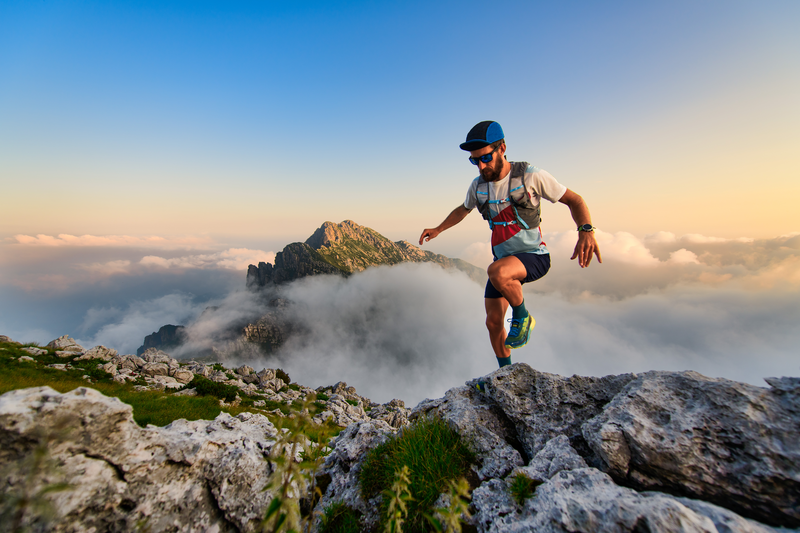
94% of researchers rate our articles as excellent or good
Learn more about the work of our research integrity team to safeguard the quality of each article we publish.
Find out more
REVIEW article
Front. Sustain. Food Syst. , 04 December 2024
Sec. Crop Biology and Sustainability
Volume 8 - 2024 | https://doi.org/10.3389/fsufs.2024.1458552
This article is part of the Research Topic Abiotic Stresses in Field Crops: Response, Impacts and Management under Climate Change Scenario View all 15 articles
Perennial ryegrass (Lolium perenne L.) is a key forage species in warm temperate to subtropical regions worldwide. Climate change poses significant challenges to agriculture, particularly through drought and heat stress, which adversely affect ryegrass yield and may be further exacerbated by global warming. Despite numerous research achievements in recent years, a comprehensive bibliometric analysis of the literature on drought and heat stress in perennial ryegrass is lacking. This study provides a quantitative analysis of relevant literature published from 1994 to 2024, utilizing the Web of Science database to evaluate global research trends and priorities. The results indicate a consistent annual growth in publication output, with China and the United States being major contributors, and the journal Crop Science publishing the most papers. Keyword analysis shows that “growth,” “endophytic fungi,” and “yield” are most frequently used in drought stress research, while “growth,” “gene,” and “leaf” are common in heat stress research. Over the past 30 years, research has mainly focused on phenotype, response mechanisms, and drought and heat resistance techniques in perennial ryegrass. Endophyte have become a hot topic in drought stress research in recent years and have also gained attention in heat stress research, suggesting future research directions in this area. Furthermore, there is a need to strengthen research on the molecular mechanisms associated with drought stress in ryegrass, as well as to explore molecular responses to heat stress. The research trend shows increasing attention to the interaction between drought and heat stress, indicating it will become an important direction for future studies. The findings of this study offer valuable insights for guiding future research on perennial ryegrass under drought and heat stress conditions and provide useful information for researchers in related fields.
Global climate change represents a serious worldwide challenge, with wide-ranging impacts, especially in agriculture. This phenomenon has profoundly affected agricultural systems by altering temperature patterns and water availability (Gong et al., 2020; Leisner, 2020). According to climate change projections, greenhouse gas emissions may lead to a global average temperature rise of 1.5–2°C, which is expected to cause more frequent and prolonged droughts in most crop-growing areas around the world (Naidoo, 2022; Zandalinas et al., 2021). In crop production, rising temperatures not only exacerbate drought problems but also increase the frequency and intensity of heat waves. These factors collectively pose significant and direct threats to ecosystems and agricultural production (Gornall et al., 2010; Mittler, 2006). These impacts not only challenge current agricultural production but also present severe tests for future food security and agricultural sustainability.
In the coming decades, global agriculture is expected to experience more severe impacts of climate change, with increased frequency and intensity of heat and drought events. These stresses could result in crop yield losses of up to 60%, depending onfactors such as the crop type, developmental stage, and the severity, type, and duration of the stress (Jedmowski et al., 2015). Drought affects crop growth by impacting nutrient and water relationships, photosynthesis, and assimilate allocation, ultimately leading to significant decreases in crop yield (Farooq et al., 2009; Praba et al., 2009). The severity of drought-induced damage is often unpredictable, influenced by factors such as rainfall patterns, soil water retention capacity, and water loss due to evapotranspiration (Huang et al., 2024). Similarly, heat stress induces a series of morphological, biochemical, and physiological changes that greatly affect plant growth and development (Anjum et al., 2011). Generally, when temperatures exceed the optimal growth range of plants by 5–10°C, irreversible cellular oxidative damage occurs, especially affecting photosystems I (PSI) and photosystems II (PSII) (Pucciariello et al., 2012; Wahid and Close, 2007; Zhao et al., 2021), leading to delayed growth and wilting (Zhao et al., 2021). Heat stress can also disrupt carbohydrate metabolism and negatively affect critical reproductive processes, including stamen and pollen development, ovule fertilization, fruit set, and seed development, ultimately leading to yield losses in various crops (Kotak et al., 2007; Liu et al., 2019; Ozga et al., 2017; Schauberger et al., 2017; Wahid et al., 2007).
Drought and heat frequently occur simultaneously, and in combination with low atmospheric humidity, drought can increase the vapor pressure deficit (VPD), accelerating the loss of water from both soil and plant tissues. To counteract increased VPD, plants usually close their stomata, which is a conservative strategy but often insufficient to meet the demands of transpirational cooling, resulting in increased tissue and canopy temperatures (Prasad et al., 2008). Similarly, water deficits caused by thermal stress require evaporative cooling to mitigate temperature effects, but under drought conditions, controlling evaporative cooling to prevent water loss makes plants more sensitive to heat stress (Craufurd et al., 2013; Steinmeyer et al., 2013). Due to certain physiological characteristics that adapt to stress, such as canopy temperature, plants may adopt similar regulatory pathways when facing drought and heat stress (Reynolds et al., 2007).
Perennial ryegrass (Lolium perenne L.) is a high-quality forage and turf grass native to Europe, Asia, and North Africa, with a cultivation history of over 100 year (Xie et al., 2020). Due to its ease of cultivation and low maintenance requirements, perennial ryegrass has been widely planted in warm temperate and subtropical regions worldwide (Yu et al., 2021). Fresh perennial ryegrass is palatable and rich in nutrients, benefiting animal digestion and absorption; its hay is rich in crude protein and contains various amino acids, vitamins, and other nutrients, aligning with sustainable animal husbandry practices (Sun et al., 2020; Zhang et al., 2020). In dairy production, perennial ryegrass is superior to many other feeds in terms of corrected milk solids and fat per hectare, and it can tolerate grazing with uniform distribution across different seasons, playing an important role in livestock production (Chapman et al., 2023; Ding et al., 2023; Janke et al., 2015). However, due to its wide geographical distribution and perennial characteristics, perennial ryegrass is highly susceptible to various environmental stresses, including drought and heat. These factors are the main limiting factors affecting its growth and productivity throughout its lifecycle (Huang et al., 2014; Zhou and Abaraha, 2007). During the sowing and seedling stages, autumn drought may prevent normal germination and growth; in the mature stage, heat and drought stress in summer may hinder its survival through the season (Shen et al., 2008; Wang et al., 2016). Drought stress leads to decreased forage quality and biomass, causing premature leaf yellowing and senescence (Rogers et al., 2019). When summer temperatures exceed 38°C, high heat can disrupt the balance between development and growth, leading to accelerated metabolic and physiological changes (Lei and Huang, 2022; Wang et al., 2016). These environmental stresses exceed the optimal growth conditions for perennial ryegrass, resulting in decreased forage yield and quality (Wang et al., 2016). Therefore, addressing global climate change (such as frequent droughts and heats) has become a key challenge in the cultivation and management of perennial ryegrass (Armstead et al., 2006; Mohammadi et al., 2017; Thomas et al., 1999). Therefore, numerous studies have emerged on drought and heat stress in perennial ryegrass, underscoring the importance of synthesizing this growing body of research to identify current trends and future development directions.
Bibliometrics provides theoretical and methodological tools for evaluating the scientific productivity, academic impact, and research frontiers of countries, institutions, or disciplines (Ninkov et al., 2022; Zhang et al., 2024). It integrates mathematics, statistics, and literature studies, focusing on a comprehensive system of quantitative knowledge. By reviewing the history of research in a particular field, bibliometric analysis offers valuable insights into the evolution of research hotspots, the establishment of collaborative networks, and the identification of emerging research directions (Zhang et al., 2024). Compared with traditional literature reviews and meta-analyses, bibliometric analysis can more comprehensively reveal the current status, frontiers, and potential future trends of a given research field (Zhou et al., 2024). However, currently, there are no reports on bibliometric research concerning drought and heat stress in perennial ryegrass. Although there is a substantial body of literature in related fields, the quantitative characteristics, development patterns, and internal relationships of this research remain unclear. To systematically and objectively summarize global research results on drought and heat stress in perennial ryegrass, this study employed mainstream bibliometric software and methods to review research progress across various growth stages of perennial ryegrass between 1994 and 2024. This process provides a deep understanding of the history and current status of drought and heat resistance research in perennial ryegrass and offers guidance for future research directions.
This study conducted a comprehensive search of the Web of Science Core Collection (WoSCC) database. For research on drought in perennial ryegrass, the following search terms were used: (“perennial ryegrass” OR “Lolium perenne L.” OR “Lolium perenne”) AND (“drought” OR “drought tolerance” OR “drought stress” etc.). For studies on heat stress in perennial ryegrass, the search terms were: (“perennial ryegrass” OR “Lolium perenne L.” OR “Lolium perenne”) AND (“heat” OR “heat tolerance” OR “heat stress” etc.). The search period spanned from January 1, 1994, to October 16, 2024. There were no restrictions on language, document type, or data category during the retrieval process. Initial screening of search results based on correlation analysis. The titles, keywords, and abstracts of literature obtained from the search results were checked to determine their relevance to perennial ryegrass. After excluding irrelevant publications, the final selection included English articles and reviews. The literature selection process and the research framework are detailed in Figure 1. A total of 680 publications on drought research of perennial ryegrass and 204 publications on heat stress research of perennial ryegrass were selected and output in text format for further analysis.
VOSviewer software (version 1.6.15) was used to analyze publication types, years, countries, institutions, journal sources, co-cited journals, and keywords co-occurrences. Data were summarized and analyzed using Microsoft Excel 2016, and relevant charts were generated. Clustering analysis was performed with VOSviewer to produce social network maps, where node size indicates the importance or frequency, and the thickness of connecting lines represents the strength of associations. Additionally, the Bibliometrix package in R was utilized to analyze author influence and trends in key keyword changes. This comprehensive approach provided an in-depth understanding of the research progress in the field of drought and heat stress in perennial ryegrass, including various bibliometric indicators such as the number of publications and citation counts.
Core indicators for evaluating academic publications include the number of publications and their citation frequency. Figure 2 shows an increasing trend in the number of publications on drought and heat stress in perennial ryegrass worldwide from 1994 to 2024. Over the past 30 years, the average number of citations per article has steadily increased and then stabilized. This trend may be partly due to newly published articles gradually replacing earlier works, as current research often takes longer to accumulate higher citation counts.
Figure 2. Annual trends in publications and citations related to drought and heat stress in perennial ryegrass (1994–2024). DT: drought stress; HT: heat stress; Mean TC per Art: average total citations per article.
The number of articles in drought research is significantly higher than in heat stress research, indicating that the academic community places greater emphasis on drought studies. The overall number of articles is rising, reflecting increasing academic interest in drought and heat stress in perennial ryegrass. This trend may signify that, as climate change and environmental challenges become more prominent, related research fields are receiving more attention and resource investment.
Figure 3A presents the differences and collaborative trends among countries involved in drought research on perennial ryegrass. Based on data from Supplementary Table S1, the graph shows 33 countries with over five published articles and their collaborative relationships. The United States ranks first globally with 182 published articles, the largest number of nodes, the widest range of domain relationships (the highest TLS), and the highest citation count of 7,658. China follows closely with 127 articles and 2,354 citations. New Zealand ranks third with 80 papers and 1,938 citations.
Figure 3. Knowledge maps of research on perennial ryegrass in countries: (A) drought stress; (B) heat stress.
Figure 3B presents the distribution and cooperation among countries in heat stress research on perennial ryegrass. Using data from Supplementary Table S2, the graph shows 30 countries with over two published articles and their collaborative relationships. The United States leads with 67 published articles, the widest domain relationships (the highest TLS), and 1,973 citations. China, as the second-largest node, has 58 articles and 1,606 citations. New Zealand ranks third with 22 articles.
These findings indicate that both China and the United States have made significant quantitative contributions to drought and heat stress research on perennial ryegrass. However, in drought research, the citation rate of Chinese articles is significantly lower than that of the United States, possibly due to a number of lower-value scientific articles. Therefore, promoting innovative exploration and reducing repetitive research is particularly important.
Figure 4A, along with Supplementary Table S3, shows the literature coupling relationships among institutions in drought research. Research institutions in New Zealand, France, the United States, and China form the core of scientific output. Massey University leads with 31 articles and occupies a central position in the network (the highest TLS). Following is the French National Institute for Agricultural Research (INRA) with 21 articles and Rutgers University with 19 articles. Notably, Aarhus University, Sichuan Agricultural University, Lanzhou University, and the University of Florida, although not in global research hubs, have significant scientific output and collaborations with numerous institutions. However, the depth and closeness of these collaborations need further strengthening.
Figure 4. Institutional collaboration networks in perennial ryegrass research: (A) drought stress; (B) heat stress.
Figure 4B, using data from Supplementary Table S4, presents the inter-institutional literature coupling analysis in heat stress research. Institutions in China, the United States, and Australia are the main contributors. Rutgers University ranks first with 15 articles and serves as a central node (the highest TLS). The Chinese Academy of Sciences and Nanjing Agricultural University each have 13 articles, playing key roles in China’s academic network. The University of Melbourne, Teagasc, and AgResearch Limited have also contributed significantly but need to enhance cooperation intensity with other institutions.
The analysis presented in Figures 3, 4 underscores the dominant roles of China and the United States in research on drought and heat stress in perennial ryegrass. Future research efforts should focus on expanding cross-regional and interdisciplinary collaborations to enhance scientific impact.
Figure 5A and Supplementary Table S5 present a co-citation analysis of 262 journals in drought research. Crop Science is the largest node with 1,516 citations, followed closely by New Phytologist with 1,101 citations and Plant Physiology with 960 citations. The map displays four clusters (red, green, blue, yellow), corresponding to environmental change and agriculture, plant physiology and genetics, microbial-plant interactions, and plant breeding and biotechnology. This demonstrates that drought resistance research covers forage production, breeding and genetics, physiological metabolism, molecular genetics, and biotechnology.
Figure 5. Co-citation knowledge maps of journals publishing research on perennial ryegrass: (A) drought stress; (B) heat stress.
In heat stress research, Figure 5B and Supplementary Table S6 show that Crop Science is the most influential journal with 438 citations, followed by Plant Physiology with 537 citations and the Journal of Experimental Botany with 259 citations. The map divides into five clusters (red, green, blue, yellow, purple), representing plant growth and physiological changes, grass development and utilization, environmental changes and agricultural research, plant physiology and biochemistry, and cell tissue structure. These research results indicate that the study of drought and heat stress on perennial ryegrass covers a wide range of fields, and Crop Science plays a crucial role in this research area.
In drought research, Supplementary Table S7 lists the top 30 most cited papers published in 24 journals. Clay and Schardl (2002) article, cited 887 times in the Web of Science database, is particularly noteworthy. It reveals the role of endophytic fungi in perennial ryegrass, emphasizing how their infection can improve drought tolerance, photosynthetic rate, and growth. Reviewing these articles shows that the first two mainly explored the effects of drought stress on growth and yield. Articles ranked 3–13 focused on physiological and biochemical responses, such as photosynthesis and hormone mechanisms. Articles ranked 14–18 delved into molecular mechanisms. Researchers also examined management strategies under drought stress, such as mixed planting (Article 19) and fertilization (Article 20). In the context of climate change, the symbiotic relationship between endophytic fungi and perennial ryegrass, especially their role in promoting plant growth and enhancing drought resistance, has become a research focus in academia (Articles 21–29). The last paper discussed drought resistance differences among varieties. These studies highlight critical research directions, involving yield, physiological and biochemical reactions, molecular regulation mechanisms, and breeding and cultivation techniques for drought resistance.
In heat stress research, the top 30 most cited articles were published in 18 journals (Supplementary Table S8). Xu et al. (2006), whose paper ranked first with 367 citations, examining physiological and biochemical responses under heat stress. Zhang et al. (2017), cited 202 times, explored melatonin’s biological functions under heat stress and its interaction with plant hormones. Kauffman et al. (2007) investigated plant hormones’ effects on photosynthetic capacity, membrane thermal stability, and heat tolerance. Summarizing these papers reveals that three focused on growth and yield effects under heat stress. Eight highly cited articles studied physiological and biochemical reactions, like osmotic regulation and oxidative stress responses. Sixteen highly cited papers analyzed molecular regulatory mechanisms. Researchers also emphasized agronomic management techniques, such as fertilization. These studies not only reveal the effects of heat stress on plant physiology and molecular levels, but also emphasize the importance of improving crop heat tolerance through agronomic management techniques, providing a scientific basis for addressing climate change.
Figure 6A displays the 20 most frequent keywords. The keyword “drought stress” has the highest frequency at 627 times, followed by “perennial ryegrass” (338 times), “growth” (183 times), “fungal endophyte” (147 times), “yield” (118 times), “CO2” (68 times), “root” (45 times), “leaf” (43 times), and “photosynthesis” (37 times). This indicates the significance of studying drought stress effects on growth and yield, and the interaction mechanisms between endophytic fungi and perennial ryegrass.
Figure 6. Keywords in drought research on perennial ryegrass: (A) most common keywords; (B) co-occurrence network.
The co-occurrence network (Figure 6B) identifies 198 keywords that appeared at least three times, grouped into four clusters: (1) Red cluster focuses on growth and physiological responses, including keywords such as “yield,” “endophytes,” “photosynthesis,” “drought survival strategies,” “physiological responses.” (2) Green cluster centers on plant hormones and molecular research, featuring keywords like “abscisic acid,” “hormone metabolism,” “antioxidant enzymes,” “Arabidopsis,” “gene expression,” “molecular identification.” (3) Blue cluster emphasizes breeding-related topics, including “interspecific hybridization,” “breeding,” and “genotype.” (4) Yellow cluster concentrates endophytes and stress responses, such as “fungal endophytes,” “seed germination,” and “environmental pressure.” This network analysis offers a comprehensive understanding of key themes. Based on Supplementary Table S7, current drought resistance research focuses on phenotype, response mechanisms, and tolerance enhancement strategies.
Figure 7A shows the 20 most frequent terms in heat stress research. “Heat stress” has the highest frequency at 141 times, followed by “perennial ryegrass” (69 times), “growth” (31 times), “gene” (20 times), “leaf” (20 times), “yield” (18 times), “Arabidopsis” (18 times), and “water-use” (16 times). This underscores the importance of studying heat stress effects on growth and molecular mechanisms.
Figure 7. Keyword analysis in heat stress research on perennial ryegrass: (A) most common keywords; (B) co-occurrence network.
The co-occurrence network (Figure 7B) identifies 84 keywords that appeared at least twice, grouped into five clusters: (1) Red cluster focuses on physiology, biochemistry, molecular mechanisms, and breeding, including keywords like “abscisic acid,” “Arabidopsis,” “genes,” “heat shock proteins,” and “antioxidant enzymes.” (2) Green cluster centers on plant growth under climate change, featuring keywords like “climate change,” “CO2,” “perennial ryegrass,” “cell membrane stability,” and “germination.” (3) Blue cluster highlights endophytic fungi and organic compounds, such as “endophytic fungi,” “alkaloids,” “ergot alkaloids,” and “chemical composition.” (4) Yellow cluster focuses on plant organic components, including “carbohydrate reserves,” “nutritional value,” and “plant components.” (5) Purple cluster features keywords related to “Temperature and humidity index.” Based on Supplementary Table S8, heat stress research emphasizes phenotype, response mechanisms, and tolerance enhancement strategies.
Figure 8A shows the analysis for drought stress from 1994 to 2024, divided into three periods: 1994–2004, 2005–2014, and 2015–2024. In the initial stage (1994–2004), six key themes were identified, with “gene expression” being the most relevant and persistent across all periods. In the second period (2005–2014), themes like “drought stress,” “yield,” “abscisic acid,” “oxidative stress,” “protein,” and “molecular characterization” emerged. “Drought stress” and “yield” showed high correlation, indicating a strong link between drought stress research and yield changes. In the third period (2015–2024), an increase in keyword variety indicates broader research fields. Notable keywords include “fungal endophyte,” “germination,” and “gene expression.” The shift from phenotypic studies to physiological, biochemical, and drought resistance improvement highlights gene expression as a consistent focus. The emergence of “fungal endophyte” suggests a future research direction. Current frontiers in drought resistance research include gene expression and endophytic fungi.
Similarly, Figure 8B presents heat stress research evolution. In the initial stage, key themes were “yield,” “perennial ryegrass,” and “CO₂.” In the second period, themes like “heat stress,” “oxidative stress,” “gene,” and “phenolic acids” emerged, with “heat stress” highly correlated with these words. In the third period, new themes like “fungal endophyte,” “Arabidopsis,” and “climate change” appeared, indicating that “fungal endophyte” may become a future focus.
The challenges posed by global climate change continue to escalate, particularly the sustained rise in environmental temperatures, which poses a serious threat to food security and crop production (Basavaraj and Rane, 2020; Shah et al., 2017). Extreme weather events such as droughts and heat waves are expected to become more frequent and intense, serving as major factors limiting the growth, geographical distribution, and yield of perennial ryegrass (Pan et al., 2018; Wang et al., 2017; Yu et al., 2013). Therefore, understanding the effects of drought and heat stress on perennial ryegrass, current research progress, and future research directions is crucial for improving its cultivation management and yield (Liu and Jiang, 2010; Yu et al., 2015). In this study, we employed bibliometric methods to analyze all English academic publications, including articles and reviews, on drought and heat stress in perennial ryegrass over the past 30 years. The bibliometric analysis shows that research in this field mainly focuses on three aspects: the effects of drought and heat stress on phenotypic changes in perennial ryegrass growth, potential response mechanisms, and studies on drought and heat resistance technologies.
The development of seeds and the growth of seedlings depend on suitable soil conditions, which vary depending on the plant type and include factors such as humidity and temperature levels (Reed et al., 2022). Drought and rising soil temperatures often have negative effects on the germination of crop seeds, involving complex physiological metabolic activities such as enzyme activity and cell division within the seeds (Huchzermeyer et al., 2022; Wafa’a, 2010). For perennial ryegrass, sufficient water is crucial for seed germination; water not only promotes enzyme absorption and activation but, when lacking, leads to delayed germination, thereby affecting the success rate of the entire germination process (Xiong et al., 2024). Additionally, different varieties of perennial ryegrass exhibit significant differences in drought resistance. For example, a study comparing the germination of four varieties under drought conditions showed that the “Solstice II” variety exhibited superior germination and seedling growth abilities under drought stress (Yilmaz and Kisakurek, 2021). Heat stress can also reduce enzyme activity and interfere with metabolic processes related to seed germination (Iqbal et al., 2024). High soil temperatures intensify transpiration, reduce the effectiveness of seed moisture, and thus delay the germination process (Zou et al., 2023). The ideal growth temperature range for perennial ryegrass is 15–24°C; beyond this range, an increase in temperature creates heat stress, limiting its application in temperate regions. Under heat stress conditions, seedling characteristics of perennial ryegrass—such as relative germination rate, germination index, vitality index, and root and seedling length—generally show a downward trend. When the temperature rises to 35°C, the germination rate sharply decreases, and the inhibitory effect of heat stress on root and stem growth becomes increasingly evident (Javaid et al., 2022). Moreover, the combined effect of drought and heat stress severely inhibits the normal physiological functions of perennial ryegrass seedlings, leading to wilting (Rahman et al., 2022).
Drought and heat stress adversely affect the growth and developmental stages of perennial ryegrass, leading to significant decreases in yield. Under such environmental pressures, perennial ryegrass exhibits notable phenotypic adaptive changes to address these challenges. Under drought stress, the lack of soil moisture directly affects plant growth and development. When perennial ryegrass experiences drought stress, its root water absorption capacity is hindered, disrupting the balance between root water uptake and canopy transpiration. As drought stress intensifies, parameters such as leaf relative water content, dark respiration rate, wilting coefficient, tiller number, and biomass of both aboveground and root systems show decreasing trends (Patel et al., 2015). Studies have shown that under severe water scarcity, drought stress can reduce the yield of fresh grass and hay by 45 and 28%, respectively (Shariatipour et al., 2022), decrease the annual harvest frequency from four to three, and reduce ryegrass biomass by up to 79% (Kemesyte et al., 2017). Water shortages in arid environments significantly alter plant morphology, revealing their drought resistance potential and water-use efficiency to some extent (Su et al., 2007). Faced with drought stress, plants typically reduce excessive water consumption by decreasing aboveground biomass while correspondingly increasing underground biomass. This strategic redistribution of biomass helps roots absorb water more effectively, thereby enhancing plant adaptability to drought stress (Huang et al., 2014; Yang et al., 2014). The root system, as a key organ for water and nutrient uptake and soil resource utilization, plays a crucial role in growth and biomass accumulation and is the first part to perceive and respond to water deficits (Wasaya et al., 2018). Under drought conditions, perennial ryegrass resists stress by forming smaller and thicker leaves and expanding the total surface area of its roots to absorb more water. Even in extreme drought conditions, increases in root length and area reveal special adaptive strategies adopted by plants in arid environments, even though root deformities and reduced numbers may occur (Jones et al., 1980).
Under heat stress conditions, when perennial ryegrass plants are subjected to high temperatures above 35°C, this extreme temperature can directly lead to plant death; leaf color changes from emerald green to withered yellow, ultimately turning brown (Chen et al., 2023; Rahman et al., 2022). Heat stress can significantly deteriorate the growth status of ryegrass plants, initially manifested as damage at the junction of the penultimate and antepenultimate leaf sheaths. Simultaneously, some leaves begin to wilt, and their color gradually turns yellow from the base to the tip, followed by damage symptoms on lodging and aging leaves. If stress persist, these injuries become irreversible, ultimately leading to decreased forage coverage (Kauffman et al., 2007; Lei et al., 2022; Yang et al., 2014). During the maturation stage, heat stress can significantly reduce the effective number of spikes per unit area. It hinders the vegetative growth of ryegrass and inhibits material transport, leading to significant decreases in pollen grain weight and protein content, seriously affecting reproductive growth. This results in reduced grain numbers per ear, affecting grain plumpness and thousand-grain weight, and ultimately decreasing yield (Jung et al., 2021). To adapt to high-temperature environments, plants cope with heat stress through a series of complex morphological adjustments. Under heat stress, plants may exhibit specific thermomorphogenic traits such as elongation of hypocotyls and petioles, growth of hypocotyl leaves, and early flowering, which contribute to enhancing photosynthesis and reproductive capacity under heat stress (Kan et al., 2023). Heat stress also leads to an increase in specific leaf area, while leaf thickness, cell layers, and chlorophyll content decrease accordingly. Heat stress intensify transpiration in leaves, increasing the risk of water loss. Therefore, plants may develop smaller and denser stomata to reduce water loss caused by transpiration (Higuchi et al., 1999). Perennial ryegrass enhances its heat tolerance through adaptive changes such as reducing plant height, narrowing leaf width, shortening leaf length, and reducing leaf area (Yang et al., 2024).
In arid environments, the diffusion of carbon dioxide through stomata and mesophyll tissues is limited, leading to a decrease in CO2 concentration within photosynthetic cells and subsequently reducing carbon fixation (Chaves et al., 2009). Under high-temperature conditions, both carbon fixation and photoreactions are adversely affected. The carbon-fixing enzyme ribulose-1,5-bisphosphate carboxylase/oxygenase (Rubisco) has a dual function, but its specificity for CO2 decreases with increasing temperature. This change enhances photorespiration, which not only consumes energy but also releases CO2, thereby reducing the efficiency of net photosynthesis (Moore et al., 2021). The chlorophyll content in leaves is directly related to photosynthetic potential and reflects the plant’s adaptability to drought stress. Drought stress leads to gradual loss of leaf water and stomatal closure, weakening the plant’s ability to absorb CO2. This results in the deactivation of the PSII reaction center, affecting the transfer of photosynthetic electrons and energy conversion, ultimately reducing overall photosynthetic efficiency (Gunasekera and Berkowitz, 1993; Medrano et al., 2010). Drought stress also increases the non-photochemical quenching of chlorophyll fluorescence (NPQ) by 15 to 47%, which helps protect PSII reaction centers from damage caused by excess energy (Koscielniak et al., 2006). Research has shown that under drought stress, the ratio of chlorophyll a to chlorophyll b increases, which helps ensure the electron supply to PSII and aligns with the excitation rate of PSI (Ashraf et al., 2011).
Under heat stress, the chlorophyll content of ryegrass decreases, relative conductivity increases, electrolyte leakage rate rises, and stomatal conductance, instantaneous water-use efficiency, and light energy-use efficiency decrease (Zhang et al., 2020). The ultrastructure of leaf cells undergoes significant changes, including chloroplast expansion, structural damage, morphological alterations, and an increase in the number of mitochondria and lipid bodies to adapt to high-temperature environments (Xu et al., 2006). Additionally, perennial ryegrass slows down chlorophyll degradation by inhibiting genes involved in chlorophyll catabolism, such as chlorophyll-a oxygenase, thereby delaying heat-induced leaf senescence (Jespersen et al., 2016; Zhang et al., 2019; Zhang et al., 2016). Studies have shown that inhibiting genes involved in chlorophyll degradation, such as pheophorbide a oxygenas, can effectively slow down the aging process of perennial ryegrass leaves under heat stress (Zhang et al., 2019). Chlorophyll a is crucial for enhancing heat tolerance, and reducing the expression of the chlorophyll catabolism gene LpNOL or applying chlorophyll derivatives can inhibit heat-induced leaf senescence (Yu et al., 2022; Zhang et al., 2019). Chlorophyll fluorescence, as a sensitive physiological indicator, can accurately reflect the maximum quantum efficiency (Fv/Fm) of PSII. Maintaining high chlorophyll content and fluorescence levels can help improve photosynthetic efficiency and enhance plant heat tolerance (Pan et al., 2018; Zhu et al., 2022). Moreover, the combined effects of drought and heat stress on photosynthesis are believed to be synergistic, as stomatal closure not only limits carbon fixation but may also lead to excess energy, exacerbating photodamage in the photosystems (Sato et al., 2024).
Under drought and heat stress, oxidative stress indicators—such as reactive oxygen species (ROS), hydrogen peroxide, and lipid peroxidation—in perennial ryegrass significantly increase, especially under combined stress conditions (Rahman et al., 2022). In chloroplasts, the reaction centers of PSI and PSII are the main sites for ROS generation; when excess light energy is present, they generate superoxide (Edreva, 2005). Mitochondria are another source of ROS production, where O2− is produced through electron leakage from complexes I and III under abiotic stress conditions (Choudhury et al., 2017). Due to the similar processes inducing ROS production under drought and heat stress, their combination synergistically increases ROS levels (Li et al., 2014). To prevent oxidative damage, plants activate ROS-scavenging mechanisms, including enzymes such as superoxide dismutase (SOD), catalase (CAT), ascorbate peroxidase, and glutathione peroxidase, as well as antioxidants like ascorbic acid and glutathione (Kuzniak, 2002). Drought and heat stress typically trigger these defense processes, but different stress conditions lead to variations in ROS levels and the induction of ROS detoxification genes, indicating specific ROS responses under specific stress combinations (Choudhury et al., 2017; Zandalinas et al., 2018). Under drought conditions, perennial ryegrass adapts by increasing the activity of SOD and CAT (Fu and Huang, 2001). Zhang et al. (2015) found that under drought treatment, resistant varieties had lower malondialdehyde (MDA) content and less cell membrane damage. To resist oxidative stress under heat stress, plants activate their antioxidant defense systems (Hamilton et al., 2012), where SOD and CAT prevent MDA accumulation, balance free radical production and scavenging, and enhance plant heat tolerance (Huang et al., 2014; Xu et al., 2011). Under adverse stresses like drought and heat, the activities of peroxidase (POD), SOD, and CAT in ryegrass leaves are enhanced, promoting ROS metabolism balance in cells and improving cell membrane stability, thereby alleviating stress-induced damage (Wang et al., 2022; Wang et al., 2019).
Under drought and heat stress, plant cells maintain osmotic balance by regulating substances such as proline, soluble sugars, and proteins. Studies have found that MDA content in different forage is positively correlated with stress degree, reflecting cell membrane damage; increased proline content enhances the drought tolerance of ryegrass (Chen and Murata, 2011; Jafari et al., 2019). As an osmotic regulator, soluble sugars increase under drought stress, helping plants adapt to arid environments. Under extreme drought conditions, increasing the content of hexose and sucrose in leaf sheaths reduces osmotic potential and enhances drought resistance in perennial ryegrass (Karsten and Macadam, 2001) Perennial ryegrass resists heat stress by maintaining high levels of proline and soluble sugar content (Sun et al., 2020). By maintaining appropriate levels of carbohydrates, including soluble sugars and fructooligosaccharides, plants protect their photosynthetic apparatus from thermal damage, maintain osmotic balance and membrane stability, and slow down leaf senescence (Wang and Xiong, 2016).
Recent studies have shown that endogenous and exogenous plant hormones play important roles in mitigating damage caused by drought and heat stress. Hormones such as abscisic acid (ABA), cytokinins (CK), and melatonin (MT) are key in the drought and heat resistance mechanisms of perennial ryegrass. ABA, as a key hormone induced by drought, regulates numerous drought-responsive genes and occupies a central position in abiotic stress signaling (Choi et al., 2000). MT pretreatment significantly slowed the decline in forage quality, photochemical efficiency, and relative water content of perennial ryegrass under drought stress, as well as the increase in relative conductivity and MDA content. MT maintains cell membrane stability by enhancing the activity of SOD, CAT, and peroxidase (Li and Du, 2019).
Under heat stress, ABA content increases, inducing the expression of resistance genes, reducing ROS damage to cell membranes, protecting plants from photoinhibition, and enhancing high-temperature tolerance (Abro et al., 2023). Cytokinins enhance the activity of membrane-protective enzymes like SOD, eliminate free radicals, reduce lipid peroxidation, maintain membrane fatty acid ratios, and protect cell membrane integrity (Wang, 2000). Maintaining stable levels of indole-3-acetic acid (IAA) and salicylic acid (SA), as well as delaying the increase of ABA and the decrease of gibberellin (GA3), can help improve plant heat tolerance (Li et al., 2020). Cytokinin and ethylene inhibitors alleviate premature leaf senescence under heat stress by upregulating the expression of LpWRKY69 and LpWRKY70 (Chen and Huang, 2022). In perennial ryegrass treated with MT, the expression of ABA synthesis pathway genes is inhibited, while the expression of CK synthesis and signaling pathway genes is upregulated, inhibiting heat-induced leaf senescence (Zhang et al., 2017). Exogenous MT application alleviates growth inhibition and leaf senescence caused by heat stress, manifested by increased tiller number, plant height, leaf chlorophyll content, and decreased transcription levels of senescence-related genes LpSAG12.1 and Lph3 (Zhang et al., 2017).
When plants face drought stress, they activate a series of complex molecular coping strategies involving numerous genes and signaling pathways. Sensors such as G protein-coupled receptors (GPCRs) and receptor-like kinases (RLKs) on the cell membrane detect external drought signals and trigger the expression of drought-responsive genes through signal transduction networks. In this chain reaction, secondary messengers like Ca2+, ROS, ABA, and transcription regulators play crucial roles (Mahmood et al., 2020). For example, GmCIPK2 enhances plant drought resistance by increasing ABA content and expression of drought-related genes, playing a role in ABA-mediated stomatal closure (Xu et al., 2021). The MAP3K18 kinase activated by ABA can directly interact with SnRK2.6 kinase and PP2C phosphatase ABI1, participating in the regulation of stomatal opening and closing in Arabidopsis and improving plant drought resistance (Tajdel et al., 2016). OsCIPK23 RNAi transgenic plants show high sensitivity to drought (Li et al., 2014); MAPKs activate SnRK2s through phosphorylation, regulating the expression of drought stress response genes (Mahmood et al., 2020). In combating drought stress, perennial ryegrass adopts complex regulatory strategies, including significantly enhanced expression of transcription factors, kinases, and E3 ubiquitin ligases, promoting ABA and stress signal transduction (Wang et al., 2023). Amiard et al. (2003) found that inositol-1-phosphate synthase (INPS) and galactinol synthase (GOLS) play key roles in regulating the content of raffinose and stachyose under drought stress. Foito et al. (2009) identified functional genes regulated under drought stress through metabolomics and transcriptomics analysis. The cytochrome P450 gene LpCYP72A15, as a potential gene for osmotic stress tolerance, demonstrates excellent antioxidant and osmoregulatory abilities, effectively enhancing drought tolerance in ryegrass (Xing et al., 2024). In arid environments, adaptive variations of genotypes such as “Accelerate,” “Icon,” and “Haymaker” form an important foundation for ryegrass drought tolerance (Majeed et al., 2023). Genome-wide association studies (GWAS) have shown that under drought stress, late embryogenesis abundant protein 3 (LpLEA3) and superoxide dismutase (LpFeSOD) play crucial roles in maintaining leaf water balance (Yu et al., 2013).
Determining the role of drought-responsive genes in perennial ryegrass adaptation through genetic transformation is significant for elucidating its drought resistance mechanisms. Transcription factors are regulatory proteins that respond to drought stress by modulating gene expression at the transcriptional level. Plants activate or inhibit specific transcription factors through MAPK and CDPK cascade signal transduction pathways, affecting cis-regulatory elements of stress-related genes to enhance or inhibit their transcription. These transcription factors include MYB, NAC, WRKY, and ERF/DREB, playing important roles in plant responses to drought stress (Baillo et al., 2019). Genes responsible for transcriptional regulation, ROS scavenging, and osmoregulation of soluble sugars and proline in perennial ryegrass seedlings (such as DREB, NAC, MYB) are crucial under drought stress (Demirkol et al., 2023; Liu and Jiang, 2010). In Arabidopsis, overexpression of the bZIP transcription factor AREB1 increases sensitivity to ABA and enhances drought tolerance (Fujita et al., 2005). Previous studies have shown that transgenic perennial ryegrass overexpressing LpHIB1 exhibits better drought tolerance, including higher relative water content, leaf water potential, chlorophyll content, and photosynthetic rate, indicating that HUB1 may play an important role in abiotic stress tolerance (Patel et al., 2015). Recent research shows that expressing the rice miR408 gene in perennial ryegrass through genetic modification can enhance drought tolerance, possibly related to leaf morphology adjustment and improved antioxidant capacity (Hang et al., 2021). Additionally, LpP5CS (pyrroline-5-carboxylate synthase) plays a crucial role in various stress responses and is a potential candidate gene for stress-related molecular breeding in perennial ryegrass. Overexpression of LpP5CS in tobacco plants, especially the mutant form LpP5CS^F128A not inhibited by proline feedback, enhances tolerance to drought stress (Cao et al., 2015). Using a yeast ectopic expression system, LpSAPK9 was identified as a candidate positive regulatory factor for drought tolerance. LpSAPK9 showed distinct expression changes in drought-tolerant ryegrass varieties, indicating that its expression level is related to drought tolerance. These results contribute to further analysis of LpSAPKs for molecular breeding of ryegrass and related grass species (Xing et al., 2022).
Identifying key regulatory genes affecting heat tolerance and exploring their mechanisms of adaptation provides references for improving heat tolerance in perennial ryegrass. The process of plant response to heat stress is complex, involving interactions among numerous transcription factors and signaling molecules, forming a regulatory network (Zhang et al., 2020). The homeodomain leucine zipper (HD-Zip) transcription factor family plays an important role in regulating plant development and responding to abiotic stresses, including heat stress. RT-PCR results showed that the expression levels of LpHOX6, LpHOX8, and LpHOX24 (HD-Zip I transcription factors) in perennial ryegrass were negatively correlated with heat tolerance, while LpHOX21 expression was positively correlated (Wang et al., 2019). Dai et al. (2017) found through sequencing and methylation analysis using sodium bisulfite that the P450 gene LpCYP72A161 in perennial ryegrass is epigenetically regulated under heat stress. Zhang et al. (2020) conducted heat tolerance identification and molecular marker experiments on 98 varieties and found that four chlorophyll catabolism genes (LpNYC1, LpNOL, LpSGR, and LpPPH) were closely related to heat tolerance. Additionally, heterologous expression of perennial ryegrass LpHSFC1b in Arabidopsis can significantly improve heat resistance in transgenic Arabidopsis (Sun et al., 2020). This study provides new insights into the epigenetic regulation of perennial ryegrass genes under heat stress.
In addition to transcriptional regulation, post-transcriptional and post-translational regulations play important roles in plant responses to heat stress. MicroRNAs (miRNAs) participate in heat stress responses by regulating transcription factor activity. Under high-temperature conditions, miRNA398 in Arabidopsis promotes the accumulation of heat shock transcription factors (HSFs) by inhibiting three ROS-scavenging enzymes, thereby enhancing heat tolerance (Guan et al., 2013). Overexpression of miRNA160 in Arabidopsis can inhibit ARF10, ARF16, and ARF17, activate a series of downstream heat shock protein (HSP) genes, and enhance heat tolerance (Lin et al., 2018). The expression of HSFA1 in Arabidopsis is also feedback-regulated by small RNAs. miR398 induced by HSFA1 inhibits the expression of ROS-scavenging genes CSD1 (copper/zinc superoxide dismutase 1), CSD2, and CCS1 (copper chaperone of SOD1), leading to excessive ROS accumulation, positively regulating HSFA1 expression and ultimately affecting heat tolerance (Guan et al., 2013). Studies have also found that DREB2A remains stable under high-temperature stress through SUMO modification, helping improve heat tolerance (Wang et al., 2020).
Plants exposed to high temperatures and/or drought produce excessive free radicals (O2−, H2O2, OH−), leading to cell damage and oxidative stress, which inhibit crucial cellular and metabolic processes (Hussain et al., 2019). These combined effects can slow plant growth and even cause wilting or death. Genetic and genomic studies have shown that many proteins and antioxidant genes are involved in plant responses to heat and drought stress (Al Khateeb et al., 2020; Bourgine and Guihur, 2021). These candidate proteins and genes protect plants from abiotic stress through cellular homeostasis, metabolic protein regulation, signal transduction, and antioxidant defense (Rahman et al., 2021; Wang et al., 2004). For example, overexpression of the TaHsfA6f gene in Arabidopsis significantly enhances tolerance to heat and drought (Bi et al., 2020). Li et al. (2014) analyzed the GmHsf family in soybean and found that Arabidopsis plants overexpressing GmHsf-34 exhibited stronger heat and drought tolerance. Ma et al. (2022) identified and characterized 16 putative Hsf genes through whole-genome bioinformatics analysis, including eight HsfA, five HsfB, and three HsfC in ryegrass. These genes mostly respond to early heat stress and also to late drought stress. Functional analysis of LmHSFA5, induced by high temperature and drought, showed that it may positively regulate heat and drought tolerance by directly activating the expression of LmHSP18.2 and LmAPX2 in ryegrass. Rahman et al. (2022) investigated the transcriptional response of HSP and antioxidant genes in perennial ryegrass under combined heat and drought stress. The study showed that oxidative stress indicators significantly increased under combined stress, while HSP70, HSP90-6, and mitochondrial small HSP26.2 showed high expression levels. Additionally, up to 90% of Hsfs in sesame have drought responsiveness (Dossa et al., 2016), and ZmHSF06 has a positive regulatory effect on drought tolerance in Arabidopsis (Li et al., 2015). Jiang et al. (2016) compared responses and gene expression differences of two perennial ryegrass varieties during deficit irrigation and growth recovery. The results showed significant differences in transcription levels of heat shock cognate protein 70 (HSC70), iron superoxide dismutase (FeSOD), and plasma membrane intrinsic protein 1 (PIP1) in leaves and stems, providing important evidence for further exploration of molecular mechanisms in drought resistance.
To resist heat stress, HSPs are induced and act as molecular chaperones to prevent protein denaturation, a process controlled by heat stress transcription factors. In the HSF gene family, the LpHSFC1b gene is significantly induced after heat treatment and is a core regulatory gene (Scharf et al., 2018). The FaHSFC1b gene of tall fescue and the LpHSFC1b gene of perennial ryegrass have been confirmed to be involved in plant heat stress responses. Overexpression of these genes can enhance heat tolerance in transgenic Arabidopsis and promote the expression of HSFA genes, HSP genes, and ABA synthesis-related gene NCED (Sundaram and Rathinasabapathi, 2010; Zhuang et al., 2018). Arabidopsis transformed with the LpHSFC1b gene showed decreased electrolyte leakage and MDA content, as well as significantly increased expression of heat stress response genes (Sun et al., 2020). RNA-seq analysis showed that the HSFA and HSFB subfamilies in ryegrass are closely related to heat tolerance (Yang et al., 2012). After 10 h of high-temperature treatment, about 25% of gene expression in ryegrass leaves showed significant changes, with genes A2, A3, A4, A6, and A9 in the HSFA subfamily and B genes in the HSFB subfamily significantly upregulated, indicating the important role of HSFA and HSFB in heat stress adaptation (Wang et al., 2017).
Under drought stress, the water absorption capacity of perennial ryegrass roots may be impaired. However, increasing the root-to-shoot ratio can enhance root water uptake, effectively mitigating the negative effects of drought stress. Adopting appropriate cultivation and management strategies can significantly improve the drought resistance of ryegrass. Although drought stress typically restricts ryegrass growth, appropriate fertilization measures can promote biomass accumulation (Ponce et al., 1993). For instance, applying suitable concentrations of potassium fertilizer in arid environments can significantly improve seed germination rates, increase leaf thickness, and enhance the root-to-shoot ratio, thereby bolstering drought resistance (Hoffman et al., 2010). The application of nitrogen fertilizer has also been shown to significantly increase the dry matter yield of ryegrass and improve feed quality (Abraha et al., 2015). Additionally, the use of silicates can alleviate physiological changes in ryegrass under drought stress, while plant growth regulators can reduce the rate of quality loss under such conditions (Hahn et al., 2008; Mahdavi et al., 2016). Spraying uniconazole has been found to reduce plant height, promote tillering, and improve stress resistance; foliar application of uniconazole enhances the drought resistance of ryegrass to varying degrees under drought stress (Ma et al., 2009). The application of rice husk biochar (RHB) improves shoot and root growth, increases chlorophyll content, and enhances photosynthetic efficiency, thereby augmenting drought tolerance (Safari et al., 2023). Methyl jasmonate (MeJA) treatment increases endogenous indole-3-acetic acid (IAA) and MeJA levels in ryegrass under drought stress, contributing to increased biomass and improved drought resistance (Wei et al., 2024). Adding sludge to the soil can significantly improve growth rates and osmotic regulation under drought stress, enhance photosynthesis and water-use efficiency, and ultimately strengthen drought resistance (Bermanec et al., 2016).
In summer, moderate application of nitrogen fertilizer can meet the energy needs of plants to resist high temperatures. Adequate phosphorus fertilization helps plants absorb water from deeper soil layers, supplementing the insufficient surface moisture caused by high temperatures. Increasing potassium fertilizer application can enhance the heat resistance of forage, possibly because potassium promotes photosynthesis, accelerates protein and starch synthesis, compensates for excessive consumption of organic matter under high temperatures, and slows the aging process of forage (Gong et al., 1998). The application of plant growth regulators can effectively improve the heat resistance of ryegrass, enabling it to survive hot summer days safely. For example, the use of chemicals such as paclobutrazol (PP333) can enhance plant heat resistance. Xiong et al. (2006) found that spraying PP333 at different concentrations on perennial ryegrass significantly reduced leaf emergence rates compared to the control group, while increasing the accumulation of free proline, relative leaf water content, soluble protein content, and enhancing the activities of ascorbic acid, peroxidase, and nitrate reductase. These changes work together to significantly enhance the heat tolerance of perennial ryegrass.
Perennial ryegrass forms mutually beneficial symbiotic relationships with endophytic fungi. The grass provides space and essential nutrients for the fungi, while endophytic fungi play key roles in promoting plant growth and enhancing resistance to biotic and abiotic stresses (Clay and Schardl, 2002; Gao et al., 2024; Zhao et al., 2024a). The impact of endophytic fungi on the resistance of perennial ryegrass has become an important research topic internationally. Numerous studies have shown that endophytic fungal infections can enhance the drought tolerance of perennial ryegrass (Kane, 2011), reduce damage caused by stress (Deng et al., 2020), and influence tillering (Ravel et al., 1997) and physiological responses. Endophytic fungi enhance the drought resistance of the host by boosting its osmotic regulation and reactive ROS scavenging systems (Ma et al., 2020). Hesse et al. (2003) reported that under drought conditions, endophytic fungi increased the root-to-shoot ratio and root dry weight of ryegrass; subsequent studies found that they significantly increased seed yield.
Endophytic fungi also play crucial roles in plant growth and resistance to environmental stresses like heat stress. They effectively eliminate harmful ROS by inducing antioxidant enzyme production, thereby enhancing the plant’s antioxidant defense system. Endophytes can regulate plant hormone levels, promote the synthesis of ABA, and enhance heat tolerance (Ding et al., 2024; Sahu et al., 2022). Research has found that inoculation with Aspergillus oryzae significantly enhances the heat and drought resistance of perennial ryegrass. After treatment with Aspergillus nidulans, ABA content in ryegrass leaves significantly increased, while salicylic acid content decreased (Li et al., 2021; Wei et al., 2023b). Inoculation with arbuscular mycorrhizal (AM) fungi can enhance the antioxidant defense capacity and hormone levels of ryegrass under heat stress. Wei et al. (2023a) found that high-temperature stress significantly inhibited the growth of perennial ryegrass. However, inoculation with AM fungi combined with melatonin treatment upregulated the expression of melatonin synthesis genes in the ryegrass root system, increased endogenous melatonin content, enhanced antioxidant enzyme activity, reduced oxidative damage, and improved osmotic regulation ability, thereby enhancing heat tolerance (Zhao et al., 2022).
Cultivating drought-resistant varieties is fundamental to improving plant drought tolerance. With the development of modern biotechnology, breeding research on perennial ryegrass has gained new opportunities and methods. Traditional breeding techniques, such as introduction, hybridization, and selection, have long improvement cycles and limited genetic material (Ahmar et al., 2020). Advances in biotechnology have provided new possibilities for cultivating drought-tolerant varieties.
Introduction is an important method for developing ryegrass production by bringing in excellent varieties from abroad or other regions, conducting adaptability assessments, and evaluating their suitability for promotion in specific areas. Among the 29 ryegrass varieties introduced by Huang et al. (2008) from Germany, “Taurus,” “Barfort,” “Gemini,” “Fastyl,” and others showed good adaptability in Sichuan; “Defo,” “Prestyl,” “Pomerol,” and others provided excellent genetic material for breeding new ryegrass varieties, accelerating the breeding process. “Winter Pasture 70” ryegrass exhibits characteristics of drought tolerance, low fertility requirements, and strong stress resistance, with yields surpassing those of Italian ryegrass and perennial ryegrass (Liu et al., 2006). Through introduction, excellent germplasm resources can be obtained. Wild and cultivated ryegrass germplasm resources can be collected and sorted, and through domestication experiments and selective breeding, valuable parents can be provided for breeding new varieties.
As a strictly cross-pollinated plant, perennial ryegrass may achieve trait improvements through mixed selection and single-plant selection methods; cyclical selection can improve ryegrass populations (Sheng and He, 2003). In an experiment conducted by Mo et al. (2009) in Guizhou, excellent plants were selected from the seed breeding population of American varieties. After repeated selection and improvement, the high-yield and high-quality “Guicao No. 1” Italian ryegrass was bred. A comparative study on the drought tolerance of nine perennial ryegrass varieties found that the dry matter content of the Norwegian germplasm was 50% higher than that of the second-best performing germplasm. After six drought cycles, the dry matter content was more than seven times higher than that of the reference variety “Grasslands Impact,” demonstrating excellent performance under drought stress (Daliya et al., 2018). A study on the genetic variation of drought resistance in 18 varieties revealed diversity among varieties and inconsistencies between survival rate and growth status. The “Sopin” variety performed the best under two drought conditions, adapting to drought by regulating starch and sugar metabolism, antioxidant enzyme activity, and transcriptional regulation mechanisms (Wang et al., 2023).
Hybrid breeding, as an effective genetic improvement method, can integrate the excellent traits of both parents. Through gene recombination and interaction, offspring with heterosis surpassing the parents’ traits may be bred (Zhao et al., 2024b). For cross-pollinated ryegrass, hybridization between different varieties of the same species is convenient, and interspecific hybridization is also feasible, often resulting in fertile hybrids. Distant hybridization has proven effective for enhancing stress resistance in perennial ryegrass. Liu et al. (2004) confirmed through isoenzyme analysis that “Changjiang No. 2” Italian ryegrass is a hybrid of “Abode” and “Ganxuan No. 1,” integrating the excellent qualities of both parents. Studying the drought tolerance of hybrid offspring from perennial ryegrass and tall fescue revealed that drought-tolerant ryegrass varieties could be developed through intergeneric hybridization (Wang and Bughrara, 2008).
Mutation breeding, as an effective means of genetic improvement, has been widely applied in drought-tolerance breeding of perennial ryegrass. Using spatial conditions for mutagenesis can generate high-frequency and significant variations, with most trait variations being heritable. Yan and Zhang (2008) observed the germination rate, phenological period, and agronomic traits of “Changjiang No. 2” Italian ryegrass seeds carried by the “Shijian 8” satellite. They found that the germination rate of the space-exposed seeds reached 98.34%, higher than the control group; the later growth rate was faster, and the coefficient of variation for various agronomic traits was significantly higher than the control. Further research showed that the endogenous hormones, ion content, and nutrient elements of perennial ryegrass seeds infected with endophytic fungi (E+) and uninfected (E−) changed after aerospace mutagenesis, these changes promoted host plant growth and ultimately improved drought resistance (Ma et al., 2020). Dong et al. (2018) successfully obtained mutant strains B9, B10, and B12 with strong drought resistance under drought stress by using EMS to induce mutations in seeds of the perennial ryegrass variety “Prime Minister.”
Global research focuses on introducing key functional genes into perennial ryegrass to improve its physiological characteristics. Given that perennial ryegrass is a cross-pollinated crop with high self-incompatibility, achieving homozygous stability of excellent genes is challenging, making genetic improvement relatively difficult. However, advances in biotechnology breeding have brought new hope. The application of genetic engineering, cell fusion, and molecular marker-assisted breeding techniques has improved breeding accuracy and speed, helping cultivate new varieties with ideal traits (Araghi et al., 2014; Foolad et al., 2001). Yang et al. (2006) transformed the DREB1B (CBF1) gene using a gene gun method and subjected 36 transgenic plants to drought treatment. After 25 days of drought, three plants showed signs of survival, and one revived after rehydration. Han et al. (2007) introduced DREB1A and BADH-CMO genes into embryogenic callus tissue of perennial ryegrass using gene gun and Agrobacterium-mediated techniques. The transgenic plants obtained showed strong tolerance to drought stress and produced fertile transgenic plants with enhanced drought resistance and normal growth. Zhang et al. (2011) cloned the Ac-1-FFT gene from ice grass using transgenic technology and transferred it into ryegrass to cultivate a new variety with enhanced drought resistance. Jaskune et al. (2020) successfully identified single nucleotide polymorphism (SNP) markers adjacent to the Phytochrome B (PhyB) and MYB41 transcription factor genes in perennial ryegrass using genome-wide association studies (GWAS). Research showed that the expression of these genes is closely related to leaf growth and yield under drought stress, playing roles in regulating drought response mechanisms. These SNP markers have the potential to become valuable resources for improving biomass and breeding strategies of perennial ryegrass under drought conditions. Akinroluyo et al. (2021) found through RNA sequencing that tetraploid lines of Italian ryegrass (Lolium multiflorum) have higher drought resistance and survival rates than diploid lines due to high expression of functional protein and dehydration protein genes. Additionally, the release of the perennial ryegrass genome has made predictive research based on genomic data possible, aiding in the selection of perennial ryegrass and providing a foundation for breeding other ryegrass species (Byrne et al., 2016).
To enhance the resistance of perennial ryegrass to heat stress, several strategies can be adopted. Firstly, introducing new ryegrass varieties with excellent heat resistance based on the natural ecological conditions of different regions is considered the most economical and effective way to improve resistance in ryegrass production. Secondly, selecting ryegrass varieties with good heat resistance through hybrid breeding, mutagenesis breeding, biotechnology breeding, or a combination of conventional and biotechnology breeding is a comprehensive strategy to prevent and address susceptibility to high-temperature stress (Kimbeng, 1999; Li et al., 2008; Soliman et al., 2021). Currently, research on heat-resistant breeding of perennial ryegrass mainly focuses on screening germplasm resources. For example, Zhang et al. (2020) subjected 98 varieties to a 24-day heat treatment at 35°C/30°C and evaluated their heat tolerance using eight physiological indicators. They successfully identified five heat-resistant and five heat-sensitive varieties. The study also showed that the perennial ryegrass variety “Emerald” has poor heat tolerance (Sun et al., 2020). Shi et al. (2010) used the conductivity method to determine the heat tolerance of 12 ryegrass varieties and found that “Esquire” and “Granddaddy” exhibited high heat tolerance after heat stress and recovery, demonstrating good heat resistance characteristics. Furthermore, Soliman et al. (2021) successfully cultivated 72 F₁ hybrid offspring by crossbreeding the heat-resistant variety “Kangaroo Valley” with the heat-sensitive variety “Norlea.” These offspring exhibit significant additive genetic variation in heat tolerance, providing valuable genetic resources for improving the heat tolerance of perennial ryegrass. In another study, Wang et al. (2016) subjected perennial ryegrass to high-temperature treatment at 40°C for 10 h and, compared with ryegrass under normal growth conditions, used RNA sequencing to identify 52 heat shock transcription factor (HSF) genes. Similarly, Wang et al. (2017) conducted RNA sequencing on perennial ryegrass treated at 35°C for 6 h and identified four candidate genes related to the C₄ carbon fixation pathway. The significant differences in heat tolerance among perennial ryegrass varieties are mainly caused by genetic diversity. Phenotypic and genetic diversity analysis is necessary for in-depth research on heat tolerance mechanisms, screening promising plant materials, and developing effective breeding strategies (Zhu et al., 2022).
In this study, we utilized bibliometric methods to comprehensively summarize and analyze the global literature on drought and heat stress in perennial ryegrass over the past 30 years. This analysis clarifies the overall knowledge framework of research in this area and provides a detailed and comprehensive reference for future studies. Our findings indicate a significant upward trend in research output during this period, with China and the United States emerging as major contributors. Notably, the journal Crop Science published the highest number of relevant papers. Keyword analysis revealed that “growth,” “endophytic fungi,” and “yield” are the most frequently used terms in drought stress research, while “growth,” “gene,” and “leaf” are prevalent in heat stress studies. Research has predominantly focused on phenotypic changes, response mechanisms, and techniques to enhance drought and heat resistance in perennial ryegrass. Endophytic fungi have become a hot topic in recent drought stress research and have also attracted considerable attention in heat stress studies, suggesting that this area may continue to develop in the future. Furthermore, there is a need to strengthen research on the functions and mechanisms of genes related to drought stress in ryegrass and to further explore molecular response mechanisms under heat stress. Trend analysis indicates that the interaction between drought and heat stress is receiving increasing attention, suggesting it will become an important direction for future research. Monitoring the hotspots and frontiers in perennial ryegrass research on drought and heat stress can help effectively identify key turning points and lay the foundation for future studies. The results of this study not only provide guidance for future research on perennial ryegrass under drought and heat stress but also offer valuable information for researchers in related fields.
RW: Writing – review & editing, Writing – original draft, Methodology. YG: Writing – review & editing, Formal analysis. JL: Writing – review & editing, Supervision, Conceptualization. XW: Writing – review & editing, Supervision, Conceptualization. YY: Writing – review & editing, Supervision, Conceptualization. HH: Writing – review & editing, Supervision, Conceptualization. ZZ: Writing – review & editing, Supervision, Conceptualization. PW: Writing – review & editing, Methodology, Funding acquisition. LZ: Writing – review & editing, Supervision, Conceptualization.
The author(s) declare that financial support was received for the research, authorship, and/or publication of this article. This study was supported by the Natural Science Foundation of China (32260351), State Key Laboratory of Microbial Technology Open Projects Fund (Project no. M2022-14), and Guizhou high-level innovative talents project [Qiankehepingtairencai-GCC (2022)022–1].
The authors declare that the research was conducted in the absence of any commercial or financial relationships that could be construed as a potential conflict of interest.
All claims expressed in this article are solely those of the authors and do not necessarily represent those of their affiliated organizations, or those of the publisher, the editors and the reviewers. Any product that may be evaluated in this article, or claim that may be made by its manufacturer, is not guaranteed or endorsed by the publisher.
The Supplementary material for this article can be found online at: https://www.frontiersin.org/articles/10.3389/fsufs.2024.1458552/full#supplementary-material
Abraha, A. B., Truter, W. F., Annandale, J. G., and Fessehazion, M. K. (2015). Forage yield and quality response of annual ryegrass (Lolium multiflorum) to different water and nitrogen levels. Afr. J. Range For. Sci. 32, 125–131. doi: 10.2989/10220119.2015.1056228
Abro, A. A., Anwar, M., Javwad, M. U., Zhang, M., Liu, F., Jiménez-Ballesta, R., et al. (2023). Morphological and physio-biochemical responses under heat stress in cotton: overview. Biotechnol. Rep 40:e00813. doi: 10.1016/j.btre.2023.e00813
Ahmar, S., Gill, R. A., Jung, K. H., Faheem, A., Qasim, M. U., Mubeen, M., et al. (2020). Conventional and molecular techniques from simple breeding to speed breeding in crop plants: recent advances and future outlook. Int. J. Mol. Sci. 21:2590. doi: 10.3390/ijms21072590
Akinroluyo, O. K., Kemesyte, V., Jaskune, K., and Statkeviciute, G. (2021). Candidate-gene expression patterns in diploid and tetraploid Lolium multiflorum spp. multiflorum cultivars under water deficit. Zemdirbyste-Agriculture 108, 363–370. doi: 10.13080/z-a.2021.108.046
Al Khateeb, W., Muhaidat, R., Alahmed, S., Al Zoubi, M. S., Al-Batayneh, K. M., El-Oqlah, A., et al. (2020). Heat shock proteins gene expression and physiological responses in durum wheat (Triticum durum) under salt stress. Physiol. Mol. Biol. Plants 26, 1599–1608. doi: 10.1007/s12298-020-00850-x
Amiard, V., Morvan-Bertrand, A., Billard, J. P., Huault, C., Keller, F., and Prud'homme, M. P. (2003). Fructans, but not the sucrosyl-galactosides, raffinose and loliose, are affected by drought stress in perennial ryegrass. Plant Physiol. 132, 2218–2229. doi: 10.1104/pp.103.022335
Anjum, S. A., Wang, L. C., Farooq, M., Hussain, M., Xue, L. L., and Zou, C. M. (2011). Brassinolide application improves the drought tolerance in maize through modulation of enzymatic antioxidants and leaf gas exchange. J. Agron. Crop Sci. 197, 177–185. doi: 10.1111/j.1439-037X.2010.00459.x
Araghi, B., Barati, M., Majidi, M. M., and Mirlohi, A. (2014). Application of half-sib mating for genetic analysis of forage yield and related traits in Bromus inermis. Euphytica 196, 25–34. doi: 10.1007/s10681-013-1011-2
Armstead, I., Donnison, I., Aubry, S., Harper, J., Hoertensteiner, S., James, C., et al. (2006). From crop to model to crop: identifying the genetic basis of the staygreen mutation in the Lolium/Festuca forage and amenity grasses. New Phytol. 172, 592–597. doi: 10.1111/j.1469-8137.2006.01922.x
Ashraf, M., Akram, N. A., Al-Qurainy, F., and Foolad, M. R. (2011). Drought tolerance: roles of organic osmolytes, growth regulators, and mineral nutrients. Adv. Agron. 111, 249–296. doi: 10.1016/b978-0-12-387689-8.00002-3
Baillo, E. H., Kimotho, R. N., Zhang, Z. B., and Xu, P. (2019). Transcription factors associated with abiotic and biotic stress tolerance and their potential for crops improvement. Genes. 10:771. doi: 10.3390/genes10100771
Basavaraj, P. S., and Rane, J. (2020). Avenues to realize potential of phenomics to accelerate crop breeding for heat tolerance. Plant Physiol. 25, 594–610. doi: 10.1007/s40502-020-00552-2
Bermanec, V., Vidakovic-Cifrek, Z., Fiket, Z., Tkalec, M., Kampic, S., and Kniewald, G. (2016). Influence of digested wastewater sludge on early growth of the perennial ryegrass (Lolium perenne L.). Environ. Earth Sci. 75:32. doi: 10.1007/s12665-015-4814-8
Bi, H. H., Zhao, Y., Li, H. H., and Liu, W. X. (2020). Wheat heat shock factor TaHsfA6f increases ABA levels and enhances tolerance to multiple abiotic stresses in transgenic plants. Int. J. Mol. Sci. 21:121. doi: 10.3390/ijms21093121
Bourgine, B., and Guihur, A. (2021). Heat shock signaling in land plants: from plasma membrane sensing to the transcription of small heat shock proteins. Front. Plant Sci. 12:710801. doi: 10.3389/fpls.2021.710801
Byrne, S. L., Nagy, I., Pfeifer, M., Armstead, I., and Asp, T. (2016). A synteny-based draft genome sequence of the forage grass Lolium perenne. Plant J. 84, 816–826. doi: 10.1111/tpj.13037
Cao, L., Han, L., Zhang, H. L., Xin, H. B., Imtiaz, M., Yi, M. F., et al. (2015). Isolation and characterization of pyrroline-5-carboxylate synthetase gene from perennial ryegrass (Lolium perenne L.). Acta Physiol. Plant. 37, 1–9. doi: 10.1007/s11738-015-1808-9
Chapman, D. F., Ludemann, C., Wims, C. M., and Kuhn-Sherlock, B. (2023). The contribution of perennial ryegrass (Lolium perenne L.) breeding to whole pasture productivity under dairy cattle grazing in New Zealand. 2. Rates of gain in production traits and economic value. Grass Forage Sci. 78, 85–100. doi: 10.1111/gfs.12589
Chaves, M. M., Flexas, J., and Pinheiro, C. (2009). Photosynthesis under drought and salt stress: regulation mechanisms from whole plant to cell. Ann. Bot. 103, 551–560. doi: 10.1093/aob/mcn125
Chen, W., and Huang, B. R. (2022). Cytokinin or ethylene regulation of heat-induced leaf senescence involving transcriptional modulation of WRKY in perennial ryegrass. Physiol. Plantarum. 174:e13766. doi: 10.1111/ppl.13766
Chen, T. H. H., and Murata, N. (2011). Glycinebetaine protects plants against abiotic stress: mechanisms and biotechnological applications. Plant Cell Environ. 34, 1–20. doi: 10.1111/j.1365-3040.2010.02232.x
Chen, Y., Sun, Y. Y., Wan, H., Chen, L., Cao, L., Zhao, B. Y., et al. (2023). Integrative analysis of transcriptome and yeast screening system identified heat stress-responding genes in ryegrass. Environ. Exp. Bot. 210:105333. doi: 10.1016/j.envexpbot.2023.105333
Choi, H., Hong, J., Ha, J., Kang, J., and Kim, S. Y. (2000). ABFs, a family of ABA-responsive element binding factors. J. Biol. Chem. 275, 1723–1730. doi: 10.1074/jbc.275.3.1723
Choudhury, F. K., Rivero, R. M., Blumwald, E., and Mittler, R. (2017). Reactive oxygen species, abiotic stress and stress combination. Plant J. 90, 856–867. doi: 10.1111/tpj.13299
Clay, K., and Schardl, C. (2002). Evolutionary origins and ecological consequences of endophyte symbiosis with grasses. Am. Nat. 160, S99–S127. doi: 10.1086/342161
Craufurd, P. Q., Vadez, V., Jagadish, S. K., Prasad, P. V., and Zaman-Allah, M. (2013). Crop science experiments designed to inform crop modeling. Agric. For. Meteorol. 170, 8–18. doi: 10.1016/j.agrformet.2011.09.003
Dai, Y., Mao, P., Tao, X., Wang, Y., Wei, C. M., and Ma, X. R. (2017). Flexible shift on gene body methylation and transcription of LpCYP72A161 exposed to temperature stress in perennial ryegrass. Environ. Exp. Bot. 143, 29–37. doi: 10.1016/j.envexpbot.2017.08.002
Daliya, C., Hofmann, R. W., Alan, S., Sathish, P., Winefield, C. S., and Moot, D. J. (2018). Intraspecific differences in long-term drought tolerance in perennial ryegrass. PLoS One 13:e0194977. doi: 10.1371/journal.pone.0194977
Demirkol, G., Felek, A. F., Asci, O. O., and Yilmaz, N. (2023). Physiological and transcriptional status of genetically diverse perennial ryegrass (Lolium perenne L.) populations under drought stress. Plant Genet. Resour. 21, 331–339. doi: 10.1017/s1479262123000795
Deng, J., Li, F., and Duan, T. Y. (2020). Claroideoglomus etunicatum reduces leaf spot incidence and improves drought stress resistance in perennial ryegrass. Australasian Plant Path. 49, 147–157. doi: 10.1007/s13313-020-00685-w
Ding, L. L., Chen, H., Wei, X., Qin, T. Y., Lei, X., Li, J. Y., et al. (2024). Soil cations explain the variation of soil extracellular activities and microbial elemental limitations on subtropical grassland, China. Polish J. Environ. Stu. 33, 4061–4070. doi: 10.15244/pjoes/176566
Ding, L. L., Tian, L. L., Li, J. Y., Zhang, Y. J., Wang, M. Y., and Wang, P. C. (2023). Grazing lowers soil multifunctionality but boosts soil microbial network complexity and stability in a subtropical grassland of China. Front. Microbiol. 13:1027097. doi: 10.3389/fmicb.2022.1027097
Dong, W. K., Lu, X. P., Jiang, H. Y., and Ma, H. L. (2018). Ems mutagenesis and drought tolerant evaluation of Loium perenne L. J. Nuclear Agric. Sci. 32, 1889–1897. doi: 10.11869/j.issn.100-8551.2018.10.1889
Dossa, K., Diouf, D., and Cisse, N. (2016). Genome-wide investigation of Hsf genes in sesame reveals their segmental duplication expansion and their active role in drought stress response. Front. Plant Sci. 7:1522. doi: 10.3389/fpls.2016.01522
Edreva, A. (2005). Generation and scavenging of reactive oxygen species in chloroplasts: a submolecular approach. Agric. Ecosyst. Environ. 106, 119–133. doi: 10.1016/j.agee.2004.10.022
Farooq, M., Wahid, A., Kobayashi, N., Fujita, D., and Basra, S. M. A. (2009). Plant drought stress: effects, mechanisms and management. Agron. Sustain. Dev. 29, 185–212. doi: 10.1051/agro:2008021
Foito, A., Byrne, S. L., Shepherd, T., Stewart, D., and Barth, S. (2009). Transcriptional and metabolic profiles of Lolium perenne L. genotypes in response to a PEG-induced water stress. Plant Biotechnol. J. 7, 719–732. doi: 10.1111/j.1467-7652.2009.00437.x
Foolad, M. R., Zhang, L. P., and Lin, G. Y. (2001). Identification and validation of QTLs for salt tolerance during vegetative growth in tomato by selective genotyping. Genome 44, 444–454. doi: 10.1139/gen-44-3-444
Fu, J. M., and Huang, B. R. (2001). Involvement of antioxidants and lipid peroxidation in the adaptation of two cool-season grasses to localized drought stress. Environ. Exp. Bot. 45, 105–114. doi: 10.1016/S0098-8472(00)00084-8
Fujita, Y., Fujita, A. M., Satoh, C. R., Maruyama, C. K., Parvez, A. M. M., and Seki, A. M. (2005). AREB1 is a transcription activator of novel abre-dependent ABA signaling that enhances drought stress tolerance in Arabidopsis. Plant Cell 17:3470, –3488. doi: 10.1105/tpc.105.035659
Gao, Y., Zhang, Y. J., Wang, P. C., and Zhao, L. L. (2024). Structure and diversity of endophytic bacteria in maize seeds and germinating roots. Microorganisms. 12:1348. doi: 10.3390/microorganisms12071348
Gong, M., Li, Y. J., and Chen, S. Z. (1998). Abscisic acid-induced thermotolerance in maize seedlings is mediated by calcium and associated with antioxidant systems. J. Plant Physiol. 153, 488–496. doi: 10.1016/S0176-1617(98)80179-X
Gong, Z. Z., Xiong, L. M., Shi, H. Z., Yang, S. H., and Zhu, J. K. (2020). Plant abiotic stress response and nutrient use efficiency. Sci. China Life Sci. 63:635, –674. doi: 10.1007/s11427-020-1683-x
Gornall, J., Betts, R., Burke, E., Clark, R., Camp, J., Willett, K., et al. (2010). Implications of climate change for agricultural productivity in the early twenty-first century. Philos. T. R. Soc. B. 365, 2973–2989. doi: 10.1098/rstb.2010.0158
Guan, Q. M., Lu, X. Y., Zeng, H. T., Zhang, Y. Y., and Zhu, J. H. (2013). Heat stress induction of miR398 triggers a regulatory loop that is critical for thermotolerance in Arabidopsis. Plant J. 74, 840–851. doi: 10.1111/tpj.12169
Gunasekera, D., and Berkowitz, G. A. (1993). Use of transgenic plants with ribulose-1, 5-bisphosphate carboxylase/oxygenase antisense DNA to evaluate the rate limitation of photosynthesis under water stress. J. Plant Physiol. 103, 629–635. doi: 10.1104/pp.103.2.629
Hahn, H., McManus, M. T., Warnstorff, K., Monahan, B. J., Young, C. A., Davies, E., et al. (2008). Neotyphodium fungal endophytes confer physiological protection to perennial ryegrass (Lolium perenne L.) subjected to a water deficit. Environ. Exp. Bot. 63, 183–199. doi: 10.1016/j.envexpbot.2007.10.021
Hamilton, C. E., Gundel, P. E., Helander, M., and Saikkonen, K. (2012). Endophytic mediation of reactive oxygen species and antioxidant activity in plants: a review. Fungal Divers. 54, 1–10. doi: 10.1007/s13225-012-0158-9
Han, L. B., Li, X., Liu, J., and Zeng, H. M. (2007). “Drought-tolerant transgenic perennial ryegrass (Lolium perenne L.) obtained via particle bombardment gene transformation of CBF3/DREB1A gene,” in II International Conference on Turfgrass Science and Management for Sports Fields, 273–282.
Hang, N., Shi, T. R., Liu, Y. R., Ye, W. X., Taier, G., Sun, Y., et al. (2021). Overexpression of Os-microRNA408 enhances drought tolerance in perennial ryegrass. Physiol. Plantarum. 172, 733–747. doi: 10.1111/ppl.13276
Hesse, U., Schöberlein, W., Wittenmayer, L., Förster, K., Warnstorff, K., Diepenbrock, W., et al. (2003). Effects of neotyphodium endophytes on growth, reproduction and drought-stress tolerance of three Lolium perenne L. genotypes. Grass Forage Sci. 58, 407–415. doi: 10.1111/j.1365-2494.2003.00393.x
Higuchi, H., Sakuratani, T., and Utsunomiya, N. (1999). Photosynthesis, leaf morphology, and shoot growth as affected by temperatures in cherimoya (Annona cherimola mill.) trees. Sci. Hortic. 80, 91–104. doi: 10.1016/S0304-4238(98)00221-0
Hoffman, L., Ebdon, J. S., Dest, W. M., and DaCosta, M. (2010). Effects of nitrogen and potassium on wear mechanisms in perennial ryegrass: I. Wear tolerance and recovery. Crop Sci. 50, 357–366. doi: 10.2135/cropsci2008.08.0473
Huang, B. R., DaCosta, M., and Jiang, Y. W. (2014). Research advances in mechanisms of turfgrass tolerance to abiotic stresses: from physiology to molecular biology. Crit. Rev. Plant Sci. 33, 141–189. doi: 10.1080/07352689.2014.870411
Huang, X. Q., Ke, S. Y., and Liu, Y. (2008). Comparative studies on adaptability of Germany ryegrass in Sichuan. Pratac. Sci. 25, 60–65. doi: 10.3969/j.issn.1001-0629.2008.10.013
Huang, H. Y., Wang, X. T., Li, J. Q., Gao, Y., Yang, Y. T., Wang, R., et al. (2024). Trends and directions in oats research under drought and salt stresses: a bibliometric analysis (1993–2023). Plan. Theory 13:1902. doi: 10.3390/plants13141902
Huang, L. K., Yan, H. D., Jiang, X. M., Yin, G. H., Zhang, X. Q., Qi, X., et al. (2014). Identification of candidate reference genes in perennial ryegrass for quantitative RT-PCR under various abiotic stress conditions. PLoS One 9:e93724. doi: 10.1371/journal.pone.0093724
Huchzermeyer, B., Menghani, E., Khardia, P., and Shilu, A. (2022). Metabolic pathway of natural antioxidants, antioxidant enzymes and ROS providence. Antioxidants. 11:761. doi: 10.3390/antiox11040761
Hussain, H. A., Men, S. N., Hussain, S., Chen, Y. L., Ali, S., Zhang, S., et al. (2019). Interactive effects of drought and heat stresses on morphophysiological attributes, yield, nutrient uptake and oxidative status in maize hybrids. Sci. Rep. 9:3890. doi: 10.1038/s41598-019-40362-7
Iqbal, B., Zhao, X., Khan, K. Y., Javed, Q., Nazar, M., Khan, I., et al. (2024). Microplastics meet invasive plants: unraveling the ecological hazards to agroecosystems. Sci. Total Environ. 906:167756. doi: 10.1016/j.scitotenv.2023.167756
Jafari, S., Garmdareh, S. E. H., and Azadegan, B. (2019). Effects of drought stress on morphological, physiological, and biochemical characteristics of stock plant (Matthiola incana L.). Sci. Hortic. 253, 128–133. doi: 10.1016/j.scienta.2019.04.033
Janke, V. D. C., Botha, P. R., Meeske, R., and Truter, W. F. (2015). Grazing capacity, milk production and milk composition of kikuyu over-sown with annual or perennial ryegrass. Afr. J. Range For. Sci. 32, 143–151. doi: 10.2989/10220119.2015.1052096
Jaskune, K., Aleliunas, A., Statkeviciute, G., Kemesyte, V., Studer, B., and Yates, S. (2020). Genome-wide association study to identify candidate loci for biomass formation under water deficit in perennial ryegrass. Front. Plant Sci. 11:570204. doi: 10.3389/fpls.2020.570204
Javaid, M. M., Mahmood, A., Alshaya, D. S., AlKahtani, M. D. F., Waheed, H., Wasaya, A., et al. (2022). Influence of environmental factors on seed germination and seedling characteristics of perennial ryegrass (Lolium perenne L.). Sci. Rep. 12:9522. doi: 10.1038/s41598-022-13416-6
Jedmowski, C., Ashoub, A., Momtaz, O., and Brüggemann, W. (2015). Impact of drought, heat, and theircombination on chlorophyll fluorescence and yield ofwild barley (Hordeum spontaneum). J. Bot. 2015, 1–9. doi: 10.1155/2015/120868
Jespersen, D., Zhang, J., and Huang, B. (2016). Chlorophyll loss associated with heat-induced senescence in bentgrass. Plant Sci. 249, 1–12. doi: 10.1016/j.plantsci.2016.04.016
Jiang, Y. W., Cui, Y., Pei, Z. Y., Liu, H. F., and Sun, S. J. (2016). Growth response and gene expression to deficit irrigation and recovery of two perennial ryegrass accessions contrasting in drought tolerance. HortScience 51, 921–926. doi: 10.21273/hortsci.51.7.921
Jones, M. B., Leafe, E. L., and Stiles, W. (1980). Water stress in field-grown perennial ryegrass. I. Its effect on growth, canopy photosynthesis and transpiration. Ann. Appl. Biol. 96, 87–101. doi: 10.1111/j.1744-7348.1980.tb04772.x
Jung, S., Estrella, N., Pfaffl, M. W., Hartmann, S., Ewald, F., and Menzel, A. (2021). Correction: impact of elevated air temperature and drought on pollen characteristics of major agricultural grass species. PLoS One 16:e0261879. doi: 10.1371/journal.pone.0261879
Kan, Y., Mu, X. R., Gao, J., Lin, H. X., and Lin, Y. S. (2023). The molecular basis of heat stress responses in plants. Mol. Plant 16, 1612–1634. doi: 10.1016/j.molp.2023.09.013
Kane, K. H. (2011). Effects of endophyte infection on drought stress tolerance of Lolium perenne accessions from the Mediterranean region. Environ. Exp. Bot. 71, 337–344. doi: 10.1016/j.envexpbot.2011.01.002
Karsten, H. D., and Macadam, J. W. (2001). Effect of drought on growth, carbohydrates, and soil water use by perennial ryegrass, tall fescue, and white clover. Crop Sci. 41, 156–166. doi: 10.2135/cropsci2001.411156x
Kauffman, G. L. III, Kneivel, D. P., and Watschke, T. L. (2007). Effects of a biostimulant on the heat tolerance associated with photosynthetic capacity, membrane thermostability, and polyphenol production of perennial ryegrass. Crop Sci. 47, 261–267. doi: 10.2135/cropsci2006.03.0171
Kemesyte, V., Statkeviciute, G., and Brazauskas, G. (2017). Perennial ryegrass yield performance under abiotic stress. Crop Sci. 57, 1935–1940. doi: 10.2135/cropsci2016.10.0864
Kimbeng, C. A. (1999). Genetic basis of crown rust resistance in perennial ryegrass, breeding strategies, and genetic variation among pathogen populations: a review. Aust. J. Exp. Agr. 39, 361–378. doi: 10.1071/ea98111
Koscielniak, J., Filek, W., and Biesaga-Koscielniak, J. (2006). The effect of drought stress on chlorophyll fluorescence in Lolium-Festuca hybrids. Acta Physiol. Plant. 28, 149–158. doi: 10.1007/s11738-006-0041-y
Kotak, S., Larkindale, J., Lee, U., Vierling, E., and Scharf, K. D. (2007). Complexity of the heat stress response in plants. Curr. Opin. Plant Biol. 10, 310–316. doi: 10.1016/j.pbi.2007.04.011
Kuzniak, E. (2002). Transgenic plants: an insight into oxidative stress tolerance mechanisms. Acta Physiol. Plant. 24, 97–113. doi: 10.1007/s11738-002-0027-3
Lei, S. H., and Huang, B. R. (2022). Metabolic regulation of α-ketoglutarate associated with heat tolerance in perennial ryegrass. Plant Physiol. Bioch. 190, 164–173. doi: 10.1016/j.plaphy.2022.09.005
Lei, S. H., Rossi, S., and Huang, B. R. (2022). Metabolic and physiological regulation of aspartic acid-mediated enhancement of heat stress tolerance in perennial ryegrass. Plan. Theory 11:199. doi: 10.3390/plants11020199
Leisner, C. P. (2020). Review: climate change impacts on food security- focus on perennial cropping systems and nutritional value. Plant Sci. 293:110412. doi: 10.1016/j.plantsci.2020.110412
Li, B. F., and Du, H. M. (2019). Mechanism analysis of melatonin pretreatment to improve drought tolerance of perennial ryegrass (Lolium perenne). Pratac Sci. 36, 666–676. doi: 10.11829/j.issn.1001-0629.2018-0397
Li, N., Euring, D., Cha, J. Y., Lin, Z., Lu, M. Z., Huang, L. J., et al. (2021). Plant hormone-mediated regulation of heat tolerance in response to global climate change. Front. Plant Sci. 11:627969. doi: 10.3389/fpls.2020.627969
Li, X., Han, L. B., and Liu, J. (2008). Review on transgenic breeding and biosafety of perennial ryegrass (Lolium perenne L.). Chin. J. Grassland. 32, 91–95. doi: 10.3724/SP.J.1035.2008.00091
Li, M., Jannasch, A. H., and Jiang, Y. (2020). Growth and hormone alterations in response to heat stress in perennial ryegrass accessions differing in heat tolerance. J. Plant Growth Regul. 39, 1022–1029. doi: 10.1007/s00344-019-10043-w
Li, J., Long, Y., Qi, G. N., Li, J., Xu, Z. J., Wu, W. H., et al. (2014). The os-akt1 channel is critical for k+ uptake in rice roots and is modulated by the rice cbl1-cipk23 complex. Plant Cell 26, 3387–3402. doi: 10.1105/tpc.114.123455
Li, X., Yang, Y. Q., Sun, X. D., Lin, H. M., Chen, J. H., Ren, J., et al. (2014). Comparative physiological and proteomic analyses of poplar (Populus yunnanensis) plantlets exposed to high temperature and drought. PLoS One 9:e107605. doi: 10.1371/journal.pone.0107605
Li, P. S., Yu, T. F., He, G. H., Chen, M., Zhou, Y. B., Chai, S. C., et al. (2014). Genome-wide analysis of the HSF family in soybean and functional identification of GmHsf-34 involvement in drought and heat stresses. BMC Genomics 15:1009. doi: 10.1186/1471-2164-15-1009
Li, H. C., Zhang, H. N., Li, G. L., Liu, Z. H., Zhang, Y. M., Zhang, H. M., et al. (2015). Expression of maize heat shock transcription factor gene ZmHsf06 enhances the thermotolerance and drought-stress tolerance of transgenic Arabidopsis. Funct. Plant Biol. 42, 1080–1091. doi: 10.1071/fp15080
Lin, J. S., Kuo, C. C., Yang, I. C., Tsai, W. A., Shen, Y. H., Lin, C. C., et al. (2018). MicroRNA160 modulates plant development and heat shock protein gene expression to mediate heat tolerance in Arabidopsis. Front. Plant Sci. 9:68. doi: 10.3389/fpls.2018.00068
Liu, S. W., and Jiang, Y. W. (2010). Identification of differentially expressed genes under drought stress in perennial ryegrass. Physiol. Plant. 139, 375–387. doi: 10.1111/j.1399-3054.2010.01374.x
Liu, Q., Luo, J., and Cao, S. H. (2006). Experiment in the introduction of herbage variety in the southern Shaanxi. J. Anhui Agric. Sci. 2, 3373–3374. doi: 10.3969/j.issn.0517-6611.2006.14.068
Liu, J., Xu, L. X., Wang, Y. B., and Han, L. B. (2019). Ethephon treatment reduced mondo grass (Ophiopogon japonicus) gas exchange rate and gene expression of Rbcs. Eur. J. Hortic. Sci. 84, 106–112. doi: 10.17660/eJHS.2019/84.2.7
Liu, M. X., Zhang, X. Q., and Yang, C. H. (2004). Analysis on the isozyme of annunl ryegrass Changiiang - II and its parents. Grass Forage Sci. 2, 20–22. doi: 10.3969/j.issn.1673-8403.2004.11.007
Ma, B. H., Lin, W. H., Gao, M., Wang, X. D., and Tian, P. (2020). Effects of salicylic acid and Epichloёon perennial ryegrass (Lolium perenne) under drought stress. Acta Pratacul. Sin. 29, 135–144. doi: 10.11686/cyxb2019103
Ma, G. J., Shen, J., Yu, H., Huang, X. B., Deng, X. L., Hu, Z. R., et al. (2022). Genome-wide identification and functional analyses of heat shock transcription factors involved in heat and drought stresses in ryegrass. Environ. Exp. Bot. 201:104968. doi: 10.1016/j.envexpbot.2022.104968
Ma, Y. H., Song, Y., and Zhang, H. R. (2009). Effects of uniconazole on the drought resistance of perennial ryegrass. Pratac. Sci. 26, 169–173. doi: 10.3969/j.issn.1001-0629.2009.05.030
Mahdavi, S., Kafi, M., Fallahi, E., Shokrpour, M., and Tabrizi, L. (2016). Water stress, nano silica, and digoxin effects on minerals, chlorophyll index, and growth in ryegrass. Int. J. Plant Prod. 10, 251–264. doi: 10.22069/IJPP.2016.2791
Mahmood, T., Khalid, S., Abdullah, M., Ahmed, Z., Shah, M. K. N., Ghafoor, A., et al. (2020). Insights into drought stress signaling in plants and the molecular genetic basis of cotton drought tolerance. Cells 9:105. doi: 10.3390/cells9010105
Majeed, Y., Rasheed, A., Awan, S. I., Xu, Z. J., Javed, S. O., Gillani, S. F. A., et al. (2023). Physicochemical response of alfalfa (Medicago sativa L.) and rye grass (Lolium perenne L.) genotypes to induce drought stress at seedling stage. Appl. Ecol. Env. Res. 21, 3335–3348. doi: 10.15666/aeer/2104_33353348
Medrano, H., Parry, M. A. J., Socias, X., and Lawlor, D. W. (2010). Long term water stress inactivates rubisco in subterranean clover. Ann. Appl. Biol. 131, 491–501. doi: 10.1111/j.1744-7348.1997.tb05176.x
Mittler, R. (2006). Abiotic stress, the field environment and stress combination. Trends Plant Sci. 11, 15–19. doi: 10.1016/j.tplants.2005.11.002
Mo, B. T., Long, Z. F., Chen, R. X., Luo, J. Y., Yang, F., and Zhang, M. J. (2009). Breeding of Guicao 1 (Lolium multiflorum). Agric. Sci. 37, 94–95. doi: 10.3969/j.issn.1001-3601.2009.02.035
Mohammadi, M. H. S., Etemadi, N., Arab, M. M., Aalifar, M., and Pessarakli, M. (2017). Molecular and physiological responses of iranian perennial ryegrass as affected by trinexapac ethyl, paclobutrazol and abscisic acid under drought stress. Plant Physiol. Bioch. 111, 129–143. doi: 10.1016/j.plaphy.2016.11.014
Moore, C. E., Meacham-Hensold, K., Lemonnier, P., Slattery, R. A., Benjamin, C., Bernacchi, C. J., et al. (2021). The effect of increasing temperature on crop photosynthesis: from enzymes to ecosystems. J. Exp. Bot. 72, 2822–2844. doi: 10.1093/jxb/erab090
Naidoo, S. (2022). Commentary on the contribution of working group iii to the sixth assessment report of the intergovernmental panel on climate change. S. Afr. J. Sci. 118, 9–10. doi: 10.17159/sajs.2022/14690
Ninkov, A., Frank, J. R., and Maggio, L. A. (2022). Bibliometrics: methods for studying academic publishing. Perspect Med. Educ. 11, 173–176. doi: 10.1007/s40037-021-00695-4
Ozga, J. A., Kaur, H., Savada, R. P., and Reinecke, D. M. (2017). Hormonal regulation of reproductive growth under normal and heat-stress conditions in legume and other model crop species. J. Exp. Bot. 68:erw464. doi: 10.1093/jxb/erw464
Pan, C. Z., Ahammed, G. J., Li, X., and Shi, K. (2018). Elevated CO2 improves photosynthesis under high temperature by attenuating the functional limitations to energy fluxes, electron transport and redox homeostasis in tomato leaves. Front. Plant Sci. 9:1739. doi: 10.3389/fpls.2018.01739
Pan, L., Meng, C., Wang, J. P., Ma, X., Fan, X. M., Yang, Z. F., et al. (2018). Integrated omics data of two annual ryegrass (Lolium multiflorum L.) genotypes reveals core metabolic processes under drought stress. BMC Plant Biol. 18:26. doi: 10.1186/s12870-018-1239-z
Patel, M., Milla-Lewis, S., Zhang, W., Templeton, K., Reynolds, W. C., Richardson, K., et al. (2015). Overexpression of ubiquitin-like LpHUB1 gene confers drought tolerance in perennial ryegrass. Plant Biotechnol. J. 13, 689–699. doi: 10.1111/pbi.12291
Ponce, R. G., Salas, M. L., and Mason, S. C. (1993). Nitrogen use efficiency by winter barley under different climatic conditions. J. Plant Nutr. 16, 1249–1261. doi: 10.1080/01904169309364610
Praba, M. L., Cairns, J. E., Babu, R. C., and Lafitte, H. R. (2009). Identification of physiological traits underlying cultivar differences in drought tolerance in rice and wheat. J. Agron. Crop Sci. 195, 30–46. doi: 10.1111/j.1439-037X.2008.00341.x
Prasad, P. V. V., Staggenborg, S. A., and Ristic, Z. (2008). Impacts of drought and/or heat stress on physiological, developmental, growth, and yield processes of crop plants. Resp. Crops Limited Water Understanding Modeling Water Stress Effects Plant Growth Proc. 1, 301–355. doi: 10.2134/advagricsystmodel1.c11
Pucciariello, C., Banti, V., and Perata, P. (2012). ROS signaling as common element in low oxygen and heat stresses. Plant Physiol. Bioch. 59, 3–10. doi: 10.1016/j.plaphy.2012.02.016
Rahman, M. A., Kabir, A. H., Song, Y., Lee, S. H., Hasanuzzaman, M., and Lee, K. W. (2021). Nitric oxide prevents Fe deficiency-induced photosynthetic disturbance, and oxidative stress in alfalfa by regulating Fe acquisition and antioxidant defense. Antioxidants. 10:1556. doi: 10.3390/antiox10101556
Rahman, M. A., Kabir, A. H., Song, Y., Lee, S. H., Hasanuzzaman, M., and Lee, K. W. (2022). Heat shock proteins and antioxidant genes involved in heat combined with drought stress responses in perennial rye grass. Life 12:1426. doi: 10.3390/life12091426
Ravel, C., Courty, C., Coudret, A., and Charmet, G. (1997). Beneficial effects of Neotyphodium lolii on the growth and the water status in perennial ryegrass cultivated under nitrogen deficiency or drought stress. Agronomie 17, 173–181. doi: 10.1051/agro:19970304
Reed, R. C., Bradford, K. J., and Khanday, I. (2022). Seed germination and vigor: ensuring crop sustainability in a changing climate. Heredity 128, 450–459. doi: 10.1038/s41437-022-00497-2
Reynolds, M. P., Pierre, C. S., Saad, A. S., Vargas, M., and Condon, A. G. (2007). Evaluating potential genetic gains in wheat associated with stress-adaptive trait expression in elite genetic resources under drought and heat stress. Crop Sci. 47, S172–S189. doi: 10.2135/cropsci2007.10.0022IPBS
Rogers, M. E., Lawson, A. R., and Kelly, K. B. (2019). Summer production and survival of perennial ryegrass (Lolium perenne) and tall fescue (Festuca arundinacea) genotypes in northern Victoria under differing irrigation management. Crop Pasture Sci. 70, 1163–1174. doi: 10.1071/cp18542
Safari, S., Nazari, F., Vafaee, Y., and Teixeira da Silva, J. A. (2023). Impact of rice husk biochar on drought stress tolerance in perennial ryegrass (Lolium perenne L.). J. Plant Growth Regul. 42, 810–826. doi: 10.1007/s00344-022-10588-3
Sahu, P. K., Jayalakshmi, K., Tilgam, J., Gupta, A., Nagaraju, Y., Kumar, A., et al. (2022). ROS generated from biotic stress: effects on plants and alleviation by endophytic microbes. Front. Plant Sci. 13:1042936. doi: 10.3389/fpls.2022.1042936
Sato, H., Mizoi, J., Shinozaki, K., and Yamaguchi-Shinozaki, K. (2024). Complex plant responses to drought and heat stress under climate change. Plant J. 117, 1873–1892. doi: 10.1111/tpj.16612
Scharf, K. D., Berberich, T., Ebersberger, I., and Nover, L. (2018). The plant heat stress transcription factor (Hsf) family: structure, function and evolution. BBA-Gene Regul. Mech. 1861:60. doi: 10.1016/j.bbagrm.2017.12.005
Schauberger, B., Archontoulis, S., Arneth, A., Balkovic, J., Ciais, P., Deryng, D., et al. (2017). Consistent negative response of US crops to high temperatures in observations and crop models. Nat. Commun. 8:13931. doi: 10.1038/ncomms13931
Shah, F., Bajwa, A. A., Usman, N., Anjum, S. A., Ayesha, F., Ali, Z., et al. (2017). Crop production under drought and heat stress: plant responses and management options. Front. Plant Sci. 8:1147. doi: 10.3389/fpls.2017.01147
Shariatipour, N., Heidari, B., Shams, Z., and Richards, C. (2022). Assessing the potential of native ecotypes of Poa pratensis L. for forage yield and phytochemical compositions under water deficit conditions. Sci. Rep. 12:1121. doi: 10.1038/s41598-022-05024-1
Shen, J. B., Xu, L. Y., Jin, X. Q., Chen, J. H., and Lu, H. F. (2008). Effect of temperature regime on germination of seed of perennial ryegrass (Lolium perenne). Grass Forage Sci. 63, 249–256. doi: 10.1111/j.1365-2494.2008.00630.x
Sheng, L. X., and He, X. Q. (2003). The development trend, introduction and breeding of forages and feed crops. Pratac. Sci. 20, 14–17. doi: 10.3969/j.issn.1001-0629.2003.05.006
Shi, Y. H., Wan, L. Q., Liu, J. N., Wang, Y. Q., Wu, X. M., and Li, X. L. (2010). Semi-lethal high temperature and heat tolerance of twelve varieties of Lolium perenne. Pratac. Sci. 30, 491–496. doi: 10.3724/SP.J.1142.2010.40491
Soliman, W. S., Abbas, A. M., Novak, S. J., Fujimori, M., Tase, K., and Sugiyama, S. I. (2021). Inheritance of heat tolerance in perennial ryegrass (Lolium perenne, Poaceae): evidence from progeny array analysis. PeerJ. 9:e11782. doi: 10.7717/peerj.11782
Steinmeyer, F. T., Lukac, M., Reynolds, M. P., and Jones, H. E. (2013). Quantifying the relationship between temperature regulation in the ear and floret development stage in wheat (Triticum aestivum L.) under heat and drought stress. Funct. Plant Biol. 40, 700–707. doi: 10.1071/fp12362
Su, K., Bremer, D. J., Keeley, S. J., and Fry, J. D. (2007). Effects of high temperature and drought on a hybrid bluegrass compared with Kentucky bluegrass and tall fescue. Crop Sci. 47, 2152–2161. doi: 10.2135/cropsci2006.12.0781
Sun, T. X., Shao, K., Huang, Y., Lei, Y. Y., Tan, L. Y., and Chan, Z. L. (2020). Natural variation analysis of perennial ryegrass in response to abiotic stress highlights LpHSFC1b as a positive regulator of heat stress. Environ. Exp. Bot. 179:104192. doi: 10.1016/j.envexpbot.2020.104192
Sundaram, S., and Rathinasabapathi, B. (2010). Transgenic expression of fern Pteris vittata glutaredoxin PvGrx5 in Arabidopsis thaliana increases plant tolerance to high temperature stress and reduces oxidative damage to proteins. Planta 231, 361–369. doi: 10.1007/s00425-009-1055-7
Tajdel, M., Mitula, F., and Ludwikow, A. (2016). Regulation of Arabidopsis MAPKKK18 by ABI1 and SnRK2, components of the ABA signaling pathway. Plant Signal. Behav. 11:e1139277. doi: 10.1080/15592324.2016.1139277
Thomas, H., Morgan, W. G., Thomas, A. M., and Ougham, H. J. (1999). Expression of the stay-green character introgressed into Lolium temulentum Ceres from a senescence mutant of Festuca pratensis. Theor. Appl. Genet. 99, 92–99. doi: 10.1007/s001220051212
Wafa’a, A. (2010). Comparative effects of drought and salt stress on germination and seedling growth of Pennisetum divisum (Gmel.) Henr. Am. J. Appl. Sci. 7, 640–646. doi: 10.3844/ajassp.2010.640.646
Wahid, A., and Close, T. J. (2007). Expression of dehydrins under heat stress and their relationship with water relations of sugarcane leaves. Biol. Plant. 51, 104–109. doi: 10.1007/s10535-007-0021-0
Wahid, A., Gelani, S., Ashraf, M., and Foolad, M. R. (2007). Heat tolerance in plants: an overview. Environ. Exp. Bot. 61, 199–223. doi: 10.1016/j.envexpbot.2007.05.011
Wang, S. G. (2000). Roles of cytokinin on stress-resistance and delaying senescence in plants. Chin. Bullet. Bot. 17:121. doi: 10.3969/j.issn.1674-3466.2000.02.004
Wang, J. P., and Bughrara, S. S. (2008). Evaluation of drought tolerance for atlas fescue, perennial ryegrass, and their progeny. Euphytica 164, 113–122. doi: 10.1007/s10681-008-9669-6
Wang, Y., Dai, Y., Tao, X., Wang, J. Z., Cheng, H. Y., Yang, H., et al. (2016). Heat shock factor genes of tall fescue and perennial ryegrass in response to temperature stress by RNA-Seq analysis. Front. Plant Sci. 6:1226. doi: 10.3389/fpls.2015.01226
Wang, F. G., Liu, Y. Y., Shi, Y. Q., Han, D. L., Wu, Y. Y., Ye, W. X., et al. (2020). SUMOylation stabilizes the transcription factor DREB2A to improve plant thermotolerance. Plant Physiol. 183, 41–50. doi: 10.1104/pp.20.00080
Wang, K. H., Liu, Y. R., Tian, J. L., Huang, K. Y., Shi, T. R., Dai, X. X., et al. (2017). Transcriptional profiling and identification of heat-responsive genes in perennial ryegrass by RNA-sequencing. Front. Plant Sci. 8:1032. doi: 10.3389/fpls.2017.01032
Wang, W. X., Vinocur, B., Shoseyov, O., and Altman, A. (2004). Role of plant heat-shock proteins and molecular chaperones in the abiotic stress response. Trends Plant Sci. 9, 244–252. doi: 10.1016/j.tplants.2004.03.006
Wang, R. M., Wang, Z. Q., and Xiang, Z. X. (2019). Effect of exogenous gamma-aminobutyric acid on the antioxidant defense system and phytohormones metabolism under high temperature stress in perennial ryegrass. Pratac. Sci. 36, 111–122. doi: 10.11829/j.issn.1001-0629.2018-0133
Wang, H., Wang, D. M., Zhang, Z. Z., Ren, H. X., Huang, W., and Xie, Z. F. (2022). Effects of exogenous melatonin on antioxidant capacity and nutrient uptake of Lolium perenne and Medicago sativa under drought stress. J. Appl. Ecol. 33, 1311–1319. doi: 10.13287/j.1001-9332.202205.004
Wang, R. M., and Xiong, X. Y. (2016). Effect of temperature stress on growth and metabolism in perennial ryegrass. Acta Pratacul. Sin. 25:81. doi: 10.11686/cyxb2015563
Wang, D., Zhang, Y. T., Chen, C. Y., Chen, R. X., Bai, X. C., Qiang, Z. Q., et al. (2023). The genetic variation in drought resistance in eighteen perennial ryegrass varieties and the underlying adaptation mechanisms. BMC Plant Biol. 23:451. doi: 10.1186/s12870-023-04460-z
Wang, J., Zhuang, L. L., Zhang, J., Yu, J. J., Yang, Z. M., and Huang, B. R. (2019). Identification and characterization of novel homeodomain leucine zipper (HD-zip) transcription factors associated with heat tolerance in perennial ryegrass. Environ. Exp. Bot. 160, 1–11. doi: 10.1016/j.envexpbot.2018.12.023
Wasaya, A., Zhang, X. Y., Fang, Q., and Yan, Z. Z. (2018). Root phenotyping for drought tolerance: a review. Agronomy 8:241. doi: 10.3390/agronomy8110241
Wei, T., Gao, H., Yang, D. Z., Zhu, G., Zhang, C. P., Wang, B. C., et al. (2024). Effects of methyl jasmonate on physiological characteristics and drought resistance of alfalfa (Medicago sativa) and ryegrass (Lolium perenne l.). J. Plant Growth Regul. 19, 1–18. doi: 10.1007/s00344-024-11469-7
Wei, H. J., He, W. Y., Wang, Y., Tang, M., and Chen, H. (2023a). The effects of arbuscular mycorrhizal fungi and melatonin on the heat tolerance of perennial ryegrass. Acta Pratacul. Sin. 32, 126–138. doi: 10.11686/cyxb2023129
Wei, H. J., Li, X., He, W. Y., Kuang, Y. X., Wang, Z. H., Hu, W. T., et al. (2023b). Arbuscular mycorrhizal symbiosis enhances perennial ryegrass growth during temperature stress through the modulation of antioxidant defense and hormone levels. Ind. Crop. Prod. 195:116412. doi: 10.1016/j.indcrop.2023.116412
Xie, L. J., Teng, K., Tan, P. H., Chao, Y. H., Li, Y. R. Z., Guo, W., et al. (2020). PacBio single-molecule long-read sequencing shed new light on the transcripts and splice isoforms of the perennial ryegrass. Mol. Gen. Genomics. 295, 475–489. doi: 10.1007/s00438-019-01635-y
Xing, J., Yang, Y. W., Zhang, Q., Yin, T. C., Zhao, R. J., Hao, G., et al. (2024). A cytochrome P450 gene, LpCYP72A15, confers drought tolerance in perennial ryegrass. Grass Forage Sci. 79, 4–16. doi: 10.1111/gfs.12642
Xing, J., Zhao, R. J., Zhang, Q., Huang, X. R., Yin, T. C., Zhang, J., et al. (2022). Genome-wide identification and characterization of the LpSAPK family genes in perennial ryegrass highlight LpSAPK9 as an active regulator of drought stress. Front. Plant Sci. 13:922564. doi: 10.3389/fpls.2022.922564
Xiong, Z. M., Cai, H., Feng, W. X., and Sun, G. P. (2006). Effects of PP333 on heat tolerance of Lolium perenne under high temperature stress. J. Yangzhou Univ. 27, 98–100. doi: 10.3969/j.issn.1671-4652.2006.04.025
Xiong, X., Dong, J. X., Li, Y. N., Zhu, G. F., and Ding, X. S. (2024). Comparison of drought resistance during germination of four perennial ryegrass varieties. J. Zhejiang Agric. Sci. 65, 2132–2137. doi: 10.16178/j.issn.0528-9017.20230740
Xu, L. X., Han, L. B., and Huang, B. R. (2011). Antioxidant enzyme activities and gene expression patterns in leaves of Kentucky bluegrass in response to drought and post-drought recovery. J. Am. Soc. Hortic. Sci. 136, 247–255. doi: 10.21273/jashs.136.4.247
Xu, M., Li, H., Liu, Z. N., Wang, X. H., Xu, P., Dai, S. J., et al. (2021). The soybean CBL-interacting protein kinase, GmCIPK2, positively regulates drought tolerance and ABA signaling. Plant Physiol. Biochem. 167, 980–989. doi: 10.1016/j.plaphy.2021.09.026
Xu, S., Li, J., Zhang, X., Wei, H., and Cui, L. (2006). Effects of heat acclimation pretreatment on changes of membrane lipid peroxidation, antioxidant metabolites, and ultrastructure of chloroplasts in two cool-season turfgrass species under heat stress. Environ. Exp. Bot. 56, 274–285. doi: 10.1016/j.envexpbot.2005.03.002
Yan, H., and Zhang, X. Q. (2008). Study on the space mutagenic effect of two herbage seeds. J. Anhui Agric. Sci. 36:580. doi: 10.3969/j.issn.0517-6611.2008.02.043
Yang, F. P., Liang, M. Q., Zhang, L. Q., Chen, X. Q., Zhang, X. D., Wang, G. Y., et al. (2006). Improvement of perennial ryegrass (Lolium perenne) tolerance to drought by gene transferring of stress-inducible transcription factor CBF1 gene. Acta Agric. Boreali-Sinica. 21, 14–18. doi: 10.3321/j.issn:1000-7091.2006.01.004
Yang, Z. M., Miao, Y. C., Yu, J. J., Liu, J., and Huang, B. R. (2014). Differential growth and physiological responses to heat stress between two annual and two perennial cool-season turfgrasses. Sci. Hortic. 170, 75–81. doi: 10.1016/j.scienta.2014.02.005
Yang, D., Zhang, Z. W., Fang, Z. F., Wang, W. L., Wu, S. M., Sun, T. X., et al. (2024). Genome-wide identification and analysis of the HD-zip transcription factors in perennial ryegrass highlight LpHOX22 and LpHOX24 as negative regulators of osmotic and heat stresses. Environ. Exp. Bot. 225:105854. doi: 10.1016/j.envexpbot.2024.105854
Yang, Y. J., Zheng, M. Z., Qin, W. Z., Chen, T., Jiang, S. G., and Liu, J. R. (2012). Responses of tall fescue and perennial ryegrass turfgrasses to drought stress and re-watering treatment. Pratac. Sci. 29, 370–376.
Yilmaz, M. B., and Kisakurek, S. (2021). Effect of drought stress on germination and early seedling growth of (Lolium perenne L.) cultivars. Ksu. Tarim. Doga. Derg. 24, 529–538. doi: 10.18016/ksutarimdoga.vi.777692
Yu, X. Q., Bai, G. H., Liu, S. W., Luo, N., Wang, Y., Richmond, D. S., et al. (2013). Association of candidate genes with drought tolerance traits in diverse perennial ryegrass accessions. J. Exp. Bot. 64, 1537–1551. doi: 10.1093/jxb/ert018
Yu, X. Q., Pijut, P. M., Byrne, S., Asp, T., Bai, G. H., and Jiang, Y. W. (2015). Candidate gene association mapping for winter survival and spring regrowth in perennial ryegrass. Plant Sci. 235, 37–45. doi: 10.1016/j.plantsci.2015.03.003
Yu, G. H., Xie, Z. N., Chen, W., Xu, B., and Huang, B. R. (2022). Knock down of NON-YELLOW COLOURING 1-like gene or chlorophyllin application enhanced chlorophyll accumulation with antioxidant roles in suppressing heat-induced leaf senescence in perennial ryegrass. J. Exp. Bot. 73, 429–444. doi: 10.1093/jxb/erab426
Yu, G. H., Xie, Z. N., Zhang, J., Lei, S. S., Lin, W. J., Xu, B., et al. (2021). NOL-mediated functional stay-green traits in perennial ryegrass (Lolium perenne L.) involving multifaceted molecular factors and metabolic pathways regulating leaf senescence. Plant J. 106, 1219–1232. doi: 10.1111/tpj.15204
Zandalinas, S. I., Fritschi, F. B., and Mittler, R. (2021). Global warming, climate change, and environmental pollution: recipe for a multifactorial stress combination disaster. Trends Plant Sci. 26, 588–599. doi: 10.1016/j.tplants.2021.02.011
Zandalinas, S. I., Mittler, R., Balfagon, D., Arbona, V., and Gomez-Cadenas, A. (2018). Plant adaptations to the combination of drought and high temperatures. Physiol. Plant. 162, 2–12. doi: 10.1111/ppl.12540
Zhang, X. Z., Ervin, E. H., Liu, Y. M., Hu, G. F., Shang, C., Fukao, T., et al. (2015). Differential responses of antioxidants, abscisic acid, and auxin to deficit irrigation in two perennial ryegrass cultivars contrasting in drought tolerance. J. Am. Soc. Hortic. Sci. 140, 562–572. doi: 10.21273/jashs.140.6.562
Zhang, X. Y., He, J. G., Sun, X. H., and Wu, J. X. (2011). Transformation of Lolium perenne with a fructan: fructan 1-fructosyltransferase gene from Agropyron cristatum and enhancement of drought tolerance in transgenic plants. Acta Pratacul. Sin. 20, 111–118.
Zhang, J., Li, H., Jiang, Y. W., Li, H. B., Zhang, Z. P., Xu, Z. P., et al. (2020). Natural variation of physiological traits, molecular markers, and chlorophyll catabolic genes associated with heat tolerance in perennial ryegrass accessions. BMC Plant Biol. 20:520. doi: 10.1186/s12870-020-02695-8
Zhang, Y., Li, J. Q., Wang, X. T., Yang, Y. T., Zhou, Z. J., Deng, X. L., et al. (2024). A bibliometric analysis review of the pennisetum (1970–2023). Front. Sust. Food Syst. 8:1405684. doi: 10.3389/fsufs.2024.1405684
Zhang, J., Shi, Y., Zhang, X. Z., Du, H. M., Xu, B., and Huang, B. R. (2017). Melatonin suppression of heat-induced leaf senescence involves changes in abscisic acid and cytokinin biosynthesis and signaling pathways in perennial ryegrass (Lolium perenne L.). Environ. Exp. Bot. 138, 36–45. doi: 10.1016/j.envexpbot.2017.02.012
Zhang, J., Xing, J., Lu, Q. Y., Yu, G. H., Xu, B., and Huang, B. (2019). Transcriptional regulation of chlorophyll-catabolic genes associated with exogenous chemical effects and genotypic variations in heat-induced leaf senescence for perennial ryegrass. Environ. Exp. Bot. 167:103858. doi: 10.1016/j.envexpbot.2019.103858
Zhang, J., Yu, G. H., Wen, W. W., Ma, X. Q., Xu, B., and Huang, B. R. (2016). Functional characterization and hormonal regulation of the PHEOPHYTINASE gene LpPPH controlling leaf senescence in perennial ryegrass. J. Exp. Bot. 67, 935–945. doi: 10.1093/jxb/erv509
Zhao, Y. L., Du, H. W., Wang, Y. K., Wang, H. L., Yang, S. Y., Li, C. H., et al. (2021). The calcium-dependent protein kinase ZmCDPK7 functions in heat-stress tolerance in maize. J. Integr. Plant Biol. 63, 510–527. doi: 10.1111/jipb.13056
Zhao, L. L., Wang, L. T., Chen, K. K., Sun, H., and Wang, P. C. (2024a). Effects of arbuscular mycorrhizal fungi on the growth and physiological performance of Sophora davidii seedling under low-phosphorus stress. J. Plant Growth Regul. 43, 2383–2395. doi: 10.1007/s00344-024-11273-3
Zhao, B. S., Yu, S. M., Lu, Y. R., Liu, S. Q., Wang, G. K., and Wang, Y. (2022). Effects of AM Fungi on growth and physiology of Lolium perenne under high temperature stress. Forestry Environ. Sci. 38, 113–119. doi: 10.3969/j.issn.1006-4427.2022.05.017
Zhao, L. L., Zhao, X., Huang, L., Liu, X. Y., and Wang, P. C. (2024b). Transcriptome analysis of Pennisetum americanum × Pennisetum purpureum and Pennisetum americanum leaves in response to high-phosphorus stress. BMC Plant Biol. 24:635. doi: 10.1186/s12870-024-05339-3
Zhou, S. P., and Abaraha, A. (2007). Response to heat stress in warm season and cool season turf grass cultivars. Sci. Res. Essays 16, 371–376. doi: 10.1002/pon.1070
Zhou, Z. J., Li, J. Q., Gao, Y., Wang, X. T., Wang, R., Huang, H. Y., et al. (2024). Research on drought stress in Medicago sativa L. from 1998 to 2023: a bibliometric analysis. Front. Plant Sci. 15:1406256. doi: 10.3389/fpls.2024.1406256
Zhu, Y. Q., Liao, Z. C., Yang, J., Ye, H. T., Han, C. Y., Xu, W. Z., et al. (2022). Genotypic and phenotypic diversity of heat tolerance among and within perennial ryegrass (Lolium perenne L.). Braz. J. Bot. 45, 1223–1234. doi: 10.1007/s40415-022-00854-9
Zhuang, L. L., Cao, W., Wang, J., Yu, J. G., Yang, Z. M., and Huang, B. R. (2018). Characterization and functional analysis of FaHsfC1b from Festuca arundinacea conferring heat tolerance in Arabidopsis. Int. J. Mol. Sci. 19:2702. doi: 10.3390/ijms19092702
Keywords: perennial ryegrass, drought stress, heat stress, bibliometrics, response mechanism
Citation: Wang R, Gao Y, Li J, Wang X, Yang Y, Huang H, Zhou Z, Wang P and Zhao L (2024) Drought and heat stress studies in perennial ryegrass: a bibliometric analysis 1994–2024. Front. Sustain. Food Syst. 8:1458552. doi: 10.3389/fsufs.2024.1458552
Received: 02 July 2024; Accepted: 18 November 2024;
Published: 04 December 2024.
Edited by:
Aliza Pradhan, National Institute of Abiotic Stress Management (ICAR), IndiaReviewed by:
Zhou Li, Sichuan Agricultural University, ChinaCopyright © 2024 Wang, Gao, Li, Wang, Yang, Huang, Zhou, Wang and Zhao. This is an open-access article distributed under the terms of the Creative Commons Attribution License (CC BY). The use, distribution or reproduction in other forums is permitted, provided the original author(s) and the copyright owner(s) are credited and that the original publication in this journal is cited, in accordance with accepted academic practice. No use, distribution or reproduction is permitted which does not comply with these terms.
*Correspondence: Puchang Wang, d2FuZ3B1Y2hhbmdAMTYzLmNvbQ==
†These authors have contributed equally to this work
Disclaimer: All claims expressed in this article are solely those of the authors and do not necessarily represent those of their affiliated organizations, or those of the publisher, the editors and the reviewers. Any product that may be evaluated in this article or claim that may be made by its manufacturer is not guaranteed or endorsed by the publisher.
Research integrity at Frontiers
Learn more about the work of our research integrity team to safeguard the quality of each article we publish.