- 1College of Life Sciences, Nanjing Agricultural University, Nanjing, China
- 2Zhenjiang Academy of Agricultural Sciences and Jiangsu Academy of Agricultural Sciences, Jurong, China
- 3Institute of Soil Science, Chinese Academy of Sciences, Nanjing, China
- 4The Collaborated Lab of Plant Molecular Ecology (Between the College of Life Sciences of Nanjing Agricultural University and the Asian Natural Environmental Science Center of the University of Tokyo), Nanjing Agricultural University, Nanjing, China
- 5National Joint Local Engineering Research Center for Rural Land Resources Use and Consolidation, Nanjing Agricultural University, Nanjing, China
Context and background: Microplastics (MPs) and heavy metals (HMs) coexist in the farmland of China.
Motivation: It still remains unclear the extent of their exposure and distribution in sweet potato fields.
Hypothesis: Polyethylene (PE) or polyamide (PA) is the main MP pollutant in contaminated sweet potato sites, and the MP abundance in low-latitude and eastern areas is highest. Methods: In this study, saturated NaCl solution, a stereo microscope, a Fourier transform infrared spectrometer, and an electrothermal digester are used for the extraction, observation, identification of MPs, and analysis of Cd elements in soil, respectively.
Methods: In this study, saturated NaCl solution, a stereo microscope, a Fourier transform infrared spectrometer, and an electrothermal digester are used for the extraction, observation, identification of MPs, and analysis of Cd elements in soil, respectively.
Results: Here, we found an average MP level of 112,400 items/kg in 30 sweet potato field sites based on the items in 5 g soil and the magnification (200x), and the maximum abundance was 197,153 items/kg in Laiyang city, Shandong province, by field survey. The distribution characteristics of MPs are middle-latitude areas < low-latitude areas, and eastern areas > central areas. Most MPs are of the fragment and film shape, which account for 47.96 and 40.22%, respectively. In order to detect MP polymers in three cities named “Liancheng,” “Huanggang,” and “Laiyang” with different degrees of development, a laser infrared imaging system was used as a novel instrument to explore the MPs larger than 10 μm.
Conclusion: The results showed that PA is the main MP pollutant in contaminated sweet potato sites, and soil texture, planting time, and urbanization processes may be the main factors affecting MP distribution. The average cadmium (Cd) concentration in 215 field sites is 0.15 mg/kg, and the local Cd pollution is existing, but the overall pollution is low. In addition, Cd concentration was negatively correlated with MP abundance. This study reveals the status of MP and also Cd pollution in sweet potato fields, which provides a theoretical basis for the safe production and utilization of sweet potato fields.
1 Introduction
Microplastic (MP) pollution in the terrestrial system is serious due to poor degradation, and the accumulation of MPs in the soil may adversely affect soil ecosystems and threaten human and animal health through the food chain (Rillig et al., 2019). Because of their low surface area, 0.1% high-density polyethylene (HDPE), 0.1% polylactic acid (PLA), and 0.001% synthetic fibers have adverse effects on the growth of plants and soil protozoa (such as earthworms), as well as the quality and moisture of soil (Boots et al., 2019; Mbachu et al., 2021). The effect of MPs on biomass and the physiology of plants, as well as the physicochemical properties of soil, depends on the MP species, size and shape (Rillig et al., 2019; Pflugmacher et al., 2020). For example, soil aggregation was reduced due to the potential for all mixed fragments, foams, films, and fiber MPs to destroy the internal structure of soil aggregates and have a potentially negative impact on soil organisms (Lozano et al., 2021). MPs can also be weathered under the conditions of hydrolysis, photo-oxidation, thermal oxidation, biodegradation, mechanical fragmentation, etc. During weathering, along with the reduction of particle size, the surface becomes rough, and functional groups are produced (Jahnke et al., 2017; Ding et al., 2020). Since little is known about the toxicity of MPs in soil over time, it is necessary to analyze the contamination status of MPs in farmland.
As a globally important food crop, sweet potatoes rank third in yield among all crops in the world and fifth in their contribution to calories in the human body (Wang et al., 2021a). China is the world’s largest sweet potato grower, with a sweet potato production area of approximately 6.6 million hectares, accounting for 70% of the world’s sweet potato cultivation area. In addition, China’s sweet potato production is approximately 100 million tons, accounting for 84.4% of the world’s total production (Gao et al., 2000; Wang et al., 2021a). In addition, sweet potatoes, as a major source of starch, can be used as a staple food or as a raw material for the production of alcohol. Therefore, relevant food safety issues cannot be ignored (Bovell-Benjamin, 2007; Ma et al., 2012; Xie et al., 2018). However, very few studies have reported on MP pollution in sweet potato fields, and their abundance, shapes, sizes, and species in fields remain unclear (Chai et al., 2020).
Cadmium (Cd) is the most contaminated heavy metal (HM) in China’s soil, accounting for 33.54 and 44.65% of soil pollution in farmland and cities, respectively (Yuan et al., 2021). Although HM contamination in sweet potato production areas has not been reported, there are few reports of the influence of Cd on sweet potato growth (Huang et al., 2020a). In these studies, sweet potato tubers grow mainly in farmland environments, and their storage roots act as the main food organs and are more likely to accumulate HMs (Lai et al., 2021). For example, some edible-type varieties can accumulate more Cd and lead (Pb) than starch-based varieties (Huang et al., 2020a). In addition, sweet potatoes with large underground biomass have higher accumulations of Cd (0.78–2.02 mg kg−1) (Lai et al., 2021). Shi et al. (2022) found that PE MPs were only found in the cortex of roots and stems, and PE MPs caused oxidative stress. Therefore, single Cd or PE treatment may pose a health risk if consumed. On the other hand, MPs exhibit great potential to increase the inhibitory effect on the growth of wheat roots for low concentrations of Cd, and the synergistic effect of MP and Cd may be attributed to the fact that aged MPs can increase the bioaccumulation of Cd in wheat (Gu et al., 2021). For example, PE MPs increase Cd uptake in lettuce by altering soil properties such as pH and dissolved organic carbon. This results in higher Cd bioaccumulation and toxicity in plants (Wang et al., 2021b). In addition, the combined pollution of MPs and Cd alters the microbial community structure and function in Pennisetum hydridum rhizosphere soil. This combination increases microbial stress and changes gene abundance related to metabolism and energy conversion (Duan et al., 2023). Another study showed that both polyester microfibers (PMFs) and co-PMFs/Cd treatments adversely affected plant growth and photosynthesis. Co-PMFs/Cd also increased the activity of antioxidant enzymes (Zeb et al., 2021). Shi et al. (2023) found that both 5% of the original PE MPs and weathered PE MPs can significantly reduce the concentration of Olsen phosphorus (P) and Olsen potassium (K) in soil, inhibit the growth of sweet potatoes, but significantly increase the Cd accumulation and glutathione (GSH) levels in the tissues of sweet potatoes, and also induce membrane lipid peroxidation, which poses a serious threat to the safe production and food safety of sweet potatoes. Therefore, Cd and MPs are our primary contaminants in this study.
Therefore, Cd and MPs coexist in farmland, and Cd could be absorbed by sweet potato from farmland soil. However, the degree of MPs and Cd pollution in sweet potato fields is unknown, and whether it poses a potential threat to the production of sweet potatoes and food safety is vital. To explore the exposure levels of MPs and Cd in sweet potato production fields in China and study their distribution and influencing factors, we explored (1) the shapes, sizes, species, and abundance of MPs in 30 sweet potato fields assessed by a stereo microscope and Fourier transform infrared (FTIR) spectrometer; (2) the sizes, numbers, and polymers of MPs in ‘Laiyang’, ‘Huanggang’, and ‘Liancheng’ assisted by a laser infrared imaging system; (3) the Cd concentration in 215 sampling sites; and also evaluated the Cd pollution status in sweet potato fields assisted by inductively coupled plasma-optical emission spectroscopy (ICP-OES).
2 Methods
2.1 Sample sites
MP and Cd pollution were investigated in 30 fields from four major sweet potato regions (northern regions, Southern regions, regions in the middle and lower reaches of the Yangtze River, and Southwest region) in China, and the size of 30 fields is between 100 and 500 m2. The 30 fields were distributed across 14 provinces, most of which were located in the central and eastern parts of China (Table 1).
2.2 Soil sample collection
For each sweet potato field, three points (two of the four corners of the rectangular field and their midpoint) were selected to collect 1 kg of surface soil samples (0–20 cm deep), which were transferred to the lab and stored in plastic bags in a 4°C refrigerator as three repetitions to prevent MP and Cd contamination. Oven-dried soil (70°C, 24 h) was sieved with a 5 mm mesh to remove stones, residues, and the roots of plants, and uniformized by mixing well before MP extraction. For each Cd pollution site, 2 kg of surface soil (0–20 cm deep) was also randomly selected from three points, and l kg of it was dried to constant weight in an oven at 60°C three times, while the remaining soils were air dried in nature for future analysis.
2.3 MP extraction
Sodium iodide (NaI) is highly efficient in extraction and recovery for MPs, but it is toxic to both the environment and humans, as well as expensive. Saturated sodium chloride (NaCl) is non-toxic and inexpensive. However, it is only suitable for the extraction of low-density MPs, and the recycling efficiency is low. The mixed extraction of NaI and NaCl is more efficient than that of NaCl solution, but the cost is also higher. In addition, because the MPs in fields are mostly low-density PE and polypropylene MPs, and in combination with the cost, we consider the use of saturated NaCl for MP extraction (Chen et al., 2022). According to Liu et al. (2018), a saturated NaCl (1.19 g cm −3) solution was used for the density separation of MPs from soil samples. Overall, 5 g of the above soil samples, which had been dried, sieved, and well mixed, were transferred to a clean glass beaker. Next, 100 mL of saturated NaCl solution was added to the beaker and mixed with each soil sample. After 2 min of ultrasonication, the soil samples were stirred evenly for 30 min, and the MPs were floated out and precipitated for 24 h. Finally, the MPs in the supernatant were collected in an Erlenmeyer flask, and the process above was repeated three times. The supernatants were filtered by a 20-μm nylon net filter and the precipitates were collected.
The precipitates on the nylon net filter were carefully transferred to an Erlenmeyer flask, mixed with 50 mL of 30% H2O2, and chemically degraded at 50°C for 72 h to completely digest the organic matter in the precipitates. The digested solution was filtered by a 20-μm nylon net filter, and then the MPs attached to filters were kept in a glass Petri dish to be stored and dried at 25 ± 2°C for observation and analysis. The MP items in 1 kg of soil were calculated based on the MP items in 5 g of soil and a magnification of 200x.
2.4 MP observation
Suspected MPs were first identified and recorded based on their shapes, surface texture, color, and luster under the stereo microscope (Stemi 2000C, Zeiss) following the methods of Liu et al. (2018) and Kor et al. (2020). After that, these plastics were picked out with tweezers for further confirmation by a FTIR (Antaris II, Thermo Fisher, Shanghai, China), which includes an ATR accessory (ATR-FTIR). Analyzing each sample by ATR-FTIR and operating the instrument in single-reflection mode provides 40 scans per particle at a resolution of 4 cm−1 in the mid-infrared range of 400 to 4,000 cm−1. When all spectra were compared to a ‘HR Hummel Polymer and Additives’ database, there were at least 70% of spectrum matches for the identification of polymer compositions. In addition, images were captured with a high-resolution camera, which allowed the counting and calculation of the abundance of MPs in each field of view after excluding non-plastic items verified by ATR-FTIR. Under the same magnification, three replicates of each sample were randomly moved to obtain 20 images, and the abundance of MPs (items/kg) was consequently calculated according to the magnification and the dry weight of the soil samples. MPs are divided into films, fragments, reticulations, and fibers based on shapes. The sizes of MPs were further grouped into class 1 (< 100 μm), class 2 (100–500 μm), class 3 (500–1,000 μm), and class 4 (> 1,000 μm) based on the longest dimension.
2.5 Identification of MP by a laser infrared imaging system
Soil samples from ‘Liancheng’, ‘Huanggang’, and ‘Laiyang’ cities, ordered from lowest to highest agricultural development degree, were selected for MP identification by a laser infrared imaging system (Agilent 8,700 LDIR, Beijing, China). One kilogram of air-dried soil samples were crushed and lightly ground before passing through a 5 mm and then 1 mm sieve. Fifty grams of sieved soil were digested with 30% H2O2 for 2–3 days until the upper liquid was clear. The precipitation was dried in an oven below 35°C. Gravity flotation was carried out with a saturated NaCl solution. Supernatants were siphoned and filtered with a 10 μm metal filter membrane. The particles on the filter membrane were rinsed with 30% H2O2 and continuously digested for several hours. Samples were filtered again with a 10 μm metal filter membrane. The membrane was placed in a thick glass tube with an aqueous ethanol solution. The tube was sonicated for 1–2 h, the ultrasonic frequency was set to the lowest, and the temperature was lower than 35°C. The concentrated samples were placed into a 2 mL glass bottle and kept at 4°C in the refrigerator. Before sampling, the liquid in the glass vials was sonicated for 10–20 min. A microdropper was used to remove approximately 20 μL of the sample and drop it on the Kevley window plate. After ethanol evaporation, the Kevley window plate with the MP sample was placed on the sample base, and the base was inserted into the sample table. Clarity software MP detection module was started, and the sample table automatically ran to the detection position and completed automatic focusing. The detection area of MPs on Kevley plate were selected, and 1800 cm−1 was set to scan the selected area quickly and accurately. The instrument automatically completed the identification positioning and photographing of the MPs in the detection area, and the identification accuracy was 1 μm. The software automatically selected the blank area without particles, collected the background spectrum, and then collected the infrared spectrum of all the identified particles in turn, completing the qualitative retrieval of the spectrum. The output results include qualitative information such as photos, sizes, and areas of all particles. Mainali et al. (2019) provided quantitative information on the number and percentage of each MP, as well as statistical information on the species distribution of MPs in different particle size ranges.
2.6 Determination of Cd in soil
For the determination of Cd in soil samples, 0.2000 ± 0.0050 g of the dried sample was sieved by a 0.15 mm stainless steel sieve, weighed, and placed in a digestion tube, mixed with 10 mL mixed acids (HNO3: HClO4 = 4:1, v/v). After digestion, the samples were resuspended in 2.5% dilute nitric acids for more than 6 h. The supernatant was filtered by a 0.45-μm microporous membrane, and the Cd concentration in the filtrate was determined by ICP-OES.
2.7 Quality control
For quality control and quality assurance (QA/QC) in MP extraction experiments, all glassware was thoroughly cleaned, washed with diluted hydrochloric acid, and rinsed with deionized water before use. The laboratory equipment and work surface were thoroughly cleaned with 70% ethanol and wiped with paper wipes before performing the analysis. Cotton lab coats were worn to prevent MP contamination, and items made of plastics (e.g., plasticware, suits, and fabrics) were avoided during this study. All the analytical instruments were calibrated prior to chemical analysis. Analyses were performed using four experimental replicates, and the results are reported as the mean ± standard deviation. Blank samples and analytical-grade reagents were used for all the analyses. The values of the blank samples were either low or below the detection limits of the corresponding method (Palansooriya et al., 2023).
For QA/QC in the Cd digestion experiment, before weighing and loading the soil samples into digestion tubes, the tubes are cleaned with dilute nitric acid (2.5%). In addition, after digestion, the digestion liquid is also filled with 2.5% nitric acid. During digestion, based on blank control (without soil samples) and a standard control (GBW07542 (GSS-51), a standard soil sample with a known Cd concentration), the recovery of Cd in the samples was 89.58%.
2.8 Statistical analysis
The data were arranged by Microsoft Excel 2010, and the figures were drawn by GraphPad Prism software 8.0. Descriptive statistics of Cd and the quantitative relationship between Cd concentration and MP abundance in sweet potato fields were performed by SPSS software 21.0, which included minimum, maximum, and average of Cd, coefficient of variation, and variance of Cd concentrations in 215 soil samples. The LSD test was used to explain the significant differences between various treatments at a p-value of <0.05.
3 Results
3.1 Exposure levels of MPs and Cd in field survey
After extracting MPs from 30 sweet potato fields and determining their amounts, a preliminary statistical result on MP pollution in sweet potato fields in China was obtained. In addition, 215 Cd-contaminated sites located above the sweet potato fields were investigated (Table 2), and a spatial distribution map of Cd-contaminated sites in sweet potato fields in China was created by ArcGIS Map 10.5 (Figure 1).
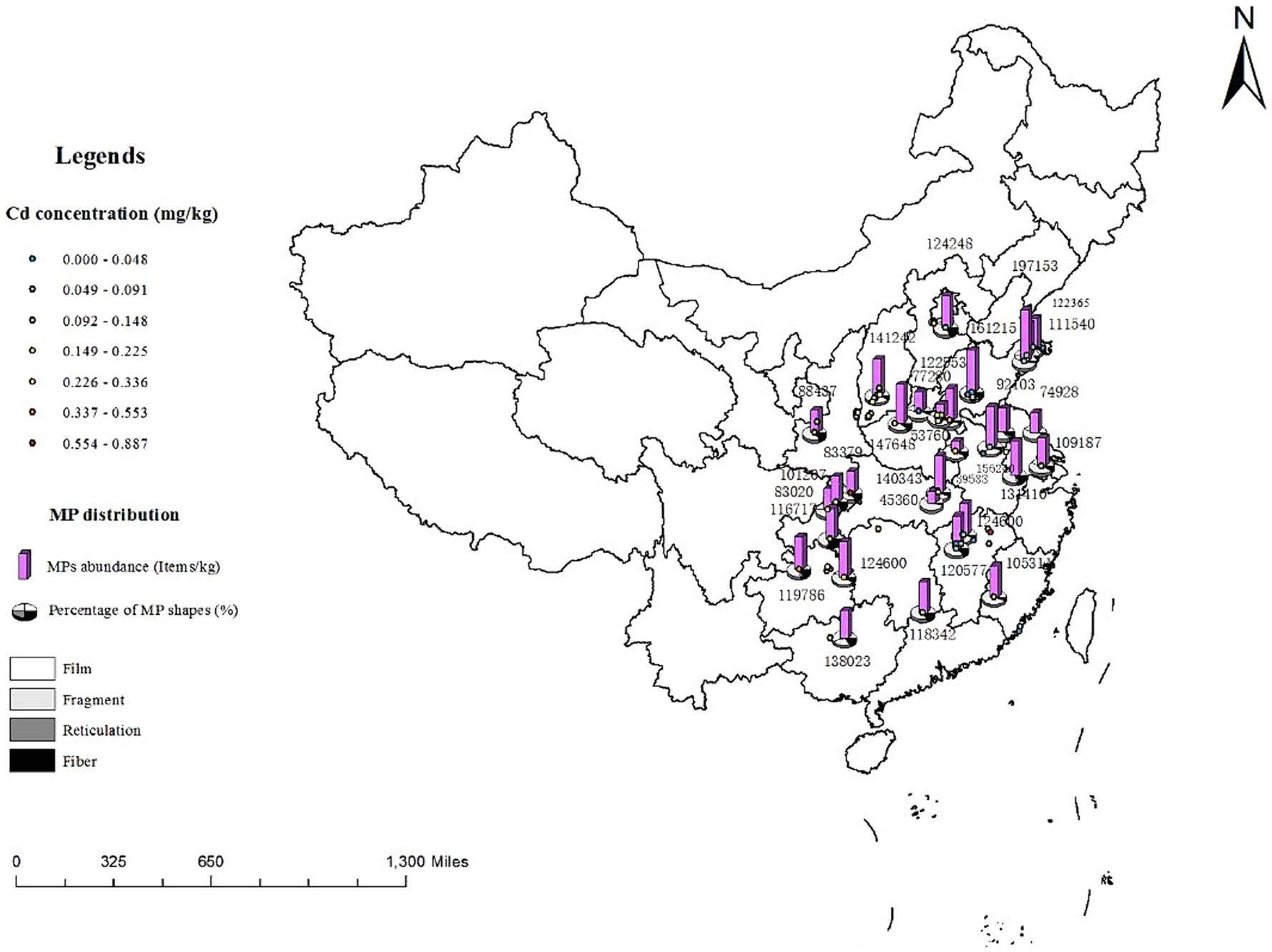
Figure 1. Spatial distribution map of Cd concentration (mg/kg), MP abundance (items/kg), and percentage of MP shapes (%) in sweet potato fields in China.
As shown in Figure 1, MPs were identified in 30 sweet potato fields, with an average level of 112,404 items/kg. MP abundance in 17 sites reached up to 120,000–200,000 items/kg. The highest MP abundance was found in Shandong province, followed by Anhui and Henan province. The abundance of MPs in the soil of Laiyang (No. 6) in Shandong province was the highest (197,153 items/kg), which was 4.99 times higher than the lowest level in the soil of Yingquan, Anhui province (No. 19; 39,533 items/kg). The average MP abundance in middle-latitude soils (30 N–60 N) was 109,280 items/kg, which was close to the average abundance of all 30 field sites and a little lower than that of low-latitude soils (0 N–30 N), where the average MP abundance was 120,995 items/kg. The average MP abundance in eastern area soils (longitude >115° E) was 116,325 items/kg, which was higher than that of central area soils (107,922 items/kg).
The average concentration of Cd in 215 soil samples was 0.15 mg/kg, which was higher than the Cd background value of Chinese soil (0.097 mg/kg) but lower than the risk screening threshold (0.3 mg/kg) of Cd in Chinese contaminated agricultural soil (GB 15618-2018, Soil environmental quality Risk control standard for soil contamination of agricultural land, Ministry of Ecology and Environment and the State Administration for Market Regulation of the People’s Republic of China, 2018). The spatial distribution map of Cd in sweet potato fields showed that the Cd pollution in central China was more serious than that in eastern China (Figure 1).
3.2 MP shapes
We categorized the MPs that were extracted from the 30 sites into four categories based on their shapes: film, fragment, fiber, or reticulation. The photos of MP shapes are shown in Supplementary Figure S1. In all MP samples, the proportion of MP shapes was fragment (47.96%) > film (40.22%) > fiber (11.02%) > reticulation (0.80%). Filmy and fragmented MPs were the two most commonly shapes, and the proportion of fibrous and reticulated MPs were relatively rarely. Especially, reticular MPs, which were only found in eight field sites (Figure 1).
3.3 MP size
The size distribution of different shapes of MPs was obtained based on their longest dimension (Figure 2). The size of 97.92% of MPs was almost smaller than 1,000 μm, and 42.35% of the MPs were less than 100 μm in size, followed by 500–1,000 μm (32.07%), 100–500 μm (23.50%), and > 1,000 μm (2.08%). A total of 77.28% of the fragmented MPs were less than 100 μm in size, followed by 100–500 μm (21.94%), 500–1,000 μm (0.78%), and > 1,000 μm (0%) MPs. For filmy MPs, 59.15% of MPs were 500–1,000 μm in size, followed by 100–500 μm (24.20%), <100 μm (13.13%), and >1,000 μm (3.52%). Compared with filmy and fragmented MPs, reticular and fibrous MPs were larger but accounted for a small proportion.
3.4 MP polymers
A novel research method of investigating the polymers of MPs was undertaken using a laser infrared imaging system (8,700 LDIR). The soil samples from ‘Liancheng,’ ‘Huanggang,’ and ‘Laiyang’ were selected for MP identification, and the degree of agricultural development was ‘Laiyang’ > ‘Huanggang’ > ‘Liancheng’. Both ‘Liancheng’ and ‘Laiyang’ have 11 polymers of MPs, 10 of which are the same. Compared with ‘Liancheng’ and ‘Laiyang’, polyvinyl chloride (PVC), polyacetal, polyurethane (PU), polycarbonate (PC), and polystyrene (PS) were not checked in ‘Huanggang.’ The main polymers of MPs in ‘Liancheng,’ ‘Huanggang,’ and ‘Laiyang’ were polyamide (PA), acrylonitrile butadiene (AB), and natural PA (NP); PA and NP; and PA, PU, NP, and AB, respectively. PA was the main MP pollutant in the contaminated sweet potato field sites, accounting for 31.1, 69.6, and 32.5% of total polymers.
3.5 Linear regression analysis of Cd concentration and MP abundance
The results of the quantitative relationship between Cd concentration and MP abundance in sweet potato fields showed that Cd concentration was negatively correlated with MP abundance, and the regression equation is y = 168943.791–98038.942 * x (y: MP abundance; x: Cd concentration, R2 = 0.949) (Table 3).
4 Discussion
The average concentration and coefficient of variation can be used to analyze whether the source of HMs is associated with human activities, and high values of both indicate that the source of HMs is closely associated with human activities (Zhou et al., 2020). In our study, the variation coefficient of Cd was large, and the maximum value exceeded the risk screening value of Cd in agricultural soil (0.3 mg/kg; GB 15618–2018), which indicated the existence of local pollution (Table 2).
The rapid increase in plastic fragments in terrestrial and marine environments has attracted increasing attention globally (Li et al., 2021). The abundance of MPs in 30 sweet potato field sites in China has been studied in this study. Compared to previous findings, MP abundance in our study was higher. It may be due to the large amount of plastic waste, plastic film, or plastic fragments that exist in our soil samples (Manta et al., 2002; Hou et al., 2019). In addition, the method of filtration through a 10 μm metal filter to obtain MP samples may also be another important factor contributing to differences in MP abundance (Manta et al., 2002). According to the statistical results from Figure 1, the average abundance of MPs in the eastern region was higher than that of the central region of China. This phenomenon was caused by the high population density and intensive human activities in the eastern region. In addition, population density, industrialization, and urbanization are all factors that affect MP abundance, and the higher the degree of urbanization, the more abundant the production and pollution channels of plastic products and the possibility of higher MP abundance (Shim and Thomposon, 2015; Fuller and Gautam, 2016; Zhou et al., 2019). We also found that the mean MP abundance in soils in middle latitudes was lower than that in soils at low latitudes (Figure 1), which due to the stronger ultraviolet radiation derived higher MPs weathering degree in low latitude areas (Yonkos et al., 2014). In addition, with the increase in agricultural development degree, the total percentage of MPs increased (Figure 3), which may be due to the difference in soil texture and planting age. Because MP abundance in sandy loam soils was significantly higher than in silty loams, facility agricultural soils may receive more MP as planting age increases (Amrutha and Warrier, 2020).
In this study, the size of 97.92% of MPs investigated was smaller than 1,000 μm, and the proportion of MPs whose size was less than 100 μm was the highest, accounting for 42.35% (Figure 2). In a study by Yuan et al. (2021), the number of MPs smaller than 1 mm in the soil near the electronic dismantling area was also close to 90%. Ríos et al. (2020) detected MPs in some waters of northern Europe and analyzed their size. They found that small MPs (100–500 μm) accounted for 48.1%, which may be due to the abundance of sunlight and ultraviolet rays. In addition, film and fragment MPs as dominant shapes in experimental field sites may mainly originate from mulch and covering plastics (Liu et al., 2018; Huang et al., 2020b; Jiang et al., 2020; Yu et al., 2021). However, even though filmy and fragmented MPs also the main shapes in our experiment, the synthetic fibers PA was the main MP pollutant in the sweet potato fields (Figure 3). Jiang et al. (2020) found that PA and PP were two major MP polymers in Wuhan soils, with percentages of 32.5 and 28.8%, respectively. Tan et al. (2019) also found that PA and PP constitute the majority of polymers in soils, while PVC and PET contribute less, which is similar to our results (Figure 3). Irrigation can cause synthetic fibers from sewage and sludge to enter farmland systems and is the primary way synthetic fibers enter the soil (Kor et al., 2020). Therefore, irrigation was supposed to be a major pathway for the PA into the agricultural soils. Based on the results of Table 3, the negative correlation between Cd concentration and MP abundance in soil may be due to the Cd adsorption capacity of MPs, and the adsorption depends upon the aging of MPs, soil pH, and salinity of the media (Yuan et al., 2019). In addition, MPs can reduce soil pH, which may be the main driver of Cd uptake by plants and lead to a Cd reduction in soil (Huang et al., 2023). Based on these results, MPs in soil are likely to be carriers of HM transfer, and the synergistic contamination of MPs and HMs may pose ecological risks and potentially adversely affect soil organisms (Manta et al., 2002).
5 Conclusion
The average MP abundance in 30 sweet potato field sites was 112,400 items/kg. The highest MP abundance was found in Shandong province, followed by Anhui and Henan province. The characteristics of MP distribution are mainly affected by ultraviolet rays and soil texture, and middle latitude areas (30 N - 60 N) (109,280 items/kg) < low latitude areas (0 N - 30 N) (120,995 items/kg); eastern areas (Longitude >115° E) (116,325 items/kg) > central areas (107,922 items/kg). Film and fragment MPs were the two main MP shapes that existed in fields, and the proportion of MPs less than 100 μm was 42.35%, followed by 500–1,000 μm (32.07%), 100–500 μm (23.50%), and > 1,000 μm (2.08%). PA as the main MP pollutant in sweet potato fields was found by a laser infrared imaging system (8,700 LDIR). Cd pollution existed in the sweet potato area, but the overall pollution level was low, and the Cd pollution in the central region was more serious than that in the eastern region of China. In addition, Cd concentration was negatively correlated with MP abundance. In future, although the degree of Cd contamination is low, the presence of MPs may exacerbate the absorption of Cd by sweet potatoes, increasing the risk of the edible safety of sweet potatoes. Therefore, it is necessary to pay attention to the effect and mechanism of MP abundance in soil on the Cd absorption in sweet potatoes.
Data availability statement
The original contributions presented in the study are included in the article/Supplementary material, further inquiries can be directed to the corresponding author.
Author contributions
LS: Data curation, Visualization, Writing – original draft, Writing – review & editing. YX: Data curation, Writing – review & editing. ZC: Data curation, Writing – review & editing. BL: Data curation, Writing – review & editing. YH: Data curation, Writing – review & editing. JL: Data curation, Writing – review & editing. FD: Writing – review & editing. YW: Writing – review & editing. ZS: Writing – review & editing. JY: Conceptualization, Writing – review & editing, Supervision. YC: Data curation, Visualization, Writing – original draft, Writing – review & editing.
Funding
The author(s) declare that financial support was received for the research, authorship, and/or publication of this article. This study is supported by the earmarked fund for CARS-10-Sweetpotato; the Scientific and Technological Innovation Fund of Carbon Emissions Peak and Neutrality of Jiangsu Provincial Department of Science and Technology (BE2022304); the National Key R&D Program of China (2021YFC1809100) and the Science and technology project of Jurong (ZA32216).
Conflict of interest
The authors declare that the research was conducted in the absence of any commercial or financial relationships that could be construed as a potential conflict of interest.
Publisher’s note
All claims expressed in this article are solely those of the authors and do not necessarily represent those of their affiliated organizations, or those of the publisher, the editors and the reviewers. Any product that may be evaluated in this article, or claim that may be made by its manufacturer, is not guaranteed or endorsed by the publisher.
Supplementary material
The Supplementary material for this article can be found online at: https://www.frontiersin.org/articles/10.3389/fsufs.2024.1420628/full#supplementary-material
References
Amrutha, K., and Warrier, A. K. (2020). The first report on the source-to-sink characterization of microplastic pollution from a riverine environment in tropical India. Sci. Total Environ. 739:140377. doi: 10.1016/j.scitotenv.2020.140377
Boots, B., Russell, C. W., and Green, D. S. (2019). Effects of microplastics in soil ecosystems: above and below ground. Environ. Sci. Technol. 53, 11496–11506. doi: 10.1021/acs.est.9b03304
Bovell-Benjamin, A. C. (2007). Sweet potato: a review of its past, present, and future role in human nutrition. Adv. Food Nutr. Res. 52, 1–59. doi: 10.1016/S1043-4526(06)52001-7
Chai, B. W., Wei, Q., She, Y. Z., Lu, G. N., and Yin, H. (2020). Soil microplastic pollution in an e-waste dismantling zone of China. Waste Manag. 118, 291–301. doi: 10.1016/j.wasman.2020.08.048
Chen, Y. L., Sun, K., Han, L. F., and Gao, B. (2022). Separation, identification, and quantification methods in soil microplastics analysis: a review. Acta Pedol. Sin. 59, 364–380. doi: 10.11766/trxb202012070566
Ding, L., Mao, R. F., Ma, S. R., Guo, X. T., and Zhu, L. Y. (2020). High temperature depended on the ageing mechanism of microplastics under different environmental conditions and its effect on the distribution of organic pollutants. Water Res. 174, 115634–115639. doi: 10.1016/j.watres.2020.115634
Duan, L. Y., Zhang, Y., Ren, X. M., Li, Y. Y., Zhang, Y. J., Zhang, H., et al. (2023). Effects of combined pollution of microplastics and cadmium on microbial community structure and function of Pennisetum hydridum rhizosphere soil. Environ. Sci. 44, 527–535. doi: 10.13227/j.hjkx.202212135
Fuller, S., and Gautam, A. (2016). A procedure for measuring microplastics using pressurized fluid extraction. Environ. Sci. Technol. 50, 5774–5780. doi: 10.1021/acs.est.6b00816
Gao, F., Gong, Y. F., and Zhang, P. B. (2000). Production and deployment of virus-free sweetpotato in China. Crop. Production. 19, 105–111. doi: 10.1016/S0261-2194(99)00085-X
Gu, X. Y., Xu, X. Y., Xian, Z. Y., Zhang, Y. S., Wang, C., and Gu, C. (2021). Joint toxicity of aged polyvinyl chloride microplastics and cadmium to the wheat plant. Environ. Chem. 40, 2633–2639. doi: 10.7524/j.issn.0254-6108.2021031505
Hou, F. Y., Dong, S. X., Xie, B. T., Zhang, H. Y., Li, A. X., and Wang, Q. M. (2019). Mulching with plastic film improved the root quality of summer-sown sweet potato (Ipomoea batatas (L). Lam.) in northern China. J. Integ. Agr. 18, 982–991. doi: 10.1016/S2095-3119(18)61994-X
Huang, F. Y., Hu, J. Z., Chen, L., Wang, Z., Sun, S. Y., Zhang, W. M., et al. (2023). Microplastics may increase the environmental risks of cd via promoting cd uptake by plants: a meta-analysis. J. Hazardous. Materials. 448:130887. doi: 10.1016/j.jhazmat.2023.130887
Huang, B., Sun, L. Y., Liu, M. R., Huang, H. Y., He, H., Han, F. X., et al. (2020b). Abundance and distribution characteristics of microplastic in plateau cultivated land of Yunnan Province, China. Environ. Sci. Pollut. Res. 28, 1675–1688. doi: 10.1007/s11356-020-10527-3
Huang, F., Zhou, H., Gu, J., Liu, C., Yang, W., Liao, B., et al. (2020a). Differences in absorption of cadmium and lead among fourteen sweet potato cultivars and health risk assessment. Ecotox. Environ. Safe. 203:111012. doi: 10.1016/j.ecoenv.2020.111012
Jahnke, A., Arp, H. P. H., Escher, B. I., Gewert, B., Gorokhova, E., Kühnel, D., et al. (2017). Reducing uncertainty and confronting ignorance about the possible impacts of weathering plastic in the marine environment. Environ. Sci. Technol. 4, 85–90. doi: 10.1021/acs.estlett.7b00008
Jiang, Y., Yang, F., Zhao, Y. N., and Wang, J. (2020). Greenland Sea gyre increases microplastic pollution in the surface waters of the Nordic seas. Sci. Total Environ. 712:136484. doi: 10.1016/j.scitotenv.2019.136484
Kor, K., Mehdinia, A., and Ershadifar, H. (2020). Neustonic microplastic pollution in the Persian Gulf. Mar. Pollut. Bull. 150:110665. doi: 10.1016/j.marpolbul.2019.110665
Lai, J., Deng, Z., Ji, X., and Luo, X. (2021). Absorption and interaction mechanisms of uranium & cadmium in purple sweet potato (Ipomoea batatas L.). J. Hazard. Mater. 400:123264. doi: 10.1016/j.jhazmat.2020.123264
Li, C., Sun, M. X., Xu, X. B., and Zhang, L. X. (2021). Characteristics and influencing factors of mulch film use for pollution control in China: microcosmic evidence from smallholder farmers. Resour. Conserv. Recy. 164:105222. doi: 10.1016/j.resconrec.2020.105222
Liu, M., Lu, S., Yang, S., Lei, L., Hu, J., Lv, W., et al. (2018). Microplastic and mesoplastic pollution infarmland soils in suburbs of Shanghai, China. Environ. Pollut. 242, 855–862. doi: 10.1016/j.envpol.2018.07.051
Lozano, Y. M., Lehnert, T., Linck, L. T., Lehmann, A., and Rillig, M. C. (2021). Microplastic shape, polymer type, and concentration affect soil properties and plant biomass. Front. Plant Sci. 12:616645. doi: 10.3389/fpls.2021.616645
Ma, D. F., Li, Q., Cao, Q. H., Niu, F. X., Xie, Y. P., Tang, J., et al. (2012). Development and prospect of sweet potato industry and industrial technology in China. Jiangsu J. Agric. Sci. 28, 969–973.
Mainali, D., Kole, M., Ghetler, A., Rault, M., and Moon, C. (2019). Rapid, automated analysis of microplastics using laser direct infrared imaging and spectroscopy. Abstr. Pap. Am. Chem. Soc. 257:135.
Manta, D. S., Angelone, M., Bellanca, A., Neri, R., and Sprovieri, M. (2002). Heavy metals in urban soils: a case study from the city of Palermo (Sicily). Italy. Sci. Total. Environ. 300, 229–243. doi: 10.1016/S0048-9697(02)00273-5
Mbachu, O., Jenkins, G., Kaparaju, P., and Pratt, C. (2021). The rise of artificial soil carbon inputs: reviewing microplastic pollution effects in the soil environment. Sci. Total Environ. 780:146569. doi: 10.1016/j.scitotenv.2021.146569
Palansooriya, K. N., Sang, M. K., EI-Naggar, A., Shi, L., Chang, S. X., Sung, J., et al. (2023). Low-density polyethylene microplastics alter chemical properties and microbial communities in agricultural soil. Sci. Rep. 13:16276. doi: 10.1038/s41598-023-42285-w
Pflugmacher, S., Sulek, A., Mader, H., Heo, J., Noh, J. H., Penttinen, O. P., et al. (2020). The influence of new and artificial aged microplastic and lleachates on the germination of Lepidium sativum L. Plan. Theory 9:339. doi: 10.3390/plants9030339
Rillig, M. C., Lehmann, A., de Souza Machado, A. A., and Yang, G. (2019). Microplastic effects on plants. New Phytol. 223, 1066–1070. doi: 10.1111/nph.15794
Ríos, M. F., Hernández-Moresino, R. D., and Galván, D. E. (2020). Assessing urban microplastic pollution in a benthic habitat of Patagonia Argentina. Mar. Pollut. Bull. 159:111491. doi: 10.1016/j.marpolbul.2020.111491
Shi, L., Chen, Z. M., Hou, Y. N., Li, J. M., Shen, Z. G., and Chen, Y. H. (2023). The original polyethylene microplastics inhibit the growth of sweet potatoes and increase the safety risk of cadmium. Front. Plant Sci. 14:1138281. doi: 10.3389/fpls.2023.1138281
Shi, L., Hou, Y. N., Chen, Z. M., Bu, Y., Zhang, X. Y., Shen, Z. G., et al. (2022). Impact of polyethylene on soil physicochemical properties and characteristics of sweet potato growth and polyethylene absorption. Chemosphere 302:134734. doi: 10.1016/j.chemosphere.2022.134734
Shim, W. J., and Thomposon, R. C. (2015). Microplastics in the ocean. Arch. Environ. Contam. Toxicol. 69, 265–268. doi: 10.1007/s00244-015-0216-x
Tan, X., Yu, X., Cai, L., Wang, J., and Peng, J. (2019). Microplastics and associated PAHs in surface water from the Feilaixia reservoir in the Beijiang River, China. Chemosphere 221, 834–840. doi: 10.1016/j.chemosphere.2019.01.022
Wang, Y. J., Qin, Y. H., Wang, S. A., Zhang, D. S., Tian, Y. T., Zhao, F. M., et al. (2021a). Species and genetic variability of sweet potato viruses in China. Phytopathology. Res. 3:20. doi: 10.1186/s42483-021-00097-8
Wang, F. L., Wang, X. X., and Song, N. N. (2021b). Polyethylene microplastics increase cadmium uptake in lettuce (Lactuca sativa L.) by altering the soil microenvironment. Sci. Total Environ. 147133:784. doi: 10.1016/j.scitotenv.2021.147133
Xie, Y. Z., Guo, X. D., Jia, Z. D., Ma, P. Y., Bian, X. F., Yu, Y., et al. (2018). Current situation and prospect of Chinese edible sweet potato breeding. Jiangsu J. Agric. Sci. 34, 1419–1424. doi: 10.3969/j.issn.1000-4440.2018.06.030
Yonkos, L. T., Friedel, E. A., Perez-Reyes, A. C., and Ghosal, V. (2014). Microplastics in four estuarine rivers in the Chesapeake Bay, USA. Environ. Sci. Technol. 2014, 14195–14202. doi: 10.1021/es5036317
Yu, L., Zhang, J. D., Liu, Y., Chen, L. Y., Tao, S., and Liu, W. X. (2021). Distribution characteristics of microplastics in agricultural soils from the largest vegetable production base in China. Sci. Total Environ. 756:143860. doi: 10.1016/j.scitotenv.2020.143860
Yuan, W. K., Liu, X. N., Wang, W. F., Di, M. X., and Wang, J. (2019). Microplastic abundance, distribution and composition in water, sediments, and wild fish from Poyang Lake, China. Ecotox. Environ. Safe. 170, 180–187. doi: 10.1016/j.ecoenv.2018.11.126
Yuan, X. H., Xue, N. D., and Han, Z. G. (2021). A meta-analysis of heavy metals pollution in farmland and urban soils in China over the past 20 years. J. Environ. Sci. 101, 217–226. doi: 10.1016/j.jes.2020.08.013
Zeb, A. R., Liu, W. T., Meng, L. Z., Lian, J. P., Wang, Q., Lian, Y. H., et al. (2021). Effects of polyester microfibers (PMFs) and cadmium on lettuce (Lactuca sativa) and the rhizospheric microbial communities: a study involving physio-biochemical properties and metabolomic profiles. J. Hazard. Mater. 424:127405. doi: 10.1016/j.jhazmat.2021.127405
Zhou, Y. F., Liu, X. N., and Wang, J. (2019). Characterization of microplastics and the association of heavy metals with microplastics in suburban soil of Central China. Sci. Total Environ. 694:133798. doi: 10.1016/j.scitotenv.2019.133798
Keywords: cadmium, distribution, field survey, laser infrared imaging system, polyamide
Citation: Shi L, Xu Y, Chen Z, Liu B, Hou Y, Li J, Dang F, Wang Y, Shen Z, Yang J and Chen Y (2024) Microplastics and cadmium pollution in Chinese sweet potato fields. Front. Sustain. Food Syst. 8:1420628. doi: 10.3389/fsufs.2024.1420628
Edited by:
Ali Riza Kosker, Çukurova University, TürkiyeCopyright © 2024 Shi, Xu, Chen, Liu, Hou, Li, Dang, Wang, Shen, Yang and Chen. This is an open-access article distributed under the terms of the Creative Commons Attribution License (CC BY). The use, distribution or reproduction in other forums is permitted, provided the original author(s) and the copyright owner(s) are credited and that the original publication in this journal is cited, in accordance with accepted academic practice. No use, distribution or reproduction is permitted which does not comply with these terms.
*Correspondence: Yahua Chen, eWFodWFjaGVuQG5qYXUuZWR1LmNu; Jinghui Yang, eWpobm4zMkAxMjYuY29t
†These authors have contributed equally to this work