- 1School of Food and Pharmacy, Zhejiang Ocean University, Zhoushan, China
- 2Faculty of Science and Technology, Charles Darwin University, Casuarina, NT, Australia
- 3Flinders Institute for Nanoscale and Technology, College of Science and Engineering, Flinders University, Bedford Park, SA, Australia
- 4Food & Nutrition Innovation Hub, RMIT University, Melbourne, VIC, Australia
- 5School of Viniculture, Lincoln University, Lincoln, New Zealand
- 6Materials Technologies and Their Applications Lab, Faculty of Science, Beni-Suef University, Beni Suef, Egypt
- 7Industrial Engineering Department, College of Engineering, King Saud University, Riyadh, Saudi Arabia
Introduction: Prime quality kamaboko (cooked surimi) is produced from Alaska pollock. The main challenge of using cheaper fish fillets, including Barramundi, is achieving similar textures.
Methods: Combined with fish gelatin, modified starch by vortex fluidic device was used to enhance the quality of kamaboko made from Barramundi.
Results: Modification by a continuous flow vortex fluidic device (VFD) significantly reduces the size of particles of starch relative to conventional homogenization. Blending this VFD-modified starch with fish gelatin resulted in a well-organized cross-linked matrix compared to products manufactured from homogenization modified starch. This led to higher gel strength of the former (1,708.32 g/cm2) relative to the latter (832.56 g/cm2). Mixing different proportions of fish gelatin and VFD-processed starch to make Barramundi kamaboko (5, 10, and 15%), yielded a product with greater chewiness relative to Alaska pollock kamaboko. Sensory evaluation indicated that the 5% product was optimal and the 15% product with the highest chewiness (56.10 N) was the least favorable.
Discussion: These results clearly indicate the potential of VFD processed starch, blended with fish gelatin to enhances the quality of kamaboko.
1 Introduction
Surimi is made by stabilizing minced white fish flesh. Cooking surimi after adjusting the water and salt content produces kamaboko. It has a firmer texture and chewier mouth feel compared to surimi. Alaska pollock produces the best quality Kamaboko and surimi (Zhiyu et al., 2024). The major problem faced in the production of these products is the expense of the raw fish. This problem can be ameliorated by using more abundant species of fish, but this may result in reduced quality (Xidong et al., 2023).
Conventionally, transgluminase is added to kamaboko made from second-grade fish species to enhance chewiness by creating cross-linking between proteins. The strengthened protein network not only provides a firmer texture, but also contributes to the structural integrity of the kamaboko during processing (Jia et al., 2019). However, the use of transgluminase in food products is regulated in many countries because of safety concerns. While transglutaminase is generally recognized as safe when used within certain limits, there are concerns about its misuse or overuse. If not properly controlled, it can lead to the cross-linking of unintended proteins and potentially causes allergenic reactions in sensitive individuals (Lerner and Matthias, 2020). Recently, the impact of natural additives on kamaboko’s texture to improve chewiness has been studied. Yaguchi et al. (2017) compared the chewiness of kamaboko made from threadfin tuna containing powders from nine different vegetables and fruits. Similarly, micro- and nano-starch were added in kamaboko made from grass carp to enhance chewiness (Li et al., 2022). Some of these natural additives did enhance the chewiness of kamaboko, but no report has yet demonstrated that this enhancement is able to match the chewiness of kamaboko produced from Alaska pollock.
The interaction between proteins and polysaccharides can alter the structure, stability, and function of biological systems (Yang et al., 2021). In the food industry, protein-polysaccharide interactions are used to stabilize emulsions and foams, improve texture and shelf life, modify viscosity and the texture of food products, create edible films and coatings etc. (Gentile, 2020). Yin et al. (2021) blended fish gelatin and xanthan gum into yoghurt, and increased its viscosity, smoothness and sensory score. Jiang et al. (2021) developed a whey protein-sodium alginate used in low-fat sausage. However, the impact of protein-polysaccharide materials on the texture of kamaboko has hitherto not been studied.
The vortex fluidic device (VFD) (Figure 1) is a thin-film processing platform with a broad variety of research and commercial applications. The development of this processing technology resulted from research into thin-film flow chemistry and microfluidics (Chen et al., 2014; Britton et al., 2017). VFD processing overcomes the restrictions of conventional batch processing by utilizing intense micro-mixing shear forces and high mass transfer (Britton et al., 2016). VFD processing has been extended (Britton et al., 2017) to small-molecule synthesis and drug delivery, as well as the manipulation of single-cell organisms. High rotational rates facilitate the formation of a dynamic thin film from the liquid inside the tube. Film thickness can be controlled by the rotational speed and the volume of liquid (Solheim et al., 2019). A model for the fluid flow in a VFD has recently been developed to describe the high mass transfer topological fluid flows (Alharbi et al., 2021). The mechanism involves a “spinning top” or “typhoon like” topological flow ~1 mm in diameter caused by a Coriolis force acting on the hemispherical base of the tube. Faraday wave eddies twisted by side wall Coriolis force into double helical flow are formed down to ~200 nm in diameter (Xu et al., 2019). The transient localized high pressures and heating caused by this intense mixing can enhance chemical reaction rates and product selectivity. The rate of enzymatic reactions has been significantly increased, with a seven-fold acceleration on average for a range of transformations (Britton et al., 2017). This phenomenon arises from a combination of Faraday pressure waves generated within the thin film, enhancing mass transport, and a collapse of the reaction transition state. Tethering enzymes to the surface of the tube can facilitate the manufacture of complex small molecules in a single pass, while maintaining continuous flow (Britton et al., 2016). VFD processing has been used to facilitate chemical reduction and elimination reactions, and fabricate new materials. For example, VFD processing has been employed to assemble C60 cones, nanotubes, spicules and rods (Britton et al., 2016; Vimalanathan et al., 2017; Alharbi et al., 2021) without the use of surfactants. The VFD is also efficient for mediating the production of diesters at room temperature (Britton et al., 2016) and enables multi-phase oxidation to occur without the need for a phase transfer catalysts or organic solvent (Pye et al., 2018). VFD processing has been employed to blend proteins and polysaccharides to achieve better organized internal structures for entrapping nutrients.
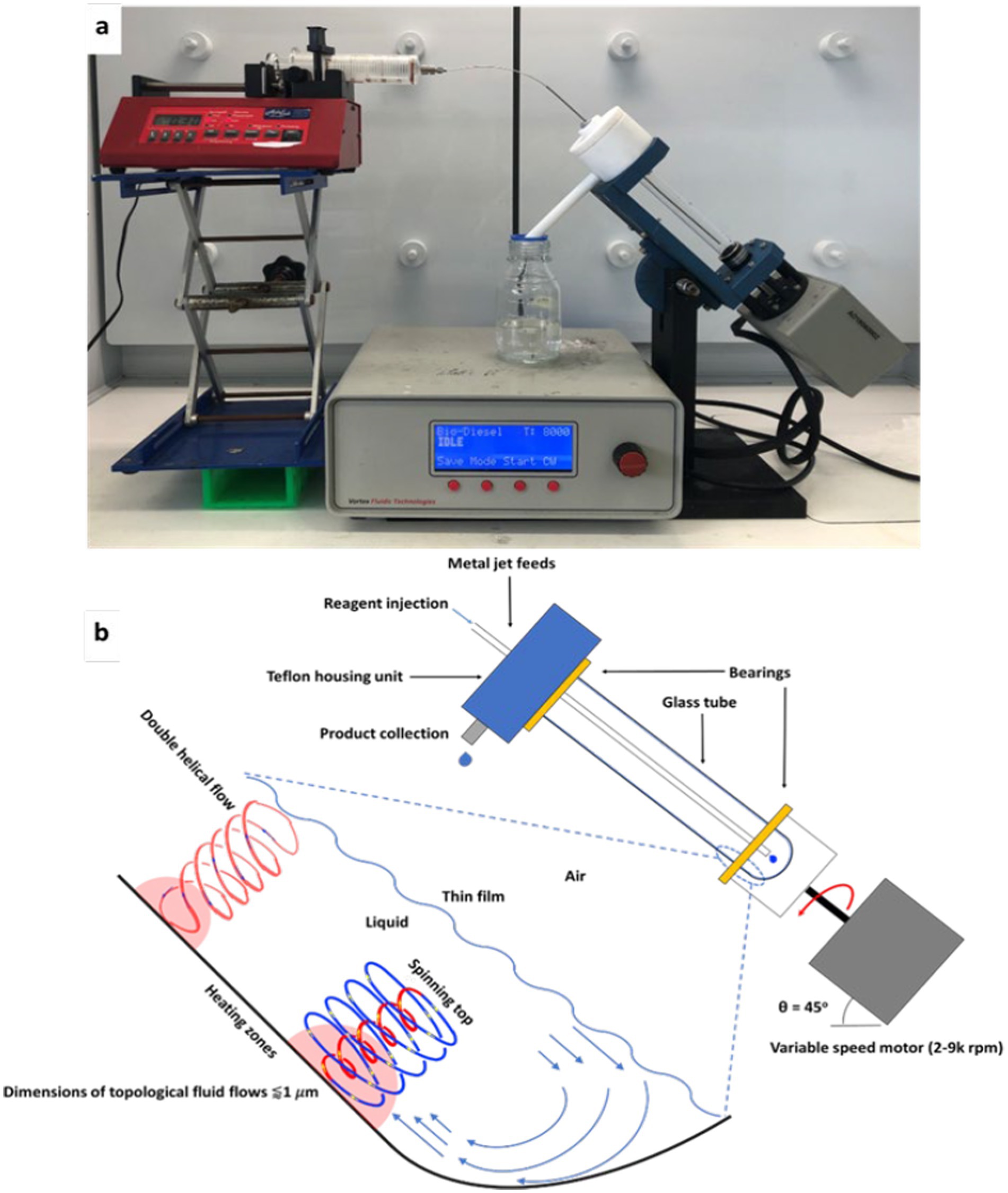
Figure 1. (a) A vortex fluidic device, (b) working operation of VFD Credit: images are reprinted from our previous publication with permission: “Sub-micron moulding topological mass transport regimes in angled vortex fluidic flow,” (Alharbi et al., 2021). “High shear spheroidal topological fluid flow induced coating of polystyrene beads with C60 spicules,” (Jellicoe et al., 2021).
The present work investigated the effect of VFD processing on the internal structure of polysaccharide / protein blended materials, with the aim of improving the quality of kamaboko produced from Barramundi. This novel approach significantly enhanced the chewiness of kamaboko made from this lower-grade fish to a level exceeding that of kamaboko made from Alaska pollock.
2 Materials and methods
2.1 Materials
Ten kg of Barramundi fillet (average weight: 1.1 kg) filleted from Barramundi fish (average weight: 4.3 kg) from the same day was obtained from a local fish market. All chemical materials were procured from Sigma-Aldrich, Inc. (St. Louis, Missouri, United States).
2.2 Modification of starch
Starch (CAS No.: 9005-25-8) solutions (10%, w/v) were treated at 10,000 rpm/min at 25°C for 10 min in a conventional homogenizer, or introduced in to a VFD quartz tube (17.5 mm ID, 20 mm OD, 18.5 cm long) through jet feeds at a flow rate of 0.2 mL/min, rotation rate of 7,000 rpm, and at a 45° tilt angle. The residence time of the sample in the VFD quartz tube was 10 min. Each solution was collected after processing, centrifuged at 4°C for 15 min at 10,000 rpm, and the supernatant freeze-dried to yield the modified starch.
2.3 VFD processing of protein-polysaccharide samples
A slight modification of the method described by Jellicoe et al. (2021) was used to prepare protein-polysaccharide samples. Fish gelatin (CAS No.: 9000-70-8) was mixed with different starch samples at a ratio of 2: 0.375 (w/w) in water to give a final concentration of 0.18 g/mL. The prepared solutions were added through jet feeds to a VFD borosilicate glass tube (20 mm OD, 17 mm ID) at a 45°tilt angle which is optimal for processing in general in the VFD and rotated at 7,000 rpm with a flow rate of 0.2 mL/min at 20–25°C. The choice of speed relates to it being optimal for this type of processing in the device. The VFD processed samples were collected and freeze-dried.
2.4 Kamaboko preparation
A slight modification of the method described by He et al. (2020) was used to prepare kamaboko. Barramundi fish fillets were minced and placed into bags with cold water at 2°C. Each bag was tumbled for 15 min at 4 rpm, left to settle for 20 min, and poured through 4-layers of cheesecloth. This washing procedure was repeated twice following the standard surimi washing procedure (He et al., 2020). To prepare surimi, the washed mince was dewatered with a wine press and the moisture content calculated by weight difference after drying in an oven (LAF Tech PTY LTD, Boronia, VIC, Australia) at 130°C for 24 h. A salt ice-water solution was sprinkled over the mince to adjust the moisture and salt content. A food processor was used to mix the water, salt and washed mince for 3–4 min at 4°C.
Kamaboko was prepared from 50 g of surimi compressed into stainless steel tubes (L: 10 cm; id: 2.5 cm) that had been sprayed internally with canola oil to reduce adhesion. Both ends of the tubes were then sealed with screw-thread caps. Tubes were refrigerated at 4°C for 24 h to obtain low temperature setting followed by heating in a steam double-jacketed kettle at 90°C for 30 min. The tubes were immediately transferred to an ice-water bath and held there for 15 min before overnight refrigeration.
2.5 Dynamic light scattering (DLS)
DLS (Nano ZS90, Malvern Instruments, Worcester, UK) was utilised to determine the particle size distribution using a He-Ne 633 nm wavelength laser at a detection angle of 173°. A Malvern Zeta Sizer was employed to measure the time-dependent variation of the light scattering.
2.6 Scanning Electron microscopy (SEM)
SEM (Inspect FEI F50 SEM (PS216), FEI Company, Hillsboro, Oregon, USA) was performed on a 2.0 mm spot size using 5.0 Ky. Liquid samples (20 μL) were dropped on a silicon substrate followed by air drying for 12 h. The freeze-dried samples were immersed for 2 min in liquid nitrogen followed by slicing into 5 mm x 5 mm x 2 mm cross sections before platinum sputtering.
2.7 Rheological measurements
The rheological properties of samples were evaluated using a Brookfield viscometer fitted with a thermos-reservoir and temperature controller (LV-DVIII, HT-60, HT-110FR, respectively; Brookfield Engineering Laboratories, Inc.; Middleboro, MA, USA). The thermal reservoir had a sample chamber and spindle (HT-2 and SC4-18 respectively). A cooling plug assembly (HT-26Y) was used to cool the system to 25°C. Viscosity profiles were measured using flow mode stepping: the shear rate of the spindle was customized from 0.01 to 500.00 rad.s−1 at each 5 s pause. For the strain sweep, shear frequency was held at 6.283 rad.s−1. The strain sweep ranged from 1% to 10,000%. For the frequency sweep, the shear strain was held at 0.4 and frequency values varied over the range 0.1 to 10 s−1.
2.8 Determination of gel strength
Gels (6.67%, w/v) were prepared by dissolving each dry sample in distilled water at 60°C for 1 h in a bloom jar, followed by cooling to 20–25°C for 15 min. The prepared solutions were kept at 4–7°C for 16–18 h and the gel strength measured using a Texture Analyzer (TA.XT2i Texture Analyzer; Stable Micro Systems, Godalming, Surrey) employing a method reported by Wainewright (1977).
2.9 Determination of kamaboko chewiness
A slight modification of the procedure described by Chou (2020) was used to determine the chewiness of kamaboko, employing a EZ Test-500 N Texture Analyzer (TA.XT2i; Stable Micro Systems, Godalming, Surrey). The Kamaboko samples were prepared by slicing into pieces (3 cm × 3 cm × 1 cm). Two compression tests were conducted on each sample at a 60 mm/min compression speed using a 1 cm rounded probe (550 N load cell, pre-test speed: 100 m/s; test speed: 5 m/s; post-test speed: 5 m/s). Data generated by two compression tests shown in Figure 2 was used to determine the chewiness of samples using the formulation shown in Table 1.
2.10 Sensory evaluation
Sensory evaluation was conducted using the protocol of Yin et al. (2021) with slight modifications. Eight-four volunteers were trained for 1 h, then for five rounds of triangle testing. Participants who scored poorly twice in the five rounds were eliminated and 56 participants were selected as panelists based on their good scores in the triangle test. Parameters including color, taste, aroma, texture and overall acceptance were measured on a hedonic scale (1: dislike extremely; 10: like extremely). Coded samples were placed randomly in front of the panelist in plastic containers. Panelists were required to rinse their palates between samples. The collected data was analysed using Sensory Management System hardware (2006) and computerized sensory software (Sensory Integrated Management System, Morristown, NJ, USA).
2.11 Statistic analysis
Date represent the means + standard deviation of three replicates. They were analyzed by analysis of variance (ANOVA) using SPSS software V23 (IBM PTY. LTD, Armonk, NY, USA). Least significant difference (LSD, 5% level) was used to separate means when a significant p-value was obtained.
3 Results and discussion
3.1 Modified starch
Samples of modified (VFD processed and conventionally homogenized) and unmodified starch were solubilized in water and particle sizes measured by dynamic light scattering (DLS). Both the modified starches had smaller particle sizes by comparison to the unmodified starch. The average particle size from VFD treatment (100–190 nm) was smaller than that obtained from homogenization (190–615 nm).
The microstructure of samples before and after modification were investigated by SEM (Figure 3). Again, the particles sizes of modified starch were smaller (Figure 4). Mechanical shear forces break down starch granules, leading to changes in physicochemical properties (Guo et al., 2023). The topological fluid flows and associated local heating generated by VFD processing (Figure 1b) generates much stronger shear force than conventional homogenization. Some of us have recently reported that VFD processing could produce 200 nm encapsulated fish oil particles, compared to the micrometer sized particles generated by homogenization (He et al., 2020).
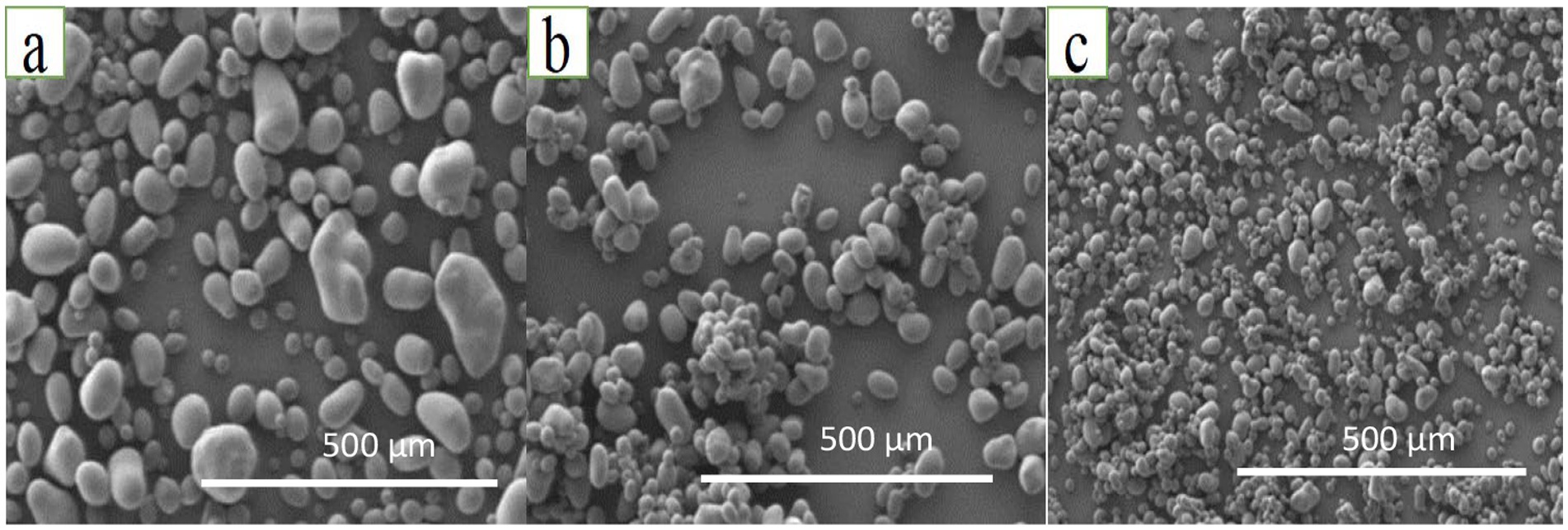
Figure 3. SEM images of starch before and after modification. (a) Original starch without modification; (b). Modified homogenized starch. (c) VFD processed starch.
The gel strengths of starch samples before and after modification are compared in Figure 5. Modified starch processed by VFD processing showed a significantly higher gel strength than other two samples. Modification such as acetylation, phosphorylation, or cross-linking is known to increase thickening and gelling characteristics (Chakraborty et al., 2022). These modifications can make starch molecules more resistant to shear forces and mechanical breakdown, resulting in higher gel strength (Compart et al., 2023). VFD-modified starch was therefore used to prepare Barramundi kamaboko, with the expectation of enhanced gel strength.
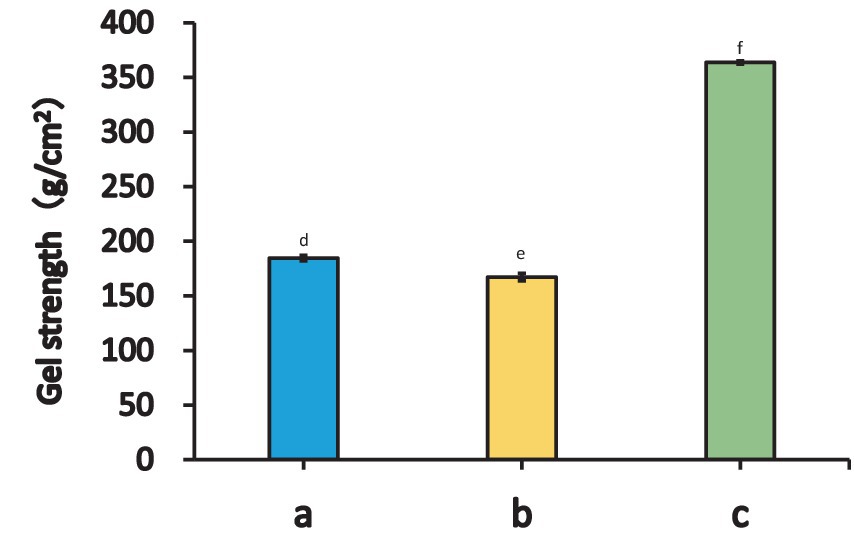
Figure 5. Gel strength of starch before and after modification (Different letters on different columns indicate significant differences of p < 0.05 based on the one-way ANOVA and LSD test). (a) Unmodified starch. (b) Homogenized starch. (c) VFD processed starch.
3.2 Kamaboko produced from Alaska pollock, Barramundi, and Barramundi with VFD-modified starch
The viscosity and chewiness of kamaboko samples prepared from the modified and unmodified ingredients are compared in Figures 6, 7, respectively. Chewiness is commonly used as a criterion for evaluating the quality of kamaboko because consumers often associate the quality of kamaboko with its texture, and chewiness serves as a key indicator of freshness, flavor retention, and overall craftsmanship (Nishinari et al., 2024). Kamaboko samples produced from Alaska pollock and Barramundi were served as the positive and negative controls, respectively. Starch and modified starch have been reported to enhance the chewiness of kamaboko produced from Alaska pollock by cross-linking the protein matrix (Jiang et al., 2021), and this enhancement is positively correlated with gel strength. To our surprise, however, the VFD-modified starch which had the highest gel strength, did not have a positive impact on the chewiness of kamaboko produced from Barramundi (Figure 7). The reason for this unexpected result became apparent on consideration of the internal structures observed by SEM (Figure 8). Unlike the kamaboko produced from Alaska pollock (positive control) with clear cross-linking in the SEM image, the corresponding image of Barramundi kamaboko made with VFD-modified starch revealed an overlaid, flaky structure, similar to that made from Barramundi without starch (negative control). This flaky structure results from the low myosin content of Barramundi fillet, the major cross-linking protein (do Vale et al., 2020). Myosin is a major cross-linking protein in muscle tissues, including fish like Barramundi, due to its role in muscle contraction and structure. Myosin is one of the two main proteins (along with actin) that make up the muscle fibers. During muscle contraction and post-mortem changes, the myosin filaments interact with actin, leading to cross-linking between these proteins (Hoi-Ting et al., 2023). When a muscle is cooked, myosin denatures, and the cross-links it forms contribute to the texture and firmness of the final product. If the myosin content is low, as with Barramundi, the structure may not be as firm, which can result in a more delicate or flaky texture in the cooked fillet (Andre, 2023).
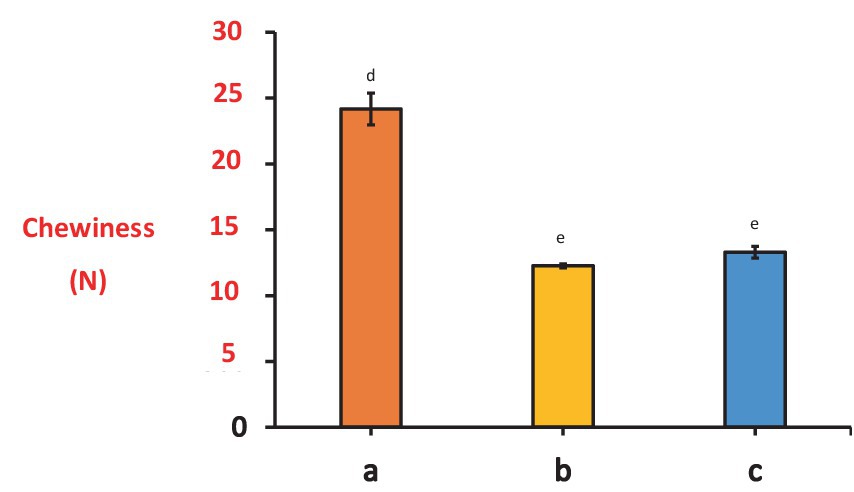
Figure 7. The chewiness of kamaboko produced by different methods (Different letters on different columns indicate significant differences of p < 0.05 based on the one-way ANOVA and LSD test). (a) Kamaboko produced from Alaska pollock. (b) Kamaboko produced from Barramundi. (c) Kamaboko produced from Barramundi with VFD-modified starch.
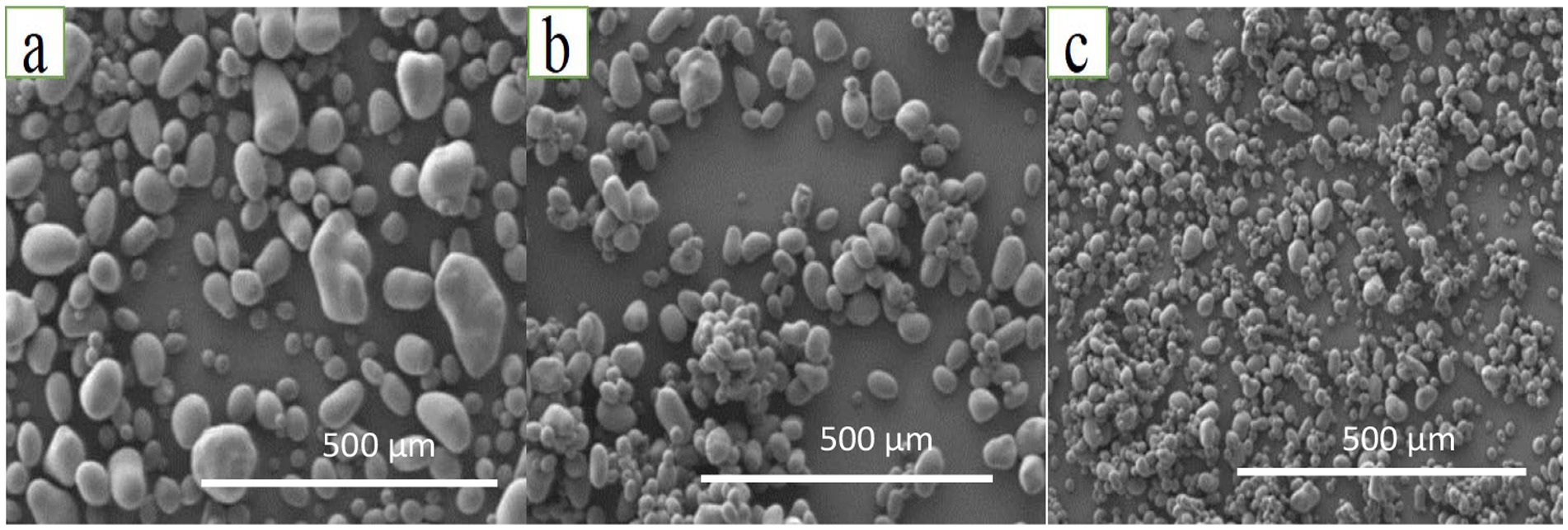
Figure 8. SEM images of kamaboko produced by different methods. (a) Kamaboko produced from Alaska pollock. (b) Kamaboko produced from Barramundi. (c) Kamaboko produced from Barramundi with VF-modified starch.
3.3 Starch-fish gelatin interaction
SEM images of the internal structure of fish gelatin blended with unmodified starch, homogenized starch and VFD-processed starch are shown in Figure 9. The cross-linking of the matrix formed with modified starch (Figures 9b,c) is more obvious than that in the image of the sample made from unmodified starch. This is presumably due to the reduced particle size of modified starch (Figures 4, 3). The larger surface area of smaller particles facilitates interaction with proteins (Kopac, 2021). The VFD-modified starch has the smallest particle size and the most obvious cross-linked matrix. This cross-linking also correlates with gel strength (Figure 10) (Wu and McClements, 2015). The sample formed from blending of fish gelatin and VFD-modified starch displayed the most cross-linking and exhibited the highest gel strength (1,708.32 g/cm2). More cross-linking of proteins is associated with higher gel strength because the cross-links form a more tightly connected and stable network within the gel. Cross-linking involves the formation of chemical or physical bonds between different protein molecules or between proteins and other components, such as starch. These bonds help to stabilize the structure of the gel by making the protein network more cohesive and resistant to deformation. The more cross-links that form, the more interconnected the network becomes. A higher cross-linked network increases the gel’s resistance to external forces, such as compression or cutting. This results in a gel with higher rigidity and strength, which is measured as gel strength. Essentially, more cross-links allow the gel to maintain its shape and structure better under stress, contributing to higher gel strength.
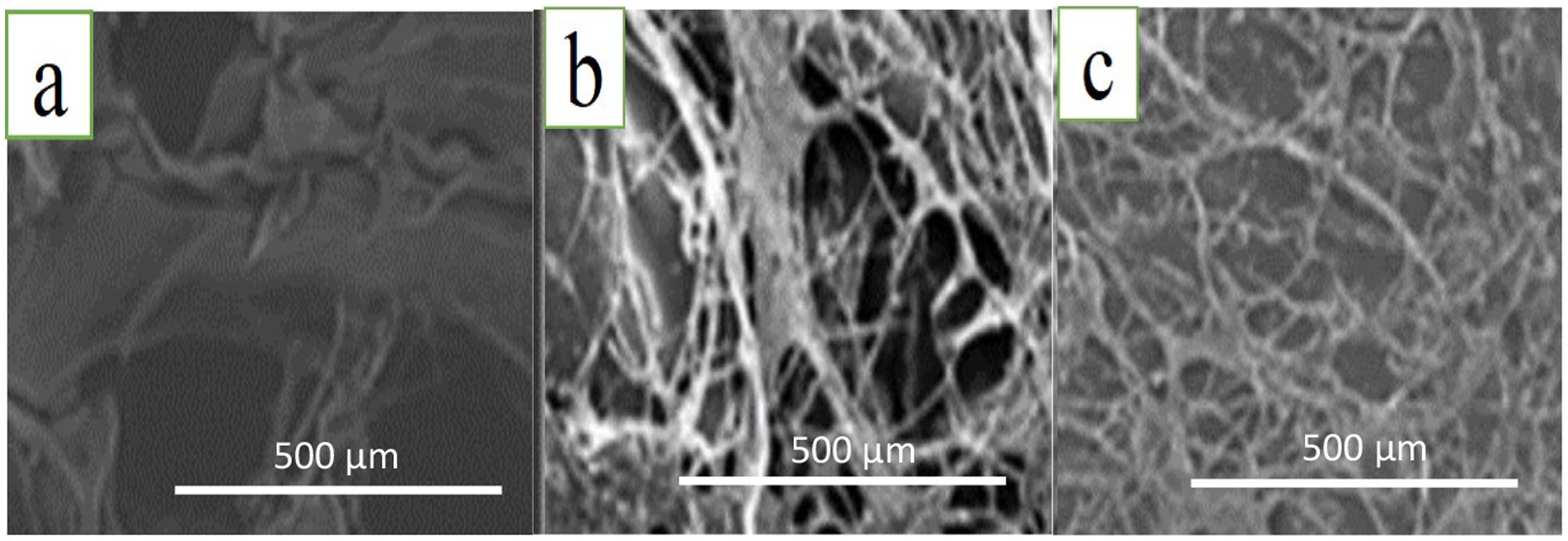
Figure 9. SEM images of different starch samples blended with fish gelatin. (a). Unmodified starch blended with fish gelatin. (b) Homogenized starch blended with fish gelatin. (c). VFD processed starch blended with fish gelatin.
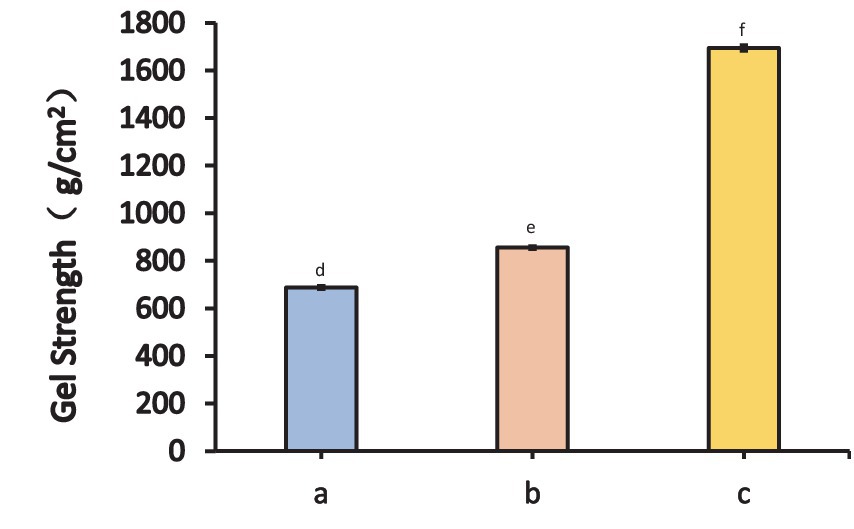
Figure 10. Gel strength of blended samples of starch with fish gelatin (Different letters on different columns indicate significant differences of p < 0.05 based on the one-way ANOVA and LSD test). (a) Unmodified starch blended with fish gelatin. (b). Homogenized starch blended with fish gelatin. (c) VFD processed starch blended with fish gelatin.
Kamaboko produced from Barramundi fillet and VFD-processed starch blended with fish gelatin
SEM images of the internal structure of kamaboko made from Barramundi fillet and 5% (w/w), 10% (w/w), and 15% (w/w) of VFD-processed starch blended with fish gelatin are shown in Figure 11. Without addition of this blended material, no regular cross-linking structure is observed, but rather the internal structure consists of irregular, overlaid flakes (Figures 7b,c). However, the addition of VFD processed starch with fish gelatin yielded a regular crosslinked structure for all three proportions (Figure 11). These cross-linked structure shown in Figure 11 is totally different from the overlaid, flaky structure of Barramundi kamaboko without fish gelatin (Figure 8b). The addition of VFD processed starch with fish gelatin yields a regular cross-linked structure of Barramundi kamaboko because the modified starch and gelatin create a foundational cross-linked network (Figure 9c) that serves as a structural framework for other proteins to attach and form a stable, organized matrix. The cross-linked structure of VFD processed starch and gelatin not only creates a stable matrix but also facilitates the attachment of other proteins, such as myosin and actin. Once the initial cross-linked framework is established by the gelatin and starch, the remaining proteins in the system have a pre-existing structure to attach to, which improves the overall integrity and regularity of the final gel. Without this foundation, the proteins are not able to organize as effectively, potentially leading to irregular, flaky textures (Mingyu et al., 2024). Furthermore, the formation of cross-linking significantly increased the chewiness of Barramundi kamaboko, exceeding that of Alaska pollock kamaboko (Figures 7, 12).
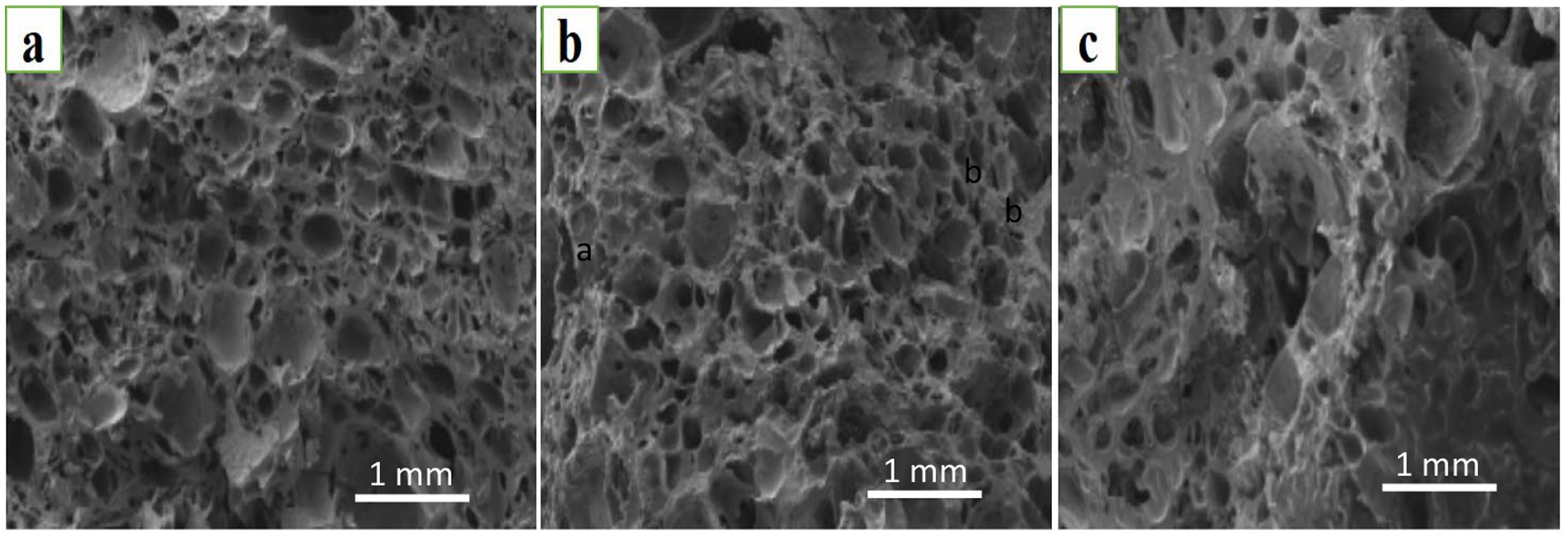
Figure 11. SEM images of kamaboko produced from different ratios of VFD processed starch blended with fish gelatin. (a) 5%; (b) 10%; (c) 15%.
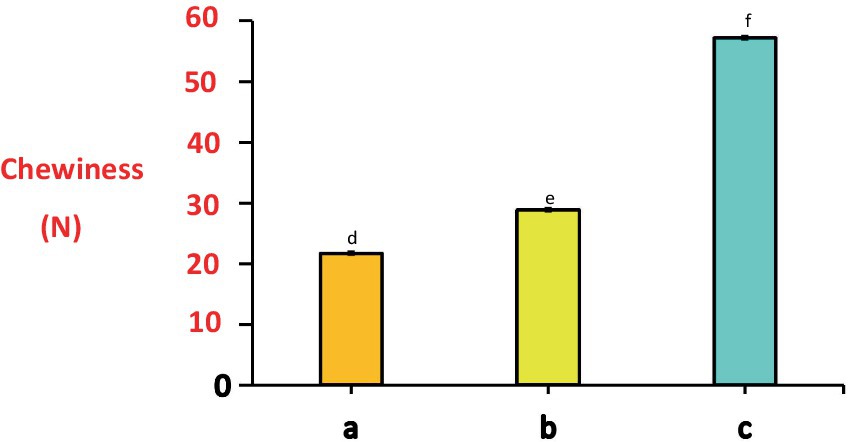
Figure 12. Chewiness of Barramundi kamaboko produced from different ratios of VFD processed starch blended with fish gelatin (Different letters on different columns indicate significant differences of p < 0.05 based on the one-way ANOVA and LSD test). (a) 5%; (b) 10%; (c) 15%.
3.4 Sensory testing
The sensory properties of kamaboko produced from Barramundi fillet and VFD-processed starch with fish gelatin (5, 10, 15%) was compared with those of kamaboko produced from Alaska pollock (Figure 13). The panelists showed a unanimous dislike for kamaboko with 15% (w/w) modified starch/fish gelatin, which we attribute to,the very high chewiness of this sample (Figure 12). While consumers enjoy a certain level of chewiness in kamaboko, excessive chewiness can detract from the eating experience and is associated with a lack of mouthfeel and freshness (Melissa and Kadri, 2021). Chewiness in food is enjoyable up to a certain point because it provides resistance and allows the consumer to experience the product’s texture. However, when the chewiness becomes excessive, it can lead to sensory fatigue, where the consumer feels like they have to chew too much to break down the food. This prolonged effort reduces the enjoyment of eating and can make the product feel laborious to consume (Xingyu et al., 2023). Other samples were given similar scores for flavor, chewiness, and overall preference. The quality of Barramundi kamaboko with 5% (w/w) and 10% (w/w) blended material matched the perceived quality of Alaska pollock kamaboko. This sensory test set up the guideline of adding percentage regarding fish gelatin in Barramundi kamaboko with VFD-processed starch for positive impact on downstream commercial utilization, which is 5–10% (w/w).
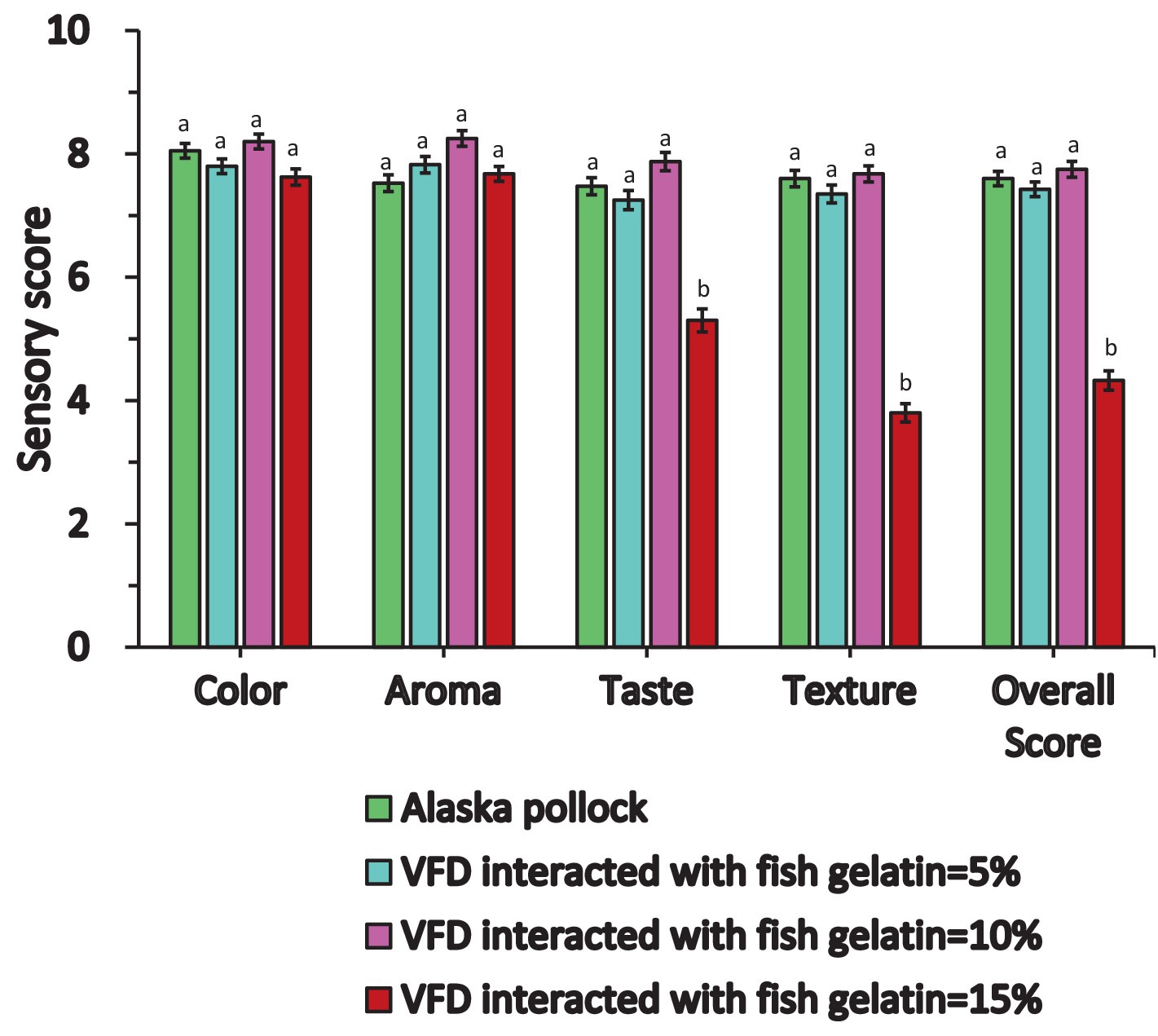
Figure 13. Sensory evaluation of different kamaboko samples (For the same group of columns, different letters on different columns indicate significant differences of p < 0.05 based on the one-way ANOVA and LSD test; columns in different groups were not compared).
4 Conclusion
This study introduces a novel application of VFD-processed starch, which, when blended with fish gelatin, significantly enhances the gel strength and chewiness of Barramundi kamaboko. VFD processing of starch reduced its particle size more efficiently than conventional homogenization. Blending this VFD-modified starch with fish gelatin resulted in material with a higher gel strength (1,708.32 g/cm2) than the corresponding homogenization-modified starch/gelatin blend (832.56 g/cm2). We attribute this difference to the enhanced cross-linking of the former. Mixing 15% (w/w) of this blended material with Barramundi resulted in kamaboko with the highest chewiness (56.10 N), exceeding that of Alaska pollock kamaboko (23.65 N). However, this sample scored poorly in sensory testing, with a marked preference for 5% (w/w) blended material in Barramundi kamaboko, which enjoyed similar sensory scores to Alaska pollock kamaboko. This study suggests that combining the smaller particle VFD processed starch with fish gelatin could be a promising additive to a variety of seafood products, particularly kamaboko. Future research should focus on scaling up this development from lab processing into industrial manufacturing.
Data availability statement
The datasets presented in this study can be found in online repositories. The names of the repository/repositories and accession number(s) can be found in the article/supplementary material.
Author contributions
JL: Resources, Software, Writing – original draft. YW: Conceptualization, Data curation, Writing – original draft. HW: Methodology, Resources, Writing – original draft. MJ: Project administration, Software, Writing – original draft. SH: Funding acquisition, Investigation, Supervision, Writing – review & editing. DY: Conceptualization, Data curation, Writing – review & editing. ST: Investigation, Methodology, Writing – review & editing. CR: Resources, Software, Writing – review & editing. CB: Resources, Software, Writing – review & editing. BT: Writing – review & editing, Resources. MA: Methodology, Software, Writing – review & editing. AE-S: Resources, Validation, Writing – review & editing.
Funding
The author(s) declare that financial support was received for the research, authorship, and/or publication of this article. The authors acknowledge support of this work by the Australian Research Council (DP230100479). The authors extend their appreciation to King Saud University for funding this work through Researchers Supporting Project number (RSP2024R133), King Saud University, Riyadh, Saudi Arabia.
Conflict of interest
The authors declare that the research was conducted in the absence of any commercial or financial relationships that could be construed as a potential conflict of interest.
Publisher’s note
All claims expressed in this article are solely those of the authors and do not necessarily represent those of their affiliated organizations, or those of the publisher, the editors and the reviewers. Any product that may be evaluated in this article, or claim that may be made by its manufacturer, is not guaranteed or endorsed by the publisher.
References
Alharbi, T. M., Jellicoe, M., Luo, X., Vimalanathan, K., Alsulami, I. K., Harbi, B. S. A., et al. (2021). Sub-micron moulding topological mass transport regimes in angled vortex fluidic flow. Nanoscale Adv. 3, 3064–3075. doi: 10.1039/D1NA00195G
Andre, T. (2023). Eccentric muscle contractions: from single muscle fibre to whole muscle mechanics. Eur. J. Phys. 475, 421–435. doi: 10.1007/s00424-023-02794-z
Britton, J., Meneghini, L. M., Raston, C. L., and Weiss, G. A. (2016). Accelerating enzymatic catalysis using vortex fluidics. Angew. Chem. Int. Ed. 55, 11387–11391. doi: 10.1002/anie.201604014
Britton, J., Smith, J. N., Raston, C. L., and Weiss, G. A. (2017). Protein folding using a vortex fluidic device. Berlin: Springer.
Chakraborty, I., N, P., Mal, S. S., Paul, U. C., Rahman, M. H., and Mazumder, N. (2022). An insight into the gelatinization properties influencing the modified starches used in food industry: a review. Food Bioprocess Technol. 15, 1195–1223. doi: 10.1007/s11947-022-02761-z
Chen, X., Smith, N. M., Iyer, K. S., and Raston, C. L. (2014). Controlling nanomaterial synthesis, chemical reactions and self assembly in dynamic thin films. Chem. Soc. Rev. 43, 1387–1399. doi: 10.1039/C3CS60247H
Chou, C. F. (2020). Evaluation of quality properties of emulsified pork sausages containing sorghum distillers grains. J. Food Process. Preserv. 44:e14968.
Compart, J., Singh, A., Fettke, J., and Apriyanto, A. (2023). Customizing starch properties: a review of starch modifications and their applications. Polymers 15:491. doi: 10.3390/polym15163491
do Vale, D. A., Vieira, C. B., de Oliveria, J. M., Vidal, M. F., de Alcântara, L. O., da Silva, A. I. M., et al. (2020). Determining the wetting capacity of the chitosan coatings from Ucides cordatus and evaluating the shelf-life quality of Scomberomorus brasiliensis fillets. Food Control 116:107329. doi: 10.1016/j.foodcont.2020.107329
Gentile, L. (2020). Protein–polysaccharide interactions and aggregates in food formulations. Curr. Opin. Colloid Interface Sci. 48, 18–27. doi: 10.1016/j.cocis.2020.03.002
Guo, L., Chen, H., Zhang, Y., Yan, S., Chen, X., and Gao, X. (2023). Starch granules and their size distribution in wheat: biosynthesis, physicochemical properties and their effect on flour-based food systems. Comput. Struct. Biotechnol. J. 21, 4172–4186. doi: 10.1016/j.csbj.2023.08.019
He, S., Joseph, N., Mirzamani, M., Pye, S. J., Al-Anataki, A. H. M., Whitten, A. E., et al. (2020). Vortex fluidic mediated encapsulation of functional fish oil featuring in situ probed small angle neutron scattering. NPJ Sci. Food 4:12. doi: 10.1038/s41538-020-00072-1
Hoi-Ting, A. H., Jeffrey, J. K., Julien, O., and Simon, M. H. (2023). Slow myosin heavy chain 1 is required for slow myofibril and muscle fibre growth but not for myofibril initiation. Dev. Biol. 499, 47–58. doi: 10.1016/j.ydbio.2023.04.002
Jellicoe, M., Vimalanathan, K., Gascooke, J. R., Luo, X., and Raston, C. L. (2021). High shear spheroidal topological fluid flow induced coating of polystyrene beads with C 60 spicules. Chem. Commun. 57, 5638–5641. doi: 10.1039/D0CC07165J
Jia, R., Jiang, Q., Kanda, M., Tokiwa, J., Nakazawa, N., Osako, K., et al. (2019). Effects of heating processes on changes in ice crystal formation, water holding capacity, and physical properties of surimi gels during frozen storage. Food Hydrocoll. 90, 254–265. doi: 10.1016/j.foodhyd.2018.12.029
Jiang, X., Chen, Q., Xiao, N., Du, Y., Feng, Q., and Shi, W. (2021). Changes in gel structure and chemical interactions of Hypophthalmichthys molitrix surimi gels: effect of setting process and different starch addition. Food Secur. 11:9. doi: 10.3390/foods11010009
Kopac, T. (2021). Protein corona, understanding the nanoparticle–protein interactions and future perspectives: a critical review. Int. J. Biol. Macromol. 169, 290–301. doi: 10.1016/j.ijbiomac.2020.12.108
Lerner, A., and Matthias, T. (2020). Processed food additive microbial transglutaminase and its cross-linked gliadin complexes are potential public health concerns in celiac disease. Int. J. Mol. Sci. 21:127. doi: 10.3390/ijms21031127
Li, X., Fan, M., Huang, Q., Zhao, S., Xiong, S., Yin, T., et al. (2022). Effect of micro- and nano-starch on the gel properties, microstructure and water mobility of myofibrillar protein from grass carp. Food Chem. 366:130579. doi: 10.1016/j.foodchem.2021.130579
Melissa, T., and Kadri, K. (2021). Sensory impairment: natural result of aging. J. Sens. Stud. 36:693. doi: 10.1111/joss.12693
Mingyu, H., Meng, Z., Tian, G., Le, C., Yue, L., Yuyang, H., et al. (2024). Assembly of soy protein-corn starch composite gels by thermal induction: structure, and properties. Food Chem. 434:137433. doi: 10.1016/j.foodchem.2023.137433
Nishinari, K., Peyron, M.-A., Yang, N., Gao, Z., Zhang, K., Fang, Y., et al. (2024). The role of texture in the palatability and food oral processing. Food Hydrocoll. 147:109095. doi: 10.1016/j.foodhyd.2023.109095
Pye, S. J., Dalgarno, S. J., Chalker, J. M., and Raston, C. L. (2018). Organic oxidations promoted in vortex driven thin films under continuous flow. Green Chem. 20, 118–124. doi: 10.1039/C7GC03352D
Solheim, T. E., Salvemini, F., Dalziel, S. B., and Raston, C. L. (2019). Neutron imaging and modelling inclined vortex driven thin films. Sci. Rep. 9:2817. doi: 10.1038/s41598-019-39307-x
Vimalanathan, K., Shrestha, R. G., Zhang, Z., Zou, J., Nakayama, T., and Raston, C. L. (2017). Surfactant-free fabrication of fullerene C60 Nanotubules under shear. Angew. Chem. 129, 8518–8521. doi: 10.1002/ange.201608673
Wu, B., and McClements, D. J. (2015). Microgels formed by electrostatic complexation of gelatin and OSA starch: potential fat or starch mimetics. Food Hydrocoll. 47, 87–93. doi: 10.1016/j.foodhyd.2015.01.021
Xidong, J., Huayu, Y., Xingying, L., Hongwei, C., and Nana, Z. (2023). Green and sustainable microwave processing of surimi seafood: a review of protein compotent interactions, mechanisms, and industrial applications. Trends Food Sci. Technol. 143:104266. doi: 10.1016/j.tifs.2023.104266
Xingyu, Z., Jinghu, Y., Shanhua, Q., and Yuyao, C. (2023). Study on texture detection of gelatin-ager composite gel based on bionic chewing. J. Food Meas. Charact. 17, 5093–5102. doi: 10.1007/s11694-023-02016-1
Xu, J., Xia, R., Zheng, L., Yuan, T., and Sun, R. (2019). Plasticized hemicelluloses/chitosan-based edible films reinforced by cellulose nanofiber with enhanced mechanical properties. Carbohydr. Polym. 224:115164. doi: 10.1016/j.carbpol.2019.115164
Wainewright, F. W. (1977). Physical tests for gelatin and gelatin products. The Science and Technology of Gelatin 507–531.
Yaguchi, S., Shimoda, M., Fukushima, H., and Maeda, T. (2017). Comparison of gel strength of kamaboko containing powders from nine different vegetables and fruits. J. Natl. Fish. Univ. 65, 1–8.
Yang, X., Li, A., Li, D., Guo, Y., and Sun, L. (2021). Applications of mixed polysaccharide-protein systems in fabricating multi-structures of binary food gels—a review. Trends Food Sci. Technol. 109, 197–210. doi: 10.1016/j.tifs.2021.01.002
Yin, M., Yang, D., Lai, S., and Yang, H. (2021). Rheological properties of xanthan-modified fish gelatin and its potential to replace mammalian gelatin in low-fat stirred yogurt. LWT 147:111643. doi: 10.1016/j.lwt.2021.111643
Keywords: vortex fluidic device (VFD), modified starch, protein-polysaccharide interaction, Barramundi kamaboko, sensory score
Citation: Lin J, Wu Y, Wang H, Jellicoe M, He S, Young DJ, Thennadil S, Raston CL, Brennan C, Tian B, Abukhadra MR and El-Sherbeeny AM (2024) Quality enhancement of Barramundi kamaboko using vortex fluidic device modified starch and fish gelatin. Front. Sustain. Food Syst. 8:1445762. doi: 10.3389/fsufs.2024.1445762
Edited by:
Elena Velickova, Saints Cyril and Methodius University of Skopje, North MacedoniaReviewed by:
Roni Nugraha, IPB University, IndonesiaPing-Hsiu Huang, Jiangsu Food & Pharmaceutical Science College, China
Poonam Singh, LaserLeap Technologies, Portugal
Copyright © 2024 Lin, Wu, Wang, Jellicoe, He, Young, Thennadil, Raston, Brennan, Tian, Abukhadra and El-Sherbeeny. This is an open-access article distributed under the terms of the Creative Commons Attribution License (CC BY). The use, distribution or reproduction in other forums is permitted, provided the original author(s) and the copyright owner(s) are credited and that the original publication in this journal is cited, in accordance with accepted academic practice. No use, distribution or reproduction is permitted which does not comply with these terms.
*Correspondence: Shan He, aGUwMDkxQGdtYWlsLmNvbQ==; c2hhbi5oZUBjZHU=; David J. Young, RGF2aWQuWW91bmdAY2R1LmVkdS5hdQ==; Suresh Thennadil, U3VyZXNoLlRoZW5uYWRpbEBjZHUuZWR1LmF1; Colin L. Raston, Y29saW4ucmFzdG9uQGZsaW5kZXJzLmVkdS5hdQ==
†These authors have contributed equally to this work and share first authorship