Corrigendum: The potential use of brewers' spent grain-based substrates as horticultural bio-fertilizers
- 1Joint Research Unit - Microbial Resource Research Infrastructure (JRU MIRRI-IT), Sassari, Italy
- 2Department of Agricultural Sciences of University of Sassari, Sassari, Italy
- 3Department of Agronomy, Food, Natural Resources and Environment – DAFNAE, University of Padua, Legnaro, PD, Italy
- 4Institute of Sciences and Technologies for Sustainable Energy and Mobility (STEMS) of the National Research Council of Italy (CNR), Turin, Italy
- 5Desertification Research Center (NRD), Sassari, Italy
Introduction: Brewers' spent grain (BSG) is the most important by-product of breweries. Currently, the primary method of recycling BSG is through its use in livestock feed production, due to its high fiber content, non-degradable protein, and water-soluble vitamins. Nonetheless, composting, vermicomposting, and biochar production pathways offer promising alternatives for managing this organic byproduct.
Methods: BSG-based substrates were evaluated as bio-fertilizers for use during the transplantation of Lactuca sativa L. and without additional fertilization at the end of the crop cycle. Biochar (B), vermicompost (V), compost (CP), and compost enriched with a microbial consortium (CPE), each mixed at 2 and 4% with peat, were compared with two control treatments: 100% peat (C1) and peat with ternary chemical fertilizer (C2). The experiment was designed with six replicates, half of which were inoculated with Sclerotinia sclerotiorum mycelium during transplantation to assess the contribution of different substrates to pathogen resistance.
Results: Overall, it was found that the control treatment C2 was the most effective, yielding the greatest plant height at the seedbed stage and the best results across all parameters at harvest. The V 4%, CP 4%, and CPE 4% treatments showed similar results without significant differences compared to the control. At the end of the seedbed phase, plants treated with CP 4% and CPE 4% exhibited the most substantial root development, with statistically significant differences from all other treatments. Moreover, the CPE 4% treatment demonstrated superior performance, resulting in statistically significant differences (p < 0.05) in the chlorophyll content (α and β) and carotenoid parameters when compared to the control treatment C2. The interaction between the treatment and the pathogen was statistically significant only for the carotenoid content in the CPE 4% treatment and for the flavonoid content in most of the treatments.
Discussion: The most promising results were obtained at the nursery phase, highlighting the potential use of processed BSG-based substrates as bio-fertilizers. These products provided plants with both a growth substrate and nutrients, effectively contributing to waste recycling and aligning with the principles of a circular economy. Additional studies are required to investigate the potential use of brewer's spent grain as bio-fertilizers in other vegetable crop species.
1 Introduction
In the era of climate change and eco-sustainability, recycling waste into valuable resources is crucial. In this context, brewers' spent grain (BSG), which is generated during the malting and mashing processes, constitutes 85% of the by-products from brewing and has an estimated annual global production of ~40 million tons (Bianco et al., 2020). BSG is a cost-effective raw material, available year-round, whose carbon and nitrogen sources make it appealing for various biotechnological processes.
Currently, the method of recycling BSG is through its use in livestock feed production, due to its high fiber content, non-degradable protein, and water-soluble vitamins. However, BSG is rapidly perishable due to its microbiological instability (high moisture content and the presence of fermentable sugars), resulting in a shelf life of 2–10 days, depending on the storage conditions. Moreover, the drying process of this biomass has a high environmental impact, which is estimated to be 495–770 kg CO2e qv t−1 BSG. Generally, 70% of BSG is used for animal feed, 10% is used for biogas production, and the remaining 20% is still disposed of in landfills, with an environmental impact of 513 kg CO2e qv t−1 BSG (Pérocheau, 2024).
In this context, composting (Tabrika et al., 2020; Vigoroso et al., 2021; Al-Tawarah et al., 2024), vermicomposting (Saba et al., 2019; Bianco et al., 2022), and biochar production (Choi et al., 2018; Manolikaki and Diamadopoulos, 2020; Xi et al., 2020) could serve as viable alternatives for organic waste management. These methods not only reduce landfill disposal but also facilitate the recycling of agronomic macronutrients (nitrogen, phosphorus, and potassium) and enhance carbon sequestration through their application in agriculture (Moretti et al., 2020).
In horticultural crops, integrating compost and vermicompost into agricultural systems positively affects soil physicochemical and biological characteristics, thereby enhancing soil quality and fertility (Urra et al., 2019; Raza et al., 2024). In particular, these kind of bio-fertilizers enhance soil fertility, improving aggregation, hydraulic conductivity, total porosity, and cation exchange capacity (CEC) (Singh et al., 2022), supplying a wide range of nutrients (N, P, K, Ca, Mg, etc.) (Cacace et al., 2022; Walia and Kaur, 2024). Moreover, previous studies (Budroni et al., 2020; Bianco et al., 2022, 2024) have highlighted that processed BSG (i.e., compost and vermicompost) are rich in microbial consortia including lactic acid bacteria (Weissella, Pediococcus), plant growth-promoting bacteria (Bacillus, Pseudomonas, Pseudoxhantomonas), biostimulant yeasts (Pichia fermentans), and fungi (Trichoderma reesei, Beauveria bassiana), which can improve soil fertility, enhance resistance to abiotic and biotic stressors, and act against fungi and insect pests.
Microbial-based fertilizers offer a solution to mitigate the environmental harm caused by excessive or improper use of chemical fertilizers, enhancing both direct and indirect plant uptake of macro- and micro-nutrients (Atieno et al., 2020). Moreover, chemical fertilizers can adversely affect the quality and safety of water, soil, and air resources, not only through their application in agriculture but also as a result of their production processes (Yin et al., 2022; Aso, 2024).
Leafy vegetables, such as lettuce, respond positively to organic fertilizers (Yassen et al., 2020). The use of these fertilizers offers several advantages, including simplicity, reliability, and cost effectiveness, all without the need for significant equipment (Radziemska et al., 2019; Yassen et al., 2020).
In our previous study (Assandri et al., 2023), we assessed the effects on lettuce growth in a short-term pot experiment, using plants as a test subject. BSG-based compost and pelletized compost, both enriched with a microbial consortium as fertilizers intended to replace chemical fertilizers, were compared. The results showed that the growth effects of BSG-based substrates are comparable to those achieved with mineral fertilizer treatments.
Nursery production is widely considered as the most reliable method compared to direct seeding for ensuring the proper development of a wide range of commercially valuable horticultural crops. Since substrates can directly or indirectly influence plant growth and development, it is crucial to identify the appropriate components of the substrate to optimize growth outcomes. Therefore, in this study, starting from the seedbed stage, a combined experimental design for the characterization of BSG-based substrates was used. This study aimed to assess: (i) the effectiveness of BSG-based substrates (compost with and without a microbial consortium, vermicompost, and biochar) at the nursery stage of Lactuca sativa L. var. capitata “Regina dei ghiacci”; (ii) the effects of these BSG-based substrates on plant productivity and physiology after being transplanted into pots without additional fertilization; and (iii) the resistance of the plants to Sclerotinia sclerotiorum (Lib.) de Bary, the pathogen responsible for collar rot disease, following transplantation.
2 Materials and methods
2.1 Brewers' spent grain-based substrates: vermicompost, compost, and biochar
The process of vermicomposting pre-treated BSG was carried out following the methodology described by Bianco et al. (2022). A single area measuring 1 × 1 m was filled with a mixture of 10 kg of pre–treated BSG and 90 kg of cow manure, supplemented with 5 kg of earthworms (Eisenia fetida, Savigny, 1826). Upon completion of the worm-cast production phase (90 days) and the subsequent curing period (30 days), fully matured vermicompost suitable for direct soil application was obtained. The composting process of BSG was carried out as described by Assandri et al. (2021). Enriched compost was obtained by incorporating a commercial microbial consortium, in the form of a wettable powder with a concentration of 3 g L−1. Biochars were produced from pre-dried BSG at 45°C for 48 h. Subsequently, the dried BSG was subjected to pyrolysis inside a carbolite furnace, Gallenkamp Muffle Furnace model, at temperatures of 350 and 550°C (Rodríguez-Vila et al., 2018). The BSG was loaded into steel containers (diameter 10.00 cm and height 18.20 cm) and covered with a perforated lid to prevent overpressure. The containers were heated in the carbolite furnace to the specified temperature and then held for 1 h before being allowed to cool overnight. The biochar was then removed (Rodríguez-Vila et al., 2022). During pyrolysis, there was no automatic air control, and low oxygen/anoxic conditions were maintained by loading feedstock into the steel containers and saturating them with feedstock materials. Moreover, the containers are closed then air cannot get inside the steel containers after closing them. BSG-based substrates used in the seedbed experiment and the soil collected from the surface horizon (0–30 cm) for the pot experiment were analyzed according to the Italian Official Methods of Soil Analysis (Ministero delle Politiche Agricole e Forestali, 1999). Microbiological analyses were performed on the BSG-based substrates following the method described by Bianco et al. (2022). The physicochemical characteristics and microbial count are shown in Tables 1, 2.
2.2 Culture-dependent microbiological analysis of soil and BSG-based substrates
Enumeration of Escherichia coli was carried out according to the ISO 16649-2 (2001). The incidence of Salmonella spp. was determined as described in ISO 6579-1 (2017). Before carrying out the pathogenicity tests with S. sclerotiorum, the soil used in the tests was analyzed to exclude the presence of any pathogens. A “soil dilution plate technique” was used (Burgess et al., 1994), where 1 g of soil was suspended in a flask containing 99 ml of 0.05% water agar. Furthermore, 1 mL of the soil suspension was spread onto Potato Dextrose Agar (PDA) plate, and three replicates were performed. The plates were incubated at 25°C with a 12 h photoperiod. After 7 days, the colonies were transferred to new PDA plates, and monosporic or monohyphal cultures were performed. The mycelium (~100 mg) from the pure cultures obtained was used the DNA extraction. Amplification of the internal transcribed spacer (ITS) region was carried out (Chen et al., 2011).
2.3 Phytotoxicity tests of BSG-based substrates
The germination test was carried out on cress (Lepidium sativum, L. purchased from Fratelli Ingegnoli, Milano, Italy) and lettuce (Lactuca sativa L. var. capitata “Regina dei ghiacci”) seeds (Pagano Domenico & figli, Salerno, Italy) according to the methodology described by Zucconi et al. (1981). Vermicompost, compost, and biochar were mixed with distilled water in a 1:10 (w/v) ratio and shaken at 150 rpm for 1 h. They were then centrifuged at 4,000 rpm for 20 min and filtered through 0.45 μm Millipore membrane filters. Dilutions were prepared from each sample using distilled water: 50% (50% extract, 50% water), 75% (75% extract, 25% water), and control (only distilled water). Whatman sterilized filter paper was moistened with 5 ml of extract and placed inside a Petri dish of 9 cm of diameter. Ten seeds of L. sativum and L. sativa were placed on the filter paper, and the Petri dishes were incubated in darkness at 25 ± 2°C. After 3 days of incubation, the percentages of germination and root length were recorded. The germination index (GI) was calculated with the mean value from three experiments as follows: GI (%) = [(Gt %)/(Gc %)] × [Lt/Lc] × 100; where Gt% and Gc% are the mean percentages of germination with the extract treatment and the control, respectively, and Lt and Lc are the mean root lengths with the extract treatment and the control, respectively (Mohamed et al., 2020).
2.4 Plant experiments and growing conditions
This short-term pot experiment took place during the winter/spring season (from February to May 2022) in a Mediterranean greenhouse at the Ottava experimental station of the University of Sassari (40°46′ 47" N, 8°29′ 45" E). The greenhouse was unheated, and the average minimum air temperature during the trial was 5°C, while the average maximum air temperature was 16°C. The average heliophany was ~421.45 min day−1.
The trial was performed using BSG-based substrates, especially vermicompost (V), compost (CP), compost enriched with the microbial consortium (CPE), and biochar obtained from 350 and 550°C pyrolysis temperatures (B350 and B550), mixed with peat at two different percentages, 2 and 4%, and compared with two control treatments, C1 and C2, consisting of 100 % peat and peat fertilized with a ternary chemical fertilizer (NPK 20–20–20), respectively (Figure 1, Table 3). In the control treatment C2, a nitrogen amount equivalent to 35 kg ha−1 was provided.
The seedbed phase extended for 43 days from seeding to transplanting, while the crop cycle lasted 58 days from transplanting to harvesting the lettuce heads. Overall, the entire experiment lasted for 101 days. In both growth phases, a completely randomized block design was adopted.
In the seedbed phase, 12 replicates per treatment were performed with five lettuce seeds for each phytocell (approximately volume 60 ml plug−1) per 2 plastic seedbeds (18 × 13.5 × 5 cm; 12 phytocells). Upon reaching the stage of three true leaves, 43 days after seeding, six plants (six replicates) per treatment were randomly selected for the evaluation of quantitative parameters (Table 5). The remaining six plants were transplanted into pots (20 × 9.5 cm), each filled with 500 g of soil, for a total of 72 pots. The phenological stage of the three true leaves and the harvesting time were assessed using the BBCH scale (Biologische Bundesantalt, Bundessortenamt, and CHemische Industrie, Berlin, Germany).
The plants were transplanted into pots without further inputs of chemical fertilizers in the control treatment and without the addition of further BSG-based substrates in the organic treatments.
During the crop cycle phase, the pots were irrigated twice a week with 500 mL of distilled water. The plants were inoculated with the pathogen (S. sclerotiorum) 10 days before transplanting, dividing the total of 72 pots into two groups: 36 pots with the pathogen (Y) and 36 pots without the pathogen (N). The inoculum was added as 3 plugs of fungal mycelium (1 cm in diameter) per pot of a 10-day-old culture of S. sclerotiorum 10 days before transplanting. Agar plugs without mycelium were used in the control pots.
2.4.1 Analytic methods for biometric parameters
At the time of transplantation (t), the following biometric parameters were evaluated: plant height (HPt), plant (PFWt), root fresh weight (RFWt), and root length (RLt). At the harvesting time, leaf number (LN), plant (PFW) and leaves (LFW) fresh weights, and leaf area per number of leaf (LA/LN) were evaluated. In addition, chlorophyll α (Chl α), β (Chl β), total carotenoids (Carot), anthocyanin (Anth), and flavonoid (Flav) were evaluated. Dry weight of the plants and root (PDWt and RDWt, respectively), plant height (HP), and root fresh weight (RFW) are shown in the Supplementary material. The dry weights were obtained after drying the plant tissues at 60°C for 72 h.
Leaf area (LA, cm2) was estimated using a planimeter (LI–COR, model 3100 area meter, Germany). The contents of chlorophyll (α and β) and carotenoids were estimated according to the method described by Lichtenthaler and Buschmann (2001) with a slight modification (Pompeiano et al., 2013).
Finally, anthocyanin and flavonoid contents were assessed using Dualex™ (FORCE–A, Orsay, France) based on the chlorophyll fluorescence excitation spectra (Cartelat et al., 2005), with five readings for each leaf.
Additionally, the nitrate content of the leaf was determined following the method outlined by da Silva et al. (2020). The filtrate was transferred to the Allsep™ Anion IC Column. An Alltech ion chromatography system, consisting of an HPLC pump, model 426, a column heater, model 630, suppressor, model 640, a conductivity detector, model 650, and an injector port with 100 μL loop, was used for anion () determination. The anion separation column was Allsep™ Anion IC Column.
2.5 Enzymatic activities
After 58 days of plant growtht, fresh root-adhering soil samples were collected from all pots receiving the same treatment, bulked together, sieved to <2 mm, and used for the analysis dehydrogenase (DHG) and urease activity (URE). The DHG activity was determined by the amount of triphenyltetrazolium red developed from soil samples treated with triphenyltetrazolium chloride at 37°C for 24 h of incubation. The URE activity was determined by quantifying the ammonia released from soil samples treated with urea during 2 h of incubation at 37°C (Alef and Nannipieri, 1995).
2.6 Statistical analysis
The analyses were conducted using R statistic software version 4.2.3 (2022–04–22 ucrt) with the utilization of the “car,” “emmens,” “multicomp,” “factoextra,” and “multicompView” packages. The data were analyzed through a generalized linear model (GLM) with a Gaussian distribution. Assumptions of homogeneity of variances, normality, and independence of the residuals were graphically checked. The two phases (seedbed and harvest) were analyzed separately because, in the first one, only the treatment was considered as a factor, while in the second experiment, both the treatment and the presence of the pathogen were considered as factors. In this case, the assessment of the significance of fixed and interacting factors in linear models were carried out using the “join_tests” of the “emmeans” package. In both experiments, the significance of the differences between mean values was assessed using the Sidak test at p < 0.05. The principal component analysis was carried out using normalized phenotypic and biochemical data collected at the end of the second experiment. Clusters represented in the PCA biplot were determined by the k–means algorithm, as implemented in the “stats” package of R software.
3 Results
3.1 Phytotoxicity: quality and safety parameters of compost, vermicompost, and biochar
All the tested BSG-based substrates showed a positive effect on germination rates of L. sativum and L. sativa seeds when used up to a concentration of 50% (Table 4), exceeding the minimum GI required as per Annex 2 of Legislative Decree 75 of 29 April 2010.1 The GI values recorded at 75% show a reduction in the germination of seeds.
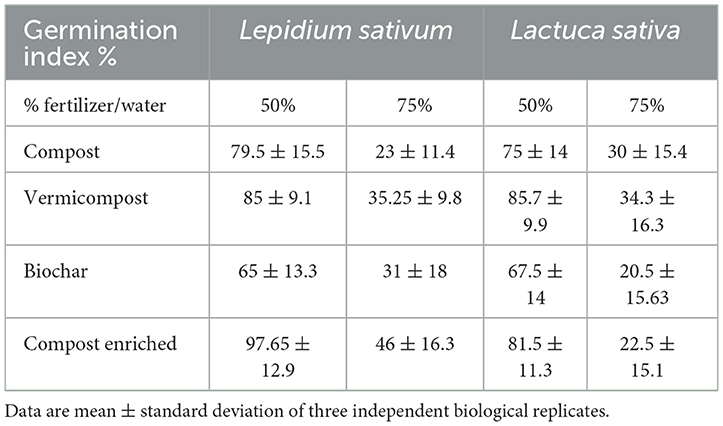
Table 4. Germination index of watercress and lettuce seeds for two percentages of horticultural fertilizer extracts.
Salmonella and E. coli were not detected in any of the tested BSG-based substrates, in compliance with Legislative Decree No. 75/2010. The molecular identification of Ottava soil confirmed the absence of lettuce pathogens.
3.2 Effects of different BSG-based substrates on lettuce growth
At the transplant time (Table 5), significant differences (p < 0.05) among treatments were found in terms of HPt, PFWt, and RLt.
The HPt ranged from 0.6 ± 0.05 cm (B350 2%) to 2.6 ± 0.55 cm (C2), with the latter proving to be the most effective treatment, statistically different from all others. On the other hand, the B350 2% treatment, despite being less effective, was only statistically different from the CP 4%, CPE 4%, and C2 treatments. The lowest RLt was observed in the B350 2% treatment, measuring 1.3 ± 0.75 cm, which was statistically different from the CP 4%, CPE 4%, and V 4% treatments. The highest RLt values were recorded at 9.0 ± 1.27 cm for the CP 4% treatment and 8.5 ± 2.43 cm for the CPE 4% treatment, both of which were statistically different from all other treatments (p < 0.05). Both control treatments (C1 and C2) showed average root length (RLt) without significant differences compared to all other BSG-based substrate treatments (p > 0.05). Similar to HPt, the highest average value for PFWt was observed under the C2 treatment at 0.08 ± 0.03 g, which was statistically different from all other treatments (p < 0.05). However, the lowest value of 0.01 g was recorded under B350 2%, B550 2%, CP 2%, and V 2% treatments. No significant differences (p > 0.05) emerged for root fresh weight (RFWt) that ranged from 0.003 ± 0.00 g in the B350 2% treatment to 0.03 ± 0.06 g under the C1 control treatment.
Considering that the edible part of L. sativa corresponds to the fresh plant material, the relative dry weight of plants and roots were also determined. In both cases, the best performing treatment was CPE 4%, while the least effective was B550 4% (Supplementary material). This analysis revealed a similar trend across the parameters evaluated.
Overall, in the absence of the pathogen, at the time of harvest (Table 6), the control treatment C2 met the expectations, proving to be the most productive treatment, with the highest fresh weight of plants (PFW), leaves (LFW), number of leaves (LN), and leaf area (LA/LN), with average values of 36.0 ± 10.43 g, 30.5 ± 9.95 g, 16.3 ± 0.58 leaves, and 390 ± 181 cm2, respectively (Figure 2). While the lowest PFW and LFW were observed in the B350 2% treatment, with 15.7 ± 0.58 g and 12.3 ± 1.15 g, respectively. Considering the parameters, such as number of leaves (NL) and LA/LN, the lowest values, 9.5 ± 1.50 and 226 ± 120 cm2, respectively, were found in the control treatment C1.
Even in the presence of biotic stress, the control treatment C2 showed the highest values in all parameters except for LA/LN. The highest leaves area (LA/LN) was recorded in the B 550 4% treatment, with an average value of 268 ± 159 cm2. On the contrary, the treatment with the lowest values for PFW, LN, and LA/LN was the control treatment C1, with average values of 8.8 ± 0.68 g, 9.0 ± 1.00 number of leaves and 126 ± 10.0 cm2, respectively. The lowest LFW was observed in the B350 2% treatment with 6.6 ± 5.39 g.
The V 4%, CPE 4%, and CPE 2% treatments exhibited statistically similar values (p > 0.05) across all four parameters, both in the absence and presence of the pathogen. Additionally, the CP (2 and 4%), B550 (2 and 4%), and B350 4% treatments did not show statistically significant differences in the PFW, LFW, and LA/LN parameters when compared to the C2 treatment.
Significant differences caused by the treatment–pathogen interaction in the carotenoid content for the CPE 4% treatment and in the flavonoid content for the majority of treatments were found (Table 7). The highest flavonoid content was recorded in the B350 2% parameter (0.84 ± 0.02 mg kg−1), showing no statistically significant difference from all other pathogen-treated treatments. While the lowest flavonoid content, statistically different from all treatments, was observed in the treatment C1 (without pathogen) with an average value of 0.30 ± 0.03 mg kg−1. The C2 treatment (0.71 ± 0.00 mg kg−1) was determined to be statistically similar to all the remaining non-inoculated treatments.
The highest chlorophyll (α and β) and carotenoid contents were measured in the CPE 4% treatment (without pathogen) with average values of 17.9 ± 4.64 mg kg−1, 5.7 ± 1.75 mg kg−1, and 1428 ± 346 mg kg−1, respectively. On the other hand, the lowest values of chlorophyll α and carotenoid contents were observed, in the presence of the pathogen, under the control treatment C2, with average values of 7.6 ± 1.85 mg kg−1 and 658 ± 149 mg kg−1, respectively.
In addition, the HPLC analysis of nitrates was conducted on lettuce leaves, both with and without the pathogen. The results showed, for all treatments, the nitrate content below the limits specified for greenhouse-grown lettuce harvested between April 1st and September 30th.2 These findings were not shown in the data.
Principal component and cluster analyses (Figure 3) allowed the subdivision of the different treatments in five different groups. Treatments included in Group 3, consisting of the C1 treatment in the presence and absence of the pathogen, exhibited the lowest mean values of all the analyzed parameters. In contrast, Cluster 4 was characterized by treatments with the highest mean values, including B550 4%, CPE 4%, CP 4%, and C2. Of particular interest is the CPE 4% treatment, which exhibited higher levels of chlorophyll α, chlorophyll β, and carotenoids.
3.3 Enzymatic activities
The DHG activity exhibited a significant increase across all pots containing BSG-based substrates, ranging from a minimum of ~1.40–fold for the V 4% treatments to a maximum of ~3.52–fold for the B350 2% treatment (Figure 4). For all BSG-based substrates (except CPE), 2% was more effective to increase DHG (between 1.61- and 2.42-fold) than 4%. On the other hand, the lowest DGH activity (214.80 μg TPF g−1 h−1) was recorded in the B550 4% treatment in the presence of the pathogen. Additionally, in this case, irrespective of the pathogen presence except for vermicompost, the lower rate (2%) of BSG-based substrates was more effective to increase DHG (between 1.22- and 3.18-fold) than the 4%.
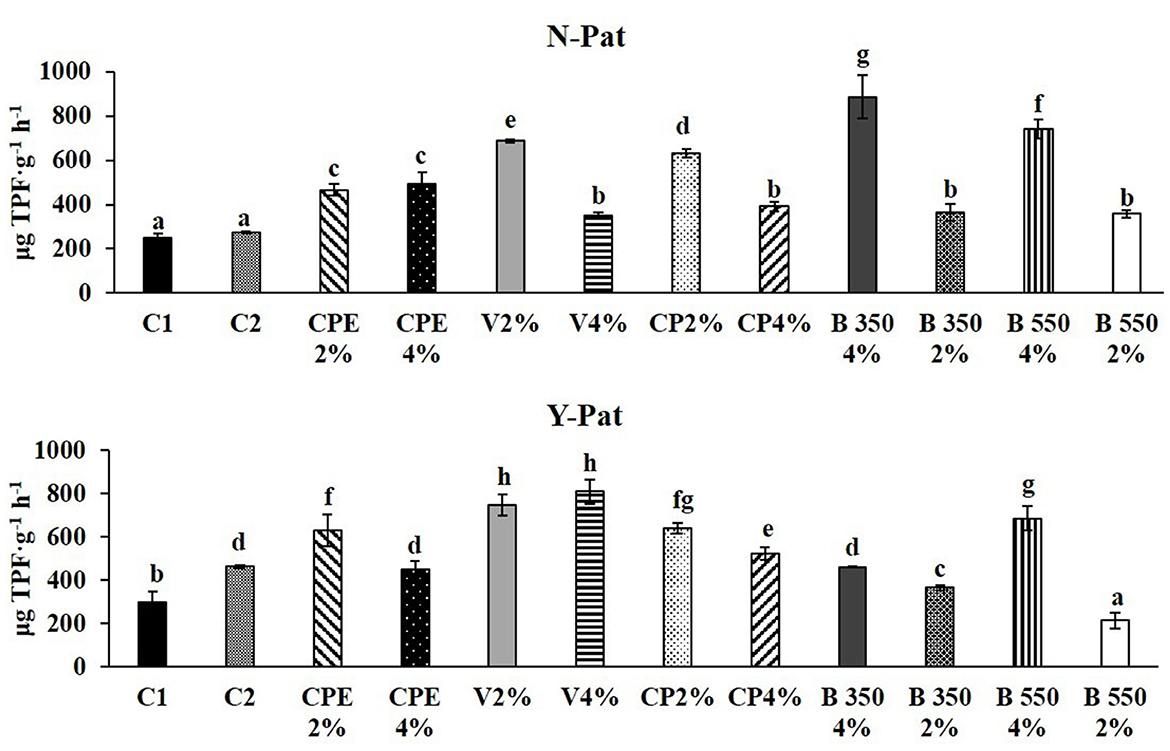
Figure 4. Enzyme activities for dehydrogenase (DHG) in fresh root-adhering soil samples, without and with the pathogen (N–Pat and Y–Pat). Data are expressed as mean ± standard deviation. The data marked with different letters indicate statistically significant differences among the treatments after plant growth, while the (p < 0.05; Fisher's least significant difference tests).
As regards the N cycle, the URE activity increased for all BSG-based substrates at 2% except for CPE and B350 (Figure 5) more than those applied at 4% and when compared to the controls (C1 and C2). The most effective BSG-based substrate to enhance URE activity was B350 4% (52.21 μg NH4-N g−1 h−1), while the less effective was B550 4% (35.03 μg NH4-N g−1 h−1).
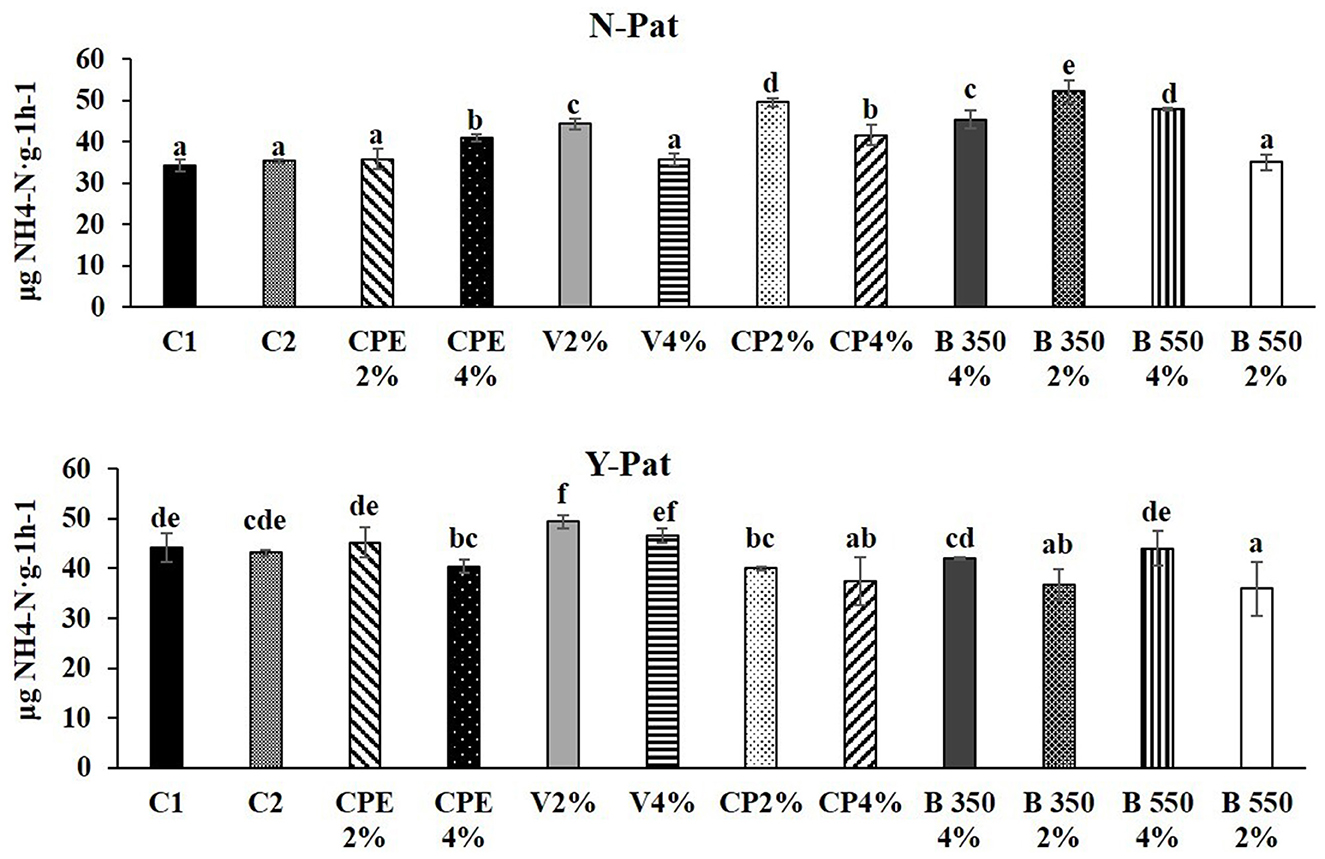
Figure 5. Enzyme activities for urease (URE) in fresh root–adhering soil samples without and with the pathogen (N–Pat and Y–Pat). Data are expressed as mean ± standard deviation. The data marked with different letters indicate statistically significant differences among the treatments after plant growth, while the (p < 0.05; Fisher's least significant difference tests).
Generally, in the presence of the pathogen, the BSG-based substrates application was not effective or reduced the URE activity compared to the controls (C1 and C2). The only exception was vermicompost, especially V 2% that increased URE by 1.18-fold compared to C1 and C2. The lowest value of the URE activity was recorded for B550 4% (35.99 μg NH4-N g−1 h−1) in the presence of the pathogen (Figure 5).
4 Discussion
BSG contains a considerable amount of nutrients that are beneficial for both animals and microorganisms (Bianco et al., 2020). In addition to nutrients, BSG is also rich in moisture content, which induces microbial spoilage, thereby posing environmental and health-associated issues. Microorganisms can be utilized to transform and valorize BSG through vermicomposting or solid-state fermentation processes, as a sustainable method to reduce the carbon footprint with a minimum use of land resources. The use of agri-food wastes-based culture media to produce bio–fertilizers rich in soil-beneficial microbial species represents a promising and environmentally friendly innovation that meets the current needs of sustainable agriculture (Nadeem et al., 2017; Nguyen et al., 2017; Guan et al., 2023; Bianco et al., 2024).
Finding substrates for horticultural crops that fully or partially substitute the use of chemical fertilizers during both the seedling and cultivation phases is essential.
In our previous study (Assandri et al., 2023), we evaluated positively the short-term benefits, not only as amendments but also as fertilizers, arising from the use of BSG-based substrates on lettuce growth. Additionally, we observed that both the pelleted compost and pelleted microbial-enriched compost contribute to a more active and diverse soil microbial community compared to NPK fertilization, leading to an improvement in soil quality.
In this study, the emphasis is on the ability of BSG-based substrates to provide nutrients during the seedbed phase, assessing their residual effects (without additional nutritional inputs) throughout the crop cycle (58 days), including an increased resistance to S. sclerotiorum in plants. The germination index (GI) of compost, enriched compost, biochar, and vermicompost, using cress and lettuce seeds, highlighted the non-phytotoxicity of BSG-based substrates even at high concentrations.
In the seedbed phase, the control treatment C2 (peat + NPK) shows the highest values in height and fresh weight parameters, likely due to the readily available nutrients (Hoque et al., 2010). Lettuce cultivated with compost (CP 4%) and enriched compost 4% (CPE 4%) showed superior root development. According to Kramer-Walter et al. (2016), roots tend to experience greater growth under conditions of limited nutrient availability. In contrast, Ryser (2006) observed that fast-growing species in productive habitats have a higher specific root length (SRL) compared to species in stressed environments. An increased nutrient supply has been linked to a higher SRL, especially when root proliferation occurs in a concentrated nutrient patch. Notably, compost treatments (at the highest percentage) exhibited greater root growth compared to other bio-fertilizer treatments. This could be attributed to the presence of humic and fulvic acids, which are characteristic components of compost (Canellas et al., 2015; Qin and Leskovar, 2020). The increase in root development is positively considered by nursery growers, as it is often correlated with improving plant transplantation success by reducing transplant stress (Bignami et al., 2023; Melito et al., 2023).
Even at the time of harvest, the control treatment C2 emerged as the best in relation to the considered productivity parameters. However, treatments with BSG-based substrates (both in the presence and absence of the pathogen), especially compost (CP), enriched compost (CPE), and 4% vermicompost (V 4%), exhibited high average values in the considered productivity parameters, with no significant differences compared to the C2 treatment. On the contrary, the less productive treatments were both in the presence of the pathogen, namely C1 for the parameters FWP, NL, and LA/LN, and B350 2% for the parameter FWL. No significant differences emerged between the treatments inoculated with the pathogen; however, it is possible to notice (Table 5) a positive growth trend of the plants in the treatments with the substrates, especially in B 550 4%, CP 4%, and CPE 4%.
A trend of higher anthocyanin production is observed in the presence of S. sclerotiorum inoculum, compared to the plants grown in the absence of the pathogen. This suggests a stress signal induced by the pathogen addition, even in the absence of characteristic symptoms on the plants. Meanwhile, the treatment–pathogen interaction was significant in the flavonoid content, highlighting significant differences between plants grown in the presence and absence of the pathogen. In general, the presence of environmental stress condition, such as the presence of pathogens can activate various defense responses in plants (Macioszek et al., 2023), including the production of chemical compounds such as flavonoids and anthocyanin (Harbart et al., 2023).
Regarding enzymatic activities, the enhanced DHG in root adhering soils could be attributed to the high nutrient content of the BSG-based substrates that could have increased the microbial activity (Garau et al., 2021; Song et al., 2023; Zhang et al., 2023), but at the same time, bio-fertilizers, especially at the higher level, could have affected the microbial community structure in terms of size and composition. This last aspect could have favored microorganisms sensitive to triphenyltetrazolium chloride (TTC) used in the DHG quantification. Wolińska et al. (2016) highlighted that TTC applied during the DHG quantification can affect the DHG activity explicating toxic effects on the microbial community in different soils.
On the other hand, the combined interaction between plants, microbial community, and bio-fertilizers affected the N cycle in root-adhering soil. Specifically, the N inputs provided by bio-fertilizers at higher rates might have satisfied the N demands of the microbial community, consequently inhibiting the stimulation of urease synthesis (Garau et al., 2019). Peng et al. (2019) observed a negative correlation between the total nitrogen content in amended soils and urease activity. Furthermore, bio-fertilizers have the capacity to immobilize ureases within the humic matrix, forming humus–enzyme complexes that can adversely impact urease activities (Lucas et al., 2018).
Positive effects resulting from the use of organic substrates, both as actual growth substrates in the seeding phase (Dhen et al., 2018; Gomah et al., 2020; Qin and Leskovar, 2020; Sarkar et al., 2021; Guan et al., 2023; Rozas et al., 2023) and as bio-fertilizers during the crop cycle (Suwor et al., 2020; Yoo et al., 2021; Melito et al., 2023; de Lima et al., 2024), were observed. Therefore, the utilization of these BSG-based substrates could be promoted as horticultural bio-fertilizer and valid alternatives to chemical fertilizers, both during the seedbed phase and throughout the crop cycle. This approach aims to reduce the risks associated with the production and use of mineral fertilizers (Papathanasiou et al., 2012; Jara-Samaniego et al., 2017; Atieno et al., 2020).
5 Conclusion
In this study, the BSG-based substrates were assessed as bio-fertilizers, providing an alternative to mineral fertilizers. The notable root development observed highlights their potential use as bio-fertilizers, particularly beneficial for promoting the growth of leafy vegetable crops during the nursery stage. These bio-fertilizers are used in organic farming as well, derived from easily manageable and controllable biological processes. This makes them well-suited for companies with limited quantities of by-products, necessitating precise dosages of self-produced bio-fertilizers.
The findings suggest the necessity for further exploration, encompassing a broader range of vegetable species, including those with extended growth cycles such as tuberous and fruit vegetables. Furthermore, future research should investigate their efficacy in mitigating potential pathogens.
Data availability statement
The raw data supporting the conclusions of this article will be made available by the authors, without undue reservation.
Ethics statement
The manuscript presents research on animals that do not require ethical approval for their study.
Author contributions
AB: Conceptualization, Data curation, Formal analysis, Methodology, Supervision, Writing—original draft, Writing—review & editing. SM: Conceptualization, Data curation, Supervision, Writing—original draft, Writing—review & editing. MG: Conceptualization, Data curation, Supervision, Writing—original draft, Writing—review & editing. VG: Formal analysis, Supervision, Writing—review & editing. GZ: Conceptualization, Data curation, Formal analysis, Supervision, Writing—original draft, Writing—review & editing. DA: Conceptualization, Data curation, Formal analysis, Supervision, Writing—original draft, Writing—review & editing. SO: Formal analysis, Methodology, Supervision, Writing—original draft, Writing—review & editing. RC: Writing—original draft, Writing—review & editing. NP: Supervision, Writing—review & editing. MB: Conceptualization, Data curation, Funding acquisition, Supervision, Validation, Writing—original draft, Writing—review & editing, Methodology.
Funding
The author(s) declare financial support was received for the research, authorship, and/or publication of this article. This work was financially supported by the Sardinia Regional Government (art.7 L.R. 16/2014 and art.10 L.R. 194/2015 CUP E77G22000470002) and within the framework of the project “Sviluppo sostenibile della birra artigianale in Sardegna”—C.U.P. J84I18000070006. The Italy's National Recovery and Resilience Plan (Project SUS-MIRRI.IT). MB and GZ acknowledge the support provided by the University of Sassari (Fondo di Ateneo per la Ricerca, 2020).
Acknowledgments
The authors thank Agostino Piredda and Benedetta Scalas (Azienda Mauro Deidda, Ottava) for providing technical support. They also extend their gratitude to Giovanni Saba (Gena Prato), Sara Saba for supporting vermicompost and compost production, and to Mario Antonello Deroma (Department of Agricultural Sciences, University of Sassari, Italy) for the chemical analysis of soil and BSG-based substrates. Special thanks to Professor Tom Sizmur of the University of Reading for providing access to laboratories, instrumentation, and expertise for the production of biochars.
Conflict of interest
The authors declare that the research was conducted in the absence of any commercial or financial relationships that could be construed as a potential conflict of interest.
Publisher's note
All claims expressed in this article are solely those of the authors and do not necessarily represent those of their affiliated organizations, or those of the publisher, the editors and the reviewers. Any product that may be evaluated in this article, or claim that may be made by its manufacturer, is not guaranteed or endorsed by the publisher.
Supplementary material
The Supplementary Material for this article can be found online at: https://www.frontiersin.org/articles/10.3389/fsufs.2024.1404914/full#supplementary-material
Footnotes
1. ^Decreto Legislativo 29 aprile 2010, n. 75. (2010). Riordino e revisione della disciplina in materia di fertilizzanti a norma dell'articolo 13 della legge 7 luglio 2009, n. 88 (in Italian language).
2. ^European Commission Regulation 1881 (2006). (2006). COMMISSION REGULATION (EC) No 1881/2006 of 19 December 2006 setting maximum levels for certain contaminants in foodstuffs.
References
Alef, K., and Nannipieri, P., (eds.). (1995). Methods in Applied Soil Microbiology and Biochemistry, 1st Edn. London; San Diego, CA: Academic Press.
Al-Tawarah, B., Alasasfa, M. A., and Mahadeen, A. Y. (2024). Efficacy of compost and vermicompost on growth, yield and nutrients content of common beans crop (Phaseolus vulgaris L.). J. Ecol. Eng. 25, 215–226. doi: 10.12911/22998993/176862
Aso, S. N. (2024). Mitigation of external costs of inorganic fertilizers with liquid fraction digestate. Biomass Convers. Bioref. 14, 2193–2209. doi: 10.1007/s13399-022-02497-y
Assandri, D., Bianco, A., Pampuro, N., Cavallo, E., Zara, G., Bardi, L., et al. (2023). Enhancing fertilizer effect of bioprocessed brewers' spent grain by microbial consortium addition. Agronomy 13:2654. doi: 10.3390/agronomy13102654
Assandri, D., Pampuro, N., Zara, G., Bianco, A., Cavallo, E., and Budroni, M. (2021). Co-composting of brewers' spent grain with animal manures and wheat straw: influence of two composting strategies on compost quality. Agronomy 11:1349. doi: 10.3390/agronomy11071349
Atieno, M., Herrmann, L., Nguyen, H. T., Phan, H. T., Nguyen, N. K., Srean, P., et al. (2020). Assessment of biofertilizer use for sustainable agriculture in the Great Mekong Region. J. Environ. Manage. 275:111300. doi: 10.1016/j.jenvman.2020.111300
Bianco, A., Budroni, M., Zara, S., Mannazzu, I., Fancello, F., and Zara, G. (2020). The role of microorganisms on biotransformation of brewers' spent grain. Appl. Microbiol. Biotechnol. 104, 8661–8678. doi: 10.1007/s00253-020-10843-1
Bianco, A., Fancello, F., Garau, M., Deroma, M., Atzori, A. S., Castaldi, P., et al. (2022). Microbial and chemical dynamics of brewers' spent grain during a low-input pre-vermicomposting treatment. Sci. Total Environ. 802:149792. doi: 10.1016/j.scitotenv.2021.149792
Bianco, A., Zara, G., Garau, M., Castaldi, P., Atzori, A. S., Deroma, M. A., et al. (2024). Microbial community assembly and chemical dynamics of raw brewers' spent grain during inoculated and spontaneous solid-state fermentation. Waste Manag. 174, 518–527. doi: 10.1016/j.wasman.2023.12.021
Bignami, C., Reyes, F., Saccaggi, M., Pane, C., Zaccardelli, M., and Ronga, D. (2023). Composts from Grapevine and Hazelnut by-products: a sustainable peat partial replacement for the growth of micropropagated Hazelnut and Raspberry in containers. Horticulturae 9:481. doi: 10.3390/horticulturae9040481
Budroni, M., Mannazzu, I., Zara, S., Saba, S., Pais, A., and Zara, G. (2020). Composition and functional profiling of the microbiota in the casts of Eisenia fetida during vermicomposting of brewers' spent grains. Biotechnol. Rep. 25:e00439. doi: 10.1016/j.btre.2020.e00439
Burgess, T., Dell, B., and Malajczuk, N. (1994). Variation in mycorrhizal development and growth stimulation by 20 Pisolithus isolates inoculated on to Eucalyptus grandis W. Hill ex Maiden. New Phytol. 127, 731–739. doi: 10.1111/j.1469-8137.1994.tb02977.x
Cacace, C., Cocozza, C., Traversa, A., Coda, R., Rizzello, C. G., Pontonio, E., et al. (2022). Potential of native and bioprocessed brewers' spent grains as organic soil amendments. Front. Sustain. Food Syst. 6:1010890. doi: 10.3389/fsufs.2022.1010890
Canellas, L. P., Olivares, F. L., Aguiar, N. O., Jones, D. L., Nebbioso, A., Mazzei, P., et al. (2015). Humic and fulvic acids as biostimulants in horticulture. Sci. Hortic. 196, 15–27. doi: 10.1016/j.scienta.2015.09.013
Cartelat, A., Cerovic, Z. G., Goulas, Y., Meyer, S., Lelarge, C., Prioul, J.-L., et al. (2005). Optically assessed contents of leaf polyphenolics and chlorophyll as indicators of nitrogen deficiency in wheat (Triticum aestivum L.). F. Crop. Res. 91, 35–49. doi: 10.1016/j.fcr.2004.05.002
Chen, Y., Prior, B. A., Shi, G., and Wang, Z. (2011). A rapid PCR-based approach for molecular identification of filamentous fungi. J. Microbiol. 49, 675–679. doi: 10.1007/s12275-011-0525-3
Choi, H.-S., Zhao, Y., Dou, H., Cai, X., Gu, M., and Yu, F. (2018). Effects of biochar mixtures with pine-bark based substrates on growth and development of horticultural crops. Hortic. Environ. Biotechnol. 59, 345–354. doi: 10.1007/s13580-018-0035-x
da Silva, D. G., de Carvalho, A. L., de Jesus Araújo, E., Rocha, M. E., Moreira, B. O., and Santos, D. L. S. (2020). Development of an analytical method for determination of nitrate in leafy vegetables using ion chromatography. OALib 7, 1–8. doi: 10.4236/oalib.1106471
de Lima, D. P., dos Santos Pinto Júnior, E., de Menezes, A. V., de Souza, D. A., de São José, V. P. B., da Silva, B. P., et al. (2024). Chemical composition, minerals concentration, total phenolic compounds, flavonoids content and antioxidant capacity in organic and conventional vegetables. Food Res. Int. 175:113684. doi: 10.1016/j.foodres.2023.113684
Dhen, N., Abed, S., ben Zouba, A., Haouala, F., and AlMohandes Dridi, B. (2018). The challenge of using date branch waste as a peat substitute in container nursery production of lettuce (Lactuca sativa L.). Int. J. Recycl. Org. Waste Agric. 7, 357–364. doi: 10.1007/s40093-018-0221-y
Garau, M., Castaldi, P., Patteri, G., Roggero, P. P., and Garau, G. (2021). Evaluation of Cynara cardunculus L. and municipal solid waste compost for aided phytoremediation of multi potentially toxic element–contaminated soils. Environ. Sci. Pollut. Res. 28, 3253–3265. doi: 10.1007/s11356-020-10687-2
Garau, M., Garau, G., Diquattro, S., Roggero, P. P., and Castaldi, P. (2019). Mobility, bioaccessibility and toxicity of potentially toxic elements in a contaminated soil treated with municipal solid waste compost. Ecotoxicol. Environ. Saf. 186:109766. doi: 10.1016/j.ecoenv.2019.109766
Gomah, H. H., Ahmed, M. M. M., Abdalla, R. M., Farghly, K. A., and Eissa, M. A. (2020). Utilization of some organic wastes as growing media for lettuce (Lactuca sativa L.) plants. J. Plant Nutr. 43, 2092–2105. doi: 10.1080/01904167.2020.1766077
Guan, T.-K., Wang, Q.-Y., Li, J.-S., Yan, H.-W., Chen, Q.-J., Sun, J., et al. (2023). Biochar immobilized plant growth-promoting rhizobacteria enhanced the physicochemical properties, agronomic characters and microbial communities during lettuce seedling. Front. Microbiol. 14:1218205. doi: 10.3389/fmicb.2023.1218205
Harbart, V., Frede, K., Fitzner, M., and Baldermann, S. (2023). Regulation of carotenoid and flavonoid biosynthetic pathways in Lactuca sativa var capitate L. in protected cultivation. Front. Plant Sci. 14:1124750. doi: 10.3389/fpls.2023.1124750
Hoque, M. M., Ajwa, H., Othman, M., Smith, R., and Cahn, M. (2010). Yield and postharvest quality of lettuce in response to nitrogen, phosphorus, and potassium fertilizers. HortScience 45, 1539–1544. doi: 10.21273/HORTSCI.45.10.1539
ISO 16649-2 (2001). Microbiology of Food and Animal Feeding Stuffs Horizontal Method for the Enumeration of Beta-Glucuronidase-Positive Escherichia coli Part 2: Colony-count Technique at 44 Degrees C Using 5-Bromo-4-Chloro-3-Indolyl Beta-D-Glucuronide.
ISO 6579-1 (2017). Microbiology of the food chain — Horizontal method for the detection, enumeration and serotyping of Salmonella — Part 1: detection of Salmonella spp. ISO/TC 34/SC 9. Microbiol. Food Chain 1:50.
Jara-Samaniego, J., Pérez-Murcia, M. D., Bustamante, M. A., Pérez-Espinosa, A., Paredes, C., López, M., et al. (2017). Composting as sustainable strategy for municipal solid waste management in the Chimborazo Region, Ecuador: suitability of the obtained composts for seedling production. J. Clean. Prod. 141, 1349–1358. doi: 10.1016/j.jclepro.2016.09.178
Kramer-Walter, K. R., Bellingham, P. J., Millar, T. R., Smissen, R. D., Richardson, S. J., and Laughlin, D. C. (2016). Root traits are multidimensional: specific root length is independent from root tissue density and the plant economic spectrum. J. Ecol. 104, 1299–1310. doi: 10.1111/1365-2745.12562
Lichtenthaler, H. K., and Buschmann, C. (2001). Chlorophylls and carotenoids measurement and UV-VIS characterization Lichtenthaler 2001. Curr. Protoc. Food Anal. Chem. 1, 1–8. doi: 10.1002/0471142913.faf0403s01
Lucas, E. G., Izquierdo, C. G., and Fernández, M. T. H. (2018). Changes in humic fraction characteristics and humus-enzyme complexes formation in semiarid degraded soils restored with fresh and composted urban wastes. A 5-year field experiment. J. Soils Sedim. 18, 1376–1388. doi: 10.1007/s11368-016-1537-8
Macioszek, V. K., Marciniak, P., and Kononowicz, A. K. (2023). Impact of Sclerotinia sclerotiorum infection on lettuce (Lactuca sativa L.) survival and phenolics content—A case study in a horticulture farm in Poland. Pathogens 12:1416. doi: 10.3390/pathogens12121416
Manolikaki, I., and Diamadopoulos, E. (2020). Agronomic potential of biochar prepared from brewery byproducts. J. Environ. Manage. 255:109856. doi: 10.1016/j.jenvman.2019.109856
Melito, S., Ronga, D., Marceddu, D., Kallikazarou, N. I., Antoniou, M. G., and Giannini, V. (2023). Organo-mineral fertilizer containing struvite from liquid digestate for Cucurbita pepo L. seedling production. J. Soil Sci. Plant Nutr. 23, 6707–6720. doi: 10.1007/s42729-023-01524-9
Ministero delle Politiche Agricole e Forestali (1999). Metodi Ufficiali di Analisi Chimica del Suolo (in Italian language). Rome.
Mohamed, O.-Z., Yassine, B., Hilali Rania, E., El Hassan, A., Abdellatif, H., and Rachid, B. (2020). Evaluation of compost quality and bioprotection potential against Fusarium wilt of date palm. Waste Manag. 113, 12–19. doi: 10.1016/j.wasman.2020.05.035
Moretti, B., Bertora, C., Grignani, C., Lerda, C., Celi, L., and Sacco, D. (2020). Conversion from mineral fertilisation to MSW compost use: Nitrogen fertiliser value in continuous maize and test on crop rotation. Sci. Total Environ. 705:135308. doi: 10.1016/j.scitotenv.2019.135308
Nadeem, S. M., Imran, M., Naveed, M., Khan, M. Y., Ahmad, M., Zahir, Z. A., et al. (2017). Synergistic use of biochar, compost and plant growth-promoting rhizobacteria for enhancing cucumber growth under water deficit conditions. J. Sci. Food Agric. 97, 5139–5145. doi: 10.1002/jsfa.8393
Nguyen, T. T. N., Wallace, H. M., Xu, C.-Y., Xu, Z., Farrar, M. B., Joseph, S., et al. (2017). Short-term effects of organo-mineral biochar and organic fertilisers on nitrogen cycling, plant photosynthesis, and nitrogen use efficiency. J. Soils Sedim. 17, 2763–2774. doi: 10.1007/s11368-017-1839-5
Papathanasiou, F., Papadopoulos, I., Tsakiris, I., and Tamoutsidis, E. (2012). Vermicompost as a soil supplement to improve growth, yield and quality of lettuce (Lactuca sativa L.). J. Food Agric. Environ. 10, 677–682.
Peng, C., Li, Q., Zhang, Z., Wu, Z., Song, X., Zhou, G., et al. (2019). Biochar amendment changes the effects of nitrogen deposition on soil enzyme activities in a Moso bamboo plantation. J. For. Res. 24, 275–284. doi: 10.1080/13416979.2019.1646970
Pérocheau, A. S. (2024). Valorisation of Brewer's spent grain : lignocellulosic fractionation and its potential for polymer and composite material applications. ChemRxiv 1–33. doi: 10.26434/chemrxiv-2024-qjdpn
Pompeiano, A., Volpi, I., Volterrani, M., and Guglielminetti, L. (2013). N source affects freeze tolerance in bermudagrass and zoysiagrass. Acta Agric. Scand. Sect. B Soil Plant Sci. 63, 341–351. doi: 10.1080/09064710.2013.781669
Qin, K., and Leskovar, D. I. (2020). Humic substances improve vegetable seedling quality and post-transplant yield performance under stress conditions. Agriculture 10:254. doi: 10.3390/agriculture10070254
Radziemska, M., Vaverková, M. D., Adamcová, D., Brtnický, M., and Mazur, Z. (2019). Valorization of fish waste compost as a fertilizer for agricultural use. Waste Biomass Valor. 10, 2537–2545. doi: 10.1007/s12649-018-0288-8
Raza, S. T., Feyissa, A., Li, R., Rene, E. R., Ali, Z., Iqbal, H., et al. (2024). Emerging technology effects on combined agricultural and eco-vermicompost. J. Environ. Manage. 352:120056. doi: 10.1016/j.jenvman.2024.120056
Rodríguez-Vila, A., Atuah, L., Abubakari, A. H., Atorqui, D. W., Abdul-Karim, A., Coole, S., et al. (2022). Effect of biochar on micronutrient availability and uptake into leafy greens in two urban tropical soils with contrasting soil pH. Front. Sustain. Food Syst. 6:821397. doi: 10.3389/fsufs.2022.821397
Rodríguez-Vila, A., Selwyn-Smith, H., Enunwa, L., Smail, I., Covelo, E. F., and Sizmur, T. (2018). Predicting Cu and Zn sorption capacity of biochar from feedstock C/N ratio and pyrolysis temperature. Environ. Sci. Pollut. Res. 25, 7730–7739. doi: 10.1007/s11356-017-1047-2
Rozas, A., Aponte, H., Maldonado, C., Contreras-Soto, R., Medina, J., and Rojas, C. (2023). Evaluation of compost and biochar as partial substitutes of peat in growing media and their influence in microbial counts, enzyme activity and Lactuca sativa L. seedling growth. Horticulturae 9:168. doi: 10.3390/horticulturae9020168
Saba, S., Zara, G., Bianco, A., Garau, M., Bononi, M., Deroma, M., et al. (2019). Comparative analysis of vermicompost quality produced from brewers' spent grain and cow manure by the red earthworm Eisenia fetida. Bioresour. Technol. 293:122019. doi: 10.1016/j.biortech.2019.122019
Sarkar, M. D., Rahman, M. J., Uddain, J., Quamruzzaman, M., Azad, M. O. K., Rahman, M. H., et al. (2021). Estimation of yield, photosynthetic rate, biochemical, and nutritional content of red leaf lettuce (Lactuca sativa L.) grown in organic substrates. Plants 10:1220. doi: 10.3390/plants10061220
Singh, V. K., Malhi, G. S., Kaur, M., Singh, G., and Jatav, H. S. (2022). “Use of organic soil amendments for improving soil ecosystem health and crop productivity,” in Ecosystem Services: Types, Management and Benefits, eds. H. Singh Jatav, and V. D. Rajput (Nova Science Publishers), 63117. Available online at: https://www.researchgate.net/publication/358770318%0AUse (accessed February, 2024).
Song, Y., Yuan, G., Wu, Q., Situ, G., Liang, C., Qin, H., et al. (2023). Change in microbial metabolic quotient under biochar amendment was associated with soil organic carbon quality, microbial community composition, and enzyme activity in bulk and rhizosphere soils in an acid rice paddy. J. Soil Sci. Plant Nutr. 23, 3149–3162. doi: 10.1007/s42729-023-01363-8
Suwor, P., Jeakkhajorn, S., and Kramchote, S. (2020). Effects of different compost manures application on growth of lettuces (Lactuca sativa L.). Int. J. Agric. Technol. 16, 1257–1266.
Tabrika, I., Azim, K., Mayad, E. H., and Zaafrani, M. (2020). Composting of tomato plant residues: improvement of composting process and compost quality by integration of sheep manure. Org. Agric. 10, 229–242. doi: 10.1007/s13165-019-00268-0
Urra, J., Alkorta, I., and Garbisu, C. (2019). Potential benefits and risks for soil health derived from the use of organic amendments in agriculture. Agronomy 9:542. doi: 10.3390/agronomy9090542
Vigoroso, L., Pampuro, N., Bagagiolo, G., and Cavallo, E. (2021). Factors influencing adoption of compost made from organic fraction of municipal solid waste and purchasing pattern: a survey of Italian professional and hobbyist users. Agronomy 11:1262. doi: 10.3390/agronomy11061262
Walia, S. S., and Kaur, T. (2024). “Influence of vermicompost on soil health,” in Earthworms and Vermicomposting (Singapore: Springer Nature), 89–107.
Wolińska, A., Zapasek, M., and Stepniewska, Z. (2016). The optimal TTC dose and its chemical reduction level. Acta Agrophys. 23, 303–314.
Xi, J., Li, H., Xi, J., Tan, S., Zheng, J., and Tan, Z. (2020). Effect of returning biochar from different pyrolysis temperatures and atmospheres on the growth of leaf-used lettuce. Environ. Sci. Pollut. Res. 27, 35802–35813. doi: 10.1007/s11356-020-09840-8
Yassen, A. A., Essa, E. M., Marzouk, N. M., and Zaghloul, S. M. (2020). Impact of vermicompost and foliar spray of vermiwash on growth, yield and nutritional status of lettuce plants. Plant Arch. 20, 449–455.
Yin, J., Li, D., Yu, J., Bai, X., Cui, W., Liu, R., et al. (2022). Environmental and economic benefits of substituting chemical potassium fertilizer with crop straw residues in China. Environ. Sci. Pollut. Res. 30, 30603–30611. doi: 10.1007/s11356-022-24128-9
Yoo, J.-H., Luyima, D., Lee, J.-H., Park, S.-Y., Yang, J.-W., An, J.-Y., et al. (2021). Effects of brewer's spent grain biochar on the growth and quality of leaf lettuce (Lactuca sativa L. var. crispa.). Appl. Biol. Chem. 64:10. doi: 10.1186/s13765-020-00577-z
Zhang, J., Fang, H., Zhao, Y., Zheng, Y., Jiang, J., and Gu, X. (2023). Responses of soil nutrients and rhizosphere microbial communities of a medicinal plant Pinellia ternata to vermicompost. 3 Biotech 13:353. doi: 10.1007/s13205-023-03780-z
Keywords: brewery by-products, microorganisms, fertilizer, compost, vermicompost, biochar, lettuce, horticulture
Citation: Bianco A, Melito S, Garau M, Giannini V, Zara G, Assandri D, Oufensou S, Coronas R, Pampuro N and Budroni M (2024) The potential use of brewers' spent grain-based substrates as horticultural bio-fertilizers. Front. Sustain. Food Syst. 8:1404914. doi: 10.3389/fsufs.2024.1404914
Received: 21 March 2024; Accepted: 03 May 2024;
Published: 23 May 2024.
Edited by:
Çagri Akyol, Ghent University, BelgiumReviewed by:
Nnanake-Abasi Offiong, Topfaith University, NigeriaWilgince Apollon, National Polytechnic Institute (IPN), Mexico
Copyright © 2024 Bianco, Melito, Garau, Giannini, Zara, Assandri, Oufensou, Coronas, Pampuro and Budroni. This is an open-access article distributed under the terms of the Creative Commons Attribution License (CC BY). The use, distribution or reproduction in other forums is permitted, provided the original author(s) and the copyright owner(s) are credited and that the original publication in this journal is cited, in accordance with accepted academic practice. No use, distribution or reproduction is permitted which does not comply with these terms.
*Correspondence: Davide Assandri, davide.assandri@stems.cnr.it