- 1National Institute of Agricultural Research, Rabat, Morocco
- 2Laboratory of Agro-Alimentary and Health, Faculty of Sciences and Techniques, Hassan First University of Settat, Settat, Morocco
- 3Higher Institute of Nursing Professions and Health Technical (ISPITS), Marrakech, Morocco
- 4Laboratory of Physical Chemistry of Processes and Materials, Faculty of Sciences and Techniques, Hassan First University, Settat, Morocco
- 5Center of Biomedical Research, Romanian Academy, Iasi, Romania
- 6Faculty of Dental Medicine, Apollonia University, Iasi, Romania
- 7Department of Biology, Faculty of Biology, Alexandru Ioan Cuza University of Iasi, Iasi, Romania
- 8Academy of Romanian Scientists, Bucharest, Romania
- 9“Ioan Haulica” Institute, Apollonia University, Iasi, Romania
- 10Faculty of Medicine, University of Medicine and Pharmacy “Grigore T. Popa”, Iasi, Romania
Introduction: Phenacoccus solenopsis Tinsley, poses a significant threat to a range of crops worldwide. This study aimed to evaluate the efficacy of entomopathogenic fungi (Alternaria murispora and Alternaria destruens) and bacteria (Streptomyces bellus-E23-2) against adult females of P. solenopsis under laboratory (26 ± 2°C) and greenhouse conditions.
Methods: Laboratory trials tested A. murispora, A. destruens (104–1010 conidia mL−1), and S. bellus E23-2 (104–1010 cfu mL−1), alone and in combination, recording mortality rates and LC50 values. Greenhouse trials tested the best lab treatments on infested potato plants, monitoring pest density and plant quality.
Results and discussion: In laboratory trials, A. murispora at 1010 conidia mL−1 was the most effective, achieved 79.7% mortality (LC50 = 1.338 × 108 conidia mL−1 after 14 days). Combination treatments significantly enhanced efficacy, with A. murispora + S. bellus E23-2 (1010 conidia mL−1 + 1010 cfu mL−1) reaching 85.3% mortality. In greenhouse trials, the combination treatments notably reduced P. solenopsis densities and increased the number of infected mealybugs, with A. murispora + S. bellus E23-2 being the most effective. These treatments did not harm plant quality, unlike imidacloprid, which reduced visual quality despite its high efficacy. Alternaria murispora and S. bellus E23-2 effectively control P. solenopsis, providing a sustainable, plant-safe alternative to chemical insecticides.
1 Introduction
The polyphagous mealybug Phenacoccus solenopsis Tinsley (Hemiptera: Pseudococcidae) is native to North America (USA), and in the past decade, it has spread to Africa, Europe, Asia, and other parts of the Americas (https://gd.eppo.int/taxon/PHENSO/distribution). This pest targets a wide range of tropical and subtropical host plants, affecting more than 200 species from 60 botanical families (Fand and Suroshe, 2015). It harms vegetables, weeds, field crops, greenhouse plants, and ornamentals, including tomato (Lycopersicon esculentum L.), cotton (Gossypium hirsutum L.), and potato (Solanum tuberosum L.) (Fand and Suroshe, 2015; Chen H. Y. et al., 2021; Chen A. et al., 2021). This mealybug infests all parts of the host plant, including leaves, roots, and branches, but it prefers roots and young leaves because of their high nutrient content (El Aalaoui and Sbaghi, 2024). It excretes large amounts of honeydew, promoting the growth of sooty mold, and injects toxins that can cause stunted growth, wilting, and, in extreme cases, the death of the entire plant (Tong et al., 2019). Additionally, P. solenopsis can transmit various viruses to important crops, including hairy virus, cocoa bud virus, cotton leaf curl virus, and cocoa spotted leaf virus (Saeed et al., 2007; Xi et al., 2019). Phenacoccus solenopsis reproduces at a high rate, both sexually and through ovoviviparity, with each female capable of laying 200 to 600 eggs in a white, waxy sac (Abbas et al., 2010; Fand and Suroshe, 2015). This mealybug can produce 12 to 15 generations each year, contributing to its rapid spread and making it challenging to control (Arif et al., 2012).
Various synthetic pesticides are used to manage P. solenopsis (Fand and Suroshe, 2015). However, the mealybug’s waxy coating and hidden nature often diminish the effectiveness of conventional pesticides, leading to limited short-term control and requiring repeated applications (Nawaz and Freed, 2022). Excessive use of these synthetic chemicals has caused significant resistance issues and has harmed the mealybug’s natural enemies, resulting in its resurgence and causing secondary pest outbreaks (Ramakrishnan et al., 1984; Cloyd and Dickinson, 2006). Given these limitations, there is a need for alternative strategies like biological control, which are more environmentally friendly. Biological control uses insect pathogens, predators, and parasitoids to effectively suppress mealybugs. This approach has proven successful with other mealybug species, such as Planococcus citri (Risso) (Singh, 2004), Maconellicoccus hirsutus (Green) (Kairo et al., 2000), and Phenacoccus manihoti Matile-Ferrero (Herren and Neuenschwander, 1991).
The use of entomopathogenic fungi (EPF) and bacteria (EPB) as potential bioinsecticides, noted for their environmental benefits, has been studied in several countries (Fand and Suroshe, 2015; Gao et al., 2017; Chen H. Y. et al., 2021; Chen A. et al., 2021). Key fungal species employed in biological control include Alternaria sp., Lecanicillium muscarium Zare & Gams, Beauveria bassiana (Balsamo), Paecilomyces farinosus (Holm ex S.F. Gray) Brown & Smith, and Metarhizium sp. (Sharma and Sharma, 2014; Gonthier et al., 2023). These fungi typically infect insects by germinating on the cuticle, penetrating the insect’s body, and eventually leading to death (Vega et al., 2012; Shin et al., 2020). Many strains of EPF have been tested against P. solenopsis, with some showing control efficacy (Nagrare et al., 2011; Fand and Suroshe, 2015). Beauveria bassiana (Bals.), Verticillium lecanii (Zimm.), and Metarhizium anisopliae (Metchnikoff) Sorokin have shown a mortality rate of 45–60% in P. solenopsis under laboratory conditions (Nagrare et al., 2011). These fungi can affect insects in various life stages due to their longevity (Gul et al., 2014).
Among the key bacteria used in biological control are Xenorhabdus spp., Streptomyces spp., Yersinia entomophaga, Chromobacterium spp., Burkholderia spp., and Bacillus spp. (Arasu et al., 2013; Ruiu, 2015; Kim et al., 2022). Streptomyces species, noted for their production of toxic proteins harmful to insects (Ganesan et al., 2018). Among microbial natural products, fungi contribute the most (40%), followed closely by Actinobacteria and single-celled bacteria (Gopalakrishnan et al., 2016). Notably, the genus Streptomyces alone accounts for a significant portion of Actinobacteria-derived products (Bérdy, 2012; Gopalakrishnan et al., 2016). These filamentous soil bacteria are prolific producers of bioactive molecules, including chitinase and protease enzymes that disrupt insects’ peritrophic membranes (Singh et al., 2011). Research highlights their role in managing major agricultural pests such as Spodoptera littoralis (Biosduval) (Lepidoptera: Noctuidae) (Bream et al., 2001), Helicoverpa armigera (Hubner) (Lepidoptera: Noctuidae), and Spodoptera litura (Fabricius) (Lepidoptera: Noctuidae) by targeting chitin in their peritrophic membranes (Binod et al., 2007). Streptomyces extracts have demonstrated significant larvicidal activity, with studies showing complete mortality of Sitophilus oryzae (Linnaeus) (Coleoptera: Curculionidae) larvae at 24 mg mL−1 concentrations (Rishikesh et al., 2013). Similarly, Streptomyces sp. AP-123 polyketide metabolite exhibited larvicidal efficacy against H. armigera and S. litura, with mortality rates of 68.41 and 60.02%, respectively, at 1,000 ppm (Arasu et al., 2013). Despite these successes, research on controlling P. solenopsis using Streptomyces species remains scarce. Environmental conditions and pest-crop specificity influence the efficacy of bacterial-derived biomolecules, which can be integrated into IPM strategies either alone, in rotation, or combined with other approaches like beneficial insects and resistant cultivars (Mazzeo et al., 2019). In addition, bio-formulations with the insect-pathogenic bacterium Photorhabdus luminescens Thomas and Poinar have proven effective in controlling P. solenopsis under laboratory conditions (Fand and Suroshe, 2015). EPB primarily infect insects through ingestion, and some produce chitinases, enzymes effective against various agricultural pests (Binod et al., 2007; Salunkhe et al., 2013; Okongo et al., 2019). The effectiveness of biological control depends on various factors such as temperature, and humidity, which affect entomopathogenic microorganisms (Sabbahi et al., 2022). EPF and EPB are often combined to create biopesticides against various insects, with studies showing both synergistic and antagonistic effects (Iqbal et al., 2019; Ebani and Mancianti, 2021; Quesada-Moraga et al., 2022). In this study, a mixture of the entomopathogenic fungi (Alternaria murispora and Alternaria destruens), along with bacteria (Streptomyces bellus-E23-2), each singly and in combination, was applied to control P. solenopsis (adult female) under laboratory and greenhouse conditions.
2 Materials and methods
2.1 Rearing of P. solenopsis
The initial culture of P. solenopsis was established on sprouted potatoes (Solanum tuberosum L.). After washing the potatoes with distilled water and drying them in the shade for 30 min, uniform-sized potatoes were placed in plastic containers (40 cm × 30 cm × 30 cm) containing soil treated with carbendazim at 5 g/kg. Water was added every other day to maintain moisture for sprout growth. After 35 days, the potatoes had 6–8 buds with sprouts measuring 6–10 cm, ideal for P. solenopsis inoculation. Mature female mealybugs were collected from an infested purslane (Portulaca oleracea L.) field in Zemamra, Morocco (32°37′48″ N, 8°42′0″ W, Elevation 165 m). The mealybugs were then transferred to the entomology lab at the National Institute of Agricultural Research (INRA), Zemamra, Morocco, and introduced to the sprouted potatoes using a camel hair brush. The inoculated containers were placed in separate entomological cages (80 cm × 80 cm × 80 cm) with mesh covers for ventilation and cross-contamination prevention. The culture was maintained at 26 ± 2°C, 60 ± 10% relative humidity, with a photoperiod of 8:16 h (L:D). To control age and increase the mealybug population, 24-h-old first-instar nymphs were transferred to similar containers. To sustain the colony, infested and uninfested sprouted potatoes were added weekly. The mealybugs settled, laid eggs, and the first-instar nymphs, or crawlers, emerged. When the F2 generation matured, adult female mealybugs were removed for bioassays.
2.2 Entomopathogenic fungi (EPF)
Two entomopathogenic fungi, Alternaria murispora (NCBI GenBank Acc. No: PP264308) and Alternaria destruens (NCBI GenBank Acc. No: PP264311), were isolated from sterilized cadavers of Dactylopius opuntiae (Cockerell) (Hemiptera: Dactylopiidae) (Moroccan biotype). Identification of these fungi was based on spore and colony morphology, with additional confirmation via ITS region sequencing. For cultivation, the fungi were grown on Sabouraud’s dextrose agar supplemented with yeast extra, following the method outlined by Goettel and Inglis (1997).
2.3 Entomopathogenic bacteria
The Streptomyces strain used in this study was Streptomyces bellus E23-2 (NCBI GenBank Acc. No: OM883988). It was isolated from soil samples collected in northwest Morocco during February and early March 2019 (Rammali et al., 2022). This strain was grown on ISP2 medium using the streak method and was stored at 4°C for short-term storage and in 20% glycerol at −20°C for long-term storage (Marimuthu et al., 2020). It exhibited antimicrobial and antioxidant activities (Rammali et al., 2022) and was also effective in laboratory and greenhouse tests for controlling D. opuntiae (Rammali et al., 2023).
2.4 Laboratory trials
Bio-efficacy trials were conducted to evaluate different treatments of entomopathogenic fungi (EPFs) and bacteria (EPBs), both alone and in combination, against adult females of P. solenopsis (Table 1). The experiments were conducted in plastic containers (15 cm × 10 cm × 5 cm), each containing a potato with 6–8 buds and sprouts measuring 6–10 cm, hosting 50 adult female mealybugs (20 days old, weighing 5.5 ± 0.5 mg, and measuring 2.4–3.5 mm). The trials were carried out under controlled conditions at 26 ± 2°C, 60 ± 10% relative humidity, and a photoperiod of 8:16 h (L:D). For the EPFs, conidial suspensions were prepared from two-week-old Potato Dextrose Agar (PDA) cultures. Conidia were harvested, suspended in sterile distilled water, and then filtered through four layers of cotton cloth. The concentration of conidia (measured in conidia per mL) was determined using a hemocytometer (HGB, Germany). The Streptomyces strain, bacterial concentrations was obtained following culturing in nutrient broth, incubating at 28°C and 150 rpm for 24 h in an orbital shaker (Kuhner Shaker Ltd., Switzerland), and subsequent centrifugation at 10,000 rpm. The bacterial cell concentration was measured at 640 nm using a spectrophotometer (Optizen 3220UV/VIS, Mecasys, South Korea) (Rammali et al., 2023). Control groups were sprayed with distilled water, while a positive control group received imipower (imidacloprid 35% SC, Nanjing Red Sun Co. Ltd.—China) applied at 0.75 cm3 L−1. Imidacloprid at a concentration of 0.75 cm3 L−1 and Chlorpyrifos were found to be the most toxic insecticides against P. solenopsis under field conditions (El-Mageed et al., 2018). A hand sprayer was used to apply 2 mL of each treatment solution as a mist over the adult female mealybugs and the potato sprouts. The insect Mortality and LC50 were recorded at 3, 6, and 14 days post-treatment. The study included 10 replicates for each treatment, with all experiments repeated twice to ensure reproducibility.
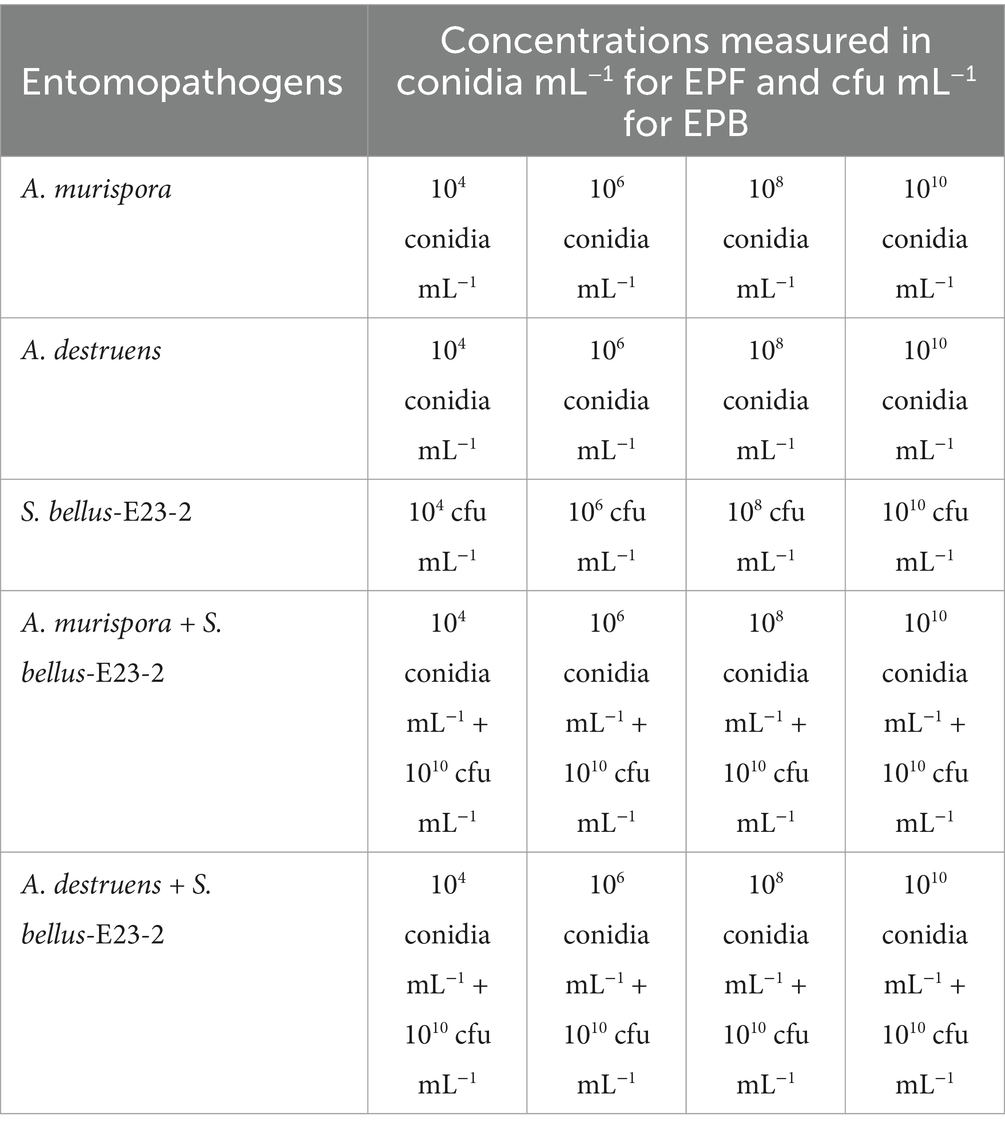
Table 1. List of tested entomopathogens fungi (EPF) and bacteria (EPB) treatments applied against adult female of P. solenopsis under laboratory conditions.
2.5 Greenhouse trials
The study was conducted in two greenhouses (11 m in length, 7 m in width, 3 m in height), located at the experimental station of the National Institute of Agricultural Research (INRA), Zemamra (32°37′48″ N, 8°42′0″ W). The environmental conditions were maintained at a temperature of 28 ± 2°C, 65% relative humidity, and under natural light conditions. The six treatments with the highest mortality rates in the laboratory were selected for the study, including A. murispora at 1010 conidia mL−1, A. destruens at 1010 conidia mL−1, S. bellus-E23-2 at 1010 cfu mL−1, A. murispora + S. bellus-E23-2 (1010 conidia mL−1 + 1010 cfu mL−1), A. murispora + S. bellus-E23-2 (108 conidia mL−1 + 1010 cfu mL−1), and A. destruens + S. bellus-E23-2 (1010 conidia mL−1 + 1010 cfu mL−1). For the experiments, three-month-old potato plants (Solanum tuberosum L.) were grown in plastic pots (33 cm diameter, 12 cm height) filled with a mixture of fine sand (2/3) and peat (1/3). These plants were artificially infested with P. solenopsis by introducing five gravid females onto each potted plant. The plants were irrigated as necessary. Twenty days after infestation, each EP treatment solution (50 mL) was applied as a mist over the infested plants using a laboratory sprayer to ensure complete coverage. Control plants were sprayed only with distilled water, while imidacloprid at a concentration of 0.75 cm3 L−1 was used as a positive control. Before treatment application, three potato leaves were randomly selected and destructively sampled per replicate per treatment to determine the starting density of the mealybug in all experiments. Ten replicates, each with ten plants, were set up for each treatment in a completely randomized experimental block design. The experiment was repeated twice in two separate greenhouses. The greenhouses were divided into chambers, and treatments were randomly assigned to chambers to minimize movements of EPs among treatments. Monitoring of the treatments began 1 week after treatment and continued on a weekly basis for 5 consecutive weeks. During each sampling date, three potato leaves were randomly selected and destructively sampled per replicate per treatment. Eggs and active stages of P. solenopsis (nymphs, cocoons, and adults), as well as the number of mummies (i.e., P. solenopsis killed by entomopathogens), were counted in the laboratory under a dissecting binocular loupe (Motic). Additionally, four adult females per leaf were selected to confirm mortality based on the presence of fungal mycelia (Cuthbertson et al., 2005) or entomopathogenic bacteria (Rammali et al., 2023). Furthermore, treated plants were monitored weekly for 5 weeks post-treatment, and a numerical scale ranging from 0 to 10 was used to describe visual quality, as reported by Gettys et al. (2021). This scale assessed plant responses in terms of quality, ranging from 0 (dead) to 10 (excellent quality). This parameter has been previously utilized in assessing various experimental factors including herbicides, salt stress, and other conditions (Gettys and Haller, 2012; Smith et al., 2014; Tootoonchi et al., 2020).
2.6 Statistical analysis
The study was conducted over 2 years (2023–2024) in both laboratory and greenhouse settings. In laboratory conditions, data on mortality rates were compared across treatments and controls using ANOVA on arcsine-transformed means. Tukey’s LSD test was used for pairwise comparisons if ANOVA showed significance at p < 0.05. LC50 values for different entomopathogen treatments were determined via Probit analysis using IBM SPSS 23.0 (SPSS Inc., Chicago, Illinois, United States). The impact of each treatment on P. solenopsis densities, mummy counts, and treated plants’ visual quality in greenhouse conditions was assessed with one-way ANOVA. Tukey’s LSD test was applied for separating treatment means in significant ANOVA cases. Mealybug counts were transformed using log10(x + 1) to address variance homogeneity. Visual quality data were arcsine-transformed to ensure a normalized distribution. Mean visual quality values for treated plants were compared with untreated controls (distilled water) using one-way ANOVA at p = 0.05, with Tukey’s LSD test for post-hoc comparisons. All analyses were performed using IBM SPSS Statistics version 23.0, maintaining a significance level of 0.05.
3 Results
3.1 Laboratory trials
The study assesses the efficacy of different entomopathogenic fungi and bacteria on P. solenopsis adult female mortality (Figure 1, Table 2). Alternaria murispora exhibited increasing mortality rates with both higher concentrations and longer exposure times, achieving 79.7% mortality at 1010 conidia mL−1 after 14 days. Alternaria destruens showed a similar pattern but was less effective, with 57.4% mortality at the same concentration and duration. S. bellus-E23-2 demonstrated increasing mortality with higher concentrations and time, reaching 58.8% at 1010 cfu mL−1 after 14 days. Combination treatments were more effective, with A. murispora + S. bellus-E23-2 achieving 85.3% mortality at 1010 conidia mL−1 + 1010 cfu mL−1 after 14 days, and A. destruens + S. bellus-E23-2 reaching 64.1% mortality under the same conditions. The positive control, Imidacloprid, showed high efficacy with 76.9% mortality after 14 days. The control group exhibited negligible mortality. All treatments were statistically significant (p < 0.0001), indicating dose-dependent effects.
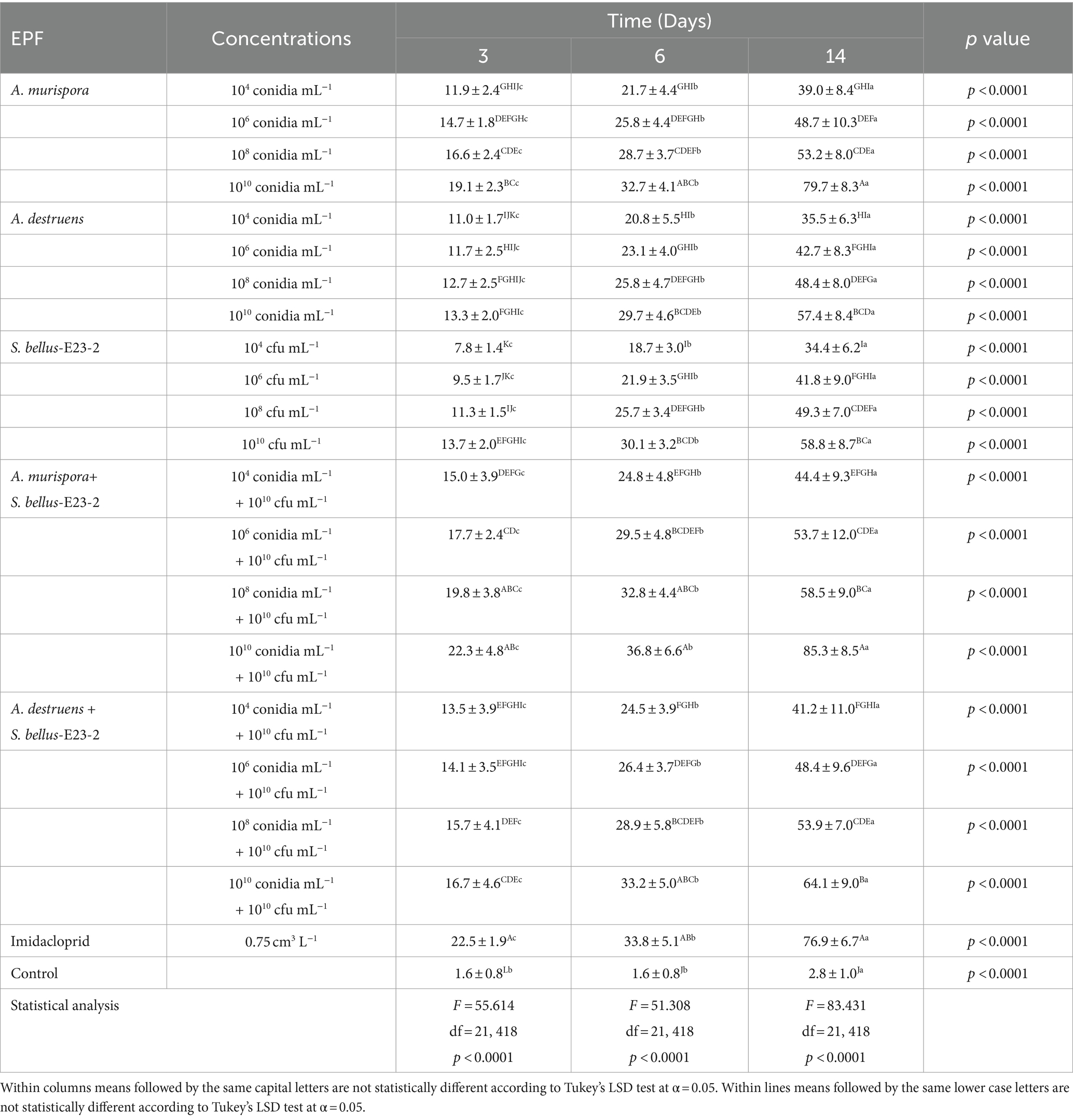
Table 2. Effects of different entomopathogenic fungi and bacteria, both alone and in combination on the percentage mortality of P. solenopsis, adult females.
Further analysis in Table 3 revealed the LC50 values for A. murispora and A. destruens, with A. murispora showing LC50 values decreasing from 3.596 × 1027 conidia mL−1 at 3 days to 1.446 × 106 conidia mL−1 at 14 days. Alternaria destruens had LC50 values from 4.303 × 1066 conidia mL−1 at 3 days to 1.338 × 108 conidia mL−1 at 14 days, indicating higher concentrations are required for efficacy. Table 4 reported the LC50 for S. bellus-E23-2, showing values of 2.462 × 1030 cfu mL−1 at 3 days, 3.646 × 1018 cfu mL−1 at 6 days, and 9.564 × 107 cfu mL−1 at 14 days. These findings illustrate the progressive effectiveness of the pathogens over time and highlight the superior efficacy of combination treatments, particularly involving A. murispora.
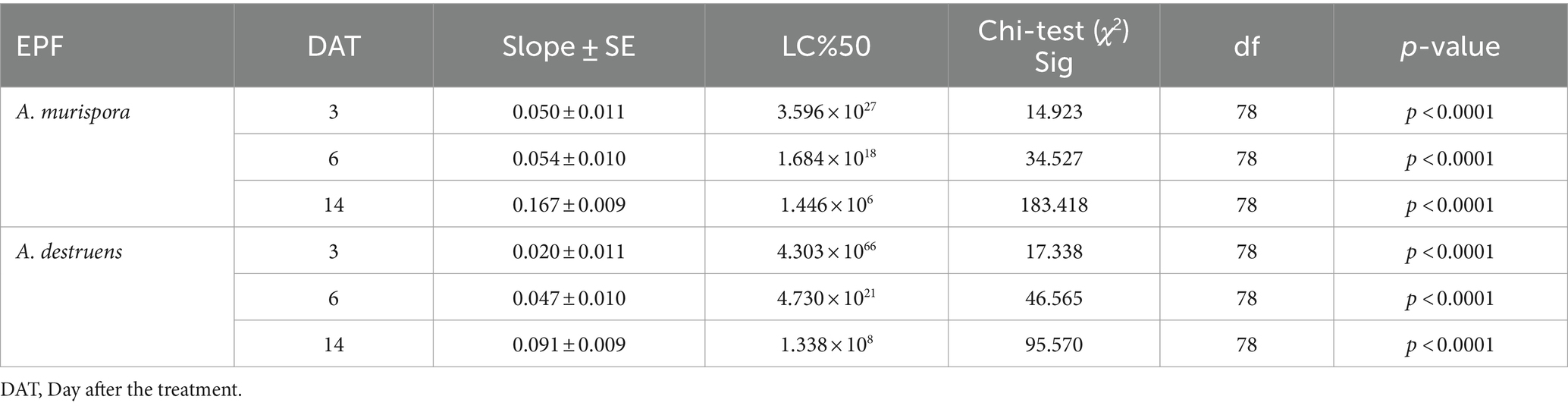
Table 3. Median lethal concentration LC50 (conidia mL−1) of P. solenopsis treated by A. murispora, and A. destruens (ANOVA, α = 0.05).

Table 4. Median lethal concentration LC50 (cfu mL−1) of P. solenopsis treated by Streptomyces bellus E23-2 strain. (ANOVA, α = 0.05).
3.2 Greenhouse trials
3.2.1 Effect of single release of A. murispora on the population density of P. solenopsis
After treatment with A. murispora at 1010 conidia mL−1, P. solenopsis densities increased from 23.3 insects per potato leaf before treatment (D0) to a peak of 297.9 in the fourth week (D4), then declined to 206.5 in the fifth week (D5) (Figure 2A). In contrast, the control (distilled water) showed an increase from 24.7 insects per leaf to a peak of 697.3 in the third week (D3), then declined to 397.3 in the fifth week. The imidacloprid treatment had much lower densities, peaking at 137.0 in the fourth week. The number of infected mealybugs (mummies) in the A. murispora treatment increased from 0.0 at D0 to a peak of 136.2 at D3, then declined to 19.7 at D5 (Figure 2B).
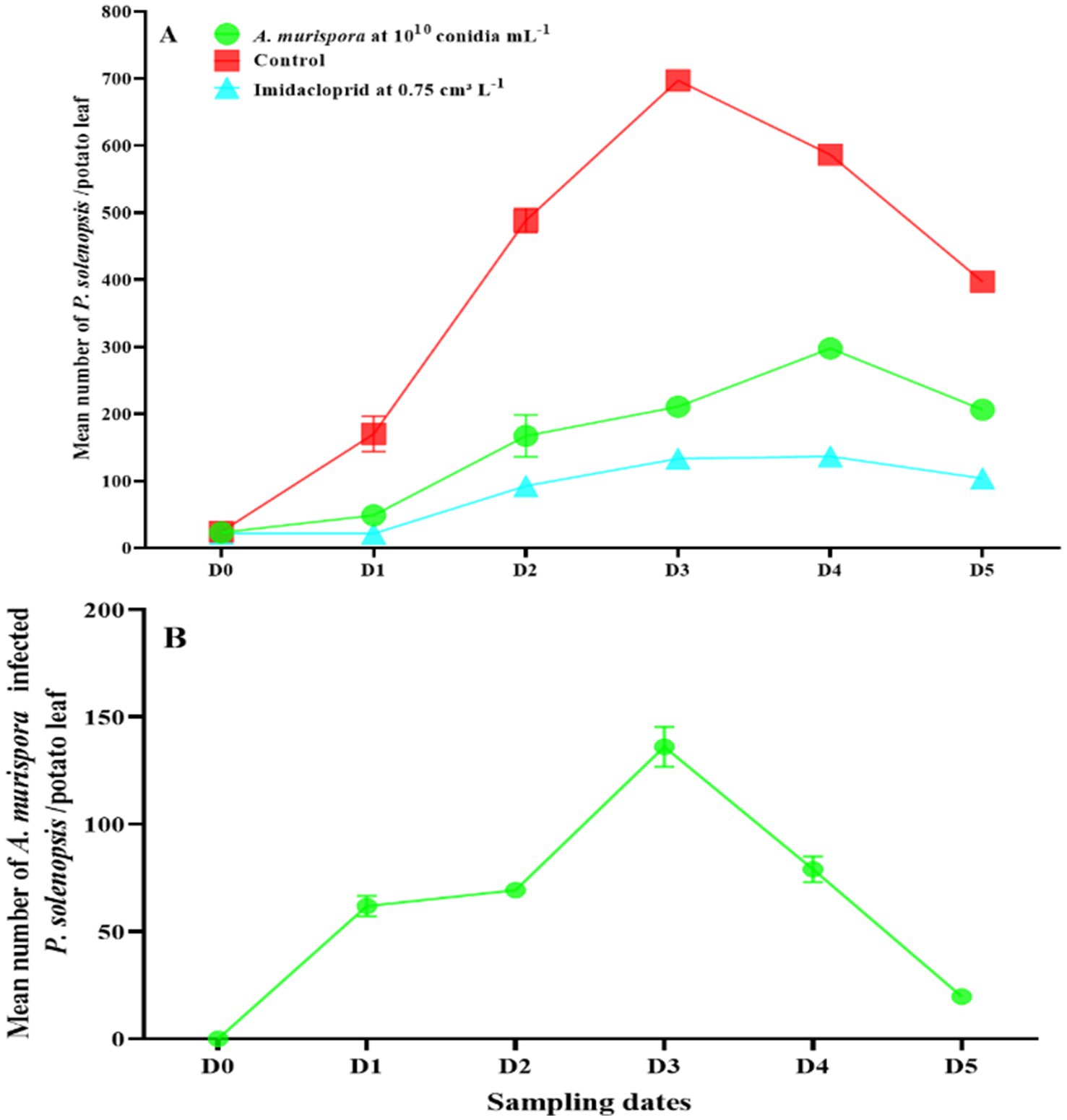
Figure 2. (A) Changes in P. solenopsis population (mean ± SE) in the control, imidacloprid, and A. murispora treatments. (B) Changes in A. murispora population (mean ± SE) in the A. murispora treatments. D0 date before A. murispora release. D1, D2, D3, D4, and D5 subsequent sampling days at one week-intervals.
3.2.2 Effect of a single release of A. destruens on the population density of P. solenopsis
After treatment with A. destruens at 1010 conidia mL−1, P. solenopsis densities increased from 22.3 insects per potato leaf before treatment (D0) to a peak of 307.8 in the fourth week, then declined to 223.6 in the fifth week (Figure 3A). The number of infected mealybugs (mummies) in the A. destruens treatment increased from 0.0 at D0 to a peak of 107.9 at D3, then declined to 12.6 at D5 (Figure 3B).
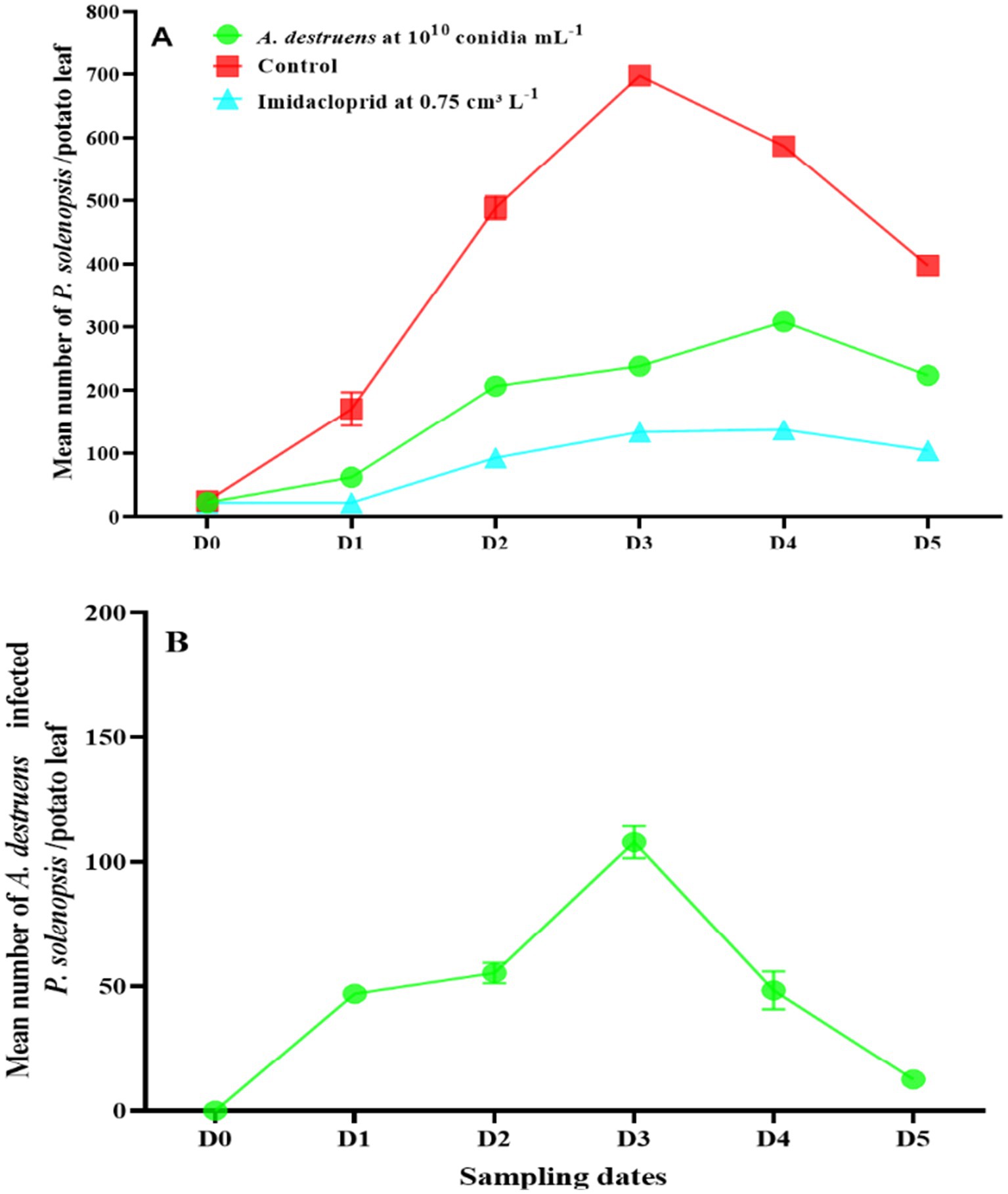
Figure 3. (A) Changes in P. solenopsis population (mean ± SE) in the control, imidacloprid, and A. destruens treatments. (B) Changes in A. destruens population (mean ± SE) in the A. destruens treatments. D0 date before A. destruens release. D1, D2, D3, D4, and D5 subsequent sampling days at one week-intervals.
3.2.3 Effect of a single release of S. bellus-E23-2 on the population density of P. solenopsis
After treatment with S. bellus-E23-2 at 1010 cfu mL−1, P. solenopsis densities increased from 22.9 insects per potato leaf before treatment (D0) to a peak of 302.2 in the fourth week (D4), then declined to 213.0 in the fifth week (D5) (Figure 4A). The number of infected mealybugs (mummies) in the S. bellus-E23-2 treatment increased from 0.0 at D0 to a peak of 122.0 at D3, then declined to 16.0 at D5 (Figure 4B).
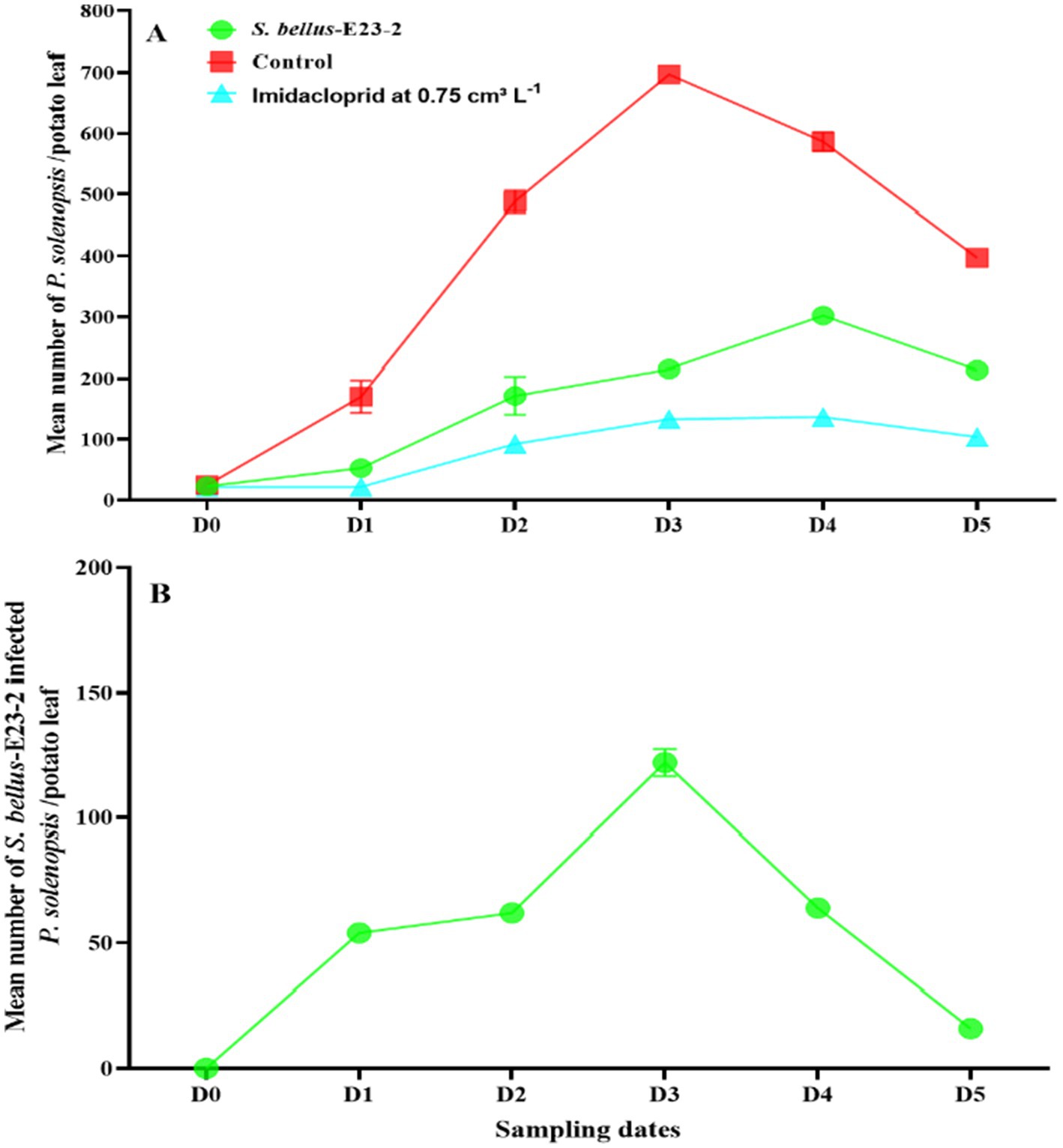
Figure 4. (A) Changes in P. solenopsis population (mean ± SE) in the control, imidacloprid, and S. bellus-E23-2 treatments. (B) Changes in S. bellus-E23-2 population (mean ± SE) in the S. bellus-E23-2 treatments. D0 date before S. bellus-E23-2 release. D1, D2, D3, D4, and D5 subsequent sampling days at one week-intervals.
3.2.4 Effect of simultaneous release of A. murispora and S. bellus-E23-2 on the population density of P. solenopsis
After simultaneous treatment with A. murispora (108 conidia mL−1) and S. bellus-E23-2 (1010 cfu mL−1), P. solenopsis densities increased from 22.8 insects per potato leaf before treatment (D0) to a peak of 177.6 in the fourth week (D4), then declined to 81.0 in the fifth week (D5) (Figure 5A). For the higher concentration treatment (1010 conidia mL−1 + 1010 cfu mL−1), densities increased from 21.9 before treatment to a peak of 156.6 in the fourth week, then declined to 68.2 in the fifth week (Figure 6A). The number of infected mealybugs (mummies) in the lower concentration treatment increased from 0.0 at D0 to a peak of 146.8 at D3, then declined to 24.5 at D5 (Figure 5B). In the higher concentration treatment, mummies increased from 0.0 at D0 to a peak of 151.4 at D3, then declined to 29.6 at D5 (Figure 6B).
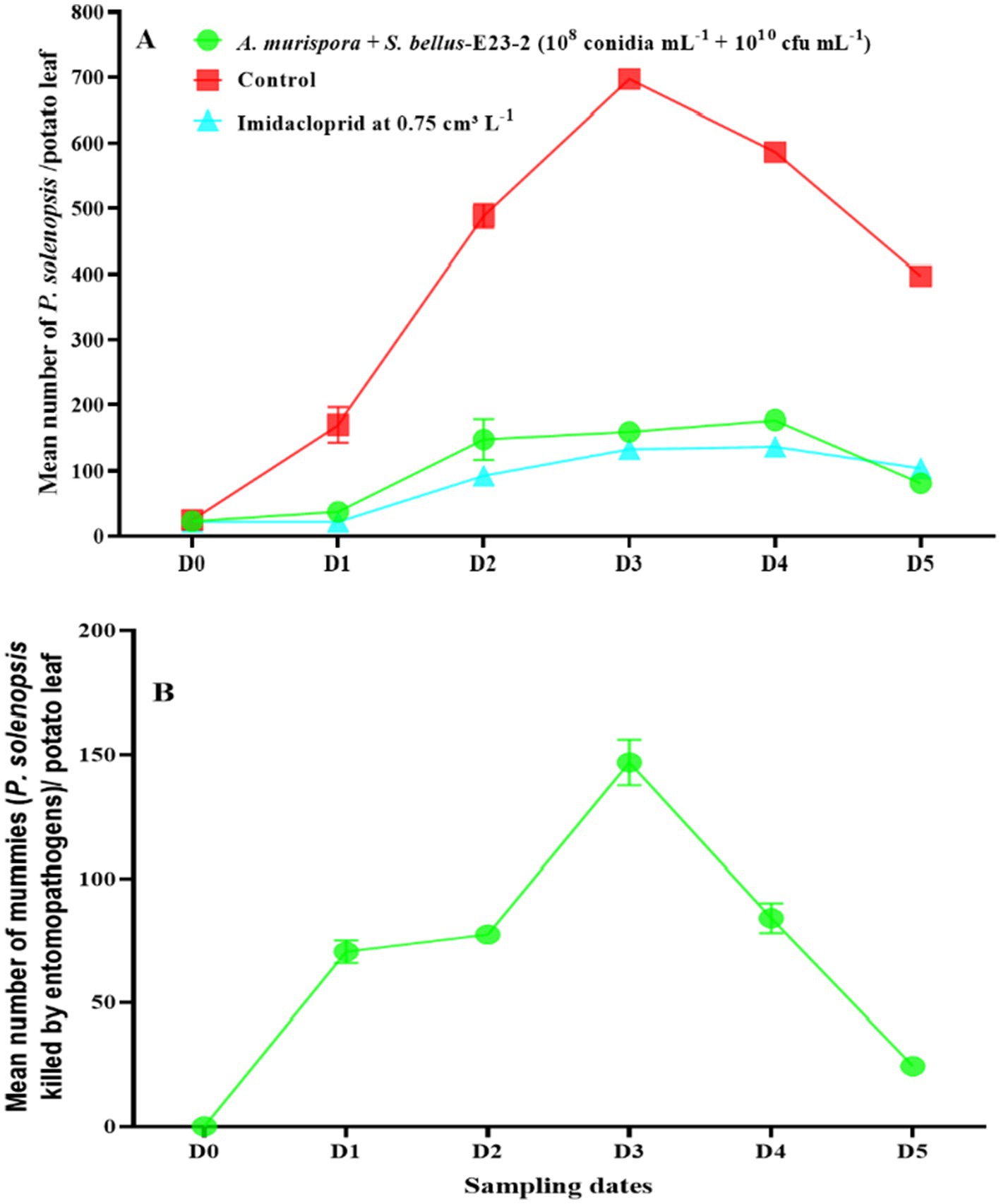
Figure 5. (A) Changes in P. solenopsis population (mean ± SE) in the control, imidacloprid, and combined A. murispora + S. bellus-E23-2 (108 conidia mL−1 + 1010 cfu mL−1) treatments. (B) Changes in A. murispora and S. bellus-E23-2 populations (mean ± SE) in the combined A. murispora + S. bellus-E23-2 (108 conidia mL−1 + 1010 cfu mL−1) treatments. D0 date before A. murispora + S. bellus-E23-2 release. D1, D2, D3, D4, and D5 subsequent sampling days at one week-intervals.
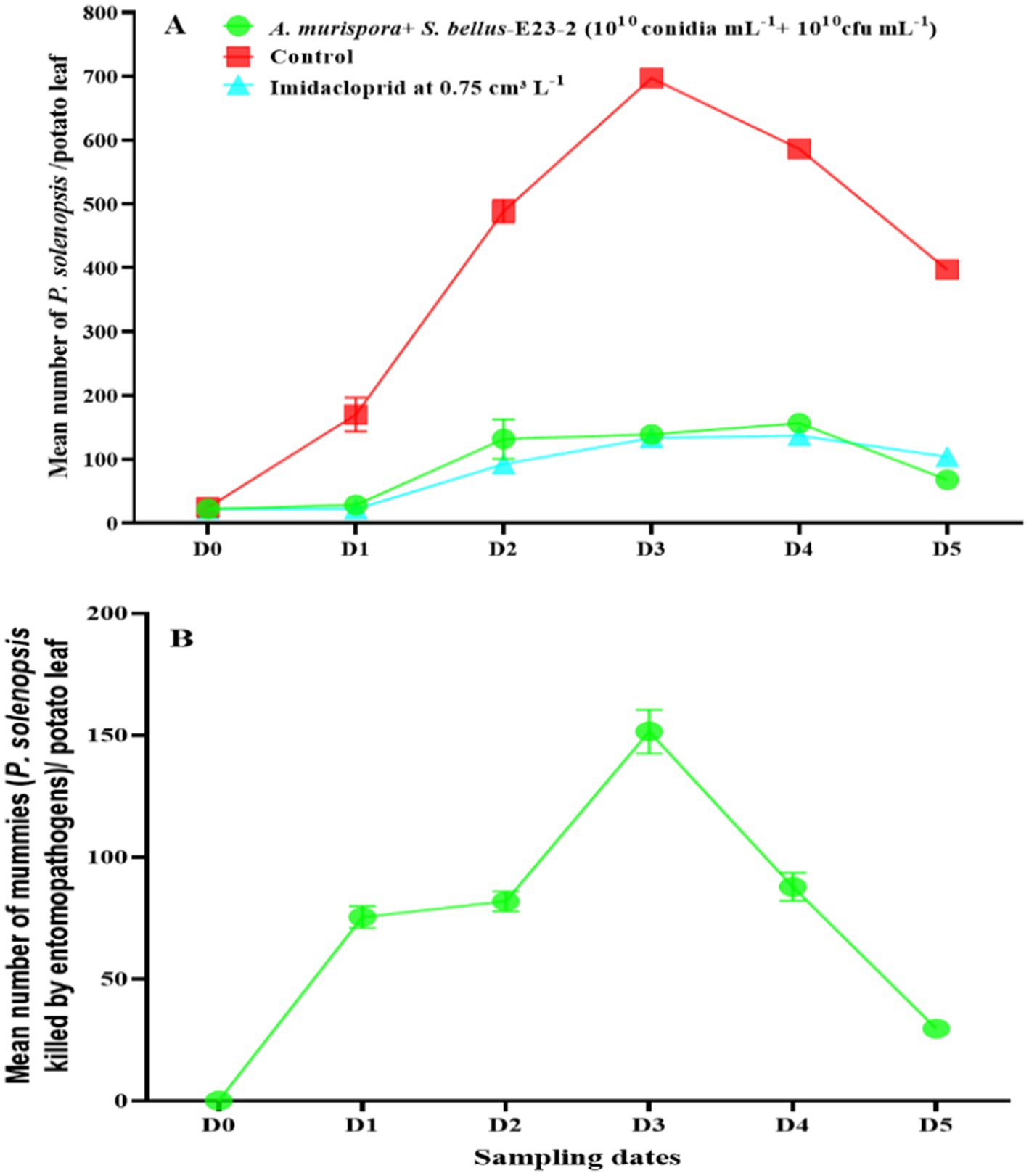
Figure 6. (A) Changes in P. solenopsis population (mean ± SE) in the control, imidacloprid, and combined A. murispora + S. bellus-E23-2 (1010 conidia mL−1 + 1010 cfu mL−1) treatments. (B) Changes in A. murispora and S. bellus-E23-2 populations (mean ± SE) in the combined A. murispora + S. bellus-E23-2 (1010 conidia mL−1 + 1010 cfu mL−1) treatments. D0 date before A. murispora + S. bellus-E23-2 release. D1, D2, D3, D4, and D5 subsequent sampling days at one week-intervals.
3.2.5 Effect of simultaneous release of A. destruens and S. bellus-E23-2 on the population density of P. solenopsis
After simultaneous treatment with A. destruens (1010 conidia mL−1) and S. bellus-E23-2 (1,010 cfu mL−1), P. solenopsis densities increased from 22.1 insects per potato leaf before treatment (D0) to a peak of 159.8 in the fourth week (D4), then declined to 71.3 in the fifth week (D5) (Figure 7A). The number of infected mealybugs (mummies) in the A. destruens and S. bellus-E23-2 treatment increased from 0.0 at D0 to a peak of 148.0 at D3, then declined to 23.0 at D5 (Figure 7B).
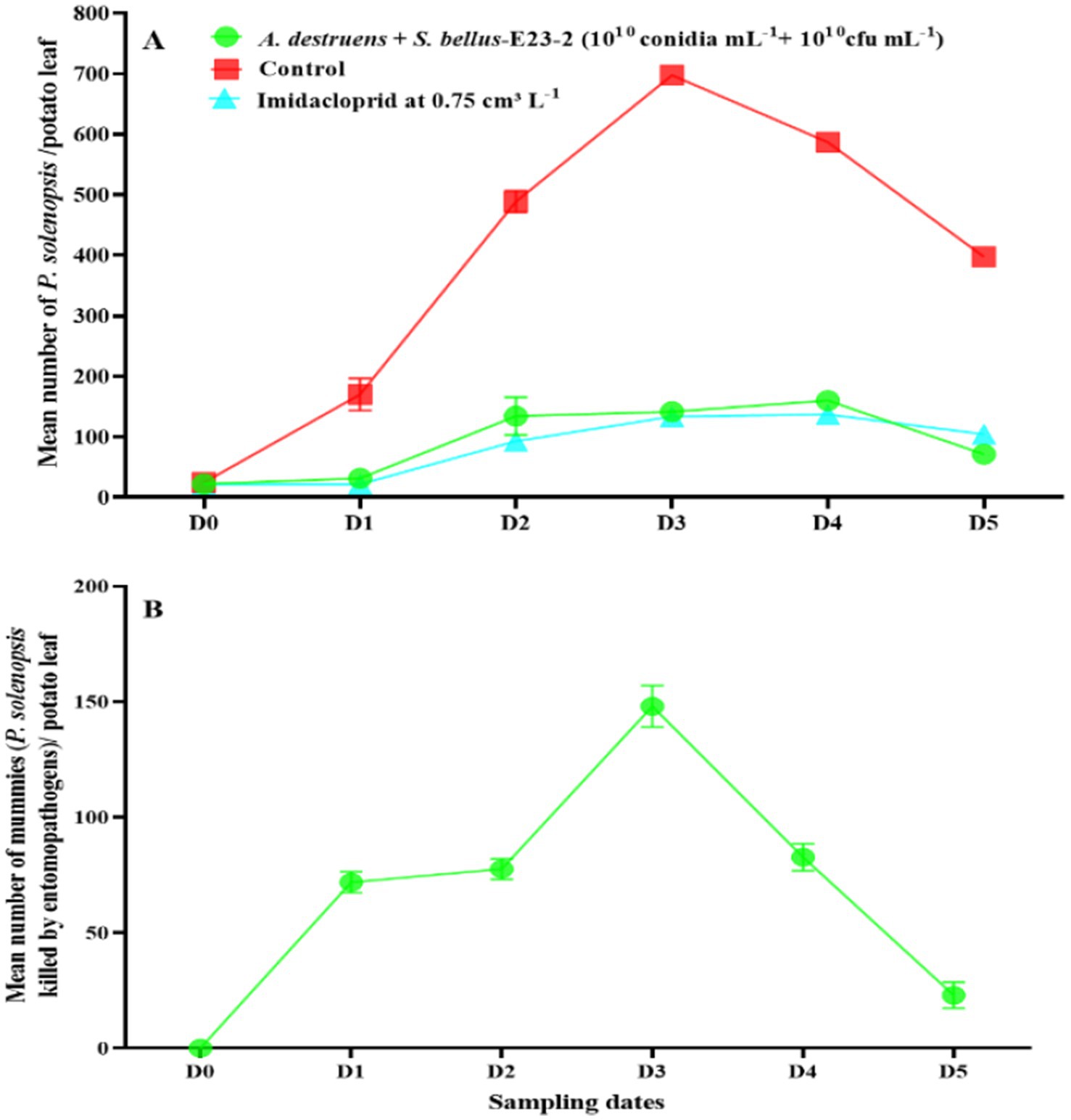
Figure 7. (A) Changes in P. solenopsis population (mean ± SE) in the control, imidacloprid, and combined A. destruens + S. bellus-E23-2 (1010 conidia mL−1 + 1010 cfu mL−1) treatments. (B) Changes in A. destruens and S. bellus-E23-2 populations (mean ± SE) in the combined A. destruens + S. bellus-E23-2 (1010 conidia mL−1 + 1010 cfu mL−1) treatments. D0 date before A. destruens + S. bellus-E23-2 release. D1, D2, D3, D4, and D5 subsequent sampling days at one week-intervals.
3.2.6 Comparison of P. solenopsis density and the number of mummies among the treatments
The control group’s density peaked at 697.3 (D3) and ended at 397.3 (D5). Imidacloprid maintained the lowest density, peaking at 137.0 5 (D4). Alternaria murispora at 1010 conidia mL−1 peaked at 297.9 (D4) and decreased to 206.5 (D5). Alternaria destruens at 1010 conidia mL−1 peaked at 307.8 (D4) and ended at 223.6 (D5). Streptomyces bellus-E23-2 at 1010 cfu mL−1 showed a peak of 302.2 0 (D4). The combinations of A. murispora + S. bellus-E23-2 (1010 conidia mL−1 + 1010 cfu mL−1), A. murispora + S. bellus-E23-2 (108 conidia mL−1 + 1010 cfu mL−1), and A. destruens + S. bellus-E23-2 (1010 conidia mL−1 + 1010 cfu mL−1) were most effective, reducing density to 68.2, 81.0, and 71.3, respectively, by the fifth week (D5) (first week F7,232 = 635.097; p < 0.0001; second week F7,232 = 698.530; p < 0.0001; third week F7,232 = 9285.563; p < 0.0001; fourth week F7,232 = 5579.626; p < 0.0001; fifth week F7,232 = 725.434; p < 0.0001). The highest number of mummies was seen with A. murispora + S. bellus-E23-2 (1010 conidia mL−1 + 1010 cfu mL−1) treatment, peaking at 151.4 (D3) (first week F5,174 = 216.693; p < 0.0001; second week F5,174 = 213.336; p < 0.0001; third week F5,174 = 131.542; p < 0.0001; fourth week F5,174 = 197.696; p < 0.0001; fifth week F5,174 = 44.518; p < 0.0001). All the tested treatments did not reduce the visual quality of the treated potato plants by 50%, and no significant difference was recorded among the control, and A. murispora at 1010 conidia mL−1, and S. bellus-E23-2 at 1010 cfu mL−1 treatments (F7,152 = 44.224; p < 0.0001), indicating the effectiveness of these treatments (Figure 8). The most promising combinations in terms of treated plants’ visual quality, in descending order, were A. murispora + S. bellus-E23-2 (108 conidia mL−1 + 1010 cfu mL−1), A. murispora + S. bellus-E23-2 (1010 conidia mL−1 + 1010 cfu mL−1), and A. destruens + S. bellus-E23-2 (1010 conidia mL−1 + 1010 cfu mL−1). Imidacloprid at 0.75 cm3 L−1 significantly affected the visual quality of the treated plants compared to the control and other treatments tested, which calls into question its validity as an effective method to combat this insect pest.
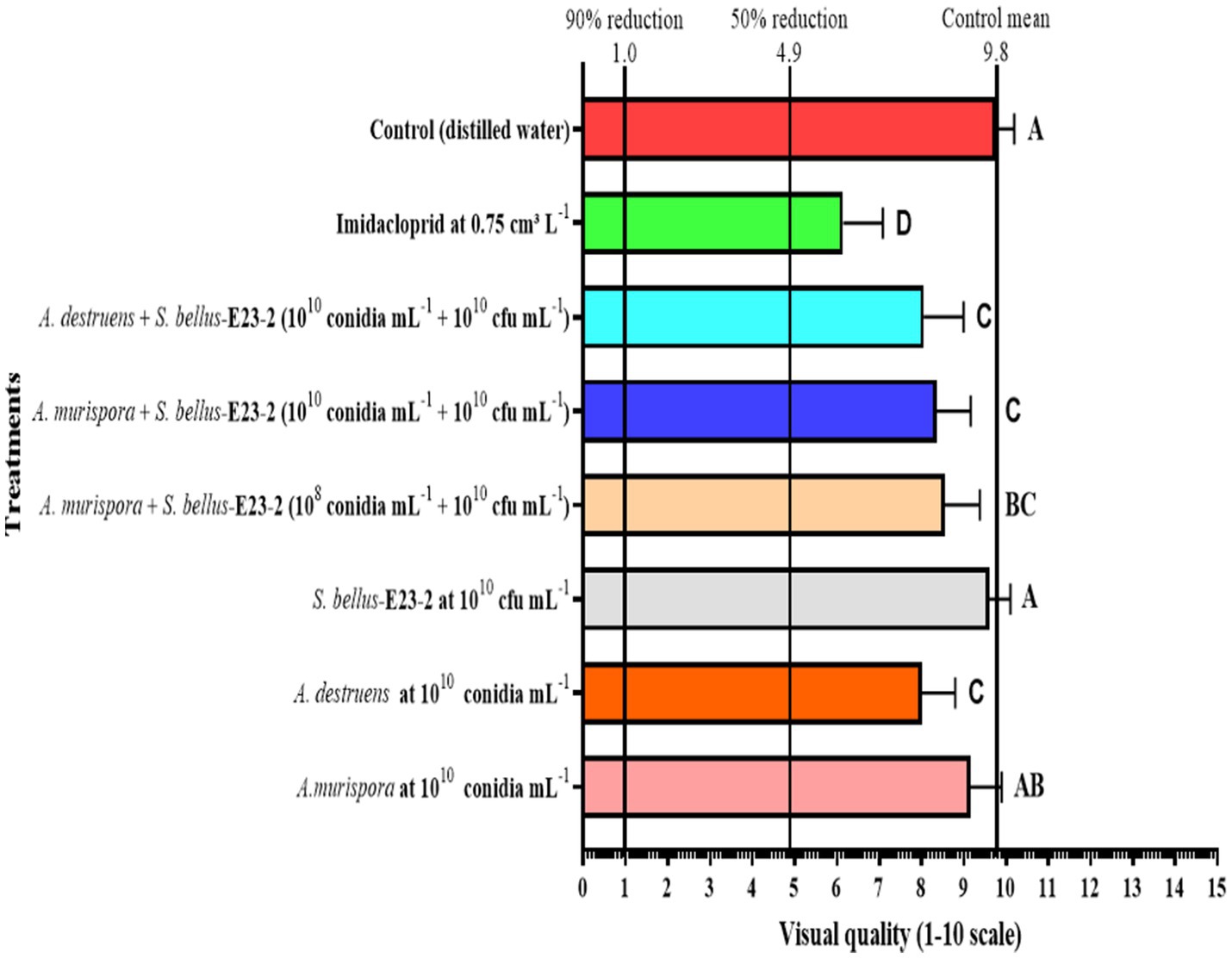
Figure 8. Visual quality of Solanum tuberosum L. plants 5 weeks after treatment. A numerical scale of 0 through 10 is used to describe the treated-plants visual quality, where 0 represents dead, and 10 indicates excellent quality. Bars represent the mean of 20 replicates. Treatments labeled with the same letter are not statistically different at p = 0.05. The right bold vertical rule indicates the mean of control plants (distilled water), whereas the central and left bold vertical rules indicate 50 and 90% reductions compared with control plants.
4 Discussion
In the present study, the efficacy of different entomopathogenic fungi and bacteria in controlling P. solenopsis was demonstrated through both laboratory and greenhouse trials. Laboratory results indicated that while single treatments with A. murispora, A. destruens, and S. bellus-E23-2 were effective in increasing mortality rates of P. solenopsis, combination treatments significantly enhanced this efficacy. The combination of A. murispora and S. bellus-E23-2 was particularly notable, achieving the highest mortality rates, which suggests a synergistic effect between the fungus and the bacterium. The greenhouse trials further validated these findings, showing that the combined treatments consistently reduced the population density of P. solenopsis more effectively than single treatments or the control. The combination treatments not only reduced pest densities but also resulted in a higher number of mummified insects, indicating successful pathogen infection and subsequent pest mortality. It is important to note that A. murispora and A. destruens are not exclusively entomopathogenic since they occupy other ecological niches in nature; A. murispora is also known as a foliar endophyte in olive trees (Nicoletti et al., 2020), and A. destruens is a parasite in Cuscuta spp.; additionally, A. destruens (strain 059) is commercially available as a herbicide in the USA and some European countries.1 These alternative roles highlight the ecological versatility of these fungi beyond their use in insect control. Regarding potential risks associated with their use as biopesticides, it is pertinent to consider the production of secondary metabolites such as mycotoxins by A. murispora and A. destruens. Current literature underscores the presence of Alternaria mycotoxins in processed plant foods and agricultural products, albeit at low concentrations, without specific legislative regulations (Chen H. Y. et al., 2021; Chen A. et al., 2021). This aspect necessitates further investigation into the metabolic profiles of A. murispora and A. destruens to evaluate any associated risks in agricultural applications. Understanding these potential risks is crucial for ensuring the safe and effective deployment of these fungi in integrated pest management strategies. Interestingly, while Imidacloprid showed high efficacy in reducing pest density, it also negatively impacted the visual quality of the treated potato plants, highlighting a significant drawback of this chemical treatment. In contrast, the biological treatments did not adversely affect plant quality, reinforcing their potential as safer and more sustainable pest management options. Our study’s findings align with and expand upon previous research exploring the use of entomopathogenic fungi and bacteria for pest control. Ujjan et al. (2015) found virulence of Metarhizium anisopliae (Metschn.) in a screen house test on cotton plants against mealybugs. Mohammadbeige and Port (2013) observed 100% mortality in long-horned grasshopper Uvarovistia zebra (Uvarov) (Orthoptera: Tettigoniidae) nymphs with B. bassiana and M. anisopliae treatments. Similarly, Herker et al. (2010) found that M. anisopliae and Paecilomyces fumosoroseus (Wise) Brown & Smith produced the highest mycosis rate and mortality against Cydia pomonella (Linnaeus), and Cydia funebrana (Treitschke) (Lepidoptera: Tortricidae) under laboratory conditions. In field applications, fungal biocontrol agents gradually minimized the density of P. solenopsis population, with M. anisopliae and B. bassiana showing particular effectiveness (Daniel and Wyss, 2010; Sahayaraj and Namachivayam, 2011).
Furthermore, screening of entomopathogenic fungi against various pests, such as Ceratothripoides claratris (Shumsher) (Thysanoptera: Thripdae), Pseudococcus cryptus Hempel (Homoptera: Pseudococcidae), and Bemisia tabaci (Gennadius) (Hemiptera: Aleyrodidae), demonstrated the efficiency of M. anisopliae (Panyasiri et al., 2007). Isaria farinosa (Holmsk.) Fries (Sordariomycetes: Hypocreales) exhibited high mortality rates against Pissodes punctatus Langor and Zhang (Coleoptera: Curculionidae) with significant mortality observed at 1 × 108 conidia mL−1 (Yang et al., 2009). Moreover, Verticillium lecanii (Zimm.) and B. bassiana foliar sprays effectively minimized mealybug populations (Tanwar et al., 2007), while B. bassiana decreased the invasion of Paracoccus marginatus Williams and Granara de Willink (Hemiptera: Pseudococcidae) under field conditions (Suresh et al., 2010). Additionally, de Souza et al. (2009) confirmed the effectiveness of B. bassiana and M. anisopliae in controlling Diaspis echinocacti (Bouché) (Hemiptera: Diaspididae). In a broader context, Alternaria spp., particularly A. alternata, have shown promising entomopathogenic properties against various pests, including thrips, Zyginidia pullula (Boheman)(Hemiptera: Cicadellidae), Oulema gallaeciana (Heyden) (Coleoptera: Chrysomelidae), Corythucha ciliata (Say):(Hemiptera: Tingidae), and aphids (Sharma and Sharma, 2014). To effectively utilize these fungi as entomopathogenic agents, accurately characterizing isolates is crucial, as their efficacy in biological control can vary significantly (Carneiro-Leão et al., 2017). Additionally, secondary metabolites produced by Streptomyces sp. play a crucial role in managing agricultural pests such as Galleria mellonella (Linnaeus) (Lepidoptera: Pyralidae), Plutella xylostella (Linnaeus) (Lepidoptera: Plutellidae), and Dactylopius opuntiae (Cockerell) (Hemiptera: Dactylopiidae) (Kim et al., 2022; Rammali et al., 2023). Bacterial chitinases and hemolysins have also demonstrated effectiveness against insects and mites (Wilson and Henderson, 2002). Bacteria primarily infect insects through ingestion, the digestive tract, and occasionally through the egg, integument, and trachea, with some entering via parasitoids and predators (Tanada and Kaya, 1993). Most bacteria isolated from insects originate from the digestive tract (Cokola, 2019), with select species showing pathogenicity towards insect hosts, garnering attention for their potential in pest control (Cokola, 2019). Within the insect digestive tract, bacteria produce enzymes such as proteinase, chitinase, and lecithinase that act on midgut cells, facilitating entry into the haemocoel (Tanada and Kaya, 1993). This invasion can lead to septicaemia and eventual mortality of the infected insect (Sabbahi et al., 2022). The integration of entomopathogenic bacteria into IPM programs necessitates precise identification of microbial agents and a thorough understanding of their bioecology and impacts on non-target organisms (Wang et al., 2016). Molecular tools are needed to distinguish isolates and monitor their presence in field conditions. Studies evaluating Streptomyces metabolites have shown high toxicity to mosquitoes while posing minimal risk to non-target organisms and ecosystems (Ganesan et al., 2018). These characteristics make Streptomyces secondary metabolites promising candidates for new-generation pesticides within IPM frameworks, balancing effectiveness with environmental safety (Chen et al., 2023). In contrast to previous findings indicating antagonistic interactions between Streptomyces species and pathogenic Alternaria such as A. alternata (Wang et al., 2020), our study examines the combined efficacy of A. murispora and S. bellus-E23-2 against P. solenopsis. Our findings reveal significant mortality among P. solenopsis adults treated with this combined approach, highlighting potential synergistic interactions between entomopathogenic fungi and bacteria. These results underscore the variable nature of microbial interactions and their contextual application in agricultural pest management. Our study contributes valuable insights into the efficacy and mechanisms of entomopathogenic fungi and bacteria in pest management. Emphasizing their effectiveness, safety, and sustainability compared to chemical treatments, we advocate for the integration of biological control agents into pest management strategies.
5 Conclusion
Our study highlights the efficacy of entomopathogenic fungi and bacteria in controlling P. solenopsis populations. Combination treatments, especially involving A. murispora and S. bellus-E23-2 (1010 conidia mL−1 + 1010 cfu mL−1), showed synergistic effects, resulting in the highest mortality rates These biological agents effectively reduced pest densities without compromising the visual quality of treated potato plants, in contrast to chemical alternatives such as imidacloprid. Future research should focus on optimizing application methods and concentrations of these biological control agents, conducting field trials to validate their effectiveness under real-world conditions, and exploring the mechanisms underlying their synergistic effects. Integrating entomopathogenic fungi and bacteria into integrated pest management strategies offers promising and sustainable solutions for controlling P. solenopsis infestations.
Data availability statement
The raw data supporting the conclusions of this article will be made available by the authors, without undue reservation.
Author contributions
MA: Writing – original draft, Writing – review & editing. SR: Writing – original draft, Writing – review & editing. FK: Writing – original draft, Writing – review & editing. RL: Writing – original draft, Writing – review & editing. GC: Writing – original draft, Writing – review & editing. VB: Writing – original draft, Writing – review & editing. AC: Writing – original draft, Writing – review & editing. AP: Writing – original draft, Writing – review & editing. ON: Writing – original draft, Writing – review & editing. BN: Writing – original draft, Writing – review & editing. MS: Writing – original draft, Writing – review & editing.
Funding
The author(s) declare that no financial support was received for the research, authorship, and/or publication of this article.
Conflict of interest
The authors declare that the research was conducted in the absence of any commercial or financial relationships that could be construed as a potential conflict of interest.
Publisher’s note
All claims expressed in this article are solely those of the authors and do not necessarily represent those of their affiliated organizations, or those of the publisher, the editors and the reviewers. Any product that may be evaluated in this article, or claim that may be made by its manufacturer, is not guaranteed or endorsed by the publisher.
Footnotes
References
Abbas, G., Arif, M. J., Ashfaq, M., Aslam, M., and Saeed, S. (2010). Host plants, distribution and overwintering of cotton mealybug (Phenacoccus solenopsis) Homoptera: Pseudococcidae. Int. J. Agric. Biol. 12, 421–425.
Arasu, M. V., Al-Dhabi, N. A., Saritha, V., Duraipandiyan, V., Muthukumar, C., and Kim, S. J. (2013). Antifeedant, larvicidal and growth inhibitory bioactivities of novel polyketide metabolite isolated from Streptomyces sp. AP-123 against Helicoverpa armigera and Spodoptera litura. BMC Microbiol. 13, 1–6. doi: 10.1186/1471-2180-13-105
Arif, M. I., Rafiq, M., Wazir, S., Mehmodd, N., and Ghaffar, A. (2012). Studies on cotton mealybug, Phenacoccus solenopsis (Pseudococcidae: Homoptera), and its natural enemies in Punjab, Pakistan. Int. J. Agric. Biol. 14, 557–562.
Bérdy, J. (2012). Thoughts and facts about antibiotics: where we are now and where we are heading. J. Antibiot. (Tokyo) 65, 385–395. doi: 10.1038/ja.2012.27
Binod, P., Sukumaran, R. K., Shirke, S. V., Rajput, J. C., and Pandey, A. (2007). Evaluation of fungal culture filtrate containing chitinase as a biocontrol agent against Helicoverpa armigera. J. Appl. Microbiol. 103, 1845–1852. doi: 10.1111/j.1365-2672.2007.03428.x
Bream, A. S., Ghazal, S. A. E. L., El-Aziz, Z. K. A., and Ibrahim, S. Y. (2001). Insecticidal activity of selected actinomycete strains against the Egyptian cotton leaf worm Spodoptera littoralis (Lepidoptera: Noctuidae). Meded. Rijksuniv. Gent Fak. Landbouwkd. Toegep. Biol. Wet. 66, 503–512.
Carneiro-Leão, M. P., Tiago, P. V., Medeiros, L. V., da Costa, A. F., and de Oliveira, N. T. (2017). Dactylopius opuntiae: control by the fusarium incarnatum–equiseti species complex and confirmation of mortality by DNA fingerprinting. J. Pestic. Sci. 90, 925–933. doi: 10.1007/s10340-017-0841-4
Chen, C. D., Hassandarvish, P., Tan, G. Y. A., AbuBakar, S., and Azman, A. S. (2023). Insecticidal activities of Streptomyces sp. KSF103 ethyl acetate extract against medically important mosquitoes and non target organisms. Sci. Rep. 13:4. doi: 10.1038/s41598-022-25387-9
Chen, H. Y., Li, H. L., Pang, H., Zhu, C. D., and Zhang, Y. Z. (2021). Investigating the parasitoid community associated with the invasive mealybug Phenacoccus solenopsis in southern China. Insects 12:290. doi: 10.3390/insects12040290
Chen, A., Mao, X., Sun, Q., Wei, Z., Li, J., You, Y., et al. (2021). Alternaria mycotoxins: an overview of toxicity, metabolism, and analysis in food. J. Agric. Food Chem. 69, 7817–7830. doi: 10.1021/acs.jafc.1c03007
Cloyd, R. A., and Dickinson, A. (2006). Effect of insecticides on mealybug destroyer (Coleoptera: Coccinellidae) and parasitoid Leptomastix dactylopii (Hymenoptera: Encyrtidae), natural enemies of Citrus mealybug (Homoptera: Pseudococcidae). J. Econ. Entomol. 99, 1596–1604. doi: 10.1093/jee/99.5.1596
Cokola, C. M. (2019). Monitoring, caractérisation moléculaire et lutte biologique contre Spodoptera frugiperda (Lepidoptera: Noctuidae). Université de Liège, Liège, Belgique, 79 p. Travail de fin d’études, Gembloux Agro-Bio Tech. Available at: http://matheo.uliege.be/handle/2268.2/8077 (accessed January 10, 2020).
Cuthbertson, A. G., Walters, K. F., and Deppe, C. (2005). Compatibility of the entomopathogenic fungus Lecanicillium muscarium and insecticides for eradication of sweet potato whitefly, Bemisia tabaci. Mycopathologia 160, 35–41. doi: 10.1007/s11046-005-6835-4
Daniel, C., and Wyss, E. (2010). Field applications of entomopathogenic fungi against Rhagoletis cerasi. J. Appl. Entomol. 134, 675–681.
de Souza, B. F., Cerqueira, D. A. M. J., Monaísy Alencar Lima, H., de Melo, R. V., Forti Broglio-Micheletti, S. M., Prédes Trindade, R. C., et al. (2009). Control of Diaspis echinocacti (Bouché, 1833) (Hemiptera: Diaspididae) in prickly-pear. Acta Hortic. 811, 223–226. doi: 10.17660/ActaHortic.2009.811.27
Ebani, V. V., and Mancianti, F. (2021). Entomopathogenic fungi and bacteria in a veterinary perspective. Biology 10:479. doi: 10.3390/biology10060479
El Aalaoui, M., and Sbaghi, M. (2024). Ecological dynamics of Phenacoccus solenopsis Tinsley (Hemiptera: Pseudococcidae) and its parasitoids in varied host environments. Phytoparasitica 52, 1–15. doi: 10.1007/s12600-024-01163-5
El-Mageed, A., Youssef, N. M., and Mostafa, M. E. (2018). Efficacy of some different insecticides against cotton mealybug, Phenacoccus solenopsis Tinsley (Hemiptera: Pseudococcidae) and its associated predators. J. Plant Prot. Pathol. 9, 351–355. doi: 10.21608/jppp.2018.41677
Fand, B. B., and Suroshe, S. S. (2015). The invasive mealybug Phenacoccus solenopsis Tinsley, a threat to tropical and subtropical agricultural and horticultural production systems–a review. Crop Prot. 69, 34–43. doi: 10.1016/j.cropro.2014.12.001
Ganesan, P., Stalin, A., Paulraj, M. G., Balakrishna, K., Ignacimuthu, S., and Al-Dhabi, N. A. (2018). Biocontrol and non-target effect of fractions and compound isolated from Streptomyces rimosus on the immature stages of filarial vector Culex quinquefasciatus say (Diptera: Culicidae) and the compound interaction with acetylcholinesterase (AChE1). Ecotoxicol. Environ. Saf. 161, 120–128. doi: 10.1016/j.ecoenv.2018.05.061
Gao, T., Wang, Z., Huang, Y., Keyhani, N. O., and Huang, Z. (2017). Lack of resistance development in Bemisia tabaci to Isaria fumosorosea after multiple generations of selection. Sci. Rep. 7, 1–11. doi: 10.1038/srep42727
Gettys, L. A., and Haller, W. T. (2012). Effect of herbicide-treated irrigation water on four vegetables. Weed Technol. 26, 272–278. doi: 10.1614/WT-D-11-00120.1
Gettys, L. A., Thayer, K. L., and Sigmon, J. W. (2021). Evaluating the effects of acetic acid and d-limonene on four aquatic plants. HortTechnology 31, 225–233. doi: 10.21273/HORTTECH04769-20
Goettel, M. S., and Inglis, D. G. (1997). “Fungi: Hyphomycetes” in Manual of techniques in insect pathology. ed. L. Lacey (London, UK: Academic Press), 213–249.
Gonthier, J., Arnó, J., Romeis, J., and Collatz, J. (2023). Few indirect effects of baculovirus on parasitoids demonstrate high compatibility of biocontrol methods against Tuta absoluta. Pest Manag. Sci. 79, 1431–1441. doi: 10.1002/ps.7314
Gopalakrishnan, S., Vadlamudi, S., Samineni, S., and Kumar, C. V. S. (2016). Plant growth-promotion and biofortification of chickpea and pigeonpea through inoculation of biocontrol potential bacteria, isolated from organic soils. Springerplus 5, 1–11. doi: 10.1186/s40064-016-3590-6
Gul, H. T., Saeed, S., and Khan, F. A. (2014). Entomopathogenic fungi as effective insect pest management tactic: a review. Appl. Sci. Bus. Econ. 1, 10–18.
Herker, M., Kleefeldt, U., and Stephan, D. (2010). Laboratory experiments with entomopathogenic fungi on artificial hideouts for biocontrol of Cydia pomonella and Cydia funebrana. In: Fördergemeinschaft Ökologischer Obstbau e.V. (FOEKO): 14th International Conference on Organic Fruit-growing, Proceedings to the Conference from February 22 to February, 24, 2010 at Hohenheim/Germany, 149–155.
Herren, H. R., and Neuenschwander, P. (1991). Biological control of cassava pests in Africa. Annu. Rev. Ent. 36, 257–283. doi: 10.1146/annurev.en.36.010191.001353
Iqbal, M., Shaheen, F. A., Mahmood, R., Rafique, M. K., Bodlah, I., Naz, F., et al. (2019). Synergistic effect of Entomopathogenic Fungi and Bacteria against pulse beetle, Callosobruchus chinensis. Pak. J. Zool. 51, 1685–1691. doi: 10.17582/journal.pjz/2019.51.5.1685.1691
Kairo, M. T. K., Pollard, G. V., Peterkin, D. D., and Lopez, V. F. (2000). Biological control of the hibiscus mealybug, Maconellicoccus hirsutus (green) (Hemiptera; Pseudococcidae) in the Caribbean. Integr. Pest Manage Rev. 5, 241–254. doi: 10.1023/A:1012997619132
Kim, J. Y., Choi, J. Y., Park, D. H., Park, M. G., Wang, M., Kim, H. J., et al. (2022). Juvenile hormone antagonistic activity of secondary metabolites from Streptomyces lactacystinicus and their insecticidal activity against Plutella xylostella. J. Asia Pac. Entomol. 25:101870.
Marimuthu, S., Karthic, C., Mostafa, A. A., Al-Enazi, N. M., Abdel-Raouf, N., and Sholkamy, E. N. (2020). Antifungal activity of Streptomyces sp. SLR03 against tea fungal plant pathogen Pestalotiopsis theae. J. King Saud. Univ. Sci. 32, 3258–3264. doi: 10.1016/j.jksus.2020.08.027
Mazzeo, G., Nucifora, S., Russo, A., and Suma, P. (2019). Dactylopius opuntiae, a new prickly pear cactus pest in the Mediterranean: an overview. Entomol. Exp. Appl. 167, 59–72. doi: 10.1111/eea.12756
Mohammadbeige, A., and Port, G. (2013). Efficacy of Beauveria bassiana and Metarhizium anisopliae against Uvarovistia zebra (Orthoptera: Tettigoniidae) via contact and ingestion. Int. J. Agric. Crop Sci. 5, 138–146.
Nagrare, V. S., Kranthi, S., Kumar, R., DharaJothi, B., Amutha, M., Deshmukh, A. J., et al. (2011). Compendium of cotton mealybugs. Nagpur, India: Central Institute for Cotton Research, 42.
Nawaz, M., and Freed, S. (2022). Pathogenicity of different isolates of entomopathogenic fungi on cotton mealybug, Phenaccocus solenopsis Tinsley. Pak. J. Zool. 54, 275–282. doi: 10.17582/journal.pjz/20161124031127
Nicoletti, R., Di Vaio, C., and Cirillo, C. (2020). Endophytic fungi of olive tree. Microorganisms. 8:1321. doi: 10.3390/microorganisms8091321
Okongo, R. N., Puri, A. K., Wang, Z., Singh, S., and Permaul, K. (2019). Comparative biocontrol ability of chitinases from bacteria and recombinant chitinases from the thermophilic fungus Thermomyces lanuginosus. J. Biosci. Bioeng. 127, 663–671. doi: 10.1016/j.jbiosc.2018.11.007
Panyasiri, C., Attathom, T., and Poehling, H. M. (2007). Pathogenicity of entomopathogenic fungi-potential candidates to control insect pests on tomato under protected cultivation in Thailand. J. Plant Dis. Prot. 114, 278–287. doi: 10.1007/BF03356230
Quesada-Moraga, E., Garrido-Jurado, I., Yousef-Yousef, M., and González-Mas, N. (2022). Multitrophic interactions of entomopathogenic fungi in BioControl. BioControl 67, 457–472. doi: 10.1007/s10526-022-10163-5
Ramakrishnan, N., Sexena, S. V., and Dhingra, S. (1984). Insecticide resistance in the population of Spodoptera litura (F.) in Andhra Pradesh. J. Pestic. Sci. 18, 23–27.
Rammali, S., El Aalaoui, M., Sbaghi, M., Dari, K., Bencharki, B., Azeroual, A., et al. (2023). Insecticidal potential of Streptomyces sp. dichloromethane extracts sp. against the cactus cochineal cochineal Dactylopius opuntiae (Cockerell). Not. Sci. Biol. 13, 1–12.
Rammali, S., Hilali, L., Dari, K., Bencharki, B., Rahim, A., Timinouni, M., et al. (2022). Antimicrobial and antioxidant activities of Streptomyces species from soils of three different cold sites in the Fez-Meknes region Morocco. Sci. Rep. 12, 1–22. doi: 10.1038/s41598-022-21644-z
Rishikesh, G. D. R., Haque, M. A., Islam, M. A. U., Rahman, M. M., and Banu, M. R. (2013). In-vitro insecticidal activity of crude extracts of Streptomyces sp. against larvae of Sitophilus oryzae. J. Drug Discov. Ther. 1, 60–63.
Ruiu, L. (2015). Insect pathogenic bacteria in integrated pest management. Insects. 6, 352–367. doi: 10.3390/insects6020352
Sabbahi, R., Hock, V., Azzaoui, K., Saoiabi, S., and Hammouti, B. (2022). A global perspective of entomopathogens as microbial biocontrol agents of insect pests. J. Agric. Food Res. 10:100376. doi: 10.1016/j.jafr.2022.100376
Saeed, S., Ahmad, M., Ahmad, M., and Kwon, Y. J. (2007). Insec ticidal control of the mealybug Phenacoccus gossypiphilous (Hemiptera: Pseudococcidae), a new pest of cotton in Pakistan. Entomol. Res. 37, 76–80. doi: 10.1111/j.1748-5967.2007.00047.x
Sahayaraj, K., and Namachivayam, S. K. R. (2011). Field evaluation of three entomopathogenic fungi on groundnut pests. Tropicultura 29, 143–147.
Salunkhe, R. B., Patil, C. D., Salunke, B. K., Rosas-Garcia, N. M., and Patil, S. V. (2013). Effect of wax degrading bacteria on life cycle of the pink hibiscus mealybug, Maconellicoccus hirsutus (green) (Hemiptera: Pseudococcidae). BioControl 58, 535–542. doi: 10.1007/s10526-013-9513-3
Sharma, I., and Sharma, A. (2014). Use of Alternaria spp. as a pest control agent: a review. World Appl. Sci. J. 31, 1869–1872.
Shin, Y.-H., Kang, S., Byun, W. S., Jeon, C.-W., Chung, B., Beom, J. Y., et al. (2020). Absolute configuration and antibiotic activity of piceamycin. J. Nat. Prod. 83, 277–285. doi: 10.1021/acs.jnatprod.9b00678
Singh, S. P. (2004). Some success stories of classical biological control of agricultural pests in India. Asia specific Association of Agricultural Institutions, FAO regional office for ASIA and the Pacific. APAARI publication 2004/2, Bangkok, Thailand, pp. 73.
Singh, R., Kapoor, V., and Kumar, V. (2011). Production of thermostable, ca+2-independent, maltose producing $α$-amylase by Streptomyces sp. MSC702 (MTCC 10772) in submerged fermentation using agro-residues as sole carbon source. Ann. Microbiol. 62, 1003–1012. doi: 10.1007/s13213-011-0340-4
Smith, H. C., Ferrell, J. A., and Koschnick, T. J. (2014). Flurprimidol performance on ornamental species in relation to trimming time and method of application. Hort Sci. 49, 1305–1308.
Suresh, S., Jothimani, R., Sivasubramanian, P., Karuppuchamy, P., Samiyappan, R., and Jonathan, E. I. (2010). Invasive mealybug of Tamil Nadu and their management. Karnataka J. Agric. Sci. 23, 6–9.
Tanwar, R. K., Jeyakumar, P., and Monga, D. (2007). Mealybugs and their management. Technical bulletin 19. New Delhi: National Centre for Integrated Pest Management, 12.
Tong, H., Ao, Y., Li, Z., Wang, Y., and Jiang, M. (2019). Invasion biology of the cotton mealybug, Phenacoccus solenopsis Tinsley: current knowledge and future directions. J. Integr. Agric. 18, 758–770. doi: 10.1016/S2095-3119(18)61972-0
Tootoonchi, M., Gettys, L., Thayer, K., Markovich, I., Sigmon, J., and Sadeghibaniani, S. (2020). Ecotypes of aquatic plant Vallisneria americana tolerate different salinity concentrations. Diversity 12:65. doi: 10.3390/d12020065
Ujjan, A. A., Khanzada, M. A., Mahar, A. Q., and Shahzad, S. (2015). Efficiency of Metarhizium spp. (Sorokīn) strains and insecticides against cotton mealybug Phenacoccus solenopsis (Tinsley). Pak. J. Zool. 47, 351–360.
Vega, F. E., Meyling, N. V., Luangsa-ard, J. J., and Blackwell, M. (2012). Fungal entomopathogens. Insect Pathol. 2, 171–220.
Wang, Q., Bosch, B. J., Vlak, J. M., Van Oers, M. M., Rottier, P. J. M., and Van Lent, J. W. M. (2016). Budded baculovirus particle structure revisited. J. Invertebr. Pathol. 134, 15–22. doi: 10.1016/j.jip.2015.12.001
Wang, M., Xue, J., Ma, J., Feng, X., Ying, H., and Xu, H. (2020). Streptomyces lydicus M01 regulates soil microbial community and alleviates foliar disease caused by Alternaria alternata on cucumbers. Front. Microbiol. 11:942. doi: 10.3389/fmicb.2020.00942
Wilson, M., and Henderson, B. (2002). Bacterial disease mechanisms, an introduction to cellular microbiology. Cambridge: Cambridge University Press.
Xi, L., Liu, D., Ma, L., Zhang, Y., Sheng, R., Zhang, S., et al. (2019). Expression patterns, molecular characterization, and response to host stress of CYP genes from Phenacoccus solenopsis (Hemiptera: Pseudococcidae). Insects 10:264. doi: 10.3390/insects10090264
Keywords: biological control, pest management, fungal pathogens, bacterial pathogens, sustainable agriculture, crop protection
Citation: El Aalaoui M, Rammali S, Kamal FZ, Lefter R, Calin G, Burlui V, Ciobică A, Petroaie AD, Novac O, Novac B and Sbaghi M (2024) Biocontrol of Phenacoccus solenopsis Tinsley using entomopathogenic fungi and bacteria. Front. Sustain. Food Syst. 8:1444917. doi: 10.3389/fsufs.2024.1444917
Edited by:
Abhinav Upadhyay, University of Connecticut, United StatesReviewed by:
Ana Paula Ramos, University of Lisbon, PortugalSwarnalee Dutta, Jeonbuk National University, Republic of Korea
Copyright © 2024 El Aalaoui, Rammali, Kamal, Lefter, Calin, Burlui, Ciobică, Petroaie, Novac, Novac and Sbaghi. This is an open-access article distributed under the terms of the Creative Commons Attribution License (CC BY). The use, distribution or reproduction in other forums is permitted, provided the original author(s) and the copyright owner(s) are credited and that the original publication in this journal is cited, in accordance with accepted academic practice. No use, distribution or reproduction is permitted which does not comply with these terms.
*Correspondence: Mohamed El Aalaoui, mohamed.elaalaoui@inra.ma; Gabriela Calin, m_gabriela2004@yahoo.com