- 1Department of Sustainability Science, School of Energy Systems, LUT University, Lappeenranta, Finland
- 2Department of Forest Sciences, Faculty of Agriculture and Forestry, University of Helsinki, Helsinki, Finland
Introduction: The reliance on fossil inputs of agricultural practices has led to maximizing yields and profitability, even at the expense of environmental sustainability. Implementing circular waste management solutions could help align the economic and environmental goals of farmers while reducing their reliance on fossil inputs. The co-application of anaerobic digestion and pyrolysis has been recognized as a potential solution to assist in the transition towards sustainable agriculture.
Objectives: This research assesses different waste management strategies of the major sidestreams generated in agroecosystems in Finland from a climate impact and financial perspective, particularly focusing on the integration of anaerobic digestion and pyrolysis as an emerging solution.
Methods: Six waste management options representing manure application, composting, incineration, anaerobic digestion, pyrolysis, and a co-application process of anaerobic digestion and pyrolysis, are simultaneously assessed with cash flow analysis and life cycle assessment.
Results: The results indicate that co-applying anaerobic digestion and pyrolysis strengthens the performance of a farm through reduced impact on climate, diversified revenue streams, and increased security of supply, even though the direct, short-term financial benefits remain marginal. The capability of co-application of anaerobic digestion and pyrolysis to reduce the climate impacts within the studied system supports the consensus that sustainable and self-sufficient agroecosystems could be supported by this solution in the future.
Conclusion: The adoption of more sustainable agricultural practices requires further expansion of technologies and additional value creation mechanisms, such as stronger employment of carbon markets, to even the economic competition and favor low-carbon operations for practitioners.
1 Introduction
Fossil-based economies are driving serious ecological challenges, such as pollution and climate change, placing the global food and forest systems at risk. At the same time, food systems contribute to a third of global anthropogenic greenhouse gas (GHG) emissions globally (Crippa et al., 2021). In Finland, the agricultural sector alone generates about 13% of annual GHG emissions (Statistics Finland, 2022). Finland’s national carbon neutrality target (Ministry of Economic Affairs and Employment, 2022) is currently falling short due to decreased forest carbon sinks and lack of new products that would sequestrate carbon, highlighting the need of sustainable solutions in the agricultural sector.
Despite efforts to implement sustainable operational models, such as regenerative farming practices, most of the agricultural operations still heavily depend on fossil inputs due to use of heavy machinery and insufficient circulation of materials. While coping with environmental concerns, agricultural entrepreneurs face vital socio-economic issues, such as rapid urbanization, ageing farming population, and declining profitability trend. In 2019, entrepreneurial income from Finnish farms decreased by around 20% compared to the previous year (Latvala et al., 2020).
To address these issues, new operational models that simultaneously prioritize environmental and financial goals while reducing reliance on fossil imports, are required. Establishing carbon sinks, closing material and energy loops, and producing long-lasting bioproducts show promise as potential ways to sequestrate carbon and reduce the reliance of fossil energy, thus improving the financial viability of rural entrepreneurs (Leppäkoski et al., 2021; Luhas et al., 2022). In the context of agricultural waste management, technologies that convert sidestreams into valuable by-products are gaining increasing interest (Demichelis et al., 2020). Treating manure and other biological waste with biorefinery processes, such as anaerobic digestion (AD) or pyrolysis (PY), has been recognized as more advanced alternative to traditional composting and incineration (Nayal et al., 2016) and favorable transition to meet circular bioeconomy requirements (Khoshnevisan et al., 2021; Leppäkoski et al., 2021; Luhas et al., 2022).
AD processes can be applied to treat organic matter (e.g., manure, bio waste, wastewater sludge), converting them into biogas and nutrient-rich digestate residue (Kaparaju and Rintala, 2011). Biogas can be used either to generate renewable energy in cogeneration of heat and electricity or by upgrading it to biomethane (Roubaud and Favrat, 2005), offering a domestic low-carbon alternative to imported fossil fuels. Utilization of AD has been increasing in Finland, reaching close to 1 TWh in 2019, representing 0.5% of Finnish renewable energy production (Huttunen et al., 2018; Virolainen-Hynnä, 2020). Agricultural feedstocks are still underemployed, with only 1% of animal manure used as biogas feedstock, while most of the commercial reactors utilize municipal and industrial wastewater sludge (Marttinen et al., 2018). Although AD is an advantageous and considerably researched technology, it suffers from technical issues, such as low methane production efficiency, high failure degree of equipment, problems with utilizing biogas digestate, and low financial performance especially on small and medium sized farms (Zhang et al., 2015; Chen and Liu, 2017).
Dry agricultural waste and lignocellulosic biomasses serve as suitable feedstocks for PY, the most widespread biochar production technology (Qian et al., 2015; Tisserant and Cherubini, 2019). Biochar is a long-lasting material which can maintain carbon in a stable form for over hundreds of years (Tisserant and Cherubini, 2019), making it an excellent carbon sink and nature-based solution for slowing down climate change (Leppäkoski et al., 2021; Tisserant et al., 2022). In the Boreal region measurements, biochar application indicated positive long-term impacts on crop yield, carbon dioxide (CO2) efflux, non-CO2 GHG emissions, and nitrogen (N) stability (Kalu et al., 2022). Bottlenecks concerning usage of PY are often related to generation of suitable feedstocks, high investment costs, regulatory issues, and unstable biochar market prices (Shackley et al., 2011; Kavitha et al., 2018; Leppäkoski et al., 2021). Recently, researchers have proposed applying AD and PY together to improve the product portfolio of both processes and relieve AD digestate treatment challenges (Fabbri and Torri, 2016; Ghysels et al., 2020; González-Arias et al., 2020; Caiardi et al., 2022).
When combining AD and PY processes, feedstocks can be routed within the system in multiple ways depending on their characteristic. Wet organic wastes are often more suitable for being digested, whereas dry lignocellulosic streams are ideal for PY. In some case, the remaining digestate of AD can be dewatered and treated in PY process, increasing the overall energy recovery and the value of the digestate (Song et al., 2021). Owing to its cellular format, biochar can improve soil quality and provide suitable conditions for microbes by retaining nutrients and water, making it an excellent component for chemical or organic fertilizers (Semida et al., 2019). In agronomic application, also biochar-enriched digestate has been demonstrated to increase plant growth in fields (Tayibi et al., 2019; Ronga et al., 2020). Biochar produced from digestate meets the IBI Biochar Standards recommendations for soil amendment (Tayibi et al., 2021) and serves as excellent material to be mixed with the liquid part of the digestate (Singh et al., 2022). Additionally, biochar acts as helpful catalyst in the AD process increasing the methane yields (Ma et al., 2019; Wu et al., 2019; Indren et al., 2020; Kaur et al., 2020; Lim et al., 2020; Sugiarto et al., 2021) and improving digester functioning by shortening the lag phase (Pan et al., 2019; Song et al., 2021). Therefore, circulating part of the produced biochar to back AD process could be beneficial also from the financial perspective.
A series-connected AD and PY, described by Song et al. (2021) and Singh et al. (2022), has been presented as a way to unlock the potential of agricultural sidestreams for significant financial and environmental benefits. With plenty of suitable agricultural and lignocellulosic residues available in the Finnish bioeconomy sector, co-application of AD and PY could relieve the environmental and socio-economic pressures of farmers. Integrating these technologies into agricultural waste management could also reduce the dependency of imported fossil-based inputs and improve the financial viability of agricultural operations with locally produced biogas, organic fertilizers, and biochar.
Although AD and PY technologies have been broadly researched separately (Sawatdeenarunat et al., 2015, 2016; Tisserant and Cherubini, 2019), their co-application has only recently begun to appear in scientific publications. Few studies have assessed their co-application with life-cycle assessment (LCA), namely in the treatment of manure (Mehta et al., 2022), sewage sludge (Mills et al., 2014; Havukainen et al., 2022), pulp and paper mill reject (Mohammadi et al., 2019), municipal solid waste (Wang et al., 2021), and food waste (Opatokun et al., 2017). It is still unknown to what extent the co-application of AD and PY could reduce the climate impacts of agricultural production. The technology combination has been assessed from the financial and environmental perspective together only in the treatment of sewage sludge (Mills et al., 2014), leaving its potential as a financially viable waste treatment option unexplored in the agricultural sector, which needs to be evidenced. Therefore, a key question remains whether this technology combination offers a viable low-carbon waste management strategy to support transition towards sustainable agriculture.
The purpose of this study is to document the financial feasibility and climate impacts of the co-application of AD and PY in the management of agricultural sidestreams, visualized in Figure 1, through cash flow analysis and LCA. Cash flow analysis and LCA are methods for evaluating the financial feasibility and environmental sustainability of a product, business, or operational model. LCA measures the environmental impacts of a project or a product by considering all the stages of the product’s life-cycle: from the extraction of raw materials to the disposal or recycling of the final product (ISO 2006a). Cash flow analysis assesses the financial viability by considering financial factors, such as capital and operational expenses, revenue generation, amortization, and inflation, allowing individuals and business practitioners to make informed financial decisions (Tham and Vélez-Pareja, 2004). This study compares the co-application of AD and PY with the utilization of business-as-usual operations (composting and incineration) and single AD, within the same research setting. This is needed to ensure the relevance of the technology combination for practitioners and compare methods of agricultural waste management from the two important perspectives: environment and economy. In a broader context, this research aims to identify optimally sustainable and financially viable waste management options for the agricultural sector.
2 Materials and methods
2.1 Scenario description
The sidestream management strategies were examined in this study for a theoretical representation of a farm-scale agroecosystem in Finland. Straw is a significant sidestream originating from Finnish agriculture, where the majority of farms focus on cultivating oats, barley, and wheat (Statistics Finland, 2024). Manure is another major agricultural sidestream with Finnish livestock farming strongly centered around milk and beef production, representing 70% of farms outside of plant production (Statistics Finland, 2024). In this study, the agroecosystem was characterized by a dairy farm with a nearby oats field. To enable further comparison of managing different type of wastes in the system, the farm was assumed to also include a greenhouse. Thus, the sidestreams originating from this agroecosystem are manure, oats straw, and greenhouse green waste.
The co-application of AD and PY was compared with management options, including manure spreading, composting, and incineration with a total of six scenarios presented in Table 1. The feedstocks listed include straw, manure, green waste, solid fraction (SF) of digestate, and liquid fraction (LF) of digestate. These treatment scenarios present more common and emerging waste management strategies. Scenario 1 (Baseline) represents a common management strategy of these feedstocks, with straw incinerated, manure spread to farmlands for nutrient recovery, and green waste composted (Laurila and Saarinen, 2014; Natural Resources Institute Finland, 2023a; Motiva, 2024a). In Scenario 2 (Compost), all the feedstocks are composted except straw, which is incinerated. In Scenario 3 (AD1), all the feedstocks are processed in a digester; then, the SF of the digestate is composted and the LF of digestate is spread to farmlands. In Scenario 4 (AD2), straw is incinerated, other feedstocks are processed in a digester, and the SF of the digestate composted and LF are spread to the farmlands. In Scenario 5 (AD1-PY), all the feedstocks are processed in a digester, after which the SF of the digestate is used in PY, and the LF of the digestate is spread to farmlands. In Scenario 6 (AD2-PY), straw is used in PY, other feedstocks are processed in a digester, and the SF and LF of the digestate used in PY are spread to farmlands. Figure 2 presents the different scenarios and the system boundary for the treatment and utilization of the sidestreams of the agroecosystem. The system boundary entails the whole utilization chain from treatment to utilization, excluding the collection of sidestreams and the construction of equipment and machinery. Detailed flow charts of individual scenarios are presented in the Supplementary material.
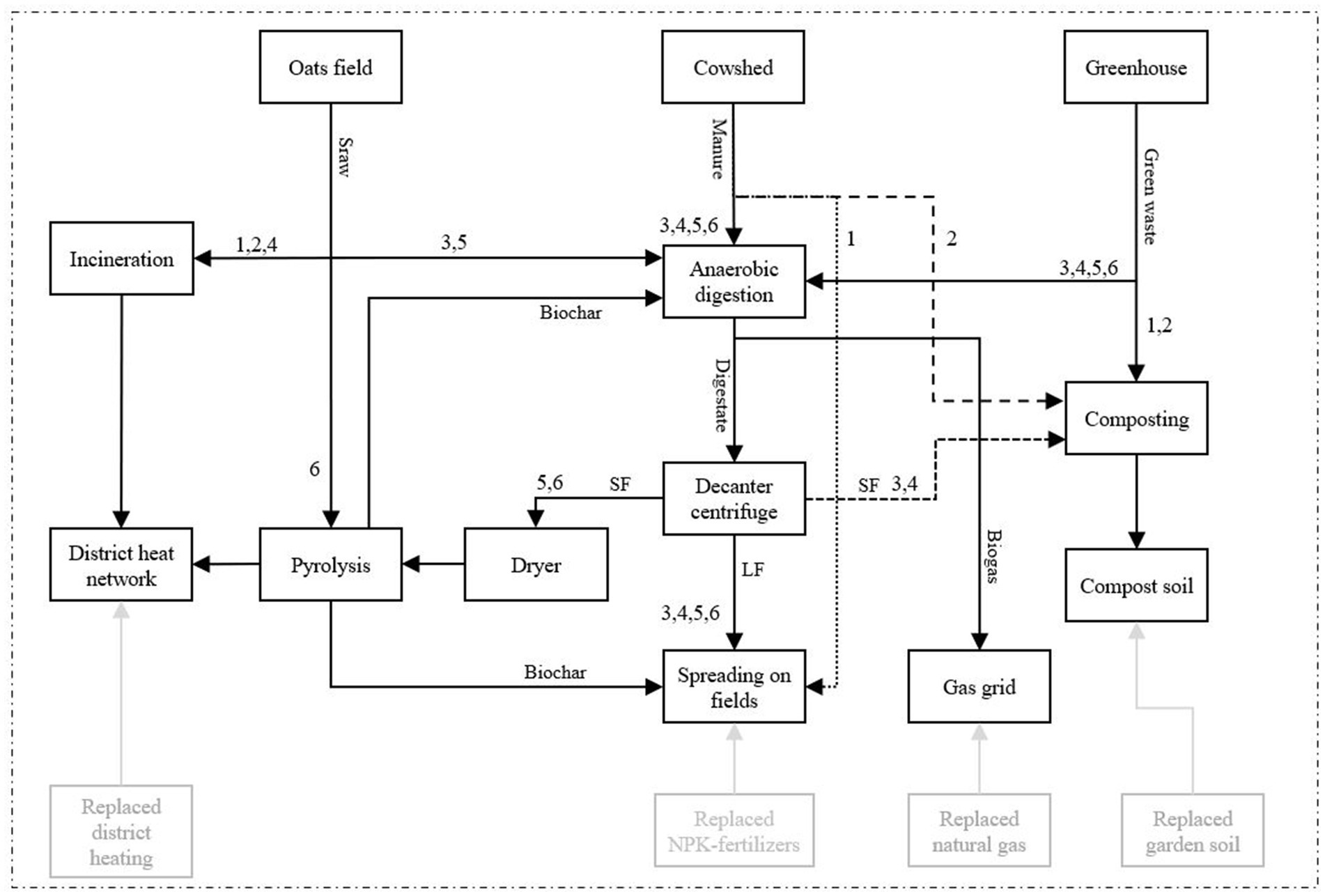
Figure 2. System boundary for the study. Numbering presents the specific flow for the waste management scenario.
2.2 Methodology
In this study, financial performance was assessed through profitability and environmental performance through climate impacts. Quantitative indicators selected for the assessment were net present value (NPV), internal rate of return (IRR), and global warming potential (GWP). Selected indicators have been previously used to assess the performance of similar operational models in the bioeconomy sector (el Kasmioui and Ceulemans, 2012; Mills et al., 2014; Li et al., 2017; Leppäkoski et al., 2021; Luhas et al., 2022). The results were further examined in the sensitivity analysis to assess the model’s robustness, identify the important input variables, and understand how changes in the variables would affect the results. The sensitivity analysis considers several parameters of different scenarios, including the inclusion of carbon markets, electricity price, initial investment, methane price, biochar price, district heat price, methane yield, biochar yield, and quantity of replaced district heat.
2.2.1 Cash flow analysis
The financial analysis was carried out through a cash flow analysis, which commonly involves various financial metrics such as NPV and IRR to determine the profitability and feasibility of an investment or a project. This study evaluated the financial performance of selected operational models through NPV and IRR, considering capital and operational costs, revenues, amortisation, and inflation. The NPV is a measure of the profitability of an investment, calculated by subtracting the initial investment from the present value of the expected cash flows. A positive NPV indicates that an investment is profitable, whereas a negative NPV indicates that it is not. NPV is determined using Eq. (1):
The IRR is used to determine the rate of return on an investment. It is the rate at which the NPV of an investment is equal to 0. A higher IRR indicates a more profitable investment and is often used to compare different investment opportunities. In Finland, the required average IRR for investments for publicly listed companies is 16% (Liljeblom and Vaihekoski, 2004).
Effects of inflation were considered in the study with evolving market prices according to the estimation of the common price development in Finland in 2023 (Finanssiala, 2023). Fluctuating prices in the uncertain market situation were considered regarding fertilizers, primary energy, and energy carriers due to the instable geopolitical state during the study. The increasing prices of fertilizers, primary energy, and energy carriers were estimated to stabilize after a couple of years and conform to the estimations on the common price development and of suppliers (Finanssiala, 2023; Autoala, 2023; Helen Oy, 2023; Nasdaq, 2023; The World Bank, 2023). The itemized costs involved in the analysis of the selected operational models are detailed in the Supplementary material according to 2023 prices. For the capital costs, amortisation with a 2% interest for 5 years in a 20-year plant lifetime was considered (Demichelis et al., 2020).
2.2.2 Life cycle assessment
The climate impacts were assessed considering the energy and resource consumption, the emissions and waste generation associated with the management of agricultural sidestream biomasses of a farm-scale agroecosystem. Assessment was performed considering the following functional unit (FU): treatment and utilization of annually generated sidestream biomasses of the agroecosystem (Table 2). A cradle-to-cradle system boundary is defined in Figure 2.
LCA was performed in accordance with the ISO 14040/44 framework (ISO 2006b) by using the Gabi LCA software. Study applied impact assessment method CML2001–Aug. 2016 with climate change as the midpoint impact category represented by GWP to evaluate the treatment technologies and their upgradation products. GWP accounts for all sources of GHG emissions, including biological sources and land-use changes but does not include any normalization or weighting. In this study, the GWP was assessed over a 100-year period.
2.3 Description of data
2.3.1 Sidestreams
The scope of this study considers a farm-scale agroecosystem that would be a representation of a dairy farm in Finland with an average inventory of the agricultural biomasses and inputs. The agroecosystem was assumed to consist of a dairy farm of 150 cows (European Commission, 2021), including a 140-hectare (ha) oats field (MTK, 2023) and a 3,000-m2 greenhouse. The annual quantities and compositions of the generated sidestream biomasses of manure, straw, and green waste are presented in Table 2.
Dairy farms often have adult and young animals that generate manure with a mean average of 28.6 m3 y−1 (Kaparaju and Rintala, 2011). Manure can be used as a fertilizer on farmlands to enrich soil with essential nutrients and organic matter, reducing fertilizer costs and increase soil fertility and leading to financial and environmental benefits (Tanskanen, 2017). While untreated manure has the potential to be an effective fertilizer, its use can lead to health and environmental risks. Spreading untreated manure to fields can be risky as antibiotic resistance genes have been found to be disseminated on Finnish animal farms (Ruuskanen et al., 2016; Muurinen et al., 2017). This practice can also lead to environmental issues, such as eutrophication and runoff into nearby waterways, disrupting local ecosystems and harming water quality (Luostarinen et al., 2020). Thus, use of untreated manure restricted by laws and regulations, such as a six month “closed period” for cold seasons, in some cases enforcing treatment of manure before spreading on farmlands (Liu et al., 2018; Enbuske et al., 2020). Manure can be pre-treated using composting or AD to also improve its nutrient content by providing a more balanced mix of nutrients for crops (Luostarinen et al., 2020). In Finland, composting has been the most common method for solid manure treatment, applied by nearly 4 % of all farms in 2016. In contrast, separation, aeration, or fermentation methods were relatively uncommon treatment options for manure (Natural Resources Institute Finland, 2023a).
Straw is generated as a by-product from crop harvesting. In Finland, the average crop yield of oats is around 4,000 kg ha−1 with approximately same quantity of straw (Leppäkoski et al., 2022; MTK, 2023; Natural Resources Institute Finland, 2023b). Laurila and Saarinen (2014) have estimated that half of the straw could be collected without decreasing the soil organic carbon (SOC). In Finland, straw has multiple uses but is most commonly applied as a component in animal feed and beddings, or burned for energy (Laurila and Saarinen, 2014; Weiser et al., 2014; Motiva, 2024a). Consensus has also been that leaving part of the straw on fields improves soil quality and SOC (Lafond et al., 2009; Lindorfer et al., 2014; Monforti et al., 2015; Singh et al., 2015). However, studies on the impacts from leaving straw on to the fields has shown conflicting results, particularly concerning its effects on the crop yield (Gabrielle and Gagnaire, 2008; Leppäkoski et al., 2022) due to its poor N/p value. The decomposition and burning of straw will release most of the carbon captured to the straw, which is why it would be better to be utilized in long-lasting products (Leppäkoski et al., 2022). While straw can be co-digested with biological waste to improve the methane yield of AD (Natural Resources Institute Finland, 2022), past studies also consider it as a desirable material to be pyrolyzed (Yanik et al., 2007; Huang et al., 2008; Kern et al., 2012; Lee et al., 2013). Pyrolyzing straw would stabilize its carbon into a more permanent form, after which it could be used as a soil conditioner (Lee et al., 2013; El-Adly et al., 2015).
In Finnish greenhouses, the production of tomatoes generates, on average, 16 kg m−2 of green waste (Marttila et al., 2021; Natural Resources Institute Finland, 2021) consisting of discarded products and plant waste. This study assumed this waste with the chemical properties of miscellaneous organic green waste (Natural Resources Institute Finland, 2022). Green waste from greenhouses is commonly in low quantities and composted for soil improvement purposes. However, composting large quantities of biowaste can cause significant CO2 emissions and N losses. With its high methane yield properties, green waste would be advantageous material for AD (Zhang et al., 2013).
2.3.2 Composting
Manure and green waste can be composted to reduce their volume, odors, and pathogens, as well as to produce a nutrient-rich soil amendment for agriculture to improve soil fertility and structure. In the management scenarios, composting is used to treat manure, green waste, and the SF of the digestate originating from biogas production to produce compost material for the replacement of garden soil. The main bulk material inputs of composting are peat, sand, and biotite, applied for required minerals (Havukainen et al., 2022). Operation of composting consumes also a small amount of electrical energy and light fuel oil. Composting requires investments in composting facilities, operational inputs, and personnel (described in detail in the Supplementary material). The water, ash, carbon, and volatile solids (VS) of the feedstocks were transferred to composting products, which were utilized to replace garden soil in this study. Table 3 presents the amounts of the operational inputs, the transfer coefficients to compost, and the emissions from composting and from the use of compost products.
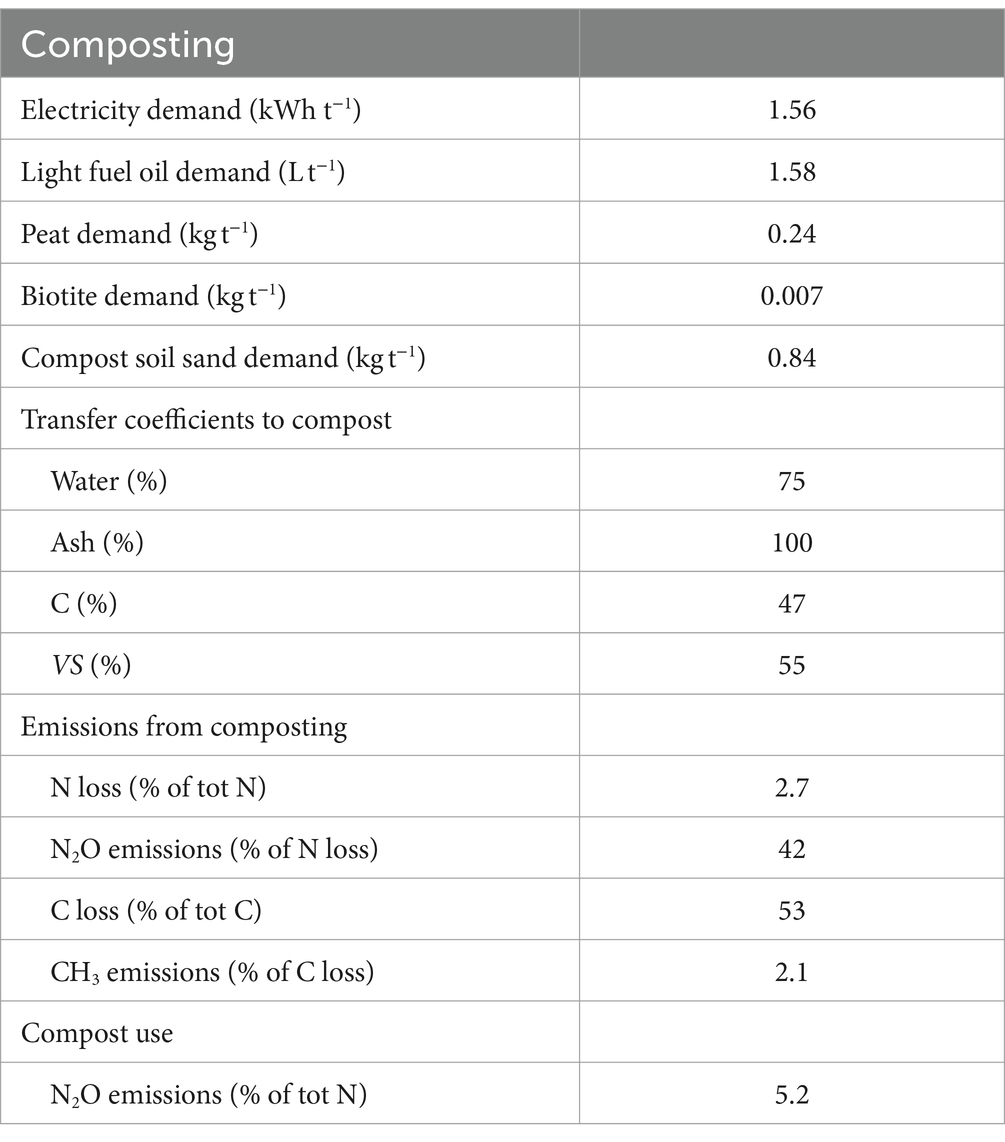
Table 3. Inventory data related to composting (Havukainen et al., 2022).
2.3.3 Anaerobic digestion
Manure and green waste can be treated in AD, where microorganisms break down organic matter in the absence of oxygen to produce biogas and digestate. Biogas can be further upgraded to biomethane. Digestate can be applied to farmlands after treatment in the hygienisation unit. The data for the AD process was gathered from Natural Resources Institute Finland’s biogas database tool (Natural Resources Institute Finland, 2022). By adjusting the digester sizes in the waste management scenarios to achieve a desired methane potential level, wet digestion with a 21 − day retention time and 4.76 kg m−3 d−1 feed rate of VS was applied for all management scenarios (Pohl et al., 2013; Natural Resources Institute Finland, 2022).
Prior to digestion, the feedstocks were diluted to 12% TS. From the produced biogas, 25% was assumed to be lost as a slip or in the burning of biogas to cover the heat demand of AD. The rest of the biogas was upgraded to methane and sold to the national gas grid (Natural Resources Institute Finland, 2022). The digester sizes, storage tank capacities, methane yield potentials, and the realized methane yields were defined according to the feedstock and process parameters presented in Table 4. Further process specifics and the financial data regarding the process are detailed in the Supplementary material.
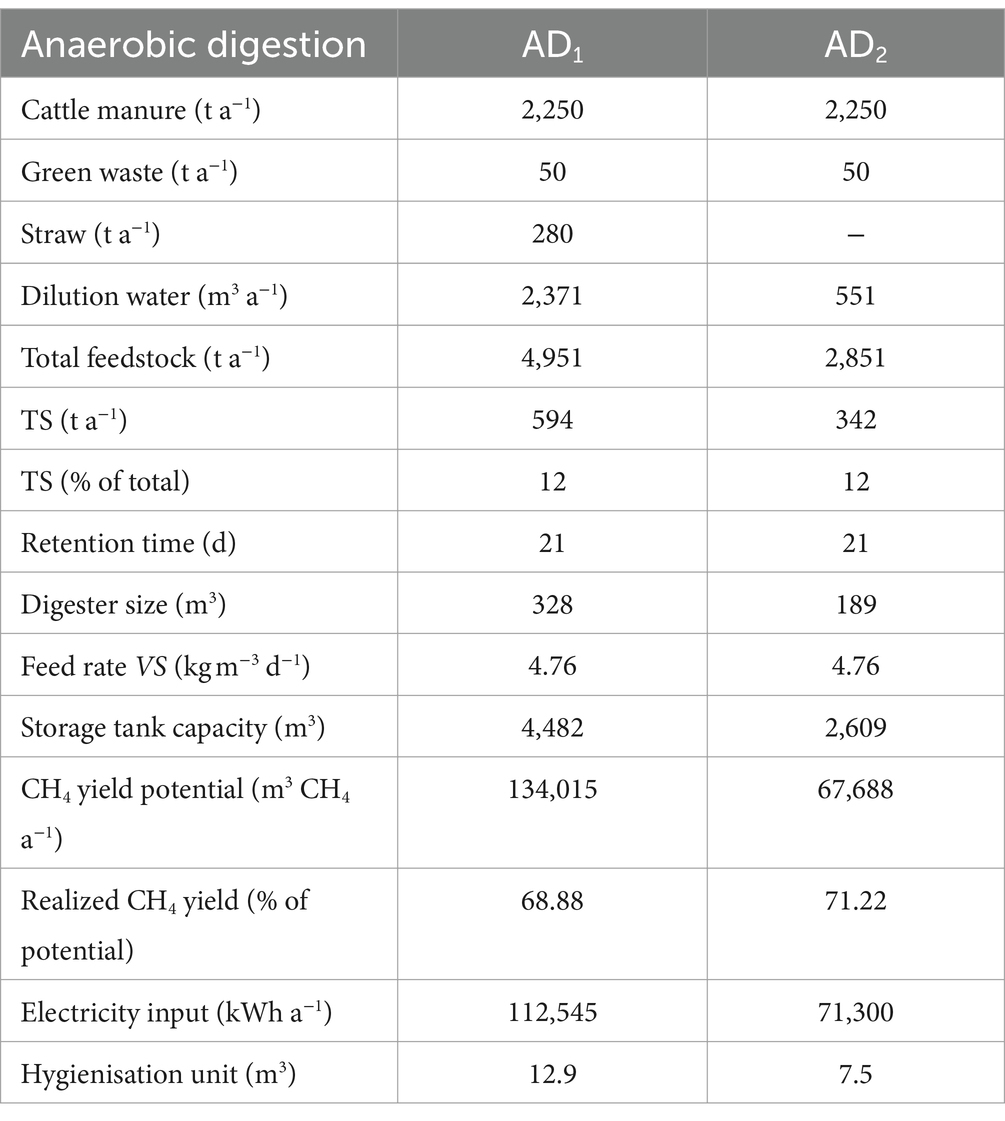
Table 4. Inventory data related to anaerobic digestion (Natural Resources Institute Finland, 2022).
Digestate quantity was higher in the AD1 scenario, where straw was digested, in comparison to AD2 scenario due to the increased amount of feedstocks and water. Decanter centrifuge, a widespread and popular technology for the separation of manure-based digestate, was used to separate the digestate into LF and SF (Al Seadi et al., 2013; Drosg et al., 2015). Separating the digestate into LF and SF improves the management of nutrients, as the high N and organic material concentrated LF can be spread on farmlands, while the P-concentrated SF can be treated by composting or pyrolysis (Czekała et al., 2017; Peltonen and Hagelberg, 2019). The LF and SF compositions are presented in Table 5. Before spreading on the fields, the LF of the digestate was sanitized in a hygienisation unit.
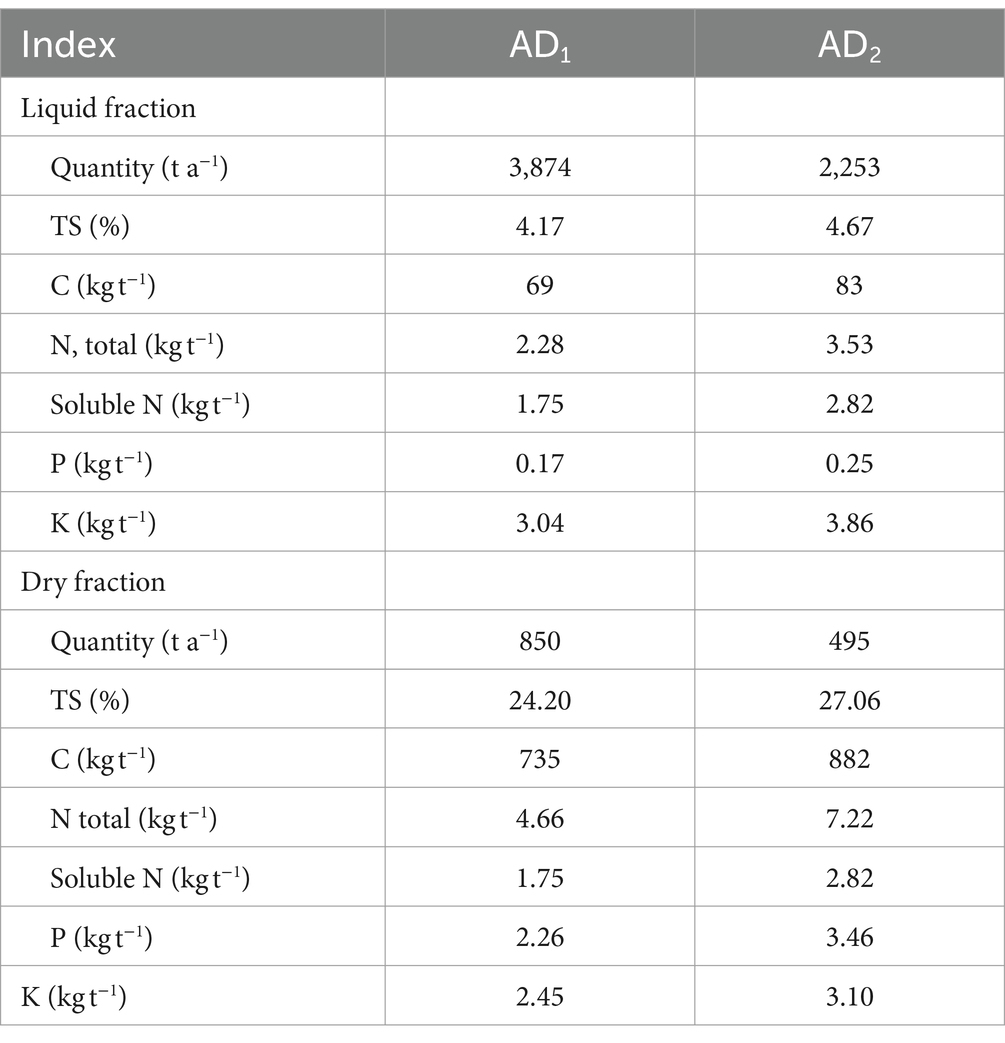
Table 5. Liquid and dry digestate fraction compositions calculated based on Al Seadi et al. (2013), Drosg et al. (2015), Natural Resources Institute Finland (2022).
2.3.4 Pyrolysis
Straw and dry digestate can be thermally treated in the PY process, leading to formation of biochar. In the treatment scenarios, PY was applied as energy self-sufficient process by utilizing other PY products as heat energy. Prior to PY, the moisture content of the SF of the digestate was reduced to 10% using a thermal dryer, which was expected to utilize the excess heat from the PY. After satisfying the heat demand for drying with the excess heat, 50% of the remaining surplus energy was estimated to replace district heating. Same approach was applied for surplus heat generated in both PY and incineration of straw. The average emission factors for district heating and electricity in Finland are 145 and 70 kgCO2 MWh−1, respectively (Motiva, 2024b).
Biochar, after mixing with the LF of the digestate, was used as a soil conditioner to store carbon into the ground. It has been assumed that after 10 years, more than 80% of carbon in the biochar can remain stable in soils (Heinonsalo, 2020). However, over longer periods biochar stability decreases. Based on a review of 34 studies (Tisserant and Cherubini, 2019), 68% of the carbon can remain in the soil after 100 years, which is applied in this study. In this study, the carbon content of biochar was averaged from three previous studies (Mašek et al., 2013; Ronsse et al., 2013; Rasa et al., 2018), resulting in a value of 72%.
Jang et al. (2018) have assessed the effects of biochar on methane production in dry dairy manure-fed AD, suggesting that relatively large quantities of biochar can improve methane yields up to 35%. In the present study, a 10% increase in the AD methane yield was assumed with 1 g L−1 addition of biochar. Pyrolyzer sizes and biochar yields were defined according to the feedstock and process parameters presented in Table 6. The financial data and more detailed specifics of the thermal dryer and PY process are further described in the Supplementary material.
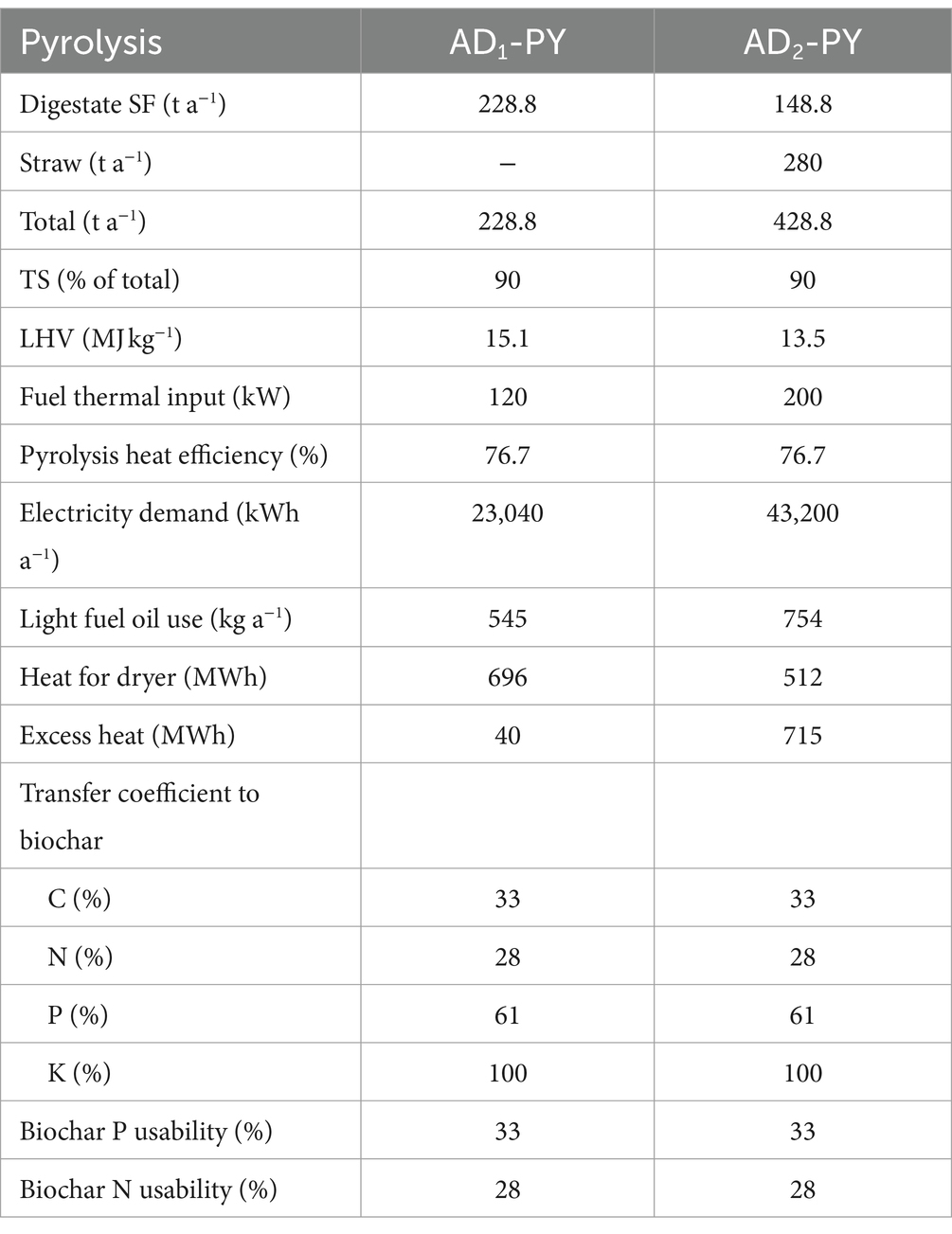
Table 6. Inventory data related to pyrolysis (Kern et al., 2012; Li and Feng, 2018; Leppäkoski et al., 2021; Havukainen et al., 2022).
2.3.5 Field application
In the scenarios, mineral fertilizers were replaced with manure, digestate, and biochar. Applying nutrients to farmlands is limited based on their N and P doses. For oats, the optimum dose of N is close to 10 t km−2 annually (Chalmers et al., 1998; Gonzales Ponce and Santin, 2001; Mohr et al., 2007). With the high P concentration limiting the use of applicable fertilizer, manure fertilization can leave N doses short (Peltonen and Hagelberg, 2019). The usage of P in farming is monitored and limited by the Finnish Food Authority (2023). The P application rate has been set to a maximum of 1.4 t km−2 y−1 for cereals by the Finnish Agri-Environmental Program (Amery and Schoumans, 2014; Finnish Food Authority, 2023).
Spreading fertilizer replacements on farmlands requires investments in manure collection, storage, and spreading equipment and machinery. In this study, storage facilities are fit to store annually produced manure or digestate quantity (Enbuske et al., 2020). Investments in the machinery required for spreading fertilizer replacements were excluded from this study, as it was a similar process in all the studied scenarios. By applying the replacements to farmlands, the use of NPK-fertilizers, namely urea, diammonium phosphate (DAP), and potassium chloride, was reduced. IPCC (2006) guidelines report N2O emissions from all fertilizers at 1%. However, in a review of 48 studies on N2O emission factors following the application of N-fertilizers, Walling and Vaneeckhaute (2020) found values ranging from as low as 0.03% to as high as 14%. Bruun et al. (2006) have modelled the N-N2O formation from total N to be around 1.8% in land use of compost and digestated municipal solid waste. This emission factor is applied in this study for all N in field spreading. Bruun et al. (2006) also estimated that carbon losses of compost and digestate can reach over 90% of total carbon 100 years after application on fields. Considering the modest carbon stability of compost, manure, and digestate, and the great uncertainty in soil carbon persistence (Heinonsalo, 2020), their carbon sequestration potential is excluded from this study.
3 Results
The scenarios for the utilization of manure, straw, and green waste were examined from a financial and climate impact perspective, and the results are presented in Table 7; Figures 3, 4. The results are described in detail in the following subsections.
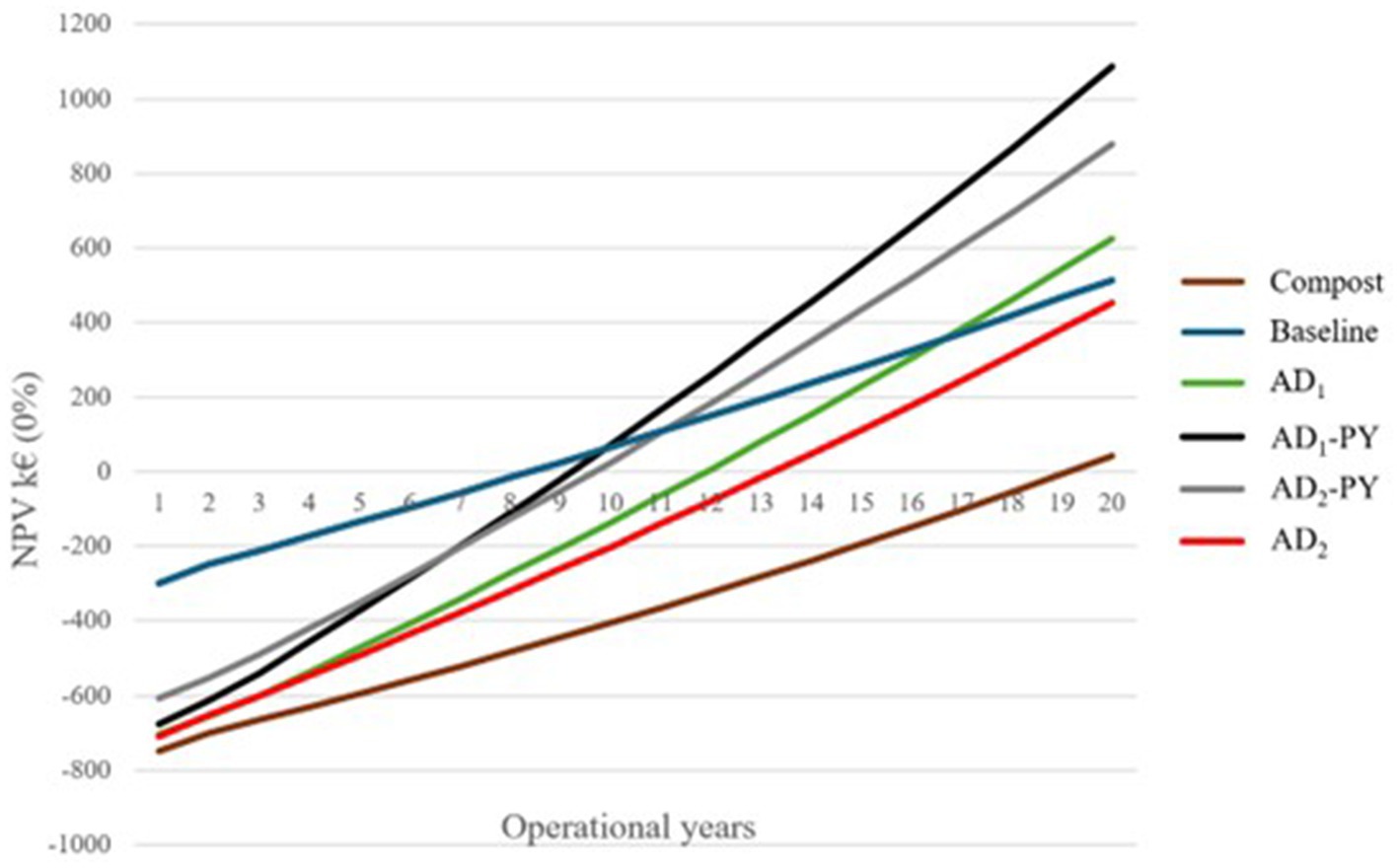
Figure 3. Accumulated cash flows without discounting for the assessed scenarios on 20-year-lifetime.
3.1 Net present value and internal rate of return
The progression of cash flow, NPV, and IRR for the treatment and utilization of agricultural sidestream biomasses are presented in Figure 3; Table 7. Baseline scenario outperformed other scenarios in financial performance based on its eight-year payback period. The baseline scenario NPV value varied between 521,734 € and − 74,862 €, with interest rates from 0 to 20%, and presented an IRR of 12.90%. As burning the straw for energy and spreading manure to farmlands without treatment can be accomplished with significantly lower investment and operational costs than AD or PY, incomes from replaced district heat and nutrients are enough to make the scenario financially attractive. Small quantities of compostable matter also obviate the demand for large investments in composting treatment. The weak financial performance of the compost scenario was presented by an IRR of 0.53% and the variation of NPV ranging from 42,514 € to −464,417 € (interest rate between 0 and 20%), demonstrating how the low return of investment of composting counterbalances the financial benefits of spreading untreated manure to farmlands.
The AD1 and AD2 scenarios presented no financial advantage, indicated by the relatively high payback periods, 12 and 13 years, respectively, due to high investment costs compared to income. These scenarios have the modest feasibility after composting, suggested by IRRs of 6.80% for AD1 and 5.21% for AD2. The NPVs ranged from 625,543 € to −333,847 € for AD1, and from 451,659 € to −361,035 € for AD2 within 0 and 20% interest rates. The feasibility of the AD process can be improved with high carbon concentrated lignocellulosic feedstock, as presented in AD2, but this operational option falls financially short compared to AD1 due to savings achieved by burning straw to replace district heating.
The co-application of AD with PY increases the feasibility of the treatment because of the high market price of biochar, improved biomethane yield, and obviation of composting treatment. These were actualized by payback periods of 9 and 9.5 years and IRRs of 11.03 and 10.17% in scenarios AD1-PY and AD2-PY, respectively. These scenarios presented NPVs between 1,085,721 € and − 230,808 € for AD1-PY, and between 877,373 € and − 223,961 € for AD2-PY within 0 and 20% interest rates, respectively, presenting improvement in the profitability compared to using AD alone.
3.2 Global warming potential
Most of the climate impacts within the examined waste management scenarios occurred with the application of nutrient-rich manure, digestate, or compost on farmlands as presented in Figure 4. Emissions are released in composting, straw burning, AD, and PY processes, and avoided by replacing district heat and natural gas with bioenergy, soil material with compost soil, and mineral fertilizers with organic fertilization. Along avoided emissions, carbon sequestration was established by storing biochar carbon to ground.
The co-application of AD and PY, presented by AD1-PY and AD2-PY, indicating the closely to carbon neutral operational models; they had the lowest GWPs of 21,636 kgCO2eq. FU−1 and − 4,122 kgCO2eq. FU−1, respectively. The low GWP of these scenarios is due to carbon sequestration with PY biochar, improved biomethane yields, and reduced quantities of feedstock composted and spread to farmlands. By burning straw in PY instead of digesting, as in AD2 − PY, the amount of carbon sequestered to farmlands in the form of biochar was increased.
The higher GWP of AD1, 95,610 kgCO2eq. FU−1, and lower GWP of AD2, 36,900 kgCO2eq. FU−1, are indicating that in this study, replacing district heat by burning straw leads to lower emissions rather than digesting it. Burning straw instead of digesting reduces the amount of compostable digestate and increases the amount of heat energy that can replace district heating. Digesting straw does improve the methane yield of AD; however, required composting of the increased amount of digestate neutralizes any benefits it brings.
Based on their climate impacts, the baseline and composting scenarios were found to be poor treatment methods, suggested by the GWPs of 76,307 kgCO2eq. FU−1 and 104,513 kgCO2eq. FU−1, respectively. These findings emphasize the importance of establishing carbon sinks through biochar application as soil conditioner and replacing high emission-intensive fossil fuels with renewable alternatives.
3.3 Parameter sensitivity
The results from financial and climate impact assessment are further analyzed in terms of most significant parameters. Table 8 presents an absolute change of the results, expressed in terms of NPV (0%) in k€ and GWP in tCO2eq FU−1 with +25% changes of the parameter value applied. For example, when the electricity price increases by 25%, the NPV (0%) of the AD1 scenario decreased by 35.7 k€, whereas in the compost scenario, the effect on the NPV (0%) is minimal. Setting a carbon market price differs from other parameter changes. Here, the price for GHG emissions is set to 100 € tCO2eq.−1 (Luhas et al., 2022), which increases the NPV (0%) for the AD2-PY scenario but decreases it for other scenarios.
The sensitivity analysis revealed that the parameters presenting the most significant sensitivity for the NPV (0%) results were initial investment, methane price, and biochar price. Electricity price fluctuations do not present remarkable changes in the results of the waste management scenarios. The results of the financial analyses showed a remarkable sensitivity to fluctuations in biochar and methane prices, whereas district heat prices present low sensitivity. GWP results are highly sensitive to changes in the methane yield and amount of district heat replaced. Methane yield fluctuation causes the most significant change in the AD1 and AD1-PY scenarios, whereas the baseline, compost, and AD2 scenarios were most sensitive to changes in the amount of replaced district heating. The AD2-PY scenario presents no remarkable sensitivity for any certain parameter in terms of GWP.
4 Discussion
The current study presents that the co-application of AD and PY effectively reduces the climate impacts of agricultural operations as a treatment option of sidestreams of straw, manure, and green waste compared to alternative operational practices. However, the financial performance of the co-application indicated a longer payback period than the baseline option of spreading untreated manure on fields. To our knowledge, this is the first report of a simultaneous financial and environmental assessment of the co-application of AD and PY against competing technologies in the agricultural context. Through cash flow analysis and LCA, we show that the co-application could offer a low-emission waste management solution for agriculture.
In terms of sidestream management, the results suggest that the digestion of straw generates slightly higher profitability compared to being burned for energy. However, by replacing fossil energy with burning straw, the climate impacts can be decreased tremendously. Additionally, it was observed that utilizing biochar in AD decreases the system-level emissions by improving methane yields but slightly decreases the overall financial performance due to lost profits from high biochar price. The results confirm that complementing AD with PY reduces the climate impacts of agricultural activities in a reasonably profitable way while improving the product portfolio in comparison to business-as-usual practices and use of single AD. Therefore, for farms already utilizing AD for treatment of agricultural sidestreams, investing in PY technology seems highly beneficial. It is worth noting that the sensitivity analysis presented greatest uncertainty for the profitability of co-application scenarios in terms of high investment costs and fluctuation in the methane price, which were less critical for the baseline scenario.
Figure 5 visualizes the scales of financial and climate impacts of different waste management strategies assessed in this study. The further on the right on the scale and the larger the bubble, the better the profitability of treatment option in terms of NPV and IRR. The lower on the y-axis, the desirable the climate impacts. Even though the baseline scenario stands out in the comparison in terms of IRR, the co-application of AD and PY is displayed as a favourable treatment option when prioritizing both financial and environmental goals. In comparison to baseline, the non-discounted NPV of the co-application scenarios was higher, indicating a positive signal for the financial attractiveness of the investment at low discount rates. The results of the financial analysis are in line with Mills et al. (2014), who assessed the co-application of AD and PY in treatment of wastewater sludge. They reported a slightly lower profitability (IRR 7.64%) for AD-PY than this study, which can be explained by their exclusion of biochar utilization. This indicates that the co-application of AD and PY could present a promising option for farmers looking to reduce or even neutralize their climate impacts without compromising excessively on profitability. However, this solution would require financial support or further development for entrepreneurs to adopt the technology.
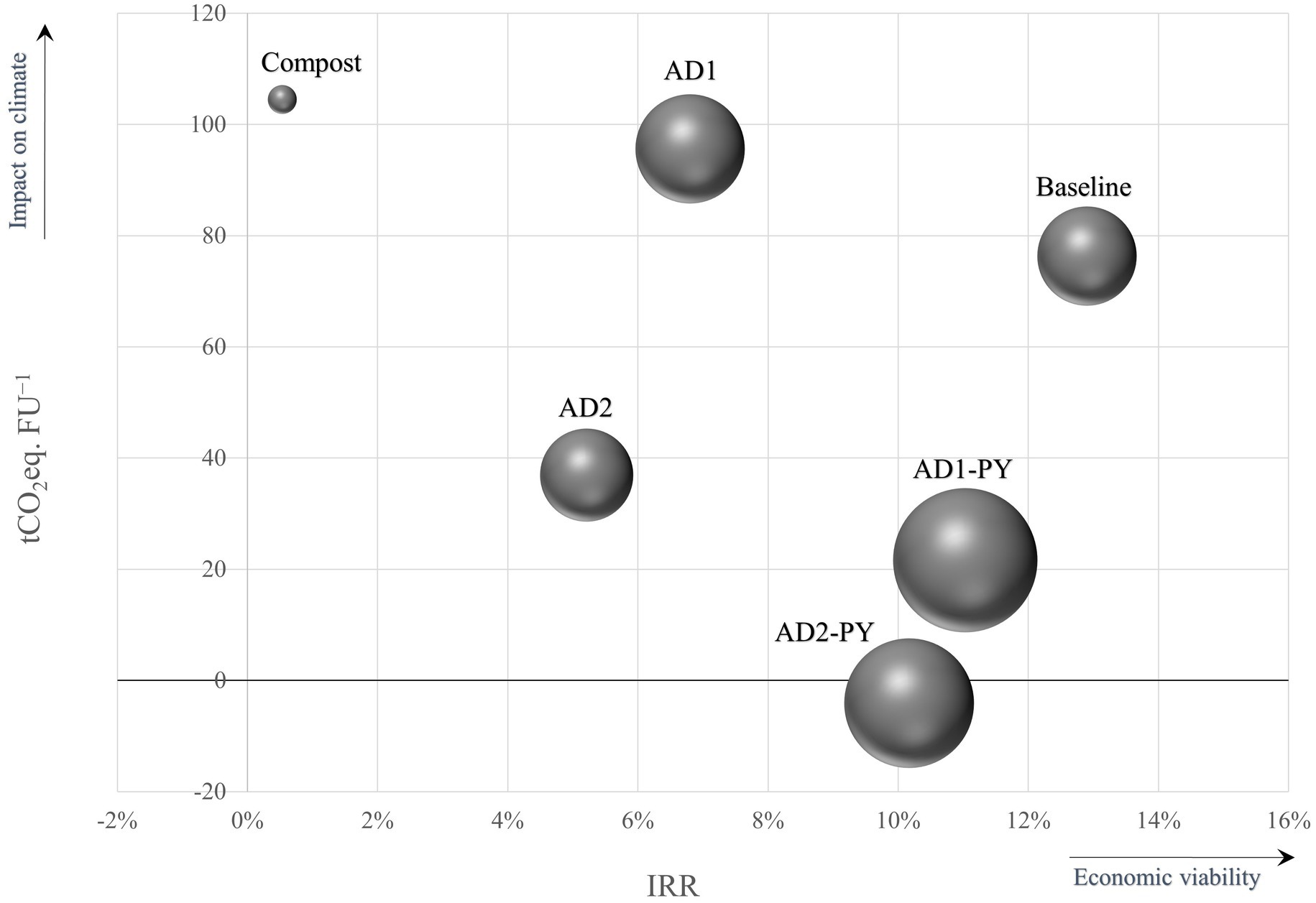
Figure 5. Waste management scenarios were compared according to based on economic viability and impact on climate.
The absolute values regarding climate impacts are challenging to compare against previous studies, especially due to the different type of FUs and system boundaries applied. Most of the related LCA studies define the FU based on kg or tonne of treated waste (Mills et al., 2014; Wang et al., 2021; Caiardi et al., 2022; Havukainen et al., 2022). In this study, the FU was set for the annual management of the sidestreams in the agroecosystem, enabling assessment of financial and climate impacts within the same FU. The FU of annual management of the sidestreams was selected due to the systemic approach that also differentiates from earlier studies. For example, Caiardi et al. (2022) did not include the N2O emissions from the field application of nutrients, and Wang et al. (2021) did not treat the LF of the digestate, resulting in lower GWP compared to in this study. Regardless of the absolute values, the interpreted results obtained here are consistent with those reported in earlier literature that assessed the GWP of AD and PY in treatment of different types of organic wastes. Similarly to the present study, coupling AD and PY processes was found to be a significantly favorable waste treatment option compared to single AD usage (Mills et al., 2014; Wang et al., 2021) or performing at least at a similar level (Opatokun et al., 2017; Caiardi et al., 2022).
The data used in the study were based on peer-reviewed publications and publicly available reports. The performance of the co-application of AD and PY can be scaled for larger systems, and the study results are reasonably applicable in other geographic locations, after considering the study limitations. However, the reliability of the results was affected by geopolitical instability, which had caused fluctuating prices of energy and fertilizers, making them difficult to predict for the future (Autoala, 2023; Helen Oy, 2023; Nasdaq, 2023; The World Bank, 2023). With changing energy prices, the profitability of the AD and PY pathways could be affected significantly. Similarly, changes in biochar price and inclusion of carbon markets could influence the results notably, as presented in Table 8. Therefore, the solution also presents potential as a financially viable strategy in a future where GHG emissions are more strongly tied to economic activities, for instance through carbon markets.
The methane production potential of straw differentiates greatly with some studies presenting typical potentials between 224- and 278-ml g−1 VS (Contreras et al., 2012; Ferreira et al., 2013; Sapci, 2013; Ferreira et al., 2014). The methane potential can vary significantly between the feedstock, reaching potentials as low as 162- and high as 336-ml g−1 VS (Dai et al., 2020). Dilution of straw has been shown to slightly improve the methane production from straw due to reduced solids concentration (Ferreira et al., 2014). As straw was not digested separately in the present study, the methane potential of 280 mL g−1 VS defined by Lei et al. (2010) for straw with anaerobic sludge was applied here. Studies also recommend heat pretreatment of straw to reach optimal methane yields in the digester (Ferreira et al., 2013, 2014). With lower straw methane yields the AD1 scenario would we notably worsen in terms of climate and financial performance. There is some uncertainty related to straw with its availability. The collection potential of straw varies regionally and is constrained by competition with other uses (Scarlat et al., 2010). The collection potential is affected by the uncertainty regarding soil improvement benefits from leaving straw in the field. Attempts to maintain SOC could also limit the collection potential of straw (Johnson et al., 2010).
The exclusion construction of machinery from the LCA with the assumptions made regarding the use of excess heat from the PY process can cause some level of uncertainty for the results (Leppäkoski et al., 2021; Marttila et al., 2021). In this study, the exclusion of transportation machinery from the financial analysis was justified based on the assumption that the spreading of manure and digestate would include the same investments in all the scenarios. Transportation of goods also found to have minimal environmental impacts in related LCA studies (Leppäkoski et al., 2021; Marttila et al., 2021; Luhas et al., 2022) but could weaken the profitability of the treatment options, especially in smaller scale applications. The study results were partly sensitive to the assumption made regarding district heat (Table 8). In this study, a thermal dryer was assumed to utilize excess heat from the PY, with 50% of remaining heat used to replace district heat. With increased heat demand of the dryer, the climate impacts of the PY scenarios could increase as less excess heat would be available to replace district heating. The present study did not consider the N2O emissions from storaging of the digestion prior to spreading on fields which could have altered the climate impacts of treatment options to some extent.
Co-application of AD and PY helps farmers reduce their resource dependence on imported goods such as fossil energy, while diversifying their revenue streams and thereby alleviating levels of uncertainty related to financial profitability. By generating additional income from the production of biochar, biogas, and organic fertilizers, the co-application of AD and PY can increase resilience in the market by minimizing vulnerability to input price fluctuations. This highlights the importance of also considering qualitative indicators such as security of supply when evaluating the best approaches for managing agricultural sidestreams in future research. Moreover, the trade-offs between different treatment options in terms of multiple environmental indicators and consideration of the co-application of PY and AD as a carbon sequestration method in carbon markets should be assessed. Some of the techno-economic benefits of the co-application of AD and PY, recognized in earlier literature (Pan et al., 2019; Song et al., 2021; Tayibi et al., 2021), were not considered in this study. Although immediate improvement in the profitability against the field application of manure was not documented in this study, by considering potential for reduced lag phase, healthier functioning of AD, or utilization of AD excess heat, could result in more financially competitive operational models, which could be addressed in future research.
While spreading untreated manure on fields presented a financial advantage, this solution has limitations, such as local legislation related to high pathogen, heavy metal, or organic pollutant levels of manure (Ruuskanen et al., 2016; Muurinen et al., 2017; Liu et al., 2018; Enbuske et al., 2020). Additionally, poorly treated manure can reduce the N reserves of the soil and weaken its fertilizing effect (Myllymäki et al., 2014). Finland’s sparse population also concentrates local livestock production heavily in certain areas, which can lead to some locational abundance of manure fertilizer (Peltonen and Hagelberg, 2019). If the amount of generated manure on a farmland is too large to use locally, farmers may have to deal with additional transportation costs to utilize the excess manure in other locations. Applying manure fertilizing serves solely the fertilization purpose, making the business-as-usual model more vulnerable to market fluctuations and stricter legislation, to which operational models with more diverse product portfolios would not be as strongly exposed to.
For the agricultural and other rural entrepreneurs, this research demonstrates the potential to achieve reductions in their climate impacts and reduce dependency on imported goods and energy by investing in the co-application of AD and PY technology. The authors, on the other hand, acknowledge that adoption of these technologies may pose challenges for individual farmers, particularly in terms of initial investment costs and technical expertise. Therefore, further education of farmers on the benefits of the co-application and on how to implement this approach effectively is necessary for its successful operation. Acknowledging the increasing concerns related to rising levels of GHG emissions, policy makers should consider strengthening governmental support mechanisms for implementing sustainable technologies that would increase domestic carbon sinks. This study highlights the importance of supportive legislation through financing options and tools, such as tax incentives and low-interest loans, as carbon market prices were shown to significantly affect the financial performances of different treatment options.
5 Conclusion
This study examined the financial and climate impacts of the co-application of AD and PY in the management of agricultural sidestreams. The results present that although this solution might not be financially most profitable for the entrepreneur, it presents potential as an effective way for agricultural operators to reduce their emissions while increasing their product portfolio and revenue streams. Establishment of AD-PY solutions instead of a single AD is estimated to increase profitability and reduce emissions. In addition, two trade-offs between financial and climate impacts were recognized; digestating straw in this system leads to improved profitability but incinerating it for heat energy would increase emissions within the system. On contrast, addition of biochar to AD process decreases the profits but lessens the impact on climate.
It is recognized that holistic assessments considering financial and environmental implications of technologies and solutions are required for recognizing best practices for sustainable low-carbon economy. Although co-application of AD and PY is not yet the most profitable solution on the market, it presents potential for cost-effective reduction of GHG emissions for the future where suitable legislation or technological development has taken place. Overall, the insights in this paper could guide decision-making processes for individual entrepreneurs and policy-makers in the agricultural sector, aligning sustainability and financial viability in operational practices and policies guiding them. To conclude, the co-application of AD and PY aligns with the principles of a circular bioeconomy by closing the loops of waste streams, thereby supporting in providing solutions for sustainable operational models for rural waste management.
Data availability statement
The original contributions presented in the study are included in the article/Supplementary material, further inquiries can be directed to the corresponding author.
Author contributions
MMa: Conceptualization, Data curation, Formal analysis, Investigation, Methodology, Software, Validation, Visualization, Writing – original draft, Writing – review & editing. JH: Conceptualization, Data curation, Investigation, Methodology, Writing – review & editing. VU: Conceptualization, Methodology, Supervision, Writing – review & editing. LL: Conceptualization, Supervision, Validation, Writing – review & editing, Project administration. MMi: Conceptualization, Funding acquisition, Methodology, Project administration, Supervision, Validation, Writing – review & editing.
Funding
The author(s) declare that financial support was received for the research, authorship, and/or publication of this article. This work was supported by the project Hämeen ilmastoviisas maaseutu- ja energiayrittäjyys (HIME) by the Lappeenranta-Lahti University of Technology, ProAgria, and the Finnish Forest Centre. The project was funded by the European Agricultural Fund for Rural Development. In addition, this work received funding from the Neles Oy 30 Years Foundation.
Conflict of interest
The authors declare that the research was conducted in the absence of any commercial or financial relationships that could be construed as a potential conflict of interest.
Publisher’s note
All claims expressed in this article are solely those of the authors and do not necessarily represent those of their affiliated organizations, or those of the publisher, the editors and the reviewers. Any product that may be evaluated in this article, or claim that may be made by its manufacturer, is not guaranteed or endorsed by the publisher.
Supplementary material
The Supplementary material for this article can be found online at: https://www.frontiersin.org/articles/10.3389/fsufs.2024.1426890/full#supplementary-material
References
Al Seadi, T., Drosg, B., Fuchs, W., Rutz, D., and Janssen, R. (2013). Biogas digestate quality and utilization, in: the biogas handbook: science. Product Appl. 267–301. doi: 10.1533/9780857097415.2.267
Amery, F., and Schoumans, O.. Agricultural phosphorus legislation in Europe (2014). Available at: https://edepot.wur.nl/300160
Autoala . The price development of gasoline and diesel 2023 [internet]. The information Center for the Automotive Industry (2023). Available at: https://www.aut.fi/tilastot/verotus_hintakehitys_ja_liikennemenot/bensiinin_ja_dieselin_hintakehitys
Bruun, S., Hansen, T. L., Christensen, T. H., Magid, J., and Jensen, L. S. (2006). Application of processed organic municipal solid waste on agricultural land–a scenario analysis. Environ. Model. Assess. 11, 251–265. doi: 10.1007/s10666-005-9028-0
Caiardi, F., Belaud, J. P., Vialle, C., Monlau, F., Tayibi, S., Barakat, A., et al. (2022). Waste-to-energy innovative system: assessment of integrating anaerobic digestion and pyrolysis technologies. Sustain. Prod. Consum. 31, 657–669. doi: 10.1016/j.spc.2022.03.021
Chalmers, A. G., Dyer, C. J., and Sylvester-Bradley, R. (1998). Effects of nitrogen fertilizer on the grain yield and quality of winter oats. J. Agric. Sci. 131, 395–407. doi: 10.1017/S0021859698006042
Chen, Q., and Liu, T. (2017). Biogas system in rural China: upgrading from decentralized to centralized? Renew. Sust. Energ. Rev. 78, 933–944. doi: 10.1016/j.rser.2017.04.113
Contreras, L. M., Schelle, H., Sebrango, C. R., and Pereda, I. (2012). Methane potential and biodegradability of rice straw, rice husk and rice residues from the drying process. Water Sci. Technol. 65, 1142–1149. doi: 10.2166/wst.2012.951
Crippa, M., Solazzo, E., Guizzardi, D., Monforti-Ferrario, F., Tubiello, F. N., and Leip, A. (2021). Food systems are responsible for a third of global anthropogenic GHG emissions. Nat. Food 2, 198–209. doi: 10.1038/s43016-021-00225-9
Czekała, W., Dach, J., Dong, R., Janczak, D., Malińska, K., Jóźwiakowski, K., et al. (2017). Composting potential of the solid fraction of digested pulp produced by a biogas plant. Biosyst. Eng. 160, 25–29. doi: 10.1016/j.biosystemseng.2017.05.003
Dai, X., Hua, Y., Liu, R., Chen, S., Li, H., and Dai, L. (2020). Biomethane production by typical straw anaerobic digestion: Deep insights of material compositions and surface properties. Bioresour. Technol 313:123643. doi: 10.1016/j.biortech.2020.123643
Demichelis, F., Laghezza, M., Chiappero, M., and Fiore, S. (2020). Technical, economic and environmental assessment of bioethanol biorefinery from waste biomass. J. Clean. Prod. 277:124111. doi: 10.1016/j.jclepro.2020.124111
Drosg, B., Fuchs, W., Seadi, T., Al, M. M., and Linke, B. (2015). Nutrient recovery by biogas Digestate processing. Brazil: IEA Bioenergy.
el Kasmioui, O., and Ceulemans, R. (2012). Financial analysis of the cultivation of poplar and willow for bioenergy. Biomass Bioenergy 43, 52–64. doi: 10.1016/j.biombioe.2012.04.006
El-Adly, R. A., Adel, Y. M., Modather, H. F., Ismail, E. A. M., and Abbas, D. (2015). Biogrease based on Biochar from Rice straw and waste cooking oil. Int. J. Adv. Pharm. Biol. Chem. 4:91.
Enbuske, T., Ollila, A., Pesonen, O., Ranta, A., and Vinblad, S.. Mäntyvaaran hevoskeskittymä kiertotalouden silmin [Internet]. (2020). Available at: https://iwwebhallinta.ulapland.fi:8443/loader.aspx?id=67fe15f9-6153-4047-b297-d03a6a0c70c6
European Commission . EU dairy farms report [internet]. (2021). Available at: https://agriculture.ec.europa.eu/system/files/2022-08/fadn-dairy-report-2021_en.pdf
Fabbri, D., and Torri, C. (2016). Linking pyrolysis and anaerobic digestion (Py-AD) for the conversion of lignocellulosic biomass. Curr. Opin. Biotechnol. 38, 167–173. doi: 10.1016/j.copbio.2016.02.004
Ferreira, L. C., Donoso-Bravo, A., Nilsen, P. J., Fdz-Polanco, F., and Pérez-Elvira, S. I. (2013). Influence of thermal pretreatment on the biochemical methane potential of wheat straw. Bioresour. Technol. 143, 251–257. doi: 10.1016/j.biortech.2013.05.065
Ferreira, L. C., Nilsen, P. J., Fdz-Polanco, F., and Pérez-Elvira, S. I. (2014). Biomethane potential of wheat straw: influence of particle size, water impregnation and thermal hydrolysis. Chem. Eng. J. 242, 254–259. doi: 10.1016/j.cej.2013.08.041
Finanssiala . The latest economic forecasts concerning Finland 2022 [Internet]. (2023). Available at: https://www.finanssiala.fi/wp-content/uploads/2023/02/fa-tilasto-talousennusteet.pdf
Finnish Food Authority . Guide to conditionality 2023 [internet]. (2023). Available at: https://www.ruokavirasto.fi/tuet/maatalous/peltotuet/ymparistokorvaus/.
Gabrielle, B., and Gagnaire, N. (2008). Life-cycle assessment of straw use in bio-ethanol production: a case study based on biophysical modelling. Biomass Bioenergy 32, 431–441. doi: 10.1016/j.biombioe.2007.10.017
Ghysels, S., Acosta, N., Estrada, A., Pala, M., de Vrieze, J., Ronsse, F., et al. (2020). Integrating anaerobic digestion and slow pyrolysis improves the product portfolio of a cocoa waste biorefinery. Sustain Energy Fuels 4, 3712–3725. doi: 10.1039/d0se00689k
Gonzales Ponce, R., and Santin, I. (2001). Competitive ability of wheat cultivars with wild oats depending on nitrogen fertilization. Agronomie 21, 119–125. doi: 10.1051/agro:2001112
González-Arias, J., Fernández, C., Rosas, J. G., Bernal, M. P., Clemente, R., Sánchez, M. E., et al. (2020). Integrating anaerobic digestion of pig slurry and thermal valorisation of biomass. Waste Biomass Valorization 11, 6125–6137. doi: 10.1007/s12649-019-00873-w
Gunaseelan, V. N. (2004). Biochemical methane potential of fruits and vegetable solid waste feedstocks. Biomass Bioenergy 26, 389–399. doi: 10.1016/j.biombioe.2003.08.006
Havukainen, J., Saud, A., Astrup, T. F., Peltola, P., and Horttanainen, M. (2022). Environmental performance of dewatered sewage sludge digestate utilization based on life cycle assessment. Waste Manag. 137, 210–221. doi: 10.1016/j.wasman.2021.11.005
Heinonsalo, J. (2020). Carbon guide - an overview of soil carbon and the basics of carbon farming. Kaarina: Paino-Kaarina.
Helen Oy . The prices of district heating 2023 [internet]. (2023) Available at: https://www.helen.fi/lammitys-ja-jaahdytys/kaukolampo/hinnat#kaukolampo-uuteen-taloon
Huang, Y. F., Kuan, W. H., Lo, S. L., and Lin, C. F. (2008). Total recovery of resources and energy from rice straw using microwave-induced pyrolysis. Bioresour. Technol. 99, 8252–8258. doi: 10.1016/j.biortech.2008.03.026
Huttunen, M., Kuittinen, V., and Lampinen, A., (2018). Finnish national biogas statistics. Data year 2017. Publications of the University of Eastern Finland. Reports and studies in forestry and natural sciences, N:o 33.
Indren, M., Birzer, C. H., Kidd, S. P., Hall, T., and Medwell, P. R. (2020). Effects of biochar parent material and microbial pre-loading in biochar-amended high-solids anaerobic digestion. Bioresour. Technol. 298:122457. doi: 10.1016/j.biortech.2019.122457
IPCC (2006). Guidelines for National Greenhouse gas Inventories Volume 4: agriculture, forestry, and other Landuse, vol. 2006. Paris: OECD Press.
ISO (2006a) ISO 14040:2006. Environmental management — life cycle assessment — principles and framework. Available at: https://www.iso.org/standard/37456.html
ISO (2006b) ISO 14044:2006. Environmental management — Life cycle assessment — Requirements and guidelines. Available at: https://www.iso.org/standard/38498.html
Jang, H. M., Choi, Y. K., and Kan, E. (2018). Effects of dairy manure-derived biochar on psychrophilic, mesophilic and thermophilic anaerobic digestions of dairy manure. Bioresour. Technol. 250, 927–931. doi: 10.1016/j.biortech.2017.11.074
Johnson, J. M. F., Karlen, D. L., and Andrews, S. S. (2010). Conservation considerations for sustainable bioenergy feedstock production: if, what, where, and how much? J. Soil Water Conserv. 65, 88A–91A. doi: 10.2489/jswc.65.4.88A
Kalu, S., Kulmala, L., Zrim, J., Peltokangas, K., Tammeorg, P., Rasa, K., et al. (2022). Potential of biochar to reduce greenhouse gas emissions and increase nitrogen use efficiency in boreal arable soils in the long-term. Front. Environ. Sci. 10:914766. doi: 10.3389/fenvs.2022.914766
Kaparaju, P., and Rintala, J. (2011). Mitigation of greenhouse gas emissions by adopting anaerobic digestion technology on dairy, sow and pig farms in Finland. Renew. Energy 36, 31–41. doi: 10.1016/j.renene.2010.05.016
Kaur, G., Johnravindar, D., and Wong, J. W. C. (2020). Enhanced volatile fatty acid degradation and methane production efficiency by biochar addition in food waste-sludge co-digestion: a step towards increased organic loading efficiency in co-digestion. Bioresour. Technol. 308:123250. doi: 10.1016/j.biortech.2020.123250
Kavitha, B., Reddy, P. V. L., Kim, B., Lee, S. S., Pandey, S. K., and Kim, K. H. (2018). Benefits and limitations of biochar amendment in agricultural soils: a review. J. Environ. Manag. 227, 146–154. doi: 10.1016/j.jenvman.2018.08.082
Kern, S., Halwachs, M., Kampichler, G., Pfeifer, C., Pröll, T., and Hofbauer, H. (2012). Rotary kiln pyrolysis of straw and fermentation residues in a 3 MW pilot plant - influence of pyrolysis temperature on pyrolysis product performance. J. Anal. Appl. Pyrolysis 97, 1–10. doi: 10.1016/j.jaap.2012.05.006
Khoshnevisan, B., Duan, N., Tsapekos, P., Awasthi, M. K., Liu, Z., Mohammadi, A., et al. (2021). A critical review on livestock manure biorefinery technologies: sustainability, challenges, and future perspectives. Renew. Sust. Energ. Rev. 135:110033. doi: 10.1016/j.rser.2020.110033
Lafond, G. P., Stumborg, M., Lemke, R., May, W. E., Holzapfel, C. B., and Campbell, C. A. (2009). Quantifying straw removal through baling and measuring the long-term impact on soil quality and wheat production. Agron. J. 101, 529–537. doi: 10.2134/agronj2008.0118x
Latvala, T., Niemi, J., and Väre, M.. Finnish Agri-food sector outlook [internet] (2020). Available at: https://jukuri.luke.fi/handle/10024/546642
Laurila, J., and Saarinen, J. (2014). Harvesting of agricultural biomass and its environmental impacts – Focusing on the regions of Southwest Finland and Satakunta [internet]. Huittinen: Satafood Kehittämisyhdistys ry.
Lee, Y., Park, J., Ryu, C., Gang, K. S., Yang, W., Park, Y. K., et al. (2013). Comparison of biochar properties from biomass residues produced by slow pyrolysis at 500°C. Bioresour. Technol. 148, 196–201. doi: 10.1016/j.biortech.2013.08.135
Lei, Z., Chen, J., Zhang, Z., and Sugiura, N. (2010). Methane production from rice straw with acclimated anaerobic sludge: effect of phosphate supplementation. Bioresour. Technol. 101, 4343–4348. doi: 10.1016/j.biortech.2010.01.083
Leppäkoski, L., Marttila, M. P., Uusitalo, V., Levänen, J., Halonen, V., and Mikkilä, M. H. (2021). Assessing the carbon footprint of biochar from willow grown on marginal lands in Finland. Sustainability 13:97. doi: 10.3390/su131810097
Leppäkoski, L., Uusitalo, V., Horn, S., Levänen, J., Kasurinen, H., and Härri, A. (2022). Assessing the climate impacts of oat straw as a raw material. Clean. Mater. 5:100133. doi: 10.1016/j.clema.2022.100133
Li, H., and Feng, K. (2018). Life cycle assessment of the environmental impacts and energy efficiency of an integration of sludge anaerobic digestion and pyrolysis. J. Clean. Prod. 195, 476–485. doi: 10.1016/j.jclepro.2018.05.259
Li, H., Jin, C., Zhang, Z., O’Hara, I., and Mundree, S. (2017). Environmental and economic life cycle assessment of energy recovery from sewage sludge through different anaerobic digestion pathways. Energy 126, 649–657. doi: 10.1016/j.energy.2017.03.068
Liljeblom, E., and Vaihekoski, M. (2004). Investment evaluation methods and required rate of return in Finnish publicly listed companies. Finn. J. Bus. Econ. 53, 9–24. Available at SSRN: https://ssrn.com/abstract=486462
Lim, E. Y., Tian, H., Chen, Y., Ni, K., Zhang, J., and Tong, Y. W. (2020). Methanogenic pathway and microbial succession during start-up and stabilization of thermophilic food waste anaerobic digestion with biochar. Bioresour. Technol. 314:123751. doi: 10.1016/j.biortech.2020.123751
Lindorfer, J., Fazeni, K., and Steinmüller, H. (2014). Life cycle analysis and soil organic carbon balance as methods for assessing the ecological sustainability of 2nd generation biofuel feedstock. Sustain Energy Technol Assess 5, 95–105. doi: 10.1016/j.seta.2013.12.003
Liu, J., Kleinman, P. J., Aronsson, H., Flaten, D., McDowell, R. W., Bechmann, M., et al. (2018). A review of regulations and guidelines related to winter manure application. Ambio 47, 657–670. doi: 10.1007/s13280-018-1012-4
Luhas, J., Marttila, M., Leppäkoski, L., Mikkilä, M., Uusitalo, V., and Linnanen, L. (2022). A financial and environmental sustainability of circular bioeconomy: a case study of short rotation coppice, biochar and greenhouse production in southern Finland. Biomass Bioenergy 163:106524. doi: 10.1016/j.biombioe.2022.106524
Luostarinen, S., Tampio, E., Laakso, J., Sarvi, M., Ylivainio, K., Riiko, K., et al. (2020). Manure processing as a pathway to enhanced nutrient recycling: report of SuMaNu platform. Nat. Res. Bioecon. Stud. 62:76.
Ma, J., Pan, J., Qiu, L., Wang, Q., and Zhang, Z. (2019). Biochar triggering multipath methanogenesis and subdued propionic acid accumulation during semi-continuous anaerobic digestion. Bioresour. Technol. 293:122026. doi: 10.1016/j.biortech.2019.122026
Marttinen, S., Venelampi, O., Iho, A., Koikkalainen, K., Lehtonen, E., and Luostarinen, S. (2018). Towards a breakthrough in nutrient recycling: State-of-the-art and recommendations for developing policy instruments in Finland. Natural resources and bioeconomy studies. Nat. Resour. Res. Finland. Available at: http://urn.fi/URN:ISBN:978-952-326-578-3
Marttila, M. P., Uusitalo, V., Linnanen, L., and Mikkilä, M. H. (2021). Agro-industrial symbiosis and alternative heating systems for decreasing the global warming potential of greenhouse production. Sustainability 13:40. doi: 10.3390/su13169040
Mašek, O., Budarin, V., Gronnow, M., Crombie, K., Brownsort, P., Fitzpatrick, E., et al. (2013). Microwave and slow pyrolysis biochar—comparison of physical and functional properties. J. Anal. Appl. Pyrolysis 100, 41–48. doi: 10.1016/j.jaap.2012.11.015
Mehta, N., Anderson, A., Johnston, C. R., and Rooney, D. W. (2022). Evaluating the opportunity for utilizing anaerobic digestion and pyrolysis of livestock manure and grass silage to decarbonise gas infrastructure: a Northern Ireland case study. Renew. Energy 196, 343–357. doi: 10.1016/j.renene.2022.06.115
Mikkilä, M., Utanun, P., Luhas, J., Horttanainen, M., and Linnanen, L. (2021). Sustainable circular bioeconomy—feasibility of recycled nutrients for biomass production within a pulp and paper integration in Indonesia, southeast asia. Sustainability 13:169. doi: 10.3390/su131810169
Mills, N., Pearce, P., Farrow, J., Thorpe, R. B., and Kirkby, N. F. (2014). Environmental & economic life cycle assessment of current & future sewage sludge to energy technologies. Waste Manag. 34, 185–195. doi: 10.1016/j.wasman.2013.08.024
Ministry of Economic Affairs and Employment (2022). Carbon-neutral Finland 2035 – National Climate and energy strategy. Helsinki: Publications of the Ministry of Economic Affairs and Employment.
Mohammadi, A., Venkatesh, G., Sandberg, M., Eskandari, S., and Granström, K. (2019). Life cycle assessment of combination of anaerobic digestion and pyrolysis: focusing on different options for biogas use. Adv. Geosci. 49, 57–66. doi: 10.5194/adgeo-49-57-2019
Mohr, R. M., Grant, C. A., May, W. E., and Stevenson, F. C. (2007). The influence of nitrogen, phosphorus and potash fertilizer application on oat yield and quality. Can. J. Soil Sci. 87, 459–468. doi: 10.4141/CJSS06022
Monforti, F., Lugato, E., Motola, V., Bodis, K., Scarlat, N., and Dallemand, J. F. (2015). Optimal energy use of agricultural crop residues preserving soil organic carbon stocks in Europe. Renew. Sust. Energ. Rev. 44, 519–529. doi: 10.1016/j.rser.2014.12.033
Motiva . Energy from fields [internet]. (2024a). Available at: https://www.motiva.fi/ratkaisut/uusiutuva_energia/bioenergia/energiaa_pelloilta
Motiva . Emission factors [internet]. (2024b) Available at: https://www.motiva.fi/ratkaisut/energiankaytto_suomessa/co2-paastokertoimet
MTK , (2023). Farm carbon flux calculator [internet]. Available at: https://hiililaskuri.mtech.fi/
Muurinen, J., Stedtfeld, R., Karkman, A., Parnanen, K., Tiedje, J., and Virta, M. (2017). Influence of manure application on the environmental resistome under Finnish agricultural practice with restricted antibiotic use. Environ. Sci. Technol. 51, 5989–5999. doi: 10.1021/acs.est.7b00551
Myllymäki, M., Särkijärvi, S., Karppinen, T., and Kumpula, H.., Virkkunen, E. . Increasing the utilization of horse manure, case study: Kainuu [internet] (2014) Available at: http://urn.fi/URN:NBN:fi-fe201502021303
Nasdaq . Natural gas [internet]. (2023). Available at: https://www.nasdaq.com/market-activity/commodities/ng:nmx
Natural Resources Institute Finland . Horticultural Statistics (2021) Available at: https://www.luke.fi/fi/tilastot/puutarhatilastot/puutarhatilastot-2021
Natural Resources Institute Finland . Agricultural information, biogas calculator 2022 [internet]. (2022). Available at: https://maatalousinfo.luke.fi/fi/laskurit/biogas
Natural Resources Institute Finland . Manure handling and spreading [internet] natural resources institute Finland. (2023a) Available at: https://www.luke.fi/en/statistics/indicators/cap-indicators/manure-handling-and-spreading#:~:text=Nearly%20all%20manure%20was%20injected.of%20the%20utilised%20agricultural%20area
Natural Resources Institute Finland Agricultural statistics, crop yield 2022 [internet]. (2023b). Available at: https://www.luke.fi/en/statistics/crop-production-statistics/crop-production-2022
Nayal, F. S., Mammadov, A., and Ciliz, N. (2016). Environmental assessment of energy generation from agricultural and farm waste through anaerobic digestion. J. Environ. Manag. 184, 389–399. doi: 10.1016/j.jenvman.2016.09.058
Opatokun, S. A., Lopez-Sabiron, A. M., Ferreira, G., and Strezov, V. (2017). Life cycle analysis of energy production from food waste through anaerobic digestion, pyrolysis and integrated energy system. Sustainability 9:804. doi: 10.3390/su9101804
Pan, J., Ma, J., Liu, X., Zhai, L., Ouyang, X., and Liu, H. (2019). Effects of different types of biochar on the anaerobic digestion of chicken manure. Bioresour. Technol. 275, 258–265. doi: 10.1016/j.biortech.2018.12.068
Pohl, M., Heeg, K., and Mumme, J. (2013). Anaerobic digestion of wheat straw – performance of continuous solid-state digestion. Bioresour. Technol. 146, 408–415. doi: 10.1016/j.biortech.2013.07.101
Qian, K., Kumar, A., Zhang, H., Bellmer, D., and Huhnke, R. (2015). Recent advances in utilization of biochar. Renew. Sust. Energ. Rev. 42, 1055–1064. doi: 10.1016/j.rser.2014.10.074
Rasa, K., Heikkinen, J., Hannula, M., Arstila, K., Kulju, S., and Hyväluoma, J. (2018). How and why does willow biochar increase a clay soil water retention capacity? Biomass Bioenergy 119, 346–353. doi: 10.1016/j.biombioe.2018.10.004
Ronga, D., Caradonia, F., Parisi, M., Bezzi, G., Parisi, B., Allesina, G., et al. (2020). Using digestate and biochar as fertilizers to improve processing tomato production sustainability. Agronomy 10:138. doi: 10.3390/agronomy10010138
Ronsse, F., Van Hecke, S., Dickinson, D., and Prins, W. (2013). Production and characterization of slow pyrolysis biochar: influence of feedstock type and pyrolysis conditions. GCB Bioenergy 5, 104–115. doi: 10.1111/gcbb.12018
Roubaud, A., and Favrat, D. (2005). Improving performances of a lean burn cogeneration biogas engine equipped with combustion prechambers. Fuel 84, 2001–2007. doi: 10.1016/j.fuel.2004.02.023
Ruuskanen, M., Muurinen, J., Meierjohan, A., Pärnänen, K., Tamminen, M., Lyra, C., et al. (2016). Fertilizing with animal manure disseminates antibiotic resistance genes to the farm environment. J. Environ. Qual. 45, 488–493. doi: 10.2134/jeq2015.05.0250
Sapci, Z. (2013). The effect of microwave pretreatment on biogas production from agricultural straws. Bioresour. Technol. 128, 487–494. doi: 10.1016/j.biortech.2012.09.094
Sawatdeenarunat, C., Nguyen, D., Surendra, K. C., Shrestha, S., Rajendran, K., Oechsner, H., et al. (2016). Anaerobic biorefinery: current status, challenges, and opportunities. Bioresour. Technol. 215, 304–313. doi: 10.1016/j.biortech.2016.03.074
Sawatdeenarunat, C., Surendra, K. C., Takara, D., Oechsner, H., and Khanal, S. K. (2015). Anaerobic digestion of lignocellulosic biomass: challenges and opportunities. Bioresour. Technol. 178, 178–186. doi: 10.1016/j.biortech.2014.09.103
Scarlat, N., Martinov, M., and Dallemand, J. F. (2010). Assessment of the availability of agricultural crop residues in the European Union: potential and limitations for bioenergy use. Waste Manag. 30, 1889–1897. doi: 10.1016/j.wasman.2010.04.016
Semida, W. M., Beheiry, H. R., Sétamou, M., Simpson, C. R., Abd El-Mageed, T. A., Rady, M. M., et al. (2019). Biochar implications for sustainable agriculture and environment: a review. S. Afr. J. Bot. 127, 333–347. doi: 10.1016/j.sajb.2019.11.015
Shackley, S., Hammond, J., Gaunt, J., and Ibarrola, R. (2011). The feasibility and costs of biochar deployment in the UK. Carbon Manag. 2, 335–356. doi: 10.4155/cmt.11.22
Singh, P., Heikkinen, J., Ketoja, E., Nuutinen, V., Palojärvi, A., Sheehy, J., et al. (2015). Tillage and crop residue management methods had minor effects on the stock and stabilization of topsoil carbon in a 30-year field experiment. Sci. Total Environ. 518-519, 337–344. doi: 10.1016/j.scitotenv.2015.03.027
Singh, R., Paritosh, K., Pareek, N., and Vivekanand, V. (2022). Integrated system of anaerobic digestion and pyrolysis for valorization of agricultural and food waste towards circular bioeconomy. Bioresour. Technol. 360:127596. doi: 10.1016/j.biortech.2022.127596
Song, J., Wang, Y., Zhang, S., Song, Y., Xue, S., Liu, L., et al. (2021). Coupling biochar with anaerobic digestion in a circular economy perspective: a promising way to promote sustainable energy, environment and agriculture development in China. Renew. Sust. Energ. Rev. 144:110973. doi: 10.1016/j.rser.2021.110973
Statistics Finland . Environment and nature. Greenhouse gas emissions in 2021 [internet] (2022). Available at: https://www.stat.fi/julkaisu/cktlf0i203azm0a519to5exzc
Statistics Finland . Agriculture, forestry and fishery [internet]. (2024) Available at: https://stat.fi/tup/suoluk/suoluk_maatalous_en.html#Crops
Sugiarto, Y., Sunyoto, N. M. S., Zhu, M., Jones, I., and Zhang, D. (2021). Effect of biochar addition on microbial community and methane production during anaerobic digestion of food wastes: the role of minerals in biochar. Bioresour. Technol. 323:124585. doi: 10.1016/j.biortech.2020.124585
Tanskanen, R. , (2017). Preliminary study on the utilization of material flows from the horse industry in South Savo as renewable energy [internet]. Available at: https://urn.fi/URN:ISBN:978-952-344-005-0
Tayibi, S., Monlau, F., Fayoud, N., Elhouda, O. A., Zeroual, Y., Hannache, H., et al. (2019). One-pot activation and pyrolysis of Moroccan Gelidium sesquipedale red macroalgae residue: production of an efficient adsorbent biochar. Biochar 1, 401–412. doi: 10.1007/s42773-019-00033-2
Tayibi, S., Monlau, F., Marias, F., Thevenin, N., Jimenez, R., Oukarroum, A., et al. (2021). Industrial symbiosis of anaerobic digestion and pyrolysis: performances and agricultural interest of coupling biochar and liquid digestate. Sci. Total Environ. 793:148461. doi: 10.1016/j.scitotenv.2021.148461
Tham, J., and Vélez-Pareja, I. (2004). Principles of cash flow valuation: an integrated market-based approach : Elsevier.
The World Bank . Commodity markets 2023 [internet]. (2023) Available at: https://www.worldbank.org/en/research/commodity-markets
Tisserant, A., and Cherubini, F. (2019). Potentials, limitations, co-benefits, and trade-offs of biochar applications to soils for climate change mitigation. Land 8:179. doi: 10.3390/LAND8120179
Tisserant, A., Morales, M., Cavalett, O., O’Toole, A., Weldon, S., Rasse, D. P., et al. (2022). Life-cycle assessment to unravel co-benefits and trade-offs of large-scale biochar deployment in Norwegian agriculture. Resour. Conserv. Recycl. 179:106030. doi: 10.1016/j.resconrec.2021.106030
Virolainen-Hynnä, A. (2020). Biogas production and use in Finland in 2030. Finnish Biocycle and Biogas Association.
Walling, E., and Vaneeckhaute, C. (2020). Greenhouse gas emissions from inorganic and organic fertilizer production and use: a review of emission factors and their variability. J. Environ. Manag. 276:111211. doi: 10.1016/j.jenvman.2020.111211
Wang, J., Okopi, S. I., Ma, H., Wang, M., Chen, R., Tian, W., et al. (2021). Life cycle assessment of the integration of anaerobic digestion and pyrolysis for treatment of municipal solid waste. Bioresour. Technol. 338:125486. doi: 10.1016/j.biortech.2021.125486
Weiser, C., Zeller, V., Reinicke, F., Wagner, B., Majer, S., Vetter, A., et al. (2014). Integrated assessment of sustainable cereal straw potential and different straw-based energy applications in Germany. Appl. Energy 114, 749–762. doi: 10.1016/j.apenergy.2013.07.016
Wu, B., Yang, Q., Yao, F., Chen, S., He, L., Hou, K., et al. (2019). Evaluating the effect of biochar on mesophilic anaerobic digestion of waste activated sludge and microbial diversity. Bioresour. Technol. 294:122235. doi: 10.1016/j.biortech.2019.122235
Yanik, J., Kornmayer, C., Saglam, M., and Yüksel, M. (2007). Fast pyrolysis of agricultural wastes: characterization of pyrolysis products. Fuel Process. Technol. 88, 942–947. doi: 10.1016/j.fuproc.2007.05.002
Zhang, C., Xiao, G., Peng, L., Su, H., and Tan, T. (2013). The anaerobic co-digestion of food waste and cattle manure. Bioresour. Technol. 129, 170–176. doi: 10.1016/j.biortech.2012.10.138
Keywords: anaerobic digestion, pyrolysis, biochar, biogas, agriculture, life cycle assessment, cash flow analysis
Citation: Marttila M, Havukainen J, Uusitalo V, Linnanen L and Mikkilä M (2024) Multicriteria analysis of sustainable agri-food waste management for an agroecosystem in Finland. Front. Sustain. Food Syst. 8:1426890. doi: 10.3389/fsufs.2024.1426890
Edited by:
Lars Stoumann Jensen, University of Copenhagen, DenmarkReviewed by:
Carlos José Álvarez-Gallego, University of Cádiz, SpainMaja Rydgård, University of Copenhagen, Denmark
Copyright © 2024 Marttila, Havukainen, Uusitalo, Linnanen and Mikkilä. This is an open-access article distributed under the terms of the Creative Commons Attribution License (CC BY). The use, distribution or reproduction in other forums is permitted, provided the original author(s) and the copyright owner(s) are credited and that the original publication in this journal is cited, in accordance with accepted academic practice. No use, distribution or reproduction is permitted which does not comply with these terms.
*Correspondence: Miika Marttila, bWlpa2EubWFydHRpbGFAbHV0LmZp