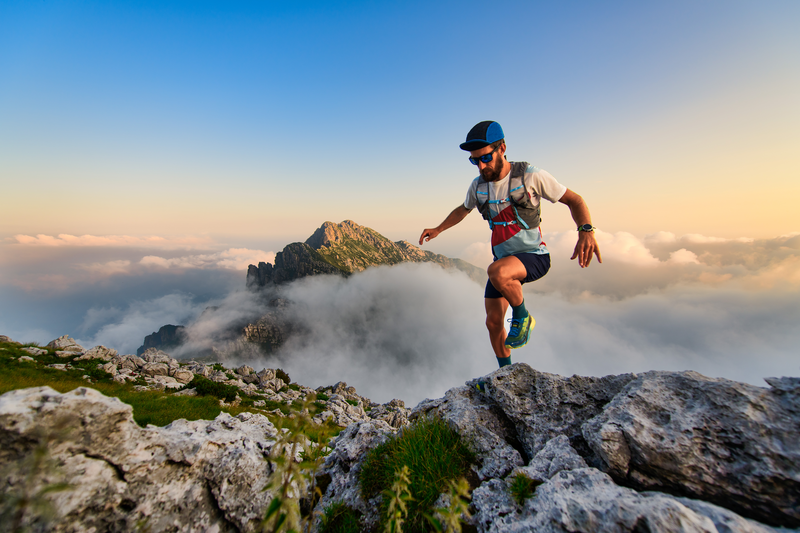
94% of researchers rate our articles as excellent or good
Learn more about the work of our research integrity team to safeguard the quality of each article we publish.
Find out more
ORIGINAL RESEARCH article
Front. Sustain. Food Syst. , 17 September 2024
Sec. Aquatic Foods
Volume 8 - 2024 | https://doi.org/10.3389/fsufs.2024.1419143
This article is part of the Research Topic Detection, Risk Analysis and Monitoring of Chemical Contaminants from Agro-Aqua Food Production and Processing: Implications on the One Health Triad View all 9 articles
Introduction: Human activities have inadvertently led to the release of harmful substances, including trace metals, into aquatic environments, with consequential impacts on aquatic organisms and potential health risks for consumers. This research assessed the presence, origins, and health implications of trace elements within the muscles of Clarias batrachus and Chrysichthys nigrodigitatus from the Bomadi and Gbotebo rivers and their surroundings in Bayelsa State, Nigeria.
Methods: Thirty samples from each fish species were collected and analyzed to conduct the study. Through the use of an atomic absorption spectrophotometer, the researchers determined the concentrations of trace metals, including iron, copper, zinc, lead, nickel, cadmium, and cobalt, in the fish tissues.
Results and discussion: The concentrations of the metals within the two fish species varied, with notable differences in zinc and cadmium levels. Utilizing statistical analyses like Pearson correlation, principal component analysis (PCA), and hierarchical cluster analysis (CA), it was established that the trace metals originated from diverse sources. The study then evaluated health risks associated with these trace elements, considering both non-carcinogenic and carcinogenic hazards for different age groups. The results indicated that children’s total target hazard quotient fell below 1 for both fish species, implying a limited tendency toward non-carcinogenic risks through lifelong fish consumption. However, in some adult fish samples, the quotient exceeded 1, indicating a higher potential for non-carcinogenic risks. Regarding carcinogenic hazards, the mean risks were generally lower than the accepted threshold, except for lead in both fish species among adults and children. Stricter values highlighted that only lead concentrations in fish from both categories were deemed acceptable. Consequently, this study highlights the importance of raising awareness among consumers who buy fish from the studied region. Moreover, consistent monitoring of potentially harmful trace elements in water, sediments, and fish is recommended to safeguard consumer health and well-being.
Fish consumption has surged significantly in recent decades due to growing awareness of its health and nutritional benefits, including its rich content of protein, essential fats, vitamins, and minerals. With the expansion of aquaculture to address declining wild fish stocks, fish has become a vital dietary staple for 3.3 billion people globally, providing over 20% of their average per capita animal protein intake [Food and Agricultural Organization of the United Nations (FAO/UN), 2020]. However, this rising demand has brought to light a critical issue: the contamination of aquatic ecosystems. As fish consumption increases, so does the potential exposure to harmful contaminants present in water bodies, raising concerns about the safety of this otherwise nutritious food source.
The pollution of aquatic environments has become a significant environmental and public health challenge, particularly as fish are prone to accumulating toxic substances such as trace metals and other pollutants (Izah et al., 2023). These contaminants can bioaccumulate and biomagnify in the food chain, with fish often serving as carriers of these hazardous substances. Consequently, the consumption of trace metals contaminated fish can lead to serious health issues, including immunotoxicity, reproductive problems, and even cancer (Tchounwou et al., 2012; Izah et al., 2016). The growing reliance on fish as a primary protein source amplifies the urgency of addressing aquatic contamination to ensure that the health benefits of fish consumption are not overshadowed by the risks associated with these environmental pollutants.
Despite this, rising river pollution from wastewater discharges, household and agricultural wastes trace metals, and unintentional chemical spills, among others, suggest that fish could be a significant source of human exposure to toxic and harmful compounds like trace metals. Trace metals in fish can transfer to humans, posing a severe health hazard. For example, cadmium has been related to heart, neurological, and reproductive issues. Exposure to a high dose of cadmium exposure might cause nausea, vomiting, stomach pain, and tiredness over a short period (Izah et al., 2016). Furthermore, poisoning associated with lead has been linked to kidney, muscular, and cardiovascular problems (Izah et al., 2016). Copper is a required component for various physiological functions in the human body, although when the concentration is beyond the permissible range, it can harm the brain, liver, and kidneys.
Despite the toxicity of these metals, they are classified as essential elements, including iron, copper, chromium, manganese, cobalt, and zinc, which implies that they are required in biological systems (Signes-Pastor et al., 2022; Sobolev et al., 2019; Izah et al., 2016). As a result of the continuous deposition of discharges from anthropogenic activities, higher concentrations of these essential elements within the systems increase to hazardous levels for the biota and ecosystems. Non-essential elements such as cadmium, lead, mercury, and arsenic are becoming a global concern due to their toxicity (Signes-Pastor et al., 2022; Sobolev et al., 2019; Bassey and Chukwu, 2019).
In the study of trace metals in environmental studies, correlational and multivariate analyses have been widely applied to determine the source of elements (Qing et al., 2015). Principal component analysis (PCA) and cluster analysis (CA) are the most commonly used multivariate analyses in environmental studies (Chandrasekaran et al., 2015). The PCA reduces data sets and extracts smaller numbers to show the variables’ association (Chandrasekaran et al., 2015). On the other hand, CA is used to group observations into two or more mutually exclusive groups based on the combination of the internal variables (Chandrasekaran et al., 2015).
To identify the human health implications of TE-contaminated fish resources, a risk evaluation (a tool for assessing, detecting, and measuring hazards, determining possible exposure pathways, and estimating the potential threat of toxins to humans) technique is often used to assess the connected hazard as food safety measures. The human health hazard evaluation of trace metals in fisheries resources is used to determine the potential adverse health impacts of human exposure to environmental hazards. Hazard identification, dose–response assessment, exposure assessment, and risk characterization are the four actions involved in human health risk assessment (Bassey and Chukwu, 2019). During the health hazard evaluation of trace metals, they are classified according to their non-carcinogenic and carcinogenic tendencies. Authors have studied the health risks associated with trace metals in various common fish samples from different locations. For example, studies have focused on Clarias batrachus, Clarias gariepinus, Hemichromis fasciatus, Chrysichthys nigrodigitatus, and Macrobrachium rosenbergii from the Densu River in Ghana (Amankwaa et al., 2021), Oreochromis niloticus, Clarias batrachus, and Chrysichthys nigrodigitatus from Lagos Lagoon (Oladapo and Taiwo, 2024), and Oreochromis niloticus, Tilapia zillii, Malapterurus electricus, Parachanna obscura, Chrysichthys nigrodigitatus, Scomber scombrus, and Trachurus trachurus from the Aba River (Ogoko et al., 2022). Additionally, research has included Chrysichthys nigrodigitatus from a floodplain river in Cross River State (Ada et al., 2012), River Oli in Kainji Lake National Park (Adelakun and Kehinde, 2019), and the Okulu River in Rivers State (Aigberua et al., 2021), Tympanotonus fuscatus from coastal region of Bayelsa state (Ogamba et al., 2016a), Clarias garepinus from Bayelsa State (Ogamba et al., 2016b), and Oreochromis niloticus and Clarias camerunensis from Ikoli creek, Bayelsa State (Ogamba et al., 2016c). In the River Nun around the Tombia community in Bayelsa State, Nigeria, Clarias batrachus and Chrysichthys nigrodigitatus have been reported to be less abundant (Gijo and Alagoa, 2022). Despite these studies, there is limited literature on the levels of trace metals and the associated health risks in these species. Furthermore, in the Bomadi River, a major tributary of the Forcados River in the Niger Delta (Izah et al., 2024), and its surrounding areas in the Ekeremor Local Government Area of Bayelsa State, a wide range of human activities take place. These include boat transportation, which serves as a primary means of travel and goods transport for local communities. Additionally, the region is characterized by significant oil and gas operations, which include both legitimate and artisanal activities. This activity, along with the presence of houseboats along the riverbanks, contributes to the complex socio-economic and environmental landscape of the area. These diverse activities can have substantial impacts on the river’s ecosystem, including potential pollution and habitat disruption.
Therefore, the aim of this study is to: (1) determine and compare the concentrations of trace metals in both fish species, (2) determine the sources of the trace metals, and (3) assess the health risks associated with them in Clarias batrachus and Chrysichthys nigrodigitatus in Bomadi and Gbotebo rivers and their environs in Bayelsa State, Nigeria.
The Bomadi and Gbotebo rivers and their environs in the Ekeremor Local Government Area of Bayelsa State are vital rivers for the inhabitants. The Forcados River receives water from all the rivers. The locals are greatly dependent on the rivers for their livelihood. This is because residents depend on fishing for a living. The river also facilitates the transportation of commodities. In addition, the rivers are used to transport wood logs to Nigeria’s western states. The neighboring oil and gas operations can be reached via the rivers. The most common plant species include elephant grass, plantains, oil palms, and Raphia palms. The ridges of the river are also frequently referenced as farmland. The region’s climate is characterized by average annual atmospheric temperatures of 28–29°C and relative humidity ranging from 50 to 95%. The wet season (7 months from April to October) and the dry season (November to March of the preceding year) is the primary, separate seasons (Agedah et al., 2015). Flooding does occur in the region, and it tends to wash leftover soil into the water bodies during the rainy season—particularly in September and October.
Thirty samples of each fish species (Chrysichthys nigrodigitatus and Clarias batrachus) from Bomadi and Gbotebo rivers and their environs (latitude 40 58′ 29″ N to 50 3′ 25″ N and longitude 50 39′ 50″ E and 50 41′ 25″ E) were purchased from local fishermen in the study area. Hence, a total of 60 fish samples (Chrysichthys nigrodigitatus denoted as BF 1–30) and (Clarias batrachus denoted as SF 1–30) were used for the study. The fish were preserved in a cooler containing ice.
The fish samples were dissected, and the muscle was obtained. The muscles were oven-dried at 60°C until the samples were completely dried.
The trace metals analysis of the fish samples was carried out following the method previously described by Aigberua et al. (2018, 2021). The fish was ground into powder. Then, it was sieved with a 2.00-mm mesh. The sieved samples were digested with a mixture of 69% NO3 Analar (BDH, Poole, United Kingdom) and 37% HCl (Sigma-Aldrich, Steinheim, Germany) in a ratio of 1:10 v/v. The mixture was heated to near dryness and allowed to cool. This was done in a fume cupboard. The resultant digest was filtered into a volumetric flask using a 110-mm-diameter filter. The filtrate was made up to the mark (20 mL) with distilled water. The filtrate was aspirated into a calibrated flame atomic absorption spectrophotometer (GBC 908 PBMT model, GBC Scientific-Australia). The level of the test metals was extrapolated from the absorbance-concentration plot. The concentration for each metal was recorded in mg/kg.
The operational conditions (Supplementary material 1, 2) and quality control methods were observed during the analysis. Quality control procedures included reagent blank aspiration, repeating the sample and analysis, and spike recovery. The recovery percentage was calculated using Equation 1. Meanwhile, spike recovery was carried out using a combination of certified reference standards and test samples. The concentration of trace metals was then calculated to determine the amount of analyte that could be recovered. The rates of recovery ranged from 92.0 to 98.3%. A quality standard check was performed after each analysis of 10 samples. The limits of detection (LOD) and limits of quantitation (LOQ) were determined using the standard deviation of the absorbance response and the slope value of the calibration curve (LOQ). The LOD, LOQ, and percentage spike recoveries of test metals are also included in Tables 1, 2. The recovery rate, LOD, and LOQ were calculated using Equations 1–3. This was done according to the methods described by Aigberua et al. (2021).
Table 1. Summary of abbreviation, units, and values used for the estimation of health risk assessment due to the consumption of muscles of the fish species.
Where MLSS is the element concentration in the spiked sample, MLU.SS is the element concentration in the unspiked sample, Std dev is the standard deviation, SCC is the slope calibration curve, 3.3 and 10 are constant factors.
When the concentration of the metals in the sample is below the equipment detection level, it is denoted as BEDL.
A research-based study on trace metal risk assessment addresses the distinctive qualities and behaviors of elements to consider when assessing human health risks. The human health risk is based on non-carcinogenic hazards (using estimated daily intake, EDIf, target hazard quotient, THQf, and total target hazard quotient, TTHQf) and carcinogenic hazards. These indices have been widely used to study the health risks of different environmental samples and foods, including fish.
The estimated daily intake of the fish (EDIf) was calculated following the method described by Arisekar et al. (2020) (Equation 4). The description of the abbreviations and corresponding figures used to assess the EDIf is shown in Table 1.
The Target Hazard Quotient of the fish (THQf) was computed using the method earlier described by Arisekar et al. (2020) and Matouke and Abdullahi (2020) (Equation 5).
Target hazard quotient is widely used to show the non-carcinogenic health effects of trace metals in ingestible items like fish tissues. When the THQf value is <1, it suggests that the possibilities of developing unfavorable health effects as a result of exposure are extremely unlikely (Arisekar et al., 2020). As a result, daily metal intake from food-fish is doubtful to aggravate adverse health effects over a person’s lifetime (Aigberua et al., 2021). When THQf is >1, it implies that adverse health effects are possible (Arisekar et al., 2020).
The sum of the individual THQf is the total target hazard quotient (TTHQf). The TTHQf values were determined using the approach outlined by Arisekar et al. (2020) and Matouke and Abdullahi (2020) (Equation 6).
Where THQf is the target hazard quotient of trace metals in the fish.
The carcinogenic risk is frequently used to describe the possibility of an individual developing cancer due to lifetime exposure to probable carcinogens from consuming food such as fish. Cadmium and lead carcinogenic hazards were determined using the approach previously described by Arisekar et al. (2020) (Equation 7).
The indices for classifying CHf are shown in Table 2.
When the element concentration in the sample is below the equipment detection level, the health risk cannot be calculated; hence, it is denoted as “Not calculated because concentration was not detected = NCCD.”
The data analysis was carried out using Minitab 17 and SPSS version 20. Data were presented as mean ± standard deviation. Student t-test was used to compare the level of trace metals in the concentration of both fish species. Multivariate analysis (principal component analysis, cluster analysis using Euclidean Distance and Ward Linkage) and Pearson correlation were used to show the connections between the measurement variables for each sample type.
Table 3 shows the relative levels of trace metals in Clarias batrachus and Chrysichthys nigrodigitatus from the Bomadi and Gbotebo rivers and their environs in Bayelsa State, Nigeria. The cadmium concentrations in Clarias batrachus and Chrysichthys nigrodigitatus ranged from 0.00–1.02 (0.10 ± 0.22) mg/kg to 0.00–1.53 (0.29 ± 0.41) mg/kg, respectively. The cadmium concentration was statistically higher in Chrysichthys nigrodigitatus than in Clarias batrachus. The overall mean concentrations of cadmium observed in both fish species were higher than the maximum permissible limit of 0.05 mg/kg by European Union (2002) (Senarathne and Pathiratne, 2007; Senarathne et al., 2006), lower than the maximum limit of 0.50 mg/kg by Food and Agricultural Organization/World Health Organization (FAO/WHO) (1989) (Elnabris et al., 2013); and at a tolerable level of 0.30 mg/kg as recommended by the median international standard (Philips, 1993; Senarathne and Pathiratne, 2007; Senarathne et al., 2006).
Table 3. Comparative levels of trace metals in Clarias batrachus and Chrysichthys nigrodigitatus from Bomadi and Gbotebo rivers and their Environs in Bayelsa State, Nigeria.
The lead mean concentration was observed in Chrysichthys nigrodigitatus [0.00–9.65 (2.06 ± 3.03) mg/kg) and Clarias batrachus (0.00–6.64 (2.06 ± 2.52) mg/kg], respectively. However, there was no statistical dissimilarity (p > 0.05, based on t-test) in the lead concentration in the two fish species. The overall mean concentrations of lead observed in both fish species were higher than the maximum permissible limit of 0.2 mg/kg by European Union (2002) (Senarathne and Pathiratne, 2007; Senarathne et al., 2006), 0.5 mg/kg by Food and Agricultural Organization/World Health Organization (FAO/WHO) (1989) (Elnabris et al., 2013) and slightly higher than the tolerable level of 2.00 mg/kg as recommended by Median International Standard (Philips, 1993; Senarathne and Pathiratne, 2007; Senarathne et al., 2006).
The mean concentrations of cobalt observed in Chrysichthys nigrodigitatus were 0.00–1.12 (0.12 ± 0.23) mg/kg, and in Clarias batrachus were 0.00–0.34 (0.06 ± 0.11) mg/kg, respectively. However, there was no significant dissimilarity (p > 0.05, based on t-test) in the cobalt concentration in both fish species. The overall mean level of cobalt showed that both fish species were lower than the WHO permissible limit of 5.00 mg/kg, as reported by Acharya et al. (2023).
Iron concentrations in Clarias batrachus and Chrysichthys nigrodigitatus ranged from 12.46–33.50 (22.60 ± 5.50) mg/kg to 12.76–37.84 (20.30 ± 6.39) mg/kg, respectively. Statistically, there was no differences (p > 0.05, based on t-test) in the mean values of both fish species.
The concentrations of copper in Clarias batrachus and Chrysichthys nigrodigitatus ranged from 0.34–2.57 (1.58 ± 0.70) mg/kg to 0.29–9.59 (1.96 ± 1.63) mg/kg, respectively. Statistically, there was no difference (p > 0.05, based on t-test) in the mean values of both fish species. The overall mean concentrations of copper observed in both fish species were slightly higher than the maximum permissible limit of 20 mg/kg recommended by the Median International Standard (Philips, 1993; Senarathne and Pathiratne, 2007; Senarathne et al., 2006) and lower than the maximum limit of 30 mg/kg recommended by Food and Agricultural Organization/World Health Organization (FAO/WHO) (1989) (Elnabris et al., 2013).
The zinc concentrations in Clarias batrachus and Chrysichthys nigrodigitatus ranged from 5.56–12.83 (8.86 ± 1.76) mg/kg to 3.37–33.22 (13.70 ± 6.59) mg/kg, respectively. Statistically, there were differences (p < 0.05, based on t-test) in the mean values of the two fish species. The overall mean concentrations of copper observed in both fish species were lower than the maximum permissible limit of 45 mg/kg recommended by the Median International Standard (Philips, 1993; Senarathne and Pathiratne, 2007; Senarathne et al., 2006) and 40 mg/kg recommended by Food and Agricultural Organization/World Health Organization (FAO/WHO) (1989) (Elnabris et al., 2013).
The concentrations of nickel in Clarias batrachus and Chrysichthys nigrodigitatus ranged from 0.00–1.67 (0.49 ± 0.51) mg/kg to 0.00–9.46 (1.23 ± 1.94) mg/kg, respectively. Statistically, there was variance (p < 0.05, based on t-test) in the mean values of the two fish species. The overall mean concentrations of nickel observed in both fish species were lower than the WHO limit of 25 mg/kg, as reported by Acharya et al. (2023).
The findings of this study have some similarity with previous studies on the concentrations of trace metals in the same fish from some Nigerian and Ghanaian rivers. For instance, Amankwaa et al. (2021) analyzed trace metals in the tissues of Clarias batrachus and Chrysichthys nigrodigitatus from the Densu River, Ghana, reporting concentrations of 1.5 mg/kg and 1.1 mg/kg for lead, 0.48 mg/kg and 0.28 mg/kg for cadmium, and 1.22 mg/kg and 1.31 mg/kg for copper, respectively. Ogoko et al. (2022) reported maximum concentrations of 0.63 mg/kg for lead, 0.81 mg/kg for chromium, and 16.91 mg/kg for iron in Chrysichthys nigrodigitatus from the Aba River. Adelakun and Kehinde (2019) studied Trace metals in the muscles of Chrysichthys nigrodigitatus from River Oli in Kainji Lake National Park, finding concentrations of 6.55 mg/kg for lead, 3.65 mg/kg for chromium, 14.56 mg/kg for zinc, 28.20 mg/kg for copper, 218.33 mg/kg for iron, and 0.001 mg/kg for cadmium. Ayotunde et al. (2012) studied trace metals in Chrysichthys nigrodigitatus and reported a trend in concentration with the order Cu > Fe > Zn > Pb > Cd, with the highest mean concentrations being copper (0.297 ± 0.022 μg/g), cadmium (0.011 μg/g), iron (0.371 μg/g), and lead (0.008 μg/g). Oladapo and Taiwo (2024) examined trace metals in fish species from Lagos Lagoon and reported mean concentrations (in mg/kg) in various organs of Chrysichthys nigrodigitatus (lead: 12.30–14.85, Copper: 9.15–11.25, zinc: 4.90–6.10, nickel: 3.90–4.73, cadmium: 2.18–3.33) and Clarias batrachus (lead: 10.40–11.25, copper: 8.75–11.45, zinc: 8.35–8.80, nickel: 1.72–1.92, cadmium: 1.30–1.45). The authors further reported pollution index for the trace metals was reported to be higher in Chrysichthys nigrodigitatus (5.27) compared to Clarias batrachus (3.26). Aigberua et al. (2021) reported cadmium, lead, iron, copper, and zinc in the range of 0.00–1.30 mg/kg, 0.00–54.70 mg/kg, 4.00–197.30 mg/kg, 0.00–73.10 mg/kg, and 9.20–35.30 mg/kg in Sarotherodon melanotheron and Chrysichthys nigrodigitatus from Okulu River in Nigeria.
The study showed no significant differences in the concentration of iron, copper, lead, and cobalt, which suggests that the sources of the metals are affecting the fish species evenly. However, apparent differences exist. Also, there was a statistical differences in the zinc, cadmium, and nickel concentrations, suggesting that they are from diverse sources. Previous studies have indicated that variations in the concentration of trace metals in fish are cause by several factors (Izah and Angaye, 2016). Olgunoğlu et al. (2015) and Izah and Angaye (2016) reported that variations in the concentration of metals in fish are affected by both external characteristics (such as level of dissolved metals, physicochemistry, dissolved oxygen, interactions between metals, seasonal and geographical differences) and internal factors (including species variability about body size, sex, development stage, breeding condition). Some other factors that affect the level of elements in fish include the mode of feeding, exposure duration, and physicochemical position of the toxicants (Aghoghovwia et al., 2016). Again, different studies have shown that organs/tissues have varying effects on the bioaccumulation of trace metals.
Depending on the locale, different fish respond differently to identical environmental conditions regarding bioaccumulation (Izah and Angaye, 2016). This may be due to the trace metal and macronutrient contamination level in the studied water body. In general, there were anomalies (although not significant for some of the trace metals) in the trace metal’s bioaccumulation and bioavailability patterns in the muscles of both fish. Eneji et al. (2011) attributed this heterogeneity to bioavailability, inherent fish processes, ecosystem trophic structure, and threshold variation.
Tables 4, 5 show the Pearson correlation of trace metals in Clarias batrachus and Chrysichthys nigrodigitatus, respectively, in Bomadi and Gbotebo rivers and environs in Bayelsa State, Nigeria. In Clarias batrachus, iron showed a negative correlation with copper (r = 0.459).Zinc negatively correlated with Cadmium (r = 0.456) at p = 0.05. Lead positively correlated with nickel (r = 0.434) at p = 0.05 (Table 4). In Chrysichthys nigrodigitatus, iron positively correlated with zinc (r = 0.398) at p = 0.05 and cobalt (r = 0.516) at p = 0.01. Copper positively correlated with nickel (r = 0.614) at p = 0.01 and cobalt (r = 0.385) at p = 0.05. The correlational analysis revealed the degree of positive and negative association between the metals. This shows the interdependence of ions (elements) in the fish. Many parameters did not show statistical association in both fish species. This could be due to the influence of chemical, physical, and biological activities within the aquatic ecosystem (Ehiemere et al., 2022). Ehiemere et al. (2022) reported that human activities influence element behavior in aquatic ecosystems. The positive correlation between elements denotes the similarity in origin (Talukder et al., 2022) or co-contamination (Chandrasekaran et al., 2015), which human activities could have influenced. Furthermore, the negative statistical correlation between the elements is an indication of lower uptake of one metal in the presence of another and vice versa (Ehiemere et al., 2022), and the different origins do not associate with the dynamics (Chandrasekaran et al., 2015) of the metals in the fish. Talukder et al. (2022) reported that the weak association between trace metals indicates that a single factor does not influence them; thus, several human and natural activities control the distribution of the elements.
Table 4. Pearson correlation (r) of trace metals in Clarias batrachus from Bomadi and Gbotebo rivers and their environs in Bayelsa State, Nigeria.
Table 5. Pearson correlation (r) of trace metals in Chrysichthys nigrodigitatus from Bomadi and Gbotebo rivers and their environs in Bayelsa State, Nigeria.
Furthermore, a weaker positive or negative correlation was observed between the trace metals in Tables 4, 5. This suggests that the trace metals may have originated from both anthropogenic and natural, leading to varied distribution patterns. Additionally, environmental factors such as pH, temperature, and redox conditions may have differentially affected the mobility and bioavailability of the metals. Moreover, biological uptake and accumulation processes can vary significantly among metals; for instance, some metals may be bioaccumulated by organisms, while others may not.
Table 6 shows the principal component analysis of trace metals in Clarias batrachus and Chrysichthys nigrodigitatus from Bomadi and Gbotebo rivers and their environs in Bayelsa State, Nigeria. The total variance explained by the Principal Component Analysis (PCA) for Clarias batrachus is 72.47%, distributed across three principal components (PCs). This indicates that the selected components effectively capture a substantial portion of the data’s variability, highlighting key patterns in the relationship between the heavy metals analyzed. For PC-1 (29.602% Variance), the first principal component, explaining 29.602% of the total variance, has strong positive statistical loadings with Iron (r = 0.799) and Zinc (r = 0.660). These elements may share common sources or exhibit similar behavior in bioaccumulation within Clarias batrachus. Conversely, PC-1 shows negative loadings with Copper (r = −0.738) and Cadmium (r = −0.568), indicating that these metals vary inversely with Iron and Zinc in this fish species. The opposing loadings suggest that the environmental factors influencing Copper and Cadmium differ from those affecting Iron and Zinc. In PC-2 (24.497% Variance), the second principal component explains 24.497% of the variance, with positive loadings on Lead (r = 0.843) and Nickel (r = 0.634). These metals are typically associated with industrial pollutants, suggesting a common source or pathway of accumulation in Clarias batrachus. PC-2 also shows a negative loading with Cobalt (r = −0.702), indicating that as Lead and Nickel levels increase, Cobalt levels tend to decrease, possibly reflecting different contamination patterns or biological interactions. In PC-3 (18.371% Variance): the third principal component accounts for 18.371% of the variance, with positive loadings on Cadmium (r = 0.562) and Nickel (r = 0.660). This component highlights the joint variation of these two metals, which may be linked to specific contamination events or sources. Additionally, there is a negative loading with Copper (r = −0.503), suggesting an inverse relationship between Copper and the other metals in this component, further emphasizing the complex interactions between these contaminants in Clarias batrachus.
Table 6. Principal component analysis of trace metals in Clarias batrachus and Chrysichthys nigrodigitatus from Bomadi and Gbotebo rivers and their Environs in Bayelsa State, Nigeria.
Similarly, for Chrysichthys nigrodigitatus, the total variance explained by PCA is 71.271%, distributed across three principal components. This analysis reveals distinct patterns in trace metal accumulation, with different metals contributing to each principal component. For PC-1 (33.246% Variance), the first principal component explains a substantial 33.246% of the variance and has positive statistical loadings with Zinc (r = 0.571), Iron (r = 0.697), Copper (r = 0.562), and Cobalt (r = 0.845). These metals appear to co-occur in Chrysichthys nigrodigitatus, possibly due to shared contamination sources or similar bioaccumulation pathways. The strong loading of Cobalt suggests its significant role in this component, highlighting the potential environmental impact on this species. In PC-2 (23.248% Variance), the second principal component accounts for 23.248% of the variance, with positive loadings on Copper (r = 0.640) and Nickel (r = 0.739). These metals might be associated with industrial or anthropogenic activities, contributing to their co-variation in this species. PC-2 also displays a negative loading with Cadmium (r = −0.526), indicating an inverse relationship between Cadmium and the other metals, which may reflect different contamination patterns or biological uptake mechanisms. In PC-3 (14.776% Variance), the third principal component explains 14.776% of the variance and has positive loadings on Cadmium (r = 0.622) and Lead (r = 0.605). This component suggests that these two metals, often linked to pollution, co-occur in Chrysichthys nigrodigitatus. Their presence in the same component underscores the potential health risks associated with the consumption of this fish species, as both metals are known for their toxicity and adverse health effects.
The PCA results for both Clarias batrachus and Chrysichthys nigrodigitatus reveal significant insights into the distribution and relationships of trace metals within these species. The variance explained by the components highlights the complexity of metal contamination and the potential for bioaccumulation in these aquatic species.
Table 7 shows the principal component (varimax with Kaiser normalization) asodiumlysis of macronutrients and trace metals in Clarias batrachus and Chrysichthys nigrodigitatus from the Bomadi and Gbotebo rivers and their environs Bayelsa State, Nigeria. The analysis of the Clarias batrachus indicates that the three principal components (F-1, F-2, F-3) account for 72.47% of the total variance in the data. This implies that these components capture a substantial portion of the variability in the concentrations of trace metals in Clarias batrachus. F-1 explained 24.653% of the Variance and has positive statistical loadings with Lead (r = 0.854), Nickel (r = 0.607), and negative Loadings with Cobalt (r = −0.722). The first factor suggests that lead and nickel concentrations are strongly correlated with each other, while cobalt shows an inverse relationship. This pattern could indicate a specific pollution source or environmental factor influencing the accumulation of these metals differently. Lead and nickel could be co-occurring due to industrial activities or runoff from contaminated sites, whereas cobalt might be associated with a different source or environmental condition. F-2 explained 23.979% of the Variance and has positive statistical loadings with Zinc (r = 0.824), and negative Loadings with Cadmium (r = −0.829). The second factor highlights a strong positive correlation between zinc and a negative relationship with cadmium. This could suggest that areas with high zinc concentrations have lower cadmium levels, possibly due to different sources or bioavailability of these metals in the aquatic environment. F-3 explained 23.839% of the Variance and has positive statistical loadings with Iron (r = 0.606), Nickel (r = 0.708), and negative Loadings with Copper (r = −0.857). The third factor shows that iron and nickel are positively correlated, while copper exhibits a strong negative loading. This relationship could reflect differing geochemical or anthropogenic processes influencing these metals’ distribution in the aquatic ecosystem.
Table 7. Principal component (Varimax with Kaiser Normalization) analysis of macronutrients and trace metals in Clarias batrachus and Chrysichthys nigrodigitatus from Bomadi and Gbotebo rivers and their environs in Bayelsa State, Nigeria.
The Chrysichthys nigrodigitatus explains 71.271% of the total variance through three factors, indicating that these factors significantly influence trace metal concentrations in this species. F-1 explained 27.035% of the Variance and has positive statistical loadings with Copper (r = 0.839), Cobalt (r = 0.512), and Nickel (r = 0.900). This factor reveals strong positive correlations between copper, cobalt, and nickel, suggesting that these metals may originate from a common source or share similar pathways in the environment. Industrial activities or mining could be potential contributors to these metal concentrations in the river systems. F-2 explained 22.707% of the Variance and has positive statistical loadings with Zinc (r = 0.670), Cadmium (r = 0.859), and Cobalt (r = 0.592). The second factor demonstrates that zinc, cadmium, and cobalt are positively correlated. This may indicate that these metals are present together in certain areas, possibly due to overlapping pollution sources or similar environmental factors that affect their bioavailability. F-3 explained 21.529% of the Variance and has positive statistical loadings with Iron (r = 0.752), and negative Loadings with Lead (r = −0.810). The third factor shows a strong positive loading for iron and a negative loading for lead, suggesting that areas with high iron concentrations might have lower lead levels. This inverse relationship could reflect differences in the sources or chemical behavior of these metals in the aquatic.
For both fish species, three components were formed. Within the same component, many of the metals did not show strong positive loading, indicating that the elements in the fish are from multiple human activities. Some identified sources of elements include agricultural activities, wastrace metals, runoff resulting from human activities on the soil, oil and gas activities, etc. It is also interesting to see that the degree of association also followed this trend. This pattern has been reported several times in the literature (Singh et al., 2015; Talukder et al., 2022; Chandrasekaran et al., 2015). Hence, the PCA could identify the sources that account for the variation in the trace metals composition of the two fish species and thereby explain the variability observed. In both fish species, some trace metals have no relation to the other components. This trend has been previously reported by Talukder et al. (2022).
A high total variance explained by the components (e.g., above 70%) indicates that the principal components effectively summarize the original data. This means that the majority of the information in the data has been captured by the three components. This high variance suggests that the identified components represent key factors influencing metal concentrations. For the Clarias batrachus (72.47% variance), this indicates that the three principal components capture 72.47% of the total variability in the concentrations of trace metals. The first factor (F-1) alone explains 24.653% of the variance, indicating that it is the most significant factor affecting trace metal levels in this species. For the Chrysichthys nigrodigitatus (71.271% variance), it also showed that three components were explained, with the first factor (F-1) contributing 27.035%, showing it is a critical factor in the trace metal distribution.
Figures 1, 2 illustrate the hierarchical cluster analyses of trace metal distributions in the muscles of two fish species, Chrysichthys nigrodigitatus and Clarias batrachus, collected from Bomadi and Gbotebo rivers and their surrounding areas in Bayelsa State, Nigeria. The analysis aims to categorize and reveal distinct patterns of trace metal accumulation within these species, providing insights into environmental contamination and metal bioaccumulation.
Figure 1. Hierarchical cluster analysis (Euclidean Distance, Ward Linkage) of trace metals in muscles of Chrysichthys nigrodigitatus from Bomadi and Gbotebo rivers and their environs in Bayelsa State, Nigeria.
Figure 2. Hierarchical cluster analysis (Euclidean Distance, Ward Linkage) of trace metals in muscles of Clarias batrachus from Bomadi and Gbotebo rivers and their environs in Bayelsa State, Nigeria.
The hierarchical cluster analysis for Chrysichthys nigrodigitatus delineates two primary clusters: Cluster A is predominantly characterized by the presence of iron. The grouping suggests that iron accumulates in a distinct manner compared to other trace metals in the fish muscles. Cluster B includes the remaining metals and is further subdivided into two sub-clusters (1 and 2). Sub-cluster 1 comprises a diverse set of metals including iron, copper, nickel, cadmium, cobalt, lead, and zinc. Within this sub-cluster, there is a further division (I and II). Sub-sub-cluster I contains lead alone. This isolation of lead indicates a unique accumulation pattern or source of lead distinct from other metals. Sub-sub-cluster II includes copper, nickel, cadmium, and cobalt. Notably, copper and cadmium are highlighted for their equal distances from each other, indicating a similar pattern of distribution or bioaccumulation. Sub-cluster 2 consists of sodium and calcium. This sub-cluster forms a separate group from the metals in Sub-cluster 1, suggesting different environmental or physiological factors influencing their accumulation.
The hierarchical cluster analysis for Clarias batrachus reveals two major clusters (A and B). Cluster A includes iron and zinc, which are grouped together at equal distances. This indicates that these two metals share similar accumulation patterns in the fish muscles. Cluster B involves the remaining metals, which are organized into two sub-clusters (1 and 2). Sub-cluster 1 contains lead alone, showing a distinct pattern of accumulation that separates it from other metals in this analysis. Sub-cluster 2 is divided into two smaller groups (1 and 2). Group 1 includes copper and nickel. These metals are found at equal distances from each other, suggesting a similar pattern of distribution or accumulation. Group 2 consists of cadmium and cobalt. These metals also exhibit equal distances between them, indicating a similar pattern of bioaccumulation.
The hierarchical cluster analyses for both fish species reveal distinct patterns of trace metal accumulation, reflecting their environmental exposure and physiological processes. For Chrysichthys nigrodigitatus, the separation of iron in Cluster A indicates a prominent role in the metal profile of this species, while the more complex grouping in Cluster B suggests varying sources or mechanisms of accumulation for other metals. The isolation of lead in Sub-sub-cluster I highlights its unique accumulation behavior, potentially linked to specific environmental factors or sources. In Clarias batrachus, the grouping of iron and zinc in Cluster A shows a similar distribution pattern, while the distinct isolation of lead in Sub-cluster 1 points to its specific accumulation characteristics. The division of Sub-cluster 2 into groups containing copper and nickel, and cadmium and cobalt, further emphasizes the varying distribution patterns and bioaccumulation tendencies of these metals. Overall, these clustering patterns provide valuable insights into the environmental contamination and bioaccumulation of trace metals in fish from these rivers.
Generally, the cluster analysis reduced the element into three groups for both fish species based on the similarity in the trace metals composition. This could help reduce the number of elements for further studies. The trace metals within the same cluster showed a high association level (Singh et al., 2015). While elements with separate collectors show dissimilarity in sources of contamination (Gyimah et al., 2018). The pollutants may be from multiple anthropogenic activities, including waste materials, oil and gas activities, agrochemicals use, soil elements leaching into the water during flooding periods, or soil erosion.
Health risks from toxicants, such as trace metals, can be both cancerous and non-cancerous. Both are frequently utilized to illustrate the various ways in which exposure to toxins can harm the body. The main determinant of whether or not a trace metals is harmful to the human body is the amount consumed daily (Aigberua et al., 2021). The various exposure routes, such as ingestion, inhalation, and skin contact, also impact daily consumption. Trace elements enter the body most commonly through the digestive system. Hence, this exposure category is included first (Aigberua et al., 2021).
Estimated daily intake of the fish for adults and children (mg/kg body weight/day) through the consumption of Clarias batrachus from Bomadi and Gbotebo rivers and their environs, Bayelsa State, Nigeria, is shown in Tables 8, 9, respectively. The EDIf of iron, copper, zinc, lead, nickel, cadmium, and cobalt ranged from 6.49–17.44 (mean 11.76), 0.18–1.34 (mean 0.82), 2.89–6.68 (mean 4.61), NCCD—3.46 (mean 1.07), NCCD—0.87 (mean 0.26), NCCD—0.53 (mean 0.05), NCCD—0.18 (mean 0.03), respectively the adult (Table 9), and 1.07–2.88 (mean 1.95), 0.03–0.22 (mean 0.14), 0.48–1.10 (mean 0.76), NCCD—0.57 (mean 0.18), NCCD—0.14 (mean 0.04), NCCD—0.09 (mean 0.01), and NCCD—0.03 (mean 0.01) for the children (Table 10). Based on the mean, the distribution of elements was in the order iron > zinc >lead > copper > nickel > cadmium > cobalt (for adults) and iron > zinc > lead > copper > nickel > cadmium = cobalt.
Table 8. EDIf for adults (mg/kg) through the consumption of Clarias batrachus from Bomadi and Gbotebo rivers and their environs in Bayelsa State, Nigeria.
Table 9. EDIf for children (mg/kg) through the consumption of Clarias batrachus from Bomadi and Gbotebo rivers and their Environs in Bayelsa State, Nigeria.
Table 10. EDIf for adults (mg/kg) through the consumption of Chrysichthys nigrodigitatus from Bomadi and Gbotebo rivers and their Environs in Bayelsa State, Nigeria.
Furthermore, the EDIf for adults and children (mg/kg body weight/day) through the consumption of Chrysichthys nigrodigitatus from the Bomadi and Gbotebo rivers and their environs in Bayelsa State, Nigeria, is shown in Tables 10, 11, respectively. The EDIf of iron, copper, zinc, lead, nickel, cadmium and cobalt from 6.64–19.70 (mean 10.57), 0.15–4.99 (mean 1.02), 1.75–17.29 (mean 7.13), NCCD – 5.02 (mean 1.07), NCCD – 4.92 (mean 0.64), NCCD – 0.80 (mean 0.15), and NCCD – 0.58 (mean 0.06) respectively the adult (Table 10), and 1.10–3.26 (mean 1.75), 0.02–0.83 (mean 0.17), 0.29–2.86 (mean 1.18), NCCD—0.83 (mean 0.18), NCCD—0.81 (mean 0.11), NCCD—0.13 (mean 0.02), and NCCD—0.10 (mean 0.01), for the children (Table 11). Based on the mean, the distribution of elements was in the order iron > zinc >lead > copper > nickel > cadmium > cobalt (for adults) and iron > zinc > lead > copper > nickel > cadmium > cobalt.
Table 11. EDIf for children (mg/kg) through the consumption of Chrysichthys nigrodigitatus from Bomadi and Gbotebo rivers and their Environs in Bayelsa State, Nigeria.
Supplementary material 3, 4 and Table 12 show the THQf for adults and children (mg/kg) through the consumption of Clarias batrachus, respectively, from the Bomadi and Gbotebo rivers and their environs in Bayelsa State, Nigeria. Iron, copper, and zinc had THQf values in the range 10−3–10−2 with a mean value of 10−2. A THQf value of 10−3 was reported in fish sample SF29 for iron, SF1, SF11, SF21, and SF22 for copper, and SF20, SF22, and SF29 for zinc for the adults (Supplementary material 3). THQf in fish samples ranged from 10−2 to 10−1 for lead and cadmium, 10−3 to 10−1 for nickel, and 10−5 to 10−3 for cobalt in adults (Table 13). Iron and zinc were in the order of 10–3 for children. Also, copper ranged from 10−4 to 10−3. Furthermore, THQf in fish samples, the elements that were detected are in the range of 10−3–10−1 for lead, 10−3–10−2 for cadmium, 10−6–10−3 for cobalt, while nickel is in the order 10−3 (Table 12).
Table 12. Non-carcinogenic health hazards for adults and children (mg/kg) through the consumption of Clarias batrachus and Chrysichthys nigrodigitatus from Bomadi and Gbotebo rivers and their Environs in Bayelsa State, Nigeria.
Table 13. Carcinogenic hazard of trace metals through the consumption of Clarias batrachus and Chrysichthys nigrodigitatus from some rivers in Bayelsa State, Nigeria.
Supplementary material 5, 6 and Table 12 show the THQf for adults and children (mg/kg) through consuming Chrysichthys nigrodigitatus, respectively, from the Bomadi and Gbotebo rivers and their environs in Bayelsa State, Nigeria. For adults, the range of THQf values in the sample detected was in the range of 10−1–101 for lead, 10−2–10−1 for nickel, and 10−3–10−2 for cobalt, while cadmium was in the order 10−1. Also, iron and zinc were in orders 10–3 (Table 12). For children, the range of THQf values in the sample detected ranged from 10−2 to 10−1 for lead and cadmium, 10−2 to 10−2 for nickel, and 10−4 to 10−3 for cobalt. Also, iron was in the order of 10−3, while zinc was in the range of 10–4–10−3 (Supplementary material 6).
Supplementary material 7 and Table 12 show the TTHQf of trace metals through the consumption of Clarias batrachus and Chrysichthys nigrodigitatus from some Bayelsa State, Nigeria rivers. The TTHQf ranged from 10−2 to 101 (mean 10−1) for adults and 10−3 to 101 (mean 10−2) for children in both fish species. The adult THQf values for each of the metals were less than 1 (<1) except for instances (BF 6; BF 14, and BF 16) where the value was >1. The adult values for TTHQf were 1, except for a few instances (BF 6; BF 10, BF 14; BF 16), where the values were also >1.0. The values reported in this study had some similarities with previous reports. TTHQf >1 has been reported in a few instances in health risk of trace metals involving Sarotherodon melanotheron and Chrysichthys nigrodigitatus from Okulu River, Nigeria (Aigberua et al., 2021). Amankwaa et al. (2021) investigated the presence of trace metals in the tissues of Clarias batrachus and Chrysichthys nigrodigitatus from the Densu River, and found that the hazard quotient for lead, cadmium, and copper in both fish species was less than 1 (<1), indicating a low risk of non-carcinogenic effects from these individual metals. However, the hazard index (HI) for the cumulative exposure to all studied metals was greater than 1 (>1), suggesting potential health concerns. Furthermore, Oladapo and Taiwo (2024) assessed the HI for heavy metals in Clarias batrachus and Chrysichthys nigrodigitatus, finding values of 6.72 × 10−4 and 6.71 × 10−4 for adults and children, respectively, for Clarias batrachus, and 1.04 × 10−3 and 1.01 × 10−3 for Chrysichthys nigrodigitatus. The authors further reported that these values are below the safety threshold of 1, indicating low non-carcinogenic risk.
Inorganic lead and cadmium have been reported as carcinogenic metals and have deleterious effects on humans. Furthermore, these metals have no known direct biological function in humans. Hence, the health hazards of these carcinogenic metals are essential to estimate the lifetime cancer hazards from consuming fish contaminated by them (Jisr et al., 2020).
Given that cadmium and lead concentrations were higher than the European Union maximum limits, there is a potentially large hazard owing to toxicity associated with the consumption of these fishes (Chrysichthys nigrodigitatus and Clarias batrachus). Therefore, in an attempt to evaluate health risks from chronic exposure to cadmium and lead in both fish species, cancer hazard values were calculated (Supplementary material 8, 9, and Table 13). For Clarias batrachus, lead, cadmium and total carcinogenic hazard values were in the range of 10−7–10−5 (mean 10−6), 10−2–10−1 (mean 10−2), and 10−6–10−1 (mean 10−2), respectively for adult, and 10−7–10−6 (mean 10−6), 10−5–10−4 (mean 10−5), and 10−7–10−4 (mean 10−5), respectively for children.
For Chrysichthys nigrodigitatus, lead, cadmium, and total carcinogenic hazard values were in the range of 10−6–10−5 (mean 10−6), 10−2–10−1 (mean 10−2) and 10−5–10−1 (mean 10−2), respectively for adult, and 10−7–10−6 (mean 10−6), 10−5–10−4 (mean 10−4) and 10−6–10−4 (mean 10−4), respectively for children.
Based on the mean values for Clarias batrachus and Chrysichthys nigrodigitatus, lead under both categories (adult and children) showed extremely low hazard, which is acceptable. Cadmium and total carcinogenic hazard mean values for both fish showed exceedingly high carcinogenic hazards for adults and moderate hazards for children, as previously classified in the literature (Uzoekwe et al., 2021; Izah et al., 2022; Ogamba et al., 2021). Generally, the mean carcinogenic hazards for individual and combined elements have an acceptable value of 10−6–10−4 as recommended by the United States Environmental Protection Agency (Arisekar et al., 2020), except for the cadmium and total carcinogenic hazards in both fish species. The fact that many of the samples meant for assessing the carcinogenic hazard of cadmium are in orders 10−2 and 10 reveals carcinogenic tendencies; hence, adverse health conditions may be possible with the lifetime consumption of fish species from the studied rivers. The health hazards of cadmium and lead have been comprehensively reported by Izah et al. (2016).
The values reported are from previous studies. For instance, Aigberua et al. (2021) reported carcinogenic hazards of cadmium and lead in the range of 10−2–10−1 and 10−4–10−2, respectively, in Sarotherodon melanotheron and Chrysichthys nigrodigitatus from Okulu river, River State, Nigeria. However, the values are different from those of other studies, for instance. Furthermore, Oladapo and Taiwo (2024) reported carcinogenic risk associated with the consumption of trace metals in Clarias batrachus and Chrysichthys nigrodigitatus as 4.10 × 10−7 for adults and 3.78 × 10−7 for children (for Clarias batrachus), and 6.10 × 10−7 for adults and 6.16 × 10−7 for children for Chrysichthys nigrodigitatus. The authors further reported a combined carcinogenic risk index indicated that consuming these fish species over a lifetime is considered safe for both adults and children. The variation in the carcinogenic tendencies could be due to the source and characteristics of the pollutants in different surface water.
Due to human activity, toxic substances such as elements find their way into aquatic environments. Aquatic organisms like fish can bio-accumulate hazardous elements in their tissues. This poses a risk to the health of humans who consume these fish since these toxic elements are then transferred into their bodies. This study investigated the levels of a variety of trace metals such as iron, copper, zinc, lead, nickel, cadmium, and cobalt in the muscles of Clarias batrachus and Chrysichthys nigrodigitatus in the Bomadi and Gbotebo rivers and their environs in Bayelsa State, Nigeria. In addition, it explored the possible dangers these elements posed to human health and the sources from which they originated. The study found no statistical differences between the mean values of both fish concerning iron, copper, lead, and cobalt. However, statistical dissimilarities exist between the fish species for zinc, nickel and cadmium. This suggests a similar trend in the bioaccumulation pattern of trace metals in tissues of both fish species. The concentration of some elements, such as cadmium, lead, zinc, and copper, exceeded some of the permissible limits given by the European Union, the median international standard, and FAO/WHO. The findings of the Pearson correlation, PCA, and Cluster analysis all point to the fact that trace metals originate from diverse anthropogenic activities. PCA indicates a complexity in the data because less than 50% of the total variance was explained by the first two components in both fish species. It was found that the THQf and the Total TTHQf for children were less than 1 both species of fish. However, for adults, the TTHQf exceeded 1 at its maximum (only) for Clarias batrachus and Chrysichthys nigrodigitatus. This evidence indicates the possibility of non-carcinogenic risks associated with the eating of fish food throughout a person’s lifetime. On the other hand, the mean carcinogenic hazards for cadmium and total carcinogenic hazards were much lower than the threshold values of 10−6 to 10−4 in adults for both fish. In addition, the utilization of strict values demonstrated that the only acceptable lead is derived from both types of fish included in both categories (adults and children). Therefore, consuming either variety of fish may put people (children and adults) at risk of being exposed to either non-carcinogenic or carcinogenic dangers. As a result, it is necessary to raise awareness among customers who buy fish food that originates from the area that is the primary focus of the inquiry. In addition, to protect consumers’ health, it is necessary to conduct routine assessments of potentially harmful elements that may be present in surface water, sediments, and fish food.
The raw data supporting the conclusions of this article will be made available by the authors, without undue reservation.
The study was approved by Bayelsa Medical University Research and Ethics Committee. The study was conducted in accordance with the local legislation and institutional requirements.
SI: Conceptualization, Data curation, Formal analysis, Funding acquisition, Investigation, Methodology, Project administration, Resources, Validation, Visualization, Writing – original draft, Writing – review & editing. GR: Conceptualization, Investigation, Supervision, Writing – review & editing. HS: Supervision, Writing – review & editing. WS: Supervision, Project administration, Writing – review & editing. OU: Supervision, Project administration, Writing – review & editing. LS: Software, Validation, Writing – review & editing.
The author(s) declare financial support was received for the research, authorship, and/or publication of this article. The authors declare that this paper is part of the first author’s PhD dissertation at Niger Delta University.
The authors declare that the research was conducted in the absence of any commercial or financial relationships that could be construed as a potential conflict of interest.
All claims expressed in this article are solely those of the authors and do not necessarily represent those of their affiliated organizations, or those of the publisher, the editors and the reviewers. Any product that may be evaluated in this article, or claim that may be made by its manufacturer, is not guaranteed or endorsed by the publisher.
The Supplementary material for this article can be found online at: https://www.frontiersin.org/articles/10.3389/fsufs.2024.1419143/full#supplementary-material
PCA, Principal component analysis; CA, Cluster analysis; THQf, Target hazard quotient for fish; TTHQf, The total target hazard quotient for fish.
Acharya, P., Muduli, P. R., and Das, M. (2023). Assessment of heavy metal accumulation in Penaeus monodon and its human health implications. Mar. Pollut. Bull. 188:114632. doi: 10.1016/j.marpolbul.2023.114632
Ada, F. B., Ekpenyong, E., and Bayim, B. P.-R. (2012). Heavy metal concentration in some fishes (Chrysichthys nigrodigitatus, Clarias gariepinus and Oreochromis niloticus) in the Great Kwa River, Cross River State, Nigeria. Global Adv. Res. J. Environ. Sci. Toxicol. 1, 183–189.
Adelakun, K. M., and Kehinde, A. S. (2019). Heavy metals bioaccumulations in Chrysichthys nigrodigitatus (silver catfish) from river oli, Kainji Lake National Park, Nigeria. Egypt. J. Aquatic Biol. Fish. 23, 253–259. doi: 10.21608/ejabf.2019.66652
Adeyemi, A. A., and Ojekunle, Z. O. (2021). Concentrations and health risk assessment of industrial heavy metals pollution in groundwater in Ogun state, Nigeria. Sci. African 11:e00666. doi: 10.1016/j.sciaf.2020.e00666
Agedah, E. C., Ineyougha, E. R., Izah, S. C., and Orutugu, L. A. (2015). Enumeration of total heterotrophic bacteria and some physico-chemical characteristics of surface water used for drinking sources in Wilberforce Island, Nigeria. J. Environ. Treat. Tech. 3, 28–34.
Aghoghovwia, O. A., Ohimain, E. I., and Izah, S. C. (2016). Bioaccumulation of heavy metals in different tissues of some commercially important fish species from Warri River, Niger Delta, Nigeria. Biotechnol. Res. 2, 25–32.
Aigberua, A. O., Izah, S. C., and Isaac, U. I. (2018). Level and health risk assessment of heavy metals in selected seasonings and culinary condiments used in Nigeria. Biol. Evid. 8, 6–15. doi: 10.5376/be.2018.08.0002
Aigberua, A. O., Izah, S. C., and Richard, G. (2021). Hazard analysis of trace metals in muscle of Sarotherodon melanotheron and Chrysichthys nigrodigitatus from Okulu River, Rivers state, Nigeria. J. Environ. Health Sustain. Dev. 6, 1340–1356. doi: 10.18502/jehsd.v6i3.7242
Amankwaa, G., Lu, Y., Liu, T., Wang, N., Luan, Y., Cao, Y., et al. (2021). Heavy metals concentration profile of an aquatic environment and health implications of human exposure to fish and prawn species from an urban river (Densu). Iran. J. Fish. Sci. 20, 529–546. doi: 10.22092/ijfs.2021.351023.0
Arisekar, U., Shakila, R. J., Shalini, R., and Jeyasekaran, G. (2020). Human health risk assessment of heavy metals in aquatic sediments and freshwater fish caught from Thamirabarani River, the Western Ghats of South Tamil Nadu. Mar. Pollut. Bull. 159:111496. doi: 10.1016/j.marpolbul.2020.111496
Ayotunde, E. O., Offem, B. O., and Ada, B. F. (2012). Heavy metal profile of water, sediment and freshwater cat fish, Chrysichthys nigrodigitatus (Siluriformes: Bagridae), of Cross River, Nigeria. Rev. Biol. Trop. 60, 1289–1301.
Bassey, O. B., and Chukwu, L. O. (2019). Health risk assessment of heavy metals in fish (Chrysichthys nigrodigitatus) from two lagoons in southwestern Nigeria. J. Toxicol. Risk Assess. 5:027. doi: 10.23937/2572-4061.1510027
Chandrasekaran, A., Ravisankar, R., Harikrishnan, N., Satapathy, K. K., Prasad, M. V. R., and Kanagasabapathy, K. V. (2015). Multivariate statistical analysis of heavy metal concentration in soils of Yelagiri Hills, Tamilnadu, India–Spectroscopical approach. Spectrochim. Acta A Mol. Biomol. Spectrosc. 137, 589–600. doi: 10.1016/j.saa.2014.08.093
Ehiemere, V. C., Ihedioha, J. N., Ekere, N. R., Ibeto, C. N., and Abugu, H. O. (2022). Pollution and risk assessment of heavy metals in water, sediment and fish (Clarias gariepinus) in a fish farm cluster in Niger Delta region, Nigeria. J. Water Health 20, 927–945. doi: 10.2166/wh.2022.003
Ekere, N. R., Yakubu, N. M., and Ihedioha, J. N. (2018). Assessment of levels and potential health risk of heavy metals in water and selected fish species from the Benue-Niger River confluence, Lokoja, Nigeria. J. Aquatic Food Product Technol. 27, 772–782. doi: 10.1080/10498850.2018.1499061
Elnabris, K. J., Muzyed, S. K., and El-Ashgar, N. M. (2013). Heavy metal concentrations in some commercially important fishes and their contribution to heavy metals exposure in Palestinian people of Gaza strip (Palestine). J. Assoc. Arab Univ. Basic Appl. Sci. 13, 44–51. doi: 10.1016/j.jaubas.2012.06.001
Eneji, I. S., Sha'Ato, R., and Annune, P. A. (2011). Bioaccumulation of heavy metals in fish (Tilapia Zilli and Clarias Gariepinus) organs from river Benue, north--Central Nigeria. Pak. J. Analy. Environ. Chem. 12, 25–31.
European Union (2002). The Commission of the European Communities, commission regulation, (EC) no. 22112002 amending regulation (EC) no. 466/2001 setting maximum levels for certain contaminants in food stuff in order to protect public health. Official Journal of the European Communities.
Food and Agricultural Organization of the United Nations (FAO/UN) (2020). The State of World Fisheries and Aquaculture 2020. Available online at: https://www.fao.org/state-of-fisheries-aquaculture#:~:text=Fish%20provided%20about%203.3%20billion,of%20all%20proteins%2C%20consumed%20globally (Accessed April 16 2022).
Food and Agricultural Organization/World Health Organization (FAO/WHO) (1989). Evaluation of certain food additives and the contaminants mercury, lead and cadmium. WHO Technical Report, Series No. 505.
Gijo, A. H., and Alagoa, K. J. (2022). Ichthyofaunal study of the river Nun around Tombia community, Niger Delta, Nigeria. Quest J. Res. Agric. Anim. Sci. 9, 01–06. doi: 10.52403/ijrr.20220237
Gyimah, E., Akoto, O., Mensah, J. K., and Bortey-Sam, N. (2018). Bioaccumulation factors and multivariate analysis of heavy metals of three edible fish species from the Barekese reservoir in Kumasi, Ghana. Environ. Monit. Assess. 190:553. doi: 10.1007/s10661-018-6938-2
Izah, S. C., Aigberua, A. O., and Richard, G. (2022). Concentration, source, and health risk of trace metals in some liquid herbal medicine sold in Nigeria. Biol. Trace Elem. Res. 200, 3009–3022. doi: 10.1007/s12011-021-02879-9
Izah, S. C., and Angaye, T. C. (2016). Heavy metal concentration in fishes from surface water in Nigeria: potential sources of pollutants and mitigation measures. Sky J. Biochem. Res. 5, 31–47.
Izah, S. C., Chakrabarty, N., and Srivastav, A. L. (2016). A review on heavy metal concentration in potable water sources in Nigeria: human health effects and mitigating measures. Expo. Health 8, 285–304. doi: 10.1007/s12403-016-0195-9
Izah, S. C., Richard, G., Stanley, H. O., Sawyer, W. E., Ogwu, M. C., and Uwaeme, O. R. (2023). Integrating the one health approach and statistical analysis for sustainable aquatic ecosystem management and trace metal contamination mitigation. ES Food Agrofor. 14:1012. doi: 10.30919/esfaf1012
Izah, S. C., Stanley, H. O., Richard, G., Sawyer, W. E., and Uwaeme, O. R. (2024). Surface water quality: a statistical perspective on the efficacy of environmental and human health assessment tools. Water Air Soil Pollut. 235:205. doi: 10.1016/j.indic.2023.100247
Jisr, N., Younes, G., El Omari, K., Hamze, M., Sukhn, C., and El-Dakdouki, M. H. (2020). Levels of heavy metals, total petroleum hydrocarbons, and microbial load in commercially valuable fish from the marine area of Tripoli, Lebanon. Environ. Monit. Assess. 192, 1–13. doi: 10.1007/s10661-020-08672-w
Joel, E. S., Maxwell, O., Adewoyin, O. O., Ehi-Eromosele, C. O., Embong, Z., and Oyawoye, F. (2018). Assessment of natural radioactivity in various commercial tiles used for building purposes in Nigeria. MethodsX 5, 8–19. doi: 10.1016/j.mex.2017.12.002
Matouke, M. M., and Abdullahi, K. L. (2020). Assessment of heavy metals contamination and human health risk in Clarias gariepinus [Burchell, 1822] collected from Jabi Lake, Abuja, Nigeria. Sci. African 7:e00292. doi: 10.1016/j.sciaf.2020.e00292
Ogamba, E. N., Charles, E. E., and Izah, S. C. (2021). Distributions, pollution evaluation and health risk of selected heavy metal in surface water of Taylor creek, Bayelsa state, Nigeria. Toxicol. Environ. Heal. Sci. 13, 109–121. doi: 10.1007/s13530-020-00076-0
Ogamba, E. N., Izah, S. C., and Isimayemiema, F. (2016b). Bioaccumulation of heavy metals in the gill and liver of a common Niger Delta wetland fish, Clarias garepinus. Br. J. Appl. Res. 1, 17–20. doi: 10.22259/2637-5338.0303004
Ogamba, E. N., Izah, S. C., and Ofoni-Ofoni, A. S. (2016c). Bioaccumulation of chromium, Lead and cadmium in the bones and tissues of Oreochromis niloticus and Clarias camerunensis from Ikoli creek, Niger Delta, Nigeria. Adv. Sci. J. Zool. 1, 13–16.
Ogamba, E. N., Izah, S. C., and Omonibo, E. (2016a). Bioaccumulation of hydrocarbon, heavy metals and minerals in Tympanotonus fuscatus from coastal region of Bayelsa state, Nigeria. Int. J. Hydrol. Res. 1, 1–7. doi: 10.18488/journal.108/2016.1.1/108.1.1.7
Ogoko, E. C., Nkoli, M., Kelle, H., Osu, C. I., and Aju, E. (2022). Health risk assessment of exposure to heavy metals in fish species consumed in aba, Abia state, Nigeria. Ovid. Univ. Ann. Chem. 33, 177–187. doi: 10.2478/auoc-2022-0026
Oladapo, F. O., and Taiwo, H. A. (2024) Human health implications of heavy metal levels in three fish species from Lagos lagoon. Oladapo, Francis Olumide and Taiwo, Helen Abisoye, human health implications of heavy metal levels in three fish species from Lagos lagoon. Available online at: https://ssrn.com/abstract=4831475 (Accessed August 05, 2024).
Olgunoğlu, M. P., Artar, E., and Olgunoğlu, İ. A. (2015). Comparison of heavy metal levels in muscle and gills of four benthic fish species from the northeastern Mediterranean Sea. Pol. J. Environ. Stud. 24, 1743–1748. doi: 10.15244/pjoes/38972
Philips, D. J. H. (1993). “Developing country aquaculture—trace chemical contaminants and public health concerns” in Environment and Quaculture in Developing Countries. ICLARl'V1 Conference Proceedings. vol 31. eds. Pullin RSV, Rosenthal H, Maclean JL, 296–311.
Qing, X., Yutong, Z., and Shenggao, L. (2015). Assessment of heavy metal pollution and human health risk in urban soils of steel industrial city (Anshan), Liaoning, Northeast China. Ecotoxicol. Environ. Saf. 120, 377–385. doi: 10.1016/j.ecoenv.2015.06.019
Sasu, D. D. (2022). Life expectancy at birth in Nigeria 2021, by gender. Available online at: https://www.statista.com/statistics/1122851/life-expectancy-in-nigeria-by-gender/#:~:text=In%202021%2C%20life%20expectancy%20at,well%20as%20in%20the%20world (Accessed February 20, 2022).
Senarathne, P., and Pathiratne, K. A. S. (2007). Accumulation of heavy metals in a food fish, Mystus gulio inhabiting Bolgoda Lake, Sri Lanka. J. Aquat. Sci. 12, 61–75. doi: 10.4038/sljas.v12i0.2214
Senarathne, P., Pathiratne, K. A. S., and Pathiratne, A. (2006). Heavy metal levels in food fish, Etroplus suratensis inhabiting Bolgoda Lake, Sri Lanka. Vidyodaya J. Sci. 13, 115–126.
Signes-Pastor, A. J., Sayarath, V., Jackson, B., Cottingham, K. L., Punshon, T., and Karagas, M. R. (2022). Dietary exposure to essential and non-essential elements during infants’ first year of life in the New Hampshire birth cohort study. Expo. Health 15, 269–279. doi: 10.1007/s12403-022-00489-x
Singh, P., Chaturvedi, R. K., Mishra, A., Kumari, L., Singh, R., Pal, D. B., et al. (2015). Assessment of ground and surface water quality along the river Varuna, Varanasi, India. Environ. Monit. Assess. 187:170. doi: 10.1007/s10661-015-4382-0
Sobolev, N., Aksenov, A., Sorokina, T., Chashchin, V., Ellingsen, D. G., Nieboer, E., et al. (2019). Essential and non-essential trace elements in fish consumed by indigenous peoples of the European Russian Arctic. Environ. Pollut. 253, 966–973. doi: 10.1016/j.envpol.2019.07.072
Talukder, R., Rabbi, M. H., Baharim, N. B., and Carnicelli, S. (2022). Source identification and ecological risk assessment of heavy metal pollution in sediments of Setiu wetland, Malaysia. Environ. Forensic 23, 241–254. doi: 10.1080/15275922.2021.1892871
Tchounwou, P. B., Yedjou, C. G., Patlolla, A. K., and Sutton, D. J. (2012). “Heavy metal toxicity and the environment” in Molecular, Clinical and Environmental Toxicology. Experientia Supplementum. ed. A. Luch, vol. 101 (Basel: Springer), 133–164.
United Statrace Metals Environmental Protection Agency (USEPA) (2012). EPA region III risk based concentration (RBC) Table 2008.
Keywords: aquatic ecosystem, environmental health, fish food, health risks, public health, water, pollution
Citation: Izah SC, Richard G, Stanley HO, Sawyer WE, Uwaeme OR and Sylva L (2024) Source and health risks of trace metals in Clarias batrachus and Chrysichthys nigrodigitatus from surface waters in Bayelsa State, Nigeria: a probabilistic model. Front. Sustain. Food Syst. 8:1419143. doi: 10.3389/fsufs.2024.1419143
Received: 17 April 2024; Accepted: 29 August 2024;
Published: 17 September 2024.
Edited by:
M. Belal Hossain, Noakhali Science and Technology University, BangladeshReviewed by:
Vignesh Sivanandham, Indian Institute of Food Processing Technology, IndiaCopyright © 2024 Izah, Richard, Stanley, Sawyer, Uwaeme and Sylva. This is an open-access article distributed under the terms of the Creative Commons Attribution License (CC BY). The use, distribution or reproduction in other forums is permitted, provided the original author(s) and the copyright owner(s) are credited and that the original publication in this journal is cited, in accordance with accepted academic practice. No use, distribution or reproduction is permitted which does not comply with these terms.
*Correspondence: Sylvester Chibueze Izah, Y2hpdmVzdGl6YWhAZ21haWwuY29t
Disclaimer: All claims expressed in this article are solely those of the authors and do not necessarily represent those of their affiliated organizations, or those of the publisher, the editors and the reviewers. Any product that may be evaluated in this article or claim that may be made by its manufacturer is not guaranteed or endorsed by the publisher.
Research integrity at Frontiers
Learn more about the work of our research integrity team to safeguard the quality of each article we publish.