- 1Department of Agricultural Sciences/Agronomy, Instituto Federal Goiano (IFGoiano), Rio Verde, Brazil
- 2Department of Animal Sciences, Instituto Federal Goiano, Rio Verde, Brazil
- 3Department of Animal Science, Universidade Federal do Maranhão, Chapadinha, Brazil
- 4Department of Plant Production, Universidade Estadual de Goiás, Ipameri, Brazil
Integrated systems are efficient technologies used for diversifying production and promoting agricultural sustainability. The benefits of double intercropping are well-established in research. With advancements in sustainable food production technology, some studies have explored triple intercropping by adding legumes. However, triple intercropping methods must be comprehensively understood. This study aimed to evaluate the production of dry mass, fermentation profile, and nutritive values of silage from maize based intercropping. The experiment was conducted in the field, in Instituto Federal Goiano, Campus Rio Verde, State of Goiás, Brazil, where we assessed silage production and quality in monoculture and intercropping systems, in a randomized block complete design with three replications. We evaluated 8 treatments, consisting of silage from: maize monoculture; Tamani guinea grass monoculture; Quênia guinea grass monoculture; Zuri guinea grass monoculture; Pigeon pea monoculture; maize + Tamani guinea grass + Pigeon pea; maize + Quênia guinea grass + Pigeon pea and maize + Zuri guinea grass + Pigeon pea. The results showed that maize silage intercropped with cultivars of Panicum maximum (Tamani, Quênia and Zuri guinea grasses) and Pigeon pea increased silage mass production by 37.8% and the crude protein content at 25.1% compared with that of the monoculture maize silage. It also improved the fermentation characteristics of silage, reducing 5.1% of pH, 32.5% of buffering capacity, 28.9% of ammoniacal nitrogen in total nitrogen, 15.7% of effluent production, and 20.0% of dry matter losses, compared with those of grass and legume silage in monoculture. Thus, the silage produced by the triple intercropping proved to be an efficient technique for sustainable forage production.
1 Introduction
The search for food security, in contrast to the need to reduce the rate of deforestation, calls for the crucial development of efficient production systems with the flexibility to meet the associated demands (Huang et al., 2019; Herrera et al., 2023). In this context, the crop–livestock integration system is a promising alternative for making better use of the soil and increasing production per unit area during the year (Bieluczyk et al., 2020; Bi et al., 2024), as it aims to diversify the production of plant species in the same area, whether in physical or chronological space (Silva and Queiroz, 2002; Wang et al., 2020).
Integrated systems are efficient, inexpensive, and sustainable technologies for intensifying food production, reducing costs and risks, and conserving natural resources (Yi et al., 2022; Huang et al., 2023). With good management, this system facilitates multiple ecosystem services through increased carbon sequestration (Ferreira et al., 2018), water and soil conservation (Maia et al., 2022), recovery of degraded pastures (Silva et al., 2018), forage supply in the off-season (Dias et al., 2020). In addition, increased nutrient cycling (Renwick et al., 2020; Silva J. A. G. et al., 2023), reduced competition with invasive plants (Mello et al., 2023), and diversification of production (Meo-Filho et al., 2022; Silva et al., 2024b).
In this context, silage production from integrated systems has yielded satisfactory results, contributing to higher silage mass production and crude protein content than those of monoculture maize silage (Ligoski et al., 2020). Intercropping maize with tropical forage crops does not reduce grain or mass yields compared with those from monoculture maize (Souza et al., 2019; Herrera et al., 2023). After cutting the crops for silage production, the forage plants regrow, providing quality pasture for the animals at a low cost (Oliveira et al., 2020) and improving land-use efficiency in a sustainable manner (Prado et al., 2023). In addition, the production of silage from integrated systems has helped minimize the problems caused by the fermentation processes of silage sourced exclusively from grasses, which is reflected in the quality of the silage (Vicente et al., 2019).
Among forage plants, the genus Panicum maximum has shown potential for making silage in integrated systems, with high productivity per unit area (Prado et al., 2023) and good nutritional characteristics (Fernandes et al., 2023), with an increase in crude protein content and dry matter digestibility (Galeano et al., 2022). Daniel et al. (2019) they emphasize that the ensiling of grasses mixed with dry rations can produce silages with minimal leachate, greater intake potential, and better digestibility of the dry matter.
In addition, the presence of legumes such as Pigeon pea in integrated systems has also proven to be an excellent sustainable option for improving the nutritional quality of silage, promoting an increase in crude protein (Nave and Corbin, 2018; Ligoski et al., 2020; Gomes et al., 2021). This reduces the need for protein/mineral supplements, especially during the dry season (Silva L. M. et al., 2023), contributing to reducing greenhouse gas emissions and increasing animal productivity (Furtado et al., 2023). In addition, the use of legumes in integrated systems is also considered a low-cost, sustainable agricultural technology, as it increases the nitrogen input to the soil through the biological fixation of atmospheric nitrogen, reducing the use of mineral nitrogen fertilizers, and ensuring greater sustainability (Epifanio et al., 2019a; Epifanio et al., 2019b), as it allows for the partial and/or total replacement of nitrogen fertilizer. Matta et al. (2024) observed that including Pigeon pea in the production system provided savings of R$1716.30 per hectare in the application of nitrogen fertilizers.
The results of double intercropping (for example, maize with tropical forage plants) are well consolidated in research (Silva et al., 2018; Souza et al., 2019; Oliveira et al., 2020; Gomes et al., 2021; Galeano et al., 2022; Herrera et al., 2023). However, with the advancement of technology for more sustainable food production, some studies have already been developed with the triple intercropping adding legumes to the system (Prado et al., 2023). Silage produced from a consortium of maize, grasses, and tropical legumes can benefit the soil–plant–animal system by ensuring plant nutrition, soil conservation, fertility maintenance, carbon sequestration (Bourscheidt et al., 2023), and improved soil health (Kumari et al., 2023) and maximizing productivity, quality, and profitability (Ligoski et al., 2020).
Therefore, we hypothesized that the triple intercropping can increase dry mass production per hectare, improve fermentation characteristics, and produce better-quality silage with a higher protein content than those of silage produced from monoculture crops. In this way, the objective was to evaluate the production of dry mass, fermentation profile, and nutritive values of maize silage intercropped with Panicum maximum cultivars and Pigeon pea.
2 Materials and methods
2.1 Description of the area and crops establishments
This experiment was conducted under field conditions, in one season, at Instituto Federal Goiano, Campus Rio Verde, located in the municipality of Rio Verde, State of Goiás, Brazil (17° 48’ S, 50° 54’ W and 832 m asl). The region’s climate, according to the Köppen-Geiger classification (Cardoso et al., 2014), is defined as tropical climate (Aw) with a dry season in winter.
Before the experiment was set up, soil samples were collected from the 0–20 cm layer for physical–chemical characterization of the soil. The soil in the experimental area was characterized as Dystroferric Red Latosol (Santos et al., 2018), with 562 g kg−1 of clay; 94 g kg−1 of silt and 344 g kg−1 sand; pH in CaCl2: 5.5; calcium (Ca): 2.70 cmolc dm−3; magnesium (Mg): 1.40 cmolc dm−3; aluminium (Al): 0.01 cmolc dm−3; hydrogen (H) + Al: 3.41 cmolc dm−3; potassium (K): 0.70 cmolc dm−3; cation exchange capacity: 8.21 cmolc dm−3; current base saturation of the soil (V1): 58.5%; phosphorus (P) (mehlich): 3.5 mg dm−3; sulfur (S): 8.6 mg dm−3; copper (Cu): 3.5 mg dm−3; zinc (Zn): 1.0 mg dm−3; iron (Fe): 17.2 mg dm−3; organic matter (O.M.): 34.7 g dm−3. During the research, rainfall, maximum, average and minimum temperature data were monitored (Figure 1). Normal rainfall distribution was observed with a total rainfall of 621 mm, an average maximum temperature of 29.5°C, an average temperature of 23.4°C and a minimum temperature of 17.3°C throughout the experiment.
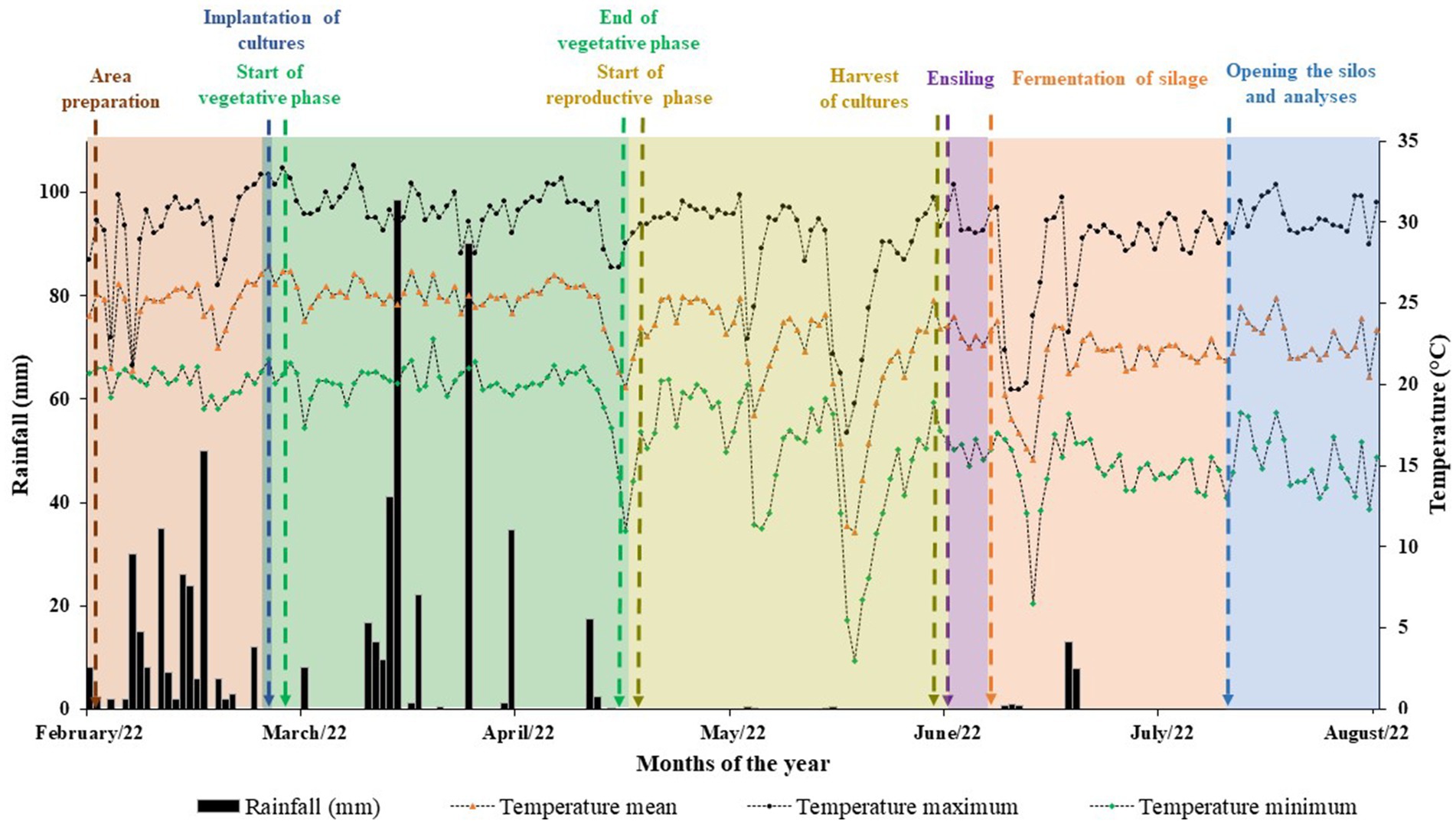
Figure 1. Monthly rainfall and minimum, average and maximum temperatures recorded from February to August 2022 in Rio Verde - GO, Brazil.
2.2 Experimental design and treatments
The experimental design used was a randomized complete block with three replications. The treatments consisted of silage: maize (Zea mays L.) monoculture; Tamani guinea grass (Panicum maximum) monoculture; Quênia guinea grass (Panicum maximum) monoculture; Zuri guinea grass (Panicum maximum) monoculture; Pigeon pea (Cajanus cajan cv. BRS Mandarim) monoculture; maize + Tamani guinea grass + Pigeon pea; maize + Quênia guinea grass + Pigeon pea and maize + Zuri guinea grass + Pigeon pea. The maize hybrid used was P4285.
Figure 2 shows the layout of the treatments and the dimensions of the plots. For the monoculture, the crops were sown at 0.90 m between rows, with an area of 21.6 m2 per plot (Figures 2a–c). In the intercropping, the maize was sown at 0.90 m and the forage and leguminous plants were sown between the rows at 0.30 m from the maize row, 3 cm deep, with an area of 21.6 m2 per plot as shown in Figure 2d. For crop ensiling, three mini silos were created per plot/treatments.
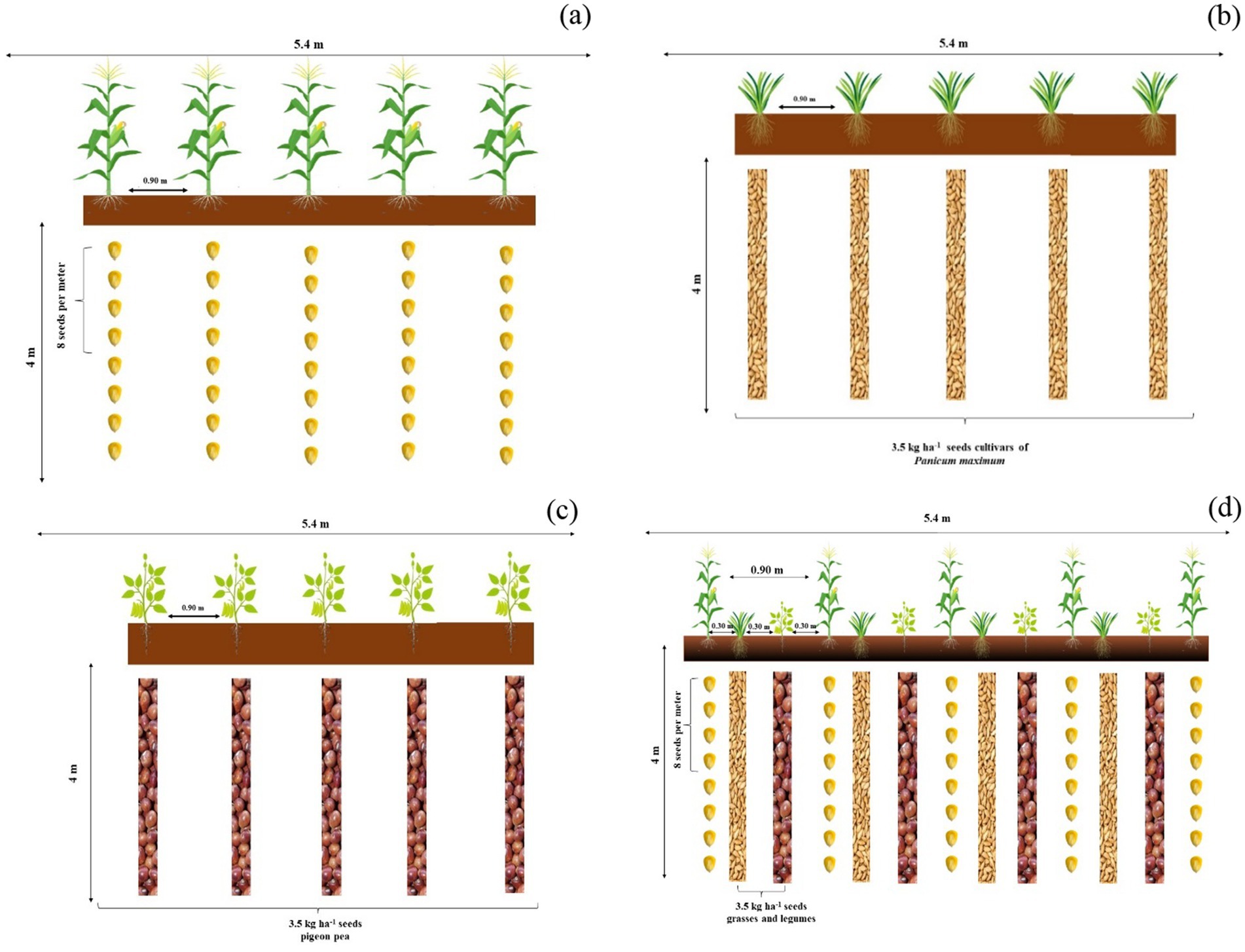
Figure 2. Layout of treatments and plot dimensions for maize in monoculture (a), cultivars of Panicum maximum in monoculture (b), Pigeon pea in monoculture (c) and maize intercropped with cultivars of Panicum and Pigeon pea (d).
All crops (monoculture and intercropping) were sown manually in the second agricultural season, on 28 February 2022, with 150 kg ha−1 of P2O5 and 20 kg ha−1 of FTE BR 12 (9% Zn; 1.8%) boron (B); 0.8% Cu; 2% manganese (Mn); 3.5% Fe and 0.1% molybdenum (Mo) applied in the planting furrow, using the sources simple superphosphate and Fritas, respectively. Eight maize seeds were used per meter and 3.5 kg seeds were used per hectare for the grasses and legumes for all cropping systems (monoculture and intercropping).
When maize plants were at the stage of three and six fully developed leaves, two top dressings were applied, in soil, with a total of 150 kg ha−1 and 80 kg ha−1 of N and K2O in urea and potassium chloride sources, respectively, the same amount of urea and potassium chloride was applied to the grass monocrops. For the intercropped systems, only half the dose of nitrogen was applied, with 75 kg ha−1 of nitrogen, in order to make use of the nitrogen through biological fixation by the Pigeon pea, and 80 kg ha−1 of K2O. As for the Pigeon pea in monoculture, only potassium fertilization of 80 kg ha−1 of K2O was used.
To control Spodoptera frugiperda and Dalbulus maidis, applications were made of the insecticides Klorpan (active ingredient Chlorpyrifos) and Connect (active ingredient Beta-cyfluthrin and Imidacloprid), at a rate of 0.4 litres ha−1 and 0.1 litres ha−1 of commercial product, respectively. Both applications were carried out manually with a knapsack sprayer.
2.3 Crop silage
The crops were harvested for silage on 4 June 2022 at 97 days after sowing (DAS), when the maize was in the pasty stage, with 332 g kg−1 of dry matter (DM). To assess dry mass production and the proportion of ensiled material (Table 1), the crops were cut separately in the field and weighed to determine the proportion of maize, forage, and leguminous plants. Part of the material was then placed in an oven at 55°C for 72 h until it reached a constant mass, for later determination of dry weight and conversion to kg ha−1.
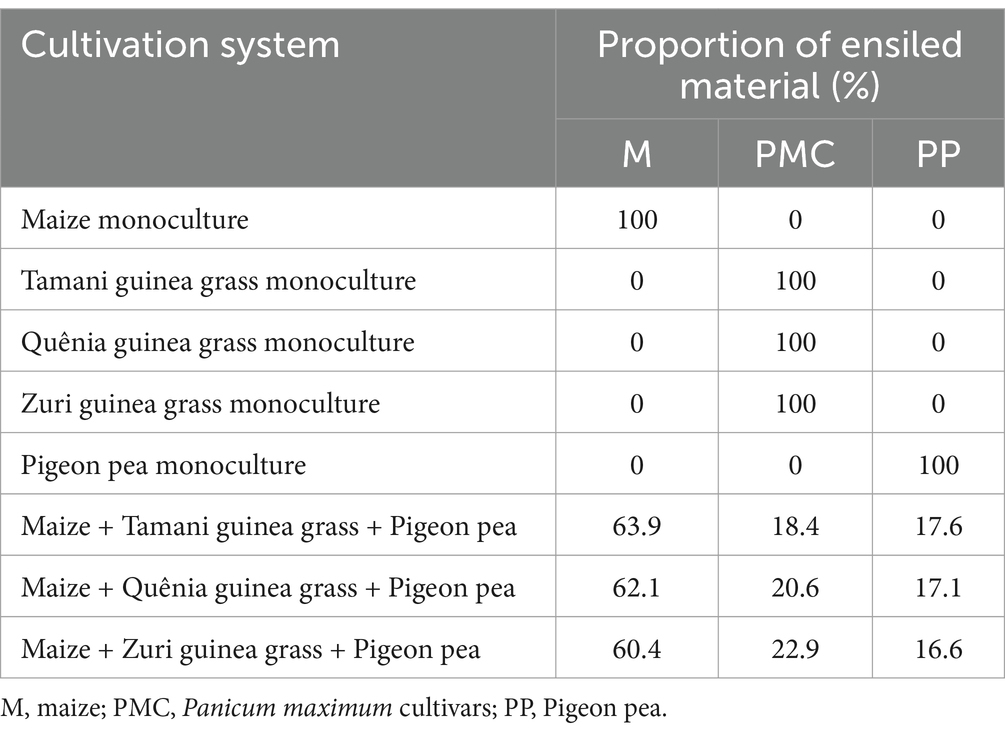
Table 1. Proportion of material ensiled from maize intercropped with Panicum maximum cultivars and Pigeon pea.
The material was ground into particles of approximately 10 mm in a forage grinder to make silage and was subsequently compacted and stored in experimental PVC silos measuring 10 cm in diameter and 40 cm in length. The silos were stored at room temperature and protected from rain and sunlight.
Bromatological analyses were carried out on the raw material (before ensiling) (Table 2) to determine dry matter (DM), crude protein (CP), lignin, ether extract (EE) and mineral matter (MM), according to the method described by AOAC (1990). Neutral detergent fiber (NDF) and acid detergent fiber (ADF) were determined according to the method described by Mertens (2002). Total digestible nutrients (TDN) were obtained using the equation, proposed by Chandler (1990). To determine the in vitro dry matter digestibility (IVDMD), the technique described by Tilley and Terry (1963) was used, adapted to the artificial rumen, developed by ANKON®, using the “Daisy incubator” instrument from Ankom Technology (in vitro true digestibility—IVTD). The rumen fluid collection was conducted via a ruminal cannula, in dairy cattle fed on pasture and corn silage, in the morning, 3 h after the animal’s feeding. The initial study was approved by the Research Ethics Committee (REC) of the Federal Goiano Institute, under protocol 53,752,405–16.
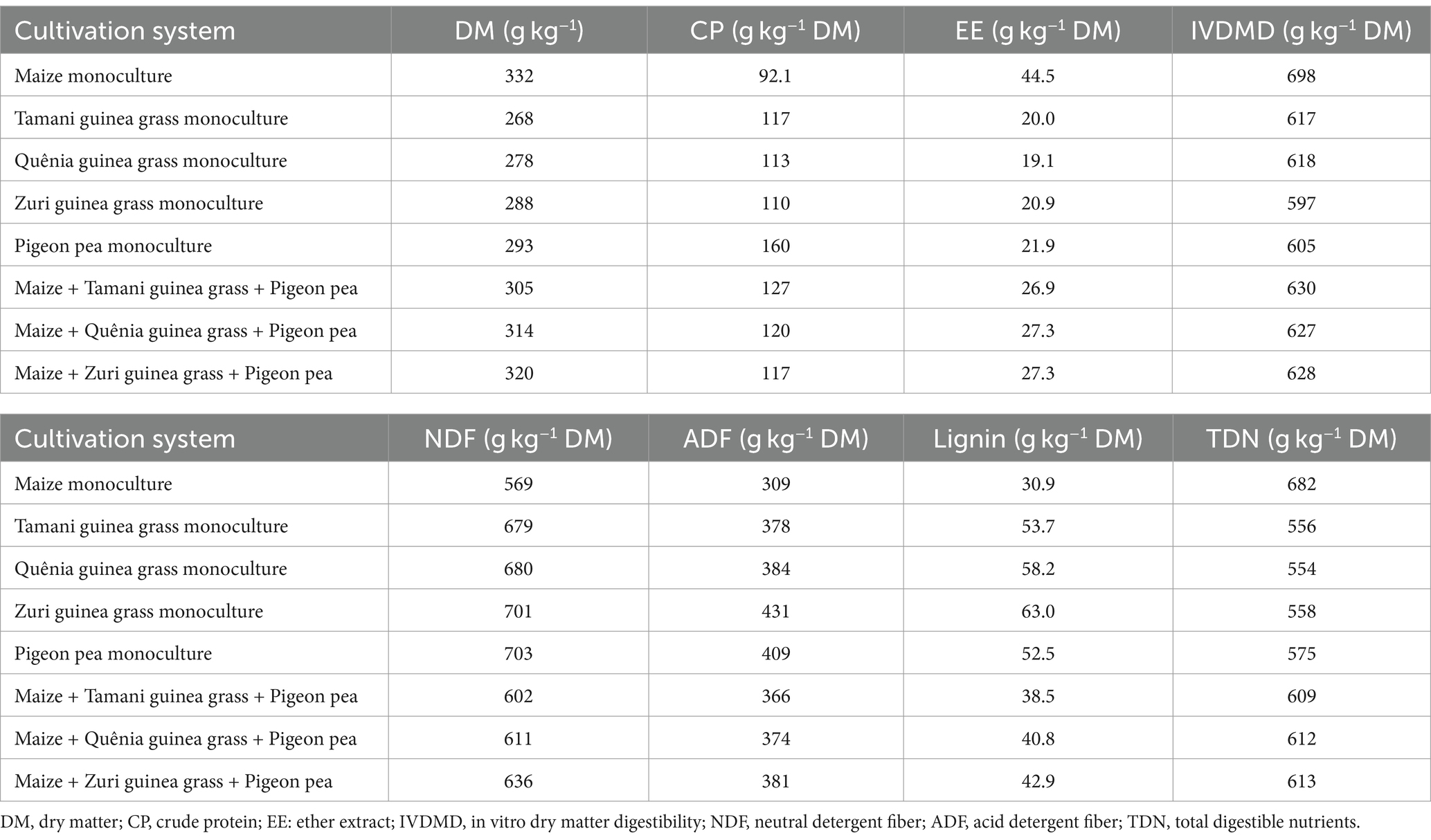
Table 2. Bromatological characteristics of maize, Panicum maximum cultivars and Pigeon pea in monoculture and intercropping before ensiling.
2.4 Opening the silos and analyzing the fermentation and bromatological characteristics of the silage
The silos were opened after 50 days of fermentation, discarding the top and bottom portions of each one. The central portion of the silo was homogenized and placed in a plastic tray. Part of the in natural silage was separated for analysis of fermentation characteristics: buffering capacity, pH and ammoniacal nitrogen in total nitrogen (N-NH3/NT), following the method described by Silva et al. (2022).
The pH and buffering capacity analyses were performed when the silos were opened to avoid changes in the expected values due to heat and humidity. For the determination of ammoniacal nitrogen, the silage was frozen in order to inactivate the activity of anaerobic bacteria, thus avoiding nitrogen volatilization, and the analysis was carried out later.
Total loss dry matter (TLDM) and effluent production were determined according to the methodology proposed by Jobim et al. (2007). Organic acids were determined using a high-performance liquid chromatograph (HPLC), according to the method described by Kung and Shaver (2001), for the determination of lactic and acetic acid.
The other portion of the material (approximately 0.5 kg) was weighed and dried in a forced ventilation oven at 55°C for 72 h. The samples were then ground in a Wiley-type knife mill with a 1 mm sieve and stored in plastic containers. Subsequently, the chemical-bromatological characteristics of the silage described above for the in natural material were analyzed.
2.5 Statistical analysis
The variables were subjected to analysis of variance using the R program version R-3.1.1 (R Core Team, 2014), using the ExpDes package (Ferreira et al., 2014). The means were compared using the Tukey test at 5% probability.
To understand the cause and effect relationship between the variables, Pearson’s correlation analysis (low: r ≤ 0.30; moderate: 0.30 < r ≤ 0.70 and high: r ˃ 0.70) and path analysis were carried out, with pH and IVDMD being considered as dependent variables due to their nutritional importance. To define the causal diagram, multiple linear regression analysis was carried out using the “Stepwise” procedure with the “backward” option (Coimbra et al., 2005; Charnet et al., 2008). Subsequently, multicollinearity was diagnosed based on the condition factor (ratio between the largest and smallest eigenvalues), with a number of conditions (NC) < 100, indicating that multicollinearity is considered weak and does not constitute a problem for the analysis (Cruz et al., 2014).
The contributions of the direct and indirect effects of the variables were quantified as a percentage. Contributions above 70% were considered to have a high direct effect (Botelho et al., 2019; Ribeiro et al., 2019). The “corrplot,” “lavaan” and “semPlot” packages of the R Development computer program were used for the statistical analyses.
3 Results
The cropping systems influenced significantly (p < 0.05) the production of dry mass for ensiling the crops (Figure 3). The highest production was obtained in intercropping systems, with an average increase of 37.8% in production compared to maize in monoculture. The Panicum maximum cultivars and Pigeon pea grown in monoculture had lower silage mass production.
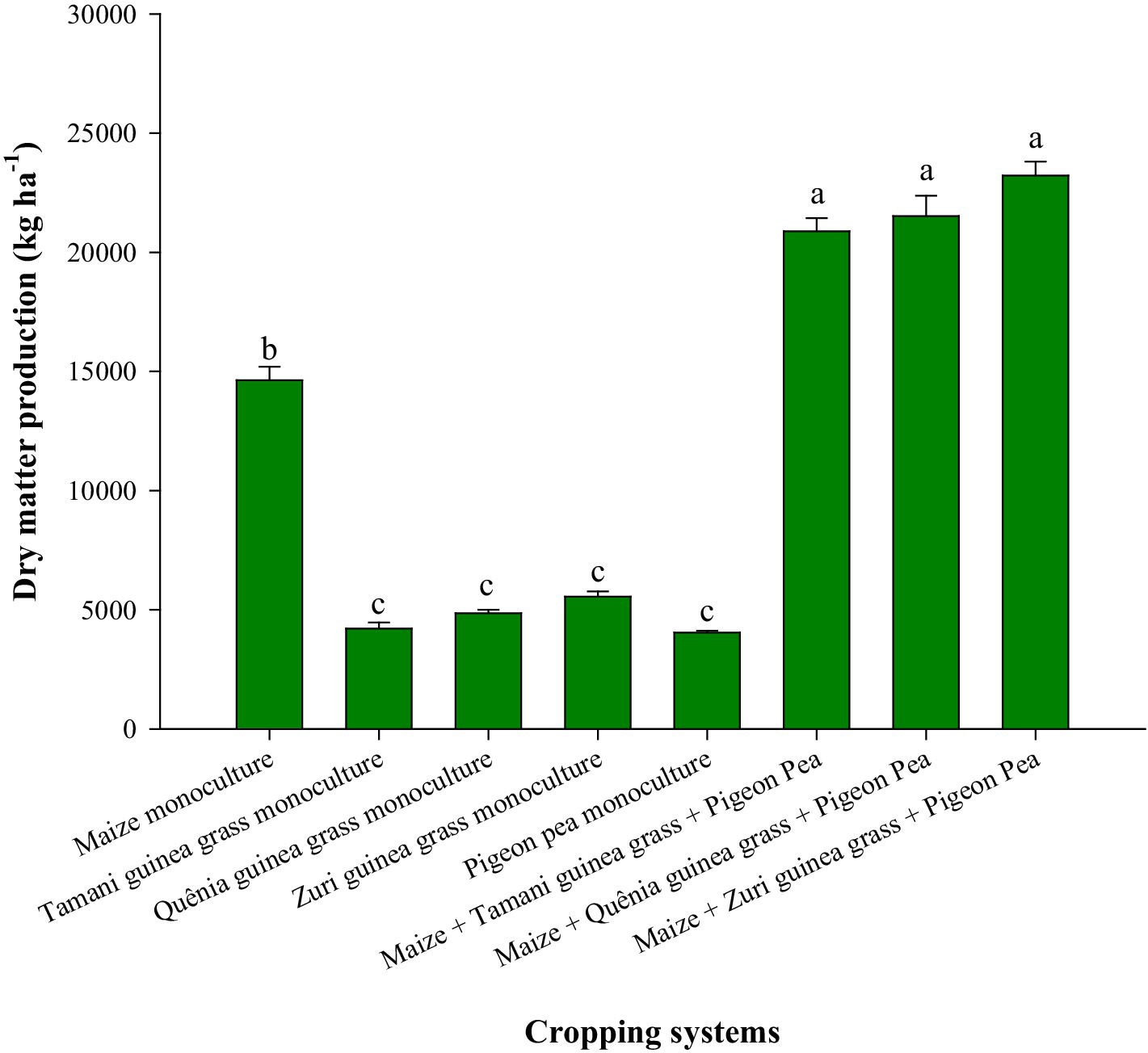
Figure 3. Dry matter production of maize silage, Panicum maximum cultivars and Pigeon peas in monoculture and intercropping. Means followed by different letters, differ by Tukey’s test (p < 0.05). The vertical bars represent the standard error of the mean.
There was a significant effect (p < 0.05) on the fermentation characteristics of the silages in relation to the cropping systems (Table 3). The highest pH, buffering capacity and N-NH3 were obtained in Pigeon pea silage, followed by silage from Panicum maximum cultivars, which showed similar results. Silage from the triple intercropping contributed to a 5.10% reduction in pH, 32.5% in buffering capacity and 28.9% in N-NH3 compared to silage from Panicum maximum cultivars and Pigeon pea in monoculture. The lowest values for these variables were observed in maize silage in monoculture. For the DM content of the crops from the different cultivation systems (Table 3), maize silage had the highest value, followed by silage from the triple intercropping. Relation to the forage plants, there was no significant effect on the DM content of the silages of Quênia guinea grass, Zuri guinea grass and Pigeon pea in monoculture. Tamani guinea grass silage in monoculture had the lowest DM content.
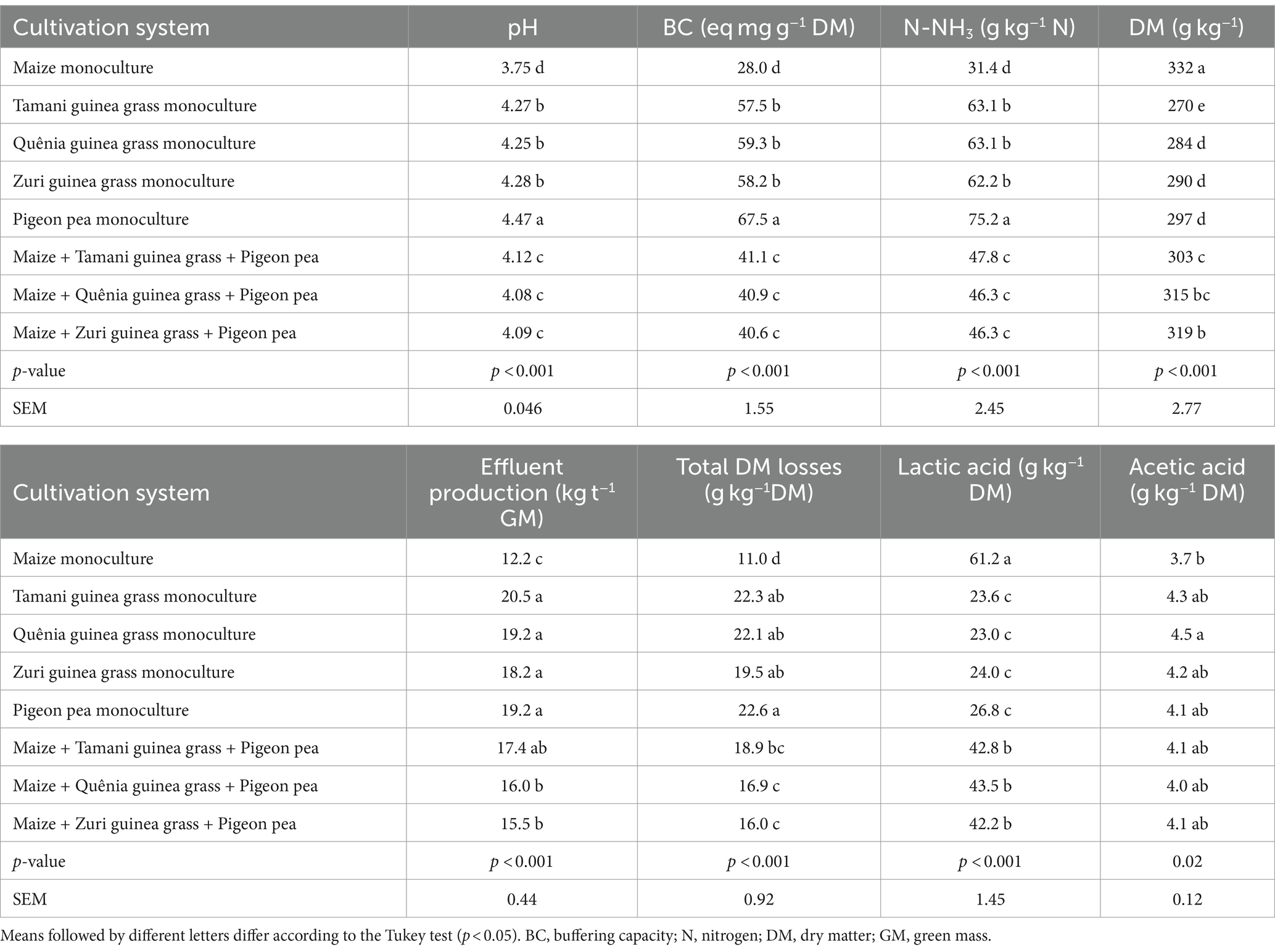
Table 3. pH, buffering capacity, N-NH3, DM, effluent production, total DM losses, lactic acid and acetic acid from maize silage, Panicum maximum cultivars and Pigeon pea in monoculture and intercropping.
Effluent production and total DM losses of the silages were also influenced (p < 0.05) by the different cropping systems (Table 3). The highest effluent production and DM losses were observed in the silages of Pigeon pea and Panicum maximum cultivars in monoculture. A 15.7% reduction in effluent production and a 20.0% reduction in DM losses were observed for silage from the consortium compared to silage from Panicum maximum cultivars and Pigeon pea grown in monoculture. For lactic acid, the highest values were observed for maize silage in monoculture, followed by the intercropping system, which proved to be efficient in increasing lactic acid production by 43.1% compared to silage from Panicum maximum cultivars and Pigeon pea, which showed the lowest values. As far as acetic acid is concerned, only monoculture Quênia guinea grass silage differed from monoculture maize silage.
The highest CP (Table 4) content were obtained in monoculture Pigeon pea silage, followed by triple intercropping silage, which provided a 25.3% increase over monoculture maize silage. Intermediate CP values were observed for the silage of Panicum maximum cultivars, especially Tamani guinea grass. On the other hand, maize silage in monoculture had the lowest value. For MM, the highest values were observed for silage from Panicum maximum cultivars, followed by silage from Pigeon pea in monoculture. There was a 27.5% reduction in MM content for silage from the intercropped system compared to silage from Panicum maximum cultivars and monoculture Pigeon pea. Maize silage had a higher EE content. The silage from the intercropping system was effective in increasing EE content by 25.0% compared to the silage from tropical forage crops grown in monoculture. The benefits of the integrated system were also observed for the IVDMD of the silages. The silage from the consortium increased digestibility by 4.90% compared to the silage from Zuri guinea grass and Pigeon pea in monoculture, which had the lowest values.
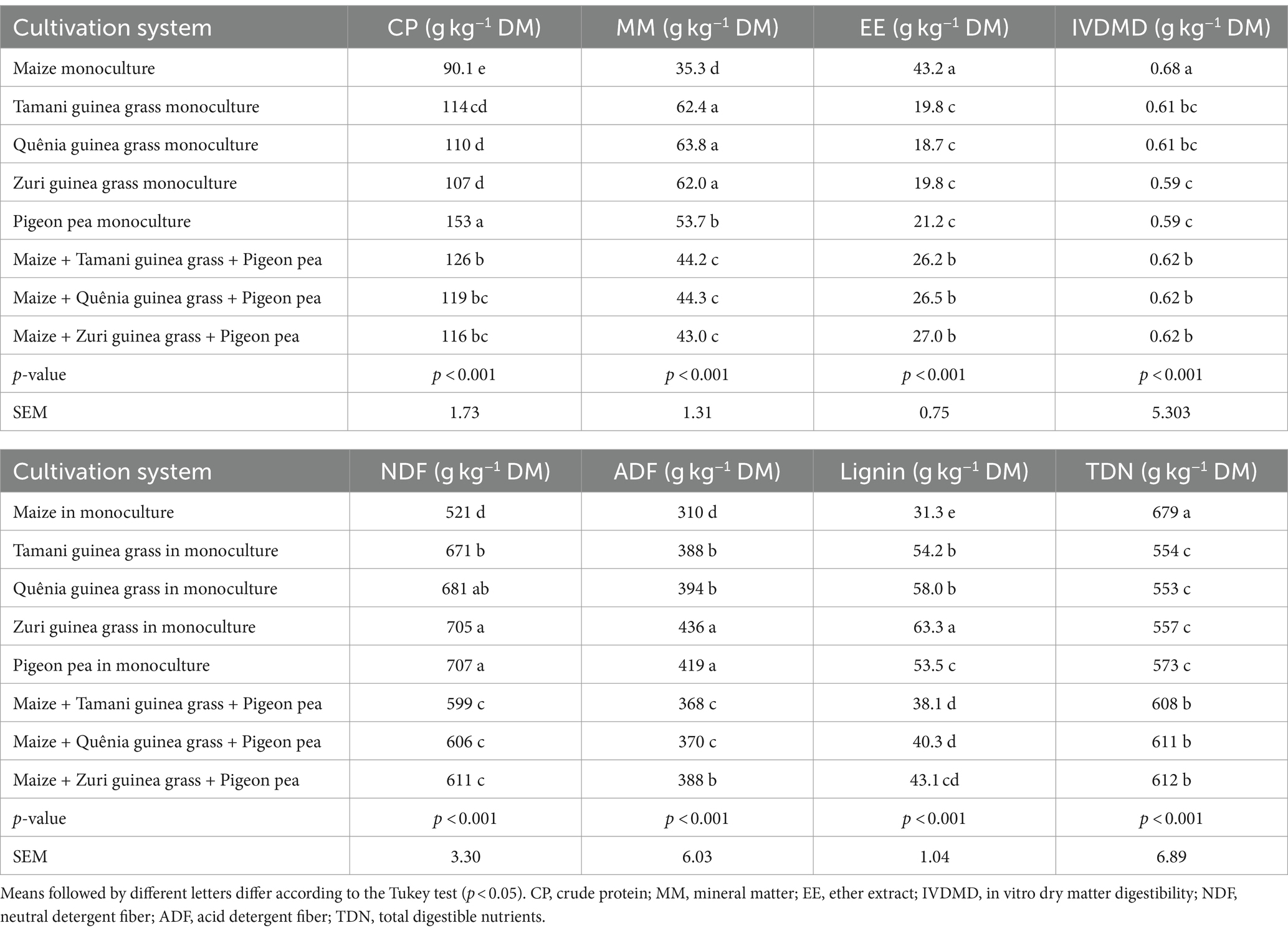
Table 4. Contents of crude protein (CP), mineral matter (MM), ether extract (EE), in vitro dry matter digestibility (IVDMD), neutral detergent fiber (NDF), acid detergent fiber (ADF), lignin and total digestible nutrients (TDN) (g kg−1 DM) of maize silage, Panicum maximum cultivars and Pigeon pea in monoculture and intercropping.
One of the great qualities of maize silage is its low fibrous fraction content and higher TDN content, as shown in the current study. Maize silage in monoculture had the lowest levels of NDF, ADF and lignin, followed by the triple intercropping. Among the forage plants, Tamani and Quênia guinea grasses in monoculture had the lowest values. Zuri guinea grass and Pigeon pea in monoculture had higher fibrous fractions. For TDN, maize silage had the highest content. The silage from the consortium contributed an increase of 8.32% compared to the silage from tropical forage crops in monoculture, which had the lowest TDN levels (Table 4).
The correlation analysis (Figure 4) showed that two groups of variables were formed, with group 1 consisting of: TDN, DM, lactic acid, EE and IVDMD. Group 2 was made up of: lignin, acetic acid, NDF, MM, ADF, effluent production, N-NH3, DM losses, buffering capacity, pH and CP. The variables within the same group showed positive correlations, and between the different groups negative correlations. Only CP showed a non-significant correlation with lignin, acetic acid, NDF and MM.
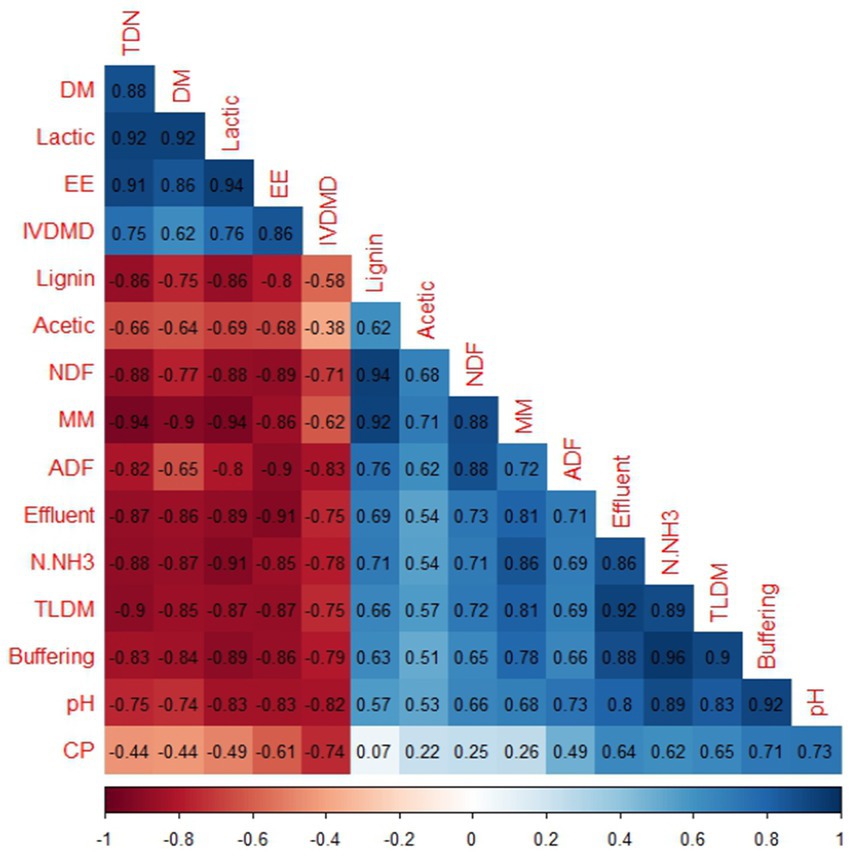
Figure 4. Pearson’s correlation between fermentation profile variables and the nutritional value of maize silage, Panicum maximum cultivars and Pigeon pea in monoculture and intercropping. Acetic: acetic acid; ADF: acid detergent fiber; Buffering: buffering capacity; CP: crude protein; DM: dry matter; EE: ether extract; Effluent: effluent production; IVDMD: in vitro dry matter digestibility; Lignin: lignin; Lactic: lactic acid; MM: mineral matter; NDF: neutral detergent fiber; N.NH3: ammoniacal nitrogen; pH; TDN: total digestible nutrients; TLDM: total loss dry matter.
The causal diagram obtained by the multiple regression analysis, using the “Stepwise” procedure with the “backward” option, showed that the variables buffering, effluent, NDF, ADF, lignin and EE were kept in the model to explain pH, with significance for all of them and with a model determination coefficient of 0.96 (Table 5). The model to explain IVDMD showed a coefficient of determination of 0.91, consisting of the variables N.NH3, DM, effluent, acetic, CP and EE, both of which were significant in the model. In the pH causal diagram (Figure 5), the correlation showed that Buffering, ADF and NDF had positive direct effects with a contribution above 50% of the correlation coefficient (Table 5). The other variables had a low direct effect on pH. The causal diagram for IVDMD (Figure 6) showed that EE had a high direct effect, and the other variables had a low effect (Table 5).
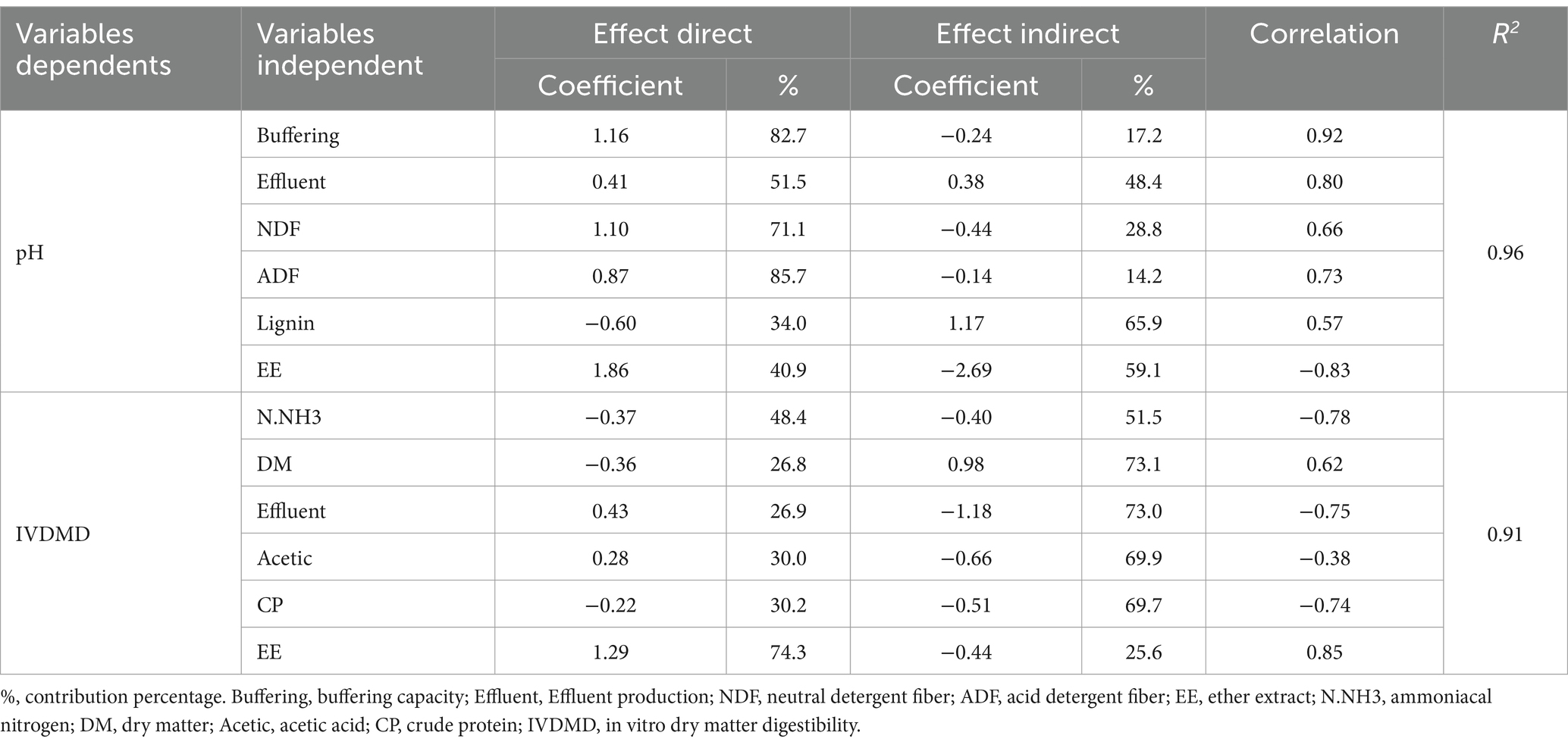
Table 5. Direct and indirect effects, correlation and coefficient of determination (R2) of the causal models.
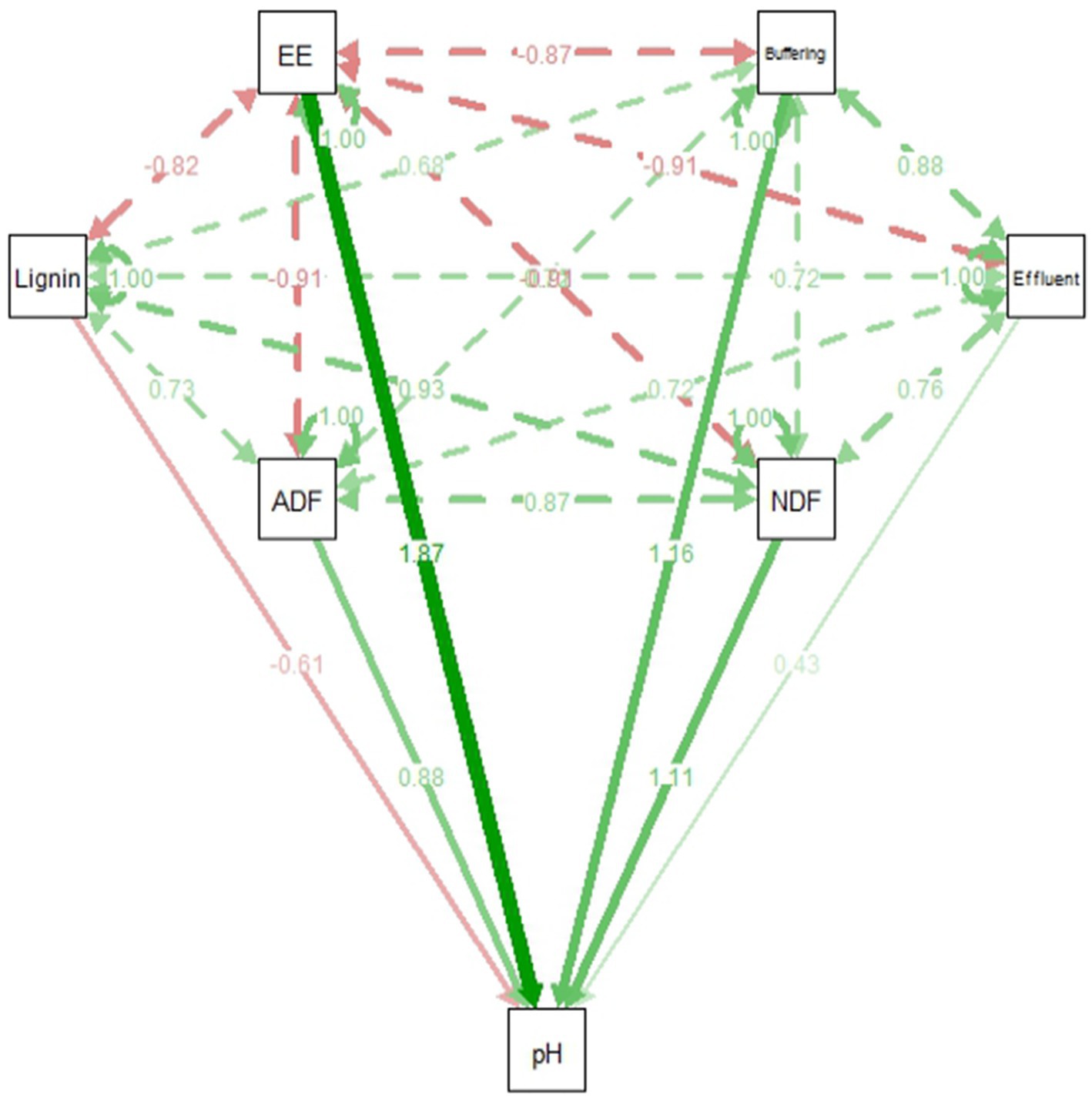
Figure 5. Causal diagram showing the correlations between the independent variables and the cause-effect relationships with pH. ADF: acid detergent fiber; Buffering: buffering capacity; EE: ether extract; Effluent: effluent production; Lignin; NDF: neutral detergent fiber.
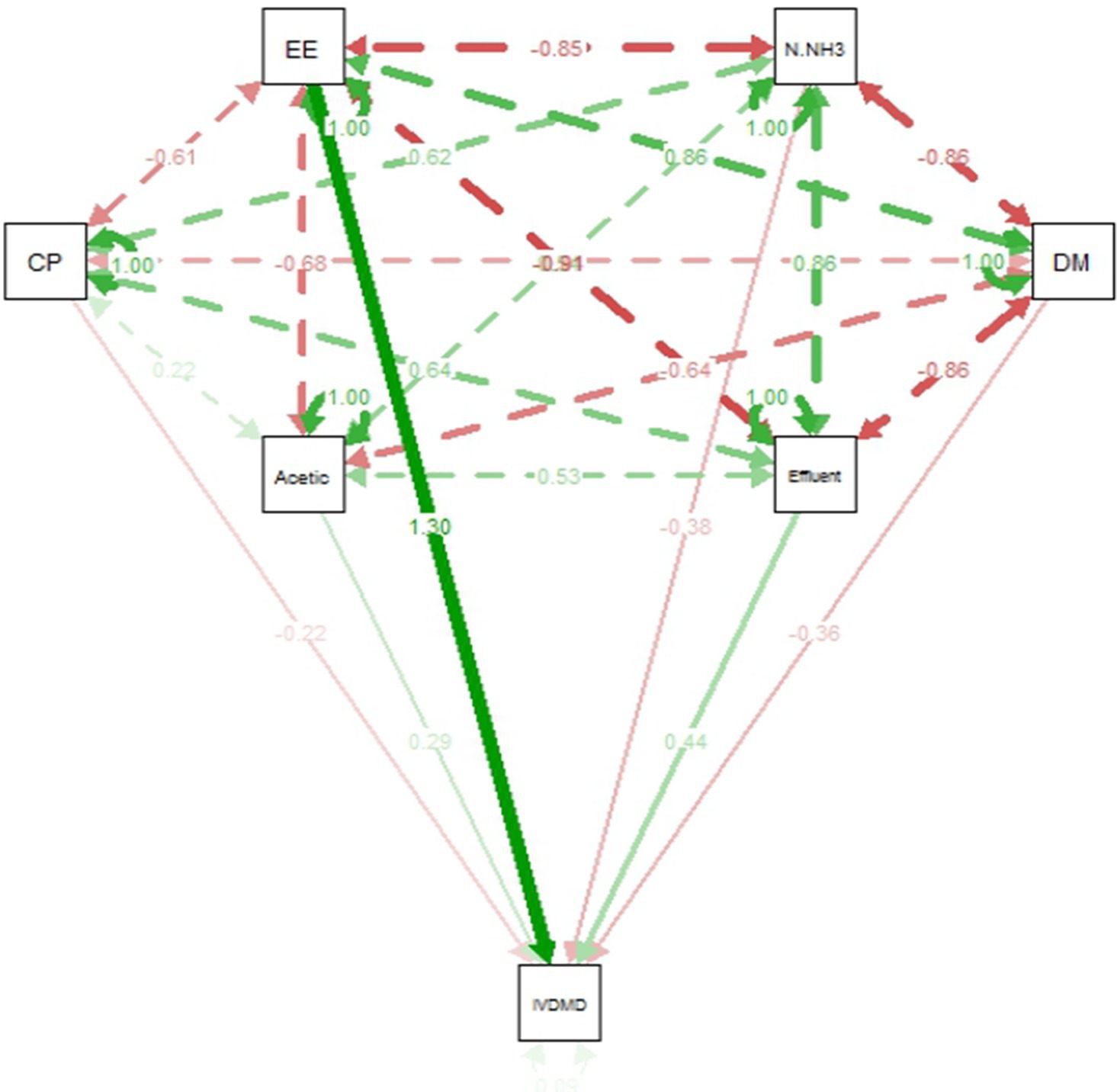
Figure 6. Causal diagram showing the correlations between the independent variables and the cause-effect relationships with IVDMD. Acetic: acetic acid; CP: crude protein; DM: dry matter; EE: ether extract; Effluent: effluent production; IVDMD: in vitro dry matter digestibility; N-NH3: ammoniacal nitrogen.
4 Discussion
A great advantages of silage production in integrated systems is the increase in silage mass production with the addition of forage crops to the system (Souza et al., 2019; Oliveira et al., 2020; Prado et al., 2023). In this context, the current study found that the higher dry mass production of intercropped systems than that of monoculture systems is because when the three crops are grown simultaneously, the area is put to better use resulting in a higher silage yield. Vicente et al. (2023) also observed greater forage production in the triple consortium due to the complementarity of the three crops grown simultaneously. In view of this result, it is worth emphasizing the importance of an integrated system of annual crops and tropical forage plants (grasses and legumes) must be emphasized, to increase the yield of dry mass for silage, compared to that from monoculture crop systems (Ligoski et al., 2020). In addition, there is the contribution of the legume in the system, with the possibility of reducing the application of nitrogen, as this, through biological fixation (Epifanio et al., 2019b), increases the supply of this macronutrient in the soil, thus contributing to the sustainability of the cultivation system and directly influencing the reduction of nitrogen fertilizer inputs (Bolson et al., 2022).
Evaluated the biomass production of maize for silage in a consortium with different populations of Brachiaria and dwarf Pigeon pea, Guimarães et al. (2017) concluded that while integration did not affect maize productivity, Pigeon pea increased the production of fresh and dry mass as plant density increased. In a recent study of silage integrated systems (triple intercropping of sorghum + Tamani guinea grass + Stylosantes Bela), Prado et al. (2023) verified the consortium systems promoted a 36.2% increase in silage mass production compared to that of the monoculture sorghum, confirming the benefits of better use of the area, which resulted in higher silage mass production.
Another advantage of silage production in integrated systems is that it provides pasture after the crops have been harvested for silage production, through the regrowth of forage plants, improving the efficiency of land use in a sustainable and economically viable way (Oliveira et al., 2020), given that the formation or recovery of pastures is costly Souza et al. (2019), making it unfeasible for most producers to adopt this practice. Integrated systems is a promising and efficient yield strategy in the global reality of agricultural and livestock production to boost production systems, contributing to improving sustainable feed production (Boote et al., 2022), can be considered an investment for future food self-sufficiency of the population (Rufino et al., 2021). The cultivation of intercropped crops is geared towards high silage production with improved nutritional quality (Ligoski et al., 2020) as well as helping to maintain sustainable agroecosystems and provide economic (Costa et al., 2016), environmental (Carvalho et al., 2016; Cruz et al., 2020; Dias et al., 2021) and social benefits (Herrera et al., 2023).
Silages from intercropping systems also positively influenced the fermentation and nutritional characteristics of silages. The triple intercropping of crops reduced the pH values by 5.10%, buffering capacity by 32.5%, and N-NH3 by 28.9% compared with those of monoculture silage from Panicum maximum cultivars and Pigeon pea. These results are relevant because these characteristics have a direct influence on fermentation, and consequently, silage quality (Oliveira et al., 2020).
The higher pH observed in monoculture Pigeon pea silage, followed by monoculture silage from Panicum maximum cultivars, can be attributed to the greater buffering capacity of legumes and grasses than those of maize, in addition to the low soluble carbohydrate and DM contents, resulting in a slow reduction of silage pH (Bao et al., 2022). pH values between 4.3 and 4.7, observed mainly in legume and grass silages, can promote the growth of proteolytic and heterofermentative microorganisms, which is undesirable in the fermentation process and influences the final quality of food (Kung et al., 2018). The pH values of monoculture and consortium maize were within an adequate range (between 3.7 and 4.2) for classifying good quality silage (Mcdonald et al., 1991). These results demonstrate the importance of producing silage in a consortium system to reduce the pH of silage made exclusively from grasses and legumes and guarantee adequate conservation of the ensiled material.
The higher buffering capacity of monoculture Pigeon pea silage can be explained by the presence of cations such as calcium (Ca2+), magnesium (Mg2+), and potassium (K+), which neutralize the organic acids produced during fermentation, making it difficult for the pH of the silage to decrease (Hawu et al., 2022). Silages from exclusive grasses also have a high buffering capacity compared to maize silage, resulting in poorly preserved forage due to inadequate fermentation (Teixeira et al., 2021).
Owing to the higher proportion of maize in the triple intercropping silage (Table 1), the buffering capacity and N-NH3 levels reduced compared to those of the silages from monoculture Panicum maximum cultivars or Pigeon pea. These results indicate that fermentation occurred properly, providing good quality silage, similar to the results observed by Souza et al. (2019) who evaluated the quality of silage from a double maize consortium with Paiaguas palisade grass. According to Kung et al. (2018), good-quality silage should have N-NH3 values below 100 g kg−1; the results of the current study for these systems were below the recommended limit, confirming the importance of producing silage from the consortium. A main factor that should be considered when determining the ideal time to cut silage is DM content, as this directly affects the fermentation process and final quality of the silage produced (Borreani et al., 2018).
Muck and Shinners (2001), stated that silages with contents lower than 300 g kg−1 DM tend to have higher effluent losses and an increase in the activity of bacteria of the Clostridium genus, resulting in low-quality silage, which is poorly accepted by animals. Results lower than those established by the aforementioned authors were observed for silage from monoculture Panicum maximum cultivars and Pigeon pea in monoculture. These suboptimal DM contents also have a negative influence on higher effluent production and total DM losses. This is due to the high humidity, low concentration of soluble carbohydrates, and high water activity, which can promote secondary fermentation, responsible for DM losses (Borreani et al., 2018). This combination of factors negatively affects the final quality of the silage (Bao et al., 2022; Silva L. M. et al., 2023) because it favors the leaching of nutrients to the bottom of the silo, resulting in the loss of nutrients from the ensiled material (Queiroz et al., 2021). In addition, the liquid produced can seep into the soil, negatively altering the natural microbiota and contributing to the emission of greenhouse gases such as nitrous oxide as it raises nitrogen levels in the soil (Araújo et al., 2020). Despite this, the losses due to effluent in the present study were less than 100 kg t−1 of forage, which can be considered within normal limits, according to Araujo et al. (2023).
Silage is the result of a microbial fermentation process in which lactic acid is considered the most effective way to quickly reduce the pH to below 4.20, while preserving the nutritional quality of the silage mass (Oliveira et al., 2021). In this context, the silage from the consortium in the current study was efficient in increasing the production of lactic acid by 43.15% compared to that from the silage from tropical forage crops in monocrops (Table 3), demonstrating that during fermentation, there was greater activity of Lactobacillus bacteria, co-producers of lactic acid, increases, ensuring a rapid drop in pH and consequent preservation of the ensiled material adequately (Meng et al., 2022), improves fermentation and the final quality of the silage.
Maize silage with legumes production aims to increase the CP content of maize silage, thereby improving the final quality of the feed provided to animals (Silva L. M. et al., 2023). In the present study, it was possible to observe that the silage from the intercropped system increased crude protein content by 25.1% compared to maize silage in monoculture (Table 4). This increase was mainly because the presence of Pigeon pea and Panicum maximum cultivars in the system, demonstrating that the use of silage through the triple intercropping in integrated food production systems allows for reduced protein supply in the diet and, consequently, feeding cost (Ribeiro et al., 2017; Gomes et al., 2021).
Corroborating these results, Edson et al. (2018) found that double consortium maize-legume silage (70,30) increased milk production and quality compared to that by maize silage alone and can thus alleviate protein-energy malnutrition in smallholder dairy products. The cost of producing a liter of milk was significantly reduced, while higher gross margins were obtained when legume silage was used compared to commercial dairy meals, demonstrating the viability of dairy businesses owing to the low cost of production. Vicente et al. (2023) observed a 22% increase in the crude protein content of the silage from the consortium system of Sorghum + Urochloa + Cajanus compared to the silage from monoculture Sorghum. In addition, the inclusion of Pigeon pea in the intercropping system meets the nutritional requirements of the animals, ensuring lower mineral supplement intake, greater daily weight gain, lower methane emissions (Furtado et al., 2023), than those from the monocropping system. We emphasize Pigeon pea contains 13.6% crude protein in the plant and 23.6% en the leaves (Oliveira et al., 2017).
For MM, the highest values were observed for silage from Panicum maximum cultivars, followed by Pigeon pea, in monoculture plants. Tropical forages (grasses and legumes) contain considerable amounts of minerals, such as calcium, potassium, and phosphorus (Oliveira et al., 2020; Hawu et al., 2022), which can be 2 to 3 times more than that of grasses. Cereals have low MM content owing to their genetic capacity (Paula et al., 2016). This result was verified in the present study, in which maize had low MM content. Bessa et al. (2018) evaluated a consortium of maize, Paiaguas palisade grass, and Pigeon pea for silage production and pasture development in the off-season and observed that the triple intercropping maintained MM levels. Ligoski et al. (2020) observed that a higher proportion of forage crops in silage increased the MM levels.
Among the monocrops, maize silage had the highest EE content. This result is associated with the higher amount of lipids contained in maize grains (Teixeira et al., 2021). However, silage from the intercropped system was effective in increasing the EE content by 25.0% compared to silage from tropical forage crops grown in monoculture systems. Thus, the results demonstrate the advantage of silage from an integrated system, given that silage from tropical forage crops has a low amount of lipids, influencing the energy content of the food (Souza et al., 2019).
Silages produced from a consortium system also proved to be effective in increasing the IVDMD compared to those of tropical forage silages in monoculture systems (Table 4), in particular, compared to those of the silages of monoculture Zuri guinea grass and Pigeon pea, which showed the lowest values. Lower digestibility can be explained by the higher concentration of fibrous materials (NDF, ADF, and lignin) in these forages, resulting in lower IVDMD (Pereira et al., 2019; Ali et al., 2022).
According to Tang et al. (2018), NDF (hemicellulose, cellulose, and lignin) and ADF (cellulose and lignin) are important quality characteristics of forage, and this quality is conditioned by low levels of these fibrous fractions in the ensiled material. Thus, the significant reduction in the levels of NDF, ADF, and lignin (Table 4) in the silage from the consortium compared to those in the monoculture Panicum maximum cultivars shows that the use of maize and Pigeon pea in combination with the three cultivars improves the quality of the silage produced in this system.
Similar behavior was observed by Souza et al. (2019), who found lower levels of NDF and ADF in double consortium systems with maize than in monoculture Paiaguas palisade grass, thus evidencing the occurrence of dilution of the fibrous fraction. Hedayati-Firoozabadi et al. (2020) also verified that a double consortium of sorghum (Sorghum bicolor) with kochia (Bassia indica) improved forage quality by reducing NDF and ADF content. This reduction in silage fiber will consequently lead to an increase in DM intake and digestibility, as fiber concentrations are negatively associated with DM intake and digestibility (Erdal et al., 2016).
The energy content of food is represented by TDN (Marques et al., 2019). In the current study, silage from the intercropped system was more efficient than silage from Panicum maximum cultivars and Pigeon pea in monoculture silage in increasing the TDN content because of the higher proportion of maize present in these silages (Table 1). Notably, TDN and CP are crucial variables for feeding ruminants and can also be the most limiting (Daniel et al., 2019).
Positive correlations between variables in the same group show that these variables are directly related; that is, an increase in one variable causes an increase in the other, either through a direct effect or an indirect effect via other variables. Negative correlations between variables in different groups indicate that they are inversely related; that is, an increase in one variable causes a reduction in the other and vice versa. These results help us better understand the relationship between the fermentation profile and the nutritional value of silage, as observed by Zeng et al. (2022).
The regression models obtained for pH and IVDMD showed high coefficients of determination (R2 > 0.90). This shows that the independent variables used in the models are important for determining pH and IVDMD. Regression models for bromatological variables are recommended if R2 is greater than 0.70 (Silva L. M. et al., 2023). Studies using path analysis with R2 lower than 0.7 have already been successfully reported in the literature, supporting the use of this analysis in silage evaluation (Ligoski et al., 2020).
The high direct effects on Buffering capacity, ADF and NDF in the pH causal diagram indicate that these variables are only weakly influenced indirectly by other variables in their correlations with pH. However, only buffering capacity and ADF have high and significant correlations with pH. Silage stability is strongly influenced by the buffering capacity of the materials that make up the silage (Hawu et al., 2022). Zeng et al. (2022) observed that ADF was undegradable during ensiling. Thus, the variables that had the greatest effect on pH were Buffering capacity and ADF. The low direct effect and high correlation of Effluent loss and EE with pH indicate that their effects occur indirectly through other variables in the model and that their use is of little importance in determining the effects of independent variables on pH.
The strong direct effect and correlation between EE and IVDMD indicate that EE has the greatest effect on IVDMD. EE represents the energy portion of the feed (Bueno et al., 2020) and, together with CP, contributes to animal feed efficiency (Ligoski et al., 2020).
Therefore, the current study supports the hypotheses raised and demonstrates the importance of the crop-livestock integration system for silage production. The intercropping of maize with Panicum maximum cultivars and Pigeon pea is a promising strategy for producing good-quality silage, recovering and/or forming pastures for use in the off-season (Oliveira et al., 2020) as well as later to produce ground cover biomass for the no-till system (Muniz et al., 2021; Silva et al., 2024b), guaranteeing food security, and promoting diversification of production in a sustainable manner (Herrera et al., 2023), with increased production per unit area during the year (Bieluczyk et al., 2020). In addition, it reduces the emission of pollutants such as methane gas (Furtado et al., 2023) and costs by purchasing mineral nitrogen fertilizers (Kutamahufa et al., 2022; Silva et al., 2024a). However, further studies should be conducted/ with other genera of grass and legumes to diversify the production system.
5 Conclusion
Panicum maximum cultivars and Pigeon pea monoculture showed lower dry mass production for silage and a restricted fermentation profile.
Maize silage in monoculture, had better fermentation of silage but a lower crude protein content.
Thus, we confirm that all Panicum cultivars can be recommended for the intercropped with maize and Pigeon pea, to increase the yield of silage mass, balance of the fermentative profile, and increase the crude protein content maize silage in monoculture, allowing for a sustainable intensification of the system of production.
Data availability statement
The original contributions presented in the study are included in the article/supplementary material, further inquiries can be directed to the corresponding author.
Ethics statement
The initial study was approved by the Research Ethics Committee (REC) of the Federal Goiano Institute, under protocol 53752405-16.
Author contributions
LS: Writing – original draft, Writing – review & editing, Conceptualization, Data curation, Investigation, Validation, Visualization. KC: Funding acquisition, Project administration, Supervision, Validation, Visualization, Writing – original draft, Writing – review & editing, Conceptualization, Formal analysis. LP: Writing – original draft, Writing – review & editing, Data curation, Investigation, Validation, Visualization. AR: Writing – original draft, Writing – review & editing, Data curation, Investigation, Validation, Visualization. ES: Writing – original draft, Writing – review & editing, Data curation, Formal analysis, Methodology, Validation, Visualization. JC: Writing – original draft, Writing – review & editing, Data curation, Investigation, Validation, Visualization. JS: Writing – original draft, Writing – review & editing, Data curation, Investigation, Validation, Visualization. AC: Writing – original draft, Writing – review & editing, Data curation, Formal analysis, Validation, Visualization. PF: Writing – original draft, Writing – review & editing, Data curation, Formal analysis, Validation, Visualization. RR: Writing – original draft, Writing – review & editing, Data curation, Formal analysis, Validation, Visualization. BSM: Writing – original draft, Writing – review & editing, Data curation, Investigation, Validation, Visualization. BFM: Writing – original draft, Writing – review & editing, Data curation, Investigation, Validation, Visualization. FR: Writing – original draft, Writing – review & editing, Data curation, Formal analysis, Validation, Visualization.
Funding
The author(s) declare that financial support was received for the research, authorship, and/or publication of this article. This work was supported by the Federal Institute of Goiás (Notice No. 19/2021 - Internal call for support for RD&I projects), the National Council for Scientific and Technological Development (CNPq) and the Coordination for the Improvement of Higher Education Personnel (CAPES), with a doctoral scholarship for Luciana Maria da Silva (process 88887.613180/2021-00). This work was also carried out with the support of the State University of Goiás (UEG), financial resource from Call for Proposals no. 01/2023 (Grant Agreement no. 51958670, SEI process no. 202300020011690).
Acknowledgments
We thank the Instituto Federal Goiano and Finep for supporting this study. The Coordination for the Improvement of Higher Education Personnel (CAPES) and National Council for Scientific and Technological Development (CNPq) for granting the PhD scholarship and research productivity.
Conflict of interest
The authors declare that the research was conducted in the absence of any commercial or financial relationships that could be construed as a potential conflict of interest.
Publisher’s note
All claims expressed in this article are solely those of the authors and do not necessarily represent those of their affiliated organizations, or those of the publisher, the editors and the reviewers. Any product that may be evaluated in this article, or claim that may be made by its manufacturer, is not guaranteed or endorsed by the publisher.
References
Ali, O., Junges, L., Gomes, E. N. O., Batistoti, J. O., Candido, A. R., Gomes, F. K., et al. (2022). Ruminal kinetics and nutritive value of Zuri grass silage harvested at different ages and added with powder molasses. Trop. Anim. Health Prod. 54:231. doi: 10.1007/s11250-022-03241-4
AOAC (1990). Official methods of analysis. Available at: https://law.resource.org/pub/us/cfr/ibr/002/aoac.methods.1.1990.pdf (Accessed November 07, 2023).
Araújo, J. A. S., Almeida, J. C. C., Reis, R. A., Carvalho, C. A. B., and Barbero, R. P. (2020). Harvest period and baking industry residue inclusion on production efficiency and chemical composition of tropical grass silage. J. Clean. Prod. 266:121953. doi: 10.1016/j.jclepro.2020.121953
Araujo, C. M. C., Galeano, E. S. J., Junior, M. A. O., Fernandes, T., Alves, J. P., Retore, M., et al. (2023). Fermentative parameters and chemical composition of mixed silages from corn-crotalaria intercropping. Anim. Feed Sci. Technol. 305:115779. doi: 10.1016/j.anifeedsci.2023.115779
Bao, J., Wang, L., and Yu, Z. (2022). Effects of different moisture levels and additives on the ensiling characteristics and in vitro digestibility of Stylosanthes silage. Animals 12:1555. doi: 10.3390/ani12121555
Bessa, S. V., Backes, C., Santos, A. J. M., Rodrigues, L. M., Teodoro, A. G., Tomazello, D. A., et al. (2018). Maize silage intercropped with grass and pigeon pea subjected to different N rates and pasture development in the offseason. Semina 39, 2501–2516. doi: 10.5433/1679-0359.2018v39n6p2501
Bi, Y., Zhou, B., Ren, P., Chen, X., Zhou, D., Yao, S., et al. (2024). Effects of Bacillus subtilis on cotton physiology and growth under water and salt stress. Agric. Water Manag. 303:109038. doi: 10.1016/j.agwat.2024.109038
Bieluczyk, W., Piccolo, M. C., Pereira, M. G., Moraes, M. T., Soltangheisi, A., Bernardi, A. C. C., et al. (2020). Integrated farming systems influence soil organic matter dynamics in southeastern Brazil. Geoderma 371:114368. doi: 10.1016/j.geoderma.2020.114368
Bolson, D. C., Jacovaci, F. A., Gritti, V. C., Bueno, A. V. I., Daniel, J. L. P., Nussio, L. G., et al. (2022). Intercropped maize-soybean silage: effects on forage yield, fermentation pattern and nutritional composition. Grassl. Sci. 68, 3–12. doi: 10.1111/grs.12323
Boote, K. J., Adesogan, A. T., Balehegn, M., Duncan, A., Muir, J. P., Dubeux, J. C., et al. (2022). Fodder development in sub-Saharan Africa: an introduction. Agron. J. 114, 1–7. doi: 10.1002/agj2.20924
Borreani, G., Tabacco, E., Schmidt, R. J., Holmes, B. J., and Muck, R. E. (2018). Silage review: factors affecting dry matter and quality losses in silages. J. Dairy Sci. 101, 3952–3979. doi: 10.3168/jds.2017-13837
Botelho, H. A., Lago, A. A., Costa, A. L., Costa, A. C., Reis Neto, R. V., Silva, Z., et al. (2019). Application of morphometric measures in estimation of body weight and discrimination of Astyanax lacustris and Astyanax fasciatus. Aquac. Res. 50, 2429–2436. doi: 10.1111/are.14196
Bourscheidt, M. L., Gomes, F. J., Pedreira, C. G., Boote, K. J., Hoogenboom, G., Pereira, D. H., et al. (2023). Highlighting the benefits of biological nitrogen fixation on agronomic, physiological, and nutritive value traits of Brachiaria grass. Eur. J. Agron. 143:126730. doi: 10.1016/j.eja.2022.126730
Bueno, A. V. I., Lazzari, G., Jobim, C. C., and Daniel, J. L. P. (2020). Ensiling total mixed ration for ruminants: a review. Agronomy 10:879. doi: 10.3390/agronomy10060879
Cardoso, M. R. D., Marcuzzo, F. F. N., and Barros, J. R. (2014). Classificação climática de Köppen-Geiger para o Estado de Goiás e o Distrito Federal. Acta Geográfica 8, 40–55. doi: 10.5654/actageo2014.0004.0016
Carvalho, W. G., Costa, K. A. P., Epifanio, P. S., Perim, R. C., Teixeira, D. A. A., and Medeiros, L. T. (2016). Silage quality of corn and sorghum added with forage peanuts. Rev. Caatinga 29, 465–472. doi: 10.1590/1983-21252016v29n224rc
Charnet, R., Luna, C. A. L., Charnet, E. M. R., and Bonvino, H. (2008). Análise de modelos de regressão linear com aplicações. 2nd Edn. Brazil: Unicamp.
Coimbra, J. L. M., Benin, G., Vieira, E. A., Oliveira, A. C. D., Carvalho, F. I. F., Guidolin, A. F., et al. (2005). Consequências da multicolinearidade sobre a análise de trilha em canola. Ciênc Rural 35, 347–352. doi: 10.1590/S0103-84782005000200015
Costa, N. R., Andreotti, M., Crusciol, C. A. C., Pariz, C. M., Lopes, K. S. M., Yokobatake, K. L. A., et al. (2016). Effect of intercropped tropical perennial grasses on the production of sorghum-based silage. Agron. J. 108, 2379–2390. doi: 10.2134/agronj2016.07.0385
Cruz, S. S. D., Andreotti, M., Pascoaloto, I. M., Lima, G. C. D., and Soares, C. D. A. (2020). Production in forage sorghum intercropped with grasses and pigeon pea at crop cutting. Rev. Ciênc. Agron. 51:e20165389. doi: 10.5935/1806-6690.20200031
Cruz, C. D., Carneiro, P. C. S., and Regazzi, A. J. (2014). Modelos biométricos aplicados ao melhoramento genético. Rev. E Ampl. Viçosa 2:688.
Daniel, J. L. P., Bernardes, T. F., Jobim, C. C., Schmidt, P., and Nussio, L. G. (2019). Production and utilization of silages in tropical areas with focus on Brazil. Grass Forage Sci. 74, 188–200. doi: 10.1111/gfs.12417
Dias, M. B. C., Costa, K. A. P., Severiano, E. C., Bilego, U., Furtini Neto, A. E., Almeida, D. P., et al. (2020). Brachiaria and Panicum maximum in an integrated crop-livestock system and second-crop corn system in succession with soybean. J. Agric. Sci. 158, 206–217. doi: 10.1017/S0021859620000532
Dias, M. B. C., Costa, K. A. P., Severiano, E. C., Bilego, U. O., Vilela, L., Souza, W. F., et al. (2021). Cattle performance with Brachiaria and Panicum maximum forages in an integrated crop-livestock system. Afr. J. Range For. Sci. 39, 230–243. doi: 10.2989/10220119.2021.1901311
Edson, C., Takarwirwa, N. N., Kuziwa, N. L., Stella, N., and Maasdorp, B. (2018). Effect of mixed maize-legume silages on milk quality and quantity from lactating smallholder dairy cows. Trop. Anim. Health Prod. 50, 1255–1260. doi: 10.1007/s11250-018-1552-4
Epifanio, P. S., Costa, K. A. P., Severiano, E. D. C., Simon, G. A., and Silva, V. R. (2019a). Nitrogen nutrition and changes in the chemical attributes of the soil for cultivars of Brachiaria brizantha intercropped with Stylosanthes in different forage systems arch. Agron. Soil Sci. 66, 1154–1169. doi: 10.1080/03650340.2019.1658867
Epifanio, P. S., Costa, K. A. P., Severiano, E. C., Souza, W. F., Teixeira, D. A. A., Silva, J. T., et al. (2019b). Productive and nutritional characteristics of Brachiaria brizantha cultivars intercropped with Stylosanthes cv. Campo Grande in different forage systems. Crop Pasture Sci. 70, 718–729. doi: 10.1071/cp18447
Erdal, S., Pamukcu, M., Curek, M., Kocaturk, M., and Dogu, O. Y. (2016). Silage yield and quality of row intercropped maize and soybean in a crop rotation following winter wheat. Arch. Agron. Soil Sci. 62, 1487–1495. doi: 10.1080/03650340.2016.1153801
Fernandes, P. B., Gonçalves, L. F., Claudio, F. L., Souza, J. A., Calgaro Júnior, G., Alves, E. M., et al. (2023). Sustainable production of maize with grass and pigeon pea intercropping. Agriculture 13:1246. doi: 10.3390/agriculture13061246
Ferreira, A. O., Amado, T. J. C., Rice, C. W., Diaz, D. A. R., Briedis, C., Inagaki, T. M., et al. (2018). Driving factors of soil carbon accumulation in Oxisols in long-term no-till systems of South Brazil. Sci. Total Environ. 622-623, 735–742. doi: 10.1016/j.scitotenv.2017.12.019
Ferreira, E. B., Cavalcanti, P. P., and Nogueira, D. A. (2014). ExpDes: an R package for ANOVA and experimental designs. Appl. Math. 5, 2952–2958. doi: 10.4236/am.2014.519280
Furtado, A. J., Abdalla Filho, A. L., Bruno, J. F., Neto, R. P., Lobo, A. A. G., Silva, G. V., et al. (2023). Pigeon pea intercropped with tropical pasture as a mitigation strategy for enteric methane emissions of Nellore steers. Animals 13:1323. doi: 10.3390/ani13081323
Galeano, E. S. J., Fernandes, T., Orrico Junior, M. A. P., Alves, J. P., Retore, M., Orrico, A. C. A., et al. (2022). Tamani grass-legume intercropping can improve productivity and composition of fodder destined to haylage or hay. Ciênc. Rural 52:e20210482. doi: 10.1590/0103-8478cr20210482
Gomes, V. C., Meirelles, P. R. L., Costa, C., Barros, J. S., Castilhos, A. M., Souza, D. M., et al. (2021). Production and quality of corn silage with forage and pigeon peas in a crop-livestock system. Semina 42, 861–876. doi: 10.5433/1679-0359.2021v42n2p861
Guimarães, F. S., Ciappina, A. L., Anjos, R. A. R., Silva, A., and Pelá, A. (2017). Consórcio guandu-milho-braquiária para integração lavoura-pecuária. Rev. Agric. Neotrop. 4, 22–27. doi: 10.32404/rean.v4i5.2218
Hawu, O., Ravhuhali, K. E., Mokoboki, H. K., Lebopa, C. K., and Sipango, N. (2022). Sustainable use of legume residues: effect on nutritive value and ensiling characteristics of maize straw silage. Sustain. For. 14:6743. doi: 10.3390/su14116743
Hedayati-Firoozabadi, A., Kazemeini, S. A., Pirasteh-Anosheh, H., Ghadiri, H., and Pessarakli, M. (2020). Forage yield and quality as affected by salt stress in different ratios of Sorghum bicolor-Bassia indica intercropping. J. Plant Nutr. 43, 2579–2589. doi: 10.1080/01904167.2020.1783301
Herrera, D. M., Peixoto, W. M., Abreu, J. G., Reis, R. H. P., Sousa, F. G., Balbinot, E., et al. (2023). Is the integration between corn and grass under different sowing modalities a viable alternative for silage? Animals 13:425. doi: 10.3390/ani13030425
Huang, H., Huang, J., Wu, Y., Zhuo, W., Song, J., Li, X., et al. (2023). The improved winter wheat yield estimation by assimilating GLASS LAI into a crop growth model with the proposed bayesian posterior-based ensemble Kalman filter. IEEE Trans. Geosci. Remote Sens. 61, 1–18. doi: 10.1109/TGRS.2023.3259742
Huang, J., Ma, H., Sedano, F., Lewis, P., Liang, S., Wu, Q., et al. (2019). Evaluation of regional estimates of winter wheat yield by assimilating three remotely sensed reflectance datasets into the coupled WOFOST–PROSAIL model. Eur. J. Agron. 102, 1–13. doi: 10.1016/j.eja.2018.10.008
Jobim, C. C., Nussio, L. G., Reis, R. A., and Schmidt, P. (2007). Avanços metodológicos na avaliação da qualidade da forragem conservada. Rev. Bras. Zootec. 36, 101–119. doi: 10.1590/S1516-35982007001000013
Kumari, V. V., Balloli, S. S., Ramana, D. B. V., Kumar, M., Maruthi, V., Prabhakar, M., et al. (2023). Crop and livestock productivity, soil health improvement and insect dynamics: impact of different fodder-based cropping systems in a rainfed region of India. Agric. Syst. 208:103646. doi: 10.1016/j.agsy.2023.103646
Kung, L., and Shaver, R. (2001). Interpretation and use of silage fermentation analyses reports. Focus Forage 3, 1–5.
Kung, L., Shaver, R. D., Grant, R. J., and Schmidt, R. J. (2018). Silage review: interpretation of chemical, microbial, and organoleptic components of silages. J. Dairy Sci. 101, 4020–4033. doi: 10.3168/jds.2017-13909
Kutamahufa, M., Matare, L., Soropa, G., Mashavakure, N., Svotwa, E., and Mashingaidze, A. B. (2022). Forage legumes exhibit a differential potential to compete against maize and weeds and to restore soil fertility in a maize-forage legume intercrop. Acta Agric. Scand. B Soil Plant Sci. 72, 127–141. doi: 10.1080/09064710.2021.1998593
Ligoski, B., Gonçalves, L. F., Cláudio, F. L., Alves, E. M., Kruger, A. M., Bizzuti, B. E., et al. (2020). Silage of intercropping corn, palisade grass, and pigeon pea increases protein content and reduces in vitro methane production. Agronomy 10:1784. doi: 10.3390/agronomy10111784
Maia, S. M. F., Medeiros, A. S., Santos, T. C., Lyra, G. B., Lal, R., Assad, E. D., et al. (2022). Potential of no-till agriculture as a nature-based solution for climate-change mitigation in Brazil. Soil Tillage Res. 220:105368. doi: 10.1016/j.still.2022.105368
Marques, K. O., Jakelaitis, A., Guimarães, K. C., Pereira, L. S., Cardoso, I. S., and Lima, S. F. (2019). Production, fermentation profile, and nutritional quality of silage from corn and soybean intercropping. Semina 40, 3143–3156. doi: 10.5433/1679-0359.2019v40n6Supl2p3143
Matta, F. D. P., Godoy, R., Oliveira, P. P. A., and Ferraz, R. S. (2024). Row spacing for pigeon pea sowing and its influence on the recovery of degraded pasture. Pesqui. Agropecu. Bras. 59:e03516. doi: 10.1590/S1678-3921.pab2024.v59.03516
Mcdonald, P. J., Henderson, A. R., and Heron, S. J. E. (1991). The biochemistry of silage. Edinburgh, UK: Mallow Chalcombe publications.
Mello, C. E., Jakelaitis, A., Silva, C. H. L., Sousa, G. D., and Silva, J. O. (2023). Glyphosate doses in the suppression of Megathyrsus maximus cv. BRS Quênia intercropped with transgenic maize. Rev. Bras. Eng. Agricola Ambient. 27, 892–899. doi: 10.1590/1807-1929/agriambi.v27n11p892-899
Meng, H., Jiang, Y., Wang, L., Wang, S., Zhang, Z., Tong, X., et al. (2022). Effects of different soybean and maize mixed proportions in a strip intercropping system on silage fermentation quality. Fermentation 8:696. doi: 10.3390/fermentation8120696
Meo-Filho, P., Berndt, A., Pezzopane, J. R. M., Pedroso, A. F., Bernardi, A. C. C., Rodrigues, P. H. M., et al. (2022). Can intensified pasture systems reduce enteric methane emissions from beef cattle in the Atlantic Forest biome? Agronomy 12:2738. doi: 10.3390/agronomy12112738
Mertens, D. R. (2002). Gravimetric determination of amylase-treated neutral detergent fiber in feeds with refluxing in beaker or crucibles: collaborative study. J. AOAC Int. 85, 1217–1240. doi: 10.1093/jaoac/85.6.1217
Muck, R. E., and Shinners, K. J. (2001). Conserved forage (silage an hay): Progress an priorities. International grassland congress. Piracicaba: FEALQ.
Muniz, M. P., Costa, K. A. P., Severiano, E. C., Bilego, U. O., Almeida, D. P., Furtini Neto, A. E., et al. (2021). Soybean yield in integrated crop–livestock system in comparison to soybean–maize succession system. J. Agric. Sci. 159, 188–198. doi: 10.1017/S0021859621000393
Nave, R. L. G., and Corbin, M. D. (2018). Forage warm-season legumes and grasses intercropped with corn as an alternative for corn silage production. Agronomy 8:199. doi: 10.3390/agronomy8100199
Oliveira, M. H., Costa, C., Pariz, C. M., Lima Meirelles, P. R., Andreotti, M., Castilhos, A. M., et al. (2021). Yield and nutritive value of mechanically processed corn silage from an integrated crop-livestock system. Semina 42, 845–860. doi: 10.5433/1679-0359.2021v42n2p845
Oliveira, S. S., Costa, K. A. P., Souza, W. F., Santos, C. B., Teixeira, D. A. A., and Silva, V. C. (2020). Production and quality of the silage of sorghum intercropped with Paiaguas palisadegrass in different forage systems and at different maturity stages. Anim. Prod. Sci. 60, 694–704. doi: 10.1071/AN17082
Oliveira, M. V. M., Demeu, A. A., Bonatti, F. K. Q., Torres, F. E., and Teodoro, P. E. (2017). Production and quality of forage under intercropping systems in the Cerrado/Pantanal ecotone. Biosci. J. 33, 341–348. doi: 10.14393/BJ-v33n2-32880
Paula, F. L. M., Menezes, L. F. G., Paris, W., Ronsani, R., Hoppen, S. M., and Ciesca, J. (2016). Produção química de silagem e composição do consórcio de milho e capim-Tanzânia. Semina 37, 1607–1616. doi: 10.5433/1679-0359.2016v37n3p1607
Pereira, D. S., Lana, R. P., Carmo, D. L., and Costa, Y. K. S. (2019). Chemical composition and fermentative losses of mixed sugarcane and pigeon pea silage. Acta Sci. Anim. Sci. 41:e43709. doi: 10.4025/actascianimsci.v41i1.43709
Prado, L. G., Costa, K. A. P., Silva, L. M., Costa, A. C., Severiano, E. C., Costa, J. V. C. P., et al. (2023). Silages of sorghum, Tamani guinea grass, and Stylosanthes in an integrated system: production and quality. Front. Sustain. Food Syst. 7:1208319. doi: 10.3389/fsufs.2023.1208319
Queiroz, F. E., Rocha Júnior, V. R., Monção, F. P., Rigueira, J. P. S., Parrella, R. A. C., Rufino, L. D. A., et al. (2021). Effect of row spacing and maturity at harvest on the fermentative profile, aerobic stability, and nutritional characteristics of biomass sorghum (BRS 716) silage in the semiarid region of Brazil. Rev. Bras. Zootec. 50:e20200254. doi: 10.37496/rbz5020200254
R Core Team (2014). R: A language and environment for statistical computing. Vienna: R Foundation for Statistical Computing.
Renwick, L. L., Kimaro, A. A., Hafner, J. M., Rosenstock, T. S., and Gaudin, A. C. (2020). Maize-pigeonpea intercropping outperforms monocultures under drought. Front. Sustain. Food Syst. 4:562663. doi: 10.3389/fsufs.2020.562663
Ribeiro, M. G., Costa, K. A. P., Souza, W. F., Cruvinel, W. S., Silva, J. T., and Santos Júnior, D. R. (2017). Silage quality of sorghum and Urochloa brizantha cultivars monocultureped or intercropped in different planting systems. Acta sci. Anim Sci. 39:243. doi: 10.4025/actascianimsci.v39i3.33455
Ribeiro, F. M., Lima, M., Costa, P. A. T., Pereira, D. M., Carvalho, T. A., Souza, T. V., et al. (2019). Associations between morphometric variables and weight and yields carcass in Pirapitinga Piaractus brachypomus. Aquac. Res. 50, 2004–2011. doi: 10.1111/are.14099
Rufino, M. C., Gachene, C. K., Diogo, R. V., Hawkins, J., Onyango, A. A., Sanogo, O. M., et al. (2021). Sustainable development of crop-livestock farms in Africa. Front. Agric. Sci. Eng. 8, 175–181. doi: 10.15302/J-FASE-2020362
Santos, H. G., Jacomine, P. K. T., Anjos, L. H. C., Oliveira, V. A., Lumbreras, J. F., Coelho, M. R., et al. (2018). Sistema Brasileiro de Classificação de Solos. Embrapa CNPS 5th Edn (Brasília: Embrapa), 356.
Silva, J. A. G., Costa, K. A. P., Severiano, E. C., Silva, A. G., Vilela, L., Leandro, W. M., et al. (2024a). Efficiency of desiccation, decomposition and release of nutrients in the biomass of forage plants of the genus Brachiaria after intercropping with sorghum in integrated systems for soybean productivity. Commun. Soil Sci. Plan. 55, 1644–1662. doi: 10.1080/00103624.2024.2323076
Silva, L. M., Costa, K. A. P., Silva, J. A. G., Costa, J. V. C. P., Costa, A. C., Severiano, E. C., et al. (2023). Fermentative profile and nutritive value of maize, legume and mixed silage. Semina 44, 1909–1926. doi: 10.5433/1679-0359.2023v44n5p1909
Silva, J. A. G., Costa, K. A. P., Silva, L. M., Severiano, E. C., Silva, F. G., Habermann, E., et al. (2023). Integrated systems improve the sustainability of soybean cultivation in the tropical region. Front. Sustain. Food Syst. 7:1224530. doi: 10.3389/fsufs.2023.1224530
Silva, J. A. G., Habermann, E., Costa, K. A. P., Silva, L. M., Severiano, E. C., Costa, A. C., et al. (2024b). Integration crop-livestock system increases the sustainability of soybean cultivation through improved soil health and plant physiology. Agric. Ecosyst. Environ. 359:108770. doi: 10.1016/j.agee.2023.108770
Silva, L. S., Laroca, J. V. S., Coelho, A. P., Gonçalves, E. C., Gomes, R. P., Pacheco, L. P., et al. (2022). Does grass-legume intercropping change soil quality and grain yield in integrated crop-livestock systems? Appl. Soil Ecol. 170:104257. doi: 10.1016/j.apsoil.2021.104257
Silva, D. J., and Queiroz, A. C. (2002). Análise de alimentos (métodos químicos e biológicos). 3rd Edn. Viçosa: Imprensa Universitária da UFV.
Silva, A., Santos, F. L. S., Barretto, V. C. M., Freitas, R. J., and Kluthcouski, J. (2018). Recovery of degraded pasture by the maize intercropping with Urochloa brizantha cv. Marandu and pigeon pea. Rev. Agric. Neotrop. 5, 39–47. doi: 10.32404/rean.v5i2.1382
Souza, W. F., Costa, K. A. P., Guarnieri, A., Severiano, E. C., Silva, J. T., Teixeira, D. A. A., et al. (2019). Production, and quality of the silage of corn intercropped with Paiaguas palisadegrass in different forage systems and maturity stages. Rev. Bras. Zootec. 48:22. doi: 10.1590/rbz4820180222
Tang, C., Yang, X., Chen, X., Ameen, A., and Xie, G. (2018). Sorghum biomass and quality and soil nitrogen balance response to nitrogen rate on semiarid marginal land. Field Crop Res. 215, 12–22. doi: 10.1016/j.fcr.2017.09.031
Teixeira, D. A. A., Costa, K. A. P., Souza, W. F., Severiano, E. C., Guimaraes, K. C., Silva, J. T., et al. (2021). Fermentation profile and nutritive value of maize silage with 'Brachiaria' species. Aust. J. Crop. Sci. 15, 695–702. doi: 10.21475/ajcs.21.15.05.p3004
Tilley, J. M. A., and Terry, R. A. (1963). A two-stage technique of the “in vitro” digestion of forage crop. J. Br. Grassl. Soc. 18, 104–111. doi: 10.1111/j.1365-2494.1963.tb00335.x
Vicente, E. R. J. S., Feba, L. T., Silva, P. C. G., Franzluebbers, A. J., and Moro, E. (2023). Production and quality of sorghum silage intercropped with marandu grass and pigeonpea. Colloq Agrariae. 19, 43–63. doi: 10.5747/ca.2023.v19.h512
Vicente, E. R. J. S., Kuwahara, F. A., Feba, L. T., Silva, P. C. G., and Moro, E. (2019). Yield of sorghum silage intercropped with pigeon pea and marandu grass in two spacings and chemical composition before and after ensiling. Aust. J. Crop. Sci. 13, 1416–1421. doi: 10.21475/ajcs.19.13.09.p1248
Wang, X., Huang, J., Feng, Q., and Yin, D. (2020). Winter wheat yield prediction at county level and uncertainty analysis in main wheat-producing regions of China with deep learning approaches. Remote Sens. 12:1744. doi: 10.3390/rs12111744
Yi, J., Li, H., Zhao, Y., Shao, M., Zhang, H., and Liu, M. (2022). Assessing soil water balance to optimize irrigation schedules of flood-irrigated maize fields with different cultivation histories in the arid region. Agric. Water Manag. 265:107543. doi: 10.1016/j.agwat.2022.107543
Keywords: Cajanus cajan cv. BRS Mandarim, integrated systems, silage fermentative profile, sustainability, triple intercropping
Citation: da Silva LM, Costa KAdP, Prado LG, Rezende AG, Severiano EdC, Costa JVCP, e Silva JAG, Costa AC, Fernandes PB, Rodrigues RC, Marques BdS, de Morais BF and Rodrigues F (2024) Maize intercropped with Panicum maximum cultivars and Pigeon pea improves silage yield and quality. Front. Sustain. Food Syst. 8:1416717. doi: 10.3389/fsufs.2024.1416717
Edited by:
Maryke T. Labuschagne, University of the Free State, South AfricaReviewed by:
Aysha Arif Chahel, Government Sadiq College Women University, Bahawalpur, PakistanRupesh Kumar Singh, University of Trás-os-Montes and Alto Douro, Portugal
Copyright © 2024 da Silva, Costa, Prado, Rezende, Severiano, Costa, e Silva, Costa, Fernandes, Rodrigues, Marques, de Morais and Rodrigues. This is an open-access article distributed under the terms of the Creative Commons Attribution License (CC BY). The use, distribution or reproduction in other forums is permitted, provided the original author(s) and the copyright owner(s) are credited and that the original publication in this journal is cited, in accordance with accepted academic practice. No use, distribution or reproduction is permitted which does not comply with these terms.
*Correspondence: Kátia Aparecida de Pinho Costa, a2F0aWEuY29zdGFAaWZnb2lhbm8uZWR1LmJy