- 1Agroscope, Environment and Resources, Life Cycle Assessment Research Group, Zürich, Switzerland
- 2Agroscope, Methods Development and Analytics, Biochemistry of Milk and Microorganisms Research Group, Bern, Switzerland
- 3Division of Human Nutrition, Wageningen University & Research, Wageningen, Netherlands
- 4Department of Health, Swiss Distant University of Applied Sciences (FFHS)/University of Applied Sciences and Arts of Southern Switzerland (SUPSI), Manno, Switzerland
Efforts towards sustainable food systems have stimulated the development of plant-based alternatives to meat and milk. However, the debate on the nutritional quality of (processed) plant products compared to animal products remains unresolved, and combined assessments are needed to assess the benefits and trade-offs of these products in future diets. Using life cycle assessment (LCA) combined with nutrient density measurements and the Digestible Indispensable Amino Acid Score (DIAAS), this case study evaluates different processing stages of soy-based products, including cooked soybeans, tofu, soy drink, and a processed soy-based meat analogue (SBMA) produced in Switzerland. The nutritional LCA (n-LCA) showed that the environmental impact of all soy-based meat alternatives was 4–20 times lower than that of beef, especially when locally sourced soy was used. The differences were smaller when compared to chicken meat. All soy-based products showed lower DIAAS compared to animal products, but the results from the combined n-LCA were always less favourable for animal products in this case study. Contribution analyses showed that despite the high level of processing, the raw materials contributed significantly to the environmental footprint of SBMA, exceeding 50% in some environmental impact categories. Moreover, comparisons within the soy-based alternatives revealed a lower environmental impact of the minimally processed products. The higher protein quality and quantity of the processed SBMA were not sufficient to offset its higher environmental impact in this case study. SBMA also contained highest level of sodium and saturated fatty acids, highlighting the need for careful food formulation. Overall, this study showed the potential of soy-based alternatives to meat and milk to reduce the environmental impact of food production whilst highlighting the importance of considering their nutritional quality and the role of processing. Overall, this research provides insight into the potential of plant-based alternatives to meat and milk for sustainable diets amid the global challenge of climate change and changing dietary patterns.
1 Introduction
Food systems are responsible for a third of global anthropogenic greenhouse gas emissions (Crippa et al., 2021), and meat and milk (including feed, direct emissions, land use and land-use change, and further supply chains) account for around 60% within the food system (Xu et al., 2021). Although livestock take up most of the world’s agricultural land (83%), they only produces 37% of the total protein and only 18% of the world’s calories (Poore and Nemecek, 2018). Meeting the Paris Agreement to cap global warming at 1.5°C requires rapid and ambitious changes to food systems (Clark et al., 2020). Replacing animal proteins with plant proteins has been seen as an effective way to reduce the environmental impact of the food system (Santo et al., 2020; Kozicka et al., 2023; Lehtonen and Rämö, 2023). However, the consumption of animal products is deeply interwoven with local culture in Switzerland, and overall consumer acceptance of plant-based alternatives is low in Europe (Michel et al., 2021; Onwezen et al., 2021; Varela et al., 2022). Recently, food processing technology has emerged in the hope of fostering solutions to the challenge of replacing animal-derived proteins with plant-derived proteins in significant quantities (Sha and Xiong, 2020; Gibney, 2021). By enhancing convenience and resemblance to traditional animal-based products, novel plant-based meat and dairy alternatives were developed and successfully introduced on the Swiss market (Bundesamt für Landwirtschaft, 2021; Coop, 2023). In particular, plant-based meat analogues (PBMAs), which mimic the organoleptic and aesthetic components of meat products, are seen as major drivers of this transformation, with market shares expected to account for 25% of the total global meat market by 2035 (Gerhardt et al., 2020).
Processed foods are often designed for ease of consumption and storage (i.e., convenience) and the World Health Organisation (WHO) promotes the need for food reformulation of convenience products because they are often energy dense and nutrient poor (World Health Organization, 2022). The PBMAs belong to Group 4 in the NOVA classification, and are thus an ultra-processed food (Monteiro, 2009) whereas traditional alternatives to meat and milk such as tofu, tempeh or soy drink are less processed. In a study by Gonzales et al. (2022), plant-based alternatives are perceived as healthier compared to their animal counterparts, even if highly processed, revealing a “halo effect” of plant-based products, without any considerations of calories, beneficial and disqualifying nutrients (Besson et al., 2020; Gonzales et al., 2022). Whereas there is a broad agreement that replacing traditional meat and milk products with plant-based alternatives will have a positive effect on the environment, their contribution to health is controversial due to their degree of processing and nutritional status (Green et al., 2022; Cordova et al., 2023) and thus, combined assessments are needed. Additionally, in recent years, the importance of protein quality has gained increasing prominence in discussions about the substitution of dairy products (Walther et al., 2022; Komatsu et al., 2023) and meat (Sonesson et al., 2017; Hertzler et al., 2020; Sousa et al., 2023a) with plant-based alternatives. The Digestible Indispensable Amino Acid Score (DIAAS) was recommended by the Food and Agriculture Organization of the United Nations (FAO) as best measure for assessing protein quality. The DIAAS determines the quantity of every indispensable amino acid (IAA) that is potentially digested at the ileal level and available to cover the requirements for IAA (Food and Agricultural Organization of the United Nations, 2013). Animal-derived proteins tend to have higher DIAAS values than proteins derived from plants (Herreman et al., 2020) which is due to deficiencies in one or more IAA and overall lower digestibility. However, processing and blending prior to human consumption also significantly alter the protein quality of plant proteins (Nosworthy et al., 2017, 2018; Adhikari et al., 2022). Additionally, the preparation (e.g., cooking) process may enhance or decrease protein digestibility and, thus, the final DIAAS (Hammer et al., 2023; Sousa et al., 2023a). A novel in vitro protein digestibility workflow based on the INFOGEST static protocol (Brodkorb et al., 2019) has been developed and validated at Agroscope (Sousa et al., 2023b). This method was recently used to investigate the DIAAS of soy products, such as tofu, soy drink (Hammer et al., 2024), and one novel soy-based meat analogue (SBMA) from soybean protein concentrate, as well as chicken meat and beef (Sousa et al., 2023a).
By combining findings on nutrient density and protein quality measurements with life cycle assessment (LCA) of animal and plant protein production systems in Switzerland, this case study aims to provide a combined evaluation of soy-based meat and milk alternatives and their animal reference products. Therefore, in addition to considering different environmental impact categories, this LCA study extends the nutritional focus by using the Nutrient Rich Food (NRF) index (Fulgoni et al., 2009), subsequently adapted to the needs of the Swiss population and modified for protein-rich products with a quality-corrected protein content (qc-protein). First described as a weighted protein score by Berardy et al. (2019) and later applied by Van Mierlo et al. (2022) and McAuliffe et al. (2023), the qc-protein is a potential protein content, taking into account DIAAS. A few other studies have presented methods to quantify the more complex nutritional value of foods that go beyond single nutrients (Drewnowski et al., 2015; Saarinen et al., 2017; Walker et al., 2018) or using the health nutritional index (Ernstoff et al., 2020; Stylianou et al., 2021), but the methods are limited to traditional food products and do not include protein quality. McLaren et al. (2021) discussed leading methodological approaches and defined recommendations for the nutritional life cycle assessment (n-LCA) of foods, including nutritional aspects, but research with nutritional functional units (FU) has only been emerging in recent years and remains scarce, mainly due to a lack of data availability in the case of protein quality (Sonesson et al., 2017; Berardy et al., 2019; Moughan, 2021). To the authors’ knowledge, this is the first attempt to evaluate multiple environmental impact categories related to processing plant proteins into plant-based meat and milk alternatives, including their specific IAA composition and digestibility, besides other nutrients.
This study follows an n-LCA approach to assess the environmental impact of three minimally processed animal-based products and four soy-based meat and milk alternatives at various processing stages, combined with nine nutrients, their corresponding recommended dietary allowance (RDA), and their DIAAS. In the same case study, the effects of processing on plant proteins are investigated and assessed in terms of additional impact burden, change in protein quality, and overall nutrient density.
2 Methods
2.1 Goal and scope
Following the ISO 14040 and 14044 (International Standard Organisation, 2006a,b) standards, this case study aims to provide a basis for communicating the nutritional composition, protein quality, and environmental impact of soy-based alternatives compared to chicken meat, beef, and cow milk. The n-LCA study is valid for products within Switzerland’s production and consumption scope. Employing a cradle-to-gate approach, the study encompasses the entire life cycle of the system, from production to the factory gate. Agricultural production constitutes the upstream system, whilst the core system involves the production of soy drink, tofu, and the focus PBMA of this case study, the SBMA, vis-à-vis meat and milk production (Figure 1). Excluded from consideration are storage, distribution, home cooking, and the end-of-life aspects of the manufactured products.
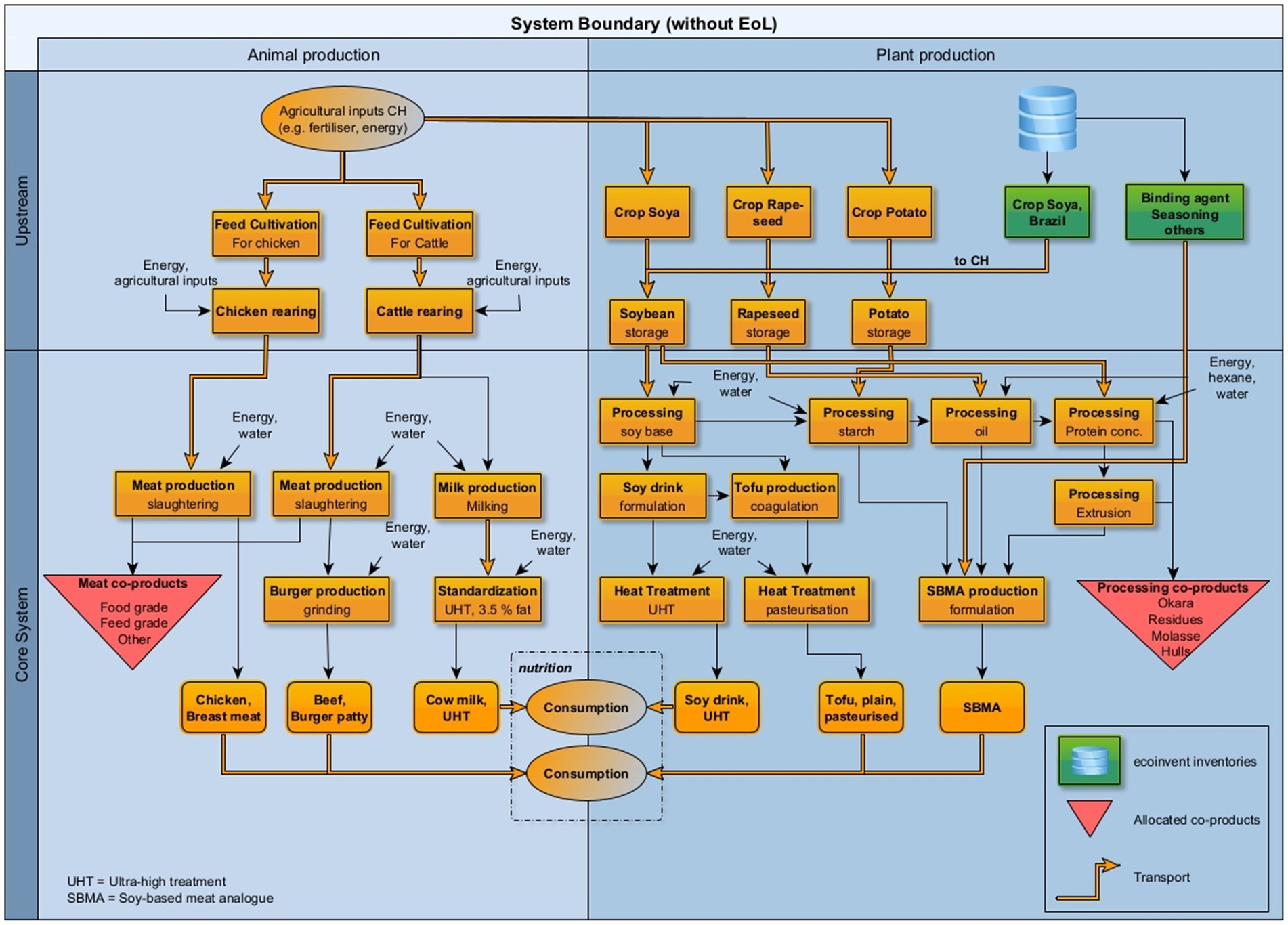
Figure 1. Flowchart of the food production system with visualisation of the upstream and core system processes within the system boundary. BR = Brazil, CH = Switzerland.
2.1.1 Nutritional functional unit
The FU provides the reference unit for the LCA (International Standard Organisation (ISO), 2006a). As nutritional delivery is a key function of food, comparisons of products under investigation were based on (a) protein quality and (b) nutrient density.
The protein quality of food products was determined by the DIAAS. Values above 100 were possible and reflected the potential to complement incomplete proteins from other sources. According to McAuliffe et al. (2023), the amount of protein in g per 100 g of food is multiplied by the DIAAS as a factor, which results in the (potential) qc-protein in 100 g of food. Given the focus on different food production systems, which generate proteins of different quality, the first FU was the production of 1 gram of qc-protein (FU: 1 g qc-protein). This nutritional FU is based on a single nutritional aspect, but the nutritional function of foods is more complex and involves a large number of nutrients. Thus, by using a method of quantifying the nutrient density of foods based on dietary requirements for several relevant nutrients, a more complex nutritional FU considering both nutritional content and nutritional demands was created. Following Green et al. (2023) and Saarinen et al. (2017), an adapted NRF score was used for nutrient density. The NRF score is calculated as the arithmetic difference between the positive and negative nutrient sub-scores and multiplied by 100 (Eq. 1). The positive sub-score is based on a variable number n of beneficial nutrients (NRn), whereas a maximum of three disqualifying nutrients (saturated fat, added sugar, and sodium) are considered in a second sub-score (LIMn), implying the need to limit their consumption. The NRn was adapted for protein-rich foods and included seven favourable nutrients: protein, fibre, and (mono-)unsaturated fatty acids, calcium (Ca), iron (Fe), zinc (Zn), and cobalamin (vitamin B12), as these are nutrients of concern in plant-based diets critical nutrients in the diet of the Swiss population (Marques-Vidal et al., 2023). To address protein quality, the introduced qc-protein was included instead of the protein content (NRprot7). As no sugar was added to any of the products, the LIMn included two disqualifying nutrients only (LIM2, saturated fatty acids and sodium). The NRprot7 and LIM2 were expressed as unweighted sums of %RDA per 100 g (Eqs. 2, 3). The Federal Food Safety and Veterinary Office recently has updated the uniform Swiss RDA (Bundesamt für Lebensmittelsicherheit und Veterinärwesen, 2022). The average metric score was used for both sexes and for the ages 18–65. Further information can be found in Supplementary material S1E. Both sub-scores were normalised to 2,000 kilocalories (kcal) by multiplication with the energy content per 100 g (E) in kcal to address different water contents. In a mixed diet, a nutrient surplus in one food product could compensate for a nutrient deficiency in another; therefore, in a single product comparison, no capping was applied (Green et al., 2023).
where: i = nutrient, j = food item
In accordance with Hallström et al. (2018) and Green et al. (2023), only the positive nutrient sub-score was employed in the LCA instead of the original NRF score (FU: 1 NRprot7). This preference arises from the argument that the function of food should not be negative, whereas the final NRF index can result in negative values, a condition deemed incongruous for an FU. Nonetheless, the LIM2 sub-score was calculated and considered in the final evaluation of the food products as an independent nutrient indicator.
For further use of the results, a reference flow with a mass-based FU (FU: 100 g food product) was also considered. All calculated results for the different FUs are available in Supplementary material S4.
2.2 Data collection
2.2.1 Life cycle inventory
The life cycle inventories of beef, chicken, and cow milk stem from Alig et al. (2012), Wolff et al. (2016), and Bystricky et al. (2014), respectively, all calculated using the Swiss Agricultural Life Cycle Assessment methodology (Nemecek et al., 2023). To allocate environmental loads between milk and meat from dairy cattle, an economic allocation from Bystricky et al. (2014) with an allocation ratio of 87:13 was chosen. The same methodology was also used for the life cycle inventories of soybean production and forms the basis of the upstream system. The data for soybeans are representative of production in the Swiss plain region with conventional production methods.
For the core system (i.e., processing into food products), the foreground data were sourced from a combination of inventories from recent databases, including Agri-Footprint v.6.3 (Tyszler et al., 2022), AGRIBALYSE v.3.1 (Asselin-Balençon et al., 2022), and ecoinvent v.3.9.1.(Wernet et al., 2016; FitzGerald and Sonderegger, 2022). Where necessary, the background inventories for the production of foods under investigation were aligned with the geographical scope of agricultural production, Switzerland. The process of converting unprocessed soybeans into soy-based products take in various production steps, including cooking, protein separation, concentration, texturisation, and ingredient formulation. A raw-to-cooked ratio for cooked soybeans was calculated considering the water content from Hammer et al. (2024). The production of tofu and soy drink was modelled based on generic ecoinvent inventories with additional economic allocation for okara production. Both products underwent thermal treatment. Ultra-high-temperature (UHT) sterilisation was applied in the case of soy drink and cow milk. SBMA production involved protein separation through ethyl alcohol extraction and, subsequently, texturisation with a twin-screw extruder and final product formulation. The SBMA, tofu, and cooked soybeans were compared with minced beef and chicken meat. Soy drink was compared with UHT-sterilised cow milk with standardised fat content (3.5%). Slaughterhouse and dairy activities were considered. Economic allocations for multi-output inventories (e.g., the side streams of protein fractionation, slaughter products, etc.) were used throughout. Detailed explanations of all the process assumptions are provided in Supplementary material S1A.
The final composition of the SBMA was determined through a linear equation system. Apart from the source of proteins and added water, the remaining sources of macronutrients (fat and carbohydrates) were assumed from the literature and personal communication (Table 1). The ingredient list was benchmarked against existing products. The linear equation and derivation of assumptions are provided in Supplementary material S1B. The environmental implications of the remaining minor ingredients (5%) were explored and excluded from the inventory if the additive share of environmental burdens was below 1%.
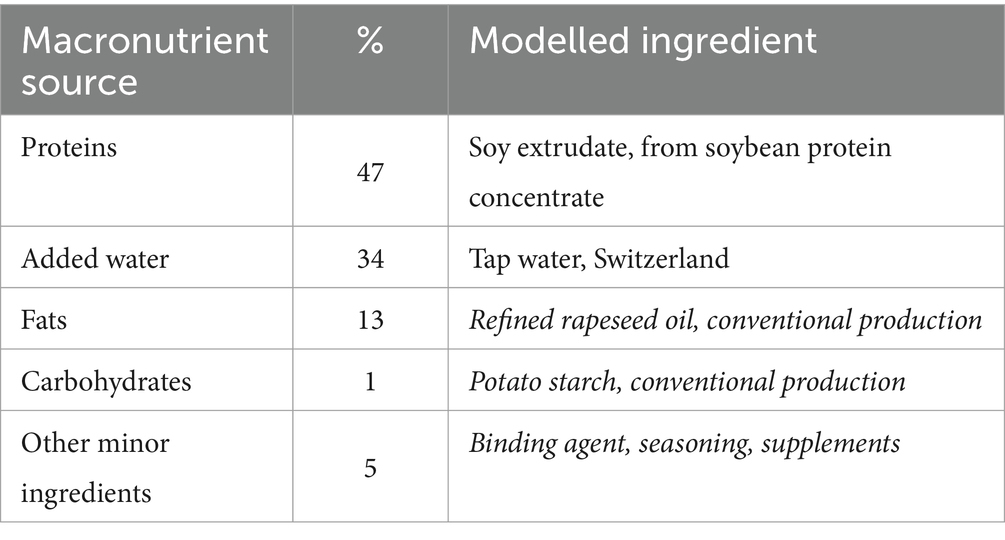
Table 1. Ingredient composition of SBMA based on linear equation approximation model—approximated ingredients are in italics.
Cow milk and soy drink had undergone UHT sterilisation process and may be stored without refrigeration, whereas meat, tofu, cooked soybeans and SBMA need to be refrigerated until consumption. As the two product categories, meat and milk, were analysed separately, cold storage was excluded in the case of meat. A detailed list of all inventories used in this study is reported in Supplementary material S2.
2.2.2 Nutritional information
Except for vitamin B12, relevant nutritional data (macronutrients and micronutrients) of soy-based products were measured in duplicate at least. Since vitamin B12 is absent in most plant sources, its concentration was set to 0 (Table 2). The nutritional data were complemented with values from the Swiss Food Composition Table (SFCT) v.6.5 (Bundesamt für Lebensmittelsicherheit und Veterinärwesen, 2023) for cooked soybeans, tofu, and soy drink. In particular, the available carbohydrates and fatty acid compositions (mono-unsaturated fatty acids, poly-unsaturated fatty acids, saturated fatty acids) were taken from the SFCT. Due to the better market reliability, sodium values for cooked soybeans, tofu, and soy drink were also taken from the SFCT, as no salt was used for the production of those products (Hammer et al., 2024). Nutritional data for SBMA was sourced from Sousa et al. (2023a) and supplemented through personal communication with the authors. For UHT-sterilized and standardised cow milk, a thorough nutritional analysis was referenced from Walther et al. (2022). Nutritional data of cooked meat products was extracted from the SFCT database, utilising the entries “Chicken, breast (schnitzel, ground), pan fried (without addition of fat and salt)” and “Beef, minced, pan fried (without addition of fat and salt).” Details on the nutritional analysis methods and SFCT sources are recorded in Supplementary material S1D.
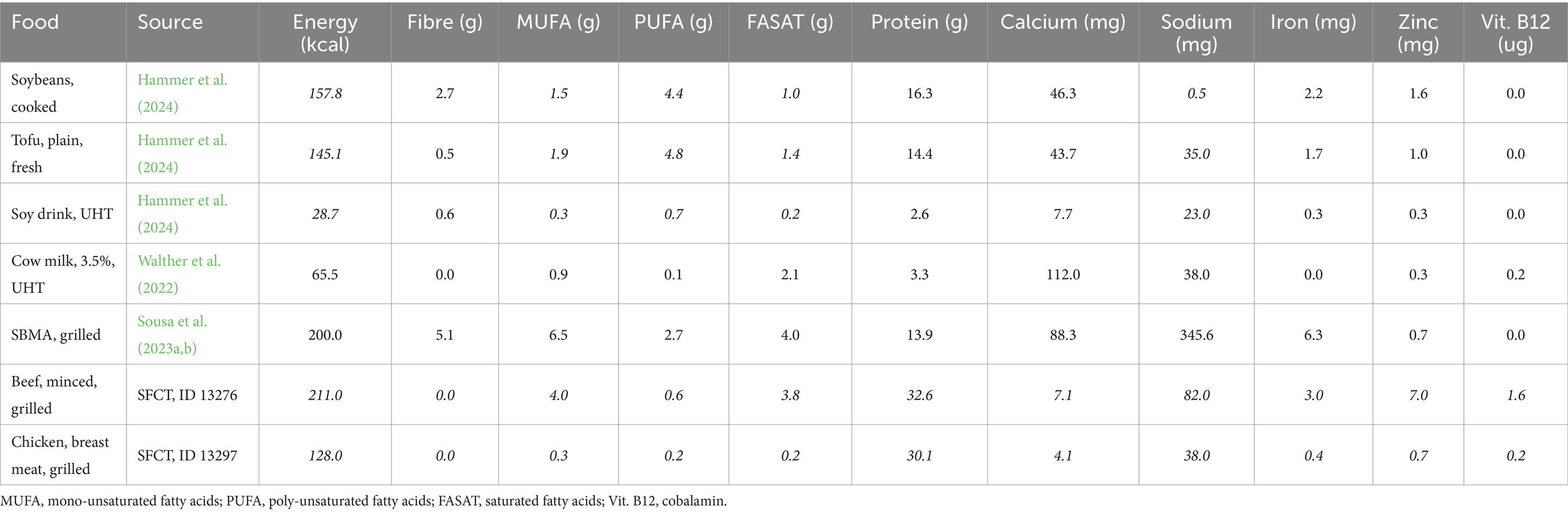
Table 2. Nutritional information used in the case study per 100 g of food product and their corresponding source—generic data from SFCT are in italics.
The amount of every IAA for all products and their digestibility, except for cow milk, were measured in duplicates (foods) or triplicates (digestibility) and calculated elsewhere (Hammer et al., 2023; Sousa et al., 2023a) with the in vitro digestibility method based on the INFOGEST in vitro digestion (Sousa et al., 2023b). For the cooked soybeans, tofu, and soy drink, three different varieties (Galice, Amandine, and Protéix) were measured by Hammer et al. (2024); thus, the arithmetic means of the IAAs and digestibility were taken for the study. In particular, the amounts of the in vitro digested IAAs were calculated based on the amount of IAAs present in 1 g of food protein (based on the total nitrogen × nitrogen conversion factor of 6.25) and multiplied by the digestibility of the individual IAA. Dividing these values by the reference amino acid requirement values (amino acid scoring pattern) for young children (6 months to 3 years; Food and Agricultural Organization of the United Nations, 2013) or older (children, young adolescents, and adults; Food and Agricultural Organization of the United Nations, 2013) resulted in the Digestible Indispensable Amino Acid Ratio for each IAA. The lowest Digestible Indispensable Amino Acid Ratio value, which therefore represents the limiting amino acid of the food, corresponds to the DIAAS, expressed as a percentage (i.e., the IAA having the lowest digestible reference ratio; ratio × 100) (Food and Agricultural Organization of the United Nations, 2013). For cow milk, IAAs were taken from Walther et al. (2022). Their DIAAS results were solely based on IAA, assuming a digestibility of 100%. They argued that the proteins in liquid form have high accessibility for digestive enzymes and can therefore be assumed to have high digestibility, so the assumption of complete digestibility (100%) is sufficient for the calculation of the DIAAS. The DIAAS calculations for cow milk in this study were complemented with the true ileal digestibility of dry milk powder from Fanelli et al. (2021), measured in vivo in pigs, as a proxy for UHT-sterilized and standardised cow milk instead. The data table of all IAA values and digestibility are provided in Supplementary material S1C.
2.3 Life cycle impact assessment
The life cycle impact assessment was performed using the software SimaPro v.9.5.0 (PRé Sustainability, 2023) and the impact methods from SALCA v.2.0.1 methodology (Douziech et al., 2024). We highlight the use of the following impact categories: the global warming potential with 100-year time horizon (GWP100) of the Intergovernmental Panel on Climate Change (2021) with carbon emissions from fossil sources and land use and land use changes; the impacts of water consumption based on the available water remaining method, which calculates stress-weighted impacts; specifically, it calculates the potential water scarcity (Water-scar) for other users in the same area (Boulay et al., 2018); the midpoints terrestrial acidification potential (Acid-ter) and freshwater eutrophication potential (Eutr-fw) from the ReCiPe Methodology 2016 (Huijbregts et al., 2017); the total land use and land occupation (Land-occ) from the ReCiPe methodology 2008 (Goedkoop et al., 2009); and the cumulative energy demand (CED) (Frischknecht et al. 2007).
For the contribution analysis, processes were categorised either in upstream system or core system based on the flow chart (Figure 1) and the relevance of agricultural production for the total environmental impact of the products was discussed.
2.4 Sensitivity analysis
Three sensitivity analyses were performed to estimate the effects of data accuracy, relevant assumptions, and constraints on the results. First, the upper and lower limits of the raw material inputs were set at ±20% of the reference flow to account for uncertainties in the production methods of the soy-based products. Second, the influence of the origin of the raw material was investigated. Therefore, the agricultural production system for soybeans was changed from Switzerland to Brazil, and transportation was adjusted accordingly (see Supplementary material S1F). Third, the reference profile for IAA was set to young children instead of profiles of older children, adolescents, and adults, which affects the DIAAS calculations (this age group was selected according to the FAO’s request for legal purposes Food and Agricultural Organization of the United Nations, 2013). For the NRprot7 calculations, the RDA values were further adjusted using the average metric score for the ages of 1–3 years and for both sexes. In the same sensitivity analysis, uncertainties in RDA values due to differences between males and females were observed, comparing the upper and lower limits of the respective recommendations with the averaged values.
3 Results
3.1 Contribution analysis
The contribution analysis carried out for the SBMA showed that despite the intensive processing involved in production, soybeans, potatoes, and rapeseeds still accounted for a significant proportion, exceeding 50% of the environmental impact in three out of six categories (Figure 2). The linear equation system explained 95% of the total mass of the SBMA (see Supplementary material S1B). Therefore, the modelled mass balance was assumed to be sufficient for describing the system. For the remaining minor ingredients, supplementation, seasoning, and binding agent methylcellulose were included in the formulation. Preliminary analysis revealed that the potential iron and vitamin B12 supplementation of the SBMA together were found to contribute below 0.1% and seasonings below 0.5% of all environmental impacts and, thus, were excluded (<1% cut-off). The binding agent carboxymethylcellulose was present in small quantities only but contributed more than 1% to the impact categories CED, GWP100, Water-scar, and Acid-ter. However, processing was more relevant than raw materials to the CED, Water-scar, and the Eutr-fw potential. In addition to the raw materials and the processing of soybeans into soy protein concentrate with subsequent extrusion and potato starch production, rapeseeds for the production of rapeseed oil accounted for the second largest share of the total environmental impact of raw material. Different sources of oil were investigated within an explorative evaluation (not shown) but rapeseed oil with overall highest contributions to environmental impact categories was kept for further investigations. The relevance of raw materials for the total environmental impact was more pronounced for tofu and soy drink (see Supplementary Figure SM1).
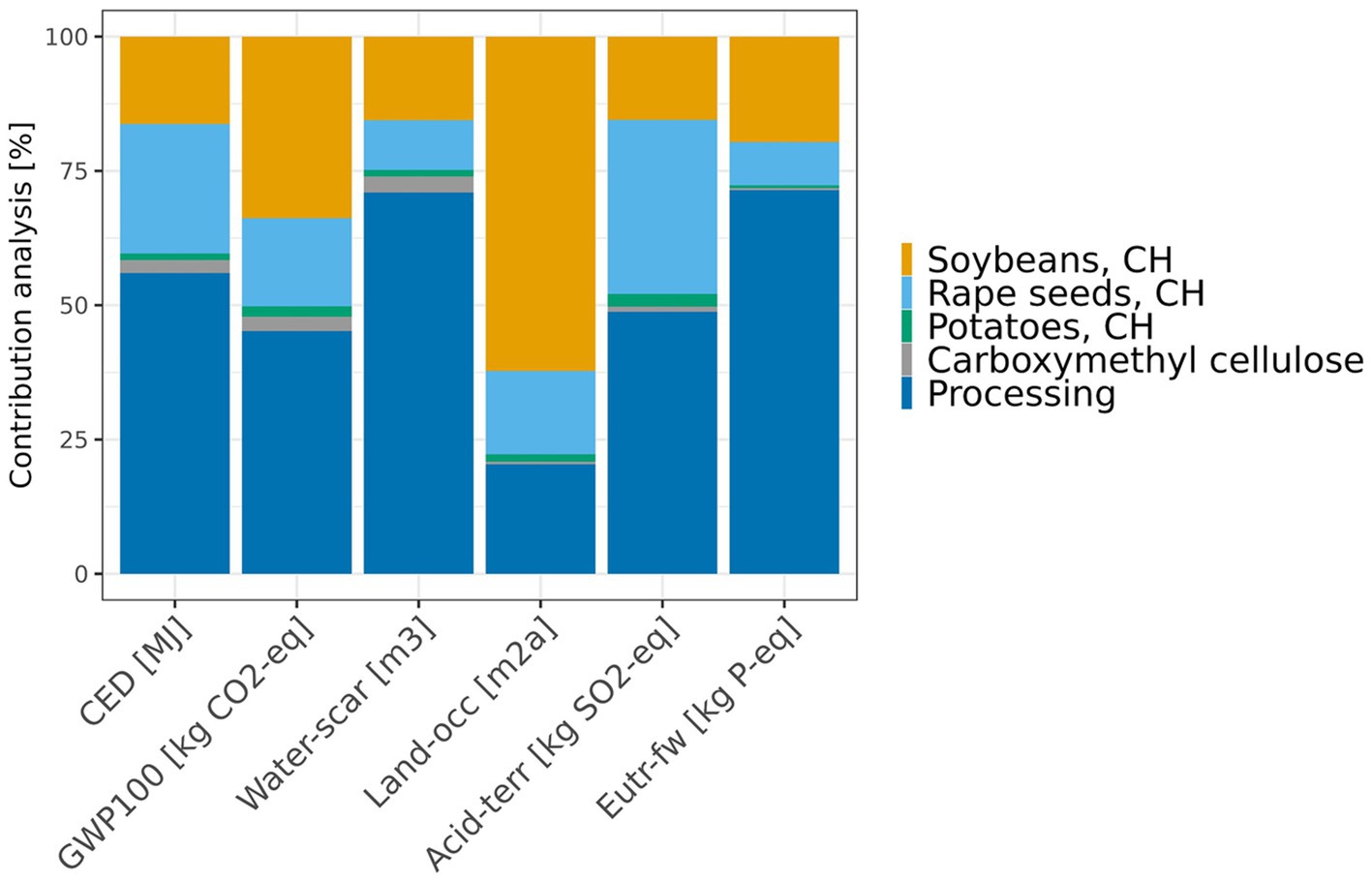
Figure 2. Contribution analysis of the raw materials and processing into the final soy-based meat analogue (SBMA) with selected environmental impact categories: Cumulative energy demand (CED), global warming potential with 100-year time horizon (GWP100), water scarcity potential (Water-scar), land use (Land-occ), terrestrial acidification potential (Acid-terr) and freshwater eutrophication potential (Eutr-fw) in Switzerland.
3.2 Life cycle impact assessment results per 1 g qc-protein
The investigated animal-based products showed DIAAS values greater than 100 with the reference protein amino acid pattern for older children (>3 years), adolescents, and adults, whereas the soy-based options were below 100 (Table 3). This resulted in lower qc-protein than protein content per 100 g of food for the soy-based products. In particular, cooked soybeans had a significantly lower qc-protein. Using the reference values for young children (6 months to 3 years), the DIAAS of the food items were lower overall, but the difference between plant- and animal-derived proteins was more pronounced (Table 3). Although tofu and soy drink reached a DIAAS value of almost 100 (98 and 99) previously, they fell to 84 and 85, respectively.
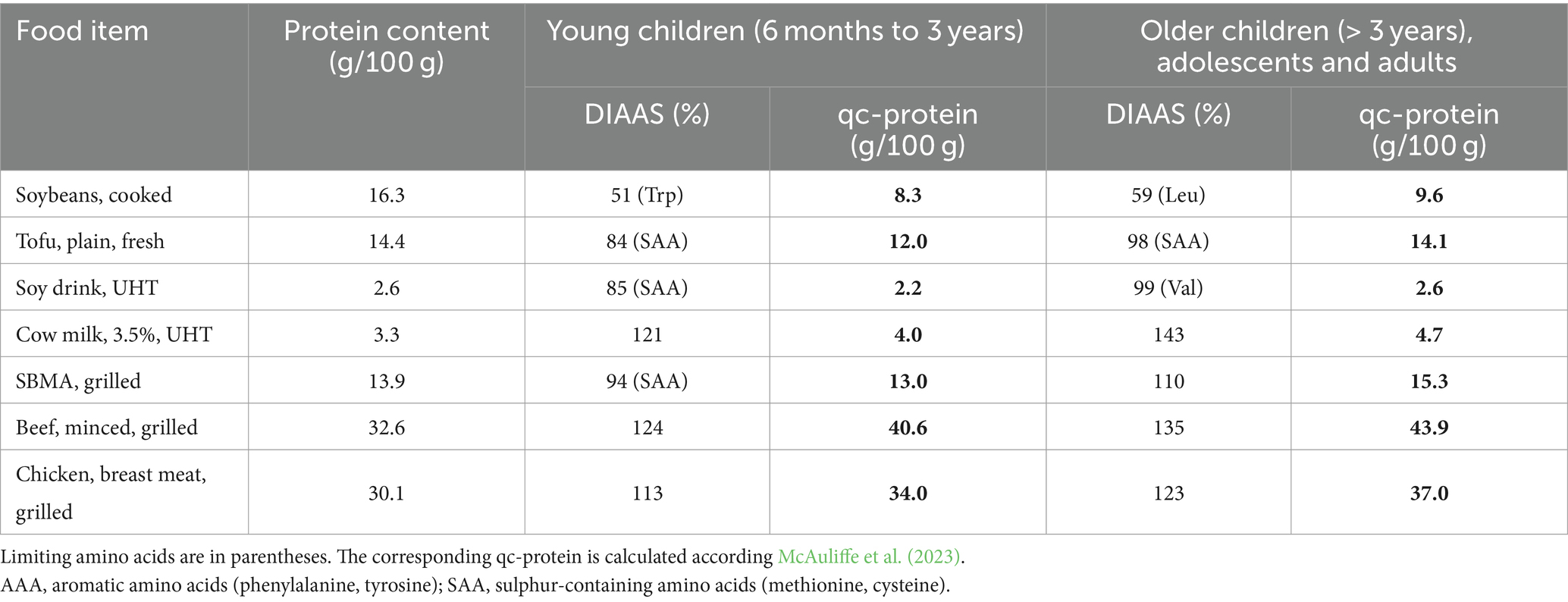
Table 3. Measured protein content, in vitro DIAAS values using the reference requirements for infants (6 months to 3 years) or children >3 years, adolescents and adults, and calculated qc-protein content.
In Figures 3–6, the environmental impacts of the soy-based meat and milk alternatives are normalised to those of beef and cow milk, respectively. Figure 3A provides a detailed comparison of soy-based meat alternatives based on 1 g of qc-protein. This analysis is an expansion of the broader comparison between meat-, dairy-, and soy-based products depicted in Figure 3B. Amongst the soy-based products, SBMA, the most processed option, exceeded the CED, Water-scar, and Eutr-fw, compared to the minimally processed soybeans (cooked soybeans) and tofu. No significant differences were observed between cooked soybeans and tofu. Compared to the reference (beef, minced), all soy-based meat alternatives performed better per gram of qc-protein. This was also true for soy drink. Apart from beef, chicken meat was presented as a meat reference with a lower environmental impact, which outperformed the soy-based products in terms of Land-occ but was worse than beef in terms of Water-scar potential. Contribution analysis showed that the environmental impact of chicken meat depends mainly on the feed composition (Wolff et al., 2016), which can change the result for the better (Water-scar) or even for the worse (Land-occ, GWP100, CED) when other feed sources are considered. In summary, the soy-based meat alternatives performed better than beef, but our modelled SBMA had higher Land-occ than chicken meat and a higher environmental impact compared to the less processed soy-based options. Soy drink was favourable compared to cow milk in all cases.
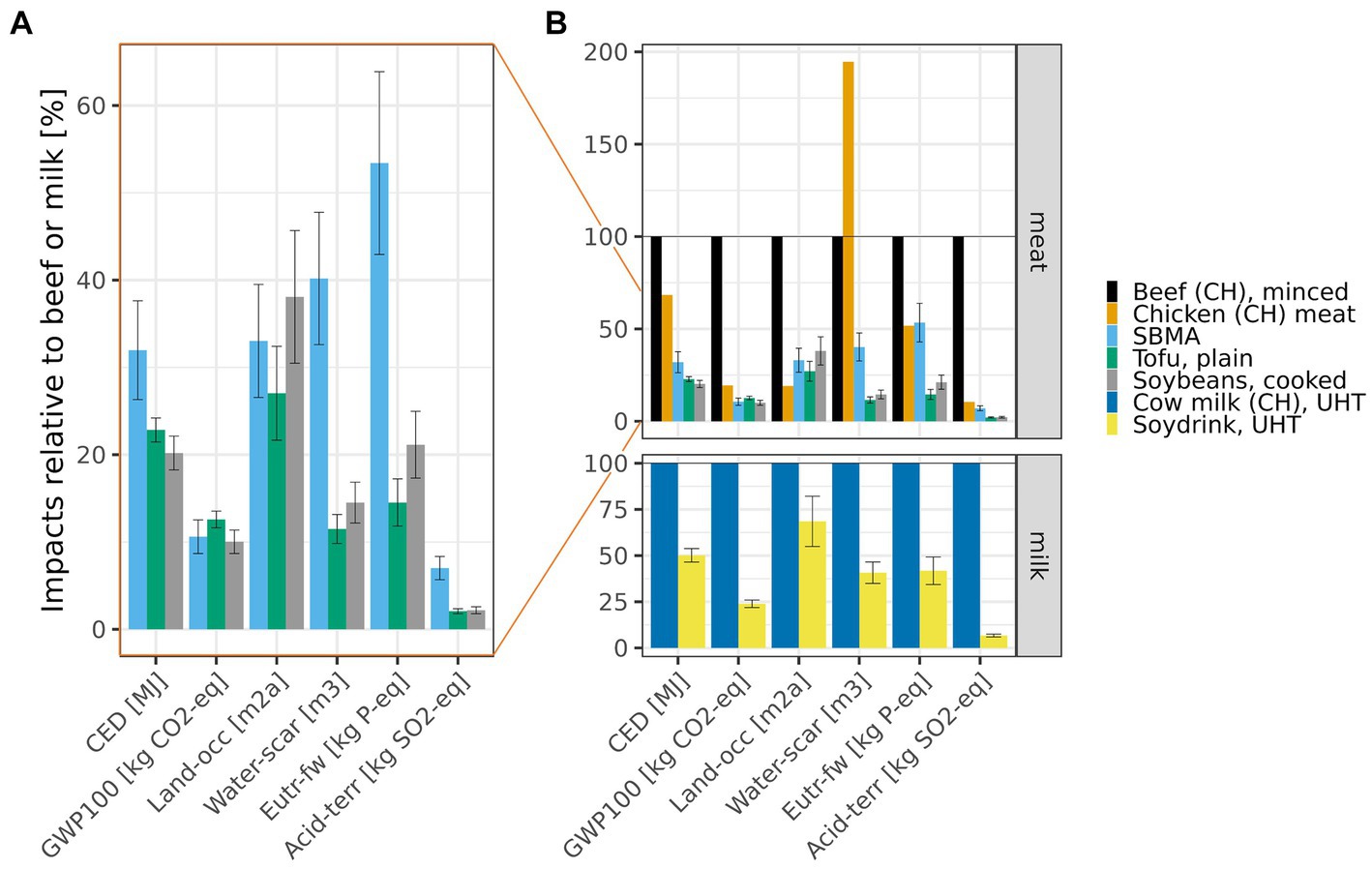
Figure 3. Comparison of selected environmental impact categories per qc-protein (g) between soy-based meat alternatives (A) and between soy-based products and references (B) relative to beef and cow milk. Error bars indicate the results of the sensitivity analysis with +/− 20% raw material input.
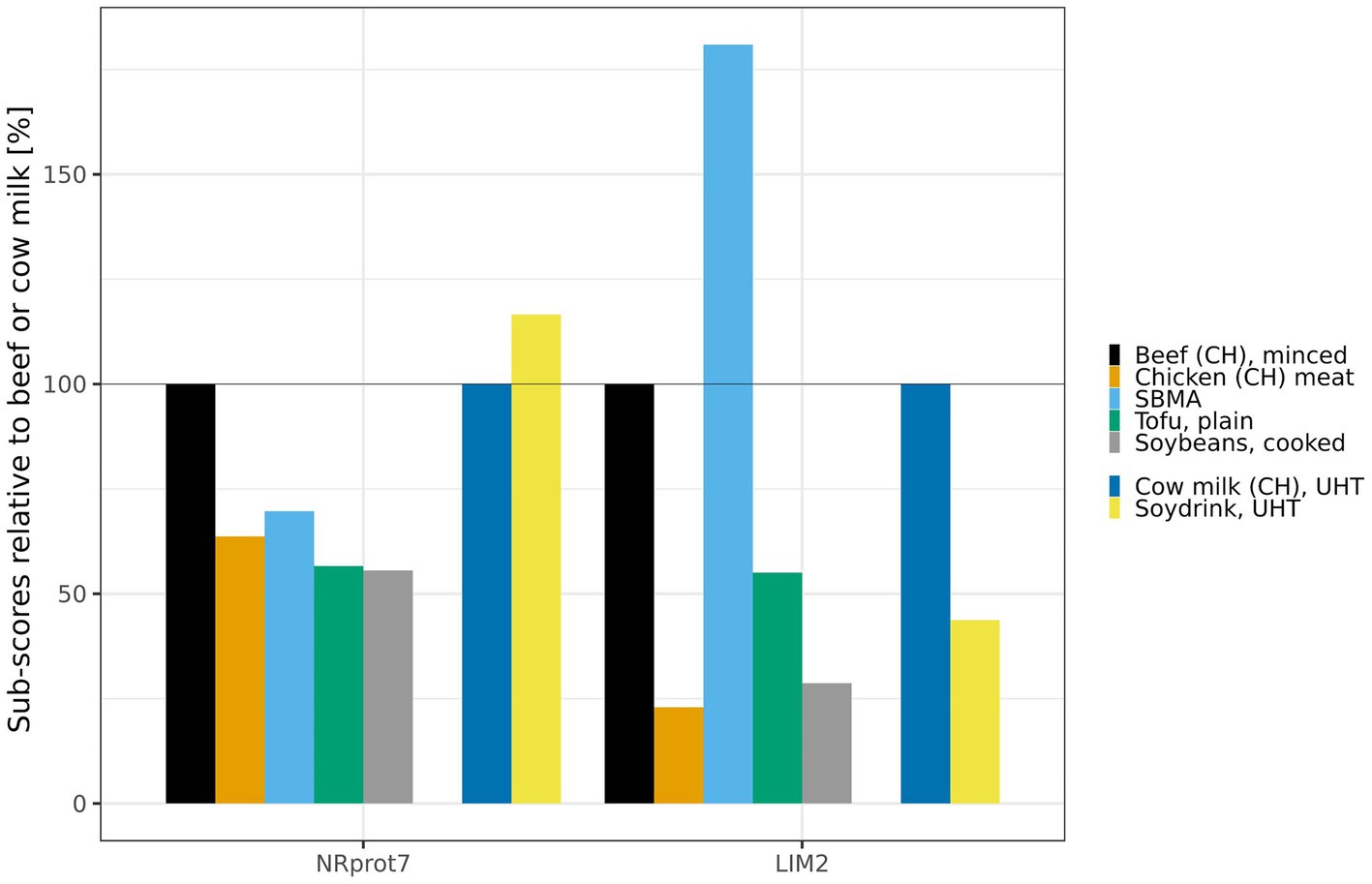
Figure 4. Comparison of the NRF sub-scores per 2,000 kcal relative to beef and cow milk—the SBMA has the highest disqualifying nutrient density score (LIM2) compared to beef, and soy drink has a higher score for beneficial nutrients (NRprot7) than cow milk.
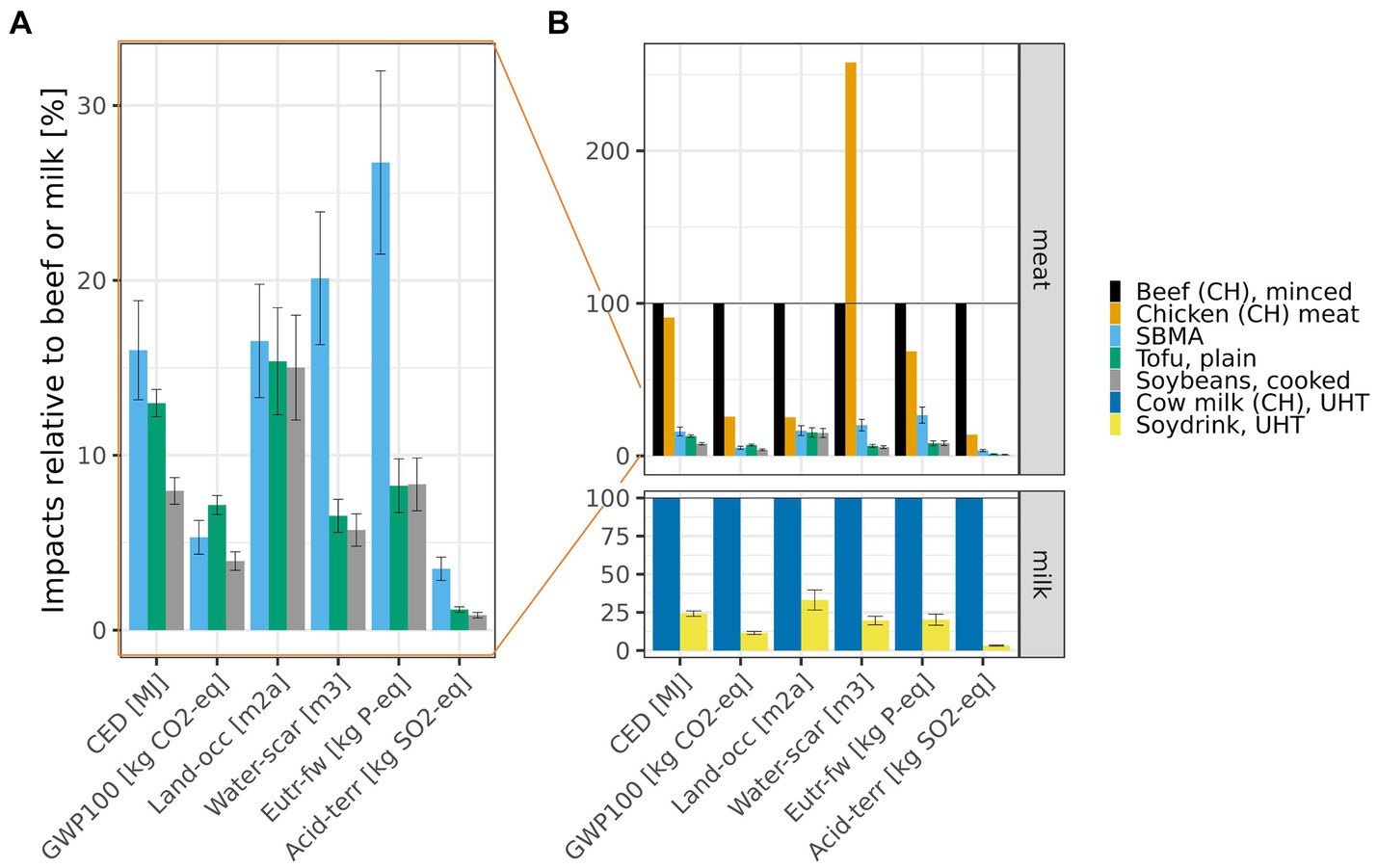
Figure 5. Comparison of selected environmental impact categories per NRprot7, normalised to 2,000 kcal, between soy-based meat alternatives (A) and between soy-based products and references (B) relative to beef and cow milk. Error bars indicate the results of the sensitivity analysis with +/− 20% raw material input.
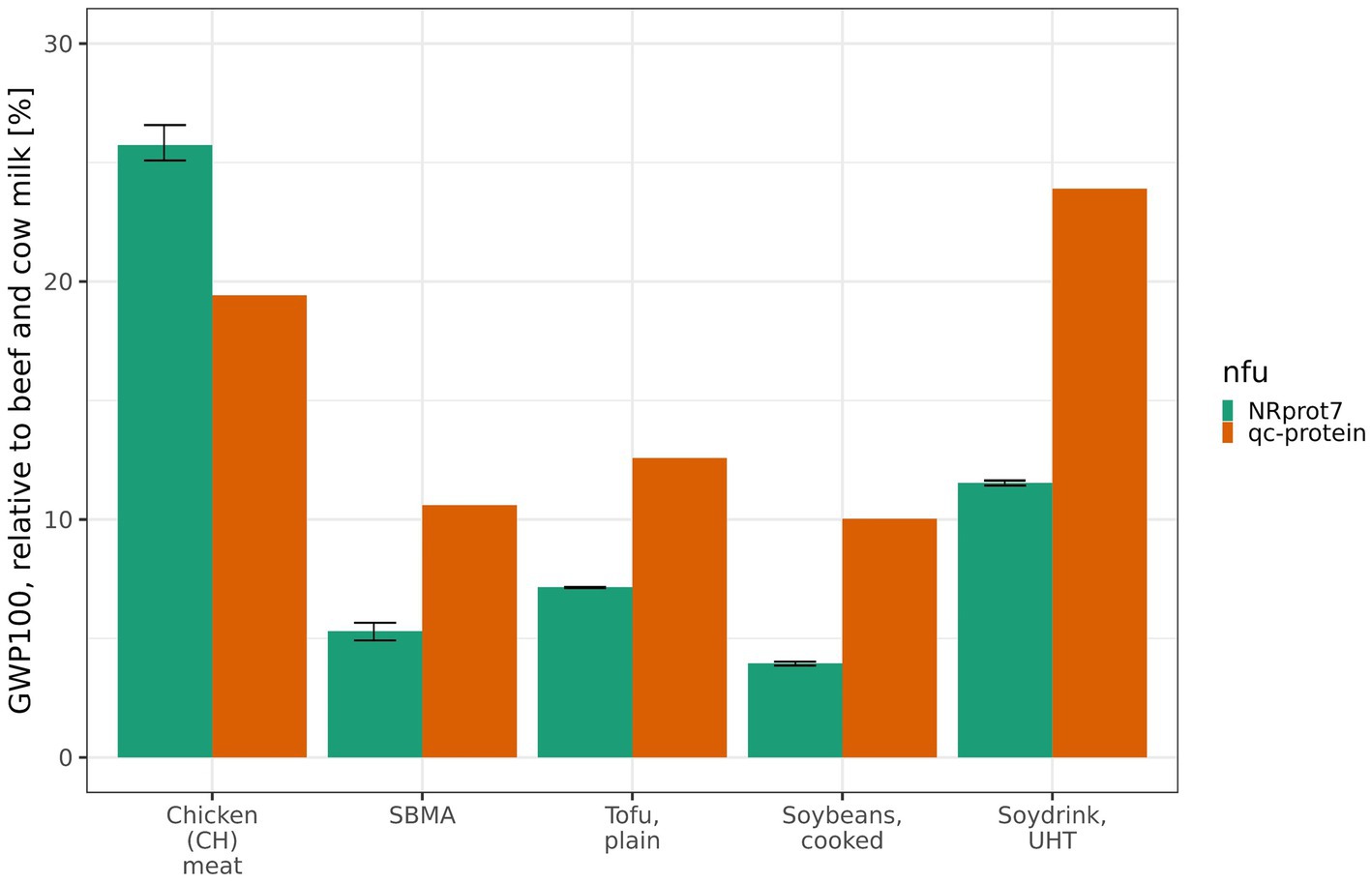
Figure 6. Comparison effect of changing nutritional FU on food items relative to beef and milk for the environmental impact category global warming potential with 100-year time horizon (GWP100). In the case of NRprot7, the error bars represent sex-related differences in RDA.
As the products had additional nutritional value besides protein, the n-LCA was accompanied by a qualitative assessment of other nutrients and total food intake (Table 4). Supplying 1 g of qc-protein required almost twice the intake of soy drink (+80%), more than twice the intake of tofu and SBMA (+266% and +210%), and five times the intake of cooked soybeans. Only chicken meat required 19% more intake than beef. The increased food intake of soy-based alternatives resulted in more calories, more saturated fatty acids, and more sodium, except for the sodium content of cooked soybeans and the total caloric intake in the case of soy drink. However, all of them were high in fibre, and the soy-based meat alternatives had higher levels of calcium and iron than the meat references (beef, minced). The zinc content was between chicken and beef. Soy drink was high in fibre, iron, and zinc, but not in calcium. All the soy-based alternatives lacked vitamin B12. In animal-based products, vitamin B12 was present in small amounts, and cow milk was high in calcium, whereas fibre was completely absent in meat and milk.
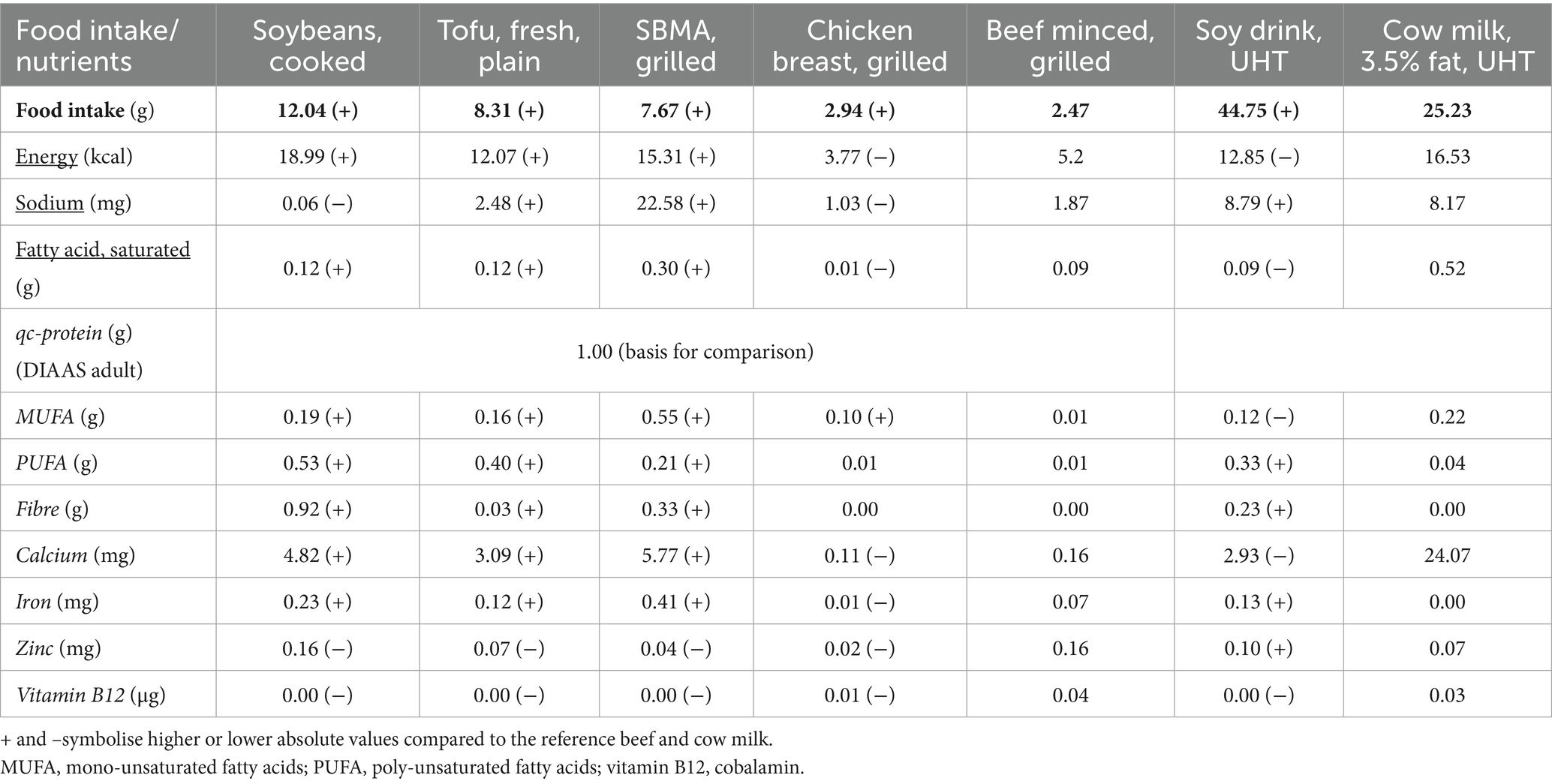
Table 4. Comparison of food amounts, needed to supply 1 g of qc-protein (in bold) and their corresponding nutrient supply of soy-based alternatives and animal-based references highlights higher amounts of beneficial (in italics) and disqualifying (underlined) nutrients for soy-based alternatives.
Lower environmental impact came at the cost of higher caloric intake in the case of soy-based meat alternatives but not for soy drink. Despite higher food intake, soy drink was lower in both, environmental impact and calories, suggesting a win–win situation. Although soy drink may be lower in environmental impact, but unfortified, it was worse as a calcium source compared to cow milk. Soy-based meat alternatives were higher in calcium and lower in environmental impact compared to the animal-based references. Beef and chicken meat were lower in monounsaturated fatty acids (MUFA) and polyunsaturated fatty acids (PUFA) and lower in saturated fatty acids compared to the soy-based meat alternatives. Soy drink was favourable for saturated fat and PUFA but not for MUFA compared to the reference. A final summary of the life cycle impact assessment results per gram of qc-protein combined with other nutrients remains difficult. To address this complexity, a second nutritional FU was applied to evaluate the environmental impact in relation to the RDA of all the nutrients considered in this study.
3.3 Life cycle impact assessment results per 1 NRprot7
Based on the function of food to provide sufficient nutrients, it can be argued that the comparison should not only consider multiple nutrients but also the corresponding food intake or caloric intake. Therefore, the soy-based alternatives were compared with meat and milk on the basis of their nutrient density, normalised to 2,000 kcal. The meat reference, beef, had the highest beneficial sub-score, whilst for the milk reference, this was not the case (Figure 4). Additionally, soy drink was lower in sodium and saturated fatty acid content than cow milk, as described in the LIM2 sub-score. Amongst the plant-based meat alternatives, only tofu and cooked soybeans showed lower LIM2 scores than beef, although not lower than chicken meat. Whereas tofu and cooked soybeans were low in sodium and saturated fat compared to beef, SBMA was higher in these disqualifying nutrients. The higher density of disqualifying nutrients in SBMA should be taken into consideration when assessing the overall evaluation of this product.
Considering all the nutrients from Table 4 to calculate NRprot7 per 2,000 kcal, the second FU now encompassed seven favourable nutrients instead of just one. In this evaluation, soy-based products, including SBMA, emerged as more efficient in nutrient provision whilst minimising environmental impact compared to the references and chicken meat (Figure 5B). Amongst the soy-based meat alternatives, the less processed options held an advantage in the environmental impact categories Water-scar, Eutr-fw, and Acid-terr, compared to SBMA, and cooked soybeans demonstrated one of the lowest GWP100 (Figure 5A). Again, soy drink as an alternative to milk performed better in all environmental impact categories compared to cow milk.
3.4 Implications of changing FU
Comparing the results of the two nutritional FUs (3.2, 3.3), it is clear that altering the FU resulted in significant shifts in the environmental impact assessment. This is illustrated by the GWP100 as a representative example of all environmental impact categories (Figure 6). In the transition from qc-protein to NRprot7 as FU, the GWP100 decreased for soy-based products in comparison to beef meat and milk, whilst it increased for chicken meat. Compared to the qc-protein supply only, animal-based products were less efficient in providing other beneficial nutrients; thus, the overall nutritional evaluation, with the exclusion of the disqualifying nutrients, was in favour of the soy-based products. The error bars on GWP100, depicted in Figure 6 for NRprot7, indicate the fluctuation of the sub-score across different sex reference values. Nevertheless, the overall variance was minor compared to the change in FU.
3.5 Sensitivity analysis
3.5.1 Soybeans from Brazil
Apart from the sensitivity of the raw materials input, which is shown in Figures 3, 5, the second sensitivity analysis delved into the origin of the raw materials. In this scenario, the origin of soybeans shifted to Brazil. The contribution analysis revealed that across all environmental impact categories except Eutr-fw, the raw material contribution to the total environmental impact of the soy-based products was greater than in the Swiss scenario (see Supplementary Figures SM2, SM3). In the assessment utilising the first nutritional FU (1 g of qc-protein), analogous conclusions were drawn regarding the comparison between beef and the soy-based meat alternatives, although not for chicken meat (see Supplementary Figure SM4). Compared to chicken meat, the Land-occ of the soy-based products decreased to the same range of chicken meat for SBMA and below for tofu and cooked soybeans. Besides these favourable changes, the GWP100 of all soy-based meat alternatives increased to the level of chicken meat. In the case of SBMA, Eutr-fw also rose to the level of chicken meat. The most significant shift occurred in the Water-scar for soy drink, which reached nearly twice (187%) the value of cow milk. In the Brazilian scenario, the findings from the assessment utilising the second FU aligned with those observed in the base scenario (see Supplementary Figure SM5). Hence, the increased Water-scar of soy drink in this scenario was offset by the overall alternation due to the change in FU, resulting in a lower (81%) Water-scar compared to cow milk.
3.5.2 Reference values: DIAAS and NRF calculated for young children
In the third sensitivity analysis, the reference values for the calculation of the DIAAS, as well as the subsequent qc-protein and NRprot7 calculations, were shifted to the requirements of young children, according to FAO recommendations (Food and Agricultural Organization of the United Nations, 2013) for DIAAS calculations, as well as RDA for young children (1 to 3 years) for NRF calculations. These adjustments elevated the total NRprot7 score (see Supplementary Table SM1) and reduced the DIAAS overall, consequently reducing the qc-protein of the products under investigation (Table 3). These effects on the n-LCA were factored into this final scenario, and the results are presented in Supplementary Figures SM6, SM7. The results of the n-LCA did not deviate significantly from the conclusions drawn from the base scenario regarding qc-protein (Figure 3) and NRprot7 (Figure 5), nor did the alteration of the RDA for young children yield significant changes in the nutritional quality assessment (see Supplementary Table SM2). Overall, no effects on the ranking due to age-dependent requirements were found.
4 Discussion
4.1 Environmental and nutritional aspects of soy-based alternatives
In terms of the environmental impact on a mass basis, the production of plant proteins appeared to be more efficient to that of animal proteins (Supplementary material S4). Due to their higher trophic level, animals have a greater need to maintain and regulate body functions, in addition to body mass production, and therefore produce higher emissions compared to plants (Poore and Nemecek, 2018). However, the nutritional quality of animal-based products can be higher, as seen in the case of proteins (Table 3). In this study, the benefits and trade-offs between soy-based meat alternatives with different levels of processing, alongside soy drink, and their animal-based counterparts were explored. The cooked soybeans, tofu, and SBMA were less nutrient dense than beef but not chicken meat (Figure 4). The high protein content and protein quality in chicken meat did not compensate enough for the absence of other beneficial nutrients, such as dietary fibre and (poly-)unsaturated fatty acids. Although SBMA may also contained elevated levels of beneficial nutrients and high-quality protein, it also exhibited high quantities of saturated fatty acids and sodium. As seen in the qualitative nutrient assessment, the processed soy-based meat alternatives had higher caloric content per g of qc-protein than their references and should therefore be consumed moderately in a holistic diet to prevent weight gain. This also indicated an advantage of minimally processed products over processed ones. With the inclusion of multiple nutrients and RDA, as in the second nutritional FU, the environmental impact was found to be lower for soy-based meat and milk alternatives than for beef and cow milk (Figure 5). The lower environmental impact of soy drink compared to cow milk was confirmed in both assessments (Figures 3, 5), whereas comparing only the soy-based meat alternatives to chicken meat, the results were nuanced. Considering the provision of high-quality protein only, chicken meat seemed to be in the same range as SBMA. The conversion of plant proteins into high-quality animal proteins is highly efficient in chicken, as seen in the generally better performance than beef. Only in the second assessment, which included nutrient density, did the SBMA perform better than chicken meat, but different nutrient density calculations could have affected the results. Given the low calcium contents in soy drink and high LIM2 values in SBMA, increased efforts to limit disqualifying nutrients in the SBMA and to implement fortification in SBMA and soy drink, where necessary, could have potentially affected the ranking substantially. Nevertheless, the preference for soy-based products remained intact when the sensitivity analysis applied +/− 20% raw material input for all scenarios, and the choice of nutritional FU in this case study did not result in a different classification of the products overall. In the scenario with soybeans sourced from Brazil, higher emissions from land use and land use change and longer transport routes were detected, but this did not affect the overall hypothesis, with three exceptions. First, the GWP100 of tofu rose to the level of chicken meat. Second, the Water-scar impact category of soy drink rose above that of milk from Swiss cows (see Supplementary Figure SM4, SM5). Third, the Land-occ of soy-based meat alternatives was reduced compared to the Swiss scenario. The higher land use in the case study was mainly caused by a lower yield in soybean production in Switzerland compared to the one on a global scale. Changing the origin of soybeans, the contribution of raw material also increased in all environmental impact categories, except Eutr-fw (see Supplementary Figures SM2, SM3). Therefore, if eutrophication into freshwater can be minimised and space is available, locally sourced soybeans are favoured over imports from Brazil. Finally, for regulatory purposes, the FAO recommends the reference amino acid pattern for young children (6 months to 3 years) for all foods (Food and Agricultural Organization of the United Nations, 2013). Applying this regulation, no effects on ranking were observed. Further, adjusting the RDA for young children (1–3 years) in the NRprot7 index did not offer new insights, and the results can therefore be considered valid for children and adults (see Supplementary Figures SM6, SM7). Nevertheless, population groups at risk for certain nutrient deficiencies, such as the elderly or pregnant women, should take the findings with caution.
Herreman et al. (2020) described the possibility of enhancing DIAAS from plant sources by complementing different plant proteins, such as potato, soy, and pea. This is in line with the findings that cooked soybeans and tofu reached lower DIAAS and lower qc-protein compared to the SBMA. It remains unclear whether the SBMA sample contained other minor protein sources besides soy protein (e.g., potato protein in potato starch), but the enhanced measured DIAAS compared to the intermediate soy protein concentrate (Sousa et al., 2023a) supported this assumption. By comparing different PBMAs and hypothetical DIAAS calculations, Van Mierlo et al. (2022) showed a beneficial effect on DIAAS and on environmental impact when mixing different ingredients. Furthermore, the simple qc-protein calculations as FU allow us to (re-)calculate results from other studies for benchmarking purposes. In this case study, soy-based alternatives were highly efficient in reducing the contribution of food to climate change by having a GWP100 between 10 and 25% of their respective references, beef and milk (Figure 3). Using data from Poore and Nemecek (2018) and DIAAS from various studies, McAuliffe et al. (2023) found similar values for GWP100 (16.0% compared to their reference product, beef from dairy cattle) and land use (13.6% of beef) in the case of tofu. This is in line with our findings for tofu, with 12.5 and 27.0% for GWP100 and Land-occ, respectively. We found no similar approach for soy drink, as the DIAAS of soy drink used here is amongst the first published (Hammer et al., 2024). A scoping review by Berardy et al. (2022) reported a mean GWP100 value between 0.4 and 1.0 kg CO2-eq per litre of soy drink. In this case study, values between 0.17 and 0.33 kg CO2-eq per litre, assuming the density of cow milk (1.01 kg/m3) for simplicity, were found. It should be noted that this case study set the system boundaries as cradle-to-gate, whereas values from Berardy et al. (2022) included packaging, (cold) distribution, use phase, and the end-of-life aspects at least partly. Finally, to benchmark the SBMA model, the absolute values for GWP100, Land-occ, and CED on a per kg of qc-protein basis were compared with the environmental impact of two commercially available PBMAs, Impossible Burger (IP) from Impossible Foods and Beyond Burger (BB) from Beyond Meat. In an explorative study, we combined data from Heller and Koleian (2018) for BB and Khan et al. (2019) for IP with an in vivo DIAAS evaluation of these burgers (Fanelli et al., 2022). GWP100 and Land-occ were assessed in both, but only the study of Heller and Koleian (2018) reported the impact category CED. Table 5 shows the environmental impact categories per kg of qc-protein of IP and BB and the SBMA from this case study. They were compared with the beef production system in Switzerland.
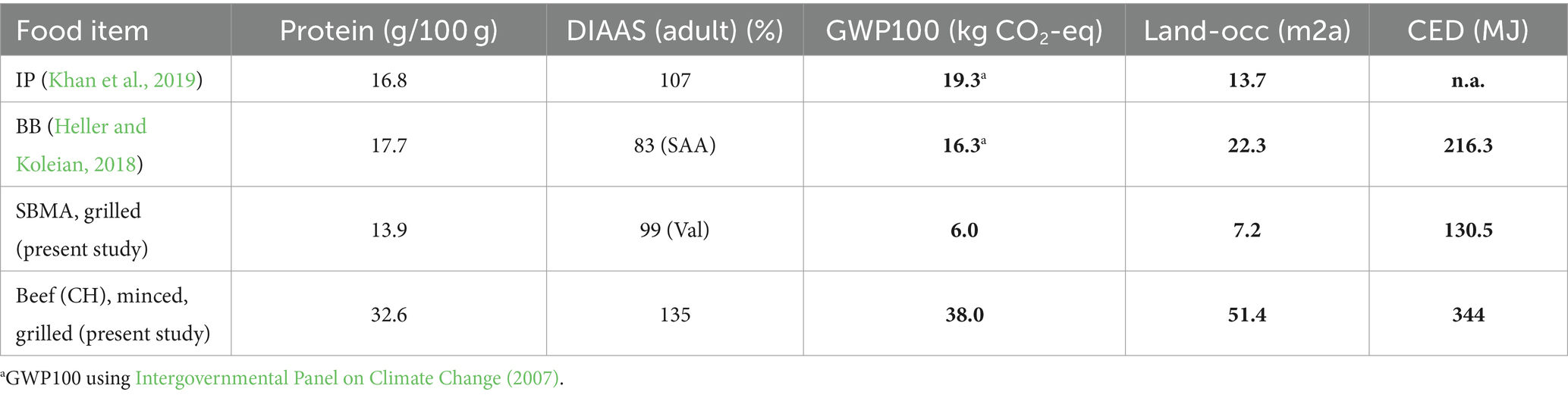
Table 5. Global warming potential with 100-year time horizon (GWP100), land use (Land-occ), and cumulative energy demand (CED) of commercial PBMAs per qc-protein (kg) and corresponding protein content and DIAAS values—in comparison with SBMA and beef from the current case study.
All PBMAs were below the beef reference in terms of environmental impact per kg of qc-protein, protein content and DIAAS, again highlighting the high environmental impact of beef despite its high protein quantity and quality. The SBMA of the case study showed a lower environmental impact compared to IP and BB. Regarding protein quality, the DIAAS of the SBMA was in the range of IP and BB. It should be noted that the protein source of BB is pea protein concentrate and rice, which generally has a lower protein quality. In Van Mierlo et al. (2022), a soy-burger was modelled using IAA content and digestibility data from the literature, resulted in a DIAAS, with a reference amino acid pattern for growing children (>3 years), adolescents, adults, of 95 (Lys) and a qc-protein of 15.4 g/100 g food. They reported a GWP100 of 13 kg CO2-eq per kg qc-protein and a Eutr-fw potential of 5.7 × 10−3 kg P-eq using ReCiPe 2008 (Goedkoop et al., 2009). Their results lied between the commercial burgers IP and BB and the SBMA in the present study (6.0 kg CO2-eq and 2.0 × 10−3 kg P-eq). The results of the life cycle impact assessment for the SBMA were, in general, lower than those reported in the literature for similar products (Table 5). The lower environmental impact could be associated with the use of different production systems and geographic scope (background data were from Swiss production mix or from European production) or higher scale technologies applied. The composition of the recipes also plays an important role. Interestingly, contribution analysis of IPs revealed that the highest contribution to GWP100 from ingredients only was the production of leghemoglobin (13%) derived from genetically modified yeast, followed by textured soy protein concentrate (11%) and sunflower oil (7%) (Khan et al., 2019). Thus, additives and supplements may contribute significantly to novel PBMAs. As the nutritional status of the SBMA in this case study indicated iron supplementation, more focus should be placed on evaluating these minor ingredients in the future, but secondary data were missing or concealed due to property rights, as in the case of the leghemoglobin process for the IP. Despite all the variations, the life cycle impact assessment results of the current study are applicable to the relative comparison of different plant-based products.
Finally, despite all the nutritional and environmental debates about different foods, plant-based products are still far too rare on plates of Swiss consumers in general. The Eat-Lancet Commission recommends 75 grams of pulses per person per day (Willett et al., 2019), but according to the national nutrition survey “MenuCH,” the average Swiss in 2014 has eaten just about nine grams per day (Bundesamt für Lebensmittelsicherheit und Veterinärwesen, 2014). As seen in this case study, higher amounts of cooked soybeans and soy-based products are needed to obtain the same amount of high-quality protein as animal-based products. Therefore, compared to current consumption patterns of minimally processed plant-based products, a shift to a more sustainable diet from a nutritional and environmental perspective will require much greater effort in terms of dietary change than is currently the case in Switzerland. The processed soy-based alternatives in this case study could help promote the replacement of animal-based products in the Swiss diet, and if disqualifying nutrients are managed, we consider them to be at least a part of the overall protein shift process.
4.2 Limitations and further research
Here, individual products were evaluated for their environmental impact and nutrient content, including DIAAS. However, foods are not consumed alone; therefore, the DIAAS should be calculated for whole meals, which could have changed the results. Further, the minerals evaluated in this study were not corrected for their bioavailability. Primary data, as assessed in this study for protein quality and digestibility, can be considered the gold standard for this scope. If not proportionate to the overall effort, data from similar foods could be used as a proxy to provide a more detailed resolution. Moreover, diet-level comparisons should be enforced to understand the impact of missing nutrients or high levels of disqualifying nutrients in a broader sense. We consider food n-LCA, at least at the meal level with multiple single products, as a way of capturing the complex multi-dimensionality of agri-food sustainability assessments, but a single product study as described here can provide further data for this approach.
The modelled SBMA captured the overall environmental impact of novel PBMAs. Nevertheless, the effects of food-grade supplementation could be assessed more precisely. The data for tofu and soy drink are available in life cycle inventory databases. However, the SBMA model is based on secondary data only, and food-grade supplements are completely missing. Here, future n-LCA could collect primary data for additional novel plant-based alternatives and supplementation to provide benchmarking in this area. Therefore, reference flows with a mass-based FU, besides nutritional information, must be provided thoroughly. As the NRF is an unweighted average of many nutrients, information about critical nutrients may be lost. In particular, when evaluating diets or meals in a food LCA, mass-based FU is favoured, but environmental impact should be assessed in line with covering the daily requirements for all nutrients through optimisation models or similar. Finally, to advance future n-LCA of plant-based alternatives to meat milk and help the protein shift as a whole, behavioural research (van den Boom et al., 2023) and the socio-economic perspective (Varela-Ortega et al., 2021) is needed alongside environmental and nutritional assessments.
5 Conclusion
The drive towards sustainable food systems has stimulated the development of novel plant-based alternatives to traditional animal-based products. Plant-based meat analogues and dairy alternatives have emerged as important players in this transition, but the current market development requires a holistic assessment from a nutritional and environmental perspective to explore the potential benefits and trade-offs they can contribute to a dietary change. This paper aims to fill this gap with a case study that simultaneously assessed the nutritional and environmental value of meat and milk alternatives at different processing stages. Including nutrient quality, this assessment considered cooked soybeans and tofu to be low and medium quality protein sources respectively, whilst the processed soy-based meat analogue was optimised for protein quantity and quality but had a higher level of disqualifying nutrients. Although protein quality increased with the level of processing, the overall nutrient density score showed that minimally processed soy and tofu were the most favourable meat alternatives. The LCA on a mass basis showed that the environmental impact of all soy-based alternatives was 4–20 times lower than that of beef, especially when locally sourced soy was used. The differences were smaller when compared to chicken meat. Amongst the soy-based alternatives, the environmental impact increased with processing. Despite the high level of processing, the raw material contributed significantly to the environmental impact of the soy-based meat analogue, exceeding 50% in several impact categories. The n-LCA of soy-based meat alternatives suggested that despite their low protein quality, the minimally processed plant proteins examined in this case study offered a promising approach to reducing the environmental impact of a diet whilst providing essential nutrients. In addition, the results indicated that soy drink had more beneficial nutrients combined with a lower disqualifying sub-score than the reference product whilst incurring dramatically smaller environmental impacts. The combined assessment of soy drink resulted in an environmental impact 4–50 times lower than that of cow milk, even when the higher nutrient content of cow milk was included in the calculations, showing an interesting win–win situation. However, consumer acceptance, an important success factor for sustainable market development, was not considered and requires further research.
Data availability statement
The original contributions presented in the study are included in the article/Supplementary material, further inquiries can be directed to the corresponding author.
Author contributions
MH: conceptualisation, data curation, formal analysis, investigation, methodology, project administration, validation, visualisation, writing – original draft, writing – review & editing. EM: Conceptualisation, Data curation, Methodology, Validation, Writing – review & editing, Investigation. LE: Conceptualisation, Data curation, Writing – review & editing. RP: Conceptualisation, Data curation, Writing – review & editing, Visualisation. LH: Data curation, Formal analysis, Validation, Writing – review & editing. TN: Funding acquisition, Project administration, Resources, Supervision, Writing – review & editing, Validation.
Funding
The author(s) declare that financial support was received for the research, authorship, and/or publication of this article. This work is supported by the OptiSignFood project, which received funding from the European Union’s Horizon 2020 Framework Programme under Grant Number 971242. Open access funding by Agroscope.
Acknowledgments
Acknowledgements are due to Cédric Furrer for help with SimaPro software and Dr. Alba Reguant Closa for their insights into nutrition, as well as to the rest of the Life Cycle Assessment research group for their interest and support. We would also like to thank the reviewers for their encouraging and constructive feedback and to the editors for facilitating the publication of this work.
Conflict of interest
The authors declare that the research was conducted in the absence of any commercial or financial relationships that could be construed as a potential conflict of interest.
Publisher’s note
All claims expressed in this article are solely those of the authors and do not necessarily represent those of their affiliated organizations, or those of the publisher, the editors and the reviewers. Any product that may be evaluated in this article, or claim that may be made by its manufacturer, is not guaranteed or endorsed by the publisher.
Supplementary material
The Supplementary material for this article can be found online at: https://www.frontiersin.org/articles/10.3389/fsufs.2024.1413802/full#supplementary-material
Abbreviations
DIAAS, Digestible indispensable amino acid score; NRprot7, Beneficial nutrient density sub-score; PBMA, Plant-based meat analogue; GWP, Global warming potential; qc-protein, Quality-corrected protein content; SBMA, Soy-based meat analogue (focus PBMA in this case study)
References
Adhikari, S., Schop, M., de Boer, I. J. M., and Huppertz, T. (2022). Protein quality in perspective: a review of protein quality metrics and their applications. Nutrients 14:947. doi: 10.3390/nu14050947
Alig, M., Grandl, F., Mieleitner, J., Nemecek, T., and Gaillard, G. (2012). Ökobilanz von Rind-, Schweine- und Geflügelfleisch. Available at: https://ira.agroscope.ch/de-CH/Page/Einzelpublikation/Download?einzelpublikationId=37100
Asselin-Balençon, A., Broekema, R., Teulon, H., Gastaldi, G., Houssier, J., Moutia, A., et al. (2022). “AGRIBALYSE 3: la base de données française d’ICV sur l’Agriculture et l’Alimentation” in Methodology for the food products. ADEME, Angers
Berardy, A., Johnston, C. S., Plukis, A., Vizcaino, M., and Wharton, C. (2019). Integrating protein quality and quantity with environmental impacts in life cycle assessment. Sustainability 11:2747. doi: 10.3390/su11102747
Berardy, A. J., Rubin-Garcia, M., and Sabate, J. (2022). A scoping review of the environmental impacts and nutrient composition of plant-based milks. Adv. Nutr. 13, 2559–2572. doi: 10.1093/advances/nmac098
Besson, T., Bouxom, H., and Jaubert, T. (2020). Halo It’s Meat! The effect of the vegetarian label on calorie perception and food choices. Ecol. Food Nutr. 59, 3–20. doi: 10.1080/03670244.2019.1652820
Boulay, A.-M., Bare, J., Benini, L., Berger, M., Lathuillière, M. J., Manzardo, A., et al. (2018). The WULCA consensus characterization model for water scarcity footprints: assessing impacts of water consumption based on available water remaining (AWARE). Int. J. Life Cycle Assess. 23, 368–378. doi: 10.1007/s11367-017-1333-8
Brodkorb, A., Egger, L., Alminger, M., Alvito, P., Assunção, R., Ballance, S., et al. (2019). INFOGEST static in vitro simulation of gastrointestinal food digestion. Nat. Protoc. 14, 991–1014. doi: 10.1038/s41596-018-0119-1
Bundesamt für Landwirtschaft (2021). Report zum Schweizer Fleischersatzmarkt, BLV Fachbereich Marktanalysen : Bundesamt für Landwirtschaft (BLW) Available at: https://www.blw.admin.ch/blw/de/home/markt/marktbeobachtung/land--und-ernaehrungswirtschaft/fleischersatz.html.
Bundesamt für Lebensmittelsicherheit und Veterinärwesen (2014). menuCH-Nationale Ernährungserhebung : Bundesamt für Lebensmittelsicherheit und Veterinärwesen (BLV) Available at: https://www.blv.admin.ch/blv/de/home/lebensmittel-und-ernaehrung/ernaehrung/menuCH.html.
Bundesamt für Lebensmittelsicherheit und Veterinärwesen (2022). Schweizer Referenzwerte für die Nährstoffzufuhr : Bundesamt für Lebensmittelsicherheit und Veterinärwesen (BLV) Available at: https://www.blv.admin.ch/blv/de/home/lebensmittel-und-ernaehrung/ernaehrung/empfehlungen-informationen/naehrstoffe/naehrstoffzufuhr-dynamische-tabelle.html.
Bundesamt für Lebensmittelsicherheit und Veterinärwesen (2023). Schweizer Nährwertdatenbank : Bundesamt für Lebensmittelsicherheit und Veterinärwesen (BLV) Available at: https://naehrwertdaten.ch/.
Bystricky, M., Nemecek, T., and Gaillard, G. (2014). Ökobilanz ausgewählter Schweizer Landwirtschaftsprodukte im Vergleich zum Import (2296-729X). Zürich: Agroscope Science Available at: https://ira.agroscope.ch/de-CH/publication/34923.
Clark, M. A., Domingo, N. G. G., Colgan, K., Thakrar, S. K., Tilman, D., Lynch, J., et al. (2020). Global food system emissions could preclude achieving the 1.5 degrees and 2 degrees C climate change targets. Science 370, 705–708. doi: 10.1126/science.aba7357
Coop. (2023). Studie über pflanzenbasierte Ernährung in der Schweiz. Available at: https://www.coop.ch/content/dam/insieme/plantbased-report-2023/Coop-Plant-Based-Food-Report-2023_D.pdf
Cordova, R., Viallon, V., Fontvieille, E., Peruchet-Noray, L., Jansana, A., Wagner, K.-H., et al. (2023). Consumption of ultra-processed foods and risk of multimorbidity of cancer and cardiometabolic diseases: a multinational cohort study. Lancet Reg. Health Eur. 35:100771. doi: 10.1016/j.lanepe.2023.100771
Crippa, M., Solazzo, E., Guizzardi, D., Monforti-Ferrario, F., Tubiello, F. N., and Leip, A. (2021). Food systems are responsible for a third of global anthropogenic GHG emissions. Nat. Food 2, 198–209. doi: 10.1038/s43016-021-00225-9
Douziech, M., Bystricky, M., Furrer, C., Gaillard, G., Lansche, J., Roesch, A., et al. (2024). Recommended method for impact assessment within the Swiss Agricultural Life Cycle Assessment (SALCA) v2.01. Agroscope Science 183. doi: 10.34776/as183e
Drewnowski, A., Rehm, C. D., Martin, A., Verger, E. O., Voinnesson, M., and Imbert, P. (2015). Energy and nutrient density of foods in relation to their carbon footprint. Am. J. Clin. Nutr. 101, 184–191. doi: 10.3945/ajcn.114.092486
Ernstoff, A., Stylianou, K. S., Sahakian, M., Godin, L., Dauriat, A., Humbert, S., et al. (2020). Towards win–win policies for healthy and sustainable diets in Switzerland. Nutrients 12:2745. doi: 10.3390/nu12092745
Fanelli, N. S., Bailey, H. M., Guardiola, L. V., and Stein, H. H. (2021). Values for Digestible Indispensable Amino Acid Score (DIAAS) determined in pigs are greater for milk than for breakfast cereals, but DIAAS values for individual ingredients are additive in combined meals. J. Nutr. 151, 540–547. doi: 10.1093/jn/nxaa398
Fanelli, N. S., Bailey, H. M., Thompson, T. W., Delmore, R., Nair, M. N., and Stein, H. H. (2022). Digestible indispensable amino acid score (DIAAS) is greater in animal-based burgers than in plant-based burgers if determined in pigs. Eur. J. Nutr. 61, 461–475. doi: 10.1007/s00394-021-02658-1
FitzGerald, D., and Sonderegger, T. (2022). Documentation of changes implemented in the ecoinvent database v3.9.1 : ecoinvent Association Available at: https://support.ecoinvent.org/ecoinvent-version-3.9.1.
Food and Agricultural Organization of the United Nations (2013). Dietary protein quality evaluation in human nutrition. Report of an FAO expert consultation. Available at. https://www.fao.org/ag/humannutrition/35978-02317b979a686a57aa4593304ffc17f06.pdf
Frischknecht, R., Jungbluth, N., Althaus, H.-J., Hischier, R., Doka, G., Bauer, C., et al. (2007). Implementation of life cycle impact assessment methods. Data v2. 0 (2007). Ecoinvent report No. 3. Ecoinvent, Dübendorf
Fulgoni, V. L. 3rd, Keast, D. R., and Drewnowski, A. (2009). Development and validation of the nutrient-rich foods index: a tool to measure nutritional quality of foods. J. Nutr. 139, 1549–1554. doi: 10.3945/jn.108.101360
Gerhardt, C., Suhlmann, G., Ziemßen, F., Donnan, D., Warschun, M., and Kühnle, H. J. (2020). How will cultivated meat and meat alternatives disrupt the agricultural and food industry. Ind. Biotechnol. 16, 262–270. doi: 10.1089/ind.2020.29227.cge
Gibney, M. J. (2021). Food technology and plant-based diets. J. Nutr. 151, 1–2. doi: 10.1093/jn/nxaa301
Goedkoop, M., Heijungs, R., Huijbregts, M., de Schryver, A., Struijs, J., and van Zelm, R. (2009). ReCiPe 2008. A life cycle impact assessment method which comprises harmonised category indicators at the midpoint and the endpoint level. First edition. Report 1: characterisation. National Institute for Public Health and the Environment (RIVM), Bilthoven
Gonzales, G. E., Berry, C., Meng, M. D., and Leary, R. B. (2022). Identifying and addressing the “health halo” surrounding plant-based meat alternatives in limited-information environments. J. Public Policy Mark. 42, 242–261. doi: 10.1177/07439156221150919
Green, A., Blattmann, C., Chen, C., and Mathys, A. (2022). The role of alternative proteins and future foods in sustainable and contextually-adapted flexitarian diets. Trends Food Sci. Technol. 124, 250–258. doi: 10.1016/j.tifs.2022.03.026
Green, A., Nemecek, T., and Mathys, A. (2023). A proposed framework to develop nutrient profiling algorithms for assessments of sustainable food: the metrics and their assumptions matter. Int. J. Life Cycle Assess. 28, 1326–1347. doi: 10.1007/s11367-023-02210-9
Hallström, E., Davis, J., Woodhouse, A., and Sonesson, U. (2018). Using dietary quality scores to assess sustainability of food products and human diets: a systematic review. Ecol. Indic. 93, 219–230. doi: 10.1016/j.ecolind.2018.04.071
Hammer, L., Moretti, D., Abbuhl-Eng, L., Kandiah, P., Hilaj, N., Portmann, R., et al. (2023). Mealworm larvae (Tenebrio molitor) and crickets (Acheta domesticus) show high total protein in vitro digestibility and can provide good-to-excellent protein quality as determined by in vitro DIAAS. Front. Nutr. 10:1150581. doi: 10.3389/fnut.2023.1150581
Hammer, L., Moretti, D., Bétrix, C.-A., Kandiah, P., Pellegri, A., Abbühl-Eng, L., et al. (2024). In vitro DIAAS of Swiss soybean cultivars using the INFOGEST model: increase in protein quality from soybean to soymilk and tofu. Food Res. Int. 178:113947. doi: 10.1016/j.foodres.2024.113947
Heller, M. C., and Koleian, G. A. (2018). Beyond meat’s beyond burger life cycle assessment: a detailed comparison between a plant-based and an animal-based protein source : University of Michigan Available at: https://css.umich.edu/sites/default/files/publication/CSS18-10.pdf.
Herreman, L., Nommensen, P., Pennings, B., and Laus, M. C. (2020). Comprehensive overview of the quality of plant- and animal-sourced proteins based on the digestible indispensable amino acid score. Food Sci. Nutr. 8, 5379–5391. doi: 10.1002/fsn3.1809
Hertzler, S. R., Lieblein-Boff, J. C., Weiler, M., and Allgeier, C. (2020). Plant proteins: assessing their nutritional quality and effects on health and physical function. Nutrients 12:3704. doi: 10.3390/nu12123704
Huijbregts, M. A. J., Steinmann, Z. J. N., Elshout, P. M. F., Stam, G., Verones, F., Vieira, M., et al. (2017). ReCiPe2016: a harmonised life cycle impact assessment method at midpoint and endpoint level. Int. J. Life Cycle Assess. 22, 138–147. doi: 10.1007/s11367-016-1246-y
Intergovernmental Panel on Climate Change (2007). Contribution of Working Group I to the Fourth Assessment Report of the Intergovernmental Panel on Climate Change. Geneva: Cambridge University Press.
Intergovernmental Panel on Climate Change (2021). Climate Change 2021: The Physical Science Basis. Contribution of Working Group I to the Sixth Assessment Report of the Intergovernmental Panel on Climate Change. Geneva: Cambridge University Press.
International Standard Organisation (2006a). ISO 14040:2006. Environmental management—Life cycle assessment—Principles and framework. Geneva: International Standard Organisation (ISO), 1–20.
International Standard Organisation (2006b). ISO 14044:2006. Environmental management—Life cycle assessment—Requirements and guidelines. Geneva: International Standard Organisation (ISO), 1–46.
Khan, S., Loyola, C., Dettling, J., Hester, J., and Moses, R. (2019). Comparative environmental LCA of the impossible burger with conventional ground beef burger : Quantis Available at: https://assets.ctfassets.net/hhv516v5f7sj/4exF7Ex74UoYku640WSF3t/cc213b148ee80fa2d8062e430012ec56/Impossible_foods_comparative_LCA.pdf.
Komatsu, Y., Tsuda, M., Wada, Y., Shibasaki, T., Nakamura, H., and Miyaji, K. (2023). Nutritional evaluation of milk-, plant-, and insect-based protein materials by protein digestibility using the INFOGEST digestion method. J. Agric. Food Chem. 71, 2503–2513. doi: 10.1021/acs.jafc.2c07273
Kozicka, M., Havlík, P., Valin, H., Wollenberg, E., Deppermann, A., Leclère, D., et al. (2023). Feeding climate and biodiversity goals with novel plant-based meat and milk alternatives. Nat. Commun. 14:5316. doi: 10.1038/s41467-023-40899-2
Lehtonen, H., and Rämö, J. (2023). Development towards low carbon and sustainable agriculture in Finland is possible with moderate changes in land use and diets. Sustain. Sci. 18, 425–439. doi: 10.1007/s11625-022-01244-6
Marques-Vidal, P., Infanger, E., Baumer, B., Van der Horst, K., Lehmann, U., Bender, N., et al. (2023). Swiss dietary recommendations: Scientific background. Bundesamt für Veterinärwesen (BLV), Bern
McAuliffe, G. A., Takahashi, T., Beal, T., Huppertz, T., Leroy, F., Buttriss, J., et al. (2023). Protein quality as a complementary functional unit in life cycle assessment (LCA). Int. J. Life Cycle Assess. 28, 146–155. doi: 10.1007/s11367-022-02123-z
McLaren, S., Berardy, A., Henderson, A., Holden, N., Huppertz, T., Jolliet, O., et al. (2021). Integration of environment and nutrition in life cycle assessment of food items: Opportunities and challenges. Rome: FAO.
Michel, F., Hartmann, C., and Siegrist, M. (2021). Consumers’ associations, perceptions and acceptance of meat and plant-based meat alternatives. Food Qual. Prefer. 87:104063. doi: 10.1016/j.foodqual.2020.104063
Monteiro, C. A. (2009). Nutrition and health. The issue is not food, nor nutrients, so much as processing. Public Health Nutr. 12, 729–731. doi: 10.1017/S1368980009005291
Moughan, P. J. (2021). Population protein intakes and food sustainability indices: the metrics matter. Glob. Food Secur. 29:100548. doi: 10.1016/j.gfs.2021.100548
Nemecek, T., Roesch, A., Bystricky, M., Jeanneret, P., Lansche, J., Stüssi, M., et al. (2023). Swiss agricultural life cycle assessment: a method to assess the emissions and environmental impacts of agricultural systems and products. Int. J. Life Cycle Assess. 29, 433–455. doi: 10.1007/s11367-023-02255-w
Nosworthy, M. G., Franczyk, A., Zimoch-Korzycka, A., Appah, P., Utioh, A., Neufeld, J., et al. (2017). Impact of processing on the protein quality of pinto bean (Phaseolus vulgaris) and buckwheat (Fagopyrum esculentum Moench) flours and blends, as determined by in vitro and in vivo methodologies. J. Agric. Food Chem. 65, 3919–3925. doi: 10.1021/acs.jafc.7b00697
Nosworthy, M. G., Medina, G., Franczyk, A. J., Neufeld, J., Appah, P., Utioh, A., et al. (2018). Effect of processing on the in vitro and in vivo protein quality of beans (Phaseolus vulgaris and Vicia faba). Nutrients 10. doi: 10.3390/nu10060671
Onwezen, M. C., Bouwman, E. P., Reinders, M. J., and Dagevos, H. (2021). A systematic review on consumer acceptance of alternative proteins: pulses, algae, insects, plant-based meat alternatives, and cultured meat. Appetite 159:105058. doi: 10.1016/j.appet.2020.105058
Poore, J., and Nemecek, T. (2018). Reducing food’s environmental impacts through producers and consumers. Science 360, 987–992. doi: 10.1126/science.aaq0216
Saarinen, M., Fogelholm, M., Tahvonen, R., and Kurppa, S. (2017). Taking nutrition into account within the life cycle assessment of food products. J. Clean. Prod. 149, 828–844. doi: 10.1016/j.jclepro.2017.02.062
Santo, R. E., Kim, B. F., Goldman, S. E., Dutkiewicz, J., Biehl, E. M. B., Bloem, M. W., et al. (2020). Considering plant-based meat substitutes and cell-based meats: a public health and food systems perspective. Front. Sustain. Food Syst. 4:134. doi: 10.3389/fsufs.2020.00134
Sha, L., and Xiong, Y. L. (2020). Plant protein-based alternatives of reconstructed meat: science, technology, and challenges. Trends Food Sci. Technol. 102, 51–61. doi: 10.1016/j.tifs.2020.05.022
Sonesson, U., Davis, J., Flysjö, A., Gustavsson, J., and Witthöft, C. (2017). Protein quality as functional unit—a methodological framework for inclusion in life cycle assessment of food. J. Clean. Prod. 140, 470–478. doi: 10.1016/j.jclepro.2016.06.115
Sousa, R., Portmann, R., Recio, I., Dubois, S., and Egger, L. (2023a). Comparison of in vitro digestibility and DIAAR between vegan and meat burgers before and after grilling. Food Res. Int. 166:112569. doi: 10.1016/j.foodres.2023.112569
Sousa, R., Recio, I., Heimo, D., Dubois, S., Moughan, P. J., Hodgkinson, S. M., et al. (2023b). In vitro digestibility of dietary proteins and in vitro DIAAS analytical workflow based on the INFOGEST static protocol and its validation with in vivo data. Food Chem. 404:134720. doi: 10.1016/j.foodchem.2022.134720
Stylianou, K. S., Fulgoni, V. L., and Jolliet, O. (2021). Small targeted dietary changes can yield substantial gains for human health and the environment. Nat. Food 2, 616–627. doi: 10.1038/s43016-021-00343-4
Tyszler, H. B. M., Braconi, M. V. P. N., and Van Rijn, N. D. J. (2022). Agri-footprint 6 methodology report. Gouda, NL: Blonk Consulting.
van den Boom, L. A. T. P., van den Broek, K. L., Kroese, F. M., Moors, E. H. M., and de Ridder, D. (2023). Mental models of the protein shift: exploring consumers’ perceptions of the transition. Appetite 187:106595. doi: 10.1016/j.appet.2023.106595
Van Mierlo, K., Baert, L., Bracquené, E., De Tavernier, J., and Geeraerd, A. (2022). Moving from pork to soy-based meat substitutes: evaluating environmental impacts in relation to nutritional values. Future Foods 5:100135. doi: 10.1016/j.fufo.2022.100135
Varela-Ortega, C., Blanco-Gutiérrez, I., Manners, R., and Detzel, A. (2021). Life cycle assessment of animal-based foods and plant-based protein-rich alternatives: a socio-economic perspective. J. Sci. Food Agric. 102, 5111–5120. doi: 10.1002/jsfa.11655
Varela, P., Arvisenet, G., Gonera, A., Myhrer, K. S., Fifi, V., and Valentin, D. (2022). Meat replacer? No thanks! The clash between naturalness and processing: an explorative study of the perception of plant-based foods. Appetite 169:105793. doi: 10.1016/j.appet.2021.105793
Walker, C., Gibney, E. R., and Hellweg, S. (2018). Comparison of environmental impact and nutritional quality among a European sample population—findings from the Food4Me study. Sci. Rep. 8:2330. doi: 10.1038/s41598-018-20391-4
Walther, B., Guggisberg, D., Badertscher, R., Egger, L., Portmann, R., Dubois, S., et al. (2022). Comparison of nutritional composition between plant-based drinks and cow’s milk. Front. Nutr. 9:2645. doi: 10.3389/fnut.2022.988707
Wernet, G., Bauer, C., Steubing, B., Reinhard, J., Moreno-Ruiz, E., and Weidema, B. (2016). The ecoinvent database version 3 (part I): overview and methodology. Int. J. Life Cycle Assess. 21, 1218–1230. doi: 10.1007/s11367-016-1087-8
Willett, W., Rockström, J., Loken, B., Springmann, M., Lang, T., Vermeulen, S., et al. (2019). Food in the Anthropocene: the EAT-lancet commission on healthy diets from sustainable food systems. Lancet 393, 447–492. doi: 10.1016/s0140-6736(18)31788-4
Wolff, V., Alig, M., Nemecek, T., and Gaillard, G. (2016). Ökobilanz verschiedener Fleischprodukte. Geflügel-, Schweine- und Rindfleisch : Agroscope Available at: https://ira.agroscope.ch/de-CH/publication/36267.
World Health Organization (2022). Reformulation of food and beverage products for healthier diets: policy brief Geneva
Keywords: plant-based, meat analogue, soy drink, DIAAS, NRF, ultra-processed food, LCA, sustainability
Citation: Herrmann M, Mehner E, Egger L, Portmann R, Hammer L and Nemecek T (2024) A comparative nutritional life cycle assessment of processed and unprocessed soy-based meat and milk alternatives including protein quality adjustment. Front. Sustain. Food Syst. 8:1413802. doi: 10.3389/fsufs.2024.1413802
Edited by:
Bradley George Ridoutt, Commonwealth Scientific and Industrial Research Organisation (CSIRO), AustraliaReviewed by:
Thom Huppertz, FrieslandCampina (Netherlands), NetherlandsLuiz H. David, Commonwealth Scientific and Industrial Research Organisation (CSIRO), Australia
Copyright © 2024 Herrmann, Mehner, Egger, Portmann, Hammer and Nemecek. This is an open-access article distributed under the terms of the Creative Commons Attribution License (CC BY). The use, distribution or reproduction in other forums is permitted, provided the original author(s) and the copyright owner(s) are credited and that the original publication in this journal is cited, in accordance with accepted academic practice. No use, distribution or reproduction is permitted which does not comply with these terms.
*Correspondence: Thomas Nemecek, thomas.nemecek@agroscope.admin.ch