- 1Department of Agronomy, Tamil Nadu Agricultural University, Coimbatore, India
- 2Department of Soil Science & Agricultural Chemistry, Tamil Nadu Agricultural University, Coimbatore, India
- 3Department of Entomology, Tamil Nadu Agricultural University, Coimbatore, India
- 4Department of Crop Physiology, Tamil Nadu Agricultural University, Coimbatore, India
Seaweed is an increasingly recognized source of secondary metabolites with a significant role as a plant biostimulant. With the rising popularity of organic farming, there is a pressing need to investigate the utilization of organic solvents derived from natural sources for seaweed extraction. Understanding the composition of these extracts is crucial for elucidating their role as biostimulants in plant growth. Hence, the present study aimed to explore the phytochemical composition and untargeted metabolomics of four extracts of Caulerpa racemosa (SW), prepared organically using vinegar, fermented buttermilk, and cow urine, in comparison with the conventionally used solvent, water. Approximately 95 volatile metabolites that belong to 22 chemical classes were identified through GC–MS analysis. The major classes detected include benzene and its substituted derivatives (24%), fatty acyls (17%), phenols (12%), benzofurans (8%), organo-oxygen compounds (6%), steroids and steroid derivatives (5%), and indole and its derivatives (4%). A significant shift in the composition of volatile metabolites was observed among the organic extracts as determined through a combination of various statistical analyses. A principal component analysis (PCA) highlighted seven principal components (PCs), which accounted for 99.9% of the total variance. Hierarchical cluster analysis delineated two distinct clusters, which signify a clear differentiation among the organic extracts. Furthermore, partial least squares discriminant analysis (PLS-DA) highlighted 49 volatile metabolites of significant importance, evident from variable importance in projection (VIP) scores of more than 1. These results underscore the intricate and distinct metabolomes inherent in Caulerpa racemosa organic extracts, hinting at their potential as biostimulants for plant growth. This study establishes a foundation for deeper investigations into seaweed extraction utilizing easily accessible organic solvents, presenting potential benefits for farmers.
1 Introduction
Organic farming is gaining traction globally as a safer and more environmentally cautious alternative to conventional farming; however, due to the nature of organic food production, it naturally has a lesser yield and requires significantly more inputs to achieve the same results as conventional farming. In lieu of this, the application of biostimulants has been confirmed to be a part of the answer to increasing yields in organic farming. Among the various types of plant biostimulants, seaweed extracts are gaining praise for their naturally high content of growth-endorsing substances and nutrients, as well as their abundance in coastal areas. Studies with different species of seaweed along with various extraction methods and their effectiveness on application against different crops have reported promising results (Khan et al., 2009; Jacomassi et al., 2022).
Examination of relevant literature exposes a marked lacuna in the areas where seaweed nutrients and bioactive compounds are extracted using organic solvents and physical processes. The advent of science in metabolomics has allowed for the study of immediate biological information generated by genomic and transcriptomic activity. There are two types of metabolomic analysis, viz., target metabolomics and untargeted metabolomics (Gorrochategui et al., 2016). The present investigation is focused on the phytochemicals and untargeted metabolomics, which will provide a list of present volatile metabolites and the data acquired can serve to prove our hypothesis and may be further utilized to assess intensive analysis of specific volatile metabolites or pathways of interest (Lee et al., 2017). Triple–quadrupole mass spectrometry-based gas chromatography (GC–MS/MS) was performed as GC/MS is the most standardized method in metabolomics (Fiehn, 2016).
The extraction process employed for seaweed determines the composition of the extracts. There are various studies exploring seaweed extraction methods, among which water is the most popular solvent followed by other common organic solvents, such as methanol, ethanol, and acetone (Godlewska et al., 2016). Extracting seaweed using microwave, ultrasound, and application of physical pressure has also been explored (Han et al., 2010). However, these processes may not always be applicable in field conditions. In present-day agriculture, the development of cost-effective biostimulant extraction processes suitable for organic farming is highly essential. Hence, the current study intends to extract seaweed utilizing vinegar, fermented buttermilk, and cow urine and assess their volatile metabolites to understand their contribution to the growth and development of crops.
2 Materials and methods
2.1 Collection and processing of seaweed
The seaweed species Caulerpa racemosa (SW) was collected from the Gulf of Mannar (Mandapam, 9.287′N, 79.178′E, India) in March 2023. Collected seaweed samples were cleaned with seawater and brought to Nammazhvar Organic Farming Research Centre, Tamil Nadu Agricultural University. Initially, tap water was used for removing dirt and debris from the seaweeds followed by a thorough rinse with deionized water. The washed seaweeds were dried at 60 ± 5°C in a hot air oven. The dried seaweed was powdered using Willy Mill and kept in a sealed container for phytochemical and GC–MS analysis.
2.2 Extraction of seaweeds with organic extracts
Locally available solvents such as water, vinegar (V), fermented buttermilk (FB), and cow urine (CU) were used for the extraction of the seaweeds. Water (WE) and vinegar (VE) extracts were prepared by adding 150 mL of solvents in 50 g (1:3 ratio) of powdered seaweed. Then, it was left for 24 h and extracted using muslin cloth. The extracts were centrifuged and the supernatant was collected by decanting; the end product was considered as a 100% seaweed extract.
To create fermented buttermilk, fresh milk from the Dairy Unit, TNAU, was allowed to ferment for 24 h with commercial yeast (30 g L−1) to make curd. At room temperature, the curd was gently churned to obtain a smooth consistency free of lumps before being left to further ferment for 48 h. It was then diluted with water in a 1:5 ratio and mixed well to make the buttermilk. Fermented buttermilk extract (FBE) was prepared by adding 200 mL of fermented buttermilk to 50 g of powdered seaweed (1:4 ratio). After this, the same procedure was followed as in water and vinegar extraction. Cow urine extraction (CUE) was done for 20 days by adding 300 mL of fresh cow urine in 50 g (1:6 ratio) of powdered seaweed. The liquid part was collected using muslin cloth and centrifuged. The supernatant was collected and used for characterization and metabolomics analysis.
2.3 Preliminary phytochemical analysis
In the organic extracts of SW, phytochemical composition such as saponins, terpenoids, steroids, phytosterols, tannins, flavonoids, phenols, alkaloids, phenolic flavonoids, and glycosides was analyzed according to the methods given by Sadasivam (1996) and Palaniyappan et al. (2023). The detailed procedure for qualitative analysis of phytochemicals is given in Table 1.
2.4 Quantitative analysis of flavonoids, phenols, and tannins
The total flavonoid content of the organic extracts was determined using the aluminum chloride technique (Hao et al., 2018). In a 0.5 mL sample, 2 mL of deionized water and 0.15 mL of NaNO2 (5%) were added and allowed to rest for 5 min. After 5 min, 0.15 mL of 10% AlCl3 was added, followed by 1 mL of 1 M NaOH. The total volume was made up to 5 mL with deionized water and incubated for 15 min. At 510 nm, the orange-yellowish color development was measured. The content of total flavonoids was estimated using the catechin standard curve and represented as mg catechin per gram (mg catechin/g) of extract.
The total phenol content was determined using the Folin–Ciocalteu (FC) technique, with adjustments suggested by Salar et al. (2012). The FC reagent (0.5 mL) was mixed with 0.1 mL of the sample. Then, the mixture was incubated at 37°C for 5 min. The volume was then raised to 10 mL by adding 1.5 mL of Na2CO3 (7.5%) and deionized water. The absorbance at 765 nm was measured with a UV–vis spectrophotometer and compared to a blank. Gallic acid was used as standard, and gallic acid equivalents per gram (mg GAE/g) were used to express the results.
The total tannin content of various organic extracts of SW was measured using the methodology described by Amorim et al. (2008). In 0.1 mL of sample, 7.5 mL of deionized water was added, followed by 0.5 mL of FC reagent and 1 mL of Na2CO3 (35%). The contents were thoroughly mixed and left as such at 25°C for 30 min and absorbance was measured at 725 nm. The tannic acid calibration curve was used to determine the total tannic acid content. The results were expressed as milligrams of tannic acid equivalents per gram (mg TAE/g).
2.5 Extraction of volatile metabolites and gas chromatography–mass spectrophotometry (GC–MS) analysis
The volatile metabolites in raw seaweed and organic solvents were extracted using methanol as a solvent. The raw seaweed powder was extracted using a 1:10 ratio of sample: methanol, organic solvents, and extracts were extracted at a 1:3 ratio. The raw seaweed:solvent suspension was shaken in an orbital shaker for 72 h and filtered through a Whatman No.1 filter paper. The organic extracts and solvents were partitioned for volatile metabolites by discarding the aqueous layer and collecting the organic layer using the separating funnel, and this partitioning was repeated 3–4 times. The volume of the pooled organic layer was reduced using a rotary evaporator, and a known volume of methanol was added and filtered through a membrane filter (0.2 μm) before injecting into the GC–MS machine for analysis.
The volatile metabolites were analyzed using GC–MS (Agilent and Model-GC7890A/MS5975C), and compound separation was done using an Agilent DB5MS column with a length of 30 m (0.25 μ internal diameter and 0.25 μ thick). The oven temperature was set at 90°C for 1 min and raised to 280°C for 23 min at a rate of 8°C per min. The gas flow rate was maintained at 1.2 mL per minute using helium gas as a carrier. The injector temperature was sustained at 250°C. In split mode, the sample was injected at the rate of 1 μL for separation by operating the mass spectrum at 70 ev.
2.6 Identification of compounds
To express the peak area normalization of the organic extracts, the area percentage was employed. Retention indices and mass spectra patterns were compared to the National Institute of Standards Technology (NIST) library for interpretation. The molecular weight, molecular formula, compound nature, and biological activity of volatile metabolites were ascertained.
2.7 Micro-plot experiment
A micro-plot study was conducted on black gram var. Vamban 11 with eight treatments at the Wetland Farm, Tamil Nadu Agricultural University, India (11.003′N latitude, 76.92′E longitude) between the 3rd week of July and 1st week of October 2023. The micro-plot experiment was conducted with a randomized block layout with three replications. Treatment includes T1—control (water only), T2—vinegar only, T3—fermented buttermilk only, T4—cow urine only, T5—water extract, T6—vinegar extract, T7—fermented buttermilk extract, T8—cow urine extracts. A micro-plot of 4 m2 was considered as one micro-plot, while plant spacing was maintained at 30 × 10 cm. Treatments were applied at 30 DAS (vegetative stage) and 50 DAS (pod development stage). During the experiment, hand weeding was done twice prior to each treatment and three irrigations were given. All the observations were taken at the time of harvest.
2.8 Statistical analysis
All the experiments were conducted in three replicates. Metabolomic analysis of volatile compounds was performed using the online program MetaboAnalyst 6.0.1 The identified volatile metabolites were exposed to univariate and multivariate analysis using normalized mean data. The data were analyzed using a one-way ANOVA, Student’s t-test (p ≤ 0.05), and a post-hoc Tukey’s honestly significant difference (HSD) test, with an adjusted p-value of 0.05. The inherent variation in the data matrix was initially investigated using PCA, which is an unsupervised method of pattern identification. Following that, PLS-DA, a supervised classification technique, was used to eliminate the dataset’s discriminating molecular features.
Abundance value was used to classify data, and most characteristic features were selected for further clustering by obtaining the greatest possible discriminating features. To highlight the variation among different volatile metabolites, we performed a hierarchical clustering analysis (HCA) using Euclidean distance and Ward’s method. A heatmap representation was also created. This was done to compare and visualize the shared characteristics between the sample replicates.
3 Results
3.1 Preliminary phytochemical analysis
Seaweeds contain different phytochemicals, which can contribute to biological activity and health benefits. Phytochemicals such as saponins, terpenoids, steroids, phytosterols, tannins, flavonoids, phenols, alkaloids, phenolic flavonoids, and glycosides were analyzed on SW and its organic extracts (Table 2). Saponins, tannins, flavonoids, phenols, and terpenoids were present in all the organic extracts. Steroids, phytosterols, glycosides, and phenolic flavonoids were not present in any of the extracts.
3.2 Quantitative analysis of flavonoids, phenols, and tannins
Flavonoid is an important dietary supplement and has good antioxidant properties (Palaniyappan et al., 2023). Research evidence showed the presence of different flavonoids in Caulerpa spp. of seaweed (Tanna et al., 2018). Results of the present investigation showed that SW flavonoid content was high (41 ± 4.24 mg catechin/g). Among the organic extracts, the FBE had the highest flavonoid (19.50 ± 0.71 mg catechin/g) content and the lowest flavonoid content in CUE (12.8 ± 2.82 mg catechin/g). Among the organic solvents, vinegar showed negative results in qualitative test; thus, quantification was not performed. However, cow urine and fermented buttermilk contain 6.14 ± 0.57 and 10.32 ± 1.68 mg catechin/g, respectively. The levels of flavonoid content are shown in Figure 1 and Supplementary Table S1.
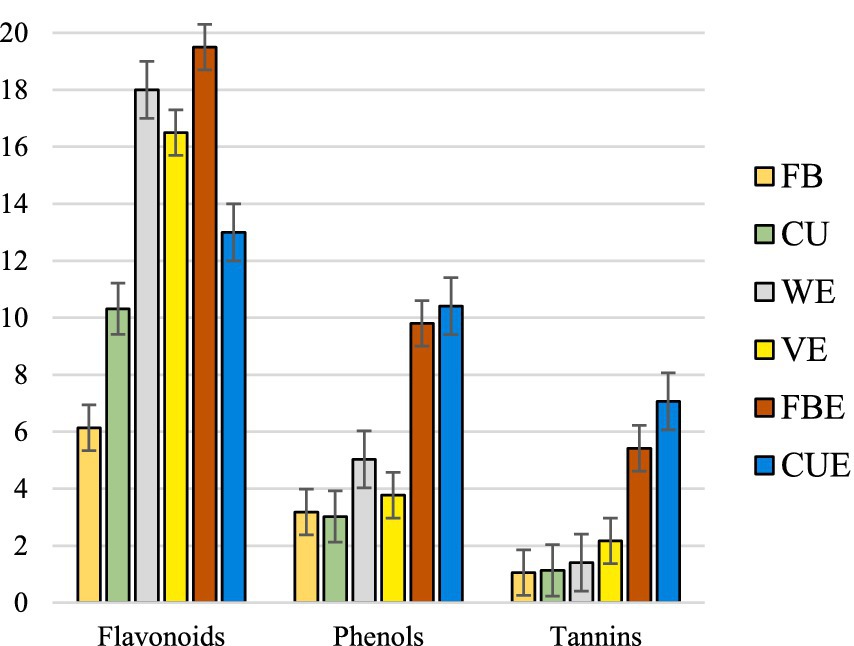
Figure 1. Quantitative analysis of total flavonoids (mg catechin/g), phenols (mg GAE/g), and tannins (mg TAE/g) content in organic extracts of SW.
The presence of phenols was reported to boost a plant defense system against ultraviolet radiation, pests and diseases, and other predators (Dai and Mumper, 2010). Generally, phenolic compounds generated through seed, root, or plant deposits may prevent pests and diseases that infect plant roots (Ndakidemi and Dakora, 2003). Phenolic compounds that accumulate in the soil determine the plant’s defense system against soil-borne pathogens, nematodes, and phytophilous insects (Dakora and Phillips, 1996). However, the structural diversity of phenolic compounds determines their activity level (Mandal et al., 2010). The results of the phenol content in the present study are shown in Figure 1 and Supplementary Table S1. Organic solvents such as FB and CU contain low phenols of 3.18 ± 0.14 and 3.03 ± 0.19 mg GAE/g, respectively. Organic extracts such as CUE and FBE exhibited higher phenol content with 10.41 ± 0.28 and 9.81 ± 0.47 mg GAE/g, respectively. Vinegar extracts of C. racemosa showed the lowest phenol content with 3.77 ± 0.12 mg GAE/g.
Tannins play a crucial role in a plant’s defense system (Petchidurai et al., 2019). The total tannin content of the present study indicated that it was high in CUE and FBE. The total tannin content of the organic extracts is shown in Figure 1 and Supplementary Table S1.
3.3 GC–MS analysis
Chromatographic analysis on the Caulerpa racemosa (SW), organic solvents used, and organic extracts revealed 95 compounds, which were classified into 22 chemical classes using the NIST (National Institute of Standards and Technology) library and HMDB (Human Metabolome Database). Details of the compounds and GC–MS chromatogram are given in Table 3 and Supplementary Figures S1–S8. The maximum number of compounds was detected in FB with 27 compounds and the lowest in vinegar with only 1 compound. Detected volatile metabolites such as octadecanoic acid and methyl ester exhibit antioxidant, anti-inflammatory, and antibacterial properties (Kumar et al., 2011; Othman et al., 2015). Hexadecanoic acid and methyl ester also exhibit anti-inflammatory, antibacterial, antioxidant, antifungal, anticandidal, nematicide, and pesticide properties (Chandrasekaran et al., 2011; Nabi et al., 2022).
To completely comprehend the variations in the chemical configuration of detected volatile metabolites in SW organic extracts, a non-targeted metabolomics analysis was performed. Major classes were benzene and substituted derivatives, fatty acyls, organo-oxygen-organooxygen compounds, and hydrocarbons with 31, 24, 14, and 18 compounds, respectively. Cumulative area percentages of the compound classes are given in Figure 2. Details of the compound under each class are given in Supplementary Table S2.
Euler Venn diagram in Figure 3 represents the number of compounds that were commonly present in the organic extracts of SW. Twenty-two compounds were found only in the SW, 1 in V, 11 in FB, and 8 in CU. Approximately 10 compounds each were identified in WE and VE, 9 compounds in FBE, and 3 compounds in CUE. SW, WE, VE, and FBE shared 3 compounds, and FB, WE, VE and FBE shared 1 compounds. Details of the common compounds found in the organic extracts are given in Supplementary Table S3.
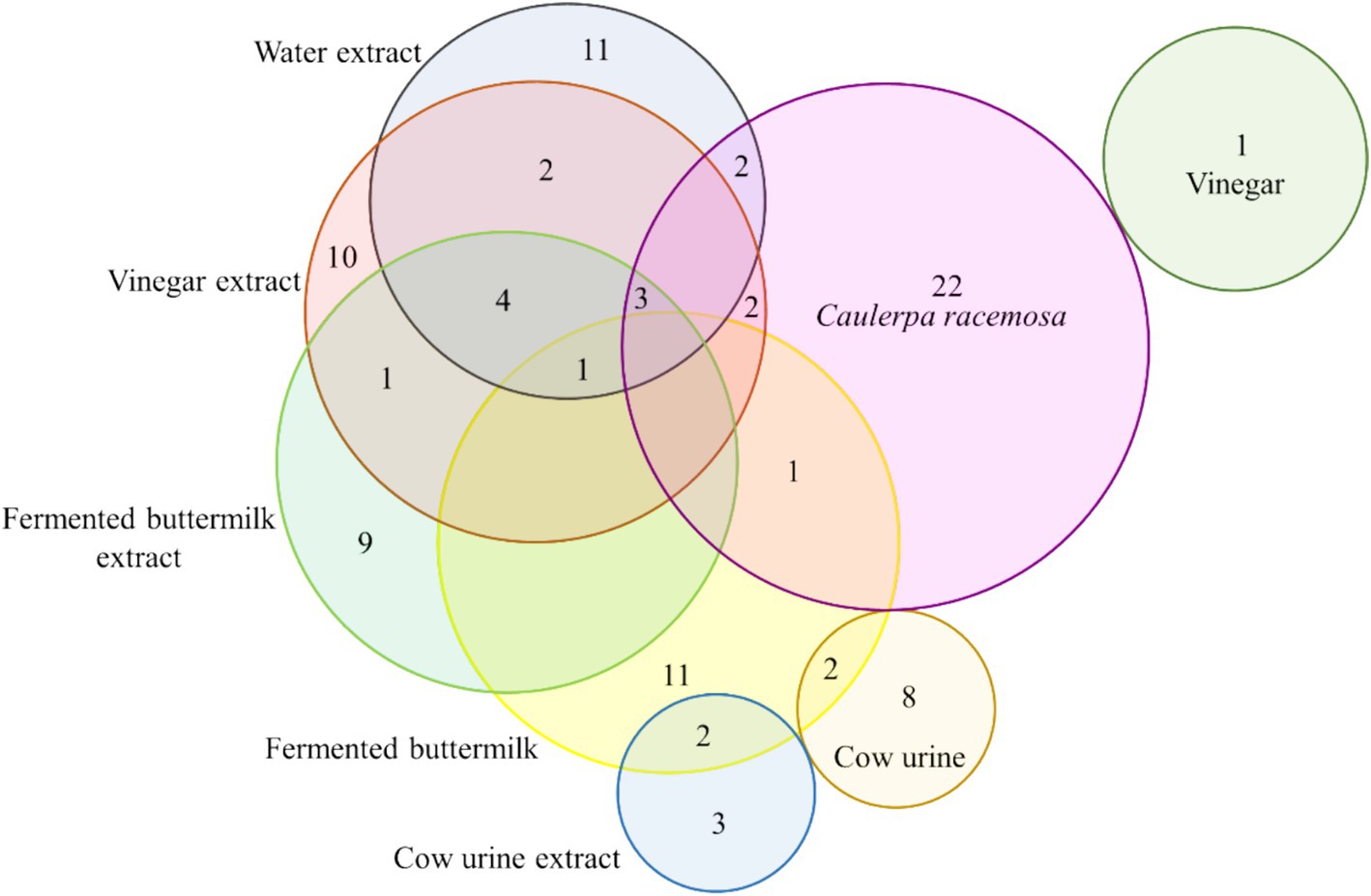
Figure 3. Euler Venn diagram showing common volatile metabolites found in SW, organic solvents, and extracts.
3.4 Univariate analysis (ANOVA)
The distribution of detected volatile metabolites in the organic extracts of SW was determined using a one-way ANOVA. The univariate analyses provide an initial assessment of features that may be significant in distinguishing the conditions under investigation. Out of 95 detected volatile metabolites, 70 showed significant differences at a p-value of 0.05 across all organic extracts (Figure 4). The change in color from yellow to orange represents an increase in the -log10(p) value, while the increase in the size of the bubble signifies an increase in the p-log value. Significant volatile metabolites and their p-values are given in Supplementary Table S4.
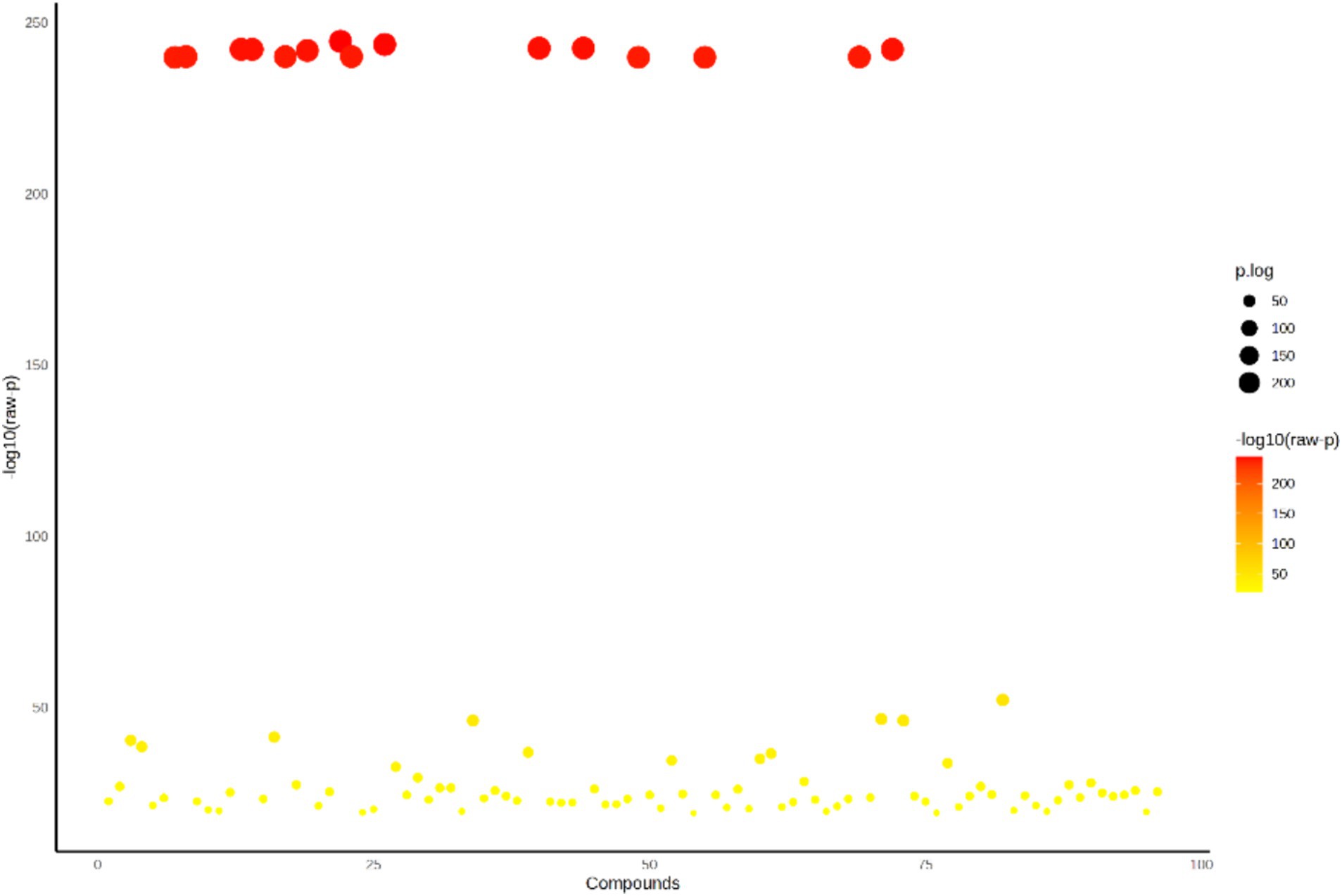
Figure 4. ANOVA plot showing significant volatile metabolites (p < 0.05) of the organic extracts of SW.
3.5 Principal component analysis (PCA)
To decrease the magnitude of the data and improve interpretability, the processed organic extracts of the SW volatile metabolites list were evaluated using chemometric statistical methods such as principal component analysis (PCA), partial least squares discriminant analysis (PLS-DA), and hierarchical clustering analysis (HCA). PCA is an unsubstantiated analysis for determining the guidelines that explain best the variation in a dataset. All 95 detected volatile metabolites were exposed to PCA and condensed to seven principal components (PCs) using normalized data to indicate volatile metabolite variances. PCs with more than one Eigenvalue were included in the study. Figure 5 depicts the score plots for PCA. According to PCA, the composition of volatile metabolites of the organic extracts of SW considerably varies statistically from each other. PCA score plot revealed that only SW was on the positive side for PC1, indicating a higher amount of volatile metabolites. Solvents such as V, FB, and CU were on the negative side for PC1; however, in PC2 FB was on the positive side. Moreover, FB and FBE were close to each other indicating similarities in volatile metabolites composition. Both CUE and VE were on the negative side of the principal component.
Selected PCs explained 99.9% of the variation as given in the scree plot. The first PC (PC1) explained 27% of the variance and was correlated with some fatty acyls and hydrocarbons. Similarly, PC2 explained 20.4% in addition to PC1 which was correlated with compounds found in SW. The PC3 explained 16.5% that was correlated with volatile metabolites of organosilicon compounds, some fatty acyls, and indoles and derivatives. PC4 explained 13.5% which was related to the benzene and substituted derivatives. PC5 explained 12% in addition to the previous principal components. PC6 and PC7 explained 7.6 and 2.8%, respectively. Details of the volatile metabolites score, loading plot, biplot, and scree plot of PCA are given in Supplementary Table S5 and Supplementary Figure S9.
3.6 Partial least squares–discriminant analysis (PLS-DA)
Subsequent to PCA, PLS-DA was performed to highlight dissimilarities among the organic extracts of SW with respect to volatile metabolite composition. The analysis showed a clear separation of different organic extracts based on the detected volatile metabolites. Additionally, the sturdiness and enactment of the model were assessed with cross-validation and transformation tests (Supplementary Table S7). Among the 95 volatile metabolites, there were 49 volatile metabolites that have VIP score higher than 1, such as hexadecanoic acid, methyl ester, 1H-indole, 1-methyl-2-phenyl-, methyltris(trimethylsiloxy)silane, 2,4,6-cycloheptatrien-1-one, 3,5-bis-trimethylsilyl-, benzamide, silane, 1,4-phenylenebis[trimethyl, p-cresol, 1,2-bis(trimethylsilyl)benzene, 1H-indole, 2,3-dihydro-4-methyl-, 1H-indole-3-ethanol, 2(3H)-furanone, dihydro-3-hydroxy-4,4-dimethyl-, benzeneacetamide, indole, 2,6-dimethylphenyl isocyanate, 2-methoxy-4-vinylphenol, benzoic acid, 2-amino-, methyl ester, octacosane, tricosane, 2-methyl-, tetracosane, 3-ethyl-, 2-tetradecene, (E)-, 1,3-dimethylcyclopentanol, 3-octadecene, (E)-, heptadecane, 2,6,10,15-tetramethyl, heptadecanoic acid, 16-methyl-, methyl ester, 2-heptadecanone, phenol, 2,6-bis(1,1-dimethylethyl), trimethyl [4-(2-methyl-4-oxo-2-pentyl) phenoxy] silane, cyclododecane, 7,9-di-tert-butyl-1-oxaspiro(4,5) deca-6,9-diene-2,8-dione, cyclotrisiloxane, hexamethyl-, benzeneacetic acid, eicosane, 9-cyclohexyl, benzoic acid, silicic acid, diethyl bis(trimethylsilyl) ester, hexatriacontane, octadecanoic acid, methyl ester, 1-methyl-3-phenylindole, 1,2-benzenediol, 3,5-bis(1,1-dimethylethyl)-, formamide, (2-acetylphenyl)-, 1-tridecene, 4-methyl-2-trimethylsilyloxy-acetophenone, undecane, eicosane, benzo[h]quinoline, 2,4-dimethyl-, benzyl nitrile, benzoic acid, methyl ester, phenol, 2,4-bis(1,1-dimethylethyl), 1-hexadecanethiol and N-methyl-1-adamantaneacetamide (Figure 6). WE, VE, and CU exhibit the highest number of volatile metabolites with high VIP scores, whereas in SW and V, almost all the volatile metabolites showed low VIP scores.
3.7 Hierarchal clustering and heatmap analysis
A hierarchical clustering analysis model was constructed using the volatile metabolites dataset to evaluate the resemblances in detecting variation within the group of organic volatile metabolites features. Following the Euclidean distance matrix for each set of comparisons quantitative values, the differential volatile metabolites were grouped and displayed as thermograms using a complete-linkage method.
The heatmap (Figure 7) depicts the distribution of the top 45 volatile metabolites among the samples. The abundance of volatile metabolites was represented in descending order using a color gradation from blue to red. A high relative abundance of volatile metabolites comprised more than half of the highlighted volatile metabolites. Cluster 1 contained SW, V, WE, VE, FBE, and CUE, where variation in volatile metabolite composition is relatively higher than in cluster 2. Cluster 2 contained FB and CU and were more similar to one another (Figure 7).
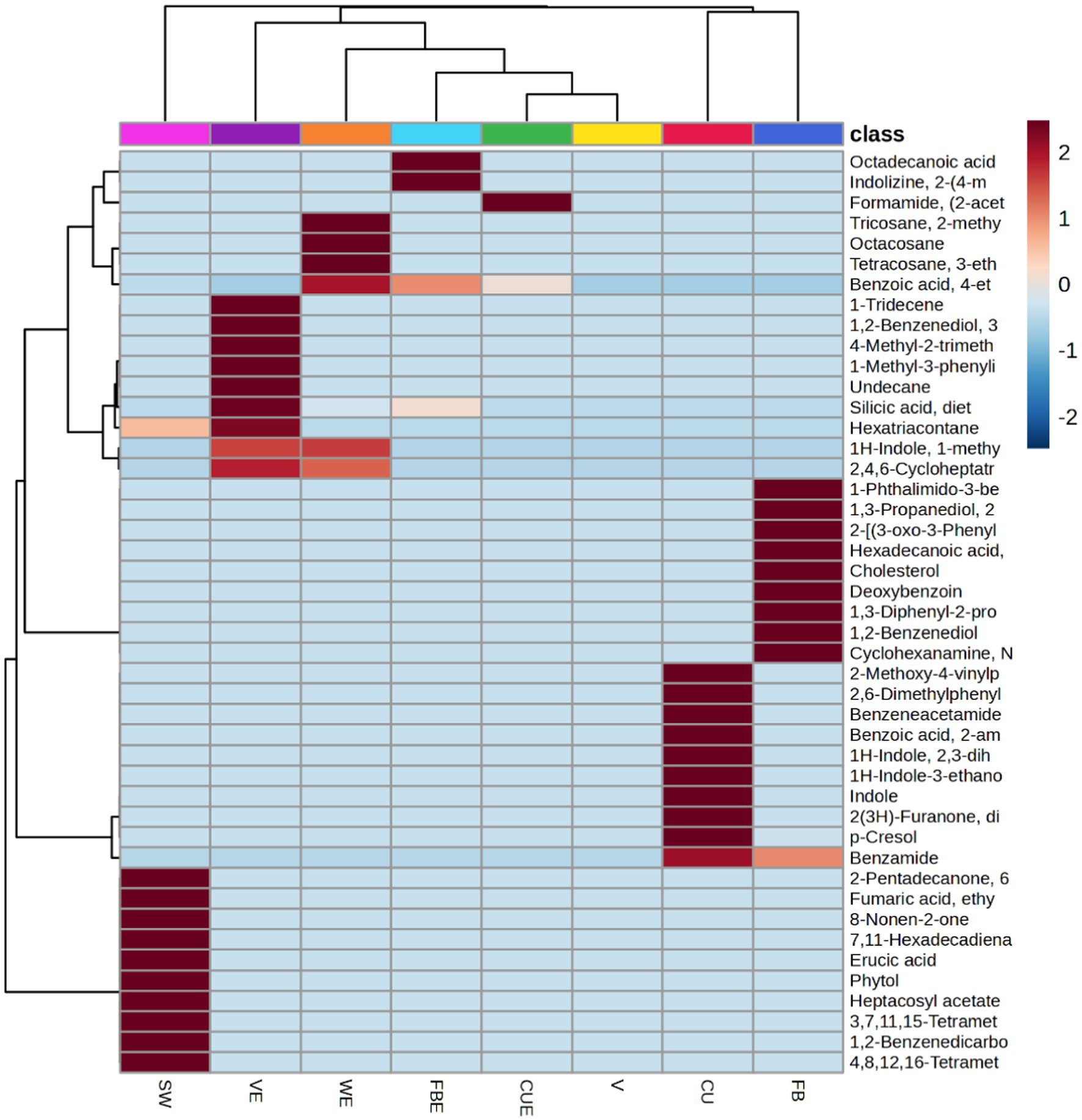
Figure 7. Heatmap showing changes top 45 volatile metabolites in organic extracts of SW. Each column represents one organic extract and the volatile metabolites were presented in rows.
3.8 Pathway analysis and pathway impacts
A pathway topology analysis was performed to determine the significant metabolic pathways in SW, and the results were presented as a bubble chart depicting the pathways of different volatile metabolites from the organic extracts (Figure 8 and Supplementary Table S6).
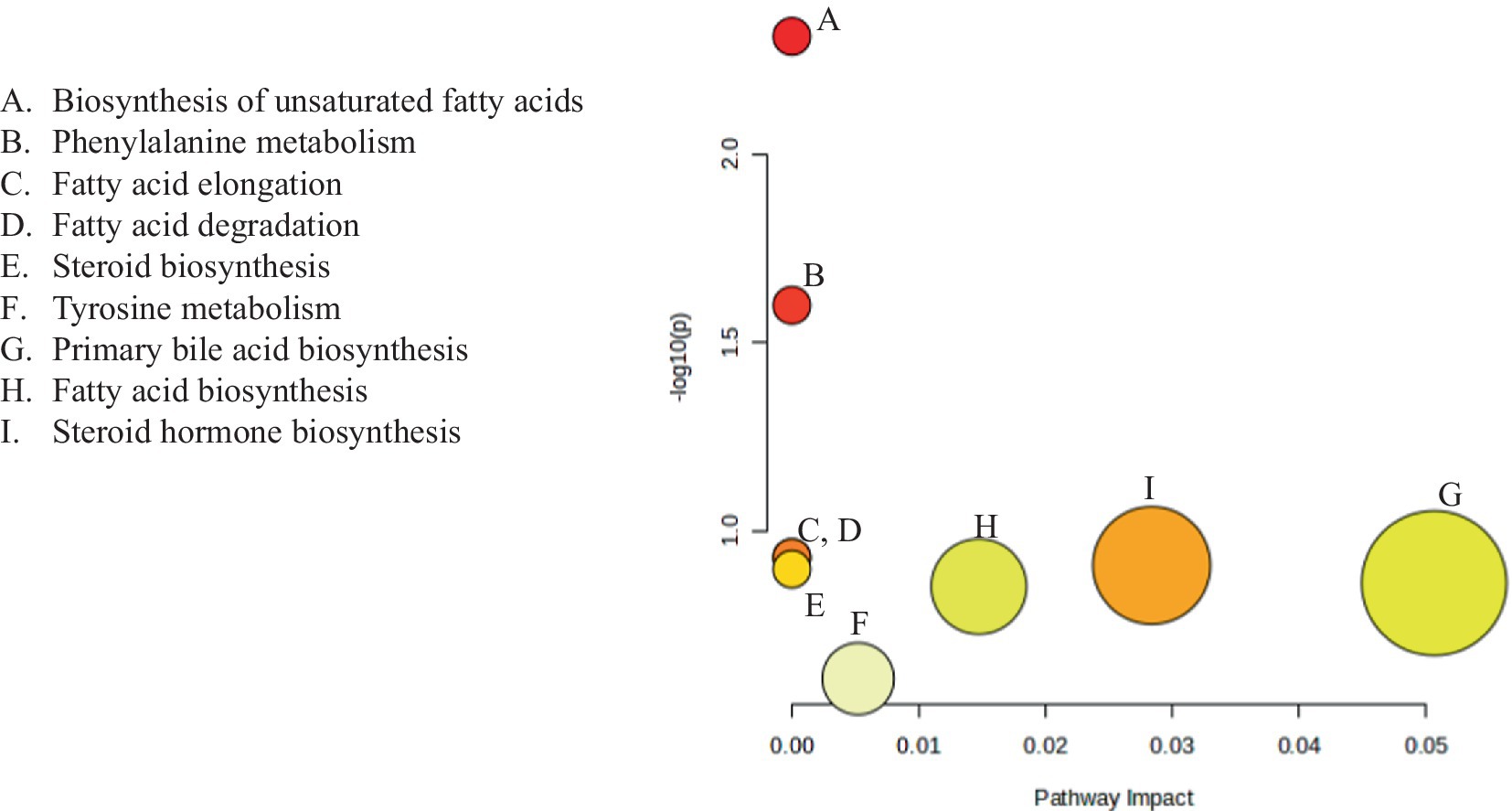
Figure 8. Metabolomic pathways of the identified volatile metabolites in organic extracts of SW. Each bubble represents one metabolomic pathway.
The Kyoto Encyclopedia of Genes and Genomes (KEGG) database revealed five significant metabolic pathways with FDR values ≤0.05. The biosynthesis pathway for unsaturated fatty acids had the highest log (p) value of 2.5284, followed by the phenylalanine metabolism biosynthesis pathway, which had a log(p) value of 1.695. The fatty acid elongation and degradation pathways had log (p) values of 1.0199, steroid biosynthesis had log(p) value of 0.99903, primary bile acid biosynthesis 0.95114, fatty acid biosynthesis 0.94221, and steroid hormone biosynthesis 0.69146.
3.9 Correlation
To understand and comprehend the relationship between the volatile metabolites better, Pearson’s correlation coefficient analysis was conducted (Figure 9). There were 1,367 significant correlation coefficients (p < 0.01, r2 ≥ 0.99) in the study, out of which 426 were positive and 904 were negative correlations. The analysis showed that compounds such as organosilicon compounds and benzofurans such as cyclosiloxane, phthalic anhydride, and benzene siloxane had the highest concentration fluctuations. The correlation of the 95 volatile metabolites was clustered into five main clusters according to the similarity in metabolomics pathways. Cluster 1 contained 25 volatile metabolites, and 15 volatile metabolites were found under Cluster 2. Clusters 3, 4, and 5 contained 16, 8, and 10 volatile metabolites, respectively.
3.10 Field experiment
From the micro-plot experiment, we confirmed that plants treated with CUE gave the highest yield, number of pods per plant, and number of branches per plant. However, plant height and dry matter production were highest in plants treated with FBE. In all the growth and yield attributes, WE- and VE-treated plants were relatively lower than CUE and FBE (Figure 10 and Supplementary Table S8).
4 Discussion
Seaweeds contain numerous kinds of organic compounds that have the potential to improve plant growth, development, and stress tolerance (Ali et al., 2021; Tinte et al., 2022). Phytochemicals improve plant defense mechanisms by releasing chemicals in the soil and plants that act as a barrier (Ndakidemi and Dakora, 2003; Dai and Mumper, 2010). The composition of flavonoids on organic extracts of SW increased in FBE might be due to the fermentation process of buttermilk. Sharma et al. (2021) also reported that fermented buttermilk increased flavonoid content in potato extract. However, an increase in the content of phenols and tannins in CUE and the decrease in VE might be attributed to the dissociation of phytochemicals at low pH. Dentinho and Bessa (2016) also reported that protein-bound and fiber-bound tannins were lower at low pH and increased at higher pH.
Among the seaweed compositions, secondary metabolites play an important role in its biological activities. Moreover, secondary metabolites act as an important weapon against plant defense systems. It also promotes the relationship of microbes, plants, insects, and higher animals (Demain and Fang, 2000). Secondary metabolites are derivatives of metabolism; hence, exploring the secondary metabolites at any given moment proposes an accurate picture of their biochemical activity (Bentley et al., 2022). A major part of the composition of volatile metabolites of biostimulants, as well as their detailed mode of action, were largely unidentified, as biostimulants are complicated and their impacts on plants are mostly due to combined metabolites activity (Bulgari et al., 2019; Ertani et al., 2018). Seaweed contains several kinds of secondary metabolites, each with its own set of biochemical properties. Consequently, the purpose of this experiment was to conduct a thorough investigation into the secondary metabolites of organic seaweed extracts that are both environmentally friendly and sustainable.
Most of the fatty acids found in the SW were extracted by the organic solvents. Fatty acids such as erucic acid exhibit antibacterial (Goc et al., 2019), antiviral (Liang et al., 2020), anti-inflammatory (Melzig and Henke, 2005), and cytotoxic activity (Altinoz et al., 2018). Compounds such as octadecanal were found to have the potential as pheromones that can attract both males and females of the Curculionidae family (Santos Neta et al., 2021). 15-Methylpalmitate showed strong acaricidal activity (Wang et al., 2009); moreover, anti-inflammatory and antioxidant activities were also observed when found in medicinal plants (Hamed et al., 2020). 7Z,10Z-Hexadecadienoic acid has bactericidal activity against gram-positive bacteria (Yuyama et al., 2020). n-Hexadecanoic acid also showed various biological activities such as antioxidants, hypocholesterolemic, nematicide, and pesticide (Sheela and Uthayakumari, 2013). cis-Vaccenic acid has antibacterial and hypolipidemic effects on rats (Hamazaki et al., 2016). Linoleic acid methyl ester also possesses properties such as anti-inflammatory, antiandrogenic, dermatitigenic hypocholesterolemic, 5-alpha reductase inhibitor, anemiagenic, and insectifuge (Surender et al., 2008; Krishnamoorthy and Subramaniam, 2014). Octadecanoic acids and methyl ester show anti-inflammatory activities (Silva et al., 2014). Among the organic extracts, FBE has the most fatty acids with five compounds. The commonly identified fatty acid, ginsenoyne K also known as shogaol was detected, which was the main compound of ginger exhibiting anti-inflammatory (Sang et al., 2009) and antioxidant (Aryanti et al., 2018) properties. Octadecanoic acid found in FBE has antibacterial and antioxidant activity (Sudharsan et al., 2011). Pentadecanoic acid also found in FBE has antibacterial and antimicrobial activity (Mujeeb et al., 2014). In particular, among all the organic extracts of SW, only the FBE extract identified specific fatty acids that were not found in other extracts. This might be due high fatty acid composition of fermented buttermilk which was used as a solvent for extraction. The biological significance of some important volatile metabolites is given in Table 4, and the functions and biological properties of various volatile metabolites in plants are shown in Table 5.
Benzene and benzene derivatives composed the second major share after fatty acyls. 2,6-Di-tert-butylhydroquinone identified in VE has antioxidant activity (Anonymous, 2014). A maximum of the benzene derivatives were identified in FB, CU, and CUE with four compounds each. Benzoic acid and methyl ester possess antibacterial properties (Al et al., 2020), Benzoic acid has a role as a plant volatile metabolite, an algal volatile metabolite, and also has antimicrobial properties (Kim et al., 2023). Benzene acetic acid is also a plant hormone (auxin), antimicrobial, antibiotic, and plant metabolite (Kim et al., 2023). Benzyl nitrile also a CUE-identified compound is an insect pheromone and animal metabolite (Kim et al., 2023).
Indole and its derivatives possess many biological activities. Generally, indole derivatives have antiviral, anti-inflammatory, anticancer, anti-HIV, antioxidant, antimicrobial, antitubercular, antidiabetic, antimalarial, and anticholinesterase activities (Kumar and Ritika., 2020). Identified indole derivatives were 1H-indole, 1-methyl-2-phenyl-, 1-methyl-2-phenylindole, 3-methyldioxyindole, indolizine, 2-(4-methylphenyl)-. Organooxygen compounds such as ethanol, 2-(octadecyloxy)- identified in SW exhibit antimicrobial activity (Jaffar et al., 2015). Eicosen-1-ol also exudes antifungal and antioxidant activity (Tayade et al., 2013). Acetic acid, the only compound identified in vinegar, is a naturally produced organic acid, which helps in the growth of plants and also improves plant tolerance toward stress while improving the uptake of water and nutrients, thus increasing the rate of photosynthesis (Rahman et al., 2024).
4.1 Pathway topology
Metabolites in living organisms have a complex relationship. These components work together to create complex pathways and networks that intertwine and govern one another’s function (Udhaya et al., 2023). As complicated as they are, they are an important link between genotype and phenotype (Fiehn, 2002). As a result, this interconnected process leads to extensive metabolome modifications (Töpfer et al., 2015). In our study, we precisely define and arrange the pathways used to interpret the variation in volatile metabolite expression in SW organic extracts.
4.2 Heatmap clustering and correlation
Based on the volatile metabolites identified, HCA was constructed to collect relevant information among the shared and distinct characteristics in the volatile metabolite profiles of the SW organic extracts. The diversity of volatile metabolite profiles was demonstrated using cluster analysis (Figure 7). The top 45 secondary volatile metabolites were selected to represent the ability of the analytical parameters to distinguish. Similarities among the dataset were created using a single linkage and correlation. More than half of the significant volatile metabolites found in organic extracts exhibit relative abundance.
The heatmap-based dendrogram shows that cluster 2 (CU and FB) is more compact, implying that the volatile metabolites were equivalent within the cluster group. Cluster 1 volatile metabolites varied more than cluster 2 samples as evidenced by their relative spacing. Variations of volatile metabolites in and among clusters might be due to the nature of the extraction solvent used. As a result, the nature of the solvents may have a direct impact on the HCA results.
4.3 PLS-DA
According to the PLS-DA score plot (Supplementary Figure S10), WE was clearly separated from other samples. Moreover, from the top 49 volatile metabolites having VIP score more than 1 were chosen from organic extracts of SW, we can see that volatile metabolites changes in organic extracts were observed compared to SW and the organic solvents (Figure 6). Comparing CU and CUE, we can clearly observe that volatile metabolites in CU were not found in CUE indicating the reaction of CU compounds with SW compounds and the transformation into new compounds. For example, the compounds of CU viz., Benzeneacetamide, Benzoic acid, 2-amino-, methyl ester, and SW hexatriacontane, Octadecanoic acid, methyl ester, and cyclododecane were not found in CUE; however, the new compounds/volatile metabolites found were benzeneacetic acid, benzoic acid, benzyl nitrile, and formamide (2-acetyl phenyl).
Those volatile metabolites found in CUE due to transformation upon extraction were found to have a beneficial impact on the growth of plants. Volatile metabolites such as benzene acetic acid, a precursor for plant hormone auxin, and benzoic acid, a plant volatile metabolite (Kim et al., 2023), found in CUE might be the transformation product of benzene acetamide and benzoic acid, 2-amino-, methyl ester of CU. Similarly, the WE obtained using deionized water from SW did not have most of the volatile metabolites identified in SW. Such volatile metabolites were phthalic anhydride, octacosane, heptadecanoic acid, 16-methyl-, methyl ester, etc. These volatile metabolites also have a positive impact on plant growth by improving antibacterial and antioxidant activities and act as a pesticide when applied to plants (Suseem and Saral, 2013; Kim et al., 2023). Similarly, in VE of SW, due to the acidity of the solvents, many volatile metabolites might have transformed into new volatile metabolites resulting in a variation of the metabolites composition of VE compared to SW. Volatile metabolites such as 1-tridecene, undecane, and methyl palmitate might be the transformed volatile metabolites of SW volatile metabolites such as 2-methyldecane, hexadecane, and 15-methylpalmitate, respectively. However, in FBE, the volatile metabolites identified were mostly observed in both FB and SW, indicating less transformation of volatile metabolites during extraction.
4.4 Micro-plot experiment
The increase in yield when black gram plants were treated with CUE might be due to the presence of benzene and benzene derivative volatile metabolites such as benzene acetic acid, which act as an auxin precursor and plant volatile metabolites (Kim et al., 2023). Benzoic acid has a role as a plant volatile metabolite, and benzyl nitrile was reported to be an insect pheromone (Kim et al., 2023). However, the increase in plant foliage when treated with FBE might be attributed to the high composition of fatty acids in FBE, which was contributed largely by the fermented buttermilk when used as a solvent for extracting the seaweed. Major fatty acids in FBE such as pentadecanoic acid and octadecanoic acid are plant volatile metabolites (Kim et al., 2023). Moreover, phthalic anhydride, which is the major volatile metabolite in FBE, acts as a precursor for amines and is used as a component of agricultural pesticides such as phaltan (Kim et al., 2023). A foliar spray of vinegar and vinegar-extracted seaweed resulted in the reduction and growth of black gram. This might be due to the presence of acetic acid, which has herbicidal properties and inhibits the growth of plants (Pujisiswanto et al., 2013). This reduction in plant growth might have affected the yield consequently.
5 Conclusion
The present study provides insight into changes in the metabolites of seaweed when extracted with organic solvents. So far, organic solvents such as vinegar, fermented buttermilk, and cow urine have not been used for extracting seaweeds. With organic agriculture and organic foods gaining popularity, our study will help in understanding the benefits of using organic solvents. Untargeted metabolomics of organic extracts of SW showed 95 volatile metabolites under 22 chemical classes exhibited different therapeutic and health benefits. Moreover, some volatile metabolites also showed beneficial effects for agricultural sectors as these volatile metabolites include plant benefiting metabolites and precursors of growth hormones, which are known to improve stress tolerance; this was proved in the micro-plot experiment of black gram. The biological activity of some volatile metabolites also showed insecticidal properties. Having biostimulants as the aim of the use of the organic extracts of SW, identification of such compounds can help contribute to the agricultural sector. Moreover, it was observed through this study that organic extracts of seaweed have benefits over traditional/conventional solvents as most of the volatile metabolites observed in conventional solvents were also observed, and on top of that, some volatile metabolites that have significant contributions in plant growth and development were also identified. Hence, this study recommends the extraction of seaweeds using organic solvents such as fermented buttermilk and cow urine.
Data availability statement
The original contributions presented in the study are included in the article/Supplementary material, further inquiries can be directed to the corresponding author/s.
Author contributions
PL: Conceptualization, Investigation, Methodology, Writing – original draft. RKr: Conceptualization, Project administration, Supervision, Writing – review & editing, Writing – original draft. PJ: Investigation, Methodology, Writing – original draft, Writing – review & editing. MS: Formal analysis, Writing – review & editing, Data curation. MD: Formal analysis, Writing – review & editing. RKa: Formal analysis, Writing – review & editing, Software.
Funding
The author(s) declare that no financial support was received for the research, authorship, and/or publication of this article.
Conflict of interest
The authors declare that the research was conducted in the absence of any commercial or financial relationships that could be construed as a potential conflict of interest.
Publisher’s note
All claims expressed in this article are solely those of the authors and do not necessarily represent those of their affiliated organizations, or those of the publisher, the editors and the reviewers. Any product that may be evaluated in this article, or claim that may be made by its manufacturer, is not guaranteed or endorsed by the publisher.
Supplementary material
The Supplementary material for this article can be found online at: https://www.frontiersin.org/articles/10.3389/fsufs.2024.1433974/full#supplementary-material
Footnotes
References
Ahmed, A., Akbar, S., and Shah, W. A. (2017). Chemical composition and pharmacological potential of aromatic water from Salix caprea inflorescence. Chin. J. Integr. Med., 1–5. doi: 10.1007/s11655-017-2781-5
Al, B. M., Makeen, H. A., Alhazmi, H. A., Syame, S. M., Abdalla, A. N., Homeida, H. E., et al. (2020). Phytochemical, cytotoxic, and antimicrobial evaluation of the fruits of miswak plant, Salvadora persica L. J. Chem. 2020, 1–11. doi: 10.1155/2020/4521951
Ali, O., Ramsubhag, A., and Jayaraman, J. (2021). Biostimulant properties of seaweed extracts in plants: implications towards sustainable crop production. Plants 10:531. doi: 10.3390/plants10030531
Altinoz, M. A., Bilir, A., and Elmaci, İ. (2018). Erucic acid, a component of Lorenzo’s oil and PPAR-δ ligand modifies C6 glioma growth and toxicity of doxorubicin. Experimental data and a comprehensive literature analysis. Chem. Biol. Interact. 294, 107–117. doi: 10.1016/j.cbi.2018.08.024
Amorim, E. L., Nascimento, J. E., Monteiro, J. M., Peixoto Sobrinho, T. J. S., Araújo, T. A., and Albuquerque, U. P. (2008). A simple and accurate procedure for the determination of tannin and flavonoid levels and some applications in ethnobotany and ethnopharmacology. Funct. Ecosyst. Commun. 2, 88–94.
Anonymous (2014). Survey of the occurrence of 2,5-Di-tertbutylhydroquinone in food contact material. Copenhagen K, Denmark: The Danish Environmental Protection Agency. Danish Ministry of the Environment, 9–10.
Aryanti, N., Nafiunisa, A., Bella, N., Sanjaya, R., Wardhani, D. H., and Kumoro, A. C. (2018). Kinetics of ultrasound-assisted extraction of anthocyanin from purple roselle calyces under different PH conditions. Chem. Chem. Technol. 4, 523–528. doi: 10.23939/chcht12.04.523
Bentley, J., Liebrich, P. Y., Farrant, J. M., Mandishonha, M., Reddy, A., and Rafudeen, M. S. (2022). Metabolomic analysis of the roots and shoots of tomato seedlings treated with the commercial seaweed-derived biostimulant Afrikelp. S. Afr. J. Bot. 147, 646–651. doi: 10.1016/j.sajb.2022.02.040
Bouchereau, A., Guénot, P., and Larher, F. (2000). Analysis of amines in plant materials. J. Chromatogr. B Biomed. Sci. Appl. 747, 49–67. doi: 10.1016/S0378-4347(00)00286-3
Bulgari, R., Franzoni, G., and Ferrante, A. (2019). Biostimulants application in horticultural crops under abiotic stress conditions. Agronomy 9:306. doi: 10.3390/agronomy9060306
Chandrasekaran, M., Senthilkumar, A., and Venkatesalu, V. (2011). Antibacterial and antifungal efficacy of fatty acid methyl esters from the leaves of Sesuvium portulacastrum L. Eur. Rev. Med. Pharmacol. Sci. 15, 775–780
Cowan, M. M. (1999). Plant products as antimicrobial agents. Clin. Microbiol. Rev. 12, 564–582. doi: 10.1128/CMR.12.4.564
Dai, J., and Mumper, R. J. (2010). Plant phenolics: extraction, analysis and their antioxidant and anticancer properties. Molecules 15, 7313–7352. doi: 10.3390/molecules15107313
Dakora, F. D., and Phillips, D. A. (1996). Diverse functions of isoflavonoids in legumes transcend ant-microbial definitions of phytoalexins. Physiol. Mol. Plant Pathol. 49, 1–20. doi: 10.1006/pmpp.1996.0035
Demain, A. L., and Fang, A. (2000). The natural functions of secondary metabolites. History Modern Biotechnol. 69, 1–39. doi: 10.1007/3-540-44964-7_1
Dentinho, M. T., and Bessa, R. J. (2016). Effect of tannin source and pH on stability of tannin-protein and fibre complexes. Revista Ciências Agrárias 39, 114–121. doi: 10.19084/RCA15062
Devi, J. A. I., Mala, V. M., and Venkateshan, N. (2020). Gas chromatography - mass spectroscopy analysis of ethyl acetate and Ethanolic extracts of Cordia obliqua wild. Int. J. Res. Pharm. Sci. 9, 1–14.
Du, Y., Fu, X., Chu, Y., Wu, P., Liu, Y., Ma, L., et al. (2022). Biosynthesis and the roles of plant sterols in development and stress responses. Int. J. Mol. Sci. 23:2332. doi: 10.3390/ijms23042332
Ertani, A., Francioso, O., Ferrari, E., Schiavon, M., and Nardi, S. (2018). Spectroscopic-chemical fingerprint and biostimulant activity of a protein-based product in solid form. Molecules 23:1031. doi: 10.3390/molecules23051031
Fiehn, O. (2002). Metabolomics—the link between genotypes and phenotypes. Plant Mol. Biol. 48, 155–171. doi: 10.1023/A:1013713905833
Fiehn, O. (2016). Metabolomics by gas chromatography–mass spectrometry: combined targeted and untargeted profiling. Curr. Protoc. Mol. Biol. 114, 30–34. doi: 10.1002/0471142727.mb3004s114
Goc, A., Niedzwiecki, A., and Rath, M. (2019). Anti-borreliae efficacy of selected organic oils and fatty acids. BMC Complement. Altern. Med. 19, 1–11. doi: 10.1186/s12906-019-2450-7
Godlewska, K., Michalak, I., Tuhy, Ł., and Chojnacka, K. (2016). Plant growth biostimulants based on different methods of seaweed extraction with water. Biomed. Res. Int. 2016, 1–11. doi: 10.1155/2016/5973760
Gorrochategui, E., Jaumot, J., Lacorte, S., and Tauler, R. (2016). Data analysis strategies for targeted and untargeted LC-MS metabolomic studies: overview and workflow. TrAC Trends Anal. Chem. 82, 425–442. doi: 10.1016/j.trac.2016.07.004
Hamazaki, K., Suzuki, N., Kitamura, K. I., Hattori, A., Nagasawa, T., Itomura, M., et al. (2016). Is vaccenic acid (18, 1t n-7) associated with an increased incidence of hip fracture? An explanation for the calcium paradox. Prostaglandins Leukot. Essent. Fat. Acids 109, 8–12. doi: 10.1016/j.plefa.2016.04.001
Hamed, A. B., Eman, M., Mantawy, W. M., El-Bakly, Y. M., and Azab, S. S. (2020). Methyl palmitate: the naturally occurring cardioprotective agent. Arch. Pharm. Sci. Ain. Shams. Univ. 4, 47–62. doi: 10.21608/APS.2020.2003.1026
Han, C., Chen, X., Xie, W., Zhu, Z., Liu, C., Chen, F., et al. (2010). Determination of hexabromocyclododecane diastereoisomers in Sargassum fusiforme and comparison of the extraction efficiency of ultrasonication, microwave-assisted extraction, Soxhlet extraction and pressurised liquid extraction. J. Sep. Sci. 33, 3319–3325. doi: 10.1002/jssc.201000558
Hao, M. H., Zhang, F., Liu, X. X., Wang, L. J., Xu, S. J., Zhang, J. H., et al. (2018). Qualitative and quantitative analysis of catechin and quercetin in flavonoids extracted from Rosa roxburghii Tratt. Trop. J. Pharm. Res. 17, 71–76. doi: 10.4314/tjpr.v17i1.11
Islam, M. T., Ali, E. S., Uddin, S. J., Shaw, S., Islam, M. A., Ahmed, M. I., et al. (2018). Phytol: a review of biomedical activities. Food Chem. Toxicol. 121, 82–94. doi: 10.1016/j.fct.2018.08.032
Ismail, G. A., Gheda, S. F., Abo-Shady, A. M., and Abdel-Karim, O. H. (2019). In vitro potential activity of some seaweeds as antioxidants and inhibitors of diabetic enzymes. Food Sci. Technol. 40, 681–691. doi: 10.1590/fst.15619
Jacomassi, L. M., Viveiros, J. D., Oliveira, M. P., Momesso, L., de Siqueira, G. F., and Crusciol, C. A. (2022). A seaweed extract-based biostimulant mitigates drought stress in sugarcane. Front. Plant Sci. 13:865291. doi: 10.3389/fpls.2022.865291
Jaffar, A., Somanath, B., and Karthi, S. (2015). Efficacy of methanolic extract of a marine ascidian, Lissoclinum bistratum for antimicrobial activity. J. Chem. Biol. Phys. Sci. 5:4119.
Khan, W., Rayirath, U. P., Subramanian, S., Jithesh, M. N., Rayorath, P., Hodges, D. M., et al. (2009). Seaweed extracts as biostimulants of plant growth and development. J. Plant Growth Regul. 28, 386–399. doi: 10.1007/s00344-009-9103-x
Kim, S., Chen, J., Cheng, T., Gindulyte, A., He, J., He, S., et al. (2023). Kim et al. 2023 update. Nucleic Acids Res. 51, D1373–D1380. doi: 10.1093/nar/gkac956
Krishnamoorthy, K., and Subramaniam, P. (2014). Phytochemical profiling of leaf, stem, and tuber parts of Solena amplexicaulis (lam.) Gandhi using GC-MS. Int. Scholarly Res. Notices 2014, 1–13. doi: 10.1155/2014/567409
Kumar, V., Bhatnagar, A. K., and Srivastava, J. N. (2011). Antibacterial activity of crude extracts of Spirulina platensis and its structural elucidation of bioactive compound. J. Med. Plants Res. 5, 7043–7048. doi: 10.5897/JMPR11.1175
Kumar, S., and Ritika,. (2020). A brief review of the biological potential of indole derivatives. Future J. Pharmaceut. Sci. 6, 1–19. doi: 10.1186/s43094-020-00141-y
Lee, D. E., Lee, S., and Singh, D. (2017). Time-resolved comparative metabolomes for Koji fermentation with brown-, white-, and giant embryo-rice. Food Chem. 231, 258–266. doi: 10.1016/j.foodchem.2017.03.119
Liang, X., Huang, Y., Pan, X., Hao, Y., Chen, X., Jiang, H., et al. (2020). Erucic acid from Isatis indigotica Fort. Suppresses influenza a virus replication and inflammation in vitro and in vivo through modulation of NF-κB and p38 MAPK pathway. J. Pharm. Anal. 10, 130–146. doi: 10.1016/j.jpha.2019.09.005
Mandal, S. M., Chakraborty, D., and Dey, S. (2010). Phenolic acids act as signaling molecules in plant-microbe symbioses. Plant Signal. Behav. 5, 359–368. doi: 10.4161/psb.5.4.10871
Melzig, M. F., and Henke, K. (2005). Inhibition of thrombin activity by selected natural products in comparison to neutrophil elastase. Planta Med. 71, 787–789. doi: 10.1055/s-2005-871253
Mujeeb, F., Bajpai, P., and Pathak, N. (2014). Phytochemical evaluation, antimicrobial activity, and determination of bioactive components from leaves of Aegle marmelos. BioMed Res. Int. 2024, 1–11. doi: 10.1155/2014/497606
Mukesi, M., Iweriebor, B. C., Obi, L. C., Nwodo, U. U., Moyo, S. R., and Okoh, A. I. (2019). The activity of commercial antimicrobials, and essential oils and ethanolic extracts of Olea europaea on Streptococcus agalactiae isolated from pregnant women. BMC Complement. Altern. Med. 19, 1–9. doi: 10.1186/s12906-019-2445-4
Nabi, M., Tabassum, N., and Ganai, B. A. (2022). Phytochemical screening and antibacterial activity of Skimmia anquetilia NP Taylor and airy Shaw: a first study from Kashmir Himalaya. Front. Plant Sci. 13:937946. doi: 10.3389/fpls.2022.937946
Ndakidemi, P. A., and Dakora, F. D. (2003). Legume seed flavonoids and nitrogenous metabolites as signals and protectants in early seedling development. Rev. Funct. Plant. Biol. 30, 729–745. doi: 10.1071/FP03042
Nisha Tripathi, N. T., Sunita Kumar, S. K., Rakesh Singh, R. S., Singh, C. J., Prashant Singh, P. S., and Varshney, V. K. (2013). Isolation and identification of γ-sitosterol by GC-MS from roots of Girardinia heterophylla. Orient. J. Chem. 29, 705–707. doi: 10.13005/ojc/290245
Osama, A., Awadelkarim, S., and Ali, A. (2017). Antioxidant activity, acetylcholinesterase inhibitory potential and phytochemical analysis of Sarcocephalus latifolius Sm. Bark used in traditional medicine in Sudan. BMC Complement. Altern. Med. 17:270. doi: 10.1186/s12906-017-1772-6
Othman, A. R., Abdullah, N., Ahmad, S., Ismail, I. S., and Zakaria, M. P. (2015). Elucidation of in-vitro anti-inflammatory bioactive compounds isolated from Jatropha curcas L. plant root. BMC Complement. Altern. Med. 15, 1–10. doi: 10.1186/s12906-015-0528-4
Pal, D., and Lal, P. (2023). “Plants showing anti-viral activity with emphasis on secondary metabolites and biological screening,” in Anti-viral metabolites from medicinal plants, reference seriesin phytochemistry. ed. D. Pal (Cham.: Springer).
Palaniyappan, S., Sridhar, A., Kari, Z. A., Téllez-Isaías, G., and Ramasamy, T. (2023). Evaluation of phytochemical screening, pigment content, in vitro antioxidant, antibacterial potential and GC-MS metabolite profiling of green seaweed Caulerpa racemosa. Mar. Drugs 21:278. doi: 10.3390/md21050278
Petchidurai, G., Nagoth, J. A., John, M. S., Sahayaraj, K., Murugesan, N., and Pucciarelli, S. (2019). Standardization and quantification of total tannins, condensed tannin and soluble phlorotannins extracted from thirty-two drifted coastal macroalgae using high performance liquid chromatography. Bioresource Technol. Reports 7:100273. doi: 10.1016/j.biteb.2019.100273
Pujisiswanto, H., Yudono, P., Sulistyaningsih, E., and Sunarminto, B. H. (2013). Effect of acetic acid as pre-plant herbicide on maize germination. ARPN J. Agric. Biol. Sci. 8, 696–701.
Rahman, M. M., Keya, S. S., Sahu, A., Gupta, A., Dhingra, A., Tran, L. S. P., et al. (2024). Acetic acid: a cheap but chief metabolic regulator for abiotic stress tolerance in plants. Stress Biology 4:34.
Renčo, M., Sasanelli, N., and Maistrello, L. (2014). “Plants as natural sources of nematicides. Nematodes: Comparative genomics, disease management and ecological importance” in Plants as natural sources of Nematicides. ed. L. M. Davis (New York: NOVA Science publisher), 115–141.
Rukhsana, K., Varghese, V., Akhilesh, V. P., Jisha Krishnan, E. K., Priya Bhaskaran, K. P., Bindu, P. U., et al. (2015). GC-MS determination of chemical components in the bioactive secretion of Anoplodesmus saussurii (Humbert, 1865). Int. J. Pharm. Sci. Res. 6, 650–653.
Saeed, N., Khan, M. R., and Shabbir, M. (2012). Antioxidant activity, total phenolic and total flavonoid contents of whole plant extracts Torilis leptophylla L. BMC Complement. Altern. Med. 12, 1–12. doi: 10.1186/1472-6882-12-221
Salar, R. K., Certik, M., and Brezova, V. (2012). Modulation of phenolic content and antioxidant activity of maize by solid state fermentation with Thamnidium Elegans CCF 1456. Biotechnol. Bioprocess Eng. 17, 109–116. doi: 10.1007/s12257-011-0455-2
Sang, S., Hong, J., Wu, H., Liu, J., Yang, C. S., Pan, M. H., et al. (2009). Increased growth inhibitory effects on human cancer cells and anti-inflammatory potency of shogaols from Zingiber officinale relative to gingerols. J. Agric. Food Chem. 57, 10645–10650. doi: 10.1021/jf9027443
Santos Neta, P. L., Fávaro, C. F., Macedo, S. O., Moura, J. I., Bello, J. E., Santos, R. R., et al. (2021). Octadecanal as the male-produced aggregation pheromone of the coconut weevil, Amerrhinus ynca (Coleoptera: Curculionidae). J. Braz. Chem. Soc. 32, 2017–2021. doi: 10.21577/0103-5053.20210087
Santos, C. C., Salvadori, M. S., Mota, V. G., Costa, L. M., de Almeida, A. A., de Oliveira, G. A., et al. (2013). Antinociceptive and antioxidant activities of phytol in vivo and in vitro models. Neurosci. J. 2013, 1–9. doi: 10.1155/2013/949452
Sharma, A., Noda, M., Sugiyama, M., Ahmad, A., and Kaur, B. (2021). Production of functional buttermilk and soymilk using Pediococcus acidilactici BD16 (alaD+). Molecules 26:4671. doi: 10.3390/molecules26154671
Sheela, D., and Uthayakumari, F. (2013). GC-MS analysis of bioactive constituents from coastal sand dune taxon Sesuvium portulacastrum (L.) L. Biosci. Discov. 4, 47–53.
Silva, R. O., Sousa, F. B. M., Damasceno, S. R., Carvalho, N. S., Silva, V. G., Oliveira, F. R. M., et al. (2014). Phytol, a diterpene alcohol, inhibits the inflammatory response by reducing cytokine production and oxidative stress. Fundam. Clin. Pharmacol. 28, 455–464. doi: 10.1111/fcp.12049
Stockwell, V. O., and Duffy, B. (2012). Use of antibiotics in plant agriculture. Rev. Sci. Technique 31, 199–210. doi: 10.20506/rst.31.1.2104
Sudharsan, S., Saravanan, R., Shanmugam, A., Vairamani, S., Kumar, R. M., Menaga, S., et al. (2011). Isolation and characterization of octadecanoic acid from the ethyl acetate root extract of Trigonella foneum graecum L. by using hydroponics method. Bioterrorism Biodefense 2, 1–4. doi: 10.4172/2157-2526.1000105
Sundarraj, S., Thangam, R., Sreevani, V., Kaveri, K., Gunasekaran, P., Achiraman, S., et al. (2012). γ-Sitosterol from Acacia nilotica L. induces G2/M cell cycle arrest and apoptosis through c-Myc suppression in MCF-7 and A549 cells. J. Ethnopharmacol. 141, 803–809. doi: 10.1016/j.jep.2012.03.014
Surender, S., Vinod, N., Sweety, J., and Gupta, Y. K. (2008). Evaluation of anti-inflammatory activity of plant lipids containing α-linolenic acid. Indian J. Exp. Biol. 46, 453–456.
Suseem, S. R., and Saral, A. M. (2013). Analysis on essential fatty acid esters of mushroom pleurotus eous and its antibacterial activity. Asian J. Pharm. Clin. Res. 6, 188–191.
Tanna, B., Choudhary, B., and Mishra, A. (2018). Metabolite profiling, antioxidant, scavenging and anti-proliferative activities of selected tropical green seaweeds reveal the nutraceutical potential of Caulerpa Spp. Algal Res. 36, 96–105. doi: 10.1016/j.algal.2018.10.019
Tayade, A. B., Dhar, P., Kumar, J., Sharma, M., Chauhan, R. S., Chaurasia, O. P., et al. (2013). Chemometric profile of root extracts of Rhodiola imbricata Edgew. With hyphenated gas chromatography mass spectrometric technique. PLoS One 8:e52797. doi: 10.1371/journal.pone.0052797
Tinte, M. M., Masike, K., Steenkamp, P. A., Huyser, J., van der Hooft, J. J. J., and Tugizimana, F. (2022). Computational metabolomics tools reveal metabolic reconfigurations underlying the effects of biostimulant seaweed extracts on maize plants under drought stress conditions. Meta 12:487. doi: 10.3390/metabo12060487
Töpfer, N., Kleessen, S., and Nikoloski, Z. (2015). Integration of metabolomics data into metabolic networks. Front. Plant Sci. 6:49. doi: 10.3389/fpls.2015.00049
Tretyn, A., and Kendrick, R. E. (1991). Acetylcholine in plants: presence, metabolism and mechanism of action. Bot. Rev. 57, 33–73. doi: 10.1007/BF02858764
Udhaya, N. D., Venkatesan, S., Senthilraja, K., Janaki, P., Prabha, B., Sangamithra, S., et al. (2023). Metabolomic analysis for disclosing nutritional and therapeutic prospective of traditional rice cultivars of Cauvery deltaic region, India. Front. Nutr. 10:1254624. doi: 10.3389/fnut.2023.1254624
Wang, Y. N., Wang, H. X., Shen, Z. J., Zhao, L. L., Clarke, S. R., Sun, J. H., et al. (2009). Methyl palmitate, an acaricidal compound occurring in green walnut husks. J. Econ. Entomol. 102, 196–202. doi: 10.1603/029.102.0128
Wessler, I., Kilbinger, H., Bittinger, F., and Kirkpatrick, C. J. (2001). The non-neuronal cholinergic system the biological role of non-neuronal acetylcholine in plants and humans. Japan. J. Pharmacol. 85, 2–10. doi: 10.1254/jjp.85.2
Yuyama, K. T., Rohde, M., Molinari, G., Stadler, M., and Abraham, W. R. (2020). Unsaturated fatty acids control biofilm formation of Staphylococcus aureus and other gram-positive bacteria. Antibiotics 9:788. doi: 10.3390/antibiotics9110788
Keywords: seaweed, organic, extraction, metabolomics, volatile metabolites
Citation: Lalruatfeli P, Krishnan R, Janaki P, Suganthy M, Djanaguiraman M and Kalpana R (2024) Unraveling the volatile metabolites and potential plant-stimulating properties of organically extracted Caulerpa racemosa. Front. Sustain. Food Syst. 8:1433974. doi: 10.3389/fsufs.2024.1433974
Edited by:
Giuseppina Rea, National Research Council (CNR), ItalyReviewed by:
Sowmyalakshmi Subramanian, McGill University, CanadaBeatriz Hernandez, Technological University of the Mixteca, Mexico
Copyright © 2024 Lalruatfeli, Krishnan, Janaki, Suganthy, Djanaguiraman and Kalpana. This is an open-access article distributed under the terms of the Creative Commons Attribution License (CC BY). The use, distribution or reproduction in other forums is permitted, provided the original author(s) and the copyright owner(s) are credited and that the original publication in this journal is cited, in accordance with accepted academic practice. No use, distribution or reproduction is permitted which does not comply with these terms.
*Correspondence: Ramanujam Krishnan, agrikrish@tnau.ac.in