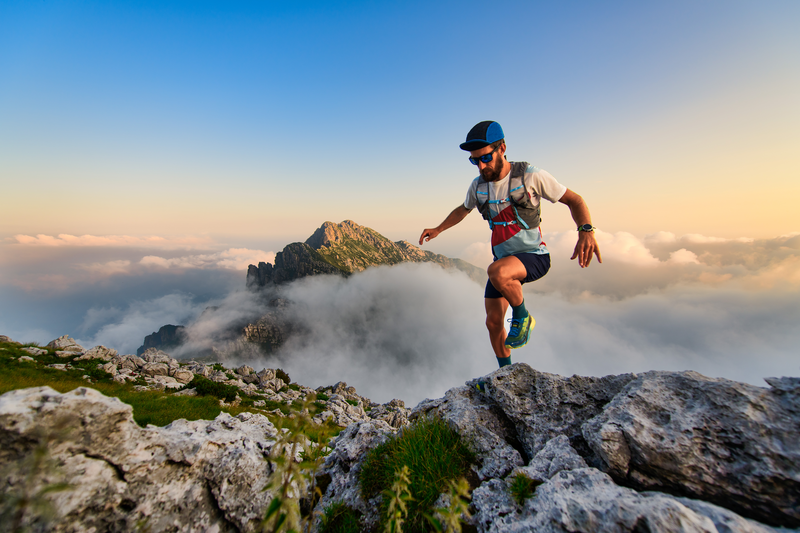
95% of researchers rate our articles as excellent or good
Learn more about the work of our research integrity team to safeguard the quality of each article we publish.
Find out more
ORIGINAL RESEARCH article
Front. Sustain. Food Syst. , 02 December 2024
Sec. Climate-Smart Food Systems
Volume 8 - 2024 | https://doi.org/10.3389/fsufs.2024.1410094
This article is part of the Research Topic Transforming African Food Systems View all 15 articles
Introduction: The adoption of climate-smart agriculture practices (CSAPs) at the plot level is a promising yet underutilized strategy in Ethiopia, where subsistence farming predominates. This study investigates the factors influencing the intensity of CSAPs on homesteads and identifies key barriers to their broader adoption.
Methods: Quantitative data were collected through household surveys. Multiple Linear Regression (MLR) analysis was employed to examine the relationship between the independent variables and farmers' decisions to intensify CSAPs.
Results: The findings reveal that slope gradient, extension contacts, farming experience, dependency ratio-induced labor, and livestock diversity significantly impact farmers' decisions to enhance CSAPs. Major barriers include insecure land tenure, poor management of open grazing, labor-intensive practices, and limited access to agricultural inputs.
Discussion: To promote the widespread implementation of CSAPs, it is imperative that these variables are prioritized in agricultural extension policies. Addressing these barriers is essential for enhancing the adoption of CSAPs, which will significantly contribute to sustainable agricultural development in Ethiopia.
In sub-Saharan nations like Ethiopia, accelerated land degradation due to deforestation, overgrazing, soil erosion, and nutrient depletion is a serious issue. Rapid population growth, limited land resources, and increased food demand since the early 20th century have exacerbated this problem (FAO, 2018; Li et al., 2021). Weak institutional arrangements and uncertain land ownership lead to inappropriate land use and farming on steep slopes (Moges et al., 2020). Intensive farming practices, such as crop residue removal, in-situ burning, and using animal manure for fuel, contribute significantly to land degradation (Moges et al., 2020; Hossain et al., 2020). These practices result in soil erosion, loss of fertility, and decreased water retention. Additionally, using animal dung for fuel, overstocking on croplands, and grazing on steep slopes can compromise crop productivity and livestock husbandry (Baiyeri et al., 2019).
Climate change, with rising temperatures and decreasing rainfall, further exacerbates land degradation (Matteoli et al., 2020; Trisos et al., 2022). This decline in land quality poses significant challenges for crop-livestock mixed farming systems. Climate-smart agriculture (CSA) offers a promising strategy to address these issues by enhancing productivity, mitigation, and adaptation (Burke et al., 2022; Trisos et al., 2022). In Ethiopia, CSAPs include land management techniques, with soil and water conservation being the most widely adopted (61.5%), followed by agroforestry and integrated soil fertility management (56.5%) (Abegaz et al., 2024).
Intensified CSAPs at homesteads can include terracing with agroforestry, legume-cereal intercropping, or rotation with straw retention, composting, and improved grazing systems (Jabbar et al., 2020; Kuyah et al., 2021; Trisos et al., 2022). Terracing alone is insufficient for controlling soil loss, maintaining moisture, preventing nutrient depletion, or increasing crop output without agroforestry or fodder strips (Debie, 2024). Combining terracing with vegetative and agronomic strategies reduces costs. Agroforestry systems, integrating trees/shrubs with crops and pasture, are sustainable and provide mitigation and adaptation benefits. Leguminous grass or shrub intercrops and forage strips on terracing enhance infiltration, soil nutrients, fodder supply, carbon sequestration, and nitrogen fixation (Matteoli et al., 2020; Horton et al., 2021; Mpairwe and Mutetikka, 2022).
In crop-livestock mixed systems, smallholder farmers should adopt diverse agroforestry-based soil conservation methods (Teklu et al., 2022). Legume-cereal intercropping or rotation improves soil fertility, reduces nitrogen inputs, and increases carbon sequestration and nitrogen fixation (Li et al., 2021; Matteoli et al., 2020; Zhao et al., 2022). Mulching crop residues promotes soil biodiversity, reduces dry spells, decreases pests, increases soil organic carbon (SOC), and boosts yields (Su et al., 2021; Kerr et al., 2022). Composting is essential for maintaining nitrogen intake and improving soil fertility, significantly impacting crop yield, and food security, and reducing greenhouse gas emissions (Demeke et al., 2017; Feliciano, 2019; Ray et al., 2020; Yang et al., 2022). However, without crop diversity and fodder strip terracing, composting can be labor-intensive (Debie, 2024).
The farming context—including topography, climate, soil type, and resource availability—is crucial in identifying the best CSAPs. Efficient irrigation systems and rainwater harvesting are vital in arid and semi-arid climates (Hussein, 2024). Crop diversification is beneficial in regions with unpredictable weather patterns (Thottadi and Singh, 2024). Various studies have explored CSAP adoption in different contexts, such as wheat production (Alemayehu et al., 2024; Geda et al., 2024), coffee-based farming (Diro et al., 2022), climate variability adaptation (Ahmed et al., 2023; Alhassan and Haruna, 2024; Kifle et al., 2022), and irrigated farming systems (Serote et al., 2021). Topography and climate significantly influence CSAP choices. Stabilized terracing with agroforestry is beneficial in hilly areas (Gashure et al., 2022), while conservation agriculture practices like crop rotation and minimal soil disturbance are effective in areas prone to soil degradation and water scarcity (Hussein, 2024). Studies show that different CSAPs are used in various locations but not often combined on a single plot. For example, Negera et al. (2022) examined techniques in the Bale-Eco region of Ethiopia, including enhanced crop variety, small-scale irrigation, integrated pest and weed control, and soil fertility management. Musafiri et al. (2022) reported on factors affecting the use of animal manure, agroforestry, soil and water conservation, crop diversification, and crop-livestock integration in Western Kenya. Kassa and Abdi (2022) identified factors influencing irrigation systems, organic manure, and tree planting in southern Ethiopia. In the central highlands of Ethiopia, Gudina and Alemu (2024) highlighted determinants affecting conservation agriculture, integrated soil fertility management, and crop diversification.
The adoption and effectiveness of CSAPs vary based on farming system contexts, types of CSAPs, and their integration on a given plot (Ma and Rahut, 2024). Jointly-used climate-smart agriculture at specific plots is recognized as a novel approach for optimizing productivity, adaptation, and carbon offsets. However, smallholder farmers in Ethiopia, where crop-livestock mixed farming is predominant, face challenges in adopting these practices. The main obstacles to expanding intensified CSAPs from homesteads to other farmlands remain unspecified. This study aims to identify the factors influencing the intensification of CSAPs at homesteads and the main obstacles to their broader adoption within watersheds. The findings are crucial for policy actions to scale up CSAPs in Ethiopia's highlands. Scaling up CSAP synergies across agroecologies is essential for achieving sustainable development goals, including greenhouse gas mitigation, climate change adaptation, poverty reduction, and biodiversity conservation.
The Azuari watershed, located between 10°42′26.399′′N to 11°9′13.462′′N latitude and 37°52′6.066′′E to 38°6′43.817′′E longitude, spans ~67,523 hectares between Addis Ababa and Bahir Dar. This watershed exemplifies the agroclimatic diversity and varied environmental and farming contexts of the Ethiopian highlands (Hurni et al., 2016). It is representative of policy frameworks supporting sustainable land management and farmer livelihoods in Ethiopia's northwest highlands (Moges et al., 2020). The elevation ranges from 1,270 to 4,043 meters, dividing the watershed into four agroecological zones: low-altitude (Kola), mid-altitude (Woina Dega), high altitude (Dega), and Wirch. These zones reflect a range of climates from warm and dry sub-humid (Kola) to cool, humid, and sub-humid (Woina Dega), temperate and humid (Dega), and humid and cold highlands (Wirch). Thus, the Azuari watershed represents the diverse agroecologies and farming systems of Ethiopia's northwest highlands.
The study area experiences a single rainy season (“Kiremt”) from June to September, with the dry season prevailing from October to March. Annual rainfall ranges from 900 mm in semiarid areas to 2,000 mm in cold and humid areas.
The watershed's major soil types include Eutric Nitosols, Cambisols, Lithosols, Luvisols, and Vertisols (FAO/IIASA/ISRIC/ISS-CAS/JRC, 2012; Fischer et al., 2008). Vertisols dominate the middle part, while Luvisols are prevalent in the upper part (Haregeweyn et al., 2017). In the lower part, dry-subhumid climates feature low-fertility, sandy Regosols (Fischer et al., 2008). Severe soil erosion in croplands, especially in the lower and higher parts, is driven by population pressure, steep slopes, and deforestation (Mequanient and Kebed, 2023). Water-induced soil erosion is the primary cause of soil degradation, impacting crop yields.
The watershed's main land uses include cultivated land, grasslands, shrublands, forests, plantations, and wetlands. Over three decades, significant areas of grasslands, forests, and shrublands have been converted to cultivated and bare land (Yimam, 2022). This conversion increases surface runoff and decreases base flow (Mekonnen and Disse, 2018). Monthly streamflow increased by 2 m3/s in wet months and decreased by 0.7 m3/s in dry months between 1990 and 2004 due to vegetation loss (Yimam, 2022). Forest degradation and agricultural encroachment have led to a 5.81% increase in wet-period streamflow and a 3.34% decline in dry-period flow (Bitew and Kebede, 2024).
Smallholder mixed farming, combining crop cultivation and livestock husbandry, is the predominant agricultural system. This system helps mitigate crop failure risks amid climate variability. Climate extremes, land degradation, and population pressure on limited resources contribute to food insecurity, increasing the sensitivity of crop-livestock mixed production to climate change, especially in lower elevations (Abeje et al., 2019).
This study used a cross-sectional survey, where data were gathered at a particular moment in time. Four kebeles (small administration units), one from the high-altitude (Selam-Abebe), two from the mid-altitude (Laytachimichael and Shegekeraniyo), and one from the low-altitude (YequaraArasema) agroecologies, were randomly selected after taking into account the elevation, farming system, climate condition, and crop pattern over the three agroecological zones (Figure 1). The smallest administrative division in Ethiopia is called a kebele, and inside a kebele is a sub-kebele. Sub-kebeles that implemented terracing practices through project initiatives or local community-based campaigns were given preference for determining the representative sample's target population. Household units can decide on composting, fodder strips for terrace stabilization, and other CSAPs. Since household heads usually make the ultimate decisions on farming techniques and resource utilization, the study looked at issues associated with using households as the unit of analysis over the sub-kebeles. The sample size is computed using Cochran (1977) formula:
Figure 1. Map showing the location of the study watershed (A) in Ethiopia's Northwest Highlands (B) between the country's capital city, Addis Ababa (AA), and regional city, Bahir Dar (BDR).
Where n is the required sample size, z is the confidence level, p estimated prevalence of farm household attributes in the area, and m- is the margin of error. With a standard value of 1.96 (z) and a 95% confidence level, the study's margin of error (m) is 5% or 0.05. Assume there is a large population but difficult to know the variability in the proportion that could adopt climate-smart intensifications in the crop-livestock mixed farming system, therefore, assume p = 0.2 (maximum variability), n = (1.96)2 (0.2(0.8)/(0.05))2 = 246 household head. A systematic random sampling technique was used to identify 246 of the 1,230 household heads in the sub-kebeles proportionately to the corresponding agroecology. A household head survey was conducted with 130 respondents from mid-altitude, 59 from low-altitude, and 57 from high-altitude agroecologies.
Data were generated after the respondents had been informed of the objectives of the research. A structured survey questionnaire was used to conduct face-to-face interviews to gather data from the participants. Well-trained four enumerators who are familiar with the local farming system delivered the questionnaire under the direct supervision of the researcher. The procedure of gathering data was conducted through two phases. In the first phase, a pre-test survey was held outside the sample areas to tailor the data collection method and issues to the research area setting. Data were collected on socioeconomic, ecological, and institutional variables, as well as the intensity of CSAPs. Plot level data included the soil fertility status and slope class. From the socioeconomic perspective, factors such as gender, farming experience, dependency ratio-induced labor, literacy level, farmland size, livestock diversity, income, and off-farm activities were taken into account. The institutional variables taken into account were access to credit associations, extension contacts, selected seed availability, distance to the nearest market, and farmland ownership status. Key informant interviews and firsthand observations provided qualitative data that was used to supplement the narrative with a variety of themes to complement the quantitative data.
The main six climate-smart agriculture practices (CSAPs)—crop rotation, crop residue retention, improved grazing, composting with inorganic fertilizers, stabilized terracing through agroforestry, and improved seed varieties—must be put into practice to increase agricultural productivity and sustainability in the Ethiopian highlands (Jirata et al., 2016; Teklu et al., 2022). The combination of these CSAPs with climate information systems is anticipated to reduce yield loss due to climate variability and farm expenses, and increase household income, food security, and resilience (Tesfaye et al., 2021). Direct observations made during the transect walks of the preliminary and main surveys provided evidence to support the primary CSAPs outlined at the national level, indicating that these practices are most common in the research watershed. Therefore, farmers were asked to rate how well their homestead adhered to the identified CSAPs with 0 representing “no” and 1 representing “yes.” The implementation of four to six major CSAPs on a particular homestead is considered intensified. For example, many farmers frequently cultivate improved maize and wheat seeds, compost/manure plus inorganic fertilizers, rotate between cereal and legume crops while retaining residue, and use agroforestry stabilized soil bunds and Fanya juu (Table 1). Summer and autumn are the best times for farmers to implement enhanced grazing systems. The average values (range from 0.17 to 1) of the number of practices implemented at the homestead were used to determine the intensity of CSAPs. The multiple linear regression (MLR) model can incorporate the values of the intensity level of CSAPs (e.g., 0.17, 0.33,…, 1) as long as these values reflect a continuous variable. The intensity values are continuous and provide measured levels of intensity. MLR can be used to model the association between these values of intensity level and other predictor variables, including covariates and categorical. Covariates (continuous) and factors (categorical) were the independent variables. For example, farmers' assessments of soil fertility status and slope steepness class were taken into account based on plot-level parameters. Socioeconomic variables were taken into consideration, such as gender, farming experience, dependency ratio-induced labor, education level, size of farmland holding, animal diversity index, income, and off-farm activities. Institutional variables such as distance to the nearest market, availability of agricultural inputs, insecurity of land tenure, training and advising possibilities through extension contacts, and access to credit associations were included.
Where n = the total number of livestock, Si = proportion of the ith animal in total livestock number. Hence, Si is the individual livestock proportion relative to the total livestock herd in a household. The Herfindahl index (HI) is calculated for LDI as = 1-HIL, where LDI is the livestock diversity index and HIL is the Herfindahl index livestock. The range of HIL values was 0.03–0.98. A value of zero denotes specialization, whereas a number greater than zero implies some degree of diversification.
The number of dependents—individuals who are normally not in the labor force, such as children under 15 and adults over 65—compared to the working-age population—those who are typically between the ages of 15 and 64—implies how the dependency ratio affects the availability of labor. The dependency ratio calculates the proportion of working-age household members (those between the ages of 15 and 64) to dependent household members (those between the ages of 0 and 14 and over 65) to determine the prospective labor supply for the labor cost of CSAPs.
Multiple Linear Regression (MLR) analysis was used to examine the relationship between the independent variables and farmers' decisions to intensify CSAPs. It can manage continuous dependent variables, regardless of the frequency at which a value occurs (Mohr et al., 2021). Verifying that the model assumptions can support the appropriateness of MLR is important in situations where the frequency of each intensity level may have an impact. The linear relationship, the lack of multicollinearity, the independence of errors, the homoscedasticity of the data, and the normality of the residuals were all confirmed before the analysis was conducted (Mohr et al., 2021; Dawson et al., 2021). MLR assumes that each predictor variable and the response variable have a linear relationship. The points in the scatter plots generally formed a straight line, suggesting a linear relationship. MLR assumes that predictor variables don't strongly correlate with one another. For this study, there was no noticeable multicollinearity because all of the VIF values were <2 and tolerance scores were above 0.6. Furthermore, a correlation matrix revealed that all correlation coefficients were <0.3, indicating that none of the predictor variables were substantially associated. The residuals (errors) must be independent for the MLR results to prove valid and robust. The value of the Durbin-Watson test in the model summary indicates 2.12, which lies between 1 and 3 implying that the residuals were independent or uncorrelated. The residuals should have constant variance at every level of the predictor variables, according to the homoscedasticity assumption of the MLR model. The assumption test result shows that homoscedasticity was indicated by the residuals' random distribution around zero. The model's predicted standardized values are plotted against the obtained standardized residuals using a random array of dots on the graph. Results demonstrate that the homoscedasticity assumption has been met since the residual variation is similar as the expected values increase along the X-axis. The model assumes that the residuals have a normal distribution. Plot values (P-P) show that the residuals were normally distributed since the points nearly followed a straight line.
The cause-effect relationship between the intensity of CSAPs with socioeconomic, institutional, and plot level variables was analyzed by a multiple linear regression (MLR) model. The model is a good choice for controlling other covariates, such as household socioeconomic, demographic, and institutional variables (Mohr et al., 2021). The equation of the regression model: yi= β0+ β1x1+ β2x2+…. + βkxk+ £, with the mean value of y given as:
where, y is the random response variable and μy is the mean value of y, β0, β1, β2, and βk are the parameters to be estimated based on the sample data, x1, x2,…,xk are the predictor variables that are assumed to be non-random or fixed and measured without error, and k is the number of predictor variables, and ε is the random error, which allows each response to deviate from the average value of y. The errors are assumed to be independent, have a mean of zero and a common variance (σ2), and are normally distributed.
The percentage distribution of the categorical variables is shown in Table 2. Farmers are thought to classify the homestead's slope and describe the fertility status of the soil. Of the total, about 70.1 and 69.1% of farmers said their homesteads had a steep slope and were fertile, respectively. Farmers stated that because of the good drainage and decreased risk of waterlogged and flooding, they often established residential villages on agricultural land with a moderate slope. Since the settlement's establishment, farmers have been able to increase the fertility of the soil on their homesteads by using sustainable land management practices. Factors such as male-headedness, literacy (at least reading and writing), lack of access to off-farm activities and credits, and interaction with extensions were shared by most respondents (Table 2). Farmers noted that training on the technical aspects and advantages of climate-smart agriculture practices (CSAPs) was often given by agricultural development agents to develop farmers' understanding and skill sets.
Table 3 shows the distribution of the continuous variables, including the total amount of farmland held, the number of years of farming experience, the dependency ratio, the diversity values of the livestock, and the annual income. For this study, the main components of developing CSAPs are agroforestry stabilized terracing, legume-cereal crop rotation with residue retention, combined use of compost and inorganic fertilizers, and an enhanced grazing system in the summer and autumn. The average value of CSAPs at the farmers' homestead spans from 0.17 to 1, with an overall average value of 0.6. Based on data, farmers engage in at least one and up to five CSAPs. The mean values of farming experience, dependency ratio, total farmland holding size, and livestock diversity were 29, 0.4, 2, and 0.49, respectively. An estimated 36,573.2 ETB was the average annual income of the respondents.
Table 3. The mean and distribution values of continuous dependent and independent variables (covariates).
The multiple linear regression models' goodness of fit was verified using the adjusted R-square statistic and the F-test significant value (Table 4). The highly significant p-value of 0.000 for the F-test in the regression model indicates that the independent factors together predict farmers' decisions to intensify CSAPs. The model's goodness of fit is demonstrated by the adjusted R-square statistic in the model summary, which indicates that significant factors account for 61% of the variation in the intensity of CSAPs. Table 4 shows that the main significant variables influencing farmers' decisions to intensify CSAPs at homestead were slope gradient, extension contacts, farming experience, dependency ratio-induce labor, and diversity in livestock husbandry. For this study, the decision of farmers to intensify CSAPs was not significantly influenced by factors, such as soil fertility status, gender, literacy level, off-farm activities, credit availability, selected seed availability, distance to the nearest market, size of farm holdings, or expected annual revenue.
Table 4. Factors influencing farmers' decision to intensify CSAPs at homesteads, and goodness of fit.
Farmers' decision to intensify CSAPs was significantly (at P < 0.001) impacted by their homesteads' moderate to steep slope characteristics. It was observed that Sesbania sesban, elephant grass, Acacia decurrens, Ficus thonningii, and Rahmnu sprinoides were used to stabilize the built terraces, such as soil bunds and Fanya juu, on the homestead with moderate to steep slope character. The steepness of the farmed land's slope had a substantial impact on the adoption of CSAPs (Ahmed et al., 2023). The findings presented in Table 4 demonstrate that farmers' decisions to enhance CSAPs on homesteads were significantly (at P < 0.001) influenced by frequent interactions with agricultural extension personnel. The acceptance of newly introduced CSAPs, like the use of improved seeds, composting, terracing, and planting of multifunctional exotic shrubs, has been greatly affected by the agriculture extension service (Kifle et al., 2022).
The intensification of CSA techniques was significantly (at P < 0.001) influenced by the husbandry of varied livestock (Table 4). Cattle, sheep, goats, chickens, horses, and donkeys are all herded inside the research area as part of the varied animal husbandry. Having a variety of cattle significantly influences a farmer's decision to use CSAPs (Bwalya et al., 2023; Debie and Ayele, 2023). The decision to strengthen CSAPs on the homestead was greatly impacted by the farmers' farming experience from the time of their productive age (Table 4). Farmers can be taught essential expertise and abilities about the integrated use of beehives, livestock herding, horticulture, and cropping patterns harmonized with favorable agroforestry through farming experience that has grown over time. Farming experience had a big impact on the degree to which farmers adopted CSAPs (Atsiaya et al., 2023; Sanogo et al., 2023). The decision of farmers to intensify CSAPs was negatively and significantly influenced (P < 0.01) by the dependency ratio, as shown in Table 4. This indicates the adoption of labor-intensive CSAPs is significantly impacted by households with a lower dependency ratio (larger productive labor size). For example, maintaining a terrace involves digging out silted soil from the channels and melding the composting materials, which may be very labor-intensive. Because of this, households with lower dependency ratios can devote more time to the ongoing upkeep of terracing and compost preparation than households with higher labor dependency composition. Farmers' decisions to use organic soil amendments are largely influenced by the low dependency ratio (Zheng et al., 2020).
The five main obstacles to the wider adoption of homestead CSAPs are the labor-intensive nature of the practices, weak management of open grazing practices, the uncertainty of land tenure, a shortage of agricultural inputs, and the lack of collaboration from stakeholders (Figure 2).
Figure 2. Significant obstacles to growing the intensity of CSAPs by the rank of the importance. Multiple responses were considered. Each barrier was ranked as “First” and “Second” responses, with the response percentages summing to 100% for each barrier.
The study shows that a primary obstacle (86%) to the watershed-level scale-up of the intensified CSAPs was the labor-intensive nature of regularly maintaining terraces, agroforestry management, composting, and improved grazing management. The weak monitoring scheme of the open grazing system was ranked as the second that prevented the successful establishment of agroforestry on the terrace segment, the strip, and distant cultivated land. Open grazing is prevalent on croplands during the winter and spring seasons. The open grazing practices of cattle, sheep, and goats destroyed the planted Sesbania sesban, elephant grass, Acacia decurrens, and Ficus thonningii on the terrace portion. At the third rank level, 67% of farmers stated that the main barrier impeding the growth of CSAPs at the watershed level was the uncertainty of agricultural land tenure. Furthermore, in the fourth and fifth rank levels, the restricted supply of agricultural inputs, such as equipment, seedlings, and better seeds (identified as the primary barrier by 55%) and weak stakeholder cooperation (indicated as the first barrier by 54%).
The use of compost and inorganic fertilizers in combination, legume-cereal crop rotation, crop residue retention, agroforestry stabilized terracing, and an improved autumn and spring grazing system are the most important identified CSAPs in the study watershed. In association with this, the three most widely applied CSAPs are conservation agriculture, crop diversification, and soil fertility management (Ali et al., 2022; Kifle et al., 2022). Crop rotations, low tillage methods, mixed cropping, planting trees, and applying manure were the most popular CSAPs among farmers (Bwalya et al., 2023; Ma and Rahut, 2024; Thottadi and Singh, 2024). Major CSAPs highlighted by Kassa and Abdi (2022) included agroforestry, organic manure, and small-scale irrigation systems. Based on the findings, farmers' decisions to intensify identified CSAPs at the homestead were significantly influenced by many factors, including the steepness of the homestead slope, regular guidance and training through extension contacts, developing awareness and skills over longer farming experiences, a low dependency ratio, and a high diversity in livestock husbandry.
The farmer's decision to use intensive CSAPs, such as forage strips or other agroforestry stabilized terracing is influenced by the homestead's steepness. The decision of farmers to use vegetative strips and terracing is significantly influenced by the steepness of the farmland (Alemayehu et al., 2024; Zeleke et al., 2024). Farmers in hilly regions could find vegetative stabilized terracing more appealing since the advantages of the terrace for changing the steep slope gradient often outweigh its expensive and labor-intensive constraints (Hilger et al., 2013).
Campaigns to construct terraces on farmlands with moderate to severe slopes were frequently started by the local community during the dry season, which lasted from January to March. However, the improperly designed campaign-based terrace, which was constructed without considering the plot owner's practical experience, did not meet the requirements and was not implemented sustainably in the cultivated field (Debie et al., 2019; Mersa et al., 2023). It was observed that terrace structures were frequently modified with plot tillers by incorporating native drainage channels and modifying slope gradients.
The result indicated that the homestead with moderate to severe slopes has maintained vegetative stabilized Fanya Juu and soil bunds because farmers are accustomed to their many advantages, which include controlling soil, water, nutrient, and crop losses; producing fodder for sheep and goats; and improving soil nutrients and crop output. Farmers were able to determine the impact of terracing on the reduction of slope gradient by observing the accumulation of deposits in the lower portion of croplands located between terraces. Natural and planted vegetative strips are effective in reducing the length of moderate and steep slopes and act as barriers to slow down runoff, thereby reducing soil erosion (Haddaway et al., 2018). The authors further noted that vegetative strips make them a popular choice among farmers due to the request being easier and less costly to implement compared to terracing. To optimize the potential for reducing soil erosion and increasing productivity, terraces should be utilized in conjunction with perennial vegetation, moisture retention, and a continuous presence of soil cover during the rainy season (Debie et al., 2019; Desta et al., 2021). Numerous ecological services are provided by stabilized terraces, such as higher soil moisture content, reduced runoff, and silt, pH, availability P, available K, and organic carbon, and enhanced grain yields (Debie et al., 2019; Tolesa et al., 2021; Deng et al., 2021). Terrace stabilizers including shrubs, annual herbaceous plants (like legumes), and trees like Faidherbia albida are examples of nitrogen-fixing plants that are essential to most CSAPs.
On the moderate to steep homestead, legume shrubs that are planted along the homestead's boundary and terrace segment are primarily pruned when they reach a productive age to provide fodder. Farmers stated that elephant grass is preferable to feeding cattle and that the best plants to feed sheep and goats are Rahmnu sprinoides, Sesbania sesban, and Acacia decurrens. One of the most widely used shrubs for fodder in Ethiopia; sesbania sesban improves the intake and digestibility of the basic diet for sheep and goats (Oosting et al., 2011; Debie, 2022). Productive natural grass developed on strips, terrace segments, homestead boundaries, and streams is commonly utilized for feeding cattle, especially oxen and cows, through the cut-and-carry grazing technique. Shrubs and trees must provide shade to shield animals from midday sunlight. Beekeeping productivity also benefits from the growth of flowering bushes along the homestead boundary and terrace segment. Shrub plants on the terrace segment may need to have deeply ingrained roots to stabilize the terrace and supply nutrients to the soil via root nodules. Farmers have observed that lowering the slope gradient significantly lowers crop damage during the early stages of growth. Consequently, farmers have developed an understanding of the detrimental effects of slope steepness on agricultural productivity, enabling them to sustain stabilized terraces over an extended period. Farmers choose to spend a significant amount of labor in upholding the practices and guarding them against free grazing because of the numerous advantages of doing so. Compost and livestock dung are applied by farmers to farmland areas situated in between terraces stabilized by grass or shrubs.
The results indicate that the husbandry of various livestock kinds at farm households leads to an increase in the amount of manure applied, which is made up of the dung of various livestock types, including horses, chickens, sheep, goats, cattle, and donkeys, to improve the fertility of their farm soil. In association with this, total livestock holding size was one of the major determinants of the adoption of CSAPs (Alemayehu et al., 2024; Zeleke et al., 2024). Cattle dung is regularly utilized to make dung cake, which is used as fuel for energy during the autumn, winter, and spring seasons. The dung of chickens, horses, donkeys, sheep, and goats is not used as fuel energy. To maximize the use of manure to improve soil fertility and be able to replace cattle dung used for fuel energy, it is essential to herd chickens, horses, donkeys, sheep, and goats. The best-integrated degree of decomposition and productivity benefits of crop grain and biomass yield were seen in co-composting or mixed use of cow, pig, and wheat straw (Fan et al., 2023). Various farming methods employ a blend of manure from pigs, cattle, and poultry (Rayne and Aula, 2020). Thompson et al. (2023) state that well-kept livestock is vital for agricultural systems and can yield significant benefits. More carbon sequestration is encouraged by manure soils (Washaya and Washaya, 2023).
According to the findings, a lower dependency ratio denotes fewer dependents per person of working age, which increases labor availability and may motivate the household to intensify CSAPs on homesteads and other farmlands to enhance livestock and crop yields. A low dependency ratio boosts labor availability, which can help with enhanced cattle husbandry practices and terrace stabilization through agroforestry and composting on a particular homestead (Debie, 2024). Having more people in the working age range in the household can help manage different CSAPs in an efficient and long-lasting way (Sharma et al., 2024). Household labor is required at large levels for CSAPs, such as manure application, composting, distribution of crop straw across the entire homestead, improved grazing management, and agroforestry management. Farmers stated that the labor cost of spreading the collected manure of various livestock on the land requires less labor cost than that of composting. During the dry season, women and young people were mostly responsible for distributing the collected manure at the homestead. Famers reported that carrying manure to scatter on a homestead needs less work compared to transporting manure to distant agricultural areas using manpower and donkeys. As per the training provided by the local administration unit's development agents, farm households with a low labor dependency ratio can devote more time to making compost from green manure, ash, and livestock manure. During the end of winter and throughout the spring, farmers can distribute manure or compost and mix it in the soil by plowing. Despite the increased labor costs associated with its production, farmers stated that compost overtakes manure in terms of improving soil fertility and crop yields for the forthcoming year. The application of compost generally had a significant and positive impact on plant nutrients, such as soil pH, EC, SOM, total N, and accessible P (Kavvadias et al., 2023). For many reasons, including low quality, inconsistent supply, and difficulties with manure collection caused by inadequate facilities and great distances between farms, manure must be properly dealt with before it can be used (Washaya and Washaya, 2023). Activators can be added to the basic constituents of compost to increase its nutritional value. Adding fungicides, viricides, nematode inhibitors, and antibacterial treatments for plants or organic materials can also improve the quality of compost (Ayilara et al., 2020). Generally, manure or compost is used to cultivate cereal crops (primarily sorghum and maize), followed by legume crops. Rotating crops between legumes and grains is a fundamental practice for managing soil.
The finding revealed that agricultural extension advice and training services had a significant impact on farmer awareness development of many advantages and integrated use of CSAPs on homesteads and other farmland. Increasing public awareness of the advantages, practical procedures, and technical know-how of CSAPs can be essential to increasing the rate of adoption. The more intensive CSAPs, including terracing, composting, improved grazing systems, and others were influenced by frequent extension contacts. The most important aspect influencing the adoption of CSAPs was having access to extension contacts (Diro et al., 2022; Xu et al., 2022; Bwalya et al., 2023 Debie and Ayele, 2023; Ferrer et al., 2023; Tadesse and Ahmed, 2023; Hussein, 2024; Ma and Rahut, 2024). Due to a lack of training and access to agricultural knowledge, farmers were found to be ill-prepared to adopt newly introduced CSAPs (Nyairo et al., 2022). Farmers stated that agricultural extension workers helped them with the functional and technical components of several CSAPs. Agricultural extension workers can also be knowledgeable on the use of improved seed, careful terracing management, making good use of terrace stabilizers for livestock fodder, beehive and livestock husbandry, and compost preparation. Farmers are familiar with the indigenous technique of legume-cereal crop rotation or intercropping. Thus, there will be more dedicated farmers who can fill their technical and knowledge gaps about the need to apply CSAPs to fulfill multiple objectives by having access to possibilities for training and advice. Farmers who have received relevant training and skills in CSAPs should be the focus of the extension program (Kifle et al., 2022).
The findings of the study showed that farmer experience across time also considerably influences the diversity, intensity, and effective management of CSAPs. Geda et al. (2024) found that the adoption of CSAPs in wheat production was influenced by farming experience. By implementing crucial technologies early on in the context of their cultivated area, farmers can develop competence in managing projected production hazards (Ainembabazi and Mugisha, 2014). Based on their local knowledge and experience, farmers commonly intercrop modified maize on their homestead with sunflowers, faba beans, peas, and common beans. The purposes of rotation or legume-cereal intercropping are widely known to farmers. The primary perceived goals of the technique are to boost crop productivity and soil fertility. Legumes and maize intercropped improved soil fertility, total nitrogen content, and grain and biomass yield of cereal crops (Gidey et al., 2024). Cereals (maize, wheat, sorghum, and teff) interplanted with oil seeds Guizotia abyssinica (named Noug locally), Ethiopian mustard, also known as gomenzer (Brassica carinata A. Braun), and linseed (flaxseed) on homestead boundary strips that touch on pathways used by people and livestock or trees (Eucalyptus globulus). Legumes and oil seed straws or wastes are utilized on the farm to mulch the soil's surface and young plants, such as Eucalyptus globulus. Straws from maize and sorghum are mostly utilized as cattle feed and fuel. The soils can be combined with a small amount of residual maize and sorghum. For the first plowing in the dry season, the collected cereals, legumes, and oil seed straws can be incorporated into the soil. Frequent plowing during the dry and spring seasons allows the organic biomass of the residue to break down and provide the soil with nutrients for the next crop's productivity. For cattle hay fed in the spring and summer, teff straw is primarily utilized. Based on their experience, the majority of farmers employ a cut-and-carry technique in the autumn and summer to improve cow grazing strategy since these seasons may require less labor than other seasons to harvest valuable feed for cattle, such as grass and shrub leaves. Weeds that grow beneath the biomass of maize crops can be harvested for cattle fodder. Post-harvest practices frequently involve establishing agroforestry and limiting unrestricted grazing on terraced homesteads. During the post-harvest time, pruning of Acacia decurrens, Sesbania sesban, and elephant grass is done on the farm to provide feed for cattle, sheep, and goats. Therefore, a combination of farmer knowledge, experience, and abilities, as well as expert scientific observation are required to properly manage multifunctional CSAPs and sustainable production of mixed agricultural systems (Debie, 2024).
The labor-intensive nature of routinely maintaining terraces and producing compost, the mishandling of open grazing practices, the uncertainty of land tenure, the scarcity of agricultural inputs and supplies, and the lack of collaboration amongst stakeholders at the lowest administrative unit were the major barriers to wider adoption of CSAPs in the watershed. Similar research conducted by Hussein (2024) demonstrates that inadequate resources, inadequate technical know-how, and poor infrastructure impede the uptake and expansion of CSAPs.
Lack of labor prohibited farmers from regularly maintaining terraces and producing large volumes of compost. The extensive and sustainable adoption of terraces is primarily driven by their technical suitability for labor requirements and farming system conditions (Debie, 2021; Sanogo et al., 2023). The preparation of large quantities of compost for a thorough treatment of their entire agricultural area has proven to be a labor-intensive operation for smallholder farmers. Farm households with a low labor dependency ratio that can maintain terraces and make compost for their homestead may not be able to extend to other cultivated fields due to their labor-intensive nature. Farmers said that carrying compost by hand is not feasible. Transporting compost or manure from houses to remote locations is one of the largest barriers to the broad application of sustainable manure management. There are additional costs of labor, financial, and time involved in transporting compost/manure/from the produced site (residential area) to distant cultivated land. Produced compost is occasionally transported from residential sites to distant croplands by donkeys.
In addition to compos, maintaining the terrace in the spring and summer seasons is labor-intensive work. Using ox drive plowing, farmers frequently tilled siltation on terrace channels. The difficulty arises when farmers throw the excavated silted soil uphill. Regular maintenance is recommended for a long-term reduction in runoff and soil loss (Belayneh et al., 2020). The technical viability of terracing needs to be evaluated concerning farmed areas that require less labor for upkeep (Debie, 2021). The amount of labor required for maintenance can be greatly decreased by stabilizing terraces with agro-forestry, grass strips, and percolation ditches (Debie et al., 2019; Belayneh et al., 2020). The problem is the absence of suitable tools and equipment to facilitate the manufacture of compost and the excavation of silted soil. The main barrier to CSAPs scaling up is limited access to appropriate farm equipment and tools to ease labor-intensive work (Barnard et al., 2015). Farmers should be given access to innovative mechanical tools and equipment to minimize the amount of labor needed for compost production and terrace maintenance. The kinds of techniques and instruments used in the manufacture and delivery of compost must minimize the labor requirements. The equipment must reduce the labor needed when siltation removal from terrace channels and tossing to upslope areas.
One of the main obstacles to the continuous use and expansion of vegetative stabilized terraces in the micro-watershed has been unrestricted open grazing throughout the post-harvest periods (winter and spring). Farmers manage their crop fields during the planting season but these fields revert to communal spaces available for livestock grazing during the dry season (Barnard et al., 2015). Livestock can be allowed to graze on the agricultural residue that remains on the cropland in substantial amounts. The owners of agricultural land under conservation are unable to monitor free grazing on their land during the post-harvesting period due to the perceived labor cost and fear of hostility from livestock owners. Under the local administration unit, farmers, administrators, and extension agents have not worked together well to restrict free grazing. The absence of implementation of local legislation that controls open cattle grazing hinders the effectiveness of the adopted land management practices (Nebere et al., 2021). Farmers were included in the terracing and vegetation plantation implementation phase, but their participation in the planning, monitoring, and evaluation phases is limited, thus constraining the institutional structure (Adego et al., 2018; Nebere et al., 2021). This issue causes established terrace stabilizers to be injured by grazers and free browsers, destabilizing and destroying the terrace in the process. Hence, to prevent many issues associated with free grazing in preserved areas, strong regulations, enforcement, and sanctions must be put in place. To achieve real and observable improvements in land management techniques, farmers are urged to participate actively in all phases of conservation initiatives (Adego et al., 2018; Nebere et al., 2021). Stall-feeding procedures, the development of fast-growing fodder grass species, and a reduction in the number of cattle all improve both the productivity of cattle and the sustainability of land management techniques (Nebere et al., 2021).
The findings showed that land tenure issues and inadequate policy strategies are the main reasons why smallholder farmers usually have limited success implementing CSAPs. Tenure-related constraints and limitations prevent the expansion of CSAPs (Autio et al., 2021; Zerssa et al., 2021; Sanogo et al., 2023). Farmers may be reluctant to invest in sustainable land management because they think that land redistribution may result from unresolved land tenure conflicts (Ege, 2017). Insufficient enforcement of local-level land use planning and unstable land tenure security result in adverse environmental consequences and a failure to manage the use of land resources in a balanced manner (Abab et al., 2023). To remedy this measures such as bylaw approval and enforcement, specific land use planning, and land tenure security must be implemented. The safety and long-term sustainability of land management practices require a bylaw establishing contractual agreements between landowners and renters. The primary driver of the broader implementation of CSAPs is the security of land tenure and land use rights (Ege, 2017; Debie, 2021; Abab et al., 2023).
The lack of collaboration among the community, local administration, and extension personnel was identified as another obstacle to the watershed-level scaling up of intensified CSAPs. It is common to see weak cooperation between farmers and agricultural extension workers when it comes to planning, monitoring, and evaluating practices. Extension agents and administrative units encourage farmers to take part in constructing terraces in the dry (winter) season and plant shrub seedlings on the terrace segments and watercourse in the summer. This suggests that farmers were forced to engage in practice time even if they were not involved in its preparation. The agricultural extension cannot successfully collaborate with farmers by making available agricultural inputs such as better seeds, fertilizers, and agricultural instruments for reducing labor costs. Sanogo et al. (2023) state that a major barrier to adopting and expanding CSAPs was the lack of agricultural inputs, such as better seeds and agroforestry seedlings.
To avert the major barriers, it is crucial to provide opportunities to hasten the CSAPs' broader implementation to enhance the food security of smallholder households (Ali et al., 2022; Kirina et al., 2022; Teklu et al., 2022). In the context of the subsistence farming system in Ethiopia's highlands, a framework for the sustainable implementation of intensified CSAPs should be developed to feed the fast-expanding population.
In Ethiopia, where subsistence farming is the main driver of the economy, the intensity of climate-smart agriculture practices (CSAPs) among smallholder farmers is still extremely low. Furthermore, it is not clear what the main obstacles are to the expansion of more intensive CSAPs. With the use of descriptive statistics and multiple linear regression models, the study aimed to identify factors that influence the intensity of CSAPs on a homestead and the main obstacles to the spread of enhanced CSAPs. The results reveal that slope gradient, extension interactions, agricultural experience, dependency ratio, and diversity in livestock husbandry were the main significant variables impacting farmers' decisions to intensify CSAPs at the homestead. The main obstacles to scaling up intensified CSAPs are labor-intensive terracing and composting, poorly managed open grazing practices, unclear land tenure, a lack of agricultural supplies and inputs, and a lack of cooperation from stakeholders at the lowest administrative unit, or “kebele.” Therefore, the agricultural extension policy must emphasize the main variables that influence farmers' decisions to intensify CSAPs as well as the primary barriers that are impeding the wider use of these practices. To achieve sustainable development goals including lowering poverty, adjusting to climate change, reducing greenhouse gas emissions, and ensuring sustainable biodiversity, it may be essential to scale up the synergies of CSAPs across agroecologies.
The original contributions presented in the study are included in the article/supplementary material, further inquiries can be directed to the corresponding author.
Ethical review and approval was not required for the study on human participants in accordance with the local legislation and institutional requirements. Written informed consent from the (patients/participants OR patients/participants legal guardian/next of kin) was not required to participate in this study in accordance with the national legislation and the institutional requirements.
ED: Conceptualization, Data curation, Formal analysis, Investigation, Methodology, Project administration, Resources, Software, Supervision, Validation, Visualization, Writing – original draft, Writing – review & editing.
The author(s) declare that no financial support was received for the research, authorship, and/or publication of this article.
The author is grateful to recognize the respondents. The manuscript acknowledges the use of Microsoft Copilot, version 1.0, for its editorial activities.
The author declares that the research was conducted in the absence of any commercial or financial relationships that could be construed as a potential conflict of interest.
All claims expressed in this article are solely those of the authors and do not necessarily represent those of their affiliated organizations, or those of the publisher, the editors and the reviewers. Any product that may be evaluated in this article, or claim that may be made by its manufacturer, is not guaranteed or endorsed by the publisher.
Abab, S. A., Senbeta, F., and Negash, T. T. (2023). The effect of land tenure institutional factors on small landholders' sustainable land management investment: evidence from the highlands of Ethiopia. Sustainability 15:9150. doi: 10.3390/su15129150
Abegaz, A., Abera, W., Jaquet, S., and Tamene, L. (2024). Adoption of climate-smart agricultural practices (CSAPs) in Ethiopia. Clim. Risk Manag. 45:100628. doi: 10.1016/j.crm.2024.100628
Abeje, M. T., Tsunekawa, A., Adgo, E., Haregeweyn, N., Nigussie, Z., Ayalew, Z., et al. (2019). Exploring drivers of livelihood diversification and its effect on adoption of sustainable land management practices in the upper blue nile basin, Ethiopia. Sustainability 11:2991. doi: 10.3390/su11102991
Adego, T., Simane, B., and Woldie, G. A. (2018). Sustainability, institutional arrangement and challenges of community-based climate-smart practices in northwest Ethiopia. Agric. Food Secur. 7:56. doi: 10.1186/s40066-018-0206-0
Ahmed, B., Haji, J., Ketema, M., and Jemal, K. (2023). Impacts and adaptation extents of climate-smart agricultural practices among smallholder farmers of Ethiopia: implication to food and nutrition security. Cogent. Econ. Finan. 11:2210911. doi: 10.1080/23322039.2023.2210911
Ainembabazi, J. H., and Mugisha, J. (2014). The role of farming experience on the adoption of agricultural technologies: evidence from smallholder farmers in Uganda. J. Dev. Stud. 50, 666–679. doi: 10.1080/00220388.2013.874556
Alemayehu, S., Ayalew, Z., Sileshi, M., and Zeleke, F. (2024). Determinants of the adoption of climate-smart agriculture practices by smallholder wheat farmers in northwestern Ethiopia. Heliyon 1:e34233. doi: 10.1016/j.heliyon.2024.e34233
Alhassan, U., and Haruna, E. U. (2024). Rural farmers' perceptions of and adaptations to climate change in Sub-Saharan Africa: Does climate-smart agriculture (CSA) matter in Nigeria and Ethiopia? Environ. Econ. Policy Stud. 26, 613–652. doi: 10.1007/s10018-023-00388-8
Ali, H., Menza, M., Hagos, F., and Haileslassie, A. (2022). Impact of climate-smart agriculture adoption on food security and multidimensional poverty of rural farm households in the Central Rift Valley of Ethiopia. Agric. Food Sec. 11:62. doi: 10.1186/s40066-022-00401-5
Atsiaya, G. O., Gido, E. O., and Sibiko, K. W. (2023). Uptake of climate-smart agricultural practices among smallholder sorghum farmers in Busia County, Kenya. Cogent. Food Agric. 9:1. doi: 10.1080/23311932.2023.2204019
Autio, A., Johansson, T., Motaroki, L., Minoia, P., and Pellikka, P. (2021). Constraints for adopting climate-smart agricultural practices among smallholder farmers in Southeast Kenya. Agric. Syst. 194:103284. doi: 10.1016/j.agsy.2021.103284
Ayilara, M. S., Olanrewaju, O. S., Babalola, O. O., and Odeyemi, O. (2020). Waste management through composting: challenges and potentials. Sustainability 12:4456. doi: 10.3390/su12114456
Baiyeri, P. K., Foleng, H. N., Machebe, N. S., and Nwobodo, C. E. (2019). “Crop-livestock interaction for sustainable agriculture,” in Innovations in Sustainable Agriculture, eds. M. Farooq, and M, Pisante (Cham: Springer).
Barnard, J., Henry, M., Emmanuel, T., and Solomon, B. (2015). Barriers to scaling up/out climate-smart agriculture and strategies to enhance adoption in Africa. The Forum for Agricultural Research in Africa, Accra, Ghana.
Belayneh, M., Yirgu, T., and Tsegaye, D. (2020). Runoff and soil loss responses of cultivated land managed with graded soil bunds of different ages in the Upper Blue Nile basin, Ethiopia. Ecol. Process. 9:66. doi: 10.1186/s13717-020-00270-5
Bitew, M. M., and Kebede, H. H. (2024). Effect of land use land cover change on stream flow in Azuari watershed of the Upper Blue Nile Basin, Ethiopia. Sustain. Water Resour. Manag. 10:112. doi: 10.1007/s40899-024-01084-5
Burke, W. J., Snapp, S. S., Peter, B. G., and Jayne, T. S. (2022). Sustainable intensification in jeopardy: transdisciplinary evidence from Malawi. Sci. Total Environ. 837:155758. doi: 10.1016/j.scitotenv.2022.155758
Bwalya, B., Mutandwa, E., and Chiluba, B. C. (2023). Awareness and use of sustainable land management practices in smallholder farming systems. Sustainability 15:14660. doi: 10.3390/su152014660
Chegere, M. J., and Stage, J. (2020). Agricultural production diversity, dietary diversity and nutritional status: Panel data evidence from Tanzania. World Develop. 129:104856. doi: 10.1016/j.worlddev.2019.104856
Dawson, D. V., Blanchette, D. R., and Pihlstrom, B. L. (2021). “Burt and Eklund's dentistry, dental practice, and the community,” in Burt and Eklund's Dentistry, Dental Practice, and the Community, 7th Edn. (St. Louis, MO: Elsevier), 131–153.
Debie, E. (2021). Smallholder farmers' decisions to the combined use of soil conservation practices in Tiwa watershed, Northwest highlands of Ethiopia. Heliyon 7:e05958. doi: 10.1016/j.heliyon.2021.e05958
Debie, E. (2022). Stakeholder perceptions on multiple ecosystem services of soil conservation practices in Tiwa Watershed, Northwest Ethiopia. J. Environ. Assess. Policy Manag. 22:2250010. doi: 10.1142/S1464333222500107
Debie, E. (2024). Effectiveness of sustainable land management initiatives in the highlands of Ethiopia. Reg. Environ. Change 24:106. doi: 10.1007/s10113-024-02263-y
Debie, E., and Ayele, A. W. (2023). Perceived determinants of smallholder households' resilience to livelihood insecurity in Goncha district, northwest highlands of Ethiopia. SAGE Open 13. doi: 10.1177/21582440231184861
Debie, E., Singh, K. N., and Belay, M. (2019). Effect of conservation structures on curbing rill erosion in micro-watersheds, northwest Ethiopia. Int. Soil Water Conserv. Res. 2, 239–247. doi: 10.1016/j.iswcr.2019.06.001
Demeke, M., Meerman, J., Scognamilo, A., Romeo, A., and Asfaw, S. (2017). Linking Farm Diversification to Household Diet Diversification From a Sample of Kenyan Ultra-Poor Farmers: ESA Working Paper No 17-01. Rome: FAO.
Deng, C., Zhang, G., Liu, Y., Nie, X., Li, Z., Liu, J., et al. (2021). Advantages and disadvantages of terracing: a comprehensive review. Int. Soil Water Conserv. Res. 9, 344–359. doi: 10.1016/j.iswcr.2021.03.002
Desta, G., Tamene, L., Abera, W., Amede, T., and Whitbread, A. (2021). Effects of land management practices and land cover types on soil loss and crop productivity in Ethiopia: A review. Int. Soil Water Conserv. Res. 9, 544–554. doi: 10.1016/j.iswcr.2021.04.008
Diro, S., Tesfaye, A., and Erko, B. (2022). Determinants of adoption of climate-smart agricultural technologies and practices in the coffee-based farming system of Ethiopia. Agric. Food Secur. 11:42. doi: 10.1186/s40066-022-00385-2
Ege, S. (2017). Land tenure insecurity in post-certification Amhara, Ethiopia. Land Use Policy 64, 56–63. doi: 10.1016/j.landusepol.2017.02.015
Fan, T., Zhang, X., Wan, Y., Deng, R., Zhu, H., Wang, X., et al. (2023). Effect of different livestock manure ratios on the decomposition process of aerobic composting of wheat straw. Agronomy 13:2916. doi: 10.3390/agronomy13122916
FAO (2018). The Future of Food and Agriculture – Alternative Pathways to 2050. Rome. 224. Licence:CC BY-NC-SA 3.0 IGO.
FAO/IIASA/ISRIC/ISS-CAS/JRC (2012). Harmonized World Soil Database (version 1.2). FAO: Rome; Laxenburg: IIASA,.
Feliciano, D. (2019). A review of the contribution of crop diversification to Sustainable Development Goal 1 “No poverty” in different world regions. Sustain. Dev. 27, 795–808. doi: 10.1002/sd.1923
Ferrer, A. J. G., Thanh, L. H., Chuong, P. H., Kiet, N. T., Trang, V. T., Duc, T. C., et al. (2023). Farming household adoption of climate-smart agricultural technologies: evidence from North-Central Vietnam. Asia Pac. J. Reg. Sci. 7, 641–663 doi: 10.1007/s41685-023-00296-5
Fischer, G., Nachtergaele, F., Prieler, S., van Velthuizen, H. T., Verelst, L., and Wiberg, D. (2008). Global Agro-ecological Zones Assessment for Agriculture (GAEZ 2008). Laxenburg: IIASA; Rome: FAO.
Gashure, S., Wana, D., and Samimi, C. (2022). Impacts of climate variability and climate-smart agricultural practices on crop production in UNESCO-designated cultural landscapes of Konso, Ethiopia. Theor. Appl. Climatol. 150, 1495–1511. doi: 10.1007/s00704-022-04244-9
Geda, M. B., Haji, J., Jemal, K., and Zeleke, F. (2024). Determinants of adoption of climate-smart agricultural technologies in wheat production in Arsi Zone, Oromia Region of Ethiopia. Discover. Food 4:8. doi: 10.1007/s44187-024-00077-9
Gidey, T., Berhe, D. H., Birhane, E., Gufi, Y., and Haileslassie, B. (2024). Intercropping maize with faba bean improves yield, income, and soil fertility in semiarid environment. Hindawi Scie. 2024:2552695. doi: 10.1155/2024/4929479
Gudina, M. H., and Alemu, E. A. (2024). Factors influencing smallholder farmers adoption of climate SMART agriculture practices in Welmera Woreda, Central Ethiopia. Front. Clim. 6:1322550. doi: 10.3389/fclim.2024.1322550
Haddaway, N. R., Brown, C., Eales, J.S, Eggers Josefsson, J., Kronvang, B., et al. (2018). The multifunctional roles of vegetated strips around and within agricultural fields. Environ. Evid. 7:14. doi: 10.1186/s13750-018-0126-2
Haregeweyn, N., Tsunekawa, A., Poesen, J., Tsubo, M., Meshesha, D. T., Fenta, A. A., et al. (2017). Comprehensive assessment of soil erosion risk for better land use planning in river basins: a case study of the Upper Blue Nile River. Sci. Total Environ. 574, 95–108 doi: 10.1016/j.scitotenv.2016.09.019
Hilger, T., Keil, A., Lippe, M., Panomtaranichagul, M., Saint-Macary, C., Zeller, M., et al. (2013). “Soil conservation on sloping land: technical options and adoption constraints,” in Sustainable Land Use and Rural Development in Southeast Asia: Innovations and Policies for Mountainous Areas. Springer Environmental Science and Engineering, eds. H. L. Fröhlich, P. Schreinemachers, K. Stahr, and G. Clemens (Berlin, Heidelberg: Springer).
Horton, P., Long, S. P., Smith, P., Banwart, S. A., and Beerling, D. J. (2021). Technologies to deliver food and climate security through agriculture. Nat. Plants 7, 250–255. doi: 10.1038/s41477-021-00877-2
Hossain, A., Krupnik, T. J., Timsina, J., Mahboob, M. G., Chaki, A. K., Farooq, M., et al. (2020). “Agricultural land degradation: processes and problems undermining future food security,” in Environment, Climate, Plant and Vegetation Growth, eds. S. Fahad, M. Hasanuzzaman, M. Alam, H. Ullah, M. Saeed, I. A. Khan, and M. Adnan (Cham: Springer). doi: 10.1007/978-3-030-49732-3_2
Hurni, H., Berhe, W. A., Chadhokar, P., Daniel, D., Gete, Z., Grunder, M., et al. (2016). Soil and Water Conservation in Ethiopia: Guidelines for Development Agents. 2nd revised Edn. Bern: Centre for Development and Environment (CDE), University of Bern, with Bern Open Publishing (BOP), 134.
Hussein, A. (2024). Climate-smart agriculture strategies for enhanced agricultural resilience and food security under a changing climate in Ethiopia. Sustain. Environ. 10:2345433 doi: 10.1080/27658511.2024.2345433
Jabbar, A., Wu, Q., Peng, J., Zhang, J., Imran, A., and Yao, L. (2020). Synergies and determinants of sustainable intensification practices in Pakistani agriculture. Land 9:110. doi: 10.3390/land9040110
Jirata, M., Grey, S., and Kilawe, E (2016). Ethiopia Climate-Smart Agriculture Scoping Study. Addis Ababa: FAO.
Kassa, B. A., and Abdi, A. T. (2022). Factors influencing the adoption of climate-smart agricultural practice by small-scale farming households in Wondo Genet, Southern Ethiopia. SAGE Open 12. doi: 10.1177/21582440221121604
Kavvadias, V., Ioannou, Z., Vavoulidou, E., and Paschalidis, C. (2023). Short-term effects of chemical fertilizer, compost, and zeolite on yield of lettuce, nutrient composition and soil properties. Agriculture 13:1022. doi: 10.3390/agriculture13051022
Kerr, B. R., Hasegawa, T., Lasco, R., Bhatt, I., Deryng, D., Farrell, A., et al. (2022). “Food, fibre, and other ecosystem products,” in Climate Change 2022: Impacts, Adaptation, and Vulnerability. Contribution of Working Group II to the Sixth Assessment Report of the Intergovernmental Panel on Climate Change, eds. H. O. Pörtner, D. C. Roberts, M. Tignor, E. S. Poloczanska, K. Mintenbeck, A. Alegría, et al. (Cambridge; New York, NY: Cambridge University Press), 713–906.
Kifle, T., Ayal, D. Y., and Mulugeta, M. (2022). Factors influencing farmers' adoption of climate-smart agriculture to respond to climate variability in Siyadebrina Wayu District, Central highland of Ethiopia. Clim. Serv. 26:100290. doi: 10.1016/j.cliser.2022.100290
Kirina, T., Groot, A., Shilomboleni, H., Ludwig, F., and Demissie, T. (2022). Scaling climate smart agriculture in East Africa: experiences and lessons. Agronomy 12:820. doi: 10.3390/agronomy12040820
Kuyah, S., Sileshi, G. W., Nkurunziza, L., Chirinda, N., Ndayisaba, P. C., Dimobe, K., et al. (2021). Innovative agronomic practices for sustainable intensification in sub-Saharan Africa. A review. Agron. Sustain. Dev. 41:16. doi: 10.1007/s13593-021-00673-4
Li, X. F., Wang, Z. G., Bao, X. G., Sun, J. H., Yang, S. C., Wang, P., et al. (2021). Long-term increased grain yield and soil fertility from intercropping. Nat. Sustain. 4, 943–950. doi: 10.1038/s41893-021-00767-7
Ma, W., and Rahut, D. B. (2024). Climate-smart agriculture: adoption, impacts, and implications for sustainable development. Mitig. Adapt. Strateg. Glob. Change 29:44. doi: 10.1007/s11027-024-10139-z
Matteoli, F., Schnetzer, J., and Jacobs, H. (2020). “Climate-Smart Agriculture (CSA): An integrated approach for climate change management in the agriculture sector,” in Handbook of Climate Change Management., eds. W. Leal Filho, J. M. Luetz, and D. Ayal (Food and Agriculture Organization of the United Nations (FAO)). doi: 10.1007/978-3-030-22759-3_148-1
Mekonnen, D. F., and Disse, M. (2018). Analyzing the future climate change of the Upper Blue Nile River basin using statistical downscaling techniques. Hydrol. Earth Syst. Sci. 22, 2391–2408. doi: 10.5194/hess-22-2391-2018
Mequanient, M. B., and Kebed, H. H. (2023). Simulation of sediment yield and evaluation of best management practices in Azuari watershed, Upper Blue Nile Basin. H2 Open J. 6, 493–506. doi: 10.2166/h2oj.2023.159
Mersa, M., Tadesse, M., and Zeray, N. (2023). Assessment of implemented physical designs and determinant factors of soil and water conservation measures: Wenago district, southern Ethiopia. Heliyon 9:e13058. doi: 10.1016/j.heliyon.2023.e13058
Moges, D. M., Kmoch, A., Bhat, H. G., and Uuema, E. (2020). Future soil loss in highland Ethiopia under changing climate and land use. Reg. Environ. Change 20:32. doi: 10.1007/s10113-020-01617-6
Mohr, D. L., Wilson, W. J., and Freund, R. J. (2021). Statistical Methods, 4th Edn.. Elsevier. doi: 10.1016/B978-0-12-823043-5.00008-4
Mpairwe, D., and Mutetikka, D. (2022). “Improved feeding for dairy cattle and poultry in smallholder crop-livestock systems,” in CAB International 2022. Sustainable Agricultural Intensification, eds. M. Bekunda, I. Hoeschle-Zeledon, and J. Odhong (Wallingford: CAB International). doi: 10.1079/9781800621602.0008
Musafiri, C. M., Kiboi, M., Macharia, J., Ng'etich, O. K., Kosgei, D. K., Mulianga, B., et al. (2022). Adoption of climate-smart agricultural practices among smallholder farmers in Western Kenya: do socioeconomic, institutional, and biophysical factors matter? Heliyon 8:e08677. doi: 10.1016/j.heliyon.2021.e08677
Nebere, H., Tolossa, D., and Bantider, A. (2021). Analyzing factors affecting the sustainability of land management practices in Mecha Woreda, Northwestern Ethiopia. Sustainability 13:7007. doi: 10.3390/su13137007
Negera, M., Alemu, T., Hagos, F., and Haileslassie, A. (2022). Determinants of adoption of climate-smart agricultural practices among farmers in Bale-Eco region. Ethiopia 8:e09824. doi: 10.1016/j.heliyon.2022.e09824
Nyairo, N. M., Pfeiffer, L., Spaulding, A., and Russel, M. (2022). Farmers' attitudes and perceptions of adoption of agricultural innovations in Kenya: a mixed methods analysis. J. Agric. Rural Dev. Tropics Subtropics 123, 147–160. doi: 10.17170/kobra-202204216055
Oosting, S. J., Mekoya, A., Fernandez-Rivera, S., and van der Zijpp, A. J. (2011). Sesbania sesban as a fodder tree in Ethiopian livestock farming systems: Feeding practices and farmers' perception of feeding effects on sheep performance. Livest. Sci. 139, 135–141. doi: 10.1016/j.livsci.2011.03.009
Ray, R. L., Grifn, R. W., Fares, A., Elhassan, A., Awal, R., Woldesenbet, S., et al. (2020). Soil CO2 emission in response to organic amendments, temperature, and rainfall. Sci. Rep. 10:5849. doi: 10.1038/s41598-020-62267-6
Rayne, N., and Aula, L. (2020). Livestock manure and the impacts on soil health: a review. Soil Syst. 4:64. doi: 10.3390/soilsystems4040064
Sanogo, K., Toure, I., Arinloye, D. A. A., Dossou-Yovo, E. R., and Bayala, J. (2023). Factors affecting the adoption of climate-smart agriculture technologies in rice farming systems in Mali, West Africa. Smart Agric. Technol. 5:100283. doi: 10.1016/j.atech.2023.100283
Serote, B., Mokgehle, S., Du Plooy, C., Mpandeli, S., Nhamo, L., and Senyolo, G. (2021). Factors influencing the adoption of climate-smart irrigation technologies for sustainable crop productivity by smallholder farmers in arid areas of South Africa. Agriculture 11:1222. doi: 10.3390/agriculture11121222
Sharma, A., Soni, R., and Soni, S. K. (2024). From waste to wealth: exploring modern composting innovations and compost valorization. J. Mater Cycles Waste Manag. 26, 20–48 doi: 10.1007/s10163-023-01839-w
Su, Y., Gabrielle, B., and Makowski, D. (2021). The impact of climate change on the productivity of conservation agriculture. Nat. Clim. Change 11, 628–633 doi: 10.1038/s41558-021-01075-w
Tadesse, B., and Ahmed, M. (2023). Impact of adoption of climate-smart agricultural practices to minimize production risk in Ethiopia: a systematic review. J. Agric. Food Res. 13:100655. doi: 10.1016/j.jafr.2023.100655
Teklu, A., Simane, B., and Bezabih, M. (2022). Effectiveness of climate-smart agriculture innovations in smallholder agriculture system in Ethiopia. Sustainability 14:16143. doi: 10.3390/su142316143
Tesfaye, K., Desta, L. T., Demissie, T., Seid, J., Haile, A., Mekonnen, K., et al. (2021). A Framework for Bundling Climate-Smart Agriculture (CSA) and Climate Information Services (CIS) in Ethiopia. AICCRA Technical Report. Addis Ababa: CGIAR Research Program on Climate Change, Agriculture and Food Security (CCAFS).
Thompson, L., Rowntree, J., Windisch, W., Waters, S. M., Shalloo, L., and Manzano, P. (2023). Ecosystem management using livestock: embracing diversity and respecting ecological principles. Anim. Front. 13:vfac094. doi: 10.1093/af/vfac094
Thottadi, B. P., and Singh, S. P. (2024). Climate-smart agriculture (CSA) adaptation, adaptation determinants, and extension services synergies: a systematic review. Mitig. Adapt. Strateg. Glob. Change 29:22. doi: 10.1007/s11027-024-10113-9
Tolesa, A., Mammo, S., and Bohnett, E. (2021). Effects of soil and water conservation structures on selected soil physicochemical properties: the case of Ejersa Lafo District, Central Highlands of Ethiopia. Hindawi Appl. Environ. Soil Sci. 2021:9910237. doi: 10.1155/2021/9910237
Trisos, C. H., Adelekan, I. O., Totin, E., Ayanlade, A., Efitre, J., Gemeda, A., et al. (2022). “Africa,” in Climate Change 2022: Impacts, Adaptation, and Vulnerability. Contribution of Working Group II to the Sixth Assessment Report of the Intergovernmental Panel on Climate Change, eds. H. O. Pörtner, D. C. Roberts, M. Tignor, E. S. Poloczanska, K. Mintenbeck, A. Alegría, et al. (Cambridge; New York, NY: Cambridge University Press), 1285–1455,
Washaya, S., and Washaya, D. D. (2023). Benefits, concerns, and prospects of using goat manure in sub-Saharan Africa. Washaya Washaya Pastoralism 13:28. doi: 10.1186/s13570-023-00288-2
Xu, Z., Li, J., and Ma, J. (2022). Impacts of extension contact on the adoption of formulated fertilizers and farm performance among large-scale farms in rural China. Land 11:1974. doi: 10.3390/land11111974
Yang, Y., Liu, H., and Lv, J. (2022). Response of N2O emission and denitrification genes to different inorganic and organic amendments. Sci. Rep. 12:3940. doi: 10.1038/s41598-022-07753-9
Yimam, A. (2022). Impact of Land Use Land Cover Dynamics on Stream Flow: A Case of Azuari Watershed, Upper Blue Nile Basin, Ethiopia (MSc. thesis). Bahir Dar University. Available at: http://ir.bdu.edu.et/handle/123456789/14650
Zeleke, G., Teshome, M., and Ayele, L. (2024). Determinants of smallholder farmers' decisions to use multiple climate-smart agricultural technologies in North Wello Zone, Northern Ethiopia. Sustainability 16:4560. doi: 10.3390/su16114560
Zerssa, G., Feyssa, D., Kim, D.-G., and Eichler-Löbermann, B. (2021). Challenges of smallholder farming in Ethiopia and opportunities by adopting climate-smart agriculture. Agriculture 11:192. doi: 10.3390/agriculture11030192
Zhao, J., Chen, J., Beillouin, D., Lambers, H., Yang, Y., Smith, P., et al. (2022). Global systematic review with meta-analysis reveals the yield advantage of legume-based rotations and their drivers. Nat. Commun. 13:4926. doi: 10.1038/s41467-022-32464-0
Keywords: farmer decision, determinants to intensified practices, climate-smart agriculture, constraints for wider adoption, Northwest Ethiopia
Citation: Debie E (2024) Intensifying homestead climate-smart agriculture and the challenges to its wider adoption in Azuari watershed, Northwest Ethiopia. Front. Sustain. Food Syst. 8:1410094. doi: 10.3389/fsufs.2024.1410094
Received: 31 March 2024; Accepted: 14 November 2024;
Published: 02 December 2024.
Edited by:
Andrew John Dougill, University of York, United KingdomReviewed by:
Bereket Tsehaye Haile, University of Pretoria, South AfricaCopyright © 2024 Debie. This is an open-access article distributed under the terms of the Creative Commons Attribution License (CC BY). The use, distribution or reproduction in other forums is permitted, provided the original author(s) and the copyright owner(s) are credited and that the original publication in this journal is cited, in accordance with accepted academic practice. No use, distribution or reproduction is permitted which does not comply with these terms.
*Correspondence: Ermias Debie, ZXJtaTI3MjAwNEBnbWFpbC5jb20=
Disclaimer: All claims expressed in this article are solely those of the authors and do not necessarily represent those of their affiliated organizations, or those of the publisher, the editors and the reviewers. Any product that may be evaluated in this article or claim that may be made by its manufacturer is not guaranteed or endorsed by the publisher.
Research integrity at Frontiers
Learn more about the work of our research integrity team to safeguard the quality of each article we publish.