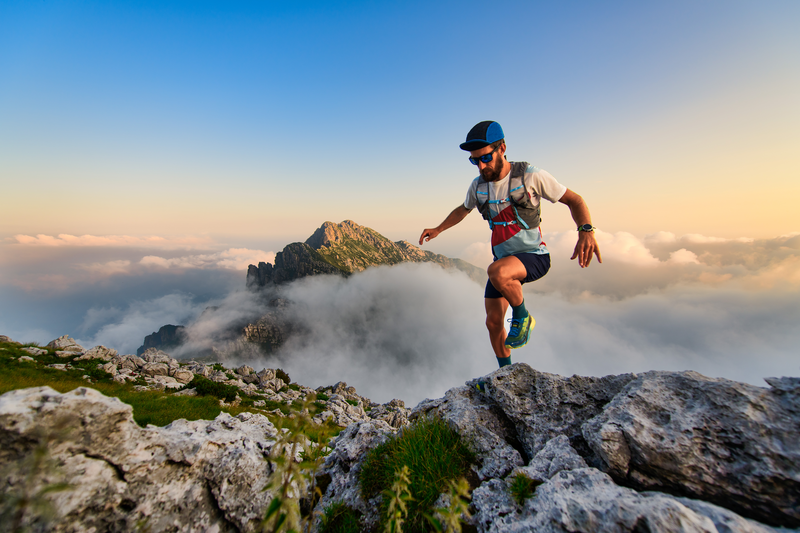
94% of researchers rate our articles as excellent or good
Learn more about the work of our research integrity team to safeguard the quality of each article we publish.
Find out more
ORIGINAL RESEARCH article
Front. Sustain. Food Syst. , 27 November 2024
Sec. Sustainable Food Processing
Volume 8 - 2024 | https://doi.org/10.3389/fsufs.2024.1459600
This article is part of the Research Topic Sustainable Active Packaging for Food Safety and Preservation: Technological, Consumer, and Environmental Perspectives View all 3 articles
Fresh fig fruits are abundant sources of antioxidants and health-beneficial compounds. However, they are highly prone to spoilage and have short postharvest life. Thus, the current study aimed to investigate the potential synergistic effect of applying Aloe vera (AV) gel combined with chitosan (CH) as a natural edible coating on fresh fig fruits’ quality and shelf life. Different coating treatments; T1—Water; T2—CH (0.5%, w/v); T3—AV (100%); T4—AV (100%) + 1% CH (25:75, v/v); T5—AV (50%) + 1% CH (25:75, v/v); T6—AV (50%) + 1% CH (40:60, v/v), were applied to fresh fig fruits utilizing dip method. Fruits were analyzed weekly to examine fruit weight loss, decay, color, texture, enzyme activity [β Galactosidase and polyphenol oxidase enzyme (PPO)], phenolic content, antioxidants, and microbial load. The combined AV and CH coatings extended the shelf life of the fig fruits up to 21 days compared to the control during storage at 2°C and 90–95% R.H. The T5 treatment has significantly retarded the fruit decay, reduced total fungal and bacterial counts, preserved total phenolics and flavonoid contents, and antioxidant activity (enzymatic and non-enzymatic), as compared to the other treatments. Meanwhile, T6 fruits showed minimum fruit weight loss and microbial load. These treatments can be applied at a large scale to improve the shelf life of fresh fig fruits.
The fig (Ficus carica L.) belongs to the Moraceae family and is one of the oldest fruit trees cultivated worldwide (Ben Temessek et al., 2023). The fruit has an increasing demand among consumers due to its high nutritional and medicinal value (Debib and Menadi, 2023; George et al., 2023). According to the current Food and Agriculture Organization of the United Nations (FAO) database, fig production has increased from 1.08 to 1.35 million tons since 2016 to meet the increasing demand (FAOSTAT, 2022). Due to their tolerance to drought and alkalinity, they grow mainly in the hot and dry climates of subtropical regions. Compared with other deciduous trees, it flourishes better in warm, humid environments with less chilling incidence (Micheloud et al., 2023).
Figs are climacteric fruits characterized by ethylene production and high respiration rate at the onset of ripening (Freiman et al., 2015). They undergo a rapid ripening process, usually taking at least 3 days to develop into a mature eatable fruit. Additionally, figs are very sensitive to microbial deterioration due to the abundance of sugars and openings (ostiole end) which easily allow the microbes to enter the fruit (Crisosto et al., 2011). To overcome these problems, figs are commonly commercialized in dried form. However, the content of bioactive compounds gets compromised compared to fresh fruits (Sandhu et al., 2023), therefore, giving a suitable postharvest treatment to fresh fig fruits is essential to decrease their susceptibility to such incidences that reduce their marketability and shelf-life.
Cold storage, modified atmosphere packaging, and edible coatings are some techniques that can be utilized to extend the postharvest life of fresh fruit (Maan et al., 2021; Abdullah et al., 2023). Still, postharvest life necessitates innovative techniques to keep fresh fig quality at peak levels (Al Shaibani et al., 2022). In this sense, refrigeration on its own is ineffective for preserving the freshness of the fruit (Nadeem et al., 2022). Alternatively, edible coatings on fresh fruits and vegetables can reduce nutritional and physicochemical changes from field to table compared to traditional preservation methods (Adiletta et al., 2022). Recently, increasing attention has been given to compounds of natural origin for coating due to the economic costs associated with food spoiling and health concerns about food safety (Kaur et al., 2023). Bio-based compounds such as chitosan (CH) and Aloe vera gel are increasingly being utilized in edible coatings to maintain the integrity of fresh fruits after harvesting (Maan et al., 2021).
Chitosan (CH), a derivate of chitin, is a high molecular weight polycationic linear polysaccharide that is derived from natural sources, including the cell walls of microorganisms, fungi, and the outer shells of crustaceans (Brasil and Siddiqui, 2019). The well-renowned biopolymer has a vast range of applications in food packaging, cosmetics, and the medical sector (Balakrishnan et al., 2023; Kulka and Sionkowska, 2023; Nguyen et al., 2023). It has been used as edible coatings and films to preserve and enhance the shelf life of fruits, vegetables, and poultry products (Adiletta et al., 2018; Fernando et al., 2023). Chitosan alone as a coating material has remarkable properties; however, combining it with other components has enhanced the overall effect of treatment (Asif et al., 2023). Several substances such as essential oils, phenolic compounds, nanoparticles, phytohormones, and plant extracts have been used in combination with CH to improve the quality and shelf life of various horticulture products (Khalil et al., 2022; Sun et al., 2022; Aazami et al., 2023). Adding other materials to the CH can exert both favorable and adverse influences. Therefore, selecting a suitable material for a positive synergetic effect is crucial.
Aloe vera (Aloe barbadensis Miller) gel has recently been reported as an edible coating for food preservation and packaging (Maan et al., 2021; Kaur et al., 2024). The transparent jelly material mainly comprises water carbohydrates, proteins, organic acids, vitamins, minerals, and bioactive compounds (Grace et al., 2015; Kahramanoğlu et al., 2019). Historically, Aloe vera gel (AV) has been known to have many therapeutic benefits and is used to treat several diseases (Embark and Abdalla, 2019; George et al., 2023). The use of AV gel has expanded at a wider scale, and it has been employed as a coating material to extend the shelf life of many horticulture products (Adhikary et al., 2023; Farooq et al., 2023; Kaur et al., 2024).
The application of AV or CH on fig fruits as a standalone treatment has been reported (Mirshekari et al., 2020; Akhila et al., 2022). However, the literature has no information regarding the combined application. Therefore, the present study investigated the synergistic effect of AV gel combined with CH as an edible coating on fig fruit quality and shelf life during cold storage for 21 days.
Fresh Aloe vera (Aloe barbadensis) leaves (disease-free) of uniform size were harvested from the AV plants cultivated in the greenhouse at UAE University, Al Ain City, United Arab Emirates (UAE). Fig fruits “Brown turkey” cv., were collected from commercial farms in Al Ain City. Fruits were harvested 50% purple skin color (the commercial maturity stage), and immediately were transported to the university laboratory.
The AV gel was extracted and prepared for coating treatment according to the protocol mentioned by (Kaur et al., 2024). The chitosan stock solution (1%, w/v) was prepared and the pH was adjusted by lactic acid (1%), and then used to make different coating formulations in combination with AV gel (Table 1). All the formulations were kept stable in sterile tubes. The homogeneity of the formulations was observed by measuring the absorbance of solutions at 600 nm just after the preparation of solutions. Afterward, absorbance from the lower and upper portions of the solution was taken after 24 h to check the homogeneity. Homogenous solutions were used to coat the fruits. Uniform fig fruits (in color and size) without defects, pests, or mechanical damage were randomly selected and distributed into 6 groups each for one treatment as mentioned in the table above. Fruits were given dip treatment for 60 s and then dried at room temperature for 2 h. Afterward, fruits were packed in plastic trays with perforated resealable plastic enclosure and placed in cold storage at 2°C and 90–95% R.H. for up to 21 days. Fig samples (10 fruits) from each treatment were taken out from storage each week for various analyses in triplicates.
Ten fruits/treatments were retrieved from cold storage weekly and weighed using an analytical balance, and visually checked for any deterioration and counted every week till the end of storage. The weight loss and decay percentages were calculated according to Equations 1 and 2, respectively (Ahmed et al., 2022).
The textural analysis of fruits was carried out using CTX USB texture analyzer, Brookfield, (USA), and the probe, trigger, deformation, and speed of penetration applied were 2 mm, 0.0100 N, 5 mm, and 1 mm/s, respectively (Ahmed et al., 2022).
Fruit color was recorded using a Hunter Lab colorimeter (Hunter Lab Inc., Reston, VA, USA), and values: L* (Lightness), a* (red, green), and b* (blue, yellow) were recorded every week of cold storage. The Hue angle, Chroma saturation, and Browning Index (BI) were calculated (Hssaini et al., 2021).
The total fungal/mold and bacterial counts on fruits were determined before cold storage and at the end of the storage period of 21 days according to the method described by (Kaur et al., 2024).
Preparation of fruit extract: Fig fruits were cut into small pieces and 10 grams of fruit pieces were taken to freeze-dry using a Labconco FreeZone freeze dryer. The lyophilized fruits were homogenized with 50 ml of Absolute methanol and left in dark conditions at room temperature shaker at 150 rpm. The next day, samples were filtered using filter paper (Whatman #1) and dried using a water bath at 45°C to evaporate the methanol, and the dry weight of the extract was recorded. The final extract was prepared by dissolving the dry fig extract in 2 ml of 50% methanol and was used for further analysis (Aldhanhani et al., 2022).
A modified method was adopted to measure fig fruit extract’s total phenolic content (TPC; Velioglu et al., 1998). An aliquot of 100 μl of fig extract was added into test tubes, then 50 μl of Folin Ciocalteu reagent and 2 ml of NaOH (6%). The tubes were vortexed and incubated in the dark for 45 min. Then the blue color developed was measured using a UV–visible spectrophotometer at 650 nm. The results were calculated according to the standard curve of gallic acid and expressed as milligram gallic acid equivalents (GAE) per 100 grams of fresh weight (mg GAE 100 g−1 FW).
The total flavonoid content was determined for fig fruit extract according to Kim et al. (2003). Briefly, an aliquot of 75 μl of NaNO2 (5%) was added to the fig extract (250 μl) and vortexed then incubated for 5 min in the dark. Afterwards, 75 μl of AlCl3 (10%) was added; the mixture was kept in the dark for 6 min. After 6 min, 500 μl of NaOH (1 M) was added, mixed by vertexing and the final volume was made to 2.5 ml with distilled water. The absorbance was measured at 510 nm using a spectrophotometer (Shimadzu, Kyoto, Japan). The results were calculated and expressed as milligram catechin per 100 grams fresh weight basis (mg CE 100 g−1 FW).
The scavenging activity of 2,2-diphenyl-1-picryl hydrazyl (DPPH) radical was determined according to the method developed by Blois in 1958 with some modifications (Kedare and Singh, 2011). Different concentrations of samples ranging from 2 to 10 mg ml−1 were mixed with 0.5 ml of DPPH (0.15 mM) in absolute methanol. After 30 min incubation in the dark at room temperature, absorbance was taken at 517 nm. A blank was prepared for each similar concentration, except that methanol was utilized instead of a DPPH solution. Each sample’s standard curve was prepared to calculate the IC50 (Half maximal inhibitory concentration) value (milligrams per milliliters of concentrated fig extract).
The radical scavenging activity of 2,2′-azino-bis-(3-ethylbenzothiazoline-6-sulfonic acid (ABTS) was determined by using a modified method (Khadhraoui et al., 2019). Stock solutions of ABTS (7.4 mM) and potassium persulphate (2.6 mM) were prepared. For each assay, a fresh ABTS solution was made. Samples (150 μl) with concentrations ranging from 0.5 to 10 mg ml−1 were combined with 2,850 μl of ABTS solution and left at room temperature for 2 h in the dark. The absorbance was then measured at 734 nm. The activity was recorded and calculated according to a Trolox standard curve ranging from 50 to 600 μM, and expressed as milligrams of Trolox equivalents (TE) per 100 grams fresh weight basis (mg TE 100 g−1 FW).
A modified method was utilized to extract the β Galactosidase enzyme from fig fruits and to assess its activity (Rastegar et al., 2012). Briefly, 5 grams of fig fruit tissue was homogenized in a sodium acetate buffer (50 mmol L−1) and sodium chloride (1 mol L−1), and polyvinylpolypyrrolidone (12 g L−1), pH 6.2. After homogenization, the mixture was stirred for 3 h at 4°C. Then centrifuged (7,500 rpm) at 4°C for 35 min. Subsequently, the supernatant (crude enzyme extract) was collected and used for enzyme activity measurement. For β Galactosidase activity analysis, 50 μl of sodium acetate buffer (0.1 M, pH 5.2) and 50 μl of р-nitrophenyl-β-D-galactopyranoside were pre-incubated at 37°C for 15 min. Then 50 μl of crude enzyme extract was added to the above mixture and allowed to react for 30 min at 37°C. The reaction was stopped by adding 200 μl of sodium carbonate solution (0.5 M). Production of free р-nitrophenol was measured at 400 nm in a Multiskan™ FC (Thermo Fisher Scientific, Waltham, MA, USA) Microplate Photometer, and β Galactosidase activity was expressed as units per gram fresh weight of fruits.
Fig fruits’ polyphenol oxidase enzyme (PPO) activity was determined every week (Daas Amiour and Hambaba, 2016). Fruit tissue (5 g) was homogenized in phosphate buffer (50 mM, pH 6.8) containing 12 g L−1 polyvinylpolypyrrolidone and stirred for 30 min at 4°C. The clear supernatant was collected after centrifuging the samples at 4°C for 30 min and labeled as crude enzyme extract. For PPO activity, 50 mM potassium phosphate buffer, pH-6.8 (150 μl), crude enzyme extract (10 μl), and freshly prepared 20 mM catechol (50 μl) were added to 96 well-plate and then kept at 37°C for 30 min. PPO activity was measured at 398 nm in a Multiskan™ FC Microplate Photometer. The results were expressed as units per gram fresh weight of fruits.
Fig fruits’ peroxidase enzyme (POD) activity was measured every week using a modified method (Zeyadi, 2019). Five grams of fig fruit tissue was homogenized in Tris–HCl buffer (50 mM, pH 7.5) containing 12 g L−1 polyvinylpolypyrrolidone and stirred for 30 min at 4°C. Then, the mixture was centrifuged, and the clear supernatant was collected to measure enzyme activity. For enzyme activity assay, the reaction mixture containing 50 mM sodium acetate buffer sample (pH 5.2), 10 μl enzyme extract, 40 mM guaiacol (freshly prepared), and 8 mM H2O2 was prepared and incubated at 37°C for 30 min and absorbance reading was taken at 470 nm utilizing a Multiskan™ FC Microplate Photometer. The results were calculated and expressed as units per gram of fresh fruit weight where one unit of peroxidase is the amount of enzyme that reduces 1.0 μM of H2O2 per minute at 37°C.
All experiments design was a complete randomized design (CRD) with three replicates. The obtained data were statistically analyzed by analysis of variance two ways-ANOVA utilizing the Minitab statistical analysis software version 21. Comparisons between means were accomplished by the Fisher LSD test at level p ≤ 0.05. Principal component analysis was done using Factoextra and FactoMineR libraries in R Studio software version 4.2.2 (Kassambara and Mundt, 2020).
Fruit weight loss results of treated and non-treated fig fruits during cold storage are presented in Figure 1. Throughout the experiment, gradual weight loss occurred in all the fruits during storage. When compared to the treated fruits, the T1 (control) fruits showed the greatest weight loss, reaching a drop of 12.38% by the end of the cold storage period. In the context of treated fruits, T6 fruits showed the least weight loss (7.40%) followed by T2 (8.98%), whereas no significant difference was observed in the weight loss of T4 (8.66%) and T5 (8.31%) at the end of storage (Figure 1). T3 (AV alone) coating was found to be the least effective compared to other coatings.
Figure 1. Effect of different edible coatings on weight loss of fig fruits during cold storage. Means with different letters in the column for each treatment are significantly different at p ≤ 0.05 using the Fisher LSD test. Values are the mean (n = 10) ± SE. T1: control; T2: CH (0.5%, w/v); T3: AV (50%, v/v) T4: AV + 1% CH (25:75, v/v), T5: 50% AV + 1% CH (25:75, v/v), T6: 50% AV + 1% CH (40:60, v/v).
Color is an essential visual attribute of food, especially for fresh fruit, that has a direct impact on the product attractiveness and acceptability by the consumers. Therefore the effect of CH and AV-based coatings on the color characteristics: L* (Lightness), Hue angle, Browning index, and Chroma (saturation) of fig fruits during cold storage are presented in Figure 2. Generally, color parameters the fruit were significantly impacted by the application of the edible coating. A color transition from dark purple to nuanced brown was observed in all the fig fruits during storage discernible through a decrease in L* and chroma values. Surprisingly, T6 fruits showed more color transition compared to the control fruit andother treated fruits. On the other hand, T2, T4, and T5 fruits showed the least color change, i.e., high L* and chroma, low hue values, and least browning.
Figure 2. Effect of different edible coatings on fig fruit color parameters: L*, Browning index, Chroma, and Hue angle during cold storage. Means with different letters in the column for each treatment are significantly different at p ≤ 0.05 using the Fisher LSD test. Values are the mean (n = 10) ± SE. T1: control; T2: CH (0.5%, w/v); T3: AV (50%, w/v), T4: AV + 1% CH (25:75, v/v), T5: 50% AV + 1% CH (25:75, v/v), T6: 50% AV + 1% CH (40:60, v/v).
In the present study, a gradual reduction in firmness was observed in all the fruits with significant differences (p ≤ 0.05) within the treatments and the storage days (Figure 3). The control fruits (T1) showed a substantial loss in firmness from day zero to the end of the storage period (2.16–1.38 N) concerning the other treatments. Among the treated fruits, T4 (2.32–1.906 N) and T5 (2.33–1.94 N) coatings considerably preserved the fruit texture and performed better than T2, T3, and T6 and the control till the end of the cold storage. While no significant differences were noticed in the texture of T2 (2.18–1.63 N), T3 (2.19–1.75 N), and T6 (2.05–1.65 N) treated fruits. The results suggest that T4 and T5 treated fruits are the best treatment for maintaining fruit firmness.
Figure 3. Effect of different edible coatings on fig fruits texture during cold storage. Means with different letters in the column for each treatment are significantly different at p ≤ 0.05 using the Fisher LSD test. Values are the mean (n = 10) ± SE. T1: control; T2: CH (0.5%, w/v); T3: AV (50%, w/v), T4: AV + 1% CH (25:75, v/v), T5: 50% AV + 1% CH (25:75, v/v), T6: 50% AV + 1% CH (40:60, v/v).
To determine the effect of edible coatings on fruit firmness, β galactosidase activity was measured in fruits every week and results are presented in Table 2. It is observed that the enzyme activity was first elevated from day 0 to day 7 in all fruits except T1, T4, and T5. At day 0, the highest activity was found in T5 (77.0 U g−1 FW) followed by T4 (76.7 U g−1 FW), T3 (73.9 U g−1 FW), T6 (68.4 U g−1 FW), and T2 (62.6 U g−1 FW) in decreasing order. However, on day 14, all treated fruits showed decreased enzyme activity except T1 (control), and then the enzyme activity increased for all fruits, except for T2, where it remained unchanged on day 21 of storage.
Table 2. Effect of different edible coatings on β-galactosidase enzyme activity (U g−1 fresh fruit weight) in fig fruits during cold storage at 2°C and 90–95% R.H. for 21 days.
The present study examined the effect of CH and AV-based coatings on preventing the microbial degradation of fig fruits. The visual inspection of fruits was done to detect the fermentative smell, mold appearance, and any other visible disorder, and the results were expressed as fruit decay percentage (Figure 4). The data showed significant differences between different treatments and storage times on fruit deterioration.
Figure 4. Effect of different edible coatings on fruit decay index during cold storage at 2°C (90–95% R.H.) for 21 days. Means with different letters in the column for each treatment are significantly different at p ≤ 0.05 using the Fisher LSD test. Values are the mean (n = 10) ± SE. T1: control; T2: CH (0.5%, w/v); T3: AV (50%, w/v), T4: AV + 1% CH (25:75, v/v), T5: 50% AV + 1% CH (25:75, v/v), T6: 50% AV + 1% CH (40:60, v/v).
Comprehensively, the fruits started to degrade on the 14th day of cold storage in all treatments and by the end of the storage, severe symptoms of spoilage were found in T1 fruits (50.3%) followed by the T3 (33%), whereas all other treated fruit had lower decay percentage with T5 fruits the least symptomatic (3.0%). At the end of the experiment after 21 days of cold storage, the T1 fruits had 83.3% decay, while T5 only had 20.5%, which means that the shelf life of the fruit had increased by 7–10 days after the T5 treatment.
To evaluate the total microbial load in the fig fruit, the total fungal count and bacterial count were measured before and after cold storage, and the results are presented in Figure 5. Overall results depicted the surge in the microbial count from day 0 to day 21 of cold storage. However, the fruits coated with CH and AV had less fungal and bacterial growth than the control (T1). In the present study, the initial ranges for total bacterial and fungal counts ranged from 3.77 to 4.62 and 3.4 to 4.10 Log10 CFUg−1 FW, respectively, then it reached 5.82–6.68 and 4.82–6.83 Log10 CFUg−1 FW, respectively at the end of storage. It is observed that all treatments including chitosan have lower bacterial and fungus growth, with T5 fruits showing the minimum microbial load (4.17, 4.82 Log10 CFUg−1 FW) as compared to T1 the control (6.67, 6.95 Log10 CFUg−1 FW). In comparison, no significant difference was found between T1 (control) and T3 (AV-treated fruit) at the end of the storage period.
Figure 5. Different edible coatings’ effect on fruits’ microbial load during cold storage at 2°C (90–95% R.H.) for 21 days. Means with different letters on the bars for each treatment are significantly different at p ≤ 0.05 using the Fisher LSD test. Values are the mean (n = 10) ± SE. T1: control; T2: CH (0.5%, w/v); T3: AV (50%, w/v), T4: AV + 1% CH (25:75, v/v), T5: 50% AV + 1% CH (25:75, v/v), T6: 50% AV + 1% CH (40:60, v/v).
The current study evaluated the effect of coatings made of CH and AV in preserving the quality of fig fruits during storage. The results showed that the coatings preserved fruits’ phenolics and flavonoid content until the end of storage (Table 3). Overall results depicted that the applied coatings have modulated the phenolic and flavonoids. The T1 (control) fruits were found with reduced TPC and TFC from the beginning and throughout the cold storage whereas the T6 fruits showed the highest TPC and TFC with 410.8 mg GAE 100 g−1 FW and 67.3 mg CE 100 g−1 FW, respectively at day zero whereas, no significant differences were found in the TPC for rest of the treatments. However, the T6 treatment reduced the phenolic and flavonoid content at day 21. At the end of storage, T5-treated fruits showed the highest TPC and TFC with 427 mg GAE 100 g−1 FW and 73 mg CE 100 g−1 FW, respectively.
Table 3. Effect of different edible coatings on total phenolic content (TPC) and total flavonoid content (TFC) in fig fruits during cold storage at 2°C and 90–95% R.H. for 21 days.
In this study, different formulations of edible films made of AV gel and CH were applied on fig fruits and the antioxidant content was measured using non-enzymatic (DPPH and ABTS; Table 4) and enzymatic (POD and PPO) methods (Table 5). DPPH is a purple color-reducing agent that reduces antioxidants and IC50 value indicates antioxidant activity in the sample were calculated. The IC50 is the concentration of antioxidant compounds in the sample that required to scavenge 50% of the initial DPPH radicals. The lower the IC50 value, the more scavenging DPPH and higher antioxidant activity. The antioxidant activity decreased during the storage in all the fruits irrespective of the treatments. However, T5 treatment showed the highest antioxidant activity with values of 5.1 mg ml−1 and 109.5 mg TE 100 g−1 FW for DPPH and ABTS assay, respectively, at the end of storage (Table 4). Meanwhile, T1 (control), followed by T6, showed the lowest antioxidant activity at the end of storage. The DPPH and ABTS results showed high similarities with the TPC and TFC values. Further validation of antioxidant activity was done by measuring the activity of antioxidant enzymes (POD and PPO) in fig fruits at weekly intervals and results are presented in Table 5. On day zero of storage, the POD activity got elevated in treated fruits compared to the control fruits and gradually increased with storage time. On the 21st day of storage, T5-treated fruits had the highest POD activity (77.3 U g−1 FW) compared to the other treatments and the control T1 (33.4 U g−1 FW). In the case of PPO activity, the opposite trend was observed. The PPO activity was comparatively higher at day 0 and then it started to decline with the storage time irrespective of the treatments. No significant differences were observed in the PPO activity within the treated fruits throughout the storage period.
Table 4. Effect of different edible coatings on antioxidant activity (ABTS; mg TE 100 g−1 FW) and (IC50, mg ml−1) in fig fruits during cold storage at 2°C and 90–95% R.H. for 21 days.
Table 5. Effect of different edible coatings on peroxidase (POD) and polyphenol oxidase (PPO) enzymes activities (U g−1 fresh FW) in fig fruits during cold storage at 2°C and 90–95% R.H. for 21 days.
The effect of different edible coatings on various physicochemical and biochemical properties of fig fruits during cold storage was analyzed using PCA (Figures 6A,B). The biplots represent the clustering of the parameters along with compound loading across the two main components. According to the eigenvalues, the cumulative variance of the first two components is explained by 72.8% (Dim 1 = 48.3% and Dim 2 = 24.5%). The variance in physiochemical properties of fig fruits is best explained by principal component 1 (Dim 1). Specifically, Dim1 represents the direction of maximum variance in the data, where the major portion of variability lies. The analysis results on each storage day of different treated fruits show significant differences that separate ellipses can see. According to both biplots, the most contributing factors are those far from the centre point. This shows that the β-Gal is the least contributing factor to the Dim1. The plots illustrate the correlation among the evaluated parameters, revealing a negative association between fruit firmness and fruit decay, as well as among TPC, TFC, and IC50. Additionally, correlations were observed among L*, Chroma, PPO, Hue, and FW. The PCA biplot (A) shows significant differences within the treatments during storage, and the plot explains small variability in T1 and T2 whereas, other treatment’s response varies significantly from day 0 to day 21 of cold storage. The second biplot describes the variability among the treatments during the whole storage period. The closely grouped bullet points indicate less variability in the treatments on day 0 of the storage period. However, the variability has increased by the end of the storage period as evidenced by the more scattered distribution of points (Figure 6B). These biplots gave a clear picture of the whole experiment.
Figure 6. (A) Principal component analysis (PCA) Biplot for the effect of postharvest application of different Treatments on various physicochemical and quality parameters of fig fruits during cold storage. (B) Principal component analysis (PCA) Biplot for the effect of storage days on various physicochemical and quality parameters of fig fruits (Treated and non-treated). Abbreviations: L, lightness; PPO, polyphenol oxidase; POD, peroxidase; BI, browning index; ABTS, 2,2′-azino-bis-(3-ethylbenzothiazoline-6-sulfonic) acid; IC50, half-maximal inhibitory concentration; FWL, fruit weight loss; β gal, β galactosidase; TBC, total bacterial count; and TFC = total fungal count.
Figs, being climacteric fruit have high respiration rates; they undergo fast deterioration after harvest. The primary affirmation of low-quality figs can be evaluated through total weight loss, palatability level, and a change in hue from purple to brown that influences the customer’s choice of purchasing (Lufu et al., 2020). Generally, fruits with a weight reduction of more than 10% from their initial weight become unviable for the market. The mechanism behind weight loss is complex, with several physiological changes such as transpiration and respiration taking place in the fruits during storage leading to water and turgidity loss, and the subsequent fruit flagging and withering (Lufu et al., 2020). Moreover, color changes in the figs are probably due to the ripening wherein ethylene hormone plays the major role. The function of ethylene is quite complex and multiplexed with other factors that synergistically trigger various metabolic pathways, influencing biochemical reactions, and causing oxidative browning in fruits during postharvest storage (Freiman et al., 2015). However, several research studies indicate that edible coatings based on CH and AV have been significantly effective in preventing weight loss and mitigating the degradation of postharvest quality in fruits. Due to the protective coat of edible layers, fruits do not lose their natural moisture easily. This phenomenon has been observed in different fruits coated with AV and CH such as mangoes, strawberries, kiwi, and peaches (Benítez et al., 2013; Pinzon et al., 2020; Shah and Hashmi, 2020; Aboryia et al., 2022). Similarly, the current results agree with the CH-coated sponge gourd (Han et al., 2014).
The role of CH and AV gel as edible coatings in delaying fruit ripening, decreasing various physiological disorders, and extending their shelf life has been reported. Furthermore, CH and AV coatings control the gas exchange in fruits during storage consequently slowing down the metabolism and pigment change. For instance, higher concentrations of AV gel have positively affected the color of strawberries (Pinzon et al., 2020). This could be due to the reduced contact with oxygen which might have inhibited the enzymatic reactions responsible for color change (Shekari et al., 2021). In another study, reduced weight loss occurs in tomatoes and guava coated with AV gel (Al-Hilifi et al., 2022; Dutta Roy et al., 2023; Farooq et al., 2023). In contrast, opposite results were found in the case of persimmon fruits coated with 50% AV gel (Saleem et al., 2022). Our findings suggest that CH and AV-based coatings have reduced discoloration, shrivelling, decay, and bioactive loss in fruits over cold storage. The CH owns the film-forming properties that reduce the water permeability across the fruit’s surface and prevent moisture loss (Kaur et al., 2024). The combination of CH and AV has retarded the fruit softening in mangoes, strawberries, guavas, papayas, and tomatoes (Adiletta et al., 2018; Mendy et al., 2019; Khatri et al., 2020; Pinzon et al., 2020). Likewise, our treatments especially, the T5 [50% AV + 1% CH (25:75, v/v)], T4 [AV + 1% CH (25:75, v/v)], and T2 (0.5% CH, w/v) have significantly preserved the physical properties of the fig fruits during cold storage.
When fruits begin to ripen, the starch components will convert to sugars, and cell wall components undergo hydrolyzation by different enzymatic actions. For example, β galactosidase (β-gal) enzyme is involved in the hydrolytic digestion of the non-reducing β-D-galactosyl moieties from the β-D-galactoside present in the cell wall, leading to fruit softening (Gross et al., 1994). The current investigation shows that the applied treatments have suppressed the β-galactosidase enzyme activity in fruits after 2 weeks of cold storage. This activity remained there in the control fruits until the 14th day. These results are highly correlated with the fruit firmness pattern. Similar results were found in the CH-coated blueberry and strawberry fruits (Gol et al., 2013; Sun et al., 2022). The coatings have prevented moisture loss from the fruits, thereby preserving the fruit’s firmness until the end of storage.
The postharvest decay in fresh fig fruits is mainly caused by fungal species from the genus: Fusarium, Alternaria, Aspergillus, Rhizopus, Penicillium, and Botrytis (Crisosto et al., 2011; Dogan et al., 2018). Fig fruits exhibit exceptional delicacy; even a minor contact can incur abrasion, eventually making them highly susceptible to microbial spoilage. However, this delicacy can be improved and protection from microorganisms can be achieved using appropriate postharvest management techniques (Crisosto et al., 2011). In the current research, the T5 (50% AV + 1% CH (25:75, v/v)) and T6 (50% AV + 1% CH (40:60, v/v)) treatments were found to be the best in retarding the microbial growth on fig fruits, thus, extending the storage life. This might be due to the specific concentrations of AV gel and CH used in this study. Despite their antimicrobial properties, the ratio of CH and AV gel used has improved their film-forming abilities which have effectively coated the fruit and retarded the microbial growth. Correspondingly, a prior study reported the role of CH in providing resistance against Botrytis cinerea in ripened strawberries and grapes (Peian et al., 2021).
Fig fruits possess a high number of bioactive compounds including anthocyanins, flavonoids (flavanols, flavones, flavanones, flavanonols, flavonols, and isoflavones), and phenolic compounds such as hydroxybenzoic acids, hydroxycinnamic acids, and hydroxy coumarins (Ammar et al., 2015). These compounds play a pivotal role in defence, imparting resistance against diverse microorganisms and reactive oxygen species. Also, they serve as a great source of antioxidants and provide several health benefits to humans (Debib and Menadi, 2023). Edible coatings have been proven to be highly effective in preserving the antioxidants in fruits, thereby enhancing their shelf life.
The CH has been documented to be involved in defence-related pathways such as jasmonic acid signalling and phenol synthesis pathways (Peian et al., 2021). Furthermore, it activates the phenylalanine ammonia-lyase; a key enzyme in phenolic acid biosynthesis and boosts the production of phenolic compounds. The application of CH and AV gel has decreased the phenolic and flavonoid loss in several fruits such as mangoes, sapota, strawberry, raspberry, and loquat during storage (Hassanpour, 2015; Adiletta et al., 2018; Khaliq et al., 2019). On the other hand, different concentrations of AV gel as coatings have been reported to preserve the TPC and TFC of tomatoes (20% AV gel) and cherries (100% AV gel) during cold storage (Serrano et al., 2017). In some cases, AV gel is used as a carrier for other elements in the coatings to maintain the fruit’s phenolic content, which AV gel alone cannot preserve. For example, adding ascorbic acid to 33% AV gel improved the phenolic and flavonoid content in strawberries compared to the AV gel alone (Sogvar et al., 2016). In the present study, AV gel (50%) combined with CH (1%) in a 40:60 ratio could not preserve the TPC and TFC content by the end of storage. However, better results were found in AV gel alone and other combinations with CH. Hence, the treatments improved the TPC and TFC in fig fruits till the end of cold storage.
The relationship between the postharvest degradation of phenolic compounds and the activity of antioxidant-related enzymes (POD and PPO) has been reported (Posmyk et al., 2009). It is suggested that they deal with many postharvest events, including discoloration, browning, and off-flavour. PPO enzymes are responsible for browning by reducing diphenols, whereas POD is involved in the transition of reactive oxygen species to hydrogen peroxide (Shekari et al., 2021). POD is a main enzyme that helps in reducing oxidative damage. Mainly, phenolic compounds are a substrate of browning-causing enzymes. In this manner, the low PPO activity is proportionate to the low consumption of phenolic compounds and the browning symptoms (Loay and Taher, 2018). It has been proposed that the inhibition of these enzymes can reduce the browning and discoloration of fruits (Maioli et al., 2020). CH treatment inhibited PPO and POD activity during cold storage in Loquat fruit and eggplant (Adiletta et al., 2018; Sharma et al., 2021). Higher POD activity was found in jujube fruits, mangoes, and berries treated with AV and CH (Xing et al., 2020; Nia et al., 2021). The current results showed that the treatments applied on fig fruits have increased the POD activity and reduced PPO activity during cold storage. Hence, it could be inferred that the CH and AV gel combinations as an edible coating are promising in maintaining the quality and extending the storage life of fig fruits.
The treatments utilized in the current study have significantly improved the shelf life of figs by delaying the fruit weight loss and microbial load on fruits during the cold storage for 21 days. Overall, all coating compositions have positively affected the fruit performance during storage however, the T5 treatment (50% AV + 1% CH (25:75, v/v)) performed better than the other treatments across the storage period. The T5 treatment significantly reduced the microbial load on fruits and retarded fruit decay. Moreover, the T5 treatment improved the fruit’s phenolic and flavonoid content and its antioxidant activity. Whereas T6 (50% AV + 1% CH (40:60, v/v)) and T2 (CH (0.5%, w/v)) treatments protected the fruit from weight loss till the end of storage. This study concludes that the coatings: T2 (CH (0.5%, w/v)), T5 (50% AV + 1% CH (25:75, v/v)), and T6 (50% AV + 1% CH (40:60, v/v)) can be used at large scale for improving the shelf life of fig fruits. Additionally, these treatments can be tried on other perishable fruits to observe their broad-spectrum effects.
The original contributions presented in the study are included in the article/supplementary material, further inquiries can be directed to the corresponding author.
NK: Formal analysis, Investigation, Methodology, Software, Writing – original draft. CS: Formal analysis, Supervision, Validation, Writing – review & editing. ZR: Formal analysis, Supervision, Validation, Writing – review & editing. ZA: Conceptualization, Data curation, Funding acquisition, Methodology, Project administration, Software, Supervision, Validation, Writing – review & editing.
The author(s) declare that financial support was received for the research, authorship, and/or publication of this article. This research was funded by United Arab Emirate University (UAEU) through the Asian Universities Alliance Joint Research Program (AUA-UAEU) in collaboration with Malaya University, project grant number 12F037.
The authors are thankful to UAEU for funding this project. The appreciation is also extended to the College of Agriculture and Veterinary Medicine, Integrative Agriculture Department, Horticulture lab (Abou Mesalam Abdalla).
The authors declare that the research was conducted in the absence of any commercial or financial relationships that could be construed as a potential conflict of interest.
All claims expressed in this article are solely those of the authors and do not necessarily represent those of their affiliated organizations, or those of the publisher, the editors and the reviewers. Any product that may be evaluated in this article, or claim that may be made by its manufacturer, is not guaranteed or endorsed by the publisher.
Aazami, M. A., Maleki, M., Rasouli, F., and Gohari, G. (2023). Protective effects of chitosan based salicylic acid nanocomposite (CS-SA NCs) in grape (Vitis vinifera cv. 'Sultana') under salinity stress. Sci. Rep. 13:883. doi: 10.1038/s41598-023-27618-z
Abdullah, A. H., Awad-Allah, M. A. A., Abd-Elkarim, N. A. A., Ahmed, Z. F. R., and Taha, E. M. A. (2023). Carboxymethyl cellulose from Banana rachis: a potential edible coating to extend the shelf life of strawberry fruit. Agriculture 13:1058. doi: 10.3390/agriculture13051058
Aboryia, M. S., El-Gioushy, S. F., Sami, R., Aljumayi, H., Alyamani, A., Almasoudi, A., et al. (2022). Synergistic effect of dipping in Aloe vera gel and mixing with chitosan or calcium chloride on the activities of antioxidant enzymes and cold storage potential of peach (Prunus persica L.) fruits. Coatings. 12:498. doi: 10.3390/coatings12040498
Adhikary, T., Gill, P. S., and Jawandha, S. K. (2023). Use of novel postharvest Aloe vera gel coating for suppression of ripening changes in Asian pears during long-term cold storage. Int. J. Food Sci. Technol. 58, 4316–4323. doi: 10.1111/ijfs.16532
Adiletta, G., Pasquariello, M. S., Zampella, L., Mastrobuoni, F., Scortichini, M., and Petriccione, M. (2018). Chitosan coating: a postharvest treatment to delay oxidative stress in loquat fruits during cold storage. Agronomy 8:54. doi: 10.3390/agronomy8040054
Adiletta, G., Zampella, L., Coletta, C., and Petriccione, M. (2022). Chitosan coating to preserve the qualitative traits and improve antioxidant system in fresh figs (Ficus carica L.). Agriculture 9:84. doi: 10.3390/agriculture9040084
Ahmed, Z. F. R., Kaur, N., Maqsood, S., and Schmeda-Hirschmann, G. (2022). Preharvest applications of chitosan, salicylic acid, and calcium chloride have a synergistic effect on quality and storability of date palm fruit (Phoenix dactylifera L.). HortScience 57, 422–430. doi: 10.21273/HORTSCI16416-21
Akhila, M., Vanajalatha, K., Joshi, V., and Kumar, S. P. (2022). Studies on effect of different surface coatings on shelf life and quality of fig (Ficus carica L.) cv. Brown Turkey. Pharm. Innov. 11, 2463–2470.
Al Shaibani, F. Y. Y., Kaur, N., and Ahmed, Z. F. R. (2022). Reducing postharvest loss by improving fruit quality, shelf life, bioactive compounds of Rutab date (Phoenix dactylifere L. cv. Barhi) using natural elicitors. Acta Hortic. 1340, 119–124. doi: 10.17660/ActaHortic.2022.1340.18
Aldhanhani, A. R. H., Kaur, N., and Ahmed, Z. F. R. (2022). Phytochemical composition, antioxidant and antibacterial activities of Ziziphus mauritiana and Ziziphus spina-christi leaf. Acta Hortic. 1353, 323–332. doi: 10.17660/ActaHortic.2022.1353.40
Alhamdan, A. M., Al Juhaimi, F. Y., Alamri, Y. A., Ahmed, I. A. M., Elamshity, M. G., Özcan, M. M., et al. (2024). Determination of changes in bioactive components, antioxidant capacity, phenolic components and mineral values of date fruits stored in different packages and temperatures for six months. J. Food Meas. Charact. 18, 4571–4591. doi: 10.1007/s11694-024-02515-9
Al-Hilifi, S. A., Al-Ali, R. M., Al-Ibresam, O. T., Kumar, N., Paidari, S., Trajkovska Petkoska, A., et al. (2022). Physicochemical, morphological, and functional characterization of edible anthocyanin-enriched Aloe vera coatings on fresh figs (Ficus carica L.). Gels 8:645. doi: 10.3390/gels8100645
Allegra, A., Sortino, G., Inglese, P., Settanni, L., Todaro, A., and Gallotta, A. (2017). The effectiveness of Opuntia ficus-indica mucilage edible coating on post-harvest maintenance of ‘Dottato’fig (Ficus carica L.) fruit. Food Packag. Shelf 12, 135–141. doi: 10.1016/j.fpsl.2017.04.010
Ammar, S., del Mar Contreras, M., Belguith-Hadrich, O., Bouaziz, M., and Segura-Carretero, A. (2015). New insights into the qualitative phenolic profile of Ficus carica L. fruits and leaves from Tunisia using ultra-high-performance liquid chromatography coupled to quadrupole-time-of-flight mass spectrometry and their antioxidant activity. RSC Adv. 5, 20035–20050. doi: 10.1039/C4RA16746E
Asif, A., Ali, M., Qadir, M., Karthikeyan, R., Singh, Z., Khangura, R., et al. (2023). Enhancing crop resilience by harnessing the synergistic effects of biostimulants against abiotic stress. Front. Plant Sci. 14:1276117. doi: 10.3389/fpls.2023.1276117
Balakrishnan, A., Appunni, S., Chinthala, M., Jacob, M. M., Vo, D. V. N., Reddy, S. S., et al. (2023). Chitosan-based beads as sustainable adsorbents for wastewater remediation: a review. Environ. Chem. Lett. 21, 1881–1905. doi: 10.1007/s10311-023-01563-9
Benítez, S., Achaerandio, I., Sepulcre, F., and Pujolà, A. (2013). Aloe vera based edible coatings improve the quality of minimally processed ‘Hayward’ kiwifruit. Postharvest Biol. Technol. 81, 29–36. doi: 10.1016/j.postharvbio.2013.02.009
Ben Temessek, M., Talbi, W., Chrifa, H., and Fattouch, S. (2023). “Fig (Ficus carica L.) production and yield” in Fig (Ficus carica): Production, processing, and properties. ed. M. F. Ramadan (Cham: Springer), 259–282.
Brasil, I. M., and Siddiqui, M. (2019). “Postharvest quality of fruits and vegetables: An overview” in Preharvest modulation of postharvest fruit and vegetable quality. ed. M. W. Siddiqui. 1st ed., ed (London, UK: Elsevier, Inc.), 1–40.
Crisosto, H., Ferguson, L., Bremer, V., Stover, E., and Colelli, G. (2011) in “Fig (Ficus carica L.)”, in postharvest biology and technology of tropical and subtropical fruits. ed. M. Y. Elhadi (Woodhead Publishing, Elsevier), 134–160e. doi: 10.1533/9780857092885.134
Daas Amiour, S., and Hambaba, L. (2016). Effect of pH, temperature and some chemicals on polyphenol oxidase and peroxidase activities in harvested Deglet nour and Ghars dates. Postharvest Biol. Technol. 111, 77–82. doi: 10.1016/j.postharvbio.2015.07.027
Debib, A., and Menadi, S. (2023). “Phenolic compounds of fresh and dried figs: characterization and health benefits” in Fig (Ficus carica): Production, processing, and properties. ed. M. F. Ramadan (Cham: Springer), 395–416.
Dogan, A., Cat, A., Catal, M., and Erkan, M. (2018). First report of Alternaria alternata causing postharvest decay in fig (Ficus carica L. cv. Bursa Siyahi) fruit in Turkey. J. Biotechnol. 280, S84–S91. doi: 10.1016/j.jbiotec.2018.06.275
Dutta Roy, D. K., Asaduzzaman, M., Saha, T., and Khatun, M. N. (2023). Physical and chemical properties of Aloe-vera coated guava (Psidium guajava) fruit during refrigerated storage. PLoS One 18:e0293553. doi: 10.1371/journal.pone.0293553
Embark, H., and Abdalla, A. (2019). A study on the effect of Aloe vera extract on the motor and exploratory activities in normal healthy rats. Assiut Vet. Med. J. 65, 47–57.
FAOSTAT. (2022). Available at: https://www.fao.org/faostat/en/#data/QCL/visualize. [Accessed July 15, 2024].
Farooq, A., Niaz, B., Saeed, F., Afzaal, M., Armghan Khalid, M., Raza, M. A., et al. (2023). Exploring the potential of Aloe vera gel-based coating for shelf-life extension and quality preservation of tomato. Int. J. Food Prop. 26, 2909–2923. doi: 10.1080/10942912.2023.2263661
Fernando, S. S., Jo, C., Mudannayake, D. C., and Jayasena, D. D. (2023). An overview of the potential application of chitosan in meat and meat products. Carbohydr. Polym. 324:121477. doi: 10.1016/j.carbpol.2023.121477
Freiman, Z. E., Rosianskey, Y., Dasmohapatra, R., Kamara, I., and Flaishman, M. A. (2015). The ambiguous ripening nature of the fig (Ficus carica L.) fruit: a gene-expression study of potential ripening regulators and ethylene-related genes. J. Exp. Bot. 66, 3309–3324. doi: 10.1093/jxb/erv140
George, T. T., Oyenihi, A. B., Oyenihi, O. R., and Obilana, A. O. (2023). “Composition and health-promoting effects of fig (Ficus carica) extracts” in Fig (Ficus carica): Production, processing, and properties. ed. M. F. Ramadan (Cham: Springer), 561–578.
Gol, N. B., Patel, P. R., and Rao, T. V. R. (2013). Improvement of quality and shelf-life of strawberries with edible coatings enriched with chitosan. Postharvest Biol. Technol. 85, 185–195. doi: 10.1016/j.postharvbio.2013.06.008
Grace, O. M., Buerki, S., Symonds, M. R. E., Forest, F., van Wyk, A. E., Smith, G. F., et al. (2015). Evolutionary history and leaf succulence as explanations for medicinal use in aloes and the global popularity of Aloe vera. BMC Evol. Biol. 15, 1–12. doi: 10.1186/s12862-015-0291-7
Gross, K. C., Starrett, D. A., and Chen, H. (1994). Rhamnogalacturonase, α-galactosidase, and β-galactosidase: potential roles in fruit softening. Acta Hortic. 398, 121–130. doi: 10.17660/ActaHortic.1995.398.12
Han, C., Zuo, J., Wang, Q., Xu, L., Zhai, B., Wang, Z., et al. (2014). Effects of chitosan coating on postharvest quality and shelf life of sponge gourd (Luffa cylindrica) during storage. Sci. Hortic. 166, 1–8. doi: 10.1016/j.scienta.2013.09.007
Hassanpour, H. (2015). Effect of Aloe vera gel coating on antioxidant capacity, antioxidant enzyme activities and decay in raspberry fruit. LWT Food Sci. 60, 495–501. doi: 10.1016/j.lwt.2014.07.049
Hssaini, L., Ouaabou, R., Charafi, J., Razouk, R., Houmanat, K., and Irchad, A. (2021). Effects of pre-storage ascorbic and salicylic acids treatments on the enzymatic browning and nutritional quality of dried fig: combined use of biochemical and ATR-FTIR analyses. Vib. Spectrosc. 115:103269. doi: 10.1016/j.vibspec.2021.103269
Kahramanoğlu, İ., Chen, C., Chen, J., and Wan, C. (2019). Chemical constituents, antimicrobial activity, and food preservative characteristics of Aloe vera gel. Agronomy 9:831. doi: 10.3390/agronomy9120831
Kassambara, A., and Mundt, F. (2020). Factoextra: extract and visualize the results of multivariate data analyses. R Package Version 1.0.7.
Kaur, N., Shahwar, D., Hassan, F. E., and Ahmed, Z. F. R. (2023). Antioxidant and antibacterial activities of date palm fruit (Phoenix dactylifera L.) in response to postharvest application with natural elicitors. Acta Hortic. 1364, 187–194. doi: 10.17660/ActaHortic.2023.1364.25
Kaur, N., Somasundram, C., Razali, Z., Mourad, A. H. I., Hamed, F., and Ahmed, Z. F. R. (2024). Aloe vera/chitosan-based edible film with enhanced antioxidant, antimicrobial, thermal, and barrier properties for sustainable food preservation. Polymers 16:242. doi: 10.3390/polym16020242
Kedare, S. B., and Singh, R. P. (2011). Genesis and development of DPPH method of antioxidant assay. JFST 48, 412–422. doi: 10.1007/s13197-011-0251-1
Khadhraoui, M., Bagues, M., Artés, F., and Ferchichi, A. (2019). Phytochemical content, antioxidant potential, and fatty acid composition of dried Tunisian fig (Ficus carica L.) cultivars. JABFQ 92:20. doi: 10.5073/JABFQ.2019.092.020
Khalil, H. A., Abdelkader, M. F. M., Loay, A. A., El-Ansary, D. O., Shaaban, F. K. M., Osman, S. O., et al. (2022). The combined effect of hot water treatment and chitosan coating on mango (Mangifera indica L. cv. Kent) fruits to control postharvest deterioration and increase fruit quality. Coatings. 12:83. doi: 10.3390/coatings12010083
Khaliq, G., Ramzan, M., and Baloch, A. H. (2019). Effect of Aloe vera gel coating enriched with Fagonia indica plant extract on physicochemical and antioxidant activity of sapodilla fruit during postharvest storage. Food Chem. 286, 346–353. doi: 10.1016/j.foodchem.2019.01.135
Khatri, D., Panigrahi, J., Prajapati, A., and Bariya, H. (2020). Attributes of Aloe vera gel and chitosan treatments on the quality and biochemical traits of post-harvest tomatoes. Sci. Hortic. 259:108837. doi: 10.1016/j.scienta.2019.108837
Kim, D. O., Chun, O. K., Kim, Y. J., Moon, H. Y., and Lee, C. Y. (2003). Quantification of polyphenolics and their antioxidant capacity in fresh plums. J. Agric. Food Chem. 51, 6509–6515. doi: 10.1021/jf0343074
Kulka, K., and Sionkowska, A. (2023). Chitosan based materials in cosmetic applications: a review. Molecules 28:1817. doi: 10.3390/molecules28041817
Loay, A. A., and Taher, M. A. (2018). Influence of edible coatings chitosan/PVP blending with salicylic acid on biochemical fruit skin browning incidence and shelf life of guava fruits cv. ‘Banati’. Sci. Hortic. 235, 424–436. doi: 10.1016/j.scienta.2018.03.008
Lufu, R., Ambaw, A., and Opara, U. L. (2020). Water loss of fresh fruit: influencing pre-harvest, harvest and postharvest factors. Sci. Hortic. 272:109519. doi: 10.1016/j.scienta.2020.109519
Maan, A. A., Ahmed, Z. F. R., Khan, M. K. I., Riaz, A., and Nazir, A. (2021). Aloe vera gel, an excellent base material for edible films and coatings. Trends Food Sci. 116, 329–341. doi: 10.1016/j.tifs.2021.07.035
Maioli, A., Gianoglio, S., Moglia, A., Acquadro, A., Valentino, D., Milani, A. M., et al. (2020). Simultaneous CRISPR/Cas9 editing of three PPO genes reduces fruit flesh Browning in Solanum melongena L. Front. Plant Sci. 11:607161. doi: 10.3389/fpls.2020.607161
Mendy, T. K., Misran, A., Mahmud, T. M. M., and Ismail, S. I. (2019). Application of Aloe vera coating delays ripening and extend the shelf life of papaya fruit. Sci. Hortic. 246, 769–776. doi: 10.1016/j.scienta.2018.11.054
Micheloud, N., Gabriel, P., Favaro, J. C., and Gariglio, N. (2023). “Agronomic strategies for fig cultivation cultivation in a temperate-humid climate zone” in Fig (Ficus carica): Production, processing, and properties. ed. M. F. Ramadan (Cham: Springer), 193–214.
Mirshekari, A., Madani, B., Wall, M., and Biggs, A. R. (2020). Aloe vera coatings maintain antioxidants of fig (Ficus carica L.) fruit during storage. Adv. Hortic. Sci. 34, 205–212. doi: 10.13128/ahsc-8255
Nadeem, A., Ahmed, Z. F. R., Hussain, S. B., Omar, A. K., Amin, M., Javed, S., et al. (2022). On-tree fruit bagging and postharvest cold storage maintain the quality of mango fruit. Horticulture 8:814. doi: 10.3390/horticulturae8090814
Nguyen, H. T. T., Do, N. H. N., Lac, H. D., Nguyen, P. L. N., and Le, P. K. (2023). Synthesis, properties, and applications of chitosan hydrogels as anti-inflammatory drug delivery system. J. Porous. Mater. 30, 655–670. doi: 10.1007/s10934-022-01371-6
Nia, A. E., Taghipour, S., and Siahmansour, S. (2021). Pre-harvest application of chitosan and postharvest Aloe vera gel coating enhances quality of table grape (Vitis vinifera L. cv. 'Yaghouti') during postharvest period. Food Chem. 347:129012. doi: 10.1016/j.foodchem.2021.129012
Peian, Z., Haifeng, J., Peijie, G., Sadeghnezhad, E., Qianqian, P., Tianyu, D., et al. (2021). Chitosan induces jasmonic acid production leading to resistance of ripened fruit against Botrytis cinerea infection. Food Chem. 337:127772. doi: 10.1016/j.foodchem.2020.127772
Pinzon, M. I., Sanchez, L. T., Garcia, O. R., Gutierrez, R., Luna, J. C., and Villa, C. C. (2020). Increasing shelf life of strawberries (Fragaria ssp) by using a Banana starch-chitosan-Aloe vera gel composite edible coating. IJFST 55, 92–98. doi: 10.1111/ijfs.14254
Posmyk, M. M., Kontek, R., and Janas, K. M. (2009). Antioxidant enzymes activity and phenolic compounds content in red cabbage seedlings exposed to copper stress. Ecotoxicol. Environ. Saf. 72, 596–602. doi: 10.1016/j.ecoenv.2008.04.024
Rastegar, S., Rahemi, M., Baghizadeh, A., and Gholami, M. (2012). Enzyme activity and biochemical changes of three date palm cultivars with different softening pattern during ripening. Food Chem. 134, 1279–1286. doi: 10.1016/j.foodchem.2012.02.208
Saleem, M. S., Ejaz, S., Anjum, M. A., Ali, S., Hussain, S., Nawaz, A., et al. (2022). Aloe vera gel coating delays softening and maintains quality of stored persimmon (Diospyros kaki Thunb.) fruits. IJFST 59, 3296–3306. doi: 10.1007/s13197-022-05412-5
Sandhu, A. K., Islam, M., Edirisinghe, I., and Burton-Freeman, B. (2023). Phytochemical composition and health benefits of figs (fresh and dried): a review of literature from 2000 to 2022. Nutrients 15:2623. doi: 10.3390/nu15112623
Serrano, M., Castillo, S., Valero, D., Valverde, J. M., Guillén, F., and Martínez-Romero, D. (2017). Effect of Aloe vera gel treatment on bioactive compounds and antioxidant activity during storage of sweet cherry. Acta Hortic. 1161, 607–612. doi: 10.17660/ActaHortic.2017.1161.97
Shah, S., and Hashmi, M. S. (2020). Chitosan–Aloe vera gel coating delays postharvest decay of mango fruit. Hortic. Environ. Biotechnol. 61, 279–289. doi: 10.1007/s13580-019-00224-7
Sharma, S., Prasad, R. N., Tiwari, S., Chaurasia, S. N. S., Shekhar, S., and Singh, J. (2021). Effect of chitosan coating on postharvest quality and enzymatic activity of eggplant (Solanum melongena L.) cultivars. JFPP 45:e15098. doi: 10.1111/jfpp.15098
Shekari, A., Hassani, R. N., Aghdam, M. S., Rezaee, M., and Jannatizadeh, A. (2021). The effects of melatonin treatment on cap browning and biochemical attributes of Agaricus bisporus during low temperature storage. Food Chem. 348:129074. doi: 10.1016/j.foodchem.2021.129074
Sogvar, O. B., Saba, M. K., and Emamifar, A. (2016). Aloe vera and ascorbic acid coatings maintain postharvest quality and reduce microbial load of strawberry fruit. Postharvest Biol. Technol. 114, 29–35. doi: 10.1016/j.postharvbio.2015.11.019
Sun, H., Hao, D., Tian, Y., Huang, Y., Wang, Y., Qin, G., et al. (2022). Effect of chitosan/thyme oil coating and UV-C on the softening and ripening of postharvest blueberry fruits. Food Secur. 11:2795. doi: 10.3390/foods11182795
Velioglu, Y. S., Mazza, G., Gao, L., and Oomah, B. D. (1998). Antioxidant activity and total phenolics in selected fruits, vegetables, and grain products. J. Agric. Food Chem. 46, 4113–4117. doi: 10.1021/jf9801973
Xing, Y., Yang, H., Guo, X., Bi, X., Liu, X., Xu, Q., et al. (2020). Effect of chitosan/Nano-TiO2 composite coatings on the postharvest quality and physicochemical characteristics of mango fruits. Sci. Hortic. 263:109135. doi: 10.1016/j.scienta.2019.109135
Keywords: fig, Aloe vera, chitosan, edible coating, shelf life, sustainability
Citation: Kaur N, Somasundram C, Razali Z and Ahmed ZFR (2024) Sustainable Aloe vera/chitosan-based edible coatings reduce postharvest loss of stored fresh figs (Ficus carica L.). Front. Sustain. Food Syst. 8:1459600. doi: 10.3389/fsufs.2024.1459600
Received: 05 July 2024; Accepted: 15 November 2024;
Published: 27 November 2024.
Edited by:
Barbara Tomadoni, Sevilla University, SpainReviewed by:
María Roberta Ansorena, Universidad Nacional de Mar del Plata, ArgentinaCopyright © 2024 Kaur, Somasundram, Razali and Ahmed. This is an open-access article distributed under the terms of the Creative Commons Attribution License (CC BY). The use, distribution or reproduction in other forums is permitted, provided the original author(s) and the copyright owner(s) are credited and that the original publication in this journal is cited, in accordance with accepted academic practice. No use, distribution or reproduction is permitted which does not comply with these terms.
*Correspondence: Zienab Fawzy Reiad Ahmed, emllbmFiLmFobWVkQHVhZXUuYWMuYWU=
Disclaimer: All claims expressed in this article are solely those of the authors and do not necessarily represent those of their affiliated organizations, or those of the publisher, the editors and the reviewers. Any product that may be evaluated in this article or claim that may be made by its manufacturer is not guaranteed or endorsed by the publisher.
Research integrity at Frontiers
Learn more about the work of our research integrity team to safeguard the quality of each article we publish.