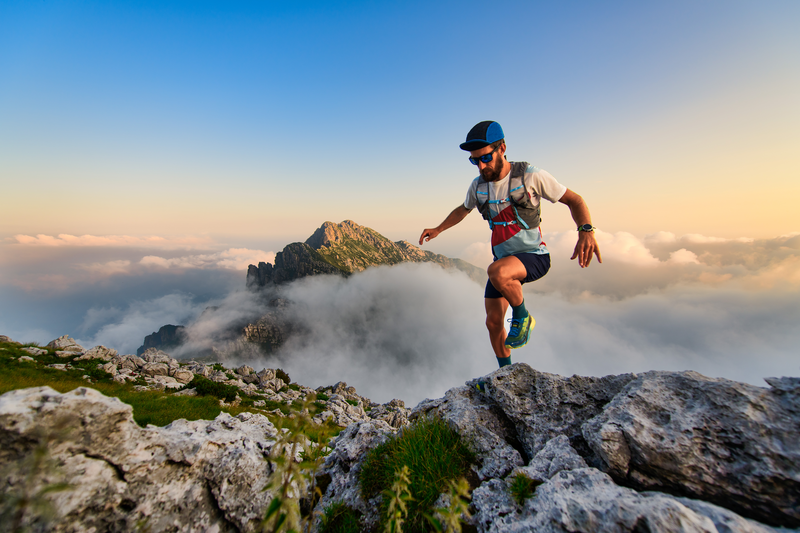
95% of researchers rate our articles as excellent or good
Learn more about the work of our research integrity team to safeguard the quality of each article we publish.
Find out more
ORIGINAL RESEARCH article
Front. Sustain. Food Syst. , 15 August 2024
Sec. Sustainable Food Processing
Volume 8 - 2024 | https://doi.org/10.3389/fsufs.2024.1434121
This article is part of the Research Topic Sustainable Drying Technologies for Foods: Enhancing Quality and Energy Efficiency View all 6 articles
This study investigated the effects of hot air drying (HAD), infrared-assisted hot air drying (IR-HAD), vacuum freeze drying (VFD), and pulsed-vacuum drying (PVD) on the drying characteristics, color, microstructure, total phenolic content (TPC), total flavonoid content (TFC), vitamin C (Vc) content, and the odor analysis using electronic nose of sea buckthorn pomace. The results showed that IR-HAD exhibited the highest drying rate and the shortest drying time (250 min). PVD exhibited the highest TPC, TFC, and Vc content while maintaining a color difference ΔE value closest to that of the fresh sample. PVD microstructure exhibited no collapse, fewer pores, and smaller cracks than the IR-HAD and VFD microstructures. Furthermore, the PVD pomace displayed the lowest reactivity to sulfides and ethanol compounds. The comprehensive analysis suggests that PVD holds promise as a drying method for sea buckthorn pomace and provides valuable insights for selecting an appropriate drying method for sea buckthorn pomace.
Sea buckthorn (Hippophae rhamnoides L.) belongs to the family Elaeagnaceae and is a dioecious deciduous shrub or small arbor with thorny characteristics. It is widely distributed in Asia and Europe (Dhyani et al., 2010; Liu et al., 2018). Due to the tolerance of the harsh environment, it has been widely planted in northwestern China and used for ecological development (Ruan et al., 2013). The berries of sea buckthorn contain effective nutrients therapeutic qualities and biological activity chemicals. Its fruits, leaves, and other parts are rich in bioactive compounds. Such as vitamins, polyphenols, flavonoids, and carotenoids, and have been used in traditional medicine. These chemicals have proven to provide a variety of health benefits, including antioxidants, anti-inflammatory and anti-tumor special (Ji et al., 2020; Gatlan and Gutt, 2021).
Globally, the agricultural food supply chain generates significant waste and by-products. Utilizing these by-products presents a challenge amidst the increasing global population and future agricultural food resource crises. The utilization of food processing by-products and the recovery of functional compounds with health benefits, ultimately replacing synthetic additives, can protect the environment from the adverse impacts of waste (Bhat, 2021; Galanakis, 2021; Luntraru et al., 2022). In the sea buckthorn industry, the focus is primarily on beverage processing and oil production. However, sea buckthorn pomace, the residue from fruit juice extraction, is often wasted, resulting in substantial resource loss. It can be used to extract the flavonoid-rich pigment sea buckthorn yellow pigment. It is an excellent high-nutrient food additive that may be used to make a variety of unique foods such as sweets, haw pieces, and sea buckthorn cakes. Additionally, it can be processed into condiments, sauces, and vinegar, highlighting its versatility (Ciesarova et al., 2020).
Fresh sea buckthorn pomace has a lot of moisture, and its active ingredients are easily oxidized and deteriorate. As a result, using appropriate drying methods to remove moisture is critical to preventing the degradation of plant chemical compounds and microbial contamination. It facilitates the extraction of bioactive chemicals, suppresses enzyme activity, and aids in the reduction of storage costs and waste (Kyriakopoulou et al., 2013; Fathi et al., 2022). However, drying can cause nutritional loss and unwanted structural changes, compromising the final product’s quality and limiting its commercial worth (An et al., 2022). Therefore, it is essential to investigate the impact of drying on the quality of the final product. The most frequent drying method used in the food industry is hot air drying (HAD). HAD utilizes high-speed airflow and convective heat transfer on the sample’s surface to facilitate moisture migration from the interior to the exterior. This technique offers advantages such as cost-effectiveness, controllability, and ease of use. However, it may lead to the loss of active ingredients in the dried product (Zhang et al., 2023). Infrared-assisted hot air drying (IR-HAD) employs infrared radiation to convert molecular vibrations into heat, enabling rapid and stable absorption. Compared to conventional HAD, IR-HAD offers shorter drying times, higher energy efficiency, and improved product quality. As a result, it has found widespread application in the food industry for various processing operations (Yao et al., 2020; Huang et al., 2021). Vacuum freeze-drying (VFD) is an emerging technology in the drying field in recent years. It utilizes sublimation for dehydration and creates a low-temperature vacuum environment to preserve the color, shape, and nutritional components of the sample to a maximum extent. However, the high cost of VFD equipment and the significant amount of drying time required to complete the process largely limit the industrial application potential of VFD (Jiang et al., 2017; An et al., 2022). Pulsed vacuum drying (PVD) is a relatively novel drying technology that has emerged in recent years. By alternating between atmospheric pressure and vacuum, PVD disrupts the vapor pressure equilibrium between the internal and external environments. This process promotes the interconnection and expansion of micropores within the sample, leading to reduced drying times. Moreover, PVD is known for its ability to effectively preserve the color and bioactive substances of the dried sample (Liu et al., 2021; Zhou et al., 2022). However, the research on drying characteristics, physicochemical properties, microstructure, and odor of sea buckthorn pomace using HAD, IR-HAD, VFD, and PVD drying methods is still limited.
Therefore, the objectives of this study are as follows: (i) Assessing the drying characteristics of sea buckthorn pomace under different drying methods; (ii) Analyzing the physicochemical properties and bioactive substances of sea buckthorn dried using different drying methods; (iii) Examined the correlations among various quality characteristics of dried sea buckthorn pomace. This study aims to maximize the utilization of waste resources in the sea buckthorn industry and produce high-value dietary fiber products for different applications. It also seeks to contribute to the extension of the sea buckthorn industry chain by providing theoretical references. The research findings can serve as a basis for selecting the optimal drying method for sea buckthorn pomace.
The raw material sample used in this study was sea buckthorn pomace (pericarp, seeds, and residual pulp), which is a by-product of sea buckthorn berry juice extraction, and was obtained from No. 170 State Farm in E-ming (Tacheng, Xinjiang, China). Sea buckthorn residues were packed in sealed bags and frozen to −25°C, then transported back to the laboratory on the same day, de-seeded, and pre-frozen for use. The average moisture mass fraction of fresh sea buckthorn pomace dried at 105°C for 24 h was obtained according to the oven drying method was 67.13 ± 0.94%(w.b.) (Tan et al., 2022). The total phenolic content (TPC) of fresh sea buckthorn pomace was measured to be 25.85 ± 0.21 mg GAE/g (Dry basis). The total flavonoid content (TFC) was determined to be 3.16 ± 0.05 mg RE/g (Dry basis), and the Vitamin C (Vc) content was found to be 92.36 ± 4.57 mg/100 g (Dry weight).
Sea buckthorn pomace is rich in total phenolic substances and vitamins, while the seeds have relatively different nutrient contents. Therefore, removing the seeds can improve the purity and effectiveness of nutrient extraction from the pomace, as well as reduce obstacles during drying and crushing, making the pomace easier to handle and process (Tan et al., 2022). After removing the seeds of sea buckthorn fruit pomace, 10 g samples were accurately weighed and placed in stainless steel metal grid trays with a loading density of 0.2 kg/m2 and a thickness of 2 mm and were dried by four drying methods, namely, HAD, IR-HAD, VFD, and PVD, which were all repeated for three times, and the average of the results was taken for analysis, and then sealed and packaged after cooling. The experimental process parameters were set based on production research, combined with relevant literature and the results of the preliminary pre-experiment, and the experimental process is shown in Figure 1.
The experiment used a hot air dryer (HAD) (DHG-9070A, Yiheng Technology Co., Ltd., Shanghai, China). The temperature of the drying medium was set at 60°C and the air velocity was set at 2 m/s. The equipment was turned on until the set value was reached and stabilized, and then the trays were put into the drying process, and the trays were placed parallel to the airflow. The samples were weighed at intervals of 30 min, and when the moisture content of the samples dropped to moisture content of less than 5%, the drying was stopped and the samples were taken out after cooling and sealed for storage.
The drying equipment used in the experiment is a short and medium-wave infrared combined hot air dryer (IR-HAD) (Taizhou Shengtai Infrared Technology Co., Ltd., Shihezi, China). The temperature of the drying medium was set at 60°C, the air velocity was kept at 3 m/s, the infrared power was set at 675 W, and according to the previous experiments, the material trays were placed at a distance of 120 mm from the infrared radiation tube, and the drying was carried out after the equipment was turned on to reach the set value and the value was stabilized. Weighing every 30 min, until the moisture content drops to less than 1%, stop drying, and after cooling, take out and seal storage.
Vacuum Freeze Drying (VFD) [CHRIST Freeze Dryer GmbH (Osterode, Germany)] was used for the experiments. Based on the previous experiments, the condenser temperature was set at −53°C, the heating plate temperature was set at 60°C, and the drying chamber pressure was set at 12 Pa. Drying was carried out after the equipment was turned on to reach the set values and the values were stabilized. Weighing was carried out every 30 min until the moisture content dropped to less than 1%, then the drying was stopped, and the samples were taken out and sealed for storage.
The equipment used for the test was a vacuum freeze drying (VFD) (Laboratory of Agricultural Product Processing Technology and Equipment, School of Mechanical and Electrical Engineering, Shihezi University, Shihezi, China), which was heated by a far-infrared radiant heating plate, with the temperature set at 60°C, the dryer holding time at atmospheric pressure was 25 min, the vacuum holding time was 5 min, and the pulsation ratio was 5:1. The equipment was turned on to reach the set value and the value is stabilized for drying. Weighing every 30 min, until the moisture content drops below 1%, stop drying, then cooling, take out, seal, and store.
The moisture ratio (MR) represents the remaining moisture content in a material, while the drying rate (DR) indicates the speed at which the material is dehydrated. The unit of drying rate is typically expressed as g/(g·h). By employing the method of weighing at regular time intervals, the MR as a function of drying time (t) and the drying rate (DR) as a function of moisture content on a dry basis (Mt) could be plotted for different drying methods during the drying process.
The dry basis moisture content (Mt) of sea buckthorn pomace during drying was calculated utilizing Eq. (1) (Ni et al., 2020):
where Wt delegates the total mass at any given drying time t (g); G is the mass of dry matter (g).
The moisture ratio (MR), which represents the moisture content of sea buckthorn pomace at drying time t, could be calculated using Eq. (2) (Geng et al., 2023):
where Mt is the dry basis moisture content of sea buckthorn pomace at drying time t (g/g); M0 is the dry basis moisture content of sea buckthorn pomace at the initial time (g/g).
DR is calculated using Eq. (3) (Jiang et al., 2020):
where DR is the drying rate [g/(g∙h)]; Mt1 is the dry basis moisture content at drying time t1 (g/g).
The color indexes of fresh and dried sea buckthorn pomace were quantitatively measured using SMY-2000SF colorimeter (Beijing Mingyang Technology Development Co., Ltd., Beijing, China). The fresh pomace was deseeded, and ground into pulp using an ice bath, and the dried sea buckthorn pomace was pulverized into a powdered form using a grinder. Color measurements were taken three times per experimental group to calculate averages. The L* value (brightness), a* value (red/green), and b* value (yellow/blue) of the sea buckthorn pomace powder were measured, and the total color difference (ΔE), color saturation (C), and hue angle (h°) were calculated to determine the degree of color difference in the material. These calculations are performed using Eqs. (4)–(6) (Wang P. et al., 2023):
Where and represent the brightness of the dried sample and fresh sample, respectively; and represent the red-green values of the dried sample and fresh sample, respectively; and represent the yellow-blue values of the dried sample and fresh sample, respectively.
S-3400 scanning electron microscope (Hitachi, Japan) was used to detect the changes in the surface microstructure of dried sea buckthorn pomace, according to the method described by Yao et al. (2020). Modifications were made for the examination of dried sea buckthorn pomace. Epidermal thin slices of the dried sea buckthorn pomace, measuring 5 mm × 5 mm, were prepared and securely affixed to the sample stage using double-sided adhesive tape. The samples were then magnified at 500× and 1,000×, with an accelerating voltage of 5 kV. At least three images were captured from three different locations on each sample.
The vitamin C (Vc) content in sea buckthorn pomace was determined using a modified version of the titration method described by Deng et al. (2017). Firstly, prepare a standard solution with a concentration of 1.0 mg/mL: prepare 2% oxalic acid to dissolve 100.00 mg of L (+) – ascorbic acid standard, add 2% oxalic acid to a 100 mL brown volumetric flask and dilute well. Next, for the extraction of Vc from the sample, a weight of 1.00 g of dried sea buckthorn pomace powder is measured and placed into a mortar for grinding until it forms a slurry. Then, a 2% oxalic acid solution is added to the slurry at a ratio of 1:20 (g/mL). The extraction is performed using a 200 W ultrasonic ice bath for 30 min. Finally, the mixture is centrifuged at 8,000 rpm for 30 min in a centrifuge (LC-LX-H185C, Lichen Bonsi Instrument Technology Co., Ltd., Shanghai, China), and the supernatant is collected. To avoid color interference in the sample solution, the Vc content was determined using the 2,6-dichlorophenol indophenol (DCPIP) back-titration method and expressed as mg/100 g of dry weight (DW). The Vc content in the sample is calculated using Eq. (7):
where A is the ascorbic acid content (mg/100 g); is the standard solution concentration (mg/mL); is the volume of standard solution consumed for titrating 2,6-dichloro indole sodium salt (5 mL) (mL); is the total volume of supernatant (mL); is the volume of supernatant consumed for titration of 2,6-dichlororel sodium salt (5 mL) (mL); is the sample dry weight (g).
The extraction process of total phenols in the samples was as follows: 1.50 g of dry powder was placed in a 50 mL test tube, 95% ethanol was added according to the material-liquid ratio of 1:25 (g/mL), and extracted in an ultrasonic apparatus at 200 W, 80°C for 35 min, cooled to room temperature, and centrifuged for 10 min (8,000 r/min). Finally, the supernatant was collected. Preparation of standard solution: gallic acid standard (10.00 mg) was mixed with 60% ethanol and diluted into a 10 mL volumetric flask to obtain a standard solution with a concentration of 1.0 mg/mL. Finally, the standard solution was diluted to 20, 40, 60, 80, 100, and 320 μg/mL. The TPC of the extract was determined by the Folin–Ciocalteu method (Tan et al., 2022). 0.4 mL of the extract and Folin-ciocalteu reagent solution (10%, 2 mL) were placed in a 10 mL test tube, sodium carbonate solution (10%, 3 mL) was added, and left to stand for 2 h. Absorbance was measured at 765 nm using a UV spectrophotometer [UV-1900i, Shimadzu Co., Ltd. (Suzhou, Suzhou, China)], and each assay was performed in triplicate. The TPC was expressed as gallic acid equivalent per g dry weight (mg GAE/g, d.b.s).
Standard solution preparation: Rutin standard (100.00 mg) was mixed with 60% ethanol and diluted into a 100 mL volumetric flask to obtain a standard solution with a concentration of 0.2 mg/mL. Finally, the standard solutions were diluted to 40, 80,160, and 320 μg/mL. The extraction method for TFC was the same as that for TPC. The TFC of the extract was determined according to the method described by Geng et al. (2023) with modification. The extract (1.5 mL) and NaNO2 solution (5%, 1.5 mL) were placed into 10 mL test tubes. After 5 min, aluminum trichloride solution (10%, 0.4 mL) was added, and it was left to stand for 5 min. After 5 min, NaOH solution (1 mol/L, 1 mL) was added and distilled water. Absorbance was measured at 510 nm using a UV spectrophotometer. Three portions were used for each determination. TFC was expressed as milligrams of rutin equivalent per gram of dry weight (mg RE/g, d.b).
Odor analysis of dried samples was performed using a PEN3.5 electronic nose (AIRSENSE Analytics GmbH, Schwerin, Germany). The electronic nose is very sensitive to aroma and can sense small aroma changes. The PEN3.5 system consists of an array of 10 sensors consisting of metal oxide semiconductors with different properties that have different sensitivities to different concentrations of gases, which are positively related to the properties and content of the odor (Li et al., 2016). Among these sensors, W1C (sensitive to aromatic compounds), W5S (sensitive to nitrogen oxides), W3C (sensitive to ammonia and aromatic compounds), W6S (sensitive to hydrogen), W5C (sensitive to hydrocarbons, aromatic compounds), W1S (sensitive to methane in the ambient environment), W1W (sensitive to sulfide, pyrazine, many terpenes such as limonene), W2S (sensitive to ethanol, some aromatic compounds, wide range), W2W (sensitive to aromatic constituents, sulfur compounds), W3S (sensitive to methane and some high concentration compounds) (Yu et al., 2022). Approximately 6.0 g of pulverized dried sea buckthorn pomace was placed in an electronic nose collection vial, left at room temperature at 25°C for 1 h, and then pumped into the sensor array at a constant rate of 300 mL/min for 180 s. All tests were performed in triplicate.
The results were expressed as mean ± standard deviation. Experimental data were analyzed by one-way analysis of variance (ANOVA) and Duncan’s multiple range test using SPSS version 21.0 (IBM Corporation, Armonk, New York, NY, United States). Statistical differences were tested at the 5% probability level (p < 0.05). OriginPro2021 (Version 9.8, OriginLab Inc., Northampton, MA, United States) was used to produce graphs and Pearson correlation analysis was used to establish correlations between various parameters of sea buckthorn pomace.
The drying kinetic curves of sea buckthorn pomace under different drying methods are shown in Figures 2A,B. The drying curves under different drying methods have similar trends, and there is a significant difference between the effects on the drying kinetics and drying time of sea buckthorn pomace (p < 0.05). Drying is the process of evaporation and diffusion of water within the material. As the drying time increases, the moisture content gradually decreases (Deng et al., 2017). The drying times of IR-HAD and VFD were the lowest (240 min) and highest (300 min). In addition, at the beginning stage of drying, the rate of water content decreased IR-HAD>HAD>PVD > VFD, and after 120 min of drying, the change of water content of different drying methods became slower and slower, and gradually stabilized, but the water content of PVD decreased faster. This phenomenon can be attributed to the continuous change of vacuum pressure which improves the mass transfer rate and allows the moisture to diffuse uniformly (Jiang et al., 2020). In the IR-HAD process, infrared radiation energy penetrates to a very small depth in the material and is then converted into heat (Huang et al., 2021). After absorbing heat, water molecules inside the sea buckthorn pomace migrate rapidly and the drying time is shorter compared to HAD (Barzegar et al., 2015). Deng et al. (2017) and Geng et al. (2023) reported similar findings regarding the drying of sea buckthorn and red pepper.
Figure 2. Drying curves (A) and drying rate curves (B) of sea buckthorn pomace under different drying methods. (a, b, c) Different lowercase letters represent significant differences at a p ≤0.05.
The DR decreased continuously with the decrease of MC, and the drying rates of different drying methods were different (Figure 2B). The drying rate of IR-HAD was significantly higher than that of HAD, VFD, and PVD at the same temperature (60°C) before the dry basis water content of 0.2 g/g (p < 0.05). During the drying process, the drying rate of the material surface is higher than that of the inner layer, resulting in rapid contraction of the sample during the early stages of drying, which affects the internal water migration (Chen et al., 2022). In the drying process, the main mechanism involves the migration of water. In the IR-HAD, the absorption of infrared radiation rapidly generates heat on the surface and inner layers of the sample. This heat is primarily converted into thermal energy through molecular vibration and rotation (Wang et al., 2017; Zheng et al., 2023).
The drying rate of VFD significantly decreased in the later stages, likely due to the difficulty in removing intracellularly bound water through alternating cycles of freezing and vacuum-atmospheric pressure. This prolongs the drying time, consistent with findings in sea buckthorn drying by Geng et al. (2023). HAD has a higher drying rate than VFD and PVD due to the initial high temperature, fast heat transfer, rapid surface temperature rise, and quick removal of humidity by hot air (Yue et al., 2023). In the later stage of drying, the drying rate of HAD is lower than that of PVD. This is due to the high-speed airflow and enhanced convective heat transfer coefficient on the HAD surface, which rapidly removes surface moisture from sea buckthorn pomace. As a result, a dense shell or layer forms on the surface, hindering internal moisture migration, evaporation, and heat transfer, leading to a reduced drying rate in the later stage.
In the early stage of drying, PVD exhibits the lowest drying rate primarily due to the relatively low efficiency of vacuum heat transfer, resulting in a slow heating rate. Additionally, the pressure pulses applied during the PVD process induce the formation of a porous structure within the material. This structure facilitates the migration of water from the interior to the exterior surface of the material. Furthermore, the continuous opening of the pressure-reducing valve during the atmospheric operation in the PVD allows cold air to enter the drying chamber. Additionally, a significant amount of heat is transferred through the vacuum pump during the vacuum-holding phase. These factors contribute to lowering the temperature of the drying chamber and the samples, ultimately impacting the drying rate of the samples (Link et al., 2017). The findings align with the data reported by Deng et al. (2017) for pulsed vacuum drying (PVD) of red chili peppers, PVD had the lowest drying rate and longest drying time compared to HAD and IR-HAD at the same temperature. However, in the final stage of PVD, the rate of drying rate decrease was significantly higher than in other methods. This can be attributed to the periodic pressure variations, which promote the movement of moisture from the material’s center to its surface (Golmohammadi et al., 2015).
The color of food holds significant importance in evaluating its visual quality and commercial value. Notably, the color superiority is closely linked to the content of Vc and TFC after the drying process (Figure 3). According to the results from Table 1, it is evident that the effect of different drying methods on the color parameters of sea buckthorn pomace is highly significant (p < 0.05). Compared to fresh samples ( =50.28 ± 0.05, a* = 30.49 ± 0.09, b* = 48.39 ± 0.11), the L* and b* of sea buckthorn pomace after drying were significantly increased and the a* was decreased, which can be explained by the degradation of flavonoids. Maximum brightness L* (57.59 ± 0.05) and b* (61.61 ± 0.10) were recorded in VFD. However, it also exhibited the highest ΔE, this phenomenon may be attributed to the fact that VFD reduces the oxidation and degradation of pigment molecules by sublimation of water directly from frozen sea buckthorn pomace under vacuum conditions, which helps maintain the stability of the pigments, resulting in higher brightness and color vibrancy (Yang et al., 2020). Torres et al. (2010) showed that VFD treatment enhanced grape brightness and that vacuum freeze-drying was the optimal method for preserving the color quality of bell peppers and blueberries.
Figure 3. Pearson correlation analysis between drying time (DT), color parameters (L*, a*, b*, ∆E, C, h°), TPC, TFC, and Vc content (Vc) of sea buckthorn pomace under different drying methods.
The lowest brightness L* (52.80 ± 0.10) was for HAD, indicating a decreased brightness. This reduction can be attributed to the prolonged exposure of the sample to oxygen, which triggered enzymatic browning reactions. Similar results were reported in HAD drying of mango (Yao et al., 2020). The higher ΔE observed in IR-HAD compared to HAD was attributed to the non-uniform internal temperature distribution caused by infrared radiation, which resulted in an uneven distribution of pigment molecules within the sea buckthorn pomace. Additionally, in the later stages of drying, the internal structure of the pomace did not reach a safe moisture content, contributing to the occurrence of surface crusting. This phenomenon promoted non-enzymatic browning and other related biochemical reactions, ultimately resulting in color deterioration.
The microstructure analysis provides valuable insights into the quality and performance changes of dried products. Scanning electron microscopy was employed to observe the effects of different drying methods on the microstructure of sea buckthorn pomace, and the results are depicted in Figure 4. The results showed that each drying method had distinct impacts on the microstructure of sea buckthorn pomace, particularly in terms of epidermal crumpling and stomatal structure and that porous materials such as fruits and vegetables formed capillary shrinkage stress within the microstructure due to evaporation of water during drying and dewatering, destruction of plasma and vesicle membranes, and loss of expansion pressure, which resulted in the shrinkage of the material in the drying process (Nour et al., 2020).
Figure 4. Scanning electron microscopy images of sea buckthorn pomace under different drying methods. Set the magnification to 500× (A) and 1.00 K × (B).
The surface of the HAD sea buckthorn seed beat was relatively smooth, and subtle tissue wrinkles could be observed, with no rupture of the cells. However, in IR-HAD, ruptured stomata, severe wrinkles, and subtle cracks were observed. These changes can be attributed to the absorption of infrared radiation, generating rapid heat on the surface and in the inner layers of the sea buckthorn seed coat, thereby accelerating moisture migration. Excessive moisture gradients within the material resulted in microstructural stresses that destroyed most of the capillaries, leading to irreversible structural changes (Shen et al., 2023).
Compared to IR-HAD, the VFD-dried sea buckthorn pomace displayed a smoother and less deformed structure. It exhibited small pores, irregular cracks, and fissures, along with numerous tiny bubbles and oil particles distributed in the epidermis. This can be attributed to the vacuum and low-temperature conditions of the VFD process. The moisture in the material transformed directly from a solid to a gaseous state, causing rapid evaporation and the formation of small pores and bubbles on the material’s surface. Similar findings were reported in studies on the freeze-drying (FD) and vacuum drying (VD) of Roselle (Hibiscus sabdariffa L.) Calyx (Juhari and Petersen, 2018; Liu et al., 2022).
It was observed that the microstructure of the samples dried under PVD showed a porous structure with ruptured stomata, presenting an obvious granularity, which was due to the rapid sublimation of water molecules under vacuum conditions, leading to a large pressure difference between the interior of the cell and its surface, which in turn caused structural damage. The appearance of microcracks was attributed to the fact that the localized stresses generated by the continuous pressure variations between the ambient pressure and the vacuum pressure exceeded the destructive strength of the berry surface and similar observations was obtained for blueberries dried by pulse vacuum drying (PVD) (Liu et al., 2022).
Vitamin C is an essential nutrient for human health and serves as an important indicator of nutritional quality in fruits and vegetables. However, it is highly susceptible to oxidative degradation caused by oxygen and heat treatment (Yao et al., 2020). The results of Vc content determination of dried sea buckthorn fruit pomace are shown in Figure 5A. The results showed that the drying led to a significant decrease in the Vc content of sea buckthorn pomace (p < 0.05). The retention of Vc in the dried pomace ranged from 67.94 to 80.81%, with the highest retention observed in PVD (74.64 ± 1.38 mg/100 g dm). This high retention can be attributed to the low oxygen partial pressure conditions and lower oxidase activity, which provide a favorable environment for the preservation of heat-sensitive components such as polyphenols, flavonoids, and Vc (Wang L. et al., 2023). In a study by Deng et al. (2017) on the effects of different drying methods on the Vc content of red pepper, it was found that the Vc content of the product obtained through PVD drying was higher compared to the other drying methods.
Figure 5. Vc content (A), TPC (B), and TFC (C) of sea buckthorn residue under different drying methods. (a, b, c, d, e) Different lowercase letters represent significant differences at a p ≤ 0.05.
The low-oxygen environment during the PVD drying process was suggested as the reason for this phenomenon. Similarly, the vacuum-low-temperature environment in VFD also helped to prevent Vc degradation. However, due to the longer drying time in VFD, the Vc content was lower than that of PVD. On the other hand, HAD exhibited the lowest Vc retention, attributed to the high air velocities promoting increased oxygen exposure to the sample, resulting in a lower Vc content in the final product. High temperatures and prolonged drying durations were identified as the main factors influencing the final Vc content of dried products (Jin et al., 2014; Roshanak et al., 2015). Wang et al. (2020) verified the degradation of Vc in drying studies on potatoes, which primarily depends on the drying time and the presence of oxygen.
Polyphenols play a significant role in determining the nutritional profile, flavor, and color attributes of dried products (Li et al., 2019). The TPC of fresh sea buckthorn pomace (Figure 5B) was measured at 25.85 ± 0.21 mg GAE/g dm. Upon drying, the TPC of sea buckthorn pomace ranged from 19.88 ± 0.20 mg GAE/g dm to 28.56 ± 0.24 mg GAE/g dm, exhibiting a notable variance in TPC under different drying conditions (p < 0.05). Research has indicated that the discrepancy in TPC is intricately linked to the method and duration of drying (Ahmed et al., 2021). Notably, the highest retention of total phenols was observed in samples subjected to PVD, possibly due to the diminished activity of oxidative and hydrolytic enzymes in vacuum and low-oxygen environments, thereby mitigating phenol loss. Conversely, the Maillard reaction occurring in other drying methods may lead to phenolic compound oxidation and subsequent phenolic compound production (Chen and Martynenko, 2018). HAD exhibited the lowest TPC content (19.88 ± 0.20 mg GAE/g dm). Various studies have demonstrated the sensitivity of polyphenolic compounds such as catechin and quercetin derivatives to temperature and oxygen (Jiang et al., 2017; Xu et al., 2020). Therefore, during the HAD process, phenolic compounds are susceptible to oxidation by oxygen and polyphenol oxidase, resulting in the generation of quinones and the formation of black-brown precipitates, ultimately leading to the degradation and reduction of color quality in fruits and vegetables (Li et al., 2019). Hence, HAD sea buckthorn pomace exhibits low color saturation. VFD ranks as the second most effective drying method in terms of total phenol retention, considered to minimize the loss of biologically active compounds owing to its low oxygen and low-temperature environment, which impedes the degradation of total phenols (An et al., 2022).
The TFC of sea buckthorn pomace under different drying conditions is shown in Figure 5C. The findings revealed increased TFC levels in dried sea buckthorn pomace compared to fresh counterparts, though without significant differences (p < 0.05). This elevation can be attributed to the effects of light, temperature, oxygen partial pressure, and water during the drying process, influencing the activity of various factors and leading to oxidation, aggregation, or decomposition of polyphenols, flavonoids, and other functional components within sea buckthorn pomace (Wang L. et al., 2023). Generally, antioxidants are higher in fresh plant material than in dried plant material due to degradation during drying. However, some studies have shown that compared to fresh plant material, freeze-dried tuberaria lignosa samples and green tea leaves dried by sunlight, microwave drying at different temperatures, and freeze-drying contain higher polyphenol and antioxidant activity (Pinela et al., 2012; Roshanak et al., 2015). In the present experiment, the higher flavonoid content in dried samples compared to fresh samples is that active enzymes in fresh samples lead to the degradation of these compounds, whereas the drying process preserves flavonoid by inhibiting enzymatic activity. Additionally, Kamiloglu et al. (2014) the increased TFC in IR-HAD samples is attributed to the radiation and penetration capability of infrared waves, which can penetrate cell interiors and break covalent bonds between polymers, facilitating the release and extraction of flavonoid and other substances. Moreover, PVD samples exhibited the highest TFC content, followed by VFD samples. This can be attributed to the low activity of oxidative enzymes under low temperatures and partial pressure of oxygen, which enables better retention of polyphenols and flavonoid under drying conditions (Lou et al., 2015). Prolonged contact with oxygen or high temperatures during drying could result in the loss of flavonoid (Wang P. et al., 2023). The difference in TFC between HAD and VFD samples was insignificant, with lower TFC observed after VFD treatment due to the prevention of heat-sensitive compound loss at lower temperatures. A similar outcome was observed in freeze-drying dragon fruits, where lower-temperature freeze-drying aided in flavonoid preservation (Dadhaneeya et al., 2023). The findings of Sim and Nyam (2019) suggested that freeze-drying reduced the loss of flavonoid antioxidants due to the low-temperature environment compared to other drying methods.
Correlation analysis between parameters aids in elucidating the underlying factors contributing to the transformation of appearance and internal components within the material. As depicted in Figure 3, the highest correlation (r = 0.98) is observed between ΔE, hue saturation C, and hue angle h°. The parameter b* demonstrates a notable influence on ΔE, exhibiting a positive correlation with the color difference. Samples with elevated h° and TFC may contain a higher concentration of anthocyanin compounds, contributing to brighter colors and increased values of h°. TFC exhibits robust antioxidant activity, capable of combating free radical damage, thereby safeguarding pigments from oxidation and preserving their natural coloration. Drying time (DT) was positively correlated with TFC, ΔE, and h° (r = 0.93; r = 0.96; r = 0.87), and significantly negatively correlated with Vc (r = −0.90). Overall, the correlations between the indicators further illustrate the shift in the intrinsic mechanism.
The electronic nose monitors volatile organic compounds (VOCs) released during the drying process in real time and accurately through sensor arrays and pattern recognition algorithms, which can provide fingerprint responses to specific volatiles (Zhu et al., 2022). By analyzing the odor characteristics, the electronic nose can quickly determine whether the food is fresh or not, whether it is contaminated by bacteria, etc., to ensure food safety, and the results can help to optimize the drying process and improve product quality (Zhang et al., 2019).
Figure 6A illustrates the radargram depicting the response of the electronic nose sensor to sea buckthorn pomace dried using various methods. The response value of the electronic nose sensor is represented as G/G0, where G denotes the headspace volatiles of the sample passing through the sensor resistance, and G0 represents the standard gas passing through the sensor resistance (Zhu et al., 2022). The sea buckthorn pomace treated with different drying methods predominantly elicited responses from sensors W1W (sensitive to inorganic sulfide), W2S (sensitive to ethanol and some aromatic compounds), and W5S (sensitive to nitrogen oxides), with varying response strengths. Notably, sensor W1W, sensitive to inorganic sulfide, exhibited the most pronounced distinguishing ability, followed by W2S and W5S. The response values of sea buckthorn pomace treated with HAD and VFD were higher than those of IR-HAD and PVD, indicating a more pronounced presence of aromatic components, inorganic sulfides, and volatile compounds such as nitrogen oxides in sea buckthorn pomace subjected to different drying methods.
Figure 6. Aroma signals of dried samples under different drying methods using an e-nose instrument: e-nose radar of sea buckthorn residue under different drying methods (A). PCA analyses of sea buckthorn residue under different drying methods and fresh sea buckthorn residue (B). PCA analyses of sea buckthorn residue between different drying methods (C).
Principal component analysis (PCA) was employed to discern odor differences between fresh and dried sea buckthorn residue. In Figure 6B, the total contribution of PC1 and PC2 amounted to 99.9%, surpassing the statistical boundaries of 85%. This suggests that PCA effectively transformed the e-nose mapping matrix, with multichannel sensors as variables, into a scoring matrix with PC1 and PC2 as variables (Sun et al., 2022). A significant disparity along the x-axis between fresh and dried sea buckthorn residue indicates that the drying process alters the taste of sea buckthorn residue. While dried sea buckthorn residue exhibits similar volatile compound characteristics, PVD demonstrates the closest resemblance to fresh samples (Figure 5B). Figure 6C shows the differences in odor between different drying methods, where the total contribution of PC1 and PC2 reached 91.0%, comprising PC1 (65.0%) and PC2 (26.0%). These results delineate the similarities and differences between various drying methods, highlighting their respective impacts on the odor of sea buckthorn residue.
Based on the aforementioned results, it is evident that HAD, IR-HAD, VFD, and PVD exert significant influences on the drying characteristics, color, microstructure, physicochemical properties, and odor of sea buckthorn pomace. The results showed that in terms of drying time, IR-HAD had the shortest drying time, and the fastest drying rate, followed by HAD and PVD, and VFD was the slowest. In terms of color and microstructure, HAD closely resembles the fresh sample, featuring a smooth surface and minimal skin wrinkling, followed by PVD, which also exhibits color proximity to the fresh sample and a porous microstructure with broken pores and distinct particle characteristics. In contrast, VFD results in the largest ΔE and damages the cell wall structure, leading to numerous surface pores larger than those observed with PVD. The ΔE of IR-HAD is smaller than that of VFD, and its microstructure exhibits irregular cracks with severe epidermal wrinkling. Furthermore, sea buckthorn pomace dried by PVD demonstrates the highest levels of Vc content, TPC content, and TFC content, while exhibiting the lowest response values to volatile components such as aromatic compounds, inorganic sulfides, and nitrogen oxides. Therefore, PVD emerges as the preferred drying method for sea buckthorn pomace due to its fast and stable drying speed, favorable appearance, and promising potential for use in production methods involving dried sea buckthorn pomace. However, further research is warranted to enhance our understanding of the regulation of the drying process and its impact on the quality characteristics of the product. Such investigations will contribute to optimizing drying techniques for sea buckthorn pomace, thereby facilitating informed decisions regarding production methods.
The raw data supporting the conclusions of this article will be made available by the authors, without undue reservation.
XZ: Conceptualization, Data curation, Formal analysis, Investigation, Methodology, Software, Validation, Visualization, Writing – original draft, Writing – review & editing. XQZ: Data curation, Formal analysis, Visualization, Writing – original draft. XL: Formal analysis, Methodology, Validation, Visualization, Writing – original draft. XJ: Data curation, Validation, Writing – original draft. QZ: Conceptualization, Funding acquisition, Project administration, Writing – review & editing. XY: Project administration, Supervision, Writing – review & editing.
The author(s) declare that financial support was received for the research, authorship, and/or publication of this article. This study was supported by Bingtuan Science and Technology Program (2023AB076 and 2023CB016), and the Bingtuan Core Technology Program (NYHXGG and 2023AA503).
We acknowledge the funding supported by the Bingtuan Science and Technology Program (2023AB076 and 2023CB016), and the Bingtuan Core Technology Program (NYHXGG and 2023AA503).
The authors declare that the research was conducted in the absence of any commercial or financial relationships that could be construed as a potential conflict of interest.
All claims expressed in this article are solely those of the authors and do not necessarily represent those of their affiliated organizations, or those of the publisher, the editors and the reviewers. Any product that may be evaluated in this article, or claim that may be made by its manufacturer, is not guaranteed or endorsed by the publisher.
Ahmed, I. A. M., Al Juhaimi, F., Özcan, M. M., Uslu, N., Babiker, E. E., Ghafoor, K., et al. (2021). A comparative study of bioactive compounds, antioxidant activity and phenolic compounds of melon (Cucumis melo L.) slices dehydrated by oven, microwave and infrared systems. J. Food Process. Preserv. 45. doi: 10.1111/jfpp.15605
An, N.-N., Sun, W.-H., Li, B.-Z., Wang, Y., Shang, N., Lv, W.-Q., et al. (2022). Effect of different drying techniques on drying kinetics, nutritional components, antioxidant capacity, physical properties and microstructure of edamame. Food Chem. 373:131412. doi: 10.1016/j.foodchem.2021.131412
Barzegar, M., Zare, D., and Stroshine, R. L. (2015). An integrated energy and quality approach to optimization of green peas drying in a hot air infrared-assisted vibratory bed dryer. J. Food Eng. 166, 302–315. doi: 10.1016/j.jfoodeng.2015.06.026
Bhat, R. (2021). “Sustainability challenges in the valorization of Agri-food wastes and by-products – ScienceDirect” in Valorization of agri-food wastes and by-products, ed. R. Bhat (Academic Press), 1–27.
Chen, Y., and Martynenko, A. (2018). Combination of hydrothermodynamic (HTD) processing and different drying methods for natural blueberry leather. Lwt 87, 470–477. doi: 10.1016/j.lwt.2017.09.030
Chen, T., Zhang, W., Liu, Y., Song, Y., Wu, L., Liu, C., et al. (2022). Water status and predictive models of moisture content during drying of soybean dregs based on LF-NMR. Molecules 27:14. doi: 10.3390/molecules27144421
Ciesarova, Z., Murkovic, M., Cejpek, K., Kreps, F., Tobolkova, B., Koplik, R., et al. (2020). Why is sea buckthorn (Hippophae rhamnoides L.) so exceptional? A review. Food Res. Int. 133:109170. doi: 10.1016/j.foodres.2020.109170
Dadhaneeya, H., Kesavan, R. K., Inbaraj, B. S., Sharma, M., Kamma, S., Nayak, P. K., et al. (2023). Impact of different drying methods on the phenolic composition, in vitro antioxidant activity, and quality attributes of dragon fruit slices and pulp. Food Secur. 12:1387. doi: 10.3390/foods12071387
Deng, L.-Z., Yang, X.-H., Mujumdar, A. S., Zhao, J.-H., Wang, D., Zhang, Q., et al. (2017). Red pepper (Capsicum annuum L.) drying: effects of different drying methods on drying kinetics, physicochemical properties, antioxidant capacity, and microstructure. Dry. Technol. 36, 893–907. doi: 10.1080/07373937.2017.1361439
Dhyani, D., Maikhuri, R. K., Misra, S., and Rao, K. S. (2010). Endorsing the declining indigenous ethnobotanical knowledge system of Seabuckthorn in central Himalaya, India. J. Ethnopharmacol. 127, 329–334. doi: 10.1016/j.jep.2009.10.037
Fathi, F., Ebrahimi, S. N., Matos, L. C., Oliveira, M. B. P. P., and Alves, R. C. (2022). Emerging drying techniques for food safety and quality: A review. Compr. Rev. Food Sci. Food Saf. 21, 1125–1160. doi: 10.1111/1541-4337.12898
Galanakis, C. M. (2021). Functionality of food components and emerging technologies. Food Secur. 10:128. doi: 10.3390/foods10010128
Gatlan, A. M., and Gutt, G. (2021). Sea buckthorn in plant based diets. An analytical approach of sea buckthorn fruits composition: nutritional value, applications, and health benefits. Int. J. Environ. Res. Public Health 18:8986. doi: 10.3390/ijerph18178986
Geng, Z., Zhu, L., Wang, J., Yu, X., Li, M., Yang, W., et al. (2023). Drying Sea buckthorn berries (Hippophae rhamnoides L.): effects of different drying methods on drying kinetics, physicochemical properties, and microstructure. Front. Nutr. 10:1106009. doi: 10.3389/fnut.2023.1106009
Golmohammadi, M., Assar, M., Rajabi-Hamaneh, M., and Hashemi, S. J. (2015). Energy efficiency investigation of intermittent paddy rice dryer: Modeling and experimental study. Food Bioprod. Process. 94, 275–283. doi: 10.1016/j.fbp.2014.03.004
Huang, D., Yang, P., Tang, X., Luo, L., and Sunden, B. (2021). Application of infrared radiation in the drying of food products. Trends Food Sci. Technol. 110, 765–777. doi: 10.1016/j.tifs.2021.02.039
Ji, M., Gong, X., Li, X., Wang, C., and Li, M. (2020). Advanced research on the antioxidant activity and mechanism of polyphenols from Hippophae species–A review. Molecules 25:917. doi: 10.3390/molecules25040917
Jiang, N., Liu, C., Li, D., Zhang, Z., Liu, C., Wang, D., et al. (2017). Evaluation of freeze drying combined with microwave vacuum drying for functional okra snacks: antioxidant properties, sensory quality, and energy consumption. LWT Food Sci. Technol. 82, 216–226. doi: 10.1016/j.lwt.2017.04.015
Jiang, D., Xiao, H., Zielinska, M., Zhu, G., Bai, T., and Zheng, Z. (2020). Effect of pulsed vacuum drying on drying kinetics and quality of roots of Panax notoginseng (Burk.) F. H. Chen (Araliaceae). Dry. Technol. 39, 2234–2251. doi: 10.1080/07373937.2020.1761827
Jin, X., Oliviero, T., van der Sman, R. G. M., Verkerk, R., Dekker, M., and van Boxtel, A. J. B. (2014). Impact of different drying trajectories on degradation of nutritional compounds in broccoli (Brassica oleracea var. italica). LWT Food Sci. Technol. 59, 189–195. doi: 10.1016/j.lwt.2014.05.031
Juhari, N., and Petersen, M. (2018). Physicochemical properties and oxidative storage stability of milled Roselle (Hibiscus sabdariffa L.) seeds. Molecules 23:385. doi: 10.3390/molecules23020385
Kamiloglu, S., Pasli, A. A., Ozcelik, B., and Capanoglu, E. (2014). Evaluating the in vitro bioaccessibility of phenolics and antioxidant activity during consumption of dried fruits with nuts. LWT Food Sci. Technol. 56, 284–289. doi: 10.1016/j.lwt.2013.11.040
Kyriakopoulou, K., Pappa, A., Krokida, M., Detsi, A., and Kefalas, P. (2013). Effects of drying and extraction methods on the quality and antioxidant activity of sea buckthorn (Hippophae rhamnoides) berries and leaves. Dry. Technol. 31, 1063–1076. doi: 10.1080/07373937.2013.773907
Li, Q., Shi, X., Zhao, Q., Cui, Y., Ouyang, J., and Xu, F. (2016). Effect of cooking methods on nutritional quality and volatile compounds of Chinese chestnut (Castanea mollissima Blume). Food Chem. 201, 80–86. doi: 10.1016/j.foodchem.2016.01.068
Li, X., Wu, X., Bi, J., Liu, X., Li, X., and Guo, C. (2019). Polyphenols accumulation effects on surface color variation in apple slices hot air drying process. Lwt 108, 421–428. doi: 10.1016/j.lwt.2019.03.098
Link, J. V., Tribuzi, G., and Laurindo, J. B. (2017). Conductive multi-flash drying of mango slices: vacuum pulse conditions on drying rate and product properties. J. Food Process. Preserv. 42:e13440. doi: 10.1111/jfpp.13440
Liu, Z.-L., Xie, L., Zielinska, M., Pan, Z., Deng, L.-Z., Zhang, J.-S., et al. (2022). Improvement of drying efficiency and quality attributes of blueberries using innovative far-infrared radiation heating assisted pulsed vacuum drying (FIR-PVD). Innovative Food Sci. Emerg. Technol. 77:102948. doi: 10.1016/j.ifset.2022.102948
Liu, Z.-L., Xie, L., Zielinska, M., Pan, Z., Wang, J., Deng, L.-Z., et al. (2021). Pulsed vacuum drying enhances drying of blueberry by altering micro-, ultrastructure and water status and distribution. Lwt 142:111013. doi: 10.1016/j.lwt.2021.111013
Liu, Y., Zhang, Y., Zhang, J., Fan, G., Tu, Y., Sun, S., et al. (2018). Rapid discrimination of sea buckthorn berries from different H. rhamnoides subspecies by multi-step IR spectroscopy coupled with multivariate data analysis. Infrared Phys. Technol. 89, 154–160. doi: 10.1016/j.infrared.2018.01.001
Lou, S.-N., Lai, Y.-C., Huang, J.-D., Ho, C.-T., Ferng, L.-H. A., and Chang, Y.-C. (2015). Drying effect on flavonoid composition and antioxidant activity of immature kumquat. Food Chem. 171, 356–363. doi: 10.1016/j.foodchem.2014.08.119
Luntraru, C. M., Apostol, L., Oprea, O. B., Neagu, M., Popescu, A. F., Tomescu, J. A., et al. (2022). Reclaim and valorization of sea buckthorn (Hippophae rhamnoides) by-product: antioxidant activity and chemical characterization. Food Secur. 11:462. doi: 10.3390/foods11030462
Ni, J., Ding, C., Zhang, Y., and Song, Z. (2020). Impact of different pretreatment methods on drying characteristics and microstructure of goji berry under electrohydrodynamic (EHD) drying process. Innovative Food Sci. Emerg. Technol. 61:102318. doi: 10.1016/j.ifset.2020.102318
Nour, V., Panaite, T. D., Corbu, A. R., Ropota, M., and Turcu, R. P. (2020). Nutritional and bioactive compounds in Dried Sea-buckthorn pomace. Erwerbs-obstbau 63, 91–98. doi: 10.1007/s10341-020-00539-1
Pinela, J., Barros, L., Dueñas, M., Carvalho, A. M., Santos-Buelga, C., and Ferreira, I. C. F. R. (2012). Antioxidant activity, ascorbic acid, phenolic compounds and sugars of wild and commercial Tuberaria lignosa samples: effects of drying and oral preparation methods. Food Chem. 135, 1028–1035. doi: 10.1016/j.foodchem.2012.05.038
Roshanak, S., Rahimmalek, M., and Goli, S. A. H. (2015). Evaluation of seven different drying treatments in respect to total flavonoid, phenolic, vitamin C content, chlorophyll, antioxidant activity and color of green tea (Camellia sinensis or C. assamica) leaves. J. Food Sci. Technol. 53, 721–729. doi: 10.1007/s13197-015-2030-x
Ruan, C. J., Rumpunen, K., and Nybom, H. (2013). Advances in improvement of quality and resistance in a multipurpose crop: sea buckthorn. Crit. Rev. Biotechnol. 33, 126–144. doi: 10.3109/07388551.2012.676024
Shen, Q., He, Z., Ding, Y., and Sun, L. (2023). Effect of different drying methods on the quality and non-volatile flavor components of Oudemansiella raphanipes. Food Secur. 12:676. doi: 10.3390/foods12030676
Sim, Y. Y., and Nyam, K. L. (2019). Effect of different drying methods on the physical properties and antioxidant activities of Hibiscus cannabinus leaves. J. Food Meas. Charact. 13, 1279–1286. doi: 10.1007/s11694-019-00043-5
Sun, Q., Yu, X., Zhang, L., Yagoub, A. E. G. A., Tang, Y., Wahia, H., et al. (2022). Effects of vacuum ultrasonic infiltration and combined drying on rehydration quality of ginger (Zingiber officinale roscoe). Ind. Crop. Prod. 187:115381. doi: 10.1016/j.indcrop.2022.115381
Tan, S., Xu, Y., Zhu, L., Geng, Z., Zhang, Q., and Yang, X. (2022). Hot air drying of Seabuckthorn (Hippophae rhamnoides L.) berries: effects of different Pretreatment methods on drying characteristics and quality attributes. Food Secur. 11:675. doi: 10.3390/foods11223675
Torres, C. D., Díaz-Maroto, M. C., Hermosín-Gutiérrez, I., and Pérez-Coello, M. S. (2010). Effect of freeze-drying and oven-drying on volatiles and phenolics composition of grape skin. Anal. Chim. Acta 660:177. doi: 10.1016/j.aca.2009.10.005
Wang, P., Li, Y., Yu, R., Huang, D., Chen, S., and Zhu, S. (2023). Effects of different drying methods on the selenium bioaccessibility and antioxidant activity of Cardamine violifolia. Food Secur. 12:758. doi: 10.3390/foods12040758
Wang, H., Liu, Z.-L., Vidyarthi, S. K., Wang, Q.-H., Gao, L., Li, B.-R., et al. (2020). Effects of different drying methods on drying kinetics, physicochemical properties, microstructure, and energy consumption of potato (Solanum tuberosum L.) cubes. Dry. Technol. 39, 418–431. doi: 10.1080/07373937.2020.1818254
Wang, L., Wen, H., Yang, N., and Li, H. (2023). Effect of vacuum freeze drying and hot air drying on dried mulberry fruit quality. PLoS One 18:e0283303. doi: 10.1371/journal.pone.0283303
Wang, J., Yang, X.-H., Mujumdar, A. S., Wang, D., Zhao, J.-H., Fang, X.-M., et al. (2017). Effects of various blanching methods on weight loss, enzymes inactivation, phytochemical contents, antioxidant capacity, ultrastructure and drying kinetics of red bell pepper (Capsicum annuum L.). Lwt 77, 337–347. doi: 10.1016/j.lwt.2016.11.070
Xu, X., Zhang, L., Feng, Y., Yagoub, A. E. G. A., Sun, Y., Ma, H., et al. (2020). Vacuum pulsation drying of okra (Abelmoschus esculentus L. Moench): better retention of the quality characteristics by flat sweep frequency and pulsed ultrasound pretreatment. Food Chem. 326:127026. doi: 10.1016/j.foodchem.2020.127026
Yang, R.-L., Li, Q., and Hu, Q.-P. (2020). Physicochemical properties, microstructures, nutritional components, and free amino acids of Pleurotus eryngii as affected by different drying methods. Sci. Rep. 10:121. doi: 10.1038/s41598-019-56901-1
Yao, L., Fan, L., and Duan, Z. (2020). Effect of different pretreatments followed by hot-air and far-infrared drying on the bioactive compounds, physicochemical property and microstructure of mango slices. Food Chem. 305:125477. doi: 10.1016/j.foodchem.2019.125477
Yu, D. X., Guo, S., Wang, J. M., Yan, H., Zhang, Z. Y., Yang, J., et al. (2022). Comparison of different drying methods on the volatile components of ginger (Zingiber officinale roscoe) by HS-GC-MS coupled with fast GC E-nose. Food Secur. 11:611. doi: 10.3390/foods11111611
Yue, Y., Zhang, Q., Wan, F., Ma, G., Zang, Z., Xu, Y., et al. (2023). Effects of different drying methods on the drying characteristics and quality of Codonopsis pilosulae slices. Food Secur. 12:323. doi: 10.3390/foods12061323
Zhang, J., Cao, J., Pei, Z., Wei, P., Xiang, D., Cao, X., et al. (2019). Volatile flavour components and the mechanisms underlying their production in golden pompano (Trachinotus blochii) fillets subjected to different drying methods: A comparative study using an electronic nose, an electronic tongue and SDE-GC-MS. Food Res. Int. 123, 217–225. doi: 10.1016/j.foodres.2019.04.069
Zhang, X., Zhang, G., Miao, Y., Sun, X., and Huang, L. (2023). Effects of different drying methods on physico-chemical properties, bioactive and taste substances of Cynomorium songaricum. Lwt 185:115159. doi: 10.1016/j.lwt.2023.115159
Zheng, Z.-A., Wang, S.-Y., Wang, H., Xiao, H., Liu, Z.-L., Pan, Y.-H., et al. (2023). Comparative study on the influence of various drying techniques on drying characteristics and physicochemical quality of garlic slices. Food Secur. 12:314. doi: 10.3390/foods12061314
Zhou, Y.-H., Pei, Y.-P., Sutar, P. P., Liu, D.-H., Deng, L.-Z., Duan, X., et al. (2022). Pulsed vacuum drying of banana: effects of ripeness on drying kinetics and physicochemical properties and related mechanism. Lwt 161:113362. doi: 10.1016/j.lwt.2022.113362
Keywords: sea buckthorn pomace, drying method, drying characteristics, quality characteristics, microstructure
Citation: Zhou X, Zhang X, Liu X, Ji X, Zhang Q and Yang X (2024) Effects of different drying techniques on sea buckthorn pomace: comprehensive assessment of drying characteristics, physicochemical properties, and odor. Front. Sustain. Food Syst. 8:1434121. doi: 10.3389/fsufs.2024.1434121
Received: 17 May 2024; Accepted: 04 July 2024;
Published: 15 August 2024.
Edited by:
Aprajeeta Jha, University of Maryland, College Park, United StatesReviewed by:
Sayantani Dutta, Universidad de Salamanca, SpainCopyright © 2024 Zhou, Zhang, Liu, Ji, Zhang and Yang. This is an open-access article distributed under the terms of the Creative Commons Attribution License (CC BY). The use, distribution or reproduction in other forums is permitted, provided the original author(s) and the copyright owner(s) are credited and that the original publication in this journal is cited, in accordance with accepted academic practice. No use, distribution or reproduction is permitted which does not comply with these terms.
*Correspondence: Xuhai Yang, eXhoXzUxM0BzaHp1LmVkdS5jbg==
Disclaimer: All claims expressed in this article are solely those of the authors and do not necessarily represent those of their affiliated organizations, or those of the publisher, the editors and the reviewers. Any product that may be evaluated in this article or claim that may be made by its manufacturer is not guaranteed or endorsed by the publisher.
Research integrity at Frontiers
Learn more about the work of our research integrity team to safeguard the quality of each article we publish.