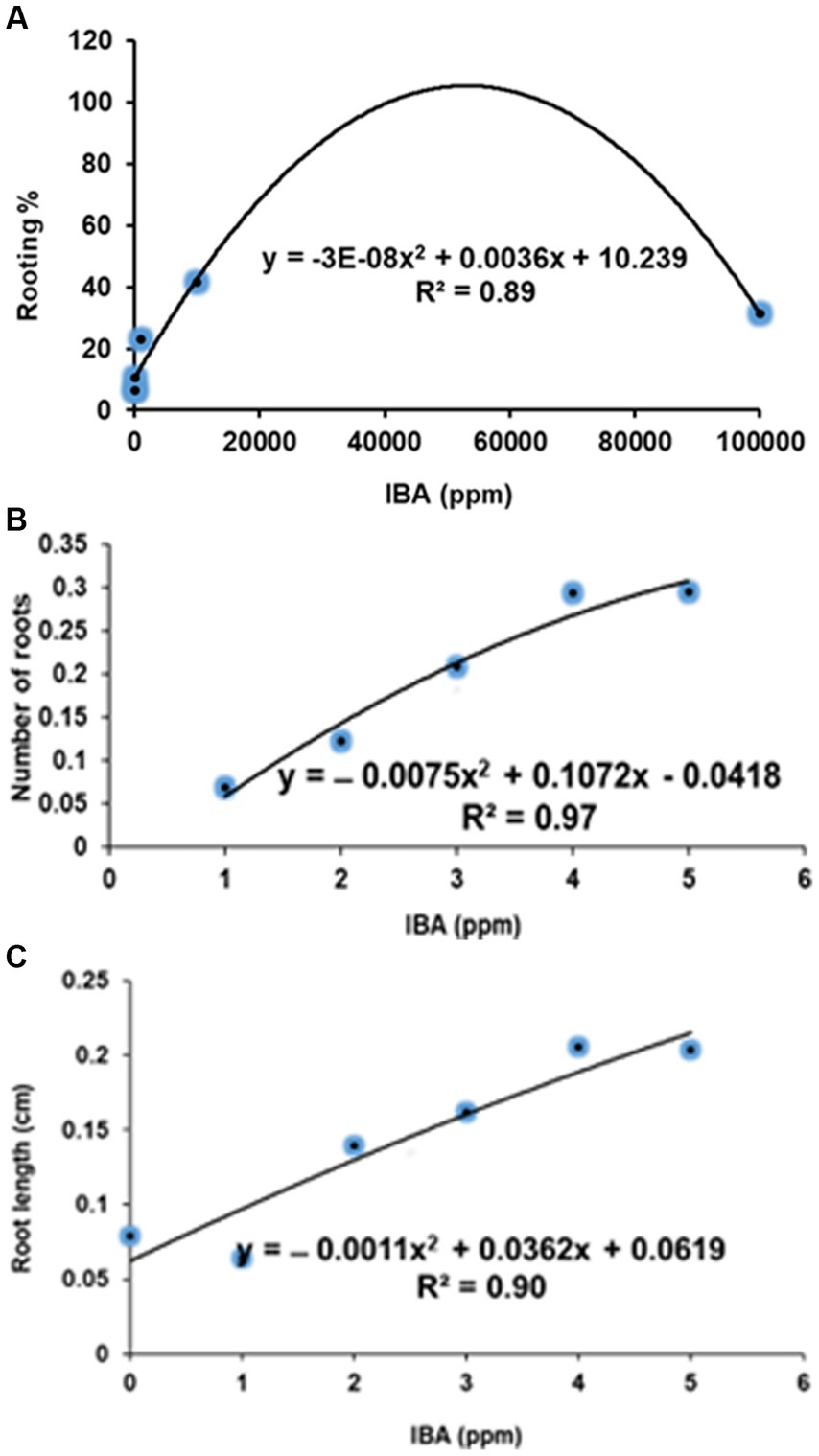
Figure 5. Quadratic relationship in number of roots (A), root length (B) and dry root mass (C) against IBA treated stem cuttings of Actinidia arguta male.
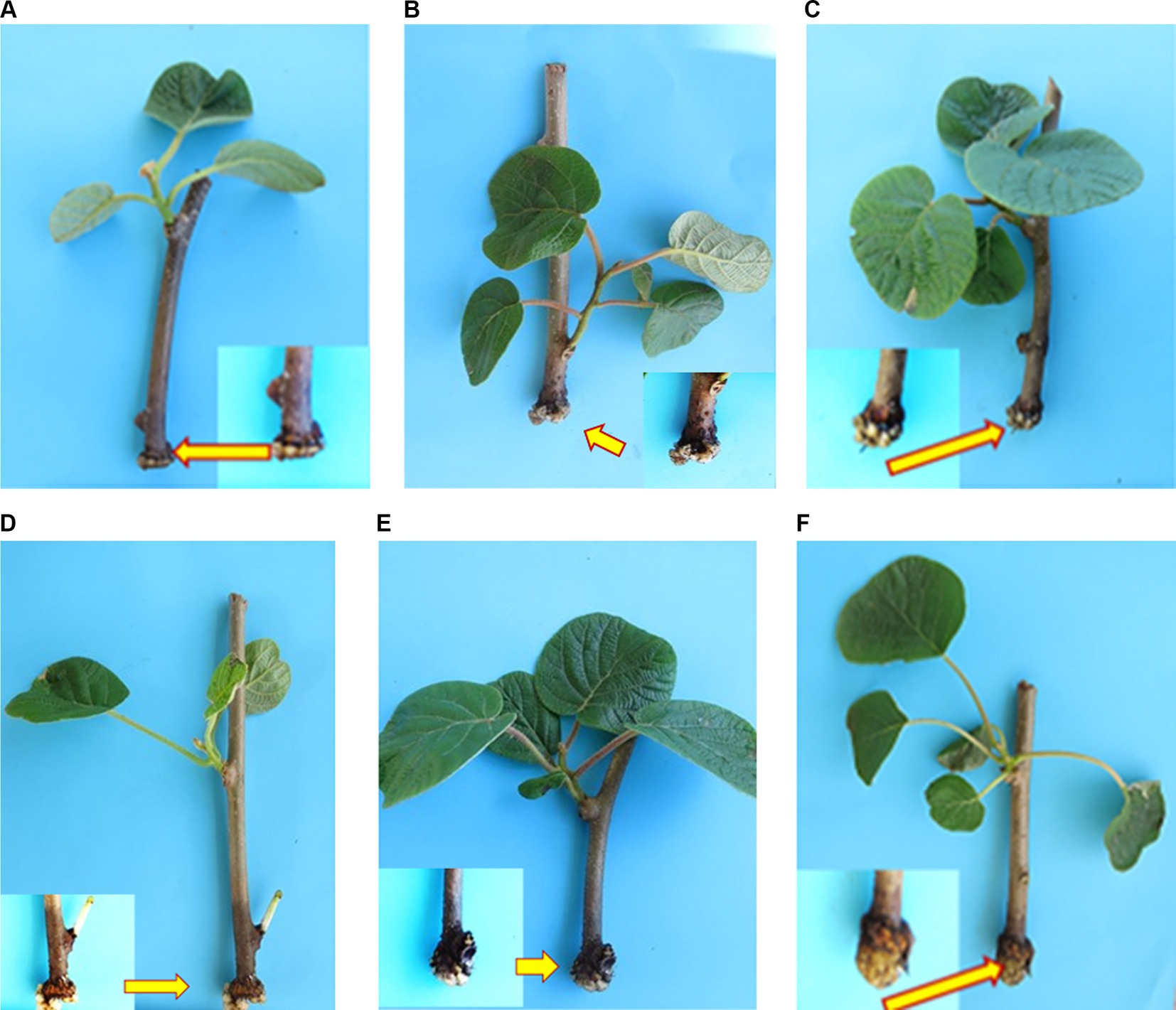
Figure 6. Representation of roots formation in response to different IBA concentrations: (A) 0 ppm; (B)10 ppm; (C) 100 ppm; (D) 1,000 ppm; (E) 10,000 ppm and (F) 100,000 ppm in Actinidia arguta male stems cuttings.
Contrarily to the number of roots produced, the IBA of 10, 100, 1,000 and 10,000 ppm, significantly increased root length (cm) by 1.000, 1.009, 0.944 and 0.871 cm, respectively, when compared to the control. However, the effects of the respective IBA at low (10 and 100 ppm) and medium (1,000 ppm) concentrations did not differ from each other (Table 3). In contrast, the root length (0.506 cm) was reduced at the highest 100,000 ppm IBA (Table 3). The longest root length (1.009 cm) was measured at the low (100 ppm) IBA concentration (Figures 5B, 6C).
The dry root mass (g) reduced by 0.038, 0.047, 0.033 and 0.016 g, when SCs were treated with concentrations of 10, 100, 1,000 and 100,000 ppm IBA, respectively. However, there were no differences among the respective IBA treatments at low (10 ppm) and medium (1,000 ppm) concentrations (Table 3). Similarly, treatments at low 10 and 100 ppm did not differ significantly from each other (Table 3), as well as treatments at 10 and 1,000 ppm IBA (Table 3). In contrast, the dry root mass (0.871 g) achieved the highest increase (0.016) at 10000 ppm IBA (Figure 6E).
The PCA revealed that PC1 and PC2 accounted for 93% of the entire variation. While PC1 accounted for 72% of the total variation, PC2 accounted for 21% of the total variation. The contribution of IBA concentrations and root growth variables to the PCA indicated rooting percentage were dominant in SCs treated at the low (10 ppm) and medium (1,000 ppm) IBA concentrations. Whereas number of roots, root length (cm) and dry root mass (g) were dominant in SCs treated with medium (10,000 ppm) IBA concentrations (Figure 7). Within PCA1, rooting percentage, number of roots, root length (cm) and dry root mass (g) showed a negative correlation, whereas within PCA2, number of roots, root length (cm) and dry root mass (g) showed a negative correlation (Figure 7). Within PCA2, rooting percentage showed a positive correlation (Figure 7).
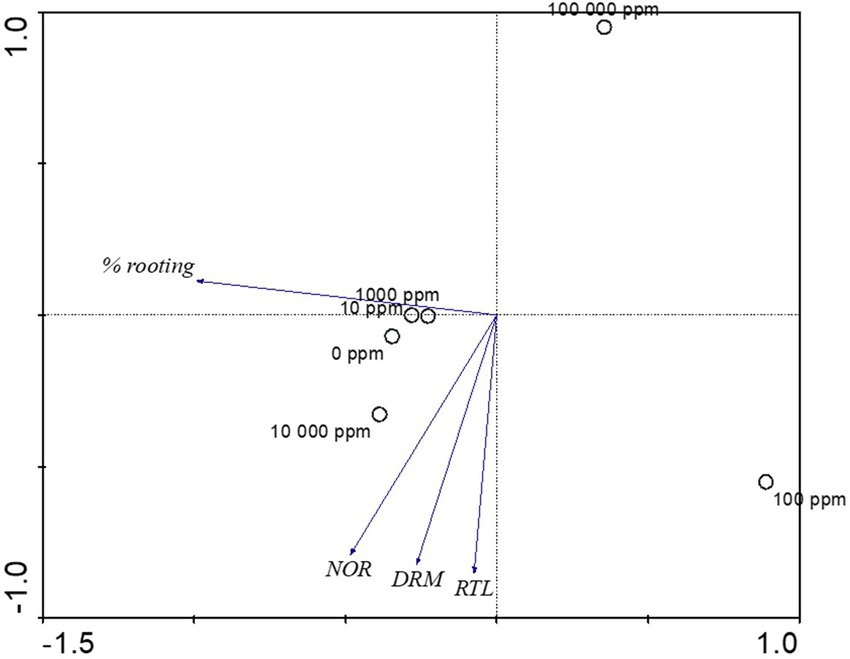
Figure 7. Biplot Principal Component Analysis (PCA) of the IBA concentrations on root growth variables in Actinidia arguta male scion stem cuttings.
4 Discussion
The findings obtained in this study demonstrated that the use of IBA treatment was beneficial for root induction in SCs of the 2 tested Actinidia spp. The effectiveness of IBA in SCs of A. deliciosa rootstock and A. arguta male scion plants, were also confirmed in findings of other kiwifruit studies (Ercisli et al., 2002; Alam et al., 2007; Peticilă et al., 2016; Zenginbal and Özcan, 2014; Peticilă et al., 2016). In this study, IBA showed its effectiveness on the below ground parameters measured, namely, rooting percentage, number of roots, root length (cm), dry root mass (g), callus percentage and size of callus depending on the tested kiwifruit plant species. In A. deliciosa SCs, significant effects were observed in all measured parameters (rooting percentage, number of roots and root length (cm), callus percentage and size of formation) except for dry root mass. In A. arguta male scion SCs, non-significant effects were shown in the rooting percentage only. No regenerative response in terms of callus formation were observed in A. arguta SCs.
The IBA treatment showed significant influence on the size of calli formation and percentage in SCs of A. deliciosa. The biggest sized-calli (2.8) was observed at highest (100,000 ppm) IBA, whereas at 100 ppm IBA, highest calli percentages (94%) was recorded. Actinidia deliciosa SCs are difficult to develop normal roots without the existence of calli formation. This was evident in the present study that SCs of A. deliciosa rootstocks, developed highest (94%) calli percentages also on the untreated SCs (0 ppm IBA). Similarly, Miri-nargesi and Sedaghathoor (2015) observed (100%) calli percentages at IBA concentration of 4,000 ppm in A. deliciosa. On the other hand, A. arguta male SCs showed better rooting response without callus development. Generally, the adventitious root formation in SCs form through dormant pre-formed root initials (Gao et al., 2023). The formation of callus as a thin layer around the basal cut end of a SCs is a consequence of wound healing and appears to stop or delay the root initiation (Davies et al., 2018). The callus formation is essential to heal and regenerate the basal cut surface of SCs to stimulate cell division and raise the contents of auxin, carbohydrate, and ethylene as a result of increased rate of respiration (Smith, 2013). Cuttings, particularly of hardwood plants, commonly grow callus followed by root formation at the physiological base of the cutting. Atak and Yalçın (2015) reported that better rooting response is achievable under conditions that do not favor callus formation, probably because dense callus have the tendency of hindering normal root emergence leading to rooting failure. These are greatly encouraged by the application of auxins such as IBA (Davies et al., 2018).
In A. deliciosa, rooting percentage increased with IBA concentrations, and the highest (42%) was evident at a medium concentration of 10,000 ppm IBA. Several authors had documented the effectiveness of IBA in rooting of kiwifruit SCs. Ercisli et al. (2003) reported the best rooting percentage (40 and 42%) in SCs of A. deliciosa ‘Hayward’ when treated with higher concentrations of 4,000 and 6,000 ppm, respectively. On the other hand, Ali et al. (2017) obtained the highest rooting percentage (56.94%) at the IBA of 3,500 ppm also in A. deliciosa SCs. Atak and Yalçın (2015) attained highest rooting percentage (37.9%) at the IBA of 5,000 ppm on A. deliciosa SCs. The observed rooting percentage in A. deliciosa SCs could be due to stimulatory effects of exogenous auxins stimulating adventitious root production in these SCs (Arya and Husen, 2022). Indole-3-butyric acid plays a significant role in mobilizing reserved food material, elongation of meristematic cells and differentiation of cambial initials into root primordial (Pandey and Husen, 2022). The physiological nature of the SCs seems also to have an influence on the rooting potential. Haissig (1979) stated that depending on the endogenous level of growth regulating substance in SCs, exogenous application of auxin IBA may be promotive, ineffective or even inhibitory for the rooting of the SCs. Therefore, the concentration of IBA selected to improve plant growth should depend on the plant species, rate of uptake and transport to target tissue, existing natural levels of auxins within the plant, sensitivity to auxins, metabolic rate, and the interaction between hormones within the plant (George et al., 2008).
The highest number of roots produced (0.295) in A. deliciosa was achieved at medium concentration of 10,000 ppm IBA. Similarly, in A. arguta SCs, the greatest number of roots (1.086) produced were at 10000 ppm IBA. The application of IBA was reported to increase the number of roots per A. arguta SCs (Beyl et al., 1995). Ali et al. (2017) observed that 3,500 ppm of IBA was effective to produce the highest number of roots (5.91) in A. deliciosa. Atak and Yalçın (2015) obtained the highest number of roots (4.9) at IBA concentration of 5,000 ppm in A. deliciosa. Application of exogenous or endogenous auxins bring about initiation of adventitious roots in the stem and the division of first root initial cells are dependent on either exogenously applied or endogenous native auxins (Pop et al., 2011). Hence, exogenous application of auxins supplements the endogenous levels of the auxins leading to enhanced rooting. The increase in the number of roots might be ascribed to auxin’s action, which triggered carbohydrate and nitrogenous material breakdown and translocation at the base of cuttings, resulting in faster cell elongation and cell division in a favorable environment. The higher number of roots formed with IBA may also be explained by differences in compartmentation of the PGR in the cells. Indole-3-butyric acids may be located in cell compartments that are more responsive to auxin as a result causing a high number of roots. Indole-3-butyric acid has been reported to improve root number (Davies et al., 2018).
The highest root length (0.301 cm) was obtained at 10000 ppm IBA in A. deliciosa SCs. Ali et al. (2017) observed that 3,500 ppm IBA induced the longest root (9.35 cm) in A. deliciosa. Atak and Yalçın (2015) attained the longest root (4.3 cm) at IBA concentration of 5,000 ppm also in A. deliciosa. Miri-nargesi and Sedaghathoor (2015) further reported that the longest root (2.01 cm) measured in A. deliciosa was related to IBA concentration of 4,000 ppm. The increase in length of root may be due to the successful rooting of IBA treated SCs (Rana and Jindal, 2001). Alam et al. (2007) reported that IBA promoted cell elongation which helped in increase in root length. Indole-3-butyric acid affects the positioning of the root outgrowth site and promotes root hair elongation (Honkanen and Dolan, 2016). Other factors such as plant species, growing media and number of nodes on SCs could lead to rooting success. Davies et al. (2018), highlighted that the length of the roots increased due to the ability of the roots to absorb water and nutrients in sufficient quantities. Hence, the increase in root length could also be due to the allocation of more nutrients for root formation.
The highest dry root mass (0.069 g) was obtained at medium concentration of 10,000 ppm in A. arguta male SCs. Similarly, Sekhukhune (2018) observed that A. arguta SCs treated with high concentrations of 10,000 ppm IBA, increased dry root mass by 0.38 g, respectively. Indole-3-butyric acid when applied in higher concentration resulted in higher dry root mass (Moalemi, 2001). The increase in root mass is due to more and longer roots (Alam et al., 2007). The IBA stimulation on initiation, growth and development of the adventitious roots depend on improving the translocation and movement of sugars to the base of SCs, and motivation of DNA, RNA and protein synthesis that are necessary for cell division (Haissig, 1979). The supplementation of IBA is to support the coupling between endogenous IAA and amino acids, which results in the synthesis of the specific proteins essential for the formation of root initiation (Ryugo and Breen, 1974; Van der Krieken et al., 1992). The effect of IBA applications during adventitious root formation may be due to modification of endogenous auxins, which play a significant role in the regulation of root development (El-banna et al., 2023). Endogenous auxins are produced from the axillary buds and transported basipetal to the base of SCs to stimulate root formation (Davies et al., 2018). Adventitious root formation is tightly controlled by the metabolism of endogenous auxins. IAA promotes root formation, while Abscisic acid (ABA) inhibits the root formation.
According to PCA, dominant root performance occurred at the medium (1,000 and 10,000 ppm) IBA concentrations in A. deliciosa rootstock. In A. arguta male scion SCs, dominant root performance occurred at the low (10 ppm) and medium (1,000 and 10,000 ppm) IBA concentrations. Rooting at low (10 and 100 ppm) concentrations of IBA may be due to induction stage of adventitious roots formation. At this stage, the cells become competent and committed to form the root primordia under the influence of IBA. Auxin IBA induces the root and helps in the establishment of carbohydrate sink that further stimulates the adventitious roots. During this phase, cytokinins show inhibitory effect (De Klerk et al., 1999). Spethmann (1997) reported that the initial weeks are crucial for root initiation and subsequent development after sticking of cuttings and endogenous biosynthesis of IAA is considered as a responsible factor in root development. At medium IBA concentrations (1,000 and 10,000 ppm) meristemoids cells started differentiating into adventitious root primordia followed by their growth and subsequent protrusion from the basal surface of the stem disks resulting in maximum root performance. There is a strong correlation between IBA content and root growth variables. The increased IBA was associated with the more development in the measured root growth variables in both plant species. However, at the highest (100,000 ppm) concentration of IBA, there was an evidence of inhibition of root growth variables due to the phytotoxic effect of the IBA. These are exposed to increasing levels of biotic or abiotic factors, responding to the pressures through density-dependent growth (DDG) patterns (Salisbury and Ross, 1992). The DDG patterns in both species (Figures 1, 5) straddled three phases, namely, stimulation, neutral and inhibition phases (Mashela and Pofu, 2017). Stimulation occurred when the concentration of IBA in root variables operated within the positive linear relation and the treatments were significant, whereas the neutral phase occurred when the concentration of IBA in root variables operated within a neutral range (no relation) and the treatments were not significant. On the other hand, inhibition phases are showing a negative linear relation with treatments characterized by having significant effects (Mashela and Pofu, 2017). As a result, the IBA concentration used in this study demonstrated the existence of the 3 phases of the DDG pattern.
5 Conclusion
It is concluded that exogenous IBA showed its effectiveness in stimulating root growth and development in both A. deliciosa and A. arguta SCs. In A. arguta, root formation was the best at 10000 ppm IBA, with no callus formation. At concentrations higher than 10,000 ppm, inhibition was evident. In A. deliciosa SCs root stimulation and development was affected due to the development of calli at the lowest IBA concentration of 10 ppm. However, the rooting percentage was dominant at 10000 ppm IBA. The findings showed that IBA at medium (10,000 ppm) concentrations is suitable for rooting in the 2 tested kiwifruit species and it is recommended for use by kiwifruit growers. Future studies should be conducted on callus management protocols and effective rooting in A. deliciosa SCs.
Data availability statement
The original contributions presented in the study are included in the article/supplementary material, further inquiries can be directed to the corresponding author.
Author contributions
MS: Writing – review & editing, Writing – original draft, Visualization, Validation, Project administration, Methodology, Investigation, Formal analysis, Data curation, Conceptualization. MM: Writing – review & editing, Supervision, Project administration.
Funding
The author(s) declare that financial support was received for the research, authorship, and/or publication of this article. The work was funded by the National Research Foundation (NRF), grant numbers (130747).
Acknowledgments
The authors are greatly indebted to the Department of Biodiversity, University of Limpopo and the Politsi nursery for the use of their facilities, as well as Nooyenskopjie farm for providing study plant material.
Conflict of interest
The authors declare that the research was conducted in the absence of any commercial or financial relationships that could be construed as a potential conflict of interest.
Publisher’s note
All claims expressed in this article are solely those of the authors and do not necessarily represent those of their affiliated organizations, or those of the publisher, the editors and the reviewers. Any product that may be evaluated in this article, or claim that may be made by its manufacturer, is not guaranteed or endorsed by the publisher.
Abbreviations
dH2O, Distilled water; DDG, Density-dependent growth; PGRs, Plant growth regulators; SCs, Stem cuttings.
References
Alam, R., Rahman, K. U., Ilyas, M., Ibrahim, M., and Rauf, M. A. (2007). Effect of indole butyric acid concentrations on the rooting of kiwi cuttings. Sarhad J. Agri 23, 293–295.
Ali, M., Iqbal, U., Mushtaq, R., Dar, S., Baba, T., Javid, R., et al. (2017). Effect of IBA on rooting of kiwi fruit cuttings under zero energy polyhouse. Veg Int. J. Plant Res. 30:165. doi: 10.5958/2229-4473.2017.00052.0
Arfaei, L., Alizadeh, M., and Zarei, H. (2021). Kiwifruit (actinidia deliciosa) propagation: influence of stock plant sexuality on root development. Int. J. Minor Fruits Med. Aroma. Plants 7, 68–72. doi: 10.53552/ijmfmap.2021.v07ii02.006
Arya, A., and Husen, A. (2022). Role of various auxins in adventitious root formation. Environ. Physiol. Chem Contr Adven Root. Cut., 213–238. doi: 10.1016/b978-0-323-90636-4.00012-x
Atak, A., and Yalçın, T. (2015). Effects of different applications on rooting of Actinidia Deliciosa “Hayward” hardwood and softwood cuttings. Acta Hortic. 117, 117–125. doi: 10.17660/actahortic.2015.1096.10
Bettoni, J. C., van der Walt, K., Souza, J. A., McLachlan, A., and Nadarajan, J. (2023). Sexual and asexual propagation of syzygium maire, a critically endangered Myrtaceae species of New Zealand. N. Z. J. Bot. 62, 35–52. doi: 10.1080/0028825x.2022.2158110
Beyl, C. A., Ghale, G., and Zhang, L. (1995). Characteristics of hardwood cuttings influence rooting of Actinidia arguta (Siebold & Zucc.) planch. HortScience 30, 973–976. doi: 10.21273/hortsci.30.5.973
da Costa, C. T., Ruedell, C. M., Fett-Neto, A. G., de Almeida, M. R., and Maraschin, J. S. F. (2013). When stress and development go hand in hand: Main hormonal controls of adventitious rooting in cuttings. Front. Plant Sci. 4. doi: 10.3389/fpls.2013.00133
Davies, F. T., Geneve, R. L., Wilson, S. B., Kester, D. E., and Hartmann, H. T. (2018). Hartmann & Kester’s plant propagation: Principles and practices. New York: Pearson.
De Klerk, G. J., Van Der Krieken, W., and De Jong, J. C. (1999). The formation of adventitious roots: new concepts, new possibilities. In Vitro Cell Dev. Biol. Plant 35, 189–199. doi: 10.1007/s11627-999-0076-z
Dutta, S. K., Layek, J., Yadav, A., Das, S. K., Rymbai, H., Mandal, S., et al. (2023). Improvement of rooting and growth in kiwifruit (actinidia deliciosa) cuttings with organic biostimulants. Heliyon 9:e17815. doi: 10.1016/j.heliyon.2023.e17815
El-Banna, M. F., Farag, N. B. B., Massoud, H. Y., and Kasem, M. M. (2023). Exogenous iba stimulated adventitious root formation of Zanthoxylum beecheyanum K. Koch stem cutting: Histo-physiological and Phytohormonal investigation. Plant Physiol. Biochem. 197:107639. doi: 10.1016/j.plaphy.2023.107639
Ercisli, S., Anapali, Ö., Esitken, A., and Sahin, Ü. (2002). The effects of IBA, rooting media and cutting collection time on rooting of kiwifruit. Eur. J. Hortic. Sci. 67, 34–38.
Ercisli, S., Esitken, A., Cangi, R., and Sahin, F. (2003). Adventitious root formation of kiwifruit in relation to sampling date, IBA and Agrobacterium rubi inoculation. J. Plant Growth Regul. 41, 133–137. doi: 10.1023/A:1027307720934
Erturk, Y., Ercisli, S., Haznedar, A., and Cakmakci, R. (2010). Effects of plant growth promoting rhizobacteria (PGPR) on rooting and root growth of kiwifruit (Actinidia deliciosa) stem cuttings. Biol. Res. 43, 91–98. doi: 10.4067/S0716-97602010000100011
Gao, L., Liu, J., Liao, L., Gao, A., Njuguna, B. N., Zhao, C., et al. (2023). Callus induction and adventitious root regeneration of cotyledon explants in peach trees. Horticulturae 9:850. doi: 10.3390/horticulturae9080850
George, E. F., Hall, M. A., and De Klerk, G. J. (2008). Plant propagation by tissue culture, vol. 1. Dordrecht: Springer.
Haissig, B. E. (1979). Influence of aryl esters of indole‐3‐acetic and indole‐3‐butyric acids on adventitious root primordium initiation and development. Physiologia. Plantarum. 47, 29–33. doi: 10.1111/j.1399-3054.1979.tb06507.x
Halder, S., Ghosh, S., Khan, R., Khan, A. A., Perween, T., and Hasan, M. A. (2019). Role of pollination in fruit crops: a review. Pharma Innov. J. 8, 695–702.
Hobbie, L., McGovern, M., Hurwitz, L. R., Pierro, A., Liu, N. Y., Bandyopadhyay, A., et al. (2000). The axr6 mutants of arabidopsis thaliana define a gene involved in auxin response and early development. Development 127, 23–32. doi: 10.1242/dev.127.1.23
Honkanen, S., and Dolan, L. (2016). Growth regulation in tip-growing cells that develop on the epidermis. Curr. Opin. Plant Biol. 34, 77–83. doi: 10.1016/j.pbi.2016.10.006
Huang, H. (ed.). (2016). “Domestication and commercialization of Actinidia,” in Kiwifruit, (Academic Press) 191–210.
Ilczuk, A., and Jacygrad, E. (2016). The effect of IBA on anatomical changes and antioxidant enzyme activity during the in vitro rooting of smoke tree (Cotinus Coggygria Scop.). Sci. Hortic. 210, 268–276. doi: 10.1016/j.scienta.2016.07.036
Kaewchangwat, N., Thanayupong, E., Jarussophon, S., Niamnont, N., Yata, T., Prateepchinda, S., et al. (2020). Coumarin-caged compounds of 1-naphthaleneacetic acid as light-responsive controlled-release plant root stimulators. J. Agric. Food Chem. 68, 6268–6279. doi: 10.1021/acs.jafc.0c00138
Li, D., Han, F., Liu, X., Lv, H., Li, L., Tian, H., et al. (2021). Localized graft incompatibility in kiwifruit: analysis of homografts and heterografts with different rootstock & scion combinations. Sci. Hortic. 283:110080. doi: 10.1016/j.scienta.2021.110080
Li, K., Tian, H., Mao, J., Khan, A., Tahir, M. M., Li, S., et al. (2022). Effect of darkness treatment on the morphology, hormone status and gene expression of developing adventitious root in apple rootstock. Plant Cell, Tissue and Organ Culture (PCTOC) 148, 331–346. doi: 10.1007/s11240-021-02192-2
Loconsole, D., Cristiano, G., and De Lucia, B. (2022). Image analysis of adventitious root quality in wild sage and glossy abelia cuttings after application of different indole-3-butyric acid concentrations. Plan. Theory 11:290. doi: 10.3390/plants11030290
Mamphiswana, N. D., Mashela, P. W., and Mdee, L. K. (2010). Distribution of total phenolics and antioxidant activity in fruit, leaf, stem and root of Monsonia burkeana. Afr. J. Agric. Res. 5, 2570–2575.
Mashela, P. W., and Pofu, K. M. (2017). Influence of cucurbitacin-containing phytonematicides on selected nutrient elements in leaf tissues of green bean under greenhouse conditions. Acta Agri. Scand. Sec. B Soil Plant Sci. 67, 743–747. doi: 10.1080/09064710.2017.1343379
Miri-nargesi, S., and Sedaghathoor, S. (2015). Study on rooting of kiwifruit cultivars (Actinidia chinensis) in different substrates and rooting hormones. J. Horti. Biol. Environ. 6, 33–42.
Moalemi, N. (2001). Effect of IBA on rooting of Duranta repens cuttings under tunnel plastic conditions. Mahallat, Iran: First Symposium of Ornamentals and Flowers of Iran, 112.
Ono, E. O., Rodrigues, J. D., and Pinho, S. Z. (2000). Studies on stem cuttings of kiwi (Actinidia chinensis pl. CV Bruno). Braz. Arch. Biol. Technol. 43. doi: 10.1590/s1516-89132000000100007
Ozcan, M., and Erisgin, E. (2000). The effects of some application on seed germination and seedling growth in kiwifruit. Bull. Pure App. Sci. 19, 25–31.
Pandey, S., and Husen, A. (2022). Role of plant stem or shoot cutting positions and hormone treatments in adventitious root formation. Environ. Physiol. Chem. Cont. Adven. Root. Cut., 367–379. doi: 10.1016/b978-0-323-90636-4.00009-x
Pant, M., Gautam, A., Chaudhary, S., Singh, A., and Husen, A. (2022). Adventitious root formation in ornamental and horticulture plants. Environ. Physiol. Chem. Cont. Adven. Root. Cutt., 455–469. doi: 10.1016/b978-0-323-90636-4.00006-4
Peticilă, A. G., Madjar, R. M., Scaeteanu, G. V., and Asanica, A. (2016). Effect of rooting hormone treatments on propagation of Actinidia sp. by hardwood cuttings. AgroLife Sci. J. 5, 112–118.
Pop, T. I., Pamfil, D., and Bellini, C. (2011). Auxin control in the formation of adventitious roots. Notulae Botanicae Horti Agrobotanici Cluj-Napoca 39:307. doi: 10.15835/nbha3916101
Rana, H. S., and Jindal, K. K. (2001). Effect of type of cutting and auxin treatment on the rooting of cuttings of some commercial kiwifruit cultivars. Haryana J. Horti. Sci 30, 3–5.
Rana, V. S., and Kumar, G. (2021). “Kiwifruit,” Temp. Fruits, eds. L. Phavaphutanon, U. Wermund, and R. Cronje, Debashis (Apple Academic Press) 417–448.
Ryugo, K., and Breen, P. J. (1974). Indole acetic acid metaholism in cuttings of plum (Prunus cerasifera x Prunus munsoniana cv. Mariana 2624). J. Am. Soc. Hortic. Sci. 99, 247–251. doi: 10.21273/JASHS.99.3.247
Saeed, Q., Xiukang, W., Haider, F. U., Kučerik, J., Mumtaz, M. Z., Holatko, J., et al. (2021). Rhizosphere bacteria in plant growth promotion, biocontrol, and bioremediation of contaminated sites: a comprehensive review of effects and mechanisms. Int. J. Mol. Sci. 22:10529. doi: 10.3390/ijms221910529
Salisbury, F. B., and Ross, C. W. (1992). Plant physiology. Inc, California: Wadsworth Publishing Co.
SAS Institute Inc (2010). SAS/STAT® 9.2 qualification tools user’s guide. Cary (NC): South Africa: SAS Institute Inc.
Sekhukhune, M. K. (2018). In vivo and in vitro propagation of Actinidia arguta and Actinidia chinensis : University of Limpopo.
Sekhukhune, M. K. (2023). Mass propagation of Actinidia species for use as rootstock, female and male scion, gender segregation of plantlets using molecular techniques and nematode status [PhD thesis]. South Africa: University of Limpopo.
Sekhukhune, M. K., Maila, M. Y., Nikolova, R. V., and Mphosi, M. S. (2018). Preliminary studies on in vivo germination of actinidia arguta and actinidia chinensis. Acta Hortic. 123, 123–132. doi: 10.17660/actahortic.2018.1204.16
Sourati, R., Sharifi, P., Poorghasemi, M., Alves Vieira, E., Seidavi, A., Anjum, N. A., et al. (2022). Effects of naphthaleneacetic acid, indole-3-butyric acid and zinc sulfate on the rooting and growth of mulberry cuttings. Int. J. Plant Biol. 13, 245–256. doi: 10.3390/ijpb13030021
Swarts, A., Matsiliza-Mlathi, B., and Kleynhans, R. (2018). Rooting and survival of Lobostemon fruticosus (l) H. Buek stem cuttings as affected by season, media and cutting position. S. Afr. J. Bot. 119, 80–85. doi: 10.1016/j.sajb.2018.08.019
Thakur, P., and Rana, V. (2011). Effect of pre-conditioning treatments, IBA and collection time on the rooting of semi-hardwood cuttings of kiwifruit, Actinidia delicosa Chev. Int. J. Farm Sci. 1, 30–36.
Van der Krieken, W. M., Breteler, H., and Visser, M. H. (1992). The effect of the conversion of indolebutyric acid into indoleacetic acid on root formation on microcuttings of Malus. Plant Cell Physiol. 33, 709–713. doi: 10.1093/oxfordjournals.pcp.a078309
Verdi, R., Bettoni, J. C., Werner, S. S., Boff, M. I., and Boff, P. (2020). Effects of the phenological stage, type of cutting and plant growth regulators on the propagation by stem cutting of poiretia latifolia Vogel, a Brazilian native medicinal plant. Revista Colombiana de Ciencias Hortícolas 14, 267–274. doi: 10.17584/rcch.2020v14i2.10917
Windauer, L. B., Insausti, P., Biganzoli, F., Benech-Arnold, R., and Izaguirre, M. M. (2016). Dormancy and germination responses of kiwifruit (actinidia deliciosa) seeds to environmental cues. Seed Sci. Res. 26, 342–350. doi: 10.1017/s0960258516000192
Keywords: Actinidia arguta, Actinidia deliciosa, in vivo, rooting hormone, rooting media, vegetative propagation
Citation: Sekhukhune MK and Maila MY (2024) Exogenous IBA stimulatory effects on root formation of Actinidia deliciosa rootstock and Actinidia arguta male scion stem cuttings. Front. Sustain. Food Syst. 8:1461871. doi: 10.3389/fsufs.2024.1461871
Edited by:
Naeem Khan, University of Florida, United StatesReviewed by:
Leo Rufato, Santa Catarina State University, BrazilSagar Datir, Naoroji Godrej Centre for Plant Research (NGCPR), India
Copyright © 2024 Sekhukhune and Maila. This is an open-access article distributed under the terms of the Creative Commons Attribution License (CC BY). The use, distribution or reproduction in other forums is permitted, provided the original author(s) and the copyright owner(s) are credited and that the original publication in this journal is cited, in accordance with accepted academic practice. No use, distribution or reproduction is permitted which does not comply with these terms.
*Correspondence: Mapogo Kgetjepe Sekhukhune, bWFwb2dvLnNla2h1a2h1bmVAdWwuYWMuemE=