- 1Qualité des Produits Animaux (QuaPA), INRAE, Centre Clermont-Auvergne Rhône Alpes, Saint Genès Champanelle, France
- 2Clermont-Auvergne University, Unité de Nutrition Humaine, Clermont-Ferrand, France
- 3Department of Food Quality and Sensory Science, Ashtown Teagasc, Dublin, Ireland
- 4Riddet Institute, Massey University, Palmerston North, New Zealand
- 5Université Paris-Saclay, INRAE, FRISE, Antony, France
- 6School of Food and Advanced Technology, Massey University, Palmerston North, New Zealand
Processing is an inevitable step in the manufacturing of animal-based foods (ABF) and animal by-products (ABP). However, our society has reached a point where our food systems have reached unsustainable levels. The impact of ABF/ABP processing on sustainability has been arguably overlooked in comparison with production. This perspective paper aims to discuss and identify research gaps regarding the assessments of the sustainability of ABF/ABF processing. First, we describe why processing techniques can have various levels of complexity, with uses that are more or less impactful on the environment depending on the products and possible synergies. In the second part, we review how impacts on sustainability have been evaluated at global and local scales using life cycle assessments (LCA). To contribute to such an approach, we suggest novel or recently introduced types of indicators that would improve future LCA studies by capturing relevant information. In the third part, we encourage a systemic view of sustainability by considering the complexity of the whole supply chains of ABF and ABP. We highlight the current gaps or challenges in evaluating sustainability across supply chains and point the readers toward recent studies that address these limitations. We hope this perspective will help improve the design of academic and industrial studies or evaluation of ABF and ABP sustainability.
1 Introduction
Animal-based foods (ABF) are currently an important source of nutrients for humanity because they provide proteins (a rich source of essential amino acids) and essential micronutrients that are readily bioaccessible (notably calcium, iron, selenium, zinc, and vitamin B12, and omega 3 and 6 from fish products). Animal by-products (ABP) also have important technical functionalities and economic values. However, our society has reached a point where our food systems have reached unsustainable levels, in terms of land usage, greenhouse gas emissions, water use, pollution, and energy consumption (Foley et al., 2011; Poore and Nemecek, 2018). Hence, it is becoming increasingly urgent to consider how food systems can be improved to meet international sustainability targets, such as described by the United Nations’ Sustainable Development Goals (United Nations General Assembly, 2015), and the planet’s boundaries (Kahiluoto et al., 2014). In this article, we will refer to the term sustainability as any practice that minimizes or reduces the environmental impacts and consumption of any kind of resource.
In this context, the sustainability of the livestock agriculture chain has been largely studied, and there is a consensus that livestock farming creates an outsized environmental impact (e.g., Foley et al., 2011; Espinosa-Marrón et al., 2022). Animal husbandry has therefore been highlighted in the media and public debates. However, processing is an inevitable step in the making of ABF and ABP, and it could also be a major driver of the impacts on sustainability.
Previous work on the sustainability of ABPs and ABFs products focuses mainly on siloed approaches, such as describing one novel processing type, discussing gain in efficiency in cold chains, etc. Such work highlights solutions targeting single aspects while the issue of sustainability is largely recognized as a systemic problem. Hence, the research gap we identified and motivated this work is the need for a holistic view of processing and its impact on sustainability which can grasp the complexity of the challenge that awaits us.
This perspective article discusses the complexity of ABF and ABP processing and their impacts on sustainability along three complementary axes. In the first section, we argue that grasping the complexity of processing techniques – and possible synergies – is an important step in proposing actions to improve sustainability. Therefore, we highlight the complexity of processing techniques by providing a non-exhaustive overview of the processing techniques and discuss how techniques can have specific dependencies such as refrigeration, storage, energy, etc. The second section reviews how ABF and ABP processing have been recently evaluated for their impacts on sustainability. Life cycle assessments can provide quantitative or semi-quantitative data to help decision-makers improve the sustainability of the processing industry. LCAs of food products and processing have increasingly incorporated indicators to account for sustainability, both at global and local scales. However, we have identified several criteria to add to LCA for future research. In the third section, we want to take a systemic approach by discussing the sustainability of supply chains for ABF and ABP processing, which we have identified as a current research gap. Our review work underlines that the analyses of environmental impacts tend to become much more complex as supply chains become global and involve many actors, but that avenues for improvements are also proposed in recent literature.
2 Main text
2.1 Disparities of processing techniques of ABF and ABP in terms of sustainability
The number of technologies developed for ABF and ABP processing has exploded in the past decades, supported by innovations in various sectors (Cooper, 1994). For example, about 50 different types of processing can be applied to meat, with a variety of machines and formulations. On the other hand, some animal products do not require any processing, or very minimal processing (such as honey or fresh eggs). Due to the variety and complexity of processes, and possible synergies, evaluating the impact of ABF and ABP products on sustainability is a difficult task. In this line of effort, this section highlights how traditional and emerging processing technologies can be regarded as low or high-energy processes, and what would be possible synergies. Of note, downstream products such as dairy products, eggs, honey, and edible animal co-products such as protein powders or functional ingredients are referred to as ABF, while ABP refers to non-edible products.
2.1.1 Animal products that are produced from animals without killing the animals or are minimally processed
Minimal processing is a trending topic that has been previously reviewed (Alzamora et al., 2016). However, the literature can be confusing about whether minimal processing should include energy-intensive or low-energy techniques. Minimal processing includes techniques such as high-intensity light pulses, ozone, or ultraviolet radiation which enhance preservation. Eggs, honey, wool, feathers, milk, naturally processed yogurt, and most modern varieties of cheese are animal products that can be obtained without the need for animal slaughter or undergoing extensive processing. These are, by definition, following the most sustainable practices and local production and distribution of these products must be encouraged.
Fermentation, including the marinade process, is an ancestral low-energy technique to process ABF, such as cheese, meat, or seafood. Fermentation can be easily scaled up or down, preserves product quality, and enhances shelf-life. At the same time, additional compounds can be added to the product, for example, to enhance nutritional properties or antioxidant potential (Fardet and Rock, 2018). Fermentation is sometimes complemented by other processes such as maturation (for some types of ham or salami and many cheese types), dehydration, curdling, or acidification.
Dairy products relying on fermentation have existed for centuries. Cheese has been produced since the 6th millennium BC (Salque et al., 2013). Many different varieties are found globally in various forms, since these products are strongly linked to tradition and customs. However, the making of dairy food products also contributes to environmental impacts such as important water use or energy consumption, especially in industrial setups for the fermentation and maturation processes, or storage at low temperatures. In some cases, the maturation process or storage can benefit from natural environments such as grottos or natural caves which keep temperature and humidity at specific levels (e.g., Verduna et al., 2020). Therefore, the energy cost of fermentation and maturation will highly depend on the itinerary routes, and the possibility of using natural environments.
2.1.2 Minimal processing with medium to high energy costs
Slaughtering for meat and fish production can be referred to as a processing technique with medium- to high resource requirements due to a large variety of associated activities. This includes the transport of animals, veterinary controls, killing equipment, cutting, aging process (maturation), packaging, cold storage/freezing, sanitary/microbiological controls, waste management, and cleaning. Of note, the management of the generated wastes and co-products (stomach contents and bones) is associated with further disposal/collection costs, sanitary restrictions, and hazards (Regulation Report, 2004). These different activities result in high costs in land occupation, energy, water, and wastewater pollution for which corrective and preventive options have been previously suggested (Genné and Derden, 2008). Small-scale farms can perform the raising, killing, and selling of products on the same site (such as chicken farms), which may reduce the environmental costs associated with transport and retailing to large distributors (which would need to maintain the cold chain). Efforts can also be made by optimizing the tenderization (aging) period of meat, which is done at low temperatures in a controlled moisture environment over an extended period (up to 40 or 50 days), depending on the species. This process can be dry- or wet-aged. The shortest maturation periods are observed for poultry (2–3 days), followed by pork and lamb (7 days), and against a minimum of 14 days for beef. Regarding fish, this period is unnecessary, and they are immediately sold fresh, frozen, or processed to extend the shelf life.
For some niche products such as Parma ham, Iberico ham, or traditionally matured cheese, very long periods of maturation are required, up to 36 months, to obtain a final product with the desired organoleptic properties. As explained above for traditional cheese, ham production can take advantage of natural resources such as caves or facilities at great altitudes, where low temperatures and constant humidity are naturally present. However, since this is not always available, processors have to invest in maturation facilities with temperature and moisture control, which will be associated with high energy costs for long maturation processes, more remarkably if the facilities are not well insulated and the systems are not used optimally (Nunes et al., 2014).
Cooking is also a type of processing. Cooking is any heat treatment applied to a food product to modify its organoleptic or physicochemical properties. Cooking can increase shelf-life, destroy possible pathogens, increase tenderness, and increase digestibility and/or acceptability by consumers. However, high cooking temperatures are also associated with the generation and accumulation of harmful compounds such as biogenic amines, heterocyclic amines, and aromatic hydrocarbons (Cross and Sinha, 2004; Barzegar et al., 2019). On the other hand, low-temperature and long-time cooking methods that prevent the generation of such compounds are being reintroduced nowadays. Heat treatments are also conducted at the factory level, to provide consumers with ready-to-eat meals, or to facilitate exports by selling added-value products. Solar cooking, or solar-energy-based cooking, is also increasing in parts of the world; however, it is still limited to individual uses. To increase the acceptance of solar cooking, a few studies are developing indoor solar cooking systems (e.g., Varun et al., 2022). Importantly, solar-based solutions applicable at industrial levels are scarce and we found no scientific literature on these systems.
Drying techniques are widely applied in the processing of animal products, from dairy products to meat, but also to produce protein isolates for example. Drying aims to preserve the nutritional values of the product while extending its shelf life, and removing the need for cold storage, as well as decreasing weight and volume. For obvious reasons drying techniques employed by the ABF processing industry can be very demanding in terms of energy, notably to control ventilation and maintain temperature and moisture at adequate levels sometimes for long periods. However, some drying processes are very cost-effective, for instance, sun drying or osmotic drying. A review specific to the osmotic treatment of meat and fish products has been previously published by Collignan et al. (2001). Spray drying is another technique used in the dairy or protein powders industry (Sovacool et al., 2021). To improve the sustainability of drying steps, hybrid drying processes have been tested, in which low-energy processes are combined with new technologies (infrared, microwave, ultrasound, or ultraviolet-assisted drying). For example, samples pretreated with ultrasound showed faster drying kinetics, reducing the energy required to remove the excess moisture (Zhu et al., 2021). In light of our research, comparative studies of drying techniques describing which synergies work the best are desirable.
Smoking is a historical preservation technique, broadly applied to meats, fish, cheese, or products derived from these sources, which also imparts a particular and distinctive flavor. Fish smoking, for example, can be performed using various smoking procedures (smoldering, liquid smoke, friction, electrostatic friction, and electrostatic smoldering), which have been previously reviewed (Varlet et al., 2007). One concern about fish smoking is that traditional processes can generate by-products such as polycyclic aromatic hydrocarbon (PAH) whose carcinogenic toxicity has been reviewed and discussed (Jinadasa et al., 2020). Hence, nowadays, smoke is mainly generated by friction, which by comparison to traditional smoking is considered safer and less prone to originate health issues: the lower temperatures for smoke generation create less harmful by-products in the smoke (Arvanitoyannis and Kotsanopoulos, 2012).
High hydrostatic pressure (HHP) processing has been proposed as an alternative to thermal pasteurization for foods with heat-sensitive properties, including meat or liquids. An intense pressure (about 400–600 MPa) is applied to the product (often a liquid product) under chilled or low temperatures (< 45°C). High-Pressure Homogenisation (HPH) is used to extract natural compounds from suspended particles, reach the stabilization of liquid foods such as milk, or provide stable emulsions. This helps to preserve the organoleptic and nutritive properties of fresh products while deactivating pathogens (Yaldagard et al., 2008). One recent example is how HHP can help optimize meat shelf-life while avoiding possible negative impacts on color, texture, and water-holding capacity (Niebuhr et al., 2020). Being a non-thermal technology, HHP is often referred to as a low-energy process or eco-friendly process because of its efficiency (Picart-Palmade et al., 2019). However, as pointed out by other authors, studies on the environmental impacts of HPP are scarce (Valsasina et al., 2017). The use of high-pressure pumps, pneumatics, and chilling equipment will necessarily imply the use of other resources with possible environmental impact. Hence, the manufacture and maintenance of HHP equipment needs to be considered, and further studies are desirable.
2.1.3 Highly processed animal-based products
We define highly processed foods (and co-products) as the result of multiple layers of processes (a.k.a.: have a complex technological itinerary). Highly processed ABF or ABP can include a wide range of products, from processed dairy products to processed meat or non-edible animal products. The applications of various techniques aims to provide microbiological control, perform the separation of components, or change/improve the properties (technological, organoleptic, etc.) of a given product. Taken independently, the techniques can be regarded as eco-friendly as they enable meeting large volume demands and reduce production costs (Picart-Palmade et al., 2019). We present some of the most relevant techniques below.
Protein extraction of animal products or co-products finds major uses in the food, medical, and pharmaceutical sectors. Protein extraction can be regarded as a medium to high-energy technique, depending on the type of products, the equipment that is involved, and the production scale. For example, whey proteins can be a natural by-product of cheese production, or it can be obtained from the industrial cultures of microorganisms, in which case it is obtained by ultrafiltration (to separate proteins from lactose and minerals) and drying. Protein extraction poses different challenges depending on their origin and the constituent elements of the matrix they are embedded in (e.g., chitin from insects, cellulose, or pectin when extracting plant proteins). Protein extraction/concentration can be performed by drying methods, protein precipitation (e.g., ammonium sulfate precipitation), solvent precipitation (e.g., using ethanol), crystallization, or enzymatic or acid/base reactions performed in bioreactors. It can be followed by purification steps, performed using advanced separation or filtration methods, such as chromatographic techniques, reverse osmosis, or electrodialysis. Some of these techniques are energetically demanding, or require solvents, but also offer potential ways to reduce the processing time or temperatures, which in some cases might mitigate the energy costs. Importantly, they can be scaled up to meet the large volumes required by the industry. Future research will need to evaluate how these different combinations of techniques impact the digestibility, safety, and nutritional values of ABF (Bhat et al., 2022). More studies on the impacts on microbiota and health in the long term are desirable.
Reverse osmosis is a membrane filtration technique that allows the selective passage of water and has been used significantly in water desalination, water treatment, and food processing applications. This processing technique has been increasingly used over the past 25 years in the dairy industry, notably in the production of whey protein, concentration of skim milk (prior to the production of milk powders), and manufacture of yogurt (El-Gazzar and Marth, 1991). It can effectively purify water, and retain substances such as fats, proteins, lactose, and minerals. This can help to improve the consistency of cheese structure, as well as shelf-life, and organoleptic properties. Large volumes of raw milk can be pre-concentrated by reverse osmosis to reduce the cost of transportation before cheese manufacturing. Proteolytic content, microbial activities, and sensory qualities of dairy products manufactured by reverse osmosis have been extensively studied. The environmental impacts of reverse osmosis were also studied (more than 30,000 papers found on Scholar one) but most studies focus on water desalination rather than food processing (3,000 studies were associated with “food processing”). As reverse osmosis enables larger processed volumes, further research is required to assess if the application of this process causes rebound effects in energy/water use and how impacts are mitigated. In this regard, we recommend a recently published article that presents the merits and demerits of membrane filtration techniques, including risks regarding potential suppliers or waste management (Deshwal et al., 2021).
Emergent technologies such as ultrasound (US), pulsed electric fields (Gómez et al., 2019), high shear cavitation, and microwaves (MW) are all used in meat and fish processing to modify the physical properties of the matrix. In particular, PEF-assisted extraction has been considered as a promising green method for extraction, osmotic treatment, drying, and freezing. It has been used to retrieve intracellular compounds of different molecular sizes from plants, mushrooms, and seaweeds, which can be used as additive compounds for ABF processing (Picart-Palmade et al., 2019). Here, the aim is to obtain novel functionality or qualities for the products, such as improving technological (structure, texture), economic, organoleptic, or nutritional aspects. Recent research shows how pulsed electric field treatment can improve seafood products parameters (Rathod et al., 2022) or help reducing salt amount used in meat processing (Bhat et al., 2020). Emergent techniques such as pulsed electric fields are however used for ABF with complex processing itinerary routes (Bhat et al., 2019), which deserve to be carefully evaluated for their impacts on sustainability.
Ultra-processing is defined here as any processing method or combination of methods aiming to fractionate whole foods into components (sugars, oils, fats, proteins, starches, fibers), which are subsequently used for new preparations. Previous studies have designed guidelines to identify ultra-processed foods and their markers (Davidou et al., 2021; Monteiro et al., 2022). Techniques we refer to include for example ultra or nano-membrane filtration techniques, supercritical fluid extraction, or hydrogenation. Ultra-processing causes an artificialization of the food matrix (Pagliai et al., 2020; Fardet and Rock, 2022), and its impacts on health have been largely studied (e.g., Pagliai et al., 2020; Suksatan et al., 2022). Ultra-processing in animal-based products is performed on meat, dairy, fish, insects, and seafood products. It enables the processing of large volumes at relatively low costs, or the valorization of sub-products that otherwise could not be sold to consumers or as animal foods, such as the collagen extracted from the bones and skin of large animals. Ultra-processing, requiring large volumes, can drive the upstream demand to produce large quantities, which can favor monoculture and intensive farm animals (Fardet and Rock, 2020; Dicken and Batterham, 2022).
Ultra-formulation, although not considered a process itself, is used to reach the desired organoleptic properties of the final product. Ultra-formulation consists of adding synthetic and purified compounds (mineral oil, hexane, etc.), additives, or aromas for texture, taste, color, and preservation (emulsifiers, antioxidants, etc.). It can be used to restore properties that have been lost during drastic processes, such as in ultra-processed food matrices (Monteiro et al., 2022). Hence, we observe that heavily formulated ABP generally results from ultra-processing. Hundreds of additives are used nowadays, and their potential impact on health is under regulatory scrutiny. However, as far as we know their environmental impacts have not been evaluated thoroughly.
A growing interest in a flexitarian diet has led to the emergence of new hybrid products that contain ingredients from both plants and animals. Hybrid meat analogs, which are prepared by co-processing plant and animal proteins, are nutritionally superior to plant-only meat analogs (Wang et al., 2022). These hybrid meat analogs differ from meat extenders, in which plant-based functional ingredients are added or blended for economic and technological reasons (Grasso and Jaworska, 2020). Animal protein-based isolates, such as whey protein isolates and concentrates, sodium caseinate, whey protein, and bone marrow are used to supplement plant protein formulations for producing hybrid meat analogs (Taylor and Walsh, 2002). Consumers perceive hybrid products as products with good texture and nutritional quality (Grasso and Jaworska, 2020), but more research is needed toward the improvement of sensory quality and health effects of these products. Plant-based meat analogs are usually manufactured through high moisture extrusion by subjecting protein dispersions to high temperatures and shear forces to achieve meat-like fibrous texture. Plant-based meat analogs usually are considered to have a lower environmental impact (Mejia et al., 2016), but the multiplicity of implied processes may lead us to question this sustainability. These products are sometimes mixed with meat to develop partially substituted meat products such as burgers, sausages, or meat mince. The leguminous proteins produce very beany flavored volatiles due to the enzymatic oxidation of polyunsaturated fatty acids (Joshi and Kumar, 2015) during thermal processing such as extrusion. This fact encourages processors to add numerous additives and flavoring agents to these products to produce a tasty product.
As an alternative to ultra-processed analogs, research is currently underway to co-process whole plant and whole low-value animal products (or co-products) to develop new hybrid structures that possess similar textural and nutritional qualities as meat. New up-and-coming technologies such as 3D food printing (Portanguen et al., 2019; Wang et al., 2022) have the potential to produce nutritionally superior analogs. Such novel techniques can be used to develop plant-based meat analogs from raw meat (such as iron-rich liver) and vegetables that answer specific requirements of people with mastication or nutritional deficiencies, such as elderly people.
Cell culture is a recently developed approach to produce cell-derived ABFs, such as meat-like or plant-like products. Such products have existed since 2013 and have been marketed since 2015. Recent research on cultured cells for meat-like products aims to recreate an assemblage of fibers and fat to mimic the technological and organoleptic properties of natural meat (e.g., Kang et al., 2021). Literature showed the translation of cell culture in the industrial sector still faces numerous challenges to reach scalability and cost-effectiveness (O'Neill et al., 2021), and poses several regulatory challenges (Post et al., 2020). The sustainability of the whole process of cultured meat is widely criticized due to the quite astonishing diversity of ingredients, equipment, and materials involved in the production (Post et al., 2020). One of the current major gaps concerns the cell media composition. The base media requires high-quality water, which is typically prepared by reverse osmosis, deionization, and filtration. It is then supplied with a cocktail of nutrients, including antifungal, antibiotic molecules, amino acids, carbohydrates, growth factors, hormones, insulin, cortisol, albumin, etc. (Post et al., 2020). Research activities and industries still rely on the controversial Fetal Bovine Serum to grow cells, and serum-free alternatives are desirable. The use of rich cell media also implies sanitary considerations due to potential contamination by opportunistic pathogens, such as mycoplasma, viruses, and bacteria (Olarerin-George and Hogenesch, 2015), which raises questions about how sanitary controls can be adequately performed in industries. In the future, in addition to engineering and sustainability challenges, the nutritional value of cell cultures needs to be addressed thoroughly.
2.2 Evaluating the environmental impacts of ABF and ABP
The past decade has been proficient in producing studies that evaluate the impacts of food products on sustainability. The LCA methods have emerged as a gold standard for providing comprehensive and understandable information. They commonly make use of indicators such as energy and water consumption, waste generation, greenhouse gas emissions such as CO2, land usage, transport distance, or eutrophication potential (Thoma et al., 2018). To draw general tendencies and characterize our food systems is a desirable goal. To do so, large meta-analysis have been analyzing the environmental costs of animal production, such as the work by Poore and Nemecek (2018), which combines 570 LCA studies across 119 countries. Their model is based on four major indicators: gas emissions, land use, water stress, and eutrophication potential. They found dairy has less impact than beef husbandry, but more than other animal production. However, the impacts of other ABF or ABP, or impacts of specific processing techniques, are not evaluated. In recent work, Clark et al. (2022) developed a model to estimate the environmental impact of 57,000 food products in the United Kingdom and Ireland based on their ingredient composition. The estimated environmental impacts account for processing, however, it does not incorporate packaging, storage, or transportation, which are essential parts of supply chains. According to this study, ABP and ABF have greater environmental impacts in comparison to other types of foods, but taking into account the nutritional values (energy and protein density, for instance) may mitigate this impact. For a given category of products (beverages, snacks, prepared foods, etc.), the authors suggested replacing the products showing a bad environmental/nutritional score with products from the same category but with better scores (Clark et al., 2022). Another group of authors performed a national study in Brazil where the carbon and water footprints were evaluated for the four NOVA food groups (Garzillo et al., 2022). The four NOVA food groups are described as unprocessed or minimally processed foods (1); processed culinary ingredients (2); processed foods (3); and ultra-processed foods (4). For each type of food product (such as meat, plant oils, cheese, or soft drinks), the ratio between dietary energy intake and carbon/water footprint was calculated. Their results highlight the impact of ultra-processed foods and in particular the impacts of meat processing in terms of CO2 and water footprint. It also suggests that food processing has a significant impact on the environment. Similarly, in a time-series study from 1987 to 2018 in Brazil, a larger group of authors concluded that “the environmental effects of the Brazilian diet have increased over the past three decades along with increased health effects from ultra-processed foods” (Da Silva et al., 2021).
The above literature took advantage of nutritional scores and environmental scores to compare ABF. To what extent these methods can be applied to ABP, in the context of supply chains, is an open question. It appears that, in general, highly processed foods and ultra-transformed foods have higher environmental impacts than less processed foods (Fanzo et al., 2021). Hence, in the context of climate change, land usage, pollution, resource consumption, and global health, there is a need to reduce reliance on animal-based processed foods.
Improving the environmental impact of ABP and ABF processing can also be done by minimizing wastes; as well as making better use of the current resources, as highlighted by several authors when it comes to harnessing the potential of ABPs (Mullen et al., 2017). The generation of suboptimal foods, food waste (or ABP waste), can be the result of physical or chemical reactions generated by poor processing or incorrect handling. Kranert et al. (2012) performed a meta-analysis of food wastes and estimated that the German food industry is responsible for approx. 14 kg of the 82 kg food waste per head and year. Technical problems during processing and quality assurance measures were pointed out as a major reason (Kranert et al., 2012). Previous studies observed that quantitative data on food waste generated during processing are scarce, and access to information is limited, especially on animal-based food (Bräutigam et al., 2014). National data stocks between countries showed great variations in what is included in the terms ‘food waste’, ‘food loss’, the studied boundaries of a given industrial process, and even variations in the type of metrics that are used (Bräutigam et al., 2014).
Interestingly, the dairy industry has greatly improved its production process efficiency while reducing energy and water consumption, wastewater and gaseous pollutants, and encouraging recycling approaches. Recent work in the dairy industry monitored the consumption of energy and water, waste generation, emissions, and effluents discharge such as pollutants in wastewater, gaseous pollutant emissions, as well as the use of cleaner fuels and the use of whey in the production of other dairy products (recycling) (e.g., Veiga et al., 2022). Whey, a co-product of cheese, is mostly processed into high-value protein and lactose products. Whey otherwise has a huge impact as an industry effluent as it is responsible for very high biochemical oxygen demand (BOD) (30,000 to 40,000 mg L−1) (Epa-Victoria 1997; Veiga et al., 2022). However, if the economic demand (market opportunities) were to drive the sole production of whey, rather than its use as a by-product of cheese, it could become a non-sustainable practice.
In Table 1, we provide some examples of studies where quantitative indicators have been used to describe specific aspects of sustainability in meat processing, such as the poultry industry, and the supply chains of meat processing. Indicators showed that reductions in specific environmental values, such as the emission of CO2 (kg CO2-eq), or in terrestrial acidification, eutrophication, water use, water depletion, energy consumption, etc. could be achieved. It demonstrates the variety of indicators that have been used and their usefulness. However, as the environmental impacts of processing can vary greatly even when considering the same product (Poore and Nemecek, 2018), comparative analyses are desirable.
LCA methods are still evolving and improving, and academics and industry bodies have a huge role to play. To be more realistic, future analyses should also include the functioning of the building/facility where processing is done. This has been done, for example, on slaughterhouses, which are very demanding in energy and water use, but also generate waste (Mozhiarasi and Natarajan, 2022). Toward this direction, the ITACA protocol is a method that proposes several criteria to measure or estimate the sustainability of buildings, and it was recently adapted to food processing facilities (Barreca and Cardinali, 2019). Criteria involve, for example, the site quality, the type of resource use (e.g., bio-sourced materials for construction), ventilation and refrigeration systems, the indoor environmental quality for workers, and broader social impacts such as services provided by the building. This novel framework establishes a solid ground to perform comparative analyses, which can encourage industries and academic research.
Moreover, LCA could include metrics describing the specificity of the economic, social, and geographic contexts, and several authors are working to fill this gap. For example, Petit and colleagues provided a metric method to include social indicators in their assessment of the sustainability and value chain of pork agrifood and trout (Petit et al., 2018). To contribute further to this collective effort, we suggest several other indicators for LCA. In particular, the type of energy mix (which is country-dependent), the reliance on energy-intensive equipment (such as pneumatic valves, ultrasound, gas, robotics), the distance/transport costs of raw materials or supply parts, the reliance on air-freighted products, or the possibility to re-use heat or recycle waste on processing sites. Studies should also consider that high-tech processing systems can have important maintenance costs or high turn-over, which questions to what extent enterprises are dependent on suppliers to maintain their operation levels. Sustainable processing solutions need to take cognizance of all these factors while maintaining a focus on product quality, nutrition, and safety.
We propose Figure 1 as an attempt to picture the aforementioned requirements and externalities linked to ABF and ABP-related processes. The Figure highlights that an increase in the degree of processing (depending on the production system, and context) calls for a larger spectrum of dependences/requirements, which together increase the footprint of processing. We also want to represent that sustainability is linked to geographical context, which will determine a number of externalities such as the energy mix, the availability of resources, expertise, and equipment (Hoang et al., 2016). For example, locally produced meat directly sold from the producer to the consumer is a good example of minimal processing: it involves slaughtering and boning, cold storage, and sometimes packaging and cutting, and occasionally all steps can be conducted on-site (e.g., for small chicken farms). This solution, however, may not meet the demand in large urban areas. Processing practices are always embedded through the local economic tissue, the network of actors, and resources. In this sense, we stress that there is no “one size fits all” solution. The craft and efficient implementation of more sustainable processing can only be done in the light of the careful evaluation of the competing interests or intrinsic opposition between the key pillars of sustainability. This viewpoint is also true to guide future policies that will help to shift away from unsustainable practices.
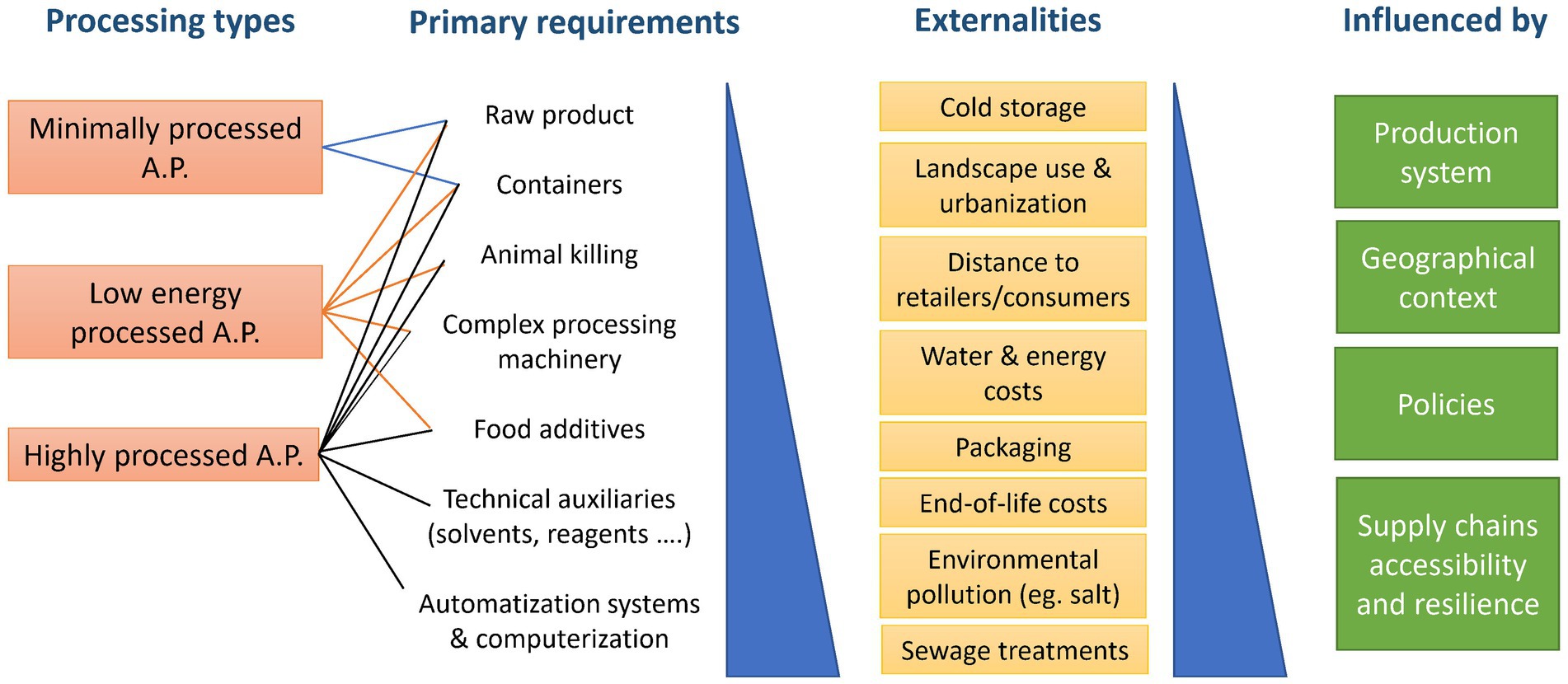
Figure 1. Processing types of food products and by-products and the major primary requirements and externalities. The blue triangles illustrate that more highly processed products require an increasing number of primary requirements (e.g., complex machinery and technical skills, automatized systems) and externalities (e.g., water and energy costs, packaging, transport such as air freight, and the origin of ingredients). Framing the sustainability of processing will require consideration of these parameters for each given product and within a specific geographical/local context.
2.3 The sustainability of supply chains for ABF and ABP processing
Sustainable food supply chains aim to achieve reduced energy and environmental footprints, while increasing positive outcomes, such as food conservation and safety. A plethora of published studies aimed to evaluate the sustainability of food systems at a global scale, using social, environmental, and economic indicators through modeling or statistical approaches (e.g., Malak-Rawlikowska et al., 2019; Béné et al., 2020). For example, Béné et al. (2020) applied quantitative statistical methods to identify possible drivers of the supply chains’ sustainability or unsustainability. In their definition of sustainability, they include social, technological, and economic indicators, such as trade exports or investments. Considering a list of 27 domains and associated indicators from carefully selected studies, they built a metric for food system sustainability and then applied it to obtain a score of sustainability for 97 countries. Then, they attempted to correlate this metric with economic and social indicators such as population growth or wealth, merchandize demand, or trade change over time. The strongest positive correlation was observed between food system sustainability and change over time in merchandize and services trade per capita. This result suggests trade improves sustainability, for it provides social services (employment etc.) and economic values, although the authors acknowledge trade can also increase the pressure on natural resources leading to environmental degradation. Malak-Rawlikowska and colleagues analyzed the sustainability of short-supply chains for 208 food producers across seven countries. Only two environmental indicators were considered, the travel distance of products and consumers. One of their results was that short supply chains can provide producers with a higher share of the value added, while strengthening local development and territorial cohesion, with lower food miles and carbon footprint (Malak-Rawlikowska et al., 2019).
While global-scale analyses provide insights about key drivers of sustainability, they also acknowledge a challenge in accessing on-site data. Arguably, obtaining such quantitative data is a tremendous amount of work, which requires important resources and effort. Subsequently, several studies have argued that the increasing globalization of food network systems has led to significant information and knowledge gaps, particularly in ABF or ABP supply chains (Hueston and McLeod, 2012; Ashe et al., 2015; Fanzo et al., 2021). These authors explain that ABF and ABP are transported and processed worldwide, with many intermediaries across the supply chain. Feeds for aquaculture, for example, can be processed from fish or fish by-products, among other ingredients, and sold globally. For these reasons, in a previous report on seafood processing published by the European Environmental Agency, Belchior and colleagues argued the sustainability of food supply chains of sea products cannot be inferred from trade data and economic indicators, even though 10 major processing enterprises account for 40% of the global seafood market (Belchior et al., 2016). Qian et al. (2022) summarize this issue by stating that “the traceability of processed food faces a different set of challenges compared to primary agro-food, because of the variety of raw materials, batch mixing, and resource transformation”. Food chain sustainability and transparency are some of the economic challenges of food chains, and we invite the reader to consult these other articles to learn more about this topic (Alao et al., 2017; Mullen et al., 2017; Soladoye et al., 2022).
To provide accurate assessments of the ABF and ABP processing and its impact on sustainability along the whole supply chain, one can focus on one specific product and perform on-site data gathering. However, such studies are difficult to find. In the Table 1, we provided examples of how LCA was applied for poultry meat chain and mutton meat supply chain. Interestingly, these studies provide specific indicators and values where efforts led to significant improvements on sustainability. Reductions in kg CO2-eq, terrestrial acidification, or other criteria, were obtained by using alternative energy fuel, or biodegradable packaging, for example (Table 1). Maïolo and colleagues recently published an exhaustive LCA of rainbow trout (Oncorhynchus mykiss) both ABF and fish by-products production and processing (Maiolo et al., 2020). The study was performed in Northern Italy, based on a dataset almost entirely on primary data gathered from supply chain actors and on-site visits. Their analysis included feed production, trout grow-out in freshwater flow-through systems, trout processing into foodstuff, and fish by-products processing into pet-food ingredients. The authors found that the environmental impacts were the highest for food-stuff and by-products processing and that the sustainability of aquafeed was also concerning. They also underlined the challenge of applying LCA on different farm systems, due to the differences in production systems (e.g., flow-through vs. recirculating system), in processing techniques, and in management. They also note that “only two among the published LCA on salmonids managed to gather data on commercial aquafeed formulation directly from feed and farming companies” which tends to explain the low prevalence of such comprehensive LCA analyses and the issues about supply chain transparency discussed above (Qian et al., 2022).
To evaluate the impacts of ABF/ABP on supply chain sustainability, another strategy is to focus on parts of the supply chain. Quantitative data analysis and modeling approaches can provide leverage to favor best practices. Mouléry and colleagues modeled the food system of beef production in an area of a 100 km radius around Avignon, France (Mouléry et al., 2022). They found that the factors driving the use of short supply chains are the number of beef-feeding areas and the connectivity between them. The authors also included the distance from the nearest slaughterhouse in their analysis. Such area-focused modeling approaches provide a strong basis for organizing supply chains, and the integration of processing, storage, and retailing is desirable.
Raw or processed meat and dairy foods are particularly dependent on refrigeration systems, which cold chains expand from processing to retailers. For example, a modeling approach on cold chains and storage of cooked ham identified leverage points to reduce food waste and energy consumption (Guillier et al., 2016). Comparative studies of designs of cold systems have been conducted, for example at the supermarket levels (Salehy et al., 2023). Interestingly, shorter cold chains and highly efficient fridges can also be coupled with better week-menu planning in collective dining facilities to help reduce energy consumption in a significant manner (Guillier et al., 2016).
A work by Batista et al. (2015) proposed a framework to evaluate the food waste scenarios and potential by-product synergies of supply chains. They termed their framework “EFOS” (Eco Food Supply Chain), which brings concepts of industrial ecology (and related industrial symbiosis) to supporting waste analysis, recycling and innovative synergies in the context of supply chains. More studies are desirable on how processing machines, refrigeration systems, waste and by-products can be recycled or re-used. Finally, retailing, consumption models and governance models are important parts of supply chains. How governance models can drive upstream more sustainable processing deserves more attention (Duncan et al., 2022).
3 Discussion
In the first section of this paper, we highlighted the complexity of the processing techniques of ABF and ABP, and described some of their externalities on sustainability. We also identified a number of research gaps and proposed future work to better evaluate the impacts of processing on sustainability. In the second section, we reviewed studies that have evaluated the environmental impacts of ABF and ABP, and their strategy to do so. We underlined the lack of exhaustive assessments of processing and building sustainability. In the last section, we saw that the supply chains of processed ABF and ABP are particularly complex. Studies highlighted that cold chain storage, in particular, has an important impact and that long supply chains, in particular for ultra-processed or ultra-formulated products, may have stronger environmental impacts. To conclude, we propose several perspectives and future research directions.
First, we propose that more holistic, detailed life cycle assessments of ABF and ABP processing, and their geographic routes, using more quantitative indicators are desirable. In particular, more work is needed to assess the social, technical, and economic externalities linked to processing, as highlighted in Figure 1. To do so, there is a need to overcome the knowledge gaps that the authors we cited pointed out, in particular knowing the processing steps of the marketed ABF and ABP. How can we encourage the measurement (or disclosure) of environmental and social impacts of all the actors involved in the manufacturing and origin of raw materials (or compounds) used in ABF and ABP processing? One way is perhaps to rely on regulatory bodies to encourage more transparency. This is starting to happen as governments and institutions, such as the FAO, aim to improve food security at a regional or national level, to anticipate and establish early warning systems in cases of climate crisis or social conflicts (Hueston and McLeod, 2012; FAO Report, 2021). For example, the traceability of animals and transformed products has been implemented in many countries, based on strategies using systematic labeling, rational encoding, and appropriate data management (Madec et al., 2001). Similar measures could be developed to evaluate the environmental and social impacts of ABF and ABP at the scale of supply chains. Moreover, how such information could be shared with consumers to help them making better choices is an open question. Nonetheless, previous studies suggested imposing more stringent policies to improve our food systems (Fanzo et al., 2021). For more information about policy frameworks in the context of ABF, we invite the reader to read the excellent book published by Thoma and colleagues (Thoma et al., 2018). Legislative aspects of ABP are discussed by Mullen and colleagues (Mullen et al., 2017).
Second, we would like to encourage further research to test the sustainability of local hubs for production, processing, storage, and distribution, and include societal aspects such as governance models (e.g., Duncan et al., 2022), consumers’ practices and choices. Implementation research and living labs should be encouraged to test a variety of processing setups integrated within local supply chains, with the aim to maximize product qualities (nutritional value, safety, and shelf-life) and reduce the negative environmental impacts along the indicators mentioned in this paper and Table 1. Such an initiative, however, will require important planning efforts at the community level. Along with this idea, the European initiative called Farm-2-Fork encourages local hubs for the production and processing of food to achieve food resilience. How this will translate locally will depend on the efforts of city and regional councils, and other regional institutions, and if funds are effectively distributed to the stakeholders with best practices.
Author contributions
AG: Writing – review & editing, Writing – original draft. AF: Writing – review & editing, Writing – original draft. CÁ: Writing – review & editing, Writing – original draft. MB: Writing – review & editing, Writing – original draft. HM: Writing – review & editing, Writing – original draft. A-MM: Writing – review & editing, Writing – original draft. LK: Writing – review & editing, Writing – original draft.
Funding
The author(s) declare that financial support was received for the research, authorship, and/or publication of this article. This work was supported by the INRAE Syalsa (“Food systems and human health”) metaprogram within the framework of the DURATRANSFO project (“Food processing and sustainability of food systems”).
Conflict of interest
The authors declare that the research was conducted in the absence of any commercial or financial relationships that could be construed as a potential conflict of interest.
Publisher’s note
All claims expressed in this article are solely those of the authors and do not necessarily represent those of their affiliated organizations, or those of the publisher, the editors and the reviewers. Any product that may be evaluated in this article, or claim that may be made by its manufacturer, is not guaranteed or endorsed by the publisher.
References
Alao, B. O., Falowo, A. B., Chulayo, A., and Muchenje, V. (2017). The potential of animal by-products in food systems: production, prospects and challenges. Sustain. 9:1089. doi: 10.3390/su9071089
Alves, E. C., Alves, I. H., Soares, B. B., Borges, A. F., Jalal, A., Jani, A. D., et al. (2023). Resource recovery of biological residues from the Brazilian poultry industry in mitigating environmental impacts: a life cycle assessment (LCA) approach. J. Clean. Prod. 416:137895. doi: 10.1016/j.jclepro.2023.137895
Alzamora, S. M., Lopez-Malo, A., Tapia, M. S., and Welti-Chanes, J. (2016). Minimally processed foods. Encycl. Food Health, 767–771. doi: 10.1016/B978-0-12-384947-2.00470-0
Arvanitoyannis, I. S., and Kotsanopoulos, K. V. (2012). Smoking of fish and seafood: history, methods and effects on physical, nutritional and microbiological properties. Food Bioprocess Technol. 5, 831–853. doi: 10.1007/s11947-011-0690-8
Barreca, F., and Cardinali, G. D. (2019). ITACAFood: a model to certificate the sustainability of food processing facilities. Sustain. For. 11:4601. doi: 10.3390/su11174601
Barzegar, F., Kamankesh, M., and Mohammadi, A. (2019). Heterocyclic aromatic amines in cooked food: a review on formation, health risk-toxicology and their analytical techniques. Food Chem. 280, 240–254. doi: 10.1016/j.foodchem.2018.12.058
Batista, L., Saes, L., and Fouto, N. (2015). Sustainability of food supply chains - mapping food waste and byproduct synergies. Proceedings of the 20th Annual Conference of The Chartered Institute of Logistics & Transport, Logistics Research Network (LRN), Derby, 9 -11 September 2015.
Belchior, C., Boteler, B., Jansen, H.M., and Piet, G.J. (2016). Seafood in Europe: a food system approach for sustainability. Report/European Environment Agency EEA Report No 25/2016. doi: 10.2800/06589
Béné, C., Fanzo, J., Prager, S. D., Achicanoy, H. A., Mapes, B. R., Alvarez, T. P., et al. (2020). Global drivers of food system (un)sustainability: a multi-country correlation analysis. PLoS One 15:e0231071. doi: 10.1371/journal.pone.0231071
Bhat, Z. F., Morton, J. D., Bekhit, A. E. D. A., Kumar, S., and Bhat, H. F. (2022). Non-thermal processing has an impact on the digestibility of the muscle proteins. Crit. Rev. Food Sci. Nutr. 62, 7773–7800. doi: 10.1080/10408398.2021.1918629
Bhat, Z. F., Morton, J. D., Mason, S. L., and Bekhit, A. E. A. (2019). Current and future prospects for the use of pulsed electric field in the meat industry. Crit. Rev. Food Sci. Nutr. 59, 1660–1674. doi: 10.1080/10408398.2018.1425825
Bhat, Z. F., Morton, J. D., Mason, S. L., and Bekhit, A. E. A. (2020). The application of pulsed electric field as a sodium reducing strategy for meat products. Food Chem. 306:125622. doi: 10.1016/j.foodchem.2019.125622
Bräutigam, K. R., Jörissen, J., and Priefer, C. (2014). The extent of food waste generation across EU-27: different calculation methods and the reliability of their results. Waste Manag. Res. 32, 683–694. doi: 10.1177/0734242X14545374
Clark, M., Springmann, M., Rayner, M., Scarborough, P., Hill, J., Tilman, D., et al. (2022). Estimating the environmental impacts of 57,000 food products. PNAS 119:e2120584119. doi: 10.1073/pnas.2120584119
Collignan, A., Bohuon, P., Deumier, F., and Poligné, I. (2001). Osmotic treatment of fish and meat products. J. Food Eng. 49, 153–162. doi: 10.1016/S0260-8774(00)00215-6
Cooper, R. G. (1994). New products: the factors that drive success. Int. Mark. Rev. 11, 60–76. doi: 10.1108/02651339410057527
Cross, A. J., and Sinha, R. (2004). Meat-related mutagens/carcinogens in the etiology of colorectal cancer. Environ. Mol. Mutagen. 44, 44–55. doi: 10.1002/em.20030
Da Silva, J. T., Garzillo, J. M. F., Rauber, F., Kluczkovski, A., Rivera, X. S., Lopes da Cruz, G., et al. (2021). Greenhouse gas emissions, water footprint, and ecological footprint of food purchases according to their degree of processing in Brazilian metropolitan areas: a time-series study from 1987 to 2018. Lancet Planet Health. 2021, e775–e785.
Davidou, S., Christodoulou, A., Frank, K., and Fardet, A. (2021). A study of ultra-processing marker profiles in 22,028 packaged ultra-processed foods using the Siga classification. J. Food Comp. Anal. 99:103848. doi: 10.1016/j.jfca.2021.103848
Deshwal, G. K., Akshit, K. S., Sharma, H., Singh, A. K., Panjagari, N. R., and Meena, G. S. (2021). Applications of reverse osmosis in dairy processing: an Indian perspective. J. Food Sci. Technol. 58, 3676–3688. doi: 10.1007/s13197-020-04958-6
Dicken, S.J., and Batterham, R.L. (2022). Ultra-processed food: A global problem requiring a global solution
Duncan, J., DeClerck, F., Báldi, A., Treyer, S., Aschemann-Witzel, J., Cuhls, K., et al. (2022). Democratic directionality for transformative food systems research. Nat Food 3, 183–186. doi: 10.1038/s43016-022-00479-x
EEA. Annual European Union greenhouse gas inventory 1990–2017 and inventory report 2019. (2019) Available at: https://www.eea.europa.eu//publications/european-union-greenhouse-gas-inventory-2019
El-Gazzar, F. E., and Marth, E. H. (1991). Ultrafiltration and reverse osmosis in dairy technology: a review. J. Food Prot. 54, 801–809. doi: 10.4315/0362-028X-54.10.801
Espinosa-Marrón, A., Adams, K., Sinno, L., Cantu-Aldana, A., Tamez, M., Marrero, A., et al. (2022). Environmental impact of animal-based food production and the feasibility of a shift toward sustainable plant-based diets in the United States. Front. Sustain. 3:841106. doi: 10.3389/frsus.2022.841106
Fanzo, J., Haddad, L., Schneider, K. R., Béné, C., Covic, N. M., Guarin, A., et al. (2021). Viewpoint: rigorous monitoring is necessary to guide food system transformation in the countdown to the 2030 global goals. Food Policy 104:100784:102163. doi: 10.1016/j.foodpol.2021.102163
Fardet, A., and Rock, E. (2018). In vitro and in vivo antioxidant potential of milks, yoghurts, fermented milks and cheeses: a narrative review of evidence. Nutr. Res. Rev. 31, 52–70. doi: 10.1017/S0954422417000191
Fardet, A., and Rock, E. (2020). Ultra-processed foods and food system sustainability: what are the links? Sustain. For. 12:6280. doi: 10.3390/su12156280
Fardet, A., and Rock, E. (2022). Chronic diseases are first associated with the degradation and artificialization of food matrices rather than with food composition: calorie quality matters more than calorie quantity. Eur. J. Nutr. 62, 2239–2253. doi: 10.1007/s00394-021-02786-8
Foley, J. A., Ramankutty, N., Brauman, K., Cassidy, E. S., Gerber, J., Jonhston, M., et al. (2011). Solutions for a cultivated planet. Nature 478, 337–342. doi: 10.1038/nature10452
Garzillo, J. M. F., Poli, V. F. S., Leite, H. M. L., Fernanda, L. F. H. M., Steele, E. M., Machado, P. P., et al. (2022). Ultra-processed food intake and diet carbon and water footprints: a national study in Brazil. Rev. Saude Publica 56:6. doi: 10.11606/s1518-8787.2022056004551
Genné, I., and Derden, A. (2008). Handbook of water and energy Management in Food Processing. Woodhead Pub. Ser. Food Sci. Technol. Nutr., 805–815. doi: 10.1533/9781845694678.6.805
Gómez, B., Munekata Paulo, E. S., Gavahian, M., Barba, F. J., Martí-Quijal, F. J., Bolumar, T., et al. (2019). Application of pulsed electric fields in meat and fish processing industries: an overview. Food Res. Int. 123, 95–105. doi: 10.1016/j.foodres.2019.04.047
Grasso, S., and Jaworska, S. (2020). Part meat and part plant: are hybrid meat products fad or future? Food Secur. 9:1888. doi: 10.3390/foods9121888
Guillier, L., Duret, S., Hoang, H. M., Flick, D., and Laguerre, O. (2016). Is food safety compatible with food waste prevention and sustainability of the food chain? Procedia Food Science 7, 125–128. doi: 10.1016/j.profoo.2016.05.001
Hueston, W., and McLeod, A. (2012). “Overview of the global food system: changes over time/space and lessons for future food safety” in Institute of Medicine (US). Improving food safety through a one health approach: Workshop summary (Washington, DC: National Academies Press (US)).
Jinadasa, B. K. K. K., Monteau, F., and Fowler, S. W. (2020). Review of polycyclic aromatic hydrocarbons (PAHs) in fish and fisheries products; a Sri Lankan perspective. Environ. Sci. Pollut. Res. 27, 20663–20674. doi: 10.1007/s11356-020-08305-2
Joshi, V., and Kumar, S. (2015). Meat analogues: plant based alternatives to meat products-a review. Int. J. Food Ferment. Technol. 5, 107–119. doi: 10.5958/2277-9396.2016.00001.5
Kahiluoto, H., Kuisma, M., Kuokkanen, A., Mikkilä, M., and Linnanen, L. (2014). Taking planetary nutrient boundaries seriously: can we feed the people? Glob. Food Sec. 3, 16–21. doi: 10.1016/j.gfs.2013.11.002
Kang, D. H., Louis, F., Liu, H., Shimoda, H., Nishiyama, Y., Nozawa, H., et al. (2021). Engineered whole cut meat-like tissue by the assembly of cell fibers using tendon-gel integrated bioprinting. Nat. Commun. 12:5059. doi: 10.1038/s41467-021-25236-9
Katajajuuri, J. M., Grönroos, J., and Usva, K. (2008). “Environmental impacts and related options for improving the chicken meat supply chain” in In: 6th international conference on LCA in the Agri-food sector. (Zurich: Natural Resource Institute Finland).
Kranert, M., Hafner, G., Barabosz, J., Schuller, H., Lebersorger, S., Leverenz, D., et al., (2012). Ermittlung der weggeworfenen Lebensmittelmengen und Vorschläge zur Verminderung der Wegwerfrate bei Lebensmitteln in Deutschland. Studie der Universität Stuttgart (gefördert vom BMELV) Stuttgart. Available at: http://www.bmelv.de/SharedDocs/Downloads/Ernaehrung/WvL/Studie_Lebensmittelabfaelle_Langfassung.pdf (Accessed February 9, 2023).
Madec, F., Geers, R., Vesseur, P., Kjeldsen, N., and Blaha, T. (2001). Traceability in the pig production chain. Rev. Sci. Tech. Off. Int. Epiz. 20, 523–537. doi: 10.20506/rst.20.2.1290
Maiolo, S., Forchino, A. A., Faccenda, F., and Pastres, R. (2020). From feed to fork – life cycle assessment on an Italian rainbow trout (Oncorhynchus mykiss) supply chain. J. Clean. Prod. 289:125155.
Malak-Rawlikowska, A., Majewski, E., Wąs, A., Borgen, S. O., Csillag, P., Donati, M., et al. (2019). Measuring the economic, environmental, and social sustainability of short food supply chains. Sustain. For. 11:4004. doi: 10.3390/su11154004
Mejia, M. A., Harwatt, H., Jaceldo-Siegl, K., Soret, S., and Sabate, J. (2016). The future of meat: exploring the nutritional qualities and environmental impacts of meat replacements. FASEB J. 30:894.8-894.8. doi: 10.1096/fasebj.30.1_supplement.894.8
Mofijur, M., Rizwanul Fattah, I. M., Senthil, K. P., Sk, Y. A. S., Ashrafur Rahman, S. M., Ahmed, S. F., et al. (2021). T.M.I. Bioenergy recovery potential through the treatment of the meat processing industry waste in Australia. J. Env. Chem. Eng. 9:105657.
Monteiro, C. A., Cannon, G., Levy, R. B., Moubarac, J. C., Louzada, M. L., Rauber, F., et al. (2022). Ultra-processed foods: what they are and how to identify them. Public Health Nutr. 22, 936–941. doi: 10.1017/S1368980018003762
Mouléry, M., Sanz Sanz, E., Debolini, M., Napoléone, C., Josselin, D., Mabire, L., et al. (2022). Self-sufficiency assessment: defining the Foodshed spatial signature of supply chains for beef in Avignon, France. Agriculture 12:419. doi: 10.3390/agriculture12030419
Mozhiarasi, V., and Natarajan, T. S. (2022). Slaughterhouse and poultry wastes: management practices, feedstocks for renewable energy production, and recovery of value added products. Biomass Convers. Biorefinery 10, 1–24. doi: 10.1007/s13399-022-02352-0
Mullen, A. M., Álvarez, C., Zeugolis, D. I., Henchion, M., O'Neill, E., and Drummond, L. (2017). Alternative uses for co-products: harnessing the potential of valuable compounds from meat processing chains. Meat Sci. 132, 90–98. doi: 10.1016/j.meatsci.2017.04.243
Niebuhr, S. E., Larson, E. M., and Dickson, J. S. (2020). The effects of high hydrostatic pressure on the color, texture and microbiology of selected pork organ meats. Adv. Food Process. Technol. 1:125.
Nunes, J., Silva, P. D., Andrade, L.P., and Gaspar, P.D. (2014). Potential of energy savings in Portuguese meat industry. Proceedings 8th International Conference on Energy and Development, Environment and Biomedicine (EDEB’14), Lisbon, Portugal.
Olarerin-George, A. O., and Hogenesch, J. B. (2015). Assessing the prevalence of mycoplasma contamination in cell culture via a survey of NCBI's RNA-seq archive. Nucleic Acids Res. 43, 2535–2542. doi: 10.1093/nar/gkv136
O'Neill, E. N., Cosenza, Z. A., Baar, K., and Block, D. E. (2021). Considerations for the development of cost-effective cell culture media for cultivated meat production. Comp. Rev. Food Sci. Food Safety 20, 686–709. doi: 10.1111/1541-4337.12678
Pagliai, G., Dinu, M., Madarena, M. P., Bonaccio, M., Iacoviello, L., and Sofi, F. (2020). Consumption of ultra-processed foods and health status: a systematic review and meta-analysis. Br. J. Nutr. 125, 308–318. doi: 10.1017/S0007114520002688
Pardo, G., Ciruelos, A., Lopez, N., Gonzalez, L., Ramos, S., and Zufia, J. (2012). “Environment improvement of a chicken product through life cycle assessment methodology” In: 8th conference on LCA in the Agri-food sector (Saint-Malo, France), 86–91.
Petit, G., Sablayrolles, C., and Yannou-Le, B. G. (2018). Combining eco-social and environmental indicators to assess the sustainability performance of a food value chain: a case study. J. Clean. Prod. 191, 135–143. doi: 10.1016/j.jclepro.2018.04.156
Picart-Palmade, L., Cunault, C., Chevalier-Lucia, D., Belleville, M.-P., and Marchesseau, S. (2019). Potentialities and limits of some non-thermal technologies to improve sustainability of food processing. Front. Nutr. 5:130. doi: 10.3389/fnut.2018.00130
Poore, J., and Nemecek, T. (2018). Reducing food’s environmental impacts through producers and consumers. Science 360, 987–992. doi: 10.1126/science.aaq0216
Portanguen, S., Tournayere, P., Sicard, J., Astruc, T., and Mirade, P. S. (2019). Toward the design of functional foods and biobased products by 3D printing: a review. Trends Food Sci. Technol. 86, 188–198. doi: 10.1016/j.tifs.2019.02.023
Post, M. J., Levenberg, S., Kaplan, D. L., Genovese, N., Fu, J., Bryant, C. J., et al. (2020). Scientific, sustainability and regulatory challenges of cultured meat. Nature Food 1, 403–415. doi: 10.1038/s43016-020-0112-z
Rathod, N. B., Kulawik, P., Ozogul, Y., Ozogul, F., and Bekhit, A. E.-D. A. (2022). Recent developments in non-thermal processing for seafood and seafood products: cold plasma, pulsed electric field and high hydrostatic pressure. Int. J. Food Sci. Technol. 57, 774–790. doi: 10.1111/ijfs.15392
Regulation Report. (2004). Regulation (EC) No 852/2004 of the European Parliament and of the Council of 29 April 2004 on the hygiene of foodstuffs. Official Journal of the European Communities. Available at: https://eur-lex.europa.eu/legal-content/FR/TXT/?uri=celex%3A32004R0852
Salehy, Y., Delahaye, A., Hoang, H. M., Fournaison, L., Cluzel, F., Leroy, Y., et al. (2023). Choosing an optimized refrigeration system based on sustainability and operational scenarios applied to four supermarket architectures in three European countries. J. Clean. Prod. 392:136307.
Salque, M., Bogucki, P. J., Pyzel, J., Sobkowiak-tabaka, I., Grygiel, R., Szmyt, M., et al. (2013). Earliest evidence for cheese making in the sixth millennium BC in northern Europe. Nature 494, 522–555.
Skunca, D., Tomasevic, I., and Djekic, I. (2015). Environmental performance of the poultry meat chain – LCA approach. Procedia Food Sci. 5, 258–261. doi: 10.1016/j.profoo.2015.09.074
Soladoye, P. O., Juárez, M., Estévez, M., Fu, Y., and Álvarez, C. (2022). Exploring the prospects of the fifth quarter in the 21st century. Compr. Rev. Food Sci. Food Saf. 21, 1439–1461. doi: 10.1111/1541-4337.12879
Sovacool, B. K., Bazilian, M., Griffiths, S., Kim, J., Foley, A., and Rooney, D. (2021). Decarbonizing the food and beverages industry: a critical and systematic review of developments, sociotechnical systems and policy options. Renew. Sust. Energ. Rev. 143:110856. doi: 10.1016/j.rser.2021.110856
Suksatan, W., Moradi, S., Naeini, F., Bagheri, R., Mohammadi, H., Talebi, S., et al. (2022). Ultra-processed food consumption and adult mortality risk: a systematic review and dose-response Meta-analysis of 207,291 participants. Nutrients 14:174. doi: 10.3390/nu14010174
Taylor, B., and Walsh, M. (2002). Development and sensory analysis of a textured whey protein meatless patty. J. Food Sci. 67, 1555–1558. doi: 10.1111/j.1365-2621.2002.tb10321.x
Thoma, G. J., Ellsworth, S. W., and Yan, M. J. (2018). Principles of green food processing (including lifecycle assessment and carbon footprint). Alternatives to conventional food processing, ed. P. Andrew London, UK: Royal Society of Chemistry, 508.
United Nations General Assembly (2015). Transforming our world: the 2030 agenda for sustainable development, 21 October 2015. Available at: https://digitallibrary.un.org/record/3923923?v=pdf
Valsasina, L., Pizzol, M., Smetana, S., Georget, E., Mathys, A., and Heinz, V. (2017). Life cycle assessment of emerging technologies: the case of milk ultra-high pressure homogenisation. J. Clean. Prod. 142, 2209–2217. doi: 10.1016/j.jclepro.2016.11.059
Varlet, V., Sérot, T., Monteau, F., Le Bizec, B., and Prost, C. (2007). Determination of PAH profiles by GC–MS/MS in salmon processed by four cold-smoking techniques. Food Addit. Contam. 24, 744–757. doi: 10.1080/02652030601139946
Varun, K., Arunchala, U. C., and Vijayan, P. K. (2022). Sustainable mechanism to popularise round the clock indoor solar cooking – part I: global status. J. Energy Storage 54:105361. doi: 10.1016/j.est.2022.105361
Veiga, L. B. E., de Souza, L. S. Q., and Vendramel, S. M. R. (2022). Environmental aspects in dairy processing, in dairy foods. Oxford, UK: Woodhead Publishing, 311–337.
Verduna, T., Blanc, S., Merlino, V. M., Cornale, P., and Battaglini, L. M. (2020). Sustainability of four dairy farming scenarios in an alpine environment: the case study of Toma di Lanzo cheese. Front. Vet. Sci. 7:569167. doi: 10.3389/fvets.2020.569167
Wang, T., Kaur, L., Furuhata, Y., Aoyama, H., and Singh, J. (2022). 3D printing of textured soft hybrid meat analogues. Food Secur. 11:478. doi: 10.3390/foods11030478
Yaldagard, M., Ali, S., Seyed, M., and Tabatabaie, F. (2008). The principles of ultra high pressure technology and its application in food processing/preservation: a review of microbiological and quality aspects. Afr. J. Biotechnol. 7, 2739–2767.
Zhang, X., Jiang, D., Li, J., Zhao, Q., and Zhang, M. (2024). Carbon emission oriented life cycle assessment and optimization strategy for meat supply chain. J. Clean. Prod. 439:140727. doi: 10.1016/j.jclepro.2024.140727
Keywords: animal products, food security, supply chain, sustainability, energy, food processing
Citation: Germond A, Fardet A, Álvarez García C, Boland M, Ming Hoang H, Mullen A-M and Kaur L (2024) Analyzing the complexity of animal products’ processing and its impact on sustainability. Front. Sustain. Food Syst. 8:1424282. doi: 10.3389/fsufs.2024.1424282
Edited by:
Guadalupe Virginia Nevárez-Moorillón, Autonomous University of Chihuahua, MexicoReviewed by:
Rogelio Sánchez Vega, Autonomous University of Chihuahua, MexicoSayantani Dutta, Universidad de Salamanca, Spain
Copyright © 2024 Germond, Fardet, Álvarez García, Boland, Ming Hoang, Mullen and Kaur. This is an open-access article distributed under the terms of the Creative Commons Attribution License (CC BY). The use, distribution or reproduction in other forums is permitted, provided the original author(s) and the copyright owner(s) are credited and that the original publication in this journal is cited, in accordance with accepted academic practice. No use, distribution or reproduction is permitted which does not comply with these terms.
*Correspondence: Arno Germond, QXJuYXVkLmdlcm1vbmRAaW5yYWUuZnI=; Lovedeep Kaur, TC5LYXVyQG1hc3NleS5hYy5ueg==
†ORCID: Arno Germond, https://orcid.org/0000-0002-2196-7566
Lovedeep Kaur, https://orcid.org/0000-0002-2789-0416